- 1Department of Infectious Disease Prevention and Control, Nanjing Bioengineering (Gene) Technology Center for Medicines, Nanjing, China
- 2Department of Infectious Disease Prevention and Control, Quzhou Center for Disease Prevention and Control, Quzhou, China
- 3School of Public Health, Nanjing Medical University, Nanjing, China
- 4College of Life Science, Nanjing Normal University, Nanjing, China
- 5Department of Occupational Health, Third Military Medical University, Chongqing, China
- 6Department of Neurosurgery, Nanjing Brain Hospital Affiliated to Nanjing Medical University, Nanjing, China
- 7School of Public Health, Bengbu Medical College, Bengbu, China
- 8School of Pharmacy, Nanjing University of Chinese Medicine, Nanjing, China
Background: There is no clear conclusion on the immunogenicity and adverse events of concomitant administration the viral respiratory infectious disease vaccines. We aimed to evaluate the impact of concomitant administering viral respiratory infectious disease vaccines on efficiencies, safety and influencing factors.
Methods: This meta-analysis included studies from PubMed, Embase, Cochrane Central Register of Clinical Trials, Web of Science, WHO COVID-19 Research, and ClinicalTrials.gov databases. Randomized controlled trials of the adult participants concomitant administered with viral respiratory infectious disease vaccine and other vaccines were included. The main outcomes were the seroconversion rate and seroprotection rate of each vaccine. Used the Mantel–Haenszel fixed effects method as the main analysis to estimate the pooled RRs and the corresponding 95% confidence intervals. The risk of bias for each trial was assessed using the Cochrane Handbook for Systematic Reviews of Interventions, while evidence certainty was evaluated using the Grading of Recommendations Assessment, Development, and Evaluation system.
Results: A total of 21 studies comprising 14060 participants with two types of vaccines were retained for the meta-analysis. Concomitant immunization reduced the geometric mean titer (RR: 0.858, 95% CI: (0.785 to 0.939)) and the geometric mean fold rise (0.754 (0.629 to 0.902)) in the SARS-COV-2 vaccine group but increased the seroconversion rate (1.033 (1.0002 to 1.067)) in the seasonal influenza vaccine group. Concomitant administration were influenced by the type of vaccine, adjuvant content, booster immunization, and age and gender of the recipient.
Conclusion: This meta-analysis suggested that the short-term protection and safety of concomitant administered were effective. Appropriate adjuvants, health promotion and counselling and booster vaccines could improve the efficiency and safety of Concomitant vaccination.
Systematic review registration: https://www.crd.york.ac.uk/PROSPERO/, identifier CRD42022343709.
Introduction
The coronavirus disease 2019 (COVID-19) pandemic, caused by severe acute respiratory syndrome coronavirus 2 (SARS-CoV-2), has become a pressing global crisis and has led to 6.5 million deaths worldwide. The SARS-CoV-2 vaccines have been successfully in inducing the neutralizing humoral and cellular immunity against the virus, thus reducing infections, hospitalizations, and deaths in clinical trials (1, 2). Safe and effective vaccines are considered viable to end the pandemic (3).
There are significant concerns that the COVID‐19 pandemic may overlap with other respiratory viruses, particularly seasonal influenza. For instance, Europe experienced a new avian influenza epidemic in June 2022 and the United States has seen an unprecedented poultry H5N1 infection, with a high zoonotic spillage risk (4, 5). These instances highlight the challenge of overlapping the COVID-19 epidemic with influenza. Concomitant vaccination is recommended for people traveling to epidemic areas with other infectious diseases or as a planned immunization for infants and children, especially during the immunization season, to reduce the burden on healthcare services (6). Vaccine concomitant administration reduces the number of hospital visits, thereby reducing stress and inconvenience for children and parents (7).
Studies on concomitant vaccination against viral respiratory infectious diseases have focused on the seasonal influenza vaccine (SIV) administered with other vaccines in adults (8, 9). With the increasing cases of COVID-19 and the widespread development of SARS-COV-2 vaccines, studies on the concomitant vaccination with SARS-COV-2 vaccines are gradually being reported (6, 10). However, due to the limited sample size, the efficacy evaluation of concomitant vaccination in randomized clinical trials (RCTs) is based on the “non-inferiority criterion,” which is ambiguous, making accurate assessment difficult. There is no clear conclusion on the immunogenicity and Ads of concomitant vaccination with viral respiratory infectious disease vaccines (VRIDVs).
Currently, there is no meta-analysis on the concomitant administration of VRIDVs in adults. This study, therefore, conducted a systematic review and meta-analysis on the differences in immunogenicity and ADs between concomitant and sequential VRIDVs administration.
Methods
Search strategy and selection criteria
We conducted a systematic review and network meta-analysis according to the Preferred Reporting Items for Systematic Reviews and Meta-Analyses (PRISMA) guidelines (Supplementary Data Sheet 1 (eTable 1)) (11). The review protocol was prospectively registered with the PROSPERO ID CRD42022343709.
We searched PubMed, Embase, Cochrane Central Register of Clinical Trials, Web of Science, WHO COVID-19 Research, and ClinicalTrials.gov databases from inception to September 10, 2022. The search was conducted in English, and the key search terms were “Concomitant vaccination,” “Concomitant administration,” “Concomitant immunization,” AND (“SARS-CoV-2 Vaccine,” OR “Influenza Vaccine,” OR “Viral Vaccine” (Supplementary Data Sheet 1 (eTable 2)).
We used a two-stage approach for literature screening: by checking the title and abstract and then going through the full-text article. Two researchers (CH and DF) independently screened the title, abstract, and full text of each article, and the discrepancies were resolved through consensus with a third researcher (YF). Studies included in the meta-analysis were those evaluating concomitant administration of adults who received VRIDV, followed by the outcome, including immunogenicity and adverse events (ADs) of the concomitant administered and sequential/alone groups in RCTs. However, studies with unreported or ambiguous outcomes of immunological efficacy, no focus on VRIDVs, and those involving children or infants were excluded from the meta-analysis. In cases where the study lacked available data, we requested additional information from the corresponding authors, upon which the study was excluded if the data were not provided.
Publication bias assessment and sensitivity analysis
The Egger regression test with a funnel plot was used to assess the publication bias in the meta-analysis of ≥8 groups, whereby p < 0.05 indicated significant asymmetry and publication bias. Moreover, sensitivity analysis was used to remove each cohort individually from the meta-analysis.
Quality assessment
The risk of bias for each trial was assessed using the Cochrane Handbook for Systematic Reviews of Interventions (12–14), while evidence certainty was evaluated using the Grading of Recommendations Assessment, Development, and Evaluation (GRADE) system (15). Two researchers (DF and MK) independently assessed the risk of bias in individual studies and the GRADE system. Discrepancies were resolved by consensus and arbitration among the authors (DF, CH, YF, MK).
Data analysis
Data on the study characteristics comprised the study setting, outcomes, study design, sample size, dropout or non-response rates, and inclusion and exclusion criteria. Participant data included age, gender, and vaccine history. Intervention-related data included the vaccine type and dosing schedule. Outcome-related data comprised the assay type, antibody measured, method of measurement, and intervals of sample collection. The categorical outcomes of the arm subgroups from multi-arm studies were combined, and the continuous outcomes from the large sample size arm were used to represent the study. Two independent researchers (DF and MK) assessed the extracted data.
We analyzed the data provided to compare the efficacy and safety of VRIDVs and reported the findings as relative risks (RRs) for binary outcomes, including seroconversion rate (SCR), seroprotection rate (SPR) and ADs. The main outcomes were the SPR and SCR of each vaccine. Since there is no common evaluation standard for the immunogenicity of SARS-CoV-2 vaccines (16), we combined the geometric mean titer (GMT), geometric mean concentrations (GMCs) and geometric mean fold rises (GMFRs) in the meta-analysis and reported as the GMT through the ratio of means (ROMs) method.
We used the Mantel–Haenszel fixed effects method as the main analysis to estimate the pooled RRs and the corresponding 95% confidence intervals (CIs), while the random-effects model was used for the heterogeneity analysis at I2 >50%. Statistical heterogeneities of the results of the included studies were determined using the DerSimonian-Laird estimator, which assessed the between-study variance I2 statistic. Heterogeneity was considered low, moderate, or high if estimated I2 was below 25%, between 25% and 50%, and above 50%, respectively. Subgroup analysis and meta-regressions were performed to examine the relationship between the outcome and baseline characteristics of the cohorts. The subgroup analysis was undertaken if at least 6 cohorts were available. All analyses were conducted on R (version 4.1.1) using the meta and metafor packages. A two-sided P value < 0.05 indicated statistical significance.
Result
The online database search yielded 2848 results, and an additional 12 were obtained from other sources. A total of 55 articles were considered for full-text assessment after removing the duplicate entries and screening the abstracts (Supplementary Data Sheet 1 (eTable 3)), among which only 21 studies comprising 14060 participants were eligible for the analysis (Figure 1, total references in Supplementary Data Sheet 1 (eTable 3)). The participants received combination or sequential vaccines with VRIDVs. Lazarus et al. included 6 subgroups (6), while Toback et al. included 2 subgroups (17) to evaluate the efficacy of SIVs and SARS-CoV-2 vaccines. Therefore, 27 groups for SIVs and 10 groups for SARS-CoV-2 vaccines were included in the meta-analysis. The main characteristics of the included studies and statistical details of the included groups are presented (Tables 1, 2; Supplementary Data Sheet 1 (eTables 8–12)).
Efficacy and safety of concomitant vaccination
We report the results of serum-immunogenicity (Figure 2; Supplementary Data Sheet 1 (eFigures 1–12)). Concomitant administration reduced the GMT (RR: 0.858, 95% CI: (0.785 to 0.939)) and GMFR (0.754 (0.629 to 0.902)) but did not interfere with the SCR (0.994 (0.969 to 1.018)) in the SARS-COV-2 vaccine group. For the SIV group, influenza tests included three strains: H1N1 and H3N2 of A strains, and B strain. Each strain was analyzed for SCR, SPR and GMT. Concomitant immunization increased the SCR by 3.3% (1.033(1.0002 to 1.067) for the H3N2 strains; however, there were no statistically significant differences were found in other results.
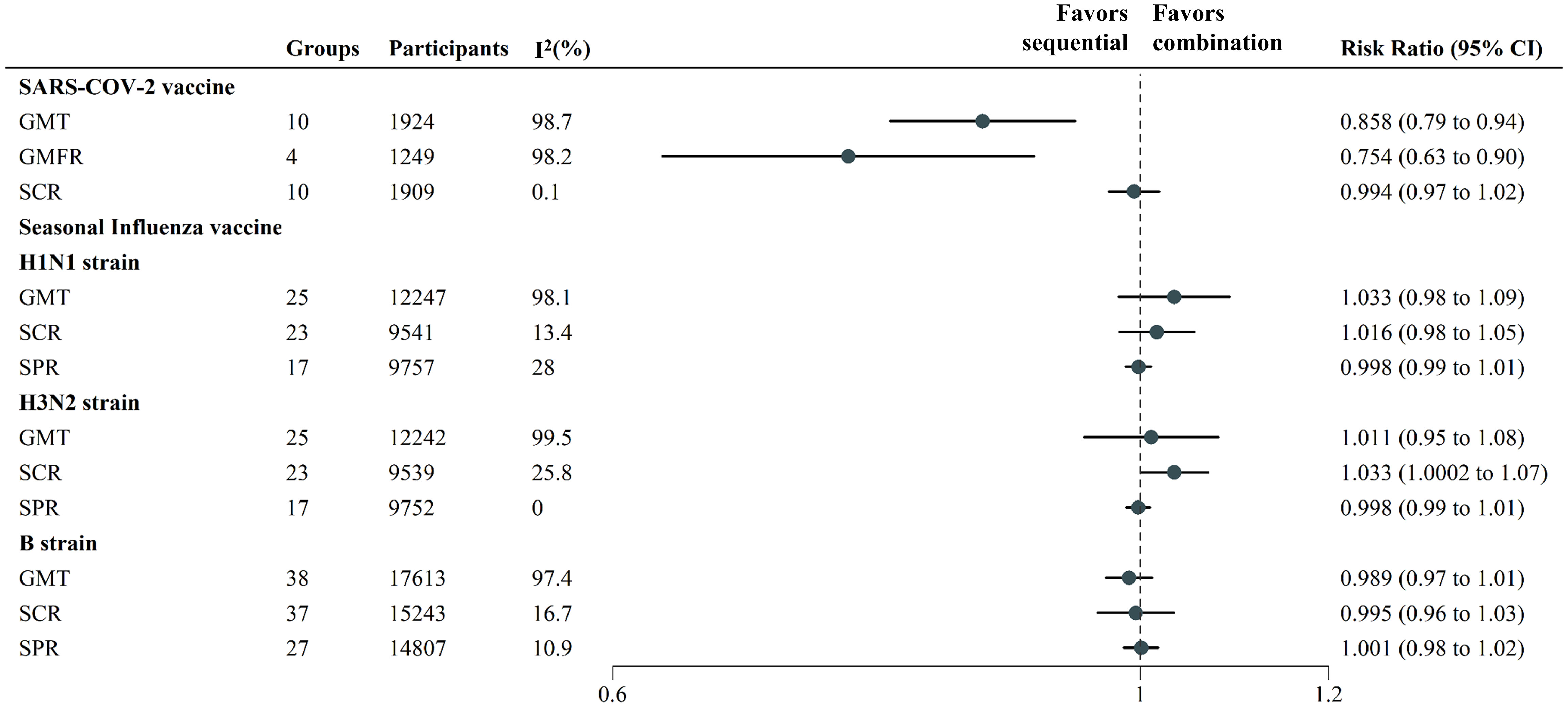
Figure 2 Pooled estimates of concomitant vaccination immunogenicity endpoints of viral respiratory infectious disease vaccines. Each point represents the respective endpoints pooled estimate, derived from Mantel–Haenszel fixed effects model and random effects model based on heterogeneity I2. The horizontal lines represent 95% CIs. In analysis of B strain, some studies analyzed 2 strain subtypes, which we combined it. A risk ratio less than 1 favors the sequential or alone vaccination. seroconversion rate (SCR), seroprotection rate (SPR), geometric mean titer (GMT) and geometric mean fold rises (GMFRs).
In the SARS-COV-2 vaccine group (Figures 3A, C; Supplementary Data Sheet 1 (eFigures 13–30)), concomitant immunization increased fatigue incidence by 11.3% (1.113 (1.005 to 1.234)) and muscle pain incidence by 17.9% (1.179 (1.031 to 1.348)) for the systemic ADs. and increased 15.8% (1.158(1.033 to 1.297)) tenderness incidence and decreased 27% (0.73 (0.556 to 0.959)) of induration incidence among the local ADs.
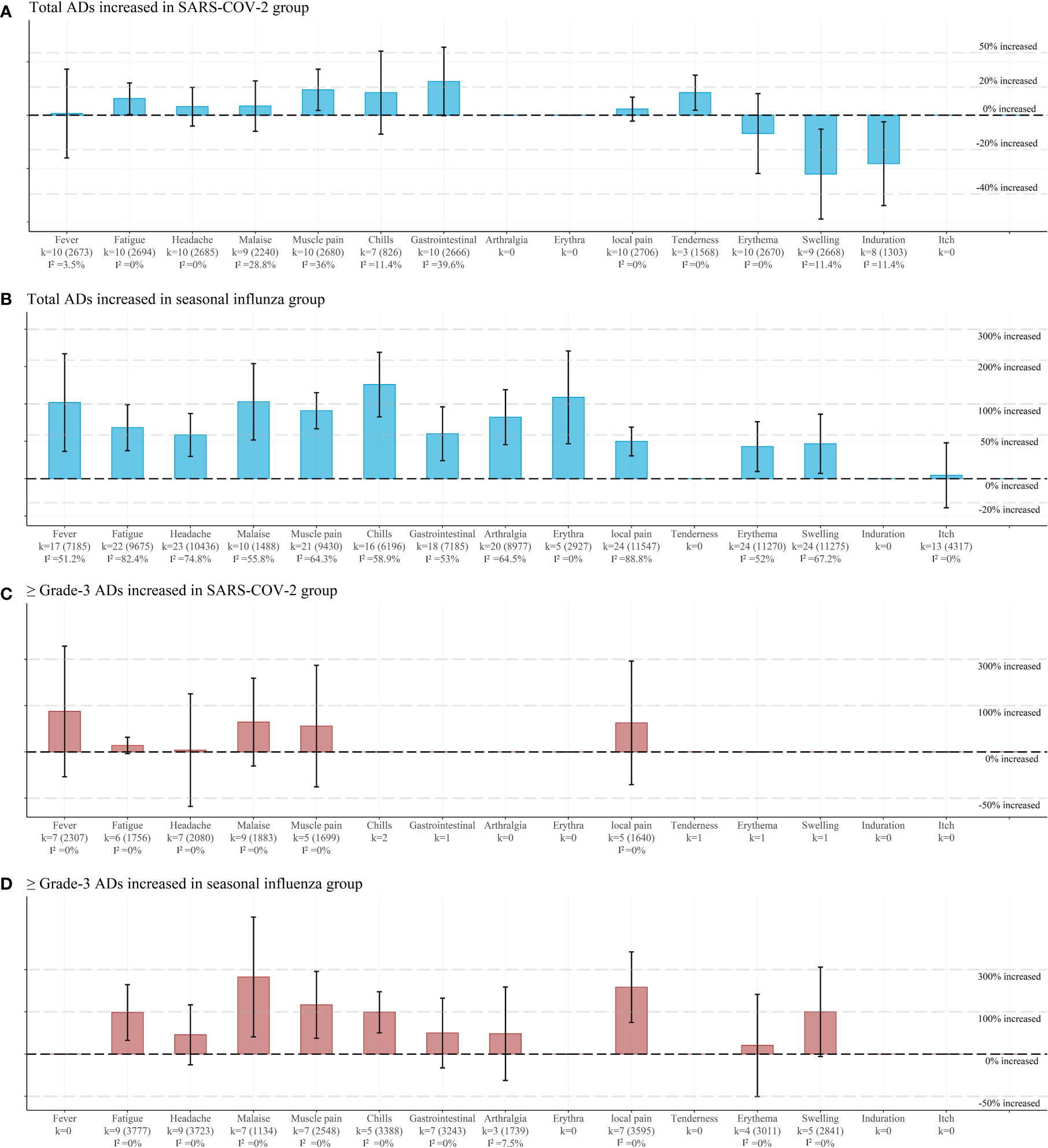
Figure 3 Pooled estimates of concomitant vaccination safety endpoints of viral respiratory infectious disease vaccines. (A) Pooled risk ratios (95% CI) for each total adverse events in SARS-COV-2 group. (B) Pooled risk ratios (95% CI) for each total adverse events in seasonal influenza group. (C) Pooled risk ratios (95% CI) for each ≥Grade-3 adverse events in SARS-COV-2 group. (D) Pooled risk ratios (95% CI) for each ≥Grade-3 adverse events in seasonal influenza group. Each point represents the respective endpoints pooled estimate, derived from Mantel–Haenszel fixed effects model and random effects model based on heterogeneity I2. The vertical lines represent 95% CIs. Each horizontal dashed line represents the increase or decrease in the incidence of adverse events for concomitant vaccination. k represents the number of studies and participants within each group. Meta-analysis when within-group studies > 3 groups.
In the SIV group (Figures 3B, D; Supplementary Data Sheet 1 (eFigures 31–52)), concomitant immunization increased several parameters of systemic ADs. These included 102.5% (2.025 (1.287 to 3.184)) of fever incidence; 60.6% (1.606 (1.297 to 1.988)) of total and 98.1% (1.981 (1.255 to 3.127)) of Grade-3 fatigue incidence; 50% (1.5 (1.229 to 1.831)) of headache incidence; 139.4%(2.394 (1.774 to 3.229)) of total and 98.7% (1.987 (1.418 to 2.784)) of Grade-3 chills incidence; 112.7% (2.127 (1.383 to 3.269)) of total rash incidence; 52.7% (1.517 (1.182 to 1.948) of gastrointestinal symptoms incidence; 76.9% (1.769 (1.372 to 2.281)) of arthralgia incidence; 87.9% (1.879 (1.589 to 2.221)) of total and 124.3% (2.243 (1.297; 3.879)) of Grade-3 muscle pain incidence; 104.2% (2.042 (1.433 to 2.909)) of total and 254.5% (3.545 (1.326 to 9.477)) of Grade-3 malaise incidence. Similarly, concomitant vaccination increased the local ADs parameters, including 98.1% (1.981 (1.255 to 3.127)) of total and 199.9% (2.998 (1.68 to 5.25)) of Grade-3 local pain, 34.7% (1.347 (1.069 to 1.696)) of redness, and 38.2% (1.382 (1.049 to 1.819)) of swelling.
Influencing factors of vaccine type
In the subgroup analysis (Supplementary Data Sheet 1 (eFigures 54–56), Supplementary Data Sheet 1 (eTables 27–48)), the GMT (p< 0.001) and GMFR (p<0.001) of the recombinant vaccine were more effective in the immunological group than those of the inactivated and split vaccine in the SARS-COV-2 vaccine group. The concomitant immunization results of the SIV group showed that the GMT (p<0.001; B strain), SCR (p=0.014; B strain), and the SPR (p=0.039; H1N1 strain) of the recombinant vaccine were more effective than those of the inactivated and split vaccines. No statistically significant results were found in the analysis of the ADs except for the local pain in the SIV group. Inactivated and split vaccines increased the ADs more than the recombinant vaccine (p=0.048).
Influencing factors of concomitant administered vaccine type
The subgroup analysis (Supplementary Data Sheet 1 (eFigures 57–59), Supplementary Data Sheet 1 (eTables 13–48)) showed that the split vaccine was more effective than GMFR (p=0.01) in the SARS-COV-2 immunogenicity group. However, no statistically significant results were found in the SIV group. For the ADs analysis of the SARS-COV-2 vaccine group, the muscle pain results showed that inactivated and recombinant vaccines increased the ADs more than the split vaccine (p=0.048). Moreover, concomitant administering SIV with recombinant and mRNA vaccines significantly increased ADs incidences, including fever (p<0.001), fatigue (p<0.001), headache (p<0.001), chills (p<0.001), muscle pain (p=0.027), and gastrointestinal symptoms (p<0.001) for systemic ADs and local pain for local ADs, than with other types of vaccines.
Influencing factors of gender proportion
In the subgroup analysis (Supplementary Data Sheet 1 (eTables 13–48)), the GMFR (p=0.01) of the SARS-COV-2 vaccine and SPR (p=0.045) of the B strain revealed that concomitant vaccination was more efficient in groups with ≥55% females. Notably, there were no statistically significant results in the ADs of the subgroups. According to meta-regression analysis, the GMT (0.21% (0.086 to 0.35%) per 1% of female proportions increased in the SARS-COV-2 vaccine group, consistent with the results of the subgroup analysis. The ADs analysis showed that gastrointestinal symptoms and malaise increased by 5.07% (1.62 to 8.51%) and 3.19% (0.38 to 6.01%), respectively, in the SIV group. However, there were no statistically significant results in the meta-regression analysis of the SARS-COV-2 vaccine ADs and SIV immunogenicity groups.
Influencing factors of mean age
The subgroup analysis (Supplementary Data Sheet 1 (eTables 13–48)) showed that the age distribution was around 18 to 80 years. The Geometric Mean Titers (GMT) and Geometric Mean Fold Rises (GMFR) in the SARS-CoV-2 vaccine immunogenicity group demonstrated a more pronounced effect of concomitant vaccination in participants with a mean age of 65 years and older, with statistical significance (p < 0.001 for both GMT and GMFR). Conversely, in the Seasonal Influenza Vaccine (SIV) immunogenicity group, no significant differences were noted in GMT and GMFR. The ADs increasing rate of concomitant vaccination was higher in the mean age < 65 group, with significantly increased fatigue (p=0.02), malaise (p=0.007) and muscle pain (p=0.02) in the SRAS-COV2 group. In contrast, the SIV group did not exhibit significant differences in these symptoms. According to meta-regression analysis, there was an increase in the GMT (0.81% (0.64 to 0.98%) per year of age and GMFR (1.7% (1.1 to 2.28%)) of the SARS-COV-2 vaccine immunogenicity group. Moreover, increase in fatigue (-0.88% (-1.69 to -0.07%)), malaise (-3.45% (-6.78 to-0.12%)) and local pain (-0.7% (-1.27 to-0.13%)) was observed in the SARS-COV-2 vaccine ADs group, but there were no statistically significant results in the SIV group.
Influencing factors of placebo used
For the subgroup analysis (Supplementary Data Sheet 1 (eTables 13–48)), in the SARS-COV-2 vaccine group, the concomitant immunization efficacy was improved upon the study use placebo, as shown by the GMT of p=0.04. Moreover, muscle pain (p=0.048) was higher in the placebo used group than in the no-placebo group. In the SIV group of the B strain group, the concomitant immunization effect was elevated by 3-4% with placebo use compared to no-placebo use in GMT and SPR; however, no differences were observed in the ADs of the SIV group. In meta-regression, the GMT of the SARS-COV-2 vaccine group increased by 7.57% (2.33 to 12.8%) with placebo used, and that of the seasonal influenza B strain group increased by 6.04% (1.75 to 10.33%). Additionally, the muscle pain of the SARS-COV-2 vaccine ADs group increased by 35.58% (0.18 to 70.97%) with placebo used. No statistically significant results were found in the ADs of the SIV group.
Influencing factors of adjuvants used
In the subgroup analysis (Supplementary Data Sheet 1 (eTables 13–48)), the SCR of the H3N2 (p=0.015) strain and B strain (p=0.006) showed that the adjuvants enhanced the concomitant immunization efficacy. Notably, statistically significant results were found in the ADs parameters, including itching (p=0.04). According to meta-regression, the SCR of H3N2 increased by 16.6% (2.42 to 30.8%) when adjuvant was used, while that of the B strain increased by 29.5% (3.94 to 55.1%) in the immunological group.
Influencing factors of booster dose used
We grouped participants according to whether the SARS-COV-2 vaccination was a first-time or a booster dose (Supplementary Data Sheet 1 (eTables 13–48)). We excluded the study by Wang et al. group, due to its design. Participants who received the first-time vaccination had a lower concomitant immunization efficiency (GMT; p<0.001 and GMFR; p<0.001) than those who received the booster dose. The increase in ADs incidences was greater in first-time vaccinated participants (fever; p < 0.001 and local pain; p < 0.001) than in those who received the booster. No statistically significant results were found in the meta-regression analysis of the groups.
Publication bias assessment and sensitivity analysis
Publication bias was found only in the chills symptoms of the SIV group (p=0.047). In the sensitivity analysis of the ADs, we found that the Herbinger et al. group had a greater impact on the results and heterogeneity of the meta-analysis and thus was excluded from the final meta-analysis.
Quality assessment
Overall, in combination with previous studies risk of bias assessments, these trials were considered low-moderate risk for bias (Supplementary Data Sheet 1 (eFigure 53)). In the GRADE system (Supplementary Data Sheet 1 (eTables 4–7)), there was low or very low grading for the SIV group and high grading for the SARS-COV-2 group in the ADs analyses. Similarly, low or very low grading was observed for GMT and GMFR results, while SCR and SPR had high or moderate grading in immunological efficacy.
Discussion
In this systematic review and meta-analysis, we evaluated the efficacy and safety of VRIDVs when concomitant administered with other vaccines. Four RCTs of the SARS-COV-2 vaccine and 21 RCTs of SIV were included in the meta-analysis. Concomitant immunization reduced the immunogenicity of GMT and GMFR in the SARS-COV-2 vaccine group by 14.2% and 24.6%, respectively. The SCR demonstrated the ability to protect the population (6, 10), both vaccine results showed that concomitant administration protected the population at the same level as sequential vaccination. Clinical studies and meta-analyses have shown that vaccine-induced production of high sero-neutralizing antibody titers declines after 3 ~ 6 months, with a progressive increase in the possibility of breakthrough infection as the titers decline and mutant strains develop (2, 3, 34, 35). Thus, serum immunoprotection declines with time, causing vaccine protection to be maintained only in higher GMT populations, suggesting that the duration of protection from concomitant vaccination is reduced.
In the analysis of the vaccine types, the concomitant administration effect of the recombinant vaccines was better than that of inactivated and split vaccines in some of the SIV group. The concomitant immunization effect of mRNA vaccines was also better than inactivated vaccines in the SARS-COV-2 vaccine group. “Heterologous effects of vaccination” can cross-reactive and bystander activate the classical adaptive immune response and improve the magnitude and durability of humoral and cellular immunity (7, 36–38). Moreover, the components of mRNA and recombinant vaccines may increase immunogenicity (3, 39), and this can potentially improve the duration and efficacy of future vaccines. In the analysis of ADs, concomitant administering SIV with the mRNA and recombinant vaccines increased the incidences of almost all systemic ADs (fever, fatigue, headache, chills, gastrointestinal symptoms, arthralgia, and muscle pain) more than the other vaccine types. In general, concomitant immunization mainly affected the systemic ADs and had less effect on the local ADs. Moreover, inactivated, and split vaccines had better safety than mRNA and recombinant vaccines when concomitant vaccination. This result is like vaccination alone.
In this review, the concomitant vaccination group showed a reduction in the GMT and GMFR among the SARS-COV-2 vaccine participants. However, no significant reduction was observed in the SIV group. Therefore, hypothesized that concomitant administration has greater effectiveness when supplemented with booster vaccination, as also reported by Lazarus et al. and Toback et al. Since most adults have a previous illness-immunity or prior vaccination against seasonal influenza because of the high prevalence of seasonal influenza, the SIV administration could be considered a booster vaccination. As such, the serum immune background of participants against influenza exhibited some reaction. In contrast, the pre-vaccination population has a very low baseline against SARS-COV-2 since covid-19 is an emerging infectious disease. According to the subgroup and meta-regression analysis of the booster vaccine in the SARS-COV-2 group, the immunogenicity of concomitant administration was lower in participants receiving the vaccine for the first time. Moreover, the immunogenicity of concomitant administration versus sequential/alone vaccination was similar to that of the booster vaccination in the SIV group, further supporting our hypothesis. The results affirm that alone vaccination is recommended as a first-time vaccination against SARS-COV-2, new emerging viruses, or other low-intensity VRIDVs, while a combination with other vaccines is a suitable option as a booster vaccination or vaccination against high-intensity VRIDVs.
Older adults exhibited a more robust tolerance to the concomitant administration of the SARS-CoV-2 vaccine compared to younger individuals, the ADs of concomitant administration significantly increased fatigue, malaise, and muscle pain among the participants with <65 years in the SARS-COV-2 vaccine group. This may also be caused by the weaker immunity of the elderly, which is not sensitive to both individual and combined vaccinations. In the SIV group, the elderly received the more suitable 3-valent SIV, while the young received 4-valent SIV. This procedure improved the immunogenicity of the elderly when vaccinated sequentially (10, 40). Consequently, we advocate for the development of vaccines offering broad coverage and prolonged protection, specifically designed for the elderly demographic.
Gender-based analysis revealed intriguing findings, one subgroup in the SARS-COV-2 group and another in the SIVs group showed higher concomitant vaccination efficacy in the group with a higher proportion of women. Notably, the meta-regression analysis showed that this observation was found only in the SARS-COV-2 vaccine group. In addition, a higher proportion of females had increased reports of gastrointestinal symptoms and malaise upon co-vaccination. Some studies reported that females have greater immune responses to vaccines and ADs than males (41, 42), potentially due to heightened sensitivity to the immune stimulation caused by ADs and the multiple antigens present in concomitant vaccination.
In the analysis of the placebo used, the concomitant immunization effect was significantly elevated in the group with placebo in the GMT of B strain and SARS-COV-2 vaccine group compared to the group without placebo. The use of a placebo increased the ADs in the muscle pain group of the SARS-COV-2 vaccine group to the point that participants were confused about their grouping after the placebo administration. People may believe that too many vaccines/antigens overload the immune system, or increase ADs, resulting in less efficacy than when the same vaccine is administered alone (43). This means that concomitant vaccination participants may have a reduced psychological burden, while those in the sequential group may have an elevated psychological burden due to the placebo, thus affecting the efficiency and safety of the administered vaccine. Therefore, educating the participants about the safety and efficacy of concomitant administration is beneficial for reducing psychological burden and improving vaccine efficacy and coverage (44).
The use of adjuvants significantly increased the immunogenicity of concomitant vaccination, with the concomitant administered vaccines being more effective in the adjuvant-containing group. The meta-regression analysis also supported these results, suggesting that adjuvants improve the immunological responses to a certain extent (17-30%). Consequently, this evidence suggests that adjuvant-containing vaccines may yield superior efficacy in concomitant administration scenarios (4).
This study has several strengths that highlight its significance. Of note, this is the first study to evaluate the efficacy and safety of the concomitant administration of SARS-COV-2 vaccines or SIVs with other vaccine in adults. Moreover, we meticulously performed meta-analyses, follow-up subgroup analyses, and meta-regressions of the primary immune and adverse outcomes to provide comprehensive and evidence-based medical data to help develop robust public health strategies. Nonetheless, this study was limited by several factors. First, some studies suggest that simple serologic testing of SARS-CoV-2 antibodies may not reflect the complexity and persistence of protective immunity and there is some testing variation in the quantitative detection (39, 45). The immunological efficacy results were only based on the serological immunological outcomes due to a lack of population outcomes, such as population protected rate and severe disease reduction rate. Thus, the study could not comprehensively reflect the protective efficiency of the discussed vaccines. Secondly, the RCTs studies included in this meta-analysis had few participants, making it difficult to statistically analyze severe ADs with very low incidences, such as anaphylaxis and myocarditis (46, 47). Therefore, future studies should analyze the efficacy and safety of concomitant administering vaccines through real-world studies to capture all vaccine ADs. Finally, this analysis focused on the SARS-COV-2 and SIV, failing to encompass other emerging VRIDVs, leading to potentially biased conclusions.
In conclusion, the results of this meta-analysis suggested that the short-term protection and safety of concomitant administered VRIDVs were reliable and did not interfere with the protection of the elderly (65-80 years old). Concomitant vaccination could improve the efficiency and safety of immunity with booster vaccines, appropriate adjuvants, and health promotion and counselling. However, the protection duration of concomitant vaccination against emerging infectious diseases could be greatly impacted by the first vaccination. Nonetheless, there is a need to develop specific vaccines for the elderly that ensure long-term protection.
Data availability statement
The original contributions presented in the study are included in the article/Supplementary Material. Further inquiries can be directed to the corresponding author.
Author contributions
DL: Data curation, Software, Visualization, Writing – original draft, Conceptualization, Investigation, Methodology, Supervision. YH: Formal analysis, Investigation, Project administration, Validation, Writing – original draft, Writing – review & editing. RX: Data curation, Supervision, Writing – original draft. MQ: Data curation, Formal analysis, Methodology, Validation, Writing – original draft. JS: Formal analysis, Project administration, Validation, Writing – original draft. CZ: Data curation, Formal analysis, Methodology, Writing – original draft. JZ: Funding acquisition, Resources, Visualization, Writing – original draft. FY: Formal analysis, Software, Writing – original draft. ZL: Methodology, Writing – original draft. YW: Validation, Writing – original draft. CFW: Validation, Writing – original draft. CHW: Formal analysis, Funding acquisition, Project administration, Resources, Validation, Writing – review & editing.
Funding
The author(s) declare financial support was received for the research, authorship, and/or publication of this article. This research has been Supported by [Military Logistics Scientific Research Program of PLA] under Grant [number BLB23J017G], [number 19SWAQ07], and [National Key Research and Development Program of China] under Grant [number 2022YFF0710100].
Acknowledgments
We are grateful to the authors of the primary studies.
Conflict of interest
The authors declare that the research was conducted in the absence of any commercial or financial relationships that could be construed as a potential conflict of interest.
Publisher’s note
All claims expressed in this article are solely those of the authors and do not necessarily represent those of their affiliated organizations, or those of the publisher, the editors and the reviewers. Any product that may be evaluated in this article, or claim that may be made by its manufacturer, is not guaranteed or endorsed by the publisher.
Supplementary material
The Supplementary Material for this article can be found online at: https://www.frontiersin.org/articles/10.3389/fimmu.2023.1259399/full#supplementary-material
Abbreviations
COVID 19, coronavirus disease 2019; SARS-CoV-2, Severe Acute Respiratory Syndrome Coronavirus 2; SIV, seasonal influenza vaccine; VRIDV, viral respiratory infectious disease vaccine; PRISMA, Preferred Reporting Items for Systematic Reviews and Meta-Analyses; RCT, randomized controlled trial; AD, adverse event; SCR, seroconversion rate; SPR, seroprotection rate; GMT, geometric mean titer; GMC, geometric mean concentration; GMFR, geometric mean fold rise; ROM, ratio of mean; 95% CIs, 95% confidence intervals; GRADE, Grading of Recommendations Assessment, Development, and Evaluation.
References
1. Garcia-Beltran WF, St DK, Hoelzemer A, Lam EC, Nitido AD, Sheehan ML, et al. Mrna-based covid-19 vaccine boosters induce neutralizing immunity against SARS-cov-2 omicron variant. Cell (2022) 185:457–66. doi: 10.1016/j.cell.2021.12.033
2. Collier AY, Yu J, Mcmahan K, Liu J, Chandrashekar A, Maron JS, et al. Differential kinetics of immune responses elicited by covid-19 vaccines. N Engl J Med (2021) 385:2010–2. doi: 10.1056/NEJMc2115596
3. Li M, Wang H, Tian L, Pang Z, Yang Q, Huang T, et al. Covid-19 vaccine development: milestones, lessons and prospects. Signal Transduct Target Ther (2022) 7:146. doi: 10.1038/s41392-022-00996-y
4. Uyeki TM, Hui DS, Zambon M, Wentworth DE, Monto AS. Influenza. Lancet (2022) 400:693–706. doi: 10.1016/S0140-6736(22)00982-5
5. Adlhoch C, Fusaro A, Gonzales JL, Kuiken T, Marangon S, Niqueux É, et al. Avian influenza overview june – september 2022. Efsa J (2022) 20:7597. doi: 10.2903/j.efsa.2022.7597
6. Lazarus R, Baos S, Cappel-Porter H, Carson-Stevens A, Clout M, Culliford L, et al. Safety and immunogenicity of concomitant administration of covid-19 vaccines (Chadox1 or bnt162b2) with seasonal influenza vaccines in adults in the uk (comflucov): a multicentre, randomised, controlled, phase 4 trial. Lancet (2021) 398:2277–87. doi: 10.1016/S0140-6736(21)02329-1
7. Domnich A, Orsi A, Trombetta CS, Guarona G, Panatto D, Icardi G. Covid-19 and seasonal influenza vaccination: cross-protection, co-administration, combination vaccines, and hesitancy. Pharm (Basel) (2022) 15:322. doi: 10.3390/ph15030322
8. Levin MJ, Buchwald UK, Gardner J, Martin J, Stek JE, Brown E, et al. Immunogenicity and safety of zoster vaccine live administered with quadrivalent influenza virus vaccine. Vaccine (2018) 36:179–85. doi: 10.1016/j.vaccine.2017.08.029
9. Song JY, Cheong HJ, Hyun HJ, Seo YB, Lee J, Wie S, et al. Immunogenicity and safety of a 13-valent pneumococcal conjugate vaccine and an mf59-adjuvanted influenza vaccine after concomitant vaccination in ≧̸60-year-old adults. Vaccine (2017) 35:313–20. doi: 10.1016/j.vaccine.2016.11.047
10. Izikson R, Brune D, Bolduc JS, Bourron P, Fournier M, Moore TM, et al. Safety and immunogenicity of a high-dose quadrivalent influenza vaccine administered concomitantly with a third dose of the mrna-1273 SARS-cov-2 vaccine in adults aged >/=65 years: a phase 2, randomised, open-label study. Lancet Respir Med (2022) 10:392–402. doi: 10.1016/S2213-2600(21)00557-9
11. Page MJ, Mckenzie JE, Bossuyt PM, Boutron I, Hoffmann TC, Mulrow CD, et al. The prisma 2020 statement: an updated guideline for reporting systematic reviews. Bmj (2021) 372:n71. doi: 10.1136/bmj.n71
12. Sterne JAC, Savović J, Page MJ, Elbers RG, Blencowe NS, Boutron I, et al. Rob 2: a revised tool for assessing risk of bias in randomised trials. Bmj (2019) 366:l4898. doi: 10.1136/bmj.l4898
13. Karyotaki E, Efthimiou O, Miguel C, Bermpohl FMG, Furukawa TA, Cuijpers P, et al. Internet-based cognitive behavioral therapy for depression. JAMA Psychiatry (2021) 78:361. doi: 10.1001/jamapsychiatry.2020.4364
14. Li J, Luo J, Pavlov I, Perez Y, Tan W, Roca O, et al. Awake prone positioning for non-intubated patients with covid-19-related acute hypoxaemic respiratory failure: a systematic review and meta-analysis. Lancet Respir Med (2022) 10:573–83. doi: 10.1016/S2213-2600(22)00043-1
15. Atkins D, Best D, Briss PA, Eccles M, Falck-Ytter Y, Flottorp S, et al. Grading quality of evidence and strength of recommendations. Bmj (2004) 328:1490. doi: 10.1136/bmj.328.7454.1490
16. Knezevic I, Mattiuzzo G, Page M, Minor P, Griffiths E, Nuebling M, et al. Who international standard for evaluation of the antibody response to covid-19 vaccines: call for urgent action by the scientific community. Lancet Microbe (2022) 3:e235–40. doi: 10.1016/S2666-5247(21)00266-4
17. Toback S, Galiza E, Cosgrove C, Galloway J, Goodman AL, Swift PA, et al. Safety, immunogenicity, and efficacy of a covid-19 vaccine (nvx-cov2373) co-administered with seasonal influenza vaccines: an exploratory substudy of a randomised, observer blinded, placebo-controlled, phase 3 trial. Lancet Respir Med (2022) 10:167–79. doi: 10.1016/S2213-2600(21)00409-4
18. Schwarz TF, Flamaing J, Rümke HC, Penzes J, Juergens C, Wenz A, et al. A randomized, double-blind trial to evaluate immunogenicity and safety of 13-valent pneumococcal conjugate vaccine given concomitantly with trivalent influenza vaccine in dults aged =65 years. Vaccine (2011) 29:5195–202. doi: 10.1016/j.vaccine.2011.05.031
19. Ofori-Anyinam O, Leroux-Roels G, Drame M, Aerssens A, Maes C, Amanullah A, et al. Immunogenicity and safety of an inactivated quadrivalent influenza vaccine co-administered with a 23-valent pneumococcal polysaccharide vaccine versus separate administration, in adults =50 years of age: results from a phase iii, randomized, non-inferiority trial. Vaccine (2017) 35:6321–8. doi: 10.1016/j.vaccine.2017.09.012
20. Sadoff J, De Paepe E, Haazen W, Omoruyi E, Bastian AR, Comeaux C, et al. Safety and immunogenicity of the ad26.rsv.pref investigational vaccine coadministered with an influenza vaccine in older adults. J Infect Dis (2021) 223:699–708. doi: 10.1093/infdis/jiaa409
21. Kerzner B, Murray AV, Cheng E, Ifle R, Harvey PR, Tomlinson M, et al. Safety and immunogenicity profile of the concomitant dministration of zostavax and inactivated influenza vaccine in adults aged 50 and older. J Am Geriatr Soc (2007) 55:1499–507. doi: 10.1111/j.1532-5415.2007.01397.x
22. Severance R, Schwartz H, Dagan R, Connor L, Li J, Pedley A, et al. Safety, tolerability, and immunogenicity of v114, a 15-valent pneumococcal conjugate vaccine, administered concomitantly with influenza vaccine in healthy adults aged =50 years: a randomized phase 3 trial (pneu-flu). Hum Vaccin Immunother (2022) 18:1–14. doi: 10.1080/21645515.2021.1976581
23. Nakashima K, Aoshima M, Ohfuji S, Yamawaki S, Nemoto M, Hasegawa S, et al. Immunogenicity of simultaneous versus sequential administration of a 23-valent pneumococcal polysaccharide vaccine and a quadrivalent influenza vaccine. Hum Vaccin Immunother (2018) 14:1923–30. doi: 10.1080/21645515.2018.1455476
24. Thompson AR, Klein NP, Downey HJ, Patterson S, Sundaraiyer V, Watson W, et al. Coadministration of 13-valent pneumococcal conjugate and quadrivalent inactivated influenza vaccines in adults previously immunized with polysaccharide pneumococcal vaccine 23: a randomized clinical trial. Hum Vaccin Immunother (2018) 15:444–51. doi: 10.1080/21645515.2018.1533777
25. Zimmermann U, Gavazzi G, Richard P, Eymin C, Soubeyrand B, Baudin M. Immunogenicity and safety of a booster dose of diphtheria, tetanus, acellular pertussis and inactivated poliomyelitis vaccine (tdap-ipv; Repevax®) administered concomitantly versus non-concomitantly with an influenza vaccine (vaxigrip®) to adults aged =60 years: an open-label, randomised trial. Vaccine (2013) 31:1496–502. doi: 10.1016/j.vaccine.2012.12.081
26. Schwarz TF, Aggarwal N, Moeckesch B, Schenkenberger I, Claeys C, Douha M, et al. Immunogenicity and safety of an adjuvanted herpes zoster subunit vaccine coadministered with seasonal influenza vaccine in adults aged 50 years or older. J Infect Dis (2017) 216:1352–61. doi: 10.1093/infdis/jix481
27. Song JY, Cheong HJ, Tsai TF, Chang H, Choi MJ, Jeon JH, et al. Immunogenicity and safety of concomitant mf59-adjuvanted influenza vaccine and 23-valent pneumococcal polysaccharide vaccine administration in older adults. Vaccine (2015) 33:4647–52. doi: 10.1016/j.vaccine.2015.05.003
28. Ortiz JR, Spearman PW, Goepfert PA, Cross K, Buddy Creech C, Chen WH, et al. Safety and immunogenicity of monovalent h7n9 influenza vaccine with as03 adjuvant given sequentially or simultaneously with a seasonal influenza vaccine: a randomized clinical trial. Vaccine (2022) 40:3253–62. doi: 10.1016/j.vaccine.2022.03.055
29. Weston WM, Friedland LR, Wu X, Howe B. Vaccination of adults 65 years of age and older with tetanus toxoid, reduced diphtheria toxoid and acellular pertussis vaccine (boostrix®): results of two randomized trials. Vaccine (2012) 30:1721–8. doi: 10.1016/j.vaccine.2011.12.055
30. Weston WM, Chandrashekar V, Friedland LR, Howe B. Safety and immunogenicity of a tetanus toxoid, reduced diphtheria toxoid, and acellular pertussis vaccine when co-administered with influenza vaccine in adults. Hum Vaccin (2009) 5:858–66. doi: 10.4161/hv.9961
31. Shenyu W, Xiaoqian D, Bo C, Xuan D, Zeng W, Hangjie Z, et al. Immunogenicity and safety of a SARS-cov-2 inactivated vaccine (coronavac) co-administered with an inactivated quadrivalent influenza vaccine: a randomized, open-label, controlled study in healthy adults aged 18 to 59 years in china. Vaccine (2022) 40:5356–5. doi: 10.1016/j.vaccine.2022.07.021
32. Frenck RW, Gurtman A, Rubino J, Smith W, van Cleeff M, Jayawardene D, et al. Randomized, controlled trial of a 13-valent pneumococcal conjugate vaccine administered concomitantly with an influenza vaccine in healthy adults. Clin Vaccine Immuno (2012) 19:126–303. doi: 10.1128/CVI.00176-
33. Herbinger K, von Sonnenburg F, Nothdurft HD, Perona P, Borkowski A, Fragapane E, et al. A phase ii study of an investigational tetravalent influenza vaccine formulation combining mf59®. Hum Vaccin Immunother (2014) 10:92–9. doi: 10.4161/hv.26495
34. Barouch DH. Covid-19 vaccines - immunity, variants, boosters. N Engl J Med (2022) 387:1011–20. doi: 10.1056/NEJMra2206573
35. Edwards AM, Baric RS, Saphire EO, Ulmer JB. Stopping pandemics before they start: lessons learned from SARS-cov-2. Science (2022) 375:1133–9. doi: 10.1126/science.abn1900
36. Debisarun PA, Gössling KL, Bulut O, Kilic G, Zoodsma M, Liu Z, et al. Induction of trained immunity by influenza vaccination - impact on covid-19. PloS Pathog (2021) 17:e1009928. doi: 10.1371/journal.ppat.1009928
37. Marin-Hernandez D, Nixon DF, Hupert N. Heterologous vaccine interventions: boosting immunity against future pandemics. Mol Med (2021) 27:54. doi: 10.1186/s10020-021-00317-z
38. Lv J, Wu H, Xu J, Liu J. Immunogenicity and safety of heterologous versus homologous prime-boost schedules with an adenoviral vectored and mrna covid-19 vaccine: a systematic review. Infect Dis Poverty (2022) 11:53. doi: 10.1186/s40249-022-00977-x
39. Sadarangani M, Marchant A, Kollmann TR. Immunological mechanisms of vaccine-induced protection against covid-19 in humans. Nat Rev Immunol (2021) 21:475–84. doi: 10.1038/s41577-021-00578-z
40. Lee JKH, Lam GKL, Shin T, Samson SI, Greenberg DP, Chit A. Efficacy and effectiveness of high-dose influenza vaccine in older adults by circulating strain and antigenic match: an updated systematic review and meta-analysis. Vaccine (2021) 39:A24–35. doi: 10.1016/j.vaccine.2020.09.004
41. Fink AL, Klein SL. Sex and gender impact immune responses to vaccines among the elderly. Physiology (2015) 39:408–16. doi: 10.1152/physiol.00035.2015
42. Klein SL. Sex influences immune responses to viruses, and efficacy of prophylaxis and treatments for viral diseases. Bioessays (2012) 30:1050–9. doi: 10.1002/bies.201200099
43. Bonanni P, Boccalini S, Bechini A, Varone O, Matteo G, Sandri F, et al. Co-administration of vaccines: a focus on tetravalent measles-mumps-rubella-varicella (mmrv) and meningococcal c conjugate vaccines. Hum Vaccin Immunother (2020) 16:1313–21. doi: 10.1080/21645515.2019.1688032
44. Lennon RP, Block RJ, Schneider EC, Zephrin L, Shah A. Underserved population acceptance of combination influenza-covid-19 booster vaccines. Vaccine (2022) 40:562–7. doi: 10.1016/j.vaccine.2021.11.097
45. Gallis JA, Turner EL. Relative measures of association for binary outcomes: challenges and recommendations for the global health researcher. Ann Glob Health (2019) 85:137. doi: 10.5334/aogh.2581
46. See I, Su JR, Lale A, Woo EJ, Guh AY, Shimabukuro TT, et al. Us case reports of cerebral venous sinus thrombosis with thrombocytopenia after ad26.cov2.s vaccination, march 2 to april 21, 2021. Jama (2021) 325:2448. doi: 10.1001/jama.2021.7517
Keywords: vaccine, concomitant administration, COVID-19, seasonal influenza, vaccine efficacy
Citation: Lu D, Han Y, Xu R, Qin M, Shi J, Zhang C, Zhang J, Ye F, Luo Z, Wang Y, Wang C and Wang C (2023) Evaluation of the efficacy, safety and influencing factors of concomitant and sequential administration of viral respiratory infectious disease vaccines: a systematic review and meta-analysis. Front. Immunol. 14:1259399. doi: 10.3389/fimmu.2023.1259399
Received: 15 July 2023; Accepted: 01 December 2023;
Published: 21 December 2023.
Edited by:
Mahbuba Rahman, McMaster University, CanadaReviewed by:
David Pejoski, Adoram Therapeutics SA, SwitzerlandHongwei Wang, Nanjing University, China
Copyright © 2023 Lu, Han, Xu, Qin, Shi, Zhang, Zhang, Ye, Luo, Wang, Wang and Wang. This is an open-access article distributed under the terms of the Creative Commons Attribution License (CC BY). The use, distribution or reproduction in other forums is permitted, provided the original author(s) and the copyright owner(s) are credited and that the original publication in this journal is cited, in accordance with accepted academic practice. No use, distribution or reproduction is permitted which does not comply with these terms.
*Correspondence: Chunhui Wang, 13912966353@139.com
†These authors share first authorship