- 1Hunan Key Laboratory of the Research and Development of Novel Pharmaceutical Preparation, Changsha Medical University, Changsha, China
- 2Department of Biochemistry and Molecular Biology, School of Basic Medicine, Changsha Medical University, Changsha, China
Diabetes is a significant chronic endocrine/metabolism disorder that can result in a number of life-threatening consequences. According to research, the gut microbiota is strongly linked to the development of diabetes, making it a viable target for diabetes treatment. The intestinal microbiota affects intestinal barrier function, organism immunity, and thus glucose metabolism and lipid metabolism. According to research, a disruption in the intestinal microbiota causes a decrease in short-chain fatty acids (SCFAs), alters the metabolism of bile acids (BAs), branched-chain amino acids (BCAAs), lipopolysaccharide (LPS), and endotoxin secretion, resulting in insulin resistance, chronic inflammation, and the progression to type 2 diabetes mellitus (T2DM). Astragali Radix is a medicinal herb of the same genus as food that has been extensively researched for treating diabetes mellitus with promising results in recent years. Polysaccharides, saponins, flavonoids, and other components are important. Among them, Astragaloside has a role in protecting the cellular integrity of the pancreas and liver, can leading to alleviation of insulin resistance and reducing blood glucose and triglyceride (TC) levels; The primary impact of Astragalus polysaccharides (APS) on diabetes is a decrease in insulin resistance, encouragement of islet cell proliferation, and suppression of islet β cell death; Astragali Radix flavonoids are known to enhance immunity, anti-inflammatory, regulate glucose metabolism and control the progression of diabetes. This study summarizes recent studies on Astragali Radix and its group formulations in the treatment of type 2 diabetes mellitus by modulating the intestinal microbiota.
1 Introduction
Diabetes mellitus is a set of endocrine illnesses defined by hyperglycemia, which can damage tissues and organs in the body due to a variety of factors (1). Type 2 diabetes mellitus (T2DM) is frequent in the middle-aged and elderly, and it accounts for more than 90% of all diabetes mellitus types (2). T2DM is strongly linked to the intestinal microbiota, according to research. The main aspects are mainly in the immune system, energy metabolism, intestinal screen function, intestinal barrier function (3). Numerous studies have shown that the occurrence of T2DM is closely related to the intestinal microbiota, which is increasingly recognized as a key factor in the development of T2DM. Diabetic patients have moderate gut ecological dysbiosis, with significant changes in the composition and diversity of their intestinal microbiota. In T2DM patients, the relative abundance of opportunistic pathogenic bacteria Clostridium increased whereas the relative abundance of butyrate-producing bacteria Roseburia, Faecalibacterium, and Eubacterium decreased (4). Dysbiosis of the intestinal microbiota may result in the release of endotoxins and the promotion of a chronic inflammatory response in the body, which may lead to insulin resistance and, eventually, diabetes (5). In terms of the immune system, intestinal microbiota and its metabolic derivatives, such as lipopolysaccharide (LPS) and short chain fatty acids (SCFAs), not only act directly on intestinal epithelial cells to modulate the immune system and so maintain immune homeostasis, but also activate downstream pathways via free fatty acid receptors and Toll-like receptors (TLRs), which are found throughout the body. TLRs and free fatty acid receptors are found throughout the body and have the ability to activate downstream pathways and have regulatory effects on the pancreas, insulin target organs, and other tissues (6, 7). Therefore, regulating intestinal microbiota can improve glucose metabolism levels, insulin resistance and increase insulin sensitivity, which is important for T2DM treatment.
It has been found that using Chinese herbal medicine to address intestinal microbiota abnormalities in the body is useful in the prevention and treatment of T2DM (8). Astragali Radix is a Chinese plant originated in Northern China that first appears in the Shennong Ben Cao Jing (9) and serves as one of the most commonly used single herbs in hypoglycemic herbal medicine. In traditional Chinese medicine (TCM), Astragali Radix can be paired with other herbs, such as ginseng and atractylodes, to participate in the composition of a variety of Chinese medicine compound. In China, Astragali Radix is not only commonly used in TCM, but is also known to be a tonic with beneficial effects on qi (maintains basic life activities and involved in the composition of the body) and yang (which fuels the body’s all physiological functions and metabolism). These functions are considered to be the original application of the herb in immunomodulation (10). And the important role of Astragali Radix in modulating immune function in humans and experimental animals is inextricably linked to the intestinal microflora and intestinal barrier function (3, 7). A variety of chemical substances have been isolated and identified from Astragali Radix, including saponins, flavonoids and polysaccharides. Astragali Radix has been used for thousands of years to treat diabetes, and its “thirst quenching” effect and disease treatment have been mentioned in several Chinese medical treatises. Modern medical research has shown that Astragali Radix can regulate the intestinal microbiota of T2DM patients, optimize the structure of intestinal microbiota, regulate the immune system and intestinal barrier function, and these are the possible mechanisms for its effective treatment of T2DM (11). Using intestinal microbiota as the target, we review the research on the interaction between Astragali Radix and intestinal microbiota for the treatment of T2DM, with the aim of providing ideas for the study of the mechanism between T2DM and intestinal microbiota and clinical development of herbal compound preparations and new drugs for T2DM treatment, as well as providing more safe and effective options for the T2DM treatment.
2 The relationship between intestinal microbiota and T2DM
The intestinal microbiota, can be considered as one of the human organs, is critical to human health. It has even been described as the “eighth organ” of humans. Intestinal microbiotas’ positive effects on the host include energy metabolism, gut protection, immune regulation, and brain function (12). Under normal conditions, the host and intestinal microbiota are in a mutually beneficial symbiotic relationship, maintaining a benign dynamic balance. When the host’s internal environment changes or pathogenic microorganisms invade, this balance will be disrupted, causing intestinal microbial, which can lead to a series of obesity and other metabolic diseases, inflammation, diabetes, etc (13). Changes in intestinal microbiota are linked to the progression of T2DM, and studies have shown that transplantation of fecal bacteria into germ-free mice in T2DM mice caused diabetic-like changes (14), and conversely, transplantation of fecal bacteria from normal mice In contrast, transplantation improves metabolic status in db/db mice (15). Because of alterations in the number of diverse bacteria in the intestinal microecology, the structure and distribution of intestinal microbiota in T2DM patients varies dramatically from those in normal persons. Allin et al. (16) showed that the abundance of Akkermansia muciniphila, Clostridium in decreased abundance and Streptococcus, Sutterella, Ruminococcus, Dorea, in increased abundance, with Ruminococcaceae, Lachnospiraceae showing a particularly strong negative correlation with fasting glucose and insulin levels. Everard et al. (17) showed that Bacteria which promote inflammation such as Bacteroides were more common in the feces T2DM patients. Larsen et al. (18) found that in diabetic patients The relative abundance of Clostridia, Firmicutes was significantly reduced and Betaproteobacteria was highly enriched. The ratios of Bacteroides-Prevotella/C.coccoides-E., Bacteroidetes/Firmicutes and plasma glucose concentration showed a significant positive correlation with plasma glucose concentration. Zhou et al. (19) found that the intestinal microbiota of rats changed dynamically with the progression of T2DM, with the abundance of Akkermansia muciniphila, Lactobacillu and butyric acid-producing-related bacteria Turicibacter decreasing as T2DM progressed, while the abundance of Allobaculum, Ruminococcus increased in abundance (as shown in Table 1; Figure 1). Some studies have also proposed that changes in the makeup and diversity of the intestinal microbiota may influence the severity of T2DM.
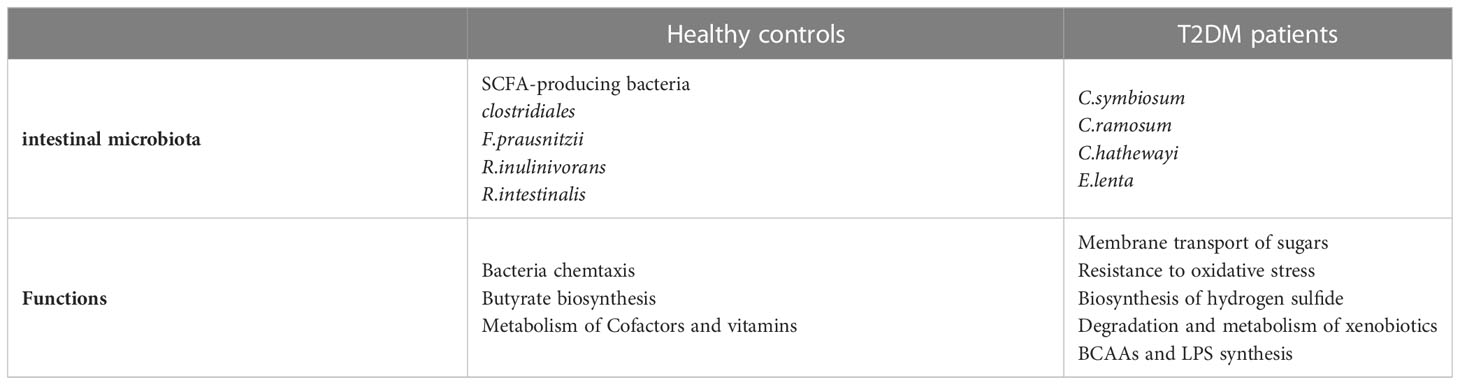
Table 1 Comparison of the intestinal microbiota and its function in patients with T2DM patients and healthy controls.
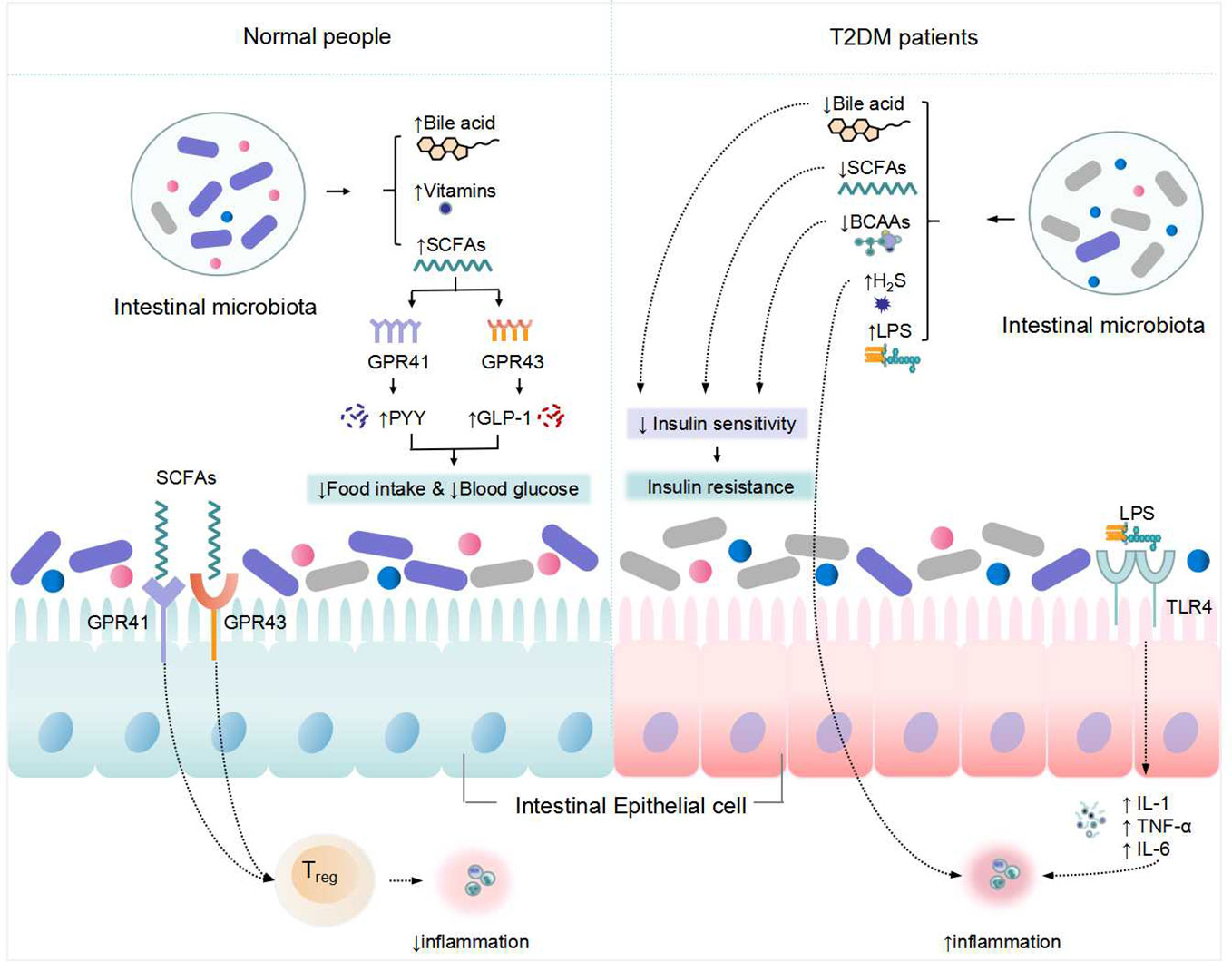
Figure 1 Changes in metabolism of the intestinal microbiota and its associated with T2DM. "↑" indicates an increase, "↓" Indicates a decrease.
2.1 The relationship between intestinal microbiota and SCFAs production
In the intestine, intestinal microbiota digest oligosaccharides, polysaccharides, peptides, proteins, and glycoproteins to create SCFAs, primarily butyric acid, propionic acid, and acetic acid. SCFAs have been proven in studies to regulate blood glucose and affect intestinal function (20). SCFAs regulate host metabolic health directly through a variety of mechanisms related to energy expenditure, appetite regulation, immune regulation, and glucose homeostasis, and its important functions are mainly in the maintenance of colonic epithelial energy supply, inhibition of pathogenic bacteria growth, regulation of the immune system and glucose and lipid metabolism (21). Bacillus, Clostridium and Bifidobacterium are the most frequent bacteria that produce SCFAs. Anaplasma is capable of producing acetate. Propionate is generated by a few dominating genera, including the basal species of mucinophilic Ackermannia, and it is also produced via the succinate route by the common and polymorphic Anaplasma Butyrate is generated by an anaerobic Clostridium species group. Butyrate, for example, has been shown to increase mitochondrial activity, prevent metabolic endotoxemia, inhibit the secretion of pro-inflammatory factors such as interleukin-6 (IL-6) and tumor necrosis factor α (TNF-α), improve insulin sensitivity, has anti-inflammatory potential, and improves intestinal barrier function (22). Acetate can also be converted to cholesterol or fatty acids, and propionate can be involved in gluconeogenesis (23). By boosting protein kinase signaling in the liver and muscle, SCFAs can improve insulin sensitivity. Gprotein-coupledreceptor-41 (GPR-41) and GPR-43 are both found in human islets, where activation of GPR43 promotes insulin secretion and activation of GPR41 acts as an inhibitor, both of which work together to maintain insulin release order and glucose homeostasis (24). SCFAs can also stimulate insulin secretion by interacting with G protein-coupled receptors that enhance Glucagon-likepeptide-1 (GLP-1) and Peptide-YY (PYY) (25). SCFAs are not only metabolites of anti-inflammatory bacteria, but they also work as immune boosters, assisting the host in fighting inflammation. Increased levels of microbial gut-derived SCFAs are regarded to be favorable to health, while gut microbial imbalance caused by intestinal inflammation and decreased SCFAs may be connected to diabetes etiology (26). intestinal microbiota disruptions may alter the synthesis and metabolism of SCFAs in vivo, resulting in decreased insulin sensitivity and disrupted insulin secretion, leading to the development of T2DM.
2.2 The correlation between the level of BCAAs and intestinal microbiota
Branched chain amino acids (BCAAs) are the basic amino acids that make up proteins, including leucine, isoleucine, and valine, and their levels in the blood alter insulin sensitivity. Horiuchi et al. (27) discovered that decreasing the protein content of mice’s food decreased insulin secretion, but increasing BCAAs in the diet restored insulin secretion and brought it back to normal levels. However, high doses of BCAAs may lead to insulin resistance. High levels of BCAAs can act as an upstream trophic signal by initiating the mTOR signal transduction pathway and inhibiting the expression of the translocator Kruppel-like factor 15 (KLF15), a key regulator of lipid, glucose, amino acid, and bile acid metabolism, resulting in increased insulin sensitivity and glycogen synthesis (28, 29). The synthesis of BCAAs in the body is intimately tied to intestinal microbiota, and the levels of BCAAs and their metabolites in the body also affect the flora (30, 31). As a result, gut flora can influence insulin sensitivity by influencing BCAAs levels, which play a vital part in the development of T2DM.
2.3 The correlation between LPS secretion and intestinal microbiota
LPS, a major component of the cell wall of Gram-negative bacteria, causes an inflammatory response (32, 33). TLRs are natural immune system receptors that play a key role in triggering an inflammatory response. TLR4 and NF-κB play critical roles in the LPS-mediated signal transduction pathway (34). TLR4 receptors detect and bind LPS, which activates the NF-κB signal transduction pathway, causing the production of proinflammatory molecules such as IL-6 and TNF-α, causing inflammation and increasing insulin resistance. Intestinal microbiota alteration can result in the production of high amounts of LPS and inflammatory chemicals, leading in chronic and persistent inflammation, structural damage, malfunction, and even apoptosis of pancreatic-cells, Consequently, insufficient insulin secretion and insulin resistance occur, leading to the development of T2DM (35).
2.4 The correlation between metabolism of BAs and intestinal microbiota
Bile acids (BAs) are an essential component of bile and the main result of cholesterol metabolic conversion in the liver. Bile acids have a vital role in intestinal absorption, transportation, and distribution of fat-soluble vitamins and lipids. It controls the network of metabolic signal transduction pathways involved in in the metabolism of glucose, lipid, drug, and energy, and facilitating lipid and fat-soluble vitamin absorption (36). Bile acid control necessitates the cooperation of the liver, the intestine, and gut bacteria. Cholesterol is processed by the liver to form primary BAs and by the action of intestinal microbiota to form secondary BAs. BAs function primarily as signaling molecules and ligands in glucose metabolism via the farne-soid X receptor (FXR) signal transduction pathway. Bile acids can activate G protein-coupled bile acid receptor 5 (TGR5) and FXR while also inhibiting gluconeogenic gene expression (37). FXR has been demonstrated to change the makeup of intestinal bacteria, boost bile acid production, and improve glucose tolerance in obese T2DM mice when the vertical trocar stomach is removed (37). TGR5 activation in intestinal L cells causes GLP-1 release, which increases insulin secretion, decreases glucagon secretion, improves liver and pancreatic function, and reduces insulin resistance and metabolic inflammation caused by a high fat diet (38). It is worth noting that the majority of the research on the aforementioned pathways has been done in rodent models. In contrast, there are differences in the composition and hydrophobicity of the bile acid metabolic pool between humans and rodents, which have a large impact on bile acid signaling properties.
2.5 Intestinal microbiota’s function in organism immunity
While boosting metabolic activities in the body, intestinal microbiota also influences host physiological functions such as immune system and intestinal epithelial cell formation, pathogen defense, and tissue homeostasis maintenance. The mucosal barrier of the intestine is a complex system combining tissue, cellular, chemical, and immunological levels. The endocrine system of the intestine and the mucosa of the intestine have a “one loss, one gain” connection. The intestinal tract is an essential component of the human immune system, intestinal inflammation is closely related to the intestinal microflora and, some researches has linked immunological problems to insulin resistance and other pathological characteristics of T2DM (39, 40). Damage to the intestinal mucosa can allow germs and their toxins to enter the body’s important tissues and organs via the bloodstream, triggering a metabolic inflammatory response. T2DM is frequently accompanied with injury to the intestinal mucosa, and the inflammatory response may also play a role in the development of T2DM (40). Intestinal immune cells are mostly found in the connective tissue of the lamina propria of the gut mucosa, which serves as a medium for communication between intestinal bacteria and the host, and are constantly impacted by intestinal microecology. The intestinal barrier normally prevents the stimulation of the immune system by pathogenic microorganisms; however, in the case of intestinal epithelial cell breakdown, abnormal tight junctions, and increased intestinal permeability, enterobacteria, viruses, and their metabolites can penetrate deeply into the lamina propria via the epithelium, stimulating adaptive immune responses and causing the release of various inflammatory factors (41). Wu et al. (42) demonstrated the most substantial effect of LPS on intestinal mucosal immunity. Endotoxemia caused changes in the ratio of the number of various lymphocytes in rat intestinal tissues. The intestinal immune system and the intestinal mucosa have a very strong connection, indicating an interdependent relationship.
The large amount of inflammatory factors released by immune disorders activates inflammatory signaling pathways in the intestinal mucosa, resulting in abnormal cell function or apoptosis and necrosis, which increases LPS and inflammatory factor leakage into the bloodstream and triggers a systemic chronic inflammatory response (43). Furthermore, intestinal immunological inflammation can affect the release of intestinal endocrine hormones such as TNF-α, which can suppress GLP-1 secretion via a receptor-dependent route, causing irregularities in glucose metabolism in the body (43).
The key cell types involved in immunological control in the immune system include Gut-associated lymphoid tissue (GALT), T-lymphocytes, B-lymphocytes, Group 3 intrinsic lymphoid cells, macrophages, and dendritic cells (41). Pattern recognition receptors (PRRs) are a class of receptors associated with the immune recognition of viruses, bacteria, fungi, or parasites and are part of the host’s innate defense system (44). LPS and multiple PAMPs activate PRRs, which sense microbes and infection factors and signal defense responses. Four classes of PRRs including transmembrane proteins, such as C-type lectin receptors (CLRs) and TLRs; cytoplasmic proteins, such as NOD-like receptors (NODs) and retinoic acid inducible gene-like receptors (RLRs) have been found (45). TLRs are the most well researched PRRs, and their recognition activates genetic programs via post-transcriptional control of transcription factors and mRNAs (46). Different microbial components of pathogens can mediate different responses of TLRs. TLRs are present in immune cells and somatic cells such as intestinal epithelial cells (47). TLR2 detects bacterial lipoproteins, whereas TLR4 recognizes bacterial LPS (as shown in Figure 2) (48). Their activation causes antigen-presenting cells to become activated, therefore combining with the autoimmune response and triggering signaling cascades in anticipation of protect against microbial invaders or heal injured tissues. Although this inflammatory reaction is necessary for eradication of infection, overactivity of TLRs can disturb immunological homeostasis, and persistent production of chemokines and pro-inflammatory cytokines may increase the likelihood of inflammation and immune disease (49, 50). Chassaing et al. (51) found that TLR5 is activated by bacterial flagellin, and mice lacking TLR5 develop colitis or metabolic syndrome, which is due to changes in the intestinal microbiota. This means that altered microflora leading to inflammatory responses can interfere with the expression of PRRs.
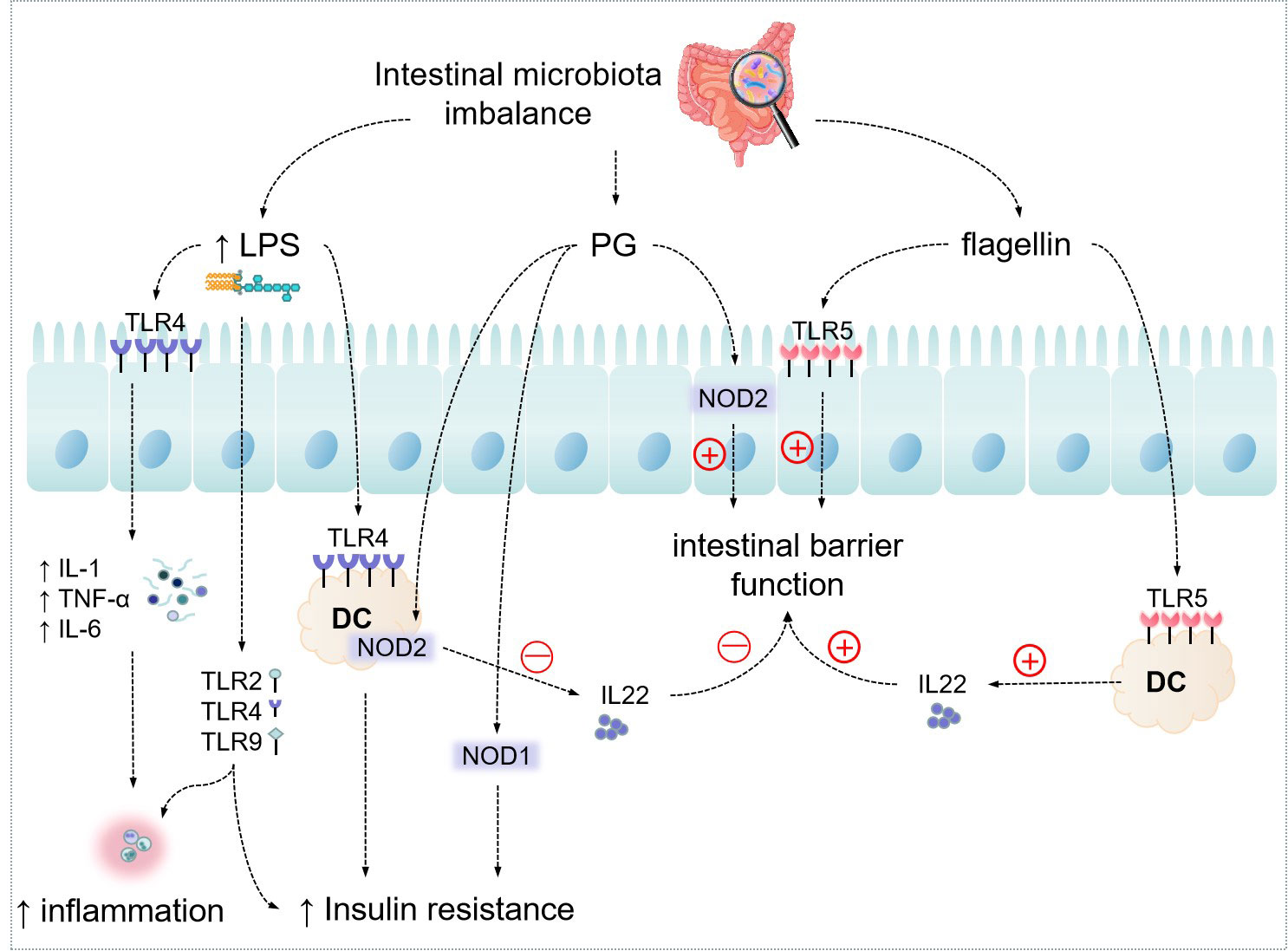
Figure 2 Effect of Intestinal microbiota on host metabolism through messenger molecules. "↑" indicates an increase.
Furthermore, multiple studies have revealed that commensal bacteria’s metabolic byproducts, such as SCFAs, influence the immunological response of gut-associated lymphoid tissues (GALTs) via epigenetic processes, operating through self-defense and the immune evasion by commensal bacteria (41).
3 Potential mechanism of Astragali Radix in the treatment of T2DM
Normal intestinal microbiota function in the body maintains the metabolic balance between microbes and the body. If this equilibrium is upset, the alteration and disorder of intestinal microbiota will result in an abnormal body metabolism in the body, including the formation of diabetes. Probiotics are living bacteria that benefit the host’s health. Prebiotics can be selectively absorbed and transformed into helpful health compounds by the host flora. Several studies have found that probiotics, prebiotics, and other microbial alteration therapies have an influence on appetite and body weight (3). The addition of probiotics to replenish reduced taxa in T2DM therapy may enhance gut metabolic activities (e.g., immunomodulation, SCFAs synthesis, and bile acid metabolism regulation) (52). Lactobacillus rhamnosus, Lactobacillus griseus, Lactobacillus casei, Lactobacillus lactis, Streptococcus thermophilus, Lactobacillus bulgaricus, and Bifidobacterium lactis have been found in studies to have anti-diabetic efficacy (53). In patients with metabolic diseases, fecal transplantation (FT) improves the sensitivity to insulin, enhances gut microbial diversity, and dramatically boosts butyric acid-producing bacteria (54, 55). Clinical trials have shown that FT can increase the sensitivity to insulin in normal person and effectively delay the decline of β-cell function and halt the progression of diabetes in diabetic patients, but the benefits are temporary and highly dependent on the individualized response of the host (56, 57). It can be demonstrated that proper intestinal microbiota modulation may regulate glucose metabolism, reduce insulin resistance, improve and stabilize blood glucose levels, and successfully cure diabetes. Astragali Radix membranaceus, a Chinese herbal medication, another aspect, has a positive impact in terms of controlling the intestinal microbiota in the body and can successfully cure T2DM in a variety of ways, including decreasing insulin resistance and lowering blood glucose.
3.1 Mechanisms of Astragali Radix in the treatment of T2DM acting on the intestinal microbiota
Diabetes mellitus is classified as Xiaokezheng in TCM, and its etiology is mostly owing to a lack of yin and fluids, as well as a predominance of dryness and heat. Diabetes mellitus is intimately linked to intestinal microbiota abnormalities in the body, according to Chinese medicine; however, intestinal microbiota disorders and spleen malfunctions are mutually causative. According to certain research, the status of normal intestinal microbiota is directly associated to the spleen (58). The ancient people believed that the spleen and stomach were the foundations of human survival, and that the spleen and stomach modified the body’s qi, blood, and vitality through eating. so the normal operation of the spleen and stomach is critical in the balance of intestinal microbiota. Bifidobacteria, Lactobacilli, and other beneficial bacteria in the intestinal tract, which can decompose food residues, metabolize them in the body in various ways, and finally transform them into essential substances to maintain body nutrition, allowing the human intestinal tract to remain balanced and healthy. Thus, managing intestinal microbiota can enhance spleen and stomach function, repair and ameliorate intestinal mucosal damage, restore intestinal wall permeability, regulate and translocate harmful bacteria in the intestinal tract, and enhancement of autoimmunity, all of which are important in combating of T2DM (59).
In recent years, many traditional Chinese herbs have been tried in combating of T2DM (60), and Astragali Radix can treat thirst primarily by “inducing clear qi to reach the lungs, harmonizing with the inhaled qi and transforming it into water, encouraging the upward movement of fluid in the stomach, and regulating the qi of the lower jiao.” Astragali Radix is thought to be a natural hypoglycemic agent with broad application potential is critical in combating of diabetes (61). Astragali Radix is a somewhat warm, sweet-tasting herb that belongs to the spleen and lung meridians and has a variety of actions include helping the body, tonifying qi and elevating yang, stimulating water retention, lowering swelling, and discharging pus and supporting sores (61). Polysaccharides, saponins, flavonoids, and other multi-class active components in Astragali Radix have strong preventative and therapeutic benefits on T2DM, including reducing insulin resistance, enhancing insulin sensitivity, antioxidant, and anti-inflammatory properties (61). By controlling intestinal microbiota abnormalities, increasing the immunological barrier function of intestinal mucosa, improving the body’s immunity, and regulating metabolites, Astragali Radix can exhibit anti-diabetic actions (61). In terms of pharmacological mechanism, Astragali Radix can increase tyrosine kinase activity, improve insulin signal transmission, and aid in combating of diabetes and its complications (61). Wang et al. (61) Astragali Radix adjusts the ratio of SCFAs-producing bacteria such as Flavonifractor_and Alloprevotella and inflammation-associated bacteria such as Alistipes and Deferribacteres. Li et al. (11) used 16S rRNA sequence determination to examine the effects of Astragali Radix on the regulation of intestinal microbiota in type 2 diabetic mice and discovered that Astragali Radix significantly improved the diversity and abundance of intestinal microbiota from diabetic mice, inducing an improving in the abundance of Bifidobacterium and Lactobacillus, thereby controlling body weight and blood glucose in type 2 diabetes mice.
3.2 Astragalus formulas in the treatment of T2DM acting on the intestinal microbiota
Astragali Radix as the main herb, together with various other herbal formulas, has also shown remarkable results in diabetes control through acting on the intestinal microbiota (as shown in Table 2). Cao et al. (62) evaluated the safety and efficacy of the new formula Qi-Jian combination (QJ) (Astragali Radix, Yang Mei, Ge Gen, and Huang Lian) in combating of type 2 diabetes by metabolomics, and showed that it can regulating blood glucose and triglyceride (TC) levels, reducing liver and kidney damage, and increasing production of SCFAs and decreasing production of BCAAs. PCoA analysis of intestinal microbiota indicated that QJ therapy significantly increased the number of Akkermansia and Bacteroidetes, reduced the number of Firmicutes (62). The system pharmacology paradigm also demonstrated that QJ worked via the PPARA, AKT1, and TP53 proteins (62). It was determined that QJ significantly reduced T2DM, and this impact was associated with changed metabolite profiles and intestinal microbiota. Xie et al. (63) showed by 16S rRNA sequence determination that the various ameliorative effects of Pi-Dan-Jian-Qing decoction (PDJQ) (Pseudostellaria heterophylla, Astragali Radix, Rhizoma atractylodes, Radix scrophulariae, Puerariae lobatae radix, Scutellaria, Coptis chinensis, Salvia miltiorrhiza, dried ginger) on T2DM, including improving hyperglycemia, hyperlipidemia and insulin resistance, improving liver and kidney function and regulating inflammatory response and oxidative stress. The mechanism of PDJQ for T2DM may be related to the improvement of intestinal microflora dysbiosis, regulation of tryptophan and histamine metabolism and citric acid cycle. The mechanisms of on T2DM are likely linked to an improvement in the dysbiosis of intestinal microbiota and modulation of tryptophan metabolism, histamine metabolism, and the TCA cycle. In addition, ELISA results showed that PDJQ decreased the levels of TNF-α, IL-1β and IL-6. PDJQ can improve the clinical symptoms of spleen deficiency and dampness-heat caused by T2DM, and can reduce both postprandial blood glucose (PBG) and fasting blood glucose (FBG) (64). The Jin-Xiao-Xiao-Ke decoction (JXXKD), first reported in Wai Tai Mi Yao Fang which was writing by Wang Tao during the Tang Dynasty (AD 752), is a traditional prescription used to treat symptoms “Xiao Ke,” which is known as T2DM in modern medicine. This prescription was used to cure “Xiao Ke” and considerably reduce symptoms like polyuria and thirst. Animal studies revealed that JXXKD dramatically reduced polyuria symptoms, lowered FBG protein levels, and improved liver fat deposition in diabetic rats (65). Guo et al. (66) mentioned in their study about the mechanism of treatment of T2DM that Drug Pair of Astragali Radix and Dioscoreae Rhizoma modulates the metabolism of BCAAs (e.g., leucine and isoleucine) and SCFAs (e.g., butyric acid and propionic acid) in vivo. low-density lipoprotein (LDL), total cholesterol (TC) and Lipid levels were reduced and high-density lipoprotein (HDL) levels were increased in T2DM rats after these treatments. In addition to this, they found that Drug Pair of Astragali Radix and Dioscoreae Rhizoma can regulate the disturbance of energy metabolism and reducing insulin resistance.
4 Mechanism of the active ingredients of Astragali Radix membranaceus in the treatment of T2DM by regulating the intestinal microbiota
Astragali Radix contains a variety of active ingredients, among which saponins and polysaccharide flavonoids have been reported most frequently, and all these types of ingredients have some therapeutic effects on T2DM, and their specific molecular mechanisms are as follows (as shown in Table 3).
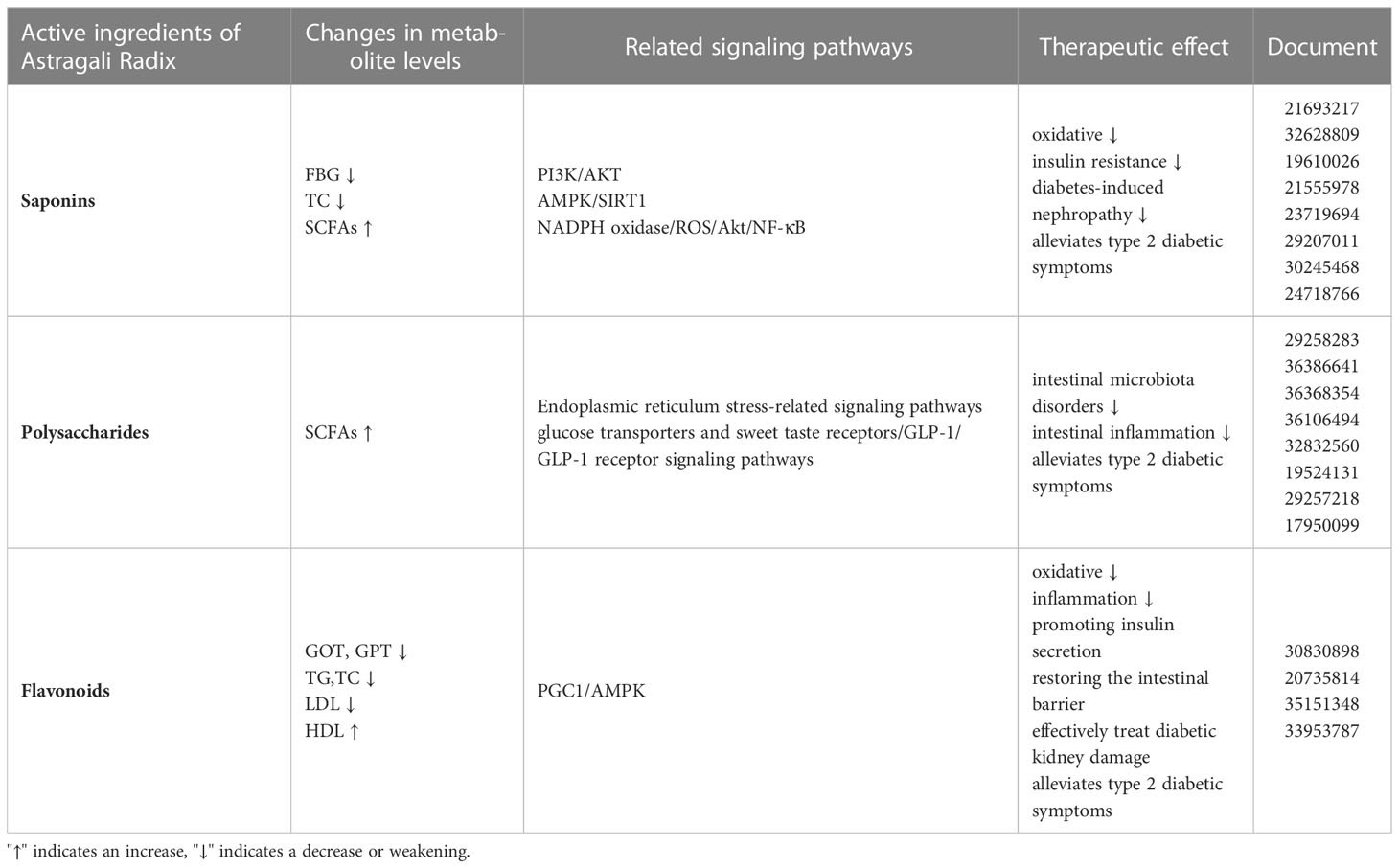
Table 3 Molecular mechanisms of the active ingredients of Astragali Radix membranaceus in the treatment of T2DM.
4.1 Saponins
Astragaloside (AS), one of the main active ingredients of Astragali Radix, which chemical nature is a kind of cycloartane-type triterpene glycoside chemical. Recent studies have shown that AS has hypoglycemic effects and may alleviate diabetic complications through several potential molecular mechanisms. Gong et al. (67) reported that AS significantly lowered blood lipid, glucose, insulin resistance, and oxidative stress levels, In T2DM rats. According to histology results, AS has a role in protecting the cellular integrity of the pancreas and liver. AS modulates the type and number of intestinal microbiota and increases butyric acid levels in diabetic mice (67). AS treatment of diabetes was found to be achieved by modulating two signaling pathways, PI3K/AKT and AMPK/SIRT1, and confirmed using insulin-resistant HepG2 cells and inhibitors (67). Cheng et al. (68) discovered that AS-IV can participate in the elevation of glucocorticoid expression in rats. Lv et al. (69) reported that the amount of glucose-6-phosphatases and glycogen phosphatases in the liver was lowered in mice with T2DM treated with AS-IV, leading to alleviation of insulin resistance and decreases in blood glucose and TC levels. He & Gong et al. (67, 70) also reported that Astragaloside IV may promoting butyric acid production by modulating the intestinal microbiota profile. Zhang et al. (71) discovered that AS-IV administration might ameliorate metabolic syndrome induced by high fructose diet in rats, resulting in lower blood pressure and improved insulin resistance. Qi et al. (72) showed that AS-IV can alleviate the Glycated albumin causes epithelial-mesenchymal transition (EMT) in the cell of Normal Rat Kidney-52E line via redox balance modulation. Similarly, He et al. (73) found that AS-IV protected rat kidney from hyperinsulinemia. The mechanisms were inhibition of TNF-α and IL-1 overproduction, reduction of oxidative stress, enhancement of transient receptor potential channel (TRPC6) expression, and inhibition of extracellular-signal-regulated kinase 1/2 (ERK1/2) activation. AS-IV reduces Diabetes-induced nephropathy by ameliorating oxidative stress and suppressing the expression of inflammatory genes driven by NF-κB in human mesangial cells (74, 75).
4.2 Polysaccharides
Like Saponins, Astragalus polysaccharides (APS) are one of the main active components of Astragali Radix. It is very closely related to intestinal microbiota. APS has a positive regulatory effect on intestinal microbiota, reduces insulin resistance and improves islet secretory cells in T2DM. It has been shown that after gavage of APS in db/db diabetic mice, the specific value of the phylum Bacteroides and Thick-walled Bacteroides in the organism was increased significantly, and the abundance of Lactobacillus and Sartorius increased, while Spirochaete mucocephalus and Treponema were growth inhibited, confirming that APS can delay glucose diffusion, treat diabetes and repair its tissue damage (76). APS not only has a positive regulatory effect on intestinal microbiota disorders, but also regulates body metabolism, improves the intestinal milieu, and plays a vital role in a wide range of properties as an example anti-inflammatory, immunomodulatory and anti-diabetic properties (77). Ming et al. (78) found that APS significantly increased serum concentrations of SCFAs, including butyric and propionic acids, by increasing the abundance of SCFA-producing genera such as Akkermansia, Coprococcus, and Oscillospira in the intestine. APS may control immune function by boosting cytokine release, promoting immune cell proliferation, and influencing immunoglobulin (Ig) production and immunological signaling (79). APS decreased oxidative stress and inflammation levels, alleviated Glucose and lipid metabolism abnormalities, and avoided organ destruction in T2DM mice, according to Chen et al. (80), the APS might preserve the intestinal barrier by reducing oxidative stress and intestinal inflammation stress, as well as inhibiting the pathogenic bacteria Shigella and promoting the development of good bacteria Allobaculum and Lactobacillus. Antibiotic test have shown that the possible glucose-lowering mechanism of APS is the restoration of the intestinal barrier by altering particular intestinal bacteria.
The pathophysiology of T2DM is mostly related to insulin resistance, islet β cell dysfunction, and insulin insufficiency. Tanase et al. (81) found that normal islet β cell actions include decreasing insulin resistance, regulating lipid metabolism, boosting insulin production, and lowering blood glucose levels. Wang et al. (82) showed through their study that APS may lower blood glucose and enhance insulin sensitivity by lowering endoplasmic reticulum stress in T2DM patients. Its method may entail decreasing ATF6 activation (caused by endoplasmic reticulum stress), reversing ATF6 translocation in cells, and inhibiting the high expression of a certain protein phosphatase. The above changes reduce the stress on the liver endoplasmic reticulum, which enhances insulin sensitivity (82). Furthermore, Wei et al. (83) found that APS can decreasing T2DM insulin resistance by maintaining or increasing miRNA-203a-3p expression, lowering the glucose-regulated protein (GRP78) expression, and modulating the endoplasmic reticulum stress-related signaling pathway protein expression. According to Seino et al. (84), APS can promotes anaerobic oxidation in muscle tissue and attenuates insulin resistance. APS has also been found in several studies to stimulate cell differentiation and induce the secretion of adiponectin, increases T2DM rat glucose transporter 4 (GLUT4) expression in adipocytes and AMPK expression in liver tissue, downregulations resistin protein and mRNA in adipocytes (85–87). Another report said that APS can improve the condition of type 2 diabetic rats by modulating the intestine-pancreatic axis and related signaling pathways (88).
Collectively, the primary impact of APS on diabetes is a decrease in insulin resistance, encouragement of islet cell proliferation, and suppression of islet β cell death. Its pharmacological impact is primarily mediated through altering the expression of associated genes and proteins (86). The growth environment of intestinal microorganisms has a high correlation with the function of the spleen and stomach. The above studies suggest that APS has various effects on anti-inflammation, promotion of ulcer tissue proliferation and repair of damaged blood vessels from the perspective of the spleen. APS plays a crucial role in the treatment and improvement of diabetes and associated disorders by regulating the microecological environment in vivo.
4.3 Flavonoids
Astragali Radix membranaceus contains flavonoids, which have been shown in recent studies to have immune-boosting, anti-inflammatory, glucose metabolism-regulating, and diabetes progression-controlling properties. According to Zhang et al. (89), a flavonoid component of Astragali Radix may effectively treat diabetic kidney damage in db/db mice by inhibiting IB and NF-B p65 and decreasing inflammatory markers in blood. Furthermore, after treatment of this component, blood glucose levels dropped in db/db obese mice, which is assumed to be connected to the anti-inflammatory impact. In mice treated with this component, serum TC levels were lowered, and glucose intolerance and insulin resistance were regulated (90). After oral administration of flavones from Astragali Radix chemistry, enriching the intestinal microbiota in STZ/high-fat diet-induced diabetic mice, flavones from Astragali Radix were shown to lower fasting blood glucose and food intake (90). In vitro experiments revealed that Astragali Radix flavonoids boosted HT22 cell survival and preserved the Normalization of the intestinal barrier function in the CaCO2 monocyte layer, a mechanism involving the PGC1/AMPK pathway (91). Flavones from Astragali Radix significantly reduced cholesterol and cholesteryl esters, TC, glutamic-oxaloacetic transaminase (GOT), glutamic-pyruvic transaminase (GPT) and LDL levels while elevating HDL levels in both in vivo and in vitro experiments. Cholesterol 7α-hydroxylase (CYP7A1) and glucose transporter 2 (GLUT2) expressions were increased significantly in the treatment of flavones from Astragali Radix, and TGR5 and FXR played key roles in regulating lipid and bile acid metabolism (92).
5 Summary and prospect
In China, TCM has been widely used in the clinical treatment of “Xiaoke” for a long time. The reason why TCM acts directly on the intestinal microbiota is inextricably linked to its oral administration. The intestinal microbiota transforms and promotes the absorption of TCM components, while at the same time, TCM can alter intestinal microbiota abundance and numbers and influence its metabolites (93). This article reviews the relationship between intestinal microorganisms and the occurrence and progression of T2DM, as well as how Astragali Radix and Astragalus formulas can be utilized to effectively treat T2DM. Astragali Radix, as a medicinal and food herb, can positively regulate the intestinal microorganisms, promotes probiotic colonization, inhibit the growth of pathogenic microorganisms, influence the differentiation and apoptosis of intestinal cells, thereby improve the intestinal barrier function and immune function.
As found by Liu et al. (76), APS altered the intestinal microbiota, such as increasing the number of Bacillus thuringiensis and Lactobacillus lactis, which improved cognitive performance of diabetic mice. By influencing the proliferation of intestinal bacteria, Astragali Radix can affect metabolites and their activities such as SCAAs, BCAAs, LPS, BAs, and others. These metabolite concentrations, which are strongly connected to intestinal barrier function, blood glucose and lipid levels, insulin sensitivity, and inflammatory levels, may account for the role of Astragali Radix in a range of metabolic diseases, including diabetes (11). The polysaccharides, flavonoids and saponins in Astragali Radix, all of which act on the intestinal microbiota, are now recognized as a possible mechanism for the treatment of diabetes mellitus. Changes in the populations of many pathogenic microorganisms are a major trigger for diabetes mellitus (62). Intestinal probiotics can regulate the metabolism of herbal ingredients and other substances in the body by acting on intestinal mucin to maintain normal intestinal barrier function. It may alleviate T2DM by regulating microbial metabolism, alleviating insulin resistance, maintaining intestinal barrier function, and reducing oxidative stress, among other mechanisms. Previous studies can help us to recognize the history and current research status of Astragali Radix in the treatment of diabetes, and lay the foundation for the future clinical application of Chinese medicine in the treatment of diabetes.
However, the intestinal microbiota is a dynamically changing and complex population and herbal medicines may bring adverse effects (94, 95). Therefore, in-depth research is needed in the study of herbal medicines acting on the gut flora to modulate the immune system for the treatment of metabolic diseases. Especially considering the individual variability, cum the effect of differences in the composition of gut microbiota and immunity in different people (96). Our research on similar signaling processes in different bacteria should continue to expand with a view to establishing standardized therapies using relevant signaling pathways or targeting molecules and better applying herbal therapeutic techniques in clinical practice.
Author contributions
MS and ML conceived the paper. MS and ML analyzed the relevance of the literature and wrote the article. TT, WT, YL, and LW revised the figures and reviewed the article. All authors contributed to the article and approved the submitted version.
Funding
This study was supported by Hunan Provincial Education Department Scientific Research Project: Hunan Education Bulletin (2019) No.353-19C0196.
Conflict of interest
The authors declare that the research was conducted in the absence of any commercial or financial relationships that could be construed as a potential conflict of interest.
Publisher’s note
All claims expressed in this article are solely those of the authors and do not necessarily represent those of their affiliated organizations, or those of the publisher, the editors and the reviewers. Any product that may be evaluated in this article, or claim that may be made by its manufacturer, is not guaranteed or endorsed by the publisher.
References
1. Mi WS, Xia Y, Bian YH. Meta-analysis of the association between aldose reductase gene (CA)n microsatellite variants and risk of diabetic retinopathy. Exp Ther Med (2019) 18:4499–509. doi: 10.3892/etm.2019.8086
2. Ren WK, Xia YY, Chen SY, Wu GY, Bazer FW, Zhou BY, et al. Glutamine metabolism in macrophages: A novel target for obesity/type 2 diabetes. Adv Nutr (2019) 10(2):321–30. doi: 10.1093/advances/nmy084
3. Krummenauer MC, Bock MP, Leivas G, Martins FA, Ramalho R, Teló HG, et al. IDF21-0087 Metabolic interventions for diabetes treatment are associated with gut microbiota changes:a systematic review. Diabetes Res Clin Pract (2022) 186(S1):109460. doi: 10.1016/J.DIABRES.2022.109460
4. Anhê FF, Barra NG, Schertzer JD. Glucose alters the symbiotic relationships between gut microbiota and host physiology. Am J Physiol Endocrinol Metab (2020) 318(2):E111–6. doi: 10.1152/ajpendo.00485.2019
5. Nima M, Shabnam R, Zahra H, Mohammadreza K, Shahin B, Ali K, et al. Human gut microbiota and its possible relationship with obesity and diabetes. Int J Diabetes Develop Countries (2020) 41:235–43. doi: 10.1007/s13410-020-00881-w
6. Hamilton MK, Boudry G, Lemay DG, Raybould HE. Changes in intestinal barrier function and gut microbiota in high-fat diet-fed rats are dynamic and region dependent. Am J Physiol - Gastrointestinal Liver Physiol (2015) 308(10):G840–51. doi: 10.1152/ajpgi.00029.2015
7. Gong J, Hu M, Huang Z, Fang K, Wang DK, Chen QJ, et al. Berberine attenuates intestinal mucosal barrier dysfunction in type 2 diabetic rats. Front Pharmacol (2017) 8:42. doi: 10.3389/fphar.2017.00042
8. Su M, Hu R, Tang T, Tang WW, Huang CX. Review of the correlation between Chinese medicine and intestinal microbiota on the efficacy of diabetes mellitus. Front Endocrinol (2022) 13:1085092. doi: 10.3389/fendo.2022.1085092
9. Peppas S, Sagris M, Bikakis I, Giannopoulos S, Tzoumas A, Kokkinidis DG, et al. A systematic review and meta-analysis on the efficacy and safety of direct oral anticoagulants in patients with peripheral artery disease. Ann Vasc Surg (2021) 80:1–11. doi: 10.1016/j.avsg.2021.07.028
10. Cho WCS, Leung KN. In vitroand in vivo immunomodulating and immunorestorative effects of Astragalus membranaceus. J Ethnopharmacol (2007) 113(1):132–41. doi: 10.1016/j.jep.2007.05.020
11. Li XY, Shen L, Ji HF. Astragalus alters gut-microbiota composition in type 2 diabetes mice: clues to its pharmacology. Diabetes Metab Syndrome Obes (2019) 12:771–8. doi: 10.2147/DMSO.S203239
12. Chen T, Zhang YQ, Zhang YY, Shan CJ, Zhang YY, Fang K, et al. Relationships between gut microbiota, plasma glucose and gestational diabetes mellitus (GDM). J Diabetes Invest (2020) 12(4):641–50. doi: 10.1111/jdi.13373
13. Salgaço MK, Oliveira LGS, Costa GN, Bianchi F, Sivieri K. Relationship between gut microbiota, probiotics, and type 2 diabetes mellitus. Appl Microbiol Biotechnol (2019) 103(23-24):9229–38. doi: 10.1007/s00253-019-10156-y
14. Yu F, Han W, Zhan GF, Li S, Jiang XH, Wang L, et al. Abnormal gut microbiota composition contributes to the development of type 2 diabetes mellitus in db/db mice. Aging (2019) 11:10454–67. doi: 10.18632/aging.102469
15. Zhang PP, Li LL, Han X, Li QW, Zhang XH, Liu JJ, et al. Fecal microbiota transplantation improves metabolism and gut microbiome composition in db/db mice. Acta Pharmacol Sin (2020) 41:678–85. doi: 10.1038/s41401-019-0330-9
16. Allin KH, Tremaroli V, Caesar R, Jensen BAH, Damgaard MTF, Bahl MI, et al. Aberrant intestinal microbiota in individuals with prediabetes. Diabetologia (2018) 61(4):810–20. doi: 10.1007/s00125-018-4550-1
17. Everard A, Cani PD. Diabetes, obesity and gut microbiota. Best Pract Res Clin Gastroenterol (2013) 27(1):73–83. doi: 10.1016/j.bpg.2013.03.007
18. Larsen N, Vogensen FK, Van Den Berg FW, Nielsen DS, Andreasen AS, Pedersen BK, et al. Gut microbiota in human adults with type 2 diabetes differs from non-diabetic adults. PloS One (2010) 5(2):e9085. doi: 10.1371/journal.pone.0009085
19. Zhou W, Xu H, Zhan L, Lu XG, Zhang LJ. Dynamic Development of fecal microbiome during the progression of diabetes mellitus in zucker diabetic fatty rats. Front Microbiol (2019) 10:232. doi: 10.3389/fmicb.2019.00232
20. Blaak EE, Canfora EE, Theis S, Frost G, Groen AK, Mithieux G, et al. Short chain fatty acids in human gut and metabolic health. Benef Microbes (2020) 11:411–55. doi: 10.3920/BM2020.0057
21. Hajar AS, Hafiz ARM, Razid SS, Muhialdin BJ, Olusegun L, Saari N. Enzymatically synthesised fructooligosaccharides from sugarcane syrup modulate the composition and short-chain fatty acid production of the human intestinal microbiota. Food Res Int (2021) 149:110677. doi: 10.1016/J.FOODRES.2021.110677
22. Alamri E, Bayomy H, Mohammedsaledh Z. The role of gut microbiome in obesity: a systematic review. Proc Nutr Soc (2022) 81(OCE1):E33–3. doi: 10.1017/S0029665122000337
23. Saad MJA, Santos A, Prada PO. Linking gut microbiota and inflammation to obesity and insulin resistance. Physiology (2016) 31(4):283–93. doi: 10.1152/physiol.00041.2015
24. Yang YY, Shi LX, Li JH, Yao LY, Xiang DX. Piperazine ferulate ameliorates the development of diabetic nephropathy by regulating endothelial nitric oxide synthase. Mol Med Rep (2019) 19:2245–53. doi: 10.3892/mmr.2019.9875
25. Sun Y, Huang YC, Ye FH, Liu WW, Jin XH, Lin KX, et al. Effects of probiotics on glycemic control and intestinal dominant flora in patients with type 2 diabetes mellitus:A protocol for systematic review and meta-analysis. Medicine (2020) 99(46):e23039. doi: 10.1097/MD.0000000000023039
26. Ma QT, Li YQ, Wang JK, Li PF, Duan YH, Dai HY, et al. Investigation of gut microbiome changes in type 1 diabetic mellitus rats based on highthroughput sequencing. BioMed Pharmacother (2020) 124:109873. doi: 10.1016/j.biopha.2020.109873
27. Horiuchi M, Takeda T, Takanashi H, Ozaki MY, Taguchi Y, Toyoshima Y, et al. Branched-chain amino acid supplementation restores reduced insulinotropic activity of a lowprotein diet through the vagus nerve in rats. Nutr Metab (2017) 14:59. doi: 10.1186/s12986-017-0215-1
28. Biswas D, Duffley L, Pulinilkunnil T. Role of branched-chain amino acid-catabolizing enzymes in intertissue signaling,metabolic remodeling, and energy homeostasis. FASEB J (2019) 33:8711–31. doi: 10.1096/fj.201802842RR
29. Liu YX, Dong WB, Shao J, Wang YB, Zhou MY, Sun HP. Branched-chain amino acid negatively regulates KLF15 expression via PI3K-AKT pathway. Front Physiol (2017) 8:853. doi: 10.3389/fphys.2017.00853
30. Han W, Li MH, Yang MY, Chen S, Lu YY, Tang TT, et al. Dietary folic acid supplementation inhibits highFat dietInduced body weight gain through gut microbiota-associated branched-chain amino acids and mitochondria in mice. J Nutr Sci vitaminol (2023) 69(2):105–20. doi: 10.3177/JNSV.69.105
31. Genton L, Pruijm M, Teta D, Bassi I, Cani PD, Gaïa N, et al. Gut barrier and microbiota changes with glycine and branched-chain amino acid supplementation in chronic haemodialysis patients. J cachexia sarcopenia Muscle (2021) 12:1527–39. doi: 10.1002/JCSM.12781
32. Picarello DM. Investigating how the LPS structure affects the immune response of gram-negative bacterial outer membranes. Biophys J (2022) 121(3S1):487a–a. doi: 10.1016/J.BPJ.2021.11.344
33. Tang L, Liu L, Li GY, Jiang PC, Wang Y, Li JM. Expression profiles of long noncoding RNAs in intranasal LPS-mediated alzheimer’s disease model in mice. BioMed Res Int (2019) 2019:9642589. doi: 10.1155/2019/9642589
34. He W, Rebello1 O, Savino R, Terracciano R, Schuster KC, Guardiola B, et al. TLR4 triggered complex inflammation in human pancreatic islets. Biochim Biophys Acta (BBA) - Mol Basis Dis (2019) 1865(1):86–97. doi: 10.1016/j.bbadis.2018.09.030
35. George L, Ramasamy T, Sirajudeen K, Manickam V. LPS-induced apoptosis is partially mediated by hydrogen sulphide in RAW 264.7 murine macrophages. Immunol investigations (2019) 48:451–65. doi: 10.1080/08820139.2019.1566355
36. Yang QY, Ouyang J, Sun FJ, Yang JD. Short-chain fatty acids: a soldier fighting against inflammation and protecting from tumorigenesis in people with diabetes. Front Immunol (2020) 11:590685(2020). doi: 10.3389/fimmu.2020.590685
37. Bozadjieva N, Heppner KM, Seeley RJ. Targeting FXR and FGF19 to treat metabolic diseases-lessons learned from bariatric surgery. Diabetes (2018) 67(9):1720–8. doi: 10.2337/dbi17-0007
38. Han L, Li T, Du M, Chang R, Zhan B, Mao X. BeneficialEffectsofPotentilla discolor bunge water extracton inflammatory cytokines releaseand gut microbiotain high-fat dietand streptozotocin induced type2 diabetic mice. Nutrients (2019) 11(3):670. doi: 10.3390/nu11030670
39. Kumar DP, Rajagopal S, Mahavadi S, Mirshahi F, Grider JR, Murthy KS, et al. Activation of transmembrane bile acid receptor TGR5 stimulates insulin secretion in pancreatic β cells. Biochem Biophys Res Commun (2012) 427(3):600–5. doi: 10.1016/j.bbrc.2012.09.104
40. Liu J, Qiao B, Deng N, Wu Y, Li DD, Tan ZJ. The diarrheal mechanism of mice with a high fat diet in a fatigued state is associated with intestinal mucosa microbiota. 3 Biotech (2023) 13(3):77. doi: 10.1007/s13205-023-03491-5
41. Martens EC, Neumann M, Desai MS. Interactions of commensal and pathogenic microorganisms with the intestinal mucosal barrier. Nat Rev Microbiol (2018) 16(8):457–70. doi: 10.1038/s41579-018-0036-x
42. Wu XX, Huang XL, Chen RR, Li T, Ye HJ, Xie W, et al. Paeoniflorin prevents intestinal barrier disruption and inhibits lipopolysaccharide (LPS)-induced inflammation in caco-2 cell monolayers. Inflammation (2019) 42(6):2215–25. doi: 10.1007/s10753-019-01085-z
43. Zhang Z, Wu X, Cao S, Wang L, Wang D, Yang H, et al. Caffeic acid ameliorates colitis in association with increased Akkermansia population in the gut microbiota of mice. Oncotarget (2016) 7(22):31790–9. doi: 10.18632/oncotarget.9306
44. Jiao YH, Wu L, Huntington ND, Zhang X. Crosstalk between gut microbiota and innate immunity and its implication in autoimmune diseases. Front Immunol (2020) 11:282. doi: 10.3389/fimmu.2020.00282
45. Spencer SP, Fragiadakis GK, Sonnenburg JL. Pursuing human-relevant gut microbiota-immune interactions. Immunity (2019) 51(2):225–39. doi: 10.1016/j.immuni.2019.08.002
46. Li D, Wu M. Pattern recognition receptors in health and diseases. Signal Transduct Targeted Ther (2021) 6(1):291. doi: 10.1038/s41392-021-00687-0
47. Kubelkova K, Macela A. Innate immune recognition: an issue more complex than expected. Front Cell Infection Microbiol (2019) 9:241. doi: 10.3389/fcimb.2019.00241
48. Fitzgerald KA, Kagan JC. Toll-like receptors and the control of immunity. Cell (2020) 180(6):1044–66. doi: 10.1016/j.cell.2020.02.041
49. Mukherjee S, Karmakar S, Babu SPS. TLR2 and TLR4 mediated host immune responses in major infectious diseases: a review. Braz J Infect Dis (2016) 20(2):193–204. doi: 10.1016/j.bjid.2015.10.011
50. Huang JZ, Guan BB, Lin LJ, Wang YP. Improvement of intestinal barrier function, gut microbiota, and metabolic endotoxemia in type 2 diabetes rats by curcumin. Bioengineered (2021) 12(2):11947–58. doi: 10.1080/21655979.2021.2009322
51. Chassaing B, Ley RE, Gewirtz AT. Intestinal epithelial cell toll-like receptor 5 regulates the intestinal microbiota to prevent low-grade inflammation and metabolic syndrome in mice. Gastroenterology (2014) 147(6):1363–77. doi: 10.1053/j.gastro.2014.08.033
52. Wang GQ, Liu J, Xia YJ, Ai LZ. Probiotics-based interventions for diabetes mellitus:a review. Food Bioscience (2021) 43:101172. doi: 10.1016/j.fbio.2021.101172
53. Singh J, Pooja, Kumar P, Singh J, Dhanda S. Impact of probiotics in alleviating type 2 diabetes risk in clinical trials: A meta-analysis study. Hum Gene (2023) 35:201149. doi: 10.1016/J.HUMGEN.2023.201149
54. Tiderencel KA, Hutcheon DA, Ziegler J. Probiotics for the treatment of type 2 diabetes:a review of randomized controlled trials. Diabetes/Metabo Res Rev (2020) 36(1):e3213. doi: 10.1002/dmrr.3213
55. Wu FF, Guo XF, Zhang JC, Zhang M, Ou ZH, Peng YZ. Phascolarctobacterium faecium abundant colonization in human gastrointestinal tract. Exp Ther Med (2017) 14:3122–6. doi: 10.3892/etm.2017.4878
56. Bastos RMC, SimplícioFilho A, SávioSilva C, Oliveira LFV, Cruz GNF, Sousa EH, et al. Fecal microbiota transplant in a pre-clinical model of type 2 diabetes mellitus, obesity and diabetic kidney disease. Int J Mol Sci (2022) 23(7):3842–2. doi: 10.3390/IJMS23073842
57. Wang H, Lu Y, Yan Y, Tian SS, Zheng DJ, Leng DJ, et al. Promising treatment for type 2 diabetes:fecal microbiota transplantation reverses insulin resistance and impaired islets. Front Cell Infection Microbiol (2019) 9:455(5). doi: 10.3389/fcimb.2019.00455
58. Ganesan K, Chung SK, Vanamala J, Xu BJ. Causal relationship between diet-induced gut microbiota changes and diabetes: a novel strategy to transplant Faecalibacterium prausnitzii in preventing diabetes. Int J Mol Sci (2018) 19(12):3720. doi: 10.3390/ijms19123720
59. Gong J, Chen G, Wang DK, Lu FE. On relation between diabetes and intestinal flora from theory of pi-wei. Chin J integrated traditional Western Med (2016) 36(4):484–7.
60. Song QB, Cheng SW, Li D, Cheng HY, Lai YS, Han QB, et al. Gut microbiota mediated hypoglycemic effect of Astragalus membranaceus polysaccharides in db/db mice. Front Pharmacol (2022) 13:1043527. doi: 10.3389/FPHAR.2022.1043527
61. Wang L, Dong XL, Qin XM, Yu Z. LiInvestigating the inter-individual variability of Astragali Radix against cisplatin-induced liver injury via 16S rRNA gene sequencing and LC/MS-based metabolomics. Phytomedicine (2022) 101:154107. doi: 10.1016/j.phymed.2022.154107
62. Gao K, Yang R, Zhang J, Zhiyong W, Caixia J, Feilong Z, et al. Effects of Qijian mixture on type 2 diabetes assessed by metabonomics, gut microbiota and network pharmacology. Pharmacol Res (2018) 130:93–109. doi: 10.1016/j.phrs.2018.01.011
63. Xie XH, Liao JB, Ai YL, Gao JM, Zhao J, Qu F, et al. Pi-dan-jian-qing decoction ameliorates type 2 diabetes mellitus through regulating the gut microbiota and serum metabolism. Front Cell Infection Microbiol (2021) 11:748872. doi: 10.3389/FCIMB.2021.748872
64. Xie XH, Zhao J, Chen YQ, Wenv WB. Efficacy of Pidan Jianqing decoction in treatment of type 2 diabetes with spleen deficiency and damp-heat syndrome. TMR Pharmacol Res (2021) 1(4):23. doi: 10.53388/TMRPR20211009023
65. Lin M, Wang L, Wan LH, Xu ZD, Li Y, Cao LY, et al. The antidiabetic effect and mechanism of jinXiaoXiaoKe decoction in type 2 diabetic goto–kakizaki rats. Clin Complement Med Pharmacol (2023) 3(1):100049. doi: 10.1016/j.ccmp.2022.100049
66. Guo Q, Niu WL, Li XJ, Guo HR, Zhang Na, Wang XF, et al. Study on hypoglycemic effect of the drug pair of astragalus radix and dioscoreae rhizoma in T2DM rats by network pharmacology and metabonomics. Molecules (2019) 24(22):4050. doi: 10.3390/molecules24224050
67. Gong P, Xiao XY, Wang S, Shi F, Liu N, Chen XF, et al. Hypoglycemic effect of astragaloside IV via modulating gut microbiota and regulating AMPK/SIRT1 and PI3K/AKT pathway. J Ethnopharmacol (2021) 281:114558–8. doi: 10.1016/J.JEP.2021.114558
68. Cheng MX, Chen ZZ, Cai YL, Liu CA, Tu B. Astragaloside IV protects against ischemia reperfusion in a murine model of orthotopic liver transplantation. Transplant Proc (2011) 43(5):1456–61. doi: 10.1016/j.transproceed.2011.02.066
69. Lv L, Wu SY, Wang GF, Zhang JJ, Pang JX, Liu ZQ, et al. Effect of Astragaloside IV on hepatic glucose-regulating enzymes in diabetic mice induced by a high-fat diet and streptozotocin. Phytotherapy Res (2010) 24(2):219–24. doi: 10.1002/ptr.2915
70. He QL, Han CP, Huang L, Yang HJ, Hu JC, Chen HX, et al. Astragaloside IV alleviates mouse slow transit constipation by modulating gut microbiota profile and promoting butyric acid generation. J Cell Mol Med (2020) 24(16):9349–61. doi: 10.1111/jcmm.15586
71. Zhang N, Wang XH, Mao SL, Zhao F. Astragaloside IV improves metabolic syndrome and endothelium dysfunction in fructose-fed rats. Molecules (2011) 16(5):3896–907. doi: 10.3390/molecules16053896
72. Qi WW, Niu JY, Qin QJ, Qiao ZD, Gu Y. Astragaloside IV attenuates glycated albumin-induced epithelial-to-mesenchymal transition by inhibiting oxidative stress in renal proximal tubular cells. Cell Stress Chaperones (2014) 19(1):105–14. doi: 10.1007/s12192-013-0438-7
73. He KQ, Li WZ, Chai XQ, Yin YY, Jiang Y, Li WP. Astragaloside IV prevents kidney injury caused by iatrogenic hyperinsulinemia in a streptozotocin-induced diabetic rat model. Int J Mol Med (2018) 41(2):1078–88. doi: 10.3892/ijmm.2017.3265
74. Chen QQ, Su Y, Ju YH, Ma KK, Li WZ, Li WP. Astragalosides IV protected the renal tubular epithelial cells from free fatty acids-induced injury by reducing oxidative stress and apoptosis. Biomed Pharmacother (2018) 108:679–86. doi: 10.1016/j.biopha.2018.09.049
75. Sun L, Li WP, Li WZ, Xiong L, Li GP, Ma R. Astragaloside IV prevents damage to human mesangial cells through the inhibition of the NADPH oxidase/ROS/Akt/N-κB pathway under high glucose conditions. Int J Mol Med (2014) 34(1):167–76. doi: 10.3892/ijmm.2014.1741
76. Liu Y, Liu W, Li J, Tang S, Wang MJ, Huang WH, et al. A polysaccharide extracted from Astragalus membranaceus residue improves cognitive dysfunction by altering gut microbiota in diabetic mice. Carbohydr Polymers (2019) 205:500–12. doi: 10.1016/j.carbpol.2018.10.041
77. Huang YC, Tsay HJ, Lu MK, Lin CH, Yeh CW, Liu HK, et al. Astragalus membranaceus-polysaccharides ameliorates obesity, hepatic steatosis, neuroinflammation and cognition impairment without affecting amyloid deposition in metabolically stressed APPswe/PS1dE9 mice. Int J Mol Sci (2017) 18(12):2746. doi: 10.3390/ijms18122746
78. Ming K, Zhuang S, Ma N, Nan S, Li QH, Ding MX, et al. Astragalus polysaccharides alleviates lipopolysaccharides-induced inflammatory lung injury by altering intestinal microbiota in mice. Front Microbiol (2022) 13:1033875. doi: 10.3389/fmicb.2022.1033875
79. An EK, Zhang W, Kwak M, Lee PCW, Jin JO. Polysaccharides from Astragalus membranaceus elicit T cell immunity by activation of human peripheral blood dendritic cells. Int J Biol Macromol (2022) 223:370–7. doi: 10.1016/j.ijbiomac.2022.11.048
80. Chen XX, Chen C, Fu X. Hypoglycemic effect of the polysaccharides from Astragalus membranaceus on type 2 diabetic mice based on the “gut microbiota-mucosal barrier”. Food Funct (2022) 13:10121–33. doi: 10.1039/D2FO02300H
81. Tanase DM, Gosav EM, Costea CF, Ciocoiu M, Lacatusu CM, Maranduca MA, et al. The intricate relationship between type 2 diabetes mellitus (T2DM), insulin resistance (IR), and nonalcoholic fatty liver disease (NAFLD). Diabetes Res (2020) 2020. doi: 10.1155/2020/3920196
82. Wang N, Zhang D, Mao X, Zou F, Jin H, Ouyang J. Astragalus polysaccharides decreased the expression of PTP1B through relieving ER stress induced activation of ATF6 in a rat model of type 2 diabetes. Mol And Cell Endocrinol (2009) 307(1-2):89–98. doi: 10.1016/j.mce.2009.03.001
83. Wei ZT, Weng SY, Wang L, Mao ZJ. Mechanism of Astragalus polysaccharides in attenuating insulin resistance in Rats with type 2 diabetes mellitus via the regulation of liver microRNA-203a-3p. Mol Med Rep (2018) 17:1617–24. doi: 10.3892/mmr.2017.8084
84. Seino Y, Hirose H, Saito I, Itoh H. High molecular weight multimer form of adiponectin as a useful marker to evaluate insulin resistance and metabolic syndrome in Japanese men. Metabolism (2007) 56(11):1493–9. doi: 10.1016/j.metabol.2007.06.015
85. Zhang RX, Qin XZ, Zhang T, Qian L, Zhang JX, Zhao JX. Astragalus polysaccharide improves insulin sensitivity via AMPK activation in 3T3-L1 adipocytes. Molecules (2018) 23(10):2711. doi: 10.3390/molecules23102711
86. Bin Ke, Ke X, Wan XS, Yang YB, Huang YJ, Jian Q. Astragalus polysaccharides attenuates TNF-α-induced insulin resistance via suppression of miR-721 and activation of PPAR-γ and PI3K/AKT in 3T3-L1 adipocytes. Am J Trans Res (2017) 9(5):2195–206.
87. Liu M, Wu K, Mao XQ, Wu Y, Ouyang JP. Astragalus polysaccharide improves insulin sensitivity in KKAy mice: Regulation of PKB/GLUT4 signaling in skeletal muscle. J Ethnopharmacol (2010) 127(1):32–7. doi: 10.1016/j.jep.2009.09.055
88. Yang ZM, Wang Y, Chen SY. Astragalus polysaccharide alleviates type 2 diabetic rats by reversing the glucose transporters and sweet taste receptors/GLP-1/GLP-1 receptor signaling pathways in the intestine-pancreatic axis. J Funct Foods (2021) 76:104310. doi: 10.1016/j.jff.2020.104310
89. Zhang YY, Tan RZ, Zhang XQ, Yu Y, Yu C. Calycosin ameliorates diabetes-induced renal inflammation via the NF-κB pathway in vitro and in vivo. Med Sci Monitor (2019) 25:1671–8. doi: 10.12659/MSM.915242
90. Hoo RL, Wong JY, Qiao C, Xu A, Xu HX, Lam SL. The effective fraction isolated from Radix Astragali alleviates glucose intolerance, insulin resistance and hypertriglyceridemia in db/db diabetic mice through its anti-inflammatory activity. Nutr Metab (2010) 7:1–12. doi: 10.1186/1743-7075-7-67
91. Li XL, Zhao TT, Gu JL, Wang Z, Lin J, Wang RS, et al. Intake of flavonoids from Astragalus membranaceus ameliorated brain impairment in diabetic mice via modulating brain-gut axis. Chin Med (2022) 17(1):22. doi: 10.1186/s13020-022-00578-8
92. Wang Z, Li XL, Hong KF, Zhao TT, Dong RX, Wang WM, et al. Total flavonoids of astragalus ameliorated bile acid metabolism dysfunction in diabetes mellitus. Phytomed Chin Med (2021) 2021:1–9. doi: 10.1155/2021/6675567
93. Wang JK, Ma QT, Li YQ, Li PF, Wang M, Wang TS, et al. Research progress on traditional Chinese medicine syndromes of diabetes mellitus. Biomedicine Pharmacotherapy (2020) 121:109565. doi: 10.1016/j.biopha.2019.109565
94. Li XY, Zhu JY, Wu Y, Tan ZJ. Correlation between kidney function and intestinal biological characteristics of adenine and folium sennaeInduced diarrhea model in mice. Turkish J Gastroenterol (2023) 34(1):4–14. doi: 10.5152/tjg.2022.211010
95. Hurley JM. Could gut flora cycles be key to treating diabetes? Sci Trans Med (2020) 12(553):eabd3618. doi: 10.1126/scitranslmed.abd3618
Keywords: Astragali Radix, active ingredients, type 2 diabetes mellitus, intestinal microbiota, immunity, metabolism
Citation: Su M, Tang T, Tang W, Long Y, Wang L and Liu M (2023) Astragalus improves intestinal barrier function and immunity by acting on intestinal microbiota to treat T2DM: a research review. Front. Immunol. 14:1243834. doi: 10.3389/fimmu.2023.1243834
Received: 21 June 2023; Accepted: 24 July 2023;
Published: 10 August 2023.
Edited by:
Zhoujin Tan, Hunan University of Chinese Medicine, ChinaReviewed by:
Xuejuan Liang, Hunan Academy of Chinese Medicine, ChinaJun Xu, Hunan Institute of Microbiology, China
Copyright © 2023 Su, Tang, Tang, Long, Wang and Liu. This is an open-access article distributed under the terms of the Creative Commons Attribution License (CC BY). The use, distribution or reproduction in other forums is permitted, provided the original author(s) and the copyright owner(s) are credited and that the original publication in this journal is cited, in accordance with accepted academic practice. No use, distribution or reproduction is permitted which does not comply with these terms.
*Correspondence: Meiling Liu, NDIxODc3NzNAcXEuY29t