- 1National Clinical Research Center for Metabolic Diseases, Metabolic Syndrome Research Center, Key Laboratory of Diabetes Immunology, Ministry of Education, Department of Metabolism and Endocrinology, The Second Xiangya Hospital of Central South University, Changsha, Hunan, China
- 2Xiangya School of Public Health, Central South University, Changsha, China
- 3Furong Laboratory, Central South University, Changsha, China
Autoimmune diseases are heterogeneous disorders believed to stem from the immune system’s inability to distinguish between auto- and foreign- antigens. B lymphocytes serve a crucial role in humoral immunity as they generate antibodies and present antigens. Dysregulation of B cell function induce the onset of autoimmune disorders by generating autoantibodies and pro-inflammatory cytokines, resulting in an imbalance in immune regulation. New research in immunometabolism shows that cellular metabolism plays an essential role in controlling B lymphocytes immune reactions by providing the energy and substrates for B lymphocytes activation, differentiation, and function. However, dysregulated immunometabolism lead to autoimmune diseases by disrupting self-tolerance mechanisms. This review summarizes the latest research on metabolic reprogramming of B lymphocytes in autoimmune diseases, identifying crucial pathways and regulatory factors. Moreover, we consider the potential of metabolic interventions as a promising therapeutic strategy. Understanding the metabolic mechanisms of B cells brings us closer to developing novel therapies for autoimmune disorders.
Introduction
Autoimmune disorders manifest when the immune system erroneously attacks the organs, tissues, or cells of the body (1). Typically, the crucial role of humoral immunity is to safeguard the body from external pathogens, ensuring a balanced state of homeostasis. However, in autoimmune disorders the immune system becomes dysregulated and attacks healthy tissue and cells, causing damage to the body (2). The incidence of autoimmune diseases is increasing globally, affecting around 5% of the world’s population, making it a major public health concern (3). Existing evidence indicates that the development of autoimmune diseases involves a multifaceted interaction among genetic predisposition, environmental risk factors (including infections, exposure to harmful chemicals, and smoking), and immune dysregulation. This intricate interplay ultimately brings about the defect of self-tolerance and the onset of these disorders (3, 4).
Recent evidence has increasingly indicated the significant role of B lymphocytes in autoimmune disorders (5–8). Autoreactive B cells function to present self-derived peptides to autoreactive T cells. This interaction triggers T cell activation and promotes the generation of pathogenic autoantibodies from B cells (9). Progress in immunometabolism research has progressively recognized the substantial function of B lymphocyte metabolism modulation in autoimmune disorders (10, 11). B cells adjust their metabolic signaling pathways such as glycolysis and oxidative phosphorylation to fulfill the energy and biosynthetic requirements essential for their proliferation and division. The regulation and reprogramming of metabolic pathways provide the necessary metabolic support for B cell function and immune responses (12). However, imbalances in immunometabolism may lead to autoreactive B cells evading self-tolerance checkpoints, thereby inducing autoimmune diseases (13). Currently, research on B cell metabolism lags behind that of T cell metabolism, and further exploration of B cell metabolism represents a challenging yet promising area of study (14). In brief, we aim to provide a comprehensive conclusion of the latest research findings, focusing on the metabolic reprogramming of B cells in autoimmune disorders. We highlight key metabolic pathways and regulatory factors that manipulate B cell homeostasis, differentiation, and function (Figure 1), and also discuss the potential of metabolic interventions as a treatment for autoimmune disorders (Figure 2).
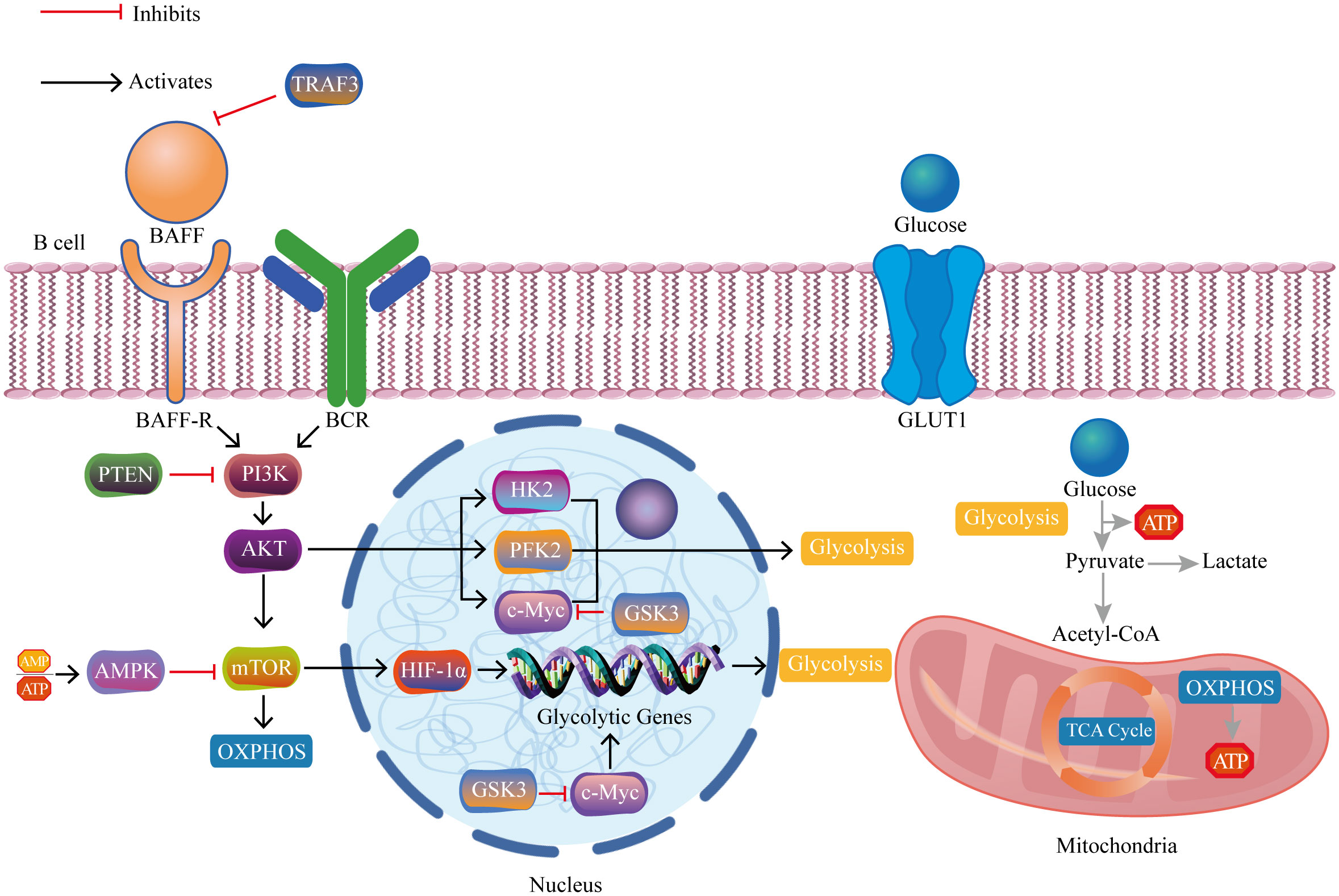
Figure 1 The crucial pathways and regulatory molecules of B cell metabolic reprogramming in autoimmune diseases. The interaction between BAFF and its receptor BAFF-R on B cell membranes triggers activation of the PI3K/AKT/mTOR/HIF-1α pathway. HIF-1α, together with c-Myc, upregulates genes associated with glycolysis, thereby promoting glucose uptake and glycolytic metabolism. TRAF3 inhibits excessive BAFF expression and its activation-related downstream cascades. PTEN inhibits PI3K and its activation-related downstream cascades. AMPK inhibits mTOR and its activation-related downstream cascades. GSK3 inhibits c-Myc and its activation-related downstream cascades. BAFF, B cell activating factor; BAFF-R, B cell activating factor receptor; BCR, B cell receptor; PI3K, phosphoinositide 3-kinase; AKT, protein kinase B; mTOR, mammalian target of rapamycin complex; HIF-1α, hypoxia-inducible factor 1-alpha; c-Myc, cellular myelocytomatosis oncogene; TRAF3, tumor necrosis factor receptor-associated factor 3; AMP, adenosine monophosphate; ATP, adenosine triphosphate; AMPK, AMP-activated protein kinase; PTEN, phosphatase and tensin homolog; GSK3, glycogen synthase kinase 3; HK2, hexokinase 2; PFK2, phosphofructokinase 2; GLUT1, glucose transport 1; TCA cycle, tricarboxylic acid cycle; OXPHOS, oxidative phosphorylation.
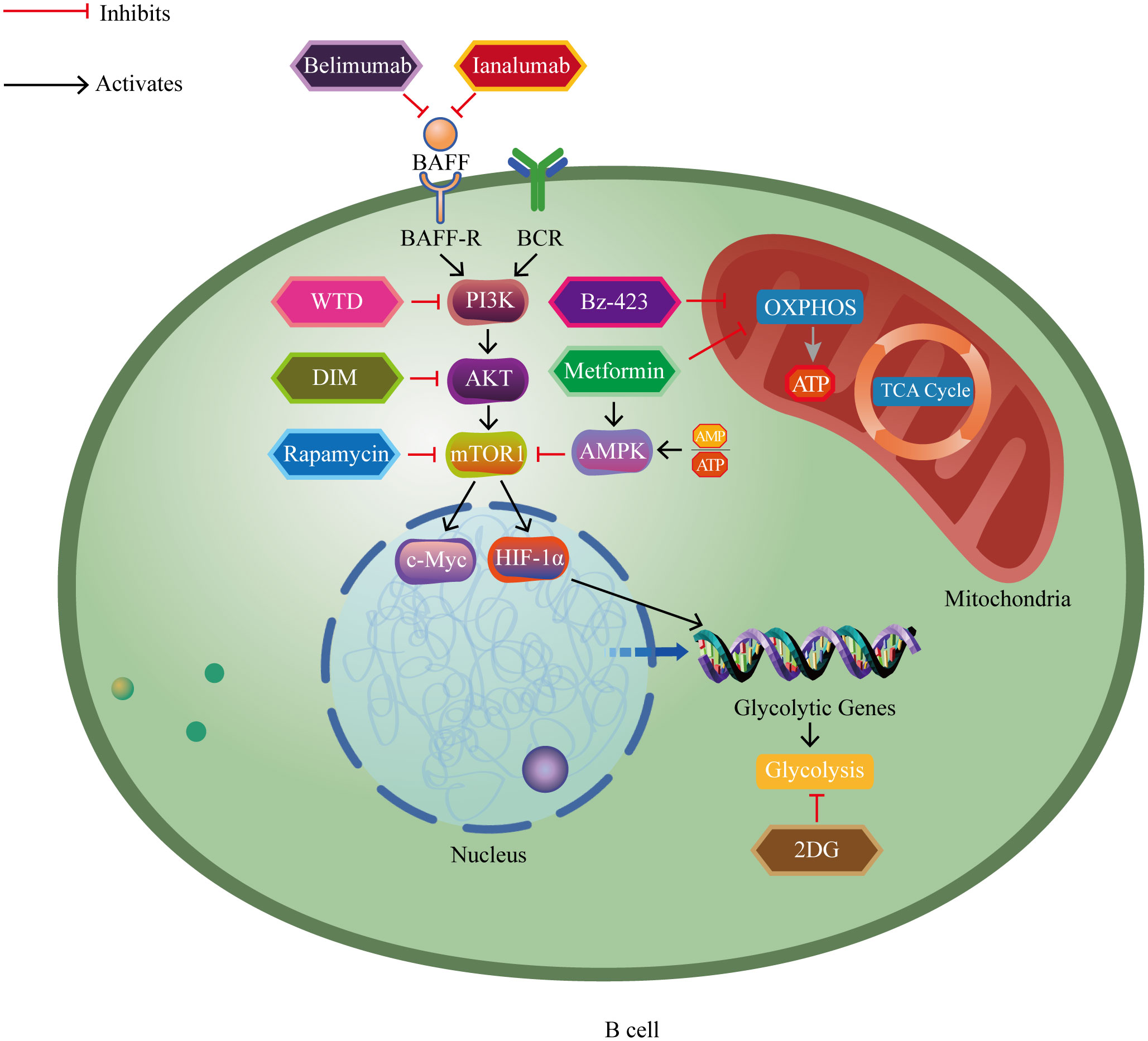
Figure 2 The impact of major metabolic drugs for treating autoimmune diseases on B cell metabolism pathways. Belimumab and ianalumab inhibit overexpression of BAFF and its activation-related downstream cascades. Rapamycin inhibits mTOR and its activation-related downstream cascades. Metformin activates AMPK, thereby inhibiting mTOR and its activation-related downstream cascades. 2DG inhibits glycolysis. WTD blocks the PI3K-AKT-mTOR-HIF-1α pathway and its activation-related downstream cascades. DIM inhibits the AKT/mTOR pathway and its activation-related downstream cascades. Metformin and Bz-423 inhibit oxidative phosphorylation. BAFF, B cell activating factor; BAFF-R, B cell activating factor receptor; BCR, B cell receptor; PI3K, phosphoinositide 3-kinase; AKT, protein kinase B; mTOR1, mammalian target of rapamycin complex 1; HIF-1α, hypoxia-inducible factor 1-alpha; c-Myc, cellular myelocytomatosis oncogene; AMP, adenosine monophosphate; ATP, adenosine triphosphate; AMPK, AMP-activated protein kinase; WTD, Wutou decoction; DIM, 3’3-Diindolylmethane; 2DG, 2-deoxy-D-glucose; Bz-423, 1,4-benzodiazepine; TCA cycle, tricarboxylic acid cycle; OXPHOS, oxidative phosphorylation.
Systemic lupus erythematosus
Glycolysis
Systemic lupus erythematosus (SLE), a refractory and chronic autoimmune disorders, affects the skin, kidneys, and blood (15). B cell tolerance checkpoints function to limit the potential harm posed by autoreactive B cell repertoire and are related to important metabolic components. Dysfunction of these checkpoints is one of the mechanisms underlying the pathogenesis of SLE. For example, metabolic imbalances may compromise the function of these checkpoints, and heightened exposure to B cell activating factor (BAFF) proves a prominent mechanism bringing about tolerance defects. Individuals diagnosed with SLE exhibit significantly elevated BAFF levels in their serum compared to healthy individuals (16). Furthermore, individuals diagnosed with lupus nephritis (LN) had elevated levels of BAFF biological activity compared to individuals diagnosed with SLE but without LN. BAFF levels are positively related to disease activity. A study suggests that SLE patients receiving intensified treatment with high-dose glucocorticoids (GCs) exhibit a significant decrease levels of BAFF in their serum. Conversely, the serum BAFF levels increase when GC dosage is reduced (17). B cell activating factor (BAFF), a type II membrane-bound category protein, primarily promotes B cell activation and differentiation by combining with receptors on B cells: B cell activating factor receptor (BAFF-R), transmembrane activator and calcium modulator and cyclophilin ligand interactor (TACI), and B cell maturation antigen (BCMA) (18). The ability of BAFF in the maintenance and enhancing of B lymphocytes immune reaction is of great importance. However, increased levels of BAFF in SLE perhaps lead to abnormal B lymphocytes activation and autoimmune dysregulation (19, 20). Transgenic mice overexpressing the BAFF gene exhibit various features, including polyclonal hypergammaglobulinemia, heightened levels of anti-double-stranded DNA (anti-dsDNA) autoantibodies and deposition of immunoglobulins in the kidneys (21–23). This phenomenon is attributed to excessive BAFF-mediated rescue of autoreactive B cells, promoting their survival and proliferation (24). Research indicates that BAFF treatment leads to an augmentation in protein levels and the expression of genes that facilitate glycolytic metabolism (25). Compared to normal B cells after stimulated by lipopolysaccharide (LPS), B cells derived from BAFF transgenic mice exhibited increased glycolytic level (26). BAFF holds a prominent position in autoimmune disorders, particularly via disrupting the B cells glucose homeostasis. Prolonged exposure to BAFF activates the Erk1/2 pathway, leading to the promotion of glycolysis, enhanced cellular proliferation, and the rescue of autoreactive B cells. BAFF’s connection with receptors activates the PI3K/AKT/mTOR pathway. It is supported by research that the aforementioned pathway displays heightened activity within the B cells of individuals affected by SLE. Moreover, it demonstrates elevated expression levels in a manner that is influenced by both age and the dosage of lupus susceptibility genes (27). Bisphenol A (BPA), a commonly found environmental chemical, disrupts endocrine function and exhibits estrogenic properties. Exposure to BPA leads to upregulation of the PI3K/AKT/mTOR signaling pathway, which induces the onset of SLE (28). The signaling pathway known as phosphoinositide 3-kinase (PI3K) holds significant importance in the processes of B cell activation and maturation. PI3K is activated upon stimulation through surface signaling receptors such as BAFF-R, toll-like receptors (TLRs), IL-4 receptors (IL-4R), CD40. This leads to the recruitment of AKT to the cell membrane, where it gets phosphorylated by phosphoinositide-dependent kinase 1 (PDK1) and the mammalian target of rapamycin complex 2 (mTORC2). The PI3K signaling pathway regulates metabolism by targeting metabolic genes such as hexokinase 2 (HK2), phosphofructokinase 2 (PFK2), and cellular myelocytomatosis oncogene (c-Myc) (29). AKT, a serine/threonine kinase, regulates over 100 known substrates, thereby collectively controlling cellular growth, metabolism, and cell cycle. Its downstream signaling nodes include mammalian target of rapamycin complex 1 (mTORC1) and glycogen synthase kinase 3 (GSK3) (30). Studies have confirmed a remarkable increase in AKT in unstimulated B cells in a SLE mouse model (27). mTOR, a serine/threonine kinase, executes cellular functions including survival, differentiation, and metabolism by forming two complexes defined as mTOR complex 1 and 2 (mTORC1 and mTORC2) (31). Although these complexes share the “ancillary” proteins of mTOR, they regulate distinct functions and can be distinguished by the distinct involvement of scaffold proteins Raptor and Rictor (32). B cells in SLE display higher mTORC1 activity, promoting plasmablasts generation (33). The overactivated B cells upregulate the expression of mTORC1, which facilitates B lymphocyte proliferation and survival. mTORC1 further enhances glycolysis, thereby allowing autoreactive B cells to evade metabolic restrictions (13, 34).
Two crucial inhibitory regulators of the PI3K/AKT/mTOR pathway, the phosphatase and tensin homolog (PTEN) and AMP-activated protein kinase (AMPK), play considerable roles in maintaining B cell homeostasis. B cells derived from individuals with SLE demonstrate diminished PTEN expression compared with B cells from healthy individuals. Furthermore, the expression level of PTEN is inversely correlated with disease activity (35). Phosphatase and tensin homolog (PTEN) is critical depressor of cellular growth and proliferation, and it also exerts a significant influence on the regulation of immune system balance. PTEN functions to negatively regulate the PI3K/AKT/mTOR pathway via its bioactivity of lipid phosphatase (36). Specifically, PTEN directly counteracts PI3K, thereby preventing B cells from becoming excessively activated (37). AMP-activated protein kinase (AMPK) functions to detect the AMP/ATP ratio within the cell, initiating the activation of AMPK while concurrently inhibiting the mTOR signaling pathway when the ratio surpasses a certain threshold (38). AMPK and mTOR signaling pathways are in a dynamic balance during the development of B cells (39).
Ping Xie’s research provides compelling evidence for the indispensable contribution of TRAF3 in maintaining the homeostasis of B lymphocytes. Specifically, TRAF3-deficient B cell in mice leads to significant expansion of peripheral B cells, resulting in elevated levels of immunoglobulinemia. This condition is accompanied by enhanced T-independent antibody responses, as well as splenomegaly and lymphadenopathy, which are hallmark features of autoimmune disorders (40). A recent study has revealed that TRAF3 knockdown attenuates lupus nephritis symptoms in mice, including urinary protein excretion and renal inflammation, implying that targeting TRAF3 presents a promising avenue for therapeutic intervention in SLE (41). The above researches show that TRAF3 loss in B cells induce autoimmune diseases under physiological conditions, while TRAF3 knockdown alleviates SLE symptoms under pathological conditions. Tumor necrosis factor receptor (TNFR)-associated factor 3 (TRAF3), a cytoplasmic adapter protein, primarily regulating B cell signaling pathways (42). TRAF3 deficiency induces the overactivation of B cells by intrinsically activating multiple pro-inflammatory pathways which increases the risk of autoimmune disorders (43). Recent studies have demonstrated that TRAF3 ablation in B cell induces glycolysis (44). TRAF3 deficiency promotes the glucose metabolism of B cells, characterized by the upregulation of glucose transport 1 (GLUT1) and hexokinase 2 (HK2).
The dysregulated activation of the AKT/GSK3β signaling pathway in the lymphocytes of individuals with SLE makes a profound contribution to the development of the disease (45). Glycogen synthase kinase-3 (GSK3), a widely expressed kinase, is known to have more than 100 substrates, impacting cellular differentiation, proliferation, survival, and transformation (46). GSK3 exerts a restraining effect on B cell activation through the suppression of metabolic activity induced by CD40 and IL-4. Furthermore, GSK3 downregulates c-Myc-dependent glycolysis regulating energy production (47). Studies have reported that GSK3 inactivation synergistically induces the transcription factors Foxo1 and c-Myc, promoting the formation of plasma cells under CD40L and IL-21 stimulation (48).
Belimumab, a BAFF inhibitor, has received approval from the FDA as the sole targeted medication that has demonstrated effective outcomes in the treatment of SLE (49). Belimumab, a recombinant human immunoglobulin G (IgG)1-l monoclonal antibody, significantly reduces disease activity, decreases the use of steroids, and improves health-related quality of life (50). Belimumab specifically targets and binds to soluble BAFF, effectively counteracting its biological effects by obstructing its contact with BAFF-R, TACI, and BCMA receptors. According to a phase III randomized controlled trial study, belimumab is a promising treatment option for lupus nephritis in East Asian populations due to its efficacy and safety (51). A randomized phase III/IV clinical trial conducted over a period of 52 weeks, demonstrates that belimumab treatment yields greater improvement in disease severity compared to placebo in African-American patients with SLE (52). A retrospective observational study found that belimumab exhibits efficacy as a viable treatment option for individuals undergoing maintenance therapy for SLE (53). A retrospective, single-center study revealed that belimumab administration in pediatric patients with childhood-onset SLE has demonstrated positive effects on laboratory parameters, disease activity reduction, and a potential decrease in glucocorticoid dosage requirements. Furthermore, belimumab has exhibited a favorable safety record (54). Povetacicept, an enhanced dual APRIL/BAFF antagonist, demonstrates remarkable improvements in multiple disease indicators in a murine lupus model (55). Compared to conventional treatment, the combination of belimumab and low-dose intravenous cyclophosphamide (CYC) therapy presents a remarkable restoration of T and B cell equilibrium and significantly reduces disease activity scores among patients diagnosed with SLE. This treatment also significantly reduces adverse events such as infections (56).
Rapamycin, a promising therapeutic approach for SLE, functions as an inhibitor of mechanistic Target of Rapamycin Complex 1 (mTORC1), based on a clinical trial (57). During a 12-month period of administering rapamycin, the condition of SLE patients showed a steady amelioration in disease activity (58). Another study also demonstrated that rapamycin can attenuate pathological changes and reduce anti-dsDNA antibody titers in a mouse model of SLE (59). Rapamycin inhibits mTORC1, which reduces cellular uptake of glucose and glutamine, and inhibits glycolysis and glutamine degradation (27).
Treatment with 2-deoxy-D-glucose (2DG) to inhibit glycolysis greatly reduced the production of T cell-independent (TI) antigen-specific antibodies (60). In vivo treatment with metformin and 2DG reversed disease biomarkers and improved autoimmune symptoms in lupus-prone mice (61). Metformin is known to activate AMPK, leading to the downregulation of mTORC1 activity, which induces ATP breakdown pathways like glycolysis and fatty acid oxidation (FAO), while inhibiting ATP synthesis pathways including gluconeogenesis and lipogenesis. Metformin will be discussed later in the oxidative phosphorylation section.
Oxidative phosphorylation
B cell metabolism like glycolysis and mitochondrial respiration were both increased in lupus-prone mice. As mentioned earlier, the increased BAFF not only upregulates glycolysis but also promotes the influx of pyruvate into the mitochondria, enhancing oxidative metabolism (26, 62) and inducing an elevation in mitochondrial membrane potential (25). BAFF activates downstream mTORC1, promoting an increase in mitochondrial mass and OXPHOS, thereby enabling the autoreactive B cells to evade energy crises (13, 34). BAFF inhibitor belimumab also has the ability to inhibit OXPHOS (20, 63).
The deficiency of TRAF3 also induces B cell hyperactivation by enhancing mitochondrial respiration, thereby increasing the risk of autoimmune diseases (43). TRAF3 ablation in B cell induces glucose uptake and oxidative phosphorylation enhancement, while mitochondrial mass and reactive oxygen species (ROS) production remain unchanged (44). Furthermore, downregulation of GSK3 attenuates oxidative respiration, thereby reducing ROS-mediated cellular toxicity (47).
Metformin is a commonly medication for managing type 2 diabetes. It reduces blood glucose levels through multiple mechanisms, including enhancing cellular glucose uptake and utilization, suppressing hepatic gluconeogenesis, and improving insulin sensitivity (64). Metformin has been found to inhibit OXPHOS. Metformin ameliorates renal dysfunction in MRL/lpr lupus-prone mice, as proved by reductions in urinary protein and blood urea nitrogen levels. Furthermore, metformin decreases IgG and complement C3 deposition, and attenuates systemic and renal inflammation in this murine model (65). Metformin alleviates the autoimmune phenotype including kidney inflammation in lupus mice via restricting B cell transformation into plasma cells (PC) and germinal centers (GC) (66). A post-hoc analysis of metformin adjunctive therapy in Chinese patients diagnosed with mild to moderate SLE revealed that metformin improves therapy outcomes in SLE patients (67). Bz-423 is also a mitochondrial metabolism inhibitor. It targets mitochondrial F1F0ATP synthase, leading to increased ROS levels, which in turn induces apoptosis in autoreactive cells, thereby suppressing disease manifestations in lupus-prone mice (68–70).
Rheumatoid arthritis
Rheumatoid arthritis (RA), an intractable autoimmune condition, affects the hands, wrists, knees, elbows, and ankles, resulting in joint pain, swelling, stiffness, and functional impairment (71). Multiple investigations have consistently demonstrated that individuals diagnosed with RA exhibit increased levels of BAFF in their serum compared to healthy control (72–74). There exists a positive relationship between disease activity and serum BAFF levels (73). Belimumab is also used to treat RA (75). A Phase II randomized, double-blind clinical trial demonstrated the curative effect of belimumab in RA patients who have failed prior treatments, and it is generally well-tolerated (76). Increased mTOR activity has been observed in RA, and inhibiting mTOR has demonstrated moderate effectiveness in decreasing joint inflammation among individuals with RA (77, 78). Wutou Decoction (WTD) restrains angiogenesis by intercepting the PI3K-AKT-mTOR-HIF-1α pathway and improves RA symptoms in collagen-induced arthritis (CIA) model rats (79). 3’3-Diindolylmethane (DIM) restrains the generation and expansion of RA fibroblast-like synoviocytes (RA-FLSs) via inhibiting the AKT/mTOR pathway, reduces TNF-α-induced cytokines, and alleviates the severity of knee joint arthritis, preventing inflammation and knee joint destruction (80). A prospective randomized controlled study showed that metformin exhibited a notable improvement in the inflammatory response, disease severity, and living quality among individuals diagnosed with RA (81). Methotrexate (MTX) is a folate analogue compound that inhibits the folate metabolism enzyme and blocks a carbon transfer reaction necessary for de novo nucleotide synthesis. It has been used for the treatment of RA for over four decades (82).
Type 1 diabetes
Type 1 diabetes (T1D), an intractable disease primarily driven by T cells, where pancreatic beta cells are targeted and destroyed by these autoreactive T cells (83, 84). Currently, there is a prevailing belief that B lymphocytes function indispensably in T1D via expressing co-stimulatory molecules and presenting antigens, which ultimately contributes to the activation of autoreactive T lymphocytes (85). Increasing evidence supports the notion that B cell development, differentiation, function, and metabolism have significant impacts on the development of T1D (86–89). Nonobese diabetic (NOD) mice serve as an exceptional model for investigating type 1 diabetes in human (90). Studies have shown that NOD mice with B cell defects are protected from T1D development (91). NOD mice lacking toll-like receptor 7 (TLR7) exhibit impaired B cell antigen presentation and antibody secretion, which inhibits activation of diabetogenic and cytotoxic T cells, and prolongs the time before T1D manifests (92). Furthermore, T1D patients have reduced PTEN expression in B cells, potentially resulting in an increased B cell autoreactivity (93). Rituximab, a selective anti-CD20 monoclonal antibody, has proved to deplete B lymphocytes effectively (94). A phase II study demonstrated that rituximab treatment in recently diagnosed T1D patients significantly lowers glycated hemoglobin levels, elevates C-peptide levels, and reduces exogenous insulin requirement (95).
Other autoimmune diseases
Primary Sjögren’s Syndrome (pSS) is distinguished by immune attack on the exocrine glands. This condition results in diminished or lacking secretions, causing dryness in the mouth and eyes (96). Immunoglobulin A (IgA) nephropathy is distinguished by the accumulation of abundant IgA proteins in the glomeruli of the kidney (97). In both pSS and IgA nephropathy, the serum levels of BAFF are elevated compared to healthy levels (72, 98). In pSS patients, an increase in the AMPK/mTORC1 activity ratio in B cells of salivary glands inhibits synthetic metabolism and increases susceptibility to oxidative stress (99). Belimumab and ianalumab, two monoclonal antibodies targeting BAFF, have been developed as potential therapies for pSS. A phase II study proved that belimumab had some curative effect and safety in the therapy of pSS (100). In a double-blind, placebo-controlled, phase II study, ianalumab was found to effectively deplete B cells and provide therapeutic benefits to pSS patients without any significant side effects (101).
Conclusion and perspective
While targeting the metabolism of autoreactive B cells is a prospective strategy for the management of autoimmune disorders, there remain several challenges to be addressed in the future. Firstly, the current understanding of the metabolic reprogramming of B cell function under physiological and pathological conditions is still incomplete and disputable, limiting the ability to precisely target specific metabolic pathways for the treatment of certain autoimmune disorders. For instance, it is generally believed that resting B lymphocytes predominantly rely on mitochondrial oxidative phosphorylation for energy production, while activated B lymphocytes tend to favor glycolysis as their metabolic pathway to rapidly provide energy for proliferation, differentiation, and other functions (102–104). However, several studies have also demonstrated that stimulated B cells do not heavily rely on glycolysis. B cell functions were not impacted by glucose restriction. On contradiction, B cell growth and differentiation were significantly hindered by either the inhibition of OXPHOS or a restriction in glutamine (105). Additionally, research has revealed that GC B cells consume more glucose and exhibit greater sensitivity to glycolysis inhibition compared to naive B cells (47, 106). Contrary to this, studies have demonstrated that GC B cells utilize glycolysis minimally, primarily relying on fatty acid oxidation to generate energy (107). As is widely known, proliferating B lymphocytes heavily rely on lactate dehydrogenase A (LDHA) for aerobic glycolysis. Nevertheless, a recent study has revealed that in naive B cells, the deletion of the glycolytic enzyme LDHA leads to impaired germinal center formation and antibody production. The impact of LDHA knockout on activated B cells is relatively minor (108). In conclusion, there is a relatively limited and controversial amount of research data regarding B cell metabolism currently. Therefore, a substantial amount of further research is required to elucidate the role of B cell metabolism in autoimmune diseases.
Secondly, most experimental studies on B cell metabolism have focused on mice rather than humans. Although valuable insights have been gained through the use of genetically modified mice, it is necessary to consider the distinction between mouse and human immune systems and metabolic pathways. For instance, B cells upregulate GLUT1 expression to increase glucose uptake in mice. However, in humans, B cell glucose uptake relies on other transporters due to relatively lower expression of GLUT1 in these cells (102, 109). Therefore, to ensure the successful application of the research findings from mice to humans, further exploration and examination are indisputably necessary.
Thirdly, traditional therapeutic approaches for autoimmune diseases involve the use of glucocorticoids and immunosuppressive agents, which may lead to various side effects such as infections, osteoporosis, and hypertension (110). Accordingly, there is a continuous search for personalized drugs with high safety profiles. Combining metabolic modulators or biologics with existing immunosuppressive agents offers a potential solution to reduce the dosage of traditional medications, thereby lowering the incidence of adverse reactions. Furthermore, there are significant individual differences in drug tolerance and therapeutic efficacy among autoimmune disease patients receiving targeted metabolic therapy. Thus, employing metabolomics and other techniques to pinpoint specific biomarkers for precise treatment may be necessary. Finally, with the rapid progress of new technologies, there is a compelling need to expand the utilization of innovative methodologies such as single-cell metabolomics with mass spectrometry and spatial transcriptomics (111) in autoimmune disease research. Traditional metabolomics studies focus on the overall metabolism of B cells or large B cell subpopulations. However, leveraging single-cell metabolomics provides a promising avenue to uncover novel, functionally distinct subsets with unique metabolic patterns and gain comprehensive insights into metabolic events (112). Understanding the spatial dynamics of B cells in physiological or disease states, including their metabolic microenvironment and interactions with other leukocyte populations, is of utmost importance for comprehending B cell functionality.
In this review, we provide a concise overview of the metabolic alterations observed in B cells in autoimmune diseases, emphasizing critical signaling pathways and molecules. Targeting and restricting B cell metabolism have emerged as potential therapeutic strategies for treating autoimmune diseases. However, the influence of B cell metabolism on autoimmune diseases warrants further investigation. Taken together, the modulation of B cell metabolism may represent a promising stratagem and viable avenue for future autoimmune disorder treatments.
Author contributions
BZ, ZZ and JL conceived and designed the structure of the literature review. JL conducted literature research, drafted the manuscript and created the figures. MZ, JH and WL commented and revised the manuscript. All authors contributed to the article and approved the submitted version.
Funding
This work was supported by the Natural Science Foundation of China (No.82170795), the Natural Science Foundation of Hunan Province (No.2021JC0003) and the Central South University Research Programme of Advanced Interdisciplinary Studies (No.2023QYJC008).
Conflict of interest
The authors declare that the research was conducted in the absence of any commercial or financial relationships that could be construed as a potential conflict of interest.
Publisher’s note
All claims expressed in this article are solely those of the authors and do not necessarily represent those of their affiliated organizations, or those of the publisher, the editors and the reviewers. Any product that may be evaluated in this article, or claim that may be made by its manufacturer, is not guaranteed or endorsed by the publisher.
Abbreviations
SLE, Systemic lupus erythematosus; BAFF, B cell activating factor; LN, lupus nephritis; GCs, glucocorticoids; BAFF-R, B cell activating factor receptor; TACI, transmembrane activator and calcium modulator and cyclophilin ligand interactor; BCMA, B cell maturation antigen; anti-dsDNA, anti-double-stranded DNA; LPS, lipopolysaccharide; BPA, Bisphenol A; PI3K, phosphoinositide 3-kinase; TLRs, toll-like receptors; IL-4R, IL-4 receptors; PDK1, phosphoinositide-dependent kinase 1; mTORC2, the mammalian target of rapamycin complex 2; HK2, hexokinase 2; PFK2, phosphofructokinase 2; c-Myc, cellular myelocytomatosis oncogene; mTORC1, the mammalian target of rapamycin complex 1; GSK3, glycogen synthase kinase 3; OXPHOS, oxidative phosphorylation; PTEN, phosphatase and tensin homolog; AMPK, AMP-activated protein kinase; TRAF3, Tumor necrosis factor receptor(TNFR)-associated factor 3; GLUT1, glucose transport 1; IgG, immunoglobulin G; CYC, cyclophosphamide; PC, plasma cells; GC, germinal centers; 2DG, 2-deoxy-D-glucose; TI, T cell-independent; FAO, fatty acid oxidation; ROS, reactive oxygen species; Bz-423, 1,4-benzodiazepine; RA, Rheumatoid arthritis; WTD, Wutou Decoction; DIM, collagen-induced arthritis(CIA)3’3-Diindolylmethane; RA-FLSs, RA fibroblast-like synoviocytes; MTX, Methotrexate; T1D, Type 1 diabetes; NOD, nonobese diabetic; TLR7, toll-like receptor 7; pSS, Primary Sjögren’s Syndrome; IgA, Immunoglobulin A; LDHA, lactate dehydrogenase A.
References
1. Marrack P, Kappler J, Kotzin BL. Autoimmune disease: why and where it occurs. Nat Med (2001) 7(8):899–905. doi: 10.1038/90935
2. Bieber K, Hundt JE, Yu X, Ehlers M, Petersen F, Karsten CM, et al. Autoimmune pre-disease. Autoimmun Rev (2023) 22(2):103236. doi: 10.1016/j.autrev.2022.103236
3. Miyauchi E, Shimokawa C, Steimle A, Desai MS, Ohno H. The impact of the gut microbiome on extra-intestinal autoimmune diseases. Nat Rev Immunol (2023) 23(1):9–23. doi: 10.1038/s41577-022-00727-y
4. Stojanovich L. Stress and autoimmunity. Autoimmun Rev (2010) 9(5):A271–6. doi: 10.1016/j.autrev.2009.11.014
5. Renaudineau Y, Garaud S, Le Dantec C, Alonso-Ramirez R, Daridon C, Youinou P. Autoreactive B cells and epigenetics. Clin Rev Allergy Immunol (2010) 39(1):85–94. doi: 10.1007/s12016-009-8174-6
6. Caielli S, Wan Z, Pascual V. Systemic lupus erythematosus pathogenesis: interferon and beyond. Annu Rev Immunol (2023) 41:533–60. doi: 10.1146/annurev-immunol-101921-042422
7. Scherer HU, van der Woude D, Toes REM. From risk to chronicity: evolution of autoreactive B cell and antibody responses in rheumatoid arthritis. Nat Rev Rheumatol (2022) 18(7):371–83. doi: 10.1038/s41584-022-00786-4
8. Yang M, Long D, Hu L, Zhao Z, Li Q, Guo Y, et al. AIM2 deficiency in B cells ameliorates systemic lupus erythematosus by regulating Blimp-1-Bcl-6 axis-mediated B-cell differentiation. Signal Transduct Target Ther (2021) 6(1):341. doi: 10.1038/s41392-021-00725-x
9. Nashi E, Wang Y, Diamond B. The role of B cells in lupus pathogenesis. Int J Biochem Cell Biol (2010) 42(4):543–50. doi: 10.1016/j.biocel.2009.10.011
10. Fu Y, Wang L, Yu B, Xu D, Chu Y. Immunometabolism shapes B cell fate and functions. Immunology (2022) 166(4):444–57. doi: 10.1111/imm.13499
11. Muschen M. Metabolic gatekeepers to safeguard against autoimmunity and oncogenic B cell transformation. Nat Rev Immunol (2019) 19(5):337–48. doi: 10.1038/s41577-019-0154-3
12. Jellusova J. The role of metabolic checkpoint regulators in B cell survival and transformation. Immunol Rev (2020) 295(1):39–53. doi: 10.1111/imr.12855
13. Raza IGA, Clarke AJ. B cell metabolism and autophagy in autoimmunity. Front Immunol (2021) 12:681105. doi: 10.3389/fimmu.2021.681105
14. Jellusova J. Metabolic control of B cell immune responses. Curr Opin Immunol (2020) 63:21–8. doi: 10.1016/j.coi.2019.11.002
15. Lou H, Ling GS, Cao X. Autoantibodies in systemic lupus erythematosus: From immunopathology to therapeutic target. J Autoimmun (2022) 132:102861. doi: 10.1016/j.jaut.2022.102861
16. Itotagawa E, Tomofuji Y, Kato Y, Konaka H, Tsujimoto K, Park J, et al. SLE stratification based on BAFF and IFN-I bioactivity for biologics and implications of BAFF produced by glomeruli in lupus nephritis. Rheumatol (Oxford) (2022) 62(5):1988–97. doi: 10.1093/rheumatology/keac528
17. Stohl W, Metyas S, Tan SM, Cheema GS, Oamar B, Xu D, et al. B lymphocyte stimulator overexpression in patients with systemic lupus erythematosus: longitudinal observations. Arthritis Rheumatol (2003) 48(12):3475–86. doi: 10.1002/art.11354
18. Kalled SL. The role of BAFF in immune function and implications for autoimmunity. Immunol Rev (2005) 204:43–54. doi: 10.1111/j.0105-2896.2005.00219.x
19. Vincent FB, Morand EF, Mackay F. BAFF and innate immunity: new therapeutic targets for systemic lupus erythematosus. Immunol Cell Biol (2012) 90(3):293–303. doi: 10.1038/icb.2011.111
20. Mockel T, Basta F, Weinmann-Menke J, Schwarting A. B cell activating factor (BAFF): Structure, functions, autoimmunity and clinical implications in Systemic Lupus Erythematosus (SLE). Autoimmun Rev (2021) 20(2):102736. doi: 10.1016/j.autrev.2020.102736
21. Gross JA, Johnston J, Mudri S, Enselman R, Dillon SR, Madden K, et al. TACI and BCMA are receptors for a TNF homologue implicated in B-cell autoimmune disease. Nature (2000) 404(6781):995–9. doi: 10.1038/35010115
22. Mackay F, Woodcock SA, Lawton P, Ambrose C, Baetscher M, Schneider P, et al. Mice transgenic for BAFF develop lymphocytic disorders along with autoimmune manifestations. J Exp Med (1999) 190(11):1697–710. doi: 10.1084/jem.190.11.1697
23. Khare SD, Sarosi I, Xia XZ, McCabe S, Miner K, Solovyev I, et al. Severe B cell hyperplasia and autoimmune disease in TALL-1 transgenic mice. Proc Natl Acad Sci U S A. (2000) 97(7):3370–5. doi: 10.1073/pnas.97.7.3370
24. Thien M, Phan TG, Gardam S, Amesbury M, Basten A, Mackay F, et al. Excess BAFF rescues self-reactive B cells from peripheral deletion and allows them to enter forbidden follicular and marginal zone niches. Immunity (2004) 20(6):785–98. doi: 10.1016/j.immuni.2004.05.010
25. Patke A, Mecklenbrauker I, Erdjument-Bromage H, Tempst P, Tarakhovsky A. BAFF controls B cell metabolic fitness through a PKC beta- and Akt-dependent mechanism. J Exp Med (2006) 203(11):2551–62. doi: 10.1084/jem.20060990
26. Caro-Maldonado A, Wang R, Nichols AG, Kuraoka M, Milasta S, Sun LD, et al. Metabolic reprogramming is required for antibody production that is suppressed in anergic but exaggerated in chronically BAFF-exposed B cells. J Immunol (2014) 192(8):3626–36. doi: 10.4049/jimmunol.1302062
27. Wu T, Qin X, Kurepa Z, Kumar KR, Liu K, Kanta H, et al. Shared signaling networks active in B cells isolated from genetically distinct mouse models of lupus. J Clin Invest. (2007) 117(8):2186–96. doi: 10.1172/JCI30398
28. Dong Y, Gao L, Sun Q, Jia L, Liu D. Increased levels of IL-17 and autoantibodies following Bisphenol A exposure were associated with activation of PI3K/AKT/mTOR pathway and abnormal autophagy in MRL/lpr mice. Ecotoxicol Environ Saf. (2023) 255:114788. doi: 10.1016/j.ecoenv.2023.114788
29. Jellusova J, Rickert RC. The PI3K pathway in B cell metabolism. Crit Rev Biochem Mol Biol (2016) 51(5):359–78. doi: 10.1080/10409238.2016.1215288
30. Revathidevi S, Munirajan AK. Akt in cancer: Mediator and more. Semin Cancer Biol (2019) 59:80–91. doi: 10.1016/j.semcancer.2019.06.002
31. Yu L, Wei J, Liu P. Attacking the PI3K/Akt/mTOR signaling pathway for targeted therapeutic treatment in human cancer. Semin Cancer Biol (2022) 85:69–94. doi: 10.1016/j.semcancer.2021.06.019
32. Geier C, Perl A. Therapeutic mTOR blockade in systemic autoimmunity: Implications for antiviral immunity and extension of lifespan. Autoimmun Rev (2021) 20(12):102984. doi: 10.1016/j.autrev.2021.102984
33. Huang N, Perl A. Metabolism as a target for modulation in autoimmune diseases. Trends Immunol (2018) 39(7):562–76. doi: 10.1016/j.it.2018.04.006
34. McAllister E, Jellusova J. BAFF signaling in B cell metabolism. Curr Opin Immunol (2021) 71:69–74. doi: 10.1016/j.coi.2021.05.011
35. Wu XN, Ye YX, Niu JW, Li Y, Li X, You X, et al. Defective PTEN regulation contributes to B cell hyperresponsiveness in systemic lupus erythematosus. Sci Transl Med (2014) 6(246):246ra99. doi: 10.1126/scitranslmed.3009131
36. Bergholz JS, Wang Q, Wang Q, Ramseier M, Prakadan S, Wang W, et al. PI3Kbeta controls immune evasion in PTEN-deficient breast tumours. Nature (2023) 617(7959):139–46. doi: 10.1038/s41586-023-05940-w
37. Taylor H, Laurence ADJ, Uhlig HH. The role of PTEN in innate and adaptive immunity. Cold Spring Harb Perspect Med (2019) 9(12). doi: 10.1101/cshperspect.a036996
38. Steinberg GR, Hardie DG. New insights into activation and function of the AMPK. Nat Rev Mol Cell Biol (2023) 24(4):255–72. doi: 10.1038/s41580-022-00547-x
39. Chun Y, Kim J. AMPK-mTOR signaling and cellular adaptations in hypoxia. Int J Mol Sci (2021) 22(18):9765. doi: 10.3390/ijms22189765
40. Xie P, Stunz LL, Larison KD, Yang B, Bishop GA. Tumor necrosis factor receptor-associated factor 3 is a critical regulator of B cell homeostasis in secondary lymphoid organs. Immunity (2007) 27(2):253–67. doi: 10.1016/j.immuni.2007.07.012
41. Sun XQ, Liu WS, Zhang HM, Xuan CY, Wang Y, Fu BB. TRAF3 plays a role in lupus nephritis by regulating Th17 cell and Treg cell balance as well as NF-kappaB signaling pathway in mice. Gen Physiol Biophys (2022) 41(2):151–8. doi: 10.4149/gpb_2022005
42. Lin WW, Hostager BS, Bishop GA. TRAF3, ubiquitination, and B-lymphocyte regulation. Immunol Rev (2015) 266(1):46–55. doi: 10.1111/imr.12299
43. Rae W, Sowerby JM, Verhoeven D, Youssef M, Kotagiri P, Savinykh N, et al. Immunodeficiency, autoimmunity, and increased risk of B cell Malignancy in humans with TRAF3 mutations. Sci Immunol (2022) 7(74):eabn3800. doi: 10.1126/sciimmunol.abn3800
44. Mambetsariev N, Lin WW, Wallis AM, Stunz LL, Bishop GA. TRAF3 deficiency promotes metabolic reprogramming in B cells. Sci Rep (2016) 6:35349. doi: 10.1038/srep35349
45. Han Y, Zeng F, Tan G, Yang C, Tang H, Luo Y, et al. Hydrogen sulfide inhibits abnormal proliferation of lymphocytes via AKT/GSK3β Signal pathway in systemic lupus erythematosus patients. Cell Physiol Biochem (2013) 31(6):795–804. doi: 10.1159/000350097
46. Beurel E, Grieco SF, Jope RS. Glycogen synthase kinase-3 (GSK3): regulation, actions, and diseases. Pharmacol Ther (2015) 148:114–31. doi: 10.1016/j.pharmthera.2014.11.016
47. Jellusova J, Cato MH, Apgar JR, Ramezani-Rad P, Leung CR, Chen C, et al. Gsk3 is a metabolic checkpoint regulator in B cells. Nat Immunol (2017) 18(3):303–12. doi: 10.1038/ni.3664
48. Lee J, Park H, Lim J, Jin HS, Park Y, Jung YJ, et al. GSK3 restrains germinal center B cells to form plasma cells. J Immunol (2021) 206(3):481–93. doi: 10.4049/jimmunol.2000908
49. Navarra SV, Guzman RM, Gallacher AE, Hall S, Levy RA, Jimenez RE, et al. Efficacy and safety of belimumab in patients with active systemic lupus erythematosus: a randomised, placebo-controlled, phase 3 trial. Lancet (2011) 377(9767):721–31. doi: 10.1016/S0140-6736(10)61354-2
50. Blair HA, Duggan ST. Belimumab: A review in systemic lupus erythematosus. Drugs (2018) 78(3):355–66. doi: 10.1007/s40265-018-0872-z
51. Yu X, Chen N, Xue J, Mok CC, Bae SC, Peng X, et al. Efficacy and safety of belimumab in patients with lupus nephritis: subgroup analyses of a phase 3 randomized trial in the east asian population. Am J Kidney Dis (2023) 81(3):294–306 e1. doi: 10.1053/j.ajkd.2022.06.013
52. Ginzler E, Guedes Barbosa LS, D’Cruz D, Furie R, Maksimowicz-McKinnon K, Oates J, et al. Phase III/IV, randomized, fifty-two-week study of the efficacy and safety of belimumab in patients of black african ancestry with systemic lupus erythematosus. Arthritis Rheumatol (2022) 74(1):112–23. doi: 10.1002/art.41900
53. Miyazaki Y, Nakayamada S, Sonomoto K, Kawabe A, Inoue Y, Okubo N, et al. Efficacy and safety of belimumab during maintenance therapy in patients with systemic lupus erythematosus. Rheumatol (Oxford). (2022) 61(9):3614–26. doi: 10.1093/rheumatology/keab953
54. Wang D, Shan C, Liu J, Zhang R, Zhu G, Gao T, et al. Efficacy and safety of belimumab for the treatment of refractory childhood-onset systemic lupus erythematosus: A single-center, real-world, retrospective study. Front Immunol (2022) 13:1067721. doi: 10.3389/fimmu.2022.1067721
55. Samy E, Wax S, Huard B, Hess H, Schneider P. Targeting BAFF and APRIL in systemic lupus erythematosus and other antibody-associated diseases. Int Rev Immunol (2017) 36(1):3–19. doi: 10.1080/08830185.2016.1276903
56. Cheng H, Zhang XY, Yang HD, Yu Z, Yan CL, Gao C, et al. Efficacy and safety of belimumab/low-dose cyclophosphamide therapy in moderate-to-severe systemic lupus erythematosus. Front Immunol (2022) 13:911730. doi: 10.3389/fimmu.2022.911730
57. Palsson-McDermott EM, O’Neill LAJ. Targeting immunometabolism as an anti-inflammatory strategy. Cell Res (2020) 30(4):300–14. doi: 10.1038/s41422-020-0291-z
58. Lai ZW, Kelly R, Winans T, Marchena I, Shadakshari A, Yu J, et al. Sirolimus in patients with clinically active systemic lupus erythematosus resistant to, or intolerant of, conventional medications: a single-arm, open-label, phase 1/2 trial. Lancet (2018) 391(10126):1186–96. doi: 10.1016/S0140-6736(18)30485-9
59. Lui SL, Yung S, Tsang R, Zhang F, Chan KW, Tam S, et al. Rapamycin prevents the development of nephritis in lupus-prone NZB/W F1 mice. Lupus (2008) 17(4):305–13. doi: 10.1177/0961203307088289
60. Choi SC, Li W, Zhang X, Kanda N, Zeumer-Spataro L, Teng X, et al. Pharmacologically inferred glycolysis and glutaminolysis requirement of B cells in lupus-prone mice. J Immunol (2022) 208(9):2098–108. doi: 10.4049/jimmunol.2100356
61. Yin Y, Choi SC, Xu Z, Perry DJ, Seay H, Croker BP, et al. NorMalization of CD4+ T cell metabolism reverses lupus. Sci Transl Med (2015) 7(274):274ra18. doi: 10.1126/scitranslmed.aaa0835
62. Zhang CX, Wang HY, Yin L, Mao YY, Zhou W. Immunometabolism in the pathogenesis of systemic lupus erythematosus. J Transl Autoimmun (2020) 3:100046. doi: 10.1016/j.jtauto.2020.100046
63. Klemm P, Müller-Ladner U, Tarner IH, Lange U, Hudowenz O. Belimumab reduces antiphospholipid antibodies in primary triple-positive antiphospholipid syndrome. Autoimmun Rev (2020) 19(8):102594. doi: 10.1016/j.autrev.2020.102594
64. Rena G, Hardie DG, Pearson ER. The mechanisms of action of metformin. Diabetologia (2017) 60(9):1577–85. doi: 10.1007/s00125-017-4342-z
65. Chen XC, Wu D, Wu HL, Li HY, Yang C, Su HY, et al. Metformin improves renal injury of MRL/lpr lupus-prone mice via the AMPK/STAT3 pathway. Lupus Sci Med (2022) 9(1):e000611. doi: 10.1136/lupus-2021-000611
66. Lee SY, Moon SJ, Kim EK, Seo HB, Yang EJ, Son HJ, et al. Metformin Suppresses Systemic Autoimmunity in Roquin(san/san) Mice through Inhibiting B Cell Differentiation into Plasma Cells via Regulation of AMPK/mTOR/STAT3. J Immunol (2017) 198(7):2661–70. doi: 10.4049/jimmunol.1403088
67. Sun F, Zhang D, Wang H, Wang H, Liu Z, Geng S, et al. Attaining treat-to-target endpoints with metformin in lupus patients: a pooled analysis. Clin Exp Rheumatol (2022) 40(9):1733–7. doi: 10.55563/clinexprheumatol/7y5ku8
68. Johnson KM, Chen X, Boitano A, Swenson L, Opipari AW Jr., Glick GD. Identification and validation of the mitochondrial F1F0-ATPase as the molecular target of the immunomodulatory benzodiazepine Bz-423. Chem Biol (2005) 12(4):485–96. doi: 10.1016/j.chembiol.2005.02.012
69. Blatt NB, Bednarski JJ, Warner RE, Leonetti F, Johnson KM, Boitano A, et al. Benzodiazepine-induced superoxide signals B cell apoptosis: mechanistic insight and potential therapeutic utility. J Clin Invest. (2002) 110(8):1123–32. doi: 10.1172/JCI0216029
70. Bednarski JJ, Warner RE, Rao T, Leonetti F, Yung R, Richardson BC, et al. Attenuation of autoimmune disease in Fas-deficient mice by treatment with a cytotoxic benzodiazepine. Arthritis Rheumatol (2003) 48(3):757–66. doi: 10.1002/art.10968
71. Smolen JS, Aletaha D, McInnes IB. Rheumatoid arthritis. Lancet (2016) 388(10055):2023–38. doi: 10.1016/S0140-6736(16)30173-8
72. Santillan-Lopez E, Munoz-Valle JF, Oregon-Romero E, Espinoza-Garcia N, Trevino-Talavera BA, Salazar-Camarena DC, et al. Analysis of TNFSF13B polymorphisms and BAFF expression in rheumatoid arthritis and primary Sjogren’s syndrome patients. Mol Genet Genomic Med (2022) 10(6):e1950. doi: 10.1002/mgg3.1950
73. Zhang LL, Xiao H, Zhang F, Wu YJ, Shu JL, Li Y, et al. BAFF, involved in B cell activation through the NF-kappaB pathway, is related to disease activity and bone destruction in rheumatoid arthritis. Acta Pharmacol Sin (2021) 42(10):1665–75. doi: 10.1038/s41401-020-00582-4
74. Bosello S, Youinou P, Daridon C, Tolusso B, Bendaoud B, Pietrapertosa D, et al. Concentrations of BAFF correlate with autoantibody levels, clinical disease activity, and response to treatment in early rheumatoid arthritis. J Rheumatol (2008) 35(7):1256–64.
75. Jin X, Ding C. Belimumab–an anti-BLyS human monoclonal antibody for rheumatoid arthritis. Expert Opin Biol Ther (2013) 13(2):315–22. doi: 10.1517/14712598.2012.758248
76. Stohl W, Merrill JT, McKay JD, Lisse JR, Zhong ZJ, Freimuth WW, et al. Efficacy and safety of belimumab in patients with rheumatoid arthritis: a phase II, randomized, double-blind, placebo-controlled, dose-ranging Study. J Rheumatol (2013) 40(5):579–89. doi: 10.3899/jrheum.120886
77. Suto T, Karonitsch T. The immunobiology of mTOR in autoimmunity. J Autoimmun (2020) 110:102373. doi: 10.1016/j.jaut.2019.102373
78. Karonitsch T, Kandasamy RK, Kartnig F, Herdy B, Dalwigk K, Niederreiter B, et al. mTOR senses environmental cues to shape the fibroblast-like synoviocyte response to inflammation. Cell Rep (2018) 23(7):2157–67. doi: 10.1016/j.celrep.2018.04.044
79. Ba X, Huang Y, Shen P, Huang Y, Wang H, Han L, et al. WTD attenuating rheumatoid arthritis via suppressing angiogenesis and modulating the PI3K/AKT/mTOR/HIF-1alpha pathway. Front Pharmacol (2021) 12:696802. doi: 10.3389/fphar.2021.696802
80. Du H, Zhang X, Zeng Y, Huang X, Chen H, Wang S, et al. A novel phytochemical, DIM, inhibits proliferation, migration, invasion and TNF-alpha induced inflammatory cytokine production of synovial fibroblasts from rheumatoid arthritis patients by targeting MAPK and AKT/mTOR signal pathway. Front Immunol (2019) 10:1620. doi: 10.3389/fimmu.2019.01620
81. Gharib M, Elbaz W, Darweesh E, Sabri NA, Shawki MA. Efficacy and safety of metformin use in rheumatoid arthritis: A randomized controlled study. Front Pharmacol (2021) 12:726490. doi: 10.3389/fphar.2021.726490
82. Zhao Z, Hua Z, Luo X, Li Y, Yu L, Li M, et al. Application and pharmacological mechanism of methotrexate in rheumatoid arthritis. BioMed Pharmacother. (2022) 150:113074. doi: 10.1016/j.biopha.2022.113074
84. Quattrin T, Mastrandrea LD, Walker LSK. Type 1 diabetes. Lancet. (2023). 401(10394):2149–62. doi: 10.1016/S0140-6736(23)00223-4
85. Bone RN, Evans-Molina C. Combination immunotherapy for type 1 diabetes. Curr Diabetes Rep (2017) 17(7):50. doi: 10.1007/s11892-017-0878-z
86. Smith MJ, Simmons KM, Cambier JC. B cells in type 1 diabetes mellitus and diabetic kidney disease. Nat Rev Nephrol. (2017) 13(11):712–20. doi: 10.1038/nrneph.2017.138
87. Bloem SJ, Roep BO. The elusive role of B lymphocytes and islet autoantibodies in (human) type 1 diabetes. Diabetologia (2017) 60(7):1185–9. doi: 10.1007/s00125-017-4284-5
88. Deng C, Xiang Y, Tan T, Ren Z, Cao C, Huang G, et al. Altered peripheral B-lymphocyte subsets in type 1 diabetes and latent autoimmune diabetes in adults. Diabetes Care (2016) 39(3):434–40. doi: 10.2337/dc15-1765
89. Liu Y, Chen Z, Qiu J, Chen H, Zhou Z. Altered tim-1 and IL-10 expression in regulatory B cell subsets in type 1 diabetes. Front Immunol (2021) 12. doi: 10.3389/fimmu.2021.773896
90. Boldison J, Hopkinson JR, Davies J, Pearson JA, Leete P, Richardson S, et al. Gene expression profiling in NOD mice reveals that B cells are highly educated by the pancreatic environment during autoimmune diabetes. Diabetologia (2023) 66(3):551–66. doi: 10.1007/s00125-022-05839-7
91. Akashi T, Nagafuchi S, Anzai K, Kondo S, Kitamura D, Wakana S, et al. Direct evidence for the contribution of B cells to the progression of insulitis and the development of diabetes in non-obese diabetic mice. Int Immunol (1997) 9(8):1159–64. doi: 10.1093/intimm/9.8.1159
92. Huang J, Peng J, Pearson JA, Efthimiou G, Hu Y, Tai N, et al. Toll-like receptor 7 deficiency suppresses type 1 diabetes development by modulating B-cell differentiation and function. Cell Mol Immunol (2021) 18(2):328–38. doi: 10.1038/s41423-020-00590-8
93. Smith MJ, Ford BR, Rihanek M, Coleman BM, Getahun A, Sarapura VD, et al. Elevated PTEN expression maintains anergy in human B cells and reveals unexpectedly high repertoire autoreactivity. JCI Insight (2019) 4(3):e123384. doi: 10.1172/jci.insight.123384
94. Rougé L, Chiang N, Steffek M, Kugel C, Croll TI, Tam C, et al. Structure of CD20 in complex with the therapeutic monoclonal antibody rituximab. Science (2020) 367(6483):1224–30. doi: 10.1126/science.aaz9356
95. Pescovitz MD, Greenbaum CJ, Krause-Steinrauf H, Becker DJ, Gitelman SE, Goland R, et al. Rituximab, B-lymphocyte depletion, and preservation of beta-cell function. N Engl J Med (2009) 361(22):2143–52. doi: 10.1056/NEJMoa0904452
96. Thorlacius GE, Bjork A, Wahren-Herlenius M. Genetics and epigenetics of primary Sjogren syndrome: implications for future therapies. Nat Rev Rheumatol (2023) 19(5):288–306. doi: 10.1038/s41584-023-00932-6
97. Liu L, Khan A, Sanchez-Rodriguez E, Zanoni F, Li Y, Steers N, et al. Genetic regulation of serum IgA levels and susceptibility to common immune, infectious, kidney, and cardio-metabolic traits. Nat Commun (2022) 13(1):6859. doi: 10.1038/s41467-022-34456-6
98. Xin G, Shi W, Xu LX, Su Y, Yan LJ, Li KS. Serum BAFF is elevated in patients with IgA nephropathy and associated with clinical and histopathological features. J Nephrol. (2013) 26(4):683–90. doi: 10.5301/jn.5000218
99. Farmer JR, Allard-Chamard H, Sun N, Ahmad M, Bertocchi A, Mahajan VS, et al. Induction of metabolic quiescence defines the transitional to follicular B cell switch. Sci Signaling (2019) 12(604):eaaw5573. doi: 10.1126/scisignal.aaw5573
100. Mariette X, Seror R, Quartuccio L, Baron G, Salvin S, Fabris M, et al. Efficacy and safety of belimumab in primary Sjogren’s syndrome: results of the BELISS open-label phase II study. Ann Rheum Dis (2015) 74(3):526–31. doi: 10.1136/annrheumdis-2013-203991
101. Dorner T, Posch MG, Li Y, Petricoul O, Cabanski M, Milojevic JM, et al. Treatment of primary Sjogren’s syndrome with ianalumab (VAY736) targeting B cells by BAFF receptor blockade coupled with enhanced, antibody-dependent cellular cytotoxicity. Ann Rheum Dis (2019) 78(5):641–7. doi: 10.1136/annrheumdis-2018-214720
102. Doughty CA, Bleiman BF, Wagner DJ, Dufort FJ, Mataraza JM, Roberts MF, et al. Antigen receptor-mediated changes in glucose metabolism in B lymphocytes: role of phosphatidylinositol 3-kinase signaling in the glycolytic control of growth. Blood (2006) 107(11):4458–65. doi: 10.1182/blood-2005-12-4788
103. Dufort FJ, Bleiman BF, Gumina MR, Blair D, Wagner DJ, Roberts MF, et al. Cutting edge: IL-4-mediated protection of primary B lymphocytes from apoptosis via Stat6-dependent regulation of glycolytic metabolism. J Immunol (2007) 179(8):4953–7. doi: 10.4049/jimmunol.179.8.4953
104. Wang T, Jiao Y, Zhang X. Immunometabolic pathways and its therapeutic implication in autoimmune diseases. Clin Rev Allergy Immunol (2021) 60(1):55–67. doi: 10.1007/s12016-020-08821-6
105. Waters LR, Ahsan FM, Wolf DM, Shirihai O, Teitell MA. Initial B cell activation induces metabolic reprogramming and mitochondrial remodeling. iScience (2018) 5:99–109. doi: 10.1016/j.isci.2018.07.005
106. Jayachandran N, Mejia EM, Sheikholeslami K, Sher AA, Hou S, Hatch GM, et al. TAPP adaptors control B cell metabolism by modulating the phosphatidylinositol 3-kinase signaling pathway: A novel regulatory circuit preventing autoimmunity. J Immunol (2018) 201(2):406–16. doi: 10.4049/jimmunol.1701440
107. Weisel FJ, Mullett SJ, Elsner RA, Menk AV, Trivedi N, Luo W,, et al. Germinal center B cells selectively oxidize fatty acids for energy while conducting minimal glycolysis. Nat Immunol(2020) 21(3):331–42. doi: 10.1038/s41590-020-0598-4
108. Sharma R, Smolkin RM, Chowdhury P, Fernandez KC, Kim Y, Cols M, et al. Distinct metabolic requirements regulate B cell activation and germinal center responses. Nat Immunol (2023) 24(8):1358–69.doi: 10.1038/s41590-023-01540-y
109. Lam WY, Becker AM, Kennerly KM, Wong R, Curtis JD, Llufrio EM, et al. Mitochondrial pyruvate import promotes long-term survival of antibody-secreting plasma cells. Immunity (2016) 45(1):60–73. doi: 10.1016/j.immuni.2016.06.011
110. Fei Y, Shi X, Gan F, Li X, Zhang W, Li M, et al. Death causes and pathogens analysis of systemic lupus erythematosus during the past 26 years. Clin Rheumatol (2014) 33(1):57–63. doi: 10.1007/s10067-013-2383-3
111. Rao A, Barkley D, França GS, Yanai I. Exploring tissue architecture using spatial transcriptomics. Nature (2021) 596(7871):211–20. doi: 10.1038/s41586-021-03634-9
Keywords: autoimmune diseases, autoimmunity, B cell, B cell metabolism, B cell differentiation and function
Citation: Li J, Zhao M, Luo W, Huang J, Zhao B and Zhou Z (2023) B cell metabolism in autoimmune diseases: signaling pathways and interventions. Front. Immunol. 14:1232820. doi: 10.3389/fimmu.2023.1232820
Received: 01 June 2023; Accepted: 09 August 2023;
Published: 23 August 2023.
Edited by:
Rui Li, University of Pennsylvania, United StatesReviewed by:
Chaohong Liu, Huazhong University of Science and Technology, ChinaLinlu Tian, Medical College of Wisconsin, United States
Copyright © 2023 Li, Zhao, Luo, Huang, Zhao and Zhou. This is an open-access article distributed under the terms of the Creative Commons Attribution License (CC BY). The use, distribution or reproduction in other forums is permitted, provided the original author(s) and the copyright owner(s) are credited and that the original publication in this journal is cited, in accordance with accepted academic practice. No use, distribution or reproduction is permitted which does not comply with these terms.
*Correspondence: Bin Zhao, binzhao@csu.edu.cn; bin.zhao@live.com; Zhiguang Zhou, zhouzhiguang@csu.edu.cn
†These authors have contributed equally to this work