- 1Department of Microbiology, Biochemistry and Immunology, Morehouse School of Medicine, Atlanta, GA, United States
- 2National Center for Emerging Zoonotic and Infectious Diseases, Center for Disease Control and Prevention (CDC), Atlanta, GA, United States
Chlamydia abortus (Cab) causes spontaneous abortion and neonatal mortality in infected ruminants and pregnant women. Most Cab infections are asymptomatic, although they can be treated with antibiotics, signifying that control of these infections may require alternative strategies, including the use of effective vaccines. However, the limitations imposed by live attenuated and inactivated vaccines further suggest that employment of subunit vaccines may need to be considered. The efficacy of a newly generated Vibrio cholerae ghost (rVCG)-based subunit vaccine harboring the N-terminal portion of the Cab Pmp18D protein (rVCG-Pmp18.3) in preventing Cab-induced abortion or neonatal mortality was evaluated in pregnant mice. Mice were intranasally (IN) immunized and boosted twice, 2 weeks apart with the vaccine, and immunized and unimmunized mice were caged with males 4 weeks postimmunization. The mice were then infected either IN or transcervically (TC) 10 days after pregnancy, and the fertility rate was determined 7 days postpartum. Eight days after delivery, the mice were sacrificed, and Cab infectivity in the lungs and spleens was evaluated by culturing tissue homogenates in tissue culture. Our results demonstrated that the vaccine induced immune effectors that mediated complete clearance of infection in the lungs and significantly reduced Cab infectivity in the spleen following IN immunization. Vaccine immunization also afforded protection against Cab-induced upper genital tract pathology (uterine dilation). Furthermore, while there was no incidence of abortion in both immunized and unimmunized mice, immunized mice were completely protected against neonatal mortality compared to unimmunized infected controls, which lost a significant percentage of their litter 7 days postpartum. Our results establish the capability of the rVCG-Pmp18.3 vaccine to prevent infection in the lungs (mucosal) and spleen (systemic) and protect mice from Cab-induced tubal pathologies and neonatal mortality, a hallmark of Cab infection in ruminants. To advance the commercial potential of this vaccine, future studies will optimize the antigen dose and the number of vaccine doses required for protection of ruminants.
Introduction
The obligate intracellular Gram-negative bacterium, Chlamydia abortus, has affinity for the placenta of both animals and humans resulting in tissue damage, inflammation, preterm labor, still birth, and late-term spontaneous abortion (1, 2). Often, infected animals are asymptomatic until late pregnancy, and in sheep, abortion usually occurs approximately 2–3 weeks before delivery (3). Pregnant women may experience flu-like symptoms, which makes it difficult to diagnose without additional background knowledge of the patients’ contact with farm animals, leading to delayed antibiotic treatment, which may result in fetal death (4–6). However, while antibiotics are effective against C. abortus infection, most infections are asymptomatic, and so infected animals remain untreated. Thus, the use of an effective vaccine is necessary to control infection and limit its zoonotic potential.
While the current live attenuated vaccines derived from Cab strain 1B were thought to prevent infection, they have been shown to contribute to abortion storms in naive flocks, raising questions regarding their attenuation (7). In addition to being hazardous to produce, the vaccines are costly and difficult to produce in large amounts. Furthermore, during implementation of these vaccines, vaccination practices cannot easily be monitored, as it is difficult to distinguish infected from vaccinated animals (DIVA) using current serodiagnostic methods (8), which is possible with subunit vaccines (9). Inactivated whole organism-based vaccines have also played a role in managing disease in sheep in Europe, but their use is limited due to lack of protection against post-parturition bacterial shedding (10). These limitations motivated the increased efforts to develop safer efficacious vaccines capable of reducing chlamydial shedding and disease-associated reproductive failures, such as subunit vaccines. Despite the above challenges, no commercial C. abortus subunit vaccine is currently available.
Among the potential C. abortus proteins predicted and/or tested as vaccine antigens include the polymorphic membrane proteins (Pmps) that are like the type V autotransporter secretion system (11, 12). In addition to playing a role in chlamydial pathogenesis, the Pmps are expressed during all stages of the chlamydial life cycle and have been reported to contain immunogenic epitopes (13), stimulate innate immune responses (14), and therefore potential vaccine targets. We previously reported that a subunit vaccine candidate consisting of the N-terminal region of the polymorphic membrane protein (Pmp) 18D and the VCG delivery platform protected mice from C. abortus genital infection (15). However, this study did not evaluate protection against infertility, abortion, or neonatal death following challenge. We have redesigned this subunit vaccine to comprise T- and B-cell epitopes of the N-terminal region of the C. abortus Pmp18D and designated it Pmp18.3. In this study, we evaluated the efficacy of the newly generated subunit vaccine antigen expressed in Vibrio cholerae ghosts (rVCG-Pmp18.3) in preventing Cab-induced abortion or neonatal mortality in pregnant mice. Our results demonstrated that the vaccine completely protected mice from Cab-induced neonatal mortality and upper genital tract pathology compared to unimmunized infected control mice, which lost a significant percentage of their litter 7 days postpartum. Furthermore, the induced immune effectors mediated complete clearance of infection in the lungs and significantly reduced Cab infectivity in the spleen in comparison with unimmunized infected control mice.
Materials and methods
Ethics statement
This study was carried out in strict accordance with the recommendations in the Guide for the Care and Use of Laboratory Animals of the National Institute of Health. The Institutional Animal Care and Use Committee (IACUC) of Morehouse School of Medicine (MSM) approved the study protocol (Protocol Number 19-09). Six-week-old female C57BL6/J mice (stock number 000664) (The Jackson Laboratory, Bar Harbor, ME) were used in this study. Mice were allowed to acclimate for 3 days in the MSM Center for Laboratory Animal Resources (CLAR) facility prior to experimentation. All immunizations and challenge were performed under isoflurane anesthesia.
Chlamydia stocks and antigens
Stock preparations of C. abortus AB7 organisms used in this study were generously provided by Dr. Teresa Garcia-Seco (VISAVET, Universidad Complutense de Madrid, Spain) and were titrated on Buffalo Green Monkey Kidney (BGMK) cell monolayers, and chlamydial elementary bodies (EBs) were purified by renografin density gradient centrifugation (Virusys Corporation, Randallstown, MD). C. abortus (Cab) antigen was prepared by exposing purified EBs to UV irradiation for 3 h and kept at −80°C pending use.
Production of the rVCG-Pmp18.3 vaccine
The rVCG-Pmp18.3 vaccine candidate was generated by genetically inactivating V. cholerae cells harboring plasmid pPmp18.3 as described previously (16). Briefly, V. cholerae O1 strain V912 cells co-harboring plasmid pPmp18.3 coding for Pmp18.3 and the lysis plasmid pDKLO1 were cultured to mid-log phase in Brain Heart Infusion broth at 37°C, and rVCG-Pmp18.3 was generated by gene E-mediated lysis. At the end of lysis, harvested cells (rVCG preparations) were washed in a low ionic buffer before being lyophilized and stored at room temperature or refrigerated until use. In previous studies, rVCG preparations stored at room temperature maintained the stability of expressed antigens and the protective efficacy of vaccine formulations (15, 17–19).
Immunization, challenge, and analysis of protection against Cab-induced abortion and neonatal mortality
Mice (10/group) were immunized intranasally (IN) with 1.5 mg of lyophilized rVCG-Pmp18.3 in 15 µl of PBS on days 0, 14, and 28 or were unimmunized (naive controls). Four weeks after the last immunization, immunized and unimmunized (nave) mice were mated with proven breeder males and weighed every 2 days for weight changes. Mice were also observed for vaginal plugs. If no plug was observed, any mouse that gained > 1.5 g over a 2-day period was considered pregnant. On day 10 of pregnancy, mice were challenged IN with 1 × 107 or TC with 1 × 106 IFUs of Cab strain AB7 in 5 ml of sucrose phosphate glutamic acid (SPG) buffer. Naive uninfected pregnant mice served as controls. Mice were observed for incidence of abortion, but not disturbed following challenge. Following delivery, the number of pups was recorded, and the mothers and pups were monitored and subsequently sacrificed on day 8. Unimmunized mice infected IN or TC between days 10 and 13 of pregnancy and similarly monitored served as infection only control group, while naive unimmunized mice mated with proven breeder males and monitored as above served as unimmunized controls.
Determination of antigen-specific mucosal and systemic humoral immune responses
Serum and vaginal wash samples were collected from immunized and naive mice at 2 and 4 weeks postimmunization and kept frozen at −80°C until analyzed. The magnitude of Cab-specific IgG2c and IgA antibodies in the samples was quantified using a previously described ELISA technique (20). Briefly, 96-well plates (Costar) were coated in triplicate overnight with either 10 μg/ml of Cab antigen or IgA or IgG2c standards (0.0, 12.5, 25, 50. 100, 250, 500, and 1,000 ng/ml) to generate a standard calibration curve in 100 ml of PBS at 4°C. The results, which were simultaneously generated with the standard curve, show data as mean concentrations (ng/ml) ± SD and denote the mean of triplicates values for each assay.
Determination of serum antibody avidity index
To evaluate the functionality of the anti-Cab IgG and IgG2c antibodies in serum samples obtained 2 and 4 weeks postimmunization, the avidity, or functional affinity, the total binding strength of the interaction between an antibody and antigen (21, 22) was evaluated using a modification of a previously described antibody ELISA assay (23) and the chaotropic agent, ammonium thiocyanate (NH4SCN). Thus, 96-well plates were coated overnight at 4°C with 10 μg/ml of Cab antigen. After washing with PBS containing 0.05% Tween 20 (PBS-T), non-specific binding sites were blocked by incubation with blocking buffer (PBS containing 5% goat serum and 1% BSA) for 1 h and then incubated with serum diluted 1:20 in blocking buffer at 37°C for 2 h. Following another wash, the wells were treated with 1M NH4SCN for 20 min at 37°C and incubated with Horseradish peroxidase (HRP)-conjugated goat anti-mouse IgG or IgG2c antibody (Southern Biotechnology, Birmingham, AL) for 1 h at 37°C. Plates were developed with the peroxidase substrate, 2,2'-azino-bis (3-ethylbenzthiazoline-6-sulfonic acid) (ABTS), and the absorbance was read at 450 nm. The relative avidity index was calculated by dividing the mean antibody concentration of samples in the presence of NH4SCN by the mean antibody concentration of samples in its absence. The relative avidity index was assessed based on the following criterion: values >50% indicate high avidity, between 30% and 49% indicate intermediate avidity, and those below 29% signify low avidity (24).
Determination of C. abortus burden in mouse spleen
Eight days after parturition, spleens were harvested, weighed, homogenized using the gentleMACs Dissociator (Miltenyi Biotech, Gaithersburg, MD), and processed following the protocol described previously (25). Briefly, homogenates were diluted in PBS containing 2 mg/ml of DEAE dextran and centrifuged at 300×g for 10 min at 4°C. Approximately 25 µl of the supernatant diluted 1:1,000 in PBS DEAE dextran was added in triplicate to McCoy cell monolayers in a 96-well plate and incubated at 37°C for 2 h followed by 30 min of centrifugation at 1,400×g. The samples were replaced with 200 µl of complete Eagle’s Minimum Essential Medium (EMEM) supplemented with 10% FBS, 0.4% glucose, 0.01% sodium pyruvate, 0.1% yeast extract, 50 mg/ml of gentamicin, 2.5 mg/ml of amphotericin B, and 200 nM of glutamine, and incubated at 37°C, 5% CO2. After 48 h, the cells were fixed with 95% ethanol and stained with Remel™ PathoDx™ Chlamydia Culture Confirmation Kit (Thermo Fisher Scientific (Waltham, MA).
Determination of C. abortus burden in mouse lungs
The lungs and livers harvested from immunized and control mice 8 days post-parturition were also weighed, and the lung tissues were homogenized using the gentleMACs Dissociator. Following centrifugation at 1,900×g for 30 min at 4°C, samples were vortexed for 30 s in 15-ml Falcon tubes containing glass beads, and supernatants (0.5 ml) were centrifuged at 2,000 rpm for 10 min at 4°C. Supernatants were diluted 1:1,000 in complete Iscove’s Modified Dulbecco’s Medium (IMDM) supplemented with 10% FBS and cycloheximide, and 200-µl aliquots were added in triplicates to confluent McCoy cell monolayers in 96-well plates. After centrifugation at 3,000 rpm for 1 h at 4°C and incubation for 2 h at 37°C in 5% CO2, the samples were replaced with 200 µl of fresh IMDM/cycloheximide and incubated further. After 48 h, the cells were fixed with 95% ethanol and stained with Remel™ PathoDx™ Chlamydia Culture Confirmation Kit (Thermo Fisher Scientific (Waltham, MA).
Evaluation of protection against C. abortus-induced upper genital tract pathology following IN or TC challenge infection
Eight days postparturition, all study mice, including immunized and unimmunized mice challenged IN or TC, were euthanized, and the intact genital tracts were excised and examined in situ for gross pathological lesions.
Results
Induction of C. abortus-specific mucosal and systemic humoral immune responses
C. abortus-specific antibody responses elicited in serum samples and vaginal secretions collected at weeks 2 and 4 post-immunization were analyzed by an antibody ELISA assay. IN immunization with rVCG-Pmp18.3 elicited significantly higher (p <0.001) concentrations of Cab-specific IgG2c and IgA antibodies in both serum (Figures 1A, C) and vaginal secretions (Figures 1B, D) in comparison with unimmunized control mice. In addition, significantly higher (p< 0.05) amounts of IgG2c (Figures 1A, B) were observed in both serum and vaginal secretions compared to amounts of IgA (Figures 1C, D). The results indicate that in line with previous findings, IN immunization with rVCG-Pmp18.3 induces antigen-specific antibodies in both systemic and mucosal tissues, with IgG2c levels being consistently higher than IgA.
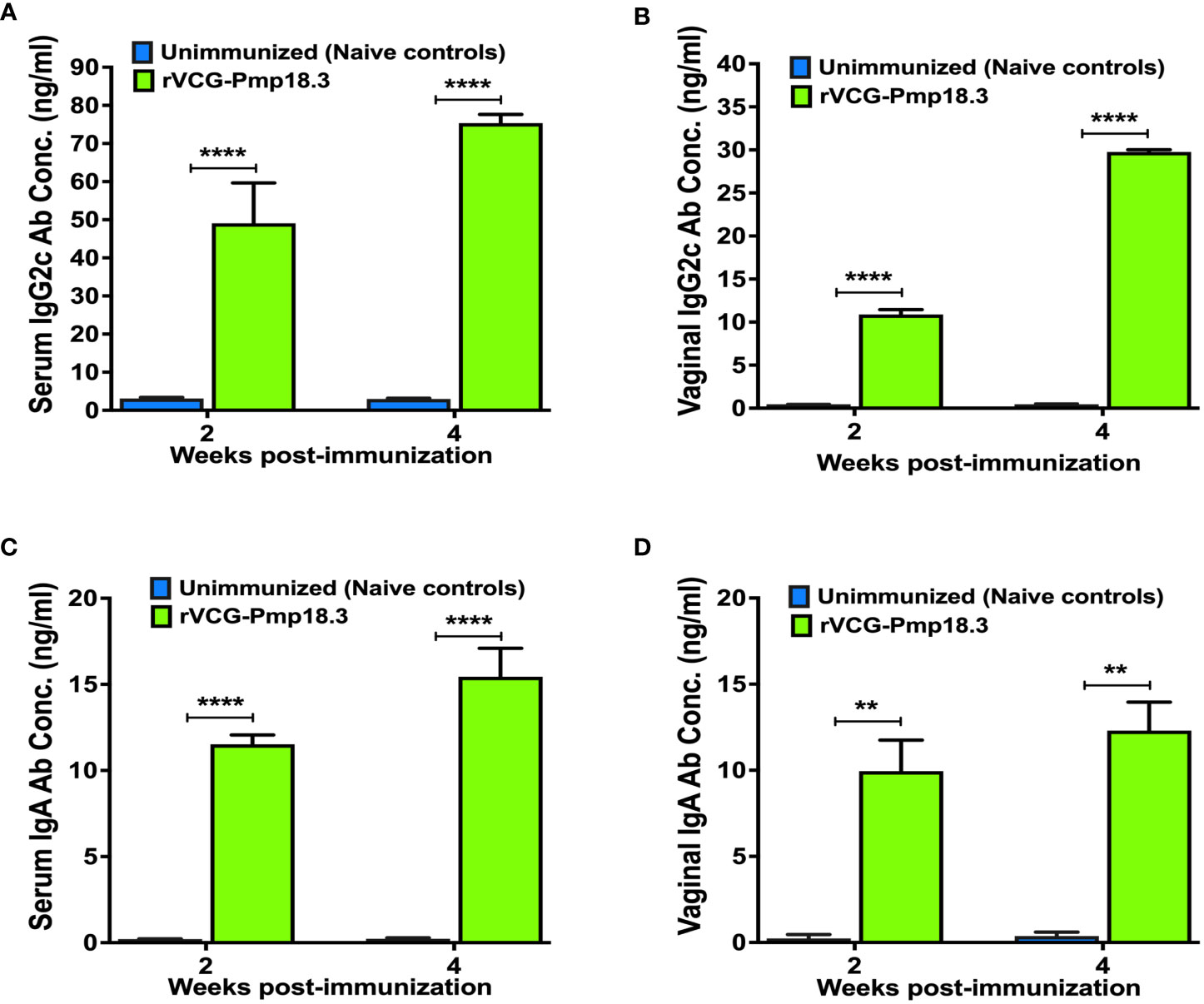
Figure 1 Chlamydia abortus-specific antibody responses elicited following IN immunization. Groups of mice were immunized intranasally, three times, 2 weeks apart. Unimmunized (naive) mice were included as controls. Serum and vaginal wash samples were obtained 2 and 4 weeks after the last immunization and pooled for each group. An antibody ELISA was used to determine the concentration of IgG2c and IgA in serum (A, C) and vaginal wash (B, D). Results were generated simultaneously with a standard curve and display data sets corresponding to absorbance values as mean concentrations (ng/ml) ± SD of triplicate cultures for each experiment. The results are from two independent experiments with similar results, and the data shown are from one of the experiments. Significant differences between groups were determined by one-way ANOVA with Tukey’s post-multiple comparison test at p** <0.01 and p**** < 0.0001.
IN immunization with rVCG-Pmp18.3 induced antigen-specific serum antibodies with high avidity
We hypothesized that the antigen-specific antibodies elicited in serum following immunization with rVCG-Pmp18.3 will have functional activity, indicated by high antigen binding capacity. Thus, the functionality of the Cab-specific IgG and IgG2c antibodies elicited in serum was established by assessing their relative avidity index using a modified antibody ELISA assay in combination with NH4SCN. The results presented in Figure 2 show that while the relative avidity index values of serum IgG antibodies were moderate (Figure 2A), those of the Th1-associated IgG2c antibodies were high (>60%) 2- and 4-weeks post-immunization and increased with time; approximately 80% 4 weeks post-immunization (Figure 2B). On the other hand, the relative avidity index values of serum IgG and IgG2c antibodies from unimmunized control were at baseline levels at both timepoints (Figures 2A, B). The results indicate that the serum Th1-associated IgG2c antibodies show higher functionality compared to total IgG antibodies.
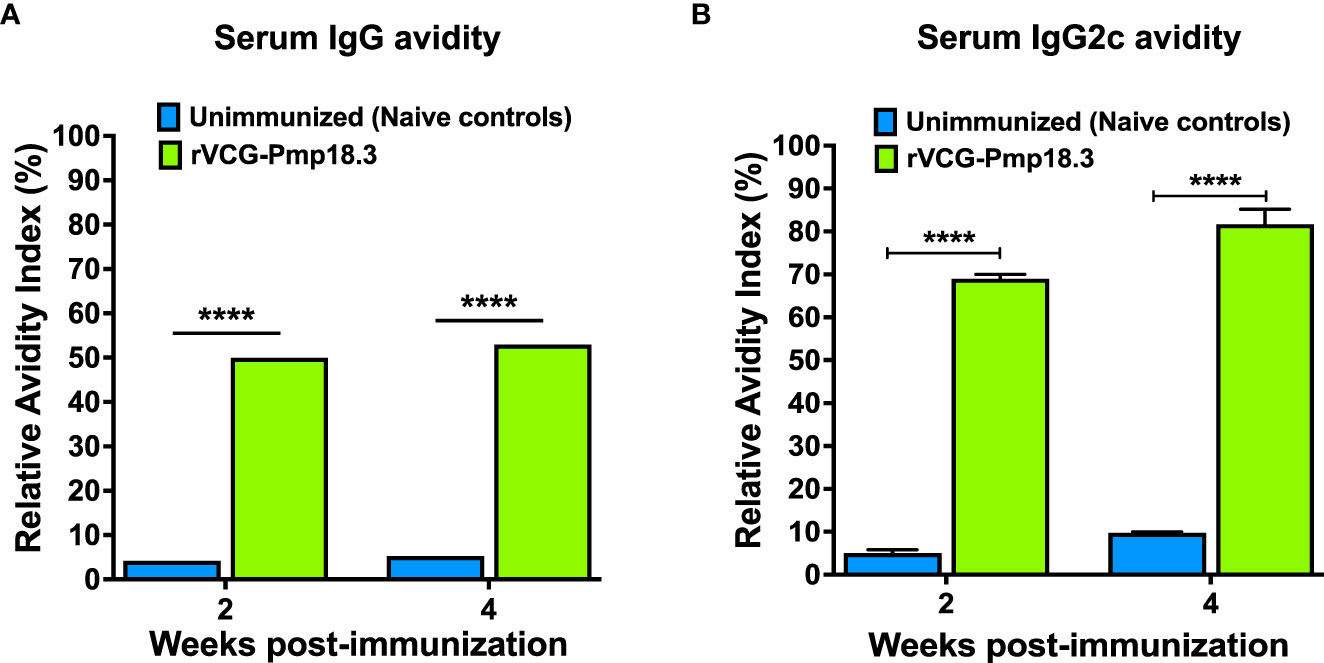
Figure 2 Relative Avidity Index of Chlamydia-specific serum IgG and IgG2c antibodies. Serum samples collected 2- and 4-weeks post-immunization were pooled, and the Relative Avidity was determined using a modified antibody ELISA assay in combination with the chaotropic agent, ammonium thiocyanate (NH4SCN). Samples diluted 1:20 were plated in triplicate, and the percent Relative Avidity Index (RAI) was calculated by dividing the concentration of treated samples by the concentration of untreated samples multiplied by 100. The data show the RAI values of serum IgG (A) and IgG2c (B) antibodies. Differences in the relative avidity between VCG-Pmp18.3 vaccine-immunized and unimmunized mice were compared by paired Student’s t-test at p**** <0.0001.
IN immunization with rVCG-Pmp18.3 confers protection against infection in mucosal and systemic tissues following respiratory challenge
To assess vaccine efficacy against infection in mucosal and systemic tissues, rVCG-Pmp18.3- immunized and unimmunized mice were challenged intranasally with live C. abortus at mid pregnancy (day 10), and the bacterial burden in harvested spleens and lungs was determined 8 days postpartum. A comparison of organ weights between immunized and unimmunized mice challenged IN or TC revealed no significant differences (p >0.05) in the mean weights of the organs, irrespective of challenge route (Figure 3A). In addition, rVCG-Pmp18.3 vaccine-immunized mice completely cleared infection in the lungs (mucosal tissue) and had significantly (p <0.0001) reduced C. abortus burden in the spleen (systemic tissue) compared to the naive control mice, which showed high levels of C. abortus IFUs at this time point following IN challenge (Figure 3B). As expected, C. abortus was not isolated from the splenic and lung tissues of the naive uninfected control mice.
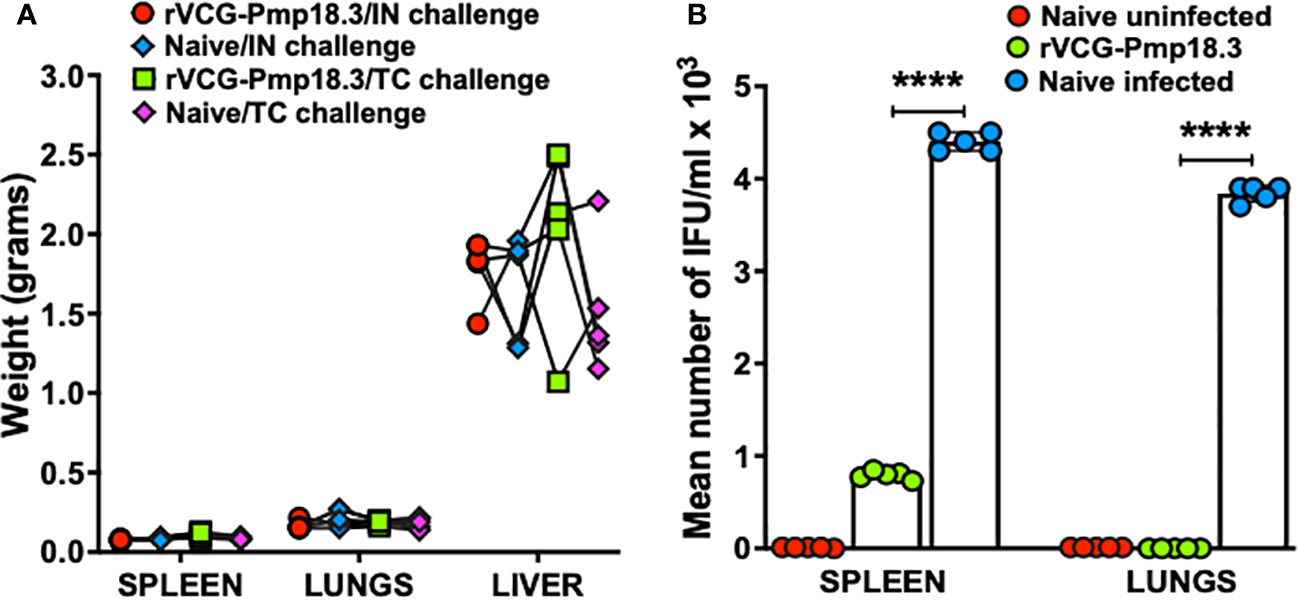
Figure 3 Protection against C. abortus infection in mucosal and systemic tissues following respiratory challenge. Groups of mice (n=5) immunized IN as described above were challenged intranasally with 1×106 IFUs of live C. abortus AB7 at mid-pregnancy. Eight days after parturition, the harvested spleens, lungs, and livers were weighed prior to homogenization, and the mean weights (grams) per group was calculated. The homogenized spleen (systemic) and lung (mucosal) tissues were inoculated on McCoy cell monolayers, and the number of IFUs recovered from each mouse was enumerated. The data show the organ weights of (A) and the recoverable IFUs from each mouse (B) per group. The horizontal bars indicate the mean recoverable IFUs from each experimental group. Differences in the recoverable IFUs between the rVCG-Pmp18.3 vaccine immunized, and live C. abortus-infected control mice were compared by paired Student’s t-test at p < 0.05.
IN immunization with rVCG-Pmp18.3 protects against neonatal mortality following challenge with C. abortus AB7
To determine vaccine efficacy against spontaneous abortion or neonatal death, rVCG-Pmp18.3 vaccine-immunized and unimmunized mice were challenged intranasally or transcervically with live C. abortus strain AB7 on day 10 of pregnancy and observed for spontaneous abortion. In addition, the fertility rates and pup survival were evaluated and compared with the naive uninfected group. The results show that neither the pregnant immunized nor the naive control mice aborted following IN infection. Figure 4 shows the fertility rate (mean number of pups per litter) for each group on the day of delivery (day 1) and on day 8 following IN infection. The fertility rates of the rVCG-Pmp18.3 vaccine-immunized (7.6), naive infected (7.2), and naive uninfected control (8.2) mice were comparable on the day of delivery. However, 8 days after delivery, the fertility rate in the naive infected group (1.0) was significantly reduced with only two of six mice still having surviving pups compared to the vaccine-immunized and naive uninfected controls in which all six mice had surviving pups. The total number of pups per group on days 1 and 8 and the percent pup survival following IN infection of pregnant mice are shown in Table 1. While the vaccine-immunized mice retained a high percentage of pup survival (100%) like the naive uninfected controls (93%), only 14% of the pups in the naive Cab-infected mice survived following IN challenge. The results indicate that immunization with the rVCG-Pmp18.3 vaccine confers protection against neonatal mortality in mice following IN challenge infection.
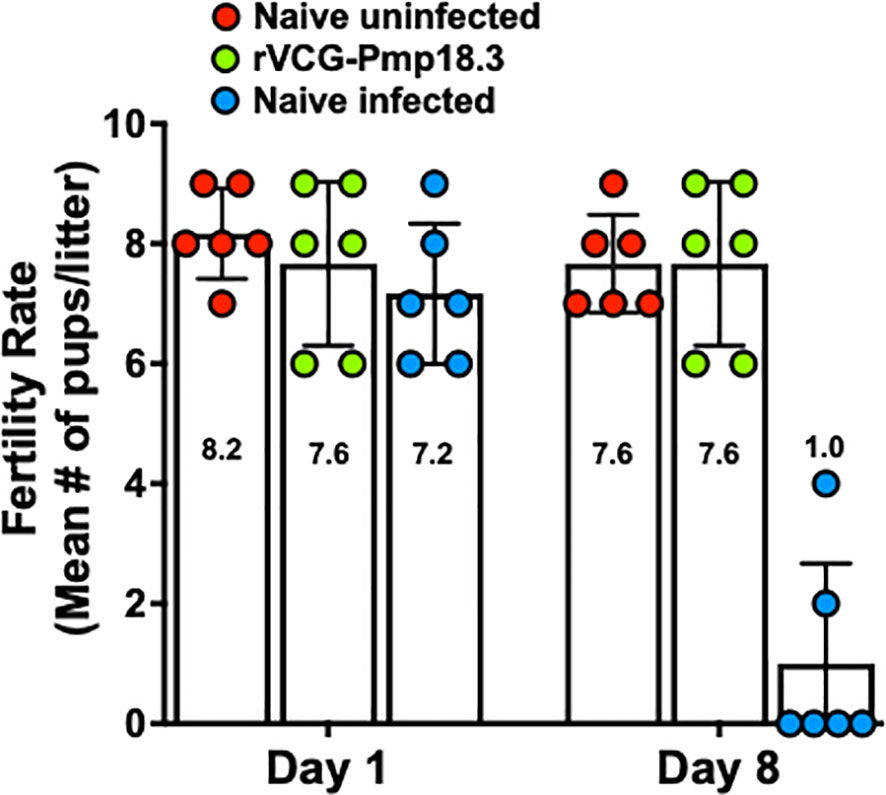
Figure 4 Protection against C. abortus-induced neonatal mortality by rVCG-Pmp18.3 vaccine following IN challenge with C. abortus AB7. Groups of mice (6/group) immunized IN with rVCG-Pmp18.3 vaccine as described above were mated with proven breeder males 3 weeks post-immunization and challenged IN with 1×106 IFUs of live C. abortus on day 10 of pregnancy. Unimmunized infected and uninfected mice served as positive and negative controls, respectively. Each symbol represents a mouse in each group. The plotted data show the mean number of pups per mouse in each group on the day of birth (day 1) (fertility rate) and at the end of the observation period (day 8) post-parturition. Numbers within each bar represent the mean number of pups per liter for each experimental group. One-way ANOVA with Tukey’s post-multiple comparison test was used to determine significant differences between groups.
We further investigated if challenge route affects the protective ability of the vaccine against neonatal death. Thus, IN immunized and unimmunized mice were challenged transcervically with live C. abortus, and the fertility rate and pup survival were evaluated as described above and compared with naive uninfected mice. Figure 5 shows the fertility rate for each group of mice on the day of delivery (day 1) and on day 8 (the end of the observation period) following TC infection. Like the IN challenged mice, none of the pregnant immunized and naive control mice aborted following TC infection. The results also showed that the fertility rate of the vaccine-immunized/TC challenged (7.0) and the naive uninfected control (8.6) mice did not differ significantly on the day of delivery. However, like the findings with the IN challenged mice, the fertility rate in the naive/TC infected group 8 days after delivery was significantly reduced from 5.5 to 2.8 (Figure 5). Table 2 shows the total number of pups per group on days 1 and 8 and the percent pup survival following TC infection of pregnant mice. The data show that whereas 53.8% of the pups in the naive TC infected mice survived, both the vaccine immunized/TC infected and naive uninfected control mice had 100% pup survival. The results indicate that route of infection has no impact on the protective efficacy of the rVCG-Pmp18.3 vaccine against neonatal mortality in mice following IN immunization. Furthermore, one of the non-immunized TC infected control mice revealed an incidence of dystocia (difficult or obstructed labor). The mouse was able to give birth to one single pup before labor was delayed due to improper positioning of the pups. Unfortunately, the single pup did not survive through the night. The mouse was observed for 24 h before being euthanized to prevent further distress, and six additional pups were found in the vaginal canal (Figure 6).
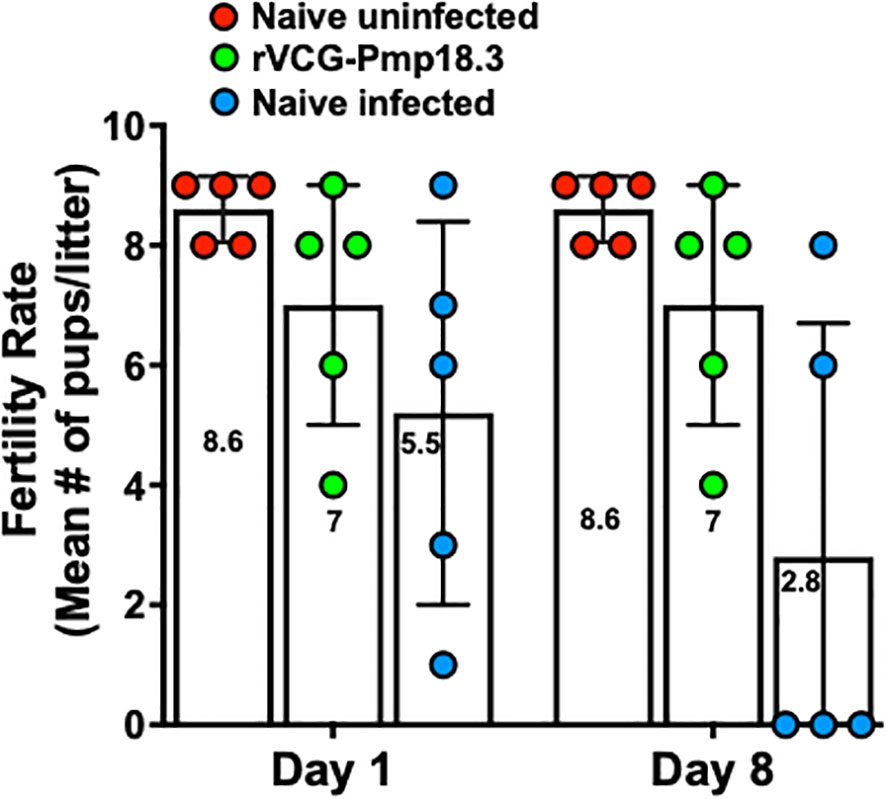
Figure 5 Protection against C. abortus-induced neonatal mortality by rVCG-Pmp18.3 vaccine following TC challenge with C. abortus AB7. Groups of mice (6/group) immunized IN with rVCG-Pmp18.3 vaccine as described above were mated with proven breeder males 3 weeks post-immunization and challenged TC with 1×106 IFUs of live C. abortus on day 10 of pregnancy. Unimmunized infected and uninfected mice served as positive and negative controls, respectively. The plotted data show the mean number of pups per mouse in each group on the day of birth (day 1) (fertility rate) and at the end of the observation period (day 8) post-parturition. Each symbol represents a mouse in each group. Numbers within each bar represent the mean number of pups per liter for each experimental group. One-way ANOVA with Tukey’s post-multiple comparison test was used to determine significant differences between groups.
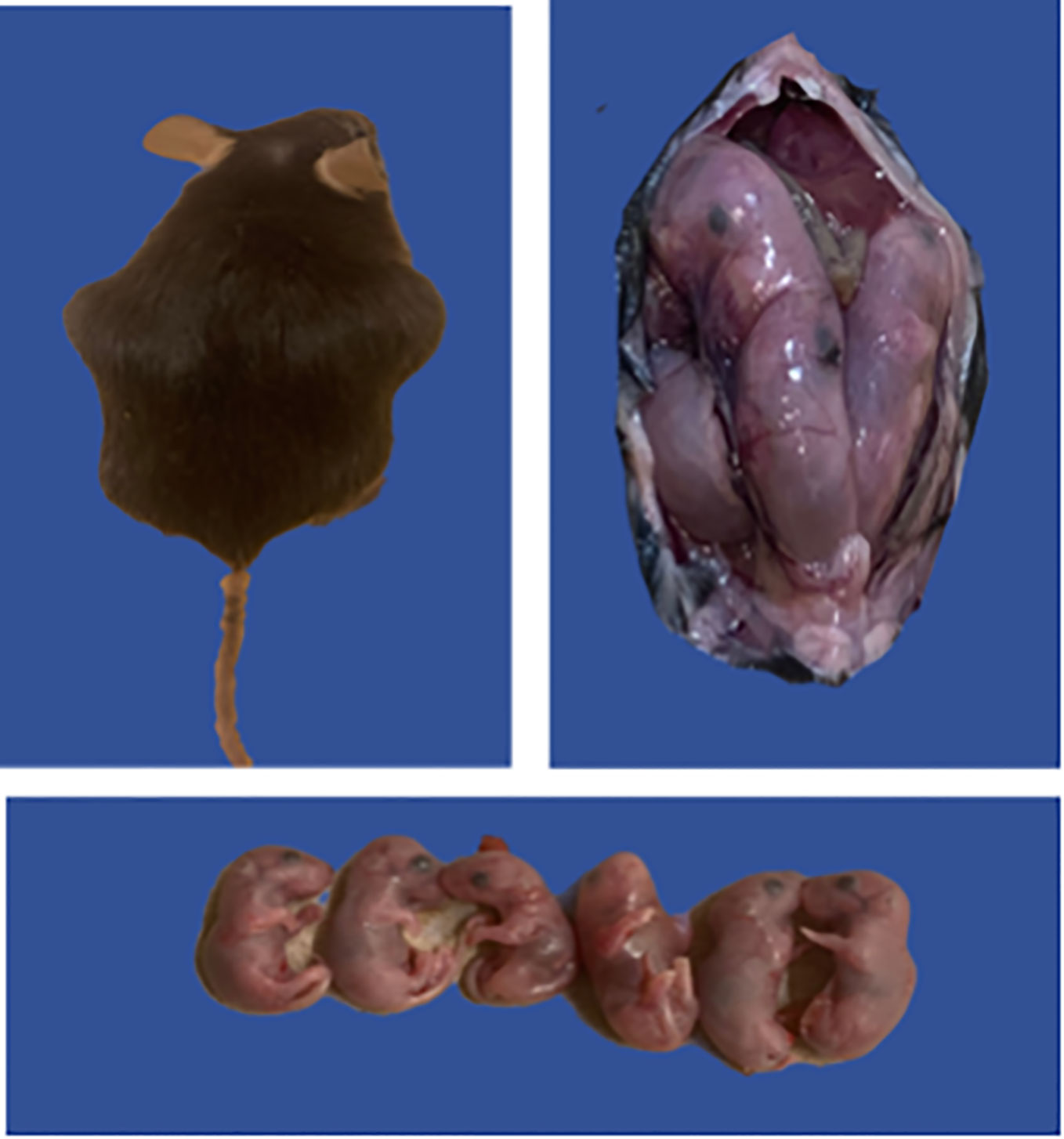
Figure 6 Possible case of C. abortus-induced dystocia and pre-term labor. Groups of mice (6/group) immunized IN with rVCG-Pmp18.3 vaccine as described above were mated with proven breeder males 3 weeks post-immunization and challenged TC with 1×106 IFUs of live C. abortus on day 10 of pregnancy. Unimmunized infected and uninfected mice served as positive and negative controls, respectively. The figure shows one of the non-immunized TC infected control mice with an incidence of dystocia (difficult or obstructed labor); the mouse attempted labor 7 days post-infection and failed to deliver six pups.
IN immunization with rVCG-Pmp18.3 protects against C. abortus-induced upper genital tract pathology following IN or TC challenge infection
The protective efficacy of the rVCG-Pmp18.3 vaccine against C. abortus-induced tubal pathology was evaluated by comparing the development of gross pathological lesions in the UGT of immunized and unimmunized mice challenged intranasally or transcervically on day 8 postpartum. As expected, no signs of gross pathological lesions were observed in the UGTs of unimmunized/uninfected control mice (Figure 7A). Interestingly, none of the genital tracts of mice challenged either IN or TC following IN immunization displayed any signs of pathological lesions (Figures 7B, C). In contrast, the genital tracts of unimmunized mice infected either IN (40%) or TC (80%) showed overt tubal dilation with black nodular lesions and dilated or swollen oviducts (Figures 7D, E). The results indicate that IN immunization with rVCG-Pmp18.3 protects against C. abortus-induced tubal pathology following IN or TC challenge.
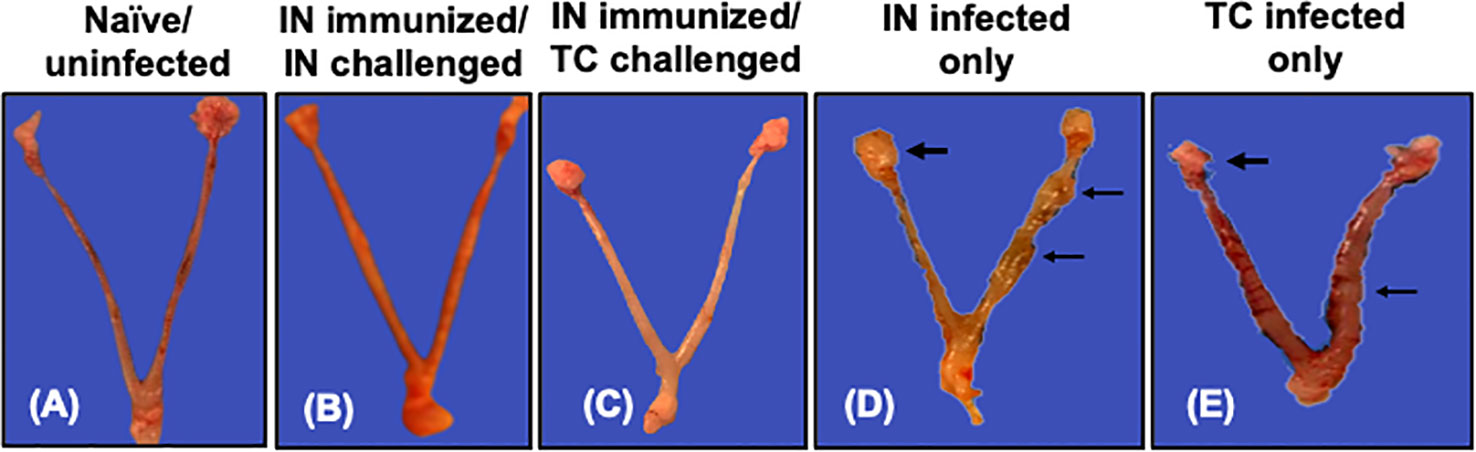
Figure 7 Protection against C. abortus-induced upper genital tract (UGT) pathology by rVCG-Pmp18.3 vaccine following IN or TC challenge infection. Groups of mice were immunized IN with rVCG-Pmp18.3 vaccine, mated with proven breeder males, and challenged IN or TC on day 10 of pregnancy as described above. Eight days after parturition, genital tracts were harvested from immunized and control mice and evaluated for the development of gross pathological lesions. Representative uterine horns of naive uninfected (A), rVCG-Pmp18.3 vaccine-immunized/IN challenged (B), and vaccine-immunized/TC challenged (C) mice with no evidence of visible pathology. Representative uterine horns of unimmunized/IN challenged (D) or TC challenged (E) control mice showing evidence of swollen inflamed oviducts (thick arrows) and tubal dilation with black nodular lesions (thin arrows).
Discussion
C. abortus infection is a significant cause of still birth and late-term spontaneous abortion in sheep. Limitations and safety concerns of the current commercially available live attenuated vaccines derived from the 1B strain of C. abortus, which have been incriminated in disease causation in farm animals, have been reported (26). Moreover, although inactivated vaccines have been shown to reduce abortions in sheep in the UK, they fail to prevent bacterial shedding and reproductive failures (27). These observations have prompted the search for new vaccine candidates, including subunit vaccines that will in addition to being efficacious obviate the safety concerns and limitations imposed by live and inactivated vaccines. Reports indicate that ewes that have aborted following Cab infection develop strong protective immunity and are protected from preterm abortion in subsequent seasons (28), suggesting that subunit vaccines that mimic such immune responses could constitute safer and effective vaccines for ruminants. Although ruminants are the natural host for C. abortus, mouse models have served as viable precursors for immune response and vaccine efficacy studies because the pathogenesis of Cab infection in sheep and mice is similar (29). We previously reported that a subunit vaccine candidate based on the N-terminal portion of the C. abortus Pmp18D and VCG protected mice against genital C. abortus infection (15). However, with regard to protection against infertility, upper genital tract pathology, spontaneous abortion, or neonatal death, the hallmark of C. abortus (Cab) infection in ruminants was not investigated.
Thus, we tested the protective efficacy of a modified rVCG-based Cab subunit vaccine comprising T- and B-cell epitopes of the Cab Pmp18D (rVCG-Pmp18.3) against abortion or neonatal mortality after Cab genital challenge infection in pregnant mice. The possibility that cellular and humoral immune effectors may contribute to resolution of Cab infection prompted us to concurrently assess the specific cell-mediated and humoral immune responses in the serum and vaginal secretions of vaccine-immunized mice. Immunological evaluation revealed that rVCG-Pmp18.3 vaccine-immunized mice elicited significant Chlamydia-specific IgA and IgG2c antibody responses in serum and vaginal secretions. Although reports indicate that anti-Cab antibodies are detected in the serum of ewes after Cab infection (30), the role of antibody in protection against ovine enzootic abortion (OEA) is incompletely understood. It has previously been suggested that antibodies may contribute to protection against secondary infection either by blocking attachment of EB to target cells or opsonizing EBs (31). However, since the magnitude of antibodies does not directly correlate with antibody functionality, the quality of elicited antibodies was determined by assessing their relative avidity. Establishing the quality of elicited antibodies is important, since antibody function has been shown to correlate with post-vaccination protection against bacterial organisms by developing long-term B-cell memory responses (32) (33). The finding that rVCG-Pmp18.3 vaccine-immunized mice elicited high avidity serum IgG2c antibodies, which are associated with Th1-type responses that are required for successful vaccination against OEA (9), suggests that these antibodies possess high functional activity.
Unlike C. trachomatis, which is genitally acquired, acquisition of Cab is mainly via the respiratory route, and following infection, it is spread through the blood and lymph to several organs, including the lungs, liver, spleen, and the placenta (34–36). Vaccine efficacy analyses against respiratory challenge with C. abortus strain AB7 revealed a significant decrease in chlamydial burden in the spleens and complete protection against challenge infection in the lungs, demonstrating the efficacy of the vaccine against mucosal and systemic infection. Change in lung weight has been established as an indicator of the severity disease in murine models of respiratory infections (37, 38). There was no correlation between lung weights and disease severity. However, lung weights were only recorded at a one-time point (day 18 post-challenge), and lung weights did not differ significantly between immunized and control mice. Moreover, reports indicate that changes in organ weights correlated with disease severity in the initial phases of infection (38–40). Furthermore, 18 days post-challenge may have been too late to observe significant differences in organ weights. To minimize contact with the mice during pregnancy, vaginal swabs were not collected, and so vaccine efficacy against genital Cab infection was not evaluated in this study.
Since Cab infections can cause upper genital tract (UGT) pathology leading to infertility or abortion and neonatal mortality (41, 42), we evaluated the protective efficacy of the vaccine against C. abortus-induced abortion or neonatal mortality in a pregnant mouse challenge model following IN and TC infection. The transcervical route has been used as a non-surgical method to bypass the cervical blockade in mice to initiate infection leading to upper genital tract inflammation (43–45). Comparison of C. abortus-induced abortion or neonatal mortality between immunized and control mice on day 8 postpartum showed that neither the immunized nor the unimmunized control mice aborted following both IN and TC challenge. These findings in mice confirm previous reports indicating that infected pregnant ewes may deliver live weak lambs, which fail to survive, cited by (46). In addition to reduced pup survival, the unimmunized mice infected TC also showed a significant reduction in fertility rate compared to those infected IN. The explanation for this disparity is uncertain given that the respiratory route is the natural route of Cab infection in ruminants leading to preterm abortion and other reproductive complications (47). On the other hand, the finding that the IN-infection route resulted in a significantly higher incidence of pup mortality in naive infected mice compared to the TC route is consistent with previous reports indicating that the respiratory route is the route by which Cab infection occurs naturally (35, 36, 47–49). The complete protection afforded by the vaccine against Cab-induced neonatal mortality in immunized mice following IN and TC challenge strongly suggests its potential as an effective vaccine for use in ruminants. Another finding in this study was a single incidence of dystocia in the TC-infected mice. This mouse attempted delivery 7 days post-challenge, approximately 18 days post-pregnancy, and was only able to deliver one weak fetus, which did not survive for 24 h. It is unclear if the TC inoculation contributed to the occurrence of dystocia in this mouse. Although dystocia is common among laboratory mice (50), the occurrence of dystocia in combination with premature delivery attempt may suggest the involvement of Cab infection.
Cab infections also induce severe UGT pathology involving inflammation and fibrosis, often leading to tissue damage and hydrosalpinx (41, 42). The absence of any evidence of gross pathological lesions in all vaccine-immunized mice following IN (natural route of infection) or TC challenge contrasts with the overt tubal dilation with black nodular lesions and dilated or swollen oviducts observed in the UGT of unimmunized mice, with greater severity occurring in TC-infected mice. These results corroborate the outcomes of a previous study that reported the protective efficacy of a rVCG-based vaccine against C. trachomatis-induced UGT pathology, involving oviduct and uterine inflammation following rectal immunization and intravaginal challenge (18). Previous reports indicate that a shift in the balance of a Th1- to a Th2-type immune response that leads to a decrease in IFN-γ and an increase in IL-4 and IL-10 production, which antagonizes and downregulates Th1-type cytokines, allows the proliferation and colonization of the placenta by Cab, triggering abortion (47). Neutrophil depletion has been reported to lead to a reduction in IFN-γ levels (51), while CD8+ T cell depletion in the spleens of experimentally infected mice led to an increase in IFN-γ secretion, which limits Cab multiplication (52). Another significant finding in this report is the observation that UGT pathological lesions, indicated by dilated uterine horns and inflamed oviducts, correlated with neonatal mortality. However, although visible gross pathology can indicate pregnancy outcomes, it is not always certain, since delivery of live offspring does not indicate the absence of Cab infection (53).
In conclusion, our study demonstrates that IN immunization with the VCG-based Cab vaccine stimulated immune effectors that mediate Cab clearance in lung (mucosal) and splenic (systemic) tissues. The study also showed that immunization with the vaccine protected against Cab-induced neonatal mortality and tubal pathologies after IN and TC challenge. The complete protection afforded by the vaccine against Cab-induced respiratory mucosal infection, neonatal mortality, and tubal pathologies, a hallmark of Cab infection in ruminants, strongly suggests its potential as an effective vaccine for ruminants. To advance the commercial potential of this vaccine, future studies will optimize the antigen dose and the number of vaccine doses required for protection of ruminants.
Data availability statement
The original contributions presented in the study are included in the article/supplementary material. Further inquiries can be directed to the corresponding author.
Ethics statement
The animal study was approved by The Institutional Animal Care and Use Committee (IACUC), Morehouse School of Medicine (MSM) (Protocol Number: 19-09). The study was conducted in accordance with the local legislation and institutional requirements.
Author contributions
FE conceived and designed the study. SR performed all the experiments. FM, TT, CB, and SL contributed to the experimental work. FE, SR, JI, and YO contributed to analysis and interpretation of data. SR and FE wrote the manuscript. All authors contributed to the article and approved the submitted version.
Funding
This work was supported by a Public Health Service grant R01AI126897 from the National Institutes of Health to FE. The investigation was conducted in a facility constructed with support from Research Facilities Improvement Grant #1 C06 RR18386 from the National Center for Research Resources, National Institutes of Health.
Acknowledgments
We gratefully acknowledge the animal care staff and veterinarians at the Center for Laboratory Animal Resources (CLAR) of Morehouse School of Medicine for their care and guidance during this study. We are also grateful for the technical support of the Core facility staff during these experiments.
Conflict of interest
The authors declare that the research was conducted in the absence of any commercial or financial relationships that could be construed as a potential conflict of interest.
Publisher’s note
All claims expressed in this article are solely those of the authors and do not necessarily represent those of their affiliated organizations, or those of the publisher, the editors and the reviewers. Any product that may be evaluated in this article, or claim that may be made by its manufacturer, is not guaranteed or endorsed by the publisher.
References
1. Bouakane A, Benchaïeb I, Rodolakis A. Abortive potency of Chlamydophila abortus in pregnant mice is not directly correlated with placental and fetal colonization levels. Infection Immun (2003) 71:7219–22. doi: 10.1128/IAI.71.12.7219-7222.2003
2. Kerr K, Entrican G, McKeever D, Longbottom D. Immunopathology of Chlamydophila abortus infection in sheep and mice. Res Veterinary Sci (2005) 78:1–7. doi: 10.1016/j.rvsc.2004.08.004
3. Longbottom D, Coulter LJ. Animal chlamydioses and zoonotic implications. J Comp Pathol (2003) 128:217–44. doi: 10.1053/jcpa.2002.0629
4. Wong SY, Gray ES, Buxton D, Finlayson J, Johnson FW. Acute placentitis and spontaneous abortion caused by chlamydia psittaci of sheep origin: a histological and ultrastructural study. J Clin Pathol (1985) 38:707–11. doi: 10.1136/jcp.38.6.707
5. Walder G, Hotzel H, Brezinka C, Gritsch W, Tauber R, Würzner R, et al. An unusual cause of sepsis during pregnancy: recognizing infection with chlamydophila abortus. Obstetrics gynecol (2005) 106:1215–7. doi: 10.1097/01.AOG.0000161060.69470.9c
6. Pichon N, Guindre L, Laroucau K, Cantaloube M, Nallatamby A, Parreau S. Chlamydia abortus in pregnant woman with acute respiratory distress syndrome. Emerg Infect Dis (2020) 26:628–9. doi: 10.3201/eid2603.191417
7. Laroucau K, Aaziz R, Vorimore F, Menard MF, Longbottom D, Denis G. Abortion storm induced by the live C. abortus vaccine 1B strain in a vaccinated sheep flock, mimicking a natural wild-type infection. Veterinary Microbiol (2018) 225:31–3. doi: 10.1016/j.vetmic.2018.09.012
8. Gerber A, Thoma R, Vretou E, Psarrou E, Kaiser C, Doherr MG, et al. Ovine Enzootic Abortion (OEA): a comparison of antibody responses in vaccinated and naturally-infected swiss sheep over a two year period. BMC Vet Res (2007) 3:24. doi: 10.1186/1746-6148-3-24
9. Entrican G, Wheelhouse N, Wattegedera SR, Longbottom D. New challenges for vaccination to prevent chlamydial abortion in sheep. Comp Immunol Microbiol Infect Dis (2012) 35:271–6. doi: 10.1016/j.cimid.2011.12.001
10. Livingstone M, Wattegedera SR, Palarea-Albaladejo J, Aitchison K, Corbett C, Sait M, et al. Efficacy of two Chlamydia abortus subcellular vaccines in a pregnant ewe challenge model for ovine enzootic abortion. Vaccines (Basel) (2021) 9. doi: 10.3390/vaccines9080898
11. Kiselev AO, Skinner MC, Lampe MF. Analysis of pmpD expression and PmpD post-translational processing during the life cycle of Chlamydia trachomatis serovars A, D, and L2. PloS One (2009) 4:e5191. doi: 10.1371/journal.pone.0005191
12. Tan C, Hsia RC, Shou H, Haggerty CL, Ness RB, Gaydos CA, et al. Chlamydia trachomatis-infected patients display variable antibody profiles against the nine-member polymorphic membrane protein family. Infect Immun (2009) 77:3218–26. doi: 10.1128/IAI.01566-08
13. Vasilevsky S, Stojanov M, Greub G, Baud D. Chlamydial polymorphic membrane proteins: regulation, function and potential vaccine candidates. Virulence (2016) 7:11–22. doi: 10.1080/21505594.2015.1111509
14. Wehrl W, Brinkmann V, Jungblut PR, Meyer TF, Szczepek AJ. From the inside out–processing of the Chlamydial autotransporter PmpD and its role in bacterial adhesion and activation of human host cells. Mol Microbiol (2004) 51:319–34. doi: 10.1046/j.1365-2958.2003.03838.x
15. Pan Q, Pais R, Ohandjo A, He C, He Q, Omosun Y, et al. Comparative evaluation of the protective efficacy of two formulations of a recombinant Chlamydia abortus subunit candidate vaccine in a mouse model. Vaccine (2015) 33:1865–72. doi: 10.1016/j.vaccine.2015.02.007
16. Eko FO, Lubitz W, McMillan L, Ramey K, Moore TT, Ananaba GA, et al. Recombinant Vibrio cholerae ghosts as a delivery vehicle for vaccinating against Chlamydia trachomatis. Vaccine (2003) 21:1694–703. doi: 10.1016/S0264-410X(02)00677-1
17. Eko FO, Okenu DN, Singh UP, He Q, Black C, Igietseme JU. Evaluation of a broadly protective Chlamydia-cholera combination vaccine candidate. Vaccine (2011) 29:3802–10. doi: 10.1016/j.vaccine.2011.03.027
18. Pais R, Omosun Y, He Q, Blas-MaChado U, Black C, Igietseme JU, et al. Rectal administration of a chlamydial subunit vaccine protects against genital infection and upper reproductive tract pathology in mice. PloS One (2017) 12:e0178537. doi: 10.1371/journal.pone.0178537
19. Pan Q, Zhang Q, Chu J, Pais R, Liu S, He C, et al. Chlamydia abortus Pmp18.1 Induces IL-1β Secretion by TLR4 Activation through the MyD88, NF-κB, and Caspase-1 Signaling Pathways. Front Cell infection Microbiol (2017) 7:514. doi: 10.3389/fcimb.2017.00514
20. Macmillan L, Ifere GO, He Q, Igietseme JU, Kellar KL, Okenu DM, et al. A recombinant multivalent combination vaccine protects against Chlamydia and genital herpes. FEMS Immunol Med Microbiol (2007) 49:46–55. doi: 10.1111/j.1574-695X.2006.00165.x
21. Goldblatt D, Pinto Vaz ARJPM, Miller E. Antibody avidity as a surrogate marker of successful priming by haemophilus influenzae type b conjugate vaccines following infant immunization. J Infect Dis (1998) 177:1112–5. doi: 10.1086/517407
22. Anttila M, Eskola J, Åhman H, Käyhty H. Differences in the avidity of antibodies evoked by four different pneumococcal conjugate vaccines in early childhood1In the study the European guidelines for Good Clinical Practice (based on directive no. 91/507/EEC) were followed. The protocol of the study was approved by the Ethics Committee of the National Public Health Institute, Helsinki, Finland. Written informed consent was obtained from the parents prior to enrolment of their children.1. Vaccine (1999) 17:1970–7. doi: 10.1016/S0264-410X(98)00458-7
23. Almanzar G, Ottensmeier B, Liese J, Prelog M. Assessment of IgG avidity against pertussis toxin and filamentous hemagglutinin via an adapted enzyme-linked immunosorbent assay (ELISA) using ammonium thiocyanate. J Immunol Methods (2013) 387:36–42. doi: 10.1016/j.jim.2012.09.008
24. Trzewikoswki de Lima G, Rodrigues TS, Portilho AI, Correa VA, Gaspar EB, De Gaspari E. Immune responses of meningococcal B outer membrane vesicles in middle-aged mice. Pathog Dis (2020) 78. doi: 10.1093/femspd/ftaa028
25. Buendía AJ, De Oca RM, Navarro JA, Sánchez J, Cuello F, Salinas J. Role of polymorphonuclear neutrophils in a murine model of Chlamydia psittaci-induced abortion. Infection Immun (1999) 67:2110–6. doi: 10.1128/IAI.67.5.2110-2116.1999
26. Wheelhouse N, Aitchison K, Laroucau K, Thomson J, Longbottom D. Evidence of Chlamydophila abortus vaccine strain 1B as a possible cause of ovine enzootic abortion. Vaccine (2010) 28:5657–63. doi: 10.1016/j.vaccine.2010.04.114
27. García-Seco T, Pérez-Sancho M, Salinas J, Navarro A, Díez-Guerrier A, García N, et al. Effect of preventive Chlamydia abortus vaccination in offspring development in sheep challenged experimentally. Front Veterinary Sci (2016) 3. doi: 10.3389/fvets.2016.00067
28. Rocchi MS, Wattegedera S, Meridiani I, Entrican G. Protective adaptive immunity to Chlamydophila abortus infection and control of ovine enzootic abortion (OEA). Vet Microbiol (2009) 135:112–21. doi: 10.1016/j.vetmic.2008.09.030
29. Caro MR, Buendía AJ, Del Rio L, Ortega N, Gallego MC, Cuello F, et al. Chlamydophila abortus infection in the mouse: a useful model of the ovine disease. Vet Microbiol (2009) 135:103–11. doi: 10.1016/j.vetmic.2008.09.029
30. Livingstone M, Entrican G, Wattegedera S, Buxton D, McKendrick IJ, Longbottom D. Antibody responses to recombinant protein fragments of the major outer membrane protein and polymorphic outer membrane protein POMP90 in chlamydophila abortus-infected pregnant sheep. Clin Diagn. Lab Immunol (2005) 12:770–7. doi: 10.1128/CDLI.12.6.770-777.2005
31. Tifrea DF, Pal S, Toussi DN, Massari P, de la Maza LM. Vaccination with major outer membrane protein proteosomes elicits protection in mice against a Chlamydia respiratory challenge. Microbes Infect (2013) 13:920–7. doi: 10.1016/j.micinf.2013.08.005
32. Alam MM, Arifuzzaman M, Ahmad SM, Hosen MI, Rahman MA, Rashu R, et al. Study of Avidity of Antigen-Specific Antibody as a Means of Understanding Development of Long-Term Immunological Memory after Vibrio cholerae O1 Infection. Clin Vaccine Immunol (2013) 20:17–23. doi: 10.1128/CVI.00521-12
33. Maslanka Susan E, Tappero Jordan W, Plikaytis Brian D, Brumberg Robert S, Dykes Janet K, Gheesling Linda L, et al. Age-dependent neisseria meningitidis serogroup C class-specific antibody concentrations and bactericidal titers in sera from young children from montana immunized with a licensed polysaccharide vaccine. Infection Immun (1998) 66:2453–9. doi: 10.1128/IAI.66.6.2453-2459.1998
34. Navarro JA, García de la Fuente JN, Sánchez J, Martínez CM, Buendía AJ, Gutiérrez-Martín CB, et al. Kinetics of infection and effects on the placenta of Chlamydophila abortus in experimentally infected pregnant ewes. Vet Pathol (2004) 41:498–505. doi: 10.1354/vp.41-5-498
35. Gutierrez J, Williams EJ, O’Donovan J, Brady C, Proctor AF, Marques PX, et al. Monitoring clinical outcomes, pathological changes and shedding of Chlamydophila abortus following experimental challenge of periparturient ewes utilizing the natural route of infection. Vet Microbiol (2011) 147:119–26. doi: 10.1016/j.vetmic.2010.06.015
36. Longbottom D, Livingstone M, Maley S, van der Zon A, Rocchi M, Wilson K, et al. Intranasal infection with Chlamydia abortus induces dose-dependent latency and abortion in sheep. PloS One (2013) 8:e57950. doi: 10.1371/journal.pone.0057950
37. Huppert M, Sun SH, Gleason-Jordon I, Vukovich KR. Lung weight parallels disease severity in experimental coccidioidomycosis. Infect Immun (1976) 14:1356–68. doi: 10.1128/iai.14.6.1356-1368.1976
38. Jiang X, Shen C, Yu H, Karunakaran KP, Brunham RC. Differences in innate immune responses correlate with differences in murine susceptibility to Chlamydia muridarum pulmonary infection. Immunology (2010) 129:556–66. doi: 10.1111/j.1365-2567.2009.03157.x
39. Huang J, Wang M-D, Lenz S, Gao D, Kaltenboeck B. IL-12 administered during <em<Chlamydia psittaci</em< Lung infection in mice confers immediate and long-term protection and reduces macrophage inflammatory protein-2 level and neutrophil infiltration in lung tissue. J Immunol (1999) 162:2217. doi: 10.4049/jimmunol.162.4.2217
40. Yuan K, Billor N. Variable selection in the chlamydia pneumoniae lung infection study. J Data Sci (2013) 11:371–87.
41. Essig A, Longbottom D. Chlamydia abortus: new aspects of infectious abortion in sheep and potential risk for pregnant women. Curr Clin Microbiol Rep (2015) 2:22–34. doi: 10.1007/s40588-015-0014-2
42. Rajeeve K, Sivadasan R. Transcervical mouse infections with chlamydia trachomatis and determination of bacterial burden. Bio Protoc (2020) 10:e3506. doi: 10.21769/BioProtoc.3506
43. Gondek DC, Olive AJ, Stary G, Starnbach MN. CD4+ T cells are necessary and sufficient to confer protection against Chlamydia trachomatis infection in the murine upper genital tract. J Immunol (2012) 189:2441–9. doi: 10.4049/jimmunol.1103032
44. Stary G, Olive A, Radovic-Moreno AF, Gondek D, Alvarez D, Basto PA, et al. VACCINES. A mucosal vaccine against Chlamydia trachomatis generates two waves of protective memory T cells. Science (2015) 348:aaa8205. doi: 10.1126/science.aaa8205
45. Pal S, Tifrea DF, Zhong G, de la Maza LM. Transcervical inoculation with chlamydia trachomatis induces infertility in HLA-DR4 transgenic and wild-type mice. Infect Immun (2018) 86. doi: 10.1128/IAI.00722-17
46. Turin L, Surini S, Wheelhouse N, Rocchi MS. Recent advances and public health implications for environmental exposure to Chlamydia abortus: from enzootic to zoonotic disease. Vet Res (2022) 53:37. doi: 10.1186/s13567-022-01052-x
47. Wattegedera SR, Livingstone M, Maley S, Rocchi M, Lee S, Pang Y, et al. Defining immune correlates during latent and active chlamydial infection in sheep. Vet Res (2020) 51:75. doi: 10.1186/s13567-020-00798-6
48. Wilsmore AJ, Parsons V, Dawson M. Experiments to demonstrate routes of transmission of ovine enzootic abortion. Br Vet J (1984) 140:380–91. doi: 10.1016/0007-1935(84)90130-1
49. Dawson M, Zaghloul A, Wilsmore AJ. Ovine enzootic abortion: experimental studies of immune responses. Res Vet Sci (1986) 40:59–64. doi: 10.1016/S0034-5288(18)30487-9
50. Wilkinson AW, Diep J, Dai S, Liu S, Ooi YS, Song D, et al. SETD3 is an actin histidine methyltransferase that prevents primary dystocia. Nature (2019) 565:372–6. doi: 10.1038/s41586-018-0821-8
51. Buendia AJ, Martinez CM, Ortega N, Del Rio L, Caro MR, Gallego MC, et al. Natural killer (NK) cells play a critical role in the early innate immune response to chlamydophila abortus infection in mice. J Comp Pathol (2004) 130:48. doi: 10.1016/S0021-9975(03)00069-0
52. Martinez CM, Buendia AJ, Sanchez J, Ortega N, Caro MR, Gallego MC, et al. Relative importance of CD4+ and CD8+ T cells in the resolution of Chlamydophila abortus primary infection in mice. J Comp Pathol (2006) 134:297–307. doi: 10.1016/j.jcpa.2005.12.002
Keywords: Chlamydia abortus, Pmp18.3, vaccine delivery, protection, neonatal mortality
Citation: Richardson S, Bell CR, Medhavi F, Tanner T, Lundy S, Omosun Y, Igietseme JU and Eko FO (2023) A novel cold-chain free VCG-based subunit vaccine protects against Chlamydia abortus-induced neonatal mortality in a pregnant mouse model. Front. Immunol. 14:1243743. doi: 10.3389/fimmu.2023.1243743
Received: 21 June 2023; Accepted: 28 September 2023;
Published: 17 October 2023.
Edited by:
Daniela Hozbor, Institute of Biotechnology and Molecular Biology (IBBM), ArgentinaReviewed by:
Vida A. Dennis, Alabama State University, United StatesWilmore C. Webley, University of Massachusetts Amherst, United States
Copyright © 2023 Richardson, Bell, Medhavi, Tanner, Lundy, Omosun, Igietseme and Eko. This is an open-access article distributed under the terms of the Creative Commons Attribution License (CC BY). The use, distribution or reproduction in other forums is permitted, provided the original author(s) and the copyright owner(s) are credited and that the original publication in this journal is cited, in accordance with accepted academic practice. No use, distribution or reproduction is permitted which does not comply with these terms.
*Correspondence: Francis O. Eko, ZmVrb0Btc20uZWR1