- 1HIV and Viral Hepatitis Research Laboratory, Instituto de Investigación Sanitaria Fundación Jiménez Díaz, Universidad Autónoma de Madrid (IIS-FJD, UAM), Madrid, Spain
- 2Hospital Universitario Rey Juan Carlos, Móstoles, Spain
- 3Department of Oncology and Cancer Institute, Fundacion Jimenez Diaz University Hospital, Autonomous University, Madrid, Spain
The immune system of people living with HIV (PLWH) is persistently exposed to antigens leading to systemic inflammation despite combination antiretroviral treatment (cART). This inflammatory milieu promotes T-cell activation and exhaustion. Furthermore, it produces diminished effector functions including loss of cytokine production, cytotoxicity, and proliferation, leading to disease progression. Exhausted T cells show overexpression of immune checkpoint molecules (ICs) on the cell surface, including programmed cell death protein 1 (PD-1), cytotoxic T-lymphocyte-associated antigen-4 (CTLA-4), T-cell immunoglobulin and mucin-domain containing-3 (TIM-3), T-cell immunoglobulin and immunoreceptor tyrosine-based inhibitory motif domain (TIGIT), and lymphocyte activation gene-3 (LAG-3). The ICs also play a crucial role in T-cell exhaustion by reducing the immune response to cancer antigens. Immunotherapy based on immune checkpoint inhibitors (ICIs) has changed the management of a diversity of cancers. Additionally, the interest in exploring this approach in the setting of HIV infection has increased, including AIDS-defining cancers and non-AIDS-defining cancers in PLWH. To date, research on this topic suggests that ICI-based therapies in PLWH could be a safe and effective approach. In this review, we provide an overview of the current literature on the potential role of ICI-based immunotherapy not only in cancer remission in PLWH but also as a therapeutic intervention to restore immune response against HIV, revert HIV latency, and attain a functional cure for HIV infection.
Introduction
Combination antiretroviral treatment (cART) has significantly improved the immune status of people living with HIV (PLWH) and has dramatically reduced HIV morbidity and mortality, transforming HIV infection into a chronic disease (1). However, cART is not curative and PLWH require lifelong treatment due to the establishment of the HIV reservoir in long-lived CD4 T cells allowing the virus to persist in a quiescent state evading immune detection (2). After many years of research in HIV infection, there is an undeniable need for therapeutic strategies that can enhance antiviral immunity and reduce the viral reservoir that is critical for permanent HIV remission.
Long-term persistence of HIV has been associated with T-cell exhaustion, which consists of T-cell functional impairment with loss of cytokine production, cytotoxicity, proliferation (3), and consequent disease progression (3–5). Exhausted T cells are characterized by an overexpression of immune checkpoint molecules (ICs) on the T-cell surface such as programmed cell death protein 1 (PD-1), cytotoxic T-lymphocyte-associated antigen-4 (CTLA-4), T-cell immunoglobulin and mucin-domain containing-3 (TIM-3), T-cell immunoglobulin and immunoreceptor tyrosine-based inhibitory motif domain (TIGIT), lymphocyte activation gene-3 (LAG-3), CD160, and 2B4 (3, 6–8). Interestingly, Banga et al. have demonstrated that PD-1 expressing memory CD4 T cells from lymph nodes are the main source of infectious viruses in PLWH (9). Similarly, another study reported that in PLWH with uncontrolled viremia, CTLA-4 expressing CD4 T cells contain higher HIV DNA levels compared with their CTLA-4 negative CD4 T-cell counterparts (10). Fromentin et al. have shown that co-expression of immune checkpoint molecules such as PD-1, LAG-3, and TIGIT in CD4 T cells leads to HIV latency during ART regimen (11).
PLWH have an increased risk of developing multiple cancers due to co-infection with certain oncogenic viruses, certain unhealthy lifestyle behaviors, and persistent immune alterations despite cART. These cancers include AIDS-defining cancers, such as non-Hodgkin lymphoma, cervical cancer, or Kaposi sarcoma, as well as non-AIDS-defining cancers such as Hodgkin lymphomas, anal cancer, lung cancer, and hepatocellular carcinoma, among others (12). Furthermore, non-HIV-related hematological malignancies such as acute leukemia, multiple myeloma, and myeloproliferative neoplasms are emerging among PLWH (13).
ICs also play an important role in T-cell exhaustion in cancer patients by decreasing the immune response against cancer antigens (3, 14). Antibodies (Abs) that specifically block ICs, also named immune checkpoint inhibitors (ICIs), including anti-CTLA-4, anti-PD-1, and anti-PD-L1, are transforming cancer therapies by enhancing anti-cancer immunity. This leads to increased survival rates in patients with various types of cancers, even in PLWH. Considering the success of ICIs in cancer therapy and taking into account that T-cell exhaustion in cancer is similar to that observed in HIV infection, the potential role of ICI-based therapies in improving T-cell responses has gained interest. ICIs can be used in the control of HIV infection as an adjuvant to standard cART to improve HIV immune response and revert virus latency.
The relevance of ICI-based therapies, in both cancer and chronic infections including HIV, is reflected in recent reviews addressing various aspects of this field (15–20). In the present review, we give a general overview of the possible impact of ICI-based treatments for cancer remission in PLWH as well as its impact on the HIV-specific immunological response to revert T-cell exhaustion and HIV latency. ICIs could represent a novel form of latency reversion agents (LRAs): on one side reactivating HIV transcription from latently infected cells which will improve HIV recognition by the immune system, while also reinvigorating HIV-specific T-cell responses. Together, this could support the eradication of HIV-infected cells.
Immune checkpoint molecules and T-cell exhaustion in HIV infection
T-cell exhaustion has been described as a hallmark of several chronic infections. This includes HIV, in which antigens persist with deleterious effects on the proliferative capacity and effector functions of antigen-specific T cells. The immune system has mechanisms to ensure a specific and controlled response to antigens controlling T-cell hyperreactivity through the expression of ICs. This includes the most studied ICs, PD-1 and CTLA-4. Engagement of these molecules with their ligands provides signals counteracting the activation of T cells after T-cell receptor (TCR) stimulation (21, 22). These ICs are upregulated upon ongoing antigen exposure which contributes to a loss of proliferating capacity and reduced production of cytokines by T cells, therefore leading these cells to a state of immune exhaustion. In addition, some studies have shown that T-cell exhaustion also exhibits impaired memory as well as metabolic and transcriptional cell abnormalities (3, 23). Furthermore, it is recognized that exhaustion can occur in immune cell types other than CD8 T cells, such as CD4 T cells (23), NK cells (24), and B cells (25).
PD-1 and its ligand receptors, PD-L1/PD-L2, are the ICs most responsible for T-cell exhaustion in chronic infections. However, other ICs, such as CTLA-4, TIM-3, TIGIT, or LAG-3 can be expressed alone or in combination to cause pronounced T-cell exhaustion. Immune exhaustion is typically characterized by the co-expression of numerous ICs by T cells. Generally, the higher the number of co-expressed ICs by T cells, the more severe the exhaustion (3, 7). Several studies in HIV infection have shown an increased expression of ICs both in CD4 and CD8 T cells. This has been associated with reduced effector functions, less virus-cell clearance, declining CD4 T-cell numbers, and disease progression (4, 5, 8, 26–28).
Unquestionably, cART has significantly prolonged the life expectancy of PLWH. However, it has been noted that suppression of viral replication with cART does not revert the increased expression of ICs such as PD-1, TIM-3, or TIGIT (26, 29), even when cART is initiated in acute infection (30). Notably, in PLWH receiving cART, the expression of PD-1 on T cells is a predictor of viral rebound following cART interruption (31) and has been associated with poor recovery of CD4 T-cell counts (32, 33).
Immune checkpoint molecules and HIV persistence
The HIV reservoir remains the principal obstacle to achieving eradication of HIV infection despite successful cART. This is mainly due to the presence of resting long-lived memory CD4 T cells harboring persistent replication-competent viruses. The homeostatic proliferation of infected CD4 T cells also contributes to the replenishment of the viral reservoir (34). Interestingly, it has been suggested that T-cell exhaustion is another crucial factor for HIV persistence (35). Khoury et al. have reported a significant correlation between the frequency of PD-1 expression on CD4 T cells and HIV persistence in rectal and blood tissues in PLWH receiving cART (36). Moreover, it has been shown that CD4 T cells expressing PD-1 in blood and lymph nodes from cART-suppressed PLWH are an important source of viral latency (9, 37, 38).
Furthermore, it has been described that the expression of T-cell exhaustion markers, including PD-1 and LAG-3, during acute HIV infection prior to the use of cART, correlates with HIV reservoir size and can predict viral rebound after treatment interruption (31). Consistent with these results, other studies have demonstrated that blood CD4 T cells expressing PD-1 alone (39) or co-expressing LAG-3 and TIGIT (11) showed a high level of integrated HIV DNA. In a recent study, Horn et al. have described that high levels of HIV DNA and PD-1 expression on CD4 T-cell subsets persist in peripheral blood and the terminal ileum of PLWH despite cART (40). Similarly, it has been shown that double-positive PD-1+CTLA-4+ memory CD4 T cells from the blood of PLWH on cART have a high frequency of HIV DNA+ cells, favoring HIV persistence (41). There is still a great deal to be discovered about how ICs induce virus latency. Evans et al. have suggested that the inhibitory effects of ICs, such as PD-1, on T-cell activation lead to a reduction of HIV transcription and RNA translation, therefore promoting HIV latent infection (39). Similar results have been found for CTLA-4, with higher levels of HIV latency in CTLA-4+ than in CTLA-4- T cells. Furthermore, downmodulation of CTLA-4 expression on T cells induced by HIV-Nef protein results in transition from latent to productive infection of these cells, supporting the role of CTLA-4 as a modulator of HIV latency (10).
A glance at therapies based on blocking immune checkpoints molecules in cancer
Exhausted T cells have been found in several malignancies, which create an immunosuppressive environment in tumor tissues, similar to what happens in HIV infection (3). Dysfunctional anti-tumor T-cell responses have been linked to the elevated and sustained expression of numerous ICs (PD-1, CTLA-4, TIM-3, TIGIT, or LAG-3) in a variety of malignancies. These ICs, which regulate T-cell function through various pathways, induce tolerance, exhaustion, and produce inhibitory signals that reduce the immune response against tumor cells (3, 14, 42–44).
The discovery that T-cell exhaustion is a reversible phenomenon has made critical advances in cancer immunotherapy. Therapy based on the blockade of ICs to reinvigorate T-cell responses has revolutionized cancer treatment. ICIs are monoclonal antibodies (mAbs) directed against ICs expressed by immune cells that prevent the inhibition of anti-cancer T cells, primarily CD8 T cells, enhancing the specific responses to tumors. In the past few years, it has been shown how the administration of ICIs can reactivate the exhausted immune system and increase survival in patients with a wide variety of cancers (45).
Several molecules have been approved for the management of advanced malignancies, including non-small cell lung cancer (NSCLC), melanoma, and head and neck cancer, among others (46–49). Ipilimumab, an ICI that selectively targets CTLA-4 and has shown promising results in the treatment of melanoma, was the first ICI approved by the FDA for use in cancer immunotherapy (47). Other mAbs for cancer therapy have been approved, including anti-PD-1 (nivolumab, pembrolizumab, and cemiplimab) and anti-PD-L1 (atezolizumab, durvalumab, and avelumab), with anti-tumor activity in several types of cancer, such as melanoma, lung cancer, Hodgkin lymphoma, metastatic anal cancer, cutaneous squamous cell carcinoma, and breast cancer, among others (46, 49–53). Importantly, combined administration of anti-CLTA-4 and anti-PD-1 has demonstrated to be a successful tool for the therapy of several cancers (44, 54–57). Currently, there are next generation ICIs other than CTLA-4 and PD-1/PD-L1. One example is anti-LAG-3 (relatlimab) which has been recently approved for metastasic melanoma treatment in combination with nivolumab (58). Others such as anti-TIGIT or anti-TIM-3, as well as new combinations of ICIs, are being explored to potentiate anti-tumor immune response in different cancer types including solid tumors, multiple myeloma, endometrial cancer, and gastric cancer, among others (NCT02913313, NCT03119428, NCT02817633, NCT03099109, NCT02608268, NCT01968109).
The inhibiting activity of ICIs on molecules with an immune system regulatory function means that ICI therapies are not without risk of toxicity and may result in a range of immune-related adverse effects (irAEs). The most common irAEs occur in the skin, endocrine system, gut, and lungs. Severe grades of irAEs, such as pneumonitis, neurotoxicity, and cardiovascular and renal toxicity have been well described in cancer patients receiving ICIs. Most of these irAEs can be treated successfully with immunosuppressor drugs, including corticosteroids (59–61). If corticosteroids are ineffective, other treatments such as infliximab or vedolizumab have been evaluated to treat these ICI side effects (62, 63).
Another aspect to consider when using ICIs for cancer treatment is the safety of their use in patients with specific associated comorbidities, such as concomitant chronic infections (64, 65) or immune-related disorders (64, 66). Overall, there is consensus that cancer patients with different comorbidities may benefit from ICI treatment. Nevertheless, precautions should be taken to reduce the occurrence of major side effects in these patients. Additionally, these precautions vary depending on the specific comorbidity.
Therapeutic potential of immune checkpoint inhibitors in HIV infection
Given the success of immunotherapy in the treatment for cancer, ICI-based immunotherapy is an intriguing field that is gaining attention as a coadjuvant to improve the immune response to HIV infection. Unfortunately, there is no vaccine or cure for this viral infection, and as stated previously, T-cell exhaustion is a primary characteristic of chronic HIV infection. The unique therapeutic approach that has demonstrated a complete eradication of HIV has been hematopoietic stem cell transplantation. This has occurred in four HIV-infected individuals with hematological malignancies who received a hematopoietic stem cell transplantation from donors homozygous for CCR5Δ32 (67–70). However, hematopoietic stem cell transplantation has a high risk of morbidity and mortality, is too complex, costly, and would therefore be inaccessible as a worldwide therapeutic strategy for the eradication of HIV. The most realistic approach is the functional cure that focuses on developing immunotherapy to boost host immunity and achieve a complete suppression of virus replication without cART. Thus, blocking ICs as a strategy to reinvigorate HIV-specific T-cell responses has raised considerable interest as explained below.
Ex vivo and in vitro studies
Different ex vivo studies have demonstrated that PD-1/PD-L1 or CTLA-4 blockade in cultured cells from PLWH can reinvigorate the proliferative ability and functionality of HIV-specific CD4 and CD8 T cells (6, 27, 71). Similarly, blockade of PD-1 or TIM-3 molecules restores cytokine production by HIV-specific CD8 T cells (4, 26, 29). Porichis et al. have shown that PD-1 blockade along with IL-10 treatment reinvigorated CD4 T cells and improved NK function, suggesting that inhibition of T-cell exhaustion can reinvigorate not only adaptive but also innate immune responses in HIV (72). Holder et al. reported that treating NK cells from PLWH with anti-TIGIT mAbs increased NK cell activity against HIV-infected CD4 T cells (73). Moreover, a very recent ex vivo study using cells from PLWH on ART has demonstrated that the combined blockade of multiple ICs, including PD-1, PD-L1, LAG-3, CTLA-4, TIM-3, and TIGIT, had a synergistic effect increasing the frequency of HIV-specific CD4 and CD8 T-cell responses when compared with the blockade of a single IC (74).
Brunet-Ratnasingh et al. measured the ability of different HIV-specific CD4 T-cell subsets to respond to PD-L1 blockade and found that follicular helper T (Tfh) cells, previously described as an important source of replication-competent HIV (75), showed low responsiveness to PD-L1 blockade while Th1, Th17, and Th22 cells significantly increased their responsiveness (76). This finding highlights the importance of considering the different lineages of HIV-specific CD4 T cells to better evaluate the potential role of ICIs for reverting T-cell dysfunctionality in PLWH.
Another interesting effect of ICIs in PLWH is the reversion of HIV latency in CD4 T cells, as demonstrated in two ex vivo studies indicating that blocking the PD-1/PD-L1 pathways with anti-PD-1 antibodies enhanced viral production and resulted in a decrease in HIV latency (39, 77). Also, an in vitro study has demonstrated that when compared to other commonly used latency-reverting drugs such as vorinostat and bryostatin, the level of latency reversion following simultaneous blockade of different ICs such as PD-1, CTLA-4, TIGIT, and TIM-3 was much higher (78).
Preclinical studies
Studies in animal models of simian immunodeficiency virus (SIV) infection have demonstrated that PD-1 or PD-L1 blockade resulted in enhanced virus-specific CD8 T-cell effector functions (79, 80) and reduced viral load (79). In ART-treated SIV-infection, the PD-1 blockade resulted in a reduction of replication-competent virus reservoirs as well as increased expansion of CXCR5-expressing and granzyme-B-producing CD8 T cells. It also offered better control of SIV-viremia following ART interruption (81). Very recently, it has been reported that in vivo PD-1 blockade after ART interruption in SIV-infected macaques was able to: a) enhance SIV-specific CD8 T-cell functions; b) induce an expansion of memory CD4 and CD8 T cells and cytolytic NK cells; and c) control viremia after interruption of ART (82). Combinatorial approaches including blockade of PD-1 and LAG-3 (7) or PD-1 and TIM-3 (83) in mice have shown that these dual blockades were able to reinvigorate CD8 T-cell responses. Importantly, in ART-treated SIV-infection the dual CTLA-4/PD-1 blockade was able to reduce the SIV reservoir size (84). This result is in accordance with another study in which blockade of CTLA-4 led to increased viral replication at mucosal sites of SIV-infected macaques (85).
Overall, the above-mentioned studies provide evidence that the blockade of some ICs, such as anti-PD-1 and/or CTLA-4 could have the potential to reverse T-cell exhaustion by improving HIV-specific immune functionality and to revert HIV-latency in PLWH (Figure 1).
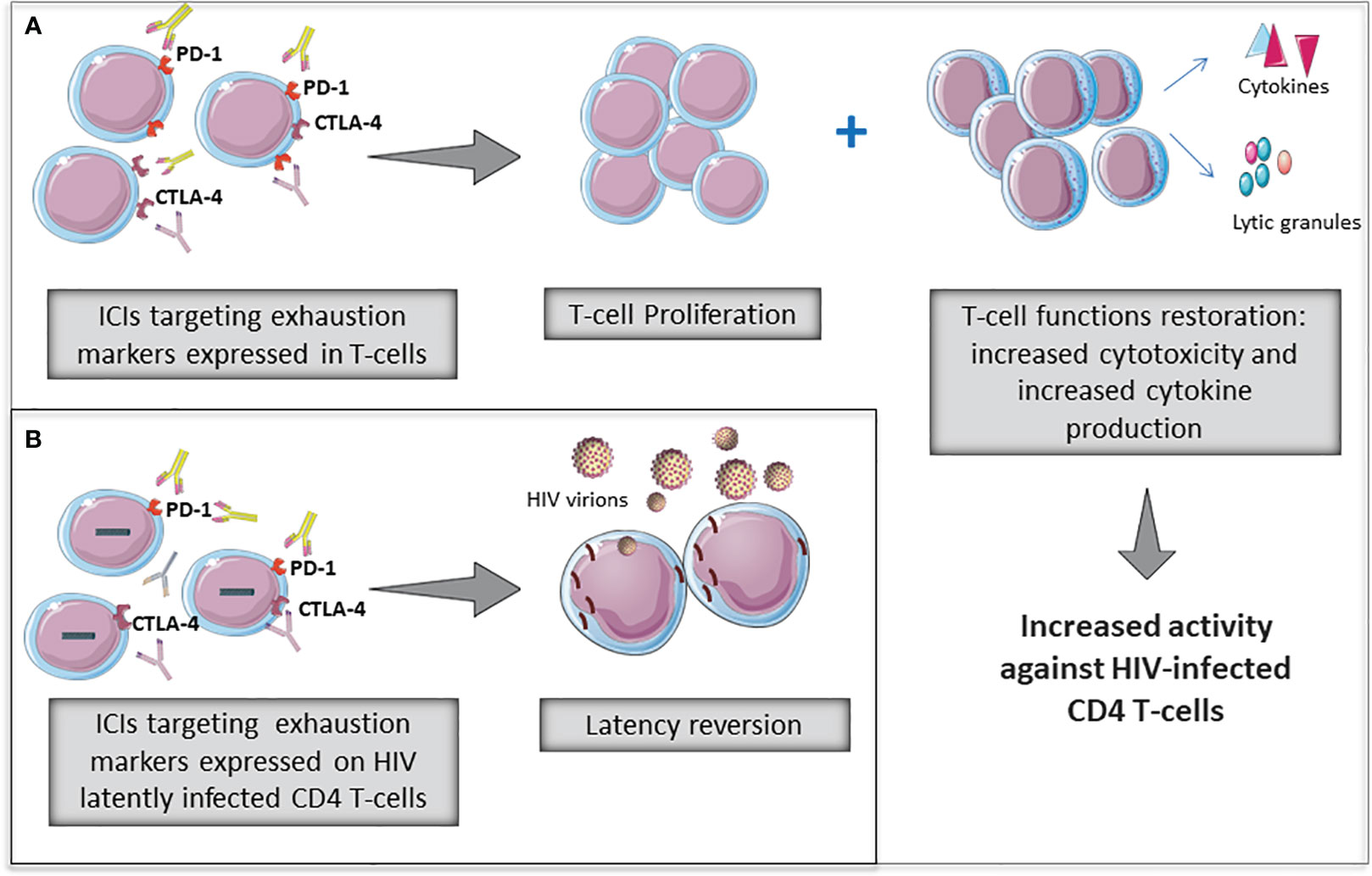
Figure 1 Potential role of immune checkpoint inhibitors (ICIs) to revert T-cell exhaustion (A) and to revert HIV latency (B) in PLWH. Some ICIs widely used in cancer therapies are being evaluated for restorating T-cell functions as well as reverting HIV-latency in PLWH.
Table 1 summarizes the ex vivo and in vivo findings reported to date focused on the role of immune checkpoint blockade in the setting of HIV/SIV infections.
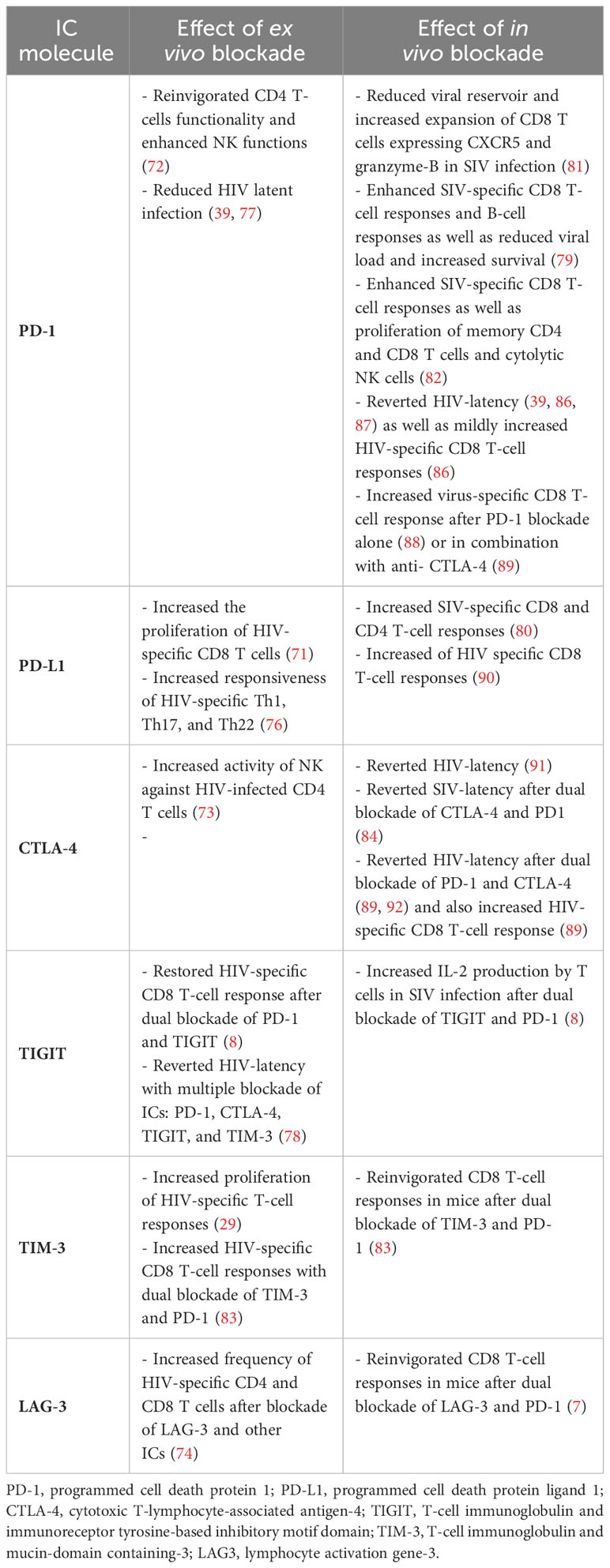
Table 1 Summary of ex vivo and in vivo studies reporting immune checkpoint blockade in the setting of HIV/SIV infections.
ICIs in PLWH with malignancies
The knowledge regarding ICI-based therapies could also be applied to PLWH with cancer, with the benefit that several ICIs have previously been established for cancer therapy with successful outcomes. However, most clinical trials studying ICIs in cancer patients have excluded patients with chronic infections such as HIV based on the concern that this population lacks sufficient T-cell immunity to benefit from therapy, as well as the risk of potential severe side effects associated with reestablishing HIV immunity with this therapy. Fortunately, accumulated experience to date from case series and clinical trials has shown that ICIs are safe in PLWH and that the incidence of adverse effects (irAEs) is not higher than in the general non-HIV-infected population, as discussed below. Only some of these studies have reported some incidence of irAEs in PLWH treated with ICIs, including hypothyroidism, pneumonitis, rash, uveitis, and allergic lung disease (93–95). Importantly, thyroiditis and autoimmune hepatitis were observed in a trial with anti-PD1, leading to the early termination of the trial (96).
Although the information about the benefits and safety of ICIs in the treatment of malignancies in PLWH is still scarce, there are some case series, retrospective cases, and cohort reports on PLWH diagnosed with malignancies and treated with ICIs. Two recent systematic reviews have summarized the case reports evaluating the safety and efficacy of ICIs in PLWH, as well as the impact in HIV progression markers in some of the studies (97, 98). Overall, the use of ICIs in PLWH was safe, and rates of adverse events were between 9% to 12%. These percentages were similar to those observed in cancer therapies in the HIV-negative population (97, 98). Regarding efficacy of ICIs against cancer in PLWH, it was highly variable depending on the tumor type, with response rates varying from 27% to 63% (97). In addition, the effect of ICIs on HIV disease markers was very modest, with 24% to 56% of patients showing increases in CD4 cell count (97, 98). In addition, data on HIV reservoirs and HIV-specific responses was available only in 7% (13/176) of PLWH of which 23% (3/13) showed improvement of HIV-specific CD8 T-cell response, and 15% (2/13) showed a decrease of HIV reservoir (98).
Interestingly, the most recent data from a retrospective study by El Zarif et al. have provided insight on the safety of ICIs among 390 PLWH with cancer that were treated with anti-PD1 or anti-PD-L1. This study demonstrated minimal ICI-related adverse events: 20% of patients had irAEs of any grade, and only 8% of patients had high grade irAEs. These data support that ICIs are safe to use in PLWH and can therefore benefit from this treatment (99).
Among the different ICIs, the most investigated in the context of PLWH with malignancies have been anti-PD-1 and anti-PD-L1. Several studies, ranging from case reports to multicenter cohorts, have demonstrated the safety of anti-PD-1 and anti-PD-L1 in PLWH suffering from different cancers. Idossa et al. and Alloghbi et al. reported three cases of PLWH on cART treated with anti-PD-1 for metastatic prostate cancer (treatment with pembrolizumab for two cases) and for advanced cutaneous squamous cell carcinoma (treatment with cemiplimab for one case), respectively. All patients responded to therapy and showed no major toxicities (100, 101).
Additionally, in two different case series studies of PLWH on cART and treated for a variety of malignancies, an excellent tolerability of anti-PD-1 and/or anti-PD-L1 therapy was observed, and all patients showed stable CD4 T-cell counts and no reactivation of HIV load during ICI therapy (93, 94). Similar results, with no evidence of ICI-related serious irAEs or negative effects on CD4 counts or viral load, were observed in two multicenter studies enrolling 20 and 30 PLWH on cART, respectively, one using anti-PD-1 (95) and the other anti-PD-L1 (102). Furthermore, a study by Galanina et al. showed an increase of CD4 T-cell counts in nine PLWH on cART who received anti-PD-1 for treating Kaposi sarcoma (103).
In addition, some of the case reports published to date have provided evidence that PLWH on cART who have received ICIs such as anti-CTLA-4 (91) or anti-PD-1 (39) for treatment of melanoma can display an increase in HIV transcription (measured by HIV-RNA in CD4 T cells), suggesting an in vivo effect of these therapies in the reversion of virus latency. Moreover, two different studies in PLWH on cART treated with anti-PD-1 for lung cancer showed not only a decrease in HIV reservoir size but also a decrease of CD4 and CD8 T-cell exhaustion with an increase in HIV-specific CD8 T-cell response (86, 87). Another case report in PLWH on cART with melanoma showed enhancement of HIV-specific CD8 T-cell response after treatment with the anti-PD-1 pembrolizumab (88). Interestingly, Lau et al. evaluated the impact on HIV-specific response and changes in HIV reservoir in three PLWH on cART after receiving repeated cycles of different ICIs (anti-PD-1, anti-PD-L1, anti-CTLA-4, or a combination of some of them) depending on their specific malignancy. These authors found that all participants showed some degree of HIV-latency reversion (evidenced by increased cell-associated unspliced HIV RNA). However, a decrease in the HIV reservoir was observed in only one patient, and an increase in virus-specific CD8 T-cell responses in another (89). On the other hand, Baron et al. reported a limited effect on the HIV reservoir using monotherapy with anti-PD-1 in PLWH cART with cancer, with a concomitant increase in expression of other ICs on T cells, suggesting the existence of compensatory mechanisms limiting the efficacy of ICI monotherapy (104). This suggests that combination therapy with several ICIs may be a better strategy to pursue a cure for HIV.
It is important to note that, currently, there are only a few clinical trials being carried out in PLWH with advanced-stage cancer (Table 2). All of these studies are in their early phases: the first is the AIDS Malignancy Consortium (AMC) 095 study, a phase I trial (NCT02408861) evaluating the safety and optimal dose of therapy with nivolumab (targeting PD-1) alone or in combination with ipilimumab (targeting CTLA-4) in PLWH on cART with advanced solid tumors. Interestingly, a substudy of this clinical trial showed that combination therapy with anti-PD-1 and anti-CTLA-4 in seven of the participants was able to revert HIV latency and in two patients was able to decrease cells containing replication-competent virus (as estimated by the quantitative viral outgrowth assay (QVOA)). These findings suggest a synergistic effect of dual therapy of ICIs on HIV latency reversion and support the hypothesis that combination therapy with several ICIs could be useful to significantly impact HIV reservoirs (92).
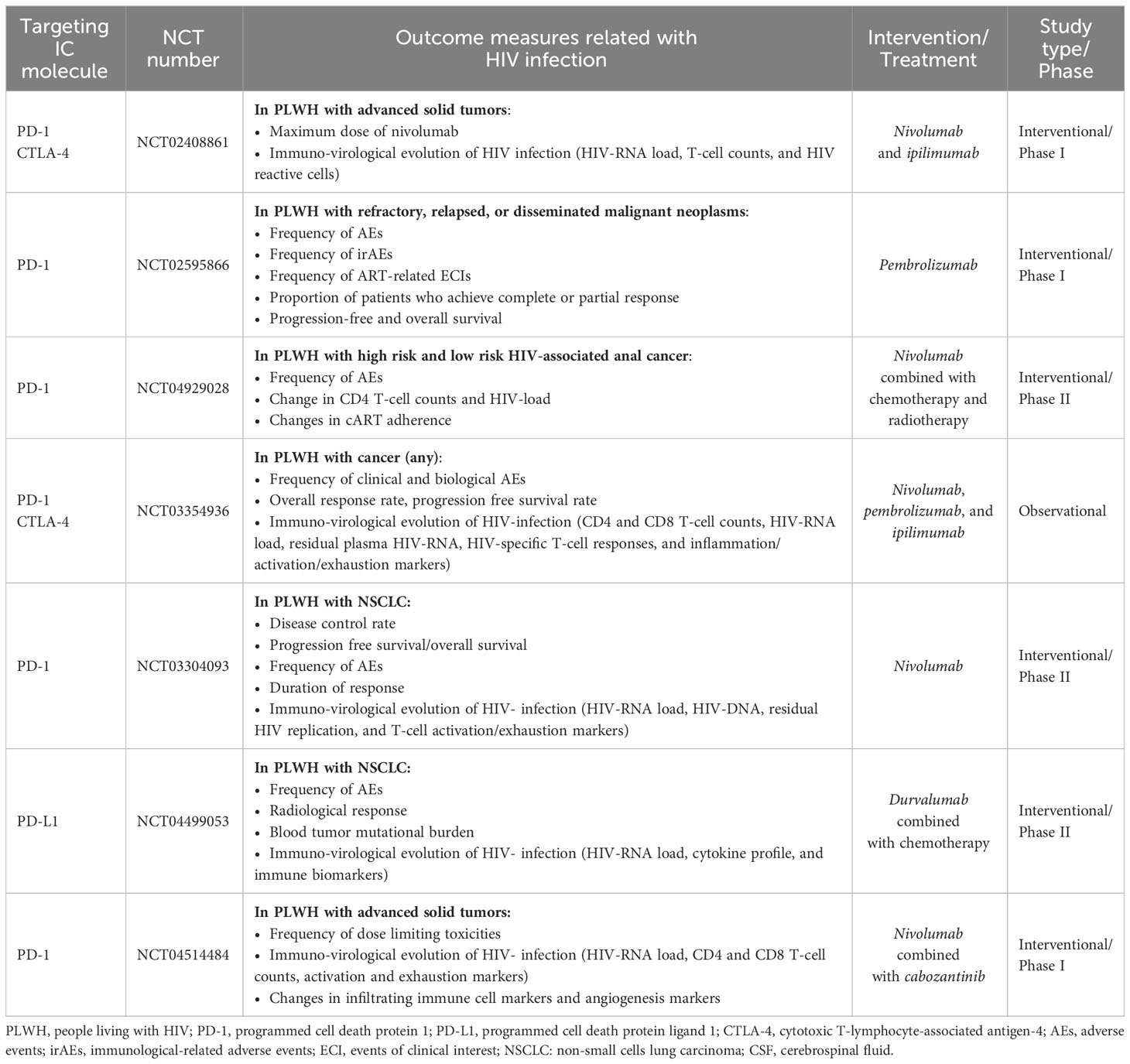
Table 2 Summary of ongoing clinical trials on immune checkpoint therapies in PLWH with malignancies.
The second study, a phase I clinical trial (NCT02595866) from the Cancer Immunotherapy Trials Network [(CITN)-12 trial] is still recruiting PLWH on cART with relapsed malignant neoplasms to evaluate the side effects of pembrolizumab. In a sub-study with 32 participants of this trial, Uldrick et al. reported that the PD-1 blockade slightly reverted HIV latency after the first cycle of anti-PD-1 treatment (105). There are two other ongoing trials; one (NCT03354936) is aimed to evaluate the safety of nivolumab or pembrolizumab (anti-PD-1) combined with ipilimumab (anti-CTLA-4) in PLWH with cancer; the other trial (NCT03304093) evaluates both the safety and efficacy of nivolumab in PLWH on cART with non-small cell lung cancer (NSCLC).
Finally, there are other ongoing clinical trials that aim to evaluate the side effects of combination therapy of ICIs with other types of anti-cancer drugs in PLWH on cART. Among these trials are: a) the phase I trial NCT04514484 assessing the side effects of the combination of nivolumab with cabozantinib (a kinase inhibitor) in PLWH with different types of malignancies; b) the phase II trial NCT04929028 that studies the side effects of nivolumab in combination with different chemotherapy drugs (e.g., capecitabine and fluorouracil) in participants with ADIS-related anal carcinoma; c) the phase II trial NCT04499053 that evaluates adverse events in PLWH with NSCLC after treatment with durvalumab (anti-PD-L1) and platinium-based chemotherapy; and d) the phase I trial NCT04902443 that investigates the safety and tolerability of combined therapy of nivolumab plus pomalidomide in PLWH with virus-associated malignancies.
Taken together, the data provided by the above mentioned studies, while limited and variable, clearly advocates for the use of ICI therapy in the PLWH population suffering from cancer. Moreover, ICI therapy in PLWH could have an additional benefit on HIV disease parameters, restoring virus-specific T-cell responses and reactivating virus production from the reservoir, mimicking the “shock and kill” concept proposed as one of the main strategies to purge the viral reservoir and cure HIV infection.
ICIs in PLWH without malignancies
Regarding the novelty and interesting field of the use of ICIs for HIV treatment in PLWH without cancer, the safety and/or efficacy of ICIs has been evaluated in some clinical trials. Two of the trials have already published results: a) the phase I trial (NCT02028403) has demonstrated that PD-L1 blockade with the BMS-936559 molecule was well tolerated and induced an increase of HIV-specific polyfunctional CD8 T-cell responses in PLWH on cART (90); b) the phase I trial (NCT03407105) using the anti-CTLA-4 ipilimumab in PLWH with uncontrolled viremia did not observe significant changes in CD4 T-cell counts and only a moderate decrease of HIV-RNA load after ICI treatment (106); c) the phase I/IIa double-blind placebo-controlled trial (NCT03787095) in PLWH on cART evaluated the efficacy of cemiplimab (anti-PD-1) and the functional profile of HIV Gag-specific CD8 T-cell responses. However, this study was stopped because two out of four participants showed irAEs at the lowest dose of cemiplimab (96).
Moreover, there are four ongoing clinical trials with anti-PD-1 in PLWH on cART without cancer (Table 3). The first trial (NCT03239899) evaluates the safety and tolerability of pembrolizumab in PLWH as well as its effect on viral load, CD4 T-cell counts, T-cell phenotype, HIV-specific T-cell responses, and cytokine and antibody profile on cerebrospinal fluid (CSF). The second trial (NCT03367754) is recruiting PLWH on cART with poor CD4 recovery and will evaluate, as the primary outcome, the safety of a single dose of pembrolizumab, and as the secondary outcome the changes in PD-1 expression on T cells. The third, a phase I/II trial (NCT05187429), is studying the safety of a single dose of nivolumab as the primary outcome, as well as the effect on PD-1 expression on T cells, HIV-specific T-cell responses, and viral rebound after analytical treatment interruption (ATI) as the secondary outcome in PLWH. Finally, the fourth trial (NCT04223804) is a double-blind placebo-controlled phase I trial that will test the pharmacokinetics and pharmacodynamics of different doses of budigalimab (ABBV-181, anti-PD-1) in PLWH on ART willing to undergo an ATI.
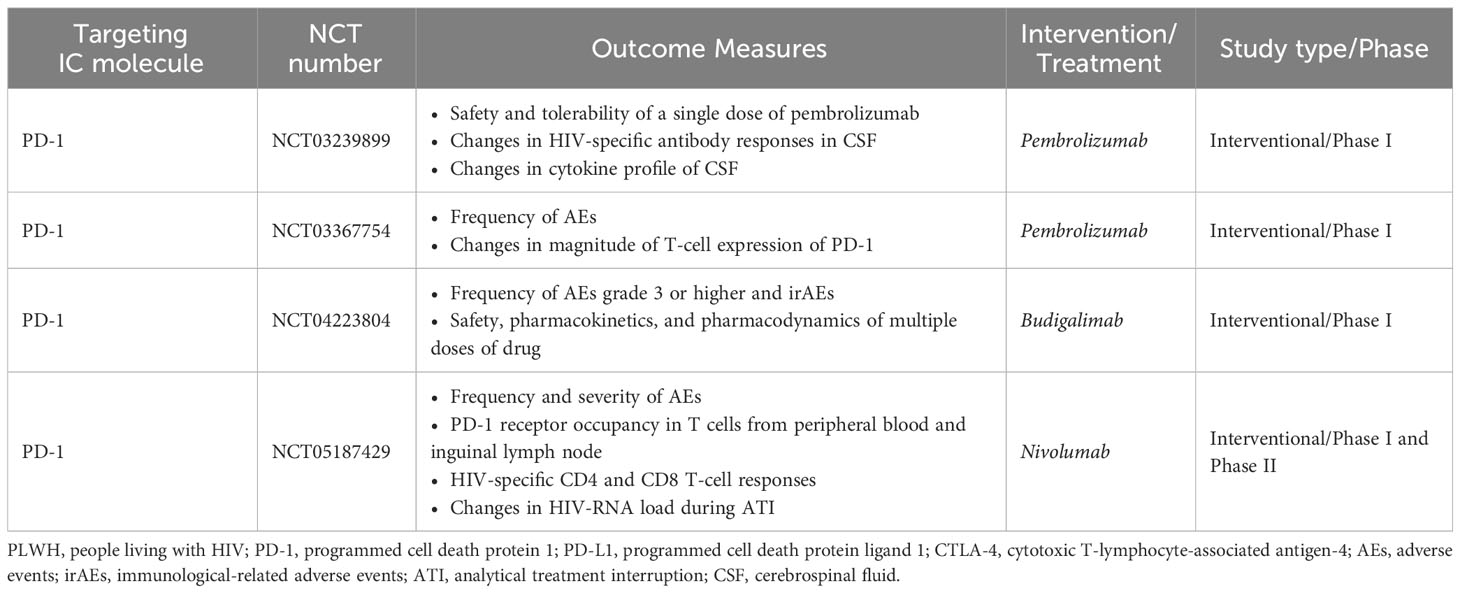
Table 3 Summary of ongoing clinical trials on immune checkpoint therapies in PLWH without malignancies.
Concluding remarks
There is compelling evidence regarding the role of ICs in the attrition of HIV-specific T-cell responses, poor CD4 T-cell recovery, and the viral persistence in PLWH despite cART. Over the past years, there have been many interesting findings related to understanding T-cell exhaustion and how to reinvigorate the HIV-specific immune response by employing mAbs blocking ICs. Without a doubt, persistent HIV latency remains the main obstacle for achieving HIV remission, and different approaches targeting this latency are under intensive investigation. Based on new data, ICI-based therapies used for cancer could be considered not only helpful to target cancer in PLWH but also beneficial to combat immune exhaustion/dysfunction and HIV-latency in PLWH. This approach could be especially attractive as adjuvant therapy in combination with cART with the goal to attain better long-term health outcomes in PLWH. The results provided by current studies are still modest, and further investigation is warranted to accurately measure the effectiveness of this approach in HIV functional cure or remission. Nevertheless, results from ongoing clinical trials will significantly contribute to clarifying the ability of ICI-based therapies to restore HIV-specific immune response and revert HIV latency.
Search strategy and selection criteria
Relevant scientific literature was surveyed to review evidence and prepare the manuscript. We searched PubMed for English language papers published until September 2023. Search terms included: “HIV disease progression”; “HIV pathogenesis”; “immune exhaustion and HIV”; “immune exhaustion and cancer”; “HIV cure”; “HIV and inflammation”; “AIDS-defining cancers”; “non-AIDS-defining cancers”; “ immune checkpoint molecules and HIV”; “ immune checkpoint molecules and cancer”; “IC and HIV”; “IC and cancer”; “immune checkpoint inhibitors and HIV”; “immune checkpoint inhibitors and cancer”; “immune checkpoint blockers and HIV”; “immune checkpoint blockers and cancer”; “ICIs and HIV”; “ICIs and Cancer”; and “ICIs and HIV and cancer”. Two authors (JMB and CR) screened abstracts for relevance and reviewed full-text articles deemed relevant to the topics addressed in the manuscript. In addition, we searched the database ClinicalTrials.gov for clinical registered trials related to immune checkpoint inhibitors. Search terms included: “HIV and ICIs”; “HIV and anti-PD1”; “HIV and anti-PD-L1”; “HIV and anti-CTLA-4”; “HIV and anti-TIGIT”; “HIV and anti-Tim-3”; and “HIV and anti-LAG-3”.
Author contributions
JB: Writing – original draft, Methodology, Writing – review & editing. CR: Writing – original draft, Methodology, Writing – review & editing. JG-F: Investigation, Visualization, Writing – review & editing. NR: Conceptualization, Investigation, Funding acquisition, Project administration, Supervision, Data curation, Writing – original draft, Validation, Visualization, Writing – review & editing.
Funding
The author(s) declare financial support was received for the research, authorship, and/or publication of this article. This work has been partially funded by the project GLD22-00197 from the Gilead Fellowship Program (Gilead Sciences, S.L.U.) and project PI19-01237 integrated into the State Plan for Scientific and Technical Research and Innovation from the General Sub-Directorate for research assessment and promotion, Spanish Carlos III Institute of Health (ISCIII) co-funded by the European Regional Development Fund (ERDF).
Acknowledgements
We especially thank Marcela Peñaloza for her effort and time devoted to proofreading and style correcting the manuscript.
Conflict of interest
The authors declare that the research was conducted in the absence of any commercial or financial relationships that could be construed as a potential conflict of interest.
Publisher’s note
All claims expressed in this article are solely those of the authors and do not necessarily represent those of their affiliated organizations, or those of the publisher, the editors and the reviewers. Any product that may be evaluated in this article, or claim that may be made by its manufacturer, is not guaranteed or endorsed by the publisher.
References
1. Deeks SG, Lewin SR, Ross AL, Ananworanich J, Benkirane M, Cannon P, et al. International AIDS Society global scientific strategy: towards an HIV cure. Nat Med (2016) 22(8):839–50. doi: 10.1038/nm.4108
2. Dahabieh MS, Battivelli E, Verdin E. Understanding HIV latency: the road to an HIV cure. Annu Rev Med (2015) 66:407–21. doi: 10.1146/annurev-med-092112-152941
3. Wherry EJ, Kurachi M. Molecular and cellular insights into T cell exhaustion. Nat Rev Immunol (2015) 15(8):486–99. doi: 10.1038/nri3862. Review.
4. Day CL, Kaufmann DE, Kiepiela P, Brown JA, Moodley ES, Reddy S, et al. PD-1 expression on HIV-specific T cells is associated with T-cell exhaustion and disease progression. Nature (2006) 443(7109):350–4. doi: 10.1038/nature05115
5. Hoffmann M, Pantazis N, Martin GE, Hickling S, Hurst J, Meyerowitz J, et al. Exhaustion of activated CD8 T cells predicts disease progression in primary HIV-1 infection. PloS Pathog (2016) 12(7):e1005661. doi: 10.1371/journal.ppat.1005661
6. Porichis F, Kwon DS, Zupkosky J, Tighe DP, McMullen A, Brockman MA, et al. Responsiveness of HIV-specific CD4 T cells to PD-1 blockade. Blood (2011) 118(4):965–74. doi: 10.1182/blood-2010-12-328070
7. Blackburn SD, Shin H, Haining WN, Zou T, Workman CJ, Polley A, et al. Coregulation of CD8+ T cell exhaustion by multiple inhibitory receptors during chronic viral infection. Nat Immunol (2009) 10(1):29–37. doi: 10.1038/ni.1679
8. Chew GM, Fujita T, Webb GM, Burwitz BJ, Wu HL, Reed JS, et al. TIGIT marks exhausted T cells, correlates with disease progression, and serves as a target for immune restoration in HIV and SIV infection. PloS Pathog (2016) 12(1):e1005349. doi: 10.1371/journal.ppat.1005349
9. Banga R, Procopio FA, Noto A, Pollakis G, Cavassini M, Ohmiti K, et al. PD-1(+) and follicular helper T cells are responsible for persistent HIV-1 transcription in treated aviremic individuals. Nat Med (2016) 22(7):754–61. doi: 10.1038/nm.4113
10. El-Far M, Ancuta P, Routy JP, Zhang Y, Bakeman W, Bordi R, et al. Nef promotes evasion of human immunodeficiency virus type 1-infected cells from the CTLA-4-mediated inhibition of T-cell activation. J Gen Virol (2015) 96(Pt 6):1463–77. doi: 10.1099/vir.0.000065
11. Fromentin R, Bakeman W, Lawani MB, Khoury G, Hartogensis W, DaFonseca S, et al. CD4+ T cells expressing PD-1, TIGIT and LAG-3 contribute to HIV persistence during ART. PloS Pathog (2016) 12(7):e1005761. doi: 10.1371/journal.ppat.1005761
12. Yarchoan R, Uldrick TS. HIV-associated cancers and related diseases. N Engl J Med (2018) 378(22):2145. doi: 10.1056/NEJMc1804812
13. Re A, Cattaneo C, Montoto S. Treatment management of haematological Malignancies in people living with HIV. Lancet Haematol (2020) 7(9):e679–89. doi: 10.1016/S2352-3026(20)30115-0
14. Topalian SL, Drake CG, Pardoll DM. Immune checkpoint blockade: a common denominator approach to cancer therapy. Cancer Cell (2015) 27(4):450–61. doi: 10.1016/j.ccell.2015.03.001
15. Gubser C, Chiu C, Lewin SR, Rasmussen TA. Immune checkpoint blockade in HIV. EBioMedicine (2022) 76:103840. doi: 10.1016/j.ebiom.2022.103840
16. Chen H, Moussa M, Catalfamo M. The role of immunomodulatory receptors in the pathogenesis of HIV infection: A therapeutic opportunity for HIV cure? Front Immunol (2020) 11:1223. doi: 10.3389/fimmu.2020.01223
17. Wykes MN, Lewin SR. Immune checkpoint blockade in infectious diseases. Nat Rev Immunol (2018) 18(2):91–104. doi: 10.1038/nri.2017.112
18. Lurain K, Ramaswami R, Yarchoan R, Uldrick TS. Anti-PD-1 and anti-PD-L1 monoclonal antibodies in people living with HIV and cancer. Curr HIV/AIDS Rep (2020) 17(5):547–56. doi: 10.1007/s11904-020-00525-y
19. Boyer Z, Palmer S. Targeting immune checkpoint molecules to eliminate latent HIV. Front Immunol (2018) 9:2339. doi: 10.3389/fimmu.2018.02339
20. Rasmussen TA, Anderson JL, Wightman F, Lewin SR. Cancer therapies in HIV cure research. Curr Opin HIV AIDS (2017) 12(1):96–104. doi: 10.1097/COH.0000000000000328
21. Parry RV, Chemnitz JM, Frauwirth KA, Lanfranco AR, Braunstein I, Kobayashi SV, et al. CTLA-4 and PD-1 receptors inhibit T-cell activation by distinct mechanisms. Mol Cell Biol (2005) 25(21):9543–53. doi: 10.1128/MCB.25.21.9543-9553.2005
22. Quigley M, Pereyra F, Nilsson B, Porichis F, Fonseca C, Eichbaum Q, et al. Transcriptional analysis of HIV-specific CD8+ T cells shows that PD-1 inhibits T cell function by upregulating BATF. Nat Med (2010) 16(10):1147–51. doi: 10.1038/nm.2232
23. Crawford A, Angelosanto JM, Kao C, Doering TA, Odorizzi PM, Barnett BE, et al. Molecular and transcriptional basis of CD4⁺ T cell dysfunction during chronic infection. Immunity (2014) 40(2):289–302. doi: 10.1016/j.immuni.2014.01.005
25. Moir S, Fauci AS. B-cell exhaustion in HIV infection: the role of immune activation. Curr Opin HIV AIDS (2014) 9(5):472–7. doi: 10.1097/COH.0000000000000092
26. Trautmann L, Janbazian L, Chomont N, Said EA, Gimmig S, Bessette B, et al. Upregulation of PD-1 expression on HIV-specific CD8+ T cells leads to reversible immune dysfunction. Nat Med (2006) 12(10):1198–202. doi: 10.1038/nm1482
27. Kaufmann DE, Kavanagh DG, Pereyra F, Zaunders JJ, Mackey EW, Miura T, et al. Upregulation of CTLA-4 by HIV-specific CD4+ T cells correlates with disease progression and defines a reversible immune dysfunction. Nat Immunol (2007) 8(11):1246–54. doi: 10.1038/ni1515
28. Tian X, Zhang A, Qiu C, Wang W, Yang Y, Qiu C, et al. The upregulation of LAG-3 on T cells defines a subpopulation with functional exhaustion and correlates with disease progression in HIV-infected subjects. J Immunol (2015) 194(8):3873–82. doi: 10.4049/jimmunol.1402176
29. Jones RB, Ndhlovu LC, Barbour JD, Sheth PM, Jha AR, Long BR, et al. Tim-3 expression defines a novel population of dysfunctional T cells with highly elevated frequencies in progressive HIV-1 infection. J Exp Med (2008) 205(12):2763–79. doi: 10.1084/jem.20081398
30. Tauriainen J, Scharf L, Frederiksen J, Naji A, Ljunggren HG, Sönnerborg A, et al. Perturbed CD8+ T cell TIGIT/CD226/PVR axis despite early initiation of antiretroviral treatment in HIV infected individuals. Sci Rep (2017) 7:40354. doi: 10.1038/srep40354
31. Hurst J, Hoffmann M, Pace M, Williams JP, Thornhill J, Hamlyn E, et al. Immunological biomarkers predict HIV-1 viral rebound after treatment interruption. Nat Commun (2015) 6:8495. doi: 10.1038/ncomms9495
32. Grabmeier-Pfistershammer K, Steinberger P, Rieger A, Leitner J, Kohrgruber N. Identification of PD-1 as a unique marker for failing immune reconstitution in HIV-1-infected patients on treatment. J Acquir Immune Defic Syndr (2011) 56(2):118–24. doi: 10.1097/QAI.0b013e3181fbab9f
33. Shive CL, Clagett B, McCausland MR, Mudd JC, Funderburg NT, Freeman ML, et al. Inflammation perturbs the IL-7 axis, promoting senescence and exhaustion that broadly characterize immune failure in treated HIV infection. J Acquir Immune Defic Syndr (2016) 71(5):483–92. doi: 10.1097/QAI.0000000000000913
34. Reeves DB, Duke ER, Wagner TA, Palmer SE, Spivak AM, Schiffer JT. A majority of HIV persistence during antiretroviral therapy is due to infected cell proliferation. Nat Commun (2018) 9(1):4811. doi: 10.1038/s41467-018-06843-5
35. El-Far M, Halwani R, Said E, Trautmann L, Doroudchi M, Janbazian L, et al. T-cell exhaustion in HIV infection. Curr HIV/AIDS Rep (2008) 5(1):13–9. doi: 10.1007/s11904-008-0003-7
36. Khoury G, Fromentin R, Solomon A, Hartogensis W, Killian M, Hoh R, et al. Human immunodeficiency virus persistence and T-cell activation in blood, rectal, and lymph node tissue in human immunodeficiency virus-infected individuals receiving suppressive antiretroviral therapy. J Infect Dis (2017) 215(6):911–9. doi: 10.1093/infdis/jix039
37. Chomont N, El-Far M, Ancuta P, Trautmann L, Procopio FA, Yassine-Diab B, et al. HIV reservoir size and persistence are driven by T cell survival and homeostatic proliferation. Nat Med (2009) 15(8):893–900. doi: 10.1038/nm.1972
38. Macatangay BJC, Gandhi RT, Jones RB, Mcmahon DK, Lalama CM, Bosch RJ, et al. T cells with high PD-1 expression are associated with lower HIV-specific immune responses despite long-term antiretroviral therapy. AIDS (2020) 34(1):15–24. doi: 10.1097/QAD.0000000000002406
39. Evans VA, van der Sluis RM, Solomon A, Dantanarayana A, McNeil C, Garsia R, et al. Programmed cell death-1 contributes to the establishment and maintenance of HIV-1 latency. AIDS (2018) 32(11):1491–7. doi: 10.1097/QAD.0000000000001849
40. Horn C, Augustin M, Ercanoglu MS, Heger E, Knops E, Bondet V, et al. HIV DNA reservoir and elevated PD-1 expression of CD4 T-cell subsets particularly persist in the terminal ileum of HIV-positive patients despite cART. HIV Med (2021) 22(5):397–408. doi: 10.1111/hiv.13031
41. Rasmussen TA, Zerbato JM, Rhodes A, Tumpach C, Dantanarayana A, McMahon JH, et al. Memory CD4+ T cells that co-express PD-1 and CTLA-4 have reduced response to activating stimuli facilitating HIV latency. Cell Rep Med (2022) 100766. doi: 10.1016/j.xcrm.2022.100766
42. Ahmadzadeh M, Johnson LA, Heemskerk B, Wunderlich JR, Dudley ME, White DE, et al. Tumor antigen-specific CD8 T cells infiltrating the tumor express high levels of PD-1 and are functionally impaired. Blood (2009) 114(8):1537–44. doi: 10.1182/blood-2008-12-195792
43. Anderson AC, Joller N, Kuchroo VK. Lag-3, tim-3, and TIGIT: co-inhibitory receptors with specialized functions in immune regulation. Immunity (2016) 44(5):989–1004. doi: 10.1016/j.immuni.2016.05.001
44. Ribas A, Wolchok JD. Cancer immunotherapy using checkpoint blockade. Science (2018) 359(6382):1350–5. doi: 10.1126/science.aar4060
45. Topalian SL, Forde PM, Emens LA, Yarchoan M, Smith KN, Pardoll DM. Neoadjuvant immune checkpoint blockade: A window of opportunity to advance cancer immunotherapy. Cancer Cell (2023) 41(9):1551–66. doi: 10.1016/j.ccell.2023.07.011
46. Brahmer J, Reckamp KL, Baas P, Crinò L, Eberhardt WEE, Poddubskaya E, et al. Nivolumab versus docetaxel in advanced squamous-cell non–small-cell lung cancer. N Engl J Med (2015) 373:1627. doi: 10.1056/NEJMoa1504627
47. Hodi FS, O’Day SJ, McDermott DF, Weber RW, Sosman JA, Haanen JB, et al. Improved survival with ipilimumab in patients with metastatic melanoma. N Engl J Med (2010) 363(8):711–23. doi: 10.1056/NEJMoa1003466
48. Siu LL, Even C, Mesia R, Remenar E, Daste A, Delord JP, et al. Safety and efficacy of durvalumab with or without tremelimumab in patients with PD-L1-low/negative recurrent or metastatic HNSCC: the phase 2 CONDOR randomized clinical trial. JAMA Oncol (2018) 5(2):195–203. doi: 10.1001/jamaoncol.2018.4628
49. Armand P, Engert A, Younes A, Fanale M, Santoro A, Zinzani PL, et al. Nivolumab for relapsed/refractory classic hodgkin lymphoma after failure of autologous hematopoietic cell transplantation: extended follow-up of the multicohort single-arm phase II CheckMate 205 trial. J Clin Oncol 36(26):2748. doi: 10.1200/JCO.2017.76.0793
50. Robert C, Long GV, Brady B, Dutriaux C, Maio M, Mortier L, et al. Nivolumab in previously untreated melanoma without BRAF mutation. N Engl J Med (2015) 372:320–30. doi: 10.1056/NEJMoa1412082
51. Ott PA, Piha-Paul SA, Munster P, Pishvaian MJ, van Brummelen EMJ, Cohen RB, et al. Safety and antitumor activity of the anti-PD-1 antibody pembrolizumab in patients with recurrent carcinoma of the anal canal. Ann Oncol (2017) 28(5):1036–41. doi: 10.1093/annonc/mdx029
52. Migden MR, Rischin D, Schmults CD, Guminski A, Hauschild A, Lewis KD, et al. PD-1 blockade with cemiplimab in advanced cutaneous squamous-cell carcinoma. N Engl J Med (2018) 379:341–51. doi: 10.1056/NEJMoa1805131
53. American Association for Cancer Research. Atezolizumab extends survival for breast cancer. Cancer Discovery (2017) 7:OF10. doi: 10.1158/2159-8290.CD-NB2017-053
54. Hellmann MD, Ciuleanu TE, Pluzanski A, Lee JS, Otterson GA, Audigier-Valette C, et al. Nivolumab plus ipilimumab in lung cancer with a high tumor mutational burden. N Engl J Med (2018) 378:2093–104. doi: 10.1056/NEJMoa1801946
55. Long GV, Atkinson V, Cebon JS, Jameson MB, Fitzharris BM, McNeil CM, et al. Standard-dose pembrolizumab in combination with reduced-dose ipilimumab for patients with advanced melanoma (KEYNOTE-029): an open-label, phase 1b trial. Lancet Oncol (2017) 18(9):1202–10. doi: 10.1016/S1470-2045(17)30428-X
56. Cella D, Grünwald V, Escudier B, Hammers HJ, George S, Nathan P, et al. Patient-reported outcomes of patients with advanced renal cell carcinoma treated with nivolumab plus ipilimumab versus sunitinib (CheckMate 214): a randomised, phase 3 trial. Lancet Oncol (2019) 20(2):297–310. doi: 10.1016/S1470-2045(18)30778-2
57. Overman MJ, Lonardi S, Wong KYM, Lenz HJ, Gelsomino F, Aglietta M, et al. Durable clinical benefit with nivolumab plus ipilimumab in DNA mismatch repair-deficient/microsatellite instability-high metastatic colorectal cancer. J Clin Oncol (2018) 36(8):773–9. doi: 10.1200/JCO.2017.76.9901
58. Tawbi HA, SChadendorf D, Lipson EJ, Ascierto PA, Matamala L, Castillo Gutiérrez E, et al. Relatlimab and nivolumab versus nivolumab in untreated advanced melanoma. N Engl J Med (2022) 386(1):24–34. doi: 10.1056/NEJMoa2109970
59. Weber JS, Hodi FS, Wolchok JD, Topalian SL, SChadendorf D, Larkin J, et al. Safety profile of nivolumab monotherapy: A pooled analysis of patients with advanced melanoma. J Clin Oncol (2017) 35(7):785–92. doi: 10.1200/JCO.2015.66.1389
60. Haanen JBAG, Carbonnel F, Robert C, Kerr KM, Peters S, Larkin J, et al. Management of toxicities from immunotherapy: ESMO Clinical Practice Guidelines for diagnosis, treatment and follow-up. Ann Oncol (2017) 28(suppl_4):iv119–42. doi: 10.1093/annonc/mdx225
61. Brahmer JR, Lacchetti C, Schneider BJ, Atkins MB, Brassil KJ, Caterino JM, et al. Management of immune-related adverse events in patients treated with immune checkpoint inhibitor therapy: american society of clinical oncology clinical practice guideline. J Clin Oncol (2018) 36(17):1714–68. doi: 10.1200/JCO.2017.77.6385
62. Yanai S, Nakamura S, Matsumoto T. Nivolumab-induced colitis treated by infliximab. Clin Gastroenterol Hepatol (2017) 15(4):e80–1. doi: 10.1016/j.cgh.2016.09.017
63. Bergqvist V, Hertervig E, Gedeon P, Kopljar M, Griph H, Kinhult S, et al. Vedolizumab treatment for immune checkpoint inhibitor-induced enterocolitis. Cancer Immunol Immunother (2017) 66(5):581–92. doi: 10.1007/s00262-017-1962-6
64. Aguilar-Company J, Lopez-Olivo MA, Ruiz-Camps I. Multidisciplinary approach to treatment with immune checkpoint inhibitors in patients with HIV, tuberculosis, or underlying autoimmune diseases. Front Med (Lausanne) (2022) 9:875910. doi: 10.3389/fmed.2022.875910
65. Tapia Rico G, Chan MM, Loo KF. The safety and efficacy of immune checkpoint inhibitors in patients with advanced cancers and pre-existing chronic viral infections (Hepatitis B/C, HIV): A review of the available evidence. Cancer Treat Rev (2020) 86:102011. doi: 10.1016/j.ctrv.2020.102011
66. Florou V, Puri S, Garrido-Laguna I, Wilky BA. Considerations for immunotherapy in patients with cancer and comorbid immune dysfunction. Ann Transl Med (2021) 9(12):1035. doi: 10.21037/atm-20-5207
67. Hütter G, Nowak D, Mossner M, Ganepola S, Müssig A, Allers K, et al. Long-term control of HIV by CCR5 Delta32/Delta32 stem-cell transplantation. N Engl J Med (2009) 360(7):692–8. doi: 10.1056/NEJMoa0802905
68. Gupta RK, Peppa D, Hill AL, Gálvez C, Salgado M, Pace M, et al. Evidence for HIV-1 cure after CCR5Δ32/Δ32 allogeneic haemopoietic stem-cell transplantation 30 months post analytical treatment interruption: a case report. Lancet HIV (2020) 7(5):e340–7. doi: 10.1016/S2352-3018(20)30069-2
69. Jensen BO, Knops E, Cords L, Lübke N, Salgado M, Busman-Sahay K, et al. In-depth virological and immunological characterization of HIV-1 cure after CCR5Δ32/Δ32 allogeneic hematopoietic stem cell transplantation. Nat Med (2023) 29(3):583–7. doi: 10.1038/s41591-023-02213-x
70. Hsu J, Van Besien K, Glesby MJ, Pahwa S, Coletti A, Warshaw MG, et al. HIV-1 remission and possible cure in a woman after haplo-cord blood transplant. Cell (2023) 186(6):1115–1126.e8. doi: 10.1016/j.cell.2023.02.030
71. Peligero C, Argilaguet J, Güerri-Fernandez R, Torres B, Ligero C, Colomer P, et al. PD-L1 blockade differentially impacts regulatory T cells from HIV-infected individuals depending on plasma viremia. PloS Pathog (2015) 11(12):e1005270. doi: 10.1371/journal.ppat.1005270
72. Porichis F, Hart MG, Massa A, Everett HL, Morou A, Richard J, et al. Immune checkpoint blockade restores HIV-specific CD4 T cell help for NK cells. J Immunol (2018) 201(3):971–81. doi: 10.4049/jimmunol.1701551
73. Holder KA, Burt K, Grant MD. TIGIT blockade enhances NK cell activity against autologous HIV-1-infected CD4+ T cells. Clin Transl Immunol (2021) 10(10):e1348. doi: 10.1002/cti2.1348
74. Chiu CY, Chang JJ, Dantanarayana AI, Solomon A, Evans VA, Pascoe R, et al. Combination immune checkpoint blockade enhances IL-2 and CD107a production from HIV-specific T cells ex vivo in people living with HIV on antiretroviral therapy. J Immunol (2022) 208(1):54–62. doi: 10.4049/jimmunol.2100367
75. Perreau M, Savoye AL, De Crignis E, Corpataux JM, Cubas R, Haddad EK, et al. Follicular helper T cells serve as the major CD4 T cell compartment for HIV-1 infection, replication, and production. J Exp Med (2013) 210(1):143–56. doi: 10.1084/jem.20121932
76. Brunet-Ratnasingham E, Morou A, Dubé M, Niessl J, Baxter AE, Tastet O, et al. Immune checkpoint expression on HIV-specific CD4+ T cells and response to their blockade are dependent on lineage and function. EBioMedicine (2022) 84:104254. doi: 10.1016/j.ebiom.2022.104254
77. Fromentin R, DaFonseca S, Costiniuk CT, El-Far M, Procopio FA, Hecht FM, et al. PD-1 blockade potentiates HIV latency reversal ex vivo in CD4(+) T cells from ART-suppressed individuals. Nat Commun (2019) 10(1):814. doi: 10.1038/s41467-019-08798-7
78. Van der Sluis RM, Kumar NA, Pascoe RD, Zerbato JM, Evans VA, Dantanarayana AI, et al. Combination immune checkpoint blockade to reverse HIV latency. J Immunol (2020) 204(5):1242–54. doi: 10.4049/jimmunol.1901191
79. Velu V, Titanji K, Zhu B, Husain S, Pladevega A, Lai L, et al. Enhancing SIV-specific immunity in vivo by PD-1 blockade. Nature (2009) 458(7235):206–10. doi: 10.1038/nature07662
80. Amancha PK, Hong JJ, Rogers K, Ansari AA, Villinger F. In vivo blockade of the programmed cell death-1 pathway using soluble recombinant PD-1-Fc enhances CD4+ and CD8+ T cell responses but has limited clinical benefit. J Immunol (2013) 191(12):6060–70. doi: 10.4049/jimmunol.1302044
81. Mylvaganam GH, Chea LS, Tharp GK, Hicks S, Velu V, Iyer SS, et al. Combination anti-PD-1 and antiretroviral therapy provides therapeutic benefit against SIV. JCI Insight (2018) 3(18):e122940. doi: 10.1172/jci.insight.122940
82. Velu V, Titanji K, Ahmed H, Shetty RD, Chennareddi LS, Freeman GJ, et al. PD-1 blockade following ART interruption enhances control of pathogenic SIV in rhesus macaques. Proc Natl Acad Sci U S A (2022) 119(33):e2202148119. doi: 10.1073/pnas.2202148119
83. Jin HT, Anderson AC, Tan WG, West EE, Ha SJ, Araki K, et al. Cooperation of Tim-3 and PD-1 in CD8 T-cell exhaustion during chronic viral infection. Proc Natl Acad Sci U S A (2010) 107(33):14733–8. doi: 10.1073/pnas.1009731107
84. Harper J, Gordon S, Chan CN, Wang H, Lindemuth E, Galardi C, et al. CTLA-4 and PD-1 dual blockade induces SIV reactivation without control of rebound after antiretroviral therapy interruption. Nat Med (2020) 26(4):519–28. doi: 10.1038/s41591-020-0782-y
85. CecChinato V, Tryniszewska E, Ma ZM, Vaccari M, Boasso A, Tsai WP, et al. Immune activation driven by CTLA-4 blockade augments viral replication at mucosal sites in simian immunodeficiency virus infection. J Immunol (2008) 180(8):5439–47. doi: 10.4049/jimmunol.180.8.5439
86. Le Garff G, Samri A, Lambert-Niclot S, Even S, Lavolé A, Cadranel J, et al. Transient HIV-specific T cells increase and inflammation in an HIV-infected patient treated with nivolumab. AIDS (2017) 31(7):1048–51. doi: 10.1097/QAD.0000000000001429
87. Guihot A, Marcelin AG, Massiani MA, Samri A, Soulié C, Autran B, et al. Drastic decrease of the HIV reservoir in a patient treated with nivolumab for lung cancer. Ann Oncol (2018) 29(2):517–8. doi: 10.1093/annonc/mdx696
88. Blanch-Lombarte O, Gálvez C, Revollo B, Jiménez-Moyano E, Llibre JM, Manzano JL, et al. Enhancement of antiviral CD8+ T-cell responses and complete remission of metastatic melanoma in an HIV-1-infected subject treated with pembrolizumab. J Clin Med (2019) 8(12):2089. doi: 10.3390/jcm8122089
89. Lau JSY, McMahon JH, Gubser C, Solomon A, Chiu CYH, Dantanarayana A, et al. The impact of immune checkpoint therapy on the latent reservoir in HIV-infected individuals with cancer on antiretroviral therapy. AIDS (2021) 35(10):1631–6. doi: 10.1097/QAD.0000000000002919
90. Gay CL, Bosch RJ, Ritz J, Hataye JM, Aga E, Tressler RL, et al. Clinical trial of the anti-PD-L1 antibody BMS-936559 in HIV-1 infected participants on suppressive antiretroviral therapy. J Infect Dis (2017) 215(11):1725–33. doi: 10.1093/infdis/jix191
91. Wightman F, Solomon A, Kumar SS, Urriola N, Gallagher K, Hiener B, et al. Effect of ipilimumab on the HIV reservoir in an HIV-infected individual with metastatic melanoma. AIDS (2015) 29(4):504–6. doi: 10.1097/QAD.0000000000000562
92. Rasmussen TA, Rajdev L, Rhodes A, Dantanarayana A, Tennakoon S, Chea S, et al. Impact of anti-PD-1 and anti-CTLA-4 on the human immunodeficiency virus (HIV) reservoir in people living with HIV with cancer on antiretroviral therapy: the AIDS Malignancy consortium 095 study. Clin Infect Dis (2021) 73(7):e1973–81. doi: 10.1093/cid/ciaa1530
93. Bari S, Muzaffar J, Chan A, Jain SR, Haider AM, Adams Curry M, et al. Outcomes of programmed cell death protein 1 (PD-1) and programmed death-ligand 1(PD-L1) inhibitor therapy in HIV patients with advanced cancer. J Oncol (2019) 2019:2989048. doi: 10.1155/2019/2989048
94. Spano JP, Veyri M, Gobert A, Guihot A, Perré P, Kerjouan M, et al. Immunotherapy for cancer in people living with HIV: safety with an efficacy signal from the series in real life experience. AIDS (2019) 33(11):F13–9. doi: 10.1097/QAD.0000000000002298
95. Uldrick TS, Gonçalves PH, Abdul-Hay M, Claeys AJ, Emu B, Ernstoff MS, et al. Assessment of the safety of pembrolizumab in patients with HIV and advanced cancer-A phase 1 study. JAMA Oncol (2019) 5(9):1332–9. doi: 10.1001/jamaoncol.2019.2244
96. Gay CL, Bosch RJ, McKhann A, Moseley KF, Wimbish CL, Hendrickx SM, et al. Suspected immune-related adverse events with an anti-PD-1 inhibitor in otherwise healthy people with HIV. J Acquir Immune Defic Syndr (2021) 87(5):e234–6. doi: 10.1097/QAI.0000000000002716
97. Cook MR, Kim C. Safety and efficacy of immune checkpoint inhibitor therapy in patients with HIV infection and advanced-stage cancer: A systematic review. JAMA Oncol (2019) 5(7):1049–54. doi: 10.1001/jamaoncol.2018.6737
98. Abbar B, Baron M, Katlama C, Marcelin AG, Veyri M, Autran B, et al. Immune checkpoint inhibitors in people living with HIV: what about anti-HIV effects? AIDS (2020) 34(2):167–75. doi: 10.1097/QAD.0000000000002397
99. El Zarif T, Nassar AH, Adib E, Fitzgerald BG, Huang J, Mouhieddine TH, et al. Safety and activity of immune checkpoint inhibitors in people living with HIV and cancer: A real-world report from the cancer therapy using checkpoint inhibitors in people living with HIV-international (CATCH-IT) consortium. J Clin Oncol (2023) 41(21):3712–23. doi: 10.1200/JCO.22.02459
100. Idossa D, Friedlander T, Paller CJ, Ryan CJ, Borno HT. Case report: clinical characteristics and outcomes of HIV positive patients with metastatic prostate cancer treated with immunotherapy: A case series and literature review. Front Oncol (2022) 12:910115. doi: 10.3389/fonc.2022.910115
101. Alloghbi A, Ninia J, Alshare B, Hotaling J, Raza S, Sukari A. Anti-PD-1 therapy using cemiplimab for advanced cutaneous squamous cell carcinoma in HIV patient: A case report. Clin Case Rep (2021) 9(12):e05228. doi: 10.1002/ccr3.5228
102. Gonzalez-Cao M, Morán T, Dalmau J, Garcia-Corbacho J, Bracht JWP, Bernabe R, et al. Assessment of the feasibility and safety of durvalumab for treatment of solid tumors in patients with HIV-1 infection: the phase 2 DURVAST study. JAMA Oncol (2020) 6(7):1063–7. doi: 10.1001/jamaoncol.2020.0465
103. Galanina N, Goodman AM, Cohen PR, Frampton GM, Kurzrock R. Successful treatment of HIV-associated kaposi sarcoma with immune checkpoint blockade. Cancer Immunol Res (2018) 6(10):1129–35. doi: 10.1158/2326-6066.CIR-18-0121
104. Baron M, Soulié C, Lavolé A, Assoumou L, Abbar B, Fouquet B, et al. Impact of anti PD-1 immunotherapy on HIV reservoir and anti-viral immune responses in people living with HIV and cancer. Cells (2022) 11(6):1015. doi: 10.3390/cells11061015
105. Uldrick TS, Adams SV, Fromentin R, Roche M, Fling SP, Gonçalves PH, et al. Pembrolizumab induces HIV latency reversal in people living with HIV and cancer on antiretroviral therapy. Sci Transl Med (2022) 14(629):eabl3836. doi: 10.1126/scitranslmed.abl3836
Keywords: immune checkpoint inhibitors, inflammation, cancer, HIV, immune exhaustion, HIV latency, immunotherapy, HIV cure
Citation: Benito JM, Restrepo C, García-Foncillas J and Rallón N (2023) Immune checkpoint inhibitors as potential therapy for reverting T-cell exhaustion and reverting HIV latency in people living with HIV. Front. Immunol. 14:1270881. doi: 10.3389/fimmu.2023.1270881
Received: 01 August 2023; Accepted: 20 November 2023;
Published: 07 December 2023.
Edited by:
Amélie Guihot, Institut National de la Santé et de la Recherche Médicale (INSERM), FranceReviewed by:
Nadia Alatrakchi, Massachusetts General Hospital and Harvard Medical School, United StatesDaniel Ramos Ram, University of Central Florida, United States
Copyright © 2023 Benito, Restrepo, García-Foncillas and Rallón. This is an open-access article distributed under the terms of the Creative Commons Attribution License (CC BY). The use, distribution or reproduction in other forums is permitted, provided the original author(s) and the copyright owner(s) are credited and that the original publication in this journal is cited, in accordance with accepted academic practice. No use, distribution or reproduction is permitted which does not comply with these terms.
*Correspondence: José M. Benito, amJlbml0bzFAaG90bWFpbC5jb20=, am9zZS5iZW5pdG9AZmpkLmVz; Norma Rallón, bm9ybWFpYm9uQHlhaG9vLmNvbQ==, bm9ybWEucmFsbG9uQGZqZC5lcw==
†These authors have contributed equally to this work