- 1Department of Dermatology & Sexually Transmitted Disease (STD), the Affiliated Hospital of Southwest Medical University, Luzhou, Sichuan, China
- 2Department of Dermatology & Sexually Transmitted Disease (STD), Chengdu First People’s Hospital, Chengdu, Sichuan, China
- 3Health Management Center, Luzhou People’s Hospital, Luzhou, China
Atopic dermatitis (AD) is a common chronic relapsing inflammatory skin disease, of which the pathogenesis is a complex interplay between genetics and environment. Although the exact mechanisms of the disease pathogenesis remain unclear, the immune dysregulation primarily involving the Th2 inflammatory pathway and accompanied with an imbalance of multiple immune cells is considered as one of the critical etiologies of AD. Tryptophan metabolism has long been firmly established as a key regulator of immune cells and then affect the occurrence and development of many immune and inflammatory diseases. But the relationship between tryptophan metabolism and the pathogenesis of AD has not been profoundly discussed throughout the literatures. Therefore, this review is conducted to discuss the relationship between tryptophan metabolism and the complex network of skin inflammatory response in AD, which is important to elucidate its complex pathophysiological mechanisms, and then lead to the development of new therapeutic strategies and drugs for the treatment of this frequently relapsing disease.
1 Atopic dermatitis
Atopic dermatitis (AD) is a chronic inflammatory skin disease that results from a complex interplay of genetics, environment, and immunity, which is characterized by intense itching, recurrent eczema lesions, and a personal or family history of atopy (1). The incidence of AD is gradually increasing with the development of the current industrialization and urbanization, affecting up to 15-30% of children and 10% of adults worldwide (2, 3). Even worse, approximately 50-70% of children with AD are at risk of developing other atopic diseases such as allergic asthma and/or allergic rhinitis in the future (4). At present, researchers believe that immune disorders play a crucial role in the pathogenesis of AD. T helper type 2 (Th2) and Th17-mediated immune disorders dominates the acute stage and chronic stages of AD respectively (5), both of them “interweave” each other to form a complex network of skin inflammation. Among them, the Th2 type immune response has attracted widespread attention and exploration. In relevant studies on the pathogenesis of AD, the pathogenic role of keratinocyte derived cytokines such as thymic stromal lymphopoietin (TSLP), interleukin-33 (IL-33) and IL-25 in inducing skin inflammation has been emphasized (6). These cytokines can promote the production of Th2 type cytokines such as IL-4, IL-5, and IL-13. Notably, IL-4 and IL-13 have previously been reported to damage the integrity of the skin barrier by inhibiting the production of key proteins such as filaggrin (Flg) and disrupting the stability of tight junctions (7, 8), leading to increased penetration of allergens and pathogens. The downstream signal transduction of both also blocks the expression of innate immune response genes such as β-defensins (7, 9), increasing the risk of skin infection with Staphylococcus aureus in AD patients. Meanwhile, IL-4 and IL-13 can drive the regeneration of eosinophils and mast cells, as well as stimulate the secretion of key cytokines IL-31 in pruritus stimulation (10). The repeated scratching behavior leads to further physical damage to the skin barrier, thereby forming a “vicious cycle” that exacerbates AD skin lesions. In addition, congenital skin barrier dysfunction and disruption of the microbiota in the skin and intestines are also considered the main contributors in driving the development of AD (11). However, the exact mechanism of AD pathogenesis mediated by intestinal microbiota is still not fully elucidated, which is presumed to be associated with tryptophan (Trp) metabolism.
Long-term itching symptoms and recurrent episodes of disease could severely affect the life quality of AD patients, and are closely related to the occurrence of negative psychology such as anxiety and depression (12). But, unfortunately, treating AD is challenging due to the high heterogeneity of the disease (13) and the limited therapeutic drugs (14). In traditional treatment regimens, topical corticosteroids are a classic first-line medication, although they could produce anti-inflammatory and immunosuppressive effects by inhibiting various inflammatory cells and cytokines, long term use may be limited by the side effects such as skin atrophy (15). The highly individualized selection of immunosuppressive drugs reduces the scope of application of the drugs and patient compliance. As an emerging treatment for AD, biological agents seem to be effective alternatives to traditional treatment. In 2017, dupilumab, a fully monoclonal antibody targeting IL-4R, became the first biological agent approved for use in adult patients in the United States due to its significant improvement in AD clinical manifestations. However, a higher incidence of herpes simplex virus infection and conjunctivitis was found in patients receiving dupilumab. Among other newly developed monoclonal antibodies, including Lebrikizumab and Tralokinumab combined with IL-13, Nemolizumab inhibiting IL-31, and Fezakinumab blocking the action of IL-22, various degrees of herpes infection, conjunctivitis, viral upper respiratory tract infection, peripheral edema, and elevated creatinine kinase levels have been found after treatment (16). Recent studies have shown that the activation of the JAK (Janus kinase)- STAT (signal transducer and activator of transcription) signaling pathway is essential for mediating downstream inflammatory cytokines in AD patients, including IL-4, IL-5, IL-13, IL-31, IL-22, and TSLP (17). These cytokines bind to immune cells, keratinocytes, and peripheral sensory neurons, leading to the spread of inflammation and itching symptoms in AD patients. Therefore, JAK inhibitors have become a new treatment strategy for AD because of immunosuppressive effects. Currently, commonly used JAK inhibitors include oral Baricinib targeting JAK1 and 2, and Upadacitinib targeting JAK1, as well as topical Tofacitinib targeting JAK1 and 3, Ruxolitinib targeting JAK1 and 2, and Delgocitinib targeting the entire JAK pathway. However, it is worth noting that, although JAK inhibitors could improve the severity of the condition and itching symptoms in AD patients, they also have the risk of increasing blood creatine phosphokinase and inducing headaches and nasopharyngitis (18). The long-term effectiveness and safety of these developing biological therapies are not yet fully understood, and with the continuous emergence of new therapies for AD, the comparison of drugs is crucial for patients to choose safe and effective treatment plans. Hence, in-depth study of the mechanism and looking for new potential treatment of AD is still valuable. Trp metabolism has recently been found to have a potential connection with the regulation of AD immune system, and greatly affect the development of other immune diseases such as metabolic syndrome, neuropsychiatric disorders and inflammatory bowel disease (19). However, throughout the literatures, the role of tryptophan metabolism in the pathogenesis of AD has not been well discussed. This article reviews the potential role of tryptophan metabolism in regulating the complex network of skin inflammatory response in AD, in order to enhance understanding of the occurrence and development of AD and seek treatment opportunities targeting tryptophan metabolism.
2 Tryptophan metabolism and its’ role on regulating skin diseases by aryl hydrocarbon receptor
Tryptophan is an essential amino acid for the human body that is mainly produced by high-protein foods such as milk, seafood, grains and peanuts, which is a biosynthetic precursor of a large number of metabolites (19). As an important intestinal metabolite, Trp metabolism in the intestine follows three main pathways (20) under homeostatic conditions: (i) the Kynurenine (Kyn) pathway (KP) via indoleamine 2,3-dioxygenase 1 (IDO1) in immune cells (mainly macrophages) and intestinal epithelial cells (IEC); (ii) the serotonin pathway via Trp hydroxylase 1 (TpH1) in enterochromaffin cells. Above two pathways mainly occur in host cells, that are predominantly but not exclusively used by the host. (iii) The direct conversion of Trp into indole, indole derivatives, and tryptamine by the intestinal microbiota via the enzyme tryptophanase (21), which produces metabolites such as indole acetic acid (IAA), indole‐3‐acetaldehyde (IAAld), indole‐3‐aldehyde (IAld), indole propionic acid (IPA) and indoleacrylic acid (IA) (Figure 1). Trp metabolites mainly exists in human feces and have been shown to maintain the integrity of the skin and intestinal barrier and immune cell homeostasis by activating aryl hydrocarbon receptor (AhR) (22, 23), thus are considered as the active biomarkers (24).
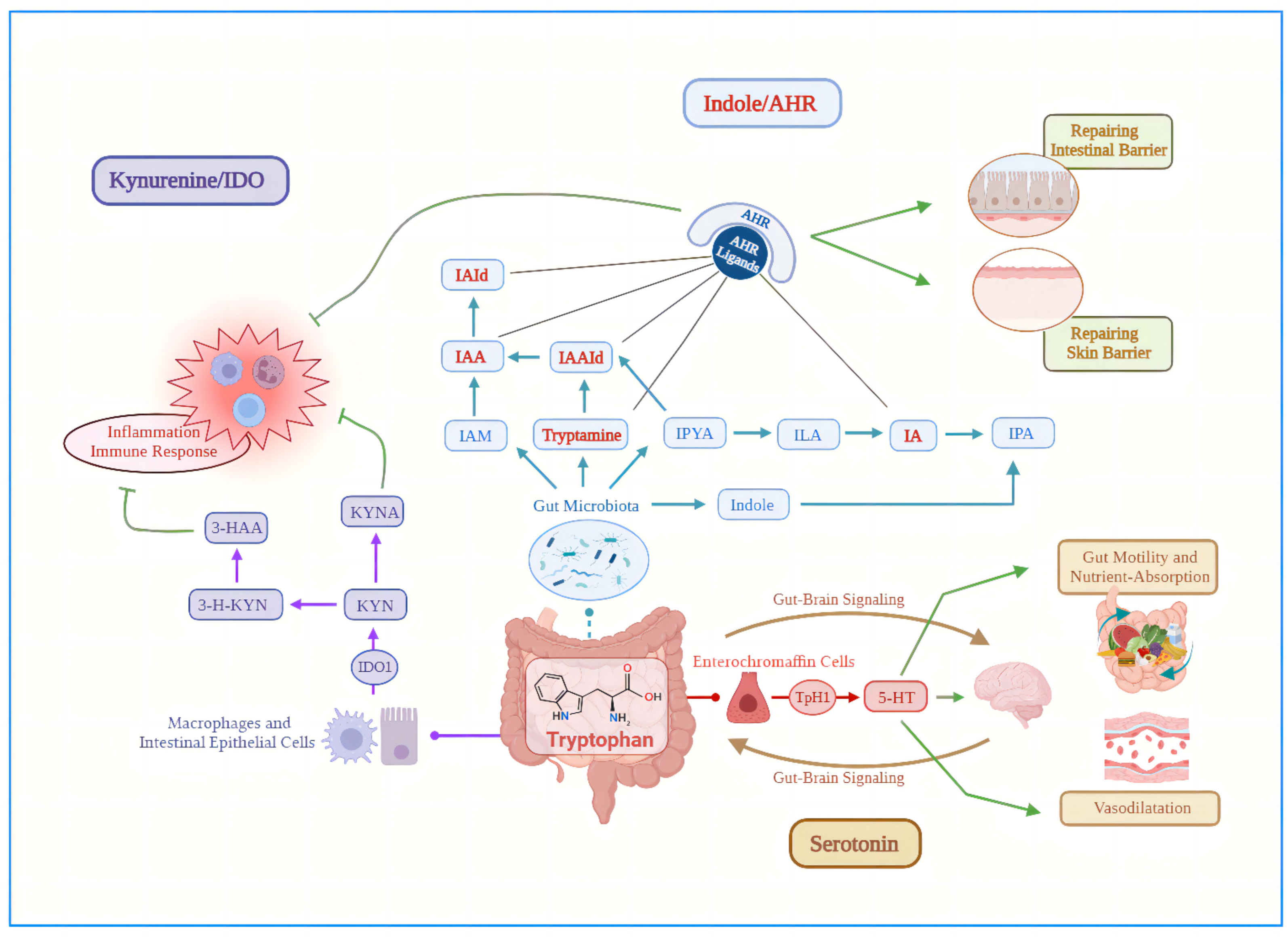
Figure 1 Three main pathways of tryptophan metabolism in the intestine under steady-state conditions. (i) Purple arrows: the Kynurenine pathway via indoleamine 2,3-dioxygenase 1 in macrophages and intestinal epithelial cells; (ii) Blue arrows: the direct conversion of Trp into indole, indole derivatives, and tryptamine by the intestinal microbiota via the enzyme tryptophanase; (iii) Red arrows: the serotonin pathway via Trp hydroxylase 1 in enterochromaffin cells. Green lines, inhibitory action; Green arrows, promotional effect; AHR, aryl hydrocarbon receptor; IA, indoleacrylic acid; IAA, indole acetic acid; IAAId, indole‐3‐acetaldehyde; IAId, indole‐3‐aldehyde; IAM, indole-3-acetamide; IDO1, indoleamine 2,3-dioxygenase 1; ILA, indole-3-lactic acid; IPA, indole propionic acid; IPYA, Indole pyruvic acid; KYN, Kynurenine; KYNA, kynurenic acid; TpH1, Trp hydroxylase 1; 3-HAA, 3-Hydroxy-Anthranilic acid; 3-H-KYN, 3-Hydroxy-Kynurenine; 5-HT, 5-hydroxytryptamine.
AhR is a ligand dependent transcription factor that could be observed in all skin cells. Although the expression levels vary in specific cell types (25), AhR signaling plays a major role in skin diseases due to its involvement in many important physiology processes such as regulating immune cells, maintaining redox balance in cells and epidermal barrier function (25–27). As a hybrid receptor, AhR could be activated by a variety of exogenous and endogenous ligands (28–31), among them, the mild and transient AhR activation caused by antioxidant phytochemicals or some tryptophan derivatives could effectively maintain healthy and complete skin barrier (32, 33). When tryptophan derivative binds to the AhR ligand to form a complex, AhR dissociates from the cytoplasm and translocates to the nucleus. The AhR nuclear translocation protein (ARNT) dimerizes with the exposed nuclear translocation site of AhR, binding to be the upregulated target gene transcription response element (34). This signaling pathway initiates the activation of the OVO-like 1 (OVOL 1) transcription factor, and subsequently enhances the expression of Flg and olein (LOR), these kind of terminal differentiation proteins have specificity for fully differentiated keratinocytes (35), helping to accelerate the final differentiation of the epidermis and the formation of the epidermal barrier (36, 37). Notably, increasing evidences suggest that the binding of tryptophan derivatives with AhR play a role in the pathogenesis or treatment of many skin diseases (38, 39), including inflammatory diseases, skin pigmentation diseases, and cancer (40, 41). As expected, tryptophan metabolism has been reported to be closely related to the occurrence and development of AD (42), the tryptophan metabolite in the AD skin lesions is significantly reduced (43) that may be associated with the weakened tryptophan metabolism in the skin microbiota by Th2 type immune response (43). Due to AhR lacking physiological ligands in the Th2-deviated environment of AD, the skin barrier damage and colonization of pathogenic microorganisms such as Staphylococcus aureus would increase, thereby exacerbating Th2 type inflammation in the lesion of AD (44, 45). Therefore, we speculate that tryptophan and its derivatives could be beneficial for the treatment of AD by appropriately activating the AhR/ARNT axis.
Tryptophan has recently been also implicated in the crosstalk between gut microbiota (GM) and host in healthy and diseased states (19, 46), and its metabolic impairment may affect the occurrence and development of many diseases, such as metabolic syndrome, neuropsychiatric disorders and inflammatory bowel disease (19). Research findings showed that, compared with the healthy control, inflammatory bowel disease (IBD) patients experienced intestinal microbiota homeostasis imbalance, while their serum tryptophan levels also significantly decreased. Interestingly, the composition of GM is significantly correlated with serum level of tryptophan, dietary supplementation with Trp can normalize the disordered GM in the IBD mouse model (47), and the AhR ligands produced subsequently by GM could alleviate the inflammatory response (48). Wilck et al. found that, Lactobacillus murinus and Lactobacillus reuteri could convert tryptophan into IAld and indole-3-lactic acid (ILA) by aromatic amino acid aminotransferase and indole lactic acid dehydrogenase (49, 50), these generator of AhR would improve the severity of colitis in mice (23). This type of approach can even be expanded to other inflammatory diseases (51), suggesting that the biological effects of Trp metabolites may be related to the interactions between gut microbiota and may become potential therapeutic targets for certain diseases. Additionally, Metghalchi et al. found that plasma level of Trp predicts the likelihood of adverse cardiovascular events in patients with acute myocardial infarction (52). Based on the characteristics of Trp metabolism influenced by pathological conditions, the use of Trp and its metabolites as biomarkers to support diagnosis and prognosis and to guide treatment options is attractive. Therefore, this article will focus on exploring the potential diagnostic and therapeutic role of tryptophan metabolism in the complex pathogenesis of AD.
3 The potential role of tryptophan metabolism in atopic diseases
AD typically begins in early childhood and is usually the first manifestation of atopic progression. While clinical symptoms of some AD children would disappear with age, some children may experience food allergy during the course of the disease, even half of AD children may develop allergic asthma and two-thirds of AD children are at risk of developing allergic rhinitis in the future. This kind of disease progression is known as the “Atopic March” (53, 54). Exploring the relationship between these atopic diseases and tryptophan can help us further understand its role in AD.
3.1 Food allergy
Although people often refer to any adverse reaction to food as an allergy, strictly speaking, food allergy (FA) is an adverse reaction to the food itself that is mediated by specific IgE antibody. Increased levels of tryptophan and indole metabolic pathway products were found in serum samples from FA children, while levels of metabolites from Kynurenine and 5-hydroxytryptamine pathways decreased with disease burden (55, 56). However, these tryptophan metabolites can downregulate the T cells activity or induce various regulatory T cell (Treg) cells to regulate immune responses at the mucosal barrier (55, 57). The reduction of tryptophan metabolites with potential anti-inflammatory effects would create a suitable environment for Th2 tilted immune responses, thereby increasing the occurrence risk of Th2 related diseases such as AD. Another study on peanut allergic mice suggests that the above mechanism is related to the activation of AhR (58, 59), but to our knowledge, there are currently no clinical studies evaluating the functional role of AhR ligands in FA.
3.2 Allergic rhinitis and asthma
Atopic diseases are highly prevalent in children worldwide, with the prevalence rates of allergic rhinitis (60, 61) and asthma (62) being approximately 14% and 15%, respectively. Consistent with food allergy, levels of tryptophan are significantly elevated in patients with allergic rhinitis and asthma (55, 63–66). Serum tryptophan levels could not only be used to distinguish between stable asthma children and healthy children, but also to distinguish between controlled and uncontrolled asthma patients (64). This kind of influence could even be traced back to the early stages of life, the tryptophan metabolite 5-hydroxyindolepyruvate in maternal amniotic fluid can determine whether the baby has wheezing attacks in the first year of life (65). The above evidences suggest the important value of tryptophan in atopic related diseases. Some researchers believe that, the high level of tryptophan in the serum of patients with atopic diseases may be related to the low activity of IDO-1 enzyme or the inhibition of IDO-2 enzyme activity (66, 67). Among them, IDO-1 is widely expressed in tumor cells and inflammatory/antigen presenting cells (APCs), induces the production of Kyn by catabolizing tryptophan, the ratio of serum Kyn to tryptophan reflects IDO-1 activity to some extent (68, 69). Kyn could up-regulate the expression of foxp3 transcription factor, increase the differentiation of Treg cells and decrease the expansion of Th17 type cells (67, 70–72). For instance, Th17 and Th1 type airway inflammation were observed in IDO deficient mice infected with the virus, characterized by significant infiltration of neutrophil, high production of IL-17 and interferon-γ (IFN-γ) and obvious airway responsiveness (73). In the asthma mouse model, activation of toll-like receptors (TLR)-9 through bacterial DNA motifs could induce the expression of IDO (74) and aromatic receptor (75, 76) to reduce the inflammatory airway hyperresponsiveness. Therefore, although the specific role of tryptophan metabolism in the pathogenesis of atopic diseases such as asthma and allergic rhinitis is still not fully understood, it cannot be denied that tryptophan metabolism and its intermediate products are the important links in regulating immune responses (19). According to research reports, administration of D-tryptophan before experimental asthma induction in mice observed an increased number of regulatory T cells in the lungs, a decreased Th2 type immune response, and an improvement in allergic airway inflammation and airway hyperresponsiveness (77). Another tryptophan metabolite, 3-Hydroxy-Anthranilic acid (3-HAA), could directly target the phosphorylation of 3-phosphoinositol dependent kinase 1 (PDK 1) to inhibit NF-κB triggered by T cell antigen receptors, leads to dysfunction and cell death of activated TH2 cells in the body, which is sufficient to suppress experimental asthma induced by TH2 immune response in mice (78). In addition, PapaMichael et al. (79) also reported a positive correlation between the tryptophan metabolite 5-hydroxyindoleacetic acid (5-HIAA) and the asthma FEV 1/FVC (forced expiratory volume in the first second/forced vital capacity) ratio, as well as a negative correlation with fractional exhaled nitric oxide (FeNO). However, further research is needed to demonstrate the therapeutic potential of tryptophan and its metabolites in patients.
In summary, the atopic diseases such as food allergy, asthma, and allergic rhinitis all have varying degrees of tryptophan metabolism abnormalities, which may even affect early immune homeostasis. The use of tryptophan metabolites as biomarkers to support the diagnosis and prognosis of atopic diseases and guide treatment choices is an attractive choice. However, despite the extensive research supporting the important role of tryptophan metabolism in the occurrence and development of atopic related diseases, the mechanism connections between these diseases are still unclear (80), the specific mechanisms of action and therapeutic potential still need to be further explored.
4 Trp metabolism as a bridge between atopic dermatitis and gut microbiota
4.1 Gut microbiota and its metabolites contribute to the development and prognosis of AD
GM is an enormous and complex ecosystem. The number of bacterial cells within the human gut outnumber the host’s cells by 10 times and the genes encoded by these bacteria even exceeds their host’s genes by more than 100 times (81). Consequently, extensive research has been conducted on the gut microbiota and its role in the various diseases and health states. It is reported that the imbalance in the diversity and composition of GM could lead to negative changes in intestinal microbial metabolism and immune response, such as affecting the host’s intestinal immune environment and barrier function, disrupting mucosal immune tolerance, and increasing the vulnerability of the host (82, 83). Actually, the connection between GM and skin inflammation was discovered as early as the 1930s (84). Up to now, numerous studies have revealed that the development of allergic diseases such as asthma, allergic rhinitis and AD is closely associated with GM disturbance (85–91). Although there are some conflicting results (92–95), lots of clinical trials have claimed that AD patients exhibit poor gut microbial diversity compared to healthy individuals (96–99), as well as a structural disorder in the gut microbiota, which includes the increased abundance of microorganisms related to inflammation and epithelial damage in the intestinal flora, such as Clostridium difficile, Coliform, pathogenic Escherichia coli, and Staphylococcus aureus (93, 97, 100–104), and the significantly decreased abundance of SCFA (short chain fatty acid) producers, such as Bifidobacteria, Fecal cocci and Fecal bacilli (92, 95, 96, 103, 105–108). Notably, SCFAs, including acetic acid, propionic acid, and butyric acid, have been proven to help maintain the balance of GM, affect immune cells, and are closely related to the remission of clinical manifestations of AD (96, 109, 110). SCFAs can bind G-Protein-Coupled Receptors (GPCRs, including GPR43, GPR41, and GPR109a) expressed on intestinal epithelial and immune cells to inhibit histone deacetylase (HDAC) (111, 112), leading to acetylation of the Foxp3 coding region in T cells, driving Treg differentiation (113, 114), downregulating expression of inflammatory cytokines (IL-6, IL-8, and tumor necrosis factor -α (TNF-α)) (115, 116), and stimulating the production of anti-inflammatory cytokines such as IL-10 by peripheral blood mononuclear cells (PBMCs) (117) which could suppresses pro-inflammatory types of Th17 and Th2 cells in turn. Therefore, intervention targeted GM and its metabolites may be an alternative method for controlling the inflammationary responses and improving the clinical symptoms of AD.
4.2 Probiotics alleviate the inflammatory response in AD by up-regulating tryptophan metabolism
In addition to SCFA, another prominent example of how microbiota affects host tissue level immune maturation is the probiotic metabolic pathway of tryptophan. As the important regulator of GM, probiotics could improve the clinical severity of patients by the reduction of pro-inflammatory cytokines such as IL-13 and IL-5 in AD skin lesions (118). It has been revealed that IL-13 is a key driver of activating Th2 type immune responses, and IL-15 is a key cytokine inducing development and survival of eosinophil (119). Thus, probiotics is expected to become an effective alternative strategy for the treatment of skin diseases based on the enormous potential in regulating immune function (120, 121). It is worth mentioning that, current research has also confirmed a close link between probiotics and tryptophan metabolism. For instance, the application of Lactobacillus reuteri (122), Lactobacillus salivary (123), and Bifidobacterium (124) significantly increased the level of tryptophan metabolites in the serum, and even accompanied by the decreased pro-inflammatory response. In fact, tryptophan, as an important regulator of mammalian inflammatory response (125), has been proven to have an immunomodulatory effect in experimental colitis or IBD patients (126). The GM obtained from IBD patients showed poor ability to produce AhR ligands (23), and in addition, sterile mice susceptible to colitis observed significant improvement in intestinal inflammation after supplementation with AhR agonists and Lactobacillus strains capable of metabolizing tryptophan (22). Similarly, administration of Bifidobacterium in AD model mice showed reduced scratching behavior and an increase in the level of Kyn (127). Therefore, considering the role of the beneficial microbiomes in tryptophan metabolism, we speculate that increasing intestinal probiotics may medicate the immune inflammatory responses and alleviate clinical symptoms of AD by producing tryptophan derivatives.
4.3 Tryptophan metabolites promote the regression of skin inflammation in AD by repairing the intestinal barrier
It has been reported that, Trp metabolism and its derivatives have many beneficial effects on intestinal epithelial barrier function mediated by GM. The intestinal barrier is made up of epithelial cells, mucous layers, T cells, IgA, and dendritic cells, collectively forming the “mucosal firewall” (128). The imbalance of GM, decreased production of SCFA, and loss of immune tolerance in the intestines of AD results in the occurrence of inflammatory reactions, increased pathogenic microorganisms and damaged intestinal barrier (92). This kind of “leaky gut” paves the way for the occurrence of AD skin inflammation by releasing toxins, food residues and pathogenic microorganisms from the damaged intestinal epithelium into the systemic circulation, inducing the release of pro-inflammatory cytokines to propel Th2 type immune responses ultimately (129–131). Nevertheless, Trp metabolites such as indole can reinforce intestinal epithelial barrier function by enhancing the expression of genes involved in preserving epithelial cell structure and function in vivo and in vitro (132, 133), IA (134) and IPA (134, 135) can enhance intestinal epithelial barrier function by reducing the expression of inflammatory factors in intestinal epithelial cells via activating the AhR and PXR (pregnenolone X receptor) receptors, respectively, to promote intestinal goblet cells differentiation and mucus production. Thus, it is supposed that the supplementation of tryptophan metabolites will play a beneficial role in AD through repairing the intestinal epithelial barrier function and consequently controlling “leaky gut” induced skin inflammation.
5 Tryptophan metabolites regulate the complex immune response in AD
Although the exact mechanisms of the disease pathogenesis remain unclear, accumulating evidence from experimental, genetic, and clinical studies indicates that impaired skin barrier and the immune dysregulation are the critical etiologies of AD (11). Although AD has long been considered to be Th2-dominated inflammation, it is also evident that its pathology is accompanied by an imbalance in immunity involving both innate immune cells such as keratinocytes, macrophages, dendritic cells, and adaptive immune cells such as Th1, Th2, Th17, Th22 and Treg cells, which interact and eventually intertwine into a complex inflammatory network. On the other hand, tryptophan metabolism has been firmly established as a key regulator of both innate and adaptive immune cells (68, 136), and its derivative receptor, AhR, is a key component of the immune response at the barrier site. Alterations in AhR activity or AhR deficiency may disrupt the immune response or impair the development and function of the epidermal barrier (137). Next, we will delve into the innate and adaptive immune mechanisms mediated by the tryptophan metabolites in the pathological process of AD.
5.1 Keratinocytes
Keratinocytes represent the first line of the host defense system by sensing pathogens via innate immune receptors, initiating antimicrobial responses and producing various cytokines and antimicrobial peptides. Among the dysregulation of immune responses in AD, keratinocytes initiate cross-talk between innate and adaptive immune responses by regulating the release of several key molecules including eotaxin/CC chemokine ligand (CCL) 11, eotaxin‐3/CCL26, monocyte chemotactic protein (MCP)‐4/CCL13, regulated on activation, normal T cell‐expressed and secreted (RANTES)/CCL5, and thymus and activation‐regulated chemokine (TARC)/CCL17, that trigger inflammatory reactions and immune responses (138). It was found that Th2 type cytokine IL-4/IL-13 could stimulate the production of IL-24 in Keratinocyte by inducing the activity of STAT 6 (139), and then reduce the expression of Flg, ultimately leading to epidermal terminal differentiation damage and barrier dysfunction in AD patients. Interestingly, AhR ligands, such as Coal tar, Glyteer and 6-formylindolo[3,2-b]carbazole (FICZ), could block the activation of STAT 6 mediated by IL-4/IL-13 and promote the expression of Flg via mild and transient activation of AhR/ARNT pathway, thus restoring barrier dysfunction (140, 141). Further experiments have proved that the defect of AhR in Keratinocyte may be the cause of worsening inflammation (142), while intense and sustained AhR activation would lead to excessive keratinization of Keratinocyte and sebocytes (43). In conclusion, the lack of AhR or the change of its activity is related to the imbalance of Keratinocyte ‘response to inflammatory stimuli, which may damage the immune response or the normal function of the epidermal barrier.
5.2 Macrophages
Macrophages (Mφ), as a natural immune cell, differentiate into two phenotypes, including the M1 phenotype activated by TLR ligands to secrete proinflammatory cytokines such as TNF-α and IL-1β, or the M2 phenotype activated by interferon or lipopolysaccharide (LPS) to secrete the anti-inflammatory cytokine such as IL-10 (143), and respond to immune responses in the AD microenvironment under different activation states. Remarkably, M2 macrophages exert immunosuppressive effects by expressing IDO to depresses T cell proliferation and stimulate regulatory T cells, which are cells with immune suppressive function (144, 145). Tryptophan metabolism has been found to affect the polarization and immune function regulation of macrophages. 5-methoxyltryptophan (5-MTP), a new endothelial factor produced by L-tryptophan metabolism that has recently been identified as a functional feature with protective and repair barriers (146), could inhibit the LPS-induced p38-MAPK pathway by interfering the binding of phosphor-p38 to peroxiredoxins (Prdx), thereby blocking the activation of macrophages and preventing the occurrence of systemic inflammation. Similarly, for other tryptophan metabolites, I3A could reduce the expression of inflammatory cytokine IL-1qwβ, TNF-α and CXCL-1 in macrophages (146); IA could stimulate IL-10 production in macrophages, thereby reducing the secretion of TNF and IL-6 (115); Kyna was later confirmed to reduce the inflammatory response induced by LPS stimulation in monocytes and Mφ through its interaction with GPR35 (147). Therefore, these findings support the idea that tryptophan metabolism could effectively control macrophage activation and “cytokine storm” in vivo, but the specific mechanism of action in AD needs further research.
5.3 Dendritic cells
As early as the 1970s, dendriticcells (DCs) has been known to coordinate immune responses by building a “bridge” between innate and adaptive immunity, while the normal physiological function of DCs could be severely affected under pathological condition, and results in the occurrence of abnormal immune responses subsequently. For instance, there are various inflammatory signals in the AD microenvironment, exposure of DCs to this immune stimulation environment would upregulate the expression of inflammatory mediators during antigen acquisition, and activate effector T cells via treated antigen peptides simultaneously (148). Despite not being the case for all DCs (149), exposure to stimuli such as IFN, TLR-4, TLR-9, TNF, and IL-1 could induce functional IDO expression in DCs, promoting tolerance in vivo and contributing to their ability to present antigens and stimulate T cells (150) either through effects of IDO on DCs or through direct action on T cells mediated by tryptophan depletion or tryptophan metabolites (151, 152). A previous study proposed initially that CD4+naïve treated T cells could transformed into FoxP 3+functional regulatory T cells upon exposure to LT/Kynurenine or IDO+ DCs (153). Additionally, the tryptophan metabolite IPA promotes the accumulation of anti-inflammatory DCs in the mesenteric lymph nodes, which was abolished by AhR antagonist (154). IPYA inhibits colon inflammation by increasing IL-10 production, reducing Th1 cell differentiation in the lamina propria of the colon, and altering the composition of DCs in the mesenteric lymph nodes (154). Therefore, we believe that the tryptophan metabolism mediated by DCs has a positive inflammatory regulatory potential in AD.
5.4 Th2 cells
The strong activation of adaptive immunity driven by Th2 cells seems to be the dominant mechanism in the acute phase of AD (155), mainly characterized by the secretion of cytokines such as IL-4, IL-5, IL-13 and IL-31, which are involved in the occurrence of keratinocyte apoptosis, inflammation and itching symptoms, to confirm the influence of T cells in the pathogenesis of AD. Previous studies have shown that tryptophan and its derivative ligand, AhR, are involved in regulating T cell populations, mediating immunosuppression, and maintaining the balance between Treg and T cells. A recent study has demonstrated that Th2-deviated environment is related to the reduction of the endogenous AhR ligand such as indole-3-aldehyde (IAId) produced by the skin symbiotic microbiota (43). IAId could promote the interaction of activated AhR receptors with TSLP, thereby inhibit the production of TSLP, and ultimately reduce the occurrence of AD-like dermatitis (43). Notably, TSLP, mainly produced by epidermal keratinocytes and fibroblasts, could stimulate Th2 differentiation by promoting the migration of DCs to the epidermis (156), playing a central role in initiating Th2 type adaptive immune responses in AD skin inflammation. In addition to IAId, significant alleviation in clinical manifestations and reversal of Th2 biased immune response were also observed after supplementation with D-tryptophan in a mice with allergic airway inflammation (77). D-tryptophan is a metabolite of Bifidobacteria, Lactobacillus and Lactococcus, strongly induces the production of anti-inflammatory factor IL-10 and reduces the secretion of IFN‐γ,IL‐12, and IL‐5 in LPS‐induced KM‐H2 (a human Hodgkin’s disease cell line) cultures, and suppress the expression of the crucial chemokine CCL17 responsible for recruitment of Th2 cells in AD skin lesions (77, 141). A newly‐identified role of D‐tryptophan consists of slowing down the production of biofilm formation in Staphylococcus aureus and Pseudomonas aeruginosa (157, 158). As is known, the skin of AD patients is more susceptible to the colonization and overgrowth of Staphylococcus aureus (159), which has been linked to the increased IgE responses and severity of AD disease (160). In addition, Yu et al. found that appropriate activation of the AhR/ARNT/Flg axis may be beneficial in treating AD (43, 161). Th2-deviated environment could significantly reduce the expression of filaments and other barrier related molecules, while the activation of the AhR/ARNT/Flg signaling pathway by rapidly metabolized AhR ligands (such as IAId or FICZ) and dioxins (2, 162) would initiate the activation of OVOL 1 transcription factors, enhance the expression of Flg and LOR, thereby contributing to accelerating the final differentiation of the epidermis and the formation of the epidermal barrier (25, 35). It should be mentioned that, FICZ, as an endogenous UVB photoproduct (28), has been found to be closely related to human skin physiology (163) that could limit the production of IL-17 and IL-22 in mouse dermatitis models by activating the AhR receptor (142, 161), this barrier-protecting effect may partially explain why UVB phototherapy is effective in treating AD and psoriasis (164, 165). Therefore, the reduction of filaments and other barrier related molecules in the Th2-deviated environment, as well as the deficiency of AhR ligands, may underlie the skin lesions in AD (117), which may compensate for the up-regulation of AhR/ARNT signal transduction pathways to weaken the occurrence of Th2 type response mediated skin inflammation. However, the excessive activation of AhR may also induce the occurrence of pruritus dermatitis (2, 166, 167).
5.5 Th22 type immune response
IL-22, the effector cytokine of Th22 type immune, has been reported to play a leading role in the pathogenesis of AD. IL-22 could inhibit epidermal differentiation and promote inflammation in AD skin lesions by inducing the secretion of IL-6, and downregulate the expression of keratinocyte fibril aggregation proteins in keratinocytes, especially, to increase the degree of epidermal damage (168), which is closely related to the severity of AD. However, the role of IL-22 in AD pathogenesis may be bidirectional, as it has been reported to have protective effects in acute viral infection of the intestinal tract but is pathogenic in the chronic inflammatory environment of AD and rheumatoid arthritis (169–172), this contradictory characteristic can even be derived into tryptophan metabolism. For instance, topical application of the tryptophan metabolic derivative FICZ could reduce the gene expression of IL-22 in a murine mite-induced dermatitis model by activating AhR receptors (161). On the other hand, IAld and I3A could actively stimulate IL-22 secretion via AhR, and the STAT3 phosphorylation is subsequently induced to accelerate proliferation of enterocytes for restoring damaged intestinal mucosa (173). Although there is evidence linking tryptophan metabolism to IL-22 secretion, the specific role of Th22 type immune response in the pathological development of AD or its connection with tryptophan require further relevant experimental confirmation.
5.6 Th17 type immune response
IL-17 is an important pro-inflammatory cytokine secreted mainly by Th17 type cells (174), could results in inflammatory reactions by inducing the production of pro-inflammatory cytokines and chemokines in keratinocytes, neutrophils and endothelial cells (175, 176). Besides that, IL-17 would reduce the expression of Flg in keratinocytes (177), thereby promoting the colonization of Staphylococcus aureus in damaged epidermal barrier of AD. The superantigen Staphylococcin B secreted by Staphylococcus aureus reversely promotes the secretion of IL-17, leading to a “vicious cycle” further destroys the skin barrier function of AD (178). As was expected, tryptophan metabolites and their AhR receptors have recently been shown to control inflammation by reducing the proliferation of Th17 lymphocytes (49, 179, 180). In mice exposed to Colitogenic DSS, the tryptophan derivative dioxin TCDD triggered AhR to inhibit proliferation of Th17 and induce differentiation of Treg (181). Similarly, the AhR ligand 3,3’-diindolyl methane (DIM) alleviated oxazolone induced experimental colitis by reducing Th2/Th17 cells and increasing Tregs (182). Increased expression of IL-17A in CD4+T cells was also observed in AhR -/- mice (183). The above evidences suggest that, the tryptophan metabolite, as an AhR ligand, is crucial for stimulating or inhibiting host immune responses and may be involved in the innate and adaptive immune response regulation of AD, although the further research about direct relationship between probiotics/tryptophan metabolites and AD is urgently needed.
6 Kyn-IDO pathway plays a crucial role in the development of AD
The Kyn - IDO pathway involved in the degradation of tryptophan was initially considered to have tolerance and immunomodulatory effects, moreover, there is mounting evidence that the Kyn-IDO pathway plays a crucial role in the development of atopy and allergy Kyn and its metabolites cover more than 90% of tryptophan metabolism, have been reported to activate AhR receptors with anti-inflammatory activity (184–187), thereupon then suppressing the activity of natural killer cells (NKT) (188) and APC such as dendritic cells (DC), monocytes, and macrophages (189, 190) in mice. Therefore, the Kyn-AhR axis has been postulated to constitute one of the factors affecting the progression of chronic inflammation (184). Further research has shown that the treatment of ovalbumin (OVA) induced asthma mice with Kyn metabolite HAA could inhibit Th 2 lung inflammation through moderately inducing apoptosis of Th 2 cells (78). In addition, IDO 1-Kyn-AhR signaling may reveal feedback loops related to inflammation and reactive oxygen species (ROS) production (191, 192). In the Kyn pathway, pro-inflammatory cytokines induce the IDO1 to create more Kyn, which in turn stimulates the expression of IDO1 by activating AhR. For example, when IFN- γ acts on intestinal epithelial cells, IDO could be induced to interfere with the expression of IL-10 receptor. Subsequently, Kyn restores the upregulation of IL-10 receptor expression by activating AhR, which significantly reduces the occurrence of intestinal inflammation (193). The above evidences indicates that the anti-inflammatory properties of the Kyn pathway is achieved through a negative feedback regulatory mechanism.
IDO is widely expressed in various types of cells, including most tumor cells, dendritic cells, macrophages, microglia, eosinophils, fibroblasts, and endothelial cells (142, 191–197), the most significant and effective inducers of IDO expression mainly are cytokines (such as IFN-γ, IFN-α, IFN-β and IL-10), as well as signaling through TLRs (198–200). IDO has long been considered to contribute significantly to the control of systemic inflammation (201), including its key role in reducing Th1 cell proliferation and inducing Treg cell differentiation (151, 189, 202), which could maintain normal immunological tolerance and limit the occurrence of tissue damage in the body. In support, enhanced CD4+ Th17 and Th1 responses were observed in airway of IDO-ablated mice following attacking by OVA and infection of human rhinovirus (hRV), characterized by significant infiltration of Th 17 and Th1 type neutrophils, high production of IL-17A, and IFN- γ, and increased collagen deposition and epithelial proliferation (73). Additionally, the direct role of IDO in inhibiting target cells by activation of Treg cells has been confirmed from Munn’s recent study (203, 204), specifically, the enhanced infiltration of effector T cells in the lung cells of IDO−/− mice coincided with a sharp decrease in CD4+CD25+FoxP3+Treg cells, which could be attributed to decreased AhR activity and impaired Kyn production (185, 205). Similarly, when co cultured with IDO+AML (Acute Myeloid Leukemia) cells, naïve T cells would convert to FoxP 3+Treg cells, and yet this conversion was completely eliminated by IDO inhibitor (206). The transformed Treg cells would subsequently suppress antigen-induced T cell responses in a “time delayed manner”, so then offsetting the overactive immune response and bringing the immune system closer to physiological equilibrium. Compared to the inhibitory effect of IDO on Th 1 cells differentiation, the effect on Th 2 cells is more complex with both inhibitory and stimulatory actions reported (207). According to the report, a asthma mouse model sensitized with ovalbumin (OVA) observed that expression of IDO inhibited Th2 type airway inflammation in the lungs (74), while the IDO-expressing and Kynurenine-producing eosinophils co cultured with Th1 or Th2 cells, a preferential decrease in Th1 response and subsequent increase in Th2 cytokine production were observed (208), indicating that IDO-expressing eosinophils may create cytotoxic metabolites that maintain an imbalance between Th1 and Th2 cell populations, and the consistent results were obtained in the study of Molano et al. (209). Notably, Kositz et al. reported that the higher tryptophan levels in atopic patients may be result from lower IDO-1 activity (66). In contrast, Th2 cytokines such as IL-4 and IL-13 inhibit the expression of IDO (210, 211), which creates favorable conditions for a Th2 tilted immune environment, thereby increasing the risk of Th2 related diseases. Although the above evidences suggest that IDO and/or its metabolites have a protective effect in atopic inflammation, there are also studies demonstrating the contradictory results that expression of IDO on eosinophils may contribute to the development of allergic inflammation (195) and was up-regulated in the AD skin (212). Under these circumstances, we suspect that the upregulation of IDO expression is a result of efforts to prevent ongoing allergic inflammation. In a few words, whether the induction of IDO can inhibit the development of allergic reactions or induce immune tolerance of allergic inflammation has begun requires further research.
7 Discussion
Previous studies have confirmed the beneficial role of tryptophan derivatives and AhR ligand in the pathogenesis or treatment of many skin diseases, including inflammatory diseases, skin pigmentation disorders and cancer (38–41, 213). Therefore, we speculate that exogenous supplementation of tryptophan derivatives (such as FICZ, IAId), or targeted intervention of GM (such as Lactobacillus reuteri, Lactobacillus salivary, Bifidobacterium and Lactobacillus), or targeted induction of the Kyn-IDO pathway to accelerate endogenous tryptophan metabolism and produce AhR ligands, may ultimately alleviate clinical symptoms of AD by suppressing abnormal immune responses. In addition to being a potential therapeutic option, tryptophan metabolites such as 5-hydroxyindolepyruvate and 5-hydroxyindoleacetic acid may become the new biomarkers supporting the diagnosis and prognosis of atopic diseases. Furthermore, supplementing tryptophan derivatives may alleviate some side effects such as herpes infections and headaches (214, 215) during the treatment of AD with biological agents. However, the biological functions involved in tryptophan metabolism are complex. The outcome of AhR activation depends on the type of cell and ligand (216, 217), stimulation or inhibition of AhR in the skin results in different immune responses (218), such as inducing overexpression of proinflammatory cytokines and ROS production to arise the development of inflammatory diseases or carcinogenesis (219), or affecting the differentiation of Treg cells, thereby promoting the immune tolerance (220, 221). Moreover, there is still insufficient evidence to support the clinical application of tryptophan and its derivatives, and the long-term efficacy and safety of their therapeutic potential are not fully understood. In summary, while the causal relationship among tryptophan metabolism, AD and GM, as well as the specific molecular mechanisms remain to be determined, we have preliminarily provided evidences that tryptophan plays an anti-inflammatory role in AD, laying the foundation for the exciting connection between tryptophan metabolism and AD immune regulation (Figure 2). We hope that this review will lead the way to further understand AD, and provide new insights into the pathogenesis and treatment direction of this disease.
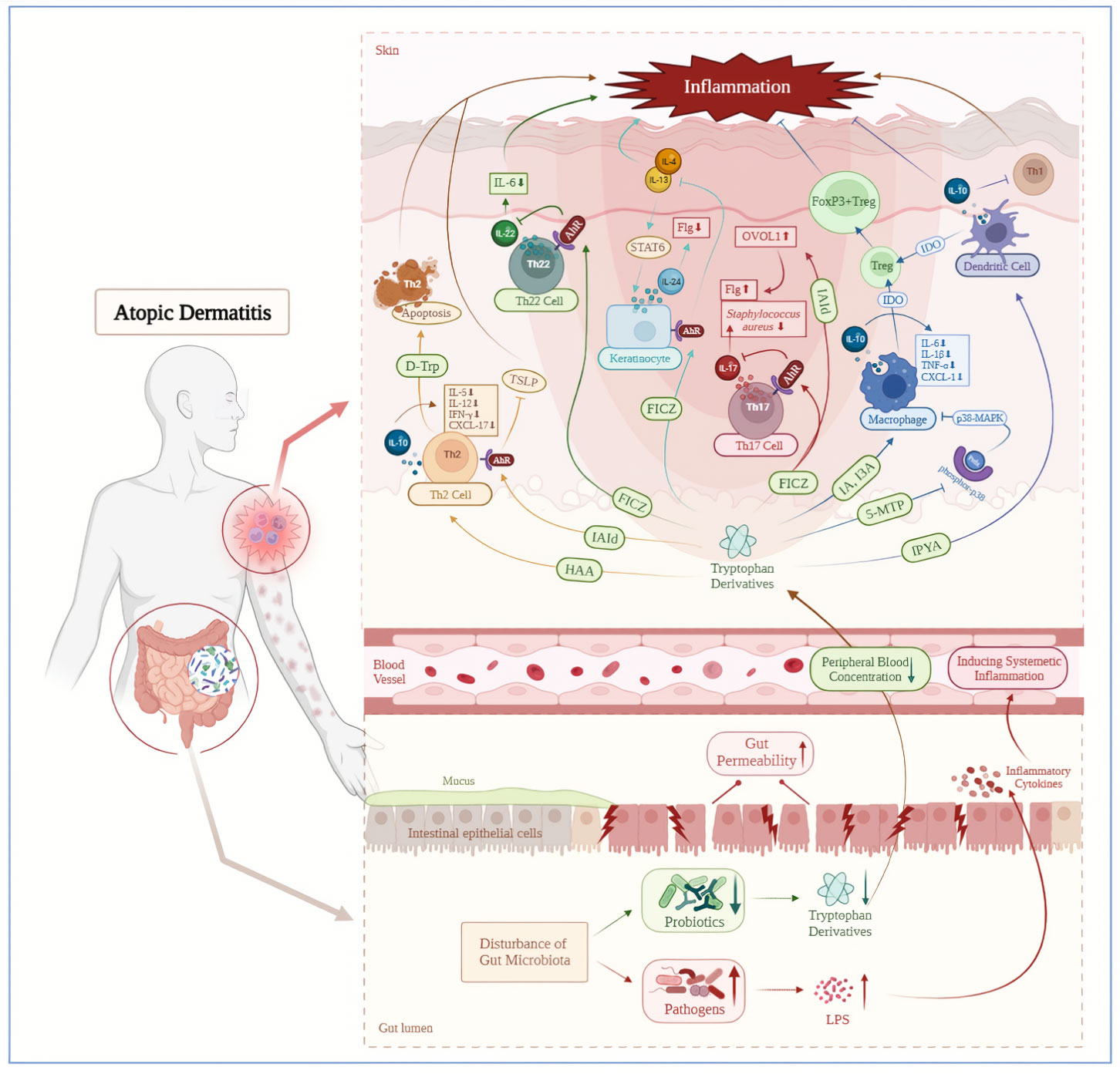
Figure 2 Decreased tryptophan metabolites mediated by gut microbiota disorder induce atopic dermatitis inflammation. AD patients are accompanied by intestinal microbiota disorders, including a downregulation of tryptophan producing probiotics and an increase in lipopolysaccharide producing pathogenic bacteria. The LPS could enter the bloodstream through increased gut permeability and cause systemic inflammation subsequently. On the contrary, downregulated tryptophan and its derivatives in the circulatory system have a decreased positive regulatory ability on the immune system, which could further exacerbate skin inflammation in AD patients. AhR, aryl hydrocarbon receptor; D-Trp, D-Tryptophan; FICZ, 6-formylindolo[3,2-b]carbazole; Flg, filaggrin; FoxP3+Treg, FoxP3+ regulatory T cell; HAA, Hydroxy-Anthranilic acid; I3A, Indole-3-aldehyde; IA, indoleacrylic acid; IAId, indole‐3‐aldehyde; IDO, indoleamine 2,3-dioxygenase; IPYA, Indole pyruvic acid; LPS, Lipopolysaccharide; OVOL1, OVO like transcriptional repressor 1; Prdx, peroxiredoxins; STAT6, signal transducer and activator of transcription 6; Treg, regulatory T cell; TSLP, thymic stromal lymphopoietin; 5-MTP,5-methoxyltryptophan.
Author contributions
YH: Writing – original draft, Visualization. LC: Writing – original draft. FL: Writing – original draft, Visualization. XX: Writing – review & editing. YO: Writing – review & editing. YD: Visualization, Writing – review & editing.
Funding
The author(s) declare financial support was received for the research, authorship, and/or publication of this article. This work was supported by the Joint project of Southwest Medical University and Luzhou science and Technology Bureau (grant no. 2021LZXNYD-Z04); General project of Southwest Medical University (grant no. 2021ZKMS027/2021ZKMS030); Sichuan Science and Technology Program (grant no. 2022YFS0631).
Conflict of interest
The authors declare that the research was conducted in the absence of any commercial or financial relationships that could be construed as a potential conflict of interest.
Publisher’s note
All claims expressed in this article are solely those of the authors and do not necessarily represent those of their affiliated organizations, or those of the publisher, the editors and the reviewers. Any product that may be evaluated in this article, or claim that may be made by its manufacturer, is not guaranteed or endorsed by the publisher.
References
1. Wallach D, Taïeb A. Atopic dermatitis/atopic eczema. Chem Immunol Allergy (2014) 100:81–96. doi: 10.1159/000358606
2. Hidaka T, Ogawa E, Kobayashi EH, Suzuki T, Funayama R, Nagashima T, et al. The aryl hydrocarbon receptor AhR links atopic dermatitis and air pollution via induction of the neurotrophic factor artemin. Nat Immunol (2017) 18(1):64–73. doi: 10.1038/ni.3614
3. Langan SM, Irvine AD, Weidinger S. Atopic dermatitis. Lancet (2020) 396(10247):345–60. doi: 10.1016/S0140-6736(20)31286-1
4. Ricci G, Patrizi A, Baldi E, Menna G, Tabanelli M, Masi M. Long-term follow-up of atopic dermatitis: retrospective analysis of related risk factors and association with concomitant allergic diseases. J Am Acad Dermatol (2006) 55(5):765–71. doi: 10.1016/j.jaad.2006.04.064
5. Kwon MS, Lim SK, Jang JY, Lee J, Park HK, Kim N, et al. Lactobacillus Sakei WIKIM30 ameliorates atopic dermatitis-like skin lesions by inducing regulatory T cells and altering gut microbiota structure in mice. Front Immunol (2018) 9:1905. doi: 10.3389/fimmu.2018.01905
6. Brandt EB, Sivaprasad U. Th2 cytokines and atopic dermatitis. J Clin Cell Immunol (2011) 2:110. doi: 10.4172/2155-9899.1000110
7. Albanesi C, Fairchild HR, Madonna S, Scarponi C, De Pità O, Leung DY, et al. IL-4 and IL-13 negatively regulate TNF and IFN induced -defensin expression through STAT-6, suppressor of cytokine signaling (SOCS)-1, and SOCS-3. J Immunol (2007) 179:984–92. doi: 10.4049/jimmunol.179.2.984
8. Sehra S, Yao Y, Howell MD, Nguyen ET, Kansas GS, Leung DY, et al. IL-4 regulates skin homeostasis and the predisposition toward allergic skin inflammation. J Immunol (2010) 184:3186–90. doi: 10.4049/jimmunol.0901860
9. Ong PY, Ohtake T, Brandt C, Strickland I, Boguniewicz M, Ganz T, et al. Endogenous antimicrobial peptides and skin infections in atopic dermatitis. N Engl J Med (2002) 347:1151–60. doi: 10.1056/NEJMoa021481
10. Tsakok T, Woolf R, Smith CH, Weidinger S, Flohr C. Atopic dermatitis: the skin barrier and beyond. Br J Dermatol (2018) 180(3):464–74. doi: 10.1111/bjd.16934
11. Peng W, Novak N. Pathogenesis of atopic dermatitis. Clin Exp Allergy (2015) 45:566–74. doi: 10.1111/cea.12495
12. Paus R, Theoharides TC, Arck PC. Neuro-immunoendocrine circuitry of the ‘Brain-skin connection’. Trends Immunol (2006) 27(1):32–9. doi: 10.1016/j.it.2005.10.002
13. Yew YW, Thyssen JP, Silverberg JI. A systematic review and meta-analysis of the regional and age-related differences in atopic dermatitis clinical characteristics. J Am Acad Derm (2019) 80:390–401. doi: 10.1016/j.jaad.2018.09.035
14. Bieber T. Atopic dermatitis: An expanding therapeutic pipeline for a complex disease. Nat Rev Drug Discov (2021) 21:21–40. doi: 10.1038/s41573-021-00266-6
15. Eichenfield LF, Tom WL, Berger TG, Krol A, Paller AS, Schwarzenberger K, et al. Guidelines of care for the management of atopic dermatitis: Section 2. Management and treatment of atopic dermatitis with topical therapies. J Am Acad Dermatol (2014) 71:116–32. doi: 10.1016/j.jaad.2014.03.023
16. Nusbaum KB, Nguyen CM, Fleischer AB. Emerging systemic therapies for atopic dermatitis: biologics. J Dermatol Treat (2022) 33(3):1269–73. doi: 10.1080/09546634.2020.1836314
17. Bao L, Zhang H, Chan LS. The involvement of the JAK-STAT signaling pathway in chronic inflammatory skin disease atopic dermatitis. JAKSTAT (2013) 2:e24137. doi: 10.4161/jkst.24137
18. Rodrigues MA, Torres T. JAK/STAT inhibitors for the treatment of atopic dermatitis. J Dermatolog Treat (2020) 31:33–40. doi: 10.1080/09546634.2019.1577549
19. Agus A, Planchais J, Sokol H. Gut microbiota regulation of tryptophan metabolism in health and disease. Cell Host Microbe (2018) 23(6):716–24. doi: 10.1016/j.chom.2018.05.003
20. Taleb S. Tryptophan dietary impacts gut barrier and metabolic diseases. Front Immunol (2019) 10:2113. doi: 10.3389/fimmu.2019.02113
21. Gutierrez-Vazquez C, Quintana FJ. Regulation of the immune response by the aryl hydrocarbon receptor. Immunity (2018) 48(1):19–33. doi: 10.1016/j.immuni.2017.12.012
22. Zelante T, Iannitti RG, Cunha C, De Luca A, Giovannini G, Pieraccini G, et al. Tryptophan catabolites from microbiota engage aryl hydrocarbon receptor and balance mucosal reactivity via interleukin-22. Immunity (2013) 39:372–85. doi: 10.1016/j.immuni.2013.08.003
23. Lamas B, Richard ML, Leducq V, Pham HP, Michel ML, Da Costa G, et al. CARD9 impacts colitis by altering gut microbiota metabolism of tryptophan into aryl hydrocarbon receptor ligands. Nat Med (2016) 22:598–605. doi: 10.1038/nm.4102
24. Dong F, Hao F, Murray IA, Smith PB, Koo I, Tindall AM, et al. Intestinal microbiota-derived tryptophan metabolites are predictive of Ah receptor activity. Gut Microbes (2020) 12:1–24. doi: 10.1080/19490976.2020.1788899
25. Esser C, Rannug A. The aryl hydrocarbon receptor in barrier organ physiology, immunology, and toxicology. Pharmacol Rev (2015) 67:259–79. doi: 10.1124/pr.114.009001
26. Lamas B, Natividad JM, Sokol H. Aryl hydrocarbon receptor and intestinal immunity. Mucosal Immunol (2018) 11(4):1024–38. doi: 10.1038/s41385-018-0019-2
27. Gao J, Xu K, Liu H, Liu G, Bai M, Peng C, et al. Impact of the gut microbiotaon intestinal immunity mediated by tryptophan metabolism. Front Cell Infect Microbiol (2018) 8:13. doi: 10.3389/fcimb.2018.00013
28. Fritsche E, Schäfer C, Calles C, Bernsmann T, Bernshausen T, Wurm M, et al. Lightening up the UV response by identification of the aryl hydrocarbon receptor as a cytoplasmatic target for ultraviolet B radiation. Proc Natl Acad Sci USA (2007) 104:8851–6. doi: 10.1073/pnas.0701764104
29. Furue M, Uchi H, Mitoma C, Hashimoto-Hachiya A, Chiba T, Ito T, et al. Antioxidants for healthy skin: The emerging role of aryl hydrocarbon receptors and nuclear factor-erythroid 2-related factor-2. Nutrients (2017) 9:223. doi: 10.3390/nu9030223
30. Magiatis P, Pappas P, Gaitanis G, Mexia N, Melliou E, Galanou M, et al. Malassezia yeasts produce a collection of exceptionally potent activators of the Ah (dioxin) receptor detected in diseased human skin. J Investig Dermatol (2013) 133:2023–30. doi: 10.1038/jid.2013.92
31. Takei K, Mitoma C, Hashimoto-Hachiya A, Takahara M, Tsuji G, Nakahara T, et al. Galactomyces fermentation filtrate prevents T helper 2-mediated reduction of filaggrin in an aryl hydrocarbon receptor-dependent manner. Clin Exp Dermatol (2015) 40:786–93. doi: 10.1111/ced.12635
32. Furue M, Hashimoto-Hachiya A, Tsuji G. Antioxidative phytochemicals accelerate epidermal terminal differentiation via the AHR-OVOL1 pathway: Implications for atopic dermatitis. Acta Derm Venereol (2018) 98:918–23. doi: 10.2340/00015555-3003
33. Lin YK, Leu YL, Yang SH, Chen HW, Wang CT, Pang JH. Anti-psoriatic effects of indigo naturalis on the proliferation and differentiation of keratinocytes with indirubin as the active component. J Dermatol Sci (2009) 54:168–74. doi: 10.1016/j.jdermsci.2009.02.007
34. Mimura J, Ema M, Sogawa K, Fujii-Kuriyama Y. Identification of a novel mechanism of regulation of Ah (dioxin) receptor function. Genes Dev (1999) 13(1):20–2. doi: 10.1101/gad.13.1.20
35. Tsuji G, Hashimoto-Hachiya A, Kiyomatsu-Oda M, Takemura M, Ohno F, Ito T, et al. Aryl hydrocarbon receptor activation restores filaggrin expression via OVOL1 in atopic dermatitis. Cell Death Dis (2017) 8:e2931. doi: 10.1038/cddis.2017.322
36. Furue M, Tsuji G, Mitoma C, Nakahara T, Chiba T, Morino-Koga S, et al. Gene regulation of filaggrin and other skin barrier proteins via aryl hydrocarbon receptor. J Dermatol Sci (2015) 80:83–8. doi: 10.1016/j.jdermsci.2015.07.011
37. Sutter CH, Bodreddigari S, Campion C, Wible RS, Sutter TR. 2,3,7,8-Tetrachlorodibenzo-p-dioxin increases the expression of genes in the human epidermal differentiation complex and accelerates epidermal barrier formation. Toxicol Sci (2011) 124:128–37. doi: 10.1093/toxsci/kfr205
38. Wirthgen E, Hoeflich A, Rebl A, Günther J. Kynurenic Acid: The janus-faced role of an immunomodulatory tryptophan metabolite and its link to pathological conditions. Front Immunol (2018) 8:1957. doi: 10.3389/fimmu.2017.01957
39. Kostyuk VA, Potapovich AI, Lulli D, Stancato A, De Luca C, Pastore S, et al. Modulation of human keratinocyte responses to solar UV by plant polyphenols as a basis for chemoprevention of non-melanoma skin cancers. Curr Med Chem (2013) 20:869–79. doi: 10.2174/0929867311320070003
40. Tsuji G, Takahara M, Uchi H, Takeuchi S, Mitoma C, Moroi Y, et al. M. An environmental contaminant, benzo(a)pyrene, induces oxidative stress-mediated interleukin-8 production in human keratinocytes via the aryl hydrocarbon receptor signaling pathway. J Dermatol Sci (2011) 62:42–9. doi: 10.1016/j.jdermsci.2010.10.017
41. Niestroy J, Barbara A, Herbst K, Rode S, van Liempt M, Roos PH. Single and concerted effects of benzo[a]pyrene and flavonoids on the AhR and Nrf2-pathway in the human colon carcinoma cell line Caco-2. Toxicol In Vitro (2011) 25:671–83. doi: 10.1016/j.tiv.2011.01.008
42. Furue M. Regulation of filaggrin, loricrin, and involucrin by IL-4, Il-13, IL-17a, Il-22, AhR, and NRF2: pathogenic implications in atopic dermatitis. Int J Mol Sci (2020) 21(15):5382. doi: 10.3390/ijms21155382
43. Yu J, Luo Y, Zhu Z, Zhou Y, Sun L, Gao J, et al. A tryptophan metabolite of the skin microbiota attenuates inflammation in patients with atopic dermatitis through the aryl hydrocarbon receptor. J Allergy Clin Immunol (2019) 143(6):2108–19.e12. doi: 10.1016/j.jaci.2018.11.036
44. Furue M, Iida K, Imaji M, Nakahara T. Microbiome analysis of forehead skin in patients with atopic dermatitis and healthy subjects: Implication of Staphylococcus and Corynebacterium. J Dermatol (2018) 45:876–7. doi: 10.1111/1346-8138.14486
45. Iwamoto K, Moriwaki M, Miyake R, Hide M. Staphylococcus aureus in atopic dermatitis: Strain-specific cell wall proteins and skin immunity. Allergol Int (2019) 68:309–15. doi: 10.1016/j.alit.2019.02.006
46. Roager HM, Licht TR. Microbial tryptophan catabolites in health and disease. Nat Commun (2018) 9(1):3294. doi: 10.1038/s41467-018-05470-4
47. Sun M, Ma N, He T, Johnston LJ, Ma X. Tryptophan (Trp) modulates gut homeostasis via aryl hydrocarbon receptor (AhR), Crit. Rev Food Sci Nutr (2020) 60(10):1760–8. doi: 10.1080/10408398.2019.1598334
48. Islam J, Sato S, Watanabe K, Watanabe T, Ardiansyah Hirahara K, Aoyama Y, et al. Dietary tryptophan alleviates dextran sodium sulfate-induced colitis through aryl hydrocarbon receptor in mice. J Nutr Biochem (2017) 42:43–50. doi: 10.1016/j.jnutbio.2016.12.019
49. Wilck N, Matus MG, Kearney SM, Olesen SW, Forslund K, Bartolomaeus H, et al. Salt-responsive gut commensal modulates T17 axis and disease. Nature (2017) 551(7682):585–9. doi: 10.1038/nature24628
50. Cervantes-Barragan L, Chai JN, Tianero MD, Di Luccia B, Ahern PP, Merriman J, et al. Induces gut intraepithelial CD4CD8αα T cells. Sci (New York NY) (2017) 357(6353):806–10. doi: 10.1126/science.aah5825
51. Rothhammer V, Mascanfroni ID, Bunse L, Takenaka MC, Kenison JE, Mayo L, et al. Type I inter-ferons and microbial metabolites of tryptophan modulate astrocyte activity and CNS inflammation via the aryl hydrocarbon receptor. Nat Med (2016) 22:586–97. doi: 10.1038/nm.4106
52. Metghalchi S, Ponnuswamy P, Simon T, Haddad Y, Laurans L, Cle´ment M, et al. Indole-amine 2,3-dioxygenase fine-tunes immune homeostasis in atherosclerosis and colitis through repression of interleukin-10 production. Cell Metab (2015) 22:460–71. doi: 10.1016/j.cmet.2015.07.004
53. Papathoma E, Triga M, Fouzas S, Dimitriou G. Cesarean section delivery and development of food allergy and atopic dermatitis in early childhood. Pediatr Allergy Immunol (2016) 27(4):419–24. doi: 10.1111/pai.12552
54. Brough HA, Liu AH, Sicherer S, Makinson K, Douiri A, Brown SJ, et al. Atopic dermatitis increases the effect of exposure to peanut antigen in dust on peanut sensitization and likely peanut allergy. J Allergy Clin Immunol (2015) 135(1):164–70. doi: 10.1016/j.jaci.2014.10.007
55. Crestani E, Harb H, Charbonnier L-M, Leirer J, Motsinger-Reif A, Rachid R, et al. Untargeted metabolomic profiling identifies disease-specific signatures in food allergy and asthma. J Allergy Clin Immunol (2020) 145:897–906. doi: 10.1016/j.jaci.2019.10.014
56. Buyuktiryaki B, Sahiner UM, Girgin G, Birben E, Soyer OU, Cavkaytar O, et al. Low indoleamine 2,3-dioxygenase activity in persistent food allergy in children. Allergy (2016) 71:258–66. doi: 10.1111/all.12785
57. Raitala A, Karjalainen J, Oja SS, Kosunen TU, Hurme M. Indoleamine 2,3-dioxygenase (IDO) activity is lower in atopic than in non-atopic individuals and is enhanced by environmental factors protecting from atopy. Mol Immunol (2006) 43:1054–6. doi: 10.1016/j.molimm.2005.06.022
58. Murray IA, Perdew GH. Ligand activation of the Ah receptor contributes to gastrointestinal homeostasis. Curr Opin Toxicol (2017) 2:15–23. doi: 10.1016/j.cotox.2017.01.003
59. Schulz VJ, Smit JJ, Willemsen KJ, Fiechter D, Hassing I, Bleumink R, et al. Activation of the aryl hydrocarbon receptor suppresses sensitization in a mouse peanut allergy model. Toxicol Sci (2011) 123:491–500. doi: 10.1093/toxsci/kfr175
60. Mims JW. Epidemiology of allergic rhinitis. Int Forum Allergy Rhinol (2014) 4:S18–20. doi: 10.1002/alr.21385
61. Aït-Khaled N, Pearce N, Anderson HR, Ellwood P, Montefort S, Shah J. Global map of the prevalence of symptoms of rhinoconjunctivitis in children: The International Study of Asthma and Allergies in Childhood (ISAAC) Phase Three. Allergy (2009) 64:123–48. doi: 10.1111/j.1398-9995.2008.01884.x
62. Loftus PA, Wise SK. Epidemiology and economic burden of asthma. Int Forum Allergy Rhinol (2015) 5:S7–S10. doi: 10.1002/alr.21547
63. Tao JL, Chen YZ, Dai QG, Tian M, Wang SC, Shan JJ, et al. Urine metabolic profiles in paediatric asthma. Respirology (2019) 24:572–81. doi: 10.1111/resp.13479
64. Saude EJ, Skappak CD, Regush S, Cook K, Ben-Zvi A, Becker A, et al. Metabolomic profiling of asthma: Diagnostic utility of urine nuclear magnetic resonance spectroscopy. J Allergy Clin Immunol (2011) 127:757–64. doi: 10.1016/j.jaci.2010.12.1077
65. Checkley W, Deza MP, Klawitter J, Romero KM, Klawitter J, Pollard SL, et al. Identifying biomarkers for asthma diagnosis using targeted metabolomics approaches. Respir Med (2016) 121:59–66. doi: 10.1016/j.rmed.2016.10.011
66. Huang Y, Chen G, Liu X, Shao Y, Gao P, Xin C, et al. Serum metabolomics study and eicosanoid analysis of childhood atopic dermatitis based on liquid chromatography–mass spectrometry. J Proteome Res (2014) 13:5715–23. doi: 10.1021/pr5007069
67. Gostner JM, Becker K, Kofler H, Strasser B, Fuchs D. Tryptophan metabolism in allergic disorders. Int Arch Allergy Immunol (2016) 169:203–15. doi: 10.1159/000445500
68. Ciprandi G, De Amici M, Tosca M, Fuchs D. Tryptophan metabolism in allergic rhinitis: the effect of pollen allergen exposure. Hum Immunol (2010) 71:911–5. doi: 10.1016/j.humimm.2010.05.017
69. Favennec M, Hennart B, Caiazzo R, Leloire A, Yengo L, Verbanck M, et al. The kynurenine pathway is activated in human obesity and shifted toward kynurenine monooxygenase activation. Obes (Silver Spring) (2015) 23:2066–74. doi: 10.1002/oby.21199
70. Romani L, Zelante T, De Luca A, Fallarino F, Puccetti P. IL-17 and therapeutic Kynurenines in pathogenic inflammation to fungi. J Immunol (2008) 180(8):5157–62. doi: 10.4049/jimmunol.180.8.5157
71. de Araújo EF, Feriotti C, Galdino NAL, Preite NW, Calich VLG, Loures FV. The IDO–AhR axis controls Th17/Treg immunity in a pulmonary model of fungal infection. Front Immunol (2017) 24:880. doi: 10.3389/fimmu.2017.00880
72. Prendergast GC, Malachowski WP, DuHadaway JB, Muller AJ. Discovery of IDO1 inhibitors: from bench to bedside. Cancer Res (2017) 77(24):6795–811. doi: 10.1158/0008-5472.CAN-17-2285
73. Hossain FMA, Park SO, Kim HJ, Eo JC, Choi JY, Tanveer M, et al. Indoleamine 2,3-dioxygenase in hematopoietic stem cell-derived cells suppresses rhinovirus-induced neutrophilic airway inflammation by regulating Th1- and Th17-type responses. Immune Netw (2021) 21(4):e26. doi: 10.4110/in.2021.21.e26
74. Hayashi T, Beck L, Rossetto C, Gong X, Takikawa O, Takabayashi K, et al. Inhibition of experimental asthma by indoleamine 2,3-dioxygenase. J Clin Investig (2004) 114:270–9. doi: 10.1172/JCI21275
75. Xu T, Zhou Y, Qiu L, Do DC, Zhao Y, Cui Z, et al. Aryl hydrocarbon receptor protects lungs from cockroach allergen–induced inflammation by modulating mesenchymal stem cells. J Immunol (2015) 195:5539–50. doi: 10.4049/jimmunol.1501198
76. Li XM, Peng J, Gu W, Guo XJ. TCDD-induced activation of aryl hydrocarbon receptor inhibits Th17 polarization and regulates non-eosinophilic airway inflammation in asthma. PloS One (2016) 11:e0150551. doi: 10.1371/journal.pone.0150551
77. Kepert I, Fonseca J, Müller C, Milger K, Hochwind K, Kostric M, et al. D-tryptophan from probiotic bacteria influences the gut microbiome and allergic airway disease. J Allergy Clin Immunol (2017) 139:1525–35. doi: 10.1016/j.jaci.2016.09.003
78. Hayashi T, Mo JH, Gong X, Rossetto C, Jang A, Beck L, et al. 3-Hydroxyanthranilic acid inhibits PDK1 activation and suppresses experimental asthma by inducing T cell apoptosis. Proc Natl Acad Sci USA (2007) 104(47):18619–24. doi: 10.1073/pnas.0709261104
79. Papamichael MM, Katsardis C, Erbas B, Itsiopoulos C, Tsoukalas D. Urinary organic acids as biomarkers in the assessment of pulmonary function in children with asthma. Nutr Res (2019) 61:31–40. doi: 10.1016/j.nutres.2018.10.004
80. Hamilton JD, Suarez-Farinas M, Dhingra N, Cardinale I, Li X, Kostic A, et al. Dupilumab improves the molecular signature in skin of patients with moderate-to-severe atopic dermatitis. J Allergy Clin Immunol (2014) 134:1293–1300. doi: 10.1016/j.jaci.2014.10.013
81. Wu GD, Lewis JD. Analysis of the human gut microbiome and association with disease. Clin Gastroenterol Hepatol (2013) 11:774–7. doi: 10.1016/j.cgh.2013.03.038
82. Gilbert JA, Blaser MJ, Caporaso JG, Jansson JK, Lynch SV, Knight R. Current understanding of the human microbiome. Nat Med (2018) 24(4):392–400. doi: 10.1038/nm.4517
83. Renz H, Brandtzaeg P, Hornef M. The impact of perinatal immune development on mucosal homeostasis and chronic inflammation. Nat Rev Immunol (2011) 12(1):9–23. doi: 10.1038/nri3112
84. Stokes JH, Pillsbury DH. The effect on the skin of emotional and nervous states: theoretical and practical consideration of a gastro-intestinal mechanism. Arch Derm Syphilol (1930) 22:962–93. doi: 10.1001/archderm.1930.01440180008002
85. Salem I, Ramser A, Isham N, Ghannoum MA. The gut microbiome as a major regulator of the gut-skin axis. Front Microbiol (2018) 9:1459. doi: 10.3389/fmicb.2018.01459
86. Stefanovic N, Flohr C, Irvine AD. The exposome in atopic dermatitis. Allergy (2020) 75(1):63–74. doi: 10.1111/all.13946
87. Stokholm J, Blaser MJ, Thorsen J, Rasmussen MA, Waage J, Vinding RK, et al. Maturation of the gut microbiome and risk of asthma in childhood. Nat Commun (2018) 9(1):141. doi: 10.1038/s41467-017-02573-2
88. Kim JE, Kim HS. Microbiome of the skin and gut in atopic dermatitis (AD): understanding the pathophysiology and finding novel management strategies. J Clin Med (2019) 8(4):444. doi: 10.3390/jcm8040444
89. Lopez-Santamarina A, Gonzalez EG, Lamas A, Mondragon ADC, Regal P, Miranda JM. Probiotics as a possible strategy for the prevention and treatment of allergies. A narrative review. Foods (2021) 10(4):701. doi: 10.3390/foods10040701
90. Zachariassen LF, Krych L, Engkilde K, Nielsen DS, Kot W, Hansen CH, et al. Sensitivity to oxazolone induced dermatitis is transferable with gut microbiota in mice. Sci Rep (2017) 7:44385. doi: 10.1038/srep44385
91. Lee SY, Lee E, Park YM, Hong SJ. Microbiome in the gut-skin axis in atopic dermatitis. Allergy Asthma Immunol Res (2018) 10(4):354. doi: 10.4168/aair.2018.10.4.354
92. Song H, Yoo Y, Hwang J, Na YC, Kim HS. Faecalibacterium prausnitzii subspecies-level dysbiosis in the human gut microbiome underlying atopic dermatitis. J Allergy Clin Immunol (2016) 137:852–60. doi: 10.1016/j.jaci.2015.08.021
93. Wang H, Li Y, Feng X, Li Y, Wang W, Qiu C, et al. Dysfunctional gut microbiota and relative co-abundance network in infantile eczema. Gut Pathog (2016) 8:36. doi: 10.1186/s13099-016-0118-0
94. Lee MJ, Kang MJ, Lee SY, Lee E, Kim K, Won S, et al. Perturbations of gut microbiome genes in infants with atopic dermatitis according to feeding type. J Allergy Clin Immunol (2018) 141:1310–9. doi: 10.1016/j.jaci.2017.11.045
95. Cait A, Cardenas E, Dimitriu PA, Amenyogbe N, Dai D, Cait J, et al. Reduced genetic potential for butyrate fermentation in the gut microbiome of infants who develop allergic sensitization. J Allergy Clin Immunol (2019) 144:1638–1647.e3. doi: 10.1016/j.jaci.2019.06.029
96. Nylund L, Nermes M, Isolauri E, Salminen S, de Vos WM, Satokari R. Severity of atopic disease inversely correlates with intestinal microbiota diversity and butyrate-producing bacteria. Allergy (2015) 70:241–4. doi: 10.1111/all.12549
97. Zimmermann P, Messina N, Mohn WW, Finlay BB, Curtis N. Association between the intestinal microbiota and allergic sensitization, eczema, and asthma: A systematic review. J Allergy Clin Immunol (2019) 143:467–85. doi: 10.1016/j.jaci.2018.09.025
98. Ye S, Yan F, Wang H, Mo X, Liu J, Zhang Y, et al. Diversity analysis of gut microbiota between healthy controls and those with atopic dermatitis in a Chinese population. J Dermatol (2021) 48:158–67. doi: 10.1111/1346-8138.15530
99. Hu C, van Meel ER, Medina-Gomez C, Kraaij R, Barroso M, Kiefte-de Jong J, et al. A population-based study on associations of stool microbiota with atopic diseases in schoolage children. J Allergy Clin Immunol (2021) 148:612–20. doi: 10.1016/j.jaci.2021.04.001
100. Melli LCFL, do Carmo-Rodrigues MS, Araújo-Filho HB, Mello CS, Tahan S, Pignatari ACC, et al. Gut microbiota of children with atopic dermatitis: Controlled study in the metropolitan region of São Paulo, Brazil. Allergol Immunopathol (2020) 48:107–15. doi: 10.1016/j.aller.2019.08.004
101. van Nimwegen FA, Penders J, Stobberingh EE, Postma DS, Koppelman GH, Kerkhof M, et al. Mode and place of delivery, gastrointestinal microbiota, and their influence on asthma and atopy. J Allergy Clin Immunol (2011) 128:948–955.e3. doi: 10.1016/j.jaci.2011.07.027
102. Penders J, Gerhold K, Stobberingh EE, Thijs C, Zimmermann K, Lau S, et al. Establishment of the intestinal microbiota and its role for atopic dermatitis in early childhood. J Allergy Clin Immunol (2013) 132:601–607.e8. doi: 10.1016/j.jaci.2013.05.043
103. Zheng H, Liang H, Wang Y, Miao M, Shi T, Yang F, et al. Altered gut microbiota composition associated with eczema in infants. PloS One (2016) 11:e0166026. doi: 10.1371/journal.pone.0166026
104. Lee E, Lee SY, Kang MJ, Kim K, Won S, Kim BJ, et al. Clostridia in the gut and onset of atopic dermatitis via eosinophilic inflammation. Ann Allergy Asthma Immunol (2016) 117:91–92.e1. doi: 10.1016/j.anai.2016.04.019
105. Kalliomäki M, Kirjavainen P, Eerola E, Kero P, Salminen S, Isolauri E. Distinct patterns of neonatal gut microflora in infants in whom atopy was and was not developing. J Allergy Clin Immunol (2001) 107:129–34. doi: 10.1067/mai.2001.111237
106. Watanabe S, Narisawa Y, Arase S, Okamatsu H, Ikenaga T, Tajiri Y, et al. Differences in fecal microflora between patients with atopic dermatitis and healthy control subjects. J Allergy Clin Immunol (2003) 111:587–91. doi: 10.1067/mai.2003.105
107. Mah KW, Björkstén B, Lee BW, van Bever HP, Shek LP, Tan TN, et al. Distinct pattern of commensal gut microbiota in toddlers with eczema. Int Arch Allergy Immunol (2006) 140:157–63. doi: 10.1159/000092555
108. Hong PY, Lee BW, Aw M, Shek LPC, Yap GC, Chua KY, et al. Comparative analysis of fecal microbiota in infants with and without eczema. PloS One (2010) 5:e9964. doi: 10.1371/journal.pone.0009964
109. Roduit C, Frei R, Ferstl R, Loeliger S, Westermann P, Rhyner C, et al. High levels of butyrate and propionate in early life are associated with protection against atopy. Allergy (2019) 74(4):799–809. doi: 10.1111/all.13660
110. Kim HJ, Lee SH, Hong SJ. Antibiotics-induced dysbiosis of intestinal microbiota aggravates atopic dermatitis in mice by altered short-chain fatty acids. Allergy Asthma Immunol Res (2020) 12(1):137–48. doi: 10.4168/aair.2020.12.1.137
111. Tan J, McKenzie C, Potamitis M, Thorburn AN, Mackay CR, Macia L. The role of short-chain fatty acids in health and disease. Adv Immunol (2014) 121:91–119. doi: 10.1016/B978-0-12-800100-4.00003-9
112. Macia L, Tan J, Vieira AT, Leach K, Stanley D, Luong S, et al. Metabolite-sensing receptors GPR43 and GPR109A facilitate dietary fibre-induced gut homeostasis through regulation of the inflammasome. Nat Commun (2015) 6:6734. doi: 10.1038/ncomms7734
113. Arpaia N, Campbell C, Fan X, Dikiy S, van der Veeken J, deRoos P, et al. Metabolites produced by commensal bacteria promote peripheral regulatory T-cell generation. Nature (2013) 504(7480):451–5. doi: 10.1038/nature12726
114. Furusawa Y, Obata Y, Fukuda S, Endo TA, Nakato G, Takahashi D, et al. Commensal microbe-derived butyrate induces the differentiation of colonic regulatory T cells. Nature (2013) 504(7480):446–50. doi: 10.1038/nature12721
115. Kendrick SFW, O’Boyle G, Mann J, Zeybel M, Palmer J, Jones DE, et al. Acetate, for key modulator of inflammatory responses in acute alcoholic hepatitis. Hepatology (2010) 51:1988–97. doi: 10.1002/hep.23572
116. Usami M, Kishimoto K, Ohata A, Miyoshi M, Aoyama M, Fueda Y, et al. Butyrate and trichostatin A attenuate nuclear factor kappaB activation and tumor necrosis factor alpha secretion and increase prostaglandin E2 secretion in human peripheral blood mononuclear cells. Nutr Res (2008) 28:321–8. doi: 10.1016/j.nutres.2008.02.012
117. Kim M, Qie Y, Park J, Kim CH. Gut microbial metabolites fuel host antibody responses. Cell Host Microbe (2016) 20:202–14. doi: 10.1016/j.chom.2016.07.001
118. Jeong DY, Ryu MS, Yang HJ, Jeong SY, Zhang T, Yang HJ, et al. Pediococcus acidilactici intake decreases the clinical severity ofAtopic dermatitis along with increasing mucin production and improving the gut microbiome in NC/Nga mice. BioMed Pharmacother (2020) 129:110488. doi: 10.1016/j.biopha.2020.110488
119. Kandikattu HK, Upparahalli Venkateshaiah S, Mishra A. Synergy of interleukin (IL)-5 and IL-18 in eosinophil mediated pathogenesis of allergic diseases. Cytokine Growth Factor Rev (2019) 47:83–98. doi: 10.1016/j.cytogfr.2019.05.003
120. Goodarzi A, Mozafarpoor S, Bodaghabadi M, Mohamadi M. The potential of probiotics for treating acne vulgaris: A review of literature on acne and microbiota. Dermatol Ther (2020) 33(3):e13279. doi: 10.1111/dth.13279
121. Yu Y, Dunaway S, Champer J, Kim J, Alikhan A. Changing our microbiome: probiotics in dermatology. Br J Dermatol (2020) 182(1):39–46. doi: 10.1111/bjd.18088
122. Liu Y, Tian X, Daniel RC, Okeugo B, Armbrister SA, Luo M, et al. Impact of probiotic Limosilactobacillus reuteri DSM 17938 on amino acid metabolism in the healthy newborn mouse. Amino Acids (2022) 54(10):1383–401. doi: 10.1007/s00726-022-03165-1
123. Xia J, Jiang S, Lv L, Wu W, Wang Q, Xu Q, et al. Modulation of the immune response and metabolism in germ-free rats colonized by the probiotic Lactobacillus salivarius LI01. Appl Microbiol Biotechnol (2021) 105(4):1629–45. doi: 10.1007/s00253-021-11099-z
124. Wiedlocha M, Marcinowicz P, Janoska-Jazdzik M, Szulc A. Gut microbiota, Kynurenine pathway and mental disorders - Review. Prog Neuropsychopharmacol Biol Psychiatry (2021) 106:110145. doi: 10.1016/j.pnpbp.2020.110145
125. Marsland BJ. Regulating inflammation with microbial metabolites. Nat Med (2016) 22(6):581–3. doi: 10.1038/nm.4117
126. Wikoff WR, Anfora AT, Liu J, Schultz PG, Lesley SA, Peters EC, et al. Metabolomics analysis reveals large effects of gut microflora on mammalian blood metabolites. Proc Natl Acad Sci USA (2009) 106:3698–703. doi: 10.1073/pnas.0812874106
127. Matsumoto M, Ebata T, Hirooka J, Hosoya R, Inoue N, Itami S, et al. Antipruritic effects of the probiotic strain LKM512 in adults with atopic dermatitis. Ann Allergy Asthma Immunol (2014) 113(2):209–216.e7. doi: 10.1016/j.anai.2014.05.002
128. Macpherson AJ, Slack E, Geuking MB, McCoy KD. The mucosal firewalls against commensal intestinal microbes. Semin Immunopathol (2009) 31(2):145–9. doi: 10.1007/s00281-009-0174-3
129. Purchiaroni F, Tortora A, Gabrielli M, Bertucci F, Gigante G, Ianiro G, et al. The role of intestinal microbiota and the immune system. Eur Rev Med Pharmacol Sci (2013) 17:323–33.
130. Seite S, Bieber T. Barrier function and microbiotic dysbiosis in atopic dermatitis. Clin Cosmet Investig Dermatol (2015) 8:479–83. doi: 10.2147/CCID.S91521
131. Johnson CC, Ownby DR. The infant gut bacterial microbiota and risk of pediatric asthma and allergic diseases. Trans Res (2017) 179:60–70. doi: 10.1016/j.trsl.2016.06.010
132. Bansal T, Alaniz RC, Wood TK, Jayaraman A. The bacterial signal indole increases epithelial-cell tight-junction resistance and attenuates indicators of inflammation. Proc Natl Acad Sci USA (2010) 107(1):228–33. doi: 10.1073/pnas.0906112107
133. Shimada Y, Kinoshita M, Harada K, Mizutani M, Masahata K, Kayama H, et al. Commensal bacteria-dependent indole production enhances epithelial barrier function in the colon. PloS One (2013) 8(11):e80604. doi: 10.1371/journal.pone.0080604
134. Wlodarska M, Luo C, Kolde R, d’Hennezel E, Annand JW, Heim CE, et al. Indoleacrylic acid produced by commensal peptostreptococcus species suppresses inflammation. Cell Host Microbe (2017) 22(1):25–37 e26. doi: 10.1016/j.chom.2017.06.007
135. Jennis M, Cavanaugh CR, Leo GC, Mabus JR, Lenhard J, Hornby PJ. Microbiota-derived tryptophan indoles increase after gastric bypass surgery and reduce intestinal permeability in vitro and in vivo. Neurogastroenterol Motil (2018) 30(2):1–12. doi: 10.1111/nmo.13178
136. Platten M, von Knebel Doeberitz N, Oezen I, Wick W, Ochs K. Cancer immunotherapy by targeting IDO1/TDO and their downstream effectors. Front Immunol (2015) 5:673. doi: 10.3389/fimmu.2014.00673
137. Ma Q. Influence of light on aryl hydrocarbon receptor signaling and consequences in drug metabolism, physiology and disease. Expert Opin Drug Metab Toxicol (2011) 7:1267–93. doi: 10.1517/17425255.2011.614947
138. Chieosilapatham P, Kiatsurayanon C, Umehara Y, Trujillo-Paez JV, Peng G, Yue H, et al. Keratinocytes: innate immune cells in atopic dermatitis. Clin Exp Immunol (2021) 204(3):296–309. doi: 10.1111/cei.13575
139. Mitamura Y, Nunomura S, Nanri Y, Ogawa M, Yoshihara T, Masuoka M, et al. The IL-13/periostin/IL-24 pathway causes epidermal barrier dysfunction in allergic skin inflammation. Allergy (2018) 73(9):1881–91. doi: 10.1111/all.13437
140. Tsuji G, Ito T, Chiba T, Mitoma C, Nakahara T, Uchi H, et al. The role of the OVOL1-OVOL2 axis in normal and diseased human skin. J Dermatol Sci (2018) 90:227–31. doi: 10.1016/j.jdermsci.2018.02.005
141. Takemura M, Nakahara T, Hashimoto-Hachiya A, Furue M, Tsuji G. Glyteer, soybean tar, impairs IL-4/Stat6 signaling in murine bone marrow-derived dendritic cells: The basis of its therapeutic effect on atopic dermatitis. Int J Mol Sci (2018) 19:1169. doi: 10.3390/ijms19041169
142. Di Meglio P, Duarte JH, Ahlfors H, Owens ND, Li Y, Villanova F, et al. Activation of the aryl hydrocarbon receptor dampens the severity of inflammatory skin conditions. Immunity (2014) 40:989–1001. doi: 10.1016/j.immuni.2014.04.019
143. Mosser DM, Edwards JP. Exploring the full spectrum of macrophage activation. Nat Rev Immunol (2008) 8(12):958–69. doi: 10.1038/nri2448
144. Wang XF, Wang HS, Wang H, Zhang F, Wang KF, Guo Q, et al. The role of indoleamine 2,3-dioxygenase (IDO) in immune tolerance: focus on macrophage polarization of THP-1 cells. Cell Immunol (2014) 289:42–8. doi: 10.1016/j.cellimm.2014.02.005
145. Savage NDL, de Boer T, Walburg KV, Joosten SA, van Meijgaarden K, Geluk A, et al. Human anti-inflammatory macrophages induce Foxp3 + GIT + CD25 + regulatory T cells, which suppress via membrane-bound TGFb-1. J Immunol (2008) 181:2220–6. doi: 10.4049/jimmunol.181.3.2220
146. Wang YF, Hsu YJ, Wu HF, Lee GL, Yang YS, Wu JY, et al. Endothelium-derived 5-methoxytryptophan is a circulating anti-inflammatory molecule that blocks systemic inflammation. Circ Res (2016) 119:222–36. doi: 10.1161/CIRCRESAHA.116.308559
147. Tiszlavicz Z, Németh B, Fülöp F, Vécsei L, Tápai K, Ocsovszky I, et al. Different inhibitory effects of Kynurenic acid and a novel Kynurenic acid analogue on tumour necrosis factor-a (TNF-a) production by mononuclear cells, HMGB1 production by monocytes and HNP1-3 secretion by neutrophils. Naunyn Schmiedebergs Arch Pharmacol (2011) 383:447–55. doi: 10.1007/s00210-011-0605-2
148. Yoshida Y, Hayakawa K, Fujishiro M, Ikeda K, Tsushima H, Hirai T, et al. Social defeat stress exacerbates atopic dermatitis through downregulation of DNA methyltransferase 1 and upregulation of C-C motif chemokine receptor 7 in skin dendritic cells. Biochem Biophys Res Commun (2020) 529(4):1073–9. doi: 10.1016/j.bbrc.2020.06.157
149. Grohmann U, Bianchi R, Belladonna ML, Silla S, Fallarino F, Fioretti MC, et al. IFN-gamma inhibits presentation of a tumor/self peptide by CD8 alpha-dendritic cells via potentiation of the CD8+ subset. J Immunol (2000) 165:1357–63. doi: 10.4049/jimmunol.165.3.1357
150. Hill M, Tanguy-Royer S, Royer P, Chauveau C, Asghar K, Tesson L, et al. IDO expands human CD4 +CD25high regulatory T cells by promoting maturation of LPS-treated dendritic cells. Eur J Immunol (2007) 37:3054–62. doi: 10.1002/eji.200636704
151. Fallarino F, Grohmng KW, Hwang KW, Orabona C, Vacca C, Bianchi R, et al. Modulation of tryptophan catabolism by regulatory T cells. Nat Immunol (2003) 4:1206–12. doi: 10.1038/ni1003
152. Mellor AL, Munn DH. IDO expression by dendritic cells: tolerance and tryptophan catabolism. Nat Rev Immunol (2004) 4:762–74. doi: 10.1038/nri1457
153. Terness P, Chuang JJ, Opelz G. The immunoregulatory role of IDO-producing human dendritic cells revisited. Trends Immunol (2006) 27:68–73. doi: 10.1016/j.it.2005.12.006
154. Aoki R, Aoki-Yoshida A, Suzuki C, Takayama Y. Indole-3-pyruvic acid, an aryl hydrocarbon receptor activator, suppresses experimental colitis in mice. J Immunol (2018) 201(12):3683–93. doi: 10.4049/jimmunol.1701734
156. Lee JK, Seok JK, Cho I, Yang G, Kim KB, Kwack SJ, et al. Topical application of celastrol alleviates atopic dermatitis symptoms mediated through the regulation of thymic stromal lymphopoietin and group 2 innate lymphoid cells. J Toxicol Environ Health A (2021) 84(22):922–31. doi: 10.1080/15287394.2021.1955785
157. Kolodkin-Gal I, Romero D, Cao S, Clardy J, Kolter R, Losick R. D-amino acids trigger biofilm disassembly. Science (2010) 328(5978):627–9. doi: 10.1126/science.1188628
158. Kao WT, Frye M, Gagnon P, Vogel JP, Chole R. D-amino acids do not inhibit pseudomonas aeruginosa biofilm formation. Laryngoscope Investig Otolaryngol (2017) 2(1):4–9. doi: 10.1002/lio2.34
159. Leung DY. New insights into atopic dermatitis: Role of skin barrier and immune dysregulation. Allergol Int (2013) 62:151–61. doi: 10.2332/allergolint.13-RAI-0564
160. Huang YJ, Marsland BJ, Bunyavanich S, O’Mahony L, Leung DY, Muraro A, et al. The microbiome in allergic disease: Current understanding and future opportunities—2017 PRACTALL document of the American Academy of Allergy, Asthma & Immunology and the European Academy of Allergy and Clinical Immunology. J Allergy Clin Immunol (2017) 139:1099–110. doi: 10.1016/j.jaci.2017.02.007
161. Kiyomatsu-Oda M, Uchi H, Morino-Koga S, Furue M. Protective role of 6-formylindolo[3,2-b] carbazole (FICZ), an endogenous ligand for arylhydrocarbon receptor, in chronic mite-induced dermatitis. J Dermatol Sci (2018) 90:284–94. doi: 10.1016/j.jdermsci.2018.02.014
162. Hong CH, Lee CH, Yu HS, Huang SK. Benzopyrene, a major polyaromatic hydrocarbon in smoke fume, mobilizes Langerhans cells and polarizes Th2/17 responses in epicutaneous protein sensitization through the aryl hydrocarbon receptor. Int Immunopharmacol (2016) 36:111–7. doi: 10.1016/j.intimp.2016.04.017
163. Rannug A, Fritsche E. The aryl hydrocarbon receptor and light. Biol Chem (2006) 387:1149–57. doi: 10.1515/BC.2006.143
164. Morita A. Current developments in phototherapy for psoriasis. J Dermatol (2018) 45:287–92. doi: 10.1111/1346-8138.14213
165. Ortiz-Salvador JM, Pérez-Ferriols A. Phototherapy in atopic dermatitis. Adv Exp Med Biol (2017) 996:279–86. doi: 10.1007/978-3-319-56017-5_23
166. Kennedy LH, Sutter CH, Leon Carrion S, Tran QT, Bodreddigari S, Kensicki E, et al. 2,3,7,8-Tetrachlorodibenzo-p-dioxin-mediated production of reactive oxygen species is an essential step in the mechanism of action to accelerate human keratinocyte differentiation. Toxicol Sci (2013) 132:235–49. doi: 10.1093/toxsci/kfs325
167. Edamitsu T, Taguchi K, Kobayashi EH, Okuyama R, Yamamoto M. Aryl hydrocarbon receptor directly regulates artemin gene expression. Mol Cell Biol (2019) 39(20):e00190−19. doi: 10.1128/MCB.00190-19
168. Lou H, Lu J, Choi EB, Oh MH, Jeong M, Barmettler S, et al. Expression of IL-22 in the skin causes Th2-biased immunity, epidermal barrier dysfunction, and pruritus via stimulating epithelial Th2 cytokines and the GRP pathway. J Immunol (2017) 198(7):2543–55. doi: 10.4049/jimmunol.1600126
169. Singh RK, Lee KM, Vujkovic−Cvijin I, Ucmak D, Farahnik B, Abrouk M, et al. The role of IL−17 in vitiligo: A review. Autoimmun Rev (2016) 15:397−404. doi: 10.1016/j.autrev.2016.01.004
170. Mattapallil MJ, Kielczewski JL, Zárate−Bladés CR, St Leger AJ, Raychaudhuri K, Silver PB, et al. Interleukin 22 ameliorates neuropathology and protects from central nervous system autoimmunity. J Autoimmun (2019) 102:65−76. doi: 10.1016/j.jaut.2019.04.017
171. Neil JA, Matsuzawa−Ishimoto Y, Kernbauer−Hölzl E, Schuster SL, Sota S, Venzon M, et al. IFN−I and IL−22 mediate protective effects of intestinal viral infection. Nat Microbiol (2019) 4:1737−1749. doi: 10.1038/s41564-019-0470-1
172. Zenewicz LA. IL−22: There is a gap in our knowledge. Immunohorizons (2018) 2:198−207. doi: 10.4049/immunohorizons.1800006
173. Hou Q, Ye L, Liu H, Huang L, Yang Q, Turner JR, et al. Lactobacillus accelerates ISCs regeneration to protect the integrity of intestinal mucosa through activation of STAT3 signaling pathway induced by LPLs secretion of IL-22. Cell Death Differ (2018) 25(9):1657–70. doi: 10.1038/s41418-018-0070-2
174. Park H, Li Z, Yang XO, Chang SH, Nurieva R, Wang YH, et al. A distinct lineage of CD4 T cells regulates tissue inflammation by producing interleukin 17[J/OL]. Nat Immunol (2005) 6(11):1133–41. doi: 10.1038/ni1261
175. Hofmann MA, Fluhr JW, Ruwwe-Glösenkamp C, Stevanovic K, Bergmann KC, Zuberbier T. Role of IL-17 in atopy-A systematic review. Clin Trans Allergy (2021) 11(6):e12047. doi: 10.1002/clt2.12047
176. Liu T, Li S, Ying S, Tang S, Ding Y, Li Y, et al. The IL-23/IL-17 pathway in inflammatory skin diseases: from bench to bedside. Front Immunol (2020) 11:594735. doi: 10.3389/fimmu.2020.594735
177. Hvid M, Vestergaard C, Kemp K, Christensen GB, Deleuran B, Deleuran M. IL-25 in atopic dermatitis: A possible link between inflammation and skin barrier dysfunction? J Invest Dermatol (2011) 131(1):150–7. doi: 10.1038/jid.2010.277
178. Sugaya M. The role of Th17-related cytokines in atopic dermatitis. Int J Mol Sci (2020) 21(4):1314. doi: 10.3390/ijms21041314
179. Rothhammer V, Mascanfroni ID, Bunse L, Takenaka MC, Kenison JE, Mayo L, et al. Type I interferons and microbial metabolites of tryptophan modulate astrocyte activity and central nervous system inflammation via the aryl hydrocarbon receptor. Nat Med (2016) 22:586–97. doi: 10.1038/nm.4106
180. Monteleone I, Rizzo A, Sarra M, Sica G, Sileri P, Biancone L, et al. Aryl hydrocarbon receptor-induced signals up-regulate IL-22 production and inhibit inflammation in the gastrointestinal tract. Gastroenterology (2011) 141(1):237–248, 48 e1. doi: 10.1053/j.gastro.2011.04.007
181. Singh NP, Singh UP, Singh B, Price RL, Nagarkatti M, Nagarkatti PS. Activation of aryl hydrocarbon receptor (AhR) leads to reciprocal epigenetic regulation of FoxP3 and IL-17 expression and amelioration of experimental colitis. PloS One (2011) 6(8):e23522. doi: 10.1371/journal.pone.0023522
182. Huang Z, Jiang Y, Yang Y, Shao J, Sun X, Chen J, et al. 3,3′-Diindolylmethane alleviates oxazolone-induced colitis through Th2/Th17 suppression and Treg induction. Mol Immunol (2013) 53(4):335–44. doi: 10.1016/j.molimm.2012.09.007
183. Rohlman D, Pham D, Yu Z, Steppan LB, Kerkvliet NI. Aryl hydrocarbon receptor−Mediated perturbations in gene expression during early stages of CD4(+) T−cell differentiation. Front Immunol (2012) 3:223. doi: 10.3389/fimmu.2012.00223
184. Opitz CA, Litzenburger UM, Sahm F, Ott M, Tritschler I, Trump S, et al. An endogenous tumour-promoting ligand of the human aryl hydrocarbon receptor. Nature (2011) 478:197–203. doi: 10.1038/nature10491
185. Mezrich JD, Fechner JH, Zhang X, Johnson BP, Burlingham WJ, Bradfield CA. An interaction between Kynurenine and the aryl hydrocarbon receptor can generate regulatory T cells. J Immunol (2010) 185:3190–8. doi: 10.4049/jimmunol.0903670
186. Wu D, Molofsky AB, Liang HE, Ricardo-Gonzalez RR, Jouihan HA, Bando JK, et al. Eosinophils sustain adipose alternatively activated macrophages associated with glucose homeostasis. Science (2011) 332:243–7. doi: 10.1126/science.1201475
187. Beamer CA, Seaver BP, Shepherd DM. Aryl hydrocarbon receptor (AhR) regulates silica-induced inflammation but not fibrosis. Toxicol Sci (2012) 126:554–68. doi: 10.1093/toxsci/kfs024
188. Frumento G, Rotondo R, Tonetti M, Damonte G, Benatti U, Ferrara GB. Tryptophan-derived catabolites are responsible for inhibition of T and natural killer cell proliferation induced by indoleamine 2,3-dioxygenase. J Exp Med (2002) 196:459–68. doi: 10.1084/jem.20020121
189. Fallarino F, Grohmann U, Vacca C, Bianchi R, Orabona C, Spreca A, et al. T cell apoptosis by tryptophan catabolism. Cell Death Differ (2002) 9:1069–77. doi: 10.1038/sj.cdd.4401073
190. Orabona C, Puccetti P, Vacca C, Bicciato S, Luchini A, Fallarino F. et al, Toward the identification of a tolerogenic signature in IDO-competent dendritic cells. Blood (2006) 107:2846–54. doi: 10.1182/blood-2005-10-4077
191. Neavin DR, Liu D, Ray B, Weinshilboum RM. The role of the aryl hydrocarbon receptor (AhR) in immune and inflammatory diseases. Int J Mol Sci (2018) 19(12):3851. doi: 10.3390/ijms19123851
192. Kaiser H, Parker E, Hamrick MW. Hamrick, Kynurenine signaling through the aryl hydrocarbon receptor: implications for aging and healthspan. Exp Gerontol (2020) 130:110797. doi: 10.1016/j.exger.2019.110797
193. Lanis JM, Alexeev EE, Curtis VF, Kitzenberg DA, Kao DJ, Battista KD, et al. Tryptophan metabolite activation of the aryl hydrocarbon receptor regulates IL-10 receptor expression on intestinal epithelia. Mucosal Immunol (2017) 10(5):1133–44. doi: 10.1038/mi.2016.133
194. Beutelspacher SC, Tan PH, McClure MO, Larkin DF, Lechler RI, George AJ. Expression of indoleamine 2,3-dioxygenase (IDO) by endothelial cells: implications for the control of alloresponses. Am J Transpl (2006) 6:1320–30. doi: 10.1111/j.1600-6143.2006.01324.x
195. Odemuyiwa SO, Ghahary A, Li Y, Puttagunta L, Lee JE, Musat-Marcu S, et al. Cutting edge: human eosinophils regulate T cell subset selection through indoleamine 2,3-dioxygenase. J Immunol (2004) 173:5909–13. doi: 10.4049/jimmunol.173.10.5909
196. Munn DH, Mellor AL. IDO and tolerance to tumors. Trends Mol Med (2004) 10:15–8. doi: 10.1016/j.molmed.2003.11.003
197. Uyttenhove C, Pilotte L, Theate I, Stroobant V, Colau D, Parmentier N, et al. Evidence for a tumoral immune resistance mechanism based on tryptophan degradation by indoleamine 2,3-dioxygenase. Nat Med (2003) 9:1269–74. doi: 10.1038/nm934
198. Agaugue S, Perrin-Cocon L, Coutant F, Andre P, Lotteau V. 1-Methyl-tryptophan can interfere with TLR signaling in dendritic cells independently of IDO activity. J Immunol (2006) 177:2061–71. doi: 10.4049/jimmunol.177.4.2061
199. Munn DH. Indoleamine 2,3-dioxygenase, tumor-induced tolerance and counter-regulation. Curr Opin Immunol (2006) 18:220–5. doi: 10.1016/j.coi.2006.01.002
200. Puccetti P, Fallarino F. Generation of T cell regulatory activity by plasmacytoid dendritic cells and tryptophan catabolism. Blood Cells Mol Dis (2008) 40:101–5. doi: 10.1016/j.bcmd.2007.06.026
201. Mellor AL, Munn DH. Tryptophan catabolism and regulation of adaptive mmunity. J Immunol (2003) 170(12):5809–13. doi: 10.4049/jimmunol.170.12.5809
202. Pallotta MT, Orabona C, Volpi C, Vacca C, Belladonna ML, Bianchi R, et al. Indoleamine 2,3-dioxygenase is a signaling protein in long-term tolerance by dendritic cells. Nat Immunol (2011) 12:870–8. doi: 10.1038/ni.2077
203. Sharma MD, Baban B, Chandler P, Hou DY, Singh N, Yagita H, et al. Plasmacytoid dendritic cells from mouse tumor-draining lymph nodes directly activate mature Tregs via indoleamine 2,3- dioxygenase. J Clin Invest (2007) 117:2570–82. doi: 10.1172/JCI31911
204. Munn DH, Sharma MD, Baban B, Harding HP, Zhang Y, Ron D, et al. GCN2 kinase in T cells mediates proliferative arrest and anergy induction in response to indoleamine 2,3-dioxygenase. Immunity (2005) 22:633–42. doi: 10.1016/j.immuni.2005.03.013
205. Munn DH, Mellor AL. Indoleamine 2,3 dioxygenase and metabolic control of immune responses. Trends Immunol (2013) 34:137–43. doi: 10.1016/j.it.2012.10.001
206. Curti A, Pandolfi S, Valzasina B, Aluigi M, Isidori A, Ferri E, et al. Modulation of tryptophan catabolism by human leukemic cells results in the conversion of CD25– into CD25+ T regulatory cells. Blood (2007) 109:2871–7. doi: 10.1182/blood-2006-07-036863
207. Xu H, Zhang GX, Ciric B, Rostami A. IDO: a double-edged sword for T(H)1/T(H)2 regulation. Immunol Lett (2008) 121:1–6. doi: 10.1016/j.imlet.2008.08.008
208. Odemuyiwa SO, Ghahary A, Li Y, Puttagunta L, Lee JE, Musat-Marcu S, et al. Cutting edge: human eosinophils regulate T cell subset selection through indoleamine 2,3-dioxygenase. J Immunol (2004) 173:5909–13. doi: 10.4049/jimmunol.173.10.5909
209. Molano A, Illarionov PA, Besra GS, Putterman C, Porcelli SA. Modulation of invariant natural killer T cell cytokine responses by indoleamine 2,3-dioxygenase. Immunol Lett (2008) 117:81–90. doi: 10.1016/j.imlet.2007.12.013
210. Musso T, Gusella G, Brooks A, Longo D, Varesio L. Interleukin-4 inhibits indoleamine 2,3-dioxygenase expression in human monocytes. Blood (1994) 83:1408–11. doi: 10.1182/blood.v83.5.1408.1408
211. Chaves AC, Cerávolo IP, Gomes JA, Zani CL, Romanha AJ, Gazzinelli RT. IL-4 and IL-13 regulate the induction of indoleamine 2,3-dioxygenase activity and the control of Toxoplasma gondii replication in human fibroblasts activated with IFN-gamma. Eur J Immunol (2001) 31:333–44. doi: 10.1002/1521-4141(200102)31:2<333::AID-IMMU333>3.0.CO;2-X
212. Ito M, Ogawa K, Takeuchi K, Nakada A, Heishi M, Suto H, et al. Gene expression of enzymes for tryptophan degradation pathway is upregulated in the skin lesions of patients with atopic dermatitis or psoriasis. J Derm Sci (2004) 36:157–64. doi: 10.1016/j.jdermsci.2004.08.012
213. Hu T, Pan Z, Yu Q, Mo X, Song N, Yan M, et al. Benzo(a)pyrene induces interleukin (IL)-6 production and reduces lipid synthesis in human SZ95 sebocytes via the aryl hydrocarbon receptor signaling pathway. Environ Toxicol Pharmacol (2016) 43:54–60. doi: 10.1016/j.etap.2016
214. Adams O, Besken K, Oberdörfer C, MacKenzie CR, Rüssing D, Däubener W. Inhibition of human herpes simplex virus type 2 by interferon gamma and tumor necrosis factor alpha is mediated by indoleamine 2,3-dioxygenase. Microbes Infect (2004) 6(9):806–12. doi: 10.1016/j.micinf.2004.04.007
215. Drummond PD. Tryptophan depletion increases nausea, headache and photophobia in migraine sufferers. Cephalalgia (2006) 26(10):1225–33. doi: 10.1111/j.1468-2982.2006.01212.x
216. Wincent E, Bengtsson J, Bardbori AM, Alsberg T, Luecke S, Rannug U, et al. A. Inhibition of cytochrome P4501-dependent clearance of the endogenous agonist FICZ as a mechanism for activation of the aryl hydrocarbon receptor. Proc Natl Acad Sci USA (2012) 109:4479–84. doi: 10.1073/pnas.1118467109
217. Sun YV, Boverhof DR, Burgoon LD, Fielden MR, Zacharewski TR. Comparative analysis of dioxin response elements in human, mouse and rat genomic sequences. Nucleic Acids Res (2004) 32:4512–23. doi: 10.1093/nar/gkh782
218. Haarmann-Stemmann T, Abel J, Fritsche E, Krutmann J. The AhR-Nrf2 pathway in keratinocytes: On the road to chemopre-vention? J Investig Dermatol (2012) 132:7–9. doi: 10.1038/jid.2011.359
219. Tsuji G, Takahara M, Uchi H, Matsuda T, Chiba T, Takeuchi S, et al. Identification of ketoconazole as an AhR-Nrf2 activator in cultured human keratinocytes: The basis of its anti-inflammatory effect. J Investig Dermatol (2012) 132:59–68. doi: 10.1038/jid.2011.194
220. di Meglio P, Perera GK, Nestle FO. The multitasking organ: Recent insights into skin immune function. Immunity (2011) 35:857–69. doi: 10.1016/j.immuni.2011.12.003
Keywords: atopic dermatitis, tryptophan, gut microbiota, immunology response, inflammation
Citation: Huang Y, Chen L, Liu F, Xiong X, Ouyang Y and Deng Y (2024) Tryptophan, an important link in regulating the complex network of skin immunology response in atopic dermatitis. Front. Immunol. 14:1300378. doi: 10.3389/fimmu.2023.1300378
Received: 05 October 2023; Accepted: 31 December 2023;
Published: 22 January 2024.
Edited by:
Rui-qun Qi, The First Affiliated Hospital of China Medical University, ChinaCopyright © 2024 Huang, Chen, Liu, Xiong, Ouyang and Deng. This is an open-access article distributed under the terms of the Creative Commons Attribution License (CC BY). The use, distribution or reproduction in other forums is permitted, provided the original author(s) and the copyright owner(s) are credited and that the original publication in this journal is cited, in accordance with accepted academic practice. No use, distribution or reproduction is permitted which does not comply with these terms.
*Correspondence: Xia Xiong, eGlvbmd4aWE3ODlAMTI2LmNvbQ==; Yongliang Ouyang, b3V5YW5neWwxOTgxQDE2My5jb20=; Yongqiong Deng, ZGVuZ3lvbmdxaW9uZzFAMTI2LmNvbQ==
†These authors have contributed equally to this work and share first authorship