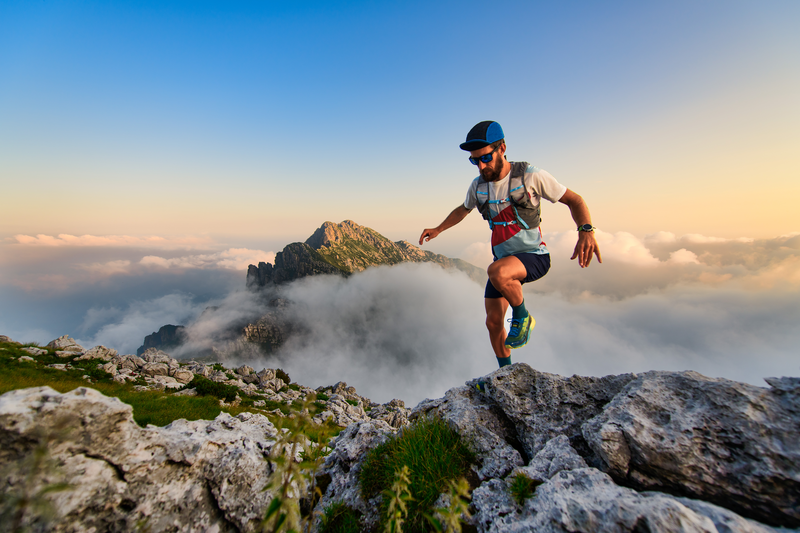
94% of researchers rate our articles as excellent or good
Learn more about the work of our research integrity team to safeguard the quality of each article we publish.
Find out more
MINI REVIEW article
Front. Immunol. , 11 July 2023
Sec. Nutritional Immunology
Volume 14 - 2023 | https://doi.org/10.3389/fimmu.2023.1233652
This article is part of the Research Topic Effects of Medicinal Homologous Foods on Immunity through Intestinal Flora View all 8 articles
It has been for thousands of years in China known medicinal homologous foods that can be employed both as foods and medicines to benefit human and animal health. These edible herbal materials perform divert roles in the regulation of metabolic disorders, cancers, and immune-related diseases. Curcumin, the primary component derived from medicinal homologous foods like curcuma longa rhizome, is reported to play vital actions in organic activities, such as the numerous pharmacological functions including anti-oxidative stress, anti-inflammation and anti/pro-apoptosis in treating various diseases. However, the potential mechanisms of curcumin-derived modulation still need to be developed and attract more attention worldwide. Given that these signal pathways are enrolled in important bioactive reactions, we collected curcumin’s last achievements predominantly on the immune-regulation signals with the underlying targetable strategies in the last 10 years. This mini-review will be helpful to accelerate curcumin and other extracts from medicinal homologous foods use in future human clinical applications.
Oxidative stress, inflammation, and apoptosis are interrelated processes that play pivotal roles in a variety of physiological and pathological conditions (1). Oxidative stress denotes an imbalance between reactive oxygen species (ROS) production and the body’s antioxidant defense mechanisms, resulting in cellular dysfunction and damage (2), while inflammation is an immune system response to harmful stimuli, such as tissue injury or pathogens, characterized by the recruitment of immune cells and the release of inflammatory mediators (3). Oxidative stress has been proven to activate pro-inflammatory signaling pathways and produce inflammatory cytokines and chemokines, thereby triggering inflammation (4). In turn, inflammation can increase oxidative stress by promoting ROS production and disrupting antioxidant defense mechanisms. Additionally, oxidative stress and inflammation can activate pro-apoptotic signaling pathways and inhibit anti-apoptotic pathways, ultimately leading to cell death (5, 6). Apoptosis, also known as programmed cell death, is a tightly controlled mechanism of cellular demise that serves a vital role in maintaining tissue homeostasis and eliminating damaged or abnormal cells (7, 8). The relationship among oxidative stress, inflammation, and apoptosis is multifaceted and complex. Dysregulation of these processes can contribute to the development and progression of various diseases, including metabolic disorders, immune-related diseases, and cancers (9–11). Therefore, it is crucial to comprehend the mechanisms underlying these processes and identify potential therapeutic targets to regulate them in preventing and treating these diseases.
The close interplay between oxidative stress, inflammation, and apoptosis has led to the investigation of medicinal homologous foods with these properties. Medicinal homologous foods epitomize a remarkable amalgamation of food and medicine. In addition to their inherent nutritional value, these foods harbor extracts imbued with distinct properties that contribute to disease prevention, treatment, and a range of healthcare benefits (12). Derived from natural sources, medicinal homologous food extracts encompass bioactive compounds with notable therapeutic potential, including polyphenols, flavonoids, terpenes, and alkaloids (13). These compounds synergistically contribute to the medicinal attributes of these foods. Curcumin (CUR) is the primary active compound found in turmeric, constituting approximately 8% of most turmeric preparations derived from curcuma longa (14). It serves as a widely used flavoring agent in food supplements and is responsible for imparting the characteristic yellow color to turmeric spice. Due to its role in oxidative stress, inflammatory response, and apoptosis, CUR shows significant pharmacological potential and has exhibited favorable effects concerning various metabolic disorders, immune-related diseases, and cancers (15, 16). Growing experimental evidence revealed that CUR had been shown to scavenge ROS, inhibit the production of pro-inflammatory cytokines, and modulate various signaling pathways involved in apoptosis (17, 18). Furthermore, CUR exhibits an excellent safety profile, with no significant adverse effects reported even at high doses (19). This feature makes it an attractive candidate for therapeutic interventions, either alone or in combination with other drugs, to enhance treatment outcomes and minimize side effects. Therefore, the investigation of CUR’s potential use in the treatment of oxidative stress, inflammation, and apoptosis can provide valuable theoretical strategies for developing it as a promising candidate for the prevention and treatment of various diseases.
Oxidative stress (OS) is a pathological state that results from an imbalance between the pro-oxidant and the antioxidant species (20). OS induces several oxidative intermediates and promotes protease secretion, and neutrophilic inflammatory infiltration, ultimately resulting in cellular senescence and various diseases, including cancer, cardiovascular disease, and neurodegenerative disorders (21, 22). Reactive oxygen species (ROS) are by-products produced during oxygen metabolism, comprising free radicals and non-free radicals that can cause oxidative damage to cellular components, including lipids, proteins, and DNA, which can disrupt cellular signaling pathways (23). As a protective mechanism, cellular antioxidant defense systems, including SOD (superoxide dismutase), peroxidases, antioxidants, and vitamins, have evolved as a protective mechanism to maintain the oxidation-reduction (redox) status by preventing the accumulation of ROS (24).
CUR is a naturally occurring compound derived from plants that exhibit anti-oxidative stress properties. Research studies have reported that CUR effectively attenuates the release of ROS in various experimental settings, including cell lines, preclinical models, and clinical samples (25–27). Pretreatment with CUR regulates the expression of antioxidant enzymes through nuclear factor erythroid 2-related factor 2 (Nrf2) signaling pathways to stabilize ROS levels (28). Nrf2 is a transcription factor that plays a critical role in the cellular response to oxidative stress by regulating the expression of genes that encode antioxidant enzymes and detoxifying proteins (29). Under normal conditions, Nrf2 is inhibited by Kelch-like ECH-associated protein 1 (Keap1), which targets Nrf2 for ubiquitination and proteasomal degradation. In response to oxidative stress conditions, specific cysteine residues within the Keap1 protein undergo oxidation, including Cys-151, Cys-273, Cys-288, Cys-297, and Cys-257, leading to the dissociation of the NRF2-Keap1 complex (30). A study conducted on the therapeutic potential of CUR in mouse skin revealed the binding of CUR to Keap1 Cys151 (31). This finding suggests that the modification of this specific amino acid by CUR may play a crucial role in releasing NRF2 from Keap1, thus highlighting its potential as a therapeutic target (32). Upon activation, Nrf2 translocates to the nucleus, binding to antioxidant response elements (AREs) and promoting the expression of ARE genes (33). The dissociation of NRF2 from the NRF2-Keap1 complex is an essential step in activating the NRF2/ARE signaling pathway, which increases the expression of antioxidant enzymes, including glutathione peroxidase (GPx), superoxide dismutase (SOD), and catalase (CAT), as well as phase II antioxidant enzymes such as heme oxygenase-1(HO-1) and NAD(P)H quinone dehydrogenase 1 (NQO1). These enzymes eliminate ROS and maintain redox homeostasis (34, 35). Studies have found that CUR enhances the activities of antioxidant enzymes through the Nrf2 pathway, which contributes to neutralizing ROS and protecting the host from damage caused by oxidative stress (36, 37).
A recent study has indicated that CUR can alleviate intestinal barrier injury and mitochondrial damage induced by oxidative stress through the activation of AMP-activated protein kinase (AMPK) pathway (38). AMPK is an important regulator of cellular energy homeostasis and is activated in response to low energy status. In addition to its role in energy metabolism, AMPK has been demonstrated to play a crucial role in the regulation of cellular stress response pathways, including the Nrf2 pathway (39). Phosphorylation of Nrf2 by AMPK results in its translocation to the nucleus and activation of Nrf2-dependent gene expression. AMPK activates Nrf2 through direct phosphorylation at Ser550, leading to the nuclear accumulation of Nrf2 (40). This phosphorylation enhances Nrf2 transcriptional activity and promotes the expression of Nrf2 target genes, such as SOD-1 and HO-1, thereby attenuating oxidative stress and inflammation (41). Therefore, the activation of AMPK enhances antioxidant defenses and provides cellular protection against oxidative stress but also represents an important mechanism for CUR to maintain cellular redox homeostasis and protect cells from oxidative stress by activating Nrf2 (42).. It is worth mentioning that CUR initiates a pro-oxidant response that stimulates the ROS and lipid MDA into less harmful products by inhibiting reduced glutathione (RGS) and GPx, which enhances cell death in cancer cells (43). Moreover, growing evidences confirm the effective suppression of gastric cancer cell proliferation by CUR through the induction of ROS generation, subsequently triggering apoptosis (44, 45). Overall, a comprehensive understanding of the intricate details of anti/pro-oxidative properties of CUR could pave the way for the development of CUR strategies aimed at preventing and treating oxidative stress-related diseases.
Inflammation is a complex biological response initiated by diverse stimuli, including pathogens, tissue damage, and cellular stress. In response to these stimuli, immune and other cells produce a diverse group of molecules known as inflammatory mediators, including cytokines, chemokines, prostaglandins, and leukotrienes (46). While inflammation and inflammatory mediators are essential for the immune response and play a role in fighting infections and promoting tissue repair, excessive or chronic inflammation may develop various diseases, such as autoimmune disorders, metabolic disorders, and cancer (47–49). Therefore, modulation of inflammatory mediators has the potential to be a promising strategy for preventing and treating these conditions.
The efficacy of CUR in modulating the inflammatory response can be attributed to its capability to regulate multiple signaling pathways and molecules involved in inflammation. Numerous studies have demonstrated that CUR exerts anti-inflammatory effects by inhibiting the activity of several pro-inflammatory mediators, including cytokines (e.g., TNF-α, IL-1β, IL-6), chemokines (e.g., CXCL8, CCL2), and enzymes (e.g., COX-2, iNOS) (50, 51). The regulation of the inflammatory response by CUR is attributed to its ability to block the NF-κB (nuclear factor kappa-light-chain-enhancer of activated B cells) pathway, which is vital in the inflammatory process (52). NF-κB, a ubiquitously present transcription factor, critically regulates the immune response, inflammation, cell proliferation, and apoptosis while participating in the expression of genes that regulate cellular responses to various stimuli, including stress, cytokines, free radicals, and microbial pathogens (53). In an inactive state, NF-κB is bound to inhibitor proteins, which prevent it from entering the nucleus and activating gene transcription. Upon activation, NF-κB translocates to the nucleus and activates the transcription of various pro-inflammatory genes. Moreover, CUR administration efficiently blocks the phosphorylation of the inhibitor of kappa B (IKK) and the inhibitor of NF-κB (IκB), which are necessary for the translocation of NF-κB into the nucleus in ulcerative colitis and cancer cells (54, 55). Furthermore, CUR also suppresses the activation of Toll-like receptors (TLRs) and downstream signaling pathways that lead to NF-κB activation (56). Overall, these findings indicate that CUR inhibits TLR activation and blocks the phosphorylation of IKK and IκB, subsequently preventing the translocation of NF-κB towards the nucleus. Ultimately, this cascade of events effectively inhibits the transcription of various pro-inflammatory genes.
The Activator Protein-1 (AP-1) pathway is an important pathway involved in CUR’s regulation of the inflammatory response (57). AP-1 is a transcription factor that promotes the transcription of several pro-inflammatory genes, such as cytokines, chemokines, and matrix metalloproteinases (MMPs) (58, 59). In line with this, Woo et al. revealed that CUR blocks AP-1 activation by inhibiting JNK, which phosphorylates and activates c-Jun (an AP-1 subunit) (60). Moreover, it has been demonstrated that CUR can directly bind to c-Jun and inhibit its DNA binding activity, thereby suppressing the expression of pro-inflammatory genes and alleviating inflammation (60). Furthermore, CUR can also depress the activity of inflammation-related enzymes (e.g., COX-2, iNOS) by inhibiting p38 MAPK signaling (61). Aside from its impact on inflammatory mediators, CUR has been observed to modulate the activity of immune cells involved in the inflammatory response, suppressing the production of pro-inflammatory cytokines TNF-α and IL-6 by macrophages and inducing the polarization of macrophages towards an anti-inflammatory M2 phenotype (62). These studies provide insights into the regulation of signaling pathways by CUR, which has been shown to inhibit the activation of NF-κB, AP-1, and MAPK, as well as the expression and activity of numerous pro-inflammatory mediators, underscoring its potential as a therapeutic agent for various inflammatory disorders.
Apoptosis, also referred to as programmed cell death, is a precisely regulated process that eliminates impaired or unneeded cells to maintain tissue homeostasis (63). Disruption of apoptosis can result in various pathological conditions, including autoimmune diseases, cancer, and neurodegeneration (11, 64, 65). Apoptosis is governed by an intricate network of signaling pathways, which include extrinsic and intrinsic pathways (66). The extrinsic pathway is triggered by the binding of extracellular ligands to death receptors (e.g., TNF, Fas receptors). In contrast, the intrinsic pathway is initiated by intracellular stress signals, such as oxidative stress and inflammatory response (67, 68). Therefore, comprehending the molecular mechanisms that regulate apoptosis and identifying effective strategies for modulating apoptosis is critical for preventing and treating diseases.
Compelling scientific and dietary evidence has demonstrated the potential of CUR to induce apoptosis in various cancer cells. For instance, CUR has been extensively investigated for its potential to inhibit the PI3K/AKT pathway in cancer cells, leading to the downregulation of downstream targets such as mTOR and inducing apoptosis (69, 70). The activation of the PI3K/AKT pathway, triggered by the production of 3’-phosphorylated phosphoinositides, is a critical signaling pathway involved in both apoptosis and cell cycle progression (71). Abnormal activation of the PI3K/AKT pathway hinders apoptosis by upregulating anti-apoptotic genes such as Bcl-2 and downregulating pro-apoptotic genes like Bax (71). In addition, CUR is validated to upregulate the expression of PTEN, a tumor suppressor that negatively regulates PI3K/AKT signaling, while downregulating the expression of genes involved in AKT activation (72). Moreover, CUR modulates upstream regulators of the PI3K/AKT/mTOR pathway, including growth factor receptors and integrins. Chiu et al. reported that CUR inhibits the activity of EGFR, a receptor tyrosine kinase that activates the PI3K/AKT pathway, leading to apoptosis induction in cancer cells (73). Aside from PI3K/AKT/mTOR pathway, the Janus kinase/signal transducer and activator of transcription (JAK/STAT) pathway is another major signaling pathway that regulates apoptosis (74). The JAK/STAT pathway is a signaling cascade that plays a critical role in the regulation of cell proliferation, differentiation, and apoptosis, and its dysregulation has been implicated in various diseases (74). In a myeloproliferative neoplasms model, CUR activates the JAK2/STAT pathway, inducing apoptosis and inhibiting proliferation, thus exerting an antitumor effect on human JAK2-mutated cells (75). Furthermore, it has been noted that CUR modulates several apoptotic pathways, including the death receptor and endoplasmic reticulum (ER) stress-induced apoptosis pathways (76). The death receptor pathway is initiated when death ligands, such as TNF and Fas ligand, bind to their respective receptors, activating caspase-8 and downstream effector caspases, ultimately leading to apoptosis (77). CUR has been shown to sensitize cancer cells to death receptor-mediated apoptosis by promoting Fas-receptor and by activation of caspase-3 and -8 in tumor cells (78). In addition, CUR activates the ER stress-induced apoptosis pathway, a critical membranous organelle during apoptosis, and participates in complex interaction with the mitochondria (79). Furthermore, in tumor therapy, CUR induces ER stress. It activates the unfolded protein response (UPR), leading to the upregulation of the pro-apoptotic proteins (CHOP and Bax) and the activation of caspase-3, which ultimately triggers apoptosis and exerts anti-tumor property (80). Experimental data have conclusively proved that CUR exerts anti-cancer effects by promoting apoptosis through several signaling pathways in cancer cells.
Intriguingly, CUR has also been demonstrated to possess inhibitory effects on apoptosis in non-cancerous diseases, expanding its potential therapeutic applications beyond cancer treatment. In diabetic cardiomyopathy, CUR inhibits apoptosis and alleviates oxidative stress by eliminating ROS levels and activating PI3K-AKT signaling pathways, resulting in the downregulation of caspase-3 and Bax protein expression (81). Moreover, CUR treatment was shown to attenuate apoptosis and inflammation by inhibiting JAK2/STAT3 and NF-κB signaling pathways, which results in the upregulation of Bcl-2, and the downregulation of Bax and caspase-3 in an acute kidney injury model (82). In addition, the treatment of CUR enhances the viability of Saos-2 cells, mitigates apoptosis, improves mitochondrial membrane function and potential, and upregulates the phosphorylation of GSK3b and protein kinase B (AKT) (83). Furthermore, in the gentamicin-induced nephrotoxicity model, CUR reverses the expression levels of ER stress markers (CHOP, calpain-2, caspase-12, and cleaved caspase-7) and decreased apoptotic protein biomarkers expression (cleaved caspase-3 and Bax) (84). Therefore, besides its anticancer effects through promoting apoptosis in cancer cells, CUR has also demonstrated the ability to inhibit apoptosis in non-cancerous diseases, such as diabetic cardiomyopathy and acute kidney injury. These significant findings underscore the multifaceted role of CUR in modulating apoptosis and establish a foundation for its therapeutic applications in diverse disease contexts.
Curcumin, a bioactive compound present in turmeric, has emerged as a potential health-promoting agent in the regulation of various diseases. This mini-review offers a comprehensive summary of recent advances in elucidating the preventive and curative effects of CUR on oxidative stress, inflammation, and apoptosis in non-cancerous diseases and cancers. Generally, CUR exhibits its antioxidant property through AMPK/Nrf2/ARE/Keap1 pathway activation, its anti-inflammatory property via NF-κB/AP-1/MAPK pathways inhibition, and its anti-apoptosis property by blocking JAK/STAT and ER stress-induced pathways while activating PI3K/AKT/mTOR pathways in non-cancerous diseases (Figure 1). Conversely, CUR demonstrates pro-oxidant, anti-inflammatory, and pro-apoptosis properties in cancers (Figure 1). Therefore, the future perspectives of CUR’s application encompass exploring novel drug formulations, investigating combination therapy approaches, conducting disease-specific clinical trials, developing targeted therapies, and integrating them with lifestyle interventions to optimize its therapeutic potential in both non-cancerous diseases and cancers. However, further research is necessary to comprehensively elucidate the underlying mechanisms of CUR in alleviating host diseases and to devise more effective clinical strategies.
Figure 1 Molecular targets of curcumin on oxidative stress, inflammatory response, and apoptosis in non-cancerous diseases and cancers. CUR exerts its effects through diverse cellular core pathways, including the AMPK/Nrf2/ARE/Keap1 pathway associated with oxidative stress, the NF-κB/AP-1/MAPK pathways related to inflammatory responses, and the PI3K/AKT/mTOR, JAK/STAT, and ER stress-induced pathways involved in apoptotic mechanisms.
DC and H-YL: conceptualization. PH, KL, X-XP, YK, T-JY, and Z-YW writing the original draft. H-YL, DC, and ZL: review and editing. All authors contributed to the article and approved the submitted version.
This work was supported by the Natural Science Foundation of Jiangsu Province (BK20200932, BK20210812, BK20220582), the National Natural Science Foundation of China (32202717, 32002243), the Open Project Program of International Joint Research Laboratory in Universities of Jiangsu Province of China for Domestic Animal Germplasm Resources and Genetic Improvement, the Priority Academic Program Development of Jiangsu Higher Education Institutions (PAPD).
The authors declare that the research was conducted in the absence of any commercial or financial relationships that could be construed as a potential conflict of interest.
All claims expressed in this article are solely those of the authors and do not necessarily represent those of their affiliated organizations, or those of the publisher, the editors and the reviewers. Any product that may be evaluated in this article, or claim that may be made by its manufacturer, is not guaranteed or endorsed by the publisher.
1. Al Olayan EM, Aloufi AS, AlAmri OD, Ola H, Moneim AEA. Protocatechuic acid mitigates cadmium-induced neurotoxicity in rats: role of oxidative stress, inflammation and apoptosis. Sci Total Environ (2020) 723:137969. doi: 10.1016/j.scitotenv.2020.137969
2. van der Pol A, van Gilst WH, Voors AA, van der Meer P. Treating oxidative stress in heart failure: past, present and future. Eur J Heart Fail (2019) 21(4):425–35. doi: 10.1002/ejhf.1320
3. Abdulkhaleq L, Assi M, Abdullah R, Zamri-Saad M, Taufiq-Yap Y, Hezmee M. The crucial roles of inflammatory mediators in inflammation: a review. Veterinary World (2018) 11(5):627. doi: 10.14202/vetworld.2018.627-635
4. Forrester SJ, Kikuchi DS, Hernandes MS, Xu Q, Griendling KK. Reactive oxygen species in metabolic and inflammatory signaling. Circ Res (2018) 122(6):877–902. doi: 10.1161/CIRCRESAHA.117.311401
5. Samimi M, Pourhanifeh MH, Mehdizadehkashi A, Eftekhar T, Asemi Z. The role of inflammation, oxidative stress, angiogenesis, and apoptosis in the pathophysiology of endometriosis: basic science and new insights based on gene expression. J Cell Physiol (2019) 234(11):19384–92. doi: 10.1002/jcp.28666
6. Askari H, Rajani SF, Poorebrahim M, Haghi-Aminjan H, Raeis-Abdollahi E, Abdollahi M. A glance at the therapeutic potential of irisin against diseases involving inflammation, oxidative stress, and apoptosis: an introductory review. Pharmacol Res (2018) 129:44–55. doi: 10.1016/j.phrs.2018.01.012
7. Carneiro BA, El-Deiry WS. Targeting apoptosis in cancer therapy. Na Rev Clin Oncol (2020) 17(7):395–417. doi: 10.1038/s41571-020-0341-y
8. Liu D, Kou X, Chen C, Liu S, Liu Y, Yu W, et al. Circulating apoptotic bodies maintain mesenchymal stem cell homeostasis and ameliorate osteopenia via transferring multiple cellular factors. Cell Res (2018) 28(9):918–33. doi: 10.1038/s41422-018-0070-2
9. Behl T, Makkar R, Sehgal A, Singh S, Sharma N, Zengin G, et al. Current trends in neurodegeneration: cross talks between oxidative stress, cell death, and inflammation. Int J Mol Sci (2021) 22(14):7432. doi: 10.3390/ijms22147432
10. Libby P, Kobold S. Inflammation: a common contributor to cancer, aging, and cardiovascular diseases–expanding the concept of cardio-oncology. Cardiovasc Res (2019) 115(5):824–9. doi: 10.1093/cvr/cvz058
11. Xu X, Lai Y, Hua Z-C. Apoptosis and apoptotic body: disease message and therapeutic target potentials. Biosci Rep (2019) 39(1):BSR20180992. doi: 10.1042/BSR20180992
12. Gong X, Ji M, Xu J, Zhang C, Li M. Hypoglycemic effects of bioactive ingredients from medicine food homology and medicinal health food species used in China. Crit Rev Food Sci Nutr (2020) 60(14):2303–26. doi: 10.1080/10408398.2019.1634517
13. Mondal S, Soumya NPP, Mini S, Sivan SK. Bioactive compounds in functional food and their role as therapeutics. Bioact Comp Health Dis (2021) 4(3):24–39. doi: 10.31989/bchd.v4i3.786
14. Shehzad A, Lee YS. Molecular mechanisms of curcumin action: signal transduction. Biofactors (2013) 39(1):27–36. doi: 10.1002/biof.1065
15. Kamal DAM, Salamt N, Yusuf ANM, Kashim MIAM, Mokhtar MH. Potential health benefits of curcumin on female reproductive disorders: a review. Nutrients (2021) 13(9):3126. doi: 10.3390/nu13093126
16. Willenbacher E, Khan SZ, Mujica SCA, Trapani D, Hussain S, Wolf D, et al. Curcumin: new insights into an ancient ingredient against cancer. Int J Mol Sci (2019) 20(8):1808. doi: 10.3390/ijms20081808
17. Abd El-Hack ME, El-Saadony MT, Swelum AA, Arif M, Abo Ghanima MM, Shukry M, et al. Curcumin, the active substance of turmeric: its effects on health and ways to improve its bioavailability. J Sci Food Agric (2021) 101(14):5747–62. doi: 10.1002/jsfa.11372
18. Gorabi AM, Razi B, Aslani S, Abbasifard M, Imani D, Sathyapalan T, et al. Effect of curcumin on proinflammatory cytokines: a meta-analysis of randomized controlled trials. Cytokine (2021) 143:155541. doi: 10.1016/j.cyto.2021.155541
19. Karthika C, Hari B, Mano V, Radhakrishnan A, Janani S, Akter R, et al. Curcumin as a great contributor for the treatment and mitigation of colorectal cancer. Exp Gerontol (2021) 152:111438. doi: 10.1016/j.exger.2021.111438
20. Nathan C, Cunningham-Bussel A. Beyond oxidative stress: an immunologist's guide to reactive oxygen species. Nat Rev Immunol (2013) 13(5):349–61. doi: 10.1038/nri3423
21. Forni C, Facchiano F, Bartoli M, Pieretti S, Facchiano A, D’Arcangelo D, et al. Beneficial role of phytochemicals on oxidative stress and age-related diseases. BioMed Res Int (2019) 2019:8748253. doi: 10.1155/2019/8748253
22. Arfin S, Jha NK, Jha SK, Kesari KK, Ruokolainen J, Roychoudhury S, et al. Oxidative stress in cancer cell metabolism. Antioxidants (2021) 10(5):642. doi: 10.3390/antiox10050642
23. Bolduc JA, Collins JA, Loeser RF. Reactive oxygen species, aging and articular cartilage homeostasis. Free Radic Biol Med (2019) 132:73–82. doi: 10.1016/j.freeradbiomed.2018.08.038
24. Juan CA, Pérez de la Lastra JM, Plou FJ, Pérez-Lebeña E. The chemistry of reactive oxygen species (ROS) revisited: outlining their role in biological macromolecules (DNA, lipids and proteins) and induced pathologies. Int J Mol Sci (2021) 22(9):4642. doi: 10.3390/ijms22094642
25. Kundur S, Prayag A, Selvakumar P, Nguyen H, McKee L, Cruz C, et al. Synergistic anticancer action of quercetin and curcumin against triple-negative breast cancer cell lines. J Cell Physiol (2019) 234(7):11103–18. doi: 10.1002/jcp.27761
26. Alikiaii B, Bagherniya M, Askari G, Sathyapalan T, Sahebkar A. Evaluation of the effect of curcumin on pneumonia: a systematic review of preclinical studies. Phytother Res (2021) 35(4):1939–52. doi: 10.1002/ptr.6939
27. Pricci M, Girardi B, Giorgio F, Losurdo G, Ierardi E, Di Leo A. Curcumin and colorectal cancer: from basic to clinical evidences. Int J Mol Sci (2020) 21(7):2364. doi: 10.3390/ijms21072364
28. Ashrafizadeh M, Ahmadi Z, Mohammadinejad R, Farkhondeh T, Samarghandian S. Curcumin activates the Nrf2 pathway and induces cellular protection against oxidative injury. Curr Mol Med (2020) 20(2):116–33. doi: 10.2174/1566524019666191016150757
29. Zuo C, Cao H, Song Y, Gu Z, Huang Y, Yang Y, et al. Nrf2: an all-rounder in depression. Redox Biol (2022) 12:102522. doi: 10.1016/j.redox.2022.102522
30. Kobayashi M, Li L, Iwamoto N, Nakajima-Takagi Y, Kaneko H, Nakayama Y, et al. The antioxidant defense system Keap1-Nrf2 comprises a multiple sensing mechanism for responding to a wide range of chemical compounds. Mol Cell Biol (2009) 29(2):493–502. doi: 10.1128/MCB.01080-08
31. Shin JW, Chun K-S, Kim D-H, Kim S-J, Kim SH, Cho N-C, et al. Curcumin induces stabilization of Nrf2 protein through Keap1 cysteine modification. Biochem Pharmacol (2020) 173:113820. doi: 10.1016/j.bcp.2020.113820
32. Kumar A, Mittal R. Nrf2: a potential therapeutic target for diabetic neuropathy. Inflammopharmacology (2017) 25:393–402. doi: 10.1007/s10787-017-0339-y
33. Nioi P, McMahon M, Itoh K, Yamamoto M, Hayes JD. Identification of a novel Nrf2-regulated antioxidant response element (ARE) in the mouse NAD (P) h: quinone oxidoreductase 1 gene: reassessment of the ARE consensus sequence. Biochem J (2003) 374(2):337–48. doi: 10.1042/bj20030754
34. Mine Y, Young D, Yang C. Antioxidative stress effect of phosphoserine dimers is mediated via activation of the Nrf2 signaling pathway. Mol Nutr Food Res (2015) 59(2):303–14. doi: 10.1002/mnfr.201400381
35. Ryoo I-g, Shin D-h, Kang K-S, Kwak M-K. Involvement of Nrf2-GSH signaling in TGFβ1-stimulated epithelial-to-mesenchymal transition changes in rat renal tubular cells. Arch Pharm Res (2015) 38:272–81. doi: 10.1007/s12272-014-0380-y
36. Meshkibaf M, Maleknia M, Noroozi S. Effect of curcumin on gene expression and protein level of methionine sulfoxide reductase a (MSRA), SOD, CAT and GPx in freund’s adjuvant inflammation-induced male rats. J Inflammation Res (2019) 12:241–9. doi: 10.2147/JIR.S212577
37. Wang X, Chang X, Zhan H, Zhang Q, Li C, Gao Q, et al. Curcumin and baicalin ameliorate ethanol-induced liver oxidative damage via the Nrf2/HO-1 pathway. J Food Biochem (2020) 44(10):e13425. doi: 10.1111/jfbc.13425
38. Cao S, Wang C, Yan J, Li X, Wen J, Hu C. Curcumin ameliorates oxidative stress-induced intestinal barrier injury and mitochondrial damage by promoting parkin dependent mitophagy through AMPK-TFEB signal pathway. Free Radic Biol Med (2020) 147:8–22. doi: 10.1016/j.freeradbiomed.2019.12.004
39. Zhou F, Wang M, Ju J, Wang Y, Liu Z, Zhao X, et al. Schizandrin a protects against cerebral ischemia-reperfusion injury by suppressing inflammation and oxidative stress and regulating the AMPK/Nrf2 pathway regulation. Am J Transl Res (2019) 11(1):199.
40. Joo MS, Kim WD, Lee KY, Kim JH, Koo JH, Kim SG. AMPK facilitates nuclear accumulation of Nrf2 by phosphorylating at serine 550. Mol Cell Biol (2016) 36(14):1931–42. doi: 10.1128/MCB.00118-16
41. Matzinger M, Fischhuber K, Pölöske D, Mechtler K, Heiss EH. AMPK leads to phosphorylation of the transcription factor Nrf2, tuning transactivation of selected target genes. Redox Biol (2020) 29:101393. doi: 10.1016/j.redox.2019.101393
42. Pu Y, Zhang H, Wang P, Zhao Y, Li Q, Wei X, et al. Dietary curcumin ameliorates aging-related cerebrovascular dysfunction through the AMPK/uncoupling protein 2 pathway. Cell Physiol Biochem (2013) 32(5):1167–77. doi: 10.1159/000354516
43. Gökçe Kütük S, Gökçe G, Kütük M, Gürses Cila HE, Nazıroğlu M. Curcumin enhances cisplatin-induced human laryngeal squamous cancer cell death through activation of TRPM2 channel and mitochondrial oxidative stress. Sci Rep (2019) 9(1):1–14. doi: 10.1038/s41598-019-54284-x
44. Shehzad A, Lee J, Huh T-L, Lee YS. Curcumin induces apoptosis in human colorectal carcinoma (HCT-15) cells by regulating expression of Prp4 and p53. Mol Cells (2013) 35:526–32. doi: 10.1007/s10059-013-0038-5
45. Liang T, Zhang X, Xue W, Zhao S, Zhang X, Pei J. Curcumin induced human gastric cancer BGC-823 cells apoptosis by ROS-mediated ASK1-MKK4-JNK stress signaling pathway. Int J Mol Sci (2014) 15(9):15754–65. doi: 10.3390/ijms150915754
46. Zhao H, Wu L, Yan G, Chen Y, Zhou M, Wu Y, et al. Inflammation and tumor progression: signaling pathways and targeted intervention. Signal Transduct Tar (2021) 6(1):263. doi: 10.1038/s41392-021-00658-5
47. Abou-Raya A, Abou-Raya S. Inflammation: a pivotal link between autoimmune diseases and atherosclerosis. Autoimmun Rev (2006) 5(5):331–7. doi: 10.1016/j.autrev.2005.12.006
48. Hotamisligil GS. Inflammation and metabolic disorders. Nature (2006) 444(7121):860–7. doi: 10.1038/nature05485
49. Yao D, Dong M, Dai C, Wu S. Inflammation and inflammatory cytokine contribute to the initiation and development of ulcerative colitis and its associated cancer. Inflammation Bowel Dis (2019) 25(10):1595–602. doi: 10.1093/ibd/izz149
50. Hedayati-Moghadam M, Hosseinian S, Paseban M, Shabgah AG, Gholizadeh J, Jamialahmadi T, et al. The role of chemokines in cardiovascular diseases and the therapeutic effect of curcumin on CXCL8 and CCL2 as pathological chemokines in atherosclerosis. Adv Exp Med Biol (2021) 1328:155–70. doi: 10.1007/978-3-030-73234-9_11
51. Lee S-Y, Cho S-S, Li Y, Bae C-S, Park KM, Park D-H. Anti-inflammatory effect of curcuma longa and allium hookeri co-treatment via NF-κB and COX-2 pathways. Sci Rep (2020) 10(1):5718. doi: 10.1038/s41598-020-62749-7
52. Wu T, Li X, Tu S, Tan W, Chen L. Curcumin protect schwann cells from inflammation response and apoptosis induced by high glucose through the NF-κB pathway. Tissue Cell (2022) 77:101873. doi: 10.1016/j.tice.2022.101873
53. Zinatizadeh MR, Schock B, Chalbatani GM, Zarandi PK, Jalali SA, Miri SR. The nuclear factor kappa b (NF-kB) signaling in cancer development and immune diseases. Genes Dis (2021) 8(3):287–97. doi: 10.1016/j.gendis.2020.06.005
54. Wang Y, Tang Q, Duan P, Yang L. Curcumin as a therapeutic agent for blocking NF-κB activation in ulcerative colitis. Immunopharm Immunot (2018) 40(6):476–82. doi: 10.1080/08923973.2018.1469145
55. Deng X-z, Geng S-s, Luo M, Chai J-j, Xu Y, Chen C-l, et al. Curcumin potentiates laryngeal squamous carcinoma radiosensitivity via NF-KB inhibition by suppressing IKKγ expression. J Recept Signal Transduct (2020) 40(6):541–9. doi: 10.1080/10799893.2020.1767649
56. Nguyen HD, Jo WH, Hoang NHM, Kim M-S. Curcumin-attenuated TREM-1/DAP12/NLRP3/Caspase-1/IL1B, TLR4/NF-κB pathways, and tau hyperphosphorylation induced by 1, 2-diacetyl benzene: an in vitro and in silico study. Neurotox Res (2022) 40(5):1272–91. doi: 10.1007/s12640-022-00535-1
57. Yen F-L, Tsai M-H, Yang C-M, Liang C-J, Lin C-C, Chiang Y-C, et al. Curcumin nanoparticles ameliorate ICAM-1 expression in TNF-α-treated lung epithelial cells through p47 phox and MAPKs/AP-1 pathways. PloS One (2013) 8(5):e63845. doi: 10.1371/journal.pone.0063845
58. Ho L-J, Lin L-C, Hung L-F, Wang S-J, Lee C-H, Chang D-M, et al. Retinoic acid blocks pro-inflammatory cytokine-induced matrix metalloproteinase production by down-regulating JNK-AP-1 signaling in human chondrocytes. Biochem Pharmacol (2005) 70(2):200–8. doi: 10.1016/j.bcp.2005.04.039
59. Qiao Y, He H, Jonsson P, Sinha I, Zhao C, Dahlman-Wright K. AP-1 is a key regulator of proinflammatory cytokine TNFα-mediated triple-negative breast cancer progression. J Biol Chem (2016) 291(10):5068–79. doi: 10.1074/jbc.M115.702571
60. Woo J-H, Park J-M, Jang J-H, Yang H, Surh Y-J, Na H-K. Curcumin induces expression of 15-hydroxyprostaglandin dehydrogenase in gastric mucosal cells and mouse stomach in vivo: AP-1 as a potential target. J Nutr Biochem (2020) 85:108469. doi: 10.1016/j.jnutbio.2020.108469
61. Joshi P, Joshi S, Semwal DK, Verma K, Dwivedi J, Sharma S. Role of curcumin in ameliorating hypertension and associated conditions: a mechanistic insight. Mol Cell Biochem (2022) 477(10):2359–85. doi: 10.1007/s11010-022-04447-8
62. Zhang J, Zheng Y, Luo Y, Du Y, Zhang X, Fu J. Curcumin inhibits LPS-induced neuroinflammation by promoting microglial M2 polarization via TREM2/TLR4/NF-κB pathways in BV2 cells. Mol Immunol (2019) 116:29–37. doi: 10.1016/j.molimm.2019.09.020
63. Meier P, Finch A, Evan G. Apoptosis in development. Nature (2000) 407(6805):796–801. doi: 10.1038/35037734
64. Alvarez-Diaz S, Dillon CP, Lalaoui N, Tanzer MC, Rodriguez DA, Lin A, et al. The pseudokinase MLKL and the kinase RIPK3 have distinct roles in autoimmune disease caused by loss of death-receptor-induced apoptosis. Immunity (2016) 45(3):513–26. doi: 10.1016/j.immuni.2016.07.016
65. Erekat NS. Apoptosis and its therapeutic implications in neurodegenerative diseases. Clin Anat (2022) 35(1):65–78. doi: 10.1002/ca.23792
66. Xu G, Shi Y. Apoptosis signaling pathways and lymphocyte homeostasis. Cell Res (2007) 17(9):759–71. doi: 10.1038/cr.2007.52
67. Schneider P, Tschopp J. Apoptosis induced by death receptors. Pharmacochemistry library (2000) 31:281–6. doi: 10.1016/S0165-7208(00)80030-6
68. Redza-Dutordoir M, Averill-Bates DA. Activation of apoptosis signalling pathways by reactive oxygen species. BBA-Mol Cell Res (2016) 1863(12):2977–92. doi: 10.1016/j.bbamcr.2016.09.012
69. Liu F, Gao S, Yang Y, Zhao X, Fan Y, Ma W, et al. Antitumor activity of curcumin by modulation of apoptosis and autophagy in human lung cancer A549 cells through inhibiting PI3K/Akt/mTOR pathway. Oncol Rep (2018) 39(3):1523–31. doi: 10.3892/or.2018.6188
70. Hamzehzadeh L, Atkin SL, Majeed M, Butler AE, Sahebkar A. The versatile role of curcumin in cancer prevention and treatment: a focus on PI3K/AKT pathway. J Cell Physiol (2018) 233(10):6530–7. doi: 10.1002/jcp.26620
71. Liu R, Chen Y, Liu G, Li C, Song Y, Cao Z, et al. PI3K/AKT pathway as a key link modulates the multidrug resistance of cancers. Cell Death Dis (2020) 11(9):797. doi: 10.1038/s41419-020-02998-6
72. Chang M, Wu M, Li H. Curcumin combined with glycyrrhetinic acid inhibits the development of hepatocellular carcinoma cells by down-regulating the PTEN/PI3K/AKT signalling pathway. Am J Transl Res (2017) 9(12):5567.
73. Chiu YJ, Yang JS, Tsai FJ, Chiu HY, Juan YN, Lo YH, et al. Curcumin suppresses cell proliferation and triggers apoptosis in vemurafenib-resistant melanoma cells by downregulating the EGFR signaling pathway. Environ Toxicol (2022) 37(4):868–79. doi: 10.1002/tox.23450
74. Hu X, Li J, Fu M, Zhao X, Wang W. The JAK/STAT signaling pathway: from bench to clinic. Signal Transduct Tarther (2021) 6(1):402. doi: 10.1038/s41392-021-00791-1
75. Petiti J, Rosso V, Lo Iacono M, Panuzzo C, Calabrese C, Signorino E, et al. Curcumin induces apoptosis in JAK2-mutated cells by the inhibition of JAK2/STAT and mTORC1 pathways. J Cell Mol Med (2019) 23(6):4349–57. doi: 10.1111/jcmm.14326
76. Lee H-P, Li T-M, Tsao J-Y, Fong Y-C, Tang C-H. Curcumin induces cell apoptosis in human chondrosarcoma through extrinsic death receptor pathway. Int Immunopharmacol (2012) 13(2):163–9. doi: 10.1016/j.intimp.2012.04.002
77. Park MY, Ha SE, Vetrivel P, Kim HH, Bhosale PB, Abusaliya A, et al. Differences of key proteins between apoptosis and necroptosis. BioMed Res Int (2021) 2021:342068. doi: 10.1155/2021/3420168
78. Ramachandran C, Rodriguez S, Ramachandran R, Nair PR, Fonseca H, Khatib Z, et al. Expression profiles of apoptotic genes induced by curcumin in human breast cancer and mammary epithelial cell lines. Anticancer Res (2005) 25(5):3293–302.
79. Shakeri A, Zirak MR, Hayes AW, Reiter R, Karimi G. Curcumin and its analogues protect from endoplasmic reticulum stress: mechanisms and pathways. Pharmacol Res (2019) 146:104335. doi: 10.1016/j.phrs.2019.104335
80. Zeng Y, Du Q, Zhang Z, Ma J, Han L, Wang Y, et al. Curcumin promotes cancer-associated fibroblasts apoptosis via ROS-mediated endoplasmic reticulum stress. Arch Biochem Biophys (2020) 694:108613. doi: 10.1016/j.abb.2020.108613
81. Ren BC, Zhang YF, Liu SS, Cheng XJ, Yang X, Cui XG, et al. Curcumin alleviates oxidative stress and inhibits apoptosis in diabetic cardiomyopathy via Sirt1-Foxo1 and PI3K-akt signalling pathways. J Cell Mol Med (2020) 24(21):12355–67. doi: 10.1111/jcmm.15725
82. Zhu H, Wang X, Wang X, Liu B, Yuan Y, Zuo X. Curcumin attenuates inflammation and cell apoptosis through regulating NF-κB and JAK2/STAT3 signaling pathway against acute kidney injury. Cell Cycle (2020) 19(15):1941–51. doi: 10.1080/15384101.2020.1784599
83. El-Desoky G, Abdel-Ghaffar A, Al-Othman Z, Habila M, Al-Sheikh Y, Ghneim H, et al. Curcumin protects against tartrazine-mediated oxidative stress and hepatotoxicity in male rats. Eur Rev Med Pharmacol Sci (2017) 21(2017):635–45.
Keywords: curcumin, oxidative stress, inflammation, apoptosis, immuno-regulation
Citation: Hu P, Li K, Peng X-X, Kan Y, Yao T-J, Wang Z-Y, Li Z, Liu H-Y and Cai D (2023) Curcumin derived from medicinal homologous foods: its main signals in immunoregulation of oxidative stress, inflammation, and apoptosis. Front. Immunol. 14:1233652. doi: 10.3389/fimmu.2023.1233652
Received: 02 June 2023; Accepted: 26 June 2023;
Published: 11 July 2023.
Edited by:
Xihong Zhou, Chinese Academy of Sciences (CAS), ChinaReviewed by:
Peng Chen, Guangzhou University of Chinese Medicine, ChinaCopyright © 2023 Hu, Li, Peng, Kan, Yao, Wang, Li, Liu and Cai. This is an open-access article distributed under the terms of the Creative Commons Attribution License (CC BY). The use, distribution or reproduction in other forums is permitted, provided the original author(s) and the copyright owner(s) are credited and that the original publication in this journal is cited, in accordance with accepted academic practice. No use, distribution or reproduction is permitted which does not comply with these terms.
*Correspondence: Hao-Yu Liu, aGFveXUubGl1QHl6dS5lZHUuY24=; Demin Cai, ZGVtaW5jYWlAeXp1LmVkdS5jbg==
†These authors have contributed equally to this work
Disclaimer: All claims expressed in this article are solely those of the authors and do not necessarily represent those of their affiliated organizations, or those of the publisher, the editors and the reviewers. Any product that may be evaluated in this article or claim that may be made by its manufacturer is not guaranteed or endorsed by the publisher.
Research integrity at Frontiers
Learn more about the work of our research integrity team to safeguard the quality of each article we publish.