- 1Translation Sciences Laboratory, Research Department, Infectious Diseases Institute, Makerere University, Kampala, Uganda
- 2Department of Medical Microbiology, School of Biomedical Sciences, College of Health Sciences, Makerere University, Kampala, Uganda
- 3Division of Infectious Diseases and International Medicine, Department of Medicine, University of Minnesota, Minneapolis, MN, United States
- 4Department of Immunology and Molecular Biology, School of Biomedical Sciences, College of Health Sciences, Makerere University, Kampala, Uganda
- 5Division of Infectious Diseases, Department of Medicine, John Hopkins University School of Medicine, Baltimore, MD, United States
- 6Department of Medicine, School of Medicine, College of Health Sciences, Makerere University, Kampala, Uganda
- 7Mucosal and Vaccine Research Program Colorado, Department of Medicine, Division of Infectious Diseases, University of Colorado Denver, Aurora, CO, United States
- 8Department of Medicine and Infectious Disease, Denver Veterans Affairs Medical Center, Denver, CO, United States
Introduction: Survival among people with HIV-associated cryptococcal meningitis (CM) remains low, particularly among women, despite the currently optimal use of antifungal drugs. Cryptococcus dissemination into the central nervous system [brain, spinal cord, and cerebrospinal fluid (CSF)] elicits the local production of cytokines, chemokines, and other biomarkers. However, no consistent diagnostic or prognostic neuroimmune signature is reported to underpin the risk of death or to identify mechanisms to improve treatment and survival. We hypothesized that distinct neuroimmune signatures in the CSF would distinguish survivors from people who died on antifungal treatment and who may benefit from tailored therapy.
Methods: We considered baseline clinical features, CSF cryptococcal fungal burden, and CSF neuroimmune signatures with survival at 18 weeks among 419 consenting adults by “gender” (168 women and 251 men by biological sex defined at birth).
Results: Survival at 18 weeks was significantly lower among women than among men {47% vs. 59%, respectively; hazard ratio (HR) = 1.4 [95% confidence interval (CI), 1.0 to 1.9; p = 0.023]}. Unsupervised principal component analysis (PCA) demonstrated divergent neuroimmune signatures by gender, survival, and intragender-specific survival. Overall, women had lower levels of programmed death ligand 1, Interleukin (IL) (IL-11RA/IL-1F30, and IL-15 (IL-15) than men (all p < 0.028). Female survivors compared with those who died expressed significant elevations in levels of CCL11 and CXCL10 chemokines (both p = 0.001), as well as increased T helper 1, regulatory, and T helper 17 cytokines (all p < 0.041). In contrast, male survivors expressed lower levels of IL-15 and IL-8 compared with men who died (p < 0.044).
Conclusions: Survivors of both genders demonstrated a significant increase in the levels of immune regulatory IL-10. In conclusion, the lower survival among women with CM was accompanied by distinct differential gender-specific neuroimmune signatures. These female and male intragender-specific survival–associated neuroimmune signatures provide potential targets for interventions to advance therapy to improve the low survival among people with HIV-associated CM.
1 Introduction
Co-infection with the fungus Cryptococcus neoformans remains an important contributor to death among people with advanced HIV/AIDS worldwide, despite the use of antifungal medications (1, 2). Mortality with HIV-associated cryptococcal meningitis (CM) varies by location based on existing HIV prevalence. In Europe and North America, with the low prevalence of people with HIV, both the incidence and mortality rates of CM are lowest (3). In contrast, in low- and middle-income countries, especially in Africa, the prevalence, incidence, and mortality with both HIV and CM are high (3–5). In Uganda, Botswana, and South Africa, the 10-week mortality rates approach 50% (3, 5–8) even in closely monitored research settings, with deaths occurring within days to weeks and, sometimes, up to months after diagnosis (2, 9, 10). These observations emphasize the importance of characterizing early immune response as a possible intervention to control fungal infection in improving survival (10).
The damage response paradigm of Casadevall and Pirofski highlights the counterbalancing contributions of pathogen vs. host response in shaping tissue injury and disease outcome (11, 12). Pathologic outcomes may result both from unrestricted pathogen growth with limited immune control or with control of the pathogen but with an exuberant immune response. This paradigm suggests that improved outcomes are achievable in the presence of therapy combining an effective pathogen-specific target drug (e.g., antifungals) with immune-based treatment to modulate immune homeostasis.
Cryptococcal dissemination into the central nervous system (CNS) tissues and cerebrospinal fluid (CSF) across the blood-brain barrier (13–15) leads to activation of resident neuroimmune cells (astrocytes, microglial cells, local macrophages, dendritic cells, and lymphocytes) and of CNS infiltrating T and B cells (16, 17). These cells produce chemoattractant proteins [e.g., CCL11/Eotaxin, CXCL10/Interferon-inducible protein 10 (IP-10) (18, 19)] and other inflammatory mediators (e.g., IL-15 and IL-8/CXCL8) (20) to induce neuroimmune activation, inflammation, and meningoencephalitis. These mediators influence fungal clearance, ensuing immunopathological processes, clinical phenotype, and outcome (21–25).
Among studies reporting cases of CM by gender (biological sex assigned at birth), the majority of CM was diagnosed among men (2, 7, 9, 16, 26–44); only four, to date, described survival by gender. Two studies done prior to optimized antiretroviral therapy (ART) initiation (delayed for 2 weeks after CM diagnosis based on the COAT Trial (9)) showed no differences in survival by gender (26, 44). In contrast, two studies done after initiation of optimized ART with antifungal therapy showed lower survival among women (7, 42). To date, no clear immunopathogenic mechanisms have been proposed to explain the divergence in survival by gender despite access to current optimal antifungal treatment and ART.
In prior studies of CM, men showed increased expression of innate chemokines and cytokines in the CSF associated with increased trafficking of innate lymphoid and myeloid cells compared with women (7). Of note, no differences were noted in soluble immune factors by survival with CM overall (7). However, these differences were not evaluated in relation to gender-specific survival. Indeed, gender-specific differences have been reported in immune responses to vaccines in both humans and animals (45, 46), with elevations in cytokine production, endocrine, and metabolic parameters in women compared with that in men. Nevertheless, in CM, the cryptococcal fungal burden, white cell counts, and protein in CSF and CD4+ T-cell numbers in blood were similar by gender (7). Moreover, the macrophage-mediated host immune evasion mechanism vomocytosis and cryptococcal intracellular proliferation in infected macrophages were similar by gender (47).
We evaluated differences in soluble cytokine, chemokine, and immunoregulatory responses to CM in CSF between women and men overall, by survival overall, and, in particular, the differences in these neuroimmune responses in relation to survival by gender. At the time of diagnosis, we determined levels of representative T helper 1 (Th1), Th2, Th17, T follicular helper (Tfh) cytokines, innate myeloid–regulating cytokines [interleukin-8 (IL-8), IL-13, and IL-15], and immune checkpoint markers [programmed death ligand 1 (PD-L1)] among people who survived or died during the 18-week follow-up. We identified discrete differences in patterns of neuroimmune mediators by gender and by intragender-specific survival at the site of infection in the CSF.
2 Materials and methods
2.1 Scope: participants, sites, and setting
We enrolled 419 participants from a retrospective cohort of 460 consenting adults with CM who were enrolled to receive meningitis treatment in the prior Adjunctive Sertraline for the Treatment of HIV-Associated Cryptococcal Meningitis trial (ClinicalTrials.gov: NCT01802385) and had their specimens and data in storage. The parent trial was conducted between 2015 and 2017 at the Infectious Diseases Institute (Administrative site) and at Mulago and Kiruddu National Referral Hospitals (patient catchment sites) in Kampala, Uganda (2, 48). The participants were selected on the basis of gender, available survival-specific data, and CSF specimens and clinical data in storage for analysis. All analyses were performed at baseline (cross-sectional analyses) with reference to the 18-week survival after CM diagnosis and study enrollment. The complete demographic, clinical, and CSF datasets were available from majority of participants (94%; 168 women and 251 men).
In the parent study, participants or their surrogates gave informed written and signed consent for their study participants under ethically approved study protocols. Enrolled were participants ≥18 years of age, with confirmed diagnosis of HIV-associated CM diagnosed as previously described (49, 50). Only participants whose survival status was known at study censoring and at study termination at 18 weeks of follow-up (90.7%; 380 of 419) were included in the survival sensitivity analyses.
2.2 Specimen preparation
CSF was drawn from lumbar punctures at diagnosis of CM prior to antifungal therapy initiation. The CSF specimens were spun to pellet out cells. The CSF supernatants were stored in a −80°C freezer prior to thawing for testing using Luminex.
2.3 Luminex cytokine and chemokine immunophenotyping
A representative sample of cytokines, chemokines, and checkpoint regulators was measured in CSF diluted in a 1:2 ratio based on the R&D Human XL Cytokines Discovery Premixed Kit platform as per the manufacturer’s recommendations (R&D, Minneapolis, MN). The Th1 cytokines were tumor necrosis factor–alpha (TNF-α), interferon-gamma (IFN-γ), interleukin-2 (IL-2), IL-12p70, with soluble CD40-Ligand/TNFSF5. The Th2 cytokines were IL-4 and IL-13. Tfh cytokines were IL-6 and IL-10. The Th17 cytokine included was IL-17A. Cytokines derived from innate lymphoid and myeloid cells were IL-15, IL-8, and IL-1 RA/IL-1 F3. The inflammatory-mediating chemokines primarily derived from microglial and astrocytes mediating neuroinflammation in CM were CXCL10 lymphoid cells mediating chemoattraction (51, 52) and CCL11 myeloid cells (Eosinophils) mediating chemoattraction (53, 54) and IL-8 neutrophils mediating activation and chemoattraction (55). The immune checkpoint molecules were PD-L1/B7-H1 (56) and immune regulatory cytokine IL-10 (57).
2.4 Statistical analysis
Data were analyzed using GraphPad Prism version 9.3.0, GraphPad Software, LLC, for Macintosh (San Diego, California, USA). The databases were compiled using Microsoft Excel. The data variability was visualized using unsupervised principal component analysis (PCA) using eigenvector covariate projection on biplots as described elsewhere (58–61). Further interrogation of the individual or independent principal component clustering and variability, factor differences, and interactions or associations of independent predictor variables by gender and by survival was performed using univariate and multivariate analyses.
Univariate analytic methods comprised pairwise comparisons using Mann–Whitney non-parametric U-test that compared arithmetic medians and unpaired parametric t-test that compared arithmetic means. In this context of non-normally distributed population variables, statistical differences were reported on the basis of the difference in the sample medians with interquartile ranges (IQRs). The univariant difference in the survival outcome or survival risk was determined using the Kaplan–Meier/log-rank test (Mantel–Cox chi-square test) or the Mann–Whitney U-test. The difference in binary outcome was determined using the Fisher’s exact chi-square test or the Mann–Whitney U-test.
In multivariate models, data were Log2-transformed to normalize the variable prior to the statistical interrogation using multivariate factor analysis and or survival-adjusted logistic regression least square models that measure the risk of likelihood (proportional hazard ratios). In all models, missingness was not imputed. In this context, statistical significance in both the univariate and the multivariate models was based on difference among variables in the original available dataset among participants. The statistical level of the significantly different covariates was reported at a power of 0.80, at a p-value <0.05, and at a 95% level of confidence. The rigor of our interrogations included a large sample size (N = 419), controlled comparative covariables at nearly a 1:1 ratio, and minimal missingness with approximately 94% data completeness in gender analyses and approximately 90% data completeness in the 18-week survival analyses.
3 Results
3.1 Baseline demographics by gender
At enrollment, all participants with CM in the current study (419 participants) were antifungal treatment–naïve, but more than half of the participants (52.3%) were ART-experienced with the median time on ART of 1.6 months (IQR, 0–22 months). However, no statistical difference in survival was observed between ART-naïve and ART-experienced participants overall and by gender (Figure 1A). The cytokines levels showed elevation with ART with IL-17A being statistically significant overall, by gender and by survival (Figure 1B). The cryptococcal CSF fungal burden was significantly lower with ART overall, by gender and by survival (Figure 1C). Survival in the parent cohort was at 50.9% (2), and survival did not differ statistically between either sertraline randomized participants or those on standard therapy alone (2).
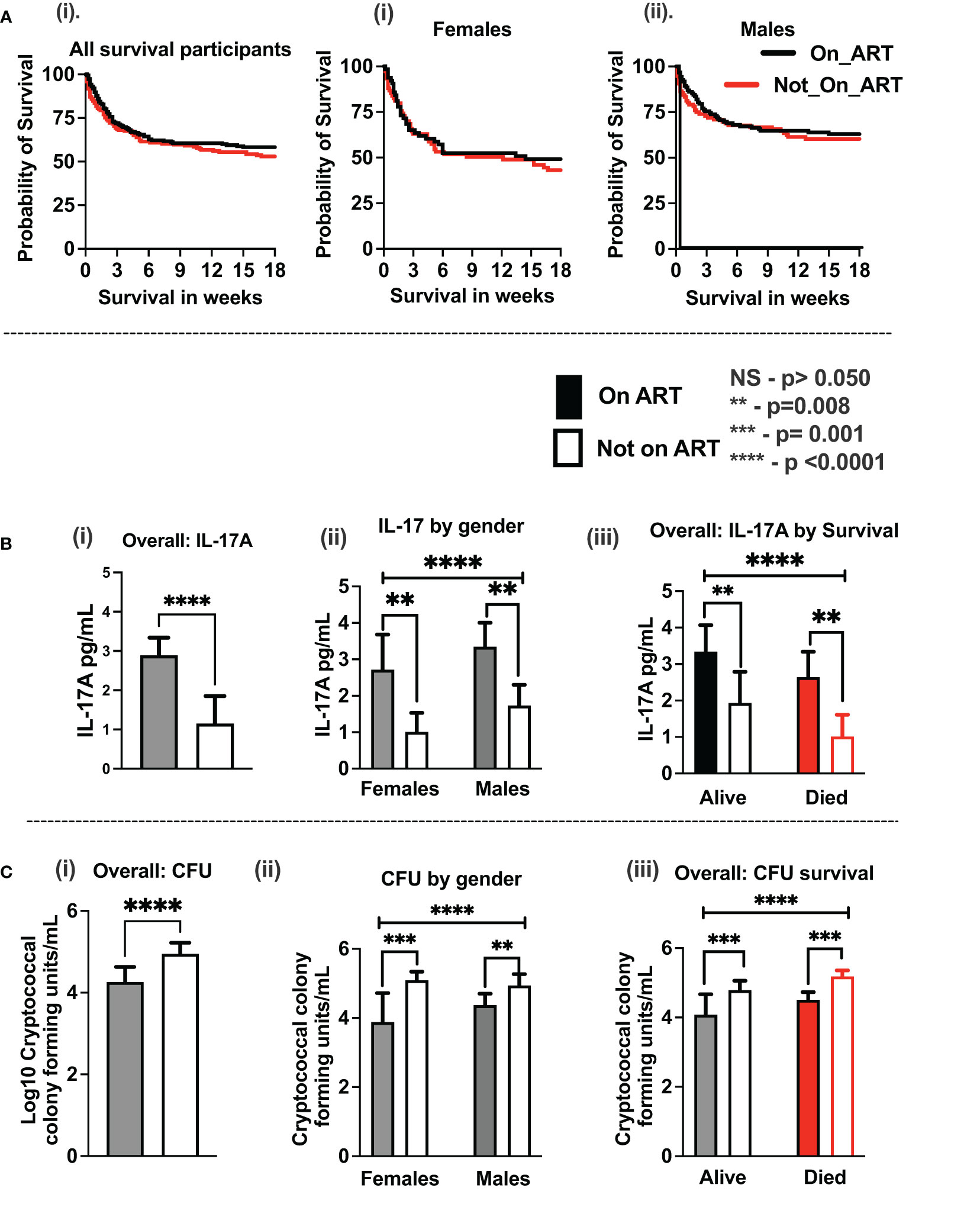
Figure 1 Association of baseline antiretroviral therapy on CSF immune response and host survival on antifungal treatment. (A) Kaplan–Meier survival by ART experience (i) and by gender (ii, iii). (B) IL-17A levels by ART experience (i), by gender (ii), and by host survival (iii). (C) CSF cryptococcal fungal burden by ART experience (i), by gender (ii), and by host survival (iii). Participants with ART status (N = 359 participants). Participants on ART (n = 188 participants) and not on ART (n = 171 participants). Females on ART (n = 65 females) and not on ART (n = 75 females). Males on ART (n = 123 males) and not on ART (n = 96 males). Asterisks (*) show statistically significant variables at p < 0.050, at 95% confidence intervals.
Consistent with published reports (Figure 2), among the 419 participants studied with HIV-associated CM, the majority were men, who were older and heavier than women (Table 1). Neurologic abnormalities predominated among clinical signs and symptoms, with almost all describing headache for 1 to 4 weeks, a third reporting changes in mental status, and a half with abnormal Glasgow Coma Score (GCS < 15), with each variable reported at comparable frequencies in men and women.
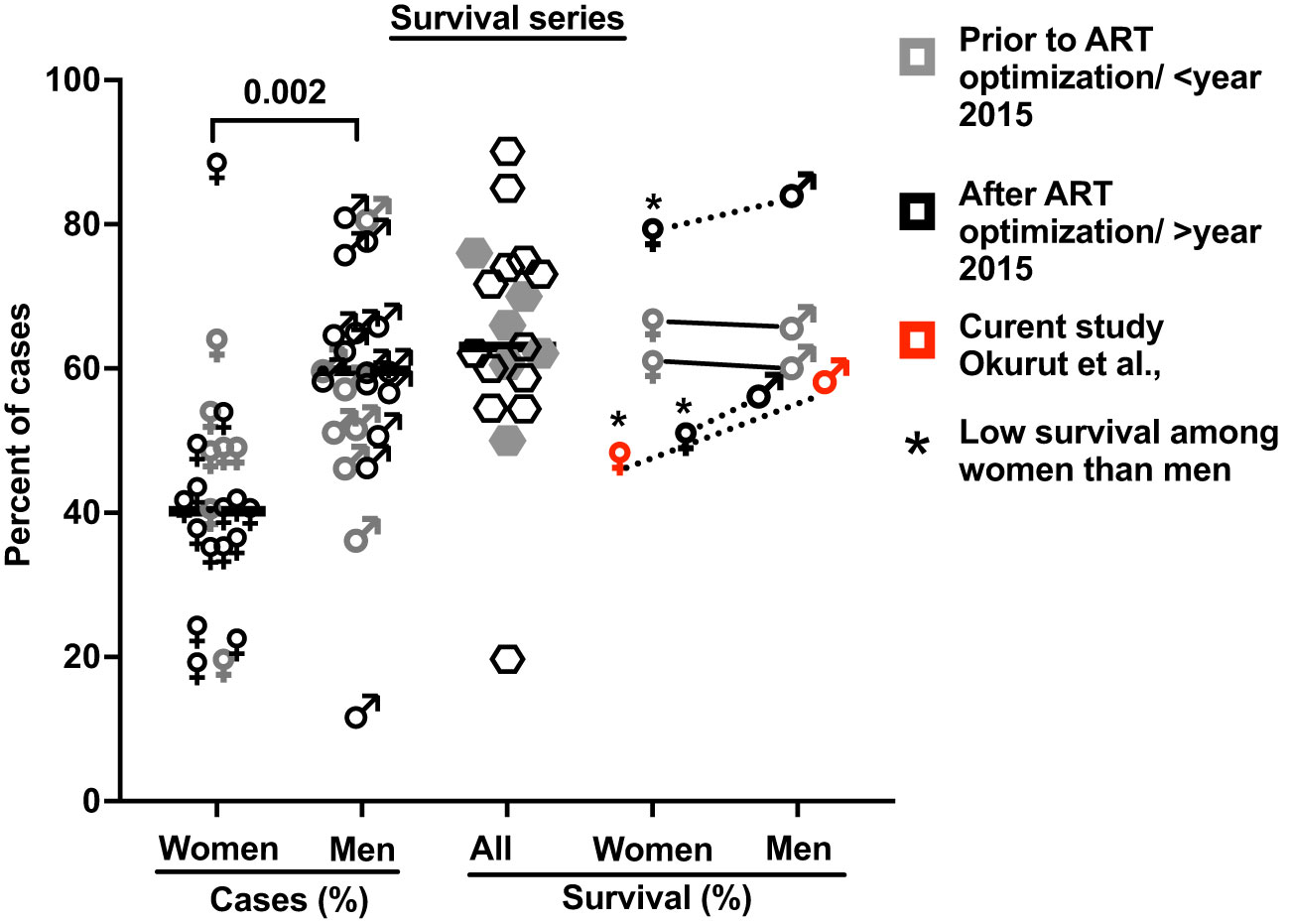
Figure 2 The proportion of cases with HIV-associated CM by gender and of related survival by gender in 21 published case series. These studies include 38,485 cases with 5,834 reported deaths, which accounted for a 15.2% case fatality rate (see Supplementary Table 1 for details and references (7, 9, 16, 26, 28–44)). Bars show median values. Five studies report survival by gender (four references and this report; Supplementary Table 1). * Shows published data with significantly low survival among women treated for cryptococcal meningitis compared to men.
As anticipated, CD4+ T-cell numbers in circulation were low but were marginally higher in women than that in men, as were platelet counts and hemoglobin levels (Table 1). CSF protein was not consistently elevated, and white blood cells (WBC) counts were low, despite a high burden of yeast. Each result was generally comparable by gender, except CSF glucose that tended to be lower among women than that among men (Table 1).
3.2 The 18-week survival on antifungal treatment
In the parent trial for this analysis, men and women from Uganda and South Africa were randomized 1:1 to receive sertraline (an antidepressant with putative antifungal activity) or standard treatment but showed no differences in survival between treatment groups at 18 weeks (2). An initial analysis by gender of an expanded data set, from which this current report is derived, showed lower 10-week survival among 400 women vs. 577 men (at 50% female survival vs. 57% male survival, respectively), although at 10 weeks unadjusted [hazard ratio (HR) was only borderline, HR = 1.20; 95% confidence interval (CI), 1.00–1.45; p ≤ 0.050] (7). These differences were greater yet, in this current subset, extended to 18 weeks of observation. Survival among women was 47% (71 of 150 women) vs. 59% among men (136 of 230 men) [Mantel–Cox proportional HR = 1.4 (95% CI, 1.0–1.9); p = 0.023) (Figure 2). Of note, survival was similar among men and women in two earlier studies reported prior to ART optimization (before 2015) but lower among women in two studies reported after ART optimization (after 2015), which include the current report (Figures 2, 3).
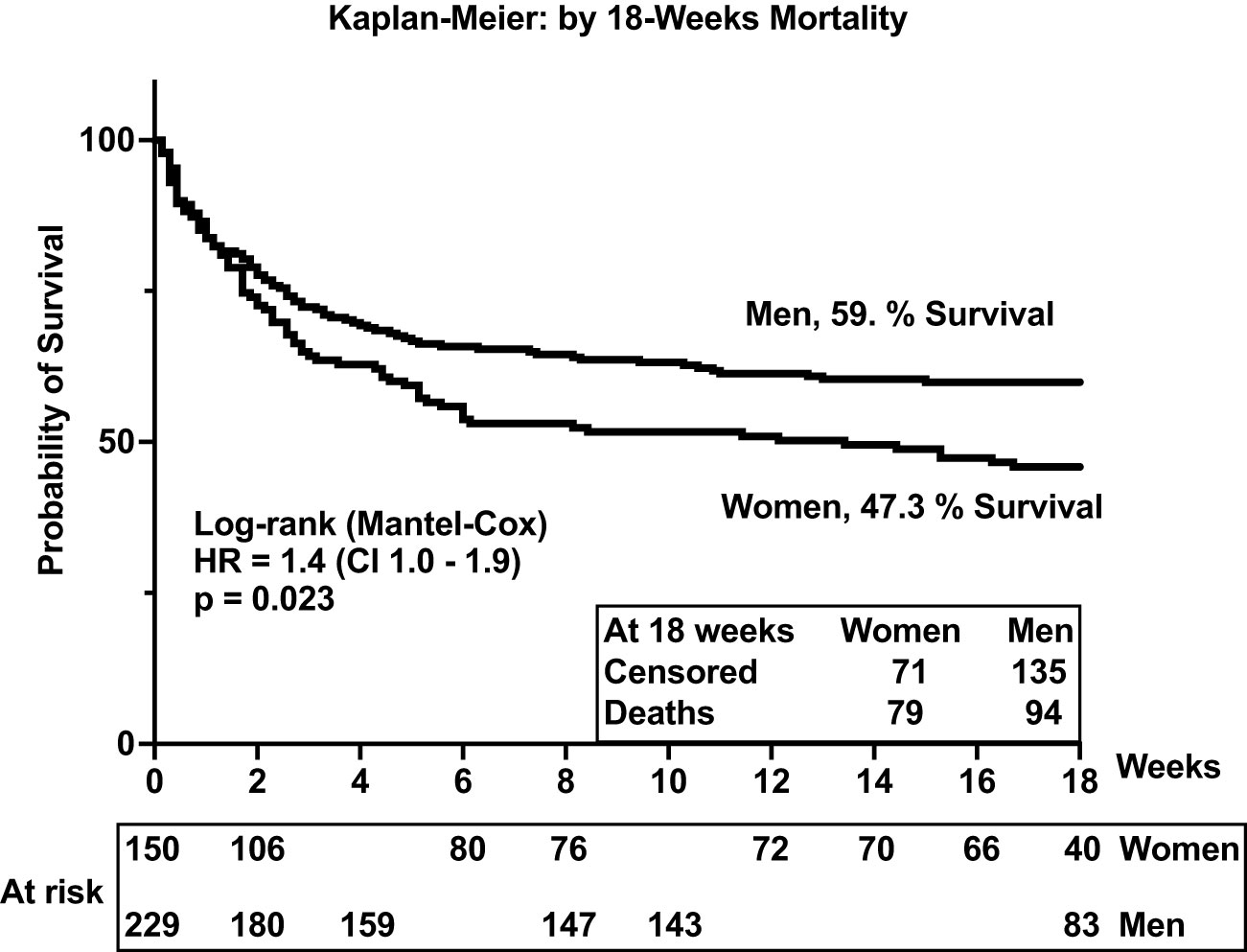
Figure 3 Survival among people with HIV-associated cryptococcal meningitis. The p-value <0.05 is statistically different.
Survival was similar by gender in the first 2 weeks of antifungal therapy (Figure 2). However, survival diverged thereafter, remaining consistently lower among women throughout the 18 weeks of observation. As noted, baseline demographics, signs and symptoms, blood and CSF analytes, and cryptococcal fungal burden were relatively similar among participants by gender and by survival (Table 1). Thus, we considered whether the concentrations and the composition of neuroimmune induced factors at the site of severe cryptococcal disease in the CSF could underlie subsequent differences in survival by gender over time of observation.
3.3 Significant differences in neuroimmune signatures in cerebrospinal fluid by survival, gender, and intragender-specific survival
At baseline, we performed unsupervised PCA as a primary approach to visualize the data variability and to explore potential unbiased differences in data clustering by gender, survival, and gender-specific survival (Figure 4). The PCA identified individual clusters that offered opportunities to structure downstream data analyses of the indicated model outcomes. The members in the cluster aggregated on the basis of common attributes of the datasets showing high variability and distinct distribution of cytokines and chemokines between women and men (Figure 4A), between survivors and participants who died (Figure 3B), and within gender survival, among women (Figure 4C) and among men (Figure 4D). Eigenvector projections on principal components 1 and 2 (PC1 and PC2, respectively) indicated a high probability of neuroimmune variables predicting association with gender and survival outcome (Figures 4A–D). High eigenvalues >5 indicate the high capability of the selected covariables in predicting model-associated outcomes (Table 2). For each of the comparative groups, almost all showed such high eigenvalues and, thereby, the separation between determinants in each group. These distinct patterns for PC1 and PC2 were closely correlated among participants by gender, subject by survival, and women by survival but less so for male survival in which three clusters were identified (correlation data not shown). Because of the notable data clustering observed in these groups, we next determined the specific neuroimmune factors contributing to the observed patterns using supervised univariate and multivariate data interrogation approaches.
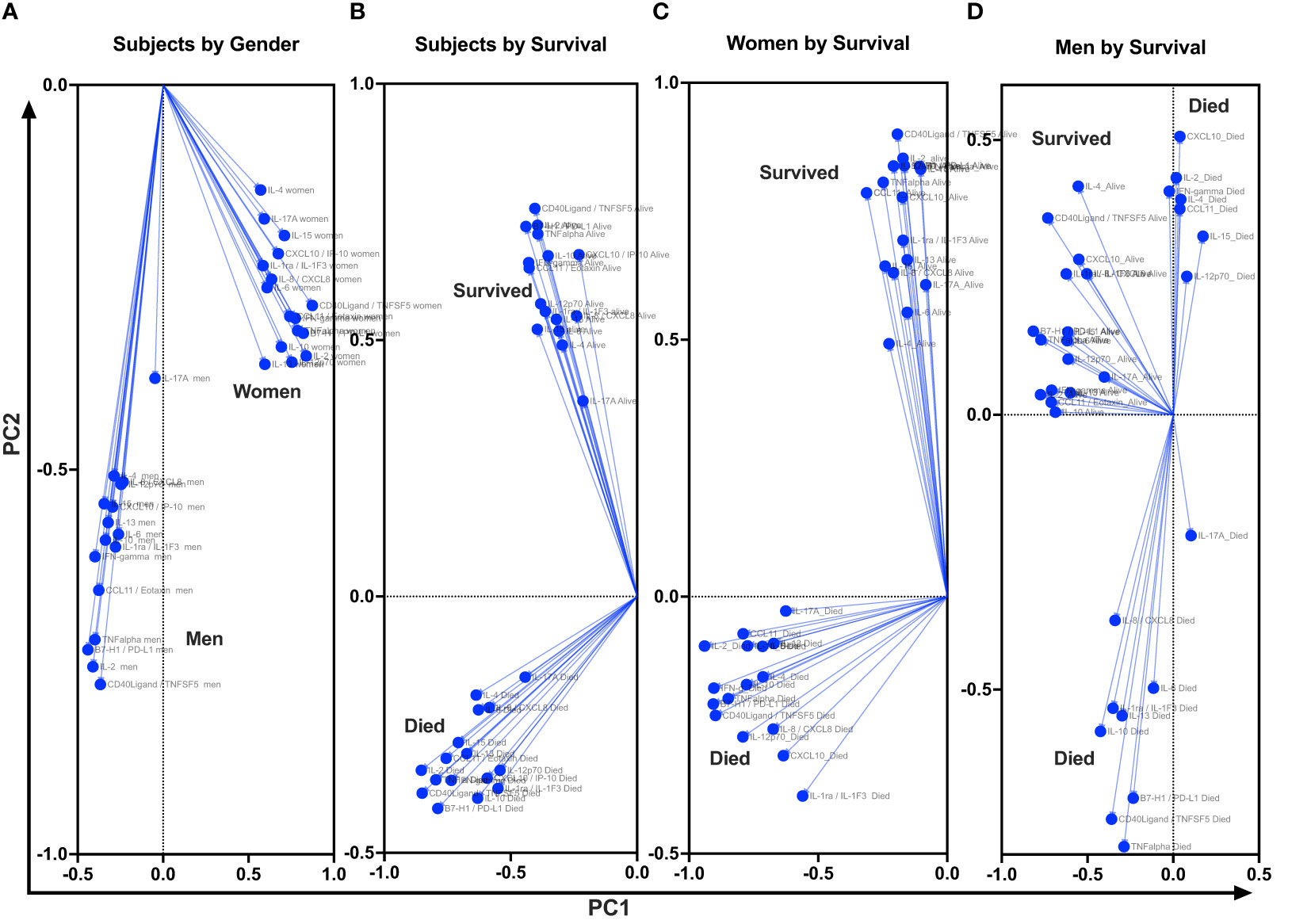
Figure 4 Projection of cytokine responses on eigenvector correlation covariates on PC1 and PC2 (axes) using unsupervised principal component analysis. Baseline cerebrospinal fluid (CSF) immune signature among participants who were diagnosed with HIV-associated CM showed distinct clusters by gender and by survival. PC, principal component. Dots show individual cytokines produced among participants projected on eigenvector correlation covariates. Variables near the center (0; zero) are uncorrelated to the model, and variables further away from the center (0; zero) are strongly correlated to the model. Variables among all cytokine projected on the variety of CSF cytokine patterns among participants by host survival (Figure 3A). The CSF secreted cytokines clustering by gender (Figure 3B). Cytokine clustering by female survival (Figure 3C). Cytokine clustering by men’s survival (Figure 3D).
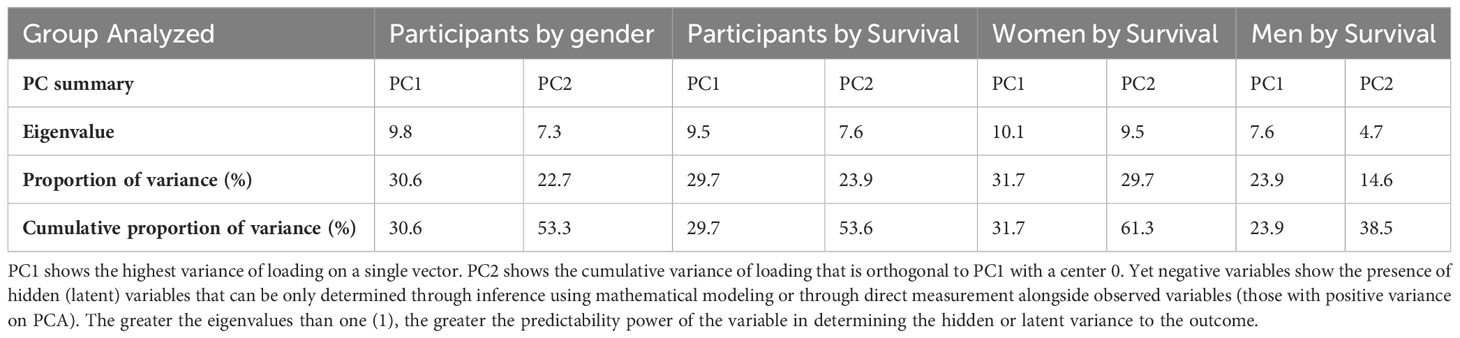
Table 2 Adjacent Table to Figure 4.
3.4 Innate neuroinflammatory cytokines in the cerebrospinal fluid differ by gender
The CSF of women with CM at baseline had significantly lower levels of selected innate cytokines than that of men, particularly IL-1RA and IL-15, TNF-α and immune checkpoint, and PD-L1 (all p < 0.050) (Table 3). The remainder of the cytokines and chemokines interrogated did not differ significantly by gender but tended to be lower still among women than that among men (Table 3). We next considered whether such gender-specific differences were associated with the differences in survival by gender.
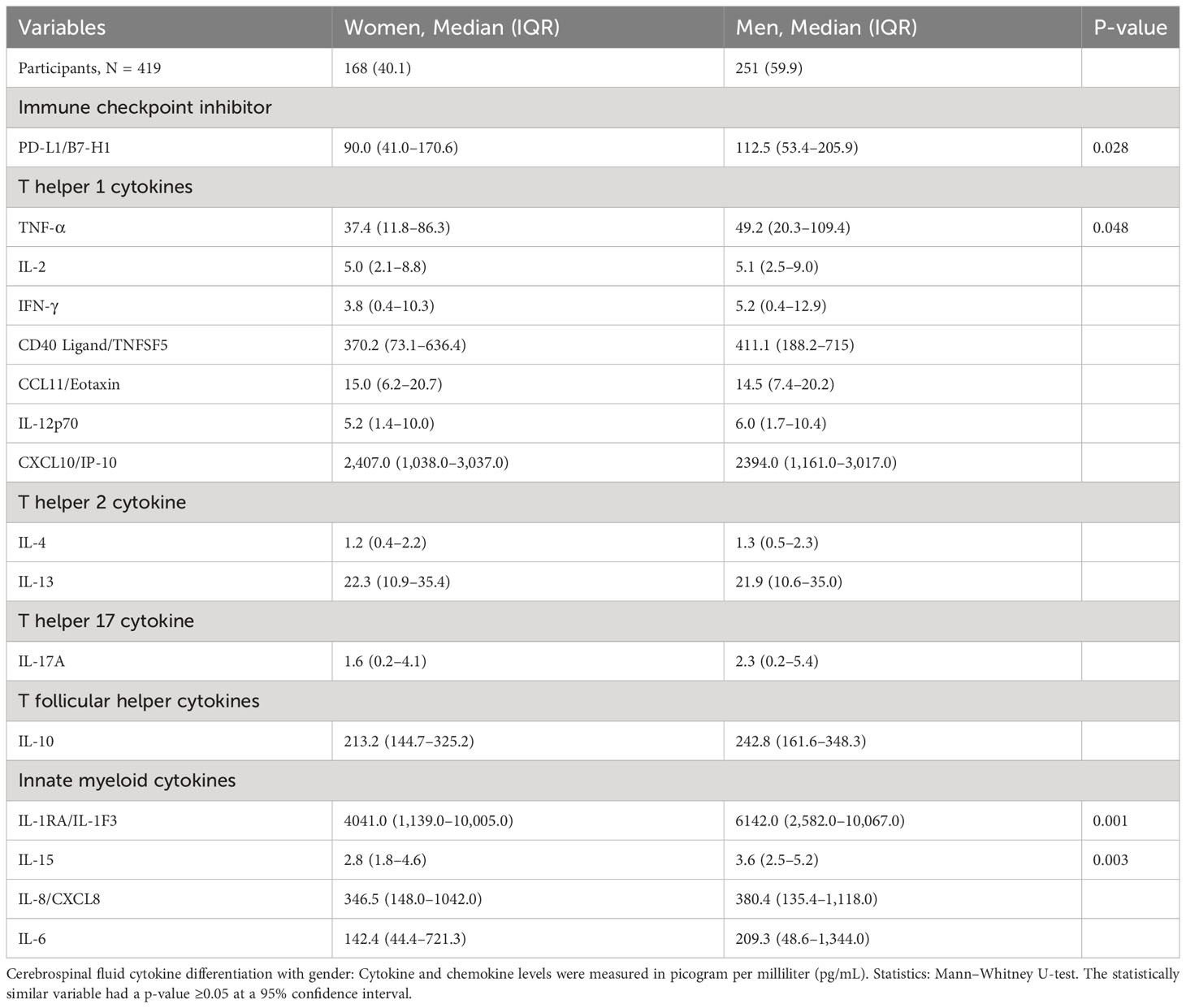
Table 3 Differences in baseline immune signature in cerebrospinal fluid exudate by gender among patients with HIV-associated cryptococcal meningitis.
3.5 Divergent baseline neuroimmune cytokine signatures predict intragender survival
3.5.1 Female gender–specific survival attributes
A number of relevant factors differed between women who survived or died during the 18-week observation. The circulating CD4+ T-cell numbers were generally very low, but median CD4+ T cells were 31 cells/µL vs. 14 cells/µL among women who survived vs. those who died (p = 0.009) but did not differ by survival in men (data not shown). Using several alternative models and after adjusting for cytokines (Table 4, model 1), CXCL10 and CCL11 consistently predicted female survival but not in men. These soluble immune factors were consistently higher in magnitude among female survivors compared with those who died (Table 5). The level of CXCL10 was significantly higher among women who survived than those who died (p = 0.013) (Table 5). By survival, CXCL10 levels in women who survived correlated with the number of CSF white cell counts (r = 0.292; 95% CI, 0.067–0.488; p = 0.010) but not in men (Supplementary Figure 1). The fungal burden [colony-forming units (CFUs)] did not correlate with CXCL10 levels by either gender or gender-specific survival (data not shown).
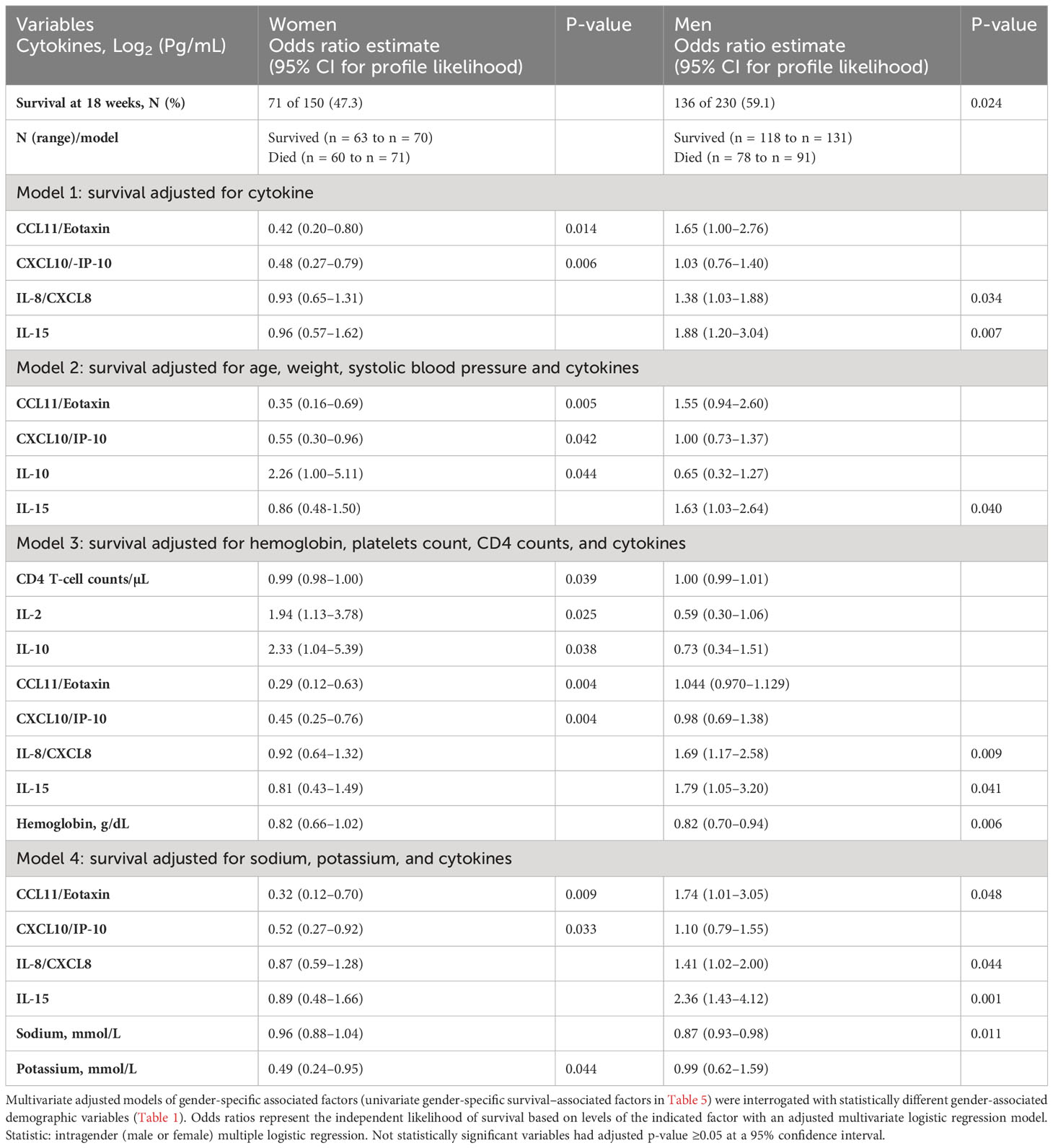
Table 4 Baseline-independent factors predicting intragender 18-week survival among patients with HIV-associated cryptococcal meningitis on antifungal therapy.
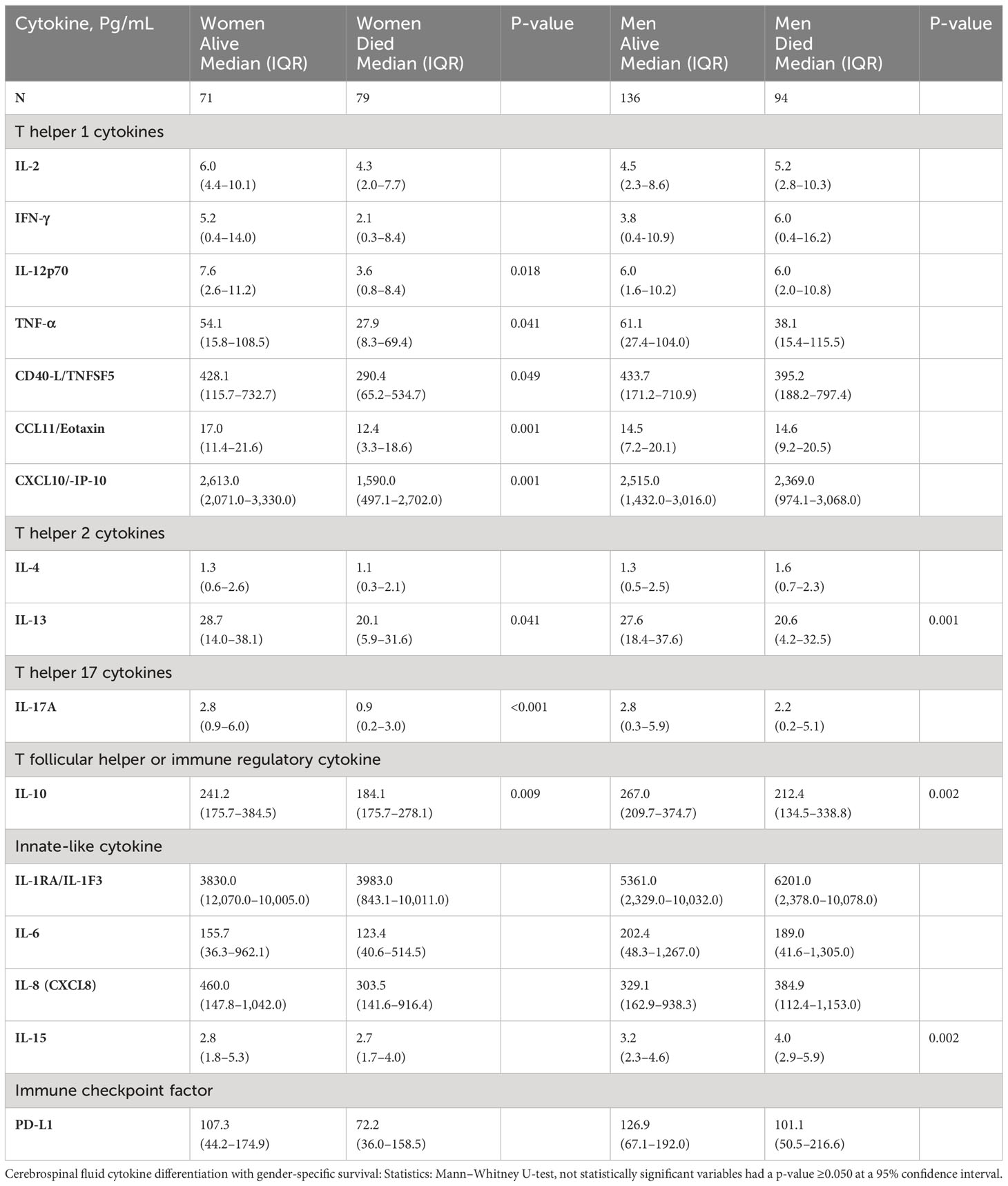
Table 5 Univariate difference in baseline levels of CSF factors associated with gender survival among patients with HIV-associated CM.
The level of CCL11 (a chemoattractant produced by activated astrocytes, lymphocytes, and macrophages) was significantly higher in female survivors than in those who died but not among men (Table 5). After adjusting for other cytokines, higher CCL11 expression still predicted female but not male survival (Table 4, model 1). T-cell–related factors (e.g., IL-12p70, IL-17A, and IFN-γ) in the univariate models were also consistently increased in women who survived compared with those who died but not in men (Table 5). The levels of other cytokines and the neutrophils chemokine IL-8 showed no difference among women by survival (Table 5). Only IL-10 level was increased in both genders among those who survived in univariable analysis (Table 5). However, after adjusting for other factors, the levels of IL-10 expression were correlated with only female survival, but not with male survival (Table 4, models 2 and 3), as was IL-2 (Table 4, model 3).
In summary, although immune parameters were lower among all women vs. men, the women who survived showed consistently elevated levels of both myeloid-derived chemokines (CCL11), lymphoid-derived chemokine (CXCL10), T-cell–derived cytokines, and the regulatory molecule PD-L1, the differences that were mostly distinct to survival in women but not in men (Supplementary Figure 1).
3.5.2 Male gender–specific survival attributes
Men who survived had significantly higher hemoglobin compared with those who died, even after adjusting for the platelet counts and CD4+ T-cell counts (Table 4, model 3). Men, but not women, who survived had significantly lower IL-15 than those who died (Table 5), even after adjusting for other cytokines (Table 4, all models). The IL-15 levels were independent of fungal burden (CFUs) and CSF white cell counts (data not shown). After adjusting for other factors, lower levels of the neutrophil chemoattractant IL-8 also consistently predicted male survival but not among women (Table 4, models 1, 3–4). Unlike in men, low levels of neither IL-15 nor IL-8 were associated with survival in women. As noted above, elevated levels of regulatory IL-10 were associated with survival in both genders but did not correlate with male survival (Supplementary Figure 1).
4 Discussion
In a large cohort of adults with CM in Uganda on optimized ART anti-fungal therapy, survival among women was significantly lower compared with that among men. Evaluating gender-specific mortality is uncommon in this context (only five including the current of 21 studies; Supplementary Table 1). Unlike in the two previous studies that showed no difference in mortality by gender reported (26, 44), the two studies showing such a difference (7, 42) were performed in people treated with optimally timed (delayed) initiation of ART that lowers mortality and with optimized antifungal therapy, which helps control fungal burden. Combining antifungal drugs with immunomodulatory interventions as new therapies could limit the fungal and immune assault on the CNS. Illustrated by high mortality among CM-infected subjects with more prominent brain lesions from magnetic resonance imaging and at autopsy who could benefit from combining antifungal drugs with immunomodulatory interventions (62–65). As proposed by Casadevall and Pirofski (11, 12), with appreciable control of fungal burden, immune responses may be a more prominent determinant of clinical outcomes.
We have identified distinct immunologic differences in CSF by gender and gender-specific survival. The signatures associated with survival in women are distinct from those in men. These differences were independent of baseline clinical features and cryptococcal fungal burden (CFUs). Most consistent in both the univariate and in multivariate gender-associated survival predictive models were the lower levels of CSF CXCL10/IP-10 and CCL11/Eotaxin that distinguished women who died from survivors but not among men. In contrast, higher levels of IL-15 and IL-8 differentiated men who died from those who survived but not among women. Several T-cell cytokines (IFN-γ, TNF-α, IL-13, and IL-17A) similarly exhibited diminished levels of expression in women who died matched survivors but not in men. Lower levels of immune regulatory IL-10 expression were linked with an increased frequency of death in both genders. Thus, the biological significance of gender-specific CSF immune signatures identified among participants with CM may underlie important immune-based mechanisms as a guide to improving antifungal treatment and survival predominantly in women.
Consistent with our univariate model observations, prior studies showed that improved survival at 2–10 weeks was associated with elevation in the levels of soluble CSF cytokines Th1 IL-2, IFN-γ, TNF-α, Th2 IL-4, and Th17 IL-17A, as well as innate IL-6 (66, 67). Soluble T-lymphokine levels in CSF are proposed to derive from CXCL10-mediated (68) and CCL11-mediated (69) stimulation of CNS-resident immune cells. Moreover, the use of cryptococcal antigens [such as Glucuroxylomannan (GXM)] to stimulate peripheral immunocytes demonstrated that patients with improved 10-week survival had increased expression of these cytokines among polyfunctional differentiated CD4 T cells (67). Other chemokines reported to predict improved survival included the elevated levels of monocytes chemotactic protein-1 and macrophages inflammatory protein-1 (66). Despite the demonstrated correlations of survival in the peripheral circulation (70, 71), the importance of characterizing immune responses in the CNS, at the foci of infection, cannot be underestimated, because CNS responses in larger studies do not often correlate with those in the peripheral circulation (70, 71). The disparity between peripheral and CNS observed responses is a characteristic indicator of local mechanisms that influences responses. Compartment-specific responses have been noted previously (16, 17) and highlight the relevance of characterizing the response in CSF. Thus, the current study is unique in characterizing the distinctive neuroimmune signatures defining survival by gender in CNS, at the foci of disease.
In theory, the microbial invasion of the CNS activates resident immune cells to produce neuroimmune cellular activating cytokines, chemoattractant chemokines, and surrounding tissue basal cellular immune mediators (prostaglandins, leukotrienes, etc.). These diverse effector cells include resident microglial, astrocytes, oligodendrocytes, CNS surveillance phagocytes (monocytes, macrophages, and neutrophils), dendritic cells, adaptive T and B cells, innate [natural killer (NK) cells], and basal barrier epithelial cells (72). Other cells may be drawn by extravasation and diapedesis through the protective vascular barriers to infiltrate the CNS in response to infection and/or to ensuing meningoencephalitis (16, 17). The consistent use of antifungal therapy in this study helps to control fungal replication and the burden of fungi (2, 9, 26, 73). With relatively effective antifungal therapy, modeling immune factors highlights the role of the immune system in determining immune-mediated damage vs. protection in the CSF and, ultimately, disease outcome (67, 74, 75).
Although cryptococcal fungal burden and other clinical parameters in blood or in CSF did not differ by gender or gender-associated survival, increased production of IL-1RA, IL-15, and PD-L1 in the CSF may have contributed to the mortality of men but not women. Lower male, but not female, survival was associated with high levels of myeloid cytokines, including the pleiotropic IL-15 and neutrophil-activating factor IL-8. In the literature, IL-8 expression derived from microglia in CNS in response to neuroinflammation (76, 77) from monocytes and autocrine IL-8 production by neutrophils enhances immune activation and cellular proliferation (20). Elevated intrathecal IL-15 and IL-8 in the CSF can enhance neutrophil-induced NETosis (neutrophils traps), which may propagate thrombotic vasculitis, cryptococcomas, and fungal occlusions in subarachnoid ventricular and arterial spaces, thereby reducing survival. Together, IL-15 and IL-8 elevation can increase local inflammation in the brain, leading to vasculitis in subarachnoid spaces; interfering with blood supply; promoting ischemia, necrosis, and infarction of the brain tissue; and potentially leading to increased risk of death. Thus, the relatively high levels of IL-15 expression among men who died and the inverse relationship of IL-15 levels with those of immune regulatory IL-10 and immune checkpoint PD-L1 among men who died highlight this dynamic functional interrelationship. Lowering levels of IL-15 may limit the mitotic division of fungal-infected macrophages that may attenuate the propagation of endogenous pathogens in quiescent cells (20), leading to lower fungal burden and improved survival.
Among neutrophil-activating factors (e.g., IL-8 and IL-15) may promote neutrophil-induced tissue injury through self-directed host and pathogen-mediated mechanisms (78, 79). Neutrophil activation is associated with the propagation of tissue necrosis, hypoxia, and nutritional supply impairment, especially among people with sepsis and multiple organ failure, which are often complicated by brain injury, e.g., stroke (78, 79). In this context, activation of neutrophil recruiting cytokines and chemokines at the foci of infection in the CNS is detrimental to the host (76, 78). Released toxic granules are destructive to connective tissues, leading to vasculitis with tissue necrosis, infarction, hypoxia, and, potentially, death. Indeed, in CM, neutrophilia in the circulation was associated with poor survival outcome (80), brain hypoxia (15, 81, 82), subarachnoid blood vessel occlusion (83), brain tissue necrosis, and cerebellar infarction (64). Subsequently, survivors of CM suffered long-term CNS abnormalities associated with impaired faculty observed in sequelae among people without HIV infection (62, 84). The persistent CNS abnormalities in sequelae noted in humans with fungal infections were similar to those observed among mouse models of Alzheimer’s disease (85). In other fatal CNS infections, such as tuberculosis and bacterial meningitis, neutrophils account for the majority of cells in the CSF (86, 87). However, neither we nor others have characterized in detail the neutrophil myeloid lineages and role among CSF white cells in the setting of CM-associated survival.
Among key differences in female intragender survival with CM, survivors tended to shed relatively higher amounts of neuroimmune-mediating soluble cytokine and chemokine responses across the panel. The higher levels of Th1 IL-2, IFN-γ, and TNF-α, as well as IL-12p70, Th17 IL-17A, and immune regulatory IL-10 may influence survival in women by distinct CXCL10 and CCL11 chemokine-stimulated mechanisms. In contrast to women who died, women with higher levels of the myeloid chemokine CCL11 and the lymphoid chemoattractant CXCL10 were more likely to survive, but not men. CXCL10 produced by astrocytes and microglia cells or by CNS-resident monocytes, macrophages, and dendritic cells stimulate Th1 cytotoxic CD8 T cells, immune modulatory CD4 T cells, regulatory T cells, NK and regulatory B cells, and regulatory T cells via its CXCR3 receptor–mediated stimulation (88). The cytotoxic response to infected macrophages is potentially enhanced in the presence of elevated chemokines in the CNS, because the infected cells respond to chemoattractant proteins at the site of infection (89, 90), whereas immune regulatory cells can modulate inflammation and cellular activation to stimulate tissue recovery (91). Thus, independent of the baseline CSF cryptococcal burden (CFUs) and the baseline clinical features, the CCL11 and CXCL10 balance is critical in immune regulation of the Th1/Th2/17 balance, modulation of CNS cellular influx that is important in better survival of host with HIV-associated CM.
IL-12 is observed as an important factor in the immune control of Cryptococcus meningitis as it is observed to influence mechanisms that inhibited cryptococcal fungal replication among Cryptococcus-infected macrophages in vitro (92). The timing of immunotherapy is likely important. In mouse models, early preemptive use of IL-12 as an adjuvant promoted survival of mice in early use after cryptococcal fungal (92), whereas the late treatment of mice with IL-12 after establishment of cryptococcal infection resulted in more mice dying from fungal infection attributable deaths (92). In the current study, IL-12p70 was elevated among female, but not in male, survivors. In the CNS, IL-12, produced prominently by dendritic cells, regulates T cells, including differentiation of the Th1 T cells and NK-cell function (93). With the prominence of T cells and B cells in the CSF with CM (16, 17), increased IL-12p70 expressed in the CSF could enhance fungal-specific immunocyte activation to control infection and improve host survival.
The Th17 cytokine, IL17A, modulated through RORγt regulatory pathway, works in tandem with Th1-modulated cytokines to regulate neuroimmune responses to CNS infections (94). In particular, IL-17A activates Th17 immunocytes in response to tissue-resident infection. In a cryptococcal model infection, IL-17 promoted the development of a majority of giant cryptococcal cells that have limited ability to cross the blood-brain barrier, leading to localized cryptococcal fungal infection with limited spread to the brain parenchyma (65, 95). Among women in our study, IL-17A was upregulated among those with better survival outcomes. Other Th1 cytokines upregulated among female survivors included IFN-γ and TNF-α and their immune-activating factor, CD40 ligand. These factors facilitate the formation of reactive phagosomes and the activation of reactive oxygen and nitrogen species that mediate endogenous killing of Cryptococci ingested by macrophages (65, 95). Collectively, these complementary immune-activating factors were each increased in association with increased survival among women. In aggregate, these novel findings, first reported herein in the setting of CM gender-specific survival, direct attention to how these results may be harnessed for targeted gender-specific immune therapy to optimize survival, which appears to operate independent of the fungal burden, clinical presentation, and current antifungal therapy.
5 Conclusion
Survival from HIV-associated CM remains significantly lower among women than that among men. We found novel female and male biological sex-distinctive survival associated CSF neuroimmune chemokine and cytokine biomarker signature at the time of HIV-associated CM clinical presentation (at diagnosis) that were independent of the baseline fungal burden and clinical features. Increased concentration of CCL11, CXCL10, and T-cell subset–associated cytokines (mediating the Th1 and Th17 pathways) was found to be uniquely associated with female intragender survival but not in men. In addition, low levels of the neutrophil-activating chemokine IL-8 and pleiotropic cytokine IL-15 were distinctively associated with male intragender survival but not in women. Increased expression of IL-10 and PD-L1 immune regulatory molecules were associated with improved survival in both genders. The increases in CCL11, CXCL10, IL-17A, and IL-12p70 neuroimmune cytokine signatures in CSF among women who survived and these markers’ positive correlation with IL-10 and PD-L1 highlight the interdependence of these biomarkers responses in shaping immune homeostasis and host survival. These novel neuroimmune biomarkers’ observations reveal the promising signals to be considered in attempts to model more robust biological sex-specific responses to improve HIV-associated CM treatment, clinical outcomes, and host survival.
6 Limitations
One limitation is that the cross-sectional, exploratory study design generated data may not explain marker and clinical variations after the baseline and prior to death outcome or study censor among survivors. The missed observations might be key to explaining the factors that underlie the observed gender-survival subjective mechanisms. The differential of myeloid vs. lymphoid white cells in the CSF compartment was largely unavailable, nor was the identification of the specific local cells producing the immune mediators. The limitations of our interpretations of the baseline cross-sectional findings can be enhanced with longitudinal data to appropriately capture and model changes in the immune profile from diagnosis to eventual survival or death.
Author’s note
An abstract CROI 2023 poster 498 from this work was presented at the Conference on Retroviruses and Opportunistic Infections at Seattle Convention Center, Seattle, Washington, 19-22 February 2023 and was published online on CROI website 96 and part of the doctoral thesis 2023 at the Makerere University 97.
Data availability statement
The original contributions presented in the study are included in the article/Supplementary Materials, further inquiries can be directed to the corresponding author.
Ethics statement
The studies involving humans were approved by Makerere University, School of Biomedical Sciences, College of Health Sciences, Higher Degrees Research, and Ethics Committee. The studies were conducted in accordance with the local legislation and institutional requirements. The human samples used in this study were acquired from primarily isolated as part of your previous study for which ethical approval was obtained. Written informed consent for participation was provided from the participants or the participants’ legal guardians/next of kin in accordance with the national legislation and institutional requirements.
Author contributions
SO: Conceptualization, Data curation, Formal Analysis, Funding acquisition, Investigation, Methodology, Project administration, Resources, Software, Supervision, Validation, Visualization, Writing – original draft, Writing – review & editing. DB: Conceptualization, Data curation, Formal Analysis, Funding acquisition, Investigation, Methodology, Project administration, Resources, Software, Supervision, Validation, Visualization, Writing – original draft, Writing – review & editing. EO: Conceptualization, Data curation, Investigation, Methodology, Resources, Validation, Visualization, Writing – review & editing. JR: Conceptualization, Data curation, Formal Analysis, Funding acquisition, Investigation, Methodology, Project administration, Resources, Software, Supervision, Validation, Visualization, Writing – original draft, Writing – review & editing. HK: Conceptualization, Investigation, Methodology, Project administration, Resources, Supervision, Validation, Visualization, Writing – review & editing. BB: Conceptualization, Formal Analysis, Investigation, Methodology, Project administration, Resources, Supervision, Validation, Visualization, Writing – original draft, Writing – review & editing. FB: Conceptualization, Investigation, Methodology, Project administration, Resources, Supervision, Validation, Visualization, Writing – review & editing. JO: Conceptualization, Data curation, Formal Analysis, Investigation, Methodology, Project administration, Resources, Supervision, Validation, Visualization, Writing – original draft, Writing – review & editing. YM: Conceptualization, Data curation, Formal Analysis, Funding acquisition, Investigation, Methodology, Project administration, Resources, Software, Supervision, Validation, Visualization, Writing – original draft, Writing – review & editing. DM: Conceptualization, Data curation, Formal Analysis, Funding acquisition, Investigation, Methodology, Project administration, Resources, Software, Supervision, Validation, Visualization, Writing – original draft, Writing – review & editing. EJ: Conceptualization, Data curation, Formal Analysis, Funding acquisition, Investigation, Methodology, Project administration, Resources, Software, Supervision, Validation, Visualization, Writing – original draft, Writing – review & editing.
Funding
The author(s) declare financial support was received for the research, authorship, and/or publication of this article. This work was supported in part by the funding from National Institutes of Health and the National Institute of Allergy and Infectious Diseases R01 AI078934, U01 AI089244, R21 NS065713, and T32 AI055433 grants to DM and DB; R01 AI108479 grant to JR, EJ, DM, and DB. National Institute of Neurologic Diseases and Stroke R01 NS086312, R25 TW009345, and K24 AI096925 grant to JR. Fogarty International Center D43 TW009771 grant to YM and R01 NS086312 grant to RJ. GlaxoSmithKline Trust in Science Africa grant COL 100044928 grant to SO. DELTAS Africa Initiative DEL-15-011 grant to THRiVE-2, DM. Wellcome Trust grants 107742/Z/15/Z grant to DM. Veterans Affairs Research Service I01CX001464 grant to EJ. The Wellcome Trust Training Health Researchers into Vocational Excellence (THRiVE) in East Africa, 087540 grant to DM. United Kingdom Medical Research Council/Wellcome Trust/Department for International Development (MRC MR/M007413/1 grant to JR and the Grand Challenges Canada S4-0296-01 grant to JR. Funding agencies had no role in study design, data collection, data analysis plan, preparation of the manuscript, or in the decision to publish.
Acknowledgments
We extend our appreciation to the study participants for taking part in the research. We thank the ASTRO trial team for the clinical management of patients and for data collection, especially Abdu Musubire, Lilian Tugume, Jane Francis Ndyetukira, Cynthia Ahimbisibwe, Florence Kugonza, Alisat Sadiq, Radha Rajasingham, Catherine Nanteza, Kiiza Tadeo Kandole, and Darlisha Williams, Edward Mpoza, Fiona Cresswell, Andrew Akampurira, Apio Alison, Caleb Skipper, Kenneth Ssebambulidde, and Mahsa Abassi. We thank the institutional support received from the Infectious Diseases Institute from Bosco Kafufu, Andrew Kambugu. We thank the PhD program mentorship support received from the Infectious Diseases Institute Capacity Building Unit, especially from Barbara D. Castelnuovo, Aidah Nanvuma, Stephen Okoboi, and the Statistics unit, especially from Agnes Kiragga. John Hopkins University, School of Medicine and Bloomberg School of Public Health, Department of Molecular Microbiology, and Immunology, especially from Robert R. Bollinger and Arturo Casadevall. University of Colorado, Denver, Anschutz Medical Campus Aurora, Division of Infectious Disease, Department of Medicine and Veteran Affairs Research Services especially from Brent Palmer, Tina Powell, and Jeremy Rakhola. We thank the statistical data analysis mentorship received from the University of Minnesota, especially from Ananta S. Bangdiwala and Kathy Hullsiek Huppler.
Conflict of interest
The authors declare that the research was conducted in the absence of any commercial or financial relationships that could be construed as a potential conflict of interest.
Publisher’s note
All claims expressed in this article are solely those of the authors and do not necessarily represent those of their affiliated organizations, or those of the publisher, the editors and the reviewers. Any product that may be evaluated in this article, or claim that may be made by its manufacturer, is not guaranteed or endorsed by the publisher.
Supplementary material
The Supplementary Material for this article can be found online at: https://www.frontiersin.org/articles/10.3389/fimmu.2023.1275443/full#supplementary-material
Supplementary Table 1 | Reported gender-Associated Survival Among People with HIV-Associated Cryptococcal Meningitis.
Supplementary Figure 1 | Spearman’s correlation of regulatory elements IL-10 and PD-L1 by gender among multivariate predictors of gender-specific survival among females and males who died on antifungal treatment. A-C correlation of IL-10 with A - CCL11/Eotaxin, B - CXCL10/IP-10, and C - IL-15 among females and males who died on antifungal treatment, (i - ii, respectively). D – correlation of IL-8 with IL-15 among females and males who died on antifungal treatment. E-H – correlation of PD-L1 with E – IL12p70, F – IL-15, G – CXCL10, and H – CCL11 among females and males who died on antifungal treatment (i - ii), respectively). Notable, among females and males who survived, the responses were similar and positively correlated across the panels.
References
1. Wake RM, Govender NP, Omar T, Nel C, Mazanderani AH, Karat AS, et al. Cryptococcal-related mortality despite fluconazole preemptive treatment in a cryptococcal antigen screen-and-treat program. Clin Infect Diseases (2020) 70(8):1683–90. doi: 10.1093/cid/ciz485
2. Rhein J, Huppler Hullsiek K, Tugume L, Nuwagira E, Mpoza E, Evans EE, et al. Adjunctive sertraline for HIV-associated cryptococcal meningitis: a randomised, placebo-controlled, double-blind phase 3 trial. Lancet Infect Diseases (2019) 19(8):843–51. doi: 10.1016/S1473-3099(19)30127-6
3. Hakyemez IN, Erdem H, Beraud G, Lurdes M, Silva-Pinto A, Alexandru C, et al. Prediction of unfavorable outcomes in cryptococcal meningitis: results of the multicenter Infectious Diseases International Research Initiative (ID-IRI) cryptococcal meningitis study. Eur J Clin Microbiol Infect Diseases (2018) 37(7):1231–40. doi: 10.1007/s10096-017-3142-1
4. Tenforde MW, Jarvis JN. HIV-associated cryptococcal meningitis: ongoing challenges and new opportunities. Lancet Infect Diseases (2019) 19(8):793–4. doi: 10.1016/S1473-3099(19)30295-6
5. Stott KE, Loyse A, Jarvis JN, Alufandika M, Harrison TS, Mwandumba HC, et al. Cryptococcal meningoencephalitis: time for action. Lancet Infect Diseases (2021) 21(9):e259–71. doi: 10.1016/S1473-3099(20)30771-4
6. Boulware DR, von Hohenberg M, Rolfes MA, Bahr NC, Rhein J, Akampurira A, et al. Human immune response varies by the degree of relative cryptococcal antigen shedding. Open Forum Infect Diseases (2016) 3(1):1–7. doi: 10.1093/ofid/ofv194
7. Stadelman AM, Ssebambulidde K, Tugume L, Pastick KA, Hullsiek KH, Lofgren S, et al. Impact of biological sex on cryptococcal meningitis mortality in Uganda and South Africa. Med Mycol (2021) 59(7):712–9. doi: 10.1093/mmy/myaa108
8. Rajasingham R, Smith RM, Park BJ, Jarvis JN, Govender NP, Chiller TM, et al. Global burden of disease of HIV-associated cryptococcal meningitis: an updated analysis. Lancet Infect Diseases (2017) 17(8):873–81. doi: 10.1016/S1473-3099(17)30243-8
9. Boulware DR, Meya DB, Muzoora C, Rolfes MA, Huppler Hullsiek K, Musubire A, et al. Timing of antiretroviral therapy after diagnosis of cryptococcal meningitis. New Engl J Med (2014) 370(26):2487–98. doi: 10.1056/nejmoa1312884
10. Kambugu A, Meya DB, Rhein J, O’Brien M, Janoff EN, Ronald AR, et al. Outcomes of cryptococcal meningitis in Uganda before and after the availability of highly active antiretroviral therapy. Clin Infect Diseases (2008) 46(11):1694–701. doi: 10.1086/587667
11. Casadevall A, Pirofski La. The damage-response framework of microbial pathogenesis. Nat Rev Microbiol (2003) 1(1):17–24. doi: 10.1038/nrmicro732
12. Casadevall A, Pirofski LA. Host-pathogen interactions: Basic concepts of microbial commensalism, colonization, infection, and disease. Infect Immunity (2000) 68(12):6511–8. doi: 10.1128/IAI.68.12.6511-6518.2000
13. Nielsen K, Cox GM, Litvintseva AP, Mylonakis E, Malliaris SD, Benjamin DK, et al. Cryptococcus neoformans α strains preferentially disseminate to the central nervous system during coinfection. Infect Immunity (2005) 73(8):4922–33. doi: 10.1128/IAI.73.8.4922-4933.2005
14. Santiago-Tirado FH, Onken MD, Cooper JA, Klein RS, Doering TL. Trojan horse transit contributes to blood-brain barrier crossing of a eukaryotic pathogen. mBio (2017) 8(1). doi: 10.1128/mBio.02183-16
15. Okurut S, Boulware DR, Olobo J, Meya DB. Landmark clinical observations and immunopathogenesis pathways linked to HIV and Cryptococcus fatal central nervous system co-infection. Mycoses (2020) 63(8):840–53. doi: 10.1111/myc.13122
16. Meya DB, Okurut S, Zziwa G, Rolfes MA, Kelsey M, Cose S, et al. Cellular immune activation in cerebrospinal fluid from Ugandans with cryptococcal meningitis and immune reconstitution inflammatory syndrome. J Infect Diseases (2015) 211(10):1597–606. doi: 10.1093/infdis/jiu664
17. Okurut S, Meya DB, Bwanga F, Olobo J, Eller MA, Cham-Jallow F, et al. B cell compartmentalization in blood and cerebrospinal fluid of HIV-infected Ugandans with cryptococcal meningitis. Infect Immunity (2020) 88(3):1–14. doi: 10.1128/IAI.00779-19
18. Elsegeiny W, Marr KA, Williamson PR. Immunology of cryptococcal infections: developing a rational approach to patient therapy. Front Immunol (2018) 9:651. doi: 10.3389/fimmu.2018.00651
19. Chang CC, Omarjee S, Lim A, Spelman T, Gosnell BI, Carr WH, et al. Chemokine levels and chemokine receptor expression in the blood and the cerebrospinal fluid of HIV-infected patientswith cryptococcal meningitis and cryptococcosis- Associated immune reconstitution inflammatory syndrome. J Infect Diseases (2013) 208(10):1604–12. doi: 10.1093/infdis/jit388
20. Perera PY, Lichy JH, Waldmann TA, Perera LP. The role of interleukin-15 in inflammation and immune responses to infection: Implications for its therapeutic use. Microbes Infect (2012) 14(3):247–61. doi: 10.1016/j.micinf.2011.10.006
21. Esher SK, Zaragoza O, Alspaugh JA. Cryptococcal pathogenic mechanisms: A dangerous trip from the environment to the brain. Memorias do Instituto Oswaldo Cruz (2018) 113(7):1–15. doi: 10.1590/0074-02760180057
22. Mora DJ, Fortunato LR, Andrade-Silva LE, Ferreira-Paim K, Rocha IH, Vasconcelos RR, et al. Cytokine profiles at admission can be related to outcome in AIDS patients with cryptococcal meningitis. PloS One (2015) 10(3):1–17. doi: 10.1371/journal.pone.0120297
23. Xu XG, Pan WH, Bi XL, Fang W, Chen M, Zhu Y, et al. Comparison of clinical features in patients with persistent and nonpersistent cryptococcal meningitis: Twelve years of clinical experience in four centers in China. CNS Neurosci Ther (2013) 19(8):625–31. doi: 10.1111/cns.12135
24. Rhein J, Hullsiek KH, Tugume L, Mpoza E, Evans EE, Kiggundu R, et al. Adjunctive sertraline for HIV-associated cryptococcal meningitis: a randomised, placebo-controlled, double-blind phase 3 trial. Adjunctive sertraline for HIV-associated cryptococcal meningitis: a randomised, placebo-controlled, double-blind phase 3 trial (2020) 19(8):843–51. doi: 10.1016/S1473-3099(19)30127-6
25. Rolfes MA, Hullsiek KH, Rhein J, Nabeta HW, Taseera K, Schutz C, et al. The effect of therapeutic lumbar punctures on acute mortality from cryptococcal meningitis. Clin Infect Diseases (2014) 59(11):1607–14. doi: 10.1093/cid/ciu596
26. Jarvis JN, Bicanic T, Loyse A, Namarika D, Jackson A, Nussbaum JC, et al. Determinants of mortality in a combined cohort of 501 patients with HIV-associated cryptococcal meningitis: Implications for improving outcomes. Clin Infect Diseases (2014) 58(5):736–45. doi: 10.1093/cid/cit794
27. Lawrence DS, Leeme T, Mosepele M, Harrison TS, Seeley J, Jarvis JN. Equity in clinical trials for hiv-associated cryptococcal meningitis: A systematic review of global representation and inclusion of patients and researchers. PloS Negl Trop Diseases (2021) 15(5):1–19. doi: 10.1371/journal.pntd.0009376
28. Pappas PG, Bustamante B, Ticona E, Hamill RJ, Johnson PC, Reboli A, et al. Recombinant interferon-γ1b as adjunctive therapy for AIDS-related acute cryptococcal meningitis. J Infect Diseases (2004) 189(12):2185–91. doi: 10.1086/420829
29. Boulware DR, Bonham SC, Meya DB, Wiesner DL, Park GS, Kambugu A, et al. Paucity of initial cerebrospinal fluid inflammation in cryptococcal meningitis is associated with subsequent immune reconstitution inflammatory syndrome. J Infect Diseases (2010) 202(6):962–70. doi: 10.1086/655785
30. Rajasingham R, Rhein J, Klammer K, Musubire A, Nabeta H, Akampurira A, et al. Epidemiology of meningitis in an HIV-infected Ugandan cohort. Am J Trop Med Hygiene (2015) 92(2):274–9. doi: 10.4269/ajtmh.14-0452
31. George IA, Santos CAQQ, Olsen MA, Powderly WG. Epidemiology of cryptococcosis and cryptococcal meningitis in a large retrospective cohort of patients after solid organ transplantation. Open Forum Infect Diseases (2017) 4(1):1–7. doi: 10.1093/ofid/ofx004
32. Rhein J, Morawski BM, Hullsiek KH, Nabeta HW, Kiggundu R, Tugume L, et al. Associated Cryptococcal Meningitis: an open-label dose-ranging study. Lancet Infect Dis (2017) 16(7):809–18. doi: 10.1016/S1473-3099(16)00074-8.Efficacy
33. Kashef Hamadani BH, Franco-Paredes C, McCollister B, Shapiro L, Beckham JD, Henao-Martínez AF. Cryptococcosis and cryptococcal meningitis: New predictors and clinical outcomes at a United States academic medical centre. Mycoses (2018) 61(5):314–20. doi: 10.1111/myc.12742
34. Meya DB, Kiragga AN, Nalintya E. Reflexive laboratory-based cryptococcal antigen screening and preemptive fluconazole therapy for cryptococcal antigenemia in HIV-infected individuals with …. J acquired … (2019) 80(2):182–9. doi: 10.1097/QAI.0000000000001894
35. Pastick KA, Bangdiwala AS, Abassi M, Flynn AG, Morawski BM, Musubire AK, et al. Seizures in human immunodeficiency virus-associated cryptococcal meningitis: predictors and outcomes. Open Forum Infect Diseases (2019) 6(11):1–7. doi: 10.1093/ofid/ofz478
36. Lakoh S, Rickman H, Sesay M, Kenneh S, Burke R, Baldeh M, et al. Prevalence and mortality of cryptococcal disease in adults with advanced HIV in an urban tertiary hospital in Sierra Leone: A prospective study. BMC Infect Diseases (2020) 20(1):1–7. doi: 10.1186/s12879-020-4862-x
37. Marr KA, Sun Y, Spec A, Lu N, Panackal A, Bennett J, et al. A multicenter, longitudinal cohort study of cryptococcosis in human immunodeficiency virus-negative people in the United States. Clin Infect Diseases (2020) 70(2):252–61. doi: 10.1093/cid/ciz193
38. Lee WJ, Ryu YJ, Moon J, Lee ST, Jung KH, Park KI, et al. Enlarged periventricular space and periventricular lesion extension on baseline brain MRI predicts poor neurological outcomes in cryptococcus meningoencephalitis. Sci Rep (2021) 11(1):1–11. doi: 10.1038/s41598-021-85998-6
39. Kalata N, Ellis J, Kanyama C, Kuoanfank C, Temfack E, Mfinanga S, et al. Short-term Mortality outcomes of HIV-Associated cryptococcal meningitis in antiretroviral therapy-naïve and -experienced patients in sub-saharan Africa. Open Forum Infect Dis (2021) 8(10):1–5. doi: 10.1093/ofid/ofab397
40. Mansoor Ae, Thompson J, Sarwari AR. Delays in lumbar puncture are independently associated with mortality in cryptococcal meningitis: a nationwide study. Infect Diseases (2021) 53(5):361–9. doi: 10.1080/23744235.2021.1889656
41. Deiss R, Loreti CV, Gutierrez AG, Filipe E, Tatia M, Issufo S, et al. High burden of cryptococcal antigenemia and meningitis among patients presenting at an emergency department in Maputo, Mozambique. PloS One (2021) 16(4 April):1–11. doi: 10.1371/journal.pone.0250195
42. Zhao T, Xu XL, Nie JM, Chen XH, Jiang ZS, Liu SQ, et al. Establishment of a novel scoring model for mortality risk prediction in HIV-infected patients with cryptococcal meningitis. BMC Infect Diseases (2021) 21(1):1–10. doi: 10.1186/s12879-021-06417-9
43. Jarvis JN, Lawrence DS, Meya DB, Kagimu E, Kasibante J, Mpoza E, et al. Single-dose liposomal amphotericin B treatment for cryptococcal meningitis. New Engl J Med (2022) 386(12):1109–20. doi: 10.1056/nejmoa2111904
44. Baldassarre R, Mdodo R, Omonge E, et al. Mortality after clinical management of aids-associated cryptococcal meningitis in Kenya. East Afr Med J (2014) 91(5):145–51.
45. Furman D, Hejblum BP, Simon N, Jojic V, Dekker CL, Thiebaut R, et al. Systems analysis of sex differences reveals an immunosuppressive role for testosterone in the response to influenza vaccination. Proc Natl Acad Sci U S A (2014) 111(2):869–74. doi: 10.1073/pnas.1321060111
46. Fink AL, Engle K, Ursin RL, Tang WY, Klein SL. Biological sex affects vaccine efficacy and protection against influenza in mice. Proc Natl Acad Sci U S A (2018) 115(49):12477–82. doi: 10.1073/pnas.1805268115
47. Garelnabi M, Taylor-Smith LM, Bielska E, Hall RA, Stones D, May RC. Quantifying donor-to-donor variation in macrophage responses to the human fungal pathogen Cryptococcus neoformans. PloS One (2018) 13(3):1–12. doi: 10.1371/journal.pone.0194615
48. Rhein J, Morawski BM, Hullsiek KH, Nabeta HW, Kiggundu R, Tugume L, et al. Efficacy of adjunctive sertraline for the treatment of HIV-associated cryptococcal meningitis: an open-label dose-ranging study. Lancet Infect Diseases (2016) 16(7):809–18. doi: 10.1016/S1473-3099(16)00074-8
49. Vidal JE, Toniolo C, Paulino A, Colombo AL, Martins MDA, Meira CS, et al. Performance of cryptococcal antigen lateral flow assay in serum, cerebrospinal fluid, whole blood, and urine in HIV-infected patients with culture-proven cryptococcal meningitis admitted at a Brazilian referral center. Rev do Instituto Medicina Trop Sao Paulo (2018) 60:e1–1. doi: 10.1590/s1678-9946201860001
50. Rajasingham R, Wake RM, Beyene T, Katende A, Letang E, Boulware DR. Cryptococcal meningitis diagnostics and screening in the era of point-of-care laboratory testing. J Clin Microbiol (2019) 57(1):e01238-18. doi: 10.1128/JCM.01238-18
51. Vazirinejad R, Ahmadi Z, Arababadi MK, Hassanshahi G, Kennedy D. The biological functions, structure and sources of CXCL10 and its outstanding part in the pathophysiology of multiple sclerosis. NeuroImmunoModulation (2014) 21(6):322–30. doi: 10.1159/000357780
52. Michlmayr D, McKimmie CS. Role of CXCL10 in central nervous system inflammation. Int J Interferon Cytokine Mediator Res (2014) 6(1):1–18. doi: 10.2147/IJICMR.S35953
53. Teixeira AL, Gama CS, Rocha NP, Teixeira MM. Revisiting the role of eotaxin-1/CCL11 in psychiatric disorders. Front Psychiatry (2018) 9:241(JUN). doi: 10.3389/fpsyt.2018.00241
54. Parajuli B, Horiuchi H, Mizuno T, Takeuchi H, Suzumura A. CCL11 enhances excitotoxic neuronal death by producing reactive oxygen species in microglia. Glia (2015) 63(12):2274–84. doi: 10.1002/glia.22892
55. Mamik MK, Ghorpade A. Chemokine CXCL8 promotes HIV-1 replication in human monocyte-derived macrophages and primary microglia via nuclear factor-κB pathway. PloS One (2014) 9(3):1–13. doi: 10.1371/journal.pone.0092145
56. Chauhan P, Lokensgard JR. Glial cell expression of PD-L1. Int J Mol Sci (2019) 20(7):1–12. doi: 10.3390/ijms20071677
57. Burmeister AR, Marriott I. The interleukin-10 family of cytokines and their role in the CNS. Front Cell Neurosci (2018) 12:458(November). doi: 10.3389/fncel.2018.00458
58. Ringnér M. What is principal component analysis? Nat Biotechnol (2008) 26(3):303–4. doi: 10.1038/nbt0308-303
59. Giuliani A. The application of principal component analysis to drug discovery and biomedical data. Drug Discovery Today (2017) 22(7):1069–76. doi: 10.1016/j.drudis.2017.01.005
60. Scriven JE, Graham LM, Schutz C, Scriba TJ, Wilkinson KA, Wilkinson RJ, et al. A glucuronoxylomannan-associated immune signature, characterized by monocyte deactivation and an increased interleukin 10 level, is a predictor of death in cryptococcal meningitis. J Infect Diseases (2016) 213(11):1725–34. doi: 10.1093/infdis/jiw007
61. Gerstein AC, Jackson KM, McDonald TR, Wang Y, Lueck BD, Bohjanen S, et al. Identification of pathogen genomic differences that impact human immune response and disease during cryptococcus neoformans infection. mBio (2019) 10(4):1–22. doi: 10.1128/mBio.01440-19
62. Traino K, Snow J, Ham L, Summers A, Segalà L, Shirazi T, et al. HIV-negative cryptococcal meningoencephalitis results in a persistent frontal-subcortical syndrome. Sci Rep (2019) 9(1):1–9. doi: 10.1038/s41598-019-54876-7
63. Vanherp L, Poelmans J, Weerasekera A, Hillen A, Croitor-Sava AR, Sorrell TC, et al. Trehalose as quantitative biomarker for in vivo diagnosis and treatment follow-up in cryptococcomas. Trans research: J Lab Clin Med (2021) 230:111–22. doi: 10.1016/j.trsl.2020.11.001
64. Leite AGB, Vidal JE, Bonasser Filho F, Nogueira RS, de Oliveira ACP. Cerebral infarction related to cryptococcal meningitis in an HIV-infected patient: case report and literature review. Braz J Infect Dis (2004) 8(2):175–9. doi: 10.1590/S1413-86702004000200008
65. Orsini J, Blaak C, Mahmoud D, Young-Gwang J. Massive cerebral edema resulting in brain death as a complication of cryptococcus neoformans meningitis. J Community Hosp Internal Med Perspectives (2015) 5(1):3–6. doi: 10.3402/jchimp.v5.26098
66. Jarvis JN, Meintjes G, Bicanic T, Buffa V, Hogan L, Mo S, et al. Cerebrospinal fluid cytokine profiles predict risk of early mortality and immune reconstitution inflammatory syndrome in HIV-associated cryptococcal meningitis. PloS Pathogens (2015) 11(4):1–17. doi: 10.1371/journal.ppat.1004754
67. Jarvis JN, Casazza JP, Stone HH, Meintjes G, Lawn SD, Levitz SM, et al. The phenotype of the cryptococcus-specific CD4+ memory T-cell response is associated with disease severity and outcome in HIV-associated cryptococcal meningitis. J Infect Diseases (2013) 207(12):1817–28. doi: 10.1093/infdis/jit099
68. Qiao X, Zhang W, Zhao W. Role of CXCL10 in spinal cord injury. Int J Med Sci (2022) 19(14):2058–70. doi: 10.7150/ijms.76694
69. Teixeira AL, Gama CS, Rocha NP, Teixeira MM. Revisiting the role of eotaxin-1/CCL11 in psychiatric disorders. Front Psychiatry (2018) 9:241. doi: 10.3389/fpsyt.2018.00241
70. Okafor EC, Hullsiek KH, Williams DA, Scriven JE, Rhein J, Nabeta HW, et al. Correlation between blood and CSF compartment cytokines and chemokines in subjects with cryptococcal meningitis. Mediators Inflammation (2020) 2020. doi: 10.1155/2020/8818044
71. Boulware DR, Meya DB, Bergemann TL, Wiesner DL, Rhein J, Musubire A, et al. Clinical features and serum biomarkers in HIV immune reconstitution inflammatory syndrome after cryptococcal meningitis: A prospective cohort study. PloS Med (2010) 7(12):1–14. doi: 10.1371/journal.pmed.1000384
72. Lobo-Silva D, Carriche GM, Castro AG, Roque S, Saraiva M. Balancing the immune response in the brain: IL-10 and its regulation. J Neuroinflamm (2016) 13(1):1–10. doi: 10.1186/s12974-016-0763-8
73. Wake RM, Govender NP, Omar T, Nel C, Mazanderani AH, Karat AS, et al. Cryptococcal-related mortality despite fluconazole pre-emptive treatment in a cryptococcal antigen (CrAg) screen-and-treat programme. Clinical infectious diseases : an official publication of the Infectious Diseases Society of America (2020) 70(8):1683–90. doi: 10.1093/cid/ciz485
74. Meya D, Okurut S, Zziwa G, Cose S, Bohjanen P, Mayanja-Kizza H, et al. Monocyte phenotype and IFN-γ-inducible cytokine responses are associated with cryptococcal immune reconstitution inflammatory syndrome. J Fungi (2017) 3(2):28. doi: 10.3390/jof3020028
75. Meya DB, Okurut S, Zziwa G, Cose S, Boulware DR, Janoff EN. HIV-associated cryptococcal immune reconstitution inflammatory syndrome is associated with aberrant T cell function and increased cytokine responses. J Fungi (Basel Switzerland) (2019) 5(2):42. doi: 10.3390/jof5020042
76. Bickel M. The role of interleukin-8 in inflammation and mechanisms of regulation. J Periodontol (1993) 64(5 Suppl):456–60. Available at: http://www.ncbi.nlm.nih.gov/pubmed/8315568
77. Ehrlich LC, Hu S, Sheng WS, Sutton RL, Rockswold GL, Peterson PK, et al. Cytokine regulation of human microglial cell IL-8 production. J Immunol (Baltimore Md: 1950) (1998) 160(4):1944–8. doi: 10.4049/jimmunol.160.4.1944
78. Rosales C. Neutrophil: A cell with many roles in inflammation or several cell types? Front Physiol (2018) 9(FEB):1–17. doi: 10.3389/fphys.2018.00113
79. Guimarães-Costa AB, Nascimento MTC, Wardini AB, Pinto-Da-Silva LH, Saraiva EM. ETosis: A microbicidal mechanism beyond cell death. J Parasitol Res (2012) 2012(Table 1). doi: 10.1155/2012/929743
80. Musubire AK, Meya DB, Rhein J, Meintjes G, Bohjanen PR, Nuwagira E, et al. Blood neutrophil counts in HIV-infected patients with cryptococcal meningitis: Association with mortality. PloS One (2018) 13(12):e0209337. doi: 10.1371/journal.pone.0209337
81. Grahl N, Shepardson KM, Chung D, Cramer RA. Hypoxia and fungal pathogenesis: To air or not to air? Eukaryotic Cell (2012) 11(5):560–70. doi: 10.1128/EC.00031-12
82. Diehl JW, Hullsiek KH, Okirwoth M, Stephens N, Abassi M, Rhein J, et al. Cerebral oximetry for detecting high-mortality risk patients with cryptococcal meningitis. Open Forum Infectious Diseases (2017) 5(6):1–7. doi: 10.1093/ofid/ofy105
83. Shimoda Y, Ohtomo S, Arai H, Ohtoh T, Tominaga T. Subarachnoid small vein occlusion due to inflammatory fibrosis—a possible mechanism for cerebellar infarction in cryptococcal meningoencephalitis: a case report. BMC Neurol (2017) 17(1):157. doi: 10.1186/s12883-017-0934-y
84. Hung CW, Chang WN, Kung CT, Tsai NW, Wang HC, Lin WC, et al. Predictors and long-term outcome of seizures in human immuno-deficiency virus (HIV)-negative cryptococcal meningitis. BMC Neurol (2014) 14(1):1–8. doi: 10.1186/s12883-014-0208-x
85. Saito T, Saido TC. Neuroinflammation in mouse models of Alzheimer’s disease. Clin Exp Neuroimmunol (2018) 9(4):211–8. doi: 10.1111/cen3.12475
86. Lu Y, Ma C, Chen R, Hu Z, Yao H, Zhang Q, et al. Development and validation of a new scoring system for the early diagnosis of tuberculous meningitis in adults. Diagn Microbiol Infect Disease (2021) 101(2):115393. doi: 10.1016/j.diagmicrobio.2021.115393
87. D’Inzeo T, Menchinelli G, De Angelis G, Fiori B, Liotti FM, Morandotti GA, et al. Implementation of the eazyplex® CSF direct panel assay for rapid laboratory diagnosis of bacterial meningitis: 32-month experience at a tertiary care university hospital. Eur J Clin Microbiol Infect Diseases (2020) 39(10):1845–53. doi: 10.1007/s10096-020-03909-5
88. Sokol CL, Luster AD. The chemokine system in innate immunity. Cold Spring Harbor Perspect Biol (2015) 7(5):1–20. doi: 10.1101/cshperspect.a016303
89. Veenstra M, León-Rivera R, Li M, Gama L, Clements JE, Berman JW. Mechanisms of CNS viral seeding by HIV+ CD14+ CD16+ monocytes: Establishment and reseeding of viral reservoirs contributing to HIV-associated neurocognitive disorders. mBio (2017) 8(5):1–15. doi: 10.1128/mBio.01280-17
90. Persidsky Y, Poluektova L. Immune privilege and HIV-1 persistence in the CNS. Immunolog Rev (2006) 213:180–94. doi: 10.1111/j.1600-065X.2006.00440.x
91. Panackal AA, Wuest SC, Lin YC, Wu T, Zhang N, Kosa P, et al. Paradoxical immune responses in non-HIV cryptococcal meningitis. PloS Pathogens (2015) 11(5):1–27. doi: 10.1371/journal.ppat.1004884
92. Kawakami K, Qureshi MH, Koguchi Y, Nakajima K, Saito A. Differential effect of Cryptococcus neoformans on the production of IL-12p40 and IL-10 by murine macrophages stimulated with lipopolysaccharide and gamma interferon. FEMS Microbiol Lett (1999) 175(1):87–94. doi: 10.1016/S0378-1097(99)00172-X
93. Trinchieri G. Interleukin-12 and the regulation of innate resistance and adaptive immunity. Nat Rev Immunol (2003) 3(2):133–46. doi: 10.1038/nri1001
94. Kuwabara T, Ishikawa F, Kondo M, Kakiuchi T. The role of IL-17 and related cytokines in inflammatory autoimmune diseases. Mediators Inflammation (2017), 2017. doi: 10.1155/2017/3908061
95. Lee SC, Casadevall A, Dickson DW. Immunohistochemical localization of capsular polysaccharide antigen in the central nervous system cells in cryptococcal meningoencephalitis. Am J Pathol (1996) 148(4):1267–74.
96. Okurut S, Okafor E, Boulware RD, Rhein J, Manabe CY, Olobo OJ, et al. Divergent neuroimmune signatures and HIV-associated cryptococcal meningitis survival. Abstract and poster retrieved on July 28, 2023 from CROI webseite. 489_ePoster (2023). Available at: https://www.croiconference.org/abstract/divergent-neuroimmune-signatures-and-hiv-associated-cryptococcal-meningitis-survival/.
Keywords: cryptococcal meningitis, cerebrospinal fluid (CSF), neuroimmune responses, chemokine CXCL10, cytokine IL-15, CCL11/Eotaxin, gender/biological sex survival, antifungal treatment
Citation: Okurut S, Boulware DR, Okafor E, Rhein J, Kajumbula H, Bagaya BS, Bwanga F, Olobo JO, Manabe YC, Meya DB and Janoff EN (2023) Divergent neuroimmune signatures in the cerebrospinal fluid predict differential gender-specific survival among patients with HIV-associated cryptococcal meningitis. Front. Immunol. 14:1275443. doi: 10.3389/fimmu.2023.1275443
Received: 19 September 2023; Accepted: 20 November 2023;
Published: 13 December 2023.
Edited by:
Hrishikesh Pandit, National Heart, Lung, and Blood Institute (NIH), United StatesReviewed by:
Aghila Rani K.G, University of Sharjah, United Arab EmiratesLucia Alves Oliveira Fraga, Juiz de Fora Federal University, Brazil
Tinashe K. Nyazika, Liverpool School of Tropical Medicine, United Kingdom
Copyright © 2023 Okurut, Boulware, Okafor, Rhein, Kajumbula, Bagaya, Bwanga, Olobo, Manabe, Meya and Janoff. This is an open-access article distributed under the terms of the Creative Commons Attribution License (CC BY). The use, distribution or reproduction in other forums is permitted, provided the original author(s) and the copyright owner(s) are credited and that the original publication in this journal is cited, in accordance with accepted academic practice. No use, distribution or reproduction is permitted which does not comply with these terms.
*Correspondence: Samuel Okurut, b2t1cnV0c0BnbWFpbC5jb20=