- 1Department of Medicine and Center for Vaccine Development and Global Health, University of Maryland School of Medicine, Baltimore, MD, United States
- 2School of Public Health, Faculty of Medicine, Tel Aviv University, Tel Aviv, Israel
- 3Institut Pasteur, Université Paris Cité, CNRS UMR3523, Unité Chimie des Biomolécules, Paris, France
- 4Institut Pasteur, Université Paris Cité, Laboratoire Innovation: Vaccins, Paris, France
- 5Department of Pediatrics and Center for Vaccine Development and Global Health, University of Maryland School of Medicine, Baltimore, MD, United States
Shigellosis is common worldwide, and it causes significant morbidity and mortality mainly in young children in low- and middle- income countries. To date, there are not broadly available licensed Shigella vaccines. A novel type of conjugate vaccine candidate, SF2a-TT15, was developed against S. flexneri serotype 2a (SF2a). SF2a-TT15 is composed of a synthetic 15mer oligosaccharide, designed to act as a functional mimic of the SF2a O-antigen and covalently linked to tetanus toxoid (TT). SF2a-TT15 was recently shown to be safe and immunogenic in a Phase 1 clinical trial, inducing specific memory B cells and sustained antibody response up to three years after the last injection. In this manuscript, we advance the study of B cell responses to parenteral administration of SF2a-TT15 to identify SF2a LPS-specific B cells (SF2a+ B cells) using fluorescently labeled bacteria. SF2a+ B cells were identified mainly within class-switched B cells (SwB cells) in volunteers vaccinated with SF2a-TT15 adjuvanted or not with aluminium hydroxide (alum), but not in placebo recipients. These cells expressed high levels of CXCR3 and low levels of CD21 suggesting an activated phenotype likely to represent the recently described effector memory B cells. IgG SF2a+ SwB cells were more abundant than IgA SF2a + SwB cells. SF2a+ B cells were also identified in polyclonally stimulated B cells (antibody secreting cells (ASC)-transformed). SF2a+ ASC-SwB cells largely maintained the activated phenotype (CXCR3 high, CD21 low). They expressed high levels of CD71 and integrin α4β7, suggesting a high proliferation rate and ability to migrate to gut associated lymphoid tissues. Finally, ELISpot analysis showed that ASC produced anti-SF2a LPS IgG and IgA antibodies. In summary, this methodology confirms the ability of SF2a-TT15 to induce long-lived memory B cells, initially identified by ELISpots, which remain identifiable in blood up to 140 days following vaccination. Our findings expand and complement the memory B cell data previously reported in the Phase 1 trial and provide detailed information on the immunophenotypic characteristics of these cells. Moreover, this methodology opens the door to future studies at the single-cell level to better characterize the development of B cell immunity to Shigella.
Introduction
Shigellosis continues to be a significant public health problem, especially in low- and middle-income countries (LMICs) where children under 5 years of age are particularly susceptible. Over 200 million cases of shigellosis occur every year in LMIC, with ~200,000 deaths reported and ~64,000 of these among young children (<5 years-old) (1). Even when not deadly, repeated bouts of diarrheal disease impair physical growth and cognitive abilities (2, 3). S. flexneri is the leading cause of endemic shigellosis in LMICs (~60% of infections) (4), while S. sonnei is the second most common cause (~25% of episodes). S. sonnei is also the leading species in high-income countries (5) and outbreaks are linked to i) young children in child-care facilities/schools (6), ii) travelers and military personnel (7, 8) and iii) men who have sex with men (9–11). Outbreaks caused by multidrug-resistant Shigella have also been reported in several high-income countries (12, 13). The main prevention strategy for shigellosis includes improved sanitation, access to clean water sources and good personal hygiene. However, Shigella requires a low bacteria inoculum to cause disease (100-1000 bacilli) which leads to frequent failures of preventive sanitary measures. Additionally, Shigella is classified as a category B agent of biodefense concern, making it a priority for the development of therapeutics and vaccines (14).
It is possible to induce protective immunity against shigellosis. For example, naturally-acquired wild-type (wt) Shigella infection confers ~70% serotype-specific immunity (15–17). Moreover, adults experimentally infected with either S. sonnei or S. flexneri are significantly protected against illness following re-challenge with the homologous strain (64-74% efficacy) (18, 19). Anti-lipopolysaccharide (LPS) antibodies appear to be critical for protection (17, 20). Despite great efforts, no licensed vaccine for shigellosis is available. Most vaccine efforts have focused on the O-antigen, a polysaccharide unit of the outer membrane LPS (21–24). Shigella detoxified LPS-protein conjugates have shown to be a potentially viable strategy (22, 25, 26). As a lead demonstration, a S. sonnei conjugate showed 74% protective efficacy in young adults (26) and 71% protective efficacy in children aged 3-4 years but was not protective in younger children (27), highlighting the need to develop improved vaccines. A novel sub-type of conjugate vaccine candidate was recently proposed. This vaccine is composed of a synthetic 15mer oligosaccharide (OS), designed to act as a functional mimic of the S. flexneri serotype 2a (SF2a) O-antigen and covalently linked to tetanus toxoid (TT) via single point attachment (herein SF2a-TT15) (24, 28). This synthetic carbohydrate-based vaccine candidate was shown to be safe and immunogenic following parenteral administration in a recent Phase I clinical trial (21). Given the importance of humoral responses in protection (29), especially antibodies against the O-antigen, it is critical to understand better how these responses are developed and persist over time, as well as the characteristics of the cells responsible for producing class-switched antibodies. In this manuscript, we expand our understanding of the B memory responses elicited by SF2a-TT15 by using a new method for the identification of Shigella-LPS-specific B cells.
Materials and methods
Labeling of whole bacteria with fluorescent dyes
SF2a (2457T) from a CVD master stock was grown into LB agar (overnight; 37°C). Next morning, cells were gently harvested from the plate using a cell scraper and 5 mL of 1X PBS. Bacteria were transferred to a 50 ml conical tube. The harvesting procedure was repeated twice and 1X PBS was added to the tube for a total of 20 mL. Bacteria was then placed into a water bath (65°C) for 60 minutes and then transferred to iced water for 5 minutes. Two mL of 10% formaldehyde were added to the bacteria and incubated at room temperature for 1 hour. The bacteria concentration was adjusted to 0.4 (O.D. 600 nm) using 1X PBS. Ten mL of bacteria (0.4 at 600 nm) were transferred to a 15 mL conical tube and centrifuged (4,300 g, 30 min at 4°C). The supernatant was carefully removed, the bacteria pellet resuspended in 15 mL of 1X PBS and centrifuged again (4,300 g, 30 minutes at 4 °C). The supernatant was removed, and the bacteria cell pellet resuspended in 0.5 mL of Pacific Blue succinimidyl ester (Molecular Probes, Eugene, OR) at a concentration of 20 μg/mL in 1X PBS. Bacteria were stained for 1 hour at room temperature (RT) in the dark. Then, bacteria were washed with 10 mL of a solution of 1% FBS in 1X PBS (4,300 g, 30 minutes at 4°C). The supernatant was discarded, the bacteria pellet was resuspended in a solution of 1% formaldehyde in 1X PBS and incubated for 1 hour at RT. The bacteria pellet was then washed twice with 10 mL of 1X PBS (4,300 g, 30 minutes at 4°C). The bacteria pellet was then resuspended in 3 mL of 1% BSA/1XPBS and the O.D. (600 nm) adjusted to 0.4. SF2a-Pacific Blue (SF2a-PB) was stored at 4°C covered from light. A similar procedure was used to label a second set of SF2a with Alexa Fluor 488 succinimidyl ester (Molecular Probes, Eugene, OR). Successful labeling of the bacteria was tested in an LSR-II flow cytometer (Beckton Dickinson, NJ) (Supplementary Figures 1A-C) using unlabeled bacteria as control. Only bacteria preparations with >90% labeled bacteria were considered appropriate for the experiments presented in this manuscript.
Ethics statements and clinical samples
The specimens used in this study were collected as part of a Phase I clinical trial assessing the safety and immunogenicity of SF2a-TT15, a synthetic carbohydrate conjugate vaccine against SF2a (28). The clinical component of the study was performed at the Clinical Research Center of Tel Aviv Sourasky Medical Center (Israel). All volunteers provided informed consent. The study is registered with ClinicalStudies.gov, NCT02797236 and has been completed. Peripheral blood mononuclear cells (PBMC) were isolated from vaccine and placebo recipients at days 0, 28, 56, 84 and 140 (Supplementary Figure 1D) using a standard Ficoll density fractionation method and cryopreserved. De-identified specimens (PBMC) from six volunteers who received SF2a-TT15 (10 µg), six that received SF2a-TT15 + Alum (10 µg) and four that received Placebo + Alum were assessed at the University of Maryland School of Medicine. Safety and immunogenicity data (humoral response) have already been reported (21).
Flow cytometry staining for SF2a-specific B cells
Cryopreserved PBMC were thawed in complete RPMI (cRPMI) [RPMI (Gibco, NY, USA) supplemented with 10% fetal bovine serum (FBS) (Gemini Bioproducts, West Sacramento, CA), 2 mM L-glutamine (Gibco, Grand Island, NY, USA), 1x non-essential amino acids (Gibco, Grand Island, NY, USA), 10 mM HEPES (Gibco, Grand Island, NY, USA), 2.5 mM sodium pyruvate, (Lonza, Walkersville, MD, USA), 100 U/mL penicillin, 100 ug/ml streptomycin (Sigma-Aldrich, St. Louis, MO, USA), 50 μg/mL gentamicin (Gibco, Grand Island, NY, USA)]. Cells were immediately stained for flow-cytometry using methods previously described (30–34). Briefly, 2x10e6 cells were plated into 12x75 mm tubes, washed twice with 1X PBS (450 g, 5 min, 4°C) and stained for viability (20 min on ice) using 100 μL of a fixable yellow staining dye (Invitrogen, USA). After 2 washes with flow cytometry staining buffer (FCSB) (0.5% FCS in 1X PBS), cells were blocked with human Fc Block (BD Pharmingen, USA). Cells were then washed with FCSB (450 g, 5 min, 4°C) and resuspended in 50 μL of a solution containing 0.6 μL of SF2a-Ax488 (O.D. 0.4 at 600 nm) plus 0.6 μL of SF2a-PB (O.D. 0.4 at 600 nm) in FCSB. PBMC were stained with bacteria for 30 minutes at RT. After 2 washes with FCSB, the cells were stained with an antibody cocktail prepared in FCSB (30 min, RT). Monoclonal antibodies (mAbs) against the following molecules were used: IgA-PE (goal polyclonal; SouthernBiotech), CD19-ECD (clone J3-119; Beckman Coulter -BC-), IgD-PerCP-Cy5.5 (clone IA6-2; BD), CD27-PE-Cy7 (clone M-T271; BD), CD14-Brillant Violet 570 (BV570) (clone M5E2; BioLegend), CD3-BV570 (clone UCHT1; BioLegend), CD56-BV570 (clone HCD56; BioLegend), IgG-BV605 (clone G18-154; BD), CD21-BV711 (clone B-ly4; BD), CXCR3-BV785 (clone 1C6/CXCR3; BD), integrin α4β7-Alexa647 (clone ACT-1; Millennium, The Takeda Oncology Co), CD38-APC-R700 (clone LS1298-4-3; BD), CD71-APC-H7 (clone M-A712; BD). After washing with FCSB, the cells were fixed with 1% PFA in 1X PBS. Samples were collected in a custom LSRII flow cytometer (BD, USA). Samples were analyzed using FlowJo (Tree Star, San Francisco, CA) (Figures 1A, 2A).
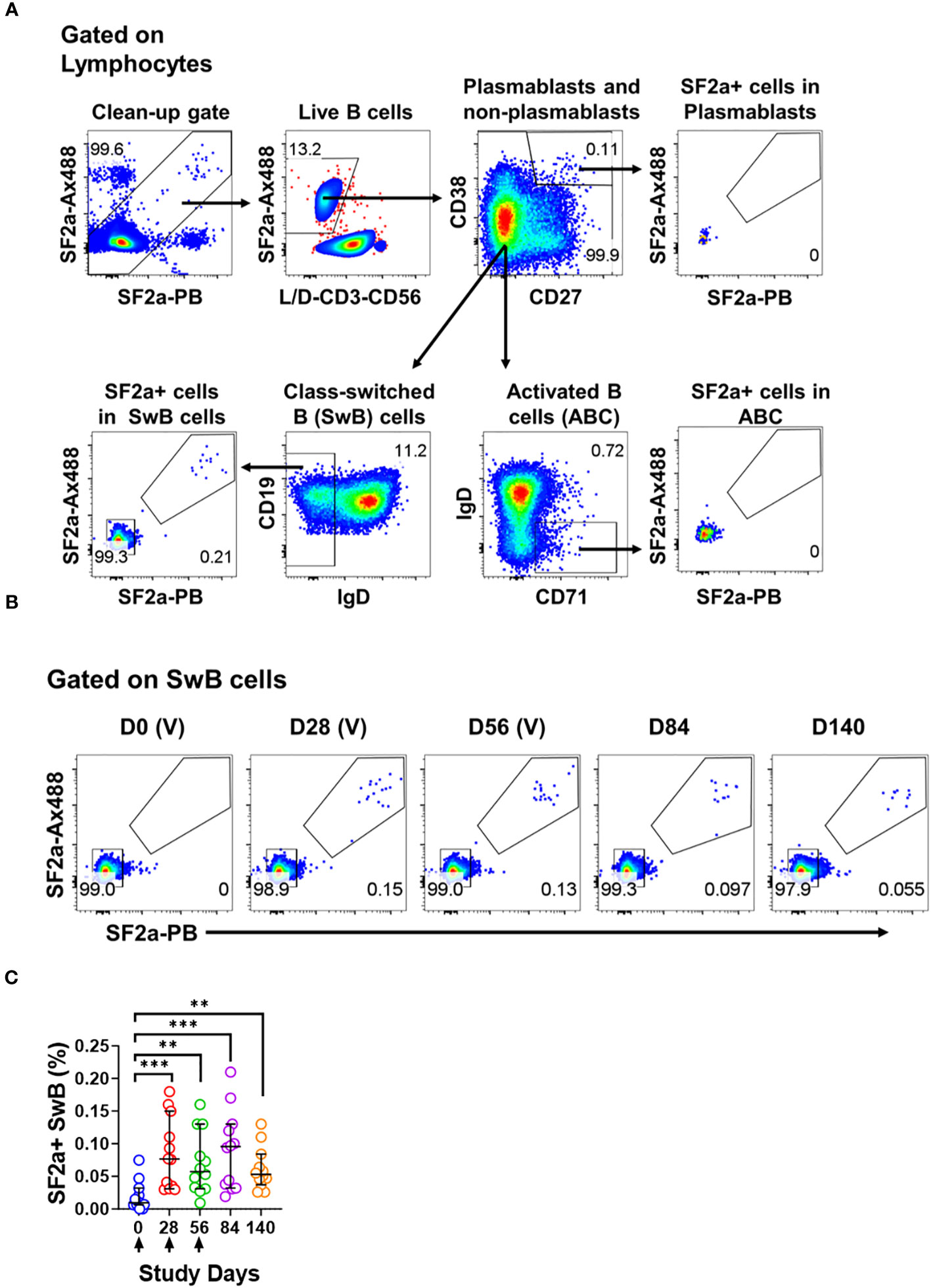
Figure 1 Identification of SF2a+ B cells in PBMC by flow cytometry. Panel (A) shows the gating strategy used to identify various B cell subsets including Plasmablasts (CD3- CD19+ CD38++, CD27++), Activated B cells (ABC; CD3- CD19+ CD27+/dim, CD38+/dim, IgD-, CD71+) and Switched B (SwB; CD3- CD19+ CD27+/dim, CD38+/dim, IgD-) cells. Note that this gating strategy eliminates non-specific SF2a binders using a clean-up gate (Top panel on the left and Supplementary Figures 1D, E). Panel (B) shows the identification and persistence of SF2a+ cells in SwB cells after vaccination in PBMC of a representative volunteer. Vaccine days are indicated as (V). Panel (C) shows the percentage of SF2a+ cells within SwB cells in volunteers that received SF2a-TT15 +/- Alum (n=12). Arrows show the vaccination days. Mean and 95CI are shown. The p values were calculated using unpaired t-tests (2-sided). **p<0.005, ***p<0.001.
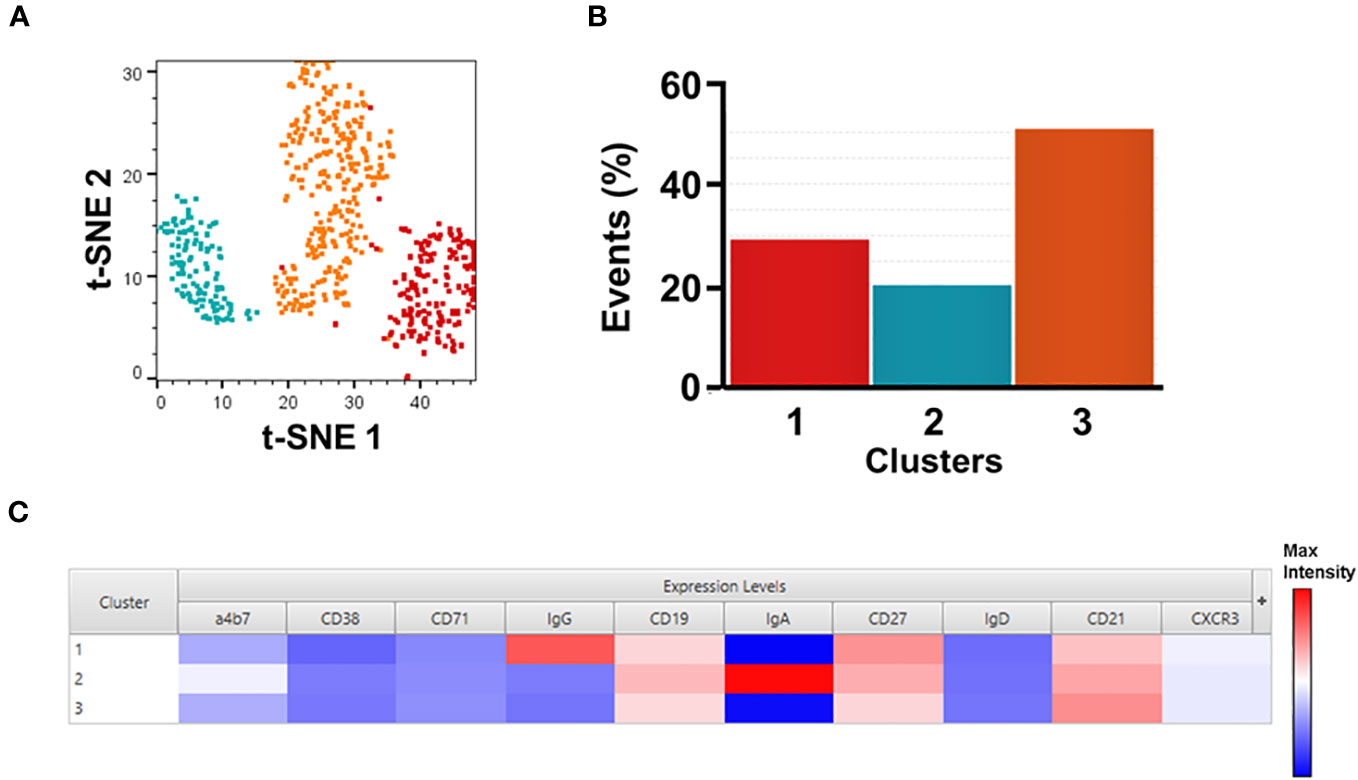
Figure 2 Unsupervised analysis of SF2a+ Sw B cells. Panel (A) shows t-SNE analysis overlaid with the X-shift analysis. The SF2a+ SwB cell population (concatenated) from all SF2a-TT15 +/- Alum vaccinated volunteers days 28-140 (no day 0) were used in the analysis. Panel (B) shows the frequency of the three cell clusters identified in the t-SNE/X-shift analysis. Panel (C) shows the expression intensity of the markers assessed (Cluster Explorer) and used to define the clusters.
Differentiation of B cells into antibody secreting cells and staining for SF2a-specific B cells
To differentiate B cells into ASC, PBMC were polyclonally expanded as described by Crotty et al. (35). In short, cryopreserved PBMC were thawed, washed with cRPMI and expanded for 5-6 days in 6-well sterile plates (2.5x10e6 cells/well) in the presence of 50 μM 2-mercaptoethanol, 1/100,000 pokeweed mitogen (PWM) (kindly provided by Dr. S. Crotty), 6 μg/mL CpG-2006 (Invivogen, CA), and 1/10,000 Staphylococcus aureus Cowan (SAC) (Sigma-Aldrich, St. Louis, MO) in cRPMI in a total volume of 2.5 mL/well. Cells were fed by adding an additional 2 mL of cRPMI after 2-3 days of incubation. On day 5-6 polyclonally stimulated cells were harvested, 2x10e6 cells plated into 12x75 mm tubes and stained for flow cytometry as described above. A similar panel to the one previously described was used. Samples were collected in a custom LSRII flow cytometer (BD, USA). Samples were analyzed using FlowJo (Tree Star, San Francisco, CA) (Figure 3A).
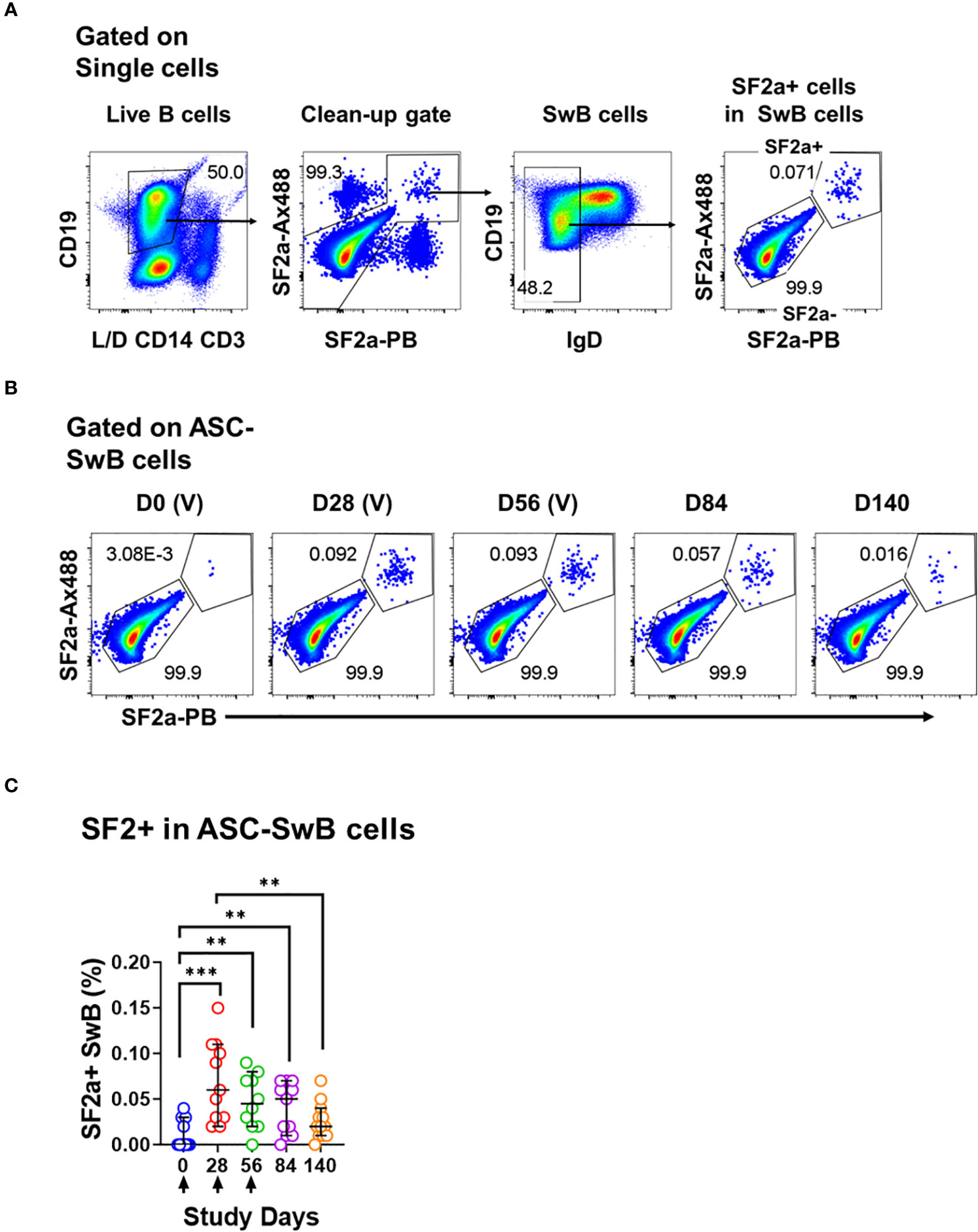
Figure 3 Identification of SF2a+ B cells in ASC by flow cytometry. (A) Shows the gating strategy used to identify SF2+ cells within SwB cells in polyclonally expanded B cells (ASC-transformed). Note that the gating strategy is different than the one used in PBMC. (B) Shows the identification and persistence of SF2a+ cells within SwB cells in ASC-transformed cells in a SF2a-TT15 +/- Alum representative volunteer. (C) Shows the percentage of SF2a+ cells within SwB cells in volunteers that received SF2a-TT15 +/- Alum (n=12). Arrows in X axis show the vaccination days. Median and 95CI are shown. The p values were calculated using unpaired t-tests (2-sided). **p<0.005, ***p<0.001, ****p<0.0001.
B memory assay
Antibody production by activated and ASC differentiated B memory cells were measured using an optimized ELISpot assay for the measurement of IgA spot forming cells (SFC). In short, after 5-6 days of polyclonal stimulation, PBMC (see above) were harvested, counted and seeded in duplicate wells of multi-screen (Cellulose Esters membrane) plates (Millipore, USA) coated with SF2a LPS (Institut Pasteur, France) or goat-anti-human IgA and IgG (Total IgA and IgG control) (Jackson ImmunoResearch, PA) as previously described (35–38). After 5 hours incubation (37°C, 5% CO2), plates were incubated with goat-anti-human IgA- and IgG- horseradish peroxidase (Jackson ImmunoResearch, PA). IgA and IgG SFC were visualized with 3-amino-9-ethylcarbazole (AEC) substrate (Calbiochem, MA). SFC were enumerated using an automated ELISpot reader (Immunospot 3B, Cellular Technologies Ltd, OH) with aid of Immunospot software version 5.0 (Cellular Technologies Ltd). Total and antigen-specific B memory SFC were calculated as SFC/10e6 cells.
Statistical methods
The D’Agostino and Pearson test was used to determine whether the data was normally distributed. The hypotheses in the study were evaluated using two sided tests and p values <0.05 without adjustment for multiple comparisons were considered statistically significant. The frequency of the cells or percentages of the various B cell subsets in vaccinated and placebo volunteers with normally distributed data were compared using unpaired student’s t-tests. Data sets without normal distribution (skewed) comparisons were done using Mann-Whitney tests. Statistical tests were performed in GraphPad Prism v9.3.0 (Boston, MA).
Results
Shigella-specific B cells in circulation after vaccination with SF2a-TT15
We used a method previously developed in our lab to efficiently label killed bacteria with fluorescent dyes (34). For all experiments described in this manuscript, we used S. flexneri 2a (2457T), a wild-type strain used in human challenges (19, 39, 40), labeled with Alexa Fluor 488 (SF2a-Ax488) or Pacific Blue (SF2a-PB) (Supplementary Figure 1C). These bacteria were used as “bait” to identify Shigella-binding B cells (henceforth SF2a-specific B cells or SF2a+ B cells) in specimens from Israeli volunteers who participated in a Phase 1 clinical trial that assessed the conjugate vaccine candidate SF2a-TT15 (21). Volunteers received three injections of SF2a-TT15 adjuvanted or not with Alum on days 0, 28 and 56. Peripheral blood mononuclear cells (PBMC) were collected on days 0, 28, 56, 84 and 140 (Supplementary Figure 1D). Safety and immunogenicity (humoral immunity) were reported elsewhere (21). In the current study, we assessed PBMC from volunteers who had received SF2a-TT15 +/- Alum (10 µg OS dose) (n=12) or the adjuvanted Placebo (Placebo + Alum, n=4).
To assess Shigella-specific B cells, we used equal amounts of bacteria labeled with each fluorochrome (Ax488 and PB; dual tag system). The bacteria were used in conjunction with a high-color flow cytometry panel to characterize different memory B cell subsets including plasmablasts (CD3- CD19+ CD27++ CD38++), activated B cells (ABC; CD3- CD19+ CD27dim/+ CD38dim/+ IgD- CD71+) and class-switched B cells (SwB; CD3- CD19- CD27dim/+ CD38dim/+ IgD-) (Figure 1A). In the gating strategy, we included a gate to eliminate non-specific bacteria binders (Figure 1A; Supplementary Figures 1E, F). The use of antigens labeled with two different fluorochromes has been proven useful to remove confounding factors such as B cells binding to the fluorochromes (41, 42).
SF2a+ cells were identified within SwB cells (memory B cells) (Figures 1A, B). Cohen et al, reported that the groups vaccinated with the 10µg dose of SF2a-TT15, adjuvanted or not, developed comparable anti-LPS IgG antibody titers (21). Similarly, the frequency of SF2a+ cells within SwB cells after day 0 was comparable in these two groups. Hence, in this manuscript, we report the data from these two groups assembled into one group (SF2a-TT15 +/- Alum; n=12). The compiled data showed that SF2a + SwB cells increased significantly after the first immunization (Figures 1B, C) and remained elevated until day 140. The frequency of these cells was significantly higher compared to placebo volunteers on days 28-140 (Supplementary Figure 2A). Like the anti-LPS IgG antibody titers described by Cohen et al. in the Phase 1 trial (21), which did not increase after the vaccine boosters (days 28 and 56), we did not see an increase the frequency of Sf2a+ SwB cells. Small fluctuations in the frequency of these cells between days 28-140 were noted, but not statistical differences were identified (Supplementary Table 1).
Immunophenotypic characteristics of Shigella-specific SwB cells
We further explored the characteristics of SF2a+ SwB cells by assessing markers associated with activation of antigen-specific B cells. CXCR3 is a chemokine receptor whose expression endows cells with the potential to home to inflamed tissues (43–46) and denotes activation. Downregulation of CD21 has been associated with cell activation and transitioning to the ASC stage (47, 48). We compared expression of CXCR3 and CD21 between SF2a+ and SF2a- (bacteria binders and non-binders, respectively) SwB cells in vaccinated volunteers (Figures 4A-C). Of note, on day 0, SF2a+ SwB cells were virtually absent therefore, comparisons of these markers with SF2a- SwB on this day were not possible. SF2a+ SwB cells showed higher expression levels of CXCR3 than SF2a- SwB cells on days 28, 56 and 84 (Figure 4B). In contrast, CD21 was downregulated in SF2a+ SwB cells on days 28, 56, 84 and 140 (Figure 4C). No differences in the expression of CD21 and CXCR3 between days 28-140 in SF2a+ SwB cells were noted. Similarly, the frequency of these markers did not change between days 28-140 in SF2a- cells. The p values of these comparisons are summarized in Supplementary Table 2.
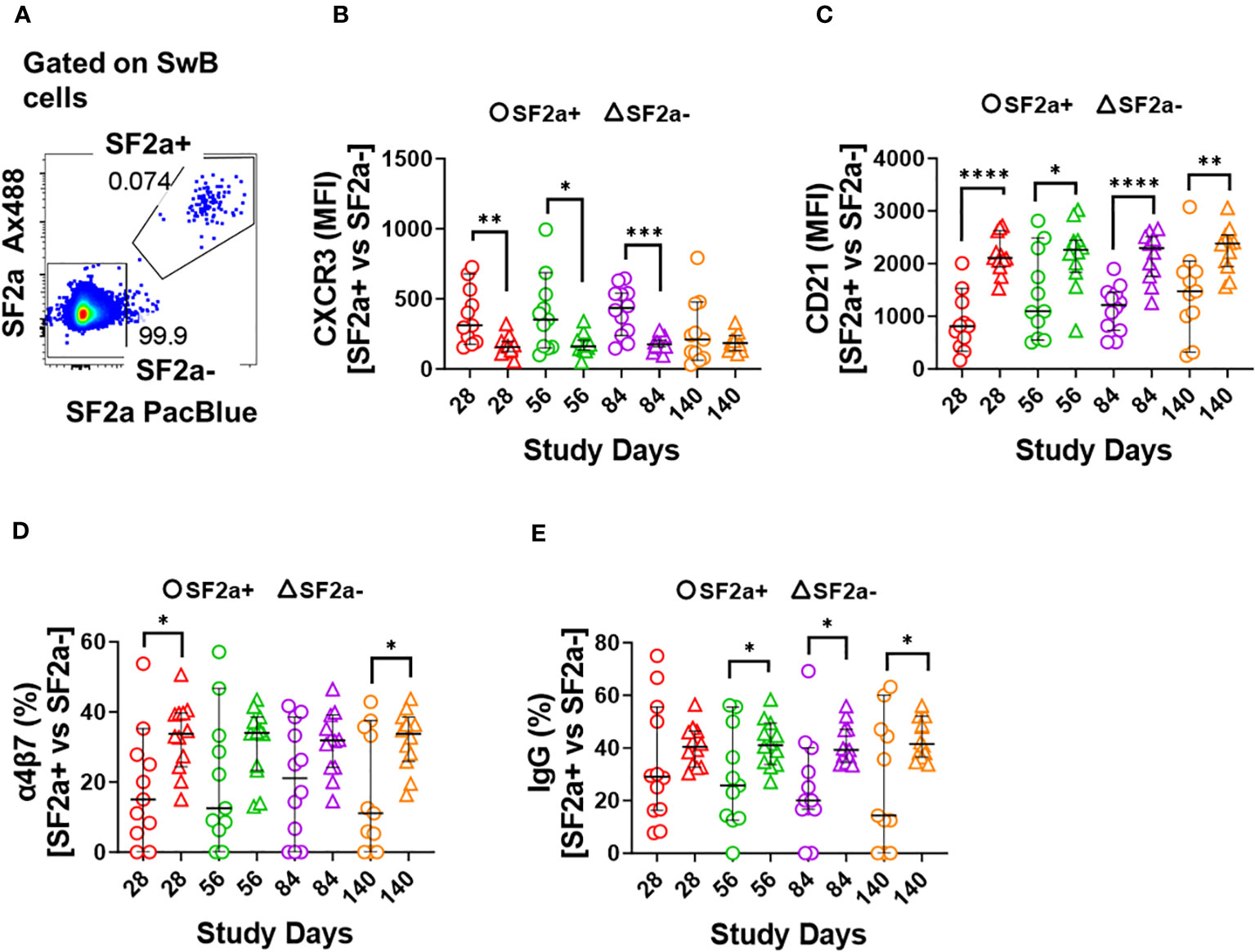
Figure 4 Characteristics of SF2a-specific B cells (SF2a+ SwB cells). Panel (A) shows an example of the gating used to identify SF2a+ and SF2a- cells within SwB cells. Data is from a representative volunteer first vaccine dose. Panels (B, C) show the Median Fluorescence Intensity (MFI) of CXCR3 and CD21, respectively, in SF2a+ and SF2a- SwB cells in vaccinated volunteers. Panels (D, E) display the percentage of SF2a+ and SF2a- SwB cells expressing integrin α4β7 and IgG, respectively. Panels (B-E) show the Median and 95CI. The p values were calculated using unpaired t-tests (2-sided). *p<0.05, **p<0.005, ***p<0.001, ****p<0.0001.
Shigella targets the intestinal mucosa, therefore, it is desirable for a vaccine to induce Shigella-specific immune cells able that migrate to gut associated lymphoid tissues (GALT). The adhesion molecule integrin α4β7 plays a crucial role in controlling lymphocyte migration to the intestine by binding to MAdCAM-1, which is expressed on Peyer’s patch high endothelial venules (HEV) and lamina propria venules, which are part of the GALT (49, 50). We thus assessed the expression of integrin α4β7 in SF2a+ and SF2a- SwB cells. Overall, SF2a+ SwB cells tended to express lower levels of integrin α4β7 than SF2a- SwB cells after vaccination. Statistically significant differences between SF2a+ and SF2a- cells were noted on days 28 and 140 (Figure 4D). We also assessed whether there were differences in IgG (Figure 4E) and IgA (Supplementary Figure 2C) expression between SF2a+ and SF2a- SwB cells. Overall, SF2a+ SwB cells tended to exhibit lower frequencies IgG expression than SF2a- SwB cells, especially on days 56, 84 and 140 (Figure 4E). SF2a+ and SF2a- SwB cells expressed IgA at similar frequencies (Supplementary Figure 2C).
To further explore the immunophenotypic characteristics of SF2a-specific B cells, we performed unsupervised analyses in concatenated SF2a+ SwB cells from all vaccinated volunteer timepoints, except day 0 since no SF2a+ SwB cells were identified at that timepoint (Figure 2A). Cells clusters rendered by a dimensionality reduction analysis (t-SNE) were assessed using X-shift. Three cell clusters were identified and the main distinctive feature between these clusters was the expression of IgG, IgA and IgG-IgA-) (Cluster 1, 2 and 3, respectively) (Cluster explorer) (Figures 2B, C). Interestingly, the IgG-IgA- cluster showed the largest frequency of SF2a+ SwB cells (Figure 2B; Supplementary Figure 2B).
Differentiation into ASC and changes in expression of activation and homing markers
One of the most critical aspects of vaccination is the induction of memory. Upon an antigen re-encounter, antigen-specific memory B cells are rapidly recruited to become ASC and these, in the case of Shigella vaccines, would be desirable to migrate to the gut. SF2a-specific SwB cells identified in PBMC showed an activated phenotype (high CXCR3 and low CD21) with limited ability to migrate to the gut, as compared to SF2a- SwB cells (Figures 4B-D). To explore whether the characteristics of these cells change when they actively secrete antibodies, we differentiated B cells from the vaccinees into ASC using a previously described protocol (ASC-transformed) (35–38) and assessed them by flow cytometry. The gating strategy was different from that used in PBMC and focused primarily in identifying SwB cells (ASC-SwB cells; CD19+/dim IgD-) (Figure 3A) since the differentiation process skews the populations towards plasmablasts and plasma cells phenotypes. Moreover, all these cells were expected to express high levels of activation and proliferation markers making it difficult to define ABCs or other B subsets. SF2a+ ASC-SwB cells were identified at every timepoint of the study, except day 0 (pre-vaccination) (Figures 3B, C). Day 28 appears to be the timepoint with the highest frequency of these cells. Interestingly, by day 140 the frequency of these cells was reduced, and no differences, as compared to day 0 were noted. Moreover, this timepoint (day 140) showed statistical differences compared to day 28 (Figure 3C; Supplementary Table 3). Since SF2a+ ASC-SwB cells were virtually absent at day 0, we report results of various activation/immunophenotyping markers from days 28 to 140. SF2a+ ASC-SwB cells (Figure 5A) showed significantly higher expression levels of CXCR3 than SF2a- ASC-SwB cells on days 28 and 56 (Figure 5B). SF2a+ ASC-SwB tended to express lower levels of CD21 than in SF2a- ASC-SwB cells across all timepoints, but statistical significance was only shown on day 28 (Figure 5C). SF2a+ ASC-SwB cells tended to have higher expression levels of integrin α4β7 than SF2a- ASC-SwB; however, statistical significance was shown only on cells from day 140 of the study (Figure 5D). Moreover, ASC SF2a+ SwB cells showed active proliferation as suggested by the higher expression levels of CD71 (Figure 5E) on days 28, 56, 84 and 140. We also assessed expression of markers associated with plasmablast and plasma cells differentiation (CD27++ and CD38++). Significantly higher percentages of CD38++CD27++ cells were observed in SF2a+ ASC-SwB cells than in SF2a- ASC-SwB cells (Figure 5F; Supplementary Figure 3B). We also compared changes in the expression of the integrin α4β7, CXCR3 and CD21 in SF2+ and SF2- SwB cells between PBMC and ASC-transformed cells. For these analyses, data from timepoints 28-140 were grouped (Supplementary Figures 4A-C). Integrin α4β7 expression increased when the cells were differentiated into ASC (Supplementary Figure 4A). Of note, SF2a+ ASC-SwB cells expressed higher levels of this molecule than SF2a- ASC-SwB cells, which contrasted with the expression of this marker in PBMC. Compared to PBMC, ASC-transformed expressed higher levels of CXCR3. Moreover, SF2a+ SwB cells in both PBMC and ASC expressed higher levels than SF2a- SwB cells (Supplementary Figure 4B). Finally, ASC differentiation decreased the expression of CD21 and SF2a+ SwB cells in both PBMC and ASC expressed the lowest levels of this marker, compared to SF2a- SwB cells (Supplementary Figure 4C).
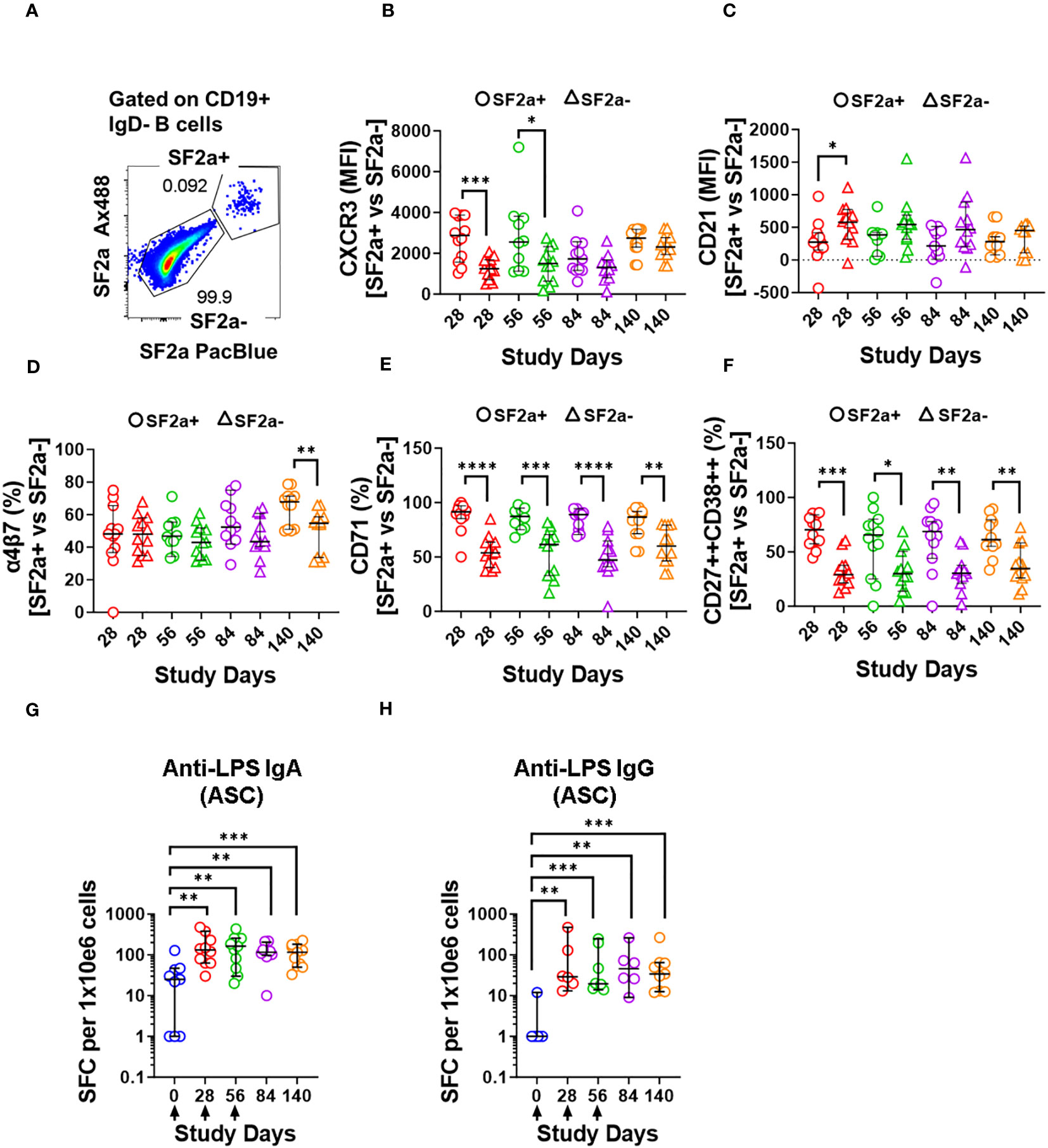
Figure 5 Characteristics of SF2a+ B cells in ASC-differentiated cells. Panel (A) shows an example of the gating used to identify SF2a+ and SF2a- in ASC-SwB cells. Data is from a representative volunteer post-vaccination. Panels (B, C) show the median fluorescence intensity (MFI) of CXCR3 and CD21 in SF2a+ and SF2a- ASC-SwB cells in SF2a-TT15 +/- Alum vaccinated volunteers. Panels (D, E) display the percentage of SF2a+ and SF2a- in ASC-SwB cells expressing integrin α4β7 and CD71. (F) displays the percentages of SF2a+ and SF2a- ASC-SwB cells with characteristics of plasmablast/plasma cells [CD27++ CD38++] (look also Supplementary Figure 3B). Panel (G, H) show the frequency of anti-LPS IgA and IgG spot forming cells -SFC- per 1x10e6 cells in volunteers that received SF2a-TT15 +/- Alum. Arrows in the X axis show the vaccination days. Panels (B-H) show the median and 95CI. The p values of panels (B-F) were calculated using unpaired t-tests (2-sided). *p<0.05, **p<0.005, ***p<0.001, ****p<0.0001. The p values of panels (G, H) were calculated using unpaired Mann Whitney tests (2-sided). **p<0.005, ***p<0.001.
Since we differentiated B cells into ASC, it was important to assess whether these cells were producing vaccine specific antibodies. To this end, we assessed the anti-LPS IgA and IgG production by ELISpot in the volunteers whose samples had sufficient cells to perform these assays. Anti-LPS IgA was assessed in 9-12 vaccinated volunteers per timepoint and in 3-4 placebo volunteers per timepoint. Anti-LPS IgG was assessed in 6-9 vaccinated and 1-3 placebo volunteer per timepoint (Supplementary Figure 5). In vaccinated volunteers, the frequency of anti-LPS IgA and IgG ASC increased significantly after day 0 (Figures 5G, H). None of the other timepoints showed statistical differences among them (Supplementary Table 4).
Discussion
The B cell compartment is critical for protection against Shigella infections (17–19, 38, 51–55). To date, development of humoral responses after Shigella infection or vaccination has been assessed using classical immunological methods such as ELISA and ELISpot. However, a better understanding of the immunophenotypic features of Shigella-specific B cells is missing. The use of molecular probes labeled with fluorescent dyes or other tags has been employed to study, in detail, the development of humoral immunity to viruses such as influenza virus and SARS-CoV-2 (56–62), but not for bacteria, to date. To address this gap, and as a proof-of-principle study, we used fluorescently labeled SF2a bacteria to identify Shigella-specific B cells in specimens from human volunteers who had received the vaccine candidate SF2a-TT15 (21).
SF2a-TT15 is a synthetic carbohydrate conjugate vaccine, whereby the glycan component is a unique 15mer oligosaccharide designed to achieve SF2a O-antigen functional mimicry. We opted to use whole bacteria as “bait” to identify antigen-specific B cells, to ensure that this vaccine induced B cells able to recognize the LPS from wild-type SF2a. Our fluorescent labeling strategy targets free amines on surface proteins of the bacteria; thus, the O-antigen was not modified during the labeling process and maintained its natural structure. We incorporated bacteria labeled with two different fluorochromes (Ax488 and PB) into high-color flow cytometry panels to reduce the possibility of measuring non-specific B cell binders which have the potential to obscure the results (Figure 1A; Supplementary Figures 1E, F). Using this strategy, we identified SF2a-specific B cells in the B memory cell compartment at every timepoint (28-140 days) after the first immunization, except day 0 (pre-vaccination) (Figures 1B, C; Supplementary Figure 2A). The absence of SF2a+ B cells in the plasmablast and ABC compartments is likely due to the timepoints available for these assessments. Antigen-specific B cells are usually identified in the plasmablast and ABC compartments ~8 days and ~2 weeks after vaccination/infection, respectively. Samples corresponding to these timepoints were not available from these vaccinees.
In PBMC, SF2a+ SwB cells expressed high levels CXCR3 and low levels of CD21. Expression of CXCR3 has been associated with the ability of cells to migrate to inflamed tissues (43–46). On the other hand, CD21 downregulation, in the context of antigen-specific B cells, has been associated with cells that left the germinal center (GC) and are primed for plasma cell differentiation (48). Importantly, CD21-low antigen-specific B cells (63, 64) have also been shown to express high levels of T-bet (65) and FcLR5 (66). CXCR3 is a transcription target of T-bet and a recent paper showed that co-expression of CXCR3 with T-bet and FcLR5 distinguishes a subset of effector memory B cells able to rapidly differentiate into antibody secreting cells (66). Taken together, the cells we identified (SF2a+ SwB cells) in SF2a-TT15 vaccinees are likely effector memory B cells, which are primed to become ASC. This would be the first report of these cells being elicited by vaccination for an enteric bacterial pathogen.
IgG and IgA expressing B cells were identified within SF2+ SwB cells in PBMC (Figure 1E; Supplementary Figure 2C). Considering that SF2a-TT15 was delivered intramuscularly, it is not surprising that IgG was the predominant isotype. The unsupervised analysis suggested that IgG was one-third more abundant than IgA (~30% vs. 20% respectively) in SF2a+ SwB cells (Figure 2B, group 1 vs. group 2). A significant percentage of SF2a+ B cells were IgG-IgA- (Figure 2; Supplementary Figure 2B). The significance of this finding is unclear at this time. Of note, SF2a- SwB cells also showed a proportion of IgG-IgA- cells (Supplementary Figure 3B). Other studies have shown similar results (significant proportion of IgG-IgA- B cells) in non-antigen-specific B cells (67–69), but no clear explanation for these findings has been provided. Future studies will address this aspect in more detail.
One critical function of memory B cells is reactivation and differentiation into ASC once a re-infection occurs. We differentiated B cells from SF2a-TT15 vaccinees into ASC to determine their ability to secrete anti-LPS IgA and IgG by ELISpot and the immunophenotypic characteristics of SF2a+ ASC-SwB cells by flow cytometry. As expected, SF2a+ ASC-SwB cells were identified only in vaccinated volunteers (Figures 3B, C; Supplementary Figure 3). These cells continued to express high levels of CXCR3 (significant on days 28 and 56) and low levels of CD21 (significant on day 28), suggesting that they maintained the characteristics of effector memory cells. Further evidence of this came from comparisons of the degree of expression of these markers between PBMC and ASC-transformed [cumulative analysis including all datapoints after vaccination (days 28 to 140)]. ASC differentiation increased the expression of CXCR3 and downregulated CD21; however, SF2a+ ASC-SwB cells showed the highest and lowest levels of CXCR3 (Supplementary Figure 4B) and CD21 expression (Supplementary Figure 4C), respectively. Interestingly, the frequency of SF2a+ ASC-SwB cells appeared to be reduced in the later timepoints, which might suggest that in some volunteers the memory compartment was contracting. Nevertheless, in several volunteers, memory B cells with the ability to produce anti-LPS IgG and IgA antibodies were still present at this timepoint, as shown by the ASC assessments (Figures 5G, H).
These results are concordant with the memory responses reported by Cohen et al. (21) in the Phase I trial, where memory responses, assessed by ELISpot, were shown to be present until day 140. A long-term follow-up of these volunteers was recently published showing that memory B cells (ELISpot) returned to almost baseline levels two years after vaccination. Nevertheless, up to 89% of the volunteers that received 10 μg OS maintained >4-fold titers of anti-SF2a LPS IgG compared to their pre-vaccination levels (70). It is important to note that antibodies detected in sera long after vaccination are produced by long-lived plasma cells (LLPC) that reside in the bone marrow (71, 72). LLPC are not memory B cells since the latter are defined as antigen-experienced quiescent B cells that can become re-activated to rapidly produce antibodies (73–75). Hence, assessment of circulating memory B cells provide only partial information on the B memory compartment. Moreover, the VDJ sequences in circulating memory B cells and serum antibodies do not always fully match due to their different cell origins. Similarly, the frequencies of circulating B cells do not always correlate with the serum antibody titers for a given antigen. We believe that the SF2a+ SwB cells identified in this manuscript represent a group of memory cells ready to be differentiated into antibody secreting cells in recall responses.
Another notable change of SF2a+ ASC-SwB cells, compared to PBMC, involved the upregulation of integrin α4β7 (Supplementary Figure 4A; Figure 4D vs. 5D). The cumulative datapoint analysis of PBMC vs. ASC showed that SF2a+ ASC-SwB cells expressed higher levels of integrin α4β7 than SF2a- ASC-SwB (Supplementary Figure 4A). This suggests that vaccination with SF2a-TT15 induces B cells that express integrin α4β7, but these are probably in circulation only for few days after vaccination. Likely, we did not identify them in circulation (PBMC samples) because by the time of blood sampling, these cells have already migrated to GALT. Nevertheless, re-activation of SF2a+ SwB cells (ASC differentiation) triggered the expression of this marker again, confirming the potential of these cells to migrate to the GALT once they start to secrete antibodies. It is also possible that SF2a-TT15 induces only limited expression of integrin α4β7 and that this marker is upregulated in ASC-transformed cells due to the intense in vitro stimulation. Future studies in which plasmablasts from SF2a-TT15 recipients are sorted based on the expression of i) gut-homing (e.g., integrin α4β7) or ii) peripheral lymphoid organ-homing (e.g., CD62L) markers and assessed for anti-LPS antibody production (76) could provide more clear answers.
As expected, all polyclonally stimulated B cells expressed high levels of CD71. However, in SF2a+ ASC-SwB cells the expression of this marker was double than in SF2a- ASC-SwB cells (Figure 3E), which suggests that SF2a+ cells were proliferating at higher rates than other differentiated non-specific ASC. Moreover, in the ELISpot assay, it was clear that anti-LPS IgA and IgG ASC increased in frequency after day 0 (Figures 5G, H).
Overall, we developed a method to identify circulating SF2a-specific B cells. Using this approach, we demonstrated that SF2a-TT15 induced circulating SF2a+ SwB cells, reflecting the induction of memory cells. These results confirm and markedly expand previous published data by Cohen et al. (21) that showed the induction of memory B cells (by ELISpot) in these volunteers. Herein, the proof-of-concept for the potential of this novel technology was achieved in samples from volunteers enrolled in a first-in-human study for SF2a-TT15, a synthetic carbohydrate-based vaccine candidate now being assayed in phase 2 clinical trials. This methodology is less labor intensive than classic ELISpot methods and could be used to easily track the presence of antigen-specific memory B cells in circulation after vaccination or infection with SF2a. Moreover, it can provide a wealth of information on the characteristics of antigen-specific B cells to enteric bacteria and allow detailed examination of the dynamic development of SF2a-specific B cells involving other subsets (e.g., ABCs). For these studies, appropriate timepoints will be collected in the future. Moreover, this methodology will be combined with other antigen probes already developed in our lab (e.g., LPS fluorescent nanoparticles (31, 32), Core-O-polysaccharides-FITC, IpaB-PacBlue, IpaD-AlexaFluor700) to study the development of B cell immunity to multiple specific Shigella antigens in specimens of volunteers vaccinated and then challenged with wild-type Shigella. The probe used to identify antigen-specific B cells (whole bacteria, LPS, or purified protein) will depend on the type of vaccine used and the antigen of interest. These reagents will also be combined with next generation sequencing for single-cell assessments of clonality to diverse antigens, and the generation of recombinant antibodies which can be used as standards across multi-center studies.
Data availability statement
The raw data supporting the conclusions of this article will be made available by the authors, without undue reservation.
Ethics statement
The requirement of ethical approval was waived by The University of Maryland Baltimore IRB for the studies on humans because the specimens received were de-identified; hence, this project was determined to be “Not human research”. The studies were conducted in accordance with the local legislation and institutional requirements. Written informed consent for participation was not required from the participants or the participants’ legal guardians/next of kin in accordance with the national legislation and institutional requirements. The human samples used in this study were acquired from primarily isolated specimens collected as part of a previous study for which ethical approval was obtained.
Author contributions
FT: Conceptualization, Formal Analysis, Funding acquisition, Investigation, Methodology, Supervision, Writing – original draft, Writing – review & editing, Project administration, Resources. JH: Formal Analysis, Methodology, Writing – original draft, Writing – review & editing. SM: Resources, Writing – review & editing. LM: Data curation, Writing – review & editing. AP: Data curation, Writing – review & editing. DC: Resources, Writing – review & editing. MS: Conceptualization, Funding acquisition, Investigation, Resources, Supervision, Writing – original draft, Writing – review & editing.
Funding
The author(s) declare financial support was received for the research, authorship, and/or publication of this article. This work was supported, in part, by NIAID, NIH, DHHS federal research grants R01 AI036525 and U19 AI082655 (Cooperative Center for Human Immunology [CCHI]) to MS and the European Union Seventh Framework Program, STOPENTERICS grant no. 261472 to DC, AP and LAM, and grant number OPP1195433 from the Bill & Melinda Gates Foundation to DC. FT was supported in part by a Pilot Project funded by the University of Maryland Claude D. Pepper Older Americans Independence Center (UM-AOCIC) from the National Institute on Aging (NIA) Claude D. Pepper Older Americans Independence Center P30-AG028747.
Acknowledgments
We are indebted to the volunteers who participated in the studies. The content is solely the responsibility of the authors and does not necessarily represent the official views of the National Institute of Allergy and Infectious Diseases or the National Institutes of Health or other granting institutions. We would like to acknowledge Ms. Regina Harley from the CVD Flow and Mass Cytometry Core. Flow cytometry experiments were performed at the Flow Cytometry and Mass Cytometry Core Facility of the University of Maryland School of Medicine Center for Innovative Biomedical Resources (CIBR), Baltimore, Maryland.
Conflict of interest
The authors declare that the research was conducted in the absence of any commercial or financial relationships that could be construed as a potential conflict of interest.
Publisher’s note
All claims expressed in this article are solely those of the authors and do not necessarily represent those of their affiliated organizations, or those of the publisher, the editors and the reviewers. Any product that may be evaluated in this article, or claim that may be made by its manufacturer, is not guaranteed or endorsed by the publisher.
Supplementary material
The Supplementary Material for this article can be found online at: https://www.frontiersin.org/articles/10.3389/fimmu.2023.1291664/full#supplementary-material
References
1. Khalil IA, Troeger C, Blacker BF, Rao PC, Brown A, Atherly DE, et al. Morbidity and mortality due to Shigella and enterotoxigenic Escherichia coli diarrhoea: the Global Burden of Disease Study 1990-2016. Lancet Infect Dis (2018) 18(11):1229–40. doi: 10.1016/S1473-3099(18)30475-4
2. Kotloff KL, Riddle MS, Platts-Mills JA, Pavlinac P, Zaidi AKM. Shigellosis. Lancet (2018) 391(10122):801–12. doi: 10.1016/S0140-6736(17)33296-8
3. Libby TE, Delawalla MLM, Al-Shimari F, MacLennan CA, Vannice KS, Pavlinac PB. Consequences of Shigella infection in young children: a systematic review. Int J Infect Dis (2023) 129:78–95. doi: 10.1016/j.ijid.2023.01.034
4. Livio S, Strockbine NA, Panchalingam S, Tennant SM, Barry EM, Marohn ME, et al. Shigella isolates from the global enteric multicenter study inform vaccine development. Clin Infect Dis (2014) 59(7):933–41. doi: 10.1093/cid/ciu468
5. Ram PK, Crump JA, Gupta SK, Miller MA, Mintz ED. Part II. Analysis of data gaps pertaining to Shigella infections in low and medium human development index countries, 1984-2005. Epidemiol Infect (2008) 136(5):577–603. doi: 10.1017/S0950268807009351
6. Scallan E, Hoekstra RM, Angulo FJ, Tauxe RV, Widdowson MA, Roy SL, et al. Foodborne illness acquired in the United States–major pathogens. Emerg Infect Dis (2011) 17(1):7–15. doi: 10.3201/eid1701.P11101
7. Kendall ME, Crim S, Fullerton K, Han PV, Cronquist AB, Shiferaw B, et al. Travel-associated enteric infections diagnosed after return to the United States, Foodborne Diseases Active Surveillance Network (FoodNet), 2004-2009. Clin Infect Dis (2012) 54 Suppl 5:S480–7. doi: 10.1093/cid/cis052
8. Ashbaugh HR, Early JM, Johnson ME, Simons MP, Graf PCF, Riddle MS, et al. A prospective observational study describing severity of acquired diarrhea among U.S. military and Western travelers participating in the Global Travelers' Diarrhea Study. Travel Med Infect Dis (2021) 43:102139. doi: 10.1016/j.tmaid.2021.102139
9. Caruso E, Wright ER, Respress ET, Evener SL, Jacobson K, Bowen A, et al. Shigellosis among gay and bisexual men: a qualitative assessment to examine knowledge, attitudes, and practices. Sex Transm Dis (2020) 47(9):596–601. doi: 10.1097/OLQ.0000000000001220
10. Thorley K, Charles H, Greig DR, Prochazka M, Mason LCE, Baker KS, et al. Emergence of extensively drug-resistant and multidrug-resistant Shigella flexneri serotype 2a associated with sexual transmission among gay, bisexual, and other men who have sex with men, in England: a descriptive epidemiological study. Lancet Infect Dis (2023) 23(6):732–9. doi: 10.1016/S1473-3099(22)00807-6
11. Charles H, Prochazka M, Thorley K, Crewdson A, Greig DR, Jenkins C, et al. Outbreak of sexually transmitted, extensively drug-resistant Shigella sonnei in the UK, 2021-22: a descriptive epidemiological study. Lancet Infect Dis (2022) 22(10):1503–10. doi: 10.1016/S1473-3099(22)00370-X
12. Baker KS, Dallman TJ, Ashton PM, Day M, Hughes G, Crook PD, et al. Intercontinental dissemination of azithromycin-resistant shigellosis through sexual transmission: a cross-sectional study. Lancet Infect Dis (2015) 15(8):913–21. doi: 10.1016/S1473-3099(15)00002-X
13. Bowen A, Grass J, Bicknese A, Campbell D, Hurd J, Kirkcaldy RD. Elevated risk for antimicrobial drug-resistant Shigella infection among men who have sex with men, United States, 2011-2015. Emerg Infect Dis (2016) 22(9):1613–6. doi: 10.3201/eid2209.160624
14. CDC. Centers for Disease Control and Prevention - Bioterrorism Agents/Diseases. Available at: https://emergency.cdc.gov/agent/agentlist-category.asp#b.
15. Taylor DN, Echeverria P, Pal T, Sethabutr O, Saiborisuth S, Sricharmorn S, et al. The role of Shigella spp., enteroinvasive Escherichia coli, and other enteropathogens as causes of childhood dysentery in Thailand. J Infect Dis (1986) 153(6):1132–8. doi: 10.1093/infdis/153.6.1132
16. Ferreccio C, Prado V, Ojeda A, Cayyazo M, Abrego P, Guers L, et al. Epidemiologic patterns of acute diarrhea and endemic Shigella infections in children in a poor periurban setting in Santiago, Chile. Am J Epidemiol (1991) 134(6):614–27. doi: 10.1093/oxfordjournals.aje.a116134
17. Cohen D, Green MS, Block C, Slepon R, Lerman Y. Natural immunity to shigellosis in two groups with different previous risks of exposure to Shigella is only partly expressed by serum antibodies to lipopolysaccharide. J Infect Dis (1992) 165(4):785–7. doi: 10.1093/infdis/165.4.785
18. Herrington DA, Van de Verg L, Formal SB, Hale TL, Tall BD, Cryz SJ, et al. Studies in volunteers to evaluate candidate Shigella vaccines: further experience with a bivalent Salmonella typhi-Shigella sonnei vaccine and protection conferred by previous Shigella sonnei disease. Vaccine (1990) 8(4):353–7. doi: 10.1016/0264-410X(90)90094-3
19. Kotloff KL, Nataro JP, Losonsky GA, Wasserman SS, Hale TL, Taylor DN, et al. A modified Shigella volunteer challenge model in which the inoculum is administered with bicarbonate buffer: clinical experience and implications for Shigella infectivity. Vaccine (1995) 13(16):1488–94. doi: 10.1016/0264-410X(95)00102-7
20. Cohen D, Ashkenazi S, Schneerson R, Farzam N, Bialik A, Meron-Sudai S, et al. Threshold protective levels of serum IgG to Shigella lipopolysaccharide: re-analysis of Shigella vaccine trials data. Clin Microbiol Infect (2023) 29(3):366–71. doi: 10.1016/j.cmi.2022.10.011
21. Cohen D, Atsmon J, Artaud C, Meron-Sudai S, Gougeon ML, Bialik A, et al. Safety and immunogenicity of a synthetic carbohydrate conjugate vaccine against Shigella flexneri 2a in healthy adult volunteers: a phase 1, dose-escalating, single-blind, randomised, placebo-controlled study. Lancet Infect Dis (2021) 21(4):546–58. doi: 10.1016/S1473-3099(20)30488-6
22. Passwell JH. Safety and immunogenicity of Shigella sonnei-CRM9 and Shigella flexneri type 2a-rEPAsucc conjugate vaccines in one- to four-year-old children. Pediatr Infect Dis J (2003) 22:701–6. doi: 10.1097/01.inf.0000078156.03697.a5
23. Phalipon A. Characterization of functional oligosaccharide mimics of the Shigella flexneri serotype 2a O-antigen: implications for the development of a chemically defined glycoconjugate vaccine. J Immunol (2006) 176:1686–94. doi: 10.4049/jimmunol.176.3.1686
24. Phalipon A, Tanguy M, Grandjean C, Guerreiro C, Belot F, Cohen D, et al. A synthetic carbohydrate-protein conjugate vaccine candidate against Shigella flexneri 2a infection. J Immunol (2009) 182(4):2241–7. doi: 10.4049/jimmunol.0803141
25. Ashkenazi S. Safety and immunogenicity of Shigella sonnei and Shigella flexneri 2a O-specific polysaccharide conjugates in children. J Infect Dis (1999) 179:1565–8. doi: 10.1086/314759
26. Cohen D, Ashkenazi S, Green MS, Gdalevich M, Robin G, Slepon R, et al. Double-blind vaccine-controlled randomised efficacy trial of an investigational Shigella sonnei conjugate vaccine in young adults. Lancet (1997) 349(9046):155–9. doi: 10.1016/S0140-6736(96)06255-1
27. Passwell JH, Ashkenzi S, Banet-Levi Y, Ramon-Saraf R, Farzam N, Lerner-Geva L, et al. Age-related efficacy of Shigella O-specific polysaccharide conjugates in 1-4-year-old Israeli children. Vaccine (2010) 28(10):2231–5. doi: 10.1016/j.vaccine.2009.12.050
28. van der Put RMF, Smitsman C, de Haan A, Hamzink M, Timmermans H, Uittenbogaard J, et al. The first-in-human synthetic glycan-based conjugate vaccine candidate against Shigella. ACS Cent Sci (2022) 8(4):449–60. doi: 10.1021/acscentsci.1c01479
29. Bernshtein B, Ndungo E, Cizmeci D, Xu P, Kovac P, Kelly M, et al. Systems approach to define humoral correlates of immunity to Shigella. Cell Rep (2022) 40(7):111216. doi: 10.1016/j.celrep.2022.111216
30. Booth JS, Toapanta FR, Salerno-Goncalves R, Patil S, Kader H, Safta A, et al. Characterization and functional properties of gastric tissue-resident memory T cells from children, adults and the elderly. Front Immunol (2014) 5. doi: 10.3389/fimmu.2014.00294
31. Toapanta FR, Bernal PJ, Fresnay S, Darton TC, Jones C, Waddington CS, et al. Oral wild-type salmonella typhi challenge induces activation of circulating monocytes and dendritic cells in individuals who develop typhoid disease. PloS Negl Trop Dis (2015) 9(6):e0003837. doi: 10.1371/journal.pntd.0003837
32. Toapanta FR, Bernal PJ, Fresnay S, Magder LS, Darton TC, Jones C, et al. Oral challenge with wild-type Salmonella typhi induces distinct changes in B cell subsets in individuals who develop typhoid disease. PloS Negl Trop Dis (2016) 10(6):e0004766. doi: 10.1371/journal.pntd.0004766
33. Toapanta FR, Bernal PJ, Kotloff KL, Levine MM, Sztein MB. T cell mediated immunity induced by the live-attenuated Shigella flexneri 2a vaccine candidate CVD 1208S in humans. J Trans Med (2018) 16(1):61. doi: 10.1186/s12967-018-1439-1
34. Toapanta FR, Bernal PJ, Sztein MB. Diverse phosphorylation patterns of B cell receptor-associated signaling in naive and memory human B cells revealed by phosphoflow, a powerful technique to study signaling at the single cell level. Front Cell Infect Microbiol (2012) 2:128. doi: 10.3389/fcimb.2012.00128
35. Crotty S, Aubert RD, Glidewell J, Ahmed R. Tracking human antigen-specific memory B cells: a sensitive and generalized ELISPOT system. J Immunol Methods (2004) 286(1-2):111–22. doi: 10.1016/j.jim.2003.12.015
36. Simon JK, Maciel M, Weld ED, Wahid R, Pasetti MF, Picking WL, et al. Antigen-specific IgA B memory cell responses to Shigella antigens elicited in volunteers immunized with live attenuated Shigella flexneri 2a oral vaccine candidates. Clin Immunol (2011) 139(2):185–92. doi: 10.1016/j.clim.2011.02.003
37. Simon JK, Wahid R, Maciel M, Picking WL, Kotloff KL, Levine MM, et al. Antigen-specific B memory cell responses to lipopolysaccharide (LPS) and invasion plasmid antigen (Ipa) B elicited in volunteers vaccinated with live-attenuated Shigella flexneri 2a vaccine candidates. Vaccine (2009) 27(4):565–72. doi: 10.1016/j.vaccine.2008.10.081
38. Wahid R, Simon JK, Picking WL, Kotloff KL, Levine MM, Sztein MB. Shigella antigen-specific B memory cells are associated with decreased disease severity in subjects challenged with wild-type Shigella flexneri 2a. Clin Immunol (2013) 148(1):35–43. doi: 10.1016/j.clim.2013.03.009
39. Taylor DN, McKenzie R, Durbin A, Carpenter C, Atzinger CB, Haake R, et al. Rifaximin, a nonabsorbed oral antibiotic, prevents shigellosis after experimental challenge. Clin Infect Dis (2006) 42(9):1283–8. doi: 10.1086/503039
40. Porter CK, Thura N, Ranallo RT, Riddle MS. The Shigella human challenge model. Epidemiol Infect (2013) 141(2):223–32. doi: 10.1017/S0950268812001677
41. Boonyaratanakornkit J, Taylor JJ. Techniques to study antigen-specific B cell responses. Front Immunol (2019) 10:1694. doi: 10.3389/fimmu.2019.01694
42. Gilman MS, Castellanos CA, Chen M, Ngwuta JO, Goodwin E, Moin SM, et al. Rapid profiling of RSV antibody repertoires from the memory B cells of naturally infected adult donors. Sci Immunol (2016) 1(6). doi: 10.1126/sciimmunol.aaj1879
43. Manz RA, Hauser AE, Hiepe F, Radbruch A. Maintenance of serum antibody levels. Annu Rev Immunol (2005) 23:367–86. doi: 10.1146/annurev.immunol.23.021704.115723
44. Phares TW, Marques CP, Stohlman SA, Hinton DR, Bergmann CC. Factors supporting intrathecal humoral responses following viral encephalomyelitis. J Virol (2011) 85(6):2589–98. doi: 10.1128/JVI.02260-10
45. Tschen SI, Stohlman SA, Ramakrishna C, Hinton DR, Atkinson RD, Bergmann CC. CNS viral infection diverts homing of antibody-secreting cells from lymphoid organs to the CNS. Eur J Immunol (2006) 36(3):603–12. doi: 10.1002/eji.200535123
46. Tsubaki T, Takegawa S, Hanamoto H, Arita N, Kamogawa J, Yamamoto H, et al. Accumulation of plasma cells expressing CXCR3 in the synovial sublining regions of early rheumatoid arthritis in association with production of Mig/CXCL9 by synovial fibroblasts. Clin Exp Immunol (2005) 141(2):363–71. doi: 10.1111/j.1365-2249.2005.02850.x
47. Knox JJ, Buggert M, Kardava L, Seaton KE, Eller MA, Canaday DH, et al. T-bet+ B cells are induced by human viral infections and dominate the HIV gp140 response. JCI Insight (2017) 2(8). doi: 10.1172/jci.insight.92943
48. Lau D, Lan LY, Andrews SF, Henry C, Rojas KT, Neu KE, et al. Low CD21 expression defines a population of recent germinal center graduates primed for plasma cell differentiation. Sci Immunol (2017) 2(7). doi: 10.1126/sciimmunol.aai8153
49. Habtezion A, Nguyen LP, Hadeiba H, Butcher EC. Leukocyte trafficking to the small intestine and colon. Gastroenterology (2016) 150(2):340–54. doi: 10.1053/j.gastro.2015.10.046
50. Lamb CA, O'Byrne S, Keir ME, Butcher EC. Gut-selective integrin-targeted therapies for inflammatory bowel disease. J Crohns Colitis (2018) 12(suppl_2):S653–S68. doi: 10.1093/ecco-jcc/jjy060
51. Cohen D, Green MS, Block C, Rouach T, Ofek I. Serum antibodies to lipopolysaccharide and natural immunity to shigellosis in an Israeli military population. J Infect Dis (1988) 157:1068–71. doi: 10.1093/infdis/157.5.1068
52. Kotloff KL. Evaluation of the safety, immunogenicity and efficacy in healthy adults of four doses of live oral hybrid Escherichia coli-Shigella flexneri 2a vaccine strain EcSf2a-2. Vaccine (1995) 13:495–502. doi: 10.1016/0264-410X(94)00011-B
53. Levine MM, Kotloff KL, Barry EM, Pasetti MF, Sztein MB. Clinical trials of Shigella vaccines: two steps forward and one step back on a long, hard road. Nat Rev Microbiol (2007) 5(7):540–53. doi: 10.1038/nrmicro1662
54. Black RE, Levine MM, Clements ML, Losonsky G, Herrington D, Berman S, et al. Prevention of shigellosis by a Salmonella typhi-Shigella sonnei bivalent vaccine. J Infect Dis (1987) 155(6):1260–5. doi: 10.1093/infdis/155.6.1260
55. Shimanovich AA, Buskirk AD, Heine SJ, Blackwelder WC, Wahid R, Kotloff KL, et al. Functional and antigen-specific serum antibody levels as correlates of protection against shigellosis in a controlled human challenge study. Clin Vaccine Immunol (2017) 24(2). doi: 10.1128/CVI.00412-16
56. Nguyen-Contant P, Embong AK, Kanagaiah P, Chaves FA, Yang H, Branche AR, et al. S protein-reactive IgG and memory B cell production after human SARS-CoV-2 infection includes broad reactivity to the S2 subunit. mBio (2020) 11(5). doi: 10.1128/mBio.01991-20
57. Zimmermann M, Rose N, Lindner JM, Kim H, Goncalves AR, Callegari I, et al. Antigen extraction and B cell activation enable identification of rare membrane antigen specific human B cells. Front Immunol (2019) 10:829. doi: 10.3389/fimmu.2019.00829
58. Cohen KW, Linderman SL, Moodie Z, Czartoski J, Lai L, Mantus G, et al. Longitudinal analysis shows durable and broad immune memory after SARS-CoV-2 infection with persisting antibody responses and memory B and T cells. Cell Rep Med (2021) 2(7):100354. doi: 10.1016/j.xcrm.2021.100354
59. Turner JS, Kim W, Kalaidina E, Goss CW, Rauseo AM, Schmitz AJ, et al. SARS-CoV-2 infection induces long-lived bone marrow plasma cells in humans. Nature (2021) 595(7867):421–5. doi: 10.1038/s41586-021-03647-4
60. Turner JS, Zhou JQ, Han J, Schmitz AJ, Rizk AA, Alsoussi WB, et al. Human germinal centres engage memory and naive B cells after influenza vaccination. Nature (2020) 586(7827):127–32. doi: 10.1038/s41586-020-2711-0
61. Jegaskanda S, Andrews SF, Wheatley AK, Yewdell JW, McDermott AB, Subbarao K. Hemagglutinin head-specific responses dominate over stem-specific responses following prime boost with mismatched vaccines. JCI Insight (2019) 4(22). doi: 10.1172/jci.insight.129035
62. Andrews SF, Chambers MJ, Schramm CA, Plyler J, Raab JE, Kanekiyo M, et al. Activation dynamics and immunoglobulin evolution of pre-existing and newly generated human memory B cell responses to influenza hemagglutinin. Immunity (2019) 51(2):398–410 e5. doi: 10.1016/j.immuni.2019.06.024
63. Ellebedy AH, Jackson KJ, Kissick HT, Nakaya HI, Davis CW, Roskin KM, et al. Defining antigen-specific plasmablast and memory B cell subsets in human blood after viral infection or vaccination. Nat Immunol (2016) 17(10):1226–34. doi: 10.1038/ni.3533
64. Andrews SF, Huang Y, Kaur K, Popova LI, Ho IY, Pauli NT, et al. Immune history profoundly affects broadly protective B cell responses to influenza. Sci Transl Med (2015) 7(316):316ra192. doi: 10.1126/scitranslmed.aad0522
65. Johnson JL, Rosenthal RL, Knox JJ, Myles A, Naradikian MS, Madej J, et al. The transcription factor T-bet resolves memory B cell subsets with distinct tissue distributions and antibody specificities in mice and humans. Immunity (2020) 52(5):842–55 e6. doi: 10.1016/j.immuni.2020.03.020
66. Nellore A, Zumaquero E, Scharer CD, Fucile CF, Tipton CM, King RG, et al. A transcriptionally distinct subset of influenza-specific effector memory B cells predicts long-lived antibody responses to vaccination in humans. Immunity (2023) 56(4):847–63 e8. doi: 10.1016/j.immuni.2023.03.001
67. Nettey L, Ballard R, Liechti T, Mason RD. OMIP 074: Phenotypic analysis of IgG and IgA subclasses on human B cells. Cytometry A (2021) 99(9):880–3. doi: 10.1002/cyto.a.24341
68. Moroney JB, Vasudev A, Pertsemlidis A, Zan H, Casali P. Integrative transcriptome and chromatin landscape analysis reveals distinct epigenetic regulations in human memory B cells. Nat Commun (2020) 11(1):5435. doi: 10.1038/s41467-020-19242-6
69. Lemarquis AL, Einarsdottir HK, Kristjansdottir RN, Jonsdottir I, Ludviksson BR. Transitional B cells and TLR9 responses are defective in selective IgA deficiency. Front Immunol (2018) 9:909. doi: 10.3389/fimmu.2018.00909
70. Meron-Sudai S, Asato V, Adler A, Bialik A, Goren S, Ariel-Cohen O, et al. A Shigella flexneri 2a synthetic glycan-based vaccine induces a long-lasting immune response in adults. NPJ Vaccines (2023) 8(1):35. doi: 10.1038/s41541-023-00624-y
71. Palm AE, Henry C. Remembrance of things past: long-term B cell memory after infection and vaccination. Front Immunol (2019) 10:1787. doi: 10.3389/fimmu.2019.01787
72. Brynjolfsson SF, Mohaddes M, Karrholm J, Wick MJ. Long-lived plasma cells in human bone marrow can be either CD19(+) or CD19(). Blood Adv (2017) 1(13):835–8. doi: 10.1182/bloodadvances.2017004481
73. Dogan I, Bertocci B, Vilmont V, Delbos F, Megret J, Storck S, et al. Multiple layers of B cell memory with different effector functions. Nat Immunol (2009) 10(12):1292–9. doi: 10.1038/ni.1814
74. Yoshida T, Mei H, Dorner T, Hiepe F, Radbruch A, Fillatreau S, et al. Memory B and memory plasma cells. Immunol Rev (2010) 237(1):117–39. doi: 10.1111/j.1600-065X.2010.00938.x
75. Pape KA, Taylor JJ, Maul RW, Gearhart PJ, Jenkins MK. Different B cell populations mediate early and late memory during an endogenous immune response. Science (2011) 331(6021):1203–7. doi: 10.1126/science.1201730
Keywords: Shigella, conjugate vaccine, synthetic carbohydrate, memory B cells, antigen-specific B cells
Citation: Toapanta FR, Hu J, Meron-Sudai S, Mulard LA, Phalipon A, Cohen D and Sztein MB (2023) Further characterization of Shigella-specific (memory) B cells induced in healthy volunteer recipients of SF2a-TT15, a Shigella flexneri 2a synthetic glycan-based vaccine candidate. Front. Immunol. 14:1291664. doi: 10.3389/fimmu.2023.1291664
Received: 09 September 2023; Accepted: 18 October 2023;
Published: 31 October 2023.
Edited by:
Francesca Micoli, GlaxoSmithKline, ItalyReviewed by:
Ahmet Eken, Erciyes University, TürkiyeGanesh Ram Visweswaran, Seattle Children’s Hospital, United States
Copyright © 2023 Toapanta, Hu, Meron-Sudai, Mulard, Phalipon, Cohen and Sztein. This is an open-access article distributed under the terms of the Creative Commons Attribution License (CC BY). The use, distribution or reproduction in other forums is permitted, provided the original author(s) and the copyright owner(s) are credited and that the original publication in this journal is cited, in accordance with accepted academic practice. No use, distribution or reproduction is permitted which does not comply with these terms.
*Correspondence: Franklin R. Toapanta, ftoapanta@som.umaryland.edu; Marcelo B. Sztein, Msztein@som.umaryland.edu