- 1Institute of Molecular Pathobiochemistry, Experimental Gene Therapy and Clinical Chemistry (IFMPEGKC), RWTH University Hospital Aachen, Aachen, Germany
- 2Institute of Neuroanatomy, RWTH University Hospital Aachen, Aachen, Germany
The human 25-kDa Lipocalin 2 (LCN2) was first identified and purified as a protein that in part is associated with gelatinase from neutrophils. This protein shows a high degree of sequence similarity with the deduced sequences of rat α2-microglobulin-related protein and the mouse protein 24p3. Based on its typical lipocalin fold, which consists of an eight-stranded, anti-parallel, symmetrical β-barrel fold structure it was initially thought that LCN2 is a circulating protein functioning as a transporter of small lipophilic molecules. However, studies in Lcn2 null mice have shown that LCN2 has bacteriostatic properties and plays a key role in innate immunity by sequestering bacterial iron siderophores. Numerous reports have further shown that LCN2 is involved in the control of cell differentiation, energy expenditure, cell death, chemotaxis, cell migration, and many other biological processes. In addition, important roles for LCN2 in health and disease have been identified in Lcn2 null mice and multiple molecular pathways required for regulation of Lcn2 expression have been identified. Nevertheless, although six putative receptors for LCN2 have been proposed, there is a fundamental lack in understanding of how these cell-surface receptors transmit and amplify LCN2 to the cell. In the present review we summarize the current knowledge on LCN2 receptors and discuss inconsistencies, misinterpretations and false assumptions in the understanding of these potential LCN2 receptors.
1 Introduction
Lipocalin 2 (LCN2; OMIM:600181), also known as neutrophil gelatinase-associated lipocalin (NGAL), siderocalin (Scn), oncogenic lipocalin 24p3, super inducible protein 24 (SIP24), uterocalin, α2-microglobulin-related protein, and neu-related lipocalin (NRL) is a 25-kDa protein involved with inflammatory response in multiple diseases. Historically, the first amino acid sequence of a LCN2 orthologue was deduced from a cDNA sequence of rat, which was isolated fortuitously whilst screening a library with a synthetic oligonucleotides designed to a sequence located in the ribosomal protein P1 (1). Based on its high degree of similarity with α2 microglobulin, rat LCN2 was initially termed α2-microglobulin-related protein (1). The identification of mouse LCN2 was reported one year later by Hraba-Renevey and colleagues (2). In their study they isolated mouse Lcn2 mRNA by differential screening as a gene that is induced by SV40 in Go-arrested primary mouse kidney cell cultures. The name 24p3 of this gene was given in a very pragmatic way and simply referred to the plate (no. 24) and the sequential assigned number of the cDNA sequence (no. 3) isolated from respective plate (Suzanne Hraba-Renevey, personal communication).
Subsequently, human LCN2 was identified another four years later and purified to apparent homogeneity from exocytosed material of phorbol myristate acetate-stimulated neutrophils and suggested to be a new member of the lipocalin family (3). This family includes small secreted proteins that share a characteristic three-dimensional fold comprised of a single eight-stranded continuously hydrogen-bonded antiparallel β-barrel (Figure 1). The calyx of this lipocalin fold is open at one end allowing uptake and binding of several small lipophilic molecules. This was the reason why LCN2 was initially thought to have transport functions. However, there is increasing evidence that many lipocalins exhibit great functional diversity and take over specialized and pleiotropic activities. This is especially documented in the numerous functions that have been reported for LCN2. Kjeldsen and coworkers already demonstrated that human LCN2 can be secreted in three different forms, namely its 25-kDa monomer, a ~46-kDa homodimer, and a ~135-kDa heterodimer composed out of LCN2 and metalloproteinase-9 (MMP-9) (3). This prompted to the assumption that LCN2 might modulate neutrophil gelatinase activity (3). The murine counterpart was identified as a superinducible protein with a molecular weight of 24-kDa (SIP24) in growth arrested, murine Balb/c 3T3 fibroblasts that become massively induced in response to mitogens, fibroblast growth factor (FGF), epidermal growth factor (EGF), and serum (5, 6). Similarly, hepatic expression of mouse LCN2/24p3 dramatically increased during turpentine-induced acute phase response and the production was significantly stimulated by tumor necrosis factor-α (TNF-α) (7). These findings suggest LCN2/SIP24/24p3 as a key systemic reactant to local or systemic inflammatory disturbances that is modulated in its expression by many factors. In line with its function as an acute-phase protein, hepatocytes were identified as a major source of LCN2 production mediating hepatoprotective effects in acute liver injury and showing positive correlation to hepatic inflammation (8, 9).
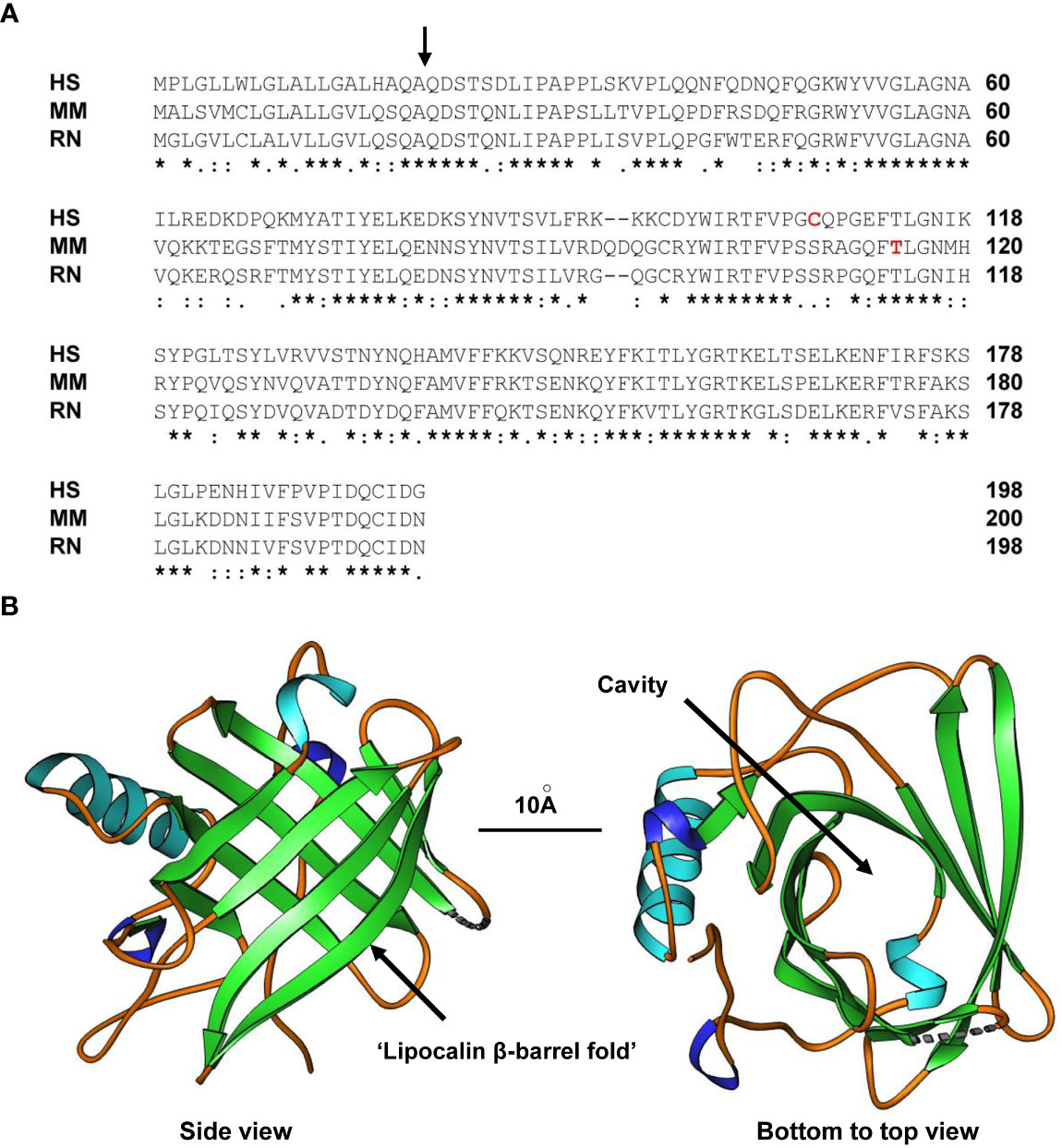
Figure 1 Structure of LCN2. (A) Alignment of human (HS), mouse (MM) and rat (RN) LCN2 proteins. The side of cleavage of the secretory signal peptide is marked by a black arrow. In addition, the tyrosine in mouse LCN2 (T115) shown to be a PKCδ phosphorylation site and the cysteine in human LCN2 (C107) shown to form the disulfide bridge with human MMP-9 is marked in red letters. An asterisk (*) indicates fully conserved residues, a colon (:) conservation between groups of strongly similar properties, and a period (.) conservation between groups of weakly similar properties. Gaps in the aligned sequences are indicated by dashes. (B) Side (left) and bottom-to-top (right) views of human LCN2 showing the typical eight-stranded, anti-parallel, symmetrical β-barrel fold that is characteristic of members of the lipocalin family. The formed cavity is supposed to permit binding of a broad array of bulky ligands. The depicted structure of the apo-form of human LCN2 determined by X-ray diffraction resolved at resolution of 2Å was generated using the Ribbons XP software (version 3.0) and structure coordinates deposited in the RCSB Protein Data Bank (4) [http://www.rcsb.org] under accession no. 3BX8. A size marker (10Å) is given.
Nowadays, there is additional ample evidence that LCN2 is a key molecule that regulates iron homeostasis. Although LCN2 itself does not have an intrinsic binding ability for iron, it can bind to siderophores, which are a group of diverse, small, high-affinity iron-chelating compounds that are secreted by microorganisms in response to iron limitation in their environment to increase iron uptake (10–12). Devireddy and coworkers proposed distinct iron-dependent activities for murine LCN2. They suggested that iron-laden LCN2 (holo-24p3) binds to a cell surface receptor termed 24p3R, is internalized, and releases its bound iron, thereby increasing the intracellular iron content (13). On the contrary, iron-free LCN2 (apo-24p3) internalized by binding to its receptor can associate with an intracellular iron chelating siderophore, take over bound iron and transfer it outside the cell, thereby reducing the overall intracellular iron concentration and inducing the BCL2-interacting protein BIM/BCL2L11 expression and stimulating cell apoptosis (13).
Studies in two independent generated gene-targeted Lcn2-deficient mice confirmed the essential iron-dependent role of LCN2 in the early stages of innate immune response (14, 15). Consistent with the function of LCN2 in regulating iron homeostasis, respective mouse models exhibited an increased sensitivity to bacterial infection under iron-limiting conditions (14, 15). It was suggested that neutrophils from Lcn2 null mice were less able to inhibit bacterial growth compared with wild type neutrophils, confirming LCN2 as a bacteriostatic factor preventing bacterial siderophore-mediated iron acquisition (15). Comparative bacterial profiling of Lcn2 null mice and wild type mice further demonstrated that the lack of LCN2 protein provokes expansion of siderophore-dependent bacterial species, resulting in significant changes in the intestinal microbiome composition and increased inflammatory activity in the gastrointestinal tract (16). Importantly, mice lacking Lcn2 displayed a persistent colonization with segmented filamentous bacteria in the ileum that were associated with signs of autolysis in apical villi and detachment of epithelial cells from the basal membrane. Moreover, we have previously speculated that the expression of LCN2 is an intrinsic “help-me” sensor that, upon injury, develops a biological activity promoting production of neutrophil-attracting chemokines (17). Since neutrophils are regarded as the first line of defense in the innate arm of the immune system, loss of LCN2 would consequently prevent the effective clearance of respective microorganisms.
Interestingly, LCN2 also plays a physiological role in reproductive biology in both sexes. Originally termed as uterocalin, LCN2 is involved in the tissue remodelling processes in the uterus during pregnancy (18). Additionally, the expression of Lcn2 varies according to hormonal fluctuations in the different estrous stages of mice (19, 20). Berger and coworkers reported that Lcn2 null females have a significantly lower pregnancy rate compared with wild type animals (15). Investigating the underlying cause, the authors found that LCN2 is an essential component in modulating the membrane properties of the sperm in the fertilization process in male mice (21). Furthermore, in mouse models, infertility of both male and female animals was shown to be associated with increased expression of LCN2 in testis and ovaries (20, 22). However, to date, the molecular pathways of LCN2 and a possible interaction with its receptor in the reproductive tract are poorly understood.
LCN2 also has critical functions in the regulation of various aspects of energy metabolism and expenditure (23). LCN2 modulates expression of genes involved in β-oxidation in adipocytes, promotes β-cell function, and counteracts obesity-induced glucose intolerance suggesting LCN2 as an endogenous compensatory signal to counteract metabolic dysregulation in obesity through its anorexigenic activity (23, 24). In the central nervous system, LCN2 is a pronounced direct appetite suppressor and satiety signal in humans and mice modulating caloric intake as well as fat and lean mass content (25, 26). In mice, LCN2 impacts mitochondrial and peroxisomal function and integrity that directly impacts hepatic triglyceride balance, oxidative stress, and apoptosis (27). Finally, LCN2 was shown to be a key modulator of hepatic lipid homeostasis that in vitro and in vivo controls the formation of intracellular lipid droplets by regulating expression of Perilipin 5 that maintains the balance between lipogenesis and lipolysis (28). All these findings suggest that LCN2, besides its immunomodulatory functions, has strong impact on many aspects in shaping the energy metabolism.
Unfortunately, all these insights into LCN2 function in innate immunity, iron homeostasis, and energy metabolism and expenditure are still descriptive. Precise mechanisms how LCN2 signals are transferred from outside into the cells are widely unknown and, although some biochemical activities and affinities were nicely demonstrated, there a numerous questions unresolved yet. In particular, the precise receptor activities and responsible pathways relevant in transmitting LCN2 inputs to intercellular targets are unknown. Actually, there are six putative LCN2 receptors, namely neutrophil gelatinase-associated lipocalin receptor (NGALR), low density lipoprotein-related protein 2 (LRP2), LRP6, melanocortin 4 receptor (MC4R), MC1R, and MC3R. The size and extent of their external ligand-binding domain, hydrophobic membrane-spanning regions, and their intracellular domains inside the cell are markedly different (Figure 2). Moreover, they are assigned to different chromosomes, reveal a marked different gene structure, and encode for mRNAs and proteins different in size (Table 1).
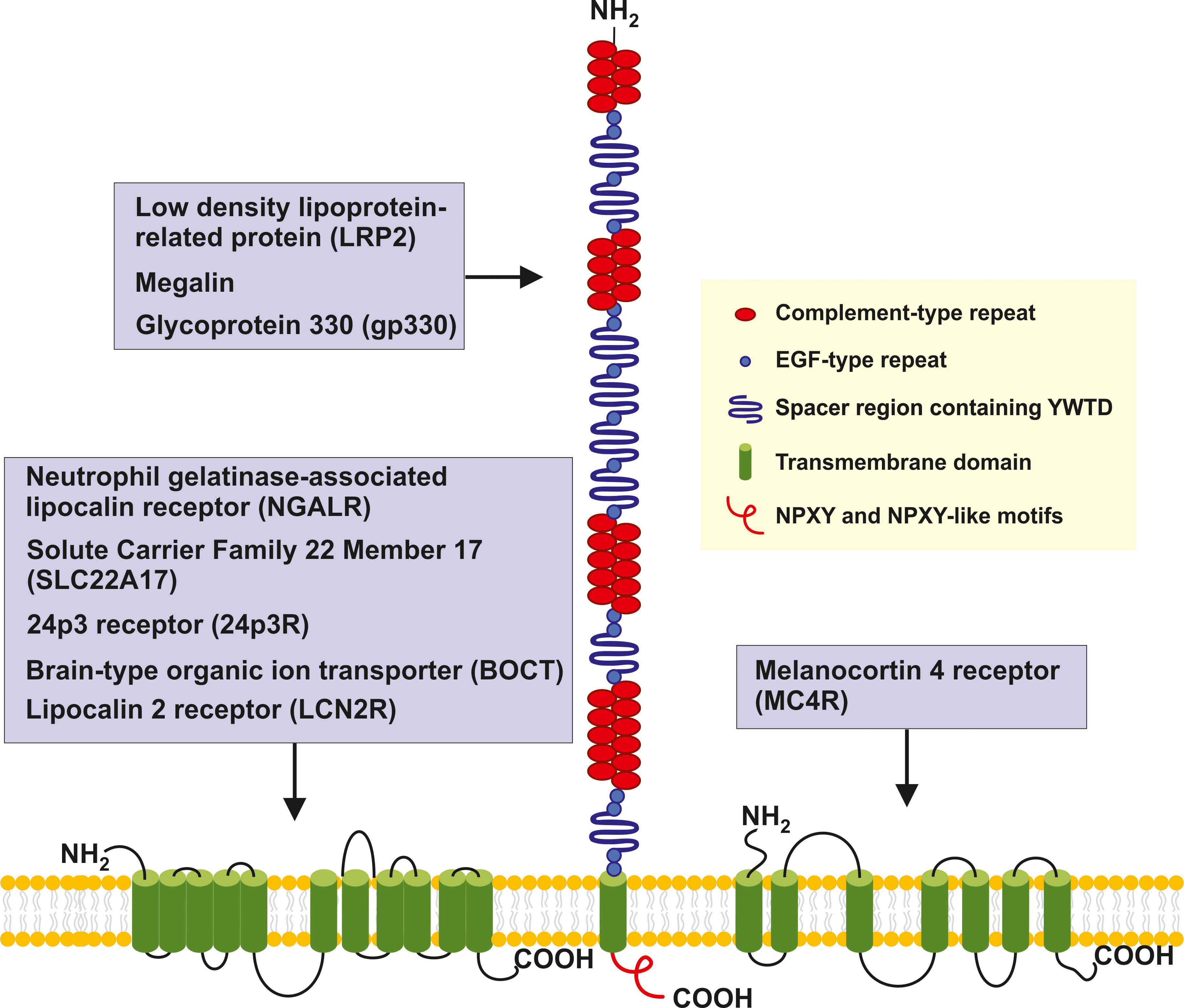
Figure 2 Structure and potential signaling pathways targeted by the putative LCN2 receptors. The three putative receptors for LCN2 are structurally different. NGALR also known as SLC22A17, 24p3, BOCT or LCN2R is a multipass 60-kDa integral membrane protein predicted to contain 11-12 transmembrane helices that are linked by extracellular and intercellular spacers of variable sizes (13, 29, 30). LRP2/megalin/gp330 is a ~4,600 amino-acid type 1 transmembrane receptor of the LDL receptor gene family characterized by extracellular domains containing four cysteine-rich clusters of complement-type repeats (i.e., the low-density lipoprotein-receptor type A repeats) that mediate ligand binding that are separated and followed by 17 epidermal growth factor type repeats and eight spacer regions that contain YWTD repeats. These are termed β-propellers that are required for pH-dependent release of bound ligands in endosomes (31). The single transmembrane of LRP2 encompassing 20 amino acids is followed by a 213 amino acid cytoplasmic tail, which contains two NPXY sequences and one NPXY-like sequence in addition to several Src-homology 3 (SH3) and one Src-homolog-2 (SH2) region sites (32). MC4R is a 332 amino acid G protein-coupled receptor (GPCR) with seven transmembrane helixes connected by alternating extracellular and intercellular loops. The recent structure of the human MC4R-GS signaling complex bound to the agonist setmalanotide determined by electron microscopy demonstrated that the seven transmembrane-spanning helixes from a bundle that forms a cavity at the cytoplasmic side to accommodate the heterotrimeric Gs protein (33, 34). For simplicity the seven helixes are drawn as stand-alone transmembrane domains.
2 Neutrophil gelatinase-associated lipocalin receptor
NGALR (OMIM: 611461) is a gene that received a number of different names historically deduced from their proposed function or from its structure. The names NGALR and LCN2R were derived from the receptor’s affinity for LCN2. NGALR2 (or NgalR-2) and NGALR3 (or NgalR-3) refer to splice variants of NGALR that share overlapping biochemical characteristics (50). The name solute carrier family 22 member 17 (SLC22A17) indicates that the receptor belongs to the solute carrier (SLC) group of membrane transport proteins that commonly contain a number of hydrophobic transmembrane α-helixes. This family currently includes about 458 members that are classified into 65 families by similarities in their characteristics, homology, functions, and structures (51, 52). The SLC22 family contains about 30 distinct multi-membrane spanning proteins, 13 of which have been localized to plasma membrane (52, 53). Beside its affinity for LCN2, SLC22A17 binds to and mediates the endocytosis of filtered protein in the kidney and assists in iron uptake but does not drive the transport of other usual substrates of the SLC22 family (52). The names BOCT, BOCT1, and BOIT are abbreviations for brain-type organic ion transporter. The BOCT sequence (acc. no. NM_021551) popped up in the initial study reporting the expression cloning of murine NGALR (i.e., 24p3R) after searching the GenBank database with two identified overlapping NGALR sequences as queries (13). Finally, the introduced acronym 24p3R indicates that this receptor has affinity for murine LCN2 termed 24p3 (13, 41). 24p3R, which is now better referenced to as NGALR, was first identified as a LCN2 receptor after three rounds of expression cloning in COS-7 cells using a cDNA library prepared from murine FL5.12 cells, which are highly sensitive to LCN2/24p3-medicated apoptosis (13).
Homozygote Ngalr mutant mice are embryonic lethal (Yukio Nakamura, personal communication), suggesting that this receptor has already important activities in the developing embryo (54, 55). It is a transmembrane signaling receptor involved in insulin receptor recycling and transport of inorganic cations/anions, amino acids, and oligopeptides. Although NGALR has been shown to be involved in endocytosis, SLC22A17 is still considered as an orphan transporter for which no specific substrates or transport mechanisms have been identified (53). Nevertheless, potential functions of NGALR have been identified in various malignancies, including cancerogenesis, kidney disease, obesity, and many other diseases (53). In particular, it has been recently shown that the LCN2/24p3R axis is highly relevant in promoting nonalcoholic steatohepatitis in high-fat diet-fed Ob/Ob mice by activating hepatic stellate cells, representing the key pro-fibrogenic cell type within the liver (56).
Binding of mouse LCN2 to murine NGALR/24p3R overexpressed in human HeLa cells was first demonstrated by ligand-cell binding experiments, in which 32P-labeled 24p3 was bound to FL5.12 cells. Based on titration curves and Scatchard plot analysis, the dissociation constant of 24p3 to NGALR was determined to ~ 92 pM (41). Direct binding of apo-LCN2 to the N-terminal part containing the first 105 residues of human NGALR was further demonstrated by solution-state biomolecular nuclear magnetic resonance (NMR) in conjunction with other biophysical methods (30). However, in this study, the binding constant of both molecules as determined by isothermal titration calorimetry and microscale thermophoresis was determined to be in the low micromolar range (~7-10 µM), while the affinity of LCN2 complexed with ferric-enterobactin was even about threefold lower (30). This suggests that the N-terminus of NGALR exhibits some specificity toward apo-LCN2, while NGALR does not or only poorly bind to holo-LCN2 (30). Finally, it should be noted that the affinity determined by NMR-based techniques is about six orders of magnitude lower than that observed in the ligand-cell binding experiments. In addition, contradictory findings showed that exogenous LCN2 had no effect on iron release or uptake in NGALR-expressing HeLa cells and relevant NGALR subdomains failed to bind LCN2, questioning the overall assumption that NGALR and LCN2 form effective receptor-ligand complexes (29). This contributes to the general discussion about problems and current standards with reproducibility and rigor in conducting measuring and evaluating binding affinities with common high-throughput technologies (57). Additionally, the interaction of a ligand with its receptor in cell-ligand binding experiments is strongly dependent on the cell system used (58), suggesting that binding characteristics of ligands and potential receptors might differ between cells analyzed. Finally, biomolecule associations and affinities are further influenced by equilibration time, temperature, half-life of complex, salt concentrations, pH, amount of active (not total) protein, and many other factors (57).
3 Low density lipoprotein-related protein 2
LRP2 (OMIM: 600073) is a large multi-ligand, type-1 transmembrane receptor that was first identified in rats by screening a rat kidney λgt 11 cDNA expression library with antiserum raised against large, negatively charged antigens isolated from rat glomeruli (35). The initially isolated cDNA clone (C1B) was then used as a probe to isolated longer λgt 11 cDNA clones. Subsequently, a 15.4 kb cDNA was assembled by several rounds of screening of a random-primed rat kidney λZAP II cDNA library that contained an uninterrupted open reading frame encoding 4660 amino acids (35). LRP2 is an endocytic receptor sharing structural similarity with the LDL receptor, which in vertebrates is the main receptor for lipoprotein uptake. Ligands for LRP2 are plasma carriers transporting vitamins and steroid hormones, which upon binding, become internalized from the apical cell surface and sorted into different cellular pathways (31). LRP2 is structurally composed of a large extracellular domain consisting of (i) complement-type repeats that have affinity for different ligands, (ii) eight β-propeller folds built by six sheets composing four antiparallel β-strands and containing up to six Tyr-Trp-Thr-Asp (YWTD) or YWTD-like repeats, (iii) flanking epidermal growth factor (EGF)-like domains, (iv) a short single membrane-spanning region, followed by (v) a small C-terminus enriched in Asn-Pro-X-Tyr (NPXY) and NPXY-like motifs required for efficient ligand-mediated receptor internalization and biological signaling (59, 60).
As its alternative name megalin indicates, full length LRP2 with its 4665-4660 amino acids in mice and humans is a giant protein with a molecular weight of ~ 600 kDa. LRP2 can physically interact with the cellular communication network factor 2 formerly known as connective tissue growth factor (CCN2/CTGF), bone morphogenetic factor-4 (BMP-4), and sonic hedgehog signaling (SHH) molecule (60). Affinity of human iron-free LCN2 (apo-LCN2) and siderophore-bound LCN2 to human LRP2 was first demonstrated by surface plasmon resonance (SPR) analysis with matrix bound LRP2. In the respective experimental setup, the dissociation constant of human LCN2 to human LRP2 was determined to ~60 nM (42), which is quite similar to the Kd determined for the interaction of murine LCN2 and MC4R which was determined to 51.39 ± 4.78 nM (43).
It was supposed that megalin functionally blocks the passage of elevated plasma LCN2 occurring in sepsis, ischemia or nephrotoxic injury into the urine (61). In line, with this suggestion the deletion of megalin in proximal tubules of murine kidney resulted in proteinurea containing large quantities of LCN2 (61). Mutated forms, in which positively charged amino acids were mutated on the surface of LCN2 without disrupting neither the typical lipocalin fold nor the ligand-binding site of LCN2, lost their affinity for LRP2, showing that the ligand-receptor binding is majorly formed by electrostatic forces and the net positive charge displayed by LCN2 (61). LRP2 has also affinity for the retinol-binding protein (RBP), α1-microglublin (α1M), odorant-binding protein IA (OBPIA), major urinary protein 6 (MUP6), and apolipoprotein M (APOM) that all belong to the lipocalin family (62, 63). Based on these findings, it might be possible that individual domains within LRP2 act as unspecific scavengers that recognize a broad range of ligands by their three-dimensional fold such as the symmetrical β-barrel fold that is a unifying characteristic of lipocalins. Nevertheless, the binding of other lipocalins with Kd values in the µM range such as RBP (Kd = 1.8 µM) and α1M (Kd = 0.42 µM) to megalin have significantly lower affinities than that of LCN2 (Kd = 60 nM) (42, 63, 64). Moreover, the affinity of purified human or rat LRP2 to other proteins such as the lipoprotein lipase is 10 to 30 fold lower (Kd = 6.1 and 2.7 nM, respectively) than that of LCN2 (65). Nevertheless, since LRP2 is involved in the endocytosis of a wide range of molecules (LCN2, siderophore-bound iron, diverse lipoproteins, hemoglobin, albumin, hormones, toxins, drugs, vitamin-binding proteins, stress-related proteins and many others), LCN2 when overexpressed during malignancies might change the affinity for other LRP2 binding partners by blocking respective binding sides (66).
Recently, mouse LRP6 composed of the same basic structural motifs as LRP2 acting as a co-receptor for Wnt was shown to specifically bind to mouse LCN2 (49). In the respective study, the interaction of LCN2 and LRP6 was shown in co-immunoprecipitation assay and it was suggested that binding of LCN2 to LRP6 effectively inhibits Wnt/β-catenin signaling. Interestingly, LRP5 which is about 70% homologous to LRP6 and also active as a co-receptor in Wnt signaling failed to bind LCN2 (49, 67). However, information about the strength of the binding of LCN2 and LRP6 and independent confirmation of observed interaction in other species than mouse is still pending.
4 Melanocortin receptors
MC4R (OMIM: 155541) belongs to a family of G protein-coupled 7-transmembrane receptors that contains five family members (termed MC1R to MC5R). The individual members of that family share a sequence homology of 38 to 60% (68). Each receptor has different specificity for melanocortins that consists of adrenocorticotropin hormone (ACTH), α-melanocyte-stimulating hormone (α-MSH), β-MSH, and γ-MSH (68). Receptor activation results in formation of cyclic AMP (cAMP) and activation of protein kinase C (PKC) that in turn provokes influx of extracellular calcium resulting in inositol triphosphate (IP3) production and downstream activation of MAPK and JAK-STAT pathways (68).
In regard to LCN2, it was further demonstrated that osteoblast-derived LCN2 can cross the blood-brain-barrier and bind to MCR4 in paraventricular nucleus neurons of the hypothalamus as assessed by application of biotinylated Lcn2 in Lcn2 null mice (43). In addition, the overexpression of MC4R in HEK293T cells dose-dependently stimulated cAMP activity with an EC50 of 1.41 ± 0.25 nM, while silencing or pharmacological inhibition of MC4R with the MC3R/MC4R antagonist SHU9119 abrogated Lcn2-activated cAMP production and signaling in the mouse hypothalamic, gonadotrophin-releasing hormone neuronal cell line GT1-7. This inhibition was independent from the two other putative Lcn2 receptors (i.e. MC1R and MC3R) because LRP2 is not expressed in GT1-7 cells and silencing of NGALR did not affected LCN2 signaling (43). Saturation and competition binding experiments in MCR4 transfected HEK293T showed that LCN2 binds to MC4R with a dissociation constant Kd of 51.39 ± 4.78 nM. This affinity is about 4.5-fold lower than that of the classical MCR ligand α-MSH.
In the same study, the authors showed that LCN2 also has affinity for MC1R (Kd = 86.96 ± 9.72 nM) and MCR3 (Kd = 82.13 ± 12.14 nM) and can stimulate cAMP production through these receptors. However, the authors suggested that MC4R is the most relevant MCR for LCN2 signaling in paraventricular and ventromedial neurons because MC1R and MC3R are not able to regulate appetite (43). Similar to MC4R, MC3R is a neural MCR that is expressed primarily in the central nervous system having important functions in regulating feed efficiencies by binding to identical ligands with similar binding affinities (69). The third putative MC receptor for LCN2, MC1R, is expressed in neurons, astrocytes, and microglia and also sensitive to the endogenous nonselective agonist α-MSH (70). The activation of MC1R can prevent neutrophil infiltration, thereby reducing the overall LCN2 content that is available within the brain (71). Furthermore, because LCN2 participates in the pathogenesis of blood-brain barrier disruption, MC1R with its lower affinity might be a kind of LCN2 sensor capable to prevent overshooting LCN2 concentrations within the brain by blocking neutrophil infiltration (72).
In addition to its activity in control of appetite, MC4R can directly impact glucose and lipid homeostasis, pain perception and even sexual behaviors by modulating of luteinizing hormone and prolactin surges in female and erectile activity in male rodents (73, 74). However, if these MC4R-driven activities can be modulated by LCN2 is not known yet.
5 Signaling pathways for putative LCN2 receptors
As discussed, there are six putative receptors supposed to have (more or less affinity) for LCN2. The receptors either belong to the group of transmembrane transporter/signaling receptors (NGALR), multi-ligand receptors (LRP2, LRP6), or G-protein coupled seven transmembrane receptors (MC4R, MC1R, MC3R). If these putative receptors are indeed bona fide LCN2 receptors, LCN2 would have many possibilities to modulate cellular signaling via binding to one of these receptors or by binding combinations of these receptors (Figure 3).
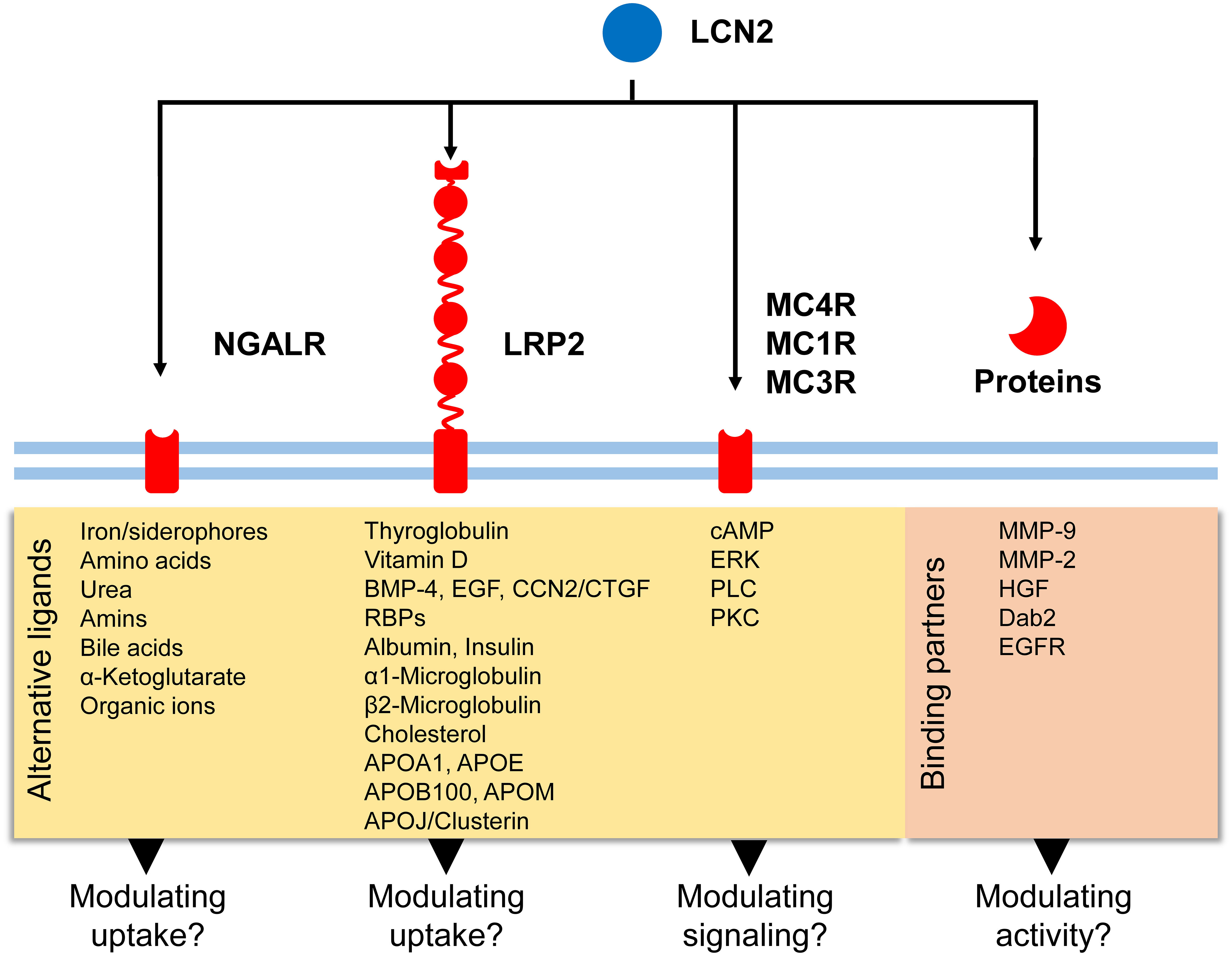
Figure 3 Potential signaling routes for LCN2. LCN2 has affinity for five putative receptors (NGALR, LRP2, MC4R, MC1R, and MC3R) and additional binding partners (e.g., MMP-9, MMP-2, HGF). Binding of LCN2 to these biomolecules can modulate activity of respective proteins/receptors or modulate signaling cascades triggered. In addition to these receptors, LCN2 was recently found to bind to LRP6 in mouse embryonic fibroblasts (49).
NGALR belongs to the SLC superfamily of proteins that can transport a wide array of molecules, including sugars, amino acids, oligopeptides, vitamins, nucleotides, metals, inorganic ions, organic anions, and drugs (52). Within the SLC group, NGALR belongs to the major facilitator superfamily (MFS) group that in particular transport organic ions including urea, amines, bile acids, and α-ketoglutarate (52). Importantly, NGALR assists in the uptake of iron and shows a more restricted substrate profile than other SLC members, suggesting that NGALR has more specialized physiological functions (75). It was suggested that NGALR contributes to adaptive osmotolerance by controlling the uptake of amino acids as osmolytes and that LCN2 downregulation counteracts increased proliferation and permanent damage of osmotically stressed cells (76). Similarly, it was proposed that murine LCN2 alters cellular iron trafficking through iron efflux via murine NGALR, thereby driving apoptosis (41). In line with this assumption, exogenous LCN2 sensitized primary cortical neuronal cells and neuroblastoma cell lines to cell death (77). Likewise, the addition of LCN2 increased intracellular reactive oxygen species formation, DNA damage, cell cycle arrest and cell death in mouse fibroblast cell line L929 (78). Additionally, in both acute and chronic white matter diseases in the brain, it could be shown that LCN2 has an inhibitory effect on the oligodendrocyte differentiation necessary for remyelination, which was mediated via SCL22A17/EGR1 signaling (79).
We showed that the lack of LCN2 is associated with endoplasmic stress, unfolded protein response, and modulation of the cellular labile chelatable iron pool (27, 80). However, there are also findings showing that LCN2 has protective and survival activity (9, 81), while other studies have shown that exogenously added LCN2 in HeLa cells that overexpress NGALR did not affect iron efflux or uptake (29). Similarly, there are numerous other studies that show conflicting data in the understanding of LCN2’ function in controlling apoptosis.
LRP2 is a multi-ligand binding receptor that has important roles during embryogenesis by supplying the fetus with nutrients and controlling sonic hedgehog signaling (31). It further serves as a receptor for thyroglobulin (82) and Vitamin D (83), has affinity for BMP-4 (83), forms specific complexes with cubulin and Dab2 that mediate the uptake of filtered proteins (e.g., vitamin-binding proteins, retinol-binding proteins, albumin, insulin, α1-microglobulin, β2-microglobulin, EGF, and lysozyme) and escape the glomerular filtration barrier and driving the endocytic uptake of clathrin-coated vesicles (84). It further has affinity for several ligands involved in cholesterol metabolism (e.g., APOA1, APOE, APOB100, APOJ/clusterin, and APOM) and is important for the endocytosis of high density lipoprotein particles (83). So there is large variety of activities that can be modulated by interacting of LCN2 with specific modules within LRP2.
As discussed, the MCRs (i.e., MC4R, MC1R, and MC3R) supposed to have affinity for LCN2 are belonging to the group of 7-transmembrane G-protein linked receptors. From these receptors MC4R has the highest affinity for LCN2. Its activation leads to increased intracellular cAMP (36, 39). Moreover, more recent studies have shown that MC4R is able to activate the extracellular regulated kinase (ERK) and modulate the activity of other G proteins receptors, most likely by sharing affinity for the same G-proteins that mediate signaling via these receptors (85, 86). This is in line with previous findings showing that the G protein Gsα is required for controlling MC4R-regulated energy expenditure and glucose metabolisms, while effects on food intake, linear growth, cholesterol metabolism, and expression of specific transcription factors in the paraventricular nucleus of the hypothalamus (i.e., Sim1, Crh) are primarily mediated by the Gq/11α subunit (87, 88). In addition to Gsα and Gq/11α, MC4R can also couple to other G proteins such as Gαi, which inhibits the adenylate cyclase activity, thereby decreasing intracellular cAMP levels, and Gαq, which activates phospholipase C (PLC) and downstream PKC (89). Presently, it is unknown if LCN2 can modulate the affinity of MC4R for G proteins. Nevertheless, LCN2 was shown to increase the formation of cAMP as efficiently as 0.5 nM α-MSH in mouse GT1-7 hypothalamic cells, but failed to activate phosphorylation of AMPK, ERK1, ERK2, or tyrosine kinase in these cells, further suggesting that cAMP formation and kinase phosphorylation might not be necessarily linked to each other (43).
So in sum, LCN2 seems to have many possibilities to interfere with cellular signaling through its putative receptors. This notion is underpinned by the finding that the overexpression of LCN2 in the esophageal squamous cell carcinoma cell line EC109 resulted in the upregulation of 167 genes and downregulation of 96 genes using a 2-fold threshold encoding genes that have further capacity to interact with thousands of other proteins (90). This study that was based on protein-protein interaction network showed that LCN2 is a highly pleiotropic protein having affinity for many other proteins, thereby causing a wide range of expression alterations in immunity-related terms, pathway-related terms, cellular response to molecules of bacterial origin, extracellular matrix organization, and cell cycle-related terms. However, there is an urgent need to clarify if these effects of LCN2 are mediated by acting as a signal-inducing ligand at a specific receptor, masking binding epitopes for other proteins on proposed LCN2 receptors, binding to other biomolecules (e.g., proteins, hormones, vitamins etc.) preventing their interaction with other proteins/receptors, or alternatively by directly or indirectly changing general cellular features (osmolarity, iron content, mitochondrial activity). It should be noted that the outcome of the induced biological effect is the sum of the different interaction levels with all receptors expressed at a specific time in a specific environment and the accessibility of possible target structures at the time of LCN2’ presence. This should results in an extreme high combinatorial diversity of LCN2 activities.
6 Unsolved issues in LCN2 receptor signaling
6.1 Experimental aspects
NGALR is expressed in many organs and tissues (13), while the expression of the neuronal MC4R receptor as well as MC1R and MC3R are more restricted and found primarily in the brain (36, 37, 39). The expression of LRP2 is high in kidney, less in lung, and low in liver, brain, heart, and skeletal muscle (35). In line with the proposed expression patterns, MC4R protein is not detectable in kidney, while NGALR protein is detectable and LRP2 protein is found in high abundance within the kidney (Figure 4). Nevertheless, based on our own experience and discussion with many colleagues, it should be mentioned that the uncritical usage of antibodies directed against the putative LCN2 receptors can lead to significant errors. Some examples of discrepant and conflicting experimental results for staining with putative LCN2 receptor antibodies are given in Figure 5, depicting data taken from the open access Human Protein Atlas resource, which uses only “validated antibodies” that have undergone a strict quality control process with defined criteria (Human Protein Atlas).
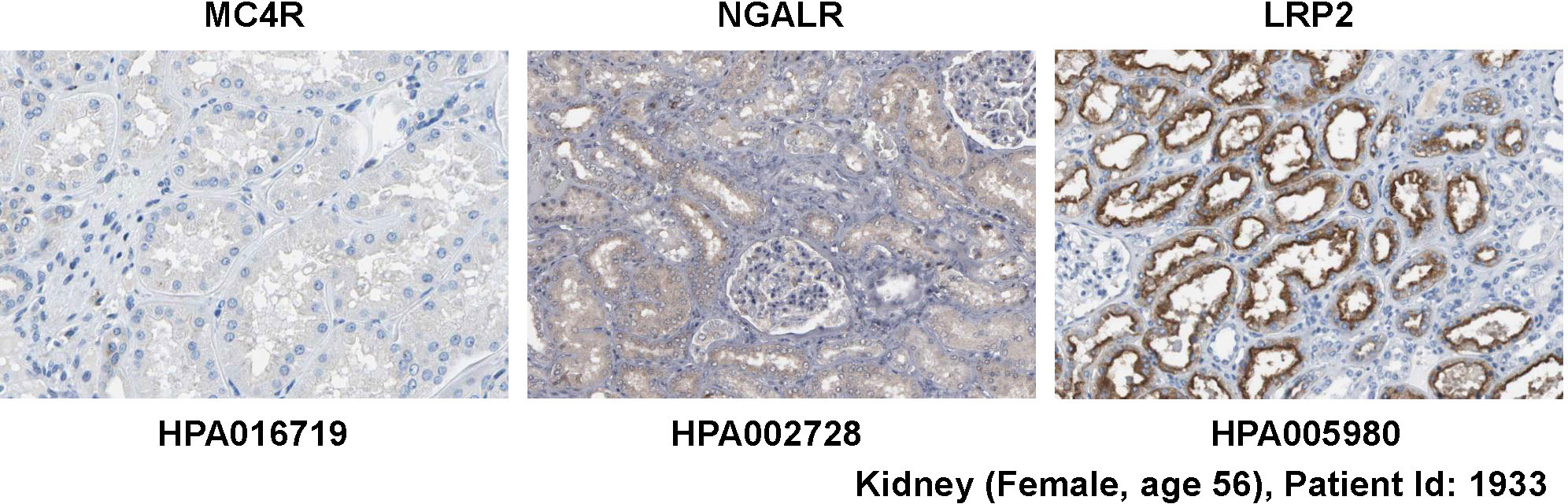
Figure 4 Expression of potential LCN2 receptors in kidney. Normal kidney tissue sections were stained with antibodies specific for MC4R, NGALR, and LRP2 showing that the kidney lacks MC4R expression. All images were taken from the Human Protein Atlas (91) database [https://www.proteinatlas.org/92]. The images can be found at: https://www.proteinatlas.org/ENSG00000166603-MC4R/tissue/kidney#img (left), https://www.proteinatlas.org/ENSG00000092096-SLC22A17/tissue/kidney#img (middle), and https://www.proteinatlas.org/ENSG00000081479-LRP2/tissue/kidney#img (right).
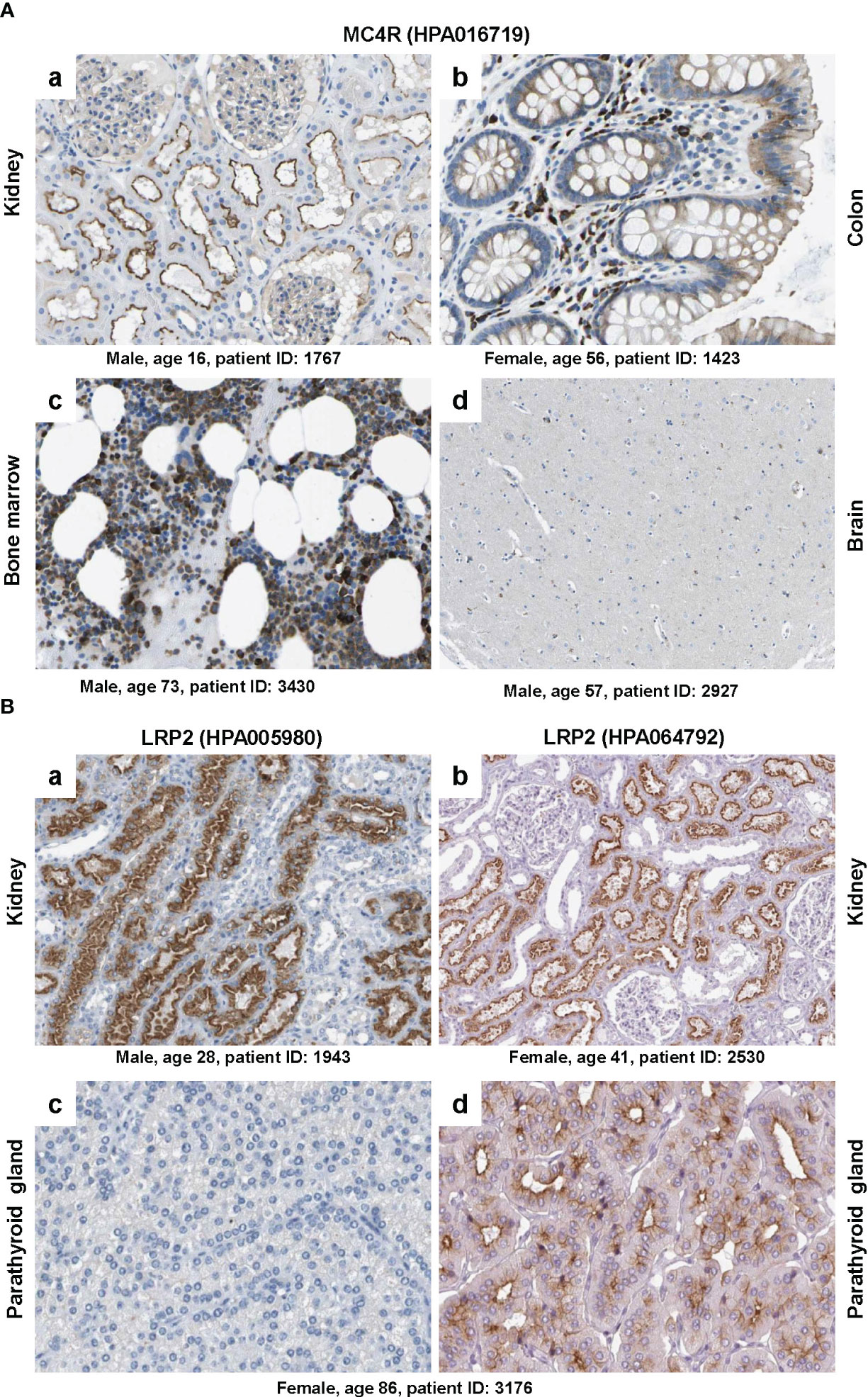
Figure 5 Immunohistochemical staining of MC4R and LRP2/megalin. (A) Tissue sections from (a) kidney, (b) colon, (c) bone marrow and (d) brain were stained with a validated antibody (HPA016719) specific for MC4R. Please note that the antibody stained tubular cells in the kidney, hematopoietic cells in the bone marrow, glandular cells in the colon, while only showing low staining in some neuronal cells in the brain. (B) Tissue sections from (a, b) kidney and (c, d) parathyroid gland were stained with two (validated) polyclonal rabbit antibodies (i.e., HPA005980 and HPA064792) directed against human LRP2. Please note that both antibodies stained similar structures in kidney, while the staining was markedly different in two sections of the parathyroid gland that were taken from the same patient. All images were taken from the Human Protein Atlas (91) database [https://www.proteinatlas.org/92]. The images depicted in (A) can be found at: (a) https://www.proteinatlas.org/ENSG00000166603-MC4R/tissue/kidney#img, (b) https://www.proteinatlas.org/ENSG00000166603-MC4R/tissue/colon#img, (c), https://www.proteinatlas.org/ENSG00000166603-MC4R/tissue/bone+marrow#img, and (d) https://www.proteinatlas.org/ENSG00000166603-MC4R/tissue/cerebral+cortex#img. The images depicted in (B) can be found at: (a, b) https://www.proteinatlas.org/ENSG00000081479-LRP2/tissue/kidney#img and (c, d) https://www.proteinatlas.org/ENSG00000081479-LRP2/tissue/parathyroid+gland#img.
6.1.1 Example 1: Cross-reactivity of antibodies directed against specific LCN2 receptors
The polyclonal rabbit affinity isolated antibody HPA016719 directed against a recombinant human MC4R peptide produced strong signals on sections taken from kidney, colon and bone marrow, while only some neural cells in the brain were positively stained for MC4R (Figure 5A). However, MC4R as a neuronal receptor should be expressed abundantly in caudal brainstem structures (73) but not in kidney, colon or bone marrow. Because HPA016719 was produced against a 44 amino acid protein epitope signature tag (PrEST) located at the N-terminal part of human MC4R (aa-9-aa52; NH2-MVNS……YEQL-COOH), one could mistakenly conclude from the observed staining pattern that parts of MC4R containing the N-terminal region of MC4R are released from the brain and taken up or bound by cells located in the kidney, colon, and bone marrow that do not themselves express MC4R. But how likely is that?
6.1.2 Example 2: Failure of an antibody to bind to its antigen
Another striking example is depicted in Figure 5B showing that staining with two polyclonal rabbit affinity isolated antibodies (HPA005980 and HPA064792), both directed against human LRP2, resulted in highly consistent results in kidney, while the parathyroid gland, known to express large quantities of LRP2 (93, 94), was only significant stained with antibody HPA064792. This finding is extremely surprising because the two antibodies were tested on sections taken from the same patient (patient ID: 3176). Of course this discrepancy might result from the fact that both antibodies were prepared against different recombinant protein fragments of human LRP2. Antibody HPA005980 was produced against PrEST consisting of aa1333 to aa1482 of human LRP2 (NH2-GFT…….STDL-COOH) and antibody HPA064792 against a PrEST spanning aa4240 to aa4331 (NH2-NND……….SVP-COOH) of human LRP2. Therefore, one could argue that the parathyroid gland expresses a shortened, N-truncated LRP2 isoform that is not recognized by antibody HP005980. But again, how likely is that assumption?
These examples are highly alarming and there are many other examples showing that uncritical usage of antibodies directed against LCN2 receptors might be a source for faulty data. Unfortunately, companies selling these antibodies propose “The antibodies that have been generated … have been tested by immunohistochemistry against hundreds of normal and disease tissues” and “The uniqueness and low cross-reactivity of the antibodies to other proteins are due to a thorough selection of antigen regions, affinity purification, and stringent selection”. It is obvious that such statements give false certainty about the quality of respective antibodies.
Moreover, there are many antibodies available for NGALR, LRP2 and MC4R that have not undergone strict quality controls. Some of them are even advertised to be specific for several species and applicable for immunohistochemistry, Western blot analysis, flow cytometry, and ELISA testing. Nevertheless, the ones that we have tested in our laboratories even failed to recognize high quantities of transiently expressed proteins in cell extracts or produced unspecific, un-interpretable background signals.
6.2 Functional aspects
There are also many functional aspects in LCN2 receptor biology that are controversially discussed or unexplained. In the following, two examples are briefly discussed to clarify what is meant.
6.2.1 Example 1: Specificity of LCN2 activities
Numerous studies have shown that the expression of LCN2 is a crucial sensor that indicates inflammation, cell damage, stress and many other factors. As such, LCN2 is an important factor contributing to the cellular microenvironment and has pivotal vial roles in cell-cell communication. Nevertheless, there are many different cell types and organs that can produce and secrete elevated quantities of LCN2 during injury into the systemic circulation. Consequently, it is questionable how overexpressed LCN2 can mediate specific effects in a specific organ or tissue. Exemplarily, the expression of LCN2 is significantly increased in several renal cell types during acute and chronic kidney injury (Figure 6). Consequently, this causes increased LCN2 quantities in the blood. Based on the fact that LCN2 can pass the blood-brain barrier (25), kidney insult should be accompanied with increased cerebral quantities of LCN2 and subsequent activation of MC4R, thereby modulating food intake and energy expenditure. However, it is already known for decades that the energy expenditure in patients with renal failure is not different from normal (95). Similarly, patients suffering from diverse liver insults have increased concentrations of LCN2 (17). Consequently, elevated concentrations of LCN2 occurring during fatty liver disease induced by elevated energy intake should limit progression of hepatic disease itself through MC4R-dependent suppression of appetite. However, quite the opposite is the case.
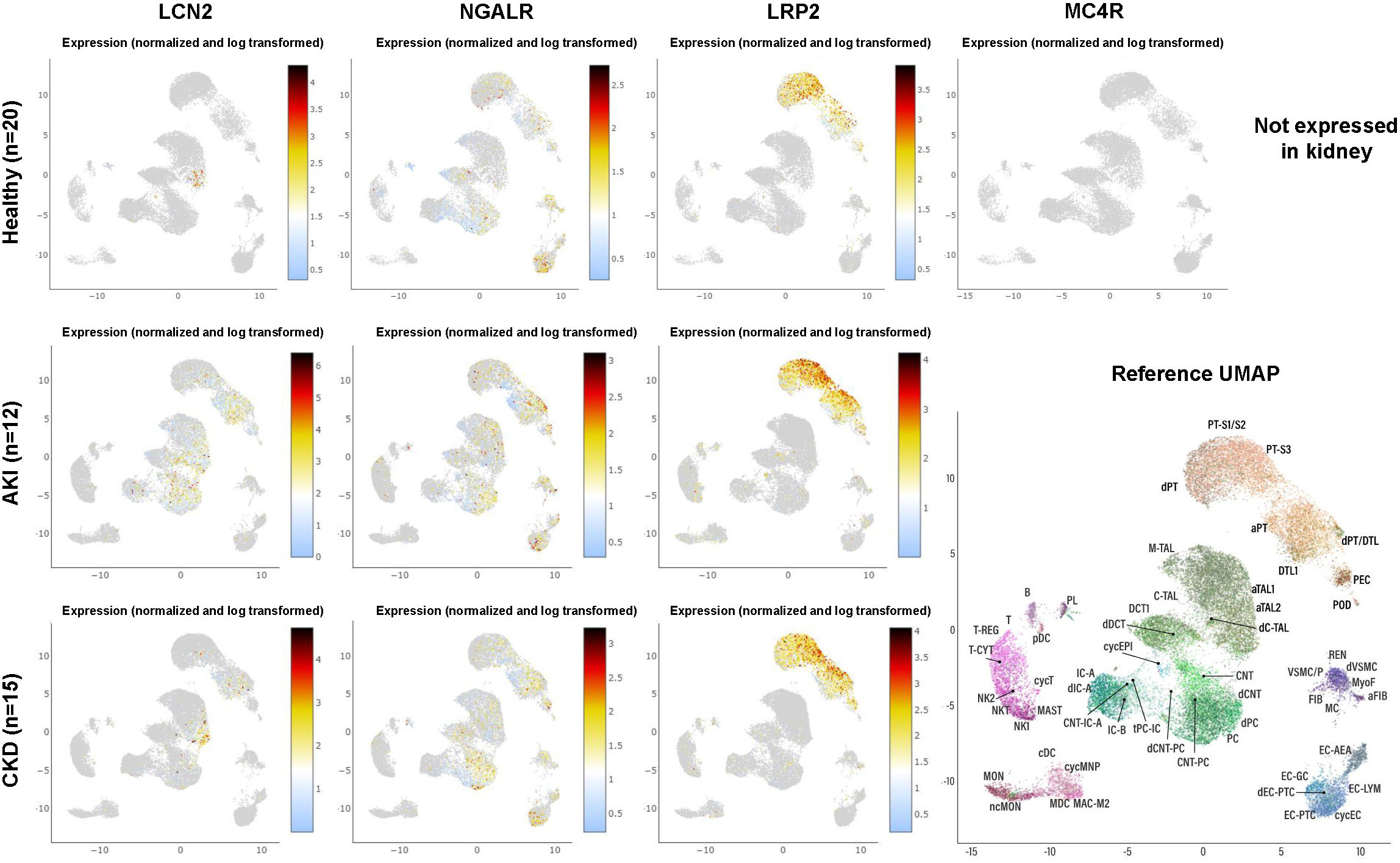
Figure 6 Expression of LCN2 and its putative receptors in human kidney in health and disease as obtained from single-cell RNA-sequencing. The dataset comprises 20 samples from 18 living donor biopsy participants taken from the Human Cell Atlas, as well as 15 biopsies from patients suffering from chronic kidney disease and 12 biopsies taken from patients suffering from acute kidney injury. For individual abbreviations depicted in the two-dimensional Reference Uniform Manifold Approximation and Projection (UMAP) image, please refer to Supplementary Table 1. Please note that the cell subsets that are capable to express LCN2 increase during renal disease, while the expression profile of the putative LCN2 receptors NGALR and LRP2 does not change. Moreover, renal expression of LRP2 is more restricted and MC4R is not expressed at all in any kidney cell fraction. The results here are in whole or part based upon data generated by KPMP [https://atlas.kpmp.org]. The respective URL address used for visualizing expression data from LCN2, NGALR, LRP2, and MC4R in healthy kidney, acute kidney injury and chronic kidney disease is: https://atlas.kpmp.org/explorer/dataviz. All data were downloaded on 20 May, 2023.
6.2.2 Example 2: Function of LCN2 in the pathogenesis of insulin resistance
In mice, the disruption of Lcn2 resulted in insulin resistance, which significantly potentiated diet-induced obesity and fatty liver disease (96). However, this finding is in contrast to other studies showing (i) that LCN2 deficiency in mice protects against developing aging- and obesity-induced insulin resistance (97), (ii) elevated quantities LCN2 contribute to insulin resistance in humans (98), and (iii) increased LCN2 expression acts as a protective mechanism to counteract obesity-induced glucose intolerance by decreasing food intake and promoting adaptive β-cell proliferation (23). These controversial findings are hardly to explain. It becomes even more complicated if the observed effects should be mediated by specific receptors. LRP2, for example, acts as an endocytic receptor for reabsorption of insulin (99) and shows increased expression in early type 2 diabetes (100). Contrarily, MC4R deficiency in humans and rodents induce insulin resistance and obesity but do not develop hyperglycaemia (101), which is in line with the finding that MC4R agonists improve insulin secretion in diabetic mice (102). However, since NGALR is supposed to have the highest affinity for LCN2 from all putative receptors, this gap of knowledge is incomprehensible. In sum, it is obvious that additional work is required to understand LCN2’s roles in the pathogenesis/prevention of insulin resistance.
6.3 Lack or wrong information about antibodies
Additional confusion results when authors miss to provide detailed product information about the antibodies used in their study. In order to be able to reproduce a published experiments, it is of fundamental importance to have information about the full antibody name, clone number (if monoclonal), manufacturer, catalogue number, lot number, working dilution, detected epitope, and strategies or references demonstrating antibody validation. Unfortunately, only a small percentage of papers in the LCN2 receptor field provide respective information. Even more, missing proper controls, nonspecific binding, or using wrong antibodies for a specific antigen provoke erroneous and inconsistent results. Two examples taken from the LCN2 receptor field should be briefly discussed.
6.3.1 Example 1
In a study from year 2020, Chen et al. (103) investigated the association of hepatic and systemic LCN2 levels in liver fibrosis, portal hypertension, and disease severity in patients with alcoholic hepatitis and in mouse subjected to ethanol or carbon tetrachloride treatments (103). In agreement with many previous studies, the authors found that LCN2 serum levels and gene expression correlated well with disease severity, liver fibrosis, and portal hypertension. Moreover, when comparing wild type and Lcn2 null mice, the authors of that study showed that mice lacking Lcn2 developed less fibrosis when subjected to carbon tetrachloride. They further showed an immunofluorescence staining for NGALR in liver specimen from patients suffering from alcohol hepatitis showing a strong upregulation of NGALR compared to healthy controls. When looking for the identity and source of antibody used in this study, the authors provide information that the NGALR antibody was from Sigma-Aldrich (#HPA049718). However, looking at the datasheet of mentioned antibody, the antibody is produced in rabbit and directed against the atypical chemokine receptor 3 (ACKR3), also known as CXC motif receptor 7 (CXCR7) or G protein-coupled receptor 159 (GPR159). Unfortunately, the respective antibody used in this study has nothing to do with NGALR, questioning the finding that NGALR is increased expression in human livers during alcohol induced liver damage.
6.3.2 Example 2
Du and colleagues reported effects of LCN2 on brain endothelial adhesion and permeability (104). In their study, they used human brain microvascular endothelial cells (HBMEC) and showed an immunohistochemistry for NGALR and LRP2. Based on the results, the authors stated that the results confirmed that both receptors are expressed in HBMEC. Unfortunately, the authors show no proper controls, they only stated in the Material and methods sections “Negative controls were incubated without primary antibodies and no immunoreactivity was observed in these controls”. In our view, the chosen control is insufficient. A typical falsity in immunohistochemistry is the use of negative controls in which the primary antibody is omitted. Results of such studies show only the potential non-specific binding of the secondary antibody on the tissue, but not that of the primary antibody. The use of serum or isotype-specific immunoglobulins in the same protein concentration as the primary antibody is suitable as a negative control for staining (105).
In addition, the chosen primary antibodies used for NGALR or LRP2 might recognize other proteins. In regard to the source of antibody the authors provide the information that the LRP2 antibody was obtained from Abcam (Cambridge, MA) and the antibody against 24p3R was obtained from Bioss (Woburn, MA). Abcam presently offers seven different unconjugated antibodies against LRP2 (#ab76969, #ab309086, #ab309087, #ab236244, #ab223754, #ab101011, and #ab56014) from which six are recommend for immunohistochemistry in human materials. Bioss sells only one unconjugated polyclonal antibody against 24p3R/SLC22A17 (bs-044R) with reactivity against human NGALR (and rat). So it is obvious that the authors of respective study used this NGALR antibody for staining, which is also likely because the paper is listed on the companies’ homepage as one reference for this antibody. But which antibody was used for LRP2 staining and how was the specificity of both antibodies tested in the respective study?
In our laboratories, we have tested several antibodies against LRP2 and NGALR. Unfortunately, most of these antibodies showed unspecific background signals in cells and tissues. For example, a rabbit polyclonal LRP2 antibody (Elabscience, #E-AB-63748) showed a nuclear and cytoplasmic staining pattern in murine brain-derived endothelial cell line bEnd3, while a typical membranous staining supposed for a receptor was missing (not shown). Therefore, we would never argue that the staining is specific for LRP2 because some nuclei stained positive and more important bEnd3 are known to express large quantities of LRP1 (106), which shares an overall sequence identity of 38.40% with murine LRP2 as determined by blastp suite-2 alignment between respective protein sequences (i.e., sequence CLUSTW (mLRP1: EDL24513.1, mLRP2: NP_001074557.1). In addition, this antibody was produced against a recombinant fusion protein of human LRP2 for which the company does not provide further information (e.g., precise epitope) in respective datasheet. Based on the occurrence of individual modules (e.g., complement-type repeats, eight β-propeller folds, EGF-like domains, NPXY motifs) within LRP2 that are also found in similar structure and arrangement in many other proteins, it would be scientifically unaccountable to claim that the stain is specific for LRP2.
Similarly, staining with a polyclonal rabbit antibody directed against a peptide located in the C-terminal region of human NGALR (Invitrogen, #PA5-103667) produced in our hands only a faint stain in murine kidney and liver tissue that was only marginal stronger than the staining with an unspecific IgG control (not shown). NGALR in mouse kidneys should be highly expressed in apical membranes of distinct distal tubular segments (i.e., the distal convoluted tubule) in renal cortex and medulla as demonstrated already one decade ago by staining of tissues with a highly specific antibody (α-CT-24p3R) directed against the peptide GALPPNASGWEQPPNSC (the first 16 amino acids of that peptide correspond to amino acid 18 to 33 of murine NGALR) (107). Of course, we could argue that distal convoluted tubules are stained over background, but based on the overall low signal is this really scientifically robust? On the contrary, NGALR expression with this antibody was detected within the seminiferous tubules in murine testis. This expression seems to be specific because we could confirm the expression of NGALR by RT-PCR and subsequent sequencing of the resulting amplicon (not shown). This would be comparable to the staining results in rat testis, where NGALR was observed in the germ cells (localized in the seminiferous tubules) (108). Unfortunately, it was not further specified which antibody (from abcam) was used. However, usage of another NGALR antibody (LS-C53320, LS Bio) that was produced using a 14 amino acid synthetic peptide taken from the last 50 amino acids from the C-terminus of human NGALR and proposed to be cross-reactive for murine NGALR (Comment by the company: test or 100% immunogen sequence identity) failed to recognize murine NGALR with the expected size of ~ 58 kDa in Western blot analysis, while showing endogenous NGALR in Hep3B and HEK293 cells and transient overexpressed NGALR (not shown). However, in addition to this band, there is one (in HEK293) or two (in Hep3B) other protein band detectable in the higher molecular size range. So, could these bands be ignored, are these artifacts arising from inadequate protein reduction, or are these cross-reactive band to other sequence related proteins?
Moreover, when performing direct immunofluorescence analysis with another rabbit polyclonal Alexa Fluor® 488-labeled antibody directed against a KLH-conjugated synthetic peptide derived from human SLC22A17 that should be cross-reactive to rat and mouse NGLAR (Bioss, #bs-0444R-A488), all weak signals for NGALR were found in kidney, liver, and testis (not shown). So what is specific and what not?
In sum, there are many experimental findings that are not easily to explain. In our laboratory, we have started to critically evaluate LCN2 receptor antibodies. We will be happy to discuss and share our experience on that topic upon reasonable request.
6.4 From mouse to men?
Work in mice has provided tremendous insight into the biology of LCN2. In particular, as research tools, mice studies have shown that Lcn2 expression is significantly increased in many organs during stressful conditions and inflammation. Moreover, the finding that LCN2 binds siderophores has unraveled LCN2 as a critical component of the innate immune systems. Similarly, the identification of different receptors that have affinity to LCN2 has shield light on potential pathways by which LCN2 transmits signals into the cells. However, it is worth considering the possibility that findings obtained in mice may not precisely occur in the same way as in humans (109). In particular, there has been an intensive discussion in the past decade if mouse models mimic human inflammatory diseases (110, 111). Systematic studies investigating the animal-to-human translational success rates have shown that the translational success is unpredictable (112). Therefore, the question arises how valuable are all these mouse findings for humans and which findings can be translated to the pathophysiology of human diseases? In this regard, the low percent identity of human and mouse LCN2 that is only 62% has to be considered (Table 2). Compared to their putative receptors sharing up to 94% sequence homology, the two LCN2 orthologs in mouse and men are rather different. Despite the common assumption that orthologs usually share the same biological functions, there have been several reports of divergence between orthologs from mice and humans (114). There are some important examples showing that LCN2 and LCN2/p24p3 as one-to-one orthologs might have dissimilar functions in regard to their biochemistry and function. Two of these examples should be briefly discussed in the following.
6.4.1 Example 1: Affinity of LCN2 to MMP-9
Previous studies have shown that human LCN2 can form tight heterodimers with human MMP-9 in human neutrophils (3, 115). Contrarily, murine LCN2 lacks the corresponding cysteine and does not associate with MMP-9 (116). MMP-9 also termed Gelatinase A has the ability to degrade extracellular matrix components (e.g., collagen, laminin, elastin, fibronection) and dysregulation of MMP-9 is associated with various diseases (117). It is associated with acute and chronic inflammatory conditions by liberation of extracellular matrix-sequestered cytokines and growth factors, enabling influx of leukocytes in the inflamed tissue, and affecting the permeability of blood brain barrier (117). The binding of human LCN2 to human MMP-9 is mediated via a disulfide bridge between free cysteine107 (C107) of human LCN2 (cf. Figure 1B) and the cysteine of the Pro-Arg-Cys-Gly-Val sequence of MMP-9 that is located in the pro-peptide domain of MMP-9 and relevant for inhibition of its catalytic site (118, 119). The binding of LCN2 provokes an enzyme-activating effect in the regulation of inflammatory and pathophysiological responses of granulocytes in the physiological activation of MMPs (118). Therefore, heterodimer formation between both proteins should have fundamental importance in the outcome of inflammatory diseases. Furthermore, the complex of LCN2 and MMP-9 plays a crucial role in the modulation of the metastatic phenotype of cancer cells that promotes cancer cell invasion and metastasis through protection of MMP-9 degradation, inducing MMP-9 activation, and increasing the overall activity of MMP-9 (120). Finally, it is most likely that the covalent binding of LCN2 and MMP-9 will change the overall affinity to putative receptors. It is hard to believe that this important function of LCN2 should be limited to the human ortholog.
6.4.2 Example 2: Posttranslational modifications
Another difference is the finding that mouse LCN2 is a PKCδ substrate and needs to be phosphorylated at T115 to be secreted from neutrophils (121). Although the surrounding residues of T115 are well-conserved between mice and humans, no reports are available demonstrating that phosphorylation at that side is necessary to mediate human LCN2 secretion from neutrophils or any other immune cell. Similarly, there are several reports demonstrating that human LCN2 is an N-glycosylated protein (122–124). However, the number of potential N-glycosylation sites is different in both species. It has been shown that bacterially expressed LCN2 has capacity to form complexes with the siderophore enterochelin and that N-glycosylation is not required for secretion of LCN2 into exosomes suggesting that this posttranslational modification might not be relevant for the activity of LCN2 (61, 124). However, it might be possible that glycosylation alters the affinity for receptors or binding partners or stability of this lipocalin. In mice it has been shown that the in vivo N-glycosylation pattern of LCN2 impacts the distribution within murine tissues (125). It is further well accepted that the structure of N-glycan is involved in the apical sorting of proteins in epithelial cells and that loss of N-glycan moieties might lead to shorter half-life (124, 125). Therefore, potential differences in posttranslational LCN2 glycosylation of murine and human LCN2 might provoke significant differences in tissue distribution and stability. Similarly, a different status of amidation in both mouse and human LCN2 might have different impact on the pathogenesis of various diseases such as inflammation, endothelial dysfunction, and hypertension (126). Consequently, high- (HMW) and low-molecular weight (LWM) species of LCN2 in mice and humans can result from different (unknown) posttranslational modifications. If these modifications are induced by similar mechanisms and provoke identical biological effects in mice and humans is still not known. Finally, experiments performed to study impact of LCN2 on cell death, mitochondrial functions, and other biological attributes could result in different findings when conducted with recombinant non-glycosylated or glycosylated LCN2. Moreover, experiments can lead to erroneous results if cells are treated with LCN2 originating from a different species differing in sequence or carrying different posttranslational modifications that will affect the activity to putative receptors.
6.5 LCN2 receptors in homeostasis and disease
Mice genetically disrupted for individual LCN2 receptors show severe phenotypes or die perinatally (44, 46–48), while mice lacking Lcn2 are viable (15). The fact that disruption of a potential LCN2 receptor provokes a more severe phenotype is not surprising, because the proposed receptors are multi-ligand binding receptors associated to many other pathways. However, the ectopic overexpression of NGALR confers on cells the ability to take up iron or undergo apoptosis, dependent upon the iron content of LCN2 (13). This finding indicates that some of the LCN2-receptor functions can be separated from others and are independent from other receptors. Nevertheless, it must be critically noticed that NGALR compared to LRP2 has about 1000 fold higher affinity for LCN2 and overexpression of NGALR or addition of LCN2 in a setting of an experiment will also modify the binding of LRP2 to LCN2. So how can we be sure that the observed biological effect (e.g. induction of apoptosis) is mediated through the NGALR axis and not through the LRP2 axis?
Moreover, numerous human diseases are associated with alterations in LCN2 receptor expression. In particular, NGALR expression was shown to be modified in myeloproliferative neoplasm (MPN), colorectal cancer (CRC), esophageal squamous cell carcinoma (ESCC), glioma, clear cell renal cell carcinoma (ccRCC), glomerulonephritis, hepatocellular carcinoma (HCC), alcoholic hepatitis, obesity, psoriasis, gastric cancer, endometrial cancer, and many other disorders (Table 3). It is obvious that modified receptor expression should have (at least in part) the same effect as altered expression of its ligand, i.e. LCN2. However, if this is in fact the case is not known yet. To further assess the level of LCN2 receptors in the disease state, screening with a validated antibody of various healthy organ systems would be strongly recommended. Certainly, a comparison between different species such as mice and humans would be advisable to clearly localize the expression of the different receptors. Finally, modified NGALR expression in disease will inevitably cause changes in the amount of LCN2 that can be bound to LRP2. This will lead to modified activity of this receptor branch. Therefore, it will be difficult/challenging/complex/problematic to estimate if disease-associated effects are mediated by altered NGALR expression or alternatively by subsequent alterations in LRP2 signaling.
7 Conclusions
LCN2 is a pleiotropic molecule involved in many biological processes including control of iron homeostasis, cell differentiation, energy expenditure, bacterial defense, cell death, chemotaxis, cell migration, and many others. Most of the biological functions of LCN2 were established in work conducted in mice. Nowadays, six potential LCN2 receptors (NGALR, LRP2, LRP6, MCR4, MCR1, and MCR3) were identified that markedly differ in regard to structure and affinity. In humans, concentrations of LCN2 in urine and serum have become increasingly relevant as a prognostic and predictive biomarker for many diseases (150, 151). Nevertheless, changes in LCN2 or LCN2 receptor expression in the course of a specific disease was mostly only reported in association or correlation studies. Actually, there is a significant gap in the understanding of the underlying mechanisms by which LCN2 and its putative receptors contribute to the initiation or progression of a disease. It could be that some findings found in experimental mouse models cannot be translated 1:1 to the human situation. Murine and human LCN2 only share a sequence identity of 62% and there are significant biochemical and functional differences between both orthologs. This might result in different affinities for putative receptors and other biomolecules supposed to interact with LCN2, resulting in modified biological effects in both species. Contradictory findings with regard to biological effects of LCN2 show that the effects of LCN2 are complex and influenced by many factors, including species, disease, sex, cell type, posttranslational modifications, receptors, and binding partners. Possibly, some of the discrepancies and inconsistencies are raised by the fact that many findings on LCN2 and its receptors were established in in vitro models and have no relevance for the in vitro situation. Finally, the usage of antibodies with unclear sensitivity and specificity may lead to data that cannot be interpreted. It cannot be excluded that incorrect expression patterns of the LCN2 receptors can also be found in the literature because the antibodies used have not been sufficiently validated. We hope that our article will help to sensitize scientists for this critical issue that may provoke the production of misleading “scientific” data.
Author contributions
RW conceived and planned the drafting of this review. All other authors have written part of this review and helped in design and drawing of images. All authors contributed to the article and approved the submitted version.
Funding
The laboratory of RW is funded by the German Research Foundation (grants WE2554/13-1, WE2554/15-1, WE 2554/17-1) and a grant from the Interdisciplinary Centre for Clinical Research within the faculty of Medicine at the RWTH Aachen University (grant PTD 1-5).
Acknowledgments
The authors are grateful for receiving unpublished information from Suzanne Hraba-Renevey (BusinessIn, Lausanne, Switzerland) and Yukio Nakamura (RIKEN BioResource Research Center, University of Tsukuba, Japan) for our article.
Conflict of interest
The authors declare that the research was conducted in the absence of any commercial or financial relationships that could be construed as a potential conflict of interest.
Publisher’s note
All claims expressed in this article are solely those of the authors and do not necessarily represent those of their affiliated organizations, or those of the publisher, the editors and the reviewers. Any product that may be evaluated in this article, or claim that may be made by its manufacturer, is not guaranteed or endorsed by the publisher.
Supplementary material
The Supplementary Material for this article can be found online at: https://www.frontiersin.org/articles/10.3389/fimmu.2023.1229885/full#supplementary-material
Glossary (in alphabetical order)
References
1. Chan YL, Paz V, Wool IG. The primary structure of rat alpha 2µ globulin-related protein. Nucleic Acids Res (1988) 16(23):11368. doi: 10.1093/nar/16.23.1136
2. Hraba-Renevey S, Türler H, Kress M, Salomon C, Weil R. SV40-induced expression of mouse gene 24p3 involves a post-transcriptional mechanism. Oncogene (1989) 4(5):601–8.
3. Kjeldsen L, Johnsen AH, Sengeløv H, Borregaard N. Isolation and primary structure of NGAL, a novel protein associated with human neutrophil gelatinase. J Biol Chem (1993) 268(14):10425–32. doi: 10.1016/S0021-9258(18)82217-7
4. RCSB. Protein Data Bank (PDB). Available at: https://www.rcsb.org/ (Accessed 11 May 2023).
5. Nilsen-Hamilton M, Hamilton RT, Adams GA. Rapid selective stimulation by growth factors of the incorporation by BALB/C 3T3 cells of [35S]methionine into a glycoprotein and five superinducible proteins. Biochem Biophys Res Commun (1982) 108(1):158–66. doi: 10.1016/0006-291x(82)91845-9
6. Davis TR, Tabatabai L, Bruns K, Hamilton RT, Nilsen-Hamilton M. Basic fibroblast growth factor induces 3T3 fibroblasts to synthesize and secrete a cyclophilin-like protein and beta 2-microglobulin. Biochim Biophys Acta (1991) 1095(2):145–52. doi: 10.1016/0167-4889(91)90077-b
7. Liu Q, Nilsen-Hamilton M. Identification of a new acute phase protein. J Biol Chem (1995) 270(38):22565–70. doi: 10.1074/jbc.270.38.22565
8. Borkham-Kamphorst E, Drews F, Weiskirchen R. Induction of lipocalin-2 expression in acute and chronic experimental liver injury moderated by pro-inflammatory cytokines interleukin-1β through nuclear factor-κB activation. Liver Int (2011) 31(5):656–65. doi: 10.1111/j.1478-3231.2011.02495.x
9. Borkham-Kamphorst E, van de Leur E, Zimmermann HW, Karlmark KR, Tihaa L, Haas U, et al. Protective effects of lipocalin-2 (LCN2) in acute liver injury suggest a novel function in liver homeostasis. Biochim Biophys Acta (2013) 1832(5):660–73. doi: 10.1016/j.bbadis.2013.01.014
10. Goetz DH, Holmes MA, Borregaard N, Bluhm ME, Raymond KN, Strong RK. The neutrophil lipocalin NGAL is a bacteriostatic agent that interferes with siderophore-mediated iron acquisition. Mol Cell (2002) 10(5):1033–43. doi: 10.1016/s1097-2765(02)00708-6
11. Yang J, Goetz D, Li JY, Wang W, Mori K, Setlik D, et al. An iron delivery pathway mediated by a lipocalin. Mol Cell (2002) 10(5):1045–56. doi: 10.1016/s1097-2765(02)00710-4
12. Kramer J, Özkaya Ö, Kümmerli R. Bacterial siderophores in community and host interactions. Nat Rev Microbiol (2020) 18(3):152–63. doi: 10.1038/s41579-019-0284-4
13. Devireddy LR, Gazin C, Zhu X, Green MR. A cell-surface receptor for lipocalin 24p3 selectively mediates apoptosis and iron uptake. Cell (2005) 123(7):1293–305. doi: 10.1016/j.cell.2005.10.027
14. Flo TH, Smith KD, Sato S, Rodriguez DJ, Holmes MA, Strong RK, et al. Lipocalin 2 mediates an innate immune response to bacterial infection by sequestrating iron. Nature (2004) 432(7019):917–21. doi: 10.1038/nature03104
15. Berger T, Togawa A, Duncan GS, Elia AJ, You-Ten A, Wakeham A, et al. Lipocalin 2-deficient mice exhibit increased sensitivity to Escherichia coli infection but not to ischemia-reperfusion injury. Proc Natl Acad Sci USA (2006) 103(6):1834–9. doi: 10.1073/pnas.0510847103
16. Klüber P, Meurer SK, Lambertz J, Schwarz R, Zechel-Gran S, Braunschweig T, et al. Depletion of Lipocalin 2 (LCN2) in mice leads to dysbiosis and persistent colonization with segmented filamentous bacteria. Int J Mol Sci (2021) 22(23):13156. doi: 10.3390/ijms222313156
17. Asimakopoulou A, Borkham-Kamphorst E, Tacke F, Weiskirchen R. Lipocalin-2 (NGAL/LCN2), a "help-me" signal in organ inflammation. Hepatology (2016) 63(2):669–71. doi: 10.1002/hep.27930
18. Liu Q, Ryon J, Nilsen-Hamilton M. Uterocalin: a mouse acute phase protein expressed in the uterus around birth. Mol Reprod Dev (1997) 46(4):507–14. doi: 10.1002/(SICI)1098-2795(199704)46:4<507
19. Huang HL, Chu ST, Chen YH. Ovarian steroids regulate 24p3 expression in mouse uterus during the natural estrous cycle and the preimplantation period. J Endocrinol (1999) 162(1):11–9. doi: 10.1677/joe.0.1620011
20. Kessel JC, Weiskirchen R, Schröder SK. Expression analysis of lipocalin 2 (LCN2) in reproductive and non-reproductive tissues of Esr1-deficient mice. Int J Mol Sci (2023) 24:9280. doi: 10.3390/ijms24119280
21. Watanabe H, Takeo T, Tojo H, Sakoh K, Berger T, Nakagata N, et al. Lipocalin 2 binds to membrane phosphatidylethanolamine to induce lipid raft movement in a PKA-dependent manner and modulates sperm maturation. Development (2014) 141(10):2157–64. doi: 10.1242/dev.105148
22. Kang Z, Qiao N, Tan Z, Tang Z, Li Y. Expression patterns and changes of the LCN2 gene in the testes of induced cryptorchidism and busulfan-treated mice. Syst Biol Reprod Med (2017) 63(6):364–9. doi: 10.1080/19396368.2017.1355416
23. Mosialou I, Shikhel S, Luo N, Petropoulou PI, Panitsas K, Bisikirska B, et al. Lipocalin-2 counteracts metabolic dysregulation in obesity and diabetes. J Exp Med (2020) 217(10):e20191261. doi: 10.1084/jem.20191261
24. Paton CM, Rogowski MP, Kozimor AL, Stevenson JL, Chang H, Cooper JA. Lipocalin-2 increases fat oxidation in vitro and is correlated with energy expenditure in normal weight but not obese women. Obes (Silver Spring) (2013) 21(12):E640–8. doi: 10.1002/oby.20507
25. Petropoulou PI, Mosialou I, Shikhel S, Hao L, Panitsas K, Bisikirska B, et al. Lipocalin-2 is an anorexigenic signal in primates. Elife (2020) 9:e58949. doi: 10.7554/eLife.58949
26. Olson B, Zhu X, Norgard MA, Levasseur PR, Butler JT, Buenafe A, et al. Lipocalin 2 mediates appetite suppression during pancreatic cancer cachexia. Nat Commun (2021) 12(1):2057. doi: 10.1038/s41467-021-22361-3
27. Asimakopoulou A, Fülöp A, Borkham-Kamphorst E, de Leur EV, Gassler N, Berger T, et al. Altered mitochondrial and peroxisomal integrity in lipocalin-2-deficient mice with hepatic steatosis. Biochim Biophys Acta Mol Basis Dis (2017) 1863(9):2093–110. doi: 10.1016/j.bbadis.2017.04.006
28. Asimakopoulou A, Borkham-Kamphorst E, Henning M, Yagmur E, Gassler N, Liedtke C, et al. Lipocalin-2 (LCN2) regulates PLIN5 expression and intracellular lipid droplet formation in the liver. Biochim Biophys Acta (2014) 1842(10):1513–24. doi: 10.1016/j.bbalip.2014.07.017
29. Correnti C, Richardson V, Sia AK, Bandaranayake AD, Ruiz M, Suryo Rahmanto Y, et al. Siderocalin/Lcn2/NGAL/24p3 does not drive apoptosis through gentisic acid mediated iron withdrawal in hematopoietic cell lines. PloS One (2012) 7(8):e43696. doi: 10.1371/journal.pone.0043696
30. Cabedo Martinez AI, Weinhäupl K, Lee WK, Wolff NA, Storch B, Żerko S, et al. Biochemical and structural characterization of the interaction between the siderocalin NGAL/LCN2 (neutrophil gelatinase-associated lipocalin/lipocalin 2) and the N-terminal domain of its endocytic receptor SLC22A17. J Biol Chem (2016) 291(6):2917–30. doi: 10.1074/jbc.M115.685644
31. Christ A, Herzog K, Willnow TE. LRP2, an auxiliary receptor that controls sonic hedgehog signaling in development and disease. Dev Dyn (2016) 245(5):569–79. doi: 10.1002/dvdy.24394
32. Christensen EI, Birn H. Megalin and cubilin: multifunctional endocytic receptors. Nat Rev Mol Cell Biol (2002) 3(4):256–66. doi: 10.1038/nrm778
33. Israeli H, Degtjarik O, Fierro F, Chunilal V, Gill AK, Roth NJ, et al. Structure reveals the activation mechanism of the MC4 receptor to initiate satiation signaling. Science (2021) 372(6544):808–14. doi: 10.1126/science.abf7958
34. Zhang H, Chen LN, Yang D, Mao C, Shen Q, Feng W, et al. Structural insights into ligand recognition and activation of the melanocortin-4 receptor. Cell Res (2021) 31(11):1163–75. doi: 10.1038/s41422-021-00552-3
35. Saito A, Pietromonaco S, Loo AK, Farquhar MG. Complete cloning and sequencing of rat gp330/"megalin," a distinctive member of the low density lipoprotein receptor gene family. Proc Natl Acad Sci USA (1994) 91(21):9725–9. doi: 10.1073/pnas.91.21.9725
36. Gantz I, Miwa H, Konda Y, Shimoto Y, Tashiro T, Watson SJ, et al. Molecular cloning, expression, and gene localization of a fourth melanocortin receptor. J Biol Chem (1993) 268(20):15174–9. doi: 10.1016/S0021-9258(18)82452-8
37. Chhajlani V, Wikberg JE. Molecular cloning and expression of the human melanocyte stimulating hormone receptor cDNA. FEBS Lett (1992) 309(3):417–20. doi: 10.1016/0014-5793(92)80820-7
38. Mountjoy KG, Robbins LS, Mortrud MT, Cone RD. The cloning of a family of genes that encode the melanocortin receptors. Science (1992) 257(5074):1248–51. doi: 10.1126/science.1325670
39. Gantz I, Konda Y, Tashiro T, Shimoto Y, Miwa H, Munzert G, et al. Molecular cloning of a novel melanocortin receptor. J Biol Chem (1993) 268(11):8246–50. doi: 10.1016/S0021-9258(18)53088-X
40. Desarnaud F, Labbe O, Eggerickx D, Vassart G, Parmentier M. Molecular cloning, functional expression and pharmacological characterization of a mouse melanocortin receptor gene. Biochem J (1994) 299(Pt 2):367–73. doi: 10.1042/bj2990367
41. Devireddy LR, Teodoro JG, Richard FA, Green MR. Induction of apoptosis by a secreted lipocalin that is transcriptionally regulated by IL-3 deprivation. Science (2001) 293(5531):829–34. doi: 10.1126/science.1061075
42. Hvidberg V, Jacobsen C, Strong RK, Cowland JB, Moestrup SK, Borregaard N. The endocytic receptor megalin binds the iron transporting neutrophil-gelatinase-associated lipocalin with high affinity and mediates its cellular uptake. FEBS Lett (2005) 579(3):773–7. doi: 10.1016/j.febslet.2004.12.031
43. Mosialou I, Shikhel S, Liu JM, Maurizi A, Luo N, He Z, et al. MC4R-dependent suppression of appetite by bone-derived lipocalin 2. Nature (2017) 543(7645):385–39. doi: 10.1038/nature21697
44. Willnow TE, Hilpert J, Armstrong SA, Rohlmann A, Hammer RE, Burns DK, et al. Defective forebrain development in mice lacking gp330/megalin. Proc Natl Acad Sci USA (1996) 93(16):8460–4. doi: 10.1073/pnas.93.16.8460
45. Schmitz C, Hilpert J, Jacobsen C, Boensch C, Christensen EI, Luft FC, et al. Megalin deficiency offers protection from renal aminoglycoside accumulation. J Biol Chem (2002) 277(1):618–22. doi: 10.1074/jbc.M109959200
46. Huszar D, Lynch CA, Fairchild-Huntress V, Dunmore JH, Fang Q, Berkemeier LR, et al. Targeted disruption of the melanocortin-4 receptor results in obesity in mice. Cell (1997) 88(1):131–41. doi: 10.1016/s0092-8674(00)81865-6
47. Healy E, Jordan SA, Budd PS, Suffolk R, Rees JL, Jackson IJ. Functional variation of MC1R alleles from red-haired individuals. Hum Mol Genet (2001) 10(21):2397–402. doi: 10.1093/hmg/10.21.2397
48. Chen AS, Marsh DJ, Trumbauer ME, Frazier EG, Guan XM, Yu H, et al. Inactivation of the mouse melanocortin-3 receptor results in increased fat mass and reduced lean body mass. Nat Genet (2000) 26(1):97–102. doi: 10.1038/79254
49. Jiang JH, Wang SY, Zhang J, Liu H, Ke KX, Jiang Y, et al. LCN2 inhibits the BMP9-induced osteogenic differentiation through reducing Wnt/β-catenin signaling via interacting with LRP6 in mouse embryonic fibroblasts. Curr Stem Cell Res Ther (2023) 18:1160–71. doi: 10.2174/1574888X18666230320091546
50. Fang WK, Xu LY, Lu XF, Liao LD, Cai WJ, Shen ZY, et al. A novel alternative spliced variant of neutrophil gelatinase-associated lipocalin receptor in oesophageal carcinoma cells. Biochem J (2007) 403(2):297–303. doi: 10.1042/BJ20060836
51. Perland E, Fredriksson R. Classification systems of secondary active transporters. Trends Pharmacol Sci (2017) 38(3):305–15. doi: 10.1016/j.tips.2016.11.008
52. Pizzagalli MD, Bensimon A, Superti-Furga G. A guide to plasma membrane solute carrier proteins. FEBS J (2021) 288(9):2784–835. doi: 10.1111/febs.15531
53. Yee SW, Giacomini KM. Emerging roles of the human solute carrier 22 family. Drug Metab Dispos (2021) 50(9):1193–210. doi: 10.1124/dmd.121.000702
54. IMPC (International Mouse Phenotyping Consortium. Gene: Slc22a12, MGI:1926225. Available at: https://www.mousephenotype.org/data/genes/MGI:1926225#phenotypes-section (Accessed 27 May 2023).
55. Groza T, Gomez FL, Mashhadi HH, Muñoz-Fuentes V, Gunes O, Wilson R, et al. The International Mouse Phenotyping Consortium: comprehensive knockout phenotyping underpinning the study of human disease. Nucleic Acids Res (2023) 51(D1):D1038–45. doi: 10.1093/nar/gkac972
56. Kim KE, Lee J, Shin HJ, Jeong EA, Jang HM, Ahn YJ, et al. Lipocalin-2 activates hepatic stellate cells and promotes nonalcoholic steatohepatitis in high-fat diet-fed Ob/Ob mice. Hepatology (2023) 77(3):888–901. doi: 10.1002/hep.32569
57. Jarmoskaite I, AlSadhan I, Vaidyanathan PP, Herschlag D. How to measure and evaluate binding affinities. Elife (2020) 9:e57264. doi: 10.7554/eLife.57264
58. Björkelund H, Gedda L, Andersson K. Comparing the epidermal growth factor interaction with four different cell lines: intriguing effects imply strong dependency of cellular context. PloS One (2011) 6(1):e16536. doi: 10.1371/journal.pone.0016536
59. Andersen OM, Dagil R, Kragelund BB. New horizons for lipoprotein receptors: communication by β-propellers. J Lipid Res (2013) 54(10):2763–74. doi: 10.1194/jlr.M039545
60. Calvier L, Herz J, Hansmann G. Interplay of low-density lipoprotein receptors, LRPs, and lipoproteins in pulmonary hypertension. JACC Basic Transl Sci (2022) 7(2):164–80. doi: 10.1016/j.jacbts.2021.09.011
61. Barasch J, Hollmen M, Deng R, Hod EA, Rupert PB, Abergel RJ, et al. Disposal of iron by a mutant form of lipocalin 2. Nat Commun (2016) 7:12973. doi: 10.1038/ncomms12973
62. Christensen EI, Moskaug JO, Vorum H, Jacobsen C, Gundersen TE, Nykjaer A, et al. Evidence for an essential role of megalin in transepithelial transport of retinol. J Am Soc Nephrol (1999) 10(4):685–95. doi: 10.1681/ASN.V104685
63. Redl B, Habeler M. The diversity of lipocalin receptors. Biochimie (2022) 192:22–9. doi: 10.1016/j.biochi.2021.09.008
64. Leheste JR, Rolinski B, Vorum H, Hilpert J, Nykjaer A, Jacobsen C, et al. Megalin knockout mice as an animal model of low molecular weight proteinuria. Am J Pathol (1999) 155(4):1361–70. doi: 10.1016/S0002-9440(10)65238-8
65. Kounnas MZ, Chappell DA, Strickland DK, Argraves WS. Glycoprotein 330, a member of the low density lipoprotein receptor family, binds lipoprotein lipase in vitro. J Biol Chem (1993) 268(19):14176–81. doi: 10.1016/S0021-9258(19)85224-9
66. Zhang J, Wang Z, Zhang H, Li S, Li J, Liu H, et al. The role of lipocalin 2 in brain injury and recovery after ischemic and hemorrhagic stroke. Front Mol Neurosci (2022) 15:930526. doi: 10.3389/fnmol.2022.930526
67. Williams BO, Insogna KL. Where Wnts went: the exploding field of Lrp5 and Lrp6 signaling in bone. J Bone Miner Res (2009) 24(2):171–8. doi: 10.1359/jbmr.081235
68. Moscowitz AE, Asif H, Lindenmaier LB, Calzadilla A, Zhang C, Mirsaeidi M. The importance of melanocortin receptors and their agonists in pulmonary disease. Front Med (Lausanne) (2019) 6:145. doi: 10.3389/fmed.2019.00145
69. Yuan XC, Tao YX. Ligands for melanocortin receptors: Beyond melanocyte-stimulating hormones and adrenocorticotropin. Biomolecules (2022) 12(10):1407. doi: 10.3390/biom12101407
70. Yu S, Doycheva DM, Gamdzyk M, Yang Y, Lenahan C, Li G, et al. Activation of MC1R with BMS-470539 attenuates neuroinflammation via cAMP/PKA/Nurr1 pathway after neonatal hypoxic-ischemic brain injury in rats. J Neuroinflamm (2021) 18(1):26. doi: 10.1186/s12974-021-02078-2
71. Xu W, Mo J, Ocak U, Travis ZD, Enkhjargal B, Zhang T, et al. Activation of melanocortin 1 receptor attenuates early brain injury in a rat model of subarachnoid hemorrhage via the suppression of neuroinflammation through AMPK/TBK1/NF-κB pathway in rats. Neurotherapeutics (2020) 17(1):294–308. doi: 10.1007/s13311-019-00772-x
72. Zhao RY, Wei PJ, Sun X, Zhang DH, He QY, Liu J, et al. Role of lipocalin 2 in stroke. Neurobiol Dis (2023) 179:106044. doi: 10.1016/j.nbd.2023.106044
73. Tao YX. The melanocortin-4 receptor: physiology, pharmacology, and pathophysiology. Endocr Rev (2010) 31(4):506–43. doi: 10.1210/er.2009-0037
74. Hall MAL, Kohut-Jackson AL, Peyla AC, Friedman GD, Simco NJ, Borland JM, et al. Melanocortin receptor 3 and 4 mRNA expression in the adult female Syrian hamster brain. Front Mol Neurosci (2023) 16:1038341. doi: 10.3389/fnmol.2023.1038341
75. Bennett KM, Liu J, Hoelting C, Stoll J. Expression and analysis of two novel rat organic cation transporter homologs, SLC22A17 and SLC22A23. Mol Cell Biochem (2011) 352(1-2):143–54. doi: 10.1007/s11010-011-0748-y
76. Probst S, Scharner B, McErlean R, Lee WK, Thévenod F. Inverse regulation of lipocalin-2/24p3 receptor/SLC22A17 and lipocalin-2 expression by tonicity, NFAT5/TonEBP and arginine vasopressin in mouse cortical collecting duct cells mCCD(cl.1): Implications for osmotolerance. Int J Mol Sci (2019) 20(21):5398. doi: 10.3390/ijms20215398
77. Lee S, Lee WH, Lee MS, Mori K, Suk K. Regulation by lipocalin-2 of neuronal cell death, migration, and morphology. J Neurosci Res (2012) 90(3):540–50. doi: 10.1002/jnr.22779
78. Li PT, Lee YC, Elangovan N, Chu ST. Mouse 24p3 protein has an effect on L929 cell viability. Int J Biol Sci (2007) 3(2):100–7. doi: 10.7150/ijbs.3.100
79. Li Q, Ru X, Yang Y, Zhao H, Qu J, Chen W, et al. Lipocalin-2-mediated insufficient oligodendrocyte progenitor cell remyelination for white matter injury after subarachnoid hemorrhage via SCL22A17 receptor/early growth response protein 1 signaling. Neurosci Bull (2022) 38(12):1457–75. doi: 10.1007/s12264-022-00906-w
80. Borkham-Kamphorst E, Van de Leur E, Haas U, Weiskirchen R. Liver parenchymal cells lacking Lipocalin 2 (LCN2) are prone to endoplasmic reticulum stress and unfolded protein response. Cell Signal (2019) 55:90–9. doi: 10.1016/j.cellsig.2019.01.001
81. Kehrer JP. Lipocalin-2: pro- or anti-apoptotic? Cell Biol Toxicol (2010) 26(2):83–9. doi: 10.1007/s10565-009-9119-9
82. Zheng G, Marino' M, Zhao J, McCluskey RT. Megalin (gp330): a putative endocytic receptor for thyroglobulin (Tg). Endocrinology (1998) 139(3):1462–5. doi: 10.1210/endo.139.3.5978
83. Kuhbandner K, Herz J, Pohlkamp T. Chapter 18 - lipoprotein receptors. In: Ridgway ND, McLeod RS, editors. Biochemistry of Lipids, Lipoproteins and Membranes, Seventh edition. Oxford, United States: Elsevier Sciences Amsterdam (2015) 583–622. doi: 10.1016/C2020-0-00089-7
84. Long KR, Rbaibi Y, Bondi CD, Ford BR, Poholek AC, Boyd-Shiwarski CR, et al. Cubilin-, megalin-, and Dab2-dependent transcription revealed by CRISPR/Cas9 knockout in kidney proximal tubule cells. Am J Physiol Renal Physiol (2022) 322(1):F14–26. doi: 10.1152/ajprenal.00259.2021
85. Inoue A, Raimondi F, Kadji FMN, Singh G, Kishi T, Uwamizu A, et al. Illuminating G-protein-coupling selectivity of GPCRs. Cell (2019) 177(7):1933–1947.e25. doi: 10.1016/j.cell.2019.04.044
86. Paisdzior S, Dimitriou IM, Schöpe PC, Annibale P, Scheerer P, Krude H, et al. Differential signaling profiles of MC4R mutations with three different ligands. Int J Mol Sci (2020) 21(4):1224. doi: 10.3390/ijms21041224
87. Li YQ, Shrestha Y, Pandey M, Chen M, Kablan A, Gavrilova O, et al. G(q/11)α and G(s)α mediate distinct physiological responses to central melanocortins. J Clin Invest (2016) 126(1):40–9. doi: 10.1172/JCI76348
88. Podyma B, Sun H, Wilson EA, Carlson B, Pritikin E, Gavrilova O, et al. The stimulatory G protein Gsα is required in melanocortin 4 receptor-expressing cells for normal energy balance, thermogenesis, and glucose metabolism. J Biol Chem (2018) 293(28):10993–1005. doi: 10.1074/jbc.RA118.003450
89. Yang LK, Tao YX. Biased signaling at neural melanocortin receptors in regulation of energy homeostasis. Biochim Biophys Acta Mol Basis Dis (2017) 1863(10 Pt A):2486–95. doi: 10.1016/j.bbadis.2017.04.010
90. Wu B, Li C, Du Z, Yao Q, Wu J, Feng L, et al. Network based analyses of gene expression profile of LCN2 overexpression in esophageal squamous cell carcinoma. Sci Rep (2014) 4:5403. doi: 10.1038/srep05403
91. The Human Protein Atlas. The open access resource for human proteins. Available at: https://www.proteinatlas.org/ (Accessed 15.4.2023).
92. Uhlen M, Oksvold P, Fagerberg L, Lundberg E, Jonasson K, Forsberg M, et al. Towards a knowledge-based human protein atlas. Nat Biotechnol (2010) 28(12):1248–50. doi: 10.1038/nbt1210-1248
93. Lundgren S, Carling T, Hjälm G, Juhlin C, Rastad J, Pihlgren U, et al. Tissue distribution of human gp330/megalin, a putative Ca(2+)-sensing protein. J Histochem Cytochem (1997) 45(3):383–92. doi: 10.1177/002215549704500306
94. Assémat E, Châtelet F, Chandellier J, Commo F, Cases O, Verroust P, et al. Overlapping expression patterns of the multiligand endocytic receptors cubilin and megalin in the CNS, sensory organs and developing epithelia of the rodent embryo. Gene Expr Patterns (2005) 6(1):69–78. doi: 10.1016/j.modgep.2005.04.014
95. Monteon FJ, Laidlaw SA, Shaib JK, Kopple JD. Energy expenditure in patients with chronic renal failure. Kidney Int (1986) 30(5):741–7. doi: 10.1038/ki.1986.250
96. Guo H, Jin D, Zhang Y, Wright W, Bazuine M, Brockman DA, et al. Lipocalin-2 deficiency impairs thermogenesis and potentiates diet-induced insulin resistance in mice. Diabetes (2010) 59(6):1376–85. doi: 10.2337/db09-1735
97. Law IK, Xu A, Lam KS, Berger T, Mak TW, Vanhoutte PM, et al. Lipocalin-2 deficiency attenuates insulin resistance associated with aging and obesity. Diabetes (2010) 59(4):872–82. doi: 10.2337/db09-1541
98. Kamble PG, Pereira MJ, Sidibeh CO, Amini S, Sundbom M, Börjesson JL, et al. Lipocalin 2 produces insulin resistance and can be upregulated by glucocorticoids in human adipose tissue. Mol Cell Endocrinol (2016) 427:124–32. doi: 10.1016/j.mce.2016.03.011
99. Orlando RA, Rader K, Authier F, Yamazaki H, Posner BI, Bergeron JJ, et al. Megalin is an endocytic receptor for insulin. J Am Soc Nephrol (1998) 9(10):1759–66. doi: 10.1681/ASN.V9101759
100. Bryniarski MA, Yee BM, Jaffri I, Chaves LD, Yu JA, Guan X, et al. Increased megalin expression in early type 2 diabetes: role of insulin-signaling pathways. Am J Physiol Renal Physiol (2018) 315(5):F1191–207. doi: 10.1152/ajprenal.00210.2018
101. de Souza Cordeiro LM, Elsheikh A, Devisetty N, Morgan DA, Ebert SN, Rahmouni K, et al. Hypothalamic MC4R regulates glucose homeostasis through adrenaline-mediated control of glucose reabsorption via renal GLUT2 in mice. Diabetologia (2021) 64(1):181–94. doi: 10.1007/s00125-020-05289-z
102. Ni Z, Wang Y, Shi C, Zhang X, Gong H, Dong Y. Islet MC4R regulates PC1/3 to improve insulin secretion in T2DM mice via the cAMP and β-arrestin-1 pathways. Appl Biochem Biotechnol (2022) 194(12):6164–78. doi: 10.1007/s12010-022-04089-y
103. Chen J, Argemi J, Odena G, Xu MJ, Cai Y, Massey V, et al. Hepatic lipocalin 2 promotes liver fibrosis and portal hypertension. Sci Rep (2020) 10(1):15558. doi: 10.1038/s41598-020-72172-7
104. Du Y, Li W, Lin L, Lo EH, Xing C. Effects of lipocalin-2 on brain endothelial adhesion and permeability. PloS One (2019) 14(7):e0218965. doi: 10.1371/journal.pone.0218965
105. Hewitt SM, Baskin DG, Frevert CW, Stahl WL, Rosa-Molinar E. Controls for immunohistochemistry: the Histochemical Society's standards of practice for validation of immunohistochemical assays. J Histochem Cytochem (2014) 62(10):693–7. doi: 10.1369/0022155414545224
106. Qosa H, Abuznait AH, Hill RA, Kaddoumi A. Enhanced brain amyloid-β clearance by rifampicin and caffeine as a possible protective mechanism against Alzheimer's disease. J Alzheimers Dis (2012) 31(1):151–65. doi: 10.3233/JAD-2012-120319
107. Langelueddecke C, Roussa E, Fenton RA, Wolff NA, Lee WK, Thévenod F. Lipocalin-2 (24p3/neutrophil gelatinase-associated lipocalin (NGAL)) receptor is expressed in distal nephron and mediates protein endocytosis. J Biol Chem (2012) 287(1):159–69. doi: 10.1074/jbc.M111.308296
108. De La Chesnaye E, Manuel-Apolinar L, Damasio L, Olivares A, Palomino MA, Santos I, et al. Expression profiling of lipocalin-2 and 24p3 receptor in murine gonads at different developmental stages. Exp Ther Med (2018) 16(1):213–21. doi: 10.3892/etm.2018.6196
109. Mestas J, Hughes CC. Of mice and not men: differences between mouse and human immunology. J Immunol (2004) 172(5):2731–8. doi: 10.4049/jimmunol.172.5.2731
110. Seok J, Warren HS, Cuenca AG, Mindrinos MN, Baker HV, Xu W, et al. Inflammation and Host Response to Injury, Large Scale Collaborative Research Program. Genomic responses in mouse models poorly mimic human inflammatory diseases. Proc Natl Acad Sci USA (2013) 110(9):3507–12. doi: 10.1073/pnas.1222878110
111. Takao K, Miyakawa T. Genomic responses in mouse models greatly mimic human inflammatory diseases. Proc Natl Acad Sci USA (2015) 112(4):1167–72. doi: 10.1073/pnas.1401965111
112. Leenaars CHC, Kouwenaar C, Stafleu FR, Bleich A, Ritskes-Hoitinga M, De Vries RBM, et al. Animal to human translation: a systematic scoping review of reported concordance rates. J Transl Med (2019) 17(1):223. doi: 10.1186/s12967-019-1976-2
113. National Library of Medicine. blastp . Available at: https://blast.ncbi.nlm.nih.gov/Blast.cgi?PAGE=Proteins&PROGRAM=blastp&BLAST_PROGRAMS=blastp&PAGE_TYPE=BlastSearch&BLAST_SPEC=blast2seq (Accessed 15.4.2023).
114. Gharib WH, Robinson-Rechavi M. When orthologs diverge between human and mouse. Brief Bioinform (2011) 12(5):436–41. doi: 10.1093/bib/bbr031
115. Triebel S, Bläser J, Reinke H, Tschesche H. A 25 kDa alpha 2-microglobulin-related protein is a component of the 125 kDa form of human gelatinase. FEBS Lett (1992) 314(3):386–8. doi: 10.1016/0014-5793(92)81511-j
116. Holmes MA, Paulsene W, Jide X, Ratledge C, Strong RK. Siderocalin (Lcn 2) also binds carboxymycobactins, potentially defending against mycobacterial infections through iron sequestration. Structure (2005) 13(1):29–41. doi: 10.1016/j.str.2004.10.009
117. Mondal S, Adhikari N, Banerjee S, Amin SA, Jha T. Matrix metalloproteinase-9 (MMP-9) and its inhibitors in cancer: A minireview. Eur J Med Chem (2020) 194:112260. doi: 10.1016/j.ejmech.2020.112260
118. Tschesche H, Zölzer V, Triebel S, Bartsch S. The human neutrophil lipocalin supports the allosteric activation of matrix metalloproteinases. Eur J Biochem (2001) 268(7):1918–28. doi: 10.1046/j.1432-1327.2001.02066.x
119. Candido S, Abrams SL, Steelman LS, Lertpiriyapong K, Fitzgerald TL, Martelli AM, et al. Roles of NGAL and MMP-9 in the tumor microenvironment and sensitivity to targeted therapy. Biochim Biophys Acta (2016) 1863(3):438–48. doi: 10.1016/j.bbamcr.2015.08.010
120. Santiago-Sánchez GS, Pita-Grisanti V, Quiñones-Díaz B, Gumpper K, Cruz-Monserrate Z, Vivas-Mejía PE. Biological functions and therapeutic potential of lipocalin 2 in cancer. Int J Mol Sci (2020) 21(12):4365. doi: 10.3390/ijms21124365
121. Weng YC, Wang G, Messing RO, Chou WH. Identification of lipocalin-2 as a PKCδ phosphorylation substrate in neutrophils. J BioMed Sci (2015) 22(1):21. doi: 10.1186/s12929-015-0129-z
122. Rudd PM, Mattu TS, Masure S, Bratt T, Van den Steen PE, Wormald MR, et al. Glycosylation of natural human neutrophil gelatinase B and neutrophil gelatinase B-associated lipocalin. Biochemistry (1999) 38(42):13937–50. doi: 10.1021/bi991162e
123. Zhao C, Ozaeta P, Fishpaugh J, Rupprecht K, Workman R, Grenier F, et al. Structural characterization of glycoprotein NGAL, an early predictive biomarker for acute kidney injury. Carbohydr Res (2010) 345(15):2252–61. doi: 10.1016/j.carres.2010.07.024
124. Borkham-Kamphorst E, Van de Leur E, Meurer SK, Buhl EM, Weiskirchen R. N-glycosylation of Lipocalin 2 is not required for secretion or exosome targeting. Front Pharmacol (2018) 9:426. doi: 10.3389/fphar.2018.00426
125. Fujiwara Y, Tsuchiya H, Sakai N, Shibata K, Fujimura A, Koshimizu TA. Lipopolysaccharide-induced inflammation or unilateral ureteral obstruction yielded multiple types of glycosylated Lipocalin 2. J Inflamm (Lond) (2016) 13:7. doi: 10.1186/s12950-016-0116-5
126. Song E, Fan P, Huang B, Deng HB, Cheung BM, Félétou M, et al. Deamidated lipocalin-2 induces endothelial dysfunction and hypertension in dietary obese mice. J Am Heart Assoc (2014) 3(2):e000837. doi: 10.1161/JAHA.114.000837
127. Bauvois B, Pramil E, Jondreville L, Chapiro E, Quiney C, Maloum K, et al. Relation of neutrophil gelatinase-associated lipocalin overexpression to the resistance to apoptosis of tumor B cells in chronic lymphocytic leukemia. Cancers (Basel) (2020) 12(8):2124. doi: 10.3390/cancers12082124
128. Nadarajan VS, Ang CH, Bee PC. Lipocalin-2 is associated with modulation of disease phenotype in a patient with concurrent JAK2-V617F and BCR-ABL mutation. Eur J Haematol (2012) 88(2):175–8. doi: 10.1111/j.1600-0609.2011.01712.x
129. Lv Z, Xu LY, Shen ZY, Zhang FR, Xu XE, Li EM. Overexpression of neutrophil gelatinase-associated lipocalin and its receptor in colorectal carcinoma: significant correlation with cell differentiation and tumour invasion. Oncol Lett (2010) 1(1):103–8. doi: 10.3892/ol_00000019
130. Cui L, Xu LY, Shen ZY, Tao Q, Gao SY, Lv Z, et al. NGALR is overexpressed and regulated by hypomethylation in esophageal squamous cell carcinoma. Clin Cancer Res (2008) 14(23):7674–81. doi: 10.1158/1078-0432.CCR-08-0420
131. Du ZP, Lv Z, Wu BL, Wu ZY, Shen JH, Wu JY, et al. Neutrophil gelatinase-associated lipocalin and its receptor: independent prognostic factors of oesophageal squamous cell carcinoma. J Clin Pathol (2011) 64(1):69–74. doi: 10.1136/jcp.2010.083907
132. Liu MF, Jin T, Shen JH, Shen ZY, Zheng ZC, Zhang ZL, et al. NGAL and NGALR are frequently overexpressed in human gliomas and are associated with clinical prognosis. J Neurooncol (2011) 104(1):119–27. doi: 10.1007/s11060-010-0486-0
133. Dekens DW, Naudé PJ, Engelborghs S, Vermeiren Y, Van Dam D, Oude Voshaar RC, et al. Neutrophil Gelatinase-Associated Lipocalin and its Receptors in Alzheimer's Disease (AD) Brain Regions: Differential Findings in AD with and without Depression. J Alzheimers Dis (2017) 55(2):763–76. doi: 10.3233/jad-160330
134. Chi Y, Remsik J, Kiseliovas V, Derderian C, Sener U, Alghader M, et al. Cancer cells deploy lipocalin-2 to collect limiting iron in leptomeningeal metastasis. Science (2020) 369(6501):276–82. doi: 10.1126/science.aaz2193
135. Liu F, Li N, Yang W, Wang R, Yu J, Wang X. The expression analysis of NGAL and NGALR in clear cell renal cell carcinoma. Gene (2018) 676:269–78. doi: 10.1016/j.gene.2018.08.060
136. Mao S, Jiang T, Shang G, Wu Z, Zhang N. Increased expression of neutrophil gelatinase-associated lipocalin receptor by interleukin-1β in human mesangial cells via MAPK/ERK activation. Int J Mol Med (2011) 27(4):555–60. doi: 10.3892/ijmm.2011.613
137. Zhang Y, Fan Y, Mei Z. NGAL and NGALR overexpression in human hepatocellular carcinoma toward a molecular prognostic classification. Cancer Epidemiol (2012) 36(5):e294–299. doi: 10.1016/j.canep.2012.05.012
138. Lee M, Kwon DY, Kim MS, Choi CR, Park MY, Kim AJ. Genome-wide association study for the interaction between BMR and BMI in obese Korean women including overweight. Nut Res Pract (2016) 10(1):115–24. doi: 10.4162/nrp.2016.10.1.115
139. Kim MJ, Kim JH, Kim MS, Yang HJ, Lee M, Kwon DY. Metabolomics associated with genome-wide association study related to the basal metabolic rate in overweight/obese Korean women. J Med Food (2019) 22(5):499–507. doi: 10.1089/jmf.2018.4310
140. Suzuki H, Nakamura Y, Matsuo K, Imaeda N, Goto C, Narita A, et al. A genome-wide association study in Japanese identified one variant associated with a preference for a Japanese dietary pattern. Eur J Clin Nutr (2021) 75(6):937–45. doi: 10.1038/s41430-020-00823-z
141. Visscher H, Rassekh SR, Sandor GS, Caron HN, van Dalen EC, Kremer LC, et al. Genetic variants in SLC22A17 and SLC22A7 are associated with anthracycline-induced cardiotoxicity in children. Pharmacogenomics (2015) 16(10):1065–76. doi: 10.2217/pgs.15.61
142. Cai Y, Wang X, Wang N, Wu J, Ma L, Xie X, et al. Correlations between tumor mutation burden and immune infiltrates and their prognostic value in pancreatic cancer by bioinformatic analysis. Life Sci (2021) 277:119505. doi: 10.1016/j.lfs.2021.119505
143. Shao S, Cao T, Jin L, Li B, Fang H, Zhang J, et al. Increased lipocalin-2 contributes to the pathogenesis of psoriasis by modulating neutrophil chemotaxis and cytokine secretion. J Invest Dermatol (2016) 136(7):1418–28. doi: 10.1016/j.jid.2016.03.002
144. Ma J, Chen J, Xue K, Yu C, Dang E, Qiao H, et al. LCN2 mediates skin inflammation in psoriasis through the SREBP2–NLRC4 axis. J Invest Dermatol (2022) 142(8):2194–2204.e2111. doi: 10.1016/j.jid.2022.01.012
145. Wei J, Gao X, Qin Y, Liu T, Kang Y. An iron metabolism-related SLC22A17 for the prognostic value of gastric cancer. Onco Targets Ther (2020) 13:12763–75. doi: 10.2147/ott.s287811
146. Wang M, Li Z, Peng Y, Fang J, Fang T, Wu J, et al. Identification of immune cells and mRNA associated with prognosis of gastric cancer. BMC Cancer (2020) 20(1):206. doi: 10.1186/s12885-020-6702-1
147. Guo X, Liang X, Wang Y, Cheng A, Zhang H, Qin C, et al. Significance of tumor mutation burden combined with immune infiltrates in the progression and prognosis of advanced gastric cancer. Front Genet (2021) 12:642608. doi: 10.3389/fgene.2021.642608
148. Miyamoto T, Asaka R, Suzuki A, Takatsu A, Kashima H, Shiozawa T. Immunohistochemical detection of a specific receptor for lipocalin2 (solute carrier family 22 member 17, SLC22A17) and its prognostic significance in endometrial carcinoma. Exp Mol Pathol (2011) 91(2):563–8. doi: 10.1016/j.yexmp.2011.06.00
149. Cao WH, Chen WP, Li J, Ye YH. Correlation of lipocalin-2 and its receptor expressions with preeclampsia. Zhonghua Fu Chan Ke Za Zhi (2012) 47(6):418–21.
150. Asaf S, Maqsood F, Jalil J, Sarfraz Z, Sarfraz A, Mustafa S, et al. Lipocalin 2-not only a biomarker: a study of current literature and systematic findings of ongoing clinical trials. Immunol Res (2023) 71:287–313. doi: 10.1007/s12026-022-09352-2
Keywords: NGALR, SLC22A17, Megalin, LRP2, MC4R, inflammation, iron
Citation: Schröder SK, Gasterich N, Weiskirchen S and Weiskirchen R (2023) Lipocalin 2 receptors: facts, fictions, and myths. Front. Immunol. 14:1229885. doi: 10.3389/fimmu.2023.1229885
Received: 27 May 2023; Accepted: 18 July 2023;
Published: 11 August 2023.
Edited by:
Masaki Hikida, Akita Univerity, JapanReviewed by:
Mario Ruiz, University of Gothenburg, SwedenKiyoshi Mori, Kyoto University Hospital, Japan
Copyright © 2023 Schröder, Gasterich, Weiskirchen and Weiskirchen. This is an open-access article distributed under the terms of the Creative Commons Attribution License (CC BY). The use, distribution or reproduction in other forums is permitted, provided the original author(s) and the copyright owner(s) are credited and that the original publication in this journal is cited, in accordance with accepted academic practice. No use, distribution or reproduction is permitted which does not comply with these terms.
*Correspondence: Ralf Weiskirchen, rweiskirchen@ukaachen.de
†These authors have contributed equally to this work