Corrigendum: Deciphering decidual leukocyte traffic with serial intravascular staining
- 1Department of Obstetrics and Gynecology, University of Wisconsin-Madison, Madison, WI, United States
- 2Wisconsin National Primate Research Center, Madison, WI, United States
- 3Department of Comparative Biosciences, University of Wisconsin-Madison, Madison, WI, United States
The decidual immunome is dynamic, dramatically changing its composition across gestation. Early pregnancy is dominated by decidual NK cells, with a shift towards T cells later in pregnancy. However, the degree, timing, and subset-specific nature of leukocyte traffic between the decidua and systemic circulation during gestation remains poorly understood. Herein, we employed intravascular staining in pregnant C57BL/6J mice and cynomolgus macaques (Macaca fascicularis) to examine leukocyte traffic into the decidual basalis during pregnancy. Timed-mated or virgin mice were tail-vein injected with labelled αCD45 antibodies 24 hours and 5 minutes before sacrifice. Pregnant cynomolgus macaques (GD155) were infused with labelled αCD45 at 2 hours or 5 mins before necropsy. Decidual cells were isolated and resulting suspensions analyzed by flow cytometry. We found that the proportion of intravascular (IVAs)-negative leukocytes (cells labeled by the 24h infusion of αCD45 or unlabeled) decreased across murine gestation while recent immigrants (24h label only) increased in mid- to late-gestation. In the cynomolgus model our data confirmed differential labeling of decidual leukocytes by the infused antibody, with the 5 min infused animal having a higher proportion of IVAs+ cells compared to the 2hr infused animal. Decidual tissue sections from both macaques showed the presence of intravascularly labeled cells, either in proximity to blood vessels (5min infused animal) or deeper into decidual stroma (2hr infused animal). These results demonstrate the value of serial intravascular staining as a sensitive tool for defining decidual leukocyte traffic during pregnancy.
1 Introduction
The maternal immune system plays a critical role at the maternal-fetal interface by providing protection against pathogens (1), maintaining tolerance towards the semi-allogeneic fetus (2, 3), and promoting vascular remodeling in the decidua (4–6). The decidua (endometrium of pregnancy underlying the placenta and fetal membranes) is a specialized mucosa that maintains a dynamic immunome. In recent years, extensive research has been directed at developing a better understanding of the leukocyte composition at the decidua in humans, mice and rhesus macaque systems using advanced flow cytometry, mass cytometry and single cell sequencing (7–13). By virtue of experimental design and necessity, these studies have all relied on single time-point snapshots of immune composition at the maternal fetal interface (decidua and placenta), limiting our understanding of immune trafficking between systemic circulation and target tissue.
The composition of the decidual (human and mouse) immunome is specific to studied gestational time-points, as early pregnancy is dominated by decidual NK (dNK) cells, with few T cells observed (14–17). This pattern shifts in late pregnancy to one that is dominated by T cells (18). Early and mid-pregnancy is of particular interest as deficits in normal dNK cell-driven vascular remodeling result in a higher risk of preeclampsia and other pregnancy pathology (19–21). Some studies have suggested that conventional NK cells can acquire a “decidua-like” phenotype upon exposure to specific cytokine cocktails (22), while others suggest that CD34+ hematopoietic precursors differentiate in human decidua in situ (23). In recent studies of dNK cells, at least one subset appears to be tissue resident (24) in a murine parabiosis model, while others might traffic into the decidua and/or expand locally in pregnancy. The question of decidual lymphocyte traffic is especially relevant as human studies suggest that as many as three dNK cells subsets exist, distinguished by cell surface receptors and transcriptional/functional programming (13). Similarly, innate lymphoid cells (ILCs) have been identified in both the murine (11, 25, 26) and human decidua (8, 27, 28) and have been associated with pre-term birth (29, 30). Although ILCs are found in circulation, they are most abundant at mucosal sites (31, 32). And, like NK cells, many novel subsets have been identified in the decidua (33, 34), however, questions remain as to whether they are recruited from the circulation or whether they proliferate and differentiate in situ.
T cells and antigen presenting cells (APCs) play a critical role in protection of and tolerance to the conceptus at the maternal-fetal interface (1, 18). From the first trimester onward, there is a gradual and progressive change in the frequency and functionality of both T and immune myeloid cells (monocytes, macrophages, dendritic cells, and granulocytes) as pregnancy progresses. Whereas T cell frequency, especially effector T cells, increases dramatically toward term, the frequency of myeloid cells remains relatively high and constant across gestation (9, 15, 35, 36). However, the origin of these gestational shifts in immune cell frequency, whether due to trafficking, differentiation or cell proliferation is unclear. It has been suggested that epigenetic regulation of chemokine expression is the primary mechanism by which entrance of T cells and exit of dendritic cells is regulated (18, 37, 38). Similarly, chemokines have also been implicated in recruitment of mucosal-associated invariant T (MAIT) cells into the intervillous space (39).
Taken together, a comprehensive model of how decidual leukocytes traffic, expand and contract in numbers during pregnancy and the peripartum period is still elusive. Development and testing of approaches to simultaneously track cellular trafficking, differentiation and function of decidual immune cells is essential for deciphering the role of the immune system in pregnancy pathology. Multiple techniques have been developed to understand how leukocytes traffic across various tissues (40, 41), such as the use of parabionts (24, 41), adoptive transfer (42) and in vivo homing assays (43), and intravital microscopy (40, 44). CFSE injection directly into the cervix has also been used to assess migration of dendritic cells in the decidua (36), although this technique only allows for a one timepoint measurement. As a means to start delineating traffic of individual cell types in the decidua, we employed serial intravascular staining (SIVS), a powerful method technique by which to assess tissue localization and traffic of lymphocytes (45–48). We illustrate that a similar approach is possible in both the mouse and non-human primates to understand leukocyte traffic in the decidua during pregnancy. Our data indicate that this technique will prove invaluable in our understanding of leukocyte traffic in the decidua, with wide applications, including integration with infectious model studies.
2 Materials and methods
2.1 Ethics, care & use of macaques
The rhesus macaques used in this study were cared for by the staff at the Wisconsin National Primate Research Center (WNPRC) according to regulations and guidelines of the University of Wisconsin Institutional Animal Care and Use Committee, which approved this study protocol (G006070) in accordance with recommendations of the Weatherall report and according to the principles described in the National Research Council’s Guide for the Care and Use of Laboratory Animals. All animals were housed in enclosures with at least 4.3, 6.0, or 8.0 sq. ft. of floor space, measuring 30, 32, or 36 inches high, and containing a tubular PVC or stainless steel perch. Each individual enclosure was equipped with a horizontal or vertical sliding door, an automatic water lixit, and a stainless steel feed hopper. All animals were fed using a nutritional plan based on recommendations published by the National Research Council. Twice daily, macaques were fed a fixed formula of extruded dry diet (2050 Teklad Global 20% Protein Primate Diet) with adequate carbohydrate, energy, fat, fiber (10%), mineral, protein, and vitamin content. Dry diets were supplemented with fruits, vegetables, and other edible foods (e.g., nuts, cereals, seed mixtures, yogurt, peanut butter, popcorn, marshmallows, etc.) to provide variety to the diet and to inspire species-specific behaviors such as foraging. To further promote psychological well-being, animals were provided with food enrichment, human-to-monkey interaction, structural enrichment, and manipulanda. Environmental enrichment objects were selected to minimize chances of pathogen transmission from one animal to another and from animals to care staff. While in the study, all animals were evaluated by trained animal care staff at least twice daily for signs of pain, distress, and illness by observing appetite, stool quality, activity level, and physical condition. Animals exhibiting abnormal presentation for any of these clinical parameters were provided appropriate care by attending veterinarians.
The female macaques described in this report were co-housed with a compatible male and observed daily for menses and breeding. Pregnancy was detected by abdominal ultrasound, and gestational age was estimated as previously described (49). For physical examinations, antibody infusions, and blood collections, dams were anesthetized with an intramuscular dose of ketamine (10 mg/kg). Blood samples from the femoral or saphenous vein were obtained using a vacutainer system or needle and syringe. Pregnant macaques were monitored daily prior to and after infusions to assess general well-being and for any clinical signs of distress.
2.2 Intravascular staining
Female and male C57BL/6J (B6) mice were purchased from Jackson laboratory (Bar Harbor, ME). B6 female mice (6-13 weeks) were used for timed mating and experiments. The day when a vaginal plug was detected in a timed mating was counted as gestational day 0.5. Mice were tail-vein injected with 6.7 μg of AF488-conjugated CD45 antibody 24 hours and 6.7 μg of AF647-conjugated CD45 antibody 5 minutes before sacrifice (Figure 1A; Supplementary Table 1). Virgin mice and pregnant mice were sacrificed, and gestational day of each decidua was recorded. For technical calibration controls, spleens of uninfused wild-type mice were also collected.
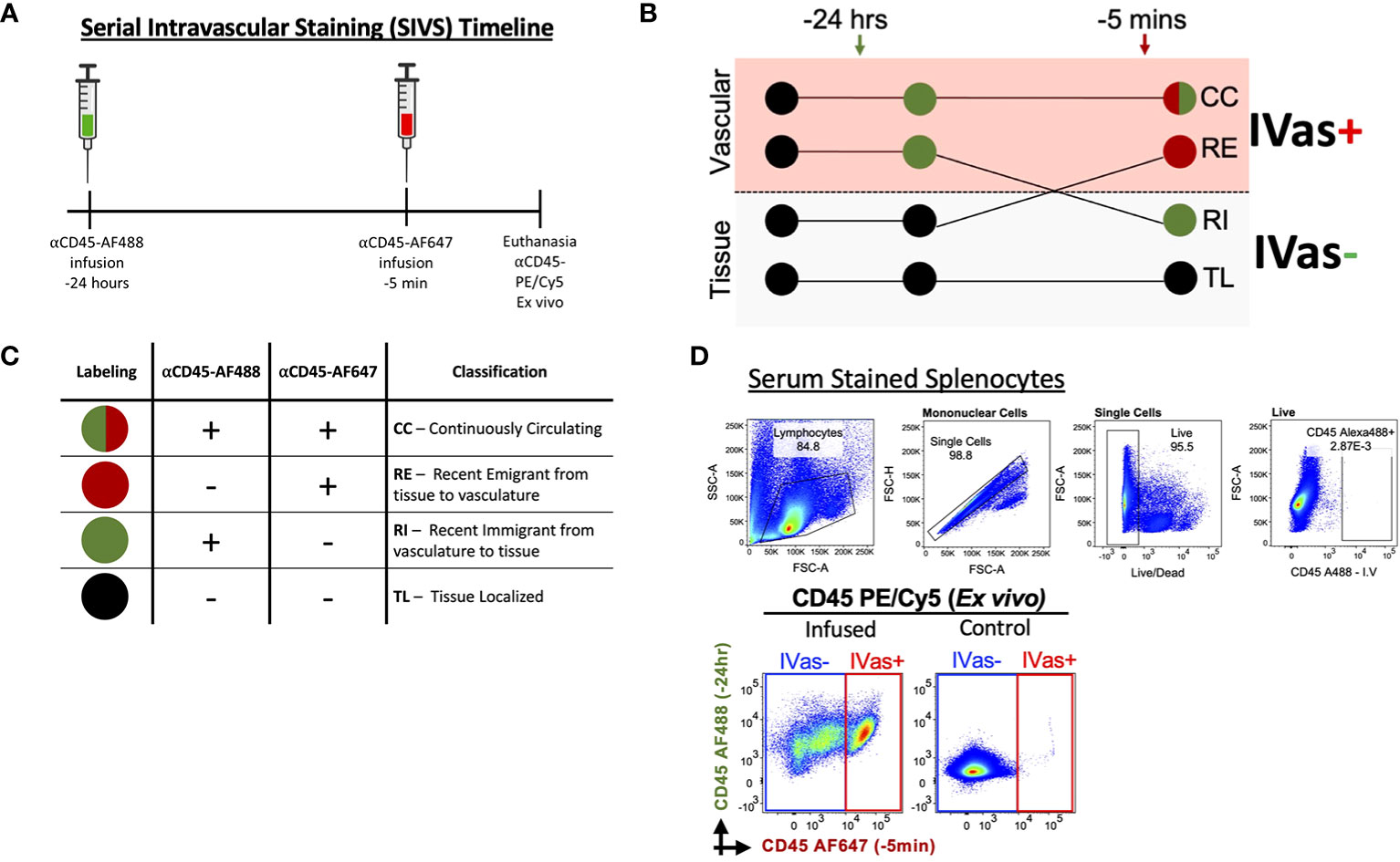
Figure 1 Serial Intravascular Staining of Leukocytes in the Mouse. (A) Experimental timeline illustrating serial infusions of αCD45 fluorochrome conjugated antibodies. (B) Schematic illustrating labeling of leukocytes by infused antibodies and (C) Interpretation of resulting intravascular labeling. (D) Top Labeling of splenocytes with serum of animal infused with αCD45-AF488 24 hours before euthanasia (plots representative of 2 experiments); Bottom Plots comparing infused animal (left) and not infused animal (right). Plots representative of 5 experiments.
Two pregnant (GD155) cynomolgus macaques (Macaca fascicularis) were infused with AF555-conjugated αCD45 (100 μg/kg) 2 hours and 5 mins before necropsy, respectively. Animals were then euthanized and decidua and placenta, as well as selected maternal tissues (spleen, lymph nodes), were collected. Peripheral blood was collected pre- and post-infusion.
2.3 Tissue processing
Mouse decidua or uteri were collected and minced with scissors in RMPI 1640 containing collagenase type V/DNAse I (Worthington Biochem). These specimens were then loaded in gentleMACS™ C tubes (Miltenyi Biotec Inc), and a specially adapted tissue dissociation program was run in a gentleMACS™ Dissociator for 30 minutes (Miltenyi Biotec Inc) (9). Spleen and Peyer’s patches (used as vascularly-permeant and mucosal lymphoid control tissues, respectively) were mechanically dissociated in RMPI 1640 containing 10% heat-inactivated FBS in gentleMACS™ C tubes by running corresponding programs for different tissue types in the gentleMACS™ Dissociator. After dissociation, homogenates were filtered through a 70 μm cell strainer, and red blood cells were lysed with ACK lysis buffer (Life Technologies). The resulting single cell suspensions were used in downstream flow cytometry applications.
Macaque decidua was dissected from the placenta and mononuclear cells were isolated as previously described (7). Maternal spleen and lymph nodes were processed as described above. Peripheral mononuclear cells (PBMCs) were isolated by gradient centrifugation. Briefly, blood was layered on Ficoll gradient media, spun, and the buffy coat was collected into RPMI. PBMCs were then washed and frozen for later analysis. In addition, tissue biopsies were collected and fixed overnight in 4% paraformaldehyde and paraffin embedded (FFPE). FFPE tissues sections (5 μm) were then stained with nuclear stain DAPI and imaged using the Nikon confocal microscope. Serial sections were stained with either hematoxylin and eosin and imaged using the Nikon Eclipse microscope.
2.4 Flow cytometry
Isolated MCs were first labeled with LIVE/DEAD® fixable blue stain (Invitrogen) according to manufacturer’s instructions. MCs were then labeled with fluorochrome-conjugated monoclonal antibodies, listed in Supplementary Table 1. Briefly, antibodies were diluted in BD Horizon Brilliant™ Stain Buffer (BD Biosciences, San Jose, CA) and used to label MCs according to the manufacturer’s instructions. Samples were then acquired using the LSR Fortessa in a 5 laser (355nm, 405nm, 488nm, 562nm, 633nm) 20-detector configuration (BD Biosciences) or in a Cytek Aurora spectral cytometer. Prior to downstream analysis, FCS files were either compensated within FlowJo or spectrally unmixed using the SpectroFlo software.
2.5 Data analysis
Manual analysis identifying well-characterized populations was performed using FlowJo v.10 software (FlowJo LLC, Ashland, OR). Dimensionality reduction was performed using the FlowSOM algorithm (50) and visualized within FlowJo. Briefly, Lineage negative, CD45-positive events were down sampled from 6 samples (2 virgin, 2 early-GD, 1 mid-GD, 1 late-GD), to 1000 cells per sample, for a total of 6000 cells (2 samples did not meet this threshold and were not down sampled). Down sampled populations were then coded for sample type and concatenated. The concatenated file was then analyzed using FlowSOM plugin within FlowJo, using only phenotypic marker expression (CD4, CD49a/b, CD103, KLRG1, and NK1.1). The resulting median fluorescence intensity (MFI) values for these markers were calculated within FlowJo for identified clusters. Cluster frequency was similarly calculated within FlowJo. Resulting data tables were coded and heatmap construction was done using the R package ComplexHeatmap (51). Clusters were annotated based on expression heatmaps, based on known expression markers (52, 53). All data are represented as median with interquartile range and statistical significance was determined by ANOVA, followed by Tukey’s post-hoc test to correct for multiple comparisons, using Prism® v. 7 (GraphPad Software, Inc, La Jolla, CA) or JMP® Pro v. 15.0.0 (SAS Institute Inc, Cary, NC), unless stated otherwise.
3 Results
3.1 Serial intravascular staining reveals leukocyte trafficking in mouse mucosal tissues
To determine if SIVS is a viable approach to studies of leukocyte traffic in virgin and pregnant mice we tested our ability to distinguish leukocytes isolated from uterus and decidua (Figure 1A). We maintained previously established nomenclature (45) and interpretation to determine whether leukocytes were designated as continuously circulating (CC), recent stromal emigrants (RE), recent immigrants (RI) to the virgin uterus/decidua, or tissue localized (TL) in the virgin uterus/decidua (Figures 1B, C). To exclude the possibility that unbound αCD45-AF488 would be circulating at the 5 min infusion time point, we tested the ability of serum from an infused animal to label splenocytes from a control animal and found that the serum did not label any splenocytes (Figure 1D, top), confirming that there was no unbound αCD45-AF488 capable of labeling leukocytes prior to euthanasia, with similar levels of background labeling compared to non-injected mice (Figure 1D, bottom).
3.2 Decidual trafficking of CD45+ leukocytes across pregnancy
We next assessed the trafficking status of total leukocytes in the decidua (Figure 2A). To determine if SIVS was capable of highlighting differences in leukocyte traffic across tissues, we assessed the proportion of CC, RE, RI, and TL leukocytes from our initial experiments and found that, indeed, we were able to detect increasing proportion of CC leukocytes in late gestation (Figure 2B, top). Furthermore, control tissues, Peyer’s patches (PPs) and spleen, chosen for relevance to pregnancy (virgin uterus, gestational decidua), a mucosal lymphocyte-rich tissue (PPs) and a vascular permeant tissue (spleen), displayed expected proportions of circulating leukocytes (47) (Figure 2B, bottom). Having established that the application of SIVS was capable of highlighting differences in the vascular status of leukocytes in murine pregnancy, we proceeded with a more detailed analysis of leukocyte traffic in the decidua (Figures 2C, D). First, we examined IVas- leukocytes, cells either labeled only by the 24h infusion of αCD45-AF488 or those that remained entirely unlabeled (Figure 1B) indicating inaccessibility to the intravascular compartment at the time of euthanasia. We found that the proportion of IVas- (RI + TL) leukocytes in the decidua decreased across gestation, while spleen and PP IVas- leukocyte proportions remained stable (Figure 2C). In agreement with other observations (54, 55), we found that there was an increase in the proportion of RI leukocytes in mid- to late-gestation (Figure 2D), suggesting an influx of leukocytes nearing parturition. A decrease in IVas- leukocytes conversely lead to a proportional increase in IVas+ (CC + RE) leukocytes (Figure 2B). This observation, coupled with a higher proportion of RI leukocytes suggests that there is increased leukocyte traffic in late gestation, supporting the concept that the decidua is actively restructured immunologically when nearing parturition (37, 54).
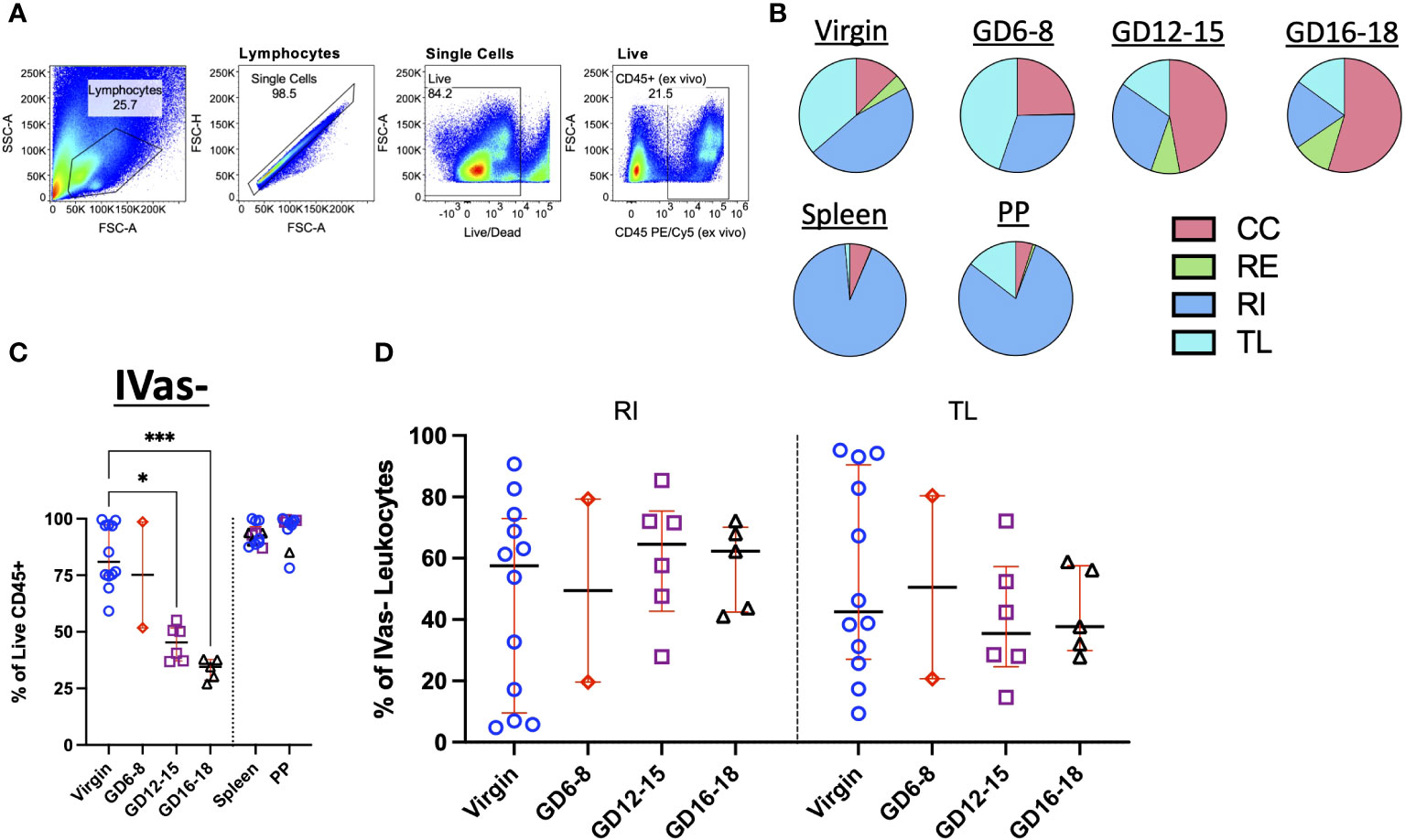
Figure 2 Trafficking Dynamics of Leukocytes Across Murine Gestation. (A) Representative gating strategy identifying total leukocytes in murine decidua. (B) Quantification of CC, RE, RI, TL leukocytes in virgin uterus, indicated gestational day (GD) decidua, spleen and Peyer’s patch (PP) of animals following infusion protocol outlined in Figure 1A. CC, continuously circulating; RE, recent emigrant; RI, recent immigrant; TL, tissue localized. Virgin, n = 5; GD6-8, n = 2; GD12-15, n = 3; GD16-18, n = 2. (C) Proportion of IVas- leukocytes from total leukocytes (Live CD45+) in the virgin uterus, early (GD6-8), mid (GD12-15), and late (GD16-18) murine gestation. Spleen and Peyer’s patch proportions also quantified for some animals. (D) Proportion of Recent Immigrant (RI) and Tissue Localized (TL) leukocytes from IVas- leukocytes across murine gestation. Shown are median with interquartile range. Virgin, n = 12; GD6-8, n = 2; GD12-15, n = 6; GD16-18, n = 5. Statistical significance was determined by One-way ANOVA followed by Tukey’s post-hoc test. *p < 0.05, ***p < 0.001.
3.3 Gestational traffic of decidual T cells and innate lymphocytes and their subsets
Both adaptive and innate lymphocytes have been implicated in having important roles in pregnancy (1, 56, 57). To determine their respective trafficking dynamics, we assayed both the T cell compartment (CD3+) and Innate Lymphocytes (decidual NK cells and ILCs) in the murine decidua. Broadly, the results indicate an increase in CC and a decrease in TL cells in both T and ILC compartments across pregnancy (Figure 3A; Supplementary Figure 1). Closer examination of the data confirmed this trend, with the proportion of IVas- (RI + TL) cells decreasing in both T cells and ILCs (Figure 3B). Interestingly, the proportion of TL T cells increased in late gestation, suggesting that T cells enter and persist (>24h) in the decidua in late gestation as has been previously observed (18, 58), while the proportion of TL ILCs remained lower across gestation (Figure 3A; Supplementary Figure 2). The majority of gestational RE and TL ILCs were NK1.1- and CD49b-negative (Figure 3C), suggesting that they are not classical NK cells or trNK cells (24, 59). Furthermore, we saw an increase in the proportion of RI ILCs that were NK1.1-CD49b+ (Figure 3C) in early gestation, suggesting that this subset of ILCs is recruited into the decidua early in pregnancy.
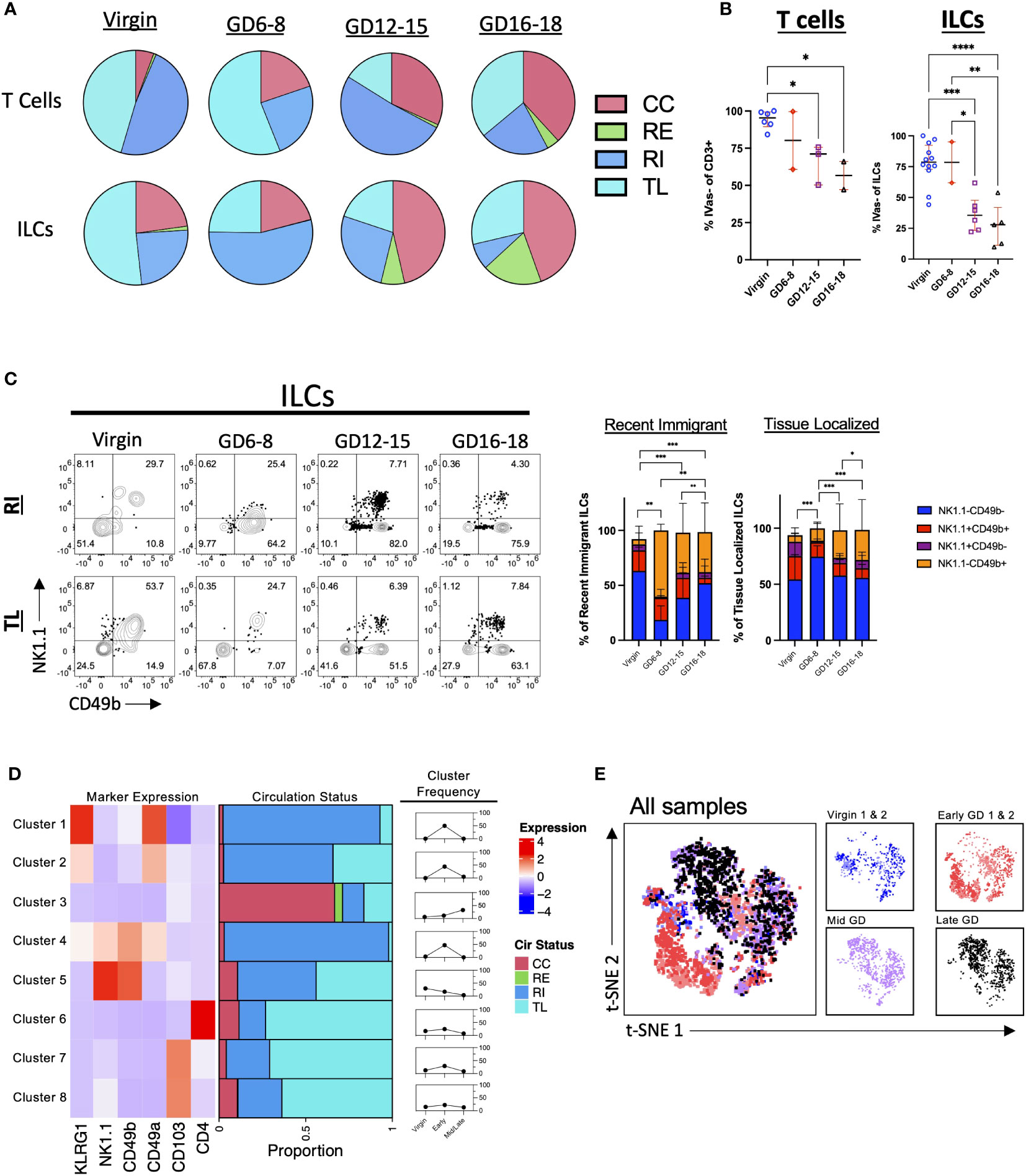
Figure 3 Pattern of T Cell and ILC Entrance and Exit Across Murine Gestation. (A) Quantification of CC, RE, RI, TL T cells and ILCs in virgin uterus and indicated GD decidua. Virgin, n = 5; GD6-8, n = 2; GD12-15, n = 3; GD16-18, n = 2. (B) Proportion of IVas- T cells (left) and ILCs (right). Shown are median with interquartile range. T cells: Virgin, n = 5; GD6-8, n = 2; GD12-15, n = 3; GD16-18, n = 2. ILCs: Virgin, n = 12; GD6-8, n = 2; GD12-15, n = 6; GD16-18, n = 5. Statistical significance was determined by One-way ANOVA followed by Tukey’s post-hoc test. *p < 0.05, **p < 0.005, ***p < 0.0005, ****p < 0.0001. (C) Distribution of NK1.1 and CD49b expression within RI and TL ILCs across gestation. Statistical significance was determined by Pearson’s chi-squared test followed by nonparametric Wilcoxon test. *p < 0.05, **p < 0.005, ***p < 0.0001. Virgin, n = 9; GD6-8, n = 2; GD12-15, n = 6; GD16-18, n = 5. (D, E) ILCs (pre-gated LiveCD45+CD3-TCRβ-CD11c-CD19-Ly6G-) from 2 virgin, 2 GD6-8 (Early), and 2 GD12-18 (Mid/Late) animals were clustered using FlowSOM (D) and visualized with t-SNE (E). (D) Left, heatmap summarizing marker expression across the 8 clusters identified. Center, bar plot summarizing the proportion of CC, RE, RI, and TL ILCs within each cluster. Right, summarizing line graphs. (E) Left, t-SNE map overlayed with animals included in analysis. Right, t-SNE maps segregated by virgin/GD. CC, continuously circulating; RE, recent emigrant; RI, recent immigrant; TL, tissue localized.
To better understand decidual ILC trafficking dynamics, we employed FlowSOM (50), a dimensionality reduction technique that allows for the unsupervised clustering of flow cytometry data sets (Supplementary Figure 3), coupled with t-SNE visualization (60) A total of 6 samples (2 virgin, 2 GD6-8, 1 GD12-15, and 1 GD16-18) were used for clustering analysis. We instructed FlowSOM to partition our dataset into 8 meta clusters (Figure 3D; Supplementary Figure 3 and Supplementary Table 2) based on marker expression. Only one cluster (Cluster 3; ILCs) had a majority of cells that were CC, with this cluster having a higher frequency in mid/late gestation (Figures 3D, E). Early pregnancy was dominated by Clusters 1, 2 (ILC2-like) and 4 (intermediate ILC1s), all CD49a+ and RI, suggesting that these subsets are recruited upon the initiation of decidualization in the mouse leading to upregulation of CD49 expression (Figure 3D). Indeed, we found that the early GD samples occupied an entire portion of the tSNE map, corresponding to cluster 4 (Figure 3E; Supplementary Figure 3B). These subsets can also represent cells that are closely associated with decidual vasculature and therefore are labeled by infused antibodies. Interestingly, TL ILCs were mostly non-NK ILCs expressing the tissue-residency marker CD103. Rather than a new decidual ILC (dILCs) subset, these TL ILCs might be in a state of transition, under the influence of the decidual stroma.
3.4 SIVS proof-of-concept in cynomolgus macaque decidua detected by flow cytometry
The non-human primate (NHP) is the ideal model for human pregnancy, sharing many important characteristics, including placenta villous morphology, placental MHC and endocrine phenotypes, and degree of spiral artery remodeling (61) that is not seen in mice. Recently, serial intravascular staining has been shown to delineate trafficking of leukocytes in the rhesus macaque (45, 46). To provide preliminary evidence that the cynomolgus macaque decidua can be effectively analyzed using SIVS we designed a pilot experiment involving one antibody (αCD45-AF555) infusion into pregnant (GD155, term GD165) cynomolgus macaques at either 2 hours (Animal #2) or 5 mins (Animal #1) before necropsy (Figure 4A) to assess intravascular (i.v.) staining of leukocytes in the decidua. We confirmed successful antibody infusions by assessing PBMC αCD45-AF555 staining pre- and post-infusion (Figure 4B) and found that the majority of circulating PBMCs were indeed stained. Next, we assessed the proportion of leukocytes that were labeled in the spleen and decidua of both infused animals (Figures 4C, D). As expected, we found that a similar proportion of splenic leukocytes were labeled in both the 2hr and 5min animals (Figure 4C), with little αCD45-AF555 signal in a control (not infused) spleen. The decidua, however, displayed differential labeling, with the decidua from the 5min infused animal having a higher proportion of labeled cells compared to the 2hr infused animal (Figure 4D).
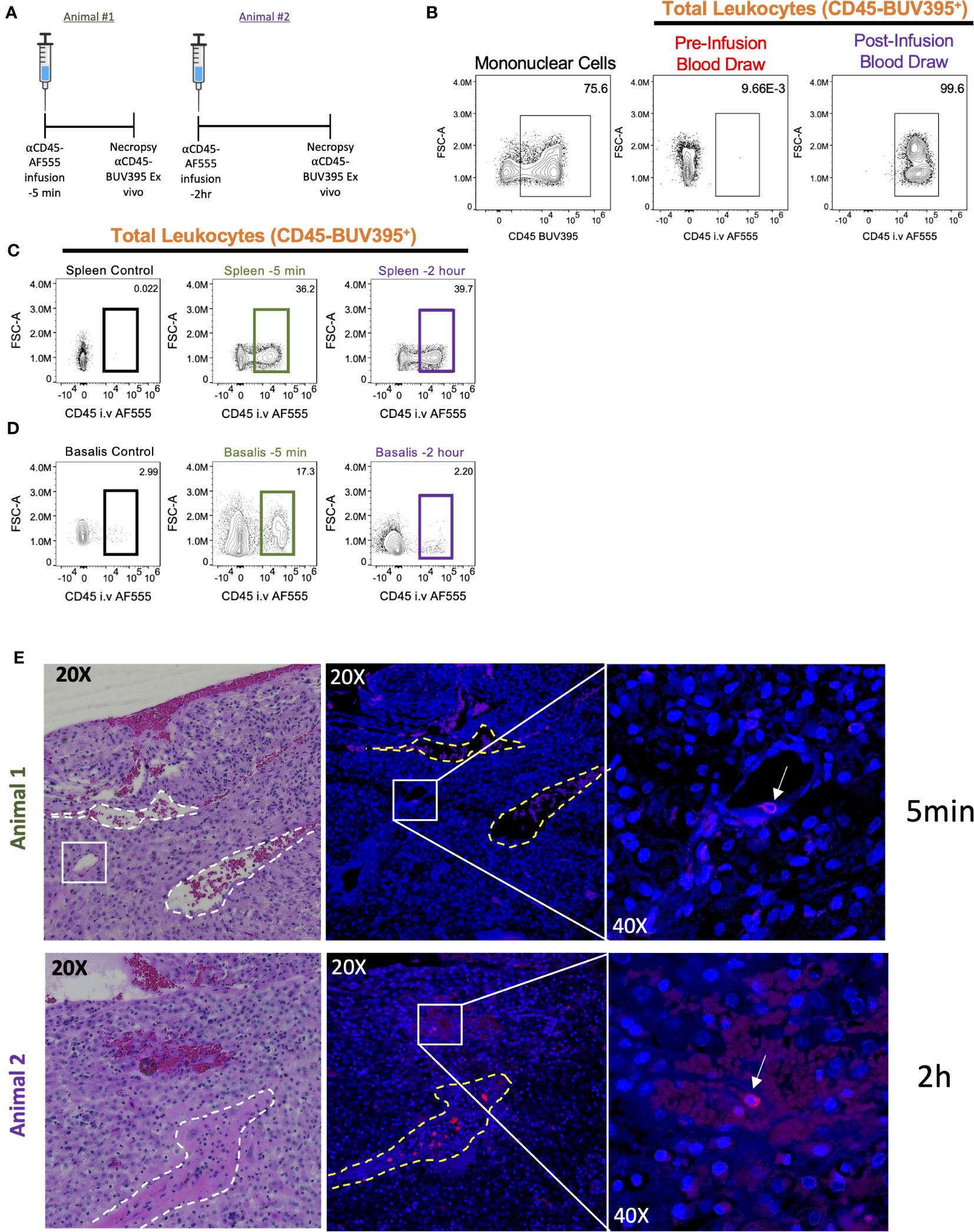
Figure 4 Intravascular staining of pregnant cynomolgus macaques. Two pregnant cynomolgus macaques were intravascularly labeled with αCD45 AF555 conjugated antibody 2 hours and 5 mins before necropsy, respectively. (A) Experimental timeline for each animal. (B) Representative gating scheme identifying total leukocytes in PBMCs before and after infusion. (C, D) Representative gating scheme identifying total leukocytes in the spleen (C) and (D) decidua. (E) Representative images of decidual sections. Serial sections from Animal 1 (top) – tissues collected 5 mins post-infusion – and Animal 2 (bottom) – tissues collected 2 hours post-infusion. H&E stained (left) section with two veins outlined (white dotted lines) and a blood vessel resembling a high endothelial venule (HEV) in white box. Fluorescent section stained with DAPI (middle) with vein outlines. Higher magnification (left) of middle panel showing a labeled leukocyte (white arrow).
3.5 Detection of architectural localization of labeled lymphocytes in cynomolgus macaque decidual sections
To explore our ability to detect architectural localization of stained lymphocytes within decidual tissue, we examined FFPE sections of this tissue from both animals. These demonstrated the presence of i.v. labeled leukocytes (Figure 4E). However, we noted that labeled leukocytes in animal #1 (infused 5 mins before necropsy) were found in the lumen of a vessel that resembles histologically a high endothelial vein, which have recently been linked to T cell trafficking in the human decidua (62). This suggests that the 5 min infusion is capturing leukocytes that are in the decidual vasculature and possibly entering the decidua stroma (Figure 4E, top left). On the other hand, we see that labeled leukocytes in animal #2 (infused 2 hours before necropsy) seem to be embedded in the decidua stroma, suggesting that these leukocytes arrived in the decidua, crossed the endothelium, and migrated into the stroma (Figure 4E, bottom left).
4 Discussion
The decidua maintains a specific immunome, which dramatically remodels across pregnancy (9, 12, 63). It has been reported, in both humans and mice, that in early pregnancy there is an abundance of dNKs, while the proportion of T cells increases in late gestation (6, 55, 56). However, limited data are available on the origin of the uterine/decidual leukocytes as well as timing of leukocyte movement through the decidua, which remain controversial. Intravascular staining is a promising tool previously used to assess the traffic of leukocytes into various tissues outside of pregnancy, in both mice (47, 48, 64) and non-human primates (45, 46). Here, we demonstrate the use of intravascular staining applied to gestation-specific trafficking of these cells in and out of murine and non-human primate decidua. First, we demonstrate the feasibility of intravascular staining in the context of murine pregnancy. Our results confirmed previously reported observations of trafficking in the murine spleen (open nature of splenic blood circulation, with majority of lymphocytes labeled) and Peyer’s patches (more restricted labeling, but open to inward and outward traffic of innate and adaptive lymphocytes) (65, 66). Next, we confirmed that the injected sub-saturating antibodies act as a pulse/chase design, with no or minimal free antibody available to bind additional leukocytes ex vivo. This also demonstrates the stability of staining of cells in vivo, with no detectable dissociation of antibody from labeled cells.
In agreement with prior studies, we find a clear contribution of circulating lymphocytes to the decidual immune compartment during pregnancy. We note a dramatic increase in the proportion of IVas+ (CC + RE) leukocytes, which, considered anatomically, implies an increased proportion of immune cells in the decidual vasculature or loosely attached to the inside of the decidual vasculature in mid to late pregnancy. While we cannot know if those cells are in the process of influx or efflux from decidual stroma based on this label alone, we note the presence of increased RI leukocytes in mid-late gestation, which argues that at least part of the CC+RE cells seen are “caught” in the act of entering the decidual compartment. This influx may contribute to decidual immune reconstruction near parturition (9, 26). Furthermore, our results are in line with the observation (24) that pregnancy orchestrates the waves of migration and residency of ILCs (including NK) subsets at different stages, and that at least a subset of lymphocytes are tissue resident.
We previously demonstrated a high level of heterogeneity among decidual immune cells driving a decidual immune signature in mouse (9) and human (7) systems. In this study, we used intravascular staining to track T cell and ILC dynamics across murine gestation. We noted the proportional decrease in IVas- (RI + TL) T cells during gestation, while CC T cells increased near parturition. These findings support the observation that CD3+ T cells and mediators are essential in creating a specific microenvironment that plays an important role in spontaneous labor at term pregnancy (55, 67, 68). Decidual NK cells (dNKs), as part of the broader ILC compartment, are the major and temporally regulated decidual inhabitants relevant to understanding pregnancy pathology. Our previous analysis of murine decidual cells demonstrated considerable heterogeneity amongst dILC subsets across pregnancy—from first trimester through term decidua (26). Like T cells, TL dILCs showed a dramatic reduction towards mid pregnancy. Interestingly, the majority of TL ILCs are NK1.1-CD49b- (ILC1-like), with this same population representing a greater proportion of RI ILCs as gestation continues (Figure 3C), suggesting that these cells are present at pregnancy initiation and are recruited as pregnancy continues. Furthermore, our clustering analysis highlighted the presence CC ILCs (Cluster 3), which increased in proportion across pregnancy, suggesting that these ILCs might be recruited near parturition in a similar fashion as T cells.
The integrins CD49a and CD103 have been identified as markers essential for resident memory T cells (TRM) maintenance and motility (69) and are expressed by tissue-resident NKs and ILC1s (52, 70). In TRM, both CD49a and CD103 are necessary for tissue retention (71, 72) and can help shape effector functions (73). Coincidentally, CD49a expression by dNKs has been linked to lower cytotoxic functions (74). TGFβ is an important factor in upregulating expression of CD49a and CD103 in both ILCs and T cells (75–77). In pregnancy, TGFβ is an important immune modulator in the decidua (78) and ex vivo treatment of peripheral NK cells with decidual stroma-derived TGFβ promotes transition to a dNK phenotype (79). This, together with our findings suggest that ILCs are recruited to the decidua and gain residency markers (CD49a and CD103) upon entrance under the influence of the unique decidual cytokine milieu.
The murine pregnancy model has the advantages of a short gestational length and significant literature and is a great tool to answer mechanistic questions using genetic manipulations. However, there are important physiological differences between murine and human pregnancy, including placental morphology and degree of placental invasiveness. The macaque represents a more faithful model of human pregnancy, sharing similar characteristics, including extensive spiral artery remodeling (80). To determine the potential of the macaque to understand how leukocytes traffic through the maternal-fetal interface, we leveraged intravascular staining, a technique recently applied in the macaque (45). Although we employed single infusions, this feasibility study shows the potential of identifying leukocytes that have recently entered the decidua as opposed to those that are resident in the stroma. This was confirmed by the spatial localization of labeled leukocytes in macaque decidua indicating temporal progression in migration of leukocytes from the decidual vasculature to deeper stroma. These findings highlight the importance of coupling flow cytometry results with imaging, when possible, as these will better inform our understanding of leukocyte trafficking in the decidua across primate pregnancy. Of course, any interpretation from serial intravascular staining experiments, both in the mouse and NHP models, should be limited to the window in which antibody infusions are done and any conclusions beyond that timepoint would need further confirmation. That is to say, assigning tissue residency to leukocytes identified as tissue localized would require more evidence (such as expression of residency markers) than that obtained simply by the lack of i.v staining.
As with most approaches, intravascular labeling has challenges. In mice, a major hurdle was the frequency of pseudopregnancies. At early gestation (GD6-8), we relied on the presence of a vaginal plug to determine whether mating had occurred. However, because pregnancy could not be confirmed this early in gestation, we had many animals assigned to the early gestation timepoint that were pseudo-pregnant. This led to the loss of animals and reagents (i.e infused antibodies) which restricted our ability to gather more replicates. In the NHP model, the major hurdle is expense. Macaques are larger thus require higher amounts of antibody to reach adequate saturation levels. Lastly, flow cytometry panel design and degree of immunophenotyping could be restricted as at least three channels need to be devoted to CD45 detection.
Overall, our data show how serial intravascular staining is a valuable tool in expanding our understanding of decidual leukocyte traffic during pregnancy. We propose that this technique will prove useful in answering outstanding questions regarding leukocyte traffic in the decidua, including transcriptional regulation dictating entrance/exit into and out of the decidua. Moving forward, similar studies can include a longer time frame between infusions and/or additional infusions to obtain better temporal resolution within waves of decidual leukocyte traffic. Future studies can also include assessment of local proliferation rates of decidual leukocytes and both chemokines and adhesion molecules involved thus providing a complete picture of leukocyte population dynamics at the maternal-fetal interface.
Data availability statement
The raw data supporting the conclusions of this article will be made available by the authors, without undue reservation.
Ethics statement
The animal study was approved by University of Wisconsin Institutional Animal Care and Use Committee. The study was conducted in accordance with the local legislation and institutional requirements.
Author contributions
JV: Conceptualization, Formal Analysis, Investigation, Methodology, Writing – original draft. MM: Writing – original draft. SB: Investigation, Writing – review & editing. LK: Investigation, Writing – review & editing. MK: Investigation, Writing – review & editing. FJ: Investigation, Writing – review & editing. RF: Investigation, Writing – review & editing. EB: Investigation, Writing – review & editing. TG: Conceptualization, Funding acquisition, Methodology, Resources, Supervision, Writing – review & editing. AS: Conceptualization, Funding acquisition, Methodology, Resources, Supervision, Writing – review & editing.
Funding
The author(s) declare financial support was received for the research, authorship, and/or publication of this article. This research was supported by NIH grants T32 HD101384 to JV, T32 GM007133 to MK, R21AI175753 to TG and AS, R01HL163623 to AS and P51 OD011106 to the Wisconsin National Primate Research Center. Additional support was provided by Wisconsin National Primate Research Center pilot grant MSN252694 to AS and TG and Burroughs Wellcome Fund Preterm Birth Initiative (1019835) to AS. The content is solely the responsibility of the authors and does not necessarily represent the official views of the NIH.
Acknowledgments
We thank the University of Wisconsin Carbone Cancer Center Flow Cytometry laboratory, supported by P30 CA014520 for use of its facilities and services for the use of the Cytek Aurora which was made possible by the National Institute of Health shared instrumentation grant 1S10OD025225-01. We thank the Wisconsin National Primate Research Center (WNPRC) Veterinary, Scientific Protocol Implementation, Pathology Service Unit, and Behavioral Management staff for assistance with animal procedures, including breeding, monitoring, surgery, and necropsy.
Conflict of interest
The authors declare that the research was conducted in the absence of any commercial or financial relationships that could be construed as a potential conflict of interest.
Publisher’s note
All claims expressed in this article are solely those of the authors and do not necessarily represent those of their affiliated organizations, or those of the publisher, the editors and the reviewers. Any product that may be evaluated in this article, or claim that may be made by its manufacturer, is not guaranteed or endorsed by the publisher.
Supplementary material
The Supplementary Material for this article can be found online at: https://www.frontiersin.org/articles/10.3389/fimmu.2023.1332943/full#supplementary-material
References
1. Ander SE, Diamond MS, Coyne CB. Immune responses at the maternal-fetal interface. Sci Immunol (2019) 4:eaat6114. doi: 10.1126/sciimmunol.aat6114
2. Robertson SA, Care AS, Moldenhauer LM. Regulatory T cells in embryo implantation and the immune response to pregnancy. J Clin Invest (2018) 128:4224–35. doi: 10.1172/JCI122182
3. Aluvihare VR, Kallikourdis M, Betz AG. Regulatory T cells mediate maternal tolerance to the fetus. Nat Immunol (2004) 5:266–71. doi: 10.1038/ni1037
4. Hanna J, Goldman-Wohl D, Hamani Y, Avraham I, Greenfield C, Natanson-Yaron S, et al. Decidual NK cells regulate key developmental processes at the human fetal-maternal interface. Nat Med (2006) 12:10. doi: 10.1038/nm1452
5. Matson BC, Caron KM. Uterine natural killer cells as modulators of the maternal-fetal vasculature. Int J Dev Biol (2014) 58:199–204. doi: 10.1387/ijdb.140032kc
6. Zhang J, Chen Z, Smith GN, Croy BA. Natural killer cell-triggered vascular transformation: maternal care before birth? Cell Mol Immunol (2011) 8:1–11. doi: 10.1038/cmi.2010.38
7. Vazquez J, Chavarria M, Li Y, Lopez GE, Stanic AK. Computational flow cytometry analysis reveals a unique immune signature of the human maternal-fetal interface. Am J Reprod Immunol (2018) 79:e12774. doi: 10.1111/aji.12774
8. Vazquez J, Chasman DA, Lopez GE, Tyler CT, Ong IM, Stanic AK. Transcriptional and functional programming of decidual innate lymphoid cells. Front Immunol (2020) 10:3065. doi: 10.3389/fimmu.2019.03065
9. Li Y, Lopez GE, Vazquez J, Sun Y, Chavarria M, Lindner PN, et al. Decidual-placental immune landscape during syngeneic murine pregnancy. Front Immunol (2018) 9:2087. doi: 10.3389/fimmu.2018.02087
10. Moström MJ, Scheef EA, Sprehe LM, Szeltner D, Tran D, Hennebold JD, et al. Immune profile of the normal maternal-fetal interface in rhesus macaques and its alteration following zika virus infection. Front Immunol (2021) 12:719810. doi: 10.3389/fimmu.2021.719810
11. Doisne J-M, Balmas E, Boulenouar S, Gaynor LM, Kieckbusch J, Gardner L, et al. Composition, development, and function of uterine innate lymphoid cells. J Immunol (2015) 195:3937–45. doi: 10.4049/jimmunol.1500689
12. van der Zwan A, van Unen V, Beyrend G, Laban S, van der Keur C, Kapsenberg HJM, et al. Visualizing dynamic changes at the maternal-fetal interface throughout human pregnancy by mass cytometry. Front Immunol (2020) 11:571300. doi: 10.3389/fimmu.2020.571300
13. Vento-Tormo R, Efremova M, Botting RA, Turco MY, Vento-Tormo M, Meyer KB, et al. Single-cell reconstruction of the early maternal–fetal interface in humans. Nature (2018) 563:347–53. doi: 10.1038/s41586-018-0698-6
14. Vargas ML, Såntos JL, Ruiz C, Montes MJ, Alemán P, García-Tortosa C, et al. Comparison of the proportions of leukocytes in early and term human decidua. Am J Reprod Immunol (1993) 29:135–40. doi: 10.1111/j.1600-0897.1993.tb00578.x
15. Williams PJ, Searle RF, Robson SC, Innes BA, Bulmer JN. Decidual leucocyte populations in early to late gestation normal human pregnancy. J Reprod Immunol (2009) 82:24–31. doi: 10.1016/j.jri.2009.08.001
16. Erlebacher A. Immunology of the maternal-fetal interface. Annu Rev Immunol (2013) 31:387–411. doi: 10.1146/annurev-immunol-032712-100003
17. DeJong CS, Maurice NJ, McCartney SA, Prlic M. Human tissue-resident memory T cells in the maternal–fetal interface. Lost soldiers or special forces? Cells (2020) 9:2699. doi: 10.3390/cells9122699
18. Nancy P, Erlebacher A. T cell behavior at the maternal-fetal interface. Int J Dev Biol (2014) 58:189–98. doi: 10.1387/ijdb.140054ae
19. Rieger L, Segerer S, Bernar T, Kapp M, Majic M, Morr A-K, et al. Specific subsets of immune cells in human decidua differ between normal pregnancy and preeclampsia - a prospective observational study. Reprod Biol Endocrinol (2009) 7:132. doi: 10.1186/1477-7827-7-132
20. Ma L, Li G, Cao G, Zhu Y, Du M, Zhao Y, et al. dNK cells facilitate the interaction between trophoblastic and endothelial cells via VEGF-C and HGF. Immunol Cell Biol (2017) 95:695–704. doi: 10.1038/icb.2017.45
21. Fraser R, Whitley GS, Johnstone AP, Host AJ, Sebire NJ, Thilaganathan B, et al. Impaired decidual natural killer cell regulation of vascular remodelling in early human pregnancies with high uterine artery resistance: NK cell regulation of vascular remodelling in pregnancy. J Pathol (2012) 228:322–32. doi: 10.1002/path.4057
22. Cerdeira AS, Rajakumar A, Royle CM, Lo A, Husain Z, Thadhani RI, et al. Conversion of peripheral blood NK cells to a decidual NK-like phenotype by a cocktail of defined factors. J Immunol (2013) 190:3939–48. doi: 10.4049/jimmunol.1202582
23. Vacca P, Vitale C, Montaldo E, Conte R, Cantoni C, Fulcheri E, et al. CD34+ hematopoietic precursors are present in human decidua and differentiate into natural killer cells upon interaction with stromal cells. Proc Natl Acad Sci (2011) 108:2402–7. doi: 10.1073/pnas.1016257108
24. Sojka DK, Yang L, Plougastel-Douglas B, Higuchi DA, Croy BA, Yokoyama WM. Cutting edge: local proliferation of uterine tissue-resident NK cells during decidualization in mice. J Immunol (2018) 201:2551–6. doi: 10.4049/jimmunol.1800651
25. Balmas E, Rana BM, Hamilton RS, Shreeve N, Kieckbusch J, Aye I, et al. Maternal group 2 innate lymphoid cells contribute to fetal growth and protection from endotoxin-induced abortion in mice. eLife (2018) 12:RP86996. doi: 10.7554/eLife.86996.1
26. Li Y, Lopez GE, Lindner PN, Parrella L, Larson M, Sun Y, et al. The role of RORγt at maternal-fetal interface during murine pregnancy. Am J Reprod Immunol (2020) 84. doi: 10.1111/aji.13250
27. Vacca P, Montaldo E, Croxatto D, Loiacono F, Canegallo F, Venturini PL, et al. Identification of diverse innate lymphoid cells in human decidua. Mucosal Immunol (2015) 8:254–64. doi: 10.1038/mi.2014.63
28. Croxatto D, Micheletti A, Montaldo E, Orecchia P, Loiacono F, Canegallo F, et al. Group 3 innate lymphoid cells regulate neutrophil migration and function in human decidua. Mucosal Immunol (2016) 9:1372–83. doi: 10.1038/mi.2016.10
29. Xu Y, Romero R, Miller D, Silva P, Panaitescu B, Theis KR, et al. Innate lymphoid cells at the human maternal-fetal interface in spontaneous preterm labor. Am J Reprod Immunol (2018) 79:e12820. doi: 10.1111/aji.12820
30. Mendes J, Rodrigues-Santos P, Areia AL, Almeida J-S, Alves V, Santos-Rosa M, et al. Type 2 and type 3 innate lymphoid cells at the maternal-fetal interface: implications in preterm birth. BMC Immunol (2021) 22:28. doi: 10.1186/s12865-021-00423-x
31. Panda SK, Colonna M. Innate lymphoid cells in mucosal immunity. Front Immunol (2019) 10:861. doi: 10.3389/fimmu.2019.00861
32. Xiong L, Nutt SL, Seillet C. Innate lymphoid cells: More than just immune cells. Front Immunol (2022) 13:1033904. doi: 10.3389/fimmu.2022.1033904
33. Miller D, Motomura K, Garcia-Flores V, Romero R, Gomez-Lopez N. Innate lymphoid cells in the maternal and fetal compartments. Front Immunol (2018) 9:2396. doi: 10.3389/fimmu.2018.02396
34. Vacca P, Vitale C, Munari E, Cassatella MA, Mingari MC, Moretta L. Human innate lymphoid cells: their functional and cellular interactions in decidua. Front Immunol (2018) 9:1897. doi: 10.3389/fimmu.2018.01897
35. Bartmann C, Segerer SE, Rieger L, Kapp M, Sütterlin M, Kämmerer U. Quantification of the predominant immune cell populations in decidua throughout human pregnancy. Am J Reprod Immunol (2014) 71:109–19. doi: 10.1111/aji.12185
36. Collins MK, Tay C-S, Erlebacher A. Dendritic cell entrapment within the pregnant uterus inhibits immune surveillance of the maternal/fetal interface in mice. J Clin Invest (2009) 119:2062–73. doi: 10.1172/JCI38714
37. Nancy P, Tagliani E, Tay C-S, Asp P, Levy DE, Erlebacher A. Chemokine gene silencing in decidual stromal cells limits T cell access to the maternal-fetal interface. Science (2012) 336:1317–21. doi: 10.1126/science.1220030
38. Liu H, Huang X, Mor G, Liao A. Epigenetic modifications working in the decidualization and endometrial receptivity. Cell Mol Life Sci (2020) 77:2091–101. doi: 10.1007/s00018-019-03395-9
39. Solders M, Kaipe H. Recruitment of MAIT cells to the intervillous space of the placenta by placenta-derived chemokines. Front Immunol (2019) 10:16. doi: 10.3389/fimmu.2019.01300
40. Hunter MC, Teijeira A, Halin C. T cell trafficking through lymphatic vessels. Front Immunol (2016) 7:613. doi: 10.3389/fimmu.2016.00613
41. Masopust D, Soerens AG. Tissue-resident T cells and other resident leukocytes. Annu Rev Immunol (2019) 37:521–46. doi: 10.1146/annurev-immunol-042617-053214
42. Rantakari P, Auvinen K, Jäppinen N, Kapraali M, Valtonen J, Karikoski M, et al. The endothelial protein PLVAP in lymphatics controls the entry of lymphocytes and antigens into lymph nodes. Nat Immunol (2015) 16:386–96. doi: 10.1038/ni.3101
43. Nieminen M, Henttinen T, Merinen M, Marttila–Ichihara F, Eriksson JE, Jalkanen S. Vimentin function in lymphocyte adhesion and transcellular migration. Nat Cell Biol (2006) 8:156–62. doi: 10.1038/ncb1355
44. Zenclussen AC, Olivieri DN, Dustin ML, Tadokoro CE. In vivo multiphoton microscopy technique to reveal the physiology of the mouse placenta. Am J Rep Immunol (2012) 68:271–8. doi: 10.1111/j.1600-0897.2012.01161.x
45. Potter EL, Gideon HP, Tkachev V, Fabozzi G, Chassiakos A, Petrovas C, et al. Measurement of leukocyte trafficking kinetics in macaques by serial intravascular staining. Sci Transl Med (2021) 13:eabb4582. doi: 10.1126/scitranslmed.abb4582
46. Mortlock RD, Wu C, Potter EL, Abraham DM, Allan DSJ, Hong SG, et al. Tissue trafficking kinetics of rhesus macaque natural killer cells measured by serial intravascular staining. Front Immunol (2022) 12:772332. doi: 10.3389/fimmu.2021.772332
47. Anderson KG, Mayer-Barber K, Sung H, Beura L, James BR, Taylor JJ, et al. Intravascular staining for discrimination of vascular and tissue leukocytes. Nat Protoc (2014) 9:209–22. doi: 10.1038/nprot.2014.005
48. Barletta KE, Cagnina RE, Wallace KL, Ramos SI, Mehrad B, Linden J. Leukocyte compartments in the mouse lung: Distinguishing between marginated, interstitial, and alveolar cells in response to injury. J Immunol Methods (2012) 375:100–10. doi: 10.1016/j.jim.2011.09.013
49. Mohr EL, Block LN, Newman CM, Stewart LM, Koenig M, Semler M, et al. Ocular and uteroplacental pathology in a macaque pregnancy with congenital Zika virus infection. PloS One (2018) 13:e0190617. doi: 10.1371/journal.pone.0190617
50. Van Gassen S, Callebaut B, Van Helden MJ, Lambrecht BN, Demeester P, Dhaene T, et al. FlowSOM: Using self-organizing maps for visualization and interpretation of cytometry data: FlowSOM. Cytometry (2015) 87:636–45. doi: 10.1002/cyto.a.22625
51. Gu Z, Eils R, Schlesner M. Complex heatmaps reveal patterns and correlations in multidimensional genomic data. Bioinformatics (2016) 32:2847–9. doi: 10.1093/bioinformatics/btw313
52. Meininger I, Carrasco A, Rao A, Soini T, Kokkinou E, Mjösberg J. Tissue-specific features of innate lymphoid cells. Trends Immunol (2020) 41:902–17. doi: 10.1016/j.it.2020.08.009
53. Lopes N, Galluso J, Escalière B, Carpentier S, Kerdiles YM, Vivier E. Tissue-specific transcriptional profiles and heterogeneity of natural killer cells and group 1 innate lymphoid cells. Cell Rep Med (2022) 3:100812. doi: 10.1016/j.xcrm.2022.100812
54. Nancy P, Siewiera J, Rizzuto G, Tagliani E, Osokine I, Manandhar P, et al. H3K27me3 dynamics dictate evolving uterine states in pregnancy and parturition. J Clin Invest (2017) 128:233–47. doi: 10.1172/JCI95937
55. Gomez-Lopez N, StLouis D, Lehr MA, Sanchez-Rodriguez EN, Arenas-Hernandez M. Immune cells in term and preterm labor. Cell Mol Immunol (2014) 11:571–81. doi: 10.1038/cmi.2014.46
56. Zhang J, Dunk C, Croy AB, Lye SJ. To serve and to protect: the role of decidual innate immune cells on human pregnancy. Cell Tissue Res (2016) 363:249–65. doi: 10.1007/s00441-015-2315-4
57. Tilburgs T, Claas FHJ, Scherjon SA. Elsevier Trophoblast Research Award Lecture: Unique Properties of Decidual T Cells and their Role in Immune Regulation during Human Pregnancy. Placenta (2010) 31:S82–6. doi: 10.1016/j.placenta.2010.01.007
58. Miller D, Gershater M, Slutsky R, Romero R, Gomez-Lopez N. Maternal and fetal T cells in term pregnancy and preterm labor. Cell Mol Immunol (2020) 17:693–704. doi: 10.1038/s41423-020-0471-2
59. Sojka DK, Plougastel-Douglas B, Yang L, Pak-Wittel MA, Artyomov MN, Ivanova Y, et al. Tissue-resident natural killer (NK) cells are cell lineages distinct from thymic and conventional splenic NK cells. eLife (2014) 3:e01659. doi: 10.7554/eLife.01659
60. Pezzotti N, Lelieveldt BPF, Maaten LVD, Hollt T, Eisemann E, Vilanova A. Approximated and user steerable tSNE for progressive visual analytics. IEEE Trans Visual Comput Graphics (2017) 23:1739–52. doi: 10.1109/TVCG.2016.2570755
61. Golos TG, Bondarenko GI, Breburda EE, Dambaeva SV, Durning M, Slukvin II. Immune and trophoblast cells at the rhesus monkey maternal-fetal interface. In: Placenta and trophoblast. New Jersey: Humana Press (2005). p. 93–108. doi: 10.1385/1-59259-989-3:93
62. Windsperger K, Vondra S, Lackner AI, Kunihs V, Haslinger P, Meinhardt G, et al. Densities of decidual high endothelial venules correlate with T-cell influx in healthy pregnancies and idiopathic recurrent pregnancy losses. Hum Reprod (2020) 35:2467–77. doi: 10.1093/humrep/deaa234
63. Bondarenko GI, Durning M, Golos TG. Immunomorphological changes in the rhesus monkey endometrium and decidua during the menstrual cycle and early pregnancy. Am J Reprod Immunol (2012) 68:309–21. doi: 10.1111/j.1600-0897.2012.01174.x
64. Patel BV, Tatham KC, Wilson MR, O’Dea KP, Takata M. In vivo compartmental analysis of leukocytes in mouse lungs. Am J Physiology-Lung Cell Mol Physiol (2015) 309:L639–52. doi: 10.1152/ajplung.00140.2015
65. Chauveau A, Pirgova G, Cheng H-W, De Martin A, Zhou FY, Wideman S, et al. Visualization of T cell migration in the spleen reveals a network of perivascular pathways that guide entry into T zones. Immunity (2020) 52:794–807.e7. doi: 10.1016/j.immuni.2020.03.010
66. Von Andrian UH, Mempel TR. Homing and cellular traffic in lymph nodes. Nat Rev Immunol (2003) 3:867–78. doi: 10.1038/nri1222
67. Gomez-Lopez N, Vega-Sanchez R, Castillo-Castrejon M, Romero R, Cubeiro-Arreola K, Vadillo-Ortega F. Evidence for a role for the adaptive immune response in human term parturition. Am J Reprod Immunol (2013) 69:212–30. doi: 10.1111/aji.12074
68. Gomez-Lopez N, Hernandez-Santiago S, Lobb AP, Olson DM, Vadillo-Ortega F. Normal and premature rupture of fetal membranes at term delivery differ in regional chemotactic activity and related chemokine/cytokine production. Reprod Sci (2013) 20:276–84. doi: 10.1177/1933719112452473
69. Mackay LK, Rahimpour A, Ma JZ, Collins N, Stock AT, Hafon M-L, et al. The developmental pathway for CD103+CD8+ tissue-resident memory T cells of skin. Nat Immunol (2013) 14:1294–301. doi: 10.1038/ni.2744
70. Vivier E, Artis D, Colonna M, Diefenbach A, Di Santo JP, Eberl G, et al. Innate lymphoid cells: 10 years on. Cell (2018) 174:1054–66. doi: 10.1016/j.cell.2018.07.017
71. Cepek K, Shaw S, Parker CM, Russell GJ, Morrow JS, Rimm DL, et al. Adhesion between epithelial cells and T lymphocytes mediated by E-cadherin and the aEB7 integrin. Nature (1994) 372:190–3. doi: 10.1038/372190a0
72. Hadley GA, Bartlett ST, Via CS, Rostapshova EA, Moainie S. The epithelial cell-specific integrin, CD103 (aEIntegrin), defines a novel subset of alloreactive CD8+CTL. J Immunol (1997) 159:3748–56. doi: 10.4049/jimmunol.159.8.3748
73. Topham DJ, Reilly EC. Tissue-resident memory CD8+ T cells: from phenotype to function. Front Immunol (2018) 9:515. doi: 10.3389/fimmu.2018.00515
74. Li H, Hou Y, Zhang S, Zhou Y, Wang D, Tao S, et al. CD49a regulates the function of human decidual natural killer cells. Am J Reprod Immunol (2019) 81:e13101. doi: 10.1111/aji.13101
75. Cortez VS, Cervantes-Barragan L, Robinette ML, Bando JK, Wang Y, Geiger TL, et al. Transforming growth factor-β Signaling guides the differentiation of innate lymphoid cells in salivary glands. Immunity (2016) 44:1127–39. doi: 10.1016/j.immuni.2016.03.007
76. Zhang N, Bevan MJ. Transforming growth factor-β Signaling controls the formation and maintenance of gut-resident memory T cells by regulating migration and retention. Immunity (2013) 39:687–96. doi: 10.1016/j.immuni.2013.08.019
77. El-Asady R, Yuan R, Liu K, Wang D, Gress RE, Lucas PJ, et al. TGF-β–dependent CD103 expression by CD8+ T cells promotes selective destruction of the host intestinal epithelium during graft-versus-host disease. J Exp Med (2005) 201:1647–57. doi: 10.1084/jem.20041044
78. Jones RL, Stoikos C, Findlay JK, Salamonsen LA. TGF-β superfamily expression and actions in the endometrium and placenta. Reproduction (2006) 132:217–32. doi: 10.1530/rep.1.01076
79. Keskin DB, Allan DSJ, Rybalov B, Andzelm MM, Stern JNH, Kopcow HD, et al. TGFβ promotes conversion of CD16+ peripheral blood NK cells into CD16- NK cells with similarities to decidual NK cells. PNAS (2007) 104:3378–83. doi: 10.1073/pnas.0611098104
Keywords: decidua, leukocytes, traffic, gestation, non-human primate, murine, T cells, ILCs
Citation: Vazquez J, Mohamed MA, Banerjee S, Keding LT, Koenig MR, Leyva Jaimes F, Fisher RC, Bove EM, Golos TG and Stanic AK (2024) Deciphering decidual leukocyte traffic with serial intravascular staining. Front. Immunol. 14:1332943. doi: 10.3389/fimmu.2023.1332943
Received: 03 November 2023; Accepted: 27 December 2023;
Published: 10 January 2024.
Edited by:
Fuyan Wang, Qingdao Hiser Hospital Affiliated of Qingdao University, ChinaReviewed by:
David Elihai Ochayon, Cincinnati Children’s Hospital Medical Center, United StatesLingling Jiang, Zhejiang University, China
Copyright © 2024 Vazquez, Mohamed, Banerjee, Keding, Koenig, Leyva Jaimes, Fisher, Bove, Golos and Stanic. This is an open-access article distributed under the terms of the Creative Commons Attribution License (CC BY). The use, distribution or reproduction in other forums is permitted, provided the original author(s) and the copyright owner(s) are credited and that the original publication in this journal is cited, in accordance with accepted academic practice. No use, distribution or reproduction is permitted which does not comply with these terms.
*Correspondence: Aleksandar K. Stanic, c3Rhbmlja29zdGljQHdpc2MuZWR1