- 1Laboratory of Medical Investigation LIM-56, Division of Dermatology, Medical School, University of Sao Paulo (USP), Sao Paulo, Brazil
- 2Post Graduation Program in Health Sciences, Santo Amaro University (UNISA), Sao Paulo, Brazil
- 3Department of Allergy and Clinical Immunology, Ajou University School of Medicine, Suwon, Republic of Korea
The regulatory T (Treg) cells constitute a functionally defined subpopulation of T cells that modulate the immune system and maintain immune tolerance through suppression of the development of autoimmune responses to self-antigens and allergic reactions to external antigens. Reduction in the number or function of Treg cells has been suggested as a key immune abnormality underlying the development of autoimmune and allergic diseases. In vitro studies have demonstrated that purified polyvalent immunoglobulin G (IgG) from multiple healthy blood donors can exert immunomodulatory effects on Treg cells. Incubation of polyvalent human IgG with purified CD4+CD25high T cells increased the intracellular expression of interleukin (IL)-10. Intravenous administration of polyvalent human IgG induced significant expansions of CD4+ Foxp3+ Treg cells and clinical improvements in patients with autoimmune diseases. In human clinical trials, intramuscular administration of autologous total IgG significantly increased the percentage of IL-10-producing CD4+ Treg cells in the peripheral blood of healthy subjects and provided significant clinical improvements in patients with atopic dermatitis. These results suggest a clinical usefulness of polyvalent IgG-induced activation of Treg cells in human subjects. This review proposes a new hypothesis for immune tolerance mechanism by integrating the pre-existing “idiotypic network theory” and “Treg cell theory” into an “anti-idiotypic Treg cell theory.” Based on this hypothesis, an “active anti-idiotypic therapy” for allergic and autoimmune diseases using autologous polyvalent IgG (as immunizing antigens) is suggested as follows: (1) Intramuscular or subcutaneous administration of autologous polyvalent IgG produces numerous immunogenic peptides derived from idiotypes of autologous IgG through processing of dendritic cells, and these peptides activate anti-idiotypic Treg cells in the same subject. (2) Activated anti-idiotypic Treg cells secrete IL-10 and suppress Th2 cell response to allergens and autoimmune T cell response to self-antigens. (3) These events can induce a long-term clinical improvements in patients with allergic and autoimmune diseases. Further studies are needed to evaluate the detailed molecular mechanism underlying polyvalent IgG-induced Treg cell activation and the clinical usefulness of this immunomodulatory therapy for autoimmune and allergic diseases.
1 Introduction
1.1 Immune tolerance and regulatory T cells
Immune tolerance is a state in which the immune system is unresponsive to foreign and self antigens that would otherwise result in a response (1). Immune tolerance is essential for maintaining immune homeostasis in healthy human subjects; defects in immune tolerance cause autoimmune and allergic diseases (1, 2).
Regulatory T (Treg) cells constitute a functionally defined subpopulation of T cells that modulate the immune system and have been suggested to play a central role in the maintenance of immune tolerance to self and foreign antigens through suppression of uncontrolled exaggerated autoimmune responses and allergic reactions that can be harmful to the host, thereby preventing the development of autoimmune and allergic diseases (1).
Treg cells are classified into natural Treg cells (nTreg cells) and induced Treg cells (iTreg cells) (3). nTreg cells arise from immature T cells in the thymus and express forkhead box P3 (Foxp3), CD4, and CD25 markers (4–6) and mediate peripheral immune tolerance through contact-dependent suppressor activity on other lymphocyte clones before they develop into full effector cells (7). iTreg cells develop after T cell maturation, do not express Foxp3, and can suppress other lymphocytes in peripheral tissues and lymph nodes but not through a contact-dependent mechanism; additionally, iTreg cells produce immunosuppressive cytokines (8). iTreg induction can be mediated through the exposure of naive CD4+ T cells to transforming growth factor-β (TGF-β), retinoic acid, and antigen presentation in peripheral tissues (3). In peripheral immune tolerance, repeated antigen exposure can also lead to the induction of iTregs (8). Among iTreg cells, the interleukin (IL)-10-producing CD4+ Treg cells (type 1 regulatory T cell: Tr1 cell) have been shown to play a key role in antigen-specific immune tolerance and can be induced through repeated administration of a specific antigen in humans (9–12). Foxp3+ Treg cells are primarily generated in the thymus (tTreg), but they can also be generated extrathymically at peripheral sites (pTreg) (13). Treg cells induce cytokine-dependent immune suppression through the secretion of IL-10 which suppresses Type 1 (Th1) and Type 2 T helper (Th2) cells (1, 2). Based on animal studies, decreased number and/or defective function (deficiency or dysfunction) of Treg cell have been suggested as a critical immune abnormality responsible for the development of autoimmune and allergic diseases (1, 2).
1.2 Polyvalent immunoglobulin G for immune regulation
Intravenous immunoglobulin G (IVIg), that is polyvalent IgG purified from the plasma pool of multiple healthy human blood donors, has been used to treat patients with primary immunodeficiency diseases associated with reduced immunoglobulin production (14, 15). Due to its immunomodulatory effects, IVIg has also been used to treat various autoimmune and allergic diseases (16, 17). Current evidence suggests that polyvalent IgG modulates the function of various immune cells, including dendritic cells (DCs), neutrophils, monocytes, macrophages, T cells, and B cells (18). Under in vitro experimental conditions, incubation of polyvalent human IgG with purified CD4+CD25high T cells increased the expression of intracellular IL-10 in these cells (19). Activation of Treg cells seems to be the central mechanism responsible for the immunomodulatory and anti-inflammatory effects of polyvalent IgG (20–22).
The induction or activation of CD4+ Treg cells by IgG may involve diverse mechanisms considering the natural structure of these molecules. Some studies demonstrated that fragment crystallizable (Fc) regions of IgG obtained from IVIg formulations may interact with Fcγ receptors and thereby mediate part of the IVIg immunoregulatory effect (23, 24), while other report indicated that Fc fragments are not involved in this effect (25). Studies using recombinant IgG Fc fragments in murine models demonstrated a reduction in collagen-induced arthritis, idiopathic thrombocytopenic purpura, and myasthenia gravis (26–28), along with some beneficial effects in murine inflammatory neuropathy (29). The immunomodulatory effect of recombinant Fc fragments observed in the mouse model of collagen-induced arthritis could not be reproduced in macaques (28). In humans, the in vivo use of IVIg Fc fragments suggested a similar immunomodulatory effect of full IVIg in children with thrombocytopenic purpura (30); however, this effect could not be reproduced in ex vivo assays using human whole blood (31), and no other study could clarify the role of Fc fragments in human diseases (32).
The IgG fragments that can react with the antigen-binding sites (idiotypes) of pathogenic antibodies (IgG autoantibodies or IgE antibodies) in IVIg formulations have been suggested to mediate several aspects of immunomodulation through not only neutralization of pathogenic antibodies but also through other mechanisms, such as inhibition of complement activation and suppression of cytokine, in autoimmune and allergic diseases (33–35).
1.3 Idiotypic network theory
The “idiotypic network theory” that was initially proposed as a mechanism of immune tolerance by Jerne in 1974 suggests that the idiotypes of autologous immunoglobulins are immunogenic enough to induce immune responses (anti-idiotypic immune response) in the same host (36, 37). In this theory, the induction of an autoimmune anti-idiotypic response to the original idiotype plays a physiological role in the development of the immune tolerance state of the host. In this theory, immune tolerance is not a passive state of the immune system not responding to self and foreign antigens, but an active state of the immune system maintained through continuous low-grade physiological autoimmune responses to idiotypes of circulating autologous immunoglobulins in mammalian hosts preventing the development of exaggerated immune responses to self or foreign antigens (bacteria, virus, allergens) harmful to the host (36, 38, 39). Immune tolerance is a state of well-controlled responsiveness of the immune system to specific substances or tissues that would otherwise have the capacity to destroy these substances or tissues (36, 38, 39). Immune tolerance is induced via exposure to specific antigens through T cell receptors (TCRs) (1, 2). In the “idiotypic network theory”, idiotypes (variable regions with antigen specific binding capacity) of polyvalent autologous immunoglobulins and TCRs act as numerous endogenous antigens that can stimulate the immune system. These antigenic stimulations maintain numerous T cells and B cells with binding specificities to numerous antigens (both autoantigens and external antigens) and ultimately maintain an immune tolerance state (immune homeostasis).
This review focuses on mechanisms underlying the immunomodulation mediated by idiotypes of polyvalent IgG (including hypervariable regions of IgG) and not those mediated by the constant region of polyvalent IgG.
1.4 Anti-idiotypic regulatory T cell theory for immune tolerance
Unfortunately, knowledge regarding the scientific link between the pre-existing “Treg cell theory” and the “idiotypic network theory” for immune tolerance mechanisms is lacking. The mechanism underlying the cause-effect relationship between Treg cell activation and anti-idiotypic immune response has not been explained as yet. The disadvantages and weaknesses in the “idiotypic network theory” include the lack of experimental evidence regarding the actual mechanism in animals or humans through which immune tolerance is maintained and the absence of a human clinical trial demonstrating its clinical usefulness in treating autoimmune and allergic diseases. Rationale supporting the existence of “anti-idiotypic T cells” can be obtained from the “idiotypic network theory” itself. The “idiotypic network theory” suggests that the idiotypes of autologous immunoglobulins are immunogenic enough to induce immune responses (anti-idiotypic B cell and T cell immune response) in the same host (36, 37). Therefore, the pre-existence of anti-idiotypic T cells specifically recognizing peptides derived from idiotypes of autologous IgG is essential to maintain an immune tolerance state according to the “idiotypic network theory”. Thus, we propose a new hypothesis regarding the immune tolerance mechanism by integrating the “idiotypic network theory” and “Treg cell theory” into an “anti-idiotypic Treg cell theory” in this review.
T cells need antigenic stimulations for activation and survival (1, 3). What are the antigens stimulating Treg cells? This review proposes an answer that major antigens stimulating Treg cells include idiotypes (or its peptides processed by antigen-presenting cells, APCs) of autologous immunoglobulin.
2 Four hypothetical models for the mechanism underlying polyvalent IgG-induced activation of Treg cells
This review proposes four hypothetical models for the mechanism underlying polyvalent IgG-induced activation of Treg cells on the basis of previous studies and our speculations (Figure 1).
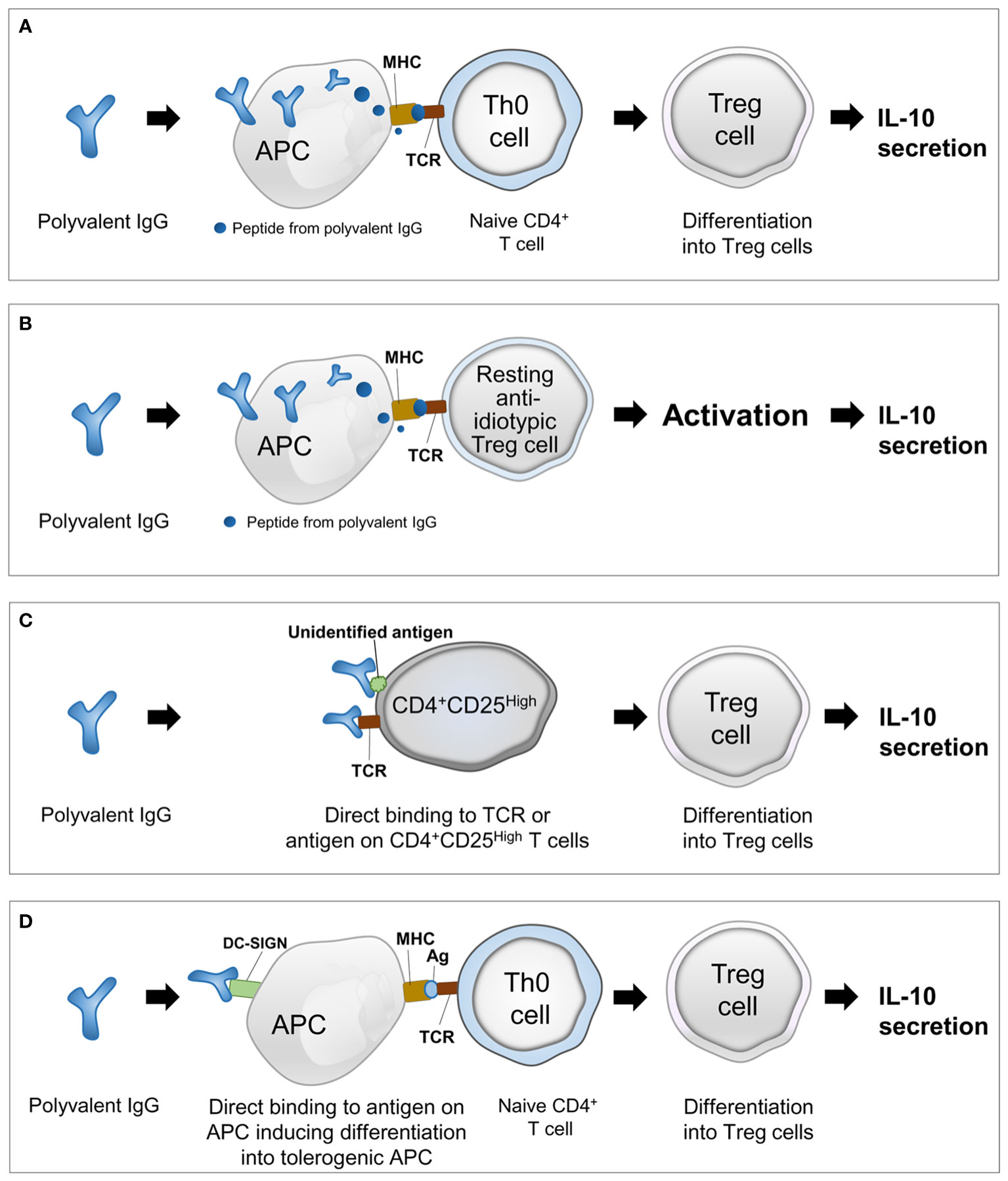
Figure 1 Four hypothetical models explaining the mechanism underlying polyvalent IgG-induced activation of regulatory T cells. (A) Presentation of peptides from polyvalent IgG by APCs and differentiation of naive T cells into Treg cells; (B) presentation of peptide idiotypes of polyvalent IgG by APCs and activation of pre-existing resting anti-idiotypic Treg cells; (C) activation of resting Treg cells by direct binding of polyvalent IgG to TCRs or unidentified antigens; and (D) direct binding of polyvalent IgG to conserved receptors or other unidentified antigens on APCs and induction of the tolerogenic phenotype of APCs facilitating differentiation of naive T cells into Treg cells. IgG, immunoglobulin G; Treg, regulatory T cell; IL-10, interleukin-10; TCR, T-cell receptor; APC, antigen-presenting cell; MHC, major histocompatibility complex; DC, dendritic cell; DC-SIGN, dendritic cell-specific intercellular adhesion molecule-3-grabbing nonintegrin.
[Model 1] Antigen presentation to naive T cells (Th0 cells): presentation of peptides from polyvalent IgG by APCs and differentiation of naive T cells into Treg cells (Figure 1A).
[Model 2] Antigen presentation to resting Treg cells: presentation of peptides from idiotypes of polyvalent IgG by APCs and activation of pre-existing resting anti-idiotypic Treg cells (Figure 1B).
[Model 3] Direct binding to antigens on T cells: activation of resting Treg cells by direct binding of polyvalent IgG to TCRs or unidentified antigens (Figure 1C).
[Model 4] Direct binding to antigens on APCs: direct binding of polyvalent IgG to conserved receptors or other unidentified antigens on APCs and induction of the tolerogenic phenotype of APCs facilitating differentiation of naive T cells into Treg cells (Figure 1D).
However, these four hypothetical models should be validated through future experiments. This review describes the limitations (unanswered questions) of these hypotheses to promote further studies on this topic by colleagues (Table 1).
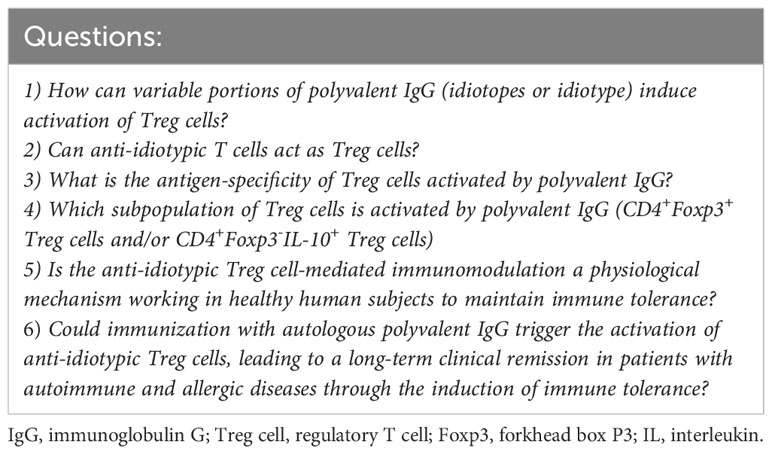
Table 1 Six general questions on the mechanism of immune modulation and activation of regulatory T cells induced by polyvalent IgG.
2.1 Hypothetical model 1 and 2: activation of Treg cells by peptides from polyvalent IgG processed by APCs (main hypotheses of authors)
APCs, including DCs, take up antigens in peripheral tissues, process them into peptides through proteolysis, and load these peptides on major histocompatibility complex (MHC) class I and II molecules (40). This review suggests that APCs, including DCs, can phagocytose polyvalent IgGs and present the peptides to naive T cells, after which the naive T cells differentiate into Treg cells [Model 1], or polyvalent IgG derived peptides activate resting anti-idiotypic Treg cells to produce IL-10 [Model 2] (Figures 1A, B). The following are past studies supporting these two hypothetical models.
In an abortion-prone mouse model, peptides produced by APCs from processing of the constant region of polyvalent IgG with a high affinity to MHC class II molecules (that can activate Treg cells and are termed as Tregitopes) can reduce the fetal death rate and increase IL-10 producing CD4+CD25+Foxp3+ Treg cells (41). In a mouse model of ovalbumin (OVA)-induced asthma, intraperitoneal administration of IVIg from healthy human blood donors could induce antigen-specific peripherally generated Foxp3+ Treg cells even after selective depletion of pre-existing CD4+CD25+Foxp3+ Treg cells and inhibit Th2 responses and airway inflammation (42). Subsequently, the same research group demonstrated that the inhibitory effect of Tregitopes could promote tolerance by activating Treg activity in a mouse model of OVA-induced asthma (43). These results suggested that Tregitopes could be active components mediating IVIg-induced anti-inflammatory effect (Model 1, Figure 1A).
In a murine model of OVA-immunization to induce OVA-specific IgG antibodies, subcutaneous injections of IVIg induced the activation and expansion of B and CD4+ T cells in the spleen and draining lymph nodes, resulted in a reduction in OVA-specific antibody production, and induced anti-IVIg IgG antibodies that mainly recognized the F(ab’)2 fragments of IVIg (44). These immunomodulatory effects could not be induced after subcutaneous injections of two human IgG monoclonal antibodies that share the constant region of IgG but not their idiotypes (44). These observations suggested that the immune responses to idiotypes of IVIg with enormous sequence diversity might produce immunomodulatory effects suppressing the production of anti-OVA IgG antibodies (44). However, in this mouse model, there was no evidence of the activation of Treg cells induced through the subcutaneous injections of IVIg (44). Investigation of the human leukocyte antigen (HLA) class II peptide repertoire from IVIg-loaded human DCs via MHC-associated peptide proteomics (MAPPs) revealed that numerous peptides derived from the hypervariable region of IgG were strongly presented (45). Surprisingly, Tregitopes derived from the constant region of IgG failed to suppress effector immune responses against a specific antigen in both human peripheral blood mononuclear cells and mouse immune cells (45). These results suggested that the immunomodulatory effects of polyvalent IgG were originated from the hypervariable region (idiotype) of IgG and not from the constant region of IgG (Tregiotopes) (Model 2, Figure 1B).
In humans, an author of this review (Nahm DH) reported that the intramuscular administration of autologous total IgG increased the percentage of IL-10-producing CD4+ T cells in the peripheral blood in 13 healthy human subjects (46). However, this report could not provide a detailed molecular mechanism underlying the autologous polyvalent IgG-mediated immunomodulation or the subpopulation of Treg cells activated by autologous polyvalent IgG.
This review provides a summary of results (subpopulation of Treg cells and mechanism) on the polyvalent IgG-induced activation of Treg cells from mouse studies (21, 42, 44, 47–51) (Table 2). Further studies are needed to evaluate the characteristics of polyvalent IgG-derived peptides activating Treg cells and subpopulations of Treg cells mediating polyvalent IgG-induced immunomodulation.
2.2 Hypothetical model 3 and 4: activation of Treg cells by direct binding of polyvalent IgG to surface receptors on T cells or APCs
Addition of polyvalent human IgG to purified CD4+CD25high T cells from healthy human subjects increased intracellular expression of IL-10 in CD4+CD25high T cells under experimental cell culture conditions; this observation indicated a direct activation of Treg cells by polyvalent IgG (20). To explain the mechanism underlying polyvalent IgG induced direct activation of T cells, the “hook without bait theory” has been proposed by an author of this review (Victor JR) (52). In this theory, direct binding of idiotype of polyvalent IgG to the TCRs or unidentified antigens on the surface of CD4+ cells can induce differentiation of Treg cells from naive T cells or activate pre-existing Treg cells to secrete IL-10 (Figure 1C). The direct interaction between polyvalent IgG and antigens on the cell membrane of CD4+ thymic T cells induced cytokine production from CD4+ thymic T cells (53). However, this study did not evaluate the activation of Treg cells by direct binding of polyvalent IgG to antigens on the cell membrane of T cells. In a co-culture model of human DCs and CD4+ T cells as well as in a mouse model of experimental autoimmune encephalomyelitis, IVIg was seen to increases Treg cells through the binding of F(ab′)2 fragments of IVIg with dendritic cell-specific intercellular adhesion molecule-3-grabbing nonintegrin (DC-SIGN) on DCs (54) (Figure 1D). A similar approach demonstrated that polyvalent IgG can specifically interact with murine DC immunoreceptors, favoring the induction of Treg cells (55). Another possibility is the direct binding of polyvalent IgG to an unidentified antigen (or conserved receptor) on the surface of APCs, and this interaction induces the changes of APCs into tolerogenic APCs, promoting the differentiation of naive CD4+ T cells into Treg cells and secretion of IL-10 (Figure 1D).
However, further studies are needed to validate these four hypothetical models of polyvalent IgG-induced Treg cell activation and the subpopulations of Treg cells (CD4+Foxp3+ T cells and/or Tr1 cells) involved in these processes.
3 Evidence supporting activation of Treg cells by polyvalent IgG in clinical trials
3.1 Evidence supporting activation of Treg cells upon intravenous administration of IVIg in human clinical trials
IVIg has been used as a replacement or immunomodulatory therapy in primary immunodeficiency, secondary immunodeficiency, hematological diseases, neuroimmunological diseases, rheumatic diseases, dermatological diseases, and other conditions including miscarriages (56). Clinical studies on IVIg therapy in primary immunodeficiency patients (57), the treatment of autoimmune rheumatic disease (58), Guillain-Barré syndrome (59), eosinophilic granulomatosis (60), Kawasaki disease (61), recurrent miscarriage (62), and repeated implantation failure (63) showed that IVIg therapy increased the percentage of Treg cells (mainly CD4+ Foxp3+ Treg cells) in the peripheral blood (Table 3). In a clinical trial, IVIg therapy in patients with common variable immunodeficiency significantly increased the number of peripheral Treg cells (expressing CD4, CD25, and low levels of CD127) and plasma levels of IL-10 within 30 minutes after the infusion of IVIg, suggesting a highly efficient interaction between polyvalent IgG and Treg cells (64). This review provides a summary of study results (subpopulation of Treg cells and mechanism) on the polyvalent IgG-induced activation of Treg cells in human clinical trials (Table 3) (46, 57–65).
3.2 Clinical application of “active anti-idiotypic therapy” with polyvalent IgG: lessons obtained from clinical trials on intramuscular injection of autologous total IgG in patients with atopic dermatitis
An author (Nahm DH) of this review has tried to develop a new anti-idiotypic therapy through intramuscular injection of autologous total IgG purified from autologous blood via affinity-chromatography using protein A bead in patients with atopic dermatitis (AD) and healthy human subjects (46, 66–70). From clinical trials in patients with AD and healthy human subjects, the novel concept of an “active anti-idiotypic therapy” was developed as described in the following sections.
3.2.1 Current knowledge on pathogenesis and treatment of atopic dermatitis
AD is an allergic skin disorder characterized by chronic allergic inflammation of the skin with clinical manifestations including itching, dry skin, exudation, and frequently associated with a personal or familial history of allergic diseases (2, 71, 72).
In patients with AD, allergic inflammation of the skin is characterized by the uptake of allergens by APCs that digest allergens and present peptides of the allergen to allergen-specific CD4+ T cells, which secrete Th2 cytokines (IL-4, IL-5, and IL-13) (73). Upon re-exposure, allergens activate Th2 cells to secrete Th2 cytokines, and allergens also bind to IgE antibodies on the mast cells and induce mast cell degranulation and release of chemical mediators, including histamine and other chemo-attractants. These cytokines and chemical mediators induce chronic allergic inflammation (Th2 inflammation) of the skin and clinical symptoms of AD (itching and eczema) (73).
Current topical and systemic immunosuppressants (corticosteroids, cyclosporine, tacrolimus, and methotrexate) nonspecifically suppress inflammatory immune cells and have limited clinical efficacy in patients with moderate-to-severe AD (2). Recently developed monoclonal antibodies to IL-4 receptor alpha or Janus kinase inhibitors suppressing Th2 cell-mediated inflammation demonstrated clinical improvements in a significant number of patients with moderate-to-severe AD (74–77). However, the clinical efficacies of current medical therapies for AD are transient and remain incomplete (71, 78). Therefore, developing a new therapeutic modality modifying the long-term clinical course of AD is needed.
3.2.2 Critical role of decreased number and/or function of Treg cells in the pathogenesis of atopic dermatitis
While Th2 cells may promote allergic inflammation, Treg cells can downregulate Th2 cell function and suppress allergic inflammations. T cell tolerance mediated by Treg cells is the key mechanism underlying immune tolerance in a healthy immune response to self and non-infectious foreign antigens. Immune dysfunction characterized by decreased Treg cell function (reflected by the decreased number and/or function of Treg cells) (79, 80) and excessive activation of Th2 cells producing IL-4 and IL-13 plays a key role in the pathogenesis of AD (2).
Therefore, immunomodulatory therapies to restore Treg cell number and/or function and Th1/Th2-cell balance might be a reasonable approach to induce a long-term treatment-free clinical remission in patients with AD (2, 81).
3.2.3 Immunomodulatory therapies that induced a long-term treatment-free clinical remission in patients with atopic dermatitis
3.2.3.1 Allergen immunotherapy
Allergen immunotherapy is a treatment that involves the repeated administration of sensitized allergens either subcutaneously or sublingually to induce allergen-specific immune tolerance in patients with allergic diseases (82, 83). Allergen immunotherapy is clinically beneficial in AD patients sensitized to house dust mites in a meta-analysis of multiple randomized clinical trials (84, 85). Clinical observational studies reported long-term treatment-free clinical remissions in patients with AD after allergen immunotherapy (86–88). Allergen immunotherapy activates allergen-specific Treg cells to downregulate Th2 cell- and IgE-mediated allergic inflammation. Treg cells are generated during allergen immunotherapy, secrete IL-10, and induce allergen-specific B cells to produce IgG4 antibodies (89). These mechanisms induce tolerance to antigens that reduce allergic symptoms. Induction of peripheral T cell tolerance by Treg cells is the key mechanism involved in allergen immunotherapy (90). Activated Treg cells release IL-10 and suppress Th2 cell-mediated allergic inflammation. However, there are still controversies regarding the main subpopulation of Treg cells mediating therapeutic effects involved in allergen immunotherapy (CD4+Foxp3+ Treg cells and/or CD4+Foxp3-IL-10+ Treg cells) (91).
3.2.3.2 Natural remission of disease in children with atopic dermatitis
More than 70% of children with AD experience natural remission of their disease before puberty (92); although the mechanism of natural remission of AD in children has not been determined yet, induction of immune tolerance due to activation of Treg cells has been suggested as a mechanism (2). Induction of immune tolerance mimicking the immunological mechanism responsible for the natural clinical remission of AD in children could be an ideal way to achieve clinical remission in patients with AD (2).
3.2.3.3 Intramuscular administration of autologous polyvalent IgG for induction of anti-idiotype immune modulation
An author of this review (Nahm DH) hypothesized that immunizing the human subjects with autologous immunoglobulin might induce Treg cell response with systemic immunomodulatory effects to prevent or treat AD. To evaluate this hypothesis, clinical trials were performed to evaluate the clinical efficacy and immunomodulatory effect of intramuscular administration of autologous polyvalent IgG (total IgG) purified from autologous blood using Protein A bead in patients with AD and healthy human subjects (46, 66–70).
In a randomized controlled trial including 51 patients with AD, 8 weekly intramuscular injections of autologous total IgG (50 mg) for 7 weeks resulted in significant clinical improvements and increased serum levels of IL-10 and interferon (IFN)-γ at week 16 (66). In this study, the percentages of IL-10- and IFN-γ-producing cells in the peripheral blood CD4+ T cells were increased after the intramuscular administration of autologous total IgG in patients with AD (66). These results suggested that intramuscular administration of low doses of autologous total IgG can activate Treg cells and Th1 cells producing IFN-γ and lead to systemic immunomodulatory effects in patients with AD (66).
In a previous clinical study, a long-term clinical improvement for more than nine months was observed in 2 of 3 patients with severe AD who received 8 intramuscular injections of autologous total IgG 50 mg (total 400 mg) for 4 weeks and were followed up for two years (69). In this study, two of three patients showed clinical improvement for more than 36 weeks after treatment with maximum decreases in clinical severity score greater than 80% from baseline. These two patients showed a long-term (more than 36 weeks) decrease in serum total IgE concentration and peripheral blood eosinophil count with maximum decreases in those values greater than 70% from baseline. No significant side effect was observed during the two years of follow-up period in all three patients (69). This observation suggests a potential of intramuscular administration of autologous total IgG in modifying the long-term disease course of AD.
Changes in T cells before and after intramuscular administration of autologous total IgG were evaluated in 13 healthy human subjects (46). Intramuscular administration of autologous total IgG (8 injections of autologous IgG 50 mg per 4 weeks; total 400 mg IgG) significantly increased the percentage of IL-10-producing CD4+ T cells in peripheral blood CD4+ T cells and the percentage of IFN-γ-producing CD3+ T cells in peripheral blood CD3+ T cells in healthy human subjects (46) (Table 3). These results suggest that intramuscular administration of autologous total IgG can activate Treg cells and IFN-γ-producing T cells in healthy subjects and that it could be a safe and effective method to activate Treg cells in human subjects (46). The significant limitations of these clinical studies are the lack of knowledge on the detailed molecular mechanism underlying Treg cell activation induced by autologous total IgG and the subpopulation of Treg cells (CD4+Foxp3+ Treg cells and/or CD4+Foxp3-IL-10+ Treg cells) activated by autologous total IgG.
An author of this review (Nahm DH) speculates that the intramuscular administration of autologous total IgG activates pre-existing anti-idiotypic Treg cells that can specifically recognize peptides derived from IgG idiotypes by their TCRs and produce IL-10, based on the observations in healthy human subjects after intramuscular injection of autologous total IgG (46). However, a direct experimental demonstration of the existence or development of anti-idiotypic Treg cells has not been provided yet. Therefore, an author (Nahm DH) of this review proposes that “anti-idiotypic Treg cell” is a missing scientific link that can integrate both “idiotypic network theory” and “Treg cell theory” to explain the mechanism underlying the maintenance of an immune tolerance state (Tables 1, 4).
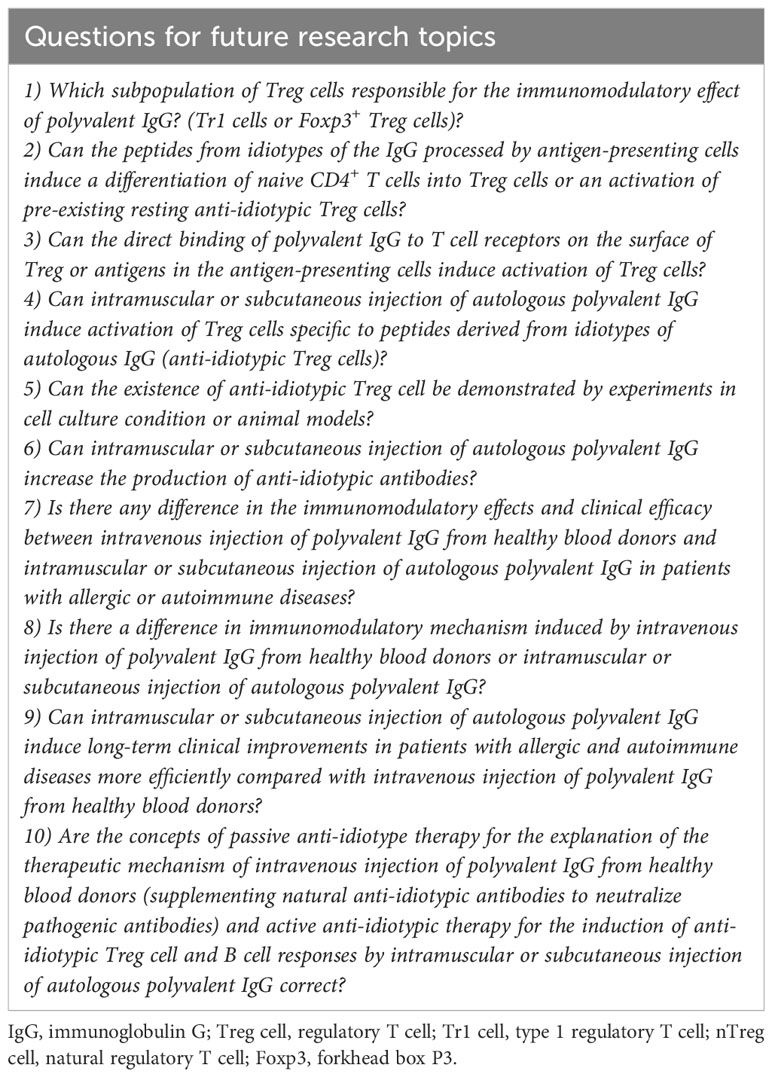
Table 4 Ten future research topics on the detailed mechanism underlying polyvalent IgG-induced activation of regulatory T cells and its clinical application.
3.2.3.4 Comparison of efficacy and mechanism of intravenous injection of heterologous polyvalent IgG and intramuscular injection of autologous total IgG in patients with atopic dermatitis
Expansion of CD4+ Foxp3+ Treg cells in the peripheral blood has been reported in patients with autoimmune diseases after intravenous administration of high-dose (1~2 g/Kg of body weight) heterologous IVIg (60, 65, 93). Continuous regular intravenous infusion of a high dose of polyvalent human IgG is needed to achieve a long-term clinical improvement in patients with autoimmune diseases (60, 91, 93). This requirement of continuous treatment has been attributed to the low concentration and low affinity of natural anti-idiotype antibodies to pathogenic autoantibodies contained in IVIg (17). Clinical trials on high-dose intravenous polyvalent IgG therapy (1~2 g/Kg of body weight per patient) in adult patients with severe AD showed no significant clinical benefit (93). However, intramuscularly administered low doses of purified autologous total IgG (8 intramuscular injections of autologous total IgG 50 mg for 4 weeks) produced potent immunomodulatory effects (increased serum levels of IL-10 and IFN- γ) and long-term clinical improvement compared to intravenous administration of a high dose of heterologous IgG (1~2 g/Kg of body weight per a patient) in adolescent and adult patients with AD (66, 93). These conflicting results from clinical trials on the intravenous administration of heterologous IgG and intramuscular administration of autologous IgG in patients with AD suggest that differences in the origin of polyvalent IgG (heterologous vs. autologous) and route of administration of polyvalent IgG (intravenous vs. intramuscular) can result in different immunomodulatory effects and clinical efficacy (66).
If polyvalent IgG is used as an “antibody” to neutralize pathogenic antibodies (IgG autoantibodies or IgE antibodies), intravenous administration of polyvalent IgG can be the preferred route of administration (66). However, if polyvalent IgG is used as an “antigen” to activate anti-idiotypic Treg cells in patients with allergic and autoimmune diseases, the intramuscular or subcutaneous administration route might be an ideal route of administration. A specific antigen administered through intravenous injection preferentially interacts with mononuclear cells in the peripheral blood, and a specific antigen administered through intramuscular or subcutaneous injection preferentially interacts with DCs (66).
Intramuscularly administered autologous polyvalent IgG may more efficiently activate pre-existing idiotype-specific Treg cells than with intramuscularly administered heterologous IgG because the pre-existence of idiotype-specific T cell to autologous IgG is an essential prerequisite of the “idiotype-network theory.” The potency of activation of Treg cells induced through intramuscular injection of heterologous polyvalent IgG can be weak because of a smaller number of pre-existing idiotype-specific Treg cells than that in case of intramuscular injection of autologous polyvalent IgG.
Further studies are necessary to evaluate the detailed mechanisms underlying systemic immunomodulation induced by intravenous or intramuscular administration of heterologous or autologous human IgG, especially on the mechanism of anti-idiotypic immunomodulation.
3.3 In vitro and animal studies about IgG-mediated anti-idiotypic immuno-modulation: lessons from experiments using IgG from donors with various immune backgrounds
3.3.1 Immunomodulatory effects induced by polyvalent IgG from donors with various immune backgrounds
In a mouse model of OVA-allergy, the passive transference of polyclonal IgG from allergen-immunized donors to non-immunized pregnant mice increased IL-10-producing CD4+ T cells in the offspring at birth and prevented neonatal OVA-sensitization (47). This effect occurs in the absence of maternal antigen immunization, suggesting that the in vivo effect was mediated by idiotypic interactions between the polyvalent IgG from allergen-immunized donors administered to the mother during pregnancy and the fetus lymphocytes (47).
A study suggested that IgG also permeates cells and interacts with intracellular molecules, inhibiting T cell activation after its interaction with nuclear and cytoplasmic components (94). These cell-penetrating antibodies, which could be detected in polyvalent IgG formulations, penetrate various mammalian cell lines and represent ~2% of polyvalent IgG. This possibility also needs to be discussed because it suggests that anti-idiotype IgG may interact with TCRs intracellularly, at an early stage of production of these receptors.
3.3.2 Experimental observations regarding IgG from patients with atopic dermatitis
It has been demonstrated that AD patients can produce detectable levels of anti-IgE autoantibodies in the IgG class (95) and that these antibodies can mediate inflammatory mediators released from basophils and mast cells (96). This observation was confirmed by two studies which showed that the functional activity of IgG anti-IgE autoantibodies from patients with AD induced the release of pro-inflammatory mediators and cytokines from human basophils and mast cells (97, 98).
Human thymic lymphocyte culture with purified IgG showed that pooled IgG from patients with AD could more effectively modulate cytokine production from thymic CD4+ T cells than did polyvalent IgG from healthy human subjects (IVIg) (99). This study demonstrates that IgG from patients with AD induced significantly higher production of IL-17 and IL-10 by intrathymic immature double positive and mature CD4+ T cells than did polyvalent IgG from healthy individuals (99). In a similar in vitro experiment, pooled IgG from patients with AD could induce thymic invariant natural killer T cells (CD1d-restricted T cells that employ an invariant TCR alpha chain and a limited repertoire of beta chains) to produce higher levels of intracellular IL-4, IL-10, and IL-17 than did polyvalent IgG from healthy non-atopic individuals (100). The IgG-reactivity toward 1152 human protein fragments was evaluated in 80 individuals (AD patients and healthy controls), and a significant differential IgG-reactivity to four antigens representing keratin-associated protein 17-1 (KRTAP17-1), heat shock protein family A member 4 (HSPA4), S100 calcium-binding proteins A12, and Z (S100A12 and S100Z) was detected (101). The reactivity to these four antigens was more frequent in the patients with severe AD (66%) than in patients with moderate AD (41%) and healthy controls (25%) (101). These observations suggest that purified IgG from patients with AD can modulate T cells more efficiently than does polyvalent IgG from healthy human subjects (IVIg) (99, 100, 102).
4 Six questions on the mechanism underlying polyvalent IgG-induced activation of Treg cells and its clinical application for the treatment of immune diseases
We asked six critical questions regarding the mechanism and its clinical application of polyvalent IgG-induced immune modulation and activation of Treg cells (Table 1). We propose one hypothesis to provide an answer to these six questions regarding the mechanism of polyvalent IgG induced activation of Treg cells and its clinical application (Box 1). These six questions and one hypothesis should be validated in future studies.
In this hypothesis, subcutaneous or intramuscular injection of autologous polyvalent IgG can induce activation of anti-idiotypic Treg cells to downregulate the production of pathogenic antibodies and exaggerated T cell responses harmful to the host and induce an immune tolerance state and ultimately induce a long-term clinical remission in patients with autoimmune and allergic diseases (Box 1). Through the perspective of the idiotypic network theory, immunization with autologous polyvalent IgG might provide a more potent immunomodulatory effects than that provided by heterologous polyvalent IgG in the treatment of autoimmune and allergic diseases.
Box 1. A new concept of active anti-idiotypic therapy with autologous polyvalent IgG for allergic and autoimmune diseases.
“Subcutaneous or intramuscular administration of autologous polyvalent IgG can activate anti-idiotypic regulatory T cells that can specifically recognize immunogenic peptides generated from idiotypes of autologous IgG by dendritic cells. The activated anti-idiotypic regulatory T cells can induce an immune tolerance state (recovery of immune homeostasis) and a long-term treatment-free clinical remission in patients with allergic and autoimmune diseases.”
IgG, immunoglobulin G.
5 Future research directions (research topics) involving the mechanism and clinical application of polyvalent IgG-induced activation of Treg cells
Polyvalent IgG can induce activation of Treg cells to produce IL-10 and exert a systemic immunomodulatory effect and clinical efficacy in patients with allergic and autoimmune diseases. However, this review also exposed a lack of current knowledge regarding the mechanism of polyvalent IgG-induced immunomodulation including Treg cell activation and its role in the development and maintenance of immune tolerance. Therefore, we provide our hypotheses (unproved speculations on the detailed mechanism) to promote further research on this important but neglected research topic by other colleagues. For future investigations, we propose research topics to answer questions on the detailed mechanism and clinical application of polyvalent IgG-induced immunomodulation (Table 4).
6 Conclusion
Experimental studies and clinical trials involving Treg cells suggest that polyvalent IgG acts as a stimulator of Treg cells. However, the mechanism underlying the activation of Treg cells by polyvalent IgG is not defined yet. We hypothesize that anti-idiotypic Treg cells can be activated via intramuscular or subcutaneous injection of autologous polyvalent IgG because idiotypes of polyvalent IgG can act as antigens. The activated anti-idiotypic Treg cells secrete IL-10, and IL-10 suppresses Th2 cell responses to allergens and autoimmune T cell responses to self-antigens and thus can induce a long-term clinical remission of allergic and autoimmune diseases. Further studies are needed to evaluate the detailed molecular mechanism underlying polyvalent IgG-induced Treg cell activation and the clinical usefulness of this immunomodulatory strategy for the treatment of allergic and autoimmune diseases.
Author contributions
All authors contributed in writing and revising the manuscript. DN: Conceptualization, Funding acquisition, Project administration, Supervision, Writing – original draft, Writing – review & editing. JV: Funding acquisition, Writing – original draft, Writing – review & editing. All authors contributed to the article and approved the submitted version.
Funding
The authors declare financial support was received for the research, authorship, and/or publication of this article. This work was supported by Basic Science Research Program through the National Research Foundation of Korea (NRF) funded by the Ministry of Education (2018R1D1A1B07045762), by the Laboratory of Medical Investigation-56, Medical School, University of São Paulo, São Paulo, Brazil (LIM-56 HC-FMUSP), by the National Council for Scientific and Technological Development (CNPq) grant# 302937/2021-8, and by the São Paulo Research Foundation (FAPESP) grant #2021/08225-8.
Acknowledgments
This collaborative work was started by DN’s initial email contact and suggestion to JV.
Conflict of interest
The authors declare that the research was conducted in the absence of any commercial or financial relationships that could be construed as a potential conflict of interest.
Publisher’s note
All claims expressed in this article are solely those of the authors and do not necessarily represent those of their affiliated organizations, or those of the publisher, the editors and the reviewers. Any product that may be evaluated in this article, or claim that may be made by its manufacturer, is not guaranteed or endorsed by the publisher.
References
1. Sakaguchi S, Yamaguchi T, Nomura T, Ono M. Regulatory T cells and immune tolerance. Cell (2008) 133(5):775–87. doi: 10.1016/j.cell.2008.05.009
2. Nahm DH. Personalized immunomodulatory therapy for atopic dermatitis: an allergist's view. Ann Dermatol (2015) 27(4):355–63. doi: 10.5021/ad.2015.27.4.355
3. Schmitt EG, Williams CB. Generation and function of induced regulatory T cells. Front Immunol (2013) 4:152. doi: 10.3389/fimmu.2013.00152
4. Sakaguchi S, Mikami N, Wing JB, Tanaka A, Ichiyama K, Ohkura N. Regulatory T cells and human disease. Annu Rev Immunol (2020) 38:541–66. doi: 10.1146/annurev-immunol-042718-041717
5. Ferreira LMR, Muller YD, Bluestone JA, Tang Q. Next-generation regulatory T cell therapy. Nat Rev Drug Discovery (2019) 18(10):749–69. doi: 10.1038/s41573-019-0041-4
6. Fontenot JD, Gavin MA, Rudensky AY. FOXP3 programs the development and function of CD4+ CD25+ regulatory T cells. Nat Immunol (2003) 4(4):330–6. doi: 10.1038/ni904
7. Riemann M, Andreas N, Fedoseeva M, Meier E, Weih D, Freytag H, et al. Central immune tolerance depends on crosstalk between the classical and alternative NF-κB pathways in medullary thymic epithelial cells. J Autoimmun (2017) 81:56–67. doi: 10.1016/j.jaut.2017.03.007
8. Xing Y, Hogquist KA. T-cell tolerance: central and peripheral. Cold Spring Harbor Perspect Biol (2012) 4(6):a006957. doi: 10.1101/cshperspect.a006957
9. Akdis CA, Akdis M, Blesken T, Wymann D, Alkan SS, Muller U, et al. Epitope-specific T cell tolerance to phospholipase A2 in bee venom immunotherapy and recovery by IL-2 and IL-15 in vitro. J Clin Invest (1996) 98(7):1676–83. doi: 10.1172/JCI118963
10. Akdis CA, Blesken T, Akdis M, Wuthrich B, Blaser K. Role of interleukin 10 in specific immunotherapy. J Clin Invest (1998) 102(1):98–106. doi: 10.1172/JCI2250
11. Francis JN, Till SJ, Durham SR. Induction of IL-10+CD4+CD25+ T cells by grass pollen immunotherapy. J Allergy Clin Immunol (2003) 111(6):1255–61. doi: 10.1067/mai.2003.1570
12. Jutel M, Akdis M, Budak F, Aebischer-Casaulta C, Wrzyszcz M, Blaser K, et al. IL-10 and TGF-beta cooperate in the regulatory T cell response to mucosal allergens in normal immunity and specific immunotherapy. Eur J Immunol (2003) 33(5):1205–14. doi: 10.1002/eji.200322919
13. Shevach EM, Thornton AM. tTregs, pTregs, and iTregs: similarities and differences. Immunol Rev (2014) 259(1):88–102. doi: 10.1111/imr.12160
14. Jolles S, Sewell WA, Misbah SA. Clinical uses of intravenous immunoglobulin. Clin Exp Immunol (2005) 142(1):1–11. doi: 10.1111/j.1365-2249.2005.02834.x
15. Buckley RH, Schiff RI. The use of intravenous immune globulin in immunodeficiency diseases. N Engl J Med (1991) 325(2):110–7. doi: 10.1056/NEJM199107113250207
16. Kazatchkine MD, Kaveri SV. Immunomodulation of autoimmune and inflammatory diseases with intravenous immune globulin. N Engl J Med (2001) 345(10):747–55. doi: 10.1056/NEJMra993360
17. Gelfand EW. Intravenous immune globulin in autoimmune and inflammatory diseases. N Engl J Med (2012) 367(21):2015–25. doi: 10.1056/NEJMra1009433
18. Galeotti C, Kaveri SV, Bayry J. IVIG-mediated effector functions in autoimmune and inflammatory diseases. Int Immunol (2017) 29(11):491–8. doi: 10.1093/intimm/dxx039
19. Kessel A, Ammuri H, Peri R, Pavlotzky ER, Blank M, Shoenfeld Y, et al. Intravenous immunoglobulin therapy affects T regulatory cells by increasing their suppressive function. J Immunol (2007) 179(8):5571–5. doi: 10.4049/jimmunol.179.8.5571
20. Maddur MS, Kaveri SV, Bayry J. Circulating normal IgG as stimulator of regulatory T cells: lessons from intravenous immunoglobulin. Trends Immunol (2017) 38(11):789–92. doi: 10.1016/j.it.2017.08.008
21. Ephrem A, Chamat S, Miquel C, Fisson S, Mouthon L, Caligiuri G, et al. Expansion of CD4+ CD25+ Regulatory T cells by intravenous immunoglobulin: A critical factor in controlling experimental autoimmune encephalomyelitis. Blood (2008) 111(2):715–22. doi: 10.1182/blood-2007-03-079947
22. Lories RJ, Casteels-Van Daele M, Ceuppens JL, Van Gool SW. Polyclonal immunoglobulins for intravenous use induce interleukin 10 release in vivo and in vitro. Ann Rheum Dis (2004) 63(6):747–8. doi: 10.1136/ard.2003.007138
23. Campbell IK, Miescher S, Branch DR, Mott PJ, Lazarus AH, Han D, et al. Therapeutic effect of IVIG on inflammatory arthritis in mice is dependent on the FC portion and independent of sialylation or basophils. J Immunol (2014) 192(11):5031–8. doi: 10.4049/jimmunol.1301611
24. Samuelsson A, Towers TL, Ravetch JV. Anti-inflammatory activity of IVIG mediated through the inhibitory FC receptor. Science (2001) 291(5503):484–6. doi: 10.1126/science.291.5503.484
25. Yu X, Vasiljevic S, Mitchell DA, Crispin M, Scanlan CN. Dissecting the molecular mechanism of IVIG therapy: the interaction between serum IgG and DC-SIGN is independent of antibody glycoform or FC domain. J Mol Biol (2013) 425(8):1253–8. doi: 10.1016/j.jmb.2013.02.006
26. Jain A, Olsen HS, Vyzasatya R, Burch E, Sakoda Y, Mérigeon EY, et al. Fully recombinant IgG2a Fc multimers (Stradomers) effectively treat collagen-induced arthritis and prevent idiopathic thrombocytopenic purpura in mice. Arthritis Res Ther (2012) 14(4):R192. doi: 10.1186/ar4024
27. Thiruppathi M, Sheng JR, Li L, Prabhakar BS, Meriggioli MN. Recombinant IgG2a Fc (M045) multimers effectively suppress experimental autoimmune myasthenia gravis. J Autoimmun (2014) 52:64–73. doi: 10.1016/j.jaut.2013.12.014
28. Zhang X, Owens J, Olsen HS, So E, Burch E, McCroskey MC, et al. A recombinant human IgG1 Fc multimer designed to mimic the active fraction of IVIG in autoimmunity. JCI Insight (2019) 4(2):e121905. doi: 10.1172/jci.insight.121905
29. Niknami M, Wang MX, Nguyen T, Pollard JD. Beneficial effect of a multimerized immunoglobulin Fc in an animal model of inflammatory neuropathy (Experimental autoimmune neuritis). J Peripher Nerv Syst (2013) 18(2):141–52. doi: 10.1111/jns5.12022
30. Debré M, Bonnet MC, Fridman WH, Carosella E, Philippe N, Reinert P, et al. Infusion of Fc gamma fragments for treatment of children with acute immune thrombocytopenic purpura. Lancet (1993) 342(8877):945–9. doi: 10.1016/0140-6736(93)92000-j
31. Käsermann F, Boerema DJ, Rüegsegger M, Hofmann A, Wymann S, Zuercher AW, et al. Analysis and functional consequences of increased Fab-sialylation of intravenous immunoglobulin (IVIG) after lectin fractionation. PloS One (2012) 7(6):e37243. doi: 10.1371/journal.pone.0037243
32. Ballow M. Mechanisms of immune regulation by IVIG. Curr Opin Allergy Clin Immunol (2014) 14(6):509–15. doi: 10.1097/ACI.0000000000000116
33. Seite J-F, Shoenfeld Y, Youinou P, Hillion S. What is the contents of the magic draft IVIg? Autoimmun Rev (2008) 7(6):435–9. doi: 10.1016/j.autrev.2008.04.012
34. Fuchs S, Feferman T, Meidler R, Brenner T, Laub O, Souroujon MC. The disease-specific arm of the therapeutic effect of intravenous immunoglobulin in autoimmune diseases: experimental autoimmune myasthenia gravis as a model. Isr Med Assoc J (2008) 10(1):58–60.
35. Wallmann J, Pali-Scholl I, Jensen-Jarolim E. Anti-ids in allergy: timeliness of a classic concept. World Allergy Organ J (2010) 3(6):195–201. doi: 10.1097/WOX.0b013e3181e61ebf
36. Shoenfeld Y. The idiotypic network in autoimmunity: antibodies that bind antibodies that bind antibodies. Nat Med (2004) 10(1):17–8. doi: 10.1038/nm0104-17
37. Jerne NK. Towards a network theory of the immune system. Ann Immunol (Paris) (1974) 125C(1-2):373–89.
38. Lopez-Requena A, Mateo De Acosta C, Vazquez AM, Perez R. Immunogenicity of autologous immunoglobulins: principles and practices. Mol Immunol (2007) 44(11):3076–82. doi: 10.1016/j.molimm.2007.01.005
39. Schulz R, Werner B, Behn U. Self-tolerance in a minimal model of the idiotypic network. Front Immunol (2014) 5:86. doi: 10.3389/fimmu.2014.00086
40. Guermonprez P, Valladeau J, Zitvogel L, Thery C, Amigorena S. Antigen presentation and T cell stimulation by dendritic cells. Annu Rev Immunol (2002) 20:621–67. doi: 10.1146/annurev.immunol.20.100301.064828
41. Kedzierska AE, Lorek D, Slawek A, Chelmonska-Soyta A. Tregitopes regulate the tolerogenic immune response and decrease the foetal death rate in abortion-prone mouse matings. Sci Rep (2020) 10(1):10531. doi: 10.1038/s41598-020-66957-z
42. Massoud AH, Kaufman GN, Xue D, Beland M, Dembele M, Piccirillo CA, et al. Peripherally generated FOXP3(+) regulatory T cells mediate the immunomodulatory effects of IVIg in allergic airways disease. J Immunol (2017) 198(7):2760–71. doi: 10.4049/jimmunol.1502361
43. Dembele M, Tao S, Massoud AH, Miah SMS, Lelias S, De Groot AS, et al. Tregitopes improve asthma by promoting highly suppressive and antigen-specific Tregs. Front Immunol (2021) 12:634509. doi: 10.3389/fimmu.2021.634509
44. Sorde L, Spindeldreher S, Palmer E, Karle A. Massive immune response against IVIg interferes with response against other antigens in mice: a new mode of action? PloS One (2017) 12(10):e0186046. doi: 10.1371/journal.pone.0186046
45. Sordé L, Spindeldreher S, Palmer E, Karle A. Tregitopes and impaired antigen presentation: Drivers of the immunomodulatory effects of IVIg? Immun Inflammation Dis (2017) 5(4):400–15. doi: 10.1002/iid3.167
46. Kwon B, Yang SJ, Cho SM, Kim ME, Nahm DH. Intramuscular administration of autologous total immunoglobulin G induces immunomodulatory effects on T cells in healthy human subjects: An open-labeled prospective single-arm trial. Med (Baltimore) (2022) 101(22):e29486. doi: 10.1097/MD.0000000000029486
47. Victor JR, Muniz BP, Fusaro AE, de Brito CA, Taniguchi EF, Duarte AJ, et al. Maternal immunization with ovalbumin prevents neonatal allergy development and up-regulates inhibitory receptor Fc gamma RIIB expression on B cells. BMC Immunol (2010) 11:11. doi: 10.1186/1471-2172-11-11
48. Lee SY, Jung YO, Ryu JG, Kang CM, Kim EK, Son HJ, et al. Intravenous immunoglobulin attenuates experimental autoimmune arthritis by inducing reciprocal regulation of Th17 and Treg cells in an interleukin-10-dependent manner. Arthritis Rheumatol (2014) 66(7):1768–78. doi: 10.1002/art.38627
49. Ramakrishna C, Newo AN, Shen YW, Cantin E. Passively administered pooled human immunoglobulins exert IL-10 dependent anti-inflammatory effects that protect against fatal HSV encephalitis. PloS Pathog (2011) 7(6):e1002071. doi: 10.1371/journal.ppat.1002071
50. Massoud AH, Guay J, Shalaby KH, Bjur E, Ablona A, Chan D, et al. Intravenous immunoglobulin attenuates airway inflammation through induction of forkhead box protein 3–positive regulatory T cells. J Allergy Clin Immunol (2012) 129(6):1656–65. e3. doi: 10.1016/j.jaci.2012.02.050
51. Othy S, Hegde P, Topçu S, Sharma M, Maddur MS, Lacroix-Desmazes S, et al. Intravenous gammaglobulin inhibits encephalitogenic potential of pathogenic T cells and interferes with their trafficking to the central nervous system, implicating sphingosine-1 phosphate receptor 1–mammalian target of rapamycin axis. J Immunol (2013) 190(9):4535–41. doi: 10.4049/jimmunol.1201965
52. Victor JR. Do different IgG repertoires play a role in B-and T-cell functional modulation during ontogeny? The “hooks without bait” theory. Immunol Cell Biol (2020) 98(7):540–8. doi: 10.1111/imcb.12335
53. de Sousa TR, Fagundes BO, Nascimento A, Fernandes LA, Sgnotto FDR, Orfali RL, et al. IgG from adult atopic dermatitis (AD) patients induces thymic IL-22 production and CLA expression on CD4+ T cells: Possible epigenetic implications mediated by miRNA. Int J Mol Sci (2022) 23(12):6867. doi: 10.3390/ijms23126867
54. Trinath J, Hegde P, Sharma M, Maddur MS, Rabin M, Vallat JM, et al. Intravenous immunoglobulin expands regulatory T cells via induction of cyclooxygenase-2-dependent prostaglandin E2 in human dendritic cells. Blood (2013) 122(8):1419–27. doi: 10.1182/blood-2012-11-468264
55. Massoud AH, Yona M, Xue D, Chouiali F, Alturaihi H, Ablona A, et al. Dendritic cell immunoreceptor: A novel receptor for intravenous immunoglobulin mediates induction of regulatory T cells. J Allergy Clin Immunol (2014) 133(3):853–63.e5. doi: 10.1016/j.jaci.2013.09.029
56. Negi VS, Elluru S, Sibéril S, Graff-Dubois S, Mouthon L, Kazatchkine MD, et al. Intravenous immunoglobulin: an update on the clinical use and mechanisms of action. J Clin Immunol (2007) 27(3):233–45. doi: 10.1007/s10875-007-9088-9
57. Abbott JK, Chan SK, MacBeth M, Crooks JL, Hancock C, Knight V, et al. Fluctuations in quality of life and immune responses during intravenous immunoglobulin infusion cycles. PloS One (2022) 17(3):e0265852. doi: 10.1371/journal.pone.0265852
58. Bayry J, Mouthon L, Kaveri SV. Intravenous immunoglobulin expands regulatory T cells in autoimmune rheumatic disease. J Rheumatol (2012) 39(2):450–1. doi: 10.3899/jrheum.111123
59. Maddur MS, Rabin M, Hegde P, Bolgert F, Guy M, Vallat JM, et al. Intravenous immunoglobulin exerts reciprocal regulation of Th1/Th17 cells and regulatory T cells in Guillain-barré syndrome patients. Immunol Res (2014) 60(2-3):320–9. doi: 10.1007/s12026-014-8580-6
60. Tsurikisawa N, Saito H, Oshikata C, Tsuburai T, Akiyama K. High-dose intravenous immunoglobulin treatment increases regulatory T cells in patients with eosinophilic granulomatosis with polyangiitis. J Rheumatol (2012) 39(5):1019–25. doi: 10.3899/jrheum.110981
61. Olivito B, Taddio A, Simonini G, Massai C, Ciullini S, Gambineri E, et al. Defective FOXP3 expression in patients with acute Kawasaki disease and restoration by intravenous immunoglobulin therapy. Clin Exp Rheumatol (2010) 28(1 Suppl 57):93–7.
62. Jafarzadeh S, Ahmadi M, Dolati S, Aghebati-Maleki L, Eghbal-Fard S, Kamrani A, et al. Intravenous immunoglobulin G treatment increases live birth rate in women with recurrent miscarriage and modulates regulatory and exhausted regulatory T cells frequency and function. J Cell Biochem (2019) 120(4):5424–34. doi: 10.1002/jcb.27821
63. Ahmadi M, Abdolmohammadi-Vahid S, Ghaebi M, Aghebati-Maleki L, Dolati S, Farzadi L, et al. Regulatory T cells improve pregnancy rate in RIF patients after additional IVIG treatment. Syst Biol Reprod Med (2017) 63(6):350–9. doi: 10.1080/19396368.2017.1390007
64. Kasztalska K, Ciebiada M, Cebula-Obrzut B, Gorski P. Intravenous immunoglobulin replacement therapy in the treatment of patients with common variable immunodeficiency disease: an open-label prospective study. Clin Drug Investig (2011) 31(5):299–307. doi: 10.1007/BF03256928
65. Wang SC, Yang KD, Lin CY, Huang AY, Hsiao CC, Lin MT, et al. Intravenous immunoglobulin therapy enhances suppressive regulatory T cells and decreases innate lymphoid cells in children with immune thrombocytopenia. Pediatr Blood Cancer (2020) 67(2):e28075. doi: 10.1002/pbc.28075
66. Nahm DH, Ye YM, Shin YS, Park HS, Kim ME, Kwon B, et al. Efficacy, safety, and immunomodulatory effect of the intramuscular administration of autologous total immunoglobulin G for atopic dermatitis: A randomized clinical trial. Allergy Asthma Immunol Res (2020) 12(6):949–63. doi: 10.4168/aair.2020.12.6.949
67. Nahm DH, Cho SM, Kim ME, Kim YJ, Jeon SY. Autologous immunoglobulin therapy in patients with severe recalcitrant atopic dermatitis: A preliminary report. Allergy Asthma Immunol Res (2014) 6(1):89–94. doi: 10.4168/aair.2014.6.1.89
68. Nahm DH, Kim ME, Cho SM. Effects of intramuscular injection of autologous immunoglobulin on clinical severity and serum IgE concentration in patients with atopic dermatitis. Dermatology (2015) 231(2):145–51. doi: 10.1159/000431173
69. Nahm DH, Ahn A, Kim ME, Cho SM, Park MJ. Autologous immunoglobulin therapy in patients with severe recalcitrant atopic dermatitis: long-term changes of clinical severity and laboratory parameters. Allergy Asthma Immunol Res (2016) 8(4):375–82. doi: 10.4168/aair.2016.8.4.375
70. Cho SM, Kim ME, Kwon B, Nahm DH. Immunomodulatory effects induced by intramuscular administration of autologous total immunoglobulin G in patients with atopic dermatitis. Int Immunopharmacol (2017) 52:1–6. doi: 10.1016/j.intimp.2017.08.020
71. Weidinger S, Novak N. Atopic dermatitis. Lancet (2016) 387(10023):1109–22. doi: 10.1016/S0140-6736(15)00149-X
72. Guttman-Yassky E, Dhingra N, Leung DY. New era of biologic therapeutics in atopic dermatitis. Expert Opin Biol Ther (2013) 13(4):549–61. doi: 10.1517/14712598.2013.758708
73. Broide DH. Immunomodulation of allergic disease. Annu Rev Med (2009) 60:279–91. doi: 10.1146/annurev.med.60.041807.123524
74. Wang FP, Tang XJ, Wei CQ, Xu LR, Mao H, Luo FM. Dupilumab treatment in moderate-to-severe atopic dermatitis: A systematic review and meta-analysis. J Dermatol Sci (2018) 90(2):190–8. doi: 10.1016/j.jdermsci.2018.01.016
75. Agache I, Song Y, Posso M, Alonso-Coello P, Rocha C, Sola I, et al. Efficacy and safety of dupilumab for moderate-to-severe atopic dermatitis: A systematic review for the EAACI biologicals guidelines. Allergy (2021) 76(1):45–58. doi: 10.1111/all.14510
76. Sedeh FB, Henning MAS, Jemec GBE, Ibler KS. Comparative efficacy and safety of monoclonal antibodies and Janus Kinase Inhibitors in moderate-to-severe atopic dermatitis: A systematic review and meta-analysis. Acta Derm Venereol (2022) 102:adv00764. doi: 10.2340/actadv.v102.2075
77. Werfel T. Novel systemic drugs in treatment of atopic dermatitis: results from phase II and phase III studies published in 2017/2018. Curr Opin Allergy Clin Immunol (2018) 18(5):432–7. doi: 10.1097/ACI.0000000000000477
78. Nankervis H, Thomas K, Delamere FM, Barbarot S, Smith S, Rogers NK, et al. What is the evidence base for atopic eczema treatments? A summary of published randomized controlled trials. Br J Dermatol (2017) 176(4):910–27. doi: 10.1111/bjd.14999
79. Agrawal R, Wisniewski JA, Woodfolk JA. The role of regulatory T cells in atopic dermatitis. Curr Probl Dermatol (2011) 41:112–24. doi: 10.1159/000323305
80. Samochocki Z, Alifier M, Bodera P, Jeziorkowska R, Rosiak E, Jurkiewicz B, et al. T-regulatory cells in severe atopic dermatitis: alterations related to cytokines and other lymphocyte subpopulations. Arch Dermatol Res (2012) 304(10):795–801. doi: 10.1007/s00403-012-1290-9
81. Eyerich K, Novak N. Immunology of atopic eczema: overcoming the Th1/Th2 paradigm. Allergy (2013) 68(8):974–82. doi: 10.1111/all.12184
82. Bousquet J, Lockey R, Malling HJ. Allergen immunotherapy: therapeutic vaccines for allergic diseases. A who position paper. J Allergy Clin Immunol (1998) 102(4 Pt 1):558–62. doi: 10.1016/s0091-6749(98)70271-4
83. Berings M, Karaaslan C, Altunbulakli C, Gevaert P, Akdis M, Bachert C, et al. Advances and highlights in allergen immunotherapy: on the way to sustained clinical and immunologic tolerance. J Allergy Clin Immunol (2017) 140(5):1250–67. doi: 10.1016/j.jaci.2017.08.025
84. Bae JM, Choi YY, Park CO, Chung KY, Lee KH. Efficacy of allergen-specific immunotherapy for atopic dermatitis: A systematic review and meta-analysis of randomized controlled trials. J Allergy Clin Immunol (2013) 132(1):110–7. doi: 10.1016/j.jaci.2013.02.044
85. Yepes-Nunez JJ, Guyatt GH, Gomez-Escobar LG, Perez-Herrera LC, Chu AWL, Ceccaci R, et al. Allergen immunotherapy for atopic dermatitis: systematic review and meta-analysis of benefits and harms. J Allergy Clin Immunol (2023) 151(1):147–58. doi: 10.1016/j.jaci.2022.09.020
86. Chait I, Allkins V. Remission of life-long atopic dermatitis after hyposensitisation to house dust mite. Practitioner (1985) 229(1405):609–12.
87. Leroy BP, Lachapelle J-M, Somville M, Jacquemin M, Saint-Remy J. Injection of allergen-antibody complexes is an effective treatment of atopic dermatitis. Dermatology (1991) 182(2):98–106. doi: 10.1159/000247754
88. Tuft L. Studies in atopic dermatitis: V. Problems in inhalant hyposensitization and results of treatment. J Allergy (1960) 31(1):1–11. doi: 10.1016/0021-8707(60)90019-8
89. Jutel M, Agache I, Bonini S, Burks AW, Calderon M, Canonica W, et al. International consensus on allergen immunotherapy II: mechanisms, standardization, and pharmacoeconomics. J Allergy Clin Immunol (2016) 137(2):358–68. doi: 10.1016/j.jaci.2015.12.1300
90. Jutel M, Akdis M, Blaser K, Akdis CA. Mechanisms of allergen specific immunotherapy–T-cell tolerance and more. Allergy (2006) 61(7):796–807. doi: 10.1111/j.1398-9995.2006.01175.x
91. Matsuda M, Terada T, Kitatani K, Kawata R, Nabe T. Roles of type 1 regulatory T (Tr1) cells in allergen-specific immunotherapy. Front Allergy (2022) 3:981126. doi: 10.3389/falgy.2022.981126
92. Hua TC, Hwang CY, Chen YJ, Chu SY, Chen CC, Lee DD, et al. The natural course of early-onset atopic dermatitis in Taiwan: A population-based cohort study. Br J Dermatol (2014) 170(1):130–5. doi: 10.1111/bjd.12603
93. Perez EE, Orange JS, Bonilla F, Chinen J, Chinn IK, Dorsey M, et al. Update on the use of immunoglobulin in human disease: A review of evidence. J Allergy Clin Immunol (2017) 139(3S):S1–S46. doi: 10.1016/j.jaci.2016.09.023
94. Sali AD, Karakasiliotis I, Evangelidou M, Avrameas S, Lymberi P. Immunological evidence and regulatory potential for cell-penetrating antibodies in intravenous immunoglobulin. Clin Transl Immunol (2015) 4(10):e42. doi: 10.1038/cti.2015.18
95. Nawata Y, Koike T, Hosokawa H, Tomioka H, Yoshida S. Anti-IgE autoantibody in patients with atopic dermatitis. J Immunol (1985) 135(1):478–82. doi: 10.4049/jimmunol.135.1.478
96. Marone G, Casolaro V, Paganelli R, Quinti I. IgG anti-IgE from atopic dermatitis induces mediator release from basophils and mast cells. J Invest Dermatol (1989) 93(2):246–52. doi: 10.1111/1523-1747.ep12277582
97. Poto R, Patella V, Criscuolo G, Marone G, Coscioni E, Varricchi G. Autoantibodies to IgE can induce the release of proinflammatory and vasoactive mediators from human cardiac mast cells. Clin Exp Med (2022) 23(4):1265–76. doi: 10.1007/s10238-022-00861-w
98. Poto R, Quinti I, Marone G, Taglialatela M, de Paulis A, Casolaro V, et al. IgG autoantibodies against IgE from atopic dermatitis can induce the release of cytokines and proinflammatory mediators from basophils and mast cells. Front Immunol (2022) 13:880412. doi: 10.3389/fimmu.2022.880412
99. Sgnotto FDR, de Oliveira MG, Lira AAL, Inoue AHS, Titz TO, Orfali RL, et al. IgG from atopic dermatitis patients induces IL-17 and IL-10 production in infant intrathymic TCD4 and TCD8 cells. Int J Dermatol (2018) 57(4):434–40. doi: 10.1111/ijd.13907
100. Santos LS, Sgnotto FDR, Sousa TR, Orfali RL, Aoki V, Duarte AJDS, et al. IgG from atopic dermatitis patients induces non-atopic infant thymic invariant natural killer T (iNKT) cells to produce IL-4, IL-17, and IL-10. Int J Dermatol (2019) 59(3):359–64. doi: 10.1111/ijd.14688
101. Mikus M, Johansson C, Acevedo N, Nilsson P, Scheynius A. The antimicrobial protein S100A12 identified as a potential autoantigen in a subgroup of atopic dermatitis patients. Clin Transl Allergy (2019) 9:6. doi: 10.1186/s13601-019-0240-4
102. Fagundes BO, de Sousa TR, Nascimento A, Fernandes LA, Sgnotto FDR, Orfali RL, et al. IgG from adult atopic dermatitis (AD) patients induces nonatopic neonatal thymic gamma-delta T cells (ΓδT) to acquire IL-22/IL-17 secretion profile with skin-homing properties and epigenetic implications mediated by miRNA. Int J Mol Sci (2022) 23(12):6872. doi: 10.3390/ijms23126872
Keywords: immunoglobulins, T-lymphocytes, immunomodulation, regulatory T cell, atopic dermatitis, immune tolerance, allergic disease, autoimmune disease
Citation: Victor JR and Nahm DH (2023) Mechanism underlying polyvalent IgG-induced regulatory T cell activation and its clinical application: Anti-idiotypic regulatory T cell theory for immune tolerance. Front. Immunol. 14:1242860. doi: 10.3389/fimmu.2023.1242860
Received: 19 June 2023; Accepted: 02 November 2023;
Published: 29 November 2023.
Edited by:
Nirupama Darshan Verma, University of New South Wales, AustraliaReviewed by:
Kjell-Olov Grönvik, Uppsala Immunobiology Lab, SwedenDongshu Zhang, Southern Medical University, China
Chang Ook Park, Yonsei University, Republic of Korea
Copyright © 2023 Victor and Nahm. This is an open-access article distributed under the terms of the Creative Commons Attribution License (CC BY). The use, distribution or reproduction in other forums is permitted, provided the original author(s) and the copyright owner(s) are credited and that the original publication in this journal is cited, in accordance with accepted academic practice. No use, distribution or reproduction is permitted which does not comply with these terms.
*Correspondence: Jefferson Russo Victor, dmljdG9yanJAdXNwLmJy; Dong-Ho Nahm, ZGhuYWhtQGFqb3UuYWMua3I=; ZGhuYWhtQGdtYWlsLmNvbQ==
†ORCID: Jefferson Russo Victor, orcid.org/0000-0001-6092-8394
Dong-Ho Nahm, orcid.org/0000-0001-5253-6577