- 1Division of Immunopathology, Department of Pathophysiology and Allergy Research, Center for Pathophysiology, Infectiology and Immunology, Medical University of Vienna, Vienna, Austria
- 2Laboratory for Immunopathology, Department of Clinical Immunology and Allergology, Sechenov First Moscow State Medical University, Moscow, Russia
- 3National Research Center, NRCI Institute of Immunology, Federal Medical-Biological Agency (FMBA) of Russia, Moscow, Russia
- 4RUDN University, Moscow, Russia
- 5Pirogov Russian National Research Medical University, Moscow, Russia
- 6Karl Landsteiner University of Healthcare, Krems, Austria
- 7Worg Pharmaceuticals, Hangzhou, China
Albumins from animals are highly cross-reactive allergens for patients suffering from immunoglobulin E (IgE)-mediated allergy. Approximately 20-30% of cat and dog allergic patients show IgE reactivity and mount IgE-mediated allergic reactions to cat and dog albumin. It is astonishing that allergic patients can develop specific IgE responses against animal albumins because these proteins exhibit a more than 70% sequence identity to human serum albumin (HSA) which is the most abundant protein in the blood of the human body. The sequence identity of cat albumin (Fel d 2) and dog albumin (Can f 3) and HSA are 82% and 80%, respectively. Given the high degree of sequence identity between the latter two allergens and HSA one would expect that immunological tolerance would prohibit IgE sensitization to Fel d 2 and Can f 3. Here we discuss two possibilities for how IgE sensitization to Fel d 2 and Can f 3 may develop. One possibility is the failed development of immune tolerance in albumin-allergic patients whereas the other possibility is highly selective immune tolerance to HSA but not to Fel d 2 and Can f 3. If the first assumption is correct it should be possible to detect HSA-specific T cell responses and HSA-containing immune complexes in sensitized patients. In the latter scenario few differences in the sequences of Fel d 2 and Can f 3 as compared to HSA would be responsible for the development of selective T cell and B cell responses towards Fel d 2 as well as Can f 3. However, the immunological mechanisms of albumin sensitization have not yet been investigated in detail although this will be important for the development of allergen-specific prevention and allergen-specific immunotherapy (AIT) strategies for allergy to albumin.
Introduction
IgE-mediated allergy to animals, in particular to cats is very common (1). A recent survey of the molecular IgE sensitization profiles in sera collected from more than 2800 children in the course of longitudinal birth cohort studies in different European regions identified the major cat allergen Fel d 1 as one of the most frequently recognized allergens (2). While Fel d 1 is an allergen that is highly specific for cats (3, 4), animals also contain cross-reactive allergens among which the serum protein albumin is the most important cross-reactive allergen known to date (5). Albumin had been described as an allergen in dogs and cats as long as 50 years ago (6, 7). In the years since, recombinant albumin allergens from dogs and cats were obtained by molecular cloning techniques. Using serum IgE from dog-allergic patients, a cDNA coding for a recombinant dog albumin fragment was isolated from a cDNA library constructed from dog liver mRNA (8). Recombinant dog albumin expressed in Escherichia coli (E. coli) has then been shown to cross-react with albumins from several different animals (9). Additionally, cat albumin could be expressed as a recombinant allergen in E. coli, and IgE recognition of Fel d 2 was found to be frequent in cat-allergic patients suffering from atopic dermatitis (10). The relevance of albumin as an allergen was demonstrated by its ability to induce specific basophil activation and immunization of mice with albumin-induced strong specific IgE sensitization (10, 11). In population-based birth cohort studies cat and dog albumin have been identified as frequently recognized allergens (12). The Swedish BAMSE birth cohort study showed a progressive increase in the prevalence of IgE sensitization to Fel d 2 and Can f 3. IgE sensitization to Fel d 2 was much more common than to Can f 3 and was detected in approximately 7% of all of the investigated children at the age of 16 years (12).
IgE cross-reactivity towards albumins from different animals has been demonstrated (9) and IgE co-sensitization has been investigated using micro-arrayed albumins from different animals by IgE serology (13). Although albumin is not the immune-dominant allergen in most of the patients suffering from animal allergy it accounts for the majority of animal-specific IgE in certain patients (13). Interestingly, T cell reactivity to albumin allergens in allergic patients has so far not been investigated in detail.
Albumins are highly abundant serum proteins with conserved primary and tertiary structure in animals and humans
Albumin belongs to a family of globular transport proteins (i.e., serum albumin, alpha-fetoprotein, Vitamin D-binding protein, afamin, extracellular matrix protein 1) in the blood which can bind various endogenous, exogenous ligands as well as drugs (14, 15) (https://albumin.org/, https://en.wikipedia.org/wiki/Albumin). Albumins are water soluble and in contrast to many other blood proteins are not glycosylated. The word albumin has its origins in the word “albumen”, which means protein, and the Latin root word “albus” meaning white, and refers to the white color of egg white when eggs are cooked. Serum albumin is synthesized in and secreted from liver cells as a single-chain protein. Human serum albumin consists of 585 amino acids and according to its sequence has a molecular mass of 66,500 Da (16). Human serum albumin contains a large number of acidic and basic amino acid residues and hence is highly soluble in aqueous solutions. As long ago as the 1930s, T. Svedberg and K. O. Petersen in Uppsala determined its molecular weight to be 66.5 kDa by ultracentrifugation (17). As the most abundant storage and transport protein in the body’s circulatory system (18), albumin has many important physiological and pharmacological functions and can be combined with many endogenous and exogenous substances such as fatty acids, amino acids, hormones, anions, cations, and drugs (19). The physiological functions of albumin include transporting ligands, maintaining plasma osmotic pressure, anti-oxidative and free radical scavenging and it is also used in disease treatment and diagnosis (20).
The three-dimensional structure of HSA as revealed by x-ray crystallography shows that the protein contains three structurally similar functional domains (domain I, II, III) which assemble in the form of a heart-shaped protein (21). Each functional domain (I-III) contains two subdomains (subdomain A and subdomain B). The hydrophobic cavities in subdomains IIA and IIIA are mainly responsible for ligand binding. The structure of HSA is stabilized by 17 disulfide bridges distributed in each tertiary region, as well as one free thiol group (21). This architecture is similar among serum albumins, in which each domain contains five or six internal disulfide bonds.
As already indicated, serum albumin plays an important role in the pharmacokinetics of drugs, especially their distribution in the human body. In 1975, G. Sudlow discovered two different types of sites on serum albumin where dansyl-L-asparagine (DNSA) and dansylsarcosirie could be displaced by drugs using fluorescent probe displacement assays (22). Fatty acids, warfarin, antioxidant metal ions, nitric oxide, and oxygen are possible ligands for the serum albumin binding sites (23–26). Most of the drugs first combine with serum albumin, and then reach the target tissue for storage and further transportation. Since albumin is synthesized in the liver, liver damage, malnutrition, and inflammation may lead to alterations in the rate of albumin synthesis (20).
Albumins as cross-reactive allergens but cross-reactivity can be limited: importance for allergy diagnosis
Fel d 2 (i.e., cat albumin) is the most frequently recognized albumin allergen (2, 27). It consists of 608 amino acids including a signal peptide comprising the first 18 amino acids, 29 alpha helices, 4 turns, and 2 beta strands (see IUIS Allergen nomenclature database: http://www.allergen.org/viewallergen.php?aid=320; Protein sequence: https://www.ncbi.nlm.nih.gov/protein/CAA59279). If one constructs a phylogenetic tree displaying evolutionary relationships of albumins from various species based on sequence features (Figure 1) it can be noticed that Fel d 2 is represented on a branch together with dog albumin, Can f 2, and HSA (Figure 1). The relationship of albumins displayed in the phylogenetic tree (Figure 1) and sequence similarity can be also observed in the alignment of amino acid sequences (Figures 2A, B). When sequences are aligned according to similarity with HSA on top, the alignment reflects to a large extent the previously observed cross-reactivity of IgE antibodies investigated in earlier immunological studies (8). These studies showed a high degree of IgE cross-reactivity between cat and dog albumin whereas IgE cross-reactivity between cat and dog albumin and bovine serum albumin, horse albumin, rat albumin, and chicken albumin (Gal d 5) were lower, respectively. However, so far no relevant IgE reactivity to HSA could be demonstrated in patients sensitized to cat and dog albumin, although Fel d 2, Can f 3 and HSA showed a high degree of sequence identity and are phylogenetically closely related (Figures 1, 2A, B). This may be due to the fact that human serum contains large amounts of HSA which will block HSA-specific IgE from binding to solid-phase bound HSA. In fact, at the level of primary structure, cat, dog, and human serum albumin all have 35 cysteines forming 17 pairs of disulfide bonds, similar to the C-(X)18-C-(X)8-C-(X)12-C-(X)14-CC-(X)9-C-(X)22-C-(X)43-CC-(X)30-C-(X)44 pattern. This highly conserved primary structure gives albumin the ability to form alpha helices, ensuring the stability of the protein. Thereby, in the tertiary structure, albumin presents an extremely stable globulin structure composed entirely of alpha helices. In the circulatory system, when the pH and other physicochemical parameters change, the large number of disulfide bonds can help albumin to reversibly change its structure, thus enabling numerous physiological functions (19). The amino acid sequences of albumin are 70% or more identical between mammals whereas sequence identities with chicken albumin are below 50% (Table 1). Again the comparison of sequence identities shows that HSA has a higher overall sequence identity to Fel d 2 and Can f 3 as compared to allergenic albumins from other albumins (Table 1). Nevertheless, IgE reactivity to albumins shown in Table 2 has been reported whereas no relevant IgE reactivity to HSA has been found in allergic patients (6, 28–44). Thus the question arises if in addition to overall sequence identity few high-impact amino acid exchanges that may affect sequential and/or conformational epitopes may have dramatic effects on IgE cross-reactivity to albumins. This would eventually explain an exquisite lack of IgE reactivity to HSA.
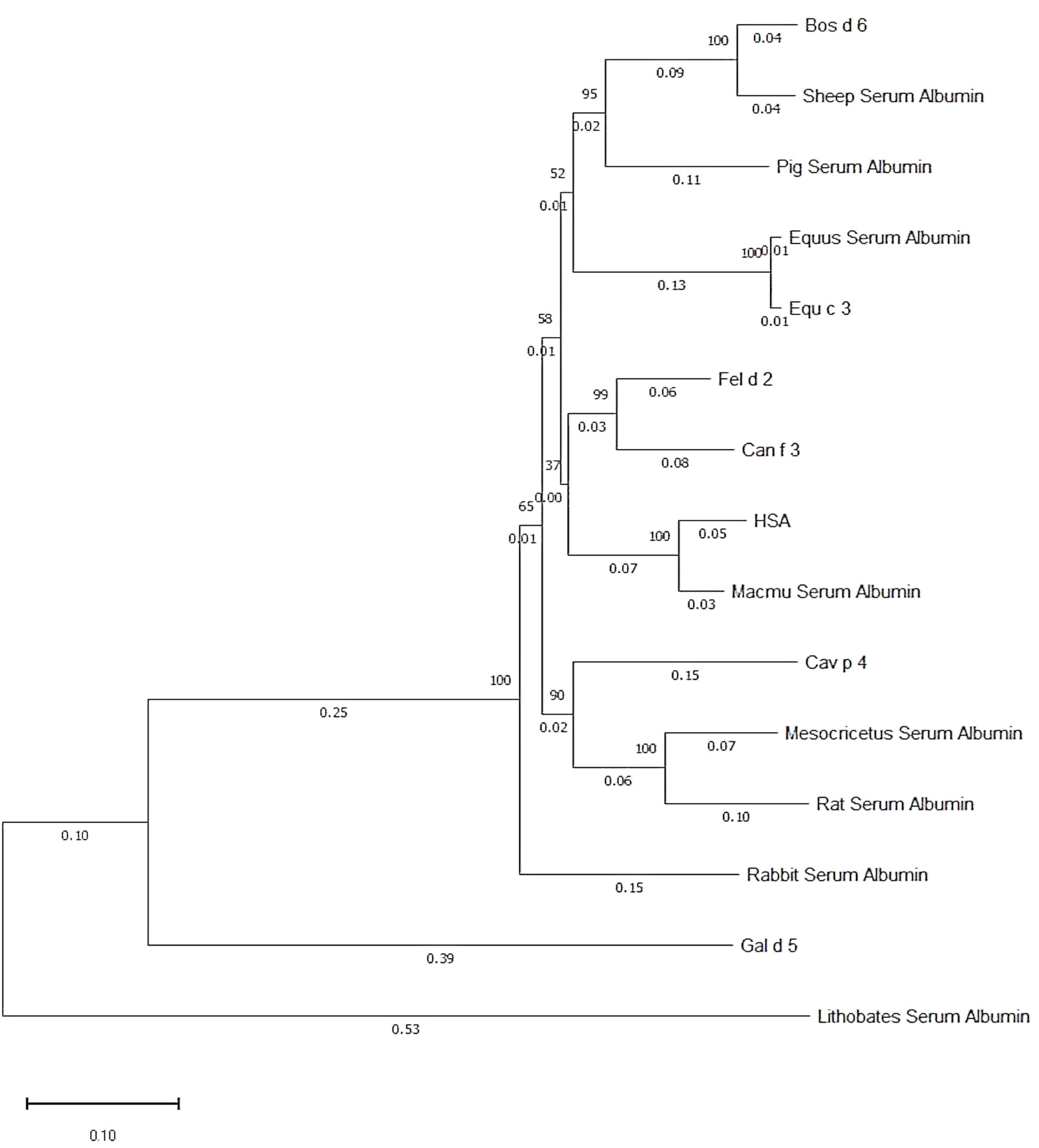
Figure 1 Phylogenetic tree of albumins from different sources. The numbers on the branch point indicate phylogenetic likelihood, whereas the numbers on the branch line indicate phylogenetic distance to branch point. Generated by Molecular Evolutionary Genetics Analysis software.
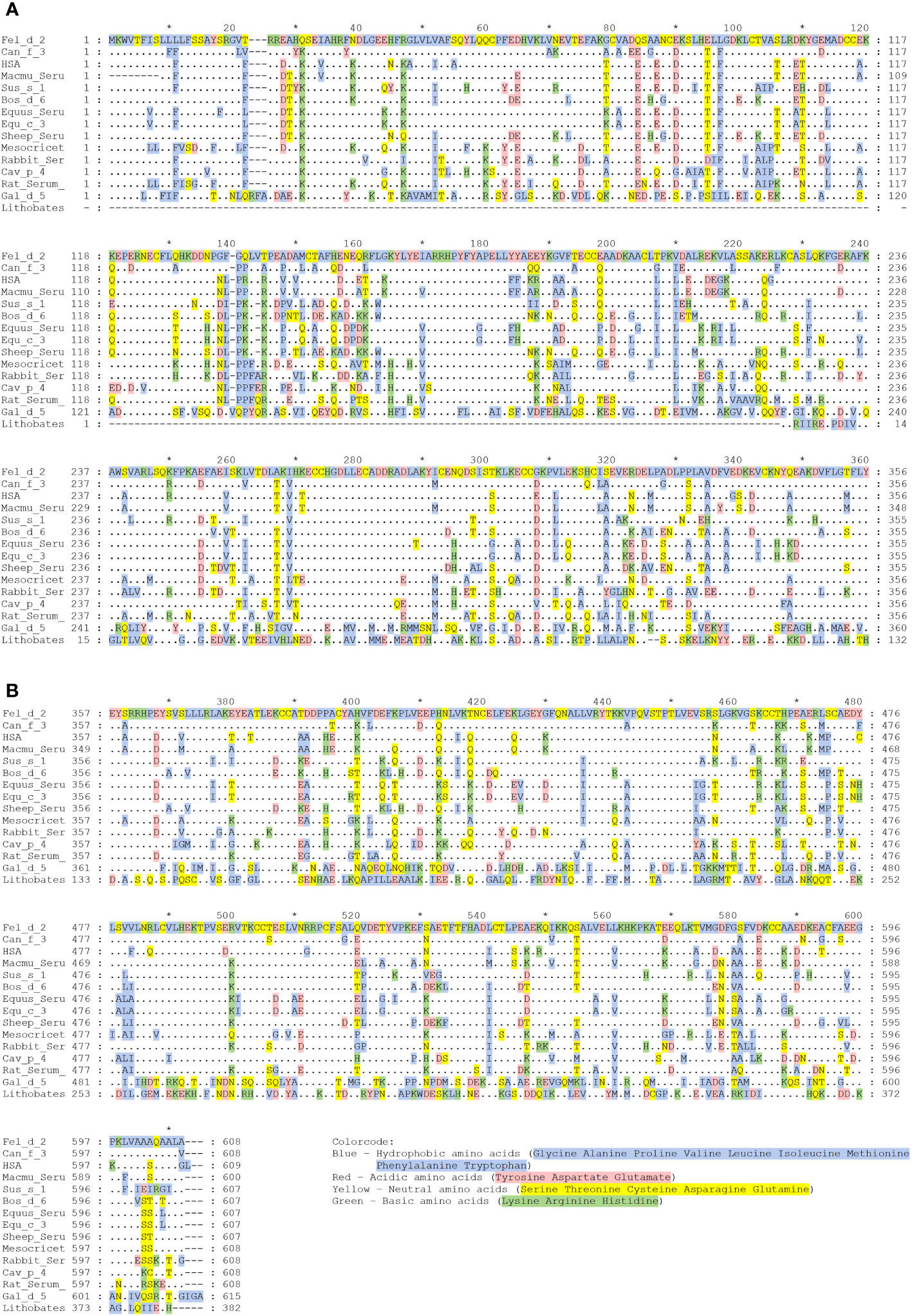
Figure 2 (A, B) Alignment of the amino acid sequence of HSA with albumins from other sources. Amino acid numbers are shown on the right and left margin and designations of albumins are given at the right margin, which are from top to bottom: Human, rhesus macaque, cat, dog, donkey, horse, pig, bovine, golden hamster, sheep, rabbit, rat, guinea pig, chicken, and American bullfrog. Amino acids identical to Fel d 2 are shown as dots, dashes indicate gaps. Amino acids are colored according to their chemical properties: Blue – Hydrophobic amino acids (Glycine, Alanine, Proline, Valine, Leucine, Isoleucine, Methionine, Phenylalanine, Tryptophan); Red – Acidic amino acids (Tyrosine, Aspartic acid, Glutamic acid); Yellow – Neutral amino acids (Serine, Threonine, Cysteine, Asparagine, Glutamine); Green – Basic amino acids (Lysine, Arginine, Histidine).
In this context, the example of pathogenesis-related allergens present in birch pollen (e.g., Bet v 1) and other plants, also known as PR10 allergens comes into mind (45, 46). IgE cross-reactivity between Bet v 1, related pollen allergens from trees belonging to the order Fagales (e., birch, alder, hazel, hornbeam), and PR10 allergens expressed in somatic plant tissues (e.g., fruits, seeds, and leaves) has been extensively described and explains varying degrees of allergic symptoms in PR10-sensitized patients (47, 48). As for albumins, IgE cross-reactivity to PR10 allergens follows more or less the overall sequence identities of the proteins, the more related to Bet v 1, the greater is the extent of IgE cross-reactivity because for most patients birch pollen and the therein contained Bet v 1 allergen, represents the genuinely sensitizing allergen source and allergen, respectively (49).
Likewise, differences in IgE reactivity in terms of specific IgE levels and presumably affinities/avidities of IgE antibodies to albumins have been observed (13). Accordingly, it has been suggested that differences in IgE reactivity to albumins from different sources may be useful for the identification of the genuinely sensitizing allergen sources among animals inducing respiratory sensitization (e.g., cats, dogs, horses) (13). Differences in IgE reactivity seem to play an even more important role when it comes to the question of whether a patient is sensitized primarily to a respiratory allergen source (e.g., cat, dog) and shows much lower secondary IgE reactivity to the corresponding allergen present in food (e.g., cow´s milk albumin) (50, 51). In this context, it has been shown, that based on allergen extract-based tests one may by mistake consider patients with IgE reactivity to BSA-containing milk as being cow´s-milk allergic although these patients were genuinely sensitized by respiratory sensitization to cat and/or dog dander (50). On the other hand, it has been demonstrated that strong IgE reactivity to BSA and BSA fragments may be indicative of primary sensitization to cow´s milk, especially in children (51).
The remaining question is why there is no relevant IgE reactivity to HSA in Fel d 2 and Can f 3-sensitized patients. Again, one possible answer to this may come from previous observations made for the cross-reactive family of the Bet v 1-related PR10 allergen family. In fact, when Cor a 1, the major allergen of hazel pollen, was characterized at the molecular level by PCR and cDNA cloning it was realized that hazel pollen contains highly IgE-reactive and allergenic Cor a 1 isoforms but at the same time, basically non-IgE reactive Cor a 1 isoforms were discovered which differed from the highly allergenic Cor a 1 isoforms only by few important amino acid exchanges (52). Likewise, highly reactive Bet v 1 isoforms were discovered which also differed only by relatively few amino acid exchanges from hypoallergenic Bet v 1 isoforms (53–55). It is therefore tempting to speculate, that one possible explanation for the lack of relevant IgE reactivity to HSA could be the occurrence of relatively few but critical differences regarding amino acids between HSA and Fel d 2 or Can f 3. In the alignment of the amino acid sequences of albumin allergens and HSA in Figure 2 we show by marking in different colors that such amino acid differences which may affect the surface charge or conformation (e.g., acidic versus basic, hydrophilic versus hydrophobic, non-conserved cysteine residues) indeed exist in Fel d 2, Can f 3 and HSA. Subsequently, we visualized amino acid differences in the model of the three-dimensional structures of Fel d 2 and HSA (Figure 3). Calculation of the hydrophilicity of the primary structure of Fel d 2 compared with HSA according to the Kyte & Doolittle algorithm showed that most (64 of 79) relevant amino acid exchanges of Fel d 2 versus HSA appeared to be in the hydrophilic fraction and accordingly can be visualized in the model of the three-dimensional structure of Fel d 2 (Figure 3). However, it has not yet been investigated if these amino acid exchanges indeed contribute to the observed differences in IgE reactivity. One possibility to investigate the effect of amino acid differences between Fel d 2 and HSA on IgE reactivity would be to conduct an IgE epitope mapping of Fel d 2 and subsequently mutate amino acids critical for IgE reactivity in Fel d 2 in HSA in order to try to render HSA IgE-reactive.
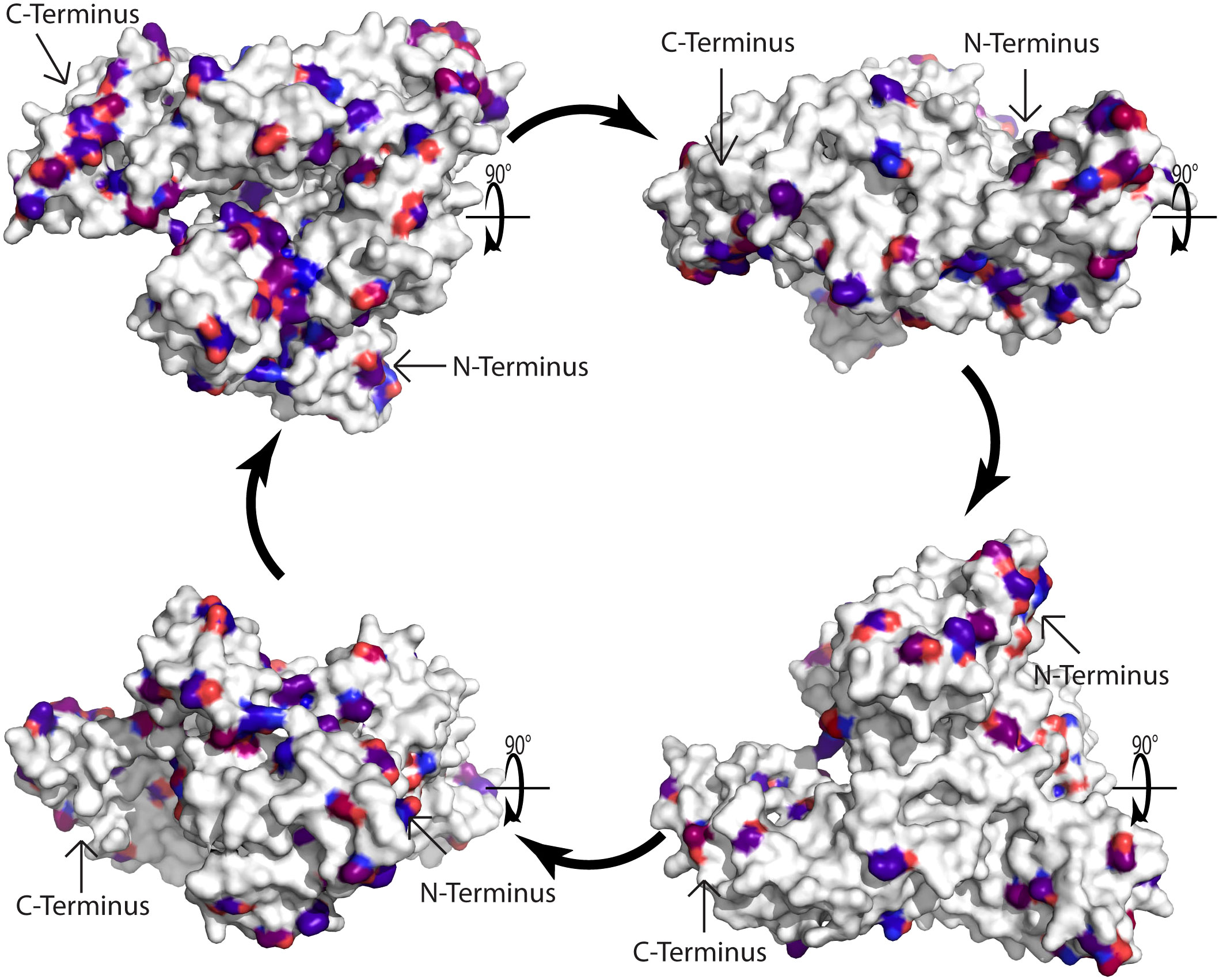
Figure 3 Model of the three-dimensional cat albumin structure. Surface representation of the model of the 3D structure of cat albumin (5yxe) as compared to human serum albumin (1ao6). In total 584 amino acids in Fel d 2 and 588 amino acids in Human serum albumin were aligned. Atoms with RMSD less than 0.972 Å were excluded. White amino acids indicate conservative regions identical to human serum albumin. Differences are represented by red and blue colors, with blue indicating more conserved mutations and red indicating less conserved mutations according to the BLOSUM90 substitution matrix. Arrows indicate the C- and N-terminus.
IgE sensitization to albumin may occur via different routes and can exhibit different clinical phenotypes of allergy
Table 2 provides an overview of albumins from different sources which have been shown to be recognized by IgE antibodies from allergic patients together with their primary and if available three-dimensional structure data and corresponding references (6, 28–44). According to population-based molecular IgE reactivity profiling available thus far, Fel d 2 and Can f 3 belong to the most frequently IgE-reactive albumins (2, 12). It thus appears that IgE sensitization to albumins occurs most frequently by the respiratory route. It has been reported that IgE recognition of Fel d 2 is more frequent in patients with atopic dermatitis (10). While this could indicate that it may be possible that sensitization can occur by the cutaneous route, it may equally be a mere sign/biomarker of strong IgE sensitization to albumin in such patients and/or broad IgE sensitization to animal allergens in general, including also albumins. Such allergens have been described also for other allergen sources as possible biomarkers for severe forms of allergy (56–58). However, IgE recognition of these biomarker allergens does not always seem to contribute to symptoms, rather it seems that broad IgE sensitization including these biomarker allergens is associated with the co-occurrence of several different allergic symptoms and severity of disease (59).
Albumin appears to have little resistance to gastrointestinal digestion and/or heating, which would be features of class 1 food allergens to which patients become sensitized by the oral route (60–62). Nevertheless, primary oral sensitization to albumins may occur, particularly when albumin is part of allergen sources that are rich in other proteins which may protect albumin against digestion. A typical example is sensitization to cow´s milk which contains besides albumin many other proteins and allergens that are highly resistant to digestion (63). In order to answer the question of whether oral sensitization to albumin present in food (e.g., milk, meat) has occurred via the gastrointestinal route molecular IgE diagnosis can be helpful. Thus certain patients may become sensitized via the gastrointestinal tract, whereas others become sensitized by the respiratory tract and it seems that the levels of allergen-specific IgE, their avidities, and the extent of cross-reactivity determine the evolvement, quality, and extent of clinical symptoms to albumins from different sources. We argue that it is useful to determine IgE levels and study IgE cross-reactivity to different albumins, for example by IgE inhibition experiments to determine the genuine allergen source which is responsible for albumin sensitization.
Such a diagnostic procedure may help clarify what type of IgE sensitization dominates in the various clinical syndromes that are attributed to IgE cross-reactivity to albumins (Table 3) (9, 12, 28, 31–34, 38, 39, 44, 64–87). These syndromes include for example the cat-pork/pork cat syndrome with gastrointestinal symptoms or skin symptoms in cat allergic patients when they eat pork or prepare pork meat dishes (28, 31, 39, 64–67). IgE sensitization to Fel d 2 may result in IgE cross-reactivity to BSA and mistakenly lead to the incorrect diagnosis of cow´s milk allergy. Simultaneous IgE reactivity to albumins from animals may be due to IgE cross-sensitization due to primary IgE sensitization to one respiratory allergen source associated with subsequent IgE cross-reactivity with albumins from other allergen sources or it can be a result of co-sensitization. Accordingly, molecular diagnosis with albumins from different allergen sources may provide information about the genuinely sensitizing allergen source and guide the prescription of allergen-specific immunotherapy (AIT). In the case of primary IgE sensitization to albumin from one allergen source AIT only to the genuinely-sensitizing source is indicated whereas in the case of co-sensitization AIT with the co-sensitizing allergen sources may be indicated if clinical relevance of the IgE sensitization has been confirmed. In this case, detection of genuine sensitization may be also confirmed by determining IgE sensitization against the genuine marker allergens of the different allergen sources (e.g., Fel d 1 for cat allergy) but this may not always be clear because even major cat and dog allergens may cross-react as shown for Fel d 7 and Can f 1 (88).
Table 3 provides a summary of clinically described syndromes in which IgE cross-reactivity to albumin plays a role (9, 12, 28, 31–34, 38, 39, 44, 64–87). The symptoms resulting from IgE cross-reactivity to albumin include the cat-pork syndrome, the cat-milk syndrome, cat-dog sensitization, allergy during artificial insemination with BSA-containing materials, the pork-chicken syndrome, the horse milk-dander syndrome, the bird-egg/egg-feather syndrome, the cow´s milk-dander-beef syndrome and horse meat allergy associated with allergy to hamsters, cats, and dogs (9, 12, 28, 31–34, 38, 39, 44, 64–87). Due to the large amounts of serum albumin in the circulatory system, connective tissues, feathers or hair, and egg yolks of birds, highly sensitive individuals develop symptoms after exposure to feathers or hair, shed by pets, and, subsequently develop symptoms when the patient is re-exposed to foods containing albumin. These symptoms are usually gastrointestinal in nature but sometimes include the skin or respiratory system as well.
The cat-pork syndrome has been widely reported in recent years, usually in people with respiratory allergies to cat dander or hair who develop acute allergic reactions to pork. It was first reported for patients eating pork after being exposed to cat hair and shown to cause digestive or even systemic allergies (28, 39, 65–67). Although cat serum albumin is not considered to be the major allergen triggering cat allergy (only 14%-23% of cat allergic patients are sensitized to Fel d 2), it is considered to be an important diagnostic marker considering that about one-third of cat allergy patients may experience symptoms upon pork ingestion (28). Cases of occupational exposure resulting in the development of cat-pork syndrome in meat processing employees have also been reported (64).
It is also possible that cat-allergic patients contain anti-BSA IgE antibodies reacting with milk. J Vicente-Serrano (85) and Karsonova (50) previously reported that patients allergic to BSA in milk may develop cross-reactivity to mammalian meat or epithelial cells, and that serum from patients allergic to cats reacts with BSA in milk.
Likewise, it was reported that cat-allergic patients had a severe anaphylactic reaction to BSA after artificial insemination with BSA-containing sperms, and after measurement of serum, IgE reactivity to serum albumin from other mammals such as cats, dogs, and pigs was found (81). Similarly, patients allergic to cats were found to have systemic symptoms due to an allergy to BSA in artificial insemination (80).
The bird-egg syndrome was first reported in 1985 when a parrot owner developed respiratory and cutaneous allergic reactions after ingesting egg yolk (83). After inhibition tests conducted by IgE-immunoblotting, serum albumin (Gal d 5), which is present in both egg yolk and parrot feathers, was identified as the relevant allergen. In fact, some patients show gradual remission of allergy to bird serum albumin after cessation of contact with bird feathers (83, 84).
For all the aforementioned syndromes, measurement of IgE levels specific for a panel of albumins from the different allergen sources by molecular diagnosis and eventually IgE inhibition experiments will in addition to the measurement of IgE sensitization to source-specific marker allergens provide information about the genuinely sensitizing allergen source for precision-medicine-based approaches for treatment and prevention.
Allergy to albumin may also occur in animals
Several observations suggest that animals may develop immunological hypersensitivity reactions to HSA. BSA and HSA have been shown to be highly immunogenic in mice in fundamental studies in immunology (89–91). Furthermore, it has been reported that dogs and/or cats receiving HSA-containing solutions developed Type III hypersensitivity reactions and formed HSA-containing immune complexes (92–95). Likewise, it was found that chronic glomerulonephritis (GN) could be induced in N/M mice by daily injections of HSA. In these mice, the glomerular lesions were similar to that observed in human membranous GN and could be characterized by intense mesangial and capillary loop immunofluorescent staining for HSA, IgG, and C3 (96).
Subcutaneous sensitization with Aluminum-hydroxide-adsorbed Can f 3 was found to induce strong Can f 3-specific IgE responses as well as Can f 3-specific IgG responses in a murine model of IgE-mediated allergy (11). HSA has been also used to sensitize rats to demonstrate that IgE sensitization can be prevented by feeding HSA via oral tolerance induction (97). Similar results were obtained by feeding BSA which prevented the development of BSA-specific hypersensitivity (98).
The aforementioned examples thus demonstrate that it is possible to induce immunological hypersensitivity and in particular IgE-mediated hypersensitivity against albumin in experimental animal models. Moreover, certain of these animal studies demonstrate that it is possible to prevent the induction of albumin-specific hypersensitivity by the induction of oral tolerance (i.e., pre-feeding of animals with albumin before sensitization).
Allergy to albumin: a case of failed tolerance or of highly selective tolerance?
Due to the similarity of HSA and animal albumins, it is quite possible that patients allergic to animal albumins also contain antibodies and T cells reactive to HSA. Indeed, the appearance of anti-HSA antibodies in patients with systemic lupus erythematosus has been reported (99). Furthermore, the presence of IgM-HSA immune complexes has been described in patients with liver disease and autonomic dysfunction (100, 101). Yet another example of possible HSA-mediated auto-reactivity is the occurrence of anti-HSA autoantibodies in patients with autoimmune bullous skin diseases (102). The development of auto-reactivity and thus the failure of HSA-specific tolerance (Figure 4) may be explained by at least two mechanisms. First, it is possible that auto-reactivity is induced by an altered self. For example, it has been shown that one can induce anti-albumin autoantibodies by immunization of animals with self-albumin which has been altered by chemical means (103, 104). In agreement with these animal models, autoantibodies specific for chemically modified HSA have been described in patients suffering from autoimmune diseases (105–107). The second possibility for the induction of adaptive immune responses against HSA patients is cross-reactivity between a structurally related foreign antigen such as Fel d 2, Can f 3, Bos d 6, or other exogenous sensitizing animal albumins. In this context, it should be noted that cases of diabetes and nephropathy due to cross-reactivity of BSA and antigenic determinants on human cells have been reported (108–112).
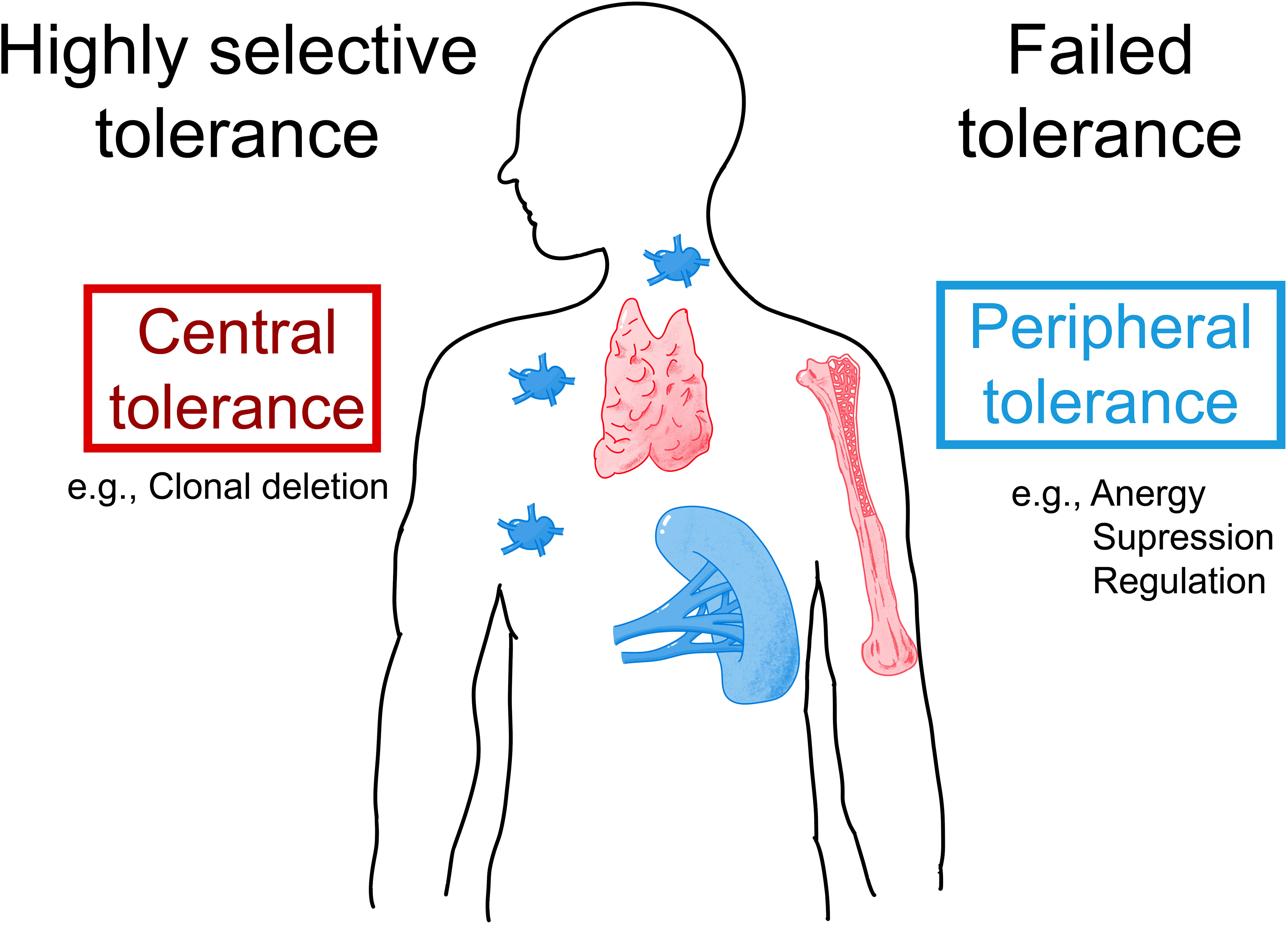
Figure 4 Possible mechanisms underlying IgE sensitization to albumins. Allergic patients who are allergic to exogenous albumins such as Fel d 2 and Can f 3 may lack IgE auto-sensitization to HSA due to highly selective central and/or peripheral tolerance to HSA (left part). Alternatively, patients with an allergy to exogenous albumins may show IgE- and T-cell cross-reactivity to HSA due to failed tolerance (right part). AIT with albumin-containing vaccines may induce and/or enhance auto-reactivity to HSA.
IgE-auto-reactivity due to cross-reactivity of foreign antigens (113–117) as well as IgE-auto-reactivity to intracellular human antigens which do not share known structural similarity with exogenous antigens (118–120) have been previously described in allergic patients. Hence, IgE auto-reactivity is known to occur in allergic patients (121).
However, so far the presence of IgE antibodies specific to HSA could not be demonstrated and we are not aware of attempts to search for HSA-specific T cells in albumin-allergic patients. The question is therefore what the reason could be that so far no IgE antibodies specific for HSA could be detected. If there were HSA-specific IgE antibodies in albumin-allergic patients, these antibodies would occur in extremely low amounts. Given the high abundance of HSA in the blood HSA-specific IgE antibodies would therefore be completely adsorbed by HSA in the form of immune complexes and accordingly will not react in tests measuring IgE reactivity to solid phase bound HSA. The only possibility to detect HSA-reactive IgE would then be to try isolating such putative IgE-HSA immune complexes but this has not yet been done successfully. If indeed such IgE-HSA immune complexes and HSA-specific T cell responses could be found in albumin-allergic patients one may consider failed immunological tolerance as a reason for albumin-specific IgE and T cell responses in albumin–allergic patients (Figure 4). If failed tolerance can be demonstrated, it will be interesting to investigate if auto-reactivity to HSA may contribute to inflammation in albumin-allergic patients.
The second possibility is that patients allergic to exogenous albumins are selectively sensitized to the exogenous albumins, but not to HSA due to highly selective tolerance (Figure 4). In this case, albumin-specific T cells and IgE would selectively react with epitopes that differ between HSA and the exogenous albumins. Accordingly, mapping of IgE and T cell epitopes of exogenous albumins would need to be done in allergic patients sensitized to these albumins to clarify whether sensitization is due to highly selective central and/or peripheral tolerance which the latter may also include the activity of B regulatory cells (122–124). In this context, it will be very interesting to determine how much the extent of the putative selective tolerance depends on differences in sequence and structure between homologous antigens. This will be important for the development of therapeutic strategies for the treatment and prevention of allergy based on tolerance induction and in particular for the selection of tolerogenic peptides for such approaches (125–127).
Can allergen-specific immunotherapy with albumin-containing vaccines induce auto-reactivity or even autoimmunity?
Besides the induction of T cell-based tolerance, AIT based on vaccines containing allergens or recombinant allergen derivatives is another possibility for allergen-specific treatment and prevention of allergy (128). The induction of allergen-specific IgG-blocking antibodies seems to be an important underlying mechanism of AIT (129). Vaccine-induced IgG antibodies are supposed to block allergens to induce IgE-mediated effector cell (e.g., mast cells, basophils, T cells) activation and boost IgE secondary IgE production (128). Currently, allergen extracts derived from natural allergen sources are mainly used for treatment (130). In the case of allergy to animal dander, especially for cat and dog allergy, natural allergen extracts that may contain albumin are used. It is thus possible that vaccination with albumin-containing allergen extracts may induce antibody and T cell responses not only against the corresponding exogenous albumins present in the vaccine but eventually also break tolerance resulting in the induction of HSA-specific antibody and T cell responses which then may lead to auto-reactivity in vaccinated subjects. At present there is no evidence for such a possibility but this topic has not yet been investigated intensively. In fact, to the best of our knowledge, it has not been studied systematically if special complications have been reported for albumin-sensitized allergic patients who had undergone AIT with albumin-containing allergen extracts. However, it has been shown that there are considerable side effects in patients who had received cat-specific AIT but it will be interesting to study if side effects due to auto-sensitization are more in albumin-reactive patients (131). Using modern molecular allergy vaccines based on recombinant allergens, recombinant allergen derivatives or allergen-derived peptides (128) will clearly be possible to eliminate the risk of inducing adaptive immunity to HSA by not including albumin and albumin-derived epitopes in the vaccines. However, it may be important to develop safe vaccines for patients with clinically relevant sensitizations to albumin. Accordingly, understanding the mechanisms of IgE-mediated allergy to albumin will be a prerequisite for the development of adequate and safe treatment strategies.
Conclusions and open questions
Albumins from various animals, especially from cats and dogs, are important allergens for allergic patients with respiratory allergy and may contribute to symptoms of food allergy upon intake of albumin-containing food. Despite a high degree of sequence and structural similarity among exogenous albumins and human serum albumin, it is not known whether albumin-allergic patients show HSA-specific auto-reactivity or have developed selective tolerance. Unraveling the mechanisms and epitopes involved in allergy to albumins is important for at least two reasons. First of all, molecular diagnostic tests based on albumins from different sources may help to identify the genuinely sensitizing allergen source and guide precision medicine-based forms of allergen-specific forms of treatment and prevention. Second, knowledge regarding possible auto-reactivity to albumin in sensitized albumin allergic patients and about the potential risks of inducing albumin-specific auto-reactivity may help improve allergen-specific forms of treatment and prevention of patients allergic to animals.
Author contributions
ZL and DT contributed to the design of the study, interpreted the findings, and wrote the manuscript. RV, AlK, and MK designed the study and contributed to the interpretation of the findings, writing and revising the manuscript. ZL, DT, IT, KR, AnK, EK, OE, MK, T-HC, MF-T, AlK, and RV provided materials, references, and/or figures/Tables, and read and revised the manuscript. All authors contributed to the article and approved the submitted version.
Funding
Funded by the Danube Allergy Research Cluster program of the Country of Lower Austria, the review of albumin-specific IgE cross-reactivity was supported by a grant of the Russian Science Foundation (the Project: No: 23-75-30016: “Allergen micro-array-based assessment of allergic sensitization profiles in the Russian Federation as a basis for personalized treatment and prevention of allergy (AllergochipRUS)”), by Worg Pharmaceuticals, Hangzhou, China and by the Megagrant of the Government of the Russian Federation, grant number 075-15-2021-632 (14.W03.31.0024). The funder was not involved in the study design, analysis, interpretation of data, the writing of this article or the decision to submit it for publication.
Acknowledgments
The authors acknowledge the support of the Medical University of Vienna, Austria, Institute of Immunology, Moscow and Sechenov First Moscow State Medical University, Moscow, Russia regarding research infrastructure.
Conflict of interest
Author T-HC was employed by the company Worg Pharmaceuticals.
The remaining authors declare that the research was conducted in the absence of any commercial or financial relationships that could be construed as a potential conflict of interest.
Publisher’s note
All claims expressed in this article are solely those of the authors and do not necessarily represent those of their affiliated organizations, or those of the publisher, the editors and the reviewers. Any product that may be evaluated in this article, or claim that may be made by its manufacturer, is not guaranteed or endorsed by the publisher.
Author disclaimer
The authors with Russian affiliation declare that they have prepared the article in their “personal capacity” and/or that they are employed at an academic/research institution where research or education is the primary function of the entity.
References
1. Konradsen JR, Fujisawa T, van Hage M, Hedlin G, Hilger C, Kleine-Tebbe J, et al. Allergy to furry animals: New insights, diagnostic approaches, and challenges. J Allergy Clin Immunol (2015) 135:616–25. doi: 10.1016/j.jaci.2014.08.026
2. Kiewiet MBG, Lupinek C, Vrtala S, Wieser S, Baar A, Kiss R, et al. A molecular sensitization map of European children reveals exposome- and climate-dependent sensitization profiles. Allergy (2023) 78(7):2007–18. doi: 10.1111/all.15689
3. Morgenstern JP, Griffith IJ, Brauer AW, Rogers BL, Bond JF, Chapman MD, et al. Amino acid sequence of Fel dI, the major allergen of the domestic cat: protein sequence analysis and cDNA cloning. Proc Natl Acad Sci USA (1991) 88:9690–4. doi: 10.1073/pnas.88.21.9690
4. Grönlund H, Saarne T, Gafvelin G, van Hage M. The major cat allergen, fel d 1, in diagnosis and therapy. Int Arch Allergy Immunol (2010) 151:265–74. doi: 10.1159/000250435
5. Caraballo L, Valenta R, Puerta L, Pomés A, Zakzuk J, Fernandez-Caldas E, et al. The allergenic activity and clinical impact of individual IgE-antibody binding molecules from indoor allergen sources. World Allergy Organ J (2020) 13:100118. doi: 10.1016/j.waojou.2020.100118
6. Yman L, Brandt R, Ponterius G. Serum albumin – an important allergen in dog epithelia extracts. Int Arch Allergy Immunol (1973) 44:358–68. doi: 10.1159/000230944
7. Ohman J, Lowell F, Bloch K. Allergens of mammalian origin: Characterization of allergen extracted from cat pelts. J Allergy Clin Immunol (1973) 52:231–41. doi: 10.1016/0091-6749(73)90061-4
8. Spitzauer S, Pandjaitan B, Söregi G, Mühl S, Ebner C, Kraft D, et al. IgE cross-reactivities against albumins in patients allergic to animals. J Allergy Clin Immunol (1995) 96:951–9. doi: 10.1016/S0091-6749(95)70233-4
9. Pandjaitan B, Swoboda I, Brandejsky-Pichler F, Rumpold H, Valenta R, Spitzauer S. Escherichia coli expression and purification of recombinant dog albumin, a cross-reactive animal allergen. J Allergy Clin Immunol (2000) 105:279–85. doi: 10.1016/S0091-6749(00)90077-0
10. Reininger R, Swoboda I, Bohle B, Hauswirth AW, Valent P, Rumpold H, et al. Characterization of recombinant cat albumin. Clin Exp Allergy (2003) 33:1695–702. doi: 10.1111/j.1365-2222.2003.01817.x
11. Vrtala S, Ball T, Spitzauer S, Pandjaitan B, Suphioglu C, Knox B, et al. Immunization with purified natural and recombinant allergens induces mouse IgG1 antibodies that recognize similar epitopes as human IgE and inhibit the human IgE-allergen interaction and allergen-induced basophil degranulation. J Immunol (1998) 160:6137–44. doi: 10.4049/jimmunol.160.12.6137
12. Asarnoj A, Hamsten C, Wadén K, Lupinek C, Andersson N, Kull I, et al. Sensitization to cat and dog allergen molecules in childhood and prediction of symptoms of cat and dog allergy in adolescence: A BAMSE/MeDALL study. J Allergy Clin Immunol (2016) 137:813–821.e7. doi: 10.1016/j.jaci.2015.09.052
13. Curin M, Swoboda I, Wollmann E, Lupinek C, Spitzauer S, van Hage M, et al. Microarrayed dog, cat, and horse allergens show weak correlation between allergen-specific IgE and IgG responses☆. J Allergy Clin Immunol (2014) 133:918–921.e6. doi: 10.1016/j.jaci.2013.10.058
14. Wikipedia contributors. Albumin (2023). Available at: https://en.wikipedia.org/wiki/Albumin (Accessed 8 June 2023).
15. Minchiotti L, Campagnoli M. Albumin (2021). Available at: https://albumin.org (Accessed 8 June 2023).
16. Minghetti PP, Ruffner DE, Kuang WJ, Dennison OE, Hawkins JW, Beattie WG, et al. Molecular structure of the human albumin gene is revealed by nucleotide sequence within q11-22 of chromosome 4. J Biol Chem (1986) 261:6747–57. doi: 10.1016/S0021-9258(19)62680-3
17. Peters J. All about albumin. Massachusetts, United States: Elsevier (1995). doi: 10.1016/B978-0-12-552110-9.X5000-4
18. Paar M, Fengler VH, Rosenberg DJ, Krebs A, Stauber RE, Oettl K, et al. Albumin in patients with liver disease shows an altered conformation. Commun Biol (2021) 4:731. doi: 10.1038/s42003-021-02269-w
19. Rosenoer VM, Oratz M, Rothschild MA. Albumin structure, function and uses. Oxford New York Toronto Paris Sydney Frankfurt: Pergamon Press (1977). p. 143.
20. Rozga J, Piatek T, Małkowski P. Human albumin: Old, new, and emerging applications. Ann Transplant (2013) 18:205–17. doi: 10.12659/AOT.889188
21. He XM, Carter DC. Atomic structure and chemistry of human serum albumin. Nature United States: Academic Press, Cambridge, Massachu (1992) 358:209–15. doi: 10.1038/358209a0
22. Sudlow GDJ, Birkett DJ, Wade DN. The characterization of two specific drug binding sites on human serum albumin. Mol Pharmacol (1975) 11:824–32.
23. Fasano M, Curry S, Terreno E, Galliano M, Fanali G, Narciso P, et al. The extraordinary ligand binding properties of human serum albumin. IUBMB Life (2005) 57:787–96. doi: 10.1080/15216540500404093
24. Fehske KJ, Müller WE, Wollert U. The location of drug binding sites in human serum albumin. Biochem Pharmacol (1981) 30:687–92. doi: 10.1016/0006-2952(81)90151-9
25. Hajili M, Pour Khalili N, Moradi R, Abdullayev Y. Targeting interface HSA through anti-cancer drugs: Molecular modeling and docking. Mater Today Proc (2021) 42:A1–9. doi: 10.1016/j.matpr.2021.05.288
26. Belinskaia DA, Goncharov NV. Theoretical and practical aspects of albumin esterase activity. Russ J Bioorg Chem (2020) 46:287–98. doi: 10.1134/S1068162020030036
27. Nilsson OB, van Hage M, Grönlund H. Mammalian-derived respiratory allergens – Implications for diagnosis and therapy of individuals allergic to furry animals. Methods (2014) 66:86–95. doi: 10.1016/j.ymeth.2013.09.002
28. Hilger C, Kohnen M, Grigioni F, Lehners C, Hentges F. Allergic cross-reactions between cat and pig serum albumin. Allergy (1997) 52:179–87. doi: 10.1111/j.1398-9995.1997.tb00972.x
29. Spitzauer S, Schweiger C, Sperr WR, Pandjaitan B, Valent P, Mühl S, et al. Molecular characterization of dog albumin as a cross-reactive allergen. J Allergy Clin Immunol (1994) 93:614–27. doi: 10.1016/S0091-6749(94)70073-7
30. Fagerberg E, Wide L. Diagnosis of hypersensitivity to dog epithelium in patients with asthma bronchiale. Int Arch Allergy Immunol (1970) 39:301–9. doi: 10.1159/000230356
31. Posthumus J, James HR, Lane CJ, Matos LA, Platts-Mills TAE, Commins SP. Initial description of pork-cat syndrome in the United States. J Allergy Clin Immunol (2013) 131:923–5. doi: 10.1016/j.jaci.2012.12.665
32. Gjesing B, Østerballe O, Schwartz B, Wahn U, Lowenstein H. Allergen-specific ige antibodies against antigenic components in cow milk and milk substitutes. Allergy (1986) 41:51–6. doi: 10.1111/j.1398-9995.1986.tb00275.x
33. Prahl P, Weeke B, Løwenstein H. Quantitative immunoelectrophoretic analysis of extract from cow hair and dander. Allergy (1978) 33:241–53. doi: 10.1111/j.1398-9995.1978.tb01544.x
34. Beretta B, Conti A, Fiocchi A, Gaiaschi A, Galli CL, Giuffrida MG, et al. Antigenic determinants of bovine serum albumin. Int Arch Allergy Immunol (2001) 126:188–95. doi: 10.1159/000049513
35. Restani P, Beretta B, Fiocchi A, Ballabio C, Galli CL. Cross-reactivity between mammalian proteins. Ann Allergy Asthma Immunol (2002) 89:11–5. doi: 10.1016/S1081-1206(10)62116-3
36. Fjeldsgaard BE, Paulsen BS. Comparison of IgE-binding antigens in horse dander and a mixture of horse hair and skin scrapings. Allergy (1993) 48:535–41. doi: 10.1111/j.1398-9995.1993.tb01111.x
37. Løwenstein H. Characterization and chemical modification of isolated allergens from horse hair and dandruff. Int Arch Allergy Immunol (1978) 57:349–57. doi: 10.1159/000232124
38. Cistero-Bahima A, Enrique E, San Miguel-Moncin MM, Alonso R, Bartra J, Fernandez-Parra B, et al. Meat allergy and cross-reactivity with hamster epithelium. Allergy (2003) 58:161–2. doi: 10.1034/j.1398-9995.2003.00056_5.x
39. Hilger C, Swiontek K, Kler S, Diederich C, Lehners C, Vogel L, et al. Evaluation of two new recombinant Guinea-pig lipocalins, Cav p 2 and Cav p 3, in the diagnosis of Guinea-pig allergy. Clin Exp Allergy (2011) 41:899–908. doi: 10.1111/j.1365-2222.2011.03726.x
40. Twiggs J, Agarwal M, Dahlberg M, Yunginger J. Immunochemical measurement of airborne mouse allergens in a laboratory animal facility. J Allergy Clin Immunol (1982) 69:522–6. doi: 10.1016/0091-6749(82)90177-4
41. Ohman J, Lowell F, Bloch K. Allergens of mammalian origin*II. Characterization of allergens extracted from rat, mouse, Guinea pig, and rabbit pelts. J Allergy Clin Immunol (1975) 55:16–24. doi: 10.1016/S0091-6749(75)80004-2
42. Wahn U, Peters T, Siraganian RP. Studies on the allergenic significance and structure of rat serum albumin. J Immunol (1980) 125:2544–9. doi: 10.4049/jimmunol.125.6.2544
43. Siraganian R, Sandberg A. Characterization of mouse allergens. J Allergy Clin Immunol (1979) 63:435–42. doi: 10.1016/0091-6749(79)90219-7
44. Quirce S, Maranon F, Umpierrez A, de las Heras M, Fernandez-Caldas E, Sastre J. Chicken serum albumin (Gal d 5 *) is a partially heat-labile inhalant and food allergen implicated in the bird-egg syndrome. Allergy (2001) 56:754–62. doi: 10.1034/j.1398-9995.2001.056008754.x
45. Breiteneder H, Pettenburger K, Bito A, Valenta R, Kraft D, Rumpold H, et al. The gene coding for the major birch pollen allergen Betv1, is highly homologous to a pea disease resistance response gene. EMBO J (1989) 8:1935–8. doi: 10.1002/j.1460-2075.1989.tb03597.x
46. Breiteneder H, Ebner C. Molecular and biochemical classification of plant-derived food allergens. J Allergy Clin Immunol (2000) 106:27–36. doi: 10.1067/mai.2000.106929
47. Niederberger V, Pauli G, Grönlund H, Fröschl R, Rumpold H, Kraft D, et al. Recombinant birch pollen allergens (rBet v 1 and rBet v 2) contain most of the IgE epitopes present in birch, alder, hornbeam, hazel, and oak pollen: a quantitative IgE inhibition study with sera from different populations. J Allergy Clin Immunol (1998) 102(4):579–91. doi: 10.1016/S0091-6749(98)70273-8
48. Ebner C, Hirschwehr R, Bauer L, Breiteneder H, Valenta R, Ebner H, et al. Identification of allergens in fruits and vegetables: IgE cross-reactivities with the important birch pollen allergens Bet v 1 and Bet v 2 (birch profilin). J Allergy Clin Immunol (1995) 95(5):962–69. doi: 10.1016/S0091-6749(95)70096-X
49. Westman M, Lupinek C, Bousquet J, Andersson N, Pahr S, Baar A, et al. Early childhood Ige reactivity to pathogenesis-related class 10 proteins predicts allergic rhinitis in adolescence. J Allergy Clin Immunol (2015) 135(5):1199–206.e1–11. doi: 10.1016/j.jaci.2014.10.042
50. Karsonova AV, Riabova KA, Khaitov MR, Elisyutina OG, Ilina N, Fedenko ES, et al. Milk-specific IgE reactivity without symptoms in albumin-sensitized cat allergic patients. Allergy Asthma Immunol Res (2021) 13:668–70. doi: 10.4168/aair.2021.13.4.668
51. Garib V, Trifonova D, Freidl R, Linhart B, Schlederer T, Douladiris N, et al. Milk allergen micro-array (MAMA) for refined detection of cow’s-milk-specific IgE sensitization. Nutrients (2023) 15:2401. doi: 10.3390/nu15102401
52. Breiteneder H, Ferreira F, Hoffmann-Sommergruber K, Ebner C, Breitenbach M, Rumpold H, et al. Four recombinant isoforms of Cor a I, the major allergen of hazel pollen, show different IgE-binding properties. Eur J Biochem (1993) 212:355–62. doi: 10.1111/j.1432-1033.1993.tb17669.x
53. Ferreira F, Hirtenlehner K, Jilek A, Godnik-Cvar J, Breiteneder H, Grimm R, et al. Dissection of immunoglobulin E and T lymphocyte reactivity of isoforms of the major birch pollen allergen Bet v 1: potential use of hypoallergenic isoforms for immunotherapy. J Exp Med (1996) 183:599–609. doi: 10.1084/jem.183.2.599
54. Arquint O, Helbling A, Crameri R, Ferreira F, Breitenbach M, Pichler WJ. Reduced in vivo allergenicity of Bet v 1d isoform, a natural component of birch pollen. J Allergy Clin Immunol (1999) 104:1239–43. doi: 10.1016/S0091-6749(99)70019-9
55. Marković-Housley Z, Degano M, Lamba D, Von Roepenack-Lahaye E, Clemens S, Susani M, et al. Crystal structure of a hypoallergenic isoform of the major birch pollen allergen Bet v 1 and its likely biological function as a plant steroid carrier. J Mol Biol (2003) 325:123–33. doi: 10.1016/S0022-2836(02)01197-X
56. Banerjee S, Resch Y, Chen KW, Swoboda I, Focke-Tejkl M, Blatt K, et al. Der p 11 is a major allergen for house dust mite-allergic patients suffering from atopic dermatitis. J Invest Dermatol (2015) 135:102–9. doi: 10.1038/jid.2014.271
57. Sarzsinszky E, Lupinek C, Vrtala S, Huang HJ, Hofer G, Keller W, et al. Expression in Escherichia coli and purification of folded rDer P20, the arginine kinase from Dermatophagoides pteronyssinus: a possible biomarker for allergic asthma. Allergy Asthma Immunol Res (2021) 13(1):154–63. doi: 10.4168/aair.2021.13.1.154
58. Huang HJ, Resch-Marat Y, Casset A, Weghofer M, Zieglmayer P, Zieglmayer R, et al. IgE recognition of the house dust mite allergen Der p 37 is associated with asthma. J Allergy Clin Immunol (2022) 149:1031–43. doi: 10.1016/j.jaci.2021.07.040
59. Riabova K, Karsonova AV, van Hage M, Käck U, Konradsen JR, Grönlund H, et al. Molecular allergen-specific IgE recognition profiles and cumulative specific IgE levels associated with phenotypes of cat allergy. Int J Mol Sci (2022) 23:6984. doi: 10.3390/ijms23136984
60. Astwood JD, Leach JN, Fuchs RL. Stability of food allergens to digestion. vitro. Nat Biotechnol (1996) 14:1269–73. doi: 10.1038/nbt1096-1269
61. Valenta R, Hochwallner H, Linhart B, Pahr S. Food allergies: the basics. Gastroenterology (2015) 148:1120–31.e4. doi: 10.1053/j.gastro.2015.02.006
62. Fuhrmann V, Huang HJ, Akarsu A, Shilovskiy I, Elisyutina O, Khaitov M, et al. From allergen molecules to molecular immunotherapy of nut allergy: A hard nut to crack. Front Immunol (2021) 12:742732. doi: 10.3389/fimmu.2021.742732
63. Hochwallner H, Schulmeister U, Swoboda I, Spitzauer S, Valenta R. Cow’s milk allergy: From allergens to new forms of diagnosis, therapy and prevention. Methods (2014) 66:22–33. doi: 10.1016/j.ymeth.2013.08.005
64. Hilger C, Swiontek K, Hentges F, Donnay C, de Blay F, Pauli G. Occupational inhalant allergy to pork followed by food allergy to pork and chicken: sensitization to hemoglobin and serum albumin. Int Arch Allergy Immunol (2010) 151(2):173–8. doi: 10.1159/000236008
65. Sabbah A, Lauret MG, Chène J, Boutet S, Drouet M. The pork-cat syndrome or crossed allergy between pork meat and cat epithelia (2). Allerg Immunol (Paris) (1994) 26:173–4.
66. Abreu C, Gomes R, Bartolome Borja B, Falcão H, Cunha L. Pork-cat syndrome? Clin Transl Allergy (2015) 5:164. doi: 10.1186/2045-7022-5-S3-P164
67. Drouet M, Lauret MG, Sabbah A. The pork-cat syndrome: effect of sensitization to cats on sensitization to pork meat. Apropos case. Allerg Immunol (Paris) (1994) 26:305–6.
68. Fuc E, Złotkowska D, Wróblewska B. Milk and meat allergens from Bos taurus β-lactoglobulin, α-casein, and bovine serum albumin: an in-vivo study of the immune response in mice. Nutrients (2019) 11:2095. doi: 10.3390/nu11092095
69. Restani P, Ballabio C, Di Lorenzo C, Tripodi S, Fiocchi A. Molecular aspects of milk allergens and their role in clinical events. Anal Bioanal Chem (2009) 395:47–56. doi: 10.1007/s00216-009-2909-3
70. Mamikoglu B. Beef, pork, and milk allergy (cross reactivity with each other and pet allergies). Otolaryngol Head Neck Surg (2005) 133:534–7. doi: 10.1016/j.otohns.2005.07.016
71. Yu J, Chen Y, Xiong L, Zhang X, Zheng Y. Conductance changes in bovine serum albumin caused by drug-binding triggered structural transitions. Materials (2019) 12:1022. doi: 10.3390/ma12071022
72. Szépfalusi Z, Ebner C, Urbanek R, Ebner H, Scheiner O, Boltz-Nitulescu G, et al. Detection of IgE antibodies specific for allergens in cow milk and cow dander. Int Arch Allergy Immunol (1993) 102:288–94. doi: 10.1159/000236538
73. Fiocchi A, Restani P, Riva E, Qualizza R, Bruni P, Restelli AR, et al. Meat allergy: I–specific IgE to BSA and OSA in atopic, beef sensitive children. J Am Coll Nutr (1995) 14:239–44. doi: 10.1080/07315724.1995.10718502
74. Restani P, Fiocchi A, Beretta B, Velonà T, Giovannini M, Galli CL. Meat allergy: III—Proteins involved and cross-reactivity between different animal species. J Am Coll Nutr (1997) 16:383–9. doi: 10.1080/07315724.1997.10718701
75. Hoffman DR. Dog and cat allergens: urinary proteins or dander proteins? Ann Allergy (1980) 45:205–6.
76. Goubran Botros H, Gregoire C, Rabillon J, David B, Dandeu JP. Cross-antigenicity of horse serum albumin with dog and cat albumins: study of three short peptides with significant inhibitory activity towards specific human IgE and IgG antibodies. Immunology (1996) 88:340–7. doi: 10.1046/j.1365-2567.1996.d01-669.x
77. Ukleja-Sokołowska N, Gawrońska-Ukleja E, Żbikowska-Gotz M, Socha E, Lis K, Sokołowski Ł, et al. Analysis of feline and canine allergen components in patients sensitized to pets. Allergy Asthma Clin Immunol (2016) 12:61. doi: 10.1186/s13223-016-0167-4
78. Curin M, Hilger C. Allergy to pets and new allergies to uncommon pets. Allergol Select (2017) 1:214–22. doi: 10.5414/alx01842e
79. Pagán JA, Postigo I, Rodríguez-Pacheco JR, Peña M, Guisantes JA, Martínez J. Bovine serum albumin contained in culture medium used in artificial insemination is an important anaphylaxis risk factor. Fertil Steril (2008) 90:2013.e17–2013.e19. doi: 10.1016/j.fertnstert.2008.05.055
80. Orta M, Ordoqui E, Aranzábal A, Fernández C, Bartolomé B, Sanz ML. Anaphylactic reaction after artificial insemination. Ann Allergy Asthma Immunol (2003) 90:446–51. doi: 10.1016/S1081-1206(10)61831-5
81. Wüthrich B, Stern A, Johansson SG. Severe anaphylactic reaction to bovine serum albumin at the first attempt of artificial insemination. Allergy (1995) 50(2):179–83. doi: 10.1111/j.1398-9995.1995.tb05077.x
82. Fanta C, Ebner C. Allergy to mare’s milk. Allergy (1998) 53:539–40. doi: 10.1111/j.1398-9995.1998.tb04095.x
83. de Maat-Bleeker F, van Dijk AG, Berrens L. Allergy to egg yolk possibly induced by sensitization to bird serum antigens. Ann Allergy (1985) 54:245–8.
84. Inomata N, Kawano K, Aihara M. Bird-egg syndrome induced by α-livetin sensitization in a budgerigar keeper: Successful induction of tolerance by avoiding exposure to avians. Allergol Int (2019) 68:282–4. doi: 10.1016/J.ALIT.2018.11.004
85. Vicente-Serrano J, Caballero ML, Rodríguez-Pérez R, Carretero P, Pérez R, Blanco JG, et al. Sensitization to serum albumins in children allergic to cow’s milk and epithelia. Pediatr Allergy Immunol (2007) 18:503–7. doi: 10.1111/j.1399-3038.2007.00548.x
86. Shingaki T, Hiraguchi Y, Tokuda R, Yamada S, Soo KJ, Teramen H, et al. Case report of a child who may have developed anaphylaxis after ingesting raw horse meat by cross-reactivity of horse and cat pelt. J Allergy Clin Immunol: Global (2023) 2(4):100139. doi: 10.1016/j.jacig.2023.100139
87. Morisset M, Arumugam K, Ollert M, Hilger C. Horse-meat allergy mediated by dog-allergy: A case report and review of the literature. Allergo J Int (2016) 25(3):30–5. doi: 10.1007/s15007-016-1074-1
88. Apostolovic D, Sanchez-Vidaurre S, Waden K, Curin M, Grundstrom J, Gafvelin G, et al. The cat lipocalin Fel d 7 and its cross-reactivity with the dog lipocalin Can f 1. Allergy (2016) 71(10):1490–5. doi: 10.1111/all.12955
89. Riley RL, Wilson LD, Germain RN, Benjamin DC. Immune responses to complex protein antigens I. MHC control of immune responses to bovine albumin. J Immunol (1982) 129:1553–8. doi: 10.4049/jimmunol.129.4.1553
90. Marusić-Galesić S, Marusić M, Pokrić B. Cellular immune response to the antigen administered as an immune complex in vivo. Immunology (1992) 75:325–9.
91. Steward MW, Stanley C, Furlong MD. Antibody affinity maturation in selectively bred high and low-affinity mice. Eur J Immunol (1986) 16:59–63. doi: 10.1002/eji.1830160112
92. Adamantos S, Chan DL, Goggs R, Humm K. Risk of immunologic reactions to human serum albumin solutions. J Small Anim Pract (2009) 50:206. doi: 10.1111/j.1748-5827.2009.00752.x
93. Powell C, Thompson L, Murtaugh RJ. Type III hypersensitivity reaction with immune complex deposition in 2 critically ill dogs administered human serum albumin. J Vet Emerg Crit Care (San Antonio) (2013) 23:598–604. doi: 10.1111/vec.12085
94. Martin LG, Luther TY, Alperin DC, Gay JM, Hines SA. Serum antibodies against human albumin in critically ill and healthy dogs. J Am Vet Med Assoc (2008) 232:1004–9. doi: 10.2460/javma.232.7.1004
95. Viganó F, Perissinotto L, Bosco VR. Administration of 5% human serum albumin in critically ill small animal patients with hypoalbuminemia: 418 dogs and 170 cats (1994-2008). J Vet Emerg Crit Care (2010) 20:237–43. doi: 10.1111/j.1476-4431.2010.00526.x
96. Quinn DG, Fennell JS, Sheils O, Gaffney EF, Feighery CF. Effect of cyclosporin on immune complex deposition in murine glomerulonephritis. Immunology (1991) 72:550–4.
97. Matthews JB, Fivaz BH, Sewell HF. Serum and salivary antibody responses and the development of oral tolerance after oral and intragastric antigen administration. Int Arch Allergy Immunol (1981) 65:107–13. doi: 10.1159/000232745
98. Thomas HC, Parrott MV. The induction of tolerance to a soluble protein antigen by oral administration. Immunology (1974) 27:631–9.
99. Nehring J, Schirmbeck LA, Friebus-Kardash J, Dubler D, Huynh-Do U, Chizzolini C, et al. Autoantibodies against albumin in patients with systemic lupus erythematosus. Front Immunol (2018) 9:2090. doi: 10.3389/fimmu.2018.02090
100. Tamura N, Suou T, Hirayama C. Anti-albumin antibodies in sera of patients with liver disease. Gastroenterol Jpn (1982) 17:469–75. doi: 10.1007/BF02774725
101. Chapman J, Maayan C, Michaelson DM. Antibodies to human serum albumin in familial dysautonomia. Int Arch Allergy Immunol (1993) 100:42–6. doi: 10.1159/000236385
102. Qian H, Cao Y, Sun J, Zu J, Ma L, Zhou H, et al. Anti-human serum albumin autoantibody may be involved in the pathogenesis of autoimmune bullous skin diseases. FASEB J (2020) 34(6):8574–95. doi: 10.1096/fj.201903247RR
103. Löwy I, Leclerc C, Chedid L. Induction of antibodies directed against self and altered-self determinants by a synthetic adjuvant, muramyl dipeptide and some of its derivatives. Immunology (1980) 39:441–50.
104. Westman E, Harris HE. Alteration of an autoantigen by chlorination, a process occurring during inflammation, can overcome adaptive immune tolerance. Scand J Immunol (2004) 59:458–63. doi: 10.1111/j.0300-9475.2004.01428.x
105. Vay D, Parodi M, Rolla R, Mottaran E, Vidali M, Bellomo G, et al. Circulating antibodies recognizing malondialdehyde-modified proteins in healthy subjects. Free Radic Biol Med (2001) 30:277–86. doi: 10.1016/S0891-5849(00)00469-X
106. Nakabo S, Hashimoto M, Ito S, Furu M, Ito H, Fujii T, et al. Carbamylated albumin is one of the target antigens of anti-carbamylated protein antibodies. Rheumatology (2017) 56(7):1217–26. doi: 10.1093/rheumatology/kex088
107. Khan M, Alam K, Ashraf GM, Rizvi MMA. Impact of hydroxyl radical modified-human serum albumin autoantigens in systemic lupus erythematosus. Curr Protein Pept Sci (2018) 19(9):881–88. doi: 10.2174/1389203718666170725115319
108. Karjalainen J, Martin JM, Knip M, Ilonen J, Robinson BH, Savilahti E, et al. A bovine albumin peptide as a possible trigger of insulin-dependent diabetes mellitus. N Engl J Med (1992) 327:302–7. doi: 10.1056/NEJM199207303270502
109. Chideckel EW. A bovine albumin peptide as a possible trigger of diabetes mellitus. N Engl J Med (1992) 327:1602–03. doi: 10.1056/NEJM199211263272217
110. Dosch HM. The possible link between insulin dependent (juvenile) diabetes mellitus and dietary cow milk. Clin Biochem (1993) 26(4):307–8. doi: 10.1016/0009-9120(93)90131-o
111. Ireland R. Bovine serum albumin: involved in childhood membranous nephropathy? Nat Rev Nephrol (2011) 7:423. doi: 10.1038/nrneph.2011.77
112. Debiec H, Lefeu F, Kemper MJ, Niaudet P, Deschenes G, Remuzzi G, et al. Early-childhood membranous nephropathy due to cationic bovine serum albumin. N Engl J Med (2011) 364(22):2101–10. doi: 10.1056/NEJMoa1013792
113. Valenta R, Duchêne M, Pettenburger K, Sillaber C, Valent P, Bettelheim P, et al. Identification of profilin as a novel pollen allergen; IgE autoreactivity in sensitized individuals. Science (1991) 253:557–60. doi: 10.1126/science.1857985
114. Mayer C, Appenzeller U, Seelbach H, Achatz G, Oberkofler H, Breitenbach M, et al. Humoral and cell-mediated autoimmune reactions to human acidic ribosomal P2 protein in individuals sensitized to aspergillus fumigatus P2 protein. J Exp Med (1999) 189:1507–12. doi: 10.1084/jem.189.9.1507
115. Mayer C, Appenzeller U, Seelbach H, Achatz G, Oberkofler H, Breitenbach M, et al. Humoral and cell-mediated autoimmunity in allergy to Aspergillus fumigatus. J Exp Med (1996) 184:265–70. doi: 10.1084/jem.184.1.265
116. Fluckiger S, Fijten H, Whitley P, Blaser K, Crameri R. Cyclophilins, a new family of cross-reactive allergens. Eur J Immunol (2002) 32:10–7. doi: 10.1002/1521-4141(200201)32:1<10::AID-IMMU10>3.0.CO;2-
117. Bunder R, Mittermann I, Herz U, Focke M, Wegmann M, Valenta R, et al. Induction of autoallergy with an environmental allergen mimicking a self protein in a murine model of experimental allergic asthma. J Allergy Clin Immunol (2004) 114(2):422–8. doi: 10.1016/j.jaci.2004.05.029
118. Valenta R, Natter S, Seiberler S, Wichlas S, Maurer D, Hess M, et al. Molecular characterization of an autoallergen, Hom s 1, identified by serum IgE from atopic dermatitis patients. J Invest Dermatol (1998) 111:1178–83. doi: 10.1046/j.1523-1747.1998.00413.x
119. Natter S, Seiberler S, Hufnagl P, Binder BR, Hirschl AM, Ring J, et al. Isolation of cDNA clones coding for IgE autoantigens with serum IgE from atopic dermatitis patients. FASEB J (1998) 12:1559–69. doi: 10.1096/fasebj.12.14.1559
120. Aichberger KJ, Mittermann I, Reininger R, Seiberler S, Swoboda I, Spitzauer S, et al. Hom s 4, an IgE-reactive autoantigen belonging to a new subfamily of calcium-binding proteins, can induce Th cell type 1-mediated autoreactivity. J Immunol (2005) 175:1286–94. doi: 10.4049/jimmunol.175.2.1286
121. Valenta R, Mittermann I, Werfel T, Garn H, Renz H. Linking allergy to autoimmune disease. Trends Immunol (2009) 30(3):109–16. doi: 10.1016/j.it.2008.12.004
122. Jansen K, Cevhertas L, Ma S, Satitsuksanoa P, Akdis M, van de Veen W. Regulatory B cells, A to Z. Allergy (2021) 76:2699–715. doi: 10.1111/all.14763
123. Ma S, Satitsuksanoa P, Jansen K, Cevhertas L, Veen W, Akdis M. B regulatory cells in allergy. Immunol Rev (2021) 299:10–30. doi: 10.1111/imr.12937
124. Akdis M, Akdis CA. Mechanisms of allergen-specific immunotherapy: Multiple suppressor factors at work in immune tolerance to allergens. J Allergy Clin Immunol (2014) 133:621–31. doi: 10.1016/j.jaci.2013.12.1088
125. Larche M, Wraith DC. Peptide-based therapeutic vaccines for allergic and autoimmune diseases. Nat Med (2005) 11:S69–76. doi: 10.1038/nm1226
126. Schurgers E, Wraith DC. Induction of tolerance to therapeutic proteins with antigen-processing independent T cell epitopes: controlling immune responses to biologics. Front Immunol (2021) 12:742695. doi: 10.3389/fimmu.2021.742695
127. Akinfenwa O, Huang H-J, Linhart B, Focke-Tejkl M, Vrtala S, Poroshina A, et al. Preventive administration of non-allergenic Bet v 1 peptides reduces allergic sensitization to major birch pollen allergen, Bet v 1. Front Immunol (2021) 12:744544. doi: 10.3389/fimmu.2021.744544
128. Dorofeeva Y, Shilovskiy I, Tulaeva I, Focke-Tejkl M, Flicker S, Kudlay D, et al. Past, present, and future of allergen immunotherapy vaccines. Allergy (2021) 76(1):131–49. doi: 10.1111/all.14300
129. Shamji MH, Valenta R, Jardetzky T, Verhasselt V, Durham SR, Wurtzen PA, et al. The role of allergen-specific IgE, IgG and IgA in allergic disease. Allergy (2021) 76:3627–41. doi: 10.1111/all.14908
130. Valenta R, Karaulov A, Niederberger V, Zhernov Y, Elisyutina O, Campana R, et al. Allergen extracts for in vivo diagnosis and treatment of allergy: Is there a future? J Allergy Clin Immunol Pract (2018) 6:1845–55.e2. doi: 10.1016/j.jaip.2018.08.032
Keywords: allergy, allergen, epitope, albumin, cross-reactivity, tolerance, antibody, immunoglobulin E (IgE)
Citation: Liu Z, Trifonova D, Tulaeva I, Riabova K, Karsonova A, Kozlov E, Elisyutina O, Khaitov M, Focke-Tejkl M, Chen T-H, Karaulov A and Valenta R (2023) Albumins represent highly cross-reactive animal allergens. Front. Immunol. 14:1241518. doi: 10.3389/fimmu.2023.1241518
Received: 16 June 2023; Accepted: 20 September 2023;
Published: 20 October 2023.
Edited by:
Christiane Hilger, Luxembourg Institute of Health, LuxembourgReviewed by:
Wayne Robert Thomas, University of Western Australia, AustraliaKavita Reginald, Sunway University, Malaysia
Copyright © 2023 Liu, Trifonova, Tulaeva, Riabova, Karsonova, Kozlov, Elisyutina, Khaitov, Focke-Tejkl, Chen, Karaulov and Valenta. This is an open-access article distributed under the terms of the Creative Commons Attribution License (CC BY). The use, distribution or reproduction in other forums is permitted, provided the original author(s) and the copyright owner(s) are credited and that the original publication in this journal is cited, in accordance with accepted academic practice. No use, distribution or reproduction is permitted which does not comply with these terms.
*Correspondence: Rudolf Valenta, UnVkb2xmLnZhbGVudGFAbWVkdW5pd2llbi5hYy5hdA==
†ORCID: Zicheng Liu, orcid.org/0009-0002-1155-954X
Daria Trifonova, orcid.org/0009-0002-4305-0529
Inna Tulaeva, orcid.org/0000-0002-5825-2687
Ksenja Riabova, orcid.org/0000-0006-0520-0936
Antonina Karsonova, orcid.org/0000-0002-8697-9816
Evgeny Kozlov, orcid.org/0000-0001-6244-1088
Olga Elisyutina, orcid.org/0000-0002-4609-2591
Musa Khaitov, orcid.org/0000-0003-4961-9640
Margarete Focke-Tejkl, orcid.org/0000-0003-3433-5071
Alexander Karaulov, orcid.org/0000-0002-1930-5424
Rudolf Valenta, orcid.org/0000-0001-5944-3365