- 1Department of Clinical Cancer Prevention, The University of Texas MD Anderson Cancer Center, Houston, TX, United States
- 2Department of Surgical Oncology, The University of Texas MD Anderson Cancer Center, Houston, TX, United States
- 3Department of Clinical Cancer Genetics, The University of Texas MD Anderson Cancer Center, Houston, TX, United States
- 4Department of Gastroenterology, Hepatology and Nutrition, The University of Texas MD Anderson Cancer Center, Houston, TX, United States
- 5Department of Gastrointestinal Medical Oncology, The University of Texas MD Anderson Cancer Center, Houston, TX, United States
- 6Department of Immunology, The University of Texas MD Anderson Cancer Center, Houston, TX, United States
Introduction: The tumor microbiome (TM) has been linked to pancreatic cancer prognosis. Specific microbes can confer tumor resistance to therapies. Early knowledge of the TM at time of diagnosis would be clinically relevant for precision therapy based on microbial composition. However, it is difficult to define the TM prior to surgical resection.
Methods: In this pilot feasibility study, patients underwent Endoscopic Ultrasound-Fine Needle Aspiration (EUS-FNA) biopsy of pancreatic adenocarcinoma. These samples were analyzed using 16S rRNA and internal transcribed spacer (ITS) sequencing for characterization of the tumor bacteria and fungi.
Result: After in silico decontamination and comparison to non-matched tumor, we were able to characterize the TM in biopsies, which was comparable to the TM from surgical specimens.
Discussion: EUS-FNA biopsy may represent a feasible modality to characterize the pancreatic TM prior to surgical resection with proper decontamination strategies and improvements in matched controls.
Introduction
There were an estimated 60,000 new cases and approximately 50,000 deaths due to pancreatic cancer (PC) in 2022 (1). Pancreatic ductal adenocarcinoma (PDAC) remains one of the most lethal of all cancers and is estimated to be the second highest cause of cancer-related mortality by 2030 (2). Endoscopic ultrasound (EUS) is used to evaluate the pancreas via transgastric and transduodenal imaging in high-risk individuals undergoing regular screening or in patients with a mass detected by other methods to confirm diagnosis (3). Samples are frequently taken for cytological analysis via fine needle aspiration (FNA) or histological assessment with core needle biopsy.
There has been an increase in the understanding of the gut and tumor microbiomes (TM) in cancer and their significant role in disease progression and responses to therapy (4–6). As a potential diagnostic tool, the gut microbiome was shown to be capable of identifying cases of PC amongst healthy controls and other benign diseases of the pancreas (7, 8). It was also determined that distinct TM signatures could be found between short- and long-term survivors of PC (4). In addition to the potential utility for diagnosis and determining prognostic factors, modulation of the gut and TM for greater immune activation and leverage against cancer has been postulated (9). PC is a heterogeneous disease process and precision oncology approaches will increasingly be required in the treatment of PC (10). In addition, manipulation of the TM by fecal microbial transplant is currently being investigated as a treatment modality against PC (NCT04975217). While the gut microbiome can be easily characterized, there is a deficiency of methods to detect the pancreatic TM prior to a surgical resection. Here we present the results of a pilot study to test feasibility of assessing bacteria and fungal populations in pancreatic tumors via EUS-FNA biopsy.
Methods
Specimen retrieval
Both 16s and ITS sequencing were performed on samples (n=5) obtained by use of Endoscopic Ultrasound and Fine Needle Aspiration biopsy (EUS-FNA biopsy) in individuals that underwent examination at M. D. Anderson Cancer Center (USA). Specimens were obtained under the informed consent of the MD Anderson Cancer Center (MDACC) IRB-approved protocol PA16-0911v07 (Analysis of the Microbiome in Patients with GI Cancer, at High Risk for GI Cancer, and Controls). Patients with known or suspected PDAC who were undergoing a clinically indicated EUS for fine needle aspiration cytology/biopsy and/or fiducials underwent two core biopsies of the pancreatic tumor for microbiome sequencing using Procore (Cook) 25G (n=4) and 22G (n=1) core biopsy needles using a transgastric (n=4) or transduodenal (n=1) approach. One of the five patients had received neoadjuvant chemotherapy (Gemcitabine/Abraxane) while the other four were treatment-naïve. Core biopsies were flash frozen, transferred in liquid nitrogen, and stored at -80 degrees Celsius until the time of DNA extraction. Patient surgical tumor samples were collected only after planned surgical resection and pathologic examination. Samples were similarly flash frozen, transferred in liquid nitrogen and stored at -80 degrees Celsius until DNA extraction. A total of 10 patient samples were obtained from pancreatectomies performed for PC from 2009 to 2019. The surgical tissue samples were collected under the Pancreas Tissue Bank, LAB00-396, and subsequently analyzed under a research-use protocol (PA16-0911).
Microbiome characterization
Sample DNAs were extracted with RNeasy Powerlyzer Tissue and Cell Kit (Qiagen, Cat. Number 69516) automated on the QIAcube Connect instruments. 16S rRNA and ITS2 gene sequencing methods were adapted from those developed for the NIH-Human Microbiome Project and the Earth Microbiome Project (11, 12). The 16S rDNA region v4 was amplified by polymerase chain reaction (PCR) and sequenced on the Illumina MiSeq platform in a 2x250 base pair paired-end protocol. The primers used for amplification contain adapters for MiSeq sequencing and single-index barcodes so that the PCR products may be pooled and sequenced directly, targeting at least 10,000 reads per sample. Raw paired-end 16S rRNA and ITS reads were merged into consensus fragments by FLASH (13) and subsequently filtered for quality (targeted error rate < 0.5%) and length (minimum 200bp) using Trimmomatic (14) and QIIME (15, 16). Spurious hits to the PhiX control genome were identified using BLASTN and removed. Passing sequences were trimmed of primers, evaluated for chimeras with UCLUST (17), and screened for human-associated contaminant using Bowtie2 (18). Chloroplast and mitochondrial contaminants were detected and filtered using the RDP classifier with a confidence threshold of 50% (19). High-quality passing 16S rRNA sequences were assigned to a high-resolution taxonomic lineage using Resphera Insight (20, 21). High-quality passing ITS sequences were clustered into OTUs by UCLUST (de novo mode) and assigned a taxonomic lineage using the RDP classifier with the UNITE database. Data analysis was carried out in R studio 2023.03.0, with the appropriate packages (plyr, ggplot). Venn diagram was performed with InteractiVenn (22).
Due to the passage of the endoscope per-orally through the esophagus and stomach and the nature of EUS-FNA biopsy (transgastric or transduodenal), there is a high propensity to be biased by microbial contaminants as well as intrinsic biases associated with 16S and ITS sequencing methods. In order to remove spurious genera commonly found as contaminants in 16S rRNA sequencing due to manipulation of samples and/or those commonly found as reagents and laboratory contaminants, we relied on curated exclusion lists previously published (4, 23) with supplementary correlation analysis against contaminant taxa to identify bacteria or fungi for exclusion. For fungi, contaminant removal was based on the identification of de novo OTUs rather than an exclusion list, and those with Spearman correlations exceeding 0.3 against any indicator contaminant OTU were also removed. Additional analysis also identified fungal genera such as Malassezia, Cystidiodontia and, Cladosporum as possible contaminants and they were later excluded (Supplementary Table S1). After contaminant filtering, 65 and 19 bacterial and fungal genera, respectively, remained in the analysis (Figure 1).
Results
At least seven bacterial genera (11%) were identified in all five samples sequenced (Actinomyces, Campylobacter, Fusobacterium, Granulicatella, Haemophilus, Prevotella, and Veilonella), with Prevotella as the most abundant (Figure 2A). When compared to fresh frozen surgical pancreatic tissue from unmatched individuals with PC (n= 10), we observed at least 35 (54%) genera in common between these two sample types (Figure 2B). This similarity is noteworthy considering that the surgical specimens were obtained from different individuals and matched irrespective of the anatomical portion of the pancreas or the collection moment (pre or post-chemotherapy treatment).
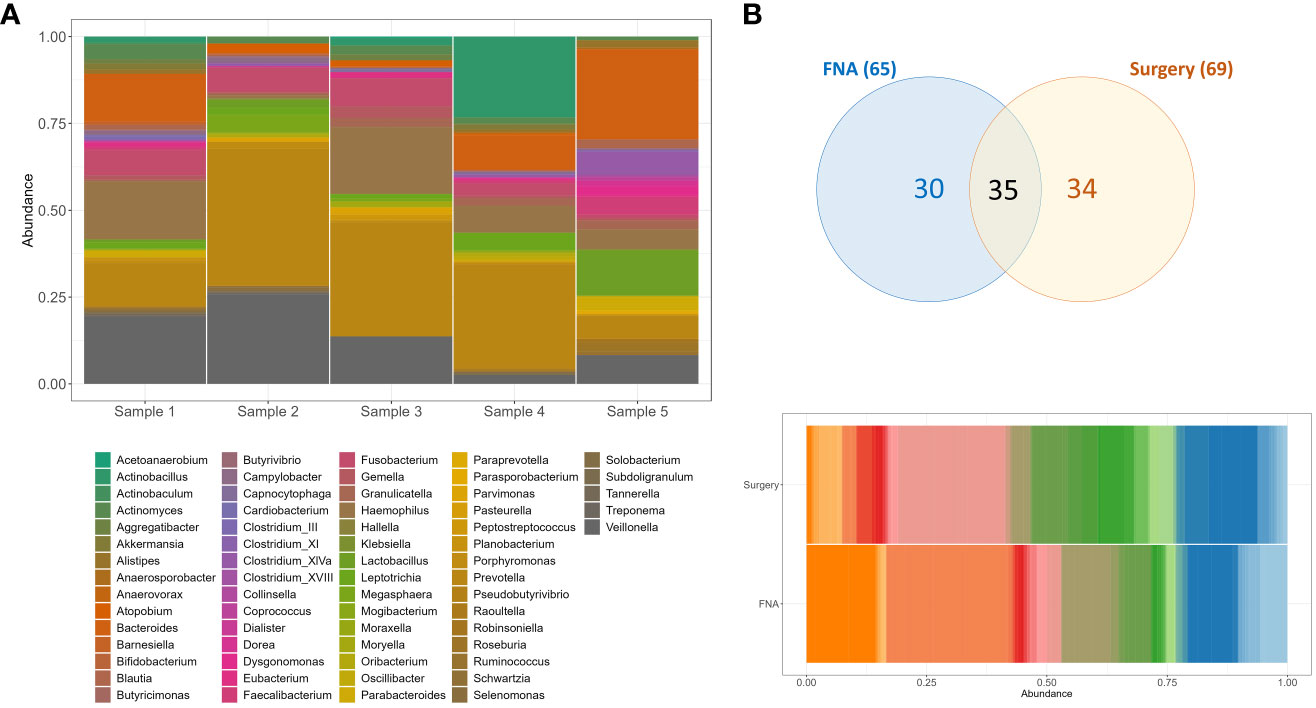
Figure 2 Bacteria genera composition of unmatched pancreatic FNA and surgical tissue samples obtained from individuals with PC. (A) Genera composition of bacteria per FNA sample. (B) Venn diagram and barplot of genera composition of surgical specimens (n=10) and FNA samples (n=5). Sample DNAs were extracted with RNeasy Powerlyzer Tissue and Cell Kit and region V4 (16s rRNA) sequenced by Illumina MiSeq v2 2x250 v1.8.
Among the unique genera (n=30) identified in the FNA biopsy samples we cannot disregard that some or most of them might represent bacteria from the oropharynx, esophagus, stomach or duodenum that could contaminate the endoscope and the pancreatic biopsy tissue during the procedure and the route used. Previous studies have identified Oribacterium, Atopobium, Klebsiella, Butyvibrio, Pasteurella, Tanerella, and Coprococcus in the mucosa derived from either duodenum or stomach samples (24–27). Other genera such as Fusobacterium, Haemophilus, Actinobacillus, Eubacterium, Actinobaculum, Bifidobacterium, Blautia, Veilonella, Prevotella and Lactobacillus were also identified in duodenum and stomach samples but were found in the FNA and the pancreatic surgical specimens as well (Supplementary Table S2) (24–27). These results are consistent with the notion that the human pancreas can share microbiota composition with gastrointestinal sites and ultimately the differential abundance of certain bacteria may be as relevant to consider as solely their presence or absence. The use of proper negative controls, amplification negative controls, and matched duodenum or stomach mucosa microbiome examination will help future decontamination strategies.
Recently, Nakano et al. (28) described the predominance of Acinetobacter and Pseudomonas in the pancreatic FNA samples in comparison to the duodenum and/or stomach mucosa from matched individuals, while in our study and many others (14, 29–31), these two genera were included on the putative contaminants list and were therefore excluded from the analysis. Additionally, these FNA samples were not compared to a matched resected tumor and it was not described whether the genera identified were solely in each sample type or shared among them (pancreatic FNA, mucosal duodenum, or stomach) (28). Similarly, Masi et al. (32) previously investigated the feasibility of using pancreatic formalin-fixed paraffin-embedded (FFPE) samples from a small number of EUS procedures to study the pancreatic microbiome and identified the existence of significant differences in the overall bacterial composition of the samples according to the route used for examination (duodenum or stomach), with Fusobacterium significantly enriched when the stomach route was used. In this sense, there is still a need to better comprehend the potential of the FNA pancreatic microbiome as a diagnostic tool for PC, including the bacterial composition of surrounding tissues and putative contaminants.
Additionally, in our cohort, two out of the five patients that underwent 16S sequencing successfully had their FNA fungal composition determined by ITS, supporting the efficacy of these minimally invasive endoscopically collected samples for complementary fungi-bacteria signature assessment. Despite the reduced number of FNAs tested, we observed relevant similarities in their fungal population even after decontamination, with high prevalence of Saccharomyces and Trichosporon (Figure 3). As most genera remain unknown for PC, there is a need to overcome experimental biases in vivo and silico associated with fungal identification by ITS sequencing in tumors.
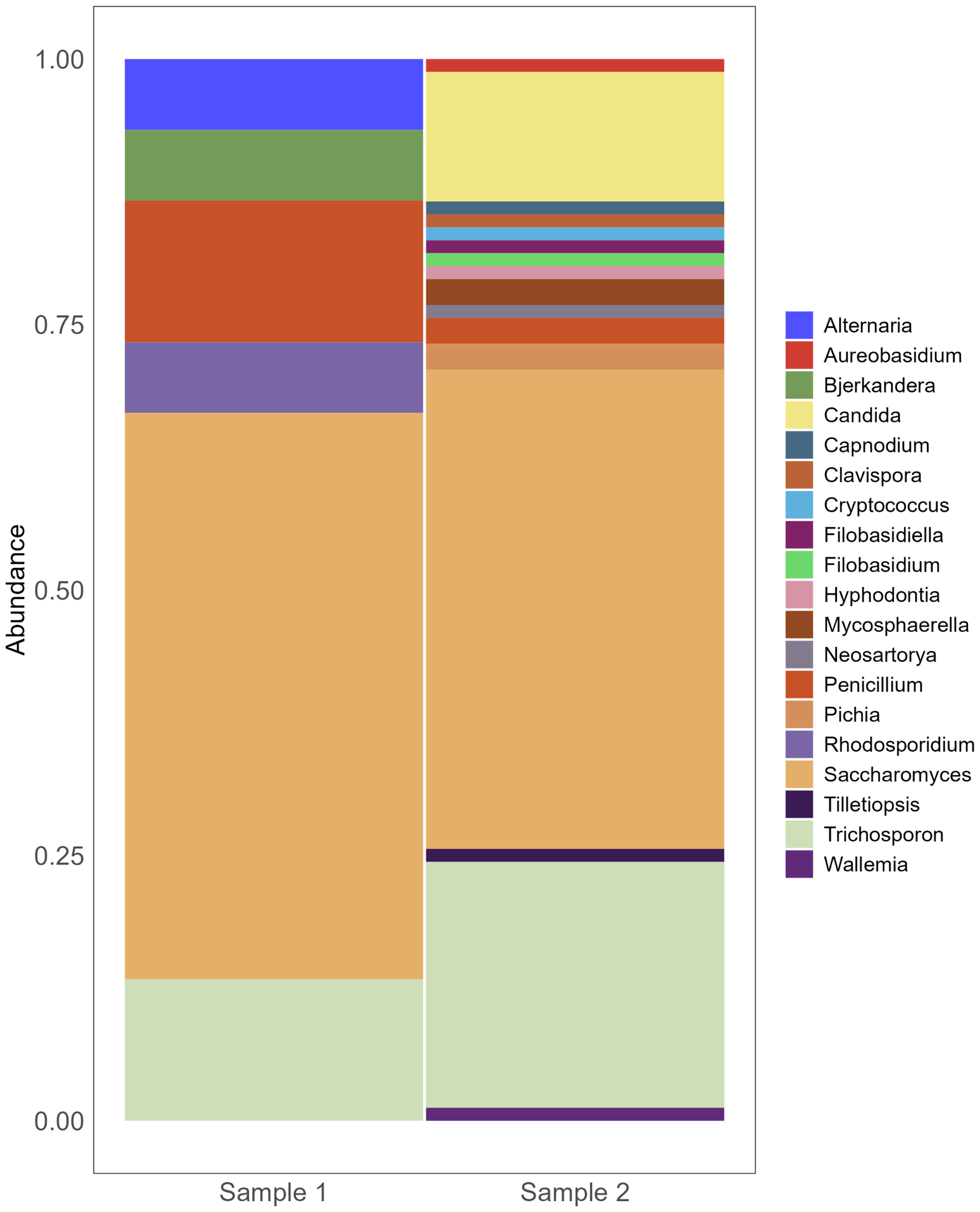
Figure 3 Fungi composition of pancreatic FNA obtained from individuals with PC. Barplot of genera composition per FNA sample individually (n=2). After DNAs extraction, ITS2 got sequenced by Illumina MiSeq v2 2x250 v1.8.
Discussion
We report the successful characterization of the pancreatic microbiome using EUS-FNA biopsy, including the first report of ITS sequencing from EUS-FNA biopsy samples. This method of microbial biopsy could be explored for use in diagnosis and prognosis. It has become the standard of practice to perform next generation sequencing (NGS) in all patients diagnosed with PC for precision oncology approaches (33). In the future, monitoring and manipulating the TM could expand individualized treatments with the understanding that certain bacterial signatures are associated with a favorable prognosis (4).
Alam et al. found that in mice, the intratumoral mycobiome of pancreatic lesions affects secretion of IL-33 which in turn induces type 2 immunity in pancreatic cancer. Both anti-fungal treatment or IL-33 suppression decreased TH2 infiltration and caused tumor regression (34, 35). These findings suggest alterations to the fungi, or mycobiome, in addition to intratumoral bacteria, may be another avenue of precision therapy.
Previously, duodenal fluid has been used as a surrogate marker for the pancreatic microbiome and it was found that patients with PDAC had lower microbial diversity in their duodenal fluid than controls with a normal pancreas (36). Techniques to accurately sample the microbiome of pancreatic lesions are urgently needed. Devices are being developed in order to meet this need (37), but conventional EUS-FNA biopsy may be a sufficient tool. It is also theoretically possible to perform immunoprofiling on the same EUS-FNA biopsy samples that are used to characterize the TM. Analysis of the microbiome and immune profile, in addition to the currently recommended NGS, could further inform directed therapies through multi-omic strategies.
We describe a characterization of the microbiome of pancreatic lesions using EUS-FNA biopsy combined with in silico decontamination. Our bacterial signature was conserved when compared to non-matched pancreatic tissue. We also used ITS sequencing to identify fungal species. Within a host-tissue background, direct sequencing of 16S rRNA and ITS amplicons offers a more direct and sensitive approach to assess microbial composition than whole-genome sequencing (WGS) or RNA-seq datasets, which are often dominated by host genomic DNA. Though samples are more routinely being taken for NGS in EUS-FNA for pancreatic lesions, the authors do not suggest extrapolating microbiome characterization from WGS data for the aforementioned reasons.
This proof of concept method could be utilized in the identification of the microbiome and mycobiome of pancreatic lesions prior to resection. Further investigation is needed with greater samples sizes, using mucosal tissue for normalization, and matched tissues for validation.
Data availability statement
The data presented in the study are deposited in the SRA under accession PRJNA1008674. The data can be found here https://www.ncbi.nlm.nih.gov/sra/?term=PRJNA1008674.
Ethics statement
The studies involving humans were approved by University of Texas MD Anderson Cancer Center- Institutional Review Board. The studies were conducted in accordance with the local legislation and institutional requirements. The participants provided their written informed consent to participate in this study.
Author contributions
RW: Conceptualization, Writing – original draft, Writing – review & editing. TB: Investigation, Methodology, Visualization, Conceptualization, Writing – original draft, Data curation, Formal Analysis. SB: Validation, Conceptualization, Formal Analysis, Investigation, Methodology, Visualization, Writing – original draft. JW: Data curation, Software, Writing – review & editing, Methodology, Validation, Visualization. MK: Investigation, Resources, Supervision, Writing – review & editing. MB: Conceptualization, Funding acquisition, Visualization, Writing – original draft, Investigation, Resources, Supervision, Writing – review & editing. FM: Methodology, Project administration, Validation, Conceptualization, Funding acquisition, Investigation, Supervision, Visualization, Writing – original draft, Writing – review & editing.
Funding
The authors declare financial support was received for the research, authorship, and/or publication of this article. RW is funded by the NIH T32 from Surgical Oncology (MDACC/PI: Funda Meric-Bernstam/Jennifer Wargo). FM received support from the National Cancer Institute (1R37CA237384), Cancer Prevention Research Institute of Texas (RP200173), Shelby Levine Pancreatic Scholar Program, V Foundation (Translational Award), Sabin Family Foundation and Robert L. Fine Cancer Research Foundation.
Conflict of interest
Author JW is founder and owner of Resphera Biosciences LLC. Author FM has a pending patent in microbiome profiling and is part of the SAB for Neologics Bio.
The remaining authors declare that the research was conducted in the absence of any commercial or financial relationships that could be construed as a potential conflict of interest.
Publisher’s note
All claims expressed in this article are solely those of the authors and do not necessarily represent those of their affiliated organizations, or those of the publisher, the editors and the reviewers. Any product that may be evaluated in this article, or claim that may be made by its manufacturer, is not guaranteed or endorsed by the publisher.
Supplementary material
The Supplementary Material for this article can be found online at: https://www.frontiersin.org/articles/10.3389/fimmu.2023.1268376/full#supplementary-material
Abbreviations
FNA, Fine Needle Aspiration; NOD, New Onset Diabetes; CT, Computed Tomography; MRCP, Magnetic Resonance Cholangiopancreatography; CA19-9, Carbohydrate antigen 19-9; IHC, Immunohistochemistry; TM, Tumor microbiome; ITS, Internal transcribed spacer.
References
1. Siegel RL, Miller KD, Fuchs HE, Jemal A. Cancer statistics, 2022. CA Cancer J Clin (2022) 72(1):7–33. doi: 10.3322/caac.21708
2. Rahib L, Wehner MR, Matrisian LM, Nead KT. Estimated projection of US cancer incidence and death to 2040. JAMA Netw Open (2021) 4(4):e214708. doi: 10.1001/jamanetworkopen.2021.4708
3. Das KK, Early D. Pancreatic cancer screening. Curr Treat Options Gastroenterol (2017) 15(4):562–75. doi: 10.1007/s11938-017-0149-8
4. Riquelme E, Zhang Y, Zhang L, Montiel M, Zoltan M, Dong W, et al. Tumor microbiome diversity and composition influence pancreatic cancer outcomes. Cell (2019) 178(4):795–806. doi: 10.1016/j.cell.2019.07.008
5. Parhi L, Alon-Maimon T, Sol A, Nejman D, Shhadeh A, Fainsod-Levi T, et al. Breast cancer colonization by Fusobacterium nucleatum accelerates tumor growth and metastatic progression. Nat Commun (2020) 11(1):3259. doi: 10.1038/s41467-020-16967-2
6. Spencer CN, McQuade JL, Gopalakrishnan V, McCulloch JA, Vetizou M, Cogdill AP, et al. Dietary fiber and probiotics influence the gut microbiome and melanoma immunotherapy response. Science (2021) 374(6575):1632–40. doi: 10.1126/science.aaz7015
7. Kartal E, Schmidt TSB, Molina-Montes E, Rodríguez-Perales S, Wirbel J, Maistrenko OM, et al. A faecal microbiota signature with high specificity for pancreatic cancer. Gut (2022) 71(7):1359–72. doi: 10.1136/gutjnl-2021-324755
8. Nagata N, Nishijima S, Kojima Y, Hisada Y, Imbe K, Miyoshi-Akiyama T, et al. Metagenomic identification of microbial signatures predicting pancreatic cancer from a multinational study. Gastroenterology (2022) 163(1):222–38. doi: 10.1053/j.gastro.2022.03.054
9. Chandra V, McAllister F. Therapeutic potential of microbial modulation in pancreatic cancer. Gut (2021) 70(8):1419–25. doi: 10.1136/gutjnl-2019-319807
10. Seo YD, Katz MHG. Preoperative therapy for pancreatic adenocarcinoma-precision beyond anatomy. Cancer (2022) 128(16):3041–56. doi: 10.1002/cncr.34273
11. Gevers D, Knight R, Petrosino JF, Huang K, McGuire AL, Birren BW, et al. The Human Microbiome Project: a community resource for the healthy human microbiome. PloS Biol (2012) 10(8):e1001377. doi: 10.1371/journal.pbio.1001377
12. Thompson LR, Sanders JG, McDonald D, Amir A, Ladau J, Locey KJ, et al. Earth Microbiome Project Consortium. A communal catalogue reveals Earth's multiscale microbial diversity. Nature (2017) 551(7681):457–63.
13. Magoč T, Salzberg SL. FLASH: fast length adjustment of short reads to improve genome assemblies. Bioinformatics (2011) 27(21):2957–63. doi: 10.1093/bioinformatics/btr507
14. Bolger AM, Lohse M, Usadel B. Trimmomatic: a flexible trimmer for Illumina sequence data. Bioinformatics (2014) 30(15):2114–20. doi: 10.1093/bioinformatics/btu170
15. Caporaso JG, Kuczynski J, Stombaugh J, Bittinger K, Bushman FD, Costello EK, et al. QIIME allows analysis of high-throughput community sequencing data. Nat Methods (2010) 7(5):335–6. doi: 10.1038/nmeth.f.303
16. Kuczynski J, Stombaugh J, Walters WA, González A, Caporaso JG, Knight R. Using QIIME to analyze 16S rRNA gene sequences from microbial communities. Curr Protoc Bioinf (2011). Dec;Chapter 10:10.7.1-10.7.20. doi: 10.1002/0471250953.bi1007s36
17. Edgar RC. Search and clustering orders of magnitude faster than BLAST. Bioinformatics (2010) 26(19):2460–1. doi: 10.1093/bioinformatics/btq461
18. Langmead B, Salzberg SL. Fast gapped-read alignment with Bowtie 2. Nat Methods (2012) 9(4):357–9. doi: 10.1038/nmeth.1923
19. Wang Q, Garrity GM, Tiedje JM, Cole JR. Naive Bayesian classifier for rapid assignment of rRNA sequences into the new bacterial taxonomy. Appl Environ Microbiol (2007) 73(16):5261–7. doi: 10.1128/AEM.00062-07
20. Drewes JL, White JR, Dejea CM, Fathi P, Iyadorai T, Vadivelu J, et al. High-resolution bacterial 16S rRNA gene profile meta-analysis and biofilm status reveal common colorectal cancer consortia. NPJ Biofilms Microbiomes (2017) 3:34. doi: 10.1038/s41522-017-0040-3
21. Daquigan N, Seekatz AM, Greathouse KL, Young VB, White JR. High-resolution profiling of the gut microbiome reveals the extent of Clostridium difficile burden. NPJ Biofilms Microbiomes (2017) 3:35. doi: 10.1038/s41522-017-0043-0
22. Heberle H, Meirelles GV, da Silva FR, Telles GP, Minghim R. InteractiVenn: a web-based tool for the analysis of sets through Venn diagrams. BMC Bioinf (2015) 16(1):169. doi: 10.1186/s12859-015-0611-3
23. Salter SJ, Cox MJ, Turek EM, Calus ST, Cookson WO, Moffatt MF, et al. Reagent and laboratory contamination can critically impact sequence-based microbiome analyses. BMC Biol (2014) 12:87. doi: 10.1186/s12915-014-0087-z
24. Nardelli C, Granata I, D'Argenio V, Tramontano S, Compare D, Guarracino MR, et al. Characterization of the duodenal mucosal microbiome in obese adult subjects by 16S rRNA sequencing. Microorganisms (2020) 8(4):485. doi: 10.3390/microorganisms8040485
25. Leite G, Morales W, Weitsman S, Celly S, Parodi G, Mathur R, et al. The duodenal microbiome is altered in small intestinal bacterial overgrowth. PloS One (2020) 15(7):e0234906. doi: 10.1371/journal.pone.0234906
26. Cervantes J, Michael M, Hong BY, Springer A, Guo H, Mendoza B, et al. Investigation of oral, gastric, and duodenal microbiota in patients with upper gastrointestinal symptoms. J Investig Med (2020), jim–2020-001642. doi: 10.1136/jim-2020-001642
27. Nardone G, Compare D. The human gastric microbiota: Is it time to rethink the pathogenesis of stomach diseases? United Eur Gastroenterol J (2015) 3(3):255–60. doi: 10.1177/2050640614566846
28. Nakano S, Kawamoto Y, Komatsu Y, Saito R, Ito K, Yamamura T, et al. Analysis of the pancreatic cancer microbiome using endoscopic ultrasound-guided fine-needle aspiration-derived samples. Pancreas (2022) 51(4):351–7. doi: 10.1097/MPA.0000000000002028
29. Kulakov LA, McAlister MB, Ogden KL, Larkin MJ, O'Hanlon JF. Analysis of bacteria contaminating ultrapure water in industrial systems. Appl Environ Microbiol (2002) 68(4):1548–55. doi: 10.1128/AEM.68.4.1548-1555.2002
30. Newsome T, Li BJ, Zou N, Lo SC. Presence of bacterial phage-like DNA sequences in commercial Taq DNA polymerase reagents. J Clin Microbiol (2004) 42(5):2264–7. doi: 10.1128/JCM.42.5.2264-2267.2004
31. Laurence M, Hatzis C, Brash DE. Common contaminants in next-generation sequencing that hinder discovery of low-abundance microbes. PloS One (2014) 9(5):e97876. doi: 10.1371/journal.pone.0097876
32. Masi AC, Oppong YEA, Haugk B, Lamb CA, Sharp L, Shaw JM, et al. Endoscopic ultrasound (EUS)-guided fine needle biopsy (FNB) formalin fixed paraffin-embedded (FFPE) pancreatic tissue samples are a potential resource for microbiota analysis. Gut (2021) 70(5):999–1001. doi: 10.1136/gutjnl-2020-322457
33. National Comprehensive Cancer Network. Pancreatic adenocarcinoma (V.1.2022). Available at: http://www.nccn.org/professionals/physician_gls/pdf/pancreaticadenocarcinoma.pdf. (Accessed February 28 2023).
34. Alam A, Levanduski E, Denz P, Villavicencio HS, Bhatta M, Alhorebi L, et al. Fungal mycobiome drives IL-33 secretion and type 2 immunity in pancreatic cancer. Cancer Cell (2022) 40(2):153–167.e11. doi: 10.1016/j.ccell.2022.01.003
35. Li X, Saxena D. The mycobiome-immune axis: The next frontier in pancreatic cancer. Cancer Cell (2022) 40(2):120–2. doi: 10.1016/j.ccell.2022.01.009
36. Kohi S, Macgregor-Das A, Dbouk M, Yoshida T, Chuidian M, Abe T, et al. Alterations in the duodenal fluid microbiome of patients with pancreatic cancer. Clin Gastroenterol Hepatol (2022) 20(2):e196–227. doi: 10.1016/j.cgh.2020.11.006
Keywords: tumor microbes, pancreatic cancer, endoscopic ultrasound, fine needle aspiration, microbiome, pancreatic adenocarcinoma
Citation: Wright RD, Bartelli TF, Baydogan S, White JR, Kim MP, Bhutani MS and McAllister F (2023) Bacterial and fungal characterization of pancreatic adenocarcinoma from Endoscopic Ultrasound-guided biopsies. Front. Immunol. 14:1268376. doi: 10.3389/fimmu.2023.1268376
Received: 27 July 2023; Accepted: 18 September 2023;
Published: 13 October 2023.
Edited by:
Scott Kenneth Durum, National Cancer Institute (NIH), United StatesReviewed by:
Ayssar A. Elamin, LIONEX GmbH, GermanyRebecca Leigh Schmidt, Colorado Mountain College, United States
Copyright © 2023 Wright, Bartelli, Baydogan, White, Kim, Bhutani and McAllister. This is an open-access article distributed under the terms of the Creative Commons Attribution License (CC BY). The use, distribution or reproduction in other forums is permitted, provided the original author(s) and the copyright owner(s) are credited and that the original publication in this journal is cited, in accordance with accepted academic practice. No use, distribution or reproduction is permitted which does not comply with these terms.
*Correspondence: Florencia McAllister, Rk1jQWxsaXN0ZXJAbWRhbmRlcnNvbi5vcmc=; Manoop S. Bhutani, bWFub29wLmJodXRhbmlAbWRhbmRlcnNvbi5vcmc=
†These authors have contributed equally to this work and share first authorship