- 1Department of Laboratory Medicine, Hallym University College of Medicine and Hallym University Sacred Heart Hospital, Anyang, Republic of Korea
- 2Animal Model Research Group, Jeonbuk Branch Institute, Korea Institute of Toxicology, Jeongeup, Republic of Korea
- 3Department of Psychology and Neuroscience, Duke University, Durham, NC, United States
Introduction: The global shortage of human blood for medical use has prompted the development of alternative blood sources. Nonhuman primates (NHPs) are commonly used owing to their physiological similarities to humans. The objective of the current study was to establish a controlled-blood-loss model in NHPs to explore their clinical and biological responses.
Methods: Blood was sequentially withdrawn from 10 cynomolgus monkeys (10, 14, 18, 22, and 25% of the total blood volume); their vital signs were monitored, and blood parameters were serially analyzed. Humoral mediators in the blood were measured using flow cytometry and enzyme-linked immunosorbent assays.
Results: In NHPs subjects to 25% blood loss and presenting with related clinical symptoms, the systolic blood pressure ratio on day 0 after bleeding was significantly lower than that of the animals from the other groups (median: 0.65 vs. 0.88, P = 0.0444). Red blood cell counts from day 0–14 and hematocrit levels from day 0–7 were markedly decreased relative to the baseline (P < 0.01). These parameters showed a direct correlation with the extent of blood loss. The levels of creatine phosphokinase, aspartate aminotransferase, and alanine aminotransferase exhibited increases in response to blood loss and had a stronger correlation with the hemoglobin ratio than the volume of blood loss. The levels of C3a and C4a, as well as interleukin (IL)-1α and IL-15, displayed a strong correlation, with no apparent association with blood loss.
Conclusion: The findings of the present study showed that only NHPs with 25% blood loss exhibited clinical decompensation and significant systolic blood pressure reduction without fatalities, suggesting that this level of blood loss is suitable for evaluating blood transfusion efficacy or other treatments in NHP models. In addition, the ratio of hemoglobin may serve as a more dependable marker for predicting clinical status than the actual volume of blood loss. Thus, our study could serve as a basis for future xenotransfusion research and to predict biological responses to massive blood loss in humans where controlled experiments cannot be ethically performed.
1 Introduction
Blood transfusions are an important aspect of substitution therapy, although the availability of blood products is often limited in low- and middle-income countries (1). According to data from 2017, 119 of the 195 analyzed countries (61%) had an insufficient blood supply. Lack of adequate blood supply has been shown to contribute to preventable mortality and morbidity in several low- and middle-income countries (2). In developed countries, the low birth rate and aging of the baby-boom generation (3) could result in a decrease in the blood donor population and eventually a shortage of blood supply.
Research on blood substitutes is actively pursued to overcome blood shortages. The objective of this field of research is to develop alternative products or technologies capable of replacing human blood in blood transfusions and an alternative means of resuscitation and oxygen delivery. Hemoglobin-based oxygen carriers, perfluorocarbon-based oxygen carriers, and synthetic blood components have been developed, and the application of stem cell-derived red blood cells (RBCs) has been explored (4, 5). Alternatively, the use of animal-derived RBCs could expand the available blood supply. Pigs have been identified as potential organ and blood-cell donors for human transplantation (xenotransplantation) and transfusion (xenotransfusion) (6). Although the lifespan of pig RBCs is lower than that of human RBCs, the size and number of pig and human RBCs are comparable (7).
Nonhuman primates (NHPs), such as monkeys, have been primarily used before human clinical trials owing to similarity in their anatomical structure and physiological characteristics to those of humans (8). Previous hemorrhage animal models using pigs or dogs include the chronic anemia and acute blood loss models (9, 10). Xenogeneic blood products would primarily be needed in emergency situations for short-term resuscitation in clinical practice. Therefore, we developed an acute blood-loss model in NHPs. We attempted to determine the point of blood loss that is clinically meaningful but not detrimental to NHPs. Hence, dose escalation was performed in a stepwise manner, beginning with a bleeding condition of 10%. To distinguish between transfusion and hemorrhage-related biological responses, we investigated hemorrhage-induced biological responses.
The main objective of the current study was to explore clinical and biological responses after bleeding in an NHP model. To understand the sequences of various biological responses in NHPs after blood loss, we analyzed the expression of hematological markers, biochemical markers, inflammatory cytokines, and complement activation products in serially drawn blood samples from NHPs. Additionally, vital signs were monitored to determine the clinical status of the NHPs.
2 Materials and methods
2.1 Study design
We used 10 cynomolgus monkeys (Macaca fascicularis; Nafovanny, Dong Nai, Vietnam) with the authorization of the Korea Institute of Toxicology Institutional Animal Care and Use Committee (IAC-22-01-0342-0037).
A schematic illustration of the study is shown in Figure 1. To develop the NHP bleeding model without lethality in experimental animals, a 10% blood loss was initially performed, which was gradually increased to 14, 18, 22, and 25% of the total blood volume, in two NHPs for each bleeding condition. The total blood volume of the NHP was calculated to be 65 mL per/kg body weight (11). The characteristics of each NHP by bleeding condition are described in Table 1. On the day of the acute blood loss intervention, NHPs were secured to a primate chair. The femoral vein was selected as the blood collection route, and blood collection was performed using the femoral triangle of the inguinal-proximal leg as an access route. Syringes were used to measure the target blood volume. The blood collection site was disinfected with 70% alcohol, and the planned volume of blood was drawn from the cephalic or femoral vein using a 21–23G needle while monitoring blood pressure. Vital signs and general symptoms, including mortality, morbidity, and changes in appearance and behavior, were observed daily for three weeks from the day of blood loss. The blood parameters of the NHPs were analyzed on days -14 (as baselines, at least 2 weeks before the intervention), 0 (before the bleeding intervention, D0BB), 0 after bleeding (immediately after the bleeding intervention, D0AB), 1, 3, 5, 7, 14, 21, and 28 (D1, D3, D5, D7, D14, D21, and D28, respectively).
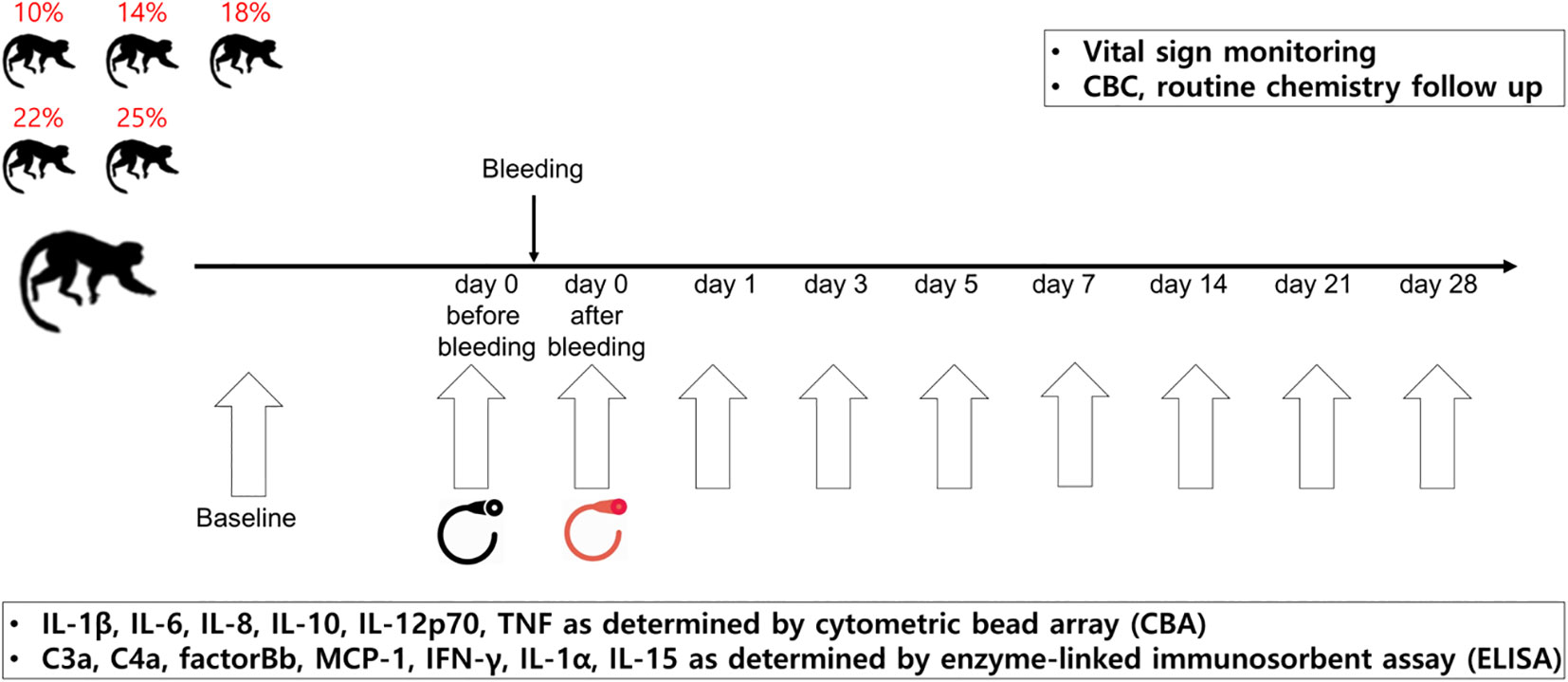
Figure 1 Study schema. The NHP bleeding model was developed using ten cynomolgus monkeys. The NHPs were bled by 10, 14, 18, 22, or 25% of their total blood volume. From the day of blood loss intervention, vital signs, general symptoms, and changes in appearance and behavior were monitored daily for three weeks. The blood parameters of the NHPs were analyzed on each day (day -14, 0 before bleeding, 0 after bleeding, 1, 3, 5, 7, 14, 21, and 28). Two NHPs were assigned per condition. NHP, nonhuman primate; CBC, complete blood count.
2.2 Measurement of the levels of biomarkers
Hematology tests were performed to determine the levels of total white blood cells (WBCs), total RBCs, hemoglobin, hematocrit, mean corpuscular volume (MCV), mean corpuscular hemoglobin concentration (MCHC), mean corpuscular hemoglobin (MCH), platelet, reticulocyte, and white blood cell differentials: neutrophils, lymphocytes, monocytes, eosinophils, and basophils. Biochemical tests were performed to estimate the levels of glucose, blood urea nitrogen (BUN), creatinine, total protein, albumin, total cholesterol, triglycerides, aspartate aminotransferase (AST), alanine aminotransferase (ALT), iron, total iron-binding capacity (TIBC), total bilirubin, alkaline phosphatase (ALP), gamma glutamyl transpeptidase (GGT), creatine phosphokinase (CK), calcium, potassium, and unsaturated iron-binding capacity (UIBC).
Using a cytometric bead array human inflammatory cytokine kit (BD Biosciences, San Diego, CA, USA), we measured plasma levels of interleukin (IL)-1β, IL-6, IL-8, IL-10, IL-12p70, and tumor necrosis factor with flow cytometry. Interferon (IFN)-γ, IL-1α, IL-15, and monocyte chemoattractant protein-1 (MCP-1) levels were measured via enzyme-linked immunosorbent assay (ELISA) using monkey IFN-γ ELISAPRO (MABTECH, Stockholm, Sweden), monkey IL-1α ELISA, monkey IL-15 ELISA (Cusabio, Wuhan, China), and monkey MCP1 ELISA kit (Abcam, Cambridge, UK), respectively. To assess the extent of complement activation, C3a,C4a and factor Bb were measured using ELISA with human C3a,C4a ELISA kit (BD Biosciences) and a Bb plus EIA kit (Quidel, San Diego, CA, USA). The detailed information is described in Table 2. Each measurement using ELISA or flow cytometry was performed in duplicate.
2.3 Statistical analysis
Statistical analyses were performed using MedCalc (version 22.0; Ostend, Belgium) and R (version 4.3.2; Vienna, Austria). The difference in the levels between baseline and a certain time point was analyzed using the Wilcoxon paired test and the difference between two groups using the Mann-Whitney test. Correlations between factors were analyzed using the Spearman’s correlation analysis. Statistical significance was set at P < 0.05 (*) and P < 0.01 (**).
3 Results
3.1 Biological characteristics of the NHPs at the baseline timepoint
The gender differences among the NHPs and the mean and standard deviation (SD) of baseline measurements segregated by gender, including the statistical significance (P value), are presented in Table 3. Notable gender-based variations were absent in most parameters, with the exception of RBC, hemoglobin, and hematocrit. However, the values of each measurement varied individually. At baseline, the levels of IL-1β, IL-6, IL-8, IL-10, IL-12p70, and tumor necrosis factor were below detectable thresholds.
3.2 Changes in measured biological parameters over time following bleeding
3.2.1 Changes in physiological parameters
To elucidate the temporal changes in each parameter while minimizing individual variability, the ratios of systolic and diastolic blood pressure (BP) values, as well as heart rate at each time point relative to the baseline, are graphically represented in Figure 2. It was observed that the systolic BP on D0AB significantly decreased when compared to the baseline (P < 0.01). NHPs in the 25%-blood-loss group exhibited various clinical symptoms, including pupillary dilation and, an inability to stand for ~1 h, accompanied by an immediate drop-in heart rate and blood pressure after blood loss. All NHPs were not administered crystalloid fluid regardless of symptom development, symptomatic NHPs were carefully monitored, and recovery was observed the following day.
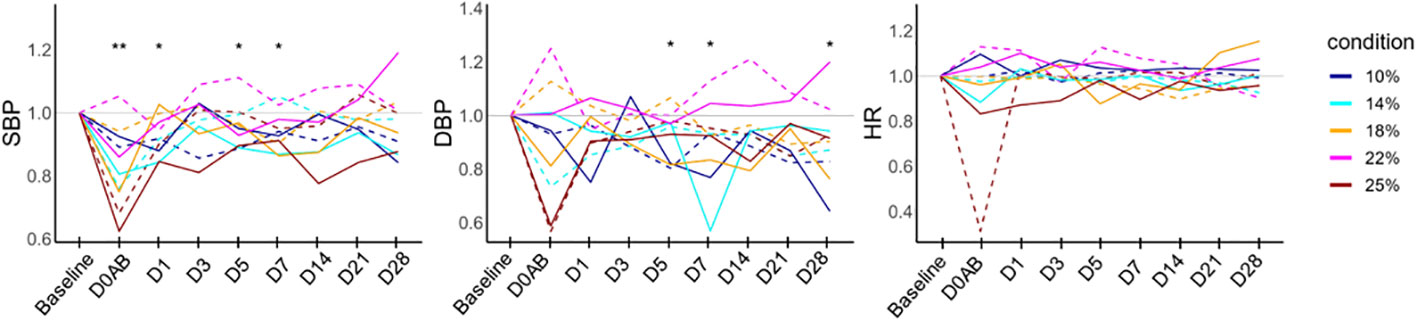
Figure 2 Vital sign monitoring in NHPs before and after controlled blood loss. Each group of NHPs (n=2) had 10%, 14%, 18%, 22%, or 25% of their total blood volume bled. The ratios to baseline levels were plotted: solid and dotted lines represent males and females, respectively. The levels at each time point were compared to the baseline levels, and the differences were analyzed using the Wilcoxon paired test. D0AB, day 0 after bleeding; NHP, nonhuman primates; SBP, systolic blood pressure; DBP, diastolic blood pressure; HR, heart rate; *P < 0.05; **P < 0.01.
3.2.2 Variations in the levels of hematological parameters over time
The ratios of hematological parameters at each time point compared to the baseline are depicted in Figure 3. On D0AB, RBC, hemoglobin, and hematocrit levels began declining, reaching their lowest on D3, followed by a gradual recovery. The levels of RBC and hemoglobin from D0AB to D14 and those of hematocrit from D0AB to D7 were significantly reduced compared to the baseline (P < 0.01). The MCV increased after bleeding, with values from D1 to D28 significantly exceeding the basal levels (P < 0.01). MCH levels on D1 and D14 were higher than baseline, whereas MCHC on D5 and D14 were lower (P < 0.01). The reticulocyte count began to rise on D3, peaked on D7 after blood loss, and then gradually approached the baseline values. However, even on D28, the reticulocyte count remained significantly higher than baseline (P < 0.05). The platelet count increased on D1, with values on D5, D7, and D14 significantly surpassing the baseline levels. The WBC, neutrophil, and monocyte counts significantly increased at D0AB but returned to the baseline levels on D1 or D3.
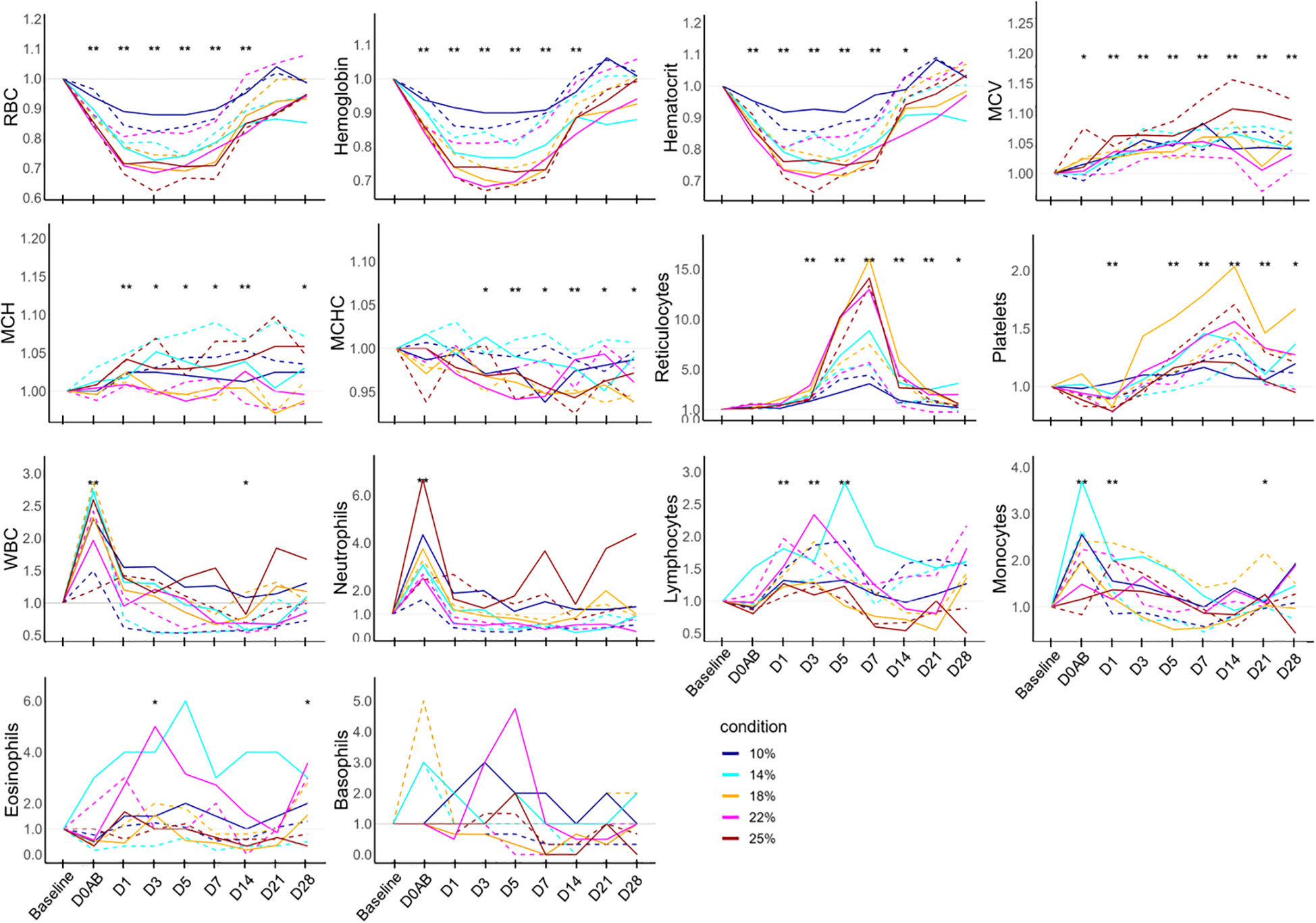
Figure 3 Hematology test results in NHPs before and after controlled blood loss. Each group of NHPs (n=2) had 10%, 14%, 18%, 22%, or 25% of their total blood volume bled. The ratios to baseline levels were plotted: solid and dotted lines represent males and females, respectively. The levels at each time point were compared to the baseline levels and the difference was analyzed using the Wilcoxon paired test. D0AB, day 0 after bleeding; RBC, red blood cell; MCV, mean corpuscular volume; MCH, mean corpuscular hemoglobin; MCHC, mean corpuscular hemoglobin concentration; WBC, white blood cell; *P < 0.05; **P < 0.01.
3.2.3 Temporal dynamics in the levels of biochemical parameters
The ratios of biological parameters at each time point compared to the baseline level are depicted in Figure 4. Glucose levels on D0AB and D1 were significantly higher than the baseline and normalized by D3. Albumin and total protein showed similar patterns of change. Their levels decreased on D0AB, reached a nadir on D1 or D3, and then began to increase. The levels of albumin and total protein from D0AB to D3 were significantly lower (P < 0.01), but total protein levels on D7 and D21 were higher than the basal levels (P < 0.05). The CK and AST levels significantly increased on D0AB and D1, peaking on D1. ALT levels significantly rose on D1 and D3, though their peak times varied. Creatinine levels increased on D0AB, while the BUN levels were variable. The iron levels significantly decreased after bleeding, remaining lower than the baseline value until D28. Accordingly, the TIBC and UIBC initially decreased but began to rise on D1 or D3, with their values on D7, D14, D21, and D28 being significantly higher than those at the baseline (P < 0.01).
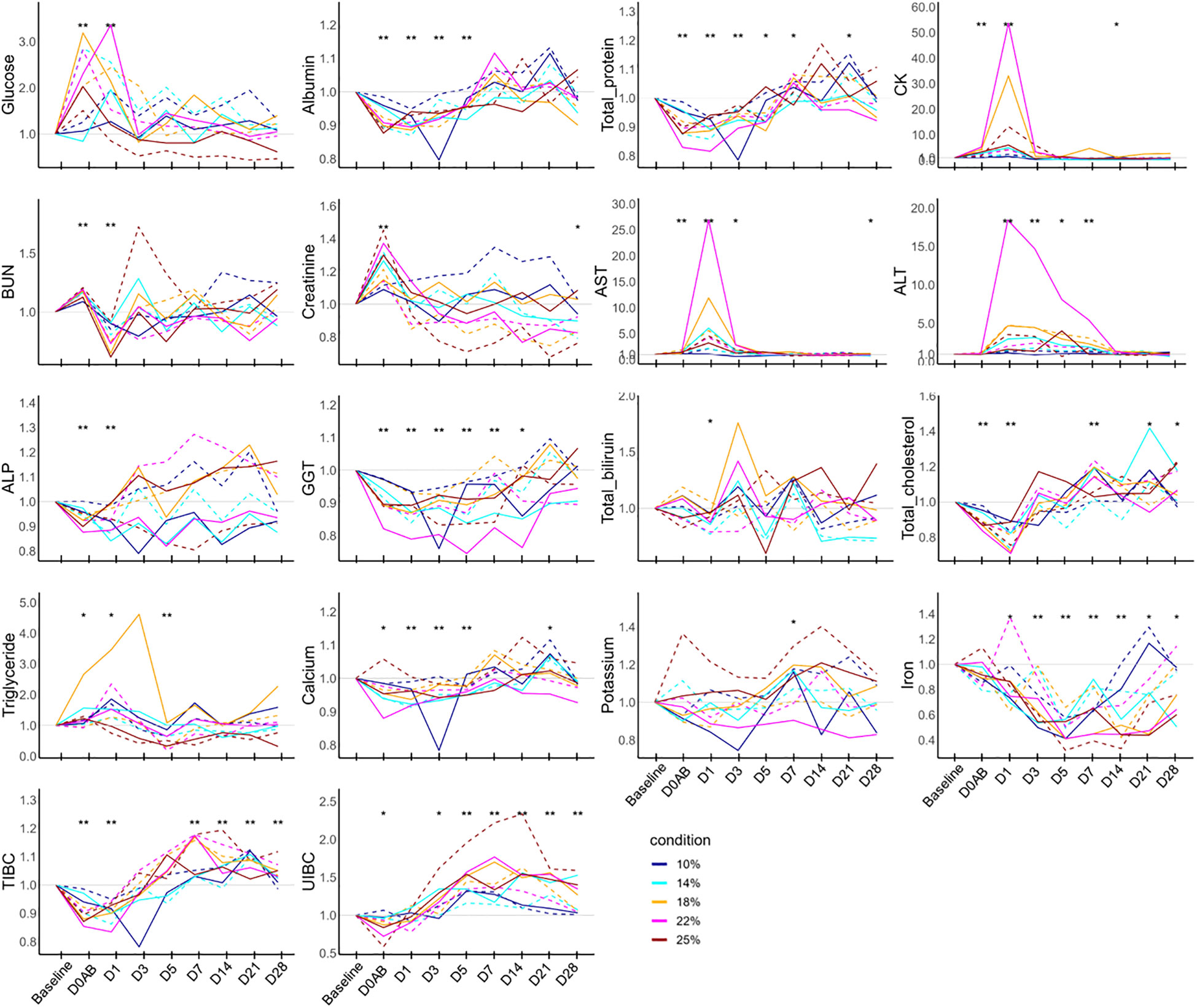
Figure 4 Biochemistry test results in NHPs before and after controlled blood loss. Each group of NHPs (n=2) had 10%, 14%, 18%, 22%, or 25% of their total blood volume bled. The ratios to baseline levels were plotted: solid and dotted lines represent males and females, respectively. The levels at each time point were compared to the baseline levels and the difference was analyzed using the Wilcoxon paired test. D0AB, day 0 after bleeding; CK, creatine phosphokinase; AST, aspartate aminotransferase; ALT, alanine aminotransferase; TIBC, total iron binding capacity; UIBC, unsaturated iron binding capacity; GGT, gamma glutamyl transpeptidase; *P < 0.05; **P < 0.01.
3.2.4 Changes in levels of immunological mediator
Because the baseline levels of immunological parameters were low, individual changes in the immunological parameters were analyzed using the net values of each parameter by subtracting the baseline values to avoid analysis distortion (Figure 5A). The levels of IL-1β, IL-6, IL-8, IL-10, IL-12p70, and tumor necrosis factor were anecdotally detected but were mostly under the detection limits. Thus, these parameters were excluded from the analysis. The levels of immunological mediators demonstrated significant increases frequently during the experiment; however, pinpointing the exact timing of these increases proved challenging due to their variable nature. The data revealed that on D0BB, the levels of IL-1α, IL-15, C3a, C4a, and MCP1 were significantly elevated compared to the baseline, independent of blood loss effects. On D1, IL-15, C3a, factor Bb, and MCP-1 also exhibited a significant increase from the baseline levels. Conversely, IFN-γ levels did not show significant variation over time. Further, a correlation analysis of these immunological parameters (as depicted in Figure 5B) indicated a strong interrelationship between IL-15, IL-1α, C3a, and C4a, while factor Bb demonstrated a moderate correlation with these parameters.
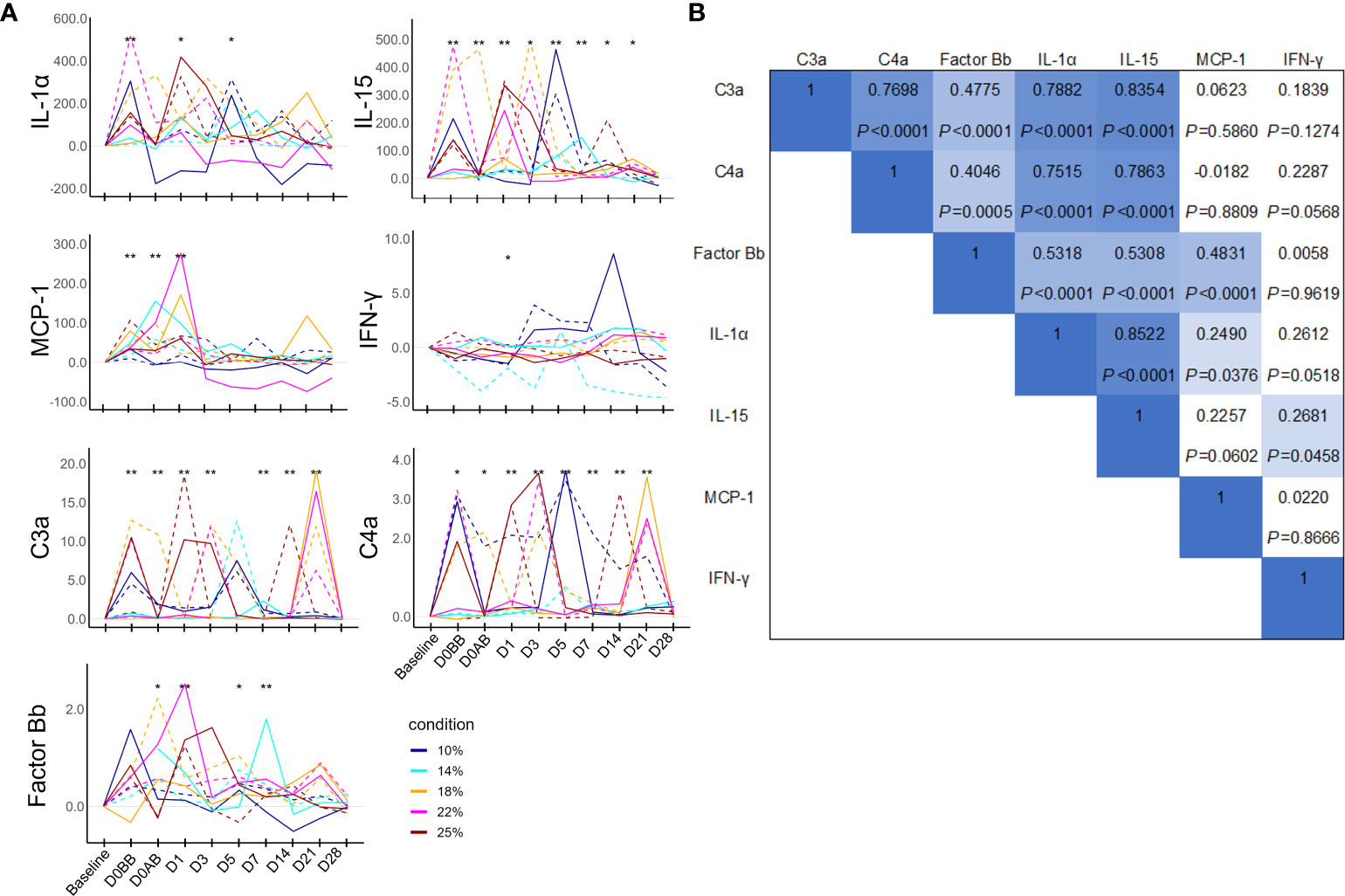
Figure 5 Humoral mediator responses in NHPs before and after controlled blood loss. Each group of NHPs (n=2) had 10%, 14%, 18%, 22%, or 25% of their total blood volume bled. (A) After subtracting baseline levels, the net values were plotted: solid and dotted lines represent males and females, respectively. The levels at each time point were compared to the baseline levels and the difference was analyzed using the Wilcoxon paired test. *P < 0.05; **P < 0.01. (B) The rho and P values of Spearman correlations between pairs of humoral mediators. IL, interleukin; MCP-1, monocyte chemoattractant protein-1; IFN, interferon.
3.3 Biological changes in response to blood loss
To investigate the influence of varying degrees of blood loss on biological responses in NHP subjects, we analyzed the relationship between the relative values (either ratio or difference) of parameters that differed significantly from baseline (P < 0.01) and the amount of blood loss. The findings are summarized in Table 4. The change in systolic BP level did not correlate with the extent of blood loss. In NHPs that experienced 25% blood loss and developed related symptoms, the systolic BP ratio on D0AB was significantly lower in this group compared to that in the others (median 0.65 vs 0.88, P = 0.0444).
Among hematological parameters, RBC-related measures including RBC, hemoglobin, hematocrit, MCHC, and reticulocyte counts correlated with the amount of blood loss. However, WBC and platelet counts (except on D1) did not show a relationship with blood loss. Biochemically, the ratios of albumin, total protein, ALP, GGT, total cholesterol, and TIBC on D0AB negatively correlated with blood loss, indicating a dilution effect due to blood loss. Conversely, increases in AST and ALT levels on D0AB and/or D1 were not related to blood loss. A positive correlation was observed between the increase in CK levels on D1 and the extent of blood loss. Analysis of the relationship between AST, ALT, and CK ratio values on D1 and the hemoglobin ratio on D1 revealed strong correlations (rho = -0.770, -0.818, and -0.915; P = 0.0092, 0.0038, and 0.0002, respectively), suggesting that a decrease in hemoglobin levels could trigger the responses of these enzymes.
Interestingly, the iron levels, which significantly decreased after blood loss, showed a strong negative correlation with the blood loss on D14. UIBC ratios on D5, D7, D21, and D28 positively correlated with blood loss, and TIBC ratios negatively correlated on D0AB but positively correlated with the blood loss on D21 and D28. Among the immunological parameters, net C3a values on D0AB and D7 negatively correlated, and net IL-15 values on D1 and D5 positively and negatively correlated, respectively, with the extent of blood loss, indicating high variability.
4 Discussion
Massive bleeding is a serious pathological condition that can lead to death without blood transfusion, making it an excellent disease model for developing RBC substitutes for blood transfusion. Investigating the therapeutic effect of RBC substitutes for blood transfusion warrants data on temporal changes in individual vitals for a simple blood loss situation. In clinical situations, massive bleeding may be associated with complex factors, such as trauma, underlying diseases, and treatment choices. Severe injury with massive bleeding has been linked to systemic inflammatory response syndrome, which is accompanied by a compensatory anti-inflammatory response characterized by elevated levels of anti-inflammatory cytokines, such as IL-10 and transforming growth factor-β, and cytokine antagonists, such as IL-1Ra (12).
Examining biological responses based on the degree of blood loss in primates, including humans, presents a considerable challenge owing to ethical issues. Considering in vivo experiments, various factors can impact subjects utilized when assessing the efficacy of blood substitutes. Our first step was to establish a blood-loss NHP model and examine the changes in hematological, biochemical, and immunological markers during blood loss. Leukocyte-related changes, especially, increased levels of neutrophils and proinflammatory cytokines (13), which are commonly seen in patients receiving blood, occurred immediately after catheterization in NHPs and were not directly related to the amount of blood loss. Subsequently, we systematically tested individual factors in a clinical situation of complex massive blood loss to explore direct biological changes.
The Advanced Trauma Life Support (ATLS) Manual provided by the American College of Surgeons, describes four classes of hemorrhage to emphasize early signs of shock (14). Based on the total blood volume, class I hemorrhage is classified as a loss of up to 15%, class II hemorrhage as 15 to 30%, class III hemorrhage is classified as a loss of 30 to 40%, and class IV hemorrhage is classified as a loss of > 40%. Symptoms of class II hemorrhage include tachycardia, tachypnea, and decreased pulse pressure. There may be a sense of coolness and clamminess on the skin as well as a delay in the degree of capillary refill. Changes in mental status are presented with class III hemorrhage. According to the ATLS manual, monitoring is recommended in cases of class I hemorrhage, and blood transfusion is recommended in cases of class II hemorrhage. In clinical practice, a transfusion trigger, based on the hemoglobin level, is used when deciding on blood transfusion (15). However, no criteria have been established for blood loss in NHPs. In this investigation, clinical symptoms of decompensation and a substantial decrease in systolic BP were observed exclusively in NHPs subjected to 25% blood loss, yet this did not result in any fatalities. Consequently, we postulate that a blood loss volume of 25% is an appropriate threshold in NHP models for assessing the efficacy of blood transfusion or alternative therapeutic interventions. Regarding blood donation protocols, it is advisable that the volume donated should not exceed 25% of the total blood volume in NHP donors to avoid inducing physiological decompensation, as evidenced by the consequences of a 25% blood loss.
RBC-related parameters demonstrated the most direct correlation with blood loss. In the ratio plots against baseline results, RBC, hemoglobin, and hematocrit exhibited nearly identical trends, reaching their nadir between D1 and D5. The lowest hemoglobin level, typically observed between 1–3 days after bleeding, was likely due to the redistribution of body fluids. This value varied between D1–D5 for each individual, with precise timing challenging to ascertain due to the insufficient frequency of blood collection. Reticulocyte counts began to rise from D3, peaking at D7 across all subjects, followed by normalization. The increase in MCV after bleeding is presumed to result from an elevated production of comparatively larger young RBCs; the bleeding increases their proportion relative to that of old RBCs with low MCV. In this study, analyzing the data as ratios to baseline proved more useful due to significant individual variation. Most soluble substances in the blood experienced a temporary dilution due to blood loss before recovery.
The levels of some enzymes, such as CK, AST, and ALT, showed reactive increases, which are hypothesized to be a complex effect of blood loss and experimental injury. The increased ratios of the levels of these enzymes after bleeding correlated more strongly with the hemoglobin ratio than with the amount of blood loss, suggesting that the hemoglobin ratio value might be a more reliable indicator for predicting clinical state than the quantity of blood loss itself. Previous human studies have detected no or only weak correlations between estimated blood loss during surgery and changes in hemoglobin (16, 17). Given that intraoperative blood loss is estimated visually, it cannot be empirically correlated with changes in hemoglobin levels. Elevated liver enzyme levels have been reported in various situations, and aminotransferases have been found to increase by 10- to 50-fold the upper limit in ischemic injuries. The increase in liver enzyme levels observed in our study appears to align with findings from previous research, indicating that acute massive bleeding leads to instantaneous tissue ischemia and elevated liver enzyme levels (18).
Blood loss led to a decrease in serum iron levels, which reached their lowest between 5–7 days after bleeding before gradually recovering; however, levels had not normalized by day 28. Notably, both TIBC and UIBC continued to rise even on day 28, indicating unresolved iron deficiency. While this study could not provide a definitive interval for blood donation, the results suggest that after a 25% blood loss, a one-month recovery period before subsequent donations might not be sufficient; iron supplementation should be considered if necessary.
The levels of IL-1α, IL-15, and MCP-1, representative proinflammatory cytokines, increased from D0BB (before bleeding), suggesting that these changes were related to the experimental process rather than the bleeding itself. This observation was similar in the increase in the WBC and neutrophil counts. The levels of the complement activation fragment C3a and C4a, which are also associated with these inflammatory cytokines, showed increases and were sometimes negatively correlated with the amount of blood loss. This may be due to a combined effect of inflammatory responses and the reduced concentrations of the C3 and C4 proteins due to blood loss.
Accumulated evidence on postoperative bleeding has shown that surgical trauma can considerably impact a patient’s immune system. Intraoperative blood loss during major surgery leads to reduced blood flow, regional hypoxia, and metabolic and microenvironmental changes, resulting in an inflammatory response characterized by proinflammatory cytokines and acute phase proteins (19). Similarly, during surgery, there is an early hyperinflammatory response involving the release of proinflammatory cytokines, such as tumor necrosis factor-alpha, IL-1, and IL-6, neutrophil activation, microvascular adhesion, as well as an uncontrolled oxidative burst of polymorphonuclear cells and macrophages (20). In previous studies, various factors that could affect the patient’s condition were not controlled owing to the surgical environment, and the precise amount of bleeding could not be measured. In the current study, we strictly controlled the amount of bleeding from 10 to 25%, depending on the body weight of the NHPs, illustrating the strength of our study.
A major limitation of our study was the small number of NHPs assigned to each condition. Although a difference was observed in the results for each condition, it is unclear whether this reflects a difference in the baseline of the individual or condition. To overcome these limitations, we statistically analyzed the increase (ratio) or change (differences) in each individual, based on their baseline values. Increasing the number of NHPs in each condition would be statistically beneficial but difficult in practice. This is a general limitation of NHP research. A previous NHP research review has shown that the number of individuals assigned to groups ranges from 1 to 8 for the smallest group size and 3 to 16 for the largest group size (21). In our NHP-based experiment, we used the relative values (ratio or difference) compared to the baseline values for the statistical analysis to minimize the impact of the limitation attributed to the small number of animals.
The implications of our study extend to predictive medicine, especially regarding the biological responses during massive blood loss. Controlled experiments on humans in emergencies, such as massive bleeding, often encounter ethical and practical constraints. Thus, our study provides a useful framework to design primate experiments on blood loss or transfusion, particularly when considering intervention effects following blood loss, and can aid in interpreting the results of related primate experiments. For future studies, we are planning a xenotransfusion experiment using porcine erythrocytes to analyze their biological effects in primates.
Data availability statement
The original contributions presented in the study are included in the article/supplementary material. Further inquiries can be directed to the corresponding author.
Ethics statement
The animal study was approved by Korea Institute of Toxicology Institutional Animal Care and Use Committee (IAC-22-01-0342-0037). The study was conducted in accordance with the local legislation and institutional requirements.
Author contributions
JR: Formal analysis, Visualization, Writing – original draft. EP: Data curation, Investigation, Methodology, Writing – review & editing. HL: Data curation, Investigation, Methodology, Writing – review & editing. JH: Project administration, Supervision, Writing – review & editing. H-SK: Data curation, Investigation, Methodology, Writing – review & editing. JP: Formal analysis, Software, Writing – review & editing. HJK: Writing – original draft, Conceptualization, Funding acquisition, Project administration, Resources, Supervision.
Funding
The author(s) declare financial support was received for the research, authorship, and/or publication of this article. This work was supported by the Institute of Civil Military Technology Cooperation funded by the Defense Acquisition Program Administration and the Ministry of Trade, Industry, and Energy of the Korean Government (Grant no. 22-CM-EC-18).
Acknowledgments
The authors acknowledge that all participated in this study. We would like to thank Editage (www.editage.com) for English language editing.
Conflict of interest
The authors declare that the research was conducted in the absence of any commercial or financial relationships that could be construed as a potential conflict of interest.
Publisher’s note
All claims expressed in this article are solely those of the authors and do not necessarily represent those of their affiliated organizations, or those of the publisher, the editors and the reviewers. Any product that may be evaluated in this article, or claim that may be made by its manufacturer, is not guaranteed or endorsed by the publisher.
Abbreviations
ALP, Alkaline phosphatase; ALT, Alanine aminotransferase; AST, Aspartate aminotransferase; ATLS, Advanced Trauma Life Support; BP, Blood pressure; BUN, Blood urea nitrogen; CK, Creatine phosphokinase; ELISA, Enzyme-linked immunosorbent assay; GGT, Gamma glutamyl transpeptidase; IACUC, Institutional Animal Care and Use Committee; IL, Interleukin; MCH, Mean corpuscular hemoglobin; MCHC, Mean corpuscular hemoglobin concentration; MCP-1, Monocyte chemoattractant protein-1; MCV, Mean corpuscular volume; NHP, Nonhuman primates; RBC, Red blood cells; TIBC, Total iron-binding capacity; UIBC, Unsaturated iron-binding capacity; WBC, White blood cell.
References
1. Roberts N, James S, Delaney M, Fitzmaurice C. The global need and availability of blood products: a modelling study. Lancet Haematol (2019) 6:e606–15. doi: 10.1016/S2352-3026(19)30200-5
2. Dzik WH. Innocent lives lost and saved: the importance of blood transfusion for children in sub-Saharan Africa. BMC Med (2015) 13:22. doi: 10.1186/s12916-014-0248-5
3. Greinacher A, Weitmann K, Schönborn L, Alpen U, Gloger D, Stangenberg W, et al. A population-based longitudinal study on the implication of demographic changes on blood donation and transfusion demand. Blood Adv (2017) 1:867–74. doi: 10.1182/bloodadvances.2017005876
4. Wang Z, Chen T, Li X, Guo B, Liu P, Zhu Z, et al. Oxygen-releasing biomaterials for regenerative medicine. J Mater Chem B (2023) 11:7300–20. doi: 10.1039/d3tb00670k
5. Han H, Rim YA, Ju JH. Recent updates of stem cell-based erythropoiesis. Hum Cell (2023) 36:894–907. doi: 10.1007/s13577-023-00872-z
6. Cooper DK, Hara H, Yazer M. Genetically engineered pigs as a source for clinical red blood cell transfusion. Clin Lab Med (2010) 30:365–80. doi: 10.1016/j.cll.2010.02.001
7. Zhu A. Introduction to porcine red blood cells: implications for xenotransfusion. Semin Hematol (2000) 37:143–9. doi: 10.1016/s0037-1963(00)90039-8
8. Nakamura T, Fujiwara K, Saitou M, Tsukiyama T. Non-human primates as a model for human development. Stem Cell Rep (2021) 16:1093–103. doi: 10.1016/j.stemcr.2021.03.021
9. Majde JA. Animal models for hemorrhage and resuscitation research. J Trauma (2003) 54(Supplement):S100–5. doi: 10.1097/01.TA.0000064503.24416.F4
10. Hildebrand F, Andruszkow H, Huber-Lang M, Pape H-C, van Griensven M. Combined hemorrhage/trauma models in pigs—current state and future Perspectives. Shock (2013) 40:247–73. doi: 10.1097/SHK.0b013e3182a3cd74
11. Diehl K-H, Hull R, Morton D, Pfister R, Rabemampianina Y, Smith D, et al. A good practice guide to the administration of substances and removal of blood, including routes and volumes. J Appl Toxicol (2001) 21:15–23. doi: 10.1002/jat.727
12. Lord JM, Midwinter MJ, Chen YF, Belli A, Brohi K, Kovacs EJ, et al. The systemic immune response to trauma: an overview of pathophysiology and treatment. Lancet (2014) 384:1455–65. doi: 10.1016/S0140-6736(14)60687-5
13. Brand A. Immunological aspects of blood transfusions. Transpl Immunol (2002) 10:183–90. doi: 10.1016/s0966-3274(02)00064-3
14. American College of Surgeons. Advanced Trauma Life Support® (ATLS®) Student Course Manual. 10th ed. Chicago: ACS American College of Surgeons (IL (2018).
15. Rahimi-Levene N, Ziv-Baran T, Peer V, Golik A, Kornberg A, Zeidenstein R, et al. Hemoglobin transfusion trigger in an internal medicine department - A ‘real world’ six year experience. PloS One (2018) 13:e0193873. doi: 10.1371/journal.pone.0193873
16. Anger H, Durocher J, Dabash R, Winikoff B. How well do postpartum blood loss and common definitions of postpartum hemorrhage correlate with postpartum anemia and fall in hemoglobin? PloS One (2019) 14:e0221216. doi: 10.1371/journal.pone.0221216
17. Jansen AJ, LeNoble PJ, Steegers EA, Van Rhenen DJ, Duvekot JJ. Relationship between haemoglobin change and estimated blood loss after delivery. BJOG (2007) 114:657–. doi: 10.1111/j.1471-0528.2007.01259.x
18. Giannini EG, Testa R, Savarino V. Liver enzyme alteration: a guide for clinicians. CMAJ Can Med Assoc J (2005) 172:367–79. doi: 10.1503/cmaj.1040752
19. Pretzsch E, Bösch F, Renz B, Werner J, Angele M, Chaudry IH. Operative trauma and blood loss — impact on tumor growth and recurrence. Shock (2021) 55:455–64. doi: 10.1097/SHK.0000000000001656
20. Menger MD, Vollmar B. Surgical trauma: hyperinflammation versus immunosuppression? Langenbecks Arch Surg (2004) 389:475–84. doi: 10.1007/s00423-004-0472-0
Keywords: biological response in hemorrhage, controlled blood loss, immune response, nonhuman primate, xenotransfusion
Citation: Roh J, Park EM, Lee H, Hwang JH, Kim H-S, Park J and Kang HJ (2024) Biological response of nonhuman primates to controlled levels of acute blood loss. Front. Immunol. 14:1286632. doi: 10.3389/fimmu.2023.1286632
Received: 31 August 2023; Accepted: 18 December 2023;
Published: 10 January 2024.
Edited by:
Timur O. Yarovinsky, Yale University, United StatesReviewed by:
Juliete A. F. Silva, Emory University, United StatesTarun Tyagi, Yale University, United States
Copyright © 2024 Roh, Park, Lee, Hwang, Kim, Park and Kang. This is an open-access article distributed under the terms of the Creative Commons Attribution License (CC BY). The use, distribution or reproduction in other forums is permitted, provided the original author(s) and the copyright owner(s) are credited and that the original publication in this journal is cited, in accordance with accepted academic practice. No use, distribution or reproduction is permitted which does not comply with these terms.
*Correspondence: Hee Jung Kang, kangheejung@hallym.ac.kr