- Medical College of Georgia, Augusta University, Augusta, GA, United States
Kynurenine (Kyn) is a circulating tryptophan (Trp) catabolite generated by enzymes including IDO1 that are induced by inflammatory cytokines such as interferon-gamma. Kyn levels in circulation increase with age and Kyn is implicated in several age-related disorders including neurodegeneration, osteoporosis, and sarcopenia. Importantly, Kyn increases with progressive disease in HIV patients, and antiretroviral therapy does not normalize IDO1 activity in these subjects. Kyn is now recognized as an endogenous agonist of the aryl hydrocarbon receptor, and AhR activation itself has been found to induce muscle atrophy, increase the activity of bone-resorbing osteoclasts, decrease matrix formation by osteoblasts, and lead to senescence of bone marrow stem cells. Several IDO1 and AhR inhibitors are now in clinical trials as potential cancer therapies. We propose that some of these drugs may be repurposed to improve musculoskeletal health in older adults living with HIV.
1 Kynurenine accumulation with aging and HIV infection
The World Health Organization estimates that 38 million people are infected with HIV worldwide, and of those 23 million currently receive antiretroviral therapy (ART). Progress in ART has enabled patients to live longer, and approximately 50% of people with HIV are older than 50 years (1). Yet, patients on ART still experience major complications and comorbidities from HIV infection. It is estimated that 20-30% of patients receiving ART experience significant muscle wasting (2, 3). Indeed, a frailty phenotype is frequently observed in HIV patients on long-term ART (4), involving declines in functional measures such as grip strength and gait speed (5, 6). In addition, both osteoporosis and sarcopenia are often observed together in older people living with HIV (7). Loss of muscle mass and strength are in turn associated with poor health outcomes ranging from accelerated disease progression to increased mortality (8, 9). The mechanisms underlying impaired muscle function and the frailty phenotype of patients with HIV are not well understood, but they do suggest an earlier onset of age-related musculoskeletal decline as compared to the HIV-naïve population, a phenomenon referred to as accentuated aging (10). A key question from the recent (2020) NIH HIV ACTION Workshop on Pathogenesis of Aging in People with HIV (10) is “Do the mechanisms and mediators of age-related end-organ disease (e.g., sarcopenia) differ in aging with and without HIV and ART?”
Tryptophan (Trp) is an essential amino acid that cannot be synthesized in humans and is only available through dietary sources. Approximately 90-95% of dietary Trp is degraded along the kynurenine (Kyn) pathway by two major enzymes; tryptophan 2, 3 dioxygenase (TDO) in the liver, and indoleamine 2, 3 dioxygenase (IDO) extrahepatically (11). A smaller (~3%) portion of Trp is metabolized along the serotonin/melatonin pathway. In the kynurenine pathway, Kyn is the first stable intermediate metabolite formed (12). Kyn has been reported to exert its functions through the Aryl hydrocarbon receptor (AhR), which acts as a transcription factor (13, 14). A number of recent studies demonstrate that Kyn increases with age (15–17). For example, Sorgdrager et al. (15) demonstrated that Kyn is increased in serum with age in humans but Kyn metabolites such as kynurenic acid (KA) and quinolinic acid (QA) also increase, suggesting that the entire Kyn pathway may be upregulated with aging. The authors found that the same metabolites also increased with age in the cerebrospinal fluid (15). Likewise, de Bie et al. showed that the Kyn/Trp ratio was significantly correlated with age in older women (16), and El Refaey et al. showed that in mice Kyn increased in an age-dependent manner (17).
Kyn has been implicated in several age-related disorders including neurodegeneration, osteoporosis, sarcopenia, and inflammation (16–20). Treatment of rodents with exogenous Kyn, either through diet or intraperitoneal injections, can induce bone loss (17, 21, 22) and muscle atrophy (19). An increase in IDO activity has been linked to an increased mortality rate in humans (23), and frailty is associated with a marked increase in the Kyn/Trp ratio (24–27). Suppressing Trp degradation and thus Kyn accumulation increases lifespan in both C. elegans (28) and Drosophila (29), and increased longevity in bats is associated with endogenous inhibition of Trp breakdown by the gut microbiome (30). Importantly, Kyn increases with progressive disease in HIV patients (31–34) along with increased serum IFN gamma (35). Furthermore, ART does not normalize IDO activity in these subjects (36, 37).
2 Mechanisms of kynurenine biogenesis with aging and HIV infection
Trp commitment to the Kyn pathway is mediated by indoleamine 2,3-dioxygenase (IDO1/IDO2) or tryptophan 2,3-dioxygenase 2 (TDO) (38–40). The expression of TDO and IDO enzymes in mammals varies not only by tissue/cellular localization but also by the mechanism of their stimulation (12). TDO is primarily found in the liver and neuronal tissue and is regulated by glucocorticoids, where it helps to maintain homeostasis of dietary Trp levels and mediate immune-related diseases and central nervous system disorders (41) The function of IDO1 was initially described as an innate mechanism of defense against microbial invasion because IDO1 could induce depletion of Trp (41). The expression and activity of IDO1 are upregulated in response to several inflammatory mediators including interferon-gamma (IFN-γ) (42), interleukin-1-beta (IL-1β) (43), tumor necrosis factor (TNF- α) (42), and prostaglandin-E2 (PGE2) (44). A substantial increase in inflammatory mediators such as IFN-γ, IL-1β, and TNF- α is seen in various organs and tissues with aging, a process termed inflammaging (45–48). The increase in these inflammatory cytokines increases TDO and IDO expression, further catabolizing Trp and increasing the accumulation of Kyn pathway metabolites (38). HIV infection has also been shown to increase inflammatory mediators enhancing IDO1 expression (49–51). Furthermore, it has been observed that HIV-infected individuals have lower circulating Trp levels despite adequate Trp dietary intake, suggesting an enhanced Trp degradation (52). This common mechanism in IDO1 activation in both aging and HIV infection might shed some light on the observed accelerated aging in HIV infected individuals (53) (Figure 1). Nuclear factor kappa B (NF-κB) activation downstream of inflammatory cytokine signaling is known to induce TDOI/DO1 (31–33, 54), which is considered among the most common mechanisms of Kyn biogenesis in the setting of systemic inflammation with aging and HIV infection.
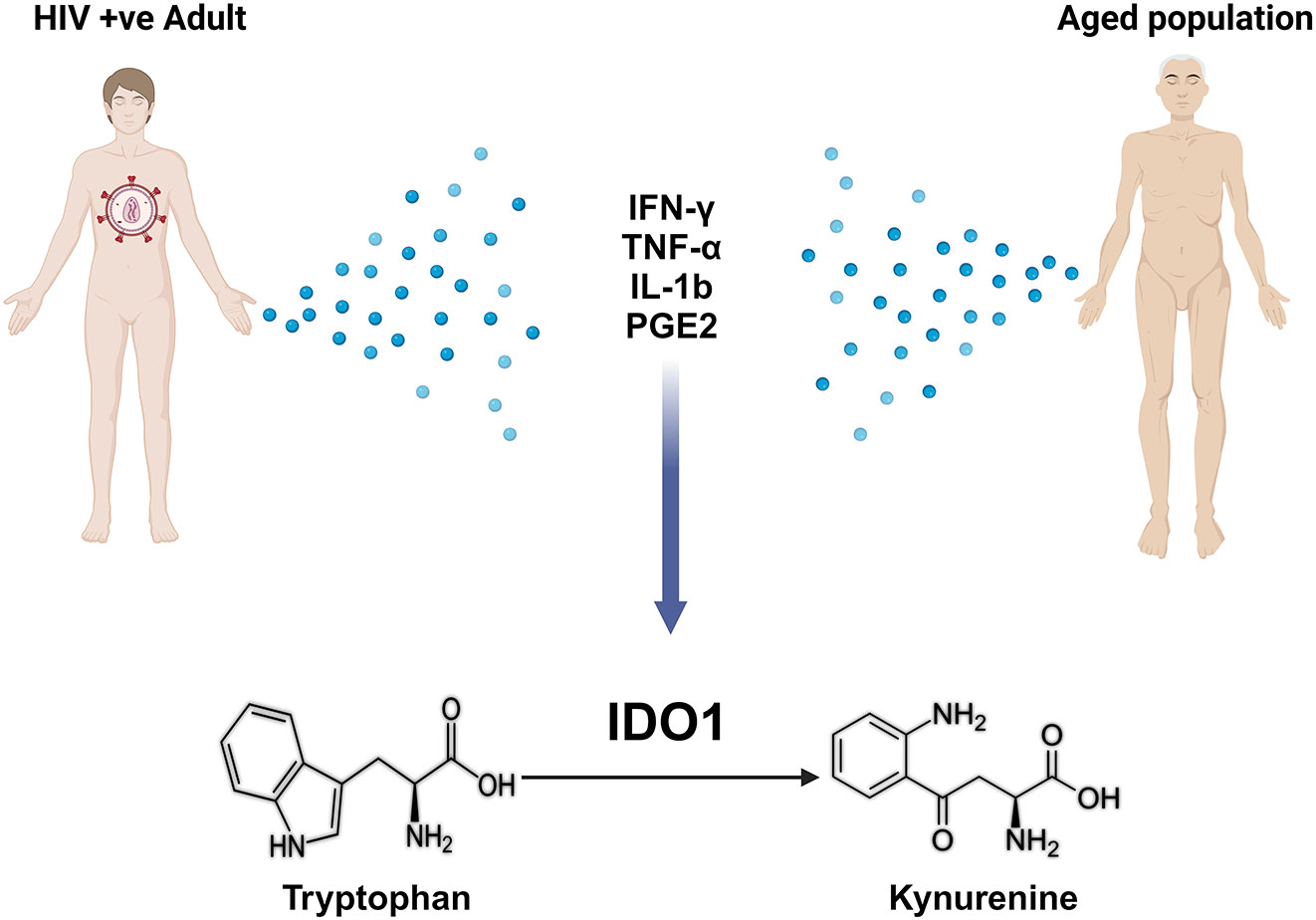
Figure 1 Shared mechanisms between aging and HIV infection in enhancing tryptophan metabolism to kynurenine via IDO1. Figure generated using BioRender.
The association between the kynurenine pathway and human immunodeficiency virus (HIV) infection has been established since 1991, when the elevated kynurenine-to-tryptophan (Kyn/Trp) ratio was observed in people living with HIV (PWH) (30). Moreover, it was also observed that, after the initial stage of infection, people receiving early ART demonstrated a rapid return of the Kyn/Trp ratio to normal concomitant with an improvement in CD4+/CD8+T cells ratio in accordance with lower mucosal inflammation (55). This observation suggested a correlation between heightened kynurenine pathway activity and compromised immune function in the context of HIV infection (33). Dendritic cells (DCs) and monocytes express the enzyme IDO-1, which catabolizes Trp into Kyn. This alters CD4+ T-cell development into regulatory T cells (Tregs) rather than T-helper (Th17) cells, which negatively affects T-cell responses. Through interferon signaling and Toll-like receptor stimulation, this altered Th17/Treg balance is directly related to elevated and sustained IDO-1 activity (56). Increased IDO-1 activity is further associated with the plasma microbial translocation markers, and the progression of HIV (57). Immunological dysfunction and ongoing immunological activation during HIV infection are both known to be significantly influenced by microbial translocation (58). A link may also exist between IDO activity and total HIV DNA in blood, pointing to a potential role for IDO in HIV persistence (57). There is also evidence that, in the case of HIV infection, gut dysbiosis is a major driver of IDO1 activity, Trp degradation, and Kyn biogenesis (59, 60). Specifically, the HIV infection itself can cause pronounced changes to the gut microbiota, leading to increased Trp degradation and elevated Kyn accumulation independent of IFN-gamma or NF-kB activity (35, 61, 62). The activation of the kynurenine pathway has been observed to occur through the generation of neurotoxic quinolinic acid in macrophages, as a direct result of the actions of the HIV proteins Tat, Nef, and gp41 (63).
3 Role of kynurenine signaling through the aryl hydrocarbon receptor in muscle and bone loss with aging and HIV infection
Kyn is an endogenous ligand of the aryl hydrocarbon receptor (AhR) (14, 23, 24, 64). AhR is bound to the molecular chaperone heat-shock protein 90 (Hsp90) and dwells there in an inactive state in the cytoplasm of the cell. Trp and its metabolite Kyn both enter the cell via transporters including solute carrier family 7 member 5 (SLC7A5) and member 11 (SLC7A11) (65) (Figure 2). Once inside the cell, Kyn can stimulate AhR translocation to the nucleus (66, 67)where AhR separates from Hsp90 and forms a dimer with the aryl hydrocarbon receptor nuclear translocator (ARNT) protein. The heterodimer binds to a specific DNA sequence called the xenobiotic response element, or XRE, in the promoter region of potential target genes (Figure 2). Previously it has been shown that Kyn’s AhR and transcriptional activation of AhR stimulates both TGF beta 1 and IDO1 expression; this creates a positive feedback loop as IDO1 can degrade tryptophan to produce more Kyn (68, 69). Moreover, AhR has been demonstrated to upregulate the expression of SLC7A5 (70, 71). Thus, the increase in SLC7A5 with kynurenine-activated Ahr probably facilitates more kynurenine entry into the cell (72).
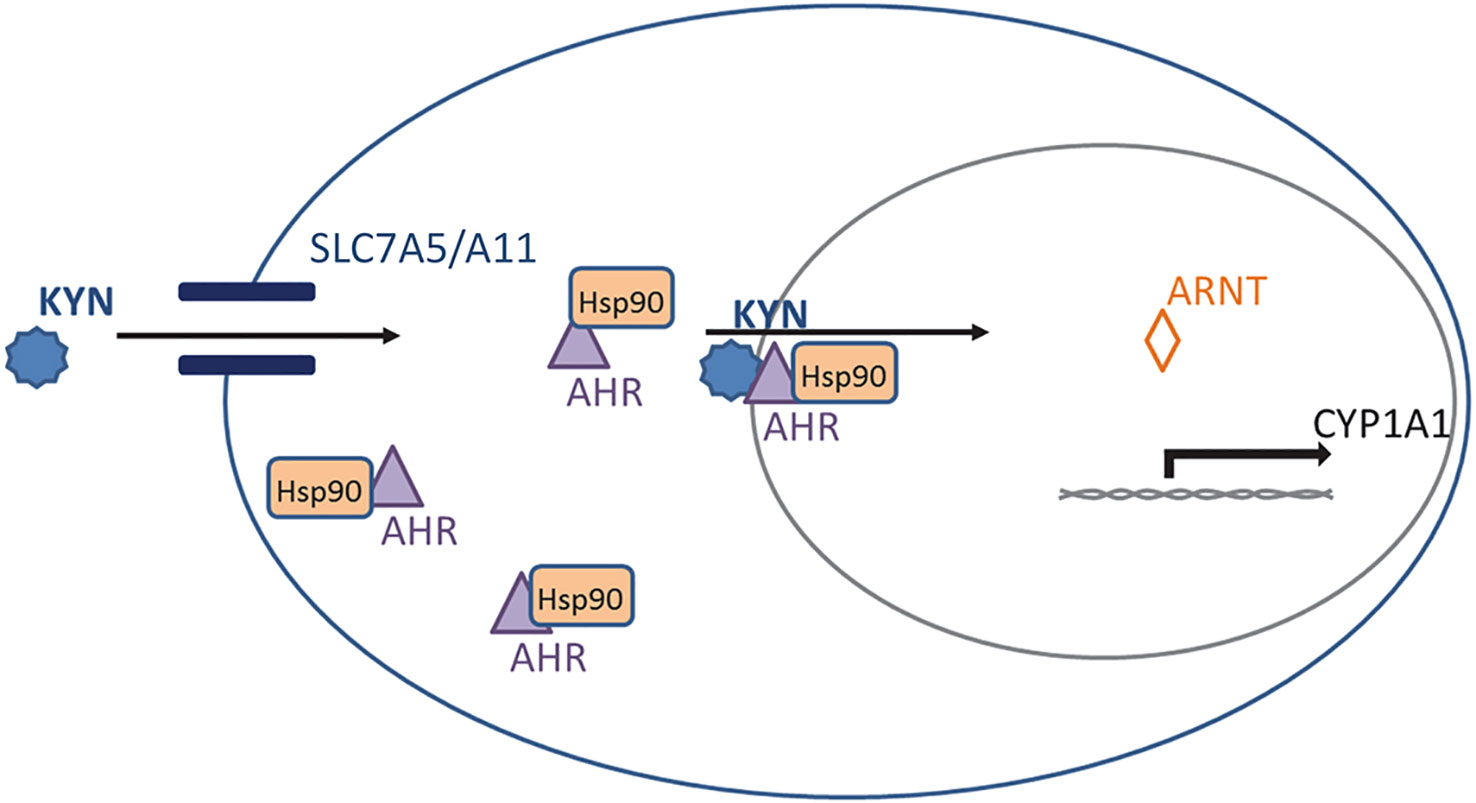
Figure 2 Kynurenine (Kyn) signaling through the aryl hydrocarbon receptor (AhR). AhR is normally bound to heat-shock protein 90 (Hsp90) in the cytoplasm. Kyn enters the cell via transporters SLC7A5 and SLC7A11 and binds the Hsp90-AhR complex. This complex translocates to the nucleus where AhR forms a complex with aryl hydrocarbon receptor nuclear translocator (Arnt) protein to activate downstream targets such as Cyp1a1.
In cases of viral infection, constitutive AhR signaling inhibits the type I interferon (IFN-I) response by upregulating the ADP-ribosylase TIPARP (73). A recent study found that AhR activation by Trp metabolites, especially Kyn, promoted HIV infection and decreased CD4+ T cell counts. AhR activates viral transcription and infection by directly binding to the HIV-1 5′ Long terminal repeat (5′-LTR) (74). Moreover, Trp metabolites increase AhR translocation to the nucleus which leads to the association with HIV 5′-LTR. Further, AhR and the HIV-1 Tat protein may bind, attracting favorable transcriptional elements that aid in infection (74). However, the role of AhR on HIV-1 activation may vary according to cell type. For example, another study found that, in macrophages, the activation of AhR is responsible for blocking HIV-1 replication by downregulating the transcription of cyclin-dependent kinase CDK1, CDK2 which ultimately exerts antiviral effects (75). Moreover, long-term exposure to AhR ligands induces hypomethylation of AhR DNA binding regions (76), and HIV infection increased hypomethylation in the promoters of numerous genes including AhR (77).
Muscle loss is frequently observed in HIV patients on ART which contributes directly to increased morbidity (2). The clinical signs of frailty, such as loss of energy, weight, and muscle mass, as well as reduced motor function and low levels of physical activity, are strikingly similar to those of advanced HIV infection (78). A key frailty mediator is a mitochondrial dysfunction which increases with aging (79). Hunt et al. (80) showed that PWH receiving contemporary ART have more skeletal muscle mitochondrial impairment than would be expected based on age alone. Activation of AhR by Kyn in the setting of aging and HIV infection is very likely a drive of muscle dysfunction in these patients. We have found that Kyn treatment in younger mice can reduce muscle size and strength with an increase in reactive oxygen species levels (19). On other hand, inhibition of IDO can increase muscle size and strength in aged mice (19). These findings are further supported by work showing that constitutive AhR overexpression increases ROS accumulation, mitochondrial dysfunction, and skeletal muscle atrophy (81). Aging and HIV infection therefore share several biological characteristics associated with musculoskeletal frailty (Figure 3).
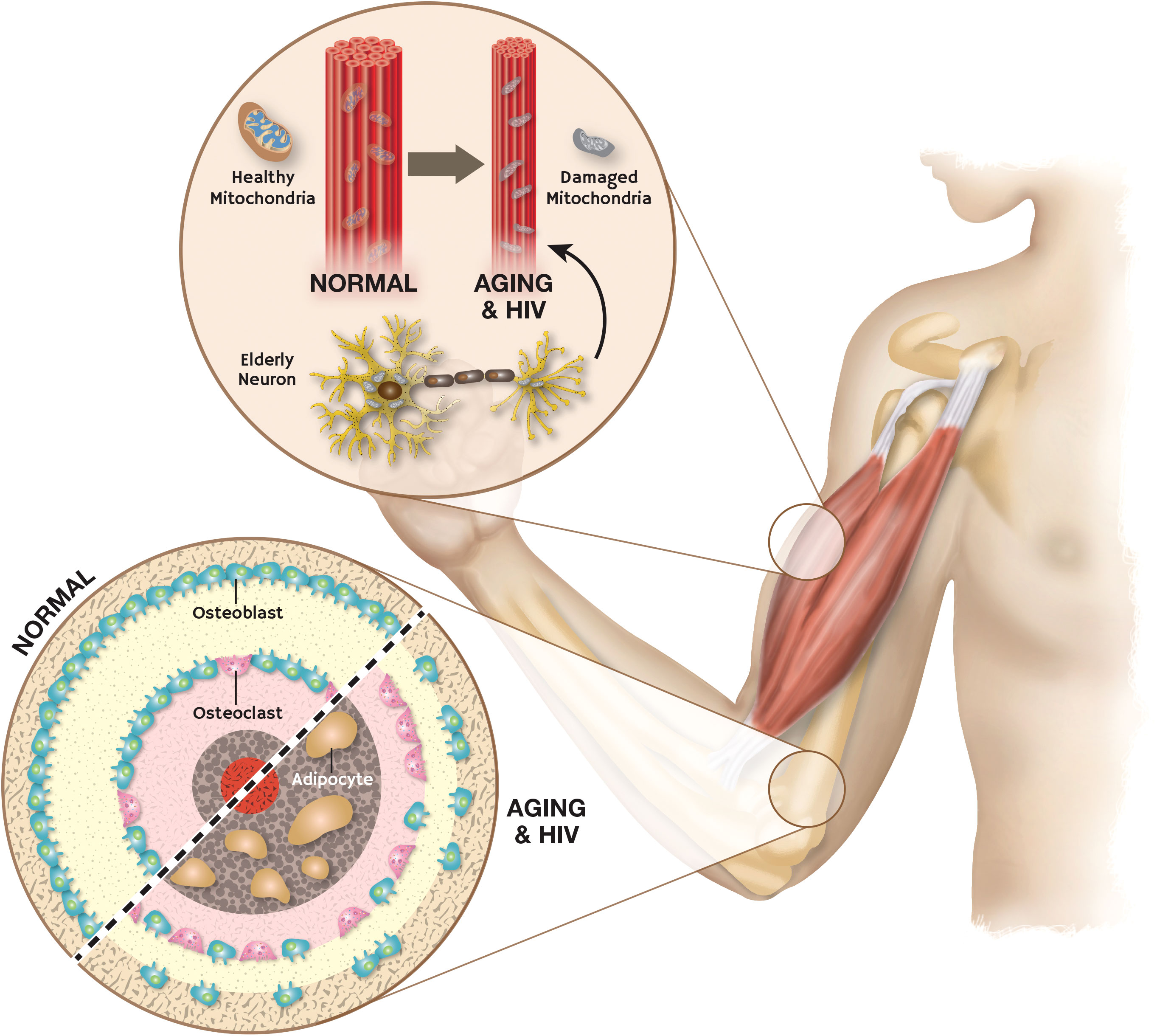
Figure 3 Aging and HIV induced damage in skeletal muscle and dysregulation of bone homeostasis: Aging and HIV cause mitochondrial damage in skeletal muscle which leads to frailty. Activation of AhR in aging and HIV causes disruption of bone homeostasis and fat deposition in skeletal muscle.
Similar findings have been made in bone that point to Kyn-induced AhR activation as a driver of bone dysfunction with aging and HIV infection. Bone mineral density (BMD) can decline rapidly in patients with HIV starting ART (82). Moreover, BMD in HIV-positive women decreases twice as quickly as compared to HIV-positive men (82). Activation of AhR signaling promotes osteoporosis with aging by stimulating the formation of osteoclasts (83, 84). While there is abundant research supporting the involvement of Kyn involvement in bone loss (17, 85–87), Eisa NH., et al. (13) further clarified that osteoclastic transcription factors are upregulated by Kyn signaling through AhR which results in an increased number of osteoclasts. Moreover, Kyn treatment in mice caused an increased serum level of the osteoclastic marker RANKL and the bone resorption marker Pyd (17). Another study reported that activation of AhR impeded the proliferation of osteoblasts derived from human mesenchymal stem cells (85), and we recently showed that AhR activation induced senescence in bone marrow stem cells (86). Kim and colleagues (87) showed the detrimental effects of Kyn on human bone metabolism where they studied bone marrow (BM) samples from patients undergoing hip surgery. They found that BM kynurenine levels increased with age and it was linked to higher fragility hip fracture risk, an increase in TRAP-5b and RANKL (two markers of bone resorption) and a decline in total femur bone mineral density (BMD). Together, these findings suggest that AhR activation in the setting of HIV infection promotes both muscle and bone loss with aging.
4 Potential strategies for therapeutic intervention
A number of experimental therapies that target the Kyn pathway are currently being tested as novel treatments for various cancers (Table 1). The majority of these, such as Epacadostat, Indoximod and Linrodostat are inhibitors of IDO1 that blunt the formation of Kyn. Others such as IK-175 and BAY2416964 are direct inhibitors of AhR activity. These therapeutics have been reported to improve outcomes in cancer patients across several different clinical trials and have shown better efficacy when used in combination with other therapeutics such as Pembrolizumab, an immune checkpoint inhibitor (CTI) (88).
Epacadostat, a highly selective and orally available inhibitor of IDO1, is currently being studied in phase 3 clinical trials following positive results from multiple phase 1 and 2 studies for a variety of cancers. In one phase 1/2 study, Epacadostat was used in combination with Pembrolizumab for patients with advanced solid tumors and showed antitumor activity and safety (89). Similar results were obtained in another phase 1/2 study of Epacadostat used with ipilimumab in patients with melanoma (90). Linrodostat is another oral inhibitor of IDO1 currently in phase 3 trials with a slightly different mechanism of action, functioning as an irreversible suicide inhibitor. In a phase 1/2 study of patients with advanced bladder cancer, the combination of nivolumab and Linrodostat was well tolerated and showed efficacy across the patient population (91). Indoximod is a Trp analogue that can suppress the IDO pathway which is normally upregulated in the absence of Trp. After demonstrating safety and efficacy in phase 1 trials of patients with advanced solid tumors (92, 93), many phase 2 clinical trials are currently active. One of these trials that have published results was a phase 2 trial investigating the addition of indoximod to patients with advanced melanoma receiving CTIs such as pembrolizumab. The therapeutic was well tolerated in this patient population and showed antitumor efficacy (94). Table 1 summarizes some of the inhibitors in clinical trials targeting AhR/IDO in cancer therapeutics.
5 Summary & conclusions
HIV is associated with accelerated or accentuated frailty. This is characterized by aging phenotypes such as cognitive impairment, cardiovascular disease, and muscle and bone loss. HIV-related proteins themselves, as well as cytokines such as IFN-gamma, increase expression of the Trp degrading enzyme IDO1, generating Kyn as a by-product of Trp degradation. Kyn and the Kyn/Trp ratio are markedly elevated in patients with HIV, even after antiretroviral therapy. Similarly, aging causes an increase in inflammatory mediators that upregulate activity of IDO1, thus promoting Trp metabolism to Kyn. However, IDO1 activity may be more pronounced in HIV infection due to gut dysbiosis which may drive IDO1 activity. Increased IDO1 is associated with microbial translocation and progression of HIV. Kyn induces senescence in bone marrow cells, reduces bone formation, and contributes to muscle atrophy and damage to the neuromuscular junction. These effects appear to be mediated in part by activation of aryl hydrocarbon receptor signaling. Small molecules that suppress Trp degradation by IDO1 have shown potential for synergizing with antiretroviral therapies to improve health outcomes. Future studies should be directed at examining AhR inhibition as a potential companion strategy.
Author contributions
SS, AE, and HB prepared the initial draft, Table and Figure 1. MH, EB and MM-L prepared Figure 2 and the subsequent draft revisions. All authors contributed to the article and approved the submitted version.
Funding
Funding for this research was provided by the US National Institutes of Health (NIAMS R01AR082307, NIA P01AG036675).
Acknowledgments
Figure 3 was skillfully prepared by Mr. Michael Cooper.
Conflict of interest
The authors declare that the research was conducted in the absence of any commercial or financial relationships that could be construed as a potential conflict of interest.
Publisher’s note
All claims expressed in this article are solely those of the authors and do not necessarily represent those of their affiliated organizations, or those of the publisher, the editors and the reviewers. Any product that may be evaluated in this article, or claim that may be made by its manufacturer, is not guaranteed or endorsed by the publisher.
References
1. Montano M, Oursler KK, Xu K, Sun YV, Marconi VC. Biological ageing with HIV infection: evaluating the geroscience hypothesis. Lancet Healthy Longev (2022) 3(3):e194–205. doi: 10.1016/S2666-7568(21)00278-6
2. Dudgeon WD, Phillips KD, Carson JA, Brewer RB, Durstine JL, Hand GA. Counteracting muscle wasting in HIV-infected individuals. HIV Med (2006) 7(5):299–310. doi: 10.1111/j.1468-1293.2006.00380.x
3. Wanke CA, Silva M, Knox TA, Forrester J, Speigelman D, Gorbach SL. Weight loss and wasting remain common complications in individuals infected with human immunodeficiency virus in the era of highly active antiretroviral therapy. Clin Infect Dis (2000) 31(3):803–5. doi: 10.1086/314027
4. Oursler KK, Iranmanesh A, Jain C, Birkett KL, Briggs BC, Garner DC, et al. Short communication: low muscle mass is associated with osteoporosis in older adults living with HIV. AIDS Res Hum Retroviruses (2020) 36(4):300–2. doi: 10.1089/aid.2019.0207
5. Schrack JA, Althoff KN, Jacobson LP, Erlandson KM, Jamieson BD, Koletar SL, et al. Accelerated longitudinal gait speed decline in HIV-infected older men. J Acquir Immune Defic Syndr (2015) 70(4):370–6. doi: 10.1097/QAI.0000000000000731
6. Schrack JA, Jacobson LP, Althoff KN, Erlandson KM, Jamieson BD, Koletar SL, et al. Effect of HIV-infection and cumulative viral load on age-related decline in grip strength. AIDS (2016) 30(17):2645–52. doi: 10.1097/QAD.0000000000001245
7. Althoff KN, Jacobson LP, Cranston RD, Detels R, Phair JP, Li X, et al. Age, comorbidities, and AIDS predict a frailty phenotype in men who have sex with men. J Gerontol A Biol Sci Med Sci (2014) 69(2):189–98. doi: 10.1093/gerona/glt148
8. Wheeler DA, Gibert CL, Launer CA, Muurahainen N, Elion RA, Abrams DI, et al. Weight loss as a predictor of survival and disease progression in HIV infection. Terry Beirn Community Programs for Clinical Research on AIDS. . J Acquir Immune Defic Syndr Hum Retrovirol (1998) 18(1):80–5. doi: 10.1097/00042560-199805010-00012
9. Scherzer R, Heymsfield SB, Lee D, Powderly WG, Tien PC, Bacchetti P, et al. Decreased limb muscle and increased central adiposity are associated with 5-year all-cause mortality in HIV infection. AIDS (2011) 25(11):1405–14. doi: 10.1097/QAD.0b013e32834884e6
10. Gabuzda D, Jamieson BD, Collman RG, Lederman MM, Burdo TH, Deeks SG, et al. Pathogenesis of aging and age-related comorbidities in people with HIV: highlights from the HIV ACTION workshop. Pathog Immun (2020) 5(1):143–74. doi: 10.20411/pai.v5i1.365
11. Jones SP, Guillemin GJ, Brew BJ. The kynurenine pathway in stem cell biology. Int J Tryptophan Res (2013) 6:57–66. doi: 10.4137/IJTR.S12626
12. Ball HJ, Jusof FF, Bakmiwewa SM, Hunt NH, Yuasa HJ. Tryptophan-catabolizing enzymes - party of three. Front Immunol (2014) 5:485. doi: 10.3389/fimmu.2014.00485
13. Eisa NH, Reddy SV, Elmansi AM, Kondrikova G, Kondrikov D, Shi XM, et al. Kynurenine promotes RANKL-induced osteoclastogenesis in vitro by activating the aryl hydrocarbon receptor pathway. Int J Mol Sci (2020) 21(21). doi: 10.3390/ijms21217931
14. Alhamad DW, Bensreti H, Dorn J, Hill WD, Hamrick MW, McGee-Lawrence ME. Aryl hydrocarbon receptor (AhR)-mediated signaling as a critical regulator of skeletal cell biology. J Mol Endocrinol (2022) 69(3):R109–R24. doi: 10.1530/JME-22-0076
15. Sorgdrager FJH, Vermeiren Y, Van Faassen M, van der Ley C, Nollen EAA, Kema IP, et al. Age- and disease-specific changes of the kynurenine pathway in Parkinson's and Alzheimer's disease. J Neurochem (2019) 151(5):656–68. doi: 10.1111/jnc.14843
16. de Bie J, Guest J, Guillemin GJ, Grant R. Central kynurenine pathway shift with age in women. J Neurochem (2016) 136(5):995–1003. doi: 10.1111/jnc.13496
17. Refaey ME, McGee-Lawrence ME, Fulzele S, Kennedy EJ, Bollag WB, Elsalanty M, et al. Kynurenine, a tryptophan metabolite that accumulates with age, induces bone loss. J Bone Miner Res (2017) 32(11):2182–93. doi: 10.1002/jbmr.3224
18. Braidy N, Guillemin GJ, Mansour H, Chan-Ling T, Grant R. Changes in kynurenine pathway metabolism in the brain, liver and kidney of aged female Wistar rats. FEBS J (2011) 278(22):4425–34. doi: 10.1111/j.1742-4658.2011.08366.x
19. Kaiser H, Yu K, Pandya C, Mendhe B, Isales CM, McGee-Lawrence ME, et al. Kynurenine, a tryptophan metabolite that increases with age, induces muscle atrophy and lipid peroxidation. Oxid Med Cell Longev (2019) 2019:9894238. doi: 10.1155/2019/9894238
20. Chatterjee P, Goozee K, Lim CK, James I, Shen K, Jacobs KR, et al. Alterations in serum kynurenine pathway metabolites in individuals with high neocortical amyloid-beta load: A pilot study. Sci Rep (2018) 8(1):8008. doi: 10.1038/s41598-018-25968-7
21. Pierce JL, Roberts RL, Yu K, Kendall RK, Kaiser H, Davis C, et al. Kynurenine suppresses osteoblastic cell energetics in vitro and osteoblast numbers in vivo. Exp Gerontol (2020) 130:110818. doi: 10.1016/j.exger.2019.110818
22. Bensreti H, Yu K, Alhamad DW, Shaver J, Kaiser H, Zhong R, et al. Orchiectomy sensitizes cortical bone in male mice to the harmful effects of kynurenine. Bone (2023) 173:116811. doi: 10.1016/j.bone.2023.116811
23. Pertovaara M, Raitala A, Lehtimaki T, Karhunen PJ, Oja SS, Jylha M, et al. Indoleamine 2,3-dioxygenase activity in nonagenarians is markedly increased and predicts mortality. Mech Ageing Dev (2006) 127(5):497–9. doi: 10.1016/j.mad.2006.01.020
24. Valdiglesias V, Marcos-Perez D, Lorenzi M, Onder G, Gostner JM, Strasser B, et al. Immunological alterations in frail older adults: A cross sectional study. Exp Gerontol (2018) 112:119–26. doi: 10.1016/j.exger.2018.09.010
25. Westbrook R, Chung T, Lovett J, Ward C, Joca H, Yang H, et al. Kynurenines link chronic inflammation to functional decline and physical frailty. JCI Insight (2020) 5(16). doi: 10.1172/jci.insight.136091
26. Jang IY, Park JH, Kim JH, Lee S, Lee E, Lee JY, et al. The association of circulating kynurenine, a tryptophan metabolite, with frailty in older adults. Aging (Albany NY) (2020) 12(21):22253–65. doi: 10.18632/aging.104179
27. Marcos-Perez D, Sanchez-Flores M, Maseda A, Lorenzo-Lopez L, Millan-Calenti JC, Strasser B, et al. Frailty status in older adults is related to alterations in indoleamine 2,3-dioxygenase 1 and guanosine triphosphate cyclohydrolase I enzymatic pathways. J Am Med Dir Assoc (2017) 18(12):1049–57. doi: 10.1016/j.jamda.2017.06.021
28. van der Goot AT, Zhu W, Vazquez-Manrique RP, Seinstra RI, Dettmer K, Michels H, et al. Delaying aging and the aging-associated decline in protein homeostasis by inhibition of tryptophan degradation. Proc Natl Acad Sci U S A (2012) 109(37):14912–7. doi: 10.1073/pnas.1203083109
29. Oxenkrug GF, Navrotskaya V, Voroboyva L, Summergrad P. Extension of life span of Drosophila melanogaster by the inhibitors of tryptophan-kynurenine metabolism. Fly (Austin) (2011) 5(4):307–9. doi: 10.4161/fly.5.4.18414
30. Ball HC, Levari-Shariati S, Cooper LN, Aliani M. Comparative metabolomics of aging in a long-lived bat: Insights into the physiology of extreme longevity. PloS One (2018) 13(5):e0196154. doi: 10.1371/journal.pone.0196154
31. Brown RR, Ozaki Y, Datta SP, Borden EC, Sondel PM, Malone DG. Implications of interferon-induced tryptophan catabolism in cancer, auto-immune diseases and AIDS. Adv Exp Med Biol (1991) 294:425–35. doi: 10.1007/978-1-4684-5952-4_39
32. Kandanearatchi A, Brew BJ. The kynurenine pathway and quinolinic acid: pivotal roles in HIV associated neurocognitive disorders. FEBS J (2012) 279(8):1366–74. doi: 10.1111/j.1742-4658.2012.08500.x
33. Huengsberg M, Winer JB, Gompels M, Round R, Ross J, Shahmanesh M. Serum kynurenine-to-tryptophan ratio increases with progressive disease in HIV-infected patients. Clin Chem (1998) 44(4):858–62. doi: 10.1093/clinchem/44.4.858
34. Baer SL, Colombo RE, Johnson MH, Wakade S, Pacholczyk G, Newman-Whitlow C, et al. Indoleamine 2,3 dioxygenase, age, and immune activation in people living with HIV. J Investig Med (2021) 69(6):1238–44. doi: 10.1136/jim-2021-001794
35. Routy JP, Mehraj V, Vyboh K, Cao W, Kema I, Jenabian MA. Clinical relevance of kynurenine pathway in HIV/AIDS: an immune checkpoint at the crossroads of metabolism and inflammation. AIDS Rev (2015) 17(2):96–106.
36. Martinez P, Tsai AC, Muzoora C, Kembabazi A, Weiser SD, Huang Y, et al. Reversal of the Kynurenine pathway of tryptophan catabolism may improve depression in ART-treated HIV-infected Ugandans. J Acquir Immune Defic Syndr (2014) 65(4):456–62. doi: 10.1097/QAI.0000000000000062
37. Chen J, Shao J, Cai R, Shen Y, Zhang R, Liu L, et al. Anti-retroviral therapy decreases but does not norMalize indoleamine 2,3-dioxygenase activity in HIV-infected patients. PloS One (2014) 9(7):e100446. doi: 10.1371/journal.pone.0100446
38. Sorgdrager FJH, Naude PJW, Kema IP, Nollen EA, Deyn PP. Tryptophan metabolism in inflammaging: from biomarker to therapeutic target. Front Immunol (2019) 10:2565. doi: 10.3389/fimmu.2019.02565
39. Savitz J. The kynurenine pathway: a finger in every pie. Mol Psychiatry (2020) 25(1):131–47. doi: 10.1038/s41380-019-0414-4
40. Jayawickrama GS, Nematollahi A, Sun G, Gorrell MD, Church WB. Inhibition of human kynurenine aminotransferase isozymes by estrogen and its derivatives. Sci Rep (2017) 7(1):17559. doi: 10.1038/s41598-017-17979-7
41. Song X, Si Q, Qi R, Liu W, Li M, Guo M, et al. Indoleamine 2,3-dioxygenase 1: A promising therapeutic target in Malignant tumor. Front Immunol (2021) 12:800630. doi: 10.3389/fimmu.2021.800630
42. Banzola I, Mengus C, Wyler S, Hudolin T, Manzella G, Chiarugi A, et al. Expression of indoleamine 2,3-dioxygenase induced by IFN-gamma and TNF-alpha as potential biomarker of prostate cancer progression. Front Immunol (2018) 9:1051. doi: 10.3389/fimmu.2018.01051
43. Zunszain PA, Anacker C, Cattaneo A, Choudhury S, Musaelyan K, Myint AM, et al. Interleukin-1beta: a new regulator of the kynurenine pathway affecting human hippocampal neurogenesis. Neuropsychopharmacology (2012) 37(4):939–49. doi: 10.1038/npp.2011.277
44. Trabanelli S, Lecciso M, Salvestrini V, Cavo M, Ocadlikova D, Lemoli RM, et al. PGE2-induced IDO1 inhibits the capacity of fully mature DCs to elicit an in vitro antileukemic immune response. J Immunol Res (2015) 2015:253191. doi: 10.1155/2015/253191
45. Alvarez-Rodriguez L, Lopez-Hoyos M, Munoz-Cacho P, Martinez-Taboada VM. Aging is associated with circulating cytokine dysregulation. Cell Immunol (2012) 273(2):124–32. doi: 10.1016/j.cellimm.2012.01.001
46. Bandres E, Merino J, Vazquez B, Inoges S, Moreno C, Subira ML, et al. The increase of IFN-gamma production through aging correlates with the expanded CD8(+high)CD28(-)CD57(+) subpopulation. Clin Immunol (2000) 96(3):230–5. doi: 10.1006/clim.2000.4894
47. Wu D, Mura C, Beharka AA, Han SN, Paulson KE, Hwang D, et al. Age-associated increase in PGE2 synthesis and COX activity in murine macrophages is reversed by vitamin E. Am J Physiol (1998) 275(3):C661–8. doi: 10.1152/ajpcell.1998.275.3.C661
48. Franceschi C, Garagnani P, Vitale G, Capri M, Salvioli S. Inflammaging and 'Garb-aging'. Trends Endocrinol Metab (2017) 28(3):199–212. doi: 10.1016/j.tem.2016.09.005
49. Sparks R, Koelle DM, Stern JE, Dhanireddy S. Elevated spontaneous interferon-gamma secretion in human immunodeficiency virus-infected persons. Open Forum Infect Dis (2017) 4(2):ofx055. doi: 10.1093/ofid/ofx055
50. De Pablo-Bernal RS, Ruiz-Mateos E, Rosado I, Dominguez-Molina B, Alvarez-Rios AI, Carrillo-Vico A, et al. TNF-alpha levels in HIV-infected patients after long-term suppressive cART persist as high as in elderly, HIV-uninfected subjects. J Antimicrob Chemother (2014) 69(11):3041–6. doi: 10.1093/jac/dku263
51. Guo H, Gao J, Taxman DJ, Ting JP, Su L. HIV-1 infection induces interleukin-1beta production via TLR8 protein-dependent and NLRP3 inflammasome mechanisms in human monocytes. J Biol Chem (2014) 289(31):21716–26. doi: 10.1074/jbc.M114.566620
52. Murray MF. Tryptophan depletion and HIV infection: a metabolic link to pathogenesis. Lancet Infect Dis (2003) 3(10):644–52. doi: 10.1016/S1473-3099(03)00773-4
53. Meir-Shafrir K, Pollack S. Accelerated aging in HIV patients. Rambam Maimonides Med J (2012) 3(4):e0025. doi: 10.5041/RMMJ.10089
54. MacCann R, Landay AL, Mallon PWG. HIV and comorbidities - the importance of gut inflammation and the kynurenine pathway. Curr Opin HIV AIDS (2023) 18(2):102–10. doi: 10.1097/COH.0000000000000782
55. Jenabian MA, El-Far M, Vyboh K, Kema I, Costiniuk CT, Thomas R, et al. Immunosuppressive tryptophan catabolism and gut mucosal dysfunction following early HIV infection. J Infect Dis (2015) 212(3):355–66. doi: 10.1093/infdis/jiv037
56. Favre D, Mold J, Hunt PW, Kanwar B, Loke P, Seu L, et al. Tryptophan catabolism by indoleamine 2,3-dioxygenase 1 alters the balance of TH17 to regulatory T cells in HIV disease. Sci Transl Med (2010) 2(32):32ra6. doi: 10.1126/scitranslmed.3000632
57. Chen J, Xun J, Yang J, Ji Y, Liu L, Qi T, et al. Plasma indoleamine 2,3-dioxygenase activity is associated with the size of the human immunodeficiency virus reservoir in patients receiving antiretroviral therapy. Clin Infect Dis (2019) 68(8):1274–81. doi: 10.1093/cid/ciy676
58. Sandler NG, Douek DC. Microbial translocation in HIV infection: causes, consequences and treatment opportunities. Nat Rev Microbiol (2012) 10(9):655–66. doi: 10.1038/nrmicro2848
59. Vesterbacka J, Rivera J, Noyan K, Parera M, Neogi U, Calle M, et al. Richer gut microbiota with distinct metabolic profile in HIV infected Elite Controllers. Sci Rep (2017) 7(1):6269. doi: 10.1038/s41598-017-06675-1
60. Wang M, Dong X, Huang Y, Su J, Dai X, Guo Y, et al. Activation of the kynurenine pathway is associated with poor outcome in Pneumocystis pneumonia patients infected with HIV: results of 2 months cohort study. BMC Infect Dis (2019) 19(1):223. doi: 10.1186/s12879-019-3851-4
61. Gelpi M, Vestad B, Raju SC, Hansen SH, Hogh J, Midttun O, et al. Association of the kynurenine pathway of tryptophan metabolism with human immunodeficiency virus-related gut microbiota alterations and visceral adipose tissue accumulation. J Infect Dis (2022) 225(11):1948–54. doi: 10.1093/infdis/jiac018
62. Vujkovic-Cvijin I, Dunham RM, Iwai S, Maher MC, Albright RG, Broadhurst MJ, et al. Dysbiosis of the gut microbiota is associated with HIV disease progression and tryptophan catabolism. Sci Transl Med (2013) 5(193):193ra91s. doi: 10.1126/scitranslmed.3006438
63. Smith DG, Guillemin GJ, Pemberton L, Kerr S, Nath A, Smythe GA, et al. Quinolinic acid is produced by macrophages stimulated by platelet activating factor, Nef and Tat. J Neurovirol (2001) 7(1):56–60. doi: 10.1080/135502801300069692
64. Ferrucci L, Fabbri E. Inflammageing: chronic inflammation in ageing, cardiovascular disease, and frailty. Nat Rev Cardiol (2018) 15(9):505–22. doi: 10.1038/s41569-018-0064-2
65. Sinclair LV, Neyens D, Ramsay G, Taylor PM, Cantrell DA. Single cell analysis of kynurenine and System L amino acid transport in T cells. Nat Commun (2018) 9(1):1981. doi: 10.1038/s41467-018-04366-7
66. Opitz CA, Litzenburger UM, Sahm F, Ott M, Tritschler I, Trump S, et al. An endogenous tumour-promoting ligand of the human aryl hydrocarbon receptor. Nature (2011) 478(7368):197–203. doi: 10.1038/nature10491
67. Yamamoto T, Hatabayashi K, Arita M, Yajima N, Takenaka C, Suzuki T, et al. Kynurenine signaling through the aryl hydrocarbon receptor maintains the undifferentiated state of human embryonic stem cells. Sci Signal (2019) 12(587). doi: 10.1126/scisignal.aaw3306
68. Vogel CF, Goth SR, Dong B, Pessah IN, Matsumura F. Aryl hydrocarbon receptor signaling mediates expression of indoleamine 2,3-dioxygenase. Biochem Biophys Res Commun (2008) 375(3):331–5. doi: 10.1016/j.bbrc.2008.07.156
69. Nuti R, Gargaro M, Matino D, Dolciami D, Grohmann U, Puccetti P, et al. Ligand binding and functional selectivity of L-tryptophan metabolites at the mouse aryl hydrocarbon receptor (mAhR). J Chem Inf Model (2014) 54(12):3373–83. doi: 10.1021/ci5005459
70. Brauze D, Zawierucha P, Kiwerska K, Bednarek K, Oleszak M, Rydzanicz M, et al. Induction of expression of aryl hydrocarbon receptor-dependent genes in human HepaRG cell line modified by shRNA and treated with beta-naphthoflavone. Mol Cell Biochem (2017) 425(1-2):59–75. doi: 10.1007/s11010-016-2862-3
71. Kim S, Dere E, Burgoon LD, Chang CC, Zacharewski TR. Comparative analysis of AhR-mediated TCDD-elicited gene expression in human liver adult stem cells. Toxicol Sci (2009) 112(1):229–44. doi: 10.1093/toxsci/kfp189
72. Kaiser H, Parker E, Hamrick MW. Kynurenine signaling through the aryl hydrocarbon receptor: Implications for aging and healthspan. Exp Gerontol (2020) 130:110797. doi: 10.1016/j.exger.2019.110797
73. Yamada T, Horimoto H, Kameyama T, Hayakawa S, Yamato H, Dazai M, et al. Constitutive aryl hydrocarbon receptor signaling constrains type I interferon-mediated antiviral innate defense. Nat Immunol (2016) 17(6):687–94. doi: 10.1038/ni.3422
74. Zhou YH, Sun L, Chen J, Sun WW, Ma L, Han Y, et al. Tryptophan metabolism activates aryl hydrocarbon receptor-mediated pathway to promote HIV-1 infection and reactivation. mBio (2019) 10(6). doi: 10.1128/mBio.02591-19
75. Kueck T, Cassella E, Holler J, Kim B, Bieniasz PD. The aryl hydrocarbon receptor and interferon gamma generate antiviral states via transcriptional repression. Elife (2018) 7. doi: 10.7554/eLife.38867
76. Habano W, Miura T, Terashima J, Ozawa S. Aryl hydrocarbon receptor as a DNA methylation reader in the stress response pathway. Toxicology (2022) 470:153154. doi: 10.1016/j.tox.2022.153154
77. Mantovani N, Defelicibus A, da Silva IT, Cicero MF, Santana LC, Arnold R, et al. Latency-associated DNA methylation patterns among HIV-1 infected individuals with distinct disease progression courses or antiretroviral virologic response. Sci Rep (2021) 11(1):22993. doi: 10.1038/s41598-021-02463-0
78. Desquilbet L, Jacobson LP, Fried LP, Phair JP, Jamieson BD, Holloway M, et al. HIV-1 infection is associated with an earlier occurrence of a phenotype related to frailty. J Gerontol A Biol Sci Med Sci (2007) 62(11):1279–86. doi: 10.1093/gerona/62.11.1279
79. Cooper JM, Mann VM, Schapira AH. Analyses of mitochondrial respiratory chain function and mitochondrial DNA deletion in human skeletal muscle: effect of ageing. J Neurol Sci (1992) 113(1):91–8. doi: 10.1016/0022-510X(92)90270-U
80. Hunt M, McNiff MM, Vincent AE, Sabin C, Winston A, Payne BAI. Skeletal muscle mitochondrial dysfunction in contemporary antiretroviral therapy: a single cell analysis. AIDS (2022) 36(14):1927–34. doi: 10.1097/QAD.0000000000003334
81. Thome T, Miguez K, Willms AJ, Burke SK, Chandran V, de Souza AR, et al. Chronic aryl hydrocarbon receptor activity phenocopies smoking-induced skeletal muscle impairment. J Cachexia Sarcopenia Muscle (2022) 13(1):589–604. doi: 10.1002/jcsm.12826
82. Erlandson KM, Lake JE, Sim M, Falutz J, Prado CM, Domingues da Silva AR, et al. Bone mineral density declines twice as quickly among HIV-infected women compared with men. J Acquir Immune Defic Syndr (2018) 77(3):288–94. doi: 10.1097/QAI.0000000000001591
83. Izawa T, Arakaki R, Mori H, Tsunematsu T, Kudo Y, Tanaka E, et al. The nuclear receptor ahR controls bone homeostasis by regulating osteoclast differentiation via the RANK/c-fos signaling axis. J Immunol (2016) 197(12):4639–50. doi: 10.4049/jimmunol.1600822
84. Park R, Madhavaram S, Ji JD. The role of aryl-hydrocarbon receptor (AhR) in osteoclast differentiation and function. Cells (2020) 9(10):2294. doi: 10.3390/cells9102294
85. Watson ATD, Nordberg RC, Loboa EG, Kullman SW. Evidence for aryl hydrocarbon receptor-mediated inhibition of osteoblast differentiation in human mesenchymal stem cells. Toxicol Sci (2019) 167(1):145–56. doi: 10.1093/toxsci/kfy225
86. Kondrikov D, Elmansi A, Bragg RT, Mobley T, Barrett T, Eisa N, et al. Kynurenine inhibits autophagy and promotes senescence in aged bone marrow mesenchymal stem cells through the aryl hydrocarbon receptor pathway. Exp Gerontol (2020) 130:110805. doi: 10.1016/j.exger.2019.110805
87. Kim BJ, Hamrick MW, Yoo HJ, Lee SH, Kim SJ, Koh JM, et al. The detrimental effects of kynurenine, a tryptophan metabolite, on human bone metabolism. J Clin Endocrinol Metab (2019) 104(6):2334–42. doi: 10.1210/jc.2018-02481
88. Peng X, Zhao Z, Liu L, Bai L, Tong R, Yang H, et al. Targeting indoleamine dioxygenase and tryptophan dioxygenase in cancer immunotherapy: clinical progress and challenges. Drug Des Devel Ther (2022) 16:2639–57. doi: 10.2147/DDDT.S373780
89. Powderly JD, Klempner SJ, Naing A, Bendell J, Garrido-Laguna I, Catenacci DVT, et al. Epacadostat plus pembrolizumab and chemotherapy for advanced solid tumors: results from the phase I/II ECHO-207/KEYNOTE-723 study. Oncologist (2022) 27(11):905–e848. doi: 10.1093/oncolo/oyac174
90. Gibney GT, Hamid O, Lutzky J, Olszanski AJ, Mitchell TC, Gajewski TF, et al. Phase 1/2 study of epacadostat in combination with ipilimumab in patients with unresectable or metastatic melanoma. J Immunother Cancer (2019) 7(1):80. doi: 10.1186/s40425-019-0562-8
91. Luke JJ, Tabernero J, Joshua A, Desai J, Varga AI, Moreno V, et al. BMS-986205, an indoleamine 2, 3-dioxygenase 1 inhibitor (IDO1i), in combination with nivolumab (nivo): Updated safety across all tumor cohorts and efficacy in advanced bladder cancer (advBC. J Clin Oncol (2019) 37(7):288–94. doi: 10.1200/JCO.2019.37.7_suppl.358
92. Soliman HH, Minton SE, Han HS, Ismail-Khan R, Neuger A, Khambati F, et al. A phase I study of indoximod in patients with advanced Malignancies. Oncotarget (2016) 7(16):22928–38. doi: 10.18632/oncotarget.8216
93. Soliman HH, Jackson E, Neuger T, Dees EC, Harvey RD, Han H, et al. A first in man phase I trial of the oral immunomodulator, indoximod, combined with docetaxel in patients with metastatic solid tumors. Oncotarget (2014) 5(18):8136–46. doi: 10.18632/oncotarget.2357
Keywords: inflammation, aryl hydrocarbon receptor, IDO1, sarcopenia, osteoporosis
Citation: Sultana S, Elengickal A, Bensreti H, de Chantemèle EB, McGee-Lawrence ME and Hamrick MW (2023) The kynurenine pathway in HIV, frailty and inflammaging. Front. Immunol. 14:1244622. doi: 10.3389/fimmu.2023.1244622
Received: 22 June 2023; Accepted: 25 August 2023;
Published: 08 September 2023.
Edited by:
Andréa Cristina Paula-Lima, University of Chile, ChileReviewed by:
Matthias Clauss, Indiana University Bloomington, United StatesJosé Luis Galaz, University of Chile, Chile
Copyright © 2023 Sultana, Elengickal, Bensreti, de Chantemèle, McGee-Lawrence and Hamrick. This is an open-access article distributed under the terms of the Creative Commons Attribution License (CC BY). The use, distribution or reproduction in other forums is permitted, provided the original author(s) and the copyright owner(s) are credited and that the original publication in this journal is cited, in accordance with accepted academic practice. No use, distribution or reproduction is permitted which does not comply with these terms.
*Correspondence: Mark W. Hamrick, mhamrick@augusta.edu