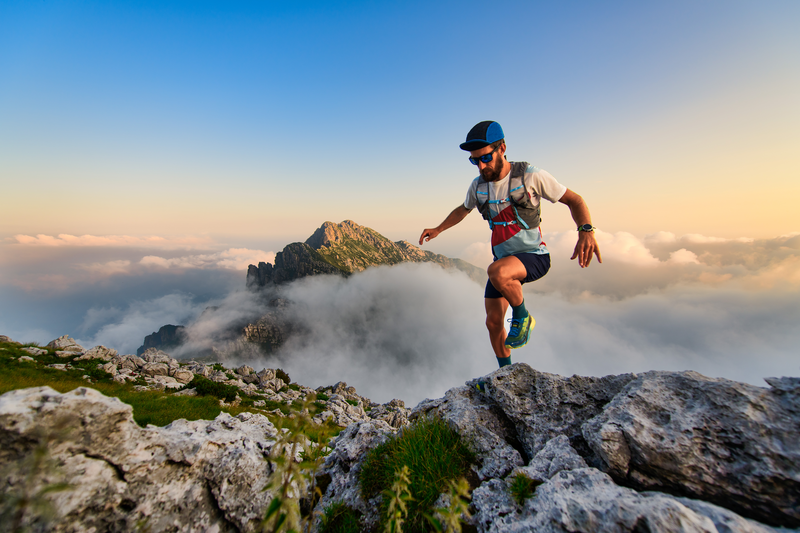
95% of researchers rate our articles as excellent or good
Learn more about the work of our research integrity team to safeguard the quality of each article we publish.
Find out more
ORIGINAL RESEARCH article
Front. Immunol. , 11 December 2023
Sec. Alloimmunity and Transplantation
Volume 14 - 2023 | https://doi.org/10.3389/fimmu.2023.1281130
Introduction: Alloimmune responses against platelet antigens, which dominantly target the major histocompatibility complex (MHC), can cause adverse reactions to subsequent platelet transfusions, platelet refractoriness, or rejection of future transplants. Platelet transfusion recipients include individuals experiencing severe bacterial or viral infections, and how their underlying health modulates platelet alloimmunity is not well understood.
Methods: This study investigated the effect of underlying inflammation on platelet alloimmunization by modelling viral-like inflammation with polyinosinic-polycytidylic acid (poly(I:C)) or gram-negative bacterial infection with lipopolysaccharide (LPS), hypothesizing that underlying inflammation enhances alloimmunization. Mice were pretreated with poly(I:C), LPS, or nothing, then transfused with non-leukoreduced or leukoreduced platelets. Alloantibodies and allogeneic MHC-specific B cell (allo-B cell) responses were evaluated two weeks later. Rare populations of allo-B cells were identified using MHC tetramers.
Results: Relative to platelet transfusion alone, prior exposure to poly(I:C) increased the alloantibody response to allogeneic platelet transfusion whereas prior exposure to LPS diminished responses. Prior exposure to poly(I:C) had equivalent, if not moderately diminished, allo-B cell responses relative to platelet transfusion alone and exhibited more robust allo-B cell memory development. Conversely, prior exposure to LPS resulted in diminished allo-B cell frequency, activation, antigen experience, and germinal center formation and altered memory B cell responses.
Discussion: In conclusion, not all inflammatory environments enhance bystander responses and prior inflammation mediated by LPS on gram-negative bacteria may in fact curtail platelet alloimmunization.
Transfusion of platelet concentrates is a critically important, life-saving procedure to treat thrombocytopenia in the context of congenital disorders, surgery, hemorrhagic trauma, and cancer therapy and continues to be developed for novel applications in regenerative medicine (1–4). A major complication emerges, however, when transfusion of allogeneic platelets is recognized by the recipient immune system as foreign, which can progress to alloimmunization against a range of alloantigens, the dominant being the major histocompatibility complex (MHC) antigens expressed on platelets and white blood cells (WBCs) (5). Long-term adverse post-transfusion sequelae of alloimmunization include platelet refractoriness to subsequent platelet transfusion (class I MHC alloimmunization) and rejection of future solid organ or hematopoietic stem cell transplants (class I and II MHC alloimmunization) in some patients (6–11).
Several practices have been adopted to mitigate the risk of alloimmunization following platelet transfusion such as reducing the immunogenicity of blood products. Modern leukoreduction practices, which filter the highly immunogenic WBCs from blood products, have decreased alloimmunization risk (12–18), however, recent estimates suggest alloantibody generation still occurs in ~4-18% of platelet recipients, where the responses are largely directed against MHC antigens on platelets and residual leukocytes (19–22). A potential complementary approach to mitigate alloimmunization risk may be to identify which recipients are at greatest risk of alloimmunization due to genetic factors or underlying health to better manage care. Transfusion recipients normally receive blood products because of a medical condition, and these conditions can create inflammatory or immunotolerant environments for blood-derived alloantigens. Advances have been made to understand the effects of underlying health on red blood cell (RBC) transfusions (23–27), but there is a dearth of research on its effects on alloimmunization to platelets.
Estimates suggest 10-14% of patients admitted to the hospital for severe bacterial or viral infections received RBC transfusions and ~6% received platelet transfusion as a component of their care (27–29). Bacterial and viral infections are synonymous with inflammation, as microorganism derived pathogen-associated molecular patterns (PAMPs) are quintessential stimulators of innate immunity (30). In a conventional immune response, adaptive immunity responds in an antigen-specific manner (31). However, ongoing infection and inflammation can elicit bystander activation where off-target adaptive immunity is activated in an antigen-independent manner due to a heightened inflammatory cytokine milieu (32, 33). Virus-mimetics such as polyinosinic-polycytidylic acid (poly(I:C)) and the bacterial component lipopolysaccharide (LPS) from gram-negative bacteria have been shown to induce bystander activation when directly injected into mice (34, 35). Therefore, patients admitted to the hospital for infection may sustain a reduced threshold for immune activation and consequently potentiate alloimmunization to subsequent exposure to platelet alloantigens during transfusion.
Conducting studies on the effect of underlying health on platelet alloimmunization is challenging clinically due to confounding factors such as the diversity of the patient population and the variability of the type and amount of blood products they receive. Furthermore, alloantigen-specific B cells (allo-B cells) are a rare population and in the presence of inflammatory signals may be further masked by non-specific activation. To address these issues, this study used murine models and MHC tetramers to track allo-B cell responses. The impact of underlying inflammation on platelet alloimmunization was evaluated using poly(I:C) to mimic a viral infection or LPS to mimic a bacterial infection prior to platelet transfusion with the hypothesis that underlying inflammation enhances alloimmunization.
Female BALB/cJ (BALB/c) recipient mice, aged 9 weeks, male and female C57Bl/6J (B6) donor mice, aged 2-12 months, and male and female B6;129P2-β2mtm1Unc/J mice (β2M-KO) were received from The Jackson Laboratory, and/or bred in-house, and maintained in a specific pathogen free vivarium at Vitalant Research Institute (San Francisco, CA) (36). Mice acclimated for at least two weeks prior to use. Research was approved by the Institutional Animal Care and Use Committee at Labcorp Early Developmental Laboratories Inc. (San Carlos, CA) under Animal Welfare Assurance A3367-01.
Poly(I:C) (Innaxon) was prepared at 500 μg/mL and LPS (Sigma) at 125 μg/mL in sterile endotoxin free saline (Innaxon). Recipient BALB/c mice received 200 μL of poly(I:C) or LPS by intraperitoneal route four hours prior to platelet transfusion (+/- 15 minutes) (Figure 1). Controls were given poly(I:C) only, LPS only, transfusion (Tx) only, or no treatment.
Blood from B6 donor mice was collected by orbital enucleation under inhalation isoflurane anesthesia into 14% citrate phosphate dextrose adenine-1, and non-leukoreduced platelets were isolated from blood after gentle centrifugation and Ficoll separation as previously described (5, 17, 37–41). Complete blood counts (CBCs) were run on the HT-5 (Heska) according to manufacturer’s instructions. The non-leukoreduced platelet product had a mean of 3.98x108 platelets/mL and 5.28x106 WBCs/mL. Recipient BALB/c mice were given 100 μL of non-leukoreduced or leukoreduced platelets by lateral tail vein I.V. injection administered within four hours of collection. Non-leukoreduced platelets were used for Figure 2. Leukoreduced platelets were used for Figure 3. Non-leukoreduced platelets were used for Figures 4–10. Leukoreduced platelets were prepared as above except they were passed through a Pall Acrodisc WBC Syringe Filter right after collection. The leukoreduced platelet product had 5.67x108 platelets/mL and WBCs were below the level of detection (<0.3x106 cells/mL). Two weeks after transfusion, serum was collected to screen for alloantibodies, and the spleen and inguinal lymph nodes in some mice were harvested for cellular analysis.
Figure 1 Experimental strategy and anti-class I and II MHC antibody screen. (A, B) Schematic diagram modelling the effect of underlying inflammation on platelet alloimmunization. B6 donor platelet was administered I.V. into recipient BALB/c mice on day zero (D0). Four hours prior (-4 Hrs) to platelet transfusion (Tx), LPS or poly(I:C) was injected into recipient BALB/c mice by intraperitoneal (I.P.) route to model underlying inflammation. Blood for serum, spleen, and lymph nodes from recipient mice were harvested on day fourteen (D14) after transfusion for analysis. (C) Schematic diagram of anti-class I MHC antibody screen. Serum from recipient BALB/c mice was used as a primary stain on B6 splenocytes, followed by a secondary antibody (2° ab) stain with a cocktail of α-Igκ, α-IgM, α-IgG1, α-IgG2a, α-IgG2b, and α-IgG3 antibodies to detect total and isotype-specific alloantibodies by flow cytometry. (D) Cells were gated on singlet, lymphocyte, and then T cells (B220-/TCRβ+) to identify bound alloantibodies on a non-Igκ, non-class II MHC population that expresses class I MHC. α-Igκ only shown as illustration. Median fluorescence intensity (MFI) of total α-Igκ alloantibodies for untreated (U) and with transfusion (Tx) sample are shown. (E) Schematic diagram of anti-class II MHC antibody screen. Protocol was similar to anti-class I MHC antibody screen except cultured dendritic cells (DC) from the bone marrow of β2M-KO mice on the B6 background were used as target cells. (F) Cells were gated on singlet, lymphocyte, and then dendritic cells (CD11c+/F4/80-) to identify bound alloantibodies on non-class I MHC population that expresses class II MHC.
Total [Igκ represents ~95% of all murine B cells (42–47)] and isotype-specific anti-donor alloantibodies were evaluated in recipient serum by flow cytometry (Figures 1C–F). Blood was collected by orbital enucleation from recipient mice, allowed to clot in room temperature for 20-30 minutes, centrifuged to collect serum, and stored at -80°C. Thawed serum was screened against B6 target splenocytes for alloantibodies against class I MHC antigens or screened against cultured dendritic cells from β2M-KO mice for alloantibodies against class II MHC antigens. Dendritic cells were cultured from the bone marrow of β2M-KO mice for 11 days with granulocyte-macrophage colony-stimulating factor (GM-CSF) every 2 or 3 days and LPS 24 hours prior to collection. Target cells were blocked with Fc receptor block (FcR, 2.4G2, Becton Dickinson Biosciences, BD), stained with 1:16 diluted recipient serum, washed, and stained with a secondary antibody cocktail containing anti-mouse B220-Alexa Fluor (AF)700 (RA3-6B2), T cell receptor (TCR)β-peridinin-chlorophyll-protein (PerCP)-Cyanine (Cy)5.5 (H57-597), immunoglobulin (Ig)κ-allophycocyanin (APC)-Cy7 (RMK-45), IgG2b-phycoerythrin (PE; RMG2b-1), IgG2a-APC (RMG2a-62), IgG1-AF488 (RMG1-1), IgM-PE-Cy7 (RMM-1) (Biolegend), and IgG3-Brilliant Violet (BV)421 (R40-82; BD) for the anti-class I antibody screen. Anti-mouse F4/80-AF700 (BM8) and CD11c PerCP-Cy5.5 (N418) were used for the anti-class II antibody screen. Median fluorescence intensity (MFI) of Igκ and each isotype were normalized by dividing each sample value by the average of the untreated group.
Spleen and lymph nodes were homogenized by enzymatic digestion with 0.4 mg/mL collagenase P (Roche), 1.6 mg/mL dispase (Invitrogen), and 0.2 mg/mL DNase I (Roche) for 20 minutes at 37°C and passed through a 100 μM filter. Homogenates were subjected to red blood cell lysis, FcR block (2.4G2, BD), viability staining (BD Live/Dead Blue), and staining with antibody cocktail in Brilliant Stain Buffer (BD). Anti-mouse antibodies included: Sca-1-BV421 (D7), Sca-1-AF488 (D7), CD19-PerCP (6D5), CD138-BV711 (281–2), IgM-BV711 (RMM-1), CD11b-BV570 (M1/70), CD73-AF594 (TY/11.8), IgD-AF594 (11-26c.2a), and B220-BV650 (RA3-6B2) (Biolegend); CD86-Brilliant Blue (BB)700 (GL1), CD5-BV750 (53-7.3), CD80-BV480 (16-10A1), PD-L2-Brilliant Ultra Violet (BUV)737 (Ty25), CD95-BUV805 (Jo2), CD93-BV605 (AA4.1), CD35-BV650 (C12), CD38-BUV563 (90/CD38), IgM-BUV615 (II/41), CD23-BUV395 (B3B4) and IgD-BUV496 (AMS9.1) (BD); B220-AF532 (RM0063-9F14, Novus Biologicals); and CD73-AF750 (496406, R&D Systems). To exclude non-B cells, the following AF700 conjugated anti-mouse antibodies were combined: F4/80 (BM8), GR-1 (RB6-8C5), CD3 (17A2), CD4 (GK1.5), CD8 (53-6.7), and CD11c (N418) (Biolegend); NKp46 (29A1.4, BD).
A syngeneic decoy (decoy, a recipient-type MHC:peptide tetramer) was used to exclude B cells specific to non-MHC components of the tetramer. Allogeneic tetramer staining was done with the same donor-type MHC molecule conjugated to two separate fluorophores, PE and APC. Double positive (PE+/APC+) events were donor-reactive MHC antigen-specific allo-B cells, whereas single positive events specific to the fluorophores were excluded. All MHC tetramers were generously provided by the National Institutes of Health (NIH) tetramer core facility which included MHC class I tetramer H-2Kb (SIINFEKL) and MHC class II tetramer I-Ab (PVSKMRMATPLLMQA) that were conjugated to PE and APC, decoy H-2Kd-AF488 (SYIPSAEKI), and decoy I-Ad-BV421 (ISQAVHAAHAEINEAGR).
Flow cytometry samples were run on a Cytek Aurora 5 Laser Cytometer with Spectroflow software (Cytek Biosciences). Cytometry data was analyzed on FlowJo software version 10.8.1 (FlowJo, LLC). Data presentation and statistics were prepared on Prism version 9.5.1 (GraphPad Software, Inc.).
Groups were compared using one-way analysis of variance followed by Tukey’s multiple comparison post-test. Significance was reported for comparisons with p<0.05. No significance was found when not indicated with an asterisk. Data were combined from 3-4 independent experiments with 3-4 mice per group except where noted; individual data points represent different mice pooled from repeated experiments unless otherwise noted.
To evaluate if underlying inflammation exacerbates the alloresponse, recipient mice were administered poly(I:C) or LPS four hours prior to non-leukoreduced or leukoreduced platelet transfusion and screened for total and isotype-specific serum anti-donor alloantibodies two weeks later (Figures 1A–F). Untreated mice, and mice that received platelet transfusion, poly(I:C), or LPS alone were evaluated as controls. In mice that were administered poly(I:C) or LPS alone, no changes in alloantibodies were detected, but a single non-leukoreduced platelet transfusion significantly increased anti-class I MHC alloantibody responses across all examined isotypes relative to untreated mice (Figure 2A). Prior exposure to poly(I:C) followed by platelet transfusion (poly(I:C)/Tx) increased total alloantibody responses whereas prior exposure to LPS followed by platelet transfusion (LPS/Tx) showed a decrease in total alloantibody responses relative to platelet transfusion alone. The elevated total alloantibody in poly(I:C)/Tx was associated with increased IgG2a and IgG3 and decreased IgG1 relative to transfusion alone. The diminished total alloantibody in LPS/Tx was associated with decreased IgM and IgG1 although a downward trend could be observed with all the other isotypes, relative to transfusion alone. Of note, the isotype of greatest magnitude in the LPS/Tx groups was IgM. Responses against class II MHC antibodies were consistent with class I except for subtle differences in IgG2b where prior poly(I:C) exposure elevated responses (Figure 2B).
Figure 2 Poly(I:C) increases and LPS decreases the alloantibody response to non-leukoreduced platelet transfusion. (A) Total and isotype-specific anti-class I MHC antibody and (B) anti-class II MHC antibody responses at 14 days post non-leukoreduced platelet transfusion. For IgG1 for anti-class I MHC antibody (A), 100 was added to the raw values prior to normalization to adjust for negative values. Normalized MFI in mice that were untreated (), received transfusion alone (
), poly(I:C) alone (
), poly(I:C) followed by transfusion (
), LPS alone (
), or LPS followed by transfusion (
). (p)<0.05 (*), p<0.01 (**), p<0.001 (***), and p<0.0001 (****).
To determine how the presence of WBCs impact the effect of underlying inflammation, the experiment was repeated with leukoreduced platelets (Figure 3). The response to leukoreduced platelet transfusion alone was similar to non-leukoreduced, although the magnitude of the responses was smaller, consistent with prior work (38, 39). Moreover, the effect of inflammatory signals on leukoreduced platelets paralleled that of non-leukoreduced platelets where prior poly(I:C) exposure showed trends of elevated responses, whereas prior LPS exposure showed trends of diminished responses for total and isotype-specific alloantibodies. As the effect with leukoreduced platelets were consistent with non-leukoreduced and since leukoreduced platelets were less immunogenic, non-leukoreduced platelets were used as a worse-case model to elicit stronger alloimmunity and examine cellular responses.
Figure 3 The effects of underlying inflammation on leukoreduced platelet transfusion are consistent with non- leukoreduced platelet transfusion, although the magnitude of the responses is smaller. Poly(I:C) increases and LPS decreases the alloantibody response to leukoreduced platelets. Normalized MFI in mice that were untreated (), received leukoreduced (LR) platelet transfusion alone (
), poly(I:C) followed by leukoreduced transfusion (
), LPS followed by leukoreduced transfusion (
), and non-leukoreduced (NLR) platelet transfusion alone (
). 10 mice per group in a single experiment. For IgG1 and IgG3, 200 was added to the raw values prior to normalization to adjust for negative values. (p)<0.05 (*), p<0.01 (**), p<0.001 (***), and p<0.0001 (****). Tick line represents mean of the group that received a leukoreduced transfusion only.
To understand the mechanism regulating the divergent alloantibody outcomes due to prior exposure to poly(I:C) or LPS, cellular responses of allo-B cells were evaluated. Platelet alloimmunization is driven by mismatched MHC between donor and recipient (in our model, H-2b in B6 mice versus H-2d in Balb/c mice). Although the strategy to identify allo-B cells by utilizing MHC tetramers has been well-established in the cardiac allograft model (48–52), it was unclear whether it could be adopted in our platelet transfusion model due to differences in dose, route of administration, and type of antigenic challenge. To evaluate the feasibility of MHC tetramers in detecting allo-B cells with platelet transfusion, allo-B cell responses were compared among B cell populations of differing specificities; allo-B cells were compared to single-fluorophore specific B cells (APC and PE), B cells specific to the non-MHC components of the tetramers (syngeneic decoy+), and the remaining B cell population (Figure 4A). Only the allo-B cell population showed significant enrichment in frequency (Figure 4B), activation (CD86+, Figure 4C), antigen experience (IgD-, Figure 4D), and germinal center (GC) formation (CD95+/CD38-, Figure 4E) after platelet transfusion when evaluated with MHC class I and MHC class II tetramers. Parallel findings were observed in the spleen and lymph node. Thus, MHC tetramers effectively identify allo-B cells in our platelet transfusion model.
Figure 4 Platelet transfusion specifically induces allo-B cell responses. (A) B cell subsets of different specificities were examined for responses in untreated mice (U) and after platelet transfusion (Tx), including B cells specific for the MHC tetramer (Tet, allo-B cells), syngeneic decoy (Decoy), single fluorophore (APC or PE), or the remaining B cell population (Neg). Normalized data shows the frequency of (B) the respective B cell subset out of total B cells, (C) CD86+ activated B cells of the respective B cell subset (see Figure 6 for gating), (D) IgD- antigen-experienced B cells of the respective B cell subset (see Figure 7 for gating), and (E) CD95+/CD38- germinal center (GC) B cells of the respective B cell subset (see Figure 8 for gating). (B–E) Results for splenic (top) and lymph node (bottom) cells were stained with MHC class I (left) and MHC class II (right) tetramers. Normalization was achieved by taking the average of the respective untreated group. Tick line falls on mean of untreated group of Neg subset. p<0.01 (**) and p<0.0001 (****).
While MHC class I is a more abundant source of antigen in our non-leukoreduced platelets (expressed by all platelets and leukocytes), class II antigen is also present (expressed on many blood leukocytes), therefore we evaluated responses to both. An intravenous route of delivery of antigen should engage systemic immunity, not a localized reaction, so spleen and lymph nodes were evaluated for confirmation. Recipient mice were treated as described above, and spleen and lymph nodes were harvested two weeks after platelet transfusion (Figure 1B). Allo-B cells were identified as singlets, lymphocytes, live cells, non-B cell-/B220+ cells, CD19+/syngeneic decoy- B cells, and tetramer double-positive events (Figure 5A).
Figure 5 Transfusion-induced expansion of alloreactive B cells is impaired following exposure to LPS. Frequency of allo-B cells (out of total B cells without syngeneic decoy events) were identified with MHC tetramers by flow cytometry two weeks after platelet transfusion. (A) Cells were first gated on singlet, lymphocytes, live cells, non-B cell-/B220+ cells, and CD19+/syngeneic decoy- B cells, then evaluated for tetramer staining. A two fluorophore (APC and PE)-one MHC tetramer strategy was used with allo-B cells identified as double positive tetramer (tet) events. (B–E) Shown is allo-B cell frequency in mice that were untreated (), received transfusion alone (
), poly(I:C) alone (
), poly(I:C) followed by transfusion (
), LPS alone (
), or LPS followed by transfusion (
). Splenic and lymph node cells were stained with MHC class I (B, C) and MHC class II (D, E) tetramers. Tick line represents mean of untreated group. (p)<0.05 (*), p<0.01 (**), p<0.001 (***), and p<0.0001 (****).
The frequency of allo-B cells was consistently low among the non-transfused control groups (untreated, Poly(I:C) only, and LPS only) (Figures 5B–E). A single platelet transfusion induced a significant increase of allo-B cells relative to untreated mice (Figures 5B–E). Relative to transfusion alone, poly(I:C)/Tx had no effect on the frequency of allo-B cells induced by platelet transfusion (Figures 5B–E). Poly(I:C)/Tx showed significantly enhanced allo-B cell frequency when compared to poly(I:C) alone (increased trend only for Figure 5C). Strikingly, LPS/Tx showed no significant differences when compared to LPS alone, and importantly, showed reduction in allo-B cell frequency relative to transfusion alone (Figures 5B, D, E, decreased trend only for Figure 5C). Similar patterns were observed in the spleen and lymph nodes, and against MHC class I and class II, though slightly more pronounced against class II.
Allo-B cell responses were next evaluated for activation by assessing upregulation of CD86, downregulation of IgD (indicating antigen-experience), and formation of GC reactions (CD38-/CD95+). These populations can be readily identified two weeks after a single platelet transfusion (Figures 6A, 7A, 8A). In the non-transfused control groups, little to no activation of allo-B cells was observed (Figures 6–8), except for the LPS only group which showed increased IgD downregulation relative to untreated mice in the spleen (Figures 7B, D, trend only in D). Two weeks after a single allogeneic platelet transfusion, the frequency of activated, antigen-experienced, and GC allo-B cells were increased compared with the untreated group (Figures 6–8). Prior exposure to poly(I:C) in the poly(I:C)/Tx group did not induce more allo-B cell activation, antigen-experience, or germinal center reactions when compared to platelet transfusion alone (Figures 6–8). This group did show significant increases in frequencies of activated, antigen-experienced, and GC allo-B cells relative to the poly(I:C) only group (Figures 6–8, increased trend only in Figure 8C). Conversely, frequencies of activated, antigen-experienced, and GC allo-B cells for the LPS/Tx group were not significantly different than the LPS only group but were significantly reduced relative to transfusion alone for responses in the spleen (Figures 6–8B, D). In the lymph node, the allo-B cell responses for the LPS/Tx group were significantly increased relative to LPS alone but were still significantly reduced relative to transfusion alone (Figures 6-8C, E, reduced trend only observed for Figures 6C, 8C). Generally, while trends were similar, responses in the lymph node were more pronounced than in the spleen, and responses against MHC class II were more pronounced than MHC class I (Figures 6–8). The levels of CD86 expression were evaluated in activated CD86+ allo-B cells by MFI, and no differences were observed when transfusion alone was compared to poly(I:C)/Tx but expression levels trended downwards in the lymph node and were significantly reduced in the spleen when transfusion alone was compared to LPS/Tx (data not shown), consistent with frequency data.
Figure 6 Transfusion-induced activation of alloreactive B cells is diminished following LPS exposure. (A) Frequency of activated CD86+ allo-B cells out of total allo-B cells was evaluated with representative examples shown of untreated and platelet transfused mice. (B–E) Shown is activated allo-B cell frequency in mice that were untreated (), received transfusion alone (
), poly(I:C) alone (
), poly(I:C) followed by transfusion (
), LPS alone (
), or LPS followed by transfusion (
). Splenic and lymph node cells were stained with MHC class I (B, C) and MHC class II (D, E) tetramers. Tick line represents mean of untreated group. (p)<0.05 (*), p<0.001 (***), and p<0.0001 (****).
Figure 7 Transfusion-induced down-regulation of IgD is impaired with prior LPS exposure. (A) Frequency of antigen experienced IgD- allo-B cells out of total allo-B cells was evaluated with representative examples shown of untreated and platelet transfused mice. (B–E) Shown is antigen experienced allo-B cell frequency in mice that were untreated (), received transfusion alone (
), poly(I:C) alone (
), poly(I:C) followed by transfusion (
), LPS alone (
), or LPS followed by transfusion (
). Splenic and lymph node cells were stained with MHC class I (B, C) and MHC class II (D, E) tetramers. Tick line represents mean of untreated group. (p)<0.05 (*), p<0.01 (**), p<0.001 (***), and p<0.0001 (****).
Figure 8 Germinal center response to transfusion is diminished with prior LPS exposure. (A) Frequency of germinal center (GC) CD95+/CD38- allo-B cells out of total allo-B cells was evaluated with representative examples shown of untreated and platelet transfused mice. (B–E) Shown is germinal center allo-B cell frequency in mice that were untreated (), received transfusion alone (
), poly(I:C) alone (
), poly(I:C) followed by transfusion (
), LPS alone (
), or LPS followed by transfusion (
). Splenic and lymph node cells were stained with MHC class I (B, C) and MHC class II (D, E) tetramers. (p)<0.05 (*), p<0.01 (**), and p<0.0001 (****).
To evaluate whether marginal zone (MZ) B cells played an important role in our model, MZ B cells were identified as CD93- (mature), CD95-/CD38+ (GC-negative), and IgM+/CD23- (MZ), and their frequencies within the total B cell and allo-B cell populations were assessed (Figure 9A). No significant differences were observed after transfusion for total MZ B cells in the spleen and lymph node (Figures 9B, C). Groups treated with LPS alone showed a significant increase in total MZ B cells (trend only in lymph node, Figures 9B, C), when compared to untreated or transfused only groups. However, this appears independent of transfusion, and others have observed LPS administration directly stimulates MZ B cells (53–55). While no significant increases in MZ allo-B cells were observed after transfusion for the respective pairs, a significant increase was seen for the Poly(I:C)/Tx group relative to the untreated and transfusion only groups (Figures 9D, E). Like total MZ B cells, MZ allo-B cells were non-specifically induced by LPS treatment (Figures 9D, E). No differences were observed in activation and IgD downregulation after transfusion in total MZ and MZ allo-B cells (data not shown). Lymph node MZ allo-B cells were too rare to quantify (data not shown).
Figure 9 LPS non-specifically enriches for marginal zone B cells. (A) Representative gating selected for CD93- mature B cells, CD95-/CD38+ non-GC (GCNeg) B cells, and IgM+/CD23- marginal zone (MZ) B cells. Shown is frequency of total MZ B cells out of total B cells in spleen (B) and lymph node (C). Frequency of marginal zone B cells within the allo-B cell subset population out of total B cells is also shown in the spleen which were stained with MHC class I (D) and MHC class II (E) tetramers. Mice were untreated (), received transfusion alone (
), poly(I:C) alone (
), poly(I:C) followed by transfusion (
), LPS alone (
), or LPS followed by transfusion (
). Tick line represents mean of untreated group. (p)<0.05 (*), p<0.01 (**), p<0.001 (***), and p<0.0001 (****).
We next evaluated the formation of memory B cells within the allo-B cell population. Total memory B cells were defined as non-GC, antigen-experienced, mature allo-B cells (Figure 10A). This population was further delineated into phenotypically and functionally distinct memory subsets by the expression of CD80 and PD-L2 (Figure 10A) (56). Functionally, CD80-/PD-L2- double-negative memory B cells readily seed GC reactions, whereas CD80+/PD-L2+ double-positive memory B cells are poised to differentiate into antibody-secreting cells (57). Although total allo-memory B cells were readily measured in the spleen and lymph node (Figures 10B–E), these events are rare, and the subset double-negative and double-positive memory B cell events were too few to quantify in the lymph node (data not shown). As seen with activation, allo-memory B cell responses were more pronounced in the lymph node compared with the spleen, and against MHC class II compared with class I (Figure 10). While Poly(I:C) alone did not alter allo-memory B cell responses (Figures 10), the LPS alone group had increased total allo-memory B cell responses in the spleen (Figures 10B, D). Compared to untreated mice, a single allogeneic transfusion showed an increase in total allo-memory B cells but was significant only in the lymph node against MHC class II (Figures 10B–E). The poly(I:C)/Tx group showed significantly elevated responses relative to poly(I:C) alone for total allo-memory B cells (Figures 10C–E, no difference in Figure 10B), and these responses trended higher than the transfusion alone group (Figures 10C–E, significant in 10C). The LPS/Tx group showed no differences in total allo-memory B cells relative to LPS only for responses against MHC class I (Figures 10B, C) and showed an increased trend for class II responses (Figures 10D, E). Relative to transfusion alone, total allo-memory B cells in the LPS/Tx group was elevated in the spleen (Figures 10B, D, significant for 10B), and trended down in the lymph node (Figures 10C, E). For allo-memory B cell responses against MHC class II, the transfusion alone and poly(I:C)/Tx groups skewed towards the CD80+/PD-L2+ double-positive subset (Figures 10H, I). In contrast, the non-specific responses against LPS (LPS only and LPS/Tx groups) skewed more towards the PDL2-/CD80- double-negative subset (Figures 10F–I).
Figure 10 Underlying inflammation induced by poly(I:C) enriches for allo-B memory cells. (A) Gating strategy for allo-B memory cells selected for CD95-/CD38+ non-GC (GCNeg) B cells, B220+/IgD- antigen (Ag) experienced B cells, and CD93- mature B cells which represents total memory B cells. Total memory B cells were then selected for CD80-/PD-L2- double negative and CD80+/PD-L2+ double positive subsets of allo-B memory cells. (B–I) Shown is frequency out of total B cells. Splenic and lymph node cells were stained with MHC class I (B, C, F, G) and MHC class II (D, E, H, I) tetramers. (B–E) Frequency of total allo-B memory cells. Frequency of CD80-/PD-L2- double negative allo-B memory cells (F, H) and CD80+/PD-L2+ double positive allo-B memory cells (G, I). Mice were untreated (), received transfusion alone (
), poly(I:C) alone (
), poly(I:C) followed by transfusion (
), LPS alone (
), or LPS followed by transfusion (
). Tick line represents mean of untreated group. (p)<0.05 (*), p<0.01 (**), p<0.001 (***), and p<0.0001 (****).
In this study, we have demonstrated that contrary to our hypothesis, not all inflammatory states enhance the alloresponse, with LPS inhibiting the response to allogeneic platelet transfusion. Using a novel, and highly sensitive tetramer staining approach, we characterized the primary B cell response to allogeneic platelet transfusion. We demonstrated that the reduction in alloantibody responses seen with LPS were accompanied by reduced activation of allo-B cells, reduced differentiation into GC B cells, and reduced PDL2+/CD80+ memory B cells. Poly(I:C), on the other hand, enhanced the alloantibody response and was associated with more robust differentiation into memory B cells in the lymph nodes.
Here we used a novel tetramer staining strategy along with our established platelet transfusion model to assess the antigen-specific B cell response to transfusion. The use of MHC tetramers to track the behavior of allo-B cells has been employed in the cardiac allograft model in mice (48–52, 58), and similarly human leukocyte antigen (HLA) tetramers were used to examine allo-B cells in clinical samples (52, 59), but this strategy had not yet been adopted in the field of transfusion medicine. We demonstrated for the first time that a single allogeneic platelet transfusion elicits robust responses from the rare, endogenous donor-reactive allogeneic MHC antigen-responsive B cell population, with increased activation, GC and memory formation. Memory responses appeared to be slightly skewed towards the CD80+/PD-L2+ double-positive subset, suggesting priming towards efficient future antibody production (57). In contrast with depletion studies from other groups showing a critical role for MZ B cells in RBC and platelet alloimmunization (60–62), we did not see much of a shift in the MZ allo-B cell population. This difference may be due in part to experimental approach, i.e. depletion versus examining endogenous responses at a set time which may have missed the peak MZ response, and/or due to differences in cell type (RBCs and leukoreduced platelets versus non-leukoreduced platelets).
Until now, there has been a paucity of information on the effect of underlying inflammation on platelet alloimmunization. Our results on the antibody response to platelet transfusion are, however, consistent with models of inflammation in RBC alloimmunization, where poly(I:C) enhanced and LPS inhibited humoral alloimmunization (23, 26, 63, 64). Within this model, poly(I:C) was shown to enhance RBC alloantigen processing by dendritic cells leading to increased CD4+ T cell activation (24). Conversely, prior exposure with LPS, consistent with the timing of LPS exposure in our model, inhibits dendritic cells and prevents them from processing RBC alloantigen which abrogates CD4+ T cell help needed for alloimmune responses (65). These data are also consistent with clinical observations of increased trends of RBC alloimmunization among patients experiencing disseminated viral disorders, and potentially decreased RBC alloimmunization among patients suffering from Gram-negative bacteremia (27).
Allo-B cell responses to platelet transfusion were significantly altered by poly(I:C) and LPS. The poly(I:C)/Tx group had allo-B cell responses that were overall similar when compared to transfusion alone, with the exception of more robust memory B cell responses in the lymph node. Like with transfusion alone, the memory cells were skewed towards the CD80+/PD-L2+ double-positive subset, which may make secondary antibody responses more efficient. The elevated primary antibody responses seen with poly(I:C)/Tx are striking and likely driven by generation of plasma cells, although we were unable to directly access this due to the rarity of plasma cell events at the timepoints analyzed. The elevated total alloantibody responses due to poly(I:C)/Tx were associated with decreased IgG1 and increased TH1-biased IgG subclasses IgG2a and IgG3 relative to transfusion alone. The IgG1 isotype is thought to be inhibitory to IgG3 aggregation which suggests inflammatory IgG3 responses were less regulated (66). This is consistent with how Poly(I:C) is known to induce viral-like responses and skewing immunity towards TH1 responses (67, 68). How poly(I:C) modulates platelet alloimmunization is unclear. Perhaps poly(I:C) engages toll-like receptor (TLR)3 which activates innate immunity that leads to anti-viral responses, which may promote increased TH1-biased alloantibody responses (67, 69). Human plasma cells express TLR3 and increase production of IgM and IgG upon TLR stimulation (70). Poly(I:C) also induces immunity through TLR3 independent pathways. In RBC alloimmunization, poly(I:C) enhances alloimmunization via MAVS pathway rather than agonizing TLR3 (71).
Unlike the heightened alloantibody response due to poly(I:C), LPS had a striking and opposing effect. LPS is known to induce TH1-like cytokine responses and favors IgG3 class switch (72, 73). However, prior exposure to LPS, a TLR4 agonist, dampened all alloantibody isotype responses, specifically IgM and IgG1, and all associated allo-B cell responses examined, which suggests LPS abrogates B cell activation in our model. In fact, of the isotypes, IgM expression was the greatest in magnitude for LPS/Tx. While counterintuitive given the established role of LPS in inflammation and bystander activation (32, 33), it is consistent with clinical reports of transient immune paralysis or “LPS tolerance” due to sepsis-induced inflammation (74–77).
As expected, LPS administration alone was sufficient to cause non-specific induction of immature/transitional (data not shown), MZ, and memory allo-B cells (78–80). We did observe an expansion of IgD- allo-B cells with LPS alone, which are likely IgDlow transitional B cells. They are unlikely activated B cells given their lack of CD86 or germinal center markers.
While trends of anti-MHC antibody responses were consistent between class I and class II, the magnitude of total anti-MHC antibody responses were ~20x greater than untreated for class II whereas they were ~10x greater for class I, although direct comparisons cannot be made. Allo-B cell responses against MHC class II were generally more robust and greater in magnitude than those against MHC class I. As MHC class I is expressed on the surface of both platelets and WBCs, whereas MHC class II is found only on a subset of WBCs, the density of exposure to MHC class I antigens from a platelet product was presumably greater (5). Despite the lower exposure, MHC class II antigens were comparatively more immunogenic than class I antigens in responses in the spleen and lymph node. One possible explanation is that the MHC class II alloantigens are directly presented to CD4+ T cells, leading to more effective T cell help for the B cell response. Minimizing these potent MHC class II responses may explain mechanistically one of the benefits of the use of leukoreduction for platelet transfusion.
Responses in the spleen mirrored those in the lymph node although surprisingly, many responses were more robust in the lymph node. This was in stark contrast to the RBC model, where no RBC consumption by macrophages or dendritic cells were observed in the lymph node and where the spleen was shown to be an essential site for alloimmunization (24, 61, 65, 81, 82). While this apparent conflict could be due to differences in platelet and RBC uptake, a study using leukoreduced platelets suggested allogeneic-specific CD4+ T cell reactions occur in the spleen and not the lymph node (83). Taken together, this suggests that the donor lymphocytes may play an important role in platelet alloimmunization, either through a different cellular source of antigen or through the addition of MHC class II antigens. Additionally, our MHC tetramer staining strategy may have provided greater sensitivity to observe rare endogenous allo-B cell events that may not have been previously appreciated. Alternatively, alloimmunity may initiate in the spleen and subsequently seed downstream responses in the lymph node.
While our study has demonstrated that different inflammatory signals can alter the alloresponse, some limitations should be considered. While our murine models enabled a controlled approach, confirmation will be needed clinically to determine if similar responses are observed with human transfusions. We also utilized non-leukoreduced platelets to examine the cellular allo-B cell responses in our study as they generate more potent immune responses. Although a similar effect of inflammatory signals on alloantibodies was observed between anti-MHC class I and class II responses with non-leukoreduced platelets and anti-MHC class I responses with leukoreduced platelets, it is possible that the lower antigen dose environment or other platelet-specific attributes of leukoreduced platelets could alter allo-B cell responses. Finally, while LPS and poly(I:C) deliver some of the critical innate immune signals seen in gram-negative bacterial infection and viral infection, respectively, an active infection would introduce additional complexity and may further alter alloimmunization outcomes.
In conclusion, these data demonstrate that not all inflammatory environments enhance bystander responses. Prior exposure to LPS on gram-negative bacteria may, in fact, curtail platelet alloimmunization. Together, these observations may inform clinical practice to better manage risk for platelet alloimmunization.
The raw data supporting the conclusions of this article will be made available by the authors, without undue reservation.
The animal study was approved by Labcorp Early Developmental Laboratories Inc. The study was conducted in accordance with the local legislation and institutional requirements.
JT: Data curation, Formal analysis, Investigation, Methodology, Project administration, Resources, Software, Supervision, Validation, Visualization, Writing – original draft, Writing – review & editing. MM: Data curation, Writing – review & editing, Investigation, Methodology, Project administration, Resources. BG: Data curation, Investigation, Methodology, Project administration, Writing – review & editing. OD: Data curation, Investigation, Methodology, Project administration, Writing – review & editing. MT: Formal analysis, Supervision, Validation, Writing – review & editing. RJ: Conceptualization, Data curation, Funding acquisition, Investigation, Methodology, Project administration, Resources, Software, Supervision, Validation, Visualization, Writing – review & editing.
The author(s) declare financial support was received for the research, authorship, and/or publication of this article. This study was supported by research funding from NIH, NHLBI (R01 HL144501) to RJ.
MHC:peptide tetramers were provided by the NIH tetramer core facility. Some figure images were generated on BioRender.
The authors declare that the research was conducted in the absence of any commercial or financial relationships that could be construed as a potential conflict of interest.
The author(s) declared that they were an editorial board member of Frontiers, at the time of submission. This had no impact on the peer review process and the final decision.
All claims expressed in this article are solely those of the authors and do not necessarily represent those of their affiliated organizations, or those of the publisher, the editors and the reviewers. Any product that may be evaluated in this article, or claim that may be made by its manufacturer, is not guaranteed or endorsed by the publisher.
1. Alves R, Grimalt R. A review of platelet-rich plasma: history, biology, mechanism of action, and classification. Skin Appendage Disord (2018) 4(1):18–24. doi: 10.1159/000477353
2. Jain A, Bedi RK, Mittal K. Platelet-rich plasma therapy: A novel application in regenerative medicine. Asian J Transfus Sci (2015) 9(2):113–4. doi: 10.4103/0973-6247.162679
3. Wang J, Zhou P, Han Y, Zhang H. Platelet transfusion for cancer secondary thrombocytopenia: Platelet and cancer cell interaction. Transl Oncol (2021) 14(4):101022. doi: 10.1016/j.tranon.2021.101022
4. Alwan F, Vendramin C, Liesner R, Clark A, Lester W, Dutt T, et al. Characterization and treatment of congenital thrombotic thrombocytopenic purpura. Blood (2019) 133(15):1644–51. doi: 10.1182/blood-2018-11-884700
5. Tran JQ, Muench MO, Heitman JW, Jackman RP. Allogeneic major histocompatibility complex antigens are necessary and sufficient for partial tolerance induced by transfusion of pathogen reduced platelets in mice. Vox Sang (2019) 114:207–15. doi: 10.1111/vox.12756
6. Howard JE, Perkins HA. The natural history of alloimmunization to platelets. Transfusion (1978) 18(4):496–503. doi: 10.1046/j.1537-2995.1978.18478251250.x
7. Itescu S, Tung TC, Burke EM, Weinberg A, Moazami N, Artrip JH, et al. Preformed IgG antibodies against major histocompatibility complex class II antigens are major risk factors for high-grade cellular rejection in recipients of heart transplantation. Circulation (1998) 98(8):786–93. doi: 10.1161/01.CIR.98.8.786
8. Massad MG, Cook DJ, Schmitt SK, Smedira NG, McCarthy JF, Vargo RL, et al. Factors influencing HLA sensitization in implantable LVAD recipients. Ann Thorac Surg (1997) 64(4):1120–5. doi: 10.1016/S0003-4975(97)00807-2
9. Moazami N, Itescu S, Williams MR, Argenziano M, Weinberg A, Oz MC. Platelet transfusions are associated with the development of anti-major histocompatibility complex class I antibodies in patients with left ventricular assist support. J Heart Lung Transplant (1998) 17(9):876–80.
10. Slichter SJ, Davis K, Enright H, Braine H, Gernsheimer T, Kao KJ, et al. Factors affecting posttransfusion platelet increments, platelet refractoriness, and platelet transfusion intervals in thrombocytopenic patients. Blood (2005) 105(10):4106–14. doi: 10.1182/blood-2003-08-2724
11. Tsau PH, Arabia FA, Toporoff B, Paramesh V, Sethi GK, Copeland JG. Positive panel reactive antibody titers in patients bridged to transplantation with a mechanical assist device: risk factors and treatment. ASAIO J (1998) 44(5):M634–7. doi: 10.1097/00002480-199809000-00067
12. Andreu G, Dewailly J, Leberre C, Quarre MC, Bidet ML, Tardivel R, et al. Prevention of HLA immunization with leukocyte-poor packed red cells and platelet concentrates obtained by filtration. Blood (1988) 72(3):964–9. doi: 10.1182/blood.V72.3.964.bloodjournal723964
13. Fisher M, Chapman JR, Ting A, Morris PJ. Alloimmunisation to HLA antigens following transfusion with leucocyte-poor and purified platelet suspensions. Vox Sang (1985) 49(5):331–5. doi: 10.1111/j.1423-0410.1985.tb00807.x
14. Murphy MF, Metcalfe P, Thomas H, Eve J, Ord J, Lister TA, et al. Use of leucocyte-poor blood components and HLA-matched-platelet donors to prevent HLA alloimmunization. Br J Haematol (1986) 62(3):529–34. doi: 10.1111/j.1365-2141.1986.tb02965.x
15. Schiffer CA, Dutcher JP, Aisner J, Hogge D, Wiernik PH, Reilly JP. A randomized trial of leukocyte-depleted platelet transfusion to modify alloimmunization in patients with leukemia. Blood (1983) 62(4):815–20. doi: 10.1182/blood.V62.4.815.815
16. van Marwijk Kooy M, van Prooijen HC, Moes M, Bosma-Stants I, Akkerman JW. Use of leukocyte-depleted platelet concentrates for the prevention of refractoriness and primary HLA alloimmunization: a prospective, randomized trial. Blood (1991) 77(1):201–5. doi: 10.1182/blood.V77.1.201.201
17. Jackman RP, Deng X, Bolgiano D, Utter GH, Schechterly C, Lebedeva M, et al. Leukoreduction and ultraviolet treatment reduce both the magnitude and the duration of the HLA antibody response. Transfusion (2014) 54(3):672–80. doi: 10.1111/trf.12317
18. Sintnicolaas K, van Marwijk Kooij M, van Prooijen HC, van Dijk BA, van Putten WL, Claas FH, et al. Leukocyte depletion of random single-donor platelet transfusions does not prevent secondary human leukocyte antigen-alloimmunization and refractoriness: a randomized prospective study. Blood (1995) 85(3):824–8. doi: 10.1182/blood.V85.3.824.bloodjournal853824
19. Trial to Reduce Alloimmunization to Platelets Study Group. Leukocyte reduction and ultraviolet B irradiation of platelets to prevent alloimmunization and refractoriness to platelet transfusions. N Engl J Med (1997) 337(26):1861–9. doi: 10.1056/NEJM199712253372601
20. Seftel MD, Growe GH, Petraszko T, Benny WB, Le A, Lee CY, et al. Universal prestorage leukoreduction in Canada decreases platelet alloimmunization and refractoriness. Blood (2004) 103(1):333–9. doi: 10.1182/blood-2003-03-0940
21. Detection of platelet-reactive antibodies in patients who are refractory to platelet transfusions, and the selection of compatible donors. Vox Sang (2003) 84(1):73–88. doi: 10.1046/j.1423-0410.2003.00259.x
22. Saris A, Kerkhoffs JL, Norris PJ, van Ham SM, Ten Brinke A, Brand A, et al. The role of pathogen-reduced platelet transfusions on HLA alloimmunization in hemato-oncological patients. Transfusion (2018) 59:470–81. doi: 10.1111/trf.15056
23. Hendrickson JE, Desmarets M, Deshpande SS, Chadwick TE, Hillyer CD, Roback JD, et al. Recipient inflammation affects the frequency and magnitude of immunization to transfused red blood cells. Transfusion (2006) 46(9):1526–36. doi: 10.1111/j.1537-2995.2006.00946.x
24. Hendrickson JE, Chadwick TE, Roback JD, Hillyer CD, Zimring JC. Inflammation enhances consumption and presentation of transfused RBC antigens by dendritic cells. Blood (2007) 110(7):2736–43. doi: 10.1182/blood-2007-03-083105
25. Yu J, Heck S, Yazdanbakhsh K. Prevention of red cell alloimmunization by CD25 regulatory T cells in mouse models. Am J Hematol (2007) 82(8):691–6. doi: 10.1002/ajh.20959
26. Hendrickson JE, Roback JD, Hillyer CD, Easley KA, Zimring JC. Discrete Toll-like receptor agonists have differential effects on alloimmunization to transfused red blood cells. Transfusion (2008) 48(9):1869–77. doi: 10.1111/j.1537-2995.2008.01801.x
27. Evers D, van der Bom JG, Tijmensen J, Middelburg RA, de Haas M, Zalpuri S, et al. Red cell alloimmunisation in patients with different types of infections. Br J Haematol (2016) 175(5):956–66. doi: 10.1111/bjh.14307
28. Roubinian NH, Escobar GJ, Liu V, Swain BE, Gardner MN, Kipnis P, et al. Trends in red blood cell transfusion and 30-day mortality among hospitalized patients. Transfusion (2014) 54(10 Pt 2):2678–86. doi: 10.1111/trf.12825
29. Karafin MS, Bruhn R, Westlake M, Sullivan MT, Bialkowski W, Edgren G, et al. Demographic and epidemiologic characterization of transfusion recipients from four US regions: evidence from the REDS-III recipient database. Transfusion (2017) 57(12):2903–13. doi: 10.1111/trf.14370
30. Tang D, Kang R, Coyne CB, Zeh HJ, Lotze MT. PAMPs and DAMPs: signal 0s that spur autophagy and immunity. Immunol Rev (2012) 249(1):158–75. doi: 10.1111/j.1600-065X.2012.01146.x
31. Iwasaki A, Medzhitov R. Regulation of adaptive immunity by the innate immune system. Science (2010) 327(5963):291–5. doi: 10.1126/science.1183021
32. Kim TS, Shin EC. The activation of bystander CD8(+) T cells and their roles in viral infection. Exp Mol Med (2019) 51(12):1–9. doi: 10.1038/s12276-019-0316-1
33. Whiteside SK, Snook JP, Williams MA, Weis JJ. Bystander T cells: A balancing act of friends and foes. Trends Immunol (2018) 39(12):1021–35. doi: 10.1016/j.it.2018.10.003
34. Tough DF, Sun S, Sprent J. T cell stimulation in vivo by lipopolysaccharide (LPS). J Exp Med (1997) 185(12):2089–94. doi: 10.1084/jem.185.12.2089
35. Boyman O. Bystander activation of CD4+ T cells. Eur J Immunol (2010) 40(4):936–9. doi: 10.1002/eji.201040466
36. Blessinger SA, Tran JQ, Jackman RP, Gilfanova R, Rittenhouse J, Gutierrez AG, et al. Immunodeficient mice are better for modeling the transfusion of human blood components than wild-type mice. PloS One (2020) 15(7):e0237106. doi: 10.1371/journal.pone.0237106
37. Jackman RP, Heitman JW, Marschner S, Goodrich RP, Norris PJ. Understanding loss of donor white blood cell immunogenicity after pathogen reduction: mechanisms of action in ultraviolet illumination and riboflavin treatment. Transfusion (2009) 49(12):2686–99. doi: 10.1111/j.1537-2995.2009.02333.x
38. Jackman RP, Muench MO, Heitman JW, Inglis HC, Law JP, Marschner S, et al. Immune modulation and lack of alloimmunization following transfusion with pathogen-reduced platelets in mice. Transfusion (2013) 53(11):2697–709. doi: 10.1111/trf.12133
39. Muench MO, Heitman JW, Inglis H, Fomin ME, Marschner S, Goodrich RP, et al. Reduced alloimmunization in mice following repeated transfusion with pathogen-reduced platelets. Transfusion (2016) 56(6):1419–29. doi: 10.1111/trf.13579
40. Tran JQ, Muench MO, Heitman JW, Jackman RP. Pathogen reduction with riboflavin and ultraviolet light induces a quasi-apoptotic state in blood leukocytes. Transfusion (2019) 59(11):3501–10. doi: 10.1111/trf.15516
41. Tran JQ, Muench MO, Jackman RP. Pathogen-reduced PRP blocks T-cell activation, induces Treg cells, and promotes TGF-beta expression by cDCs and monocytes in mice. Blood Adv (2020) 4(21):5547–61. doi: 10.1182/bloodadvances.2020002867
42. Larijani M, Chen S, Cunningham LA, Volpe JM, Cowell LG, Lewis SM, et al. The recombination difference between mouse kappa and lambda segments is mediated by a pair-wise regulation mechanism. Mol Immunol (2006) 43(7):870–81. doi: 10.1016/j.molimm.2005.06.038
43. Medina CA, Teale JM. Restricted kappa chain expression in early ontogeny: biased utilization of V kappa exons and preferential V kappa-J kappa recombinations. J Exp Med (1993) 177(5):1317–30. doi: 10.1084/jem.177.5.1317
44. Kalled SL, Brodeur PH. Preferential rearrangement of V kappa 4 gene segments in pre-B cell lines. J Exp Med (1990) 172(2):559–66. doi: 10.1084/jem.172.2.559
45. Kalled SL, Brodeur PH. Utilization of V kappa families and V kappa exons. Implications for the available B cell repertoire. J Immunol (1991) 147(9):3194–200.
46. Ramsden DA, Baetz K, Wu GE. Conservation of sequence in recombination signal sequence spacers. Nucleic Acids Res (1994) 22(10):1785–96. doi: 10.1093/nar/22.10.1785
47. Ramsden DA, Paige CJ, Wu GE. Kappa light chain rearrangement in mouse fetal liver. J Immunol (1994) 153(3):1150–60. doi: 10.4049/jimmunol.153.3.1150
48. Chen J, Wang Q, Yin D, Vu V, Sciammas R, Chong AS. Cutting edge: CTLA-4Ig inhibits memory B cell responses and promotes allograft survival in sensitized recipients. J Immunol (2015) 195(9):4069–73. doi: 10.4049/jimmunol.1500940
49. Chen J, Yin H, Xu J, Wang Q, Edelblum KL, Sciammas R, et al. Reversing endogenous alloreactive B cell GC responses with anti-CD154 or CTLA-4Ig. Am J Transplant. (2013) 13(9):2280–92. doi: 10.1111/ajt.12350
50. Khiew SH, Jain D, Chen J, Yang J, Yin D, Young JS, et al. Transplantation tolerance modifies donor-specific B cell fate to suppress de novo alloreactive B cells. J Clin Invest. (2020) 130(7):3453–66. doi: 10.1172/JCI132814
51. Yang J, Chen J, Young JS, Wang Q, Yin D, Sciammas R, et al. Tracing donor-MHC class II reactive B cells in mouse cardiac transplantation: delayed CTLA4-ig treatment prevents memory alloreactive B-cell generation. Transplantation (2016) 100(8):1683–91. doi: 10.1097/TP.0000000000001253
52. Young JS, McIntosh C, Alegre ML, Chong AS. Evolving approaches in the identification of allograft-reactive T and B cells in mice and humans. Transplantation (2017) 101(11):2671–81. doi: 10.1097/TP.0000000000001847
53. Oliver AM, Martin F, Gartland GL, Carter RH, Kearney JF. Marginal zone B cells exhibit unique activation, proliferative and immunoglobulin secretory responses. Eur J Immunol (1997) 27(9):2366–74. doi: 10.1002/eji.1830270935
54. Lopes-Carvalho T, Kearney JF. Development and selection of marginal zone B cells. Immunol Rev (2004) 197:192–205. doi: 10.1111/j.0105-2896.2004.0112.x
55. Groeneveld PH, Erich T, Kraal G. In vivo effects of LPS on B lymphocyte subpopulations. Migration of marginal zone-lymphocytes and IgD-blast formation in the mouse spleen. Immunobiology (1985) 170(5):402–11. doi: 10.1016/S0171-2985(85)80064-4
56. Tomayko MM, Steinel NC, Anderson SM, Shlomchik MJ. Cutting edge: Hierarchy of maturity of murine memory B cell subsets. J Immunol (2010) 185(12):7146–50. doi: 10.4049/jimmunol.1002163
57. Zuccarino-Catania GV, Sadanand S, Weisel FJ, Tomayko MM, Meng H, Kleinstein SH, et al. CD80 and PD-L2 define functionally distinct memory B cell subsets that are independent of antibody isotype. Nat Immunol (2014) 15(7):631–7. doi: 10.1038/ni.2914
58. Kwun J, Oh BC, Gibby AC, Ruhil R, Lu VT, Kim DW, et al. Patterns of de novo allo B cells and antibody formation in chronic cardiac allograft rejection after alemtuzumab treatment. Am J Transplant (2012) 12(10):2641–51. doi: 10.1111/j.1600-6143.2012.04181.x
59. Song S, Manook M, Kwun J, Jackson AM, Knechtle SJ, Kelsoe G. Allo-specific humoral responses: new methods for screening donor-specific antibody and characterization of HLA-specific memory B cells. Front Immunol (2021) 12:705140. doi: 10.3389/fimmu.2021.705140
60. Couvidou A, Angenieux C, Ruch L, Mangin PH, Gachet C, Maitre B. Marginal zone B cells are responsible for the production of alloantibodies following platelet transfusion in mice. Blood Adv (2022) 7:1356–65. doi: 10.1182/bloodadvances.2022008411
61. Patel SR, Gibb DR, Girard-Pierce K, Zhou X, Rodrigues LC, Arthur CM, et al. Marginal zone B cells induce alloantibody formation following RBC transfusion. Front Immunol (2018) 9:2516. doi: 10.3389/fimmu.2018.02516
62. Zerra PE, Patel SR, Jajosky RP, Arthur CM, McCoy JW, Allen JWL, et al. Marginal zone B cells mediate a CD4 T-cell-dependent extrafollicular antibody response following RBC transfusion in mice. Blood (2021) 138(8):706–21. doi: 10.1182/blood.2020009376
63. Escamilla-Rivera V, Liu J, Gibb DR, Santhanakrishnan M, Liu D, Forsmo JE, et al. Poly(I:C) causes failure of immunoprophylaxis to red blood cells expressing the KEL glycoprotein in mice. Blood (2020) 135(22):1983–93. doi: 10.1182/blood.2020005018
64. Elayeb R, Tamagne M, Bierling P, Noizat-Pirenne F, Vingert B. Red blood cell alloimmunization is influenced by the delay between Toll-like receptor agonist injection and transfusion. Haematologica (2016) 101(2):209–18. doi: 10.3324/haematol.2015.134171
65. Calabro S, Gallman A, Gowthaman U, Liu D, Chen P, Liu J, et al. Bridging channel dendritic cells induce immunity to transfused red blood cells. J Exp Med (2016) 213(6):887–96. doi: 10.1084/jem.20151720
66. Collins AM. IgG subclass co-expression brings harmony to the quartet model of murine IgG function. Immunol Cell Biol (2016) 94(10):949–54. doi: 10.1038/icb.2016.65
67. Jacobs BL, Langland JO. When two strands are better than one: the mediators and modulators of the cellular responses to double-stranded RNA. Virology (1996) 219(2):339–49. doi: 10.1006/viro.1996.0259
68. Visciano ML, Tagliamonte M, Tornesello ML, Buonaguro FM, Buonaguro L. Effects of adjuvants on IgG subclasses elicited by virus-like particles. J Transl Med (2012) 10:4. doi: 10.1186/1479-5876-10-4
69. Takeda K, Akira S. Toll-like receptors. Curr Protoc Immunol (2015) 109:14 2 1– 2 0. doi: 10.1002/0471142735.im1412s109
70. Dorner M, Brandt S, Tinguely M, Zucol F, Bourquin JP, Zauner L, et al. Plasma cell toll-like receptor (TLR) expression differs from that of B cells, and plasma cell TLR triggering enhances immunoglobulin production. Immunology (2009) 128(4):573–9. doi: 10.1111/j.1365-2567.2009.03143.x
71. Jash A, Usaneerungrueng C, Howie HL, Qiu A, Luckey CJ, Zimring JC, et al. Antibodies to low-copy number RBC alloantigen convert a tolerogenic stimulus to an immunogenic stimulus in mice. Front Immunol (2021) 12:629608. doi: 10.3389/fimmu.2021.629608
72. Mukherjee S, Chen LY, Papadimos TJ, Huang S, Zuraw BL, Pan ZK. Lipopolysaccharide-driven Th2 cytokine production in macrophages is regulated by both MyD88 and TRAM. J Biol Chem (2009) 284(43):29391–8. doi: 10.1074/jbc.M109.005272
73. Deenick EK, Hasbold J, Hodgkin PD. Switching to IgG3, IgG2b, and IgA is division linked and independent, revealing a stochastic framework for describing differentiation. J Immunol (1999) 163(9):4707–14. doi: 10.4049/jimmunol.163.9.4707
74. Ertel W, Keel M, Neidhardt R, Steckholzer U, Kremer JP, Ungethuem U, et al. Inhibition of the defense system stimulating interleukin-12 interferon-gamma pathway during critical Illness. Blood (1997) 89(5):1612–20. doi: 10.1182/blood.V89.5.1612
75. Weijer S, Lauw FN, Branger J, van den Blink B, van der Poll T. Diminished interferon-gamma production and responsiveness after endotoxin administration to healthy humans. J Infect Dis (2002) 186(12):1748–53. doi: 10.1086/345675
76. Lauw FN, ten Hove T, Dekkers PE, de Jonge E, van Deventer SJ, van der Poll T. Reduced Th1, but not Th2, cytokine production by lymphocytes after in vivo exposure of healthy subjects to endotoxin. Infect Immun (2000) 68(3):1014–8. doi: 10.1128/IAI.68.3.1014-1018.2000
77. Gould MP, Greene JA, Bhoj V, DeVecchio JL, Heinzel FP. Distinct modulatory effects of LPS and CpG on IL-18-dependent IFN-gamma synthesis. J Immunol (2004) 172(3):1754–62. doi: 10.4049/jimmunol.172.3.1754
78. LeBien TW, Tedder TF. B lymphocytes: how they develop and function. Blood (2008) 112(5):1570–80. doi: 10.1182/blood-2008-02-078071
79. Meyer-Bahlburg A, Andrews SF, Yu KO, Porcelli SA, Rawlings DJ. Characterization of a late transitional B cell population highly sensitive to BAFF-mediated homeostatic proliferation. J Exp Med (2008) 205(1):155–68. doi: 10.1084/jem.20071088
80. Zhang J, Liu YJ, MacLennan IC, Gray D, Lane PJ. B cell memory to thymus-independent antigens type 1 and type 2: the role of lipopolysaccharide in B memory induction. Eur J Immunol (1988) 18(9):1417–24. doi: 10.1002/eji.1830180918
81. Hendrickson JE, Saakadze N, Cadwell CM, Upton JW, Mocarski ES, Hillyer CD, et al. The spleen plays a central role in primary humoral alloimmunization to transfused mHEL red blood cells. Transfusion (2009) 49(8):1678–84. doi: 10.1111/j.1537-2995.2009.02200.x
82. Hendrickson JE, Eisenbarth SC, Tormey CA. Red blood cell alloimmunization: new findings at the bench and new recommendations for the bedside. Curr Opin Hematol (2016) 23(6):543–9. doi: 10.1097/MOH.0000000000000277
Keywords: platelets, alloimmunization, underlying health, inflammation, poly(I:C), lipopolysaccharide, alloantibodies
Citation: Tran JQ, Muench MO, Gaillard B, Darst O, Tomayko MM and Jackman RP (2023) Polyinosinic: polycytidylic acid induced inflammation enhances while lipopolysaccharide diminishes alloimmunity to platelet transfusion in mice. Front. Immunol. 14:1281130. doi: 10.3389/fimmu.2023.1281130
Received: 21 August 2023; Accepted: 20 November 2023;
Published: 11 December 2023.
Edited by:
Jeffrey J. Pu, Harvard Medical School, United StatesReviewed by:
James C. Zimring, University of Virginia, United StatesCopyright © 2023 Tran, Muench, Gaillard, Darst, Tomayko and Jackman. This is an open-access article distributed under the terms of the Creative Commons Attribution License (CC BY). The use, distribution or reproduction in other forums is permitted, provided the original author(s) and the copyright owner(s) are credited and that the original publication in this journal is cited, in accordance with accepted academic practice. No use, distribution or reproduction is permitted which does not comply with these terms.
*Correspondence: Rachael P. Jackman, cmphY2ttYW5Adml0YWxhbnQub3Jn
Disclaimer: All claims expressed in this article are solely those of the authors and do not necessarily represent those of their affiliated organizations, or those of the publisher, the editors and the reviewers. Any product that may be evaluated in this article or claim that may be made by its manufacturer is not guaranteed or endorsed by the publisher.
Research integrity at Frontiers
Learn more about the work of our research integrity team to safeguard the quality of each article we publish.