- 1Department of Epidemiology, School of Public Health, Southern Medical University, Guangzhou, China
- 2Institute of Evidence-Based Medicine, Heping Hospital Affiliated to Changzhi Medical College, Changzhi, China
- 3Office of Emergency, Shenzhen Center for Disease Control and Prevention, Shenzhen, China
- 4Third Class of 2019 of Clinical Medicine, Suzhou Medical College, Soochow University, Suzhou, Jiangsu, China
- 5Department of Biochemistry, Changzhi Medical College, Changzhi, China
- 6Department of Infectious Diseases, Nanfang Hospital, Southern Medical University, Guangzhou, China
We conducted a retrospective cohort study to evaluate the transmission risk of severe acute respiratory syndrome coronavirus 2 (SARS-CoV-2) Omicron BA.2 variant and the effectiveness of inactivated COVID-19 vaccine boosters in Shenzhen during a BA.2 outbreak period from 1 February to 21 April 2022. A total of 1,248 individuals were infected with the BA.2 variant, and 7,855 close contacts were carefully investigated. The risk factors for the high secondary attack rate of SARS-CoV-2 infection were household contacts [adjusted odds ratio (aOR): 1.748; 95% confidence interval (CI): 1.448, 2.110], younger individuals aged 0–17 years (aOR: 2.730; 95% CI: 2.118, 3.518), older persons aged ≥60 years (aOR: 1.342; 95% CI: 1.135, 1.588), women (aOR: 1.442; 95% CI: 1.210, 1.718), and the subjects exposed to the post-onset index cases (aOR: 8.546; 95% CI: 6.610, 11.050), respectively. Compared with the unvaccinated and partially vaccinated individuals, a relatively low risk of secondary attack was found for the individuals who received booster vaccination (aOR: 0.871; 95% CI: 0.761, 0.997). Moreover, a high transmission risk was found for the index cases aged ≥60 years (aOR: 1.359; 95% CI: 1.132, 1.632), whereas a relatively low transmission risk was observed for the index cases who received full vaccination (aOR: 0.642; 95% CI: 0.490, 0.841) and booster vaccination (aOR: 0.676; 95% CI: 0.594, 0.770). Compared with full vaccination, booster vaccination of inactivated COVID-19 vaccine showed an effectiveness of 24.0% (95% CI: 7.0%, 37.9%) against BA.2 transmission for the adults ≥18 years and 93.7% (95% CI: 72.4%, 98.6%) for the adults ≥60 years, whereas the effectiveness was 51.0% (95% CI: 21.9%, 69.3%) for the individuals of 14 days to 179 days after booster vaccination and 51.2% (95% CI: 37.5%, 61.9%) for the non-household contacts. The estimated mean values of the generation interval, serial interval, incubation period, latent period, and viral shedding period were 2.7 days, 3.2 days, 2.4 days, 2.1 days, and 17.9 days, respectively. In summary, our results confirmed that the main transmission route of Omicron BA.2 subvariant was household contact, and booster vaccination of the inactivated vaccines was relatively effective against BA.2 subvariant transmission in older people.
Introduction
The severe acute respiratory syndrome coronavirus 2 (SARS-CoV-2) Omicron (B.1.1.529) variant was first identified in South Africa on 24 November 2021; it was categorized as a variant of concern on 26 November 2021, by the World Health Organization (1). In January 2022, over 98% of new infections were caused by the Omicron variant worldwide (2). Moreover, the Omicron variant had over 50 mutations and 26–35 amino acids on the spike protein that differed from all previous variants (3). Furthermore, Omicron had stronger transmissibility than other variants, making infection more difficult to control (4–6). Between February and April 2022, an Omicron BA.2 sub-lineage outbreak occurred in Shenzhen, Guangdong, China.
It is crucial to understand the transmission characteristics of the Omicron BA.2 sub-lineage in order to develop strategies to prevent future epidemic or outbreaks. Moreover, a clearer understanding of the Omicron BA.2 sub-lineage will aid in understanding the time-interval distribution of the crucial spreading events. For instance, knowing the incubation period or the time from infection to clinical symptoms can help us determine the necessary durations of isolation and quarantine periods for the close contacts (7). In addition, the generation interval (GI) or the time from index case infection to secondary attack infection (SAI) may provide insight into the measures needed to track close contacts (8). Therefore, it is essential to analyze the distribution of the time intervals of the key spreading events for the Omicron BA.2 sub-lineage (9, 10).
Several studies have reported the vaccine effectiveness (VE) of inactivated COVID-19 vaccines against Omicron BA.2 with regard to various infection outcomes in China (11–15). Although one study evaluated VE against Omicron BA.5.2 sub-lineage transmission in China (16), more studies are definitely needed to answer the questions about the extent to which the inactivated vaccines are related to the risk of transmission (17). In this study, we conducted a retrospective cohort study to evaluate the characteristics, dynamics, and risk of transmission of SARS-CoV-2 Omicron variant infection, to estimate the effectiveness of inactivated vaccine boosters against transmission in Shenzhen during an Omicron BA.2 sub-lineage outbreak period from 1 February to 21 April 2022.
Methods
Study setting and design
This study was conducted as a retrospective cohort study and included all individuals with laboratory-confirmed infections and their close contacts between 1 February and 21 April 2022, in Shenzhen, Guangdong, China, during an Omicron BA.2 sub-lineage outbreak period. This report was developed using the S1 STROBE Checklist. The first Omicron BA.2–infected individual was identified on 1 February 2022, in Shenzhen, after which the variant quickly spread among the population. In response to the epidemic of COVID-19, including Omicron infection, very stringent preventive measures were quickly implemented and included by the local government (1): identification and isolation of SARS-CoV-2–infected subjects and their close contacts. All the infected patients were asked to stay and treated in the hospitals of infectious diseases, whereas all the close contacts (i.e., household and non-household) were exclusively quarantined in the specific facilities to receive medical observation and screening for SARS-CoV-2 every 2–3 days by reverse transcription polymerase chain reaction (RT-PCR) tests for SARS-CoV-2 to monitor whether they were infected with SARS-CoV-2 (2); in the areas of COVID-19 epidemic, people were asked to stay in the home and screen for SARS-CoV-2 every 2–3 days to identify any potential SARS-CoV-2 infection due to potential delays in viral load reaching detectable levels; and (3) when necessary, some districts or even the whole city may be locked down to prevent the expansion of COVID-19 epidemic. These measures influenced the transmission and resulted in lower secondary attack rate of SARS-CoV-2 BA.2 infection.
SARS-CoV-2 infections
Data on Omicron BA.2 sub-lineage–infected individuals and their close contacts were retrospectively collected between 1 February and 21 April 2022, from Shenzhen Center for Disease Control and Prevention in China. For individuals with BA.2 sub-lineage infection, we extracted data, which mainly included age, sex, history of exposure, contact setting, onset date of clinical symptoms, first positive test date, serial PCR test results, and history of COVID-19 vaccination. In this study, SARS-CoV-2 infection was indicated by a positive nucleic acid amplification test regardless of illness severity. Asymptomatic infection referred to a positive nucleic acid amplification test without clinical symptoms (12). Symptomatic COVID-19 was defined as PCR-confirmed infection with any COVID-19 clinical symptom. COVID-19 pneumonia was diagnosed on the basis of characteristics of chest computed tomography imaging.
Close contacts and transmission pairs
Close contacts were individuals in the same exposure settings within proximity of a COVID-19–infected individual without any effective protection (18). Exposure settings for close contacts included household and non-household settings (19). In this study, individuals at risk of exposure were considered to be close contacts of confirmed cases. Those close contacts who eventually tested positive for COVID-19 were treated as infected individuals (infectees) and their index cases (who were originally confirmed to have COVID-19) as infectors. We extracted these epidemiologically linked infectees and infector pairs and their individual data for all transmission pairs. Figure 1 shows a flowchart of the sample selection procedure for transmission pairs.
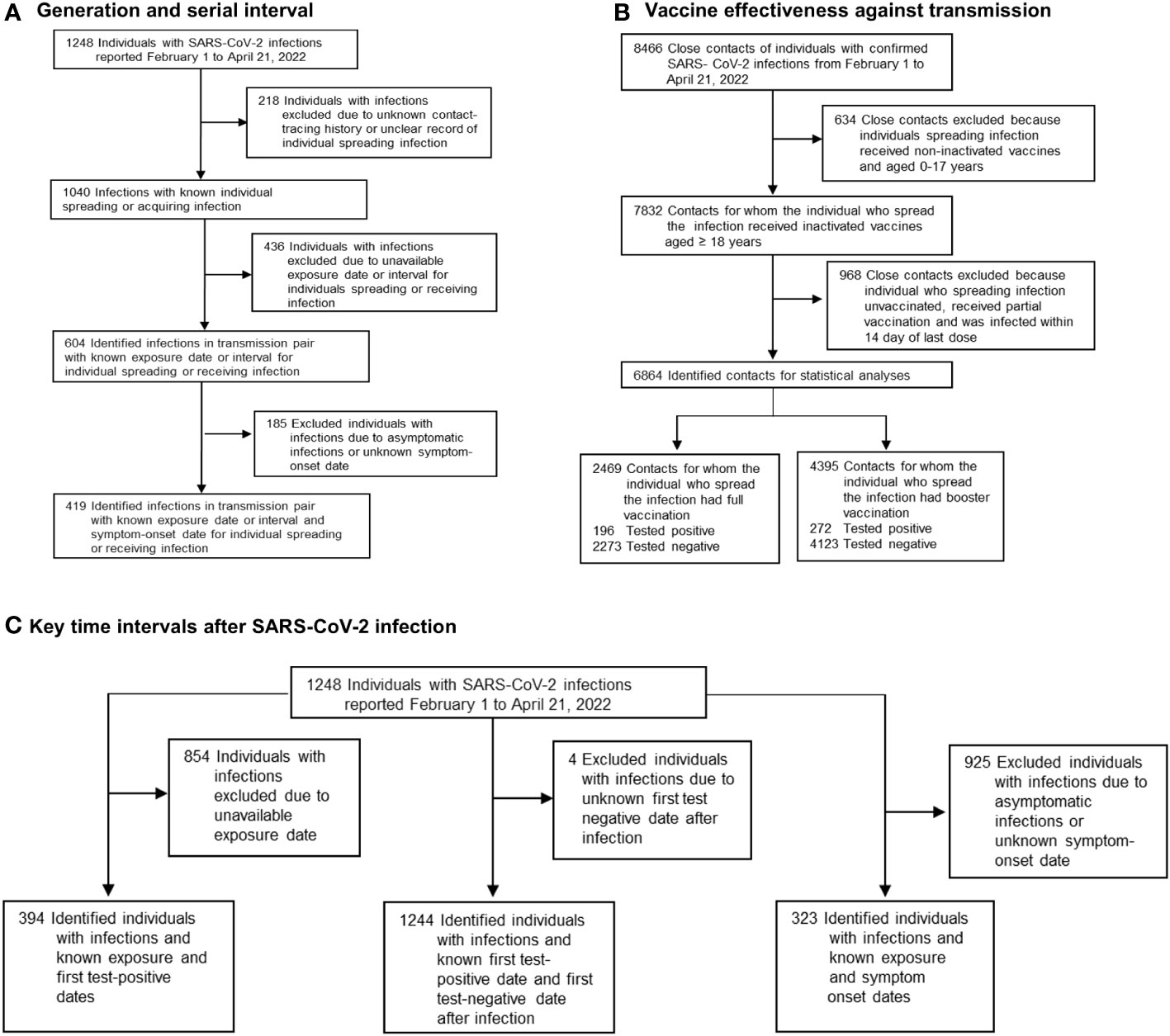
Figure 1 Flowchart of sample selection in this study. (A) The sample selection procedure for transmission pairs that were used for estimating generation and serial interval is presented. (B) The sample selection procedure for eligible close contacts who were used for estimating vaccine effectiveness against transmission is presented. (C) The sample selection procedure for eligible SARS-CoV-2 infections that were used for estimating the period from exposure to viral shedding, viral shedding period, and incubation period is presented.
Vaccination status
We classified the individuals infected with the BA.2 sub-lineage and their close contacts into an unvaccinated group, a partially vaccinated group, a fully vaccinated group, and a booster-vaccinated group based on their electronic COVID-19 vaccination records. The unvaccinated group had received no COVID-19 vaccines before their last known contact with a confirmed infected individual or COVID-19 onset time. The partial vaccination group included those who had their first dose of viral vector (non-replicating) COVID-19 vaccines <14 days, their first dose of inactivated COVID-19 vaccine ≥0 days or their second dose of inactivated COVID-19 vaccine <14 days, or their first and second doses of protein subunit COVID-19 vaccines ≥0 days or third dose of protein subunit COVID-19 vaccine <14 days before their last known contact or symptom onset time. Full vaccination included those ≥14 days after their first dose of viral vector (non-replicating) COVID-19 vaccine, ≥14 days after their second dose of inactivated COVID-19 vaccine, ≥14 days after their third dose of protein subunit COVID-19 vaccine, and <7 days after booster vaccination before their last known contact or onset time. The booster vaccination group included those ≥7 days after their second dose of viral vector (non-replicating) COVID-19 vaccine and ≥7 days after their third dose of inactivated COVID-19 vaccine or other third dose of heterologous COVID-19 vaccine before their last known contact or onset time. To evaluate the inactivated COVID-19 VE against transmission, the full vaccination group included only those ≥14 days after their second dose, excluding those 0–7 days after their third dose, and the booster vaccination group included only those ≥14 days after their third dose because index cases who received their booster vaccination within 7–13 days were absent in this study.
Statistical analysis
Baseline characteristics were calculated as the number (%) for categorical variables and the median [interquartile range (IQR)] for continuous variables. Differences in proportions and median values were analyzed with the chi-squared and Wilcoxon tests, respectively. The secondary attack rate (7, 20) was estimated by dividing the number of individuals with secondary infections by the overall number of close contacts related to index cases. Moreover, we analyzed the proportion of cases of supercritical transmission (SCT), which referred to cases with an individual transmission number ≥1 (21, 22).
To assess the risk of SAI with the Omicron BA.2 sub-lineage, a stepwise logistic regression model was used involving age, age of index case, sex, sex of index case, contact setting, type of index case, COVID-19 vaccination status of close contacts, COVID-19 vaccination status of index case, and exposure to index case before or after onset. Of the index cases in this study, most were in the groups with full and booster vaccinations with inactivated COVID-19 vaccines. Therefore, we focused on evaluating the effectiveness of inactivated vaccine boosters against transmission compared with that of full vaccination. The crude odds ratio (OR) was calculated by applying a univariate logistic regression model, and the adjusted OR (aOR) was calculated using a multivariable ordinary logistic regression model by adjusting for potential confounding variables, including sex of index case and their close contacts, age of index case and their close contacts, contact setting, COVID-19 vaccination status of close contacts, and exposure to an index case before or after onset. The crude or adjusted VE (aVE) was calculated as (1 − OR) × 100%, and the OR is the odds ratio for the incidence of secondary infection (23–25). Subgroups were evaluated on the basis of age, time interval of COVID-19 vaccination, and contact setting. All statistical analyses were conducted with IBM SPSS Statistics 25.0.
Then, the time intervals between key events were estimated involving GI, serial interval (SI), latent period, viral shedding period, and incubation period with gamma distributions using a Bayesian framework (16). All of these analyses were performed with the rstan package in R software (R Foundation for Statistical Computing, Vienna, Austria). GI was estimated as the mean duration between the time of SAI with known exposure time and time of infection of index case. SI was estimated as the mean duration between the onset time of clinical symptoms in index cases and the onset time of clinical symptoms in secondary cases generated by those index cases. Moreover, we estimated the distribution of the latent period, from exposure to viral shedding, using the first positive test date as an alternative to the time of viral shedding; we included cases with a known exposure date or a first positive PCR test date. Furthermore, we estimated the viral shedding period using the first positive PCR test date and the first negative test date as the end date of viral shedding. Finally, the incubation period was estimated using symptomatic cases with a known exposure date. Subgroup analyses were conducted according to contact setting, age, symptom status, and vaccination status. We estimated the daily instantaneous reproduction number (Rt) with the EpiEstim package (7) in R software.
Results
In this study, we identified 7,855 uninfected close contacts {4,822 men [61.4%]; mean age, 36.1 [standard deviation (SD): 13.0] years} and 1,248 individuals infected with Omicron BA.2 [710 men (56.9%); mean age, 34.4 (SD: 16.5) years]. Of the infected individuals, 203 (16.3%) were asymptomatic and 1,045 (83.7%) were symptomatic (Supplementary Table 1). Among the 1,045 symptomatic cases, 975 (93.3%) presented with mild symptoms and 70 (6.7%) had COVID-19 pneumonia (Supplementary Table 1). The most frequently reported symptoms were pyrexia [573 (60.5%) of 947], cough [341 (36.0%)], pharyngeal pain [223 (23.5%)], and fatigue [135 (14.3%)] (Supplementary Table 2 and Supplementary Figure 1). The number of daily infections continuously increased until reaching a peak of 107 infections on 15 March 2022. Thereafter, the number of daily infections gradually decreased to one infection on 21 April 2022 (Supplementary Figure 2).
Characteristics of close contacts and index cases
We included 8,466 close contacts associated with 644 index cases. Among the 644 index cases, 368 (57.1%) were men, and the median age was 35.0 years (IQR: 27.0, 47.0), with 545 (84.6%) index cases aged between 20 years and 59 years (Table 1). The majority of index cases had mild COVID-19 (510, 79.2%) or asymptomatic infection (101, 15.7%), whereas the remaining cases had COVID-19 pneumonia (33, 5.1%) (Table 1). Among the index cases, there were 66 (10.3%), 29 (4.5%), 232 (36.0%), and 317 (49.2%) cases in the unvaccinated, partially vaccinated, fully vaccinated, and booster-vaccinated groups, respectively (Table 1). We also found that the cycle threshold (Ct) values for the first positive test were 24.0 (IQR: 20.0, 29.0) and 23.0 (IQR: 18.0, 28.0) for the ORF1ab and N genes, respectively (Table 1). Finally, significant differences (P < 0.05) were observed for age and Ct value of the first positive test for the ORF1ab or N gene between the transmitted index cases (216, 33.5%) and non-transmitted index cases (428, 66.5%) (Table 1).
Among the 8,466 close contacts, 5,150 (52.8%) were men, with a median age of 34.0 years (IQR: 27.0, 45.0 years) and 7,402 (87.4%) individuals aged between 20 years and 59 years (Table 2). In terms of contact setting, there were 1,568 (18.5%) and 6,898 (81.5%) household and non-household settings, respectively (Table 2). Contact frequency was categorized as “occasionally or sometimes” for 7,258 (85.7%) individuals and “frequently” for 1,208 (14.3%) (Table 2). Moreover, 737 (8.7%) close contacts did not receive any COVID-19 vaccines, and the percentages in the partial, full, and booster vaccination groups were 3.3%, 36.8%, and 51.1%, respectively (Table 2). Furthermore, of the 8,466 close contacts, 611 {7.2% [95% confidence interval (CI): 6.7%, 7.8%]} were secondarily infected, 85 [1.0% (95% CI: 0.8%, 1.2%)] were asymptomatic, 499 [5.9% (95% CI: 5.4%, 6.4%)] had mild COVID-19, and 27 [0.3% (95% CI: 0.2% to 0.5%)] had COVID-19 pneumonia. Of these 611 cases, 85 (13.9%) presented with no clinical symptoms and 526 (86.1%) presented with clinical symptoms (Table 2). Finally, significant differences (P < 0.05) were found for age, gender, contact setting, contact frequency, and COVID-19 vaccination status between the SAI and non-infected groups (Table 2).
Incidence of SAI and factors associated with transmission risk
Of the 8,466 close contacts, 611 were SAI cases caused by Omicron BA.2, with an incidence of 7.2% (95% CI: 6.7%, 7.8%) (Table 3). There were two secondary attack and spreading infection peaks in the groups of individuals aged 0–9 years old and ≥60 years old (Supplementary Figure 3). Moreover, the SCT rate was higher for individuals spreading infection among the group of individuals aged ≥60 years old [26.8% (95% CI: 15.7%, 41.9%)] (Supplementary Table 3).
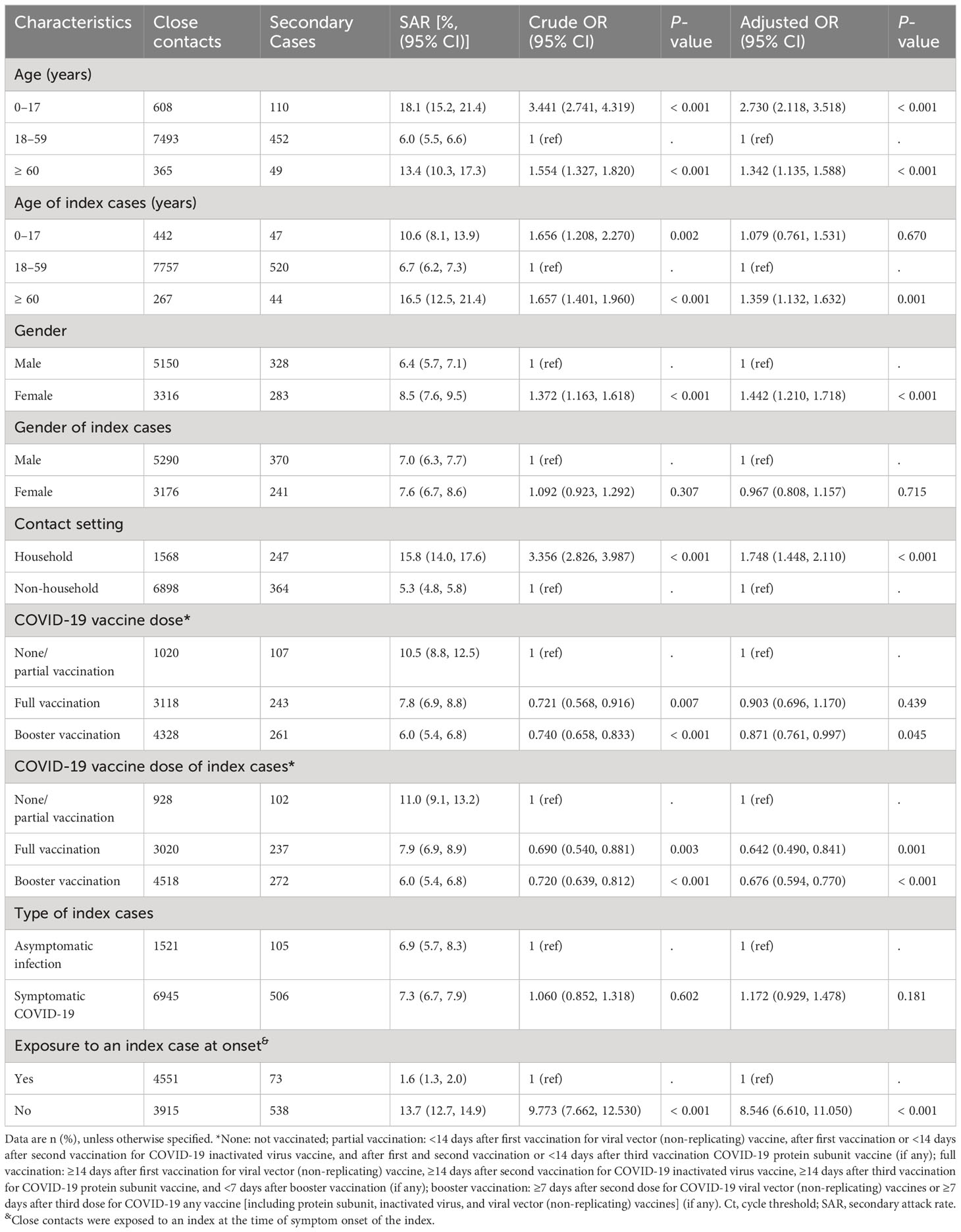
Table 3 Estimating the association of demographic and behavioral factors with the risk of acquiring and transmitting SARS-CoV-2 Omicron BA.2.
Next, we found that the close contacts aged 0–17 years old [aOR: 2.730 (95% CI: 2.118, 3.518)] and ≥60 years old [aOR: 1.342 (95% CI: 1.135, 1.588)] had a higher SAI risk than those aged 18–59 years old (Table 3). Moreover, we observed that female contacts had a slightly higher SAI risk (aOR: 1.442; 95% CI: 1.210, 1.718) than the male contacts (Table 3). Among the exposure settings, household contacts were associated with a higher risk of SAI (aOR: 1.748; 95% CI: 1.448, 2.110) versus non-household contacts (Table 3). A lower SAI rate was found for the close contacts who received full vaccination compared with those who were unvaccinated and partially vaccinated [7.8% (95% CI: 6.9%, 8.8%) versus 10.5% (95% CI: 8.8% to 12.5%)] (Table 3), but this difference was not significant according to multivariate analysis. However, a lower risk of SAI was found for the close contacts who received booster vaccination (aOR: 0.871; 95% CI: 0.761, 0.997) compared with unvaccinated and partially vaccinated close contacts (Table 3). Moreover, the close contacts with exposure to an index case after onset had a higher SAI risk (aOR: 8.546; 95% CI: 6.610, 11.05) compared with those exposed to an index case before onset (Table 3). The frequency of contact was not separately evaluated due to multicollinearity with the household contacts.
A higher transmission rate was also found for the index cases aged 0–17 years [10.6% (95% CI: 8.1%, 13.9%)] compared with those aged 18–59 years [6.7% (95% CI: 6.2%, 7.3%)] (Table 3). A relatively high transmission risk was found for index cases aged ≥60 years (aOR: 1.359; 95% CI: 1.132, 1.632) compared with those aged 18–59 years. In terms of COVID-19 vaccination status of index cases, a lower transmission risk was observed for index cases who received full vaccination (aOR: 0.642; 95% CI: 0.490, 0.841) and booster vaccination (aOR: 0.676; 95% CI: 0.594, 0.770) compared with those who were unvaccinated and partially vaccinated.
VE against transmission of the Omicron BA.2 variant
Overall, 6,864 close contacts were related to 502 index cases who spread infections; their data were analyzed to assess VE of inactivated COVID-19 vaccines against BA.2 transmission for those aged ≥18 years old (Table 4). Of the close contacts, 2,469 (36.0%) and 4,395 close contacts (64.0%) received full and booster vaccinations, respectively. Among index cases, 184 (36.7%) and 318 (63.3%) individuals received full and booster vaccinations, respectively. Overall, the aVE was 24.0% (95% CI: 7.0%, 37.9%) against BA.2 transmission for booster vaccination compared with full vaccination. An aVE against transmission of 93.7% (95% CI: 72.4%, 98.6%) was found among those aged ≥60 years. Moreover, the aVE against transmission remained high 14 to 179 days after booster vaccination [51.0% (95% CI: 21.9%, 69.3%)]. We also found that the aVE [51.2% (95% CI: 37.5%, 61.9%)] against transmission was high for the non-household contact setting.
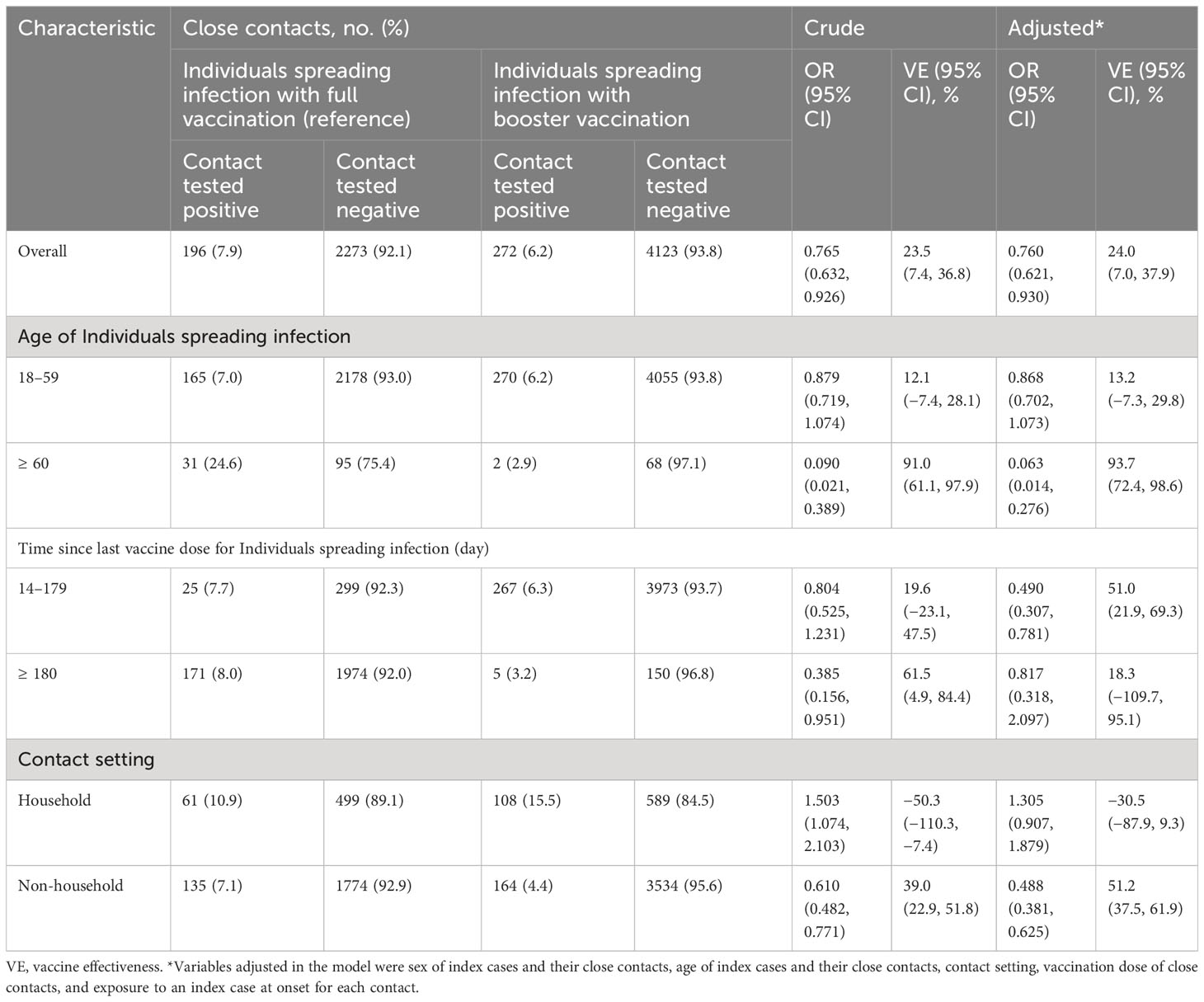
Table 4 Inactivated vaccine effectiveness of booster vaccination vs full vaccination against transmission.
Estimation of time intervals between key events
We analyzed data from 604 transmission pairs to estimate the GI, which was 2.7 days [97.5% credible interval (CrI): −0.8 to 6.7 days] (Table 5; Figure 2A). In a subgroup analysis based on contact setting, the mean GI estimate was longer for non-household settings than for household settings [2.9 days (97.5% CrI: −0.8 to 7.0 days) versus 2.4 days (97.5% CrI: −0.9 to 6.0 days), respectively] (Supplementary Table 4). Moreover, 419 transmission pairs were used to estimate SI, which was 3.2 days (97.5% Crl: −0.6 to 7.4 days) (Table 5; Figure 2B). According to subgroup analysis based on contact setting, the mean SI estimate was similar in non-household and household settings [3.2 days (97.5% CrI: −0.7 to 7.6 days) versus 3.2 days (97.5% CrI: −0.5 to 7.2 days)] (Supplementary Table 4). Furthermore, 323 transmission pairs were used to estimate the mean incubation period of 2.4 days (97.5% CrI: 0.0 to 9.9 days) (Table 5; Figure 2C). Individuals aged 18–59 years had a longer mean incubation period than the overall average (Supplementary Table 4). The mean latent period was 2.1 days (97.5% CrI: 0.0 to 9.7 days) (Table 5; Figure 2D) based on an analysis of the data from 394 transmission pairs, although individuals aged 0–17 years had a shorter mean latent period (Supplementary Table 4). Finally, we found that the mean viral shedding period was 17.9 days (97.5% CrI: 9.4, 28.5 days) (Table 5; Figure 2E) according to analysis of data from 1,244 individuals with BA.2 infection. A shorter mean viral shedding period (Supplementary Table 4) was observed among asymptomatic individuals aged 0–17 years. The estimated reproduction number declined from 2.8 on 14 February 2022 to 0.4 on 21 April 2022 (Figure 3).
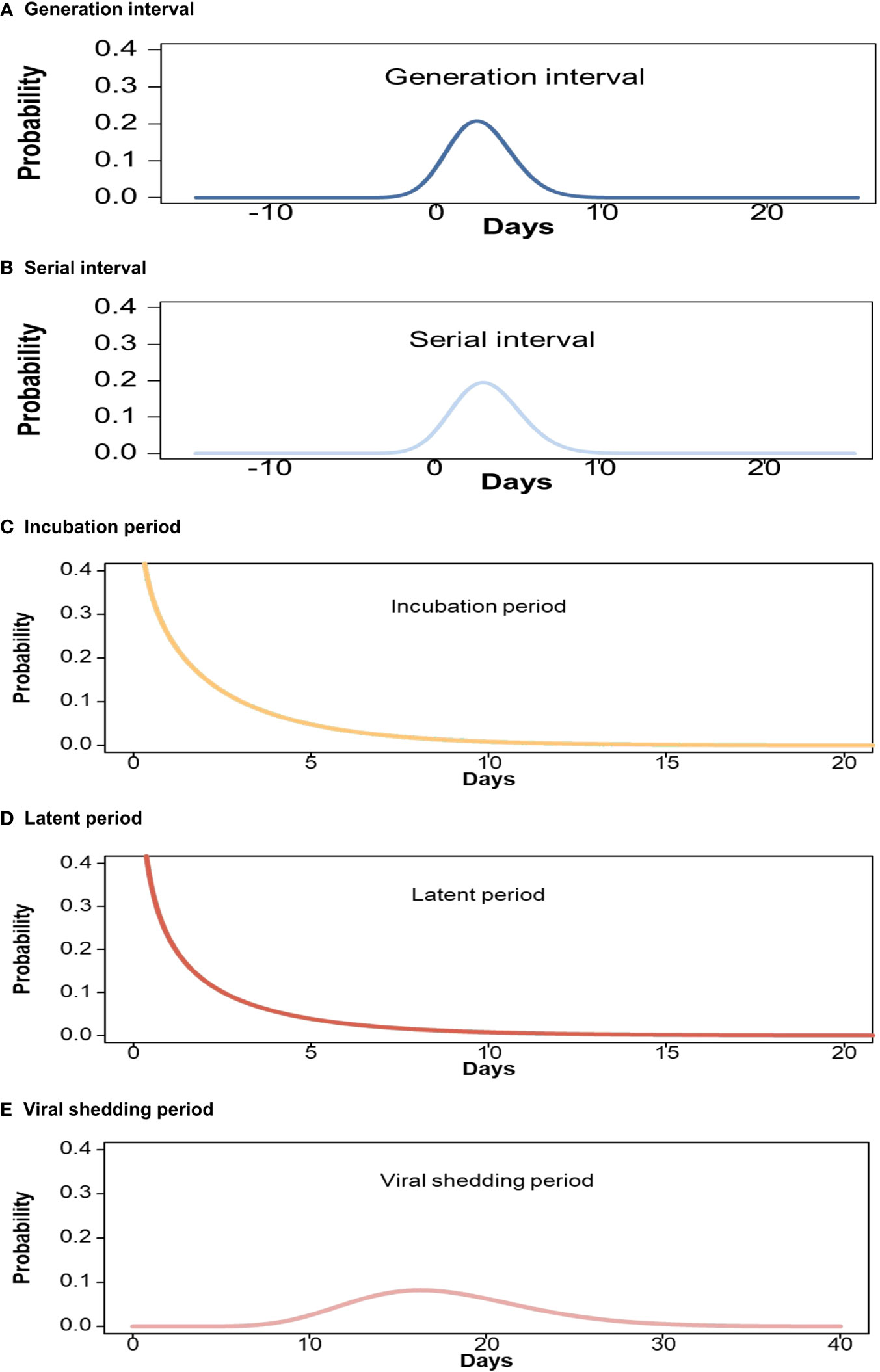
Figure 2 Estimated cumulative γ distributions of key time intervals for Omicron BA.2. (A) Generation interval; (B) Serial interval; (C) Incubation period; (D) Latent period; (E) Viral shedding period.
Discussion
In this study, we conducted a comprehensive analysis to evaluate the characteristics, dynamics, and risk of transmission of BA.2 infection. Moreover, we estimated the effectiveness of inactivated COVID-19 vaccine boosters against transmission in Shenzhen, China.
The secondary attack rate was 7.2% among close contacts with BA.2 infection. No significant difference was found for the transmission risk related to the type of index case or gender of index case; however, female close contacts showed a higher SAI risk. Moreover, individuals aged 0–17 years old and ≥60 years old had a higher SAI and transmission incidence compared with those aged 18–59 years old. Although this difference was not significant for the index cases aged 0–17 years old compared with those aged 18–59 years old, a higher SAI incidence was found for index cases aged 0–17 years. Furthermore, we observed that close contact exposure to an index case after onset was associated with a higher SAI risk than exposure to an index case before onset. This may be because post-onset index cases may carry a higher viral load. Moreover, household contacts had a higher SAI risk than non-household contacts. SAI incidence was 15.8% among household contacts, which was not consistent with the incidence of SAI in Denmark in a published study (21). This may be because the local government in Shenzhen immediately compulsorily isolated household contacts at designated facilities after the index cases were diagnosed, resulting in a relatively low secondary attack rate compared with published study in Denmark (21). In addition, we observed that individuals who received booster vaccination had a lower risk of SAI compared with unvaccinated or only partial vaccinated subjects although the effectiveness was not very high. Several studies have reported a lower VE against Omicron BA.2 infection than other variants of SARS-CoV-2 (26–31).
The VE against transmission is rarely reported (7, 16, 32). We found that a lower risk of SAI for close contacts was associated with index cases who received full or booster vaccinations compared with index cases in the unvaccinated and partially vaccinated groups. The results further showed the importance of increasing vaccine coverage to mitigate the spread of COVID-19 (33). We then excluded individuals who were unvaccinated or partially vaccinated because most index cases [549 of 644 individuals (85.2%)] and their close contacts [7,446 of 8,466 individuals (88.0%)] in this study had received at least two doses of COVID-19 vaccines. Moreover, individuals aged 0–17 years old were not included in the analysis of VE against transmission because they were not covered by the booster immunization campaigns in China. Furthermore, we excluded those who received non-inactivated COVID-19 vaccines because most individuals in this study had received the inactivated COVID-19 vaccines (>90%). Therefore, we focused on evaluating the inactivated VE against transmission for the individuals with booster vaccination versus full vaccination.
The overall aVE was 24.0% against BA.2 transmission for booster vaccination compared with full vaccination indicating no effective protection. Our estimated overall VE against BA.2 transmission was consistent with the previous estimates of 28.9% for Omicron BA.5.2 variant [18]. However, an aVE of 93.7% against transmission was found among those aged ≥60 years older, but not in those aged 18–59 years older when comparing the booster versus full vaccination groups. As we know, the current COVID-19 vaccines are less effective in preventing SARS-CoV-2 Omicron infection but are useful in mitigating the severity of COVID-19 especially in old adults (26–31). Because of the high frequency of complications, such as hypertension and diabetes mellitus, senior adults are more easily to suffer from severe COVID-19. Moreover, our study indicated that family members and household contacts were prone to being infected with SARS-CoV-2. Old adults are more often to stay in home and became susceptible to SARS-CoV-2 infection. We indeed observed two secondary infection peaks in children and old adults. These factors might contribute to the observed high effectiveness in the age group of ≥60 years. A moderate level of protection was observed against the transmission in non-household contact setting, whereas no significantly protective effect was found in household contact setting. Moreover, a moderate level of protection was observed against the transmission at 14 to 179 days after booster vaccination, whereas no any effectiveness was found against the transmission at ≥180 days after booster vaccination. The results indicate the protective effect was waning over time against BA.2 transmission. Wang et al. [18] observed inactivated vaccines booster immunization provided ineffective protection against BA.5.2 transmission at over 90 days after booster vaccination. As we know, SARS-CoV-2 has been evolving and even the Omicron sub-lineages are constantly emerging, such as XXB, EG.5, and HV.1 (1). The emerging variants and sub-lineages showed new features including different virulence, transmission capability and resistance to the existing neutralizing immunity, which, in turn, require the continuous assessment of VE against emerging SARS-VoV-2 variants. Furthermore, new COVID-19 vaccines including different SARS-CoV-2 variants, i.e., bivalent or trivalent COVID-19 vaccines, have been developing and implemented in response to emerging Omicron sub-lineage according to WHO recommendation. However, the VE remains to be evaluated by using real world data. Therefore, we emphasize the importance of continuously evaluating VE for emerging Omicron subvariants. Overall, these results indicated that inactivated vaccine boosters can effectively prevent the transmission of index cases, especially in the population aged ≥60 years.
The data on exposure history were collected on the basis of in-depth epidemiological investigations, allowing us to provide a fitting estimation of the distribution of the time intervals of key transmission events. We noted that there was considerable uncertainty in the previous estimates generated prior to the emergence of SARS-CoV-2 variants of concern, and previous studies reported ranges from 3.0 to 7.8 days for the SI (33–39) and from 4.8 to 8.0 days for the incubation period (33, 40–45). In this study, we observed that the mean SI was 3.2 days for the BA.2 variant. Moreover, the mean incubation period estimates were shorter (2.4 days) than previous estimates for BA.1 (3.2–4.6 days) (9), BA.2 (4.4 days) (10), and BA.5 (5.7 days) (16). Only three studies (10, 16, 33) provided GI estimates because it was difficult to obtain the required information on the infection dates of both the index cases and their close contacts. We found a mean GI of 2.7 days, which was shorter than those of the previous Alpha (4.7 days) and Delta (5.5 days) variants (33), but longer than that of the Omicron BA.1 variant (2.4 days) (10). Moreover, it was slightly shorter than that of the Omicron BA.5 variant (2.8 days) (16). Finally, we found that the mean latent and viral shedding periods were approximately 2.1 days and 17.9 days, respectively. Previously uncertain estimated time intervals for key events may have resulted from small sample sizes and possible sampling biases (46, 47). Our data were based on an entire epidemic wave and had a larger sample size. These factors may mean that this study does not have the same bias and may provide stable results (47).
In this study, we evaluated the characteristics, dynamics, and risk of Omicron BA.2 transmission based on a major outbreak of the epidemic. It was the first study to comprehensively evaluate Omicron BA.2 sub-lineage SAI and transmission risk, and the first study to assess the effectiveness of inactivated COVID-19 vaccine boosters against the transmission of SARS-CoV-2 Omicron variant in comparison with a full schedule of vaccination without boosters. However, there were also several limitations. First, we used the positive PCR test (Ct value < 40) to estimate the time intervals of key effects. The use of PCR test positivity may impact the accuracy of transmission dynamics assessments due to its imperfect sensitivity caused by the potential delays in viral load reaching detectable levels. However, at present, PCR test is the gold standard for diagnosing SARS-CoV-2 infection. In addition, in our study, all the close contacts received multiple tests during medical observation period, which increased the possibility of early diagnosis of SARS-CoV-2 infection and made up for the low sensitivity of PCR. Second, different countries implemented different measures to control the COVID-19 outbreak. Those different measures may influence the incidence of SAI among close contacts and limit the generalization of our results. Third, our estimates of the high VE for adults of over 60 years old may be associated with a relatively small sample size. Further studies are needed to identify our findings. Fourth, because our data relied on the epidemiological contact-tracing data, we could not always accurately reconstruct the entire transmission chain and fully avoid recall bias.
In summary, the main Omicron BA.2 subvariant transmission setting was in households, and the effectiveness of inactivated vaccine boosters against BA.2 subvariant transmission was relatively high in older people. These findings indicate the importance of continuously assessing VE against different Omicron variant sub-lineages as they quickly evolve and mutate.
Data availability statement
The original contributions presented in the study are included in the article/Supplementary Material. Further inquiries can be directed to the corresponding authors.
Ethics statement
The study involving human participants were reviewed and approved by the China CDC Ethical Review Committee (approval number 202210). Written informed consent was waived because the data in the study were collected from administrative requirements of disease control and surveillance by Shenzhen Center for Disease Control and Prevention as required by the National Health Commission. Analytical data sets were de-linked and anonymized in this study.
Author contributions
XH: Conceptualization, Data curation, Formal analysis, Methodology, Project administration, Validation, Writing – original draft, Writing – review & editing. YXL: Conceptualization, Data curation, Investigation, Writing – original draft, Writing – review & editing. YHL: Formal analysis, Writing – review & editing. JY: Writing – review & editing. WG: Data curation, Investigation, Writing – review & editing. JW: Data curation, Investigation, Writing – review & editing. YL: Investigation, Writing – review & editing. JS: Writing – review & editing. XZ: Conceptualization, Investigation, Project administration, Supervision, Writing – original draft, Writing – review & editing. ST: Conceptualization, Project administration, Supervision, Writing – original draft, Writing – review & editing.
Funding
The author(s) declare financial support was received for the research, authorship, and/or publication of this article. This study was supported by Special Project for Research and Development in Key areas of Guangdong Province (2022B1111020006) and Emergency Key Program of Guangzhou Laboratory, Project Evaluation of Effectiveness of COVID-19 Vaccine against SARS-CoV-2 Outbreak in China (SRPG22–009).
Acknowledgments
We thank Amanda Holland, PhD, from Liwen Bianji (Edanz) (www.liwenbianji.cn) for editing the English text of a draft of this manuscript. We thank all staffs involved in the prevention and control of COVID-19 at the Shenzhen Center for Disease Control and Prevention.
Conflict of interest
The authors declare that the research was conducted in the absence of any commercial or financial relationships that could be construed as a potential conflict of interest.
Publisher’s note
All claims expressed in this article are solely those of the authors and do not necessarily represent those of their affiliated organizations, or those of the publisher, the editors and the reviewers. Any product that may be evaluated in this article, or claim that may be made by its manufacturer, is not guaranteed or endorsed by the publisher.
Supplementary material
The Supplementary Material for this article can be found online at: https://www.frontiersin.org/articles/10.3389/fimmu.2023.1290279/full#supplementary-material
References
1. WHO. Currently circulating variants of concern (VOCs). World Health Organization (2023). Available at: https://www.who.int/activities/tracking-SARS-CoV-2-variants.
2. WHO. Weekly epidemiological update on COVID-19-21 September 2022. World Health Organization. Available at: https://www.who.int/publications/m/item/weekly-epidemiological-update-on-covid-19---21-september-2022.
3. Ren SY, Wang WB, Gao RD, Zhou AM. Omicron variant (B.1.1.529) of SARS-CoV-2: Mutation, infectivity, transmission, and vaccine resistance. World J Clin cases (2022) 10:1–11. doi: 10.12998/wjcc.v10.i1.1
4. Tian D, Sun Y, Xu H, Ye Q. The emergence and epidemic characteristics of the highly mutated SARS-CoV-2 Omicron variant. J Med Virol (2022) 94:2376–83. doi: 10.1002/jmv.27643
5. Kraemer MUG, Pybus OG, Fraser C, Cauchemez S, Rambaut A, Cowling BJ. Monitoring key epidemiological parameters of SARS-CoV-2 transmission. Nat Med (2021) 27:1854–5. doi: 10.1038/s41591-021-01545-w
6. Farahat RA, Abdelaal A, Umar TP, El-Sakka AA, Benmelouka AY, Albakri K, et al. The emergence of SARS-CoV-2 Omicron subvariants: current situation and future trends. Infez Med (2022) b30:480–94. doi: 10.53854/liim-3004-2
7. Kang M, Xin H, Yuan J, Ali ST, Liang Z, Zhang J, et al. Transmission dynamics and epidemiological characteristics of SARS-CoV-2 Delta variant infections in Guangdong, China, May to June 2021. Euro Surveill (2022) 27:2100815. doi: 10.2807/1560-7917.ES.2022.27.10.2100815
8. Ganyani T, Kremer C, Chen D, Torneri A, Faes C, Wallinga J, et al. Estimating the generation interval for coronavirus disease (COVID-19) based on symptom onset data, March 2020. Euro Surveill (2020) 25:12–9. doi: 10.2807/1560-7917.ES.2020.25.17.2000257
9. Backer JA, Eggink D, Andeweg SP, Veldhuijzen IK, van Maarseveen N, Vermaas K, et al. Shorter serial intervals in SARS-CoV-2 cases with Omicron BA.1 variant compared with Delta variant, the Netherlands, 13 to 26 December 2021. Euro Surveill (2022) 27:2200042. doi: 10.2807/1560-7917.ES.2022.27.6.2200042
10. Mefsin YM, Chen D, Bond HS, Lin Y, Cheung JK, Wong JY, et al. Epidemiology of infections with SARS-coV-2 omicron BA.2 variant, hong kong, january-march 2022. Emerg Infect Dis (2022) 28:1856–8. doi: 10.3201/eid2809.220613
11. McMenamin ME, Nealon J, Lin Y, Wong JY, Cheung JK, Lau EHY, et al. Vaccine effectiveness of one, two, and three doses of BNT162b2 and CoronaVac against COVID-19 in Hong Kong: a population-based observational study. Lancet Infect Dis (2022) 22:1435–43. doi: 10.1016/S1473-3099(22)00345-0
12. Huang Z, Xu S, Liu J, Wu L, Qiu J, Wang N, et al. Effectiveness of inactivated and Ad5-nCoV COVID-19 vaccines against SARS-CoV-2 Omicron BA. 2 variant infection, severe illness, and death. BMC Med (2022) 20:400. doi: 10.1186/s12916-022-02606-8
13. Wan EYF, Mok AHY, Yan VKC, Wang B, Zhang R, Hong SN, et al. Vaccine effectiveness of BNT162b2 and CoronaVac against SARS-CoV-2 Omicron BA.2 infection, hospitalisation, severe complications, cardiovascular disease and mortality in patients with diabetes mellitus: a case control study. J Infect (2022) 85:e140–4. doi: 10.1016/j.jinf.2022.08.008
14. Cheng FWT, Fan M, Wong CKH, Chui CSL, Lai FTT, Li X, et al. The effectiveness and safety of mRNA (BNT162b2) and inactivated (CoronaVac) COVID-19 vaccines among individuals with chronic kidney diseases. Kidney Int (2022) 102:922–5. doi: 10.1016/j.kint.2022.07.018
15. Tang L, Wang FZ, Rodewald LE, Wang XY, Liu SY, Liu QQ, et al. Real-world effectiveness of primary series and booster doses of inactivated COVID-19 vaccine against Omicron BA.2 variant infection in China: a retrospective cohort study. J Infect Dis (2023) 228:261–9. doi: 10.1093/infdis/jiad090
16. Wang K, Guo Z, Zeng T, Sun S, Lu Y, Wang J, et al. Transmission characteristics and inactivated vaccine effectiveness against transmission of SARS-coV-2 omicron BA.5 variants in urumqi, China. JAMA Netw Open (2023) 6:e235755. doi: 10.1001/jamanetworkopen.2023.5755
17. de Gier B, Andeweg S, Joosten R, Ter Schegget R, Smorenburg N, van de Kassteele J, et al. Vaccine effectiveness against SARS-CoV-2 transmission and infections among household and other close contacts of confirmed cases, the Netherlands, February to May 2021. Euro Surveill (2021) 26:2100640. doi: 10.2807/1560-7917.ES.2021.26.31.2100640
18. National Health Commission of the People’s Republic of China. The prevention and Control Scheme of COVID-19. Available at: https://www.nhc.gov.cn/jkj/s3577/202105/6f1e8ec6c4a540d99fafef52fc86d0f8/files/4a860a7e85d14d55a22fbab0bbe77cd9.pdf.
19. Hu S, Wang W, Wang Y, Litvinova M, Luo K, Ren L, et al. Infectivity, susceptibility, and risk factors associated with SARS-CoV-2 transmission under intensive contact tracing in Hunan, China. Nat Commun (2021) 12:1533. doi: 10.1038/s41467-021-21710-6
20. Madewell ZJ, Yang Y, Longini IM Jr, Halloran ME, Dean NE. Household secondary attack rates of SARS-CoV-2 by variant and vaccination status: an updated systematic review and meta-analysis. JAMA Netw Open (2022) 5:e229317. doi: 10.1001/jamanetworkopen.2022.9317
21. Zhao S, Chong MKC, Ryu S, Guo Z, He M, Chen B, et al. Characterizing superspreading potential of infectious disease: decomposition of individual transmissibility. PloS Comput Biol (2022) 18:e1010281. doi: 10.1371/journal.pcbi.1010281
22. Nishiura H, Yan P, Sleeman CK, Mode CJ. Estimating the transmission potential of supercritical processes based on the final size distribution of minor outbreaks. J Theor Biol (2012) 294:48–55. doi: 10.1016/j.jtbi.2011.10.039
23. Jackson ML, Nelson JC. The test-negative design for estimating influenza vaccine effectiveness. Vaccine (2013) 31:2165–8. doi: 10.1016/j.vaccine.2013.02.053
24. Bond HS, Sullivan SG, Cowling BJ. Regression approaches in the test-negative study design for assessment of influenza vaccine effectiveness. Epidemiol Infect (2016) 144:1601–11. doi: 10.1017/S095026881500309X
25. Cowling BJ, Perera RA, Fang VJ, Chan KH, Wai W, So HC, et al. Incidence of influenza virus infections in children in Hong Kong in a 3-year randomized placebo-controlled vaccine study, 2009-2012. Clin Infect Dis (2014) 59:517–24. doi: 10.1093/cid/ciu356
26. Lopez Bernal J, Andrews N, Gower C, Robertson C, Stowe J, Tessier E, et al. Effectiveness of the Pfizer-BioNTech and Oxford-AstraZeneca vaccines on covid-19 related symptoms, hospital admissions, and mortality in older adults in England: test negative case-control study. BMJ (2021) 373:n1088. doi: 10.1136/bmj.n1088
27. Andrews N, Stowe J, Kirsebom F, Toffa S, Rickeard T, Gallagher E, et al. Covid-19 vaccine effectiveness against the omicron (B.1.1.529) variant. N Engl J Med (2022) 386:1532–46. doi: 10.1056/NEJMoa2119451
28. Zeng B, Gao L, Zhou Q, Yu K, Sun F. Effectiveness of COVID-19 vaccines against SARS-CoV-2 variants of concern: a systematic review and meta-analysis. BMC Med (2022) 20:200. doi: 10.1186/s12916-022-02397-y
29. Sacco C, Del Manso M, Mateo-Urdiales A, Rota MC, Petrone D, Riccardo F, et al. Effectiveness of BNT162b2 vaccine against SARS-CoV-2 infection and severe COVID-19 in children aged 5-11 years in Italy: a retrospective analysis of January-April, 2022. Lancet (2022) 400:97–103. doi: 10.1016/S0140-6736(22)01185-0
30. Tseng HF, Ackerson BK, Luo Y, Sy LS, Talarico CA, Tian Y, et al. Effectiveness of mRNA-1273 against SARS-CoV-2 Omicron and Delta variants. Nat Med (2022) 28(5):1063–71. doi: 10.1038/s41591-022-01753-y
31. McMenamin ME, Nealon J, Lin Y, Wong JY, Cheung JK, Lau EHY, et al. Vaccine effectiveness of one, two, and three doses of BNT162b2 and CoronaVac against COVID-19 in Hong Kong: a population-based observational study. Lancet Infect Dis (2022) 22:1435–43. doi: 10.1016/S1473-3099(22)00345-0
32. de Gier B, Andeweg S, Backer JA. RIVM COVID-19 surveillance and epidemiology team; Hahné SJ, van den Hof S, et al. Vaccine effectiveness against SARS-CoV-2 transmission to household contacts during dominance of Delta variant (B.1.617.2), the Netherlands, August to September 2021. Euro Surveill (2021). 26:2100977. doi: 10.2807/1560-7917.ES.2021.26.44.2100977
33. Li Q, Guan X, Wu P, Wang X, Zhou L, Tong Y, et al. Early transmission dynamics in Wuhan, China, of novel coronavirus–infected pneumonia. N Engl J Med (2020) 382:1199–207. doi: 10.1056/NEJMoa2001316
34. Du Z, Xu X, Wu Y, Wang L, Cowling BJ, Meyers LA. Serial interval of COVID-19 among publicly reported confirmed cases. Emerg Infect Dis (2020) 26:1341–3. doi: 10.3201/eid2606.200357
35. Nishiura H, Linton NM, Akhmetzhanov AR. Serial interval of novel coronavirus (COVID-19) infections. Int J Infect Dis (2020) 93:284–6. doi: 10.1016/j.ijid.2020.02.060
36. Danis K, Epaulard O, Bénet T, Gaymard A, Campoy S, Botelho-Nevers E, et al. Cluster of coronavirus disease 2019 (COVID-19) in the French Alps, February 2020. Clin Infect Dis (2020) 71:825–32. doi: 10.1093/cid/ciaa424
37. He X, Lau EHY, Wu P, Deng X, Wang J, Hao X, et al. Temporal dynamics in viral shedding and transmissibility of COVID-19. Nat Med (2020) 26:672–5. doi: 10.1038/s41591-020-0869-5
38. Prete CA, Buss L, Dighe A, Porto VB, da Silva Candido D, Ghilardi F, et al. Serial interval distribution of SARS-CoV-2 infection in Brazil. J Travel Med (2021) 28:taaa115. doi: 10.1093/jtm/taaa115
39. You C, Deng Y, Hu W, Sun J, Lin Q, Zhou F, et al. Estimation of the time-varying reproduction number of COVID19 outbreak in China. Int J Hyg Environ Health (2020) 228:113555. doi: 10.1016/j.ijheh.2020.113555
40. Zhang J, Litvinova M, Wang W, Wang Y, Deng X, Chen X, et al. Evolving epidemiology and transmission dynamics of coronavirus disease 2019 outside Hubei province, China: a descriptive and modelling study. Lancet Infect Dis (2020) 20:793–802. doi: 10.1016/S14733099(20)30230-9
41. Linton NM, Kobayashi T, Yang Y, Hayashi K, Akhmetzhanov AR, Jung SM, et al. Incubation period and other epidemiological characteristics of 2019 novel coronavirus infections with right truncation: a statistical analysis of publicly available case data. J Clin Med (2020) 9:538. doi: 10.3390/jcm9020538
42. Backer JA, Klinkenberg D, Wallinga J. Incubation period of 2019 novel coronavirus (2019-nCoV) infections among travellers from Wuhan, China, 20–28 January 2020. Euro Surveill (2020) 25:2000062. doi: 10.2807/1560-7917.ES.2020.25.5.2000062
43. Bi Q, Wu Y, Mei S, Ye C, Zou X, Zhang Z, et al. Epidemiology and transmission of COVID-19 in 391 cases and 1286 of their close contacts in Shenzhen, China: a retrospective cohort study. Lancet Infect Dis (2020) 20:911–9. doi: 10.1016/S1473-3099(20)30287-5
44. Tian S, Hu N, Lou J, Chen K, Kang X, Xiang Z, et al. Characteristics of COVID-19 infection in beijing. J Infect (2020) 80:401–6. doi: 10.1016/j.jinf.2020.02.018
45. Xu T, Chen C, Zhu Z, Cui M, Chen C, Dai H, et al. Clinical features and dynamics of viral load in imported and non-imported patients with COVID-19. Int J Infect Dis (2020) 94:68–71. doi: 10.1016/j.ijid.2020.03.022
46. Nishiura H, Linton NM, Akhmetzhanov AR. Serial interval of novel coronavirus (COVID-19) infections. Int J Infect Dis (2020) 93:284–6. doi: 10.1016/j.ijid.2020.02.060
Keywords: COVID-19, vaccines, effectiveness, Omicron (B.1.1.529), BA.2 subvariant, transmission
Citation: He X, Liao Y, Liang Y, Yu J, Gao W, Wan J, Liao Y, Su J, Zou X and Tang S (2024) Transmission characteristics and inactivated vaccine effectiveness against transmission of the SARS-CoV-2 Omicron BA.2 variant in Shenzhen, China. Front. Immunol. 14:1290279. doi: 10.3389/fimmu.2023.1290279
Received: 07 September 2023; Accepted: 13 December 2023;
Published: 08 January 2024.
Edited by:
Chuanxi Fu, Zhejiang Chinese Medical University, ChinaReviewed by:
Roberto Dias de Oliveira, State University of Mato Grosso do Sul, BrazilSivaram Gunisetty, Emory University, United States
Copyright © 2024 He, Liao, Liang, Yu, Gao, Wan, Liao, Su, Zou and Tang. This is an open-access article distributed under the terms of the Creative Commons Attribution License (CC BY). The use, distribution or reproduction in other forums is permitted, provided the original author(s) and the copyright owner(s) are credited and that the original publication in this journal is cited, in accordance with accepted academic practice. No use, distribution or reproduction is permitted which does not comply with these terms.
*Correspondence: Xuan Zou, OTE0NDk0NTU3QHFxLmNvbQ==; Shixing Tang, dGFtZ3NoaXhpbmdAc211LmVkdS5jbg==
†These authors have contributed equally to this work and share first authorship