- 1Unit of Clinical and Experimental Immunology, IRCCS Humanitas Research Hospital, Rozzano, Milan, Italy
- 2Department of Medical Biotechnologies and Translational Medicine (BioMeTra), University of Milan, Milan, Italy
- 3Department of Biomedical Sciences, Humanitas University, Milan, Italy
- 4Laboratory of Translational Immunology, IRCCS Humanitas Research Hospital, Milan, Italy
- 5Bone Marrow Transplant Unit, IRCCS Humanitas Research Hospital, Milan, Italy
- 6Molecular Biology Section, Clinical Investigation Laboratory, IRCCS Humanitas Research Hospital, Milan, Italy
- 7Institute for Virology, University Hospital Essen, University of Duisburg-Essen, Essen, Germany
Haploidentical hematopoietic stem cell transplantation (h-HSCT) is a therapeutic option to cure patients affected by hematologic malignancies. The kinetics and the quality of immune-reconstitution (IR) impact the clinical outcome of h-HSCT and limit the onset of life-threatening Human Cytomegalovirus (HCMV) infection/reactivation. Natural Killer (NK) cells are the first lymphocytes that recover after h-HSCT and they can provide rapid innate immune responses against opportunistic pathogens. By performing a longitudinal single-cell analysis of multiparametric flow-cytometry data, we show here that the persistence at high frequencies of CD158b1b2jneg/NKG2Apos/NKG2Cneg/NKp30pos/NKp46pos (KIRneg) NK cells is associated with HCMV infection/reactivation control. These KIRneg NK cells are “unlicensed”, and are not terminal-differentiated lymphocytes appearing early during IR and mainly belonging to CD56bright/CD16neg and CD56bright/CD16pos subsets. KIRneg NK cells are enriched in oxidative and glucose metabolism pathways, produce interferon-γ, and are endowed with potent antiviral activity against HCMV ex vivo. Decreased frequencies of KIRneg NK cells early during IR are associated with clinically relevant HCMV replication. Taken together, our findings indicate that the prolonged persistence of KIRneg NK cells after h-HSCT could serve as a biomarker to better predict HCMV infection/reactivation. This phenomenon also paves the way to optimize anti-viral immune responses by enriching post-transplant donor lymphocyte infusions with KIRneg NK cells.
1 Introduction
Human cytomegalovirus (HCMV) is a β-herpesvirus that establishes lifelong latent infections and is estimated to affect about 60% of the western population. While the reactivation of HCMV is physiologically controlled by the immune system, it represents a severe condition in immune-compromised patients, including those receiving an allogeneic hematopoietic stem cell transplantation (HSCT) (1–5). Haploidentical HSCT (h-HSCT) with post-transplant Cyclophosphamide (PT-Cy) is one of the most effective platforms to cure hematologic malignancies and allows to find a donor for nearly every patient in need, including those aged 60 years or older, thanks to the reduced toxicity of the conditioning regimens (6, 7). In this context, the kinetics and quality of innate immune reconstitution (IR) emerged as predictors of transplant outcomes and adverse reaction onset (8). Natural Killer (NK) cells are the first lymphocytes to recover in the first month after h-HSCT and play essential roles in clearing residual leukemic cells and in limiting post-transplant complications (9–12). Despite the availability of diagnostics and antiviral drugs, productive HCMV replication, as a consequence of infection/reactivation (HCMV-I/R), represents one of the main life-threatening adverse events after h-HSCT (1).
HCMV greatly impacts NK cell homeostasis, maturation, and IR following HSCT by promoting the expansion of NKG2Cpos NK cells with adaptive properties (13–15). Accordingly, we previously confirmed that HCMV-I/R after h-HSCT also accelerates the maturation of NK cells in a h-HSCT setting. Moreover, we demonstrated that HCMV-I/R after h-HSCT is associated with the appearance of mature and adaptive-like CD158b1b2jpos/NKG2Aneg/NKG2Cpos/NKp30lo NK cells that have impaired anti-viral effector-functions. The expansion of these adaptive-like and functionally impaired NK cells was associated not only with an increased reactivation of HCMV, but also with a higher probability of acute Graft versus Host Disease (GvHD) onset (16).
However, very little is known about the possible appearance or expansion of other NK cell subsets able to control viral infections soon after h-HSCT. Herein, by undertaking a longitudinal profile of immune-reconstituting NK cells by high-dimensional flow cytometry, we identified an NK cell subpopulation expanded early after h-HSCT that could protect h-HSCT recipients from HCMV-I/R.
2 Materials and methods
2.1 Patient recruitment and sample collection
This study included a total of 30 consecutive patients (Table 1) affected by hematologic malignancies who underwent a T-cell replete h-HSCT with post-transplant Cyclophosphamide (16) and their relative donors who were enrolled at the Bone Marrow Transplant Unit, IRCCS Humanitas Research Hospital, Rozzano, Milan, Italy between 2011 and 2016.
Written informed consent was obtained from all patients and donors prior to sample collection, in accordance with the Declaration of Helsinki. Protocol approval was obtained from the Institutional Review Boards of the Clinical and Research Institute Humanitas (Approval 24/18).
The HCMV-I/R was evaluated in the peripheral blood of both donors and recipients before the transplant and monitored weekly in the recipients after h-HSCT by assessing the HCMV viral load through real-time PCR (CMV R-GENE, Argene, Biomérieux). When a viral load greater than 2,000 IU/mL was detected, recipients were considered as HCMV-reactivated. Preemptive therapy (Foscarnet 90 mg/kg or Ganciclovir 5 mg/kg and/or Valganciclovir, twice a day for 2 weeks) was given to reactivated patients with a viral load exceeding 4,000 IU/mL. All patients included in this study were enrolled before the introduction of Letermovir as HCMV prophylaxis.
Peripheral blood samples were obtained from both donors and recipients before h-HSCT, and from the recipients monthly until 1-year post-h-HSCT. Peripheral blood mononuclear cells (PBMCs) were isolated using standardized density gradient techniques (Lympholyte-H, Cedarlane) and frozen in liquid nitrogen according to standard procedures.
2.2 Polychromatic flow cytometry and PhenoGraph analysis
To minimize variability, the flow cytometry experiments were conducted in batches on frozen cells. Frozen cells were thawed and stained for 15 minutes at room temperature (RT) with Zombie Aqua™ Fixable Viability Kit (Biolegend) and 20 minutes at RT with fluorescent-conjugated monoclonal antibody mix (16).
Samples were acquired at BD FACSymphony A5 flow cytometer (BD Bioscience).
Flow Cytometry Standard (FCS) 3.0 files were imported into FlowJo software (version 9.9.6., TreeStar) and analyzed by standard manual gating strategy. NK cells were identified among viable single cells as Lineageneg (CD3neg/CD14neg/CD4neg/CD15neg/CD20neg/CD19neg/CD33neg/CD34neg/CD203cneg/FCϵRneg) lymphocytes. Two thousand NK cells/sample were imported into FlowJo (v 10.2.0) to perform a biexponential transformation. Data were then exported in Python (v 3.7.3) and subjected to the Cytophenograph pipeline (http://github.com/luglilab/Cytophenograph) (16). The K-value was set at 45. New CSV files were then saved, and data were further analyzed in FlowJo (v 10.2.0). Clusters with a frequency lower than 0.5% were excluded from the analysis. Metaclustering was then performed using the gplots R package and PhenoGraph clusters were visualized using the Uniform Manifold Approximation and Projection (UMAP) dimension reduction technique.
2.3 In vitro HCMV infections
The EndoGRO HUVEC (MilliporeSigma) were cultured in cell flasks precoated with Collagen I (Corning) at 105-106 cells/mL by using the endothelial cell growth medium-2 (EGM-2) (Lonza) containing 5% FBS (Lonza), 1% Penicillin-Streptomycin (Invitrogen), and 1% UltraGlutamine (Lonza). The bacterial artificial chromosome (BAC) clone of the HCMV strain TB40/E expressing enhanced green fluorescent protein (EGFP) (17) was used to infect HUVEC at a multiplicity of infection (MOI) of 1 for 12 hours.
CD158b1b2jneg NK cells were purified from PBMCs of NR and R recipients at 8-12 months after h-HSCT (Supplementary Table 2) by using a BD FACSAria III cell sorter (BD Bioscience). FACS-sorted CD158b1b2jneg NK cells were then incubated overnight at 37°C in 5% CO2 in RPMI medium containing 10% FBS, 1% Penicillin-Streptomycin, and 1% UltraGlutamine (RPMI complete) and supplemented with 100 IU/mL rhIL-2 and 100 IU/mL rhIL-12. CD158b1b2jneg NK cells were then cocultured with infected HUVECs for 72 hours in RPMI complete medium containing rhIL-2 (200 IU/mL). The images were acquired with the DMi8 Wide-Field Microscope (Leica). For each well, four representative fields were analyzed by counting the number of fluorescent-infected HUVECs by using ImageJ software (NIH).
2.4 Enzyme-linked immunosorbent assay
Interferon-γ (IFN-γ) production was quantified on the supernatant of purified CD158b1b2jneg NK cells co-cultured for 72 hours in the presence of HCMV-infected HUVEC using the Human IFN-gamma DuoSet ELISA (R&D Systems), according to manufacturer’s instructions.
2.5 Total RNA sequencing
FACS-sorted CD158b1b2jneg NK cells were lysed in 49 μL of RLT buffer (Qiagen) containing 1 μL of RNAse inhibitor (Thermo Fisher Scientific) and stored at -80°C.
The MicroRNAeasy KitTM with DNAse (Qiagen) was used to purify total RNA, which was then quantified by a Nanodrop 2000 (Thermo Fisher Scientific). Starting from 0.5 ng of high-quality total RNA with an RNA Integrity Number (RIN) greater than 6, assessed using a 4200 Tape Station (Agilent), libraries were prepared with the SMART-Seq Stranded Kit (Clontech-Takara). Libraries were then multiplexed in equimolar pools and sequenced by using a NextSeq-550 Illumina Platform. At least 60 million 75bp-paired-end reads per sample were generated. Read alignment, differential gene expression, and functional enrichment analyses were performed as previously described (16).
2.6 Statistics
GraphPad PRISM (version 9.0) and R statistical software were used to perform statistical analysis. Different variables among samples were compared by unpaired, two-tailed Student’s t-test. Different variables within the same sample were compared by using a paired, two-tailed Student’s t-test. Results are presented as the mean ± standard deviation (SD) or as the mean ± standard error of mean (SEM) when repeated sampling was considered. Two-sided or one-sided P values were considered significant when P ≤ 0.05.
3 Results
3.1 Patient characteristics
A total of 30 patients affected by hematologic diseases and receiving a T-cell replete h-HSCT with PT-Cy were enrolled in this study (Table 1; Supplementary Table 1). Peripheral blood mononuclear cells (PBMCs) were collected from recipients at different time points after transplant till one year after and from their relative HSC donors (16). HCMV-I/R was observed in 10/30 (33.3%) haplo-HSCT recipients with median onset at +40.5 days after h-HSCT (range 21-116).
Acute GvHD requiring systemic therapy was observed in 6/10 HCMV not-reactivated and 12/20 HCMV reactivated patients with a median day of onset at day 41 (range 19-104). Chronic GvHD was observed in 4/30 patients, three of whom belong to the HCMV reactivated group.
3.2 Longitudinal analysis of NK cells identifies NK cell clusters persisting in NR patients
We first performed a longitudinal analysis of high-dimensional flow cytometry data on circulating NK cells. To investigate the differences in NK cell immune reconstitution according to HCMV-I/R, h-HSCT recipients were stratified into two groups, namely either experiencing (R, n=20) or not (NR, n=10) the HCMV-I/R that generally occurs within the first 2-3 months after h-HSCT. NK cell data were next concatenated, analyzed with Cytophenograph pipeline, and visualized using Uniform Manifold Approximation and Projection (UMAP) (Figure 1A). These analyses identified 28 clusters of phenotypically distinct NK cells (16). We next focused our attention on four clusters (2, 3, 7, and 8) detectable at high frequencies during the first weeks after h-HSCT prior to HCMV-I/R in NR and R (Figure 1B). We observed that the percentages of these NK cell clusters started to decline in R at 3-4 months after h-HSCT, while their frequencies remained high in NR even after 12 months from the transplant (Figure 1C). A common phenotype of clusters 2, 3, 7, and 8 was the absence of Killer Immunoglobulin-like Receptors (KIR) CD158b1b2j, low expression of NKG2C, and high levels of NKG2A, NKp30, and NKp46.
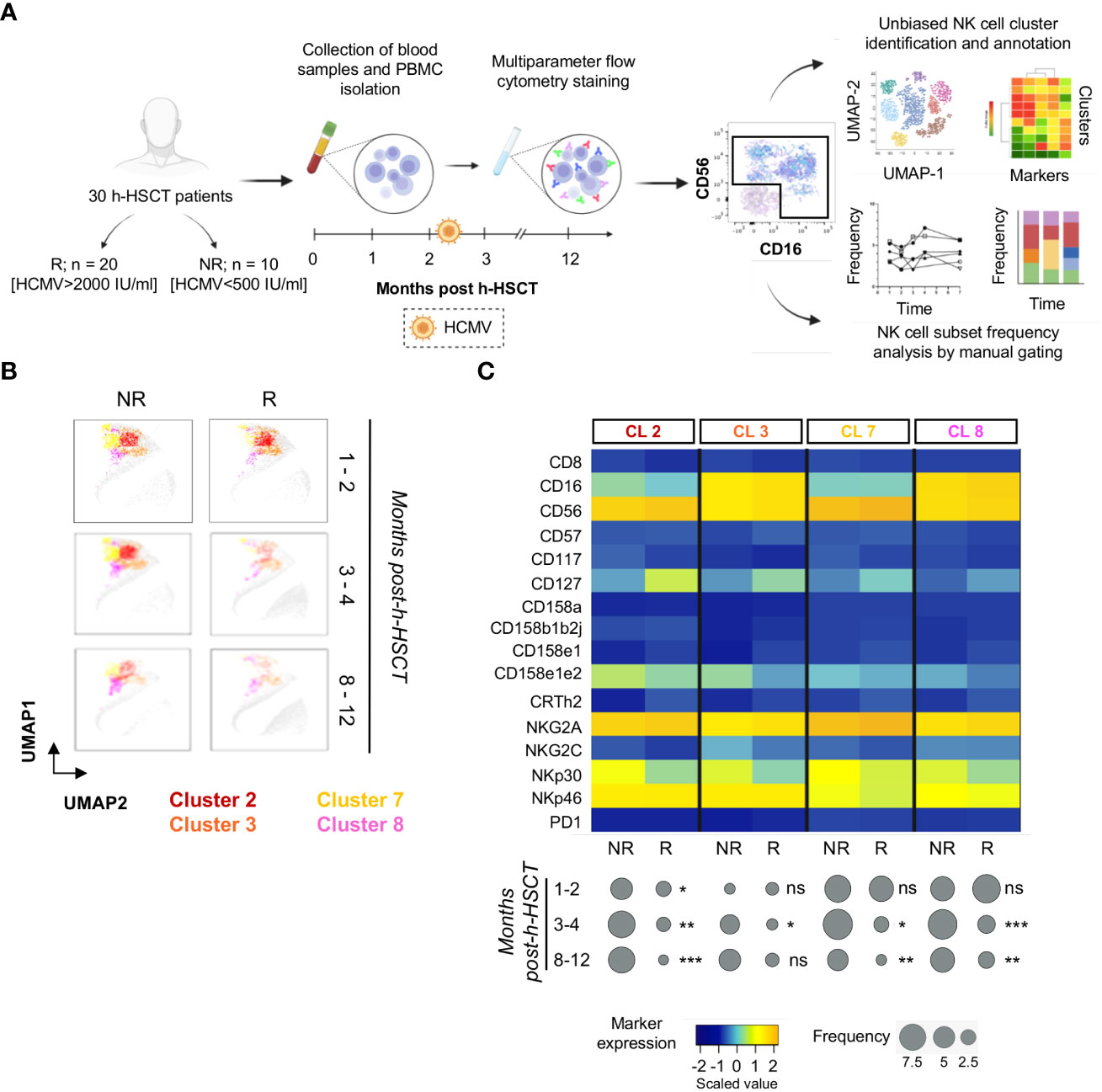
Figure 1 NK cells from HCMV not-reactivated recipients are characterized by a CD158b1b2jneg/NKG2Apos/NKG2Cneg/NKp30pos/NKp46pos phenotype. (A) Experimental workflow: peripheral blood mononuclear cells (PBMCs) were isolated from a total of 30 patients affected by hematologic diseases and receiving a T-cell replete h-HSCT with post-transplant Cyclophosphamide longitudinally for 1 year after h-HSCT. Patients were stratified by HCMV not-reactivated (NR) or reactivated (R). PBMCs were next stained and analyzed by multiparametric flow cytometry. NK cells, gated as viable Lineageneg lymphocytes, were examined by PhenoGraph (upper panel), an unbiased method that uses marker expression to define the k-nearest neighbor for each single cell and then group and stratify cells in clusters, as well as by classical manual gating strategy (lower panel) to evaluate their phenotype and frequencies over time of the different NK cell subpopulations. (B) UMAP plots showing the distribution of clusters 2, 3, 7, and 8 in NR (left panels) and R (right panels) recipients before (1-2 months), soon after (3-4 months), and late after (8-12 months) HCMV infection/reactivation. NK cell clusters were overlaid with the total NK cell distribution (gray background). (C) Heatmap displaying the expression of NK cell markers on clusters (CL) 2, 3, 7, and 8 in NR (n=10) and in R (n=20) h-HSCT patients (upper panel). The median frequencies of each PhenoGraph NK cell cluster in NR and R patients at 1-2, 3-4, and 8-12 months after h-HSCT is shown in balloon plots (lower panel). Student's t-test NR vs R, *p<0.05, **p<0.01, ***p<0.001, ns: not significant.
3.3 CD158b1b2jneg/NKG2Apos/NKG2Cneg/NKp30pos/NKp46pos NK cells are expanded in NR patients
The manual gating strategy analysis confirmed that CD158b1b2jneg NK cells were significantly enriched in NR patients, who also expressed high levels of NKG2A, NKp30, and NKp46 and lower levels of NKG2C (Figure 2A).
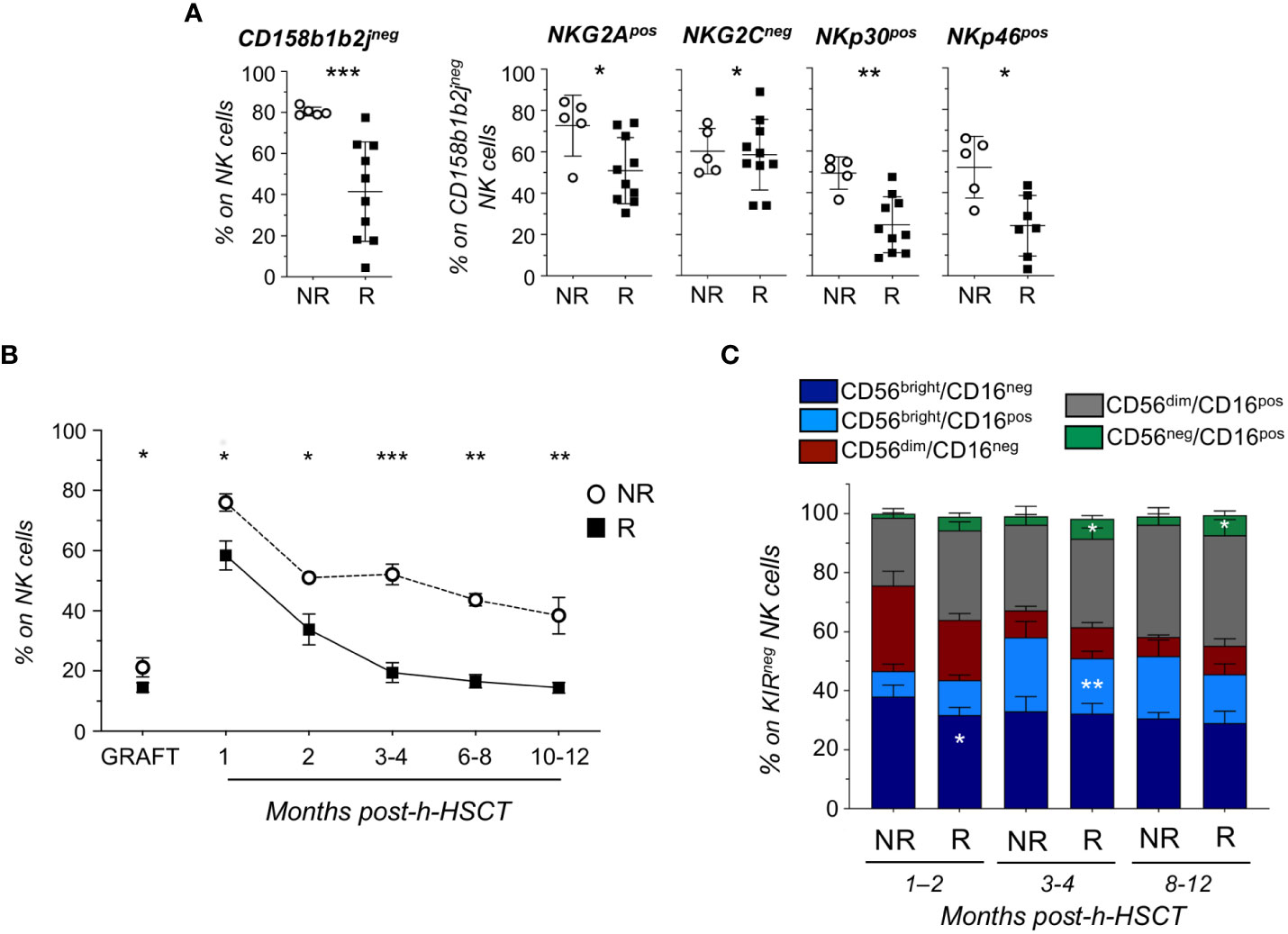
Figure 2 Longitudinal analysis reveals that KIRneg NK cells persist in HCMV not-reactivated recipients up to 1-year post-h-HSCT and are mainly composed by less differentiated subsets. (A) Relative frequencies of CD158b1b2jneg on total NK cells (left panel) and of NKG2Apos, NKG2Cneg, NKp30pos, and NKp46pos on CD158b1b2jneg NK cells in NR (○; n=5) and R (▪; n=10) recipients at 8-12 months after h-HSCT. (B) Summary statistical graph depicting the frequency (%, mean ± SEM) of CD158b1b2jneg/NKG2Apos/NKG2Cneg/NKp30pos/NKp46pos NK cells on total NK cells in NR (○; n=10) and R (▪; n=20) recipients and their grafts (NR=6; R=13) at 1, 2, 3-4, 6-8, and 10-12 months after the h-HSCT. (C) Histograms showing the NK cell subset distribution (%; mean ± SD) within CD158b1b2jneg/NKG2Apos/NKG2Cneg/NKp30pos/NKp46pos (KIRneg) NK cells in NR and R at 1-2, 3-4, and 8-12 months after h-HSCT. Student’s t-test NR vs R, *p<0.05, **p<0.01, ***p<0.001.
By combining these markers differentially expressed in R and NR, we identified a novel CD158b1b2jneg/NKG2Apos/NKG2Cneg/NKp30pos/NKp46pos (KIRneg) NK cell subset. This NK cell subpopulation was detectable at significantly higher frequencies in NR compared to R at all time points analyzed. Moreover, the percentage of KIRneg NK cells started to decrease in R after 2 months from the transplant and further declined after the onset of HCMV-I/R. Conversely, circulating KIRneg NK cells persisted more in NR compared to R even 12 months after the transplant (Figure 2B; Supplementary Figure 1). Of note, we also found significantly higher amounts of KIRneg NK cells in the grafts of NR compared to those of R (Figure 2B), regardless of their HCMV serostatus and donor age (Supplementary Figure 2).
By assessing the NK cell subset distribution among CD158b1b2jneg/NKG2Apos/NKG2Cneg/NKp30pos/NKp46pos NK cells, we observed that they mainly belonged to less mature CD56bright/CD16neg, CD56bright/CD16pos, and CD56dim/CD16neg NK cell subsets in both NR and R and at all time points considered (10, 18). Moreover, we demonstrated that CD56bright/CD16neg and CD56bright/CD16pos NK cells with a CD158b1b2jneg/NKG2Apos/NKG2Cneg/NKp30pos/NKp46pos phenotype were present at significantly higher frequencies in NR compared to R at 1-2 months and 3-4 months after h-HSCT, respectively. In clear contrast and in line with previous findings (8, 19), our data showed that HCMV-I/R induced a significant expansion of terminally differentiated CD56neg/CD16pos NK cells in R, considering both total and KIRneg NK cells (Figure 2C; Supplementary Figure 3A).
We next focused our attention on CD56bright/CD16pos NK cells, a neglected subset of unknown origin, which is present at very low frequency under homeostatic conditions or during post-h-HSCT IR (10, 18, 20). Our data demonstrated that CD56bright/CD16pos NK cell frequencies and absolute counts were significantly higher in NR in the first months after the transplant (Figure 2C; Supplementary Figures 3A, B). Phenotypic features of CD56bright/CD16pos NK cells place them in an intermediary maturation stage between CD56bright/CD16neg and CD56dim/CD16pos in term of differentiation markers (CD117, CD127, T-bet, EOMES, and CD57), KIRs (CD158b1b2j and CD158e1e2), activating and inhibitory receptors (NKG2A, NKG2C, NKp30, NKp46, and NKp44), and cytolytic potential (PerforinA and GranzymeB) (Figure 3).
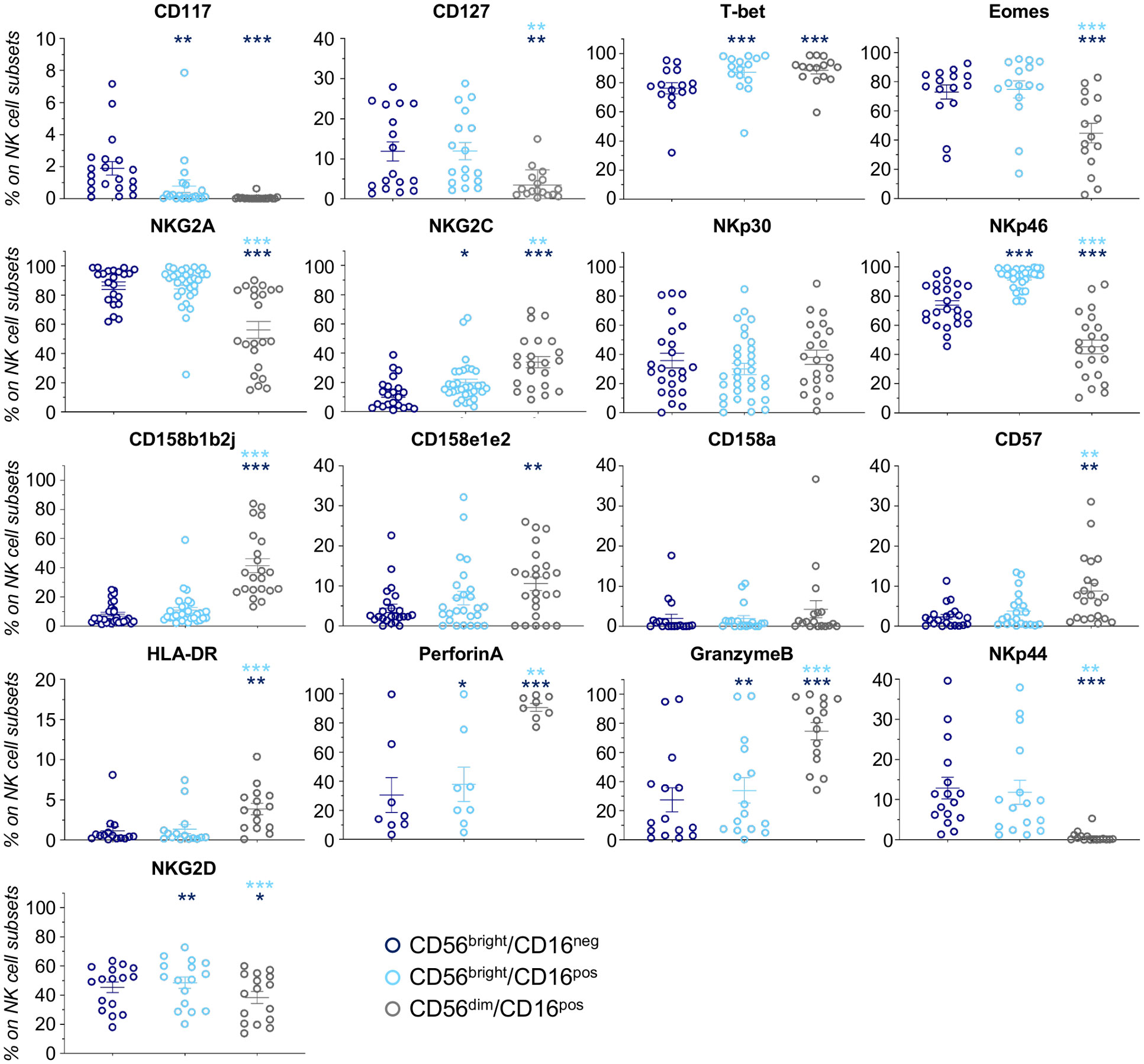
Figure 3 NK cell markers in CD56bright/CD16neg, CD56bright/CD16pos, and CD56dim/CD16pos NK cells. Scatter plots showing expression (%; mean ± SEM) of markers involved in NK cell differentiation (CD117, CD127, T-bet, and Eomes), maturation/licensing (NKG2A, NKG2C, CD158b1b2j, CD158e1e2, CD158a, and CD57), activation (NKp44 and HLA-DR), and function (PerforinA, GranzymeB, NKp30, and NKp46) in h-HSCT patients at 3-4 months after the transplant. CD56bright/CD16neg (blue) CD56bright/CD16pos (light blue) and CD56dim/CD16pos (gray) Paired t-test, *p<0.05, **p<0.01, ***p<0.001 (light blue vs CD56bright/CD16neg, blue vs CD56bright/CD16pos).
3.4 KIRneg NK cells show a higher anti-viral potential
To assess the functional relevance of KIRneg NK cells in controlling HCMV infection, we investigated their ability to prevent HCMV replication ex vivo (16, 20). To obtain enough purified NK cells that should recapitulate those NK cell clusters highly enriched in NR, CD158b1b2jneg NK cells were FACS-sorted from NR (n=4) and R (n=4) at 8-12 months (Supplementary Table 2) and co-cultured with HUVEC cells infected with the BAC clone of the HCMV strain TB40/E expressing EGFP for a maximum of 72 hours. Every 24 hours cells were observed with a microscope and the number of green infected HUVEC cells was counted. Our results first showed that CD158b1b2jneg NK cells diminished the HCMV spread in permissive endothelial cells. Of note, CD158b1b2jneg NK cells from NR were able to completely suppress HCMV-infection ex vivo (Figures 4A, B). Accordingly, our data also demonstrated that CD158b1b2jneg NK cells purified from NR were able to release higher amounts of INF-γ after co-culture with HCMV-infected HUVEC compared to R (Figure 4C).
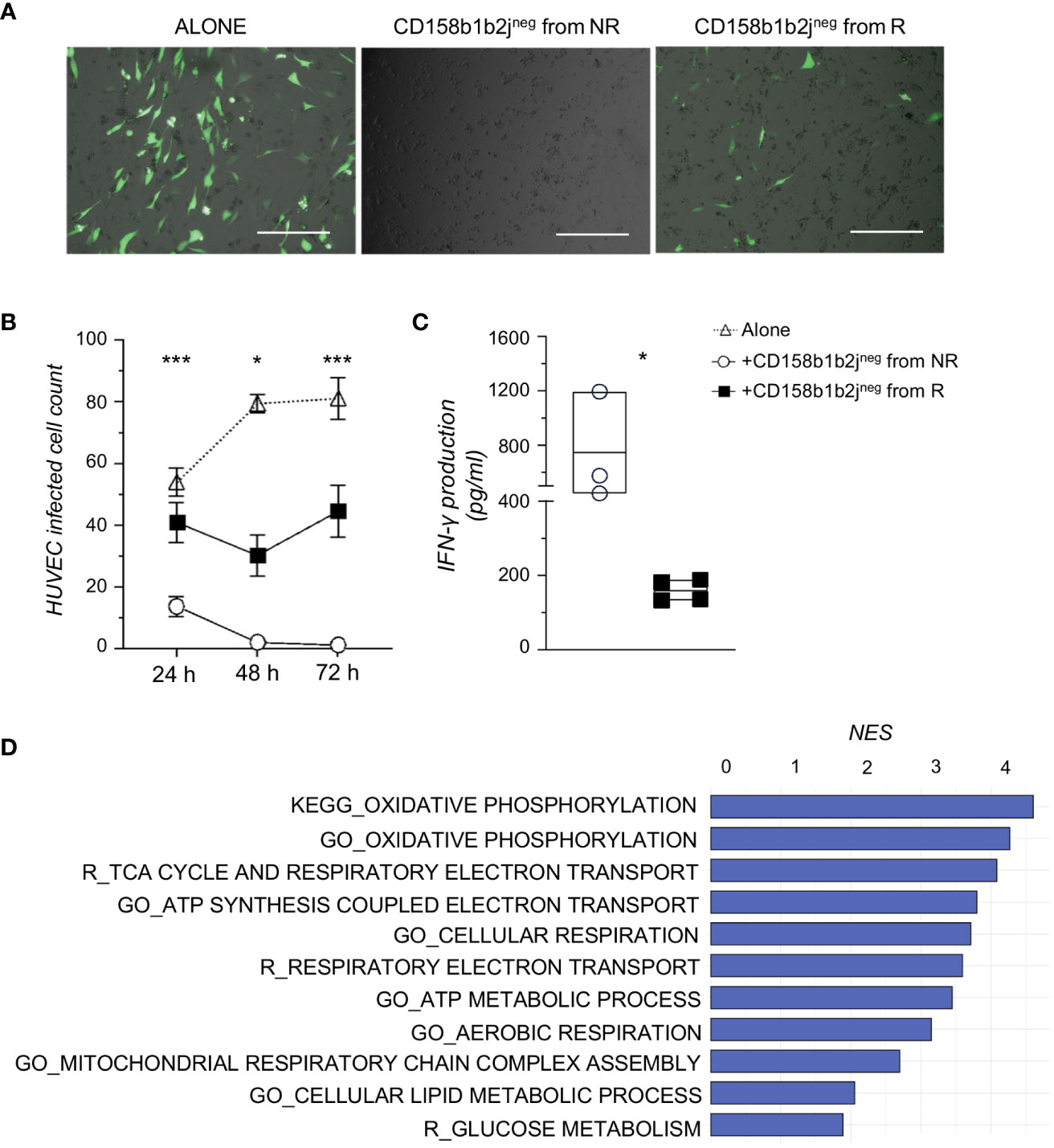
Figure 4 CD158b1b2jneg NK cells from NR better control HCMV infection and have higher metabolic functions than those from R. (A) Representative overlayed images of immunofluorescence and bright field pictures of HUVEC infected with TB40:EGFP (MOI=1), a BAC clone of the HCMV strain TB40/E expressing EGFP, and co-cultured for 48 hours alone (left panel) or in the presence of CD158b1b2jneg NK cells from NR (middle panel) or R (right panel). Scale bar 400 μm. (B) Summary statistical graph displaying the number of TB40:EGFP-infected HUVEC remained after 24, 48, and 72 hours (h) of culture alone or in the presence of CD158b1b2jneg NK cells from NR (n=4) or R (n=4) patients. Student’s t-test NR vs R, *p<0.05, **p<0.01, ***p<0.001. (C) Summary statistical graph showing the IFN-γ production (pg/ml) by purified CD158b1b2jneg NK cells from NR (o) and R (▪) after 72 hours of co-culture with TB40:Egfp HUVEC-infected cells and assessed using the Human IFN-gamma DuoSet ELISA (R&D Systems). Student’s t-testNR vs R, *p<0.05. (D) Gene sets, with a False Discovery Rate < 0.05 and involved in energy metabolism, more enriched in CD158b1b2jneg NK cells from NR compared to R as retrieved from Gene Ontology (GO_), KEGG, and Reactome (R_) databases. NES=Normalized Enrichment Score.
To better assess the functional properties of our newly disclosed NK cell subset, we investigated the transcriptional profile of purified CD158b1b2jneg NK cells from NR and R by RNA sequencing. Our analyses identified 1327 differentially expressed genes (DEGs; p-value <0.05), 72 of which had a p-value adjusted<0.05. We next performed a Gene Set Enrichment Analysis (GSEA) and found that CD158b1b2jneg NK cells from NR were more enriched in pathways associated with oxidative and glucose metabolisms compared to R (Figure 4D).
4 Discussion
Haploidentical HSCT with PT-Cy represents a therapeutic platform with curative potential for patients affected by high-risk hematologic malignancies and enables the rapid sourcing of a donor for almost every patient in need. Due to the prolonged immunodeficiency post-h-HSCT, the clinical outcome is still hampered by opportunistic viral infections, mainly caused by new infections or from reactivations of HCMV (21).
In a h-HSCT setting, we and others previously reported that NK cells are the first donor-derived lymphocytes to immune-reconstitute, thus conferring a certain degree of immune protection against pathogens and leukemic cells early after transplant (10–12).
Moreover, we and others demonstrated that, in patients who underwent h-HSCT, the homeostasis of NK cells is influenced by the occurrence of HCMV-I/R, which accelerates the maturation of NK cells and drives the expansion of NKG2Cpos NK cells endowed with adaptive traits (15). In this context, a recent study investigating the NKG2C genotype as a factor predicting the h-HSCT outcome showed that NKG2C homozygosity in the donor ameliorates HCMV clearance in the recipient and promotes the quantitative and qualitative reconstitution of adaptive NKG2Cpos NK cells (22). On the other hand, we demonstrated that HCMV-I/R can also mediate the expansion of dysfunctional CD158b1b2jpos/NKG2Aneg/NKG2Cpos/NKp30lo NK cells (16).
Herein, we identified a novel CD158b1b2jneg/NKG2Apos/NKG2Cneg/NKp30pos/NKp46pos NK cell subset persisting in HCMV not-reactivated patients and characterized by a strong anti-viral potential.
Our unbiased computational analysis of single cell flow cytometry data demonstrated that the frequencies and absolute counts of KIRneg NK cells are higher in NR than in R before HCMV-I/R occurrence. These KIRneg NK cells further decline in R after the occurrence of HCMV-I/R because of the expansion of KIRpos adaptive NK cells in R at the expense of KIRneg ones (15, 16). Of note, we also found significantly higher amounts of KIRneg NK cells in the grafts of NR compared to those of R, regardless of their HCMV serostatus and donor age. This observation could be of clinical utility since lower frequencies of KIRneg NK cells in potential familiar donors may be predictive of HCMV-I/R in the recipient. Thus, the assessment of KIRneg NK cells’ frequencies can be implemented, together with other NK cell properties, including the NKG2C genotype (22), KIR genotype, and alloreactivity (23, 24), for the screening of patient relatives to select optimal donors whose graft helps to prevent post-transplant HCMV-I/R and to ameliorate the clinical outcome of recipients in terms of engraftment, fast immune-reconstitution, and prevention of disease relapse and GvHD onset.
In contrast to mature CD158b1b2jpos/NKG2Aneg/NKG2Cpos/NKp30lo NK cells expanded in R, which are mainly composed by CD56dim/CD16pos and CD56neg/CD16pos NK cell subsets (16), KIRneg NK cells are in earlier stages of differentiation as they mainly belong to CD56bright/CD16neg and CD56bright/CD16pos subsets. Of particular significance are CD56bright/CD16pos NK cells, a neglected subset of unknown origin, which is present at very low frequency under homeostatic conditions or during post-h-HSCT IR (10, 18, 20). By investigating the expression of markers involved in NK cell differentiation, maturation/licensing, activation, and function, we herein demonstrated that CD56bright/CD16pos NK cells are an intermediate step of differentiation between CD56bright/CD16neg and CD56dim/CD16pos NK cells rather than CD56dim/CD16pos NK cells upregulating CD56 in response to the cytokine storm occurring in patients undergoing h-HSCT (20, 25).
In line with previous findings made in a CMV mouse model (26), our data reveal in a clinical setting that “not terminally-differentiated” KIRneg NK cells are involved in protection from HCMV-I/R. Indeed, the persistence of KIRneg NK cells in NR till 1-year post-h-HSCT indicates that they could participate in efficient HCMV control following h-HSCT. On the contrary, KIRneg NK cells decline in R after HCMV-I/R onset, suggesting that the decreased frequencies of these NK cells, together with the parallel increase of KIRpos NK cells (16), could predispose an individual to HCMV-I/R and could predict the onset of this opportunistic condition.
In agreement, our ex vivo model of HCMV infection clearly demonstrated that, contrary to CD158b1b2jpos NK cells (16), CD158b1b2jneg NK cells from both NR and R limited the HCMV spread in permissive endothelial cells, even at 1-year after the transplant and far from the reactivation events. Of note, CD158b1b2jneg NK cells from NR completely abolish HCMV-infection. This could be due to the higher NKG2A-education of CD158b1b2jneg NK cells from R compared to NR, as well as to the higher expression of the natural cytotoxicity receptors NKp30 and NKp46 (27). In accordance, we also found that INF-γ production was significantly higher in CD158b1b2jneg NK cells purified from NR compared to R, thus corroborating once more the anti-viral ability of NR-derived KIRneg NK cells.
Recent findings indicate that cellular metabolism could determine the functional differences in NK cells and that an enhanced functional activity has been associated with the NK cell’s ability to efficiently utilize glucose to fuel glycolysis and oxidative phosphorylation (27). In agreement, our RNA-sequencing analyses clearly demonstrated that CD158b1b2jneg NK cells from NR are characterized by a superior metabolic function that could be responsible for the enhanced anti-viral activity compared to CD158b1b2jneg NK cells from R.
In summary, we show here that the chronic persistence of KIRneg NK cells in h-HSCT patients is associated with HCMV control. This phenomenon is due to the higher anti-viral potential and superior metabolic functions of this NK cell subset. These “not terminally-differentiated” and “NKG2A educated” KIRneg NK cells can persist for several months in NR, while keeping high surface levels of the checkpoint inhibitor NKG2A. Further investigations are warranted to identify the mechanism(s) ensuring the persistence and the homeostasis of KIRneg NK cells in NR and to address if the NKG2A blockade may further improve their anti-viral potential (10). Moreover, although the data obtained could have a clinical utility in predicting the onset of HCMV-I/R and in potentially identifying the best donor among patient relatives whose graft helps to prevent post-transplant HCMV-I/R, to confirm and validate our findings a larger prospective study is necessary to allow patient stratification based on the disease stage and category, HCMV serostatus, and HCMV-I/R severity. Our findings may enable the optimization of post-transplant Donor-Lymphocyte Infusions by enriching or selecting “unlicensed” KIRneg NK cells for superior HCMV-I/R control.
Data availability statement
The datasets presented in this study can be found in online repositories. The names of the repository/repositories and accession number(s) can be found below: https://www.ncbi.nlm.nih.gov/geo/, GSE160362.
Ethics statement
The studies involving humans were approved by Institutional Review Boards of the Clinical and Research Institute Humanitas (Approval 24/18) Comitato etico indipendente IRCCS Istituto Clinico Humanitas. The studies were conducted in accordance with the local legislation and institutional requirements. The participants provided their written informed consent to participate in this study.
Author contributions
CDV: Conceptualization, Formal Analysis, Investigation, Methodology, Supervision, Writing – original draft, Writing – review & editing, Project administration. NC: Formal Analysis, Investigation, Methodology, Writing – original draft. MC: Formal Analysis, Investigation, Methodology, Writing – review & editing. ST: Data curation, Formal Analysis, Writing – review & editing. EZ: Formal Analysis, Investigation, Methodology, Writing – review & editing. SP: Data curation, Formal Analysis, Software, Writing – review & editing. AF: Investigation, Methodology, Writing – review & editing. JM: Resources, Writing – review & editing. CP: Resources, Writing – review & editing. DaM: Resources, Writing – review & editing. BS: Resources, Writing – review & editing. RM: Resources, Writing – review & editing. VTKL-T: Methodology, Resources, Writing – review & editing. MT: Funding acquisition, Methodology, Resources, Writing – review & editing. LC: Resources, Writing – review & editing. SB: Resources, Writing – review & editing. AS: Resources, Supervision, Writing – review & editing. DoM: Formal Analysis, Funding acquisition, Project administration, Supervision, Writing – original draft, Writing – review & editing.
Funding
The author(s) declare financial support was received for the research, authorship, and/or publication of this article. This work is supported by the Associazione Italiana per la Ricerca sul Cancro (IG 2018-21567 to DoM), Intramural Research Funding of Istituto Clinico Humanitas (to DoM), and the Italian Ministry of Health (Bando Ricerca Finalizzata PE-2016-02363915 to DoM). MT receives funding from the Deutsche Forschungsgemeinschaft (DFG) through RTG1949, TR1208/1-1, and TR1208/2-1. MC is a recipient of the Leonelli AIRC fellowship (26580). MC and AF are recipients of competitive fellowships awarded by the PhD program of Experimental Medicine from University of Milan. ST is a recipient of a competitive fellowship awarded by the Data Science in Medicine and Nutrition (DASMEN) Ph.D program at Humanitas University. The purchase of a FACSymphony A5 was defrayed in part by a grant from the Italian Ministry of Health (Agreement 82/2015).
Acknowledgments
We wish to thank all the patients and their relatives for their participation in this study. We also thank the nurses of the Hematology and Bone Marrow Transplant Unit.
Conflict of interest
The authors declare that the research was conducted in the absence of any commercial or financial relationships that could be construed as a potential conflict of interest.
The author(s) declared that they were an editorial board member of Frontiers, at the time of submission. This had no impact on the peer review process and the final decision.
Publisher’s note
All claims expressed in this article are solely those of the authors and do not necessarily represent those of their affiliated organizations, or those of the publisher, the editors and the reviewers. Any product that may be evaluated in this article, or claim that may be made by its manufacturer, is not guaranteed or endorsed by the publisher.
Supplementary material
The Supplementary Material for this article can be found online at: https://www.frontiersin.org/articles/10.3389/fimmu.2023.1266051/full#supplementary-material
Supplementary Table 1 | Statistical analysis of NR and R patient characteristics. (related to Table 1).
Supplementary Table 2 | Characteristics of patients used in in vitro infection experiments. (related to in vitro infection experiments).
Supplementary Figure 1 | Longitudinal analysis of KIRneg NK cell absolute counts in NR and R. (related to Figure 2B).
Supplementary Figure 2 | KIRneg NK cell frequency in donor grafts. (related to Figure 2B).
Supplementary Figure 3 | NK cell subset distribution in recipients experiencing or not a HCMV-I/R after h-HSCT. (related to Figure 2C).
References
1. Griffiths P, Reeves M. Pathogenesis of human cytomegalovirus in the immunocompromised host. Nat Rev Microbiol (2021) 19(12):759–73. doi: 10.1038/s41579-021-00582-z
2. Stern L, Withers B, Avdic S, Gottlieb D, Abendroth A, Blyth E, et al. Human cytomegalovirus latency and reactivation in allogeneic hematopoietic stem cell transplant recipients. Front Microbiol (2019) 10:1186. doi: 10.3389/fmicb.2019.01186
3. Williams L, Cirrone F, Cole K, Abdul-Hay M, Luznik L, Al-Homsi AS. Post-transplantation cyclophosphamide: from HLA-haploidentical to matched-related and matched-unrelated donor blood and marrow transplantation. Front Immunol (2020) 11:636. doi: 10.3389/fimmu.2020.00636
4. Forte E, Zhang Z, Thorp EB, Hummel M. Cytomegalovirus latency and reactivation: an intricate interplay with the host immune response. Front Cell Infect Microbiol (2020) 10:130. doi: 10.3389/fcimb.2020.00130
5. Paludan SR, Bowie AG, Horan KA, Fitzgerald KA. Recognition of herpesviruses by the innate immune system. Nat Rev Immunol (2011) 11(2):143–54. doi: 10.1038/nri2937
6. Luznik L, O'Donnell PV, Symons HJ, Chen AR, Leffell MS, Zahurak M, et al. HLA-haploidentical bone marrow transplantation for hematologic Malignancies using nonmyeloablative conditioning and high-dose, posttransplantation cyclophosphamide. Biol Blood Marrow Transplant. (2008) 14(6):641–50. doi: 10.1016/j.bbmt.2008.03.005
7. Locatelli F, Pende D, Maccario R, Mingari MC, Moretta A, Moretta L. Haploidentical hemopoietic stem cell transplantation for the treatment of high-risk leukemias: how NK cells make the difference. Clin Immunol (2009) 133(2):171–8. doi: 10.1016/j.clim.2009.04.009
8. Zaghi E, Calvi M, Di Vito C, Mavilio D. Innate immune responses in the outcome of haploidentical hematopoietic stem cell transplantation to cure hematologic Malignancies. Front Immunol (2019) 10:2794. doi: 10.3389/fimmu.2019.02794
9. Rambaldi B, Kim HT, Reynolds C, Chamling Rai S, Arihara Y, Kubo T, et al. Impaired T- and NK-cell reconstitution after haploidentical HCT with posttransplant cyclophosphamide. Blood Adv (2021) 5(2):352–64. doi: 10.1182/bloodadvances.2020003005
10. Roberto A, Di Vito C, Zaghi E, Mazza EMC, Capucetti A, Calvi M, et al. The early expansion of anergic NKG2A(pos)/CD56(dim)/CD16(neg) natural killer represents a therapeutic target in haploidentical hematopoietic stem cell transplantation. Haematologica (2018) 103(8):1390–402. doi: 10.3324/haematol.2017.186619
11. Ruggeri L, Capanni M, Urbani E, Perruccio K, Shlomchik WD, Tosti A, et al. Effectiveness of donor natural killer cell alloreactivity in mismatched hematopoietic transplants. Science (2002) 295(5562):2097–100. doi: 10.1126/science.1068440
12. Russo A, Oliveira G, Berglund S, Greco R, Gambacorta V, Cieri N, et al. NK cell recovery after haploidentical HSCT with posttransplant cyclophosphamide: dynamics and clinical implications. Blood (2018) 131(2):247–62. doi: 10.1182/blood-2017-05-780668
13. Della Chiesa M, Muccio L, Moretta A. CMV induces rapid NK cell maturation in HSCT recipients. Immunol Lett (2013) 155(1-2):11–3. doi: 10.1016/j.imlet.2013.09.020
14. Foley B, Cooley S, Verneris MR, Pitt M, Curtsinger J, Luo X, et al. Cytomegalovirus reactivation after allogeneic transplantation promotes a lasting increase in educated NKG2C+ natural killer cells with potent function. Blood (2012) 119(11):2665–74. doi: 10.1182/blood-2011-10-386995
15. Della Chiesa M, Falco M, Muccio L, Bertaina A, Locatelli F, Moretta A. Impact of HCMV infection on NK cell development and function after HSCT. Front Immunol (2013) 4:458. doi: 10.3389/fimmu.2013.00458
16. Zaghi E, Calvi M, Puccio S, Spata G, Terzoli S, Peano C, et al. Single-cell profiling identifies impaired adaptive NK cells expanded after HCMV reactivation in haploidentical HSCT. JCI Insight (2021) 6(12):e146973. doi: 10.1172/jci.insight.146973
17. Sampaio KL, Cavignac Y, Stierhof YD, Sinzger C. Human cytomegalovirus labeled with green fluorescent protein for live analysis of intracellular particle movements. J Virol (2005) 79(5):2754–67. doi: 10.1128/JVI.79.5.2754-2767.2005
18. Di Vito C, Mikulak J, Mavilio D. On the way to become a natural killer cell. Front Immunol (2019) 10:1812. doi: 10.3389/fimmu.2019.01812
19. De Angelis C, Mancusi A, Ruggeri L, Capanni M, Urbani E, Velardi A, et al. Expansion of CD56-negative, CD16-positive, KIR-expressing natural killer cells after T cell-depleted haploidentical hematopoietic stem cell transplantation. Acta Haematol (2011) 126(1):13–20. doi: 10.1159/000323661
20. Michel T, Poli A, Cuapio A, Briquemont B, Iserentant G, Ollert M, et al. Human CD56bright NK cells: an update. J Immunol (2016) 196(7):2923–31. doi: 10.4049/jimmunol.1502570
21. Mohty R, Brissot E, Battipaglia G, Ruggeri A, Dulery R, Bonnin A, et al. Infectious complications after post-transplantation cyclophosphamide and anti-thymocyte globulin-based haploidentical stem cell transplantation. Br J Haematol (2019) 187(3):e64–e8. doi: 10.1111/bjh.16189
22. Yu XX, Shang QN, Liu XF, He M, Pei XY, Mo XD, et al. Donor NKG2C homozygosity contributes to CMV clearance after haploidentical transplantation. JCI Insight (2022) 7(3):e149120. doi: 10.1172/jci.insight.149120
23. Solomon SR, Aubrey MT, Zhang X, Piluso A, Freed BM, Brown S, et al. Selecting the best donor for haploidentical transplant: impact of HLA, killer cell immunoglobulin-like receptor genotyping, and other clinical variables. Biol Blood Marrow Transplant. (2018) 24(4):789–98. doi: 10.1016/j.bbmt.2018.01.013
24. Zou J, Kongtim P, Srour SA, Greenbaum U, Schetelig J, Heidenreich F, et al. Donor selection for KIR alloreactivity is associated with superior survival in haploidentical transplant with PTCy. Front Immunol (2022) 13:1033871. doi: 10.3389/fimmu.2022.1033871
25. Melenhorst JJ, Tian X, Xu D, Sandler NG, Scheinberg P, Biancotto A, et al. Cytopenia and leukocyte recovery shape cytokine fluctuations after myeloablative allogeneic hematopoietic stem cell transplantation. Haematologica (2012) 97(6):867–73. doi: 10.3324/haematol.2011.053363
26. Orr MT, Murphy WJ, Lanier LL. 'Unlicensed' natural killer cells dominate the response to cytomegalovirus infection. Nat Immunol (2010) 11(4):321–7. doi: 10.1038/ni.1849
Keywords: haploidentical hematopoietic stem cell transplantation, natural killer cells, immune reconstitution, human cytomegalovirus, viral immunity
Citation: Di Vito C, Coianiz N, Calvi M, Terzoli S, Zaghi E, Puccio S, Frigo A, Mariotti J, De Philippis C, Mannina D, Sarina B, Mineri R, Le-Trilling VTK, Trilling M, Castagna L, Bramanti S, Santoro A and Mavilio D (2024) Persistence of KIRneg NK cells after haploidentical hematopoietic stem cell transplantation protects from human cytomegalovirus infection/reactivation. Front. Immunol. 14:1266051. doi: 10.3389/fimmu.2023.1266051
Received: 24 July 2023; Accepted: 19 December 2023;
Published: 10 January 2024.
Edited by:
Yi Luo, Zhejiang University, ChinaReviewed by:
Jeremy Rubinstein, Cincinnati Children’s Hospital Medical Center, United StatesMeng Lv, Peking University People’s Hospital, China
Copyright © 2024 Di Vito, Coianiz, Calvi, Terzoli, Zaghi, Puccio, Frigo, Mariotti, De Philippis, Mannina, Sarina, Mineri, Le-Trilling, Trilling, Castagna, Bramanti, Santoro and Mavilio. This is an open-access article distributed under the terms of the Creative Commons Attribution License (CC BY). The use, distribution or reproduction in other forums is permitted, provided the original author(s) and the copyright owner(s) are credited and that the original publication in this journal is cited, in accordance with accepted academic practice. No use, distribution or reproduction is permitted which does not comply with these terms.
*Correspondence: Clara Di Vito, Y2xhcmEuZGlfdml0b0BodW1hbml0YXNyZXNlYXJjaC5pdA==; Domenico Mavilio, ZG9tZW5pY28ubWF2aWxpb0B1bmltaS5pdA==; ZG9tZW5pY28ubWF2aWxpb0BodW1hbml0YXMuaXQ=