- 1Department of Pharmacy, The First Hospital of Jiaxing, First Affiliated Hospital of Jiaxing University, Jiaxing, China
- 2College of Materials and Textile Engineering, Jiaxing University, Jiaxing, China
Allergen-specific immunotherapy (AIT) describes the establishment of peripheral tolerance through repeated allergen exposure, which qualifies as the only curative treatment for allergic diseases. Although conventional subcutaneous immunotherapy (SCIT) and sublingual immunotherapy (SLIT) have been approved to treat respiratory allergies clinically, the progress made is far from satisfactory. Epicutaneous immunotherapy (EPIT) exploits the skin’s immune properties to modulate immunological response, which is emerging as a promising alternative and has shown effectiveness in many preclinical and clinical studies for both respiratory and food allergies. It is worth noting that the stratum corneum (SC) barrier impedes the effective delivery of allergens, while disrupting the SC layer excessively often triggers unexpected Th2 immune responses. This work aims to comprehend the immunological mechanisms of EPIT, and summarize the innovative system for sufficient delivery of allergens as well as tolerogenic adjuvants. Finally, the safety, acceptability, and cost-effectiveness of these innovative delivery systems are discussed, which directs the development of future immunotherapies with all desirable characteristics.
Introduction
Immunoglobulin E (IgE)-mediated allergies affect more than 30% of the population worldwide that brings a considerable medical and socioeconomic burden (1). Current allergen-specific immunotherapy (AIT) describes the establishment of peripheral tolerance through repeated allergen exposure, which qualifies as the only curative treatment for allergic diseases (2, 3). Conventional AITs, such as subcutaneous immunotherapy (SCIT) and sublingual immunotherapy (SLIT) have been approved to treat allergic patients, but they require frequent dosing over long-term, and often provoke undesirable systemic or local reactions (4, 5). Therefore, only <5% of allergy sufferers choice AIT as second-line treatment. A critical need remains safety, efficacy and acceptability of AIT, which is largely dependent on how the allergen is presented to immune system, emphasizing the innovative route and form of allergen administration (6, 7).
Epicutaneous immunotherapy (EPIT) exerts tolerogenic effects by applying allergens topically on intact or pre-treated skin. Within the epidermis and dermis, allergens are taken up by skin antigen presenting cells (APCs), such as Langerhans cells (LCs) and dermal dendritic cells (DCs) (8, 9), subsequently migrate through the dermis to local draining lymph nodes (LNs), where they can elicit T cell polarization and tolerance (10). The first study of successful EPIT dates back to 1921, and it was observed that applying allergens onto scarified skin reduced allergic symptoms in patients with a horse allergy (11). The development of tolerogenic effects is mediated by CD4+ CD25+ Foxp3+ Tregs and secreting TGF-β. Mouse experiments showed that Tregs required CTLA-4 surface marker expression but not IL-10 (12). Both humoral and T-cellular effects have been noticed after EPIT, including the generation of Tregs, the induction of specific IgG2a and a diminished Th2 immune response (13).
EPIT has shown varying degrees of success in phase II/III clinical trials (13, 14), and simultaneously satisfies the urgent need of needle-free and self-administration. Since allergens are introduced into epidermis, the non-vascularized skin layer can lower the antigen leakage into systemic circulation and minimize the risk of adverse events, promoting the clinical medication safety (14–16). Moreover, EPIT fully exploits the skin’s innate immune properties, and the high density of APCs in epidermis and dermis implies smaller amounts of antigen to activate immune response, compared to subcutaneous injections (17, 18). However, the exist of stratum corneum (SC) impedes epidermal delivery of allergen via intact skin, whereas damaging this barrier often results in unwanted Th2 responses (19, 20). Thus, the desirable EPIT must keep a balance between skin disruption and allergen delivery. In this work, we review the immunological mechanisms, and summarize the innovative delivery system for allergens as well as tolerogenic adjuvants in preclinical and clinical EPIT (Tables 1, 2). The safety, acceptability, and cost-effectiveness of these novel systems are appraised (Table 3), which directs the development of future immunotherapies with all desirable characteristics.
The Viaskin epicutaneous delivery system
Initially, the skin was prepared by adhesive tape-stripping to remove SC and enhance solvent allergen delivery, whereas the majority of adults reported local reactions and systemic Th2 response, with more than 70% eczema at the treated area (21–23). To minimize Th2 immune responses, EPIT is performed onto intact skin through Viaskin epicutaneous delivery system (DBV Technologies, France) in most preclinical and clinical trials (14). It is engineered by electrostatic spraying powdered allergens onto a transparent plastic membrane. When applied on the skin, Viaskin creates an enclosed chamber and utilizes transepidermal water loss to increase permeability of SC (Figure 1A) (13, 17).
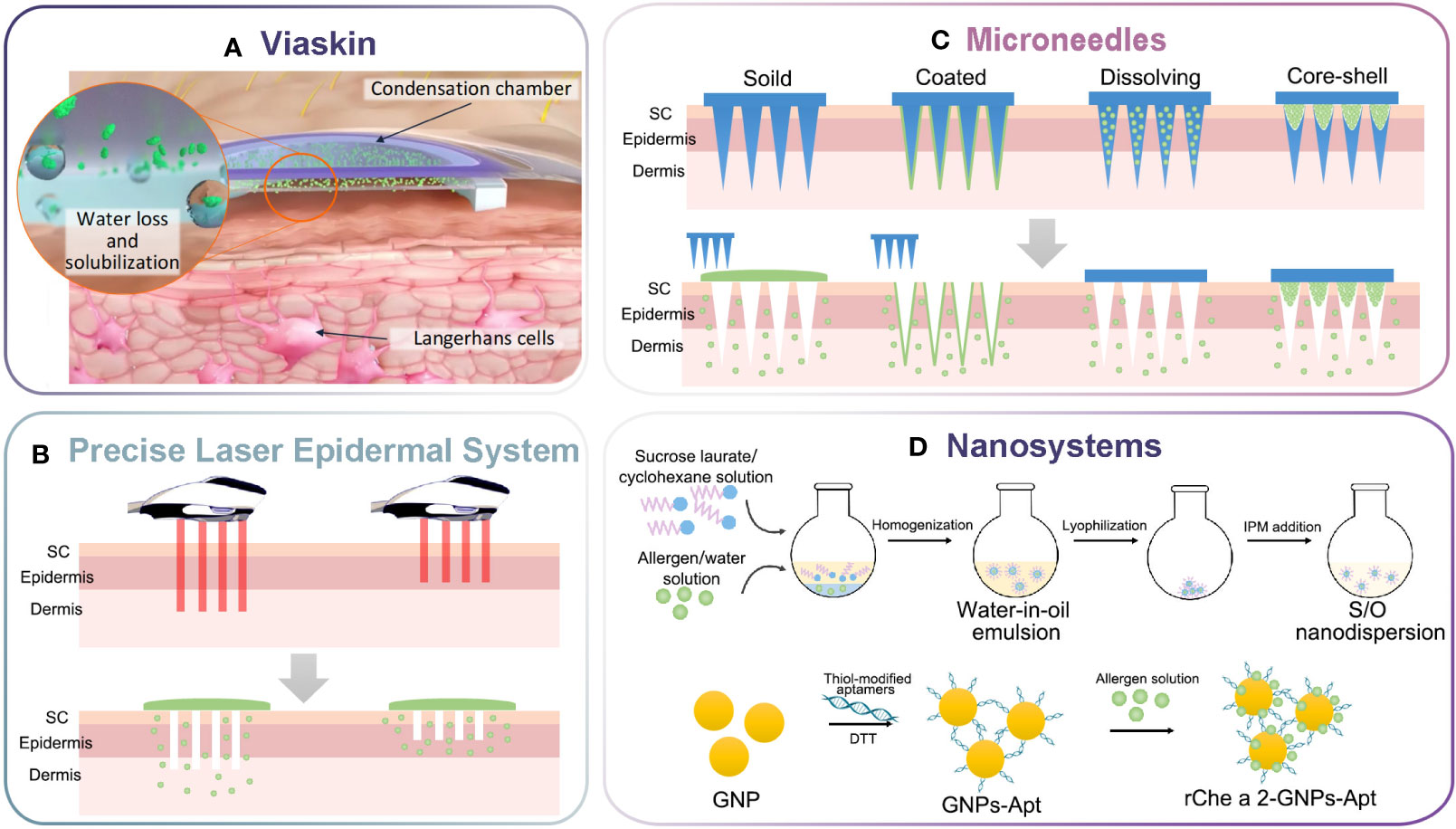
Figure 1 Schematic illustration of innovative delivery systems for EPIT. (A) Viaskin system (https://dbv-technologies.com). (B) Precise laser epidermal system. (C) Microneedle delivery system. (D) Nano-drug delivery system.
Mondoulet and Dioszeghy group evaluated Viaskin system in mouse models with respiratory and food allergies. In mice sensitized to house dust mite (HDM), pollen, ovalbumin (OVA) or peanut, EPIT had equivalent curative effect to SCIT, considered as the reference immunotherapy (45). The repeated applications of Viaskin patches should be performed on intact skin to insure the safety and efficacy, but not stripped skin (20). What was more, EPIT was at least as efficient as SLIT in mice sensitized to Phleum pratense pollen (46). The EPIT-induced Tregs were still effective eight weeks after the end of treatment, whereas that in SLIT lost their suppressive activities (12). In peanut-sensitized mice, the Tregs induction by EPIT also mediated long-term protection from eosinophilic disorders (47).
The characteristics of EPIT trials were summarized in Table 1. The Viaskin patches were preferentially developed for the treatment of food allergies. In phase I study (NCT01170286), the safety and tolerability of Viaskin peanut were evaluated at doses of 20, 50, 200 or 500 μg, as well as placebo. The localized treatment-emergent adverse events (L-TEAE) that emerged during the study were primarily mild to moderate, affecting 86.3% of peanut-treated patients compared to 60% of placebo-treated individuals. Peanut EPIT through Viaskin system on intact skin was safe and well tolerated, with high adherence by participants (24). Subsequent phase II studies were conducted to evaluate therapy efficacy and dose variation effect on adults or children. Both studies demonstrated significant absolute difference in response rates of 53.6% in children aged 6-11, and 38.9% in adolescents aged 12-17, with daily 24 h application (250 µg) for 12 months (25). The compliance rate was more than 97% across all cohorts. However, adults showed no significant response to treatment. To assess the efficacy and adverse events further, a phase 3, randomized, double-blind, placebo-controlled trial was conducted with children aged 4-11 years (NCT02636699). Under daily treatment with Viaskin peanut (250 µg) for 12 months, the responder rate was found to be 35.3% in the peanut group, compared to only 13.6% with placebo, but did not meet the prespecified lower bound of the confidence interval criterion for a positive trial result (28). The incidence rate of TEAE was 95.4% in peanut group vs 89% in placebo group, and all of them were mild or moderate severity. Then, subjects who successfully completed the 12-months study were enrolled in an additional 2 years with daily treatment (NCT03013517). At month 36, 75.9% of patients demonstrated increased eliciting dose compared with baseline, and 51.8% of subjects reached an eliciting dose of ≥1000 mg, compared with 40.4% at month 12. There was no treatment-related epinephrine use during treatment. The compliance for Viaskin was high (96.9%), and withdrawals were lower than 1% due to TEAE. For children younger than 4 years of age, a phase 3 trial was also carried out (NCT03211247). The primary efficacy end point result was observed in 67.0% of toddlers in the intervention group, compared to 33.5% with placebo (31). Additionally, Viaskin has been used on intact skin to treat children with cow’s milk allergy and milk-induced eosinophilic esophagitis (23, 27, 48).
Viaskin-mediated EPIT successfully increases tolerance for food allergy without any serious anaphylaxis incidents during study, suggesting the advantages of high safety and evident efficacy. The comparison of efficiency between EPIT and SCIT in clinical is not available, because SCIT for food allergy has been abandoned given the significant rate of severe, systemic reactions. Similarly, there is no head-to-head comparison of Viaskin-EPIT to other routes of administration, except in mouse models. What is more, Viaskin-EPIT is easy to use with minimal restrictions on daily activities, resulting in high compliance rates. However, the SC poses a major barrier, and passive diffusion of allergens through intact skin was less than 10% after 24 h application (49). It can explain the moderate efficacy in clinical trials, whereas the prolonged application leads to significant skin irritation. On the other hand, the morphological differences of skins might cause less consistent outcomes across different populations (25). This suggests that responses to Viaskin-mediated EPIT might be more robust in younger patients, so the phase 3 trial only limits to children (26). Moreover, Viaskin patch is restricted to deliver allergen powders only, so it is challenging to introduce tolerogenic adjuvants in the system.
Precise laser epidermal system
Ablative fractional laser (AFL) creates microchannels in epidermal or dermal layers to enhance transcutaneous drug delivery, particularly for biomacromolecules (Figure 1B). It targets hydrophilic cutaneous tissue and emits energy to explosive evaporation of water, which creates aqueous micropores (about 50-150 µm in diameter) in the skin with minimized thermal tissue damage (50). Subsequently, the aqueous solution or powder of allergens are applied onto the created micropores. The commercially available precise laser epidermal system (P.L.E.A.S.E.) allow to vary the density and depth of micropores, resulting from the adjustable parameters such as the number of microchannels per surface area, number of pulses per microchannel (51). Unlike tape-stripping, the P.L.E.A.S.E. device achieve selective ablation of epidermal and dermal layers, enabling more individualized skin targeting (52).
In early studies, the aqueous solution of OVA and Phl p 5 were applied onto the AFL-treated skin. Immunizations with OVA or Phl p 5 led to a distinct Th2 immune response, while the CpG addition manipulated it towards Th1 milieu, with increased IgG2a secretion and decreased IgE, IL-4, IL-5 and IL-13 (33, 53). We inferred that micropores with depths of 30-40 µm went through the epidermis to basement membrane, and the allergen exposure to dermis might induce sensitization (17). Alternatively, Korotchenko et al. created micropores with well-defined depth and density using P.L.E.A.S.E. device to facilitate the delivery and uptake of topically applied allergens, which was emerging as an alternative to classical SCIT or SLIT for HDM-induced lung inflammation (32).
The powder delivered within the microchannels could be hydrated by interstitial fluid, then dissolved and spread over the epidermis with minimal leakage to circulating system, whereas aqueous allergens diffused quickly (34). As captured by confocal microscopy, the GFP+ APCs were attracted and accumulated around individual microchannel. It increased rapidly and reached the peak on 3 days, and declined over 6 to 10 days (54, 55). Motivated by these hypotheses, Kumar et al. used P.L.E.A.S.E. to generate micropores with a size of 50-75 μm in base diameter and 20-30 μm in depth, then a mixture powder of OVA, 1,25-Dixydroxyvitamin D3 (VD3), and CpG was applied onto the microchannels for 2 h. The topical application promoted high levels of epidermal delivery, and the retained powder was beneficial to create an “antigen-depot” effect and stimulated the immune system continuously for a long time. Only three times of EPIT significantly suppressed airway hyperresponsiveness and lung inflammation of OVA-sensitized mice, which was unattainable by SCIT. The EPIT with OVA-CpG-VD3 induced higher Tregs, and favored IgG2a expression from Th1-biased immune response (34).
Enhanced and controlled activation of DC may be achieved through the specific targeting of surface receptors such as C-type lectin receptors. The P.L.E.A.S.E. device allows for effective delivery of DC-targeted allergens into the epidermis, thereby increasing the immune protection and reducing side effects. In a study by Korotchenko et al., laminarin-ovalbumin neoglycoconjugates (LamOVA) were synthesized using a 2-step reductive amination method, followed by analysis of their immunogenicity, allergenicity, and therapeutic efficacy. Laminarin conjugation to OVA significantly facilitated uptake by bone marrow-derived dendritic cells (BMDCs), leading to their activation. The LamOVA conjugates showed a five-fold reduction in IgE binding capacity, while their immunogenicity increased to three-fold. What was more, EPIT with LamOVA induced higher IgG and suppressed lung inflammation, which was equally effective as SCIT, although the latter was associated with elevated IgE and Th2 cytokines (18). Similarly, mannan conjugates are known to enhance allergen uptake by skin DCs, particularly LCs and CD14+ dermal DCs (56). For instance, Mannan-Bet v 1 was synthesized using mild oxidation and reductive amination, then applied to P.L.E.A.S.E.-treated mouse skin (57). The allergenicity of Mannan-Bet v 1 was about six orders of magnitude lower than that of soluble Bet v 1, making it less likely to trigger IgE-dependent responses. Furthermore, the same conjugation approach could be applied to hypoallergenic Phl p 5 (33).
Briefly, the precise laser epidermal system increased the quantity of antigen delivered into the epidermis without systemic passage. As a needle-free and painless approach, it has potential to replace standard methods due to the improved safety and optimal compliance. From a research point of view, the adaptable parameters will allow us to investigate the underlying immunological mechanisms and design the vaccination strategies rationally. Moreover, tailored DC targeting with polysaccharide conjugation improved uptake and increased the level of DC activation specifically (52). Despite the potential benefits of P.L.E.A.S.E. device in therapy, challenges such as inconvenience and high cost still need to be addressed before it can be widely practiced in self-administration.
Microneedle delivery system
Microneedles have emerged as an attractive platform for transdermal or topical drug delivery, comprising solid, coated, dissolving, and hollow microneedles. These systems can pierce through SC layer perpendicularly, and selectively deliver the allergens and adjuvants into epidermis or dermis for uptake by skin APCs (58). Since the performance is not affected by skin permeabilities between children and adults, microneedles allow strict dose control, and reduce application time from 24 h to 3-5 min (59). This not only improves the reliability and compliance of immunotherapy but also renders them painless, non-invasive, safe, and tolerable across all age groups (60). The following sections highlights the microneedles as an innovative delivery system in EPIT (Figure 1C).
In earlier studies, solid microneedles were employed to penetrate the skin resembling AFL, followed by topically allergens application. However, these studies were not involved in sensitized animal models. Coated microneedles were introduced next, in which the allergen was coated on the surface and rapidly dissolved in the aqueous environment of skin tissue, allowing for freely delivery of allergens into epidermis/dermis. Studies by Gill group utilized stainless-steel, dip-coated microneedles for the treatment of respiratory and food allergies in mouse models (35, 36, 39–41). In a pioneering study, the peanut protein-coated microneedles (5 μg) were performed once weekly for 3 weeks in peanut-sensitized mice (35). After oral challenge, clinical symptoms of peanut-induced anaphylaxis were significantly reduced following treatment, accompanied with the higher IgG1 and IgG2a. The analysis of splenocyte culture supernatants showed elevated levels of IL-2 and IFN-γ, but decreased levels of IL-4 and IL-5 compared to untreated group, indicating a Th1 dominant response. Landers et al. directly compared the therapeutic benefit of coated microneedles (11 μg) to EPIT (100 μg) in peanut sensitized mice and found that treatment with microneedles was safe and resulted in enhanced desensitization compared to EPIT (36). The mice with coated microneedles were better protected from body temperature drop and anaphylaxis symptom scores upon oral challenge, while those with EPIT exhibited allergic symptoms. These findings suggested that coated microneedles had the potential to deliver allergens into the skin, thereby improving immune modulation and efficacy.
The Gill group also evaluated both the therapeutic and prophylactic capabilities of coated microneedles in mouse models of airway allergy (39–41). In a comparison between microneedles (OVA + CpG) and SCIT (OVA + Alum), mice receiving OVA + CpG were found to induce higher levels of OVA-specific IgG1 compared to those treated with SCIT (41). The therapeutic capacity was evidenced by the suppression of airway inflammation upon intranasal OVA challenge, with reduction of eosinophils in the lung tissues, and low deposition of mucus inside lung bronchioles. Coated microneedles also demonstrated prophylactic efficacy to prevent the allergy progression of mice that were vaccinated with OVA + CpG, as confirmed by the regulated Th2 cytokines and anti-inflammatory cytokines in BALF (40). In another study, Gill et al. examined the effectiveness of microneedles in delivering Der p1 into mouse skin to prevent the development of Der p1-induced airway allergy (39). Post challenge, mice vaccinated with Der p 1 + CpG-coated microneedles showed a greater IgG2a response than the Der p 1-coated microneedles and SCIT groups, suggesting the beneficial role of CpG for allergy vaccination. Microneedles were minimally invasive, painless, and self-administration, making them a user-friendly alternative to SCIT. However, the system used in these studies were about 700 μm in length, which increased the possibility that allergen spreading in the dermis could reach the bloodstream and cause anaphylaxis.
The dissolving microneedles were demonstrated to avoid biohazardous wastes and promote drug loading (59). Park et al. designed Der f 1-loaded sodium hyaluronate microneedles using the droplet-born air blowing method, and compared their efficacy to conventional SCIT in murine asthma model (38). The Der f 1-loaded biodegradable microneedles alleviated airway hyperresponsiveness, eosinophilic infiltration, goblet cell hyperplasia and subepithelial fibrosis. These changes were more significant in the low-dose (10 μg) microneedle group than in high-dose (100 μg) SCIT group. In another study, a powder-laden, dissolving microneedle arrays (PLD-MNA) were engineered to deliver the aforementioned powder allergens into epidermis. The outer shell of PLD-MNA was made of highly biocompatible carboxymethyl cellulose, and the concave was filled directly with powder of peanut protein, VD3 and CpG. Notably, the immunogenicity of lyophilized allergens was fully preserved, while more than 50% immunogenicity could be lost with repeated dissolving and drying during the fabrication of coated and dissolving microneedles. After skin insertion, PLD-MNA deposited the powder in to epidermis with minimal leakage to the circulation system and attracted a large number of APCs (54). In the preclinical study, microneedle-mediated immunotherapy produced enhanced outcomes when compared to sham treatments, with lower peanut-specific serum IgE, and decreased infiltration of intestinal mucosal mast cells and eosinophils. PLD-MNA required only 6 treatments and one-fifth of therapeutic dose, with improved outcomes compared to 12 intradermal immunotherapies.
Nano-drug delivery system
Nano-based drug delivery systems can interact with skin components in a way, offering an attractive alternative for overcoming the limited skin penetration of molecules (61). A variety of drug nanocarriers, such as lipid nanoparticles, organic-inorganic nanoparticles, dendrimers and micelles, have been developed for both topical and transdermal delivery of therapeutics. In this review, we focus specifically on the performance of nanosystems in EPIT.
The solid-in-oil (S/O) and gel-in-oil (G/O) nanodispersion was proposed as a means of improving the dispersibility of hydrophilic allergens into an oil phase, thereby breaking down the skin barrier and enhancing drug permeability (62). Initially, Kitaoka et al. incorporated CpG with OVA into S/O nanodispersion for transcutaneous immunization, and investigated the effects on Th1/Th2 immune balance (63). It was noting that the permeability of ~40 kDa proteins remained lower than that of small size after application (62, 64). Subsequently, β-lactoglobulin (BLG) nanoparticles were prepared and dispersed into oil isopropyl myristate (IPM) with the aid of Sucrose laurate L-195 (Figure 1D). The S/O nanodispersion enhanced the skin permeation of BLG into the dermis, and skewed the immune response toward Th1 immunity, indicating the amelioration of allergic symptoms. This effect was reinforced when resiquimod (R848) was included in IPM (42). Likewise, the T cell epitope peptide (7CrpR) was efficiently delivered via the S/O nanodispersion and decreased IgE levels in pollinosis model mice. The addition of immunomodulator R848 shifted the Th1/Th2-balance toward Th1-type immunity significantly, demonstrating potential for alleviating Japanese cedar pollinosis (43, 65).
Koushki et al. developed a targeted delivery system comprised of functionalized gold nanoparticles along with the DC-specific aptamers and rChe a 2 allergen (rChe a 2-GNPs-Apt) (Figure 1D) (44). Additionally, skin-penetrating peptides (SPP) were topically applied to enhance skin permeability and therapeutic efficacy. Results demonstrated that the rChe a 2-GNPs-Apt stimulated the secretion of IFN-γ, TGF-β, and IL-10 in splenocyte culture supernatants, reduced IgE, IL-4 and IL-17a levels, as well as eosinophil cell counts in nasal lavage fluid (NALF), compared to non-targeted groups. Besides, microemulsions have great potential as a protein-containing drug delivery system due to their natural penetration enhancement, thermodynamic stability, and solubilization capacity. Kiselmann et al. focused on the bee-venom phospholipase A2 (Api m 1) and created a skin-friendly microemulsion via Phase Diagram via Micro Plate Dilution (PDMPD)-method, allowing the model allergen to penetrate epidermal layers and reach LC and dDC. However, subsequent in vivo studies were needed to investigated the protective therapeutic effects of Api m 1/microemulsion (66).
We highlight the potential of nanosystems in skin permeation and allergens/adjuvants delivery. However, there is still much to be understood. For instance, the physicochemical properties of nanocarriers including size, shape, rigidity, and surface charge can interact with the biological components and affect skin penetration. In this sense, better understanding on their physicochemical properties is necessary for precise epidermal delivery. Moreover, the antigen presentation, maturation and migration, and induction of T-cell differentiation requires proximity between APCs and nanoparticles. The presence of nanoparticles can alter APC functions, which is essential for a well-oriented and effective immune response. Besides, only a few nanocarriers have been approved as pharmaceutical products, due to issues like large-scale synthesis, stability. Bringing a nanosystem for EPIT from bench to market remains a lengthy process.
Conclusion
AIT remains the only disease-modifying treatment capable of achieving long-term desensitization in allergy patients. Although various AIT systems, such as SCIT and SLIT, have been clinically approved to treat respiratory allergies, their frequent local or systemic side effects, as well as the substantial time, monetary, and effort commitments of patients pose certain limitations. Skin-based AIT systems have emerged as an alternative option in many preclinical and clinical studies. While no EPIT platform has been FDA-approved up to date, numerous encouraging studies suggest that EPIT could be a future trend for both respiratory and food allergies. The Viaskin, precise laser epidermal system, microneedles and nano-drug delivery system can sufficiently deliver allergens into epidermis with minimal skin reaction and rewrites the immunological response. Furthermore, addition of adjuvants in EPIT represents another strategy to enhance efficacy and safety. Overall, the innovative delivery systems for EPIT will allow for convenient, non-invasive, and self-administrable modalities of treatment.
Author contributions
All the authors participate in initial discussion and composition of the review. ZW wrote the first draft and summarized Table 1, 2. LW summarized the characteristics of these innovative delivery systems in Table 3 and Figure 1. WW provided useful suggestions and finalized the review. All authors contributed to drafting this review and approved its final version.
Funding
This work is supported by Jiaxing Science and Technology Planning Project (2023AY11012), “Pioneer” and “Leading Goose” R&D Program of Zhejiang Province (2023C01201), Jiaxing Key Discipline of Medicine (2023-ZC-008) and Basic Public Welfare Research Project of Zhejiang Province (LGD21H310003).
Acknowledgments
The authors gratefully acknowledge the Jiaxing Science and Technology Planning Project (2023AY11012), “Pioneer” and “Leading Goose” R&D Program of Zhejiang Province (2023C01201), Jiaxing Key Discipline of Medicine (2023-ZC-008) and Basic Public Welfare Research Project of Zhejiang Province (LGD21H310003).
Conflict of interest
The authors declare that the research was conducted in the absence of any commercial or financial relationships that could be construed as a potential conflict of interest.
Publisher's note
All claims expressed in this article are solely those of the authors and do not necessarily represent those of their affiliated organizations, or those of the publisher, the editors and the reviewers. Any product that may be evaluated in this article, or claim that may be made by its manufacturer, is not guaranteed or endorsed by the publisher.
References
1. Cardona V, Ansotegui IJ, Ebisawa M, El-Gamal Y, Fernandez Rivas M, Fineman S, et al. World allergy organization anaphylaxis guidance 2020. World Allergy Organ J (2020) 13(10):100472. doi: 10.1016/j.waojou.2020.100472
2. Celebi Sozener Z, Mungan D, Cevhertas L, Ogulur I, Akdis M, Akdis C. Tolerance mechanisms in allergen immunotherapy. Curr Opin Allergy Clin Immunol (2020) 20(6):591–601. doi: 10.1097/ACI.0000000000000693
3. Dorofeeva Y, Shilovskiy I, Tulaeva I, Focke-Tejkl M, Flicker S, Kudlay D, et al. Past, presence, and future of allergen immunotherapy vaccines. Allergy (2021) 76(1):131–49. doi: 10.1111/all.14300
4. Penagos M, Durham SR. Allergen immunotherapy for long-term tolerance and prevention. J Allergy Clin Immunol (2022) 149(3):802–11. doi: 10.1016/j.jaci.2022.01.007
5. Nelson HS. Allergy immunotherapy: future directions for the 2020s. Allergy Asthma Proc (2020) 41(5):314–25. doi: 10.2500/aap.2020.41.200041
6. Pfutzner W, Mobs C. Ait: new avenues in allergen immunotherapy. Handb Exp Pharmacol (2022) 268:135–49. doi: 10.1007/164_2021_514
7. Incorvaia C, Al-Ahmad M, Ansotegui IJ, Arasi S, Bachert C, Bos C, et al. Personalized medicine for allergy treatment: allergen immunotherapy still a unique and unmatched model. Allergy (2021) 76(4):1041–52. doi: 10.1111/all.14575
8. Luo Y, Wang S, Liu X, Wen H, Li W, Yao X. Langerhans cells mediate the skin-induced tolerance to ovalbumin via langerin in a murine model. Allergy (2019) 74(9):1738–47. doi: 10.1111/all.13813
9. Wavrin S, Mondoulet L, Dioszeghy V, Puteaux E, Ligouis M, Dhelft V, et al. Allergen capture by dcs during epicutaneous immunotherapy is enhanced by modulating the dose and the surface area of the patch. J Allergy Clin Immunol (2017) 139(2):AB257. doi: 10.1016/j.jaci.2016.12.827
10. Laoubi L, Lacoffrette M, Valsesia S, Lenief V, Guironnet-Paquet A, Mosnier A, et al. Epicutaneous allergen immunotherapy induces a profound and selective modulation in skin dendritic-cell subsets. J Allergy Clin Immunol (2022) 150(5):1194–208. doi: 10.1016/j.jaci.2022.05.025
11. Lanser BJ, Leung DYM. The current state of epicutaneous immunotherapy for food allergy: A comprehensive review. Clin Rev Allergy Immunol (2018) 55(2):153–61. doi: 10.1007/s12016-017-8650-3
12. Dioszeghy V, Mondoulet L, Puteaux E, Dhelft V, Ligouis M, Plaquet C, et al. Differences in phenotype, homing properties and suppressive activities of regulatory T cells induced by epicutaneous, oral or sublingual immunotherapy in mice sensitized to peanut. Cell Mol Immunol (2017) 14(9):770–82. doi: 10.1038/cmi.2016.14
13. Langlois A, Graham F, Begin P. Epicutaneous peanut patch device for the treatment of peanut allergy. Expert Rev Clin Immunol (2019) 15(5):449–60. doi: 10.1080/1744666X.2019.1593138
14. Xiong L, Lin J, Luo Y, Chen W, Dai J. The efficacy and safety of epicutaneous immunotherapy for allergic diseases: A systematic review and meta-analysis. Int Arch Allergy Immunol (2020) 181(3):170–82. doi: 10.1159/000504366
15. Guttman-Yassky E, Zhou L, Krueger JG. The skin as an immune organ: tolerance versus effector responses and applications to food allergy and hypersensitivity reactions. J Allergy Clin Immunol (2019) 144(2):362–74. doi: 10.1016/j.jaci.2019.03.021
16. Doebel T, Voisin B, Nagao K. Langerhans cells - the macrophage in dendritic cell clothing. Trends Immunol (2017) 38(11):817–28. doi: 10.1016/j.it.2017.06.008
17. Bird JA, Sanchez-Borges M, Ansotegui IJ, Ebisawa M, Ortega Martell JA. Skin as an immune organ and clinical applications of skin-based immunotherapy. World Allergy Organ J (2018) 11(1):38. doi: 10.1186/s40413-018-0215-2
18. Korotchenko E, Schiessl V, Scheiblhofer S, Schubert M, Dall E, Joubert IA, et al. Laser-facilitated epicutaneous immunotherapy with hypoallergenic beta-glucan neoglycoconjugates suppresses lung inflammation and avoids local side effects in a mouse model of allergic asthma. Allergy (2021) 76(1):210–22. doi: 10.1111/all.14481
19. Senti G, Kundig TM. Novel delivery routes for allergy immunotherapy: intralymphatic, epicutaneous, and intradermal. Immunol Allergy Clin North Am (2016) 36(1):25–37. doi: 10.1016/j.iac.2015.08.006
20. Mondoulet L, Dioszeghy V, Puteaux E, Ligouis M, Dhelft V, Letourneur F, et al. Intact skin and not stripped skin is crucial for the safety and efficacy of peanut epicutaneous immunotherapy (Epit) in mice. Clin Transl Allergy (2012) 2(1):22. doi: 10.1186/2045-7022-2-22
21. Senti G, Graf N, Haug S, Rüedi N, von Moos S, Sonderegger T, et al. Epicutaneous allergen administration as a novel method of allergen-specific immunotherapy. J Allergy Clin Immunol (2009) 124(5):997–1002. doi: 10.1016/j.jaci.2009.07.019
22. Senti G, von Moos S, Tay F, Graf N, Sonderegger T, Johansen P, et al. Epicutaneous allergen-specific immunotherapy ameliorates grass pollen-induced rhinoconjunctivitis: A double-blind, placebo-controlled dose escalation study. J Allergy Clin Immunol (2012) 129(1):128–35. doi: 10.1016/j.jaci.2011.08.036
23. Senti G, von Moos S, Tay F, Graf N, Johansen P, Kundig TM. Determinants of efficacy and safety in epicutaneous allergen immunotherapy: summary of three clinical trials. Allergy (2015) 70(6):707–10. doi: 10.1111/all.12600
24. Jones SM, Agbotounou WK, Fleischer DM, Burks AW, Pesek RD, Harris MW, et al. Safety of epicutaneous immunotherapy for the treatment of peanut allergy: A phase 1 study using the viaskin patch. J Allergy Clin Immunol (2016) 137(4):1258. doi: 10.1016/j.jaci.2016.01.008
25. Sampson HA, Shreffler WG, Yang WH, Sussman GL, Brown-Whitehorn TF, Nadeau KC, et al. Effect of varying doses of epicutaneous immunotherapy vs placebo on reaction to peanut protein exposure among patients with peanut sensitivity a randomized clinical trial. J Am Med Assoc (2017) 318(18):1798–809. doi: 10.1001/jama.2017.16591
26. Jones SM, Sicherer SH, Burks AW, Leung DYM, Lindblad RW, Dawson P, et al. Epicutaneous immunotherapy for the treatment of peanut allergy in children and young adults. J Allergy Clin Immunol (2017) 139(4):1242. doi: 10.1016/j.jaci.2016.08.017
27. Spergel JM, Elci OU, Muir AB, Liacouras CA, Wilkins BJ, Burke D, et al. Efficacy of epicutaneous immunotherapy in children with milk-induced eosinophilic esophagitis. Clin Gastroenterol Hepatol (2020) 18(2):328. doi: 10.1016/j.cgh.2019.05.014
28. Fleischer DM, Greenhawt M, Sussman G, Begin P, Nowak-Wegrzyn A, Petroni D, et al. Effect of epicutaneous immunotherapy vs placebo on reaction to peanut protein ingestion among children with peanut allergy the pepites randomized clinical trial. J Am Med Assoc (2019) 321(10):946–55. doi: 10.1001/jama.2019.1113
29. Greenhawt M, Kim EH, Campbell DE, Green TD, Lambert R, Fleischer DM. Improvements in eliciting dose across baseline sensitivities following 12 months of epicutaneous immunotherapy (Epit) in peanut-allergic children aged 4 to 11 years. J Allergy Clin Immunol Pract (2020) 8(9):3219–21. doi: 10.1016/j.jaip.2020.05.030
30. Fleischer DM, Shreffler WG, Campbell DE, Green TD, Anvari S, Assa’ad A, et al. Long-term, open-label extension study of the efficacy and safety of epicutaneous immunotherapy for peanut allergy in children: people 3-year results. J Allergy Clin Immunol (2020) 146(4):863–74. doi: 10.1016/j.jaci.2020.06.028
31. Fleischer D, Green T, Sampson H, Meney J, Bahnson H, Burks AW. Efficacy and safety of epicutaneous immunotherapy (Epit) for peanut allergy in subjects aged 1-3 years with and without concomitant food allergies in the epitope study. J Allergy Clin Immunol (2023) 151(2). doi: 10.1016/j.jaci.2022.12.088
32. Korotchenko E, Moya R, Scheiblhofer S, Joubert IA, Horejs-Hoeck J, Hauser M, et al. Laser-facilitated epicutaneous immunotherapy with depigmented house dust mite extract alleviates allergic responses in a mouse model of allergic lung inflammation. Allergy (2020) 75(5):1217–28. doi: 10.1111/all.14164
33. Hessenberger M, Weiss R, Weinberger EE, Boehler C, Thalhamer J, Scheiblhofer S. Transcutaneous delivery of cpg-adjuvanted allergen via laser-generated micropores. Vaccine (2013) 31(34):3427–34. doi: 10.1016/j.vaccine.2012.09.086
34. Kumar MNK, Zhou C, Wu MX. Laser-facilitated epicutaneous immunotherapy to ige-mediated allergy. J Control Release (2016) 235:82–90. doi: 10.1016/j.jconrel.2016.05.057
35. Shakya AK, Ingrole RSJ, Joshi G, Uddin MJ, Anvari S, Davis CM, et al. Microneedles coated with peanut allergen enable desensitization of peanut sensitized mice. J Control Release (2019) 314:38–47. doi: 10.1016/j.jconrel.2019.09.022
36. Landers JJ, Janczak KW, Shakya AK, Zarnitsyn V, Patel SR, Baker JR, et al. Targeted allergen-specific immunotherapy within the skin improves allergen delivery to induce desensitization to peanut. Immunotherapy (2022) 14(7):539–52. doi: 10.2217/imt-2021-0206
37. Yu Y, KIran Kumar MN, Wu MX. Delivery of allergen powder for safe and effective epicutaneous immunotherapy. J Allergy Clin Immunol (2020) 145(2):597–609. doi: 10.1016/j.jaci.2019.11.022
38. Park KH, Oh EY, Han H, Kim JD, Kim SJ, Jeong KY, et al. Efficacy of transdermal immunotherapy with biodegradable microneedle patches in a murine asthma model. Clin Exp Allergy (2020) 50(9):1084–92. doi: 10.1111/cea.13688
39. Shakya AK, Lee CH, Gill HS. Coated microneedle-based cutaneous immunotherapy prevents der P 1-induced airway allergy in mice. J Allergy Clin Immunol (2018) 142(6):2007–11 e3. doi: 10.1016/j.jaci.2018.08.017
40. Shakya AK, Lee CH, Gill HS. Cutaneous vaccination with coated microneedles prevents development of airway allergy. J Control Release (2017) 265:75–82. doi: 10.1016/j.jconrel.2017.08.012
41. Shakya AK, Lee CH, Gill HS. Microneedle-mediated allergen-specific immunotherapy for the treatment of airway allergy in mice. Mol Pharm (2020) 17(8):3033–42. doi: 10.1021/acs.molpharmaceut.0c00447
42. Kitaoka M, Xiao W, Kong Q, Tahara Y, Kamiya N, Goto M. A solid-in-oil nanodispersion system for transcutaneous immunotherapy of cow’s milk allergies. Pharmaceutics (2020) 12(3). doi: 10.3390/pharmaceutics12030205
43. Kitaoka M, Naritomi A, Kawabe Y, Kamihira M, Kamiya N, Goto M. Transcutaneous pollinosis immunotherapy using a solid-in-oil nanodispersion system carrying T cell epitope peptide and R848. Bioeng Transl Med (2017) 2(1):102–8. doi: 10.1002/btm2.10048
44. Koushki K, Varasteh AR, Shahbaz SK, Sadeghi M, Mashayekhi K, Ayati SH, et al. Dc-specific aptamer decorated gold nanoparticles: A new attractive insight into the nanocarriers for allergy epicutaneous immunotherapy. Int J Pharm (2020) 584:119403. doi: 10.1016/j.ijpharm.2020.119403
45. Mondoulet L, Dioszeghy V, Ligouis M, Dhelft V, Dupont C, Benhamou PH. Epicutaneous immunotherapy on intact skin using a new delivery system in a murine model of allergy. Clin Exp Allergy (2010) 40(4):659–67. doi: 10.1111/j.1365-2222.2009.03430.x
46. Mondoulet L, Dioszeghy V, Ligouis M, Dhelft V, Puteaux E, Dupont C, et al. Epicutaneous immunotherapy compared with sublingual immunotherapy in mice sensitized to pollen (Phleum pratense). ISRN Allergy (2012) 2012:375735. doi: 10.5402/2012/375735
47. Dioszeghy V, Mondoulet L, Dhelft V, Ligouis M, Puteaux E, Dupont C, et al. The regulatory T cells induction by epicutaneous immunotherapy is sustained and mediates long-term protection from eosinophilic disorders in peanut-sensitized mice. Clin Exp Allergy (2014) 44(6):867–81. doi: 10.1111/cea.12312
48. Dupont C, Kalach N, Soulaines P, Legoue-Morillon S, Piloquet H, Benhamou PH. Cow’s milk epicutaneous immunotherapy in children: A pilot trial of safety, acceptability, and impact on allergic reactivity. J Allergy Clin Immunol (2010) 125(5):1165–7. doi: 10.1016/j.jaci.2010.02.029
49. Fleischer DM, Spergel JM, Kim EH, Campbell DE, Green TD, Bee KJ, et al. Evaluation of daily patch application duration for epicutaneous immunotherapy for peanut allergy. Allergy Asthma Proc (2020) 41(4):278–84. doi: 10.2500/aap.2020.41.200045
50. Giunchedi P, Maestri M, Gavini E, Dionigi P, Rassu G. Transarterial chemoembolization of hepatocellular carcinoma–agents and drugs: an overview. Part 2. Expert Opin Drug Deliv (2013) 10(6):799–810. doi: 10.1517/17425247.2013.796359
51. Tripp CH, Voit H, An A, Seidl-Philipp M, Krapf J, Sigl S, et al. Laser-assisted epicutaneous immunization to target human skin dendritic cells. Exp Dermatol (2021) 30(9):1279–89. doi: 10.1111/exd.14346
52. Herve PL, Dhelft V, Plaquet C, Rousseaux A, Bouzereau A, Gaulme L, et al. Epidermal micro-perforation potentiates the efficacy of epicutaneous vaccination. J Control Release (2019) 298:12–26. doi: 10.1016/j.jconrel.2019.02.004
53. Weiss R, Hessenberger M, Kitzmuller S, Bach D, Weinberger EE, Krautgartner WD, et al. Transcutaneous vaccination via laser microporation. J Control Release (2012) 162(2):391–9. doi: 10.1016/j.jconrel.2012.06.031
54. Chen X, Kositratna G, Zhou C, Manstein D, Wu MX. Micro-fractional epidermal powder delivery for improved skin vaccination. J Control Release (2014) 192:310–6. doi: 10.1016/j.jconrel.2014.08.006
55. Wang Y, Kong Y, Wu MX. Innovative systems to deliver allergen powder for epicutaneous immunotherapy. Front Immunol (2021) 12:647954. doi: 10.3389/fimmu.2021.647954
56. Weinberger EE, Himly M, Myschik J, Hauser M, Altmann F, Isakovic A, et al. Generation of hypoallergenic neoglycoconjugates for dendritic cell targeted vaccination: A novel tool for specific immunotherapy. J Control Release (2013) 165(2):101–9. doi: 10.1016/j.jconrel.2012.11.002
57. MaChado Y, Duinkerken S, Hoepflinger V, Mayr M, Korotchenko E, Kurtaj A, et al. Synergistic effects of dendritic cell targeting and laser-microporation on enhancing epicutaneous skin vaccination efficacy. J Control Release (2017) 266:87–99. doi: 10.1016/j.jconrel.2017.09.020
58. Zhi D, Yang T, Zhang T, Yang M, Zhang S, Donnelly RF. Microneedles for gene and drug delivery in skin cancer therapy. J Control Release (2021) 335:158–77. doi: 10.1016/j.jconrel.2021.05.009
59. Amani H, Shahbazi MA, D’Amico C, Fontana F, Abbaszadeh S, Santos HA. Microneedles for painless transdermal immunotherapeutic applications. J Control Release (2021) 330:185–217. doi: 10.1016/j.jconrel.2020.12.019
60. Icardi G, Orsi A, Ceravolo A, Ansaldi FJHV. Current evidence on intradermal influenza vaccines administered by soluvia™ Licensed micro injection system. Hum Vaccin Immunother (2012) 8 (1):67–75. doi: 10.4161/hv.8.1.18419
61. Tiwari N, Osorio-Blanco ER, Sonzogni A, Esporrin-Ubieto D, Wang H, Calderon M. Nanocarriers for skin applications: where do we stand? Angew Chem Int Ed Engl (2022) 61(3):e202107960. doi: 10.1002/anie.202107960
62. Tahara Y, Honda S, Kamiya N, Piao H, Hirata A, Hayakawa E, et al. A solid-in-oil nanodispersion for transcutaneous protein delivery. J Control Release (2008) 131(1):14–8. doi: 10.1016/j.jconrel.2008.07.015
63. Kitaoka M, Naritomi A, Hirakawa Y, Kamiya N, Goto M. Transdermal immunization using solid-in-oil nanodispersion with cpg oligodeoxynucleotide adjuvants. Pharm Res (2015) 32(4):1486–92. doi: 10.1007/s11095-014-1554-5
64. Pissuwan D, Nose K, Kurihara R, Kaneko K, Tahara Y, Kamiya N, et al. A solid-in-oil dispersion of gold nanorods can enhance transdermal protein delivery and skin vaccination. Small (2011) 7(2):215–20. doi: 10.1002/smll.201001394
65. Kitaoka M, Shin Y, Kamiya N, Kawabe Y, Kamihira M, Goto M. Transcutaneous peptide immunotherapy of Japanese cedar pollinosis using solid-in-oil nanodispersion technology. AAPS PharmSciTech (2015) 16(6):1418–24. doi: 10.1208/s12249-015-0333-x
Keywords: epicutaneous immunotherapy, delivery systems, stratum corneum barrier, Viaskin, precise laser epidermal system, microneedles, nanocarriers
Citation: Wang Z, Wu L and Wang W (2023) Innovative delivery systems for epicutaneous immunotherapy. Front. Immunol. 14:1238022. doi: 10.3389/fimmu.2023.1238022
Received: 10 June 2023; Accepted: 09 August 2023;
Published: 22 August 2023.
Edited by:
Jamie Marie Orengo, Regeneron Pharmaceuticals, Inc., United StatesReviewed by:
Mei X. Wu, Massachusetts General Hospital and Harvard Medical School, United StatesCopyright © 2023 Wang, Wu and Wang. This is an open-access article distributed under the terms of the Creative Commons Attribution License (CC BY). The use, distribution or reproduction in other forums is permitted, provided the original author(s) and the copyright owner(s) are credited and that the original publication in this journal is cited, in accordance with accepted academic practice. No use, distribution or reproduction is permitted which does not comply with these terms.
*Correspondence: Wei Wang, zjxuwangwei@163.com