- 1Infection and Immunity Research Group (INIM), Institut Investigació Sanitària Pere Virgili (IISPV), Tarragona, Spain
- 2Infection and Immunity Research Group (INIM), Hospital Universitari de Tarragona Joan XXIII (HJ23), Tarragona, Spain
- 3Universitat Rovira i Virgili (URV), Tarragona, Spain
- 4Centro de Investigación Biomédica en Red de Enfermedades Infecciosas (CIBERINFEC), Instituto de Salud Carlos III, Madrid, Spain
- 5Lluita contra les Infeccions, Hospital Universitari Germans Trias i Pujol, Badalona, Spain
- 6Universitat Autònoma de Barcelona, Barcelona, Spain
- 7Universitat de Vic - Universitat Central de Catalunya, Vic, Spain
- 8Eurecat, Centre Tecnològic de Catalunya, Centre for Omic Sciences, Joint Unit Eurecat-Universitat Rovira i Virgili, Unique Scientific and Technical Infrastructure (ICTS), Reus, Spain
- 9HIV Unit, Hospital Clínic-Institut d'Investigacions Biomèdiques August Pi i Sunyer (IDIBAPS), University of Barcelona, Barcelona, Spain
- 10Department of Infectious Diseases, University Hospital Ramón y Cajal, Instituto Ramón y Cajal de Investigación Sanitaria (IRYCIS), Madrid, Spain
- 11Universidad de Alcalá (UAH), Madrid, Spain
Antiretroviral therapy (ART) induces persistent suppression of HIV-1 replication and gradual recovery of T-cell counts, and consequently, morbidity and mortality from HIV-related illnesses have been significantly reduced. However, in approximately 30% of people living with HIV (PLHIV) on ART, CD4+ T-cell counts fail to normalize despite ART and complete suppression of HIV viral load, resulting in severe immune dysfunction, which may represent an increased risk of clinical progression to AIDS and non-AIDS events as well as increased mortality. These patients are referred to as “immune inadequate responders”, “immunodiscordant responders” or “immune nonresponders (INR)”. The molecular mechanisms underlying poor CD4+ T-cell recovery are still unclear. In this sense, the use of omics sciences has shed light on possible factors involved in the activity and metabolic dysregulation of immune cells during the failure of CD4+ T-cell recovery in INR. Moreover, identification of key molecules by omics approaches allows for the proposal of potential biomarkers or therapeutic targets to improve CD4+ T-cell recovery and the quality of life of these patients. Hence, this review aimed to summarize the information obtained through different omics concerning the molecular factors and pathways associated with the INR phenotype to better understand the complexity of this immunological status in HIV infection.
1 Introduction
Effective lifelong antiretroviral therapy (ART) substantially reduces the incidence of acquired immunodeficiency syndrome (AIDS)-related morbidity and mortality in people living with HIV (PLHIV) and transforms HIV infection into a chronic disease by reducing viraemia to undetectable levels and increasing CD4+ T-cell counts in peripheral blood (1). However, approximately 30% of ART-treated PLHIV individuals do not achieve optimal CD4+ T-cell reconstitution despite achieving complete suppression of HIV replication. They are termed nonresponders, poor immunological recoverees (PIR), discordant responders or immunological nonresponders (INR) (2). There are no universal criteria for INR definition, but several factors have been traditionally linked to INR phenotype, such as age, male sex, drug consumption, late ART introduction, or cytokine storm. The clinical risk is intrinsically related to the current CD4+ T-cell count, and of note, the World Health Organization (WHO) considers PLHIV with CD4+ T-cell counts less than 200 cells/µL to be the development of advanced HIV disease (AHD) (2–5). Thus, INR are at higher risk of morbidity and mortality for both AIDS and non-AIDS events compared to HIV-infected individuals who have achieved complete immune reconstitution (6, 7), representing an important barrier to achieving the UNAIDS goals to end the AIDS epidemic by 2030 (6). And although much research has been focused on CD4+T-cell reconstitution on ART, the biological and molecular mechanisms determining whether an individual will become an INR are not well understood.
The underlying mechanisms of the INR condition are complicated and not limited to a low CD4+ T-cell count (Figure 1A). INR is characterized by severe immune dysfunction that includes malfunctioning and decreased cell production in lymphopoietic tissue, altered frequencies of immune regulators such as regulatory T cells and Th17 cells, residual viral replication, T-cell exhaustion and senescence, perturbation of cytokine secretion, mitochondrial disruption, gut dysbiosis, and specific genetic or metabolic characteristics (7, 15–18). However, none of these independent factors can fully explain the mechanism of INR, which seems to be multifactorial. And in this sense, omics sciences have become a powerful tool for obtaining information in different fields, such as genetics, proteomics, or metabolomics, through reproducible quantification of several molecules in different bio-organic fluids and biological samples. Integration of all these molecules with other immunological, molecular, and biochemical data results in deep knowledge of the behavior of the cells in both physiological and pathological states (19) and allows for the identification of many immunotherapeutic targets for the development of new therapies (20). For this reason, this review aimed to collect the main information obtained in different omics studies carried out on the INR phenotype that will help to advance knowledge on the pathophysiology of poor immunological recovery in PLHIV and to gain insights that will help in the generation of new interventional therapeutic strategies.
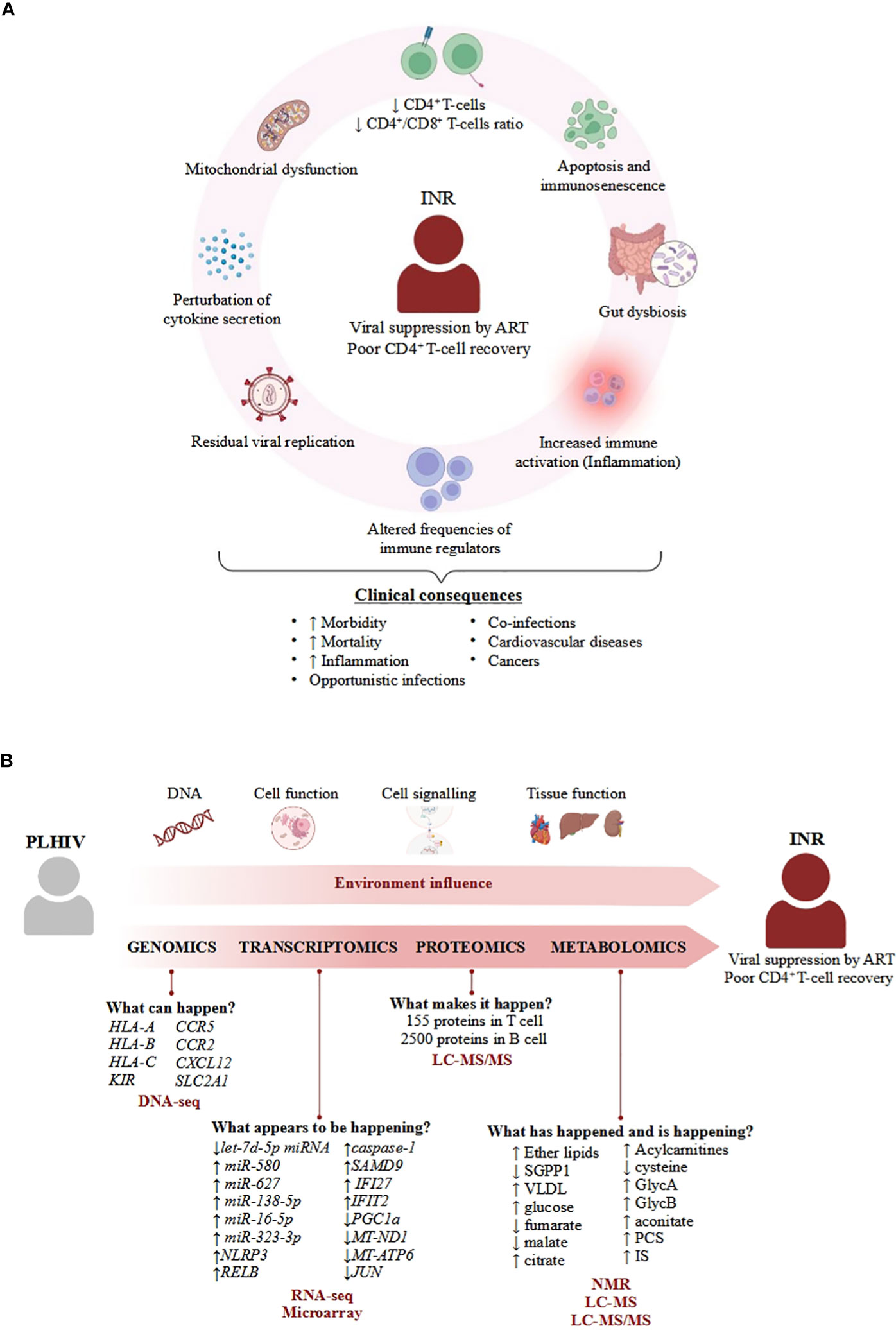
Figure 1 Overview of the physiological and molecular features of INR condition. (A) Important processes describing INR that enhance clinical consequences. The mechanisms of the INR condition are characterized by a set of biological processes that eventually lead to different pathologies such as cancers (8, 9), cardiovascular diseases (10), opportunistic infections (11, 12) or other coinfections (13, 14), contributing to the increased mortality and morbidity associated to INR condition. (B) Overview of applicability of omics science in INR. Omics can answer biological questions of different layers of knowledge to identify factors that confer a specific environment in the INR phenotype in PLHIV, such as 1) understanding the influence of several SNPs in the specific response to infection; 2) analysing perturbations of cell function under infection; 3) how cell communication activates the immune system; and 4) how the coordination among cells allows tissues to perform their function correctly. The combination of these factors provided by different omics adds complexity to understanding the real causes of poor CD4+ T-cell recovery in INR patients, even though these patients achieve viral suppression by antiretroviral therapy (ART). Each molecule associated with the INR phenotype is listed below with the omics approach used to identify it, as well as the different techniques (in red bold) used in the different studies identified in this review.
2 Genomics: the blueprint for an INR phenotype
Genomics refers to the study of the total or part of the genetic sequence information and chemical modifications of an organism, respectively, which includes the identification of key genes and functional elements (21). The use of genomic analysis allows for a better understanding of the molecular mechanisms involved in health interventions and environmental factors in disease, which can be widely used for developing future therapeutic strategies (21). Concretely, different single-nucleotide polymorphisms (SNPs) in genes have been related to some molecular processes, such as T-cell expansion, lymphocyte migration, the cell cycle, survival, and apoptosis, which in turn are associated with the magnitude of CD4+ T-cell count recovery during ART (Figure 1B) (22–26).
For instance, several SNPs in human leukocyte antigen (HLA) class I have been associated with lower CD4+ T-cell counts. HLA class I is formed by 20 genes classified into A, B, and C subtypes, and their function is to activate immune cells by presenting pathogen-derived peptides present in the membrane of cells to T lymphocytes (27). El-Beeli et al. reported that PLHIV with poor CD4+ T-cell recovery, characterized by low levels of nadir CD4+ T-cells (<200 cells/ul compared with 300 cells/ul of IR), showed a significantly higher proportion of HLA-A68 and HLA-B15 alleles (Table 1) (28). HLA-B13 was correlated with the highest risk of immune failure because of its association with less than 100 cells/ul of nadir CD4+ T-cells before ART and with not exceeding 300 cells/ul after 2 years of ART. (Table 1) (29). Moreover, while the homozygous HLA-Bw6 epitope promotes CD4+ T-cell recovery, the homozygous HLA-Bw4 epitope was associated with poor CD4+ T-cell recovery once ART was initiated (Table 1) (52). In contrast, the HLA-Bw4 epitope has been associated with suppression of HIV-1 viraemia and the maintenance of a normal CD4+ T-cell count in HIV patients not receiving ART. The causes of this different role of the HLA-Bw4 epitope depending on the presence or absence of ART are still unclear (53). Moreover, interaction of HLA class I with killer immunoglobulin-like receptors (KIR), which are present on the surface of natural killer (NK) cells, modulates their activity and is associated with a specific immune response (54). Along this line, the study of Soria et al. investigated the different roles of KIR gene polymorphisms and their HLA class I ligands related to their effect on the immunological response to ART. They postulated that INRs showed higher rates of lower allele frequencies of KIR2DL3 and KIR2DS2 than in complete responders. A high frequency of KIR2DL3 is related to a lower inhibitory potential and therefore better NK activation, resulting in more efficient control of viral infection. Of note, a low allele frequency of HLA-Cw*04:01 was found in INRs, and hence, the KIR2DS2+/HLA-Cw*04:01+ multi-allele complex was highly associated with poor recovery of CD4+ T-cells (Table 1) (30).
In addition, cytokines, chemokines, and their receptors play a key role in HIV infection by activating the immune system and acting as factors related to HIV pathogenesis (55). For instance, C-C chemokine receptor type 5 (CCR5), which is expressed on the surface of lymphocytes and other cell types, plays a key role in HIV infection due to its major involvement in virus entry and cell-to-cell spread (56). The delta-32 (CCR5Δ32) mutation affecting CCR5 that causes a deletion in the receptor sequence prevents entry of the virus into a cell, and consequently, homozygous delta-32 individuals are substantially resistant to HIV infection (57). PLHIV carrying the heterozygous CCR5Δ32 mutation also had a significantly lower CD4+ T-cell count at pre-treatment and insufficient recovery of CD4+ T-cell count during ART compared to those homozygous CCR5Δ32 individuals and with those without the mutation (Table 1) (31). Therefore, aberrant CCR5 protein blocks the ability to co-stimulate CD4+ T-cell activation by inducing T-cell proliferation and differentiation (58, 59). Additionally, several SNPs in genes encoding cytokines and chemokines and their receptors are important in HIV infection and associated with inflammatory INR status, such as IFNγ rs2430561, IL19 rs2243191, CXCL12 (SDF-1) rs1801157, MCP-1 (CCR2) rs1799864_814, and fractalkine (CX3CR1) rs373278_814 and rs3732379. CCR2 rs1799864-AG and CXCL12 rs1801157-TT genotypes are considered prognostic markers of poor immunological recovery, whereas the CX3CR1 249I polymorphism is related to earlier immune failure (Table 1) (32–34, 60).
To activate the immune system, Toll-like receptors (TLRs), a family of type I transmembrane pattern recognition receptors (PRRs), are important due to their capacity to initiate the innate immune response by sensing conserved molecular patterns for early immune recognition of a pathogen or endogenous molecules (61). Specifically, the SNP TLR91635AA has been associated with lower CD4+ T-cell counts and higher immune activation of CD4+ and CD8+ T-cells, which is related to higher plasma levels of interferon-inducible protein 10 (IP10), suggesting a link between the TLR9 polymorphism and inflammation (62). Moreover, not only inflammation but also metabolic disorders are involved in the immune response, as glucose metabolism is important for the proper function of CD4+ T cells through the glucose transporter GLUT1 (63). The GLUT1 gene is up-regulated by the activation of the phosphoinositide 3-kinase (PI3K)/protein kinase B (AKT)/mammalian target of rapamycin (mTOR) pathway (PI3K/AKT/mTOR) by IL-7 (64). The up-regulation of the SLC2A1 gene, which encodes GLUT1, promotes the growth and differentiation of these cells through increasing glucose uptake and the activation of glycolysis (63). In a cohort of PLHIV receiving ART, the polymorphism rs1385129 SLC2A1 allele, which does not change the amino acid sequence, was significantly associated with lower counts and slower recovery of CD4+ T-cells (Table 1) (35). In fact, the homozygous rs1385129 SLC2A1 allele and the rs1130214 AKT allele were associated with the major presence of GLUT1 receptor and increased glycolytic activity in CD4+ T-cells.
Remarkably, mitochondrial DNA also seems to be involved in the CD4+ T-cell count recovery. The mitochondrial haplogroup is defined as a population that shares a similar mtDNA sequence. There are four major haplogroups, HV, U, JT, and IWX, and several minor ones such as H, V, J, and T (65). Slow AIDS progression is associated with haplogroup H and better CD4+ T-cell reconstruction, whereas haplogroups J and T are associated with poor CD4+ T-cell recovery (66, 67).
In 2019, Greenblatt et al. performed whole-exome genome sequence analysis on 48 women with good CD4+ T-cell recovery compared to 42 INR to find exome sequence variations associated with CD4+ T-cell recovery. In this study, 41 genes harboring variations were detected using combined multivariate and collapsing (CMC) and kernel-based adaptive collapsing (KBAC) methods. Interestingly, 11 genes associated with CD4+ recovery had unknown functions (68), which is evidence that more studies related to genetic function are required to understand their role in patients with INR status.
3 Transcriptomics: the regulation of INR blueprint
Transcriptomic studies focus on exploring the expression of the genome. These studies quantify RNA molecules under specific conditions due to the fact that gene profiles are affected by different factors such as the stage of development, physiological and environmental factors, and different pathologies (69).
Many studies in recent years have revealed that, due to their chemical stability and anti-RNase action, endogenous circulating miRNAs are reliable blood biomarkers of disease progression (Figure 1B). In this regard, miRNAs play key roles related to the regulation of different viral diseases, including HIV, affecting many developmental pathways and cellular processes (70). Through analysis of the different miRNAs profiles in INR compared to immunological responders (IR) before and after 24 months of viral suppression (VS), researchers identified 299 miRNAs at baseline and 321 after 24 months of VS that were differentially expressed between INR and IR groups. All these miRNAs affect the function of genes involved in several biological processes, such as cell binding and cellular and organelle structure (36). Remarkably, Fu et al. found that expression of the let-7d-5p miRNA was significantly down-regulated in the INR group compared to the IR group, and they proposed the let-7d-5p miRNA as a good biomarker to predict INR condition (Table 1) (36). Interestingly, miRNA let-7d-5p is a member of the miRNA let-7 family, which is involved in a wide range of human physiological activities, such as cell development and differentiation and/or regulation of the immune response (71). Specifically, some members of the let-7 family can act as regulators of erythroid differentiation (72). Likewise, erythroid cells that express V-domain Immunoglobulin (Ig) Suppressor of T Cell Activation (VISTA) on their surface promote development of regulatory T cells (Tregs) through cytokine TGF-β production (73). This fact might explain the relation between down-regulation of let-7d-5p miRNA and poor recovery of CD4+ T cells in INR.
Another study by Lv et al. identified a panel of five up-regulated miRNAs whose expression negatively correlated with CD4+ T-cell count after one year on ART (37). The authors of this study proposed a panel of these five miRNAs (miR-580, miR-627, miR-138-5p, miR-16-5p, and miR-323-3p) as a good biomarker to predict a poor immune response to ART, obtaining a model with 92.9% sensitivity and 91.3% specificity (Table 1) (37). Of note, upregulation of miR-627 reduces proliferation of CD4+ T-cells through regulation of Ca2+ transport, which plays a key role in CD4+ T-cell development (37, 74). Also, miR-323-3p can act for itself as a biomarker related to the characteristic failure of immune cell recovery in INR patients (75). Similarly, up-regulation of NLRP3 and caspase-1 expression has been associated with immunological nonresponse (76). NLRP3 is a component of the inflammasome complex, and it is essential for activation of the innate immune system against a pathogen (77). In the study of Bandera et al., NLRP3 triggering caspase-1 activation and IL-1β cytokine secretion resulted in inflammatory and pyroptotic cell death, which could explains the failure of immune recovery and the chronic inflammatory state of INR (76).
Liu et al. used RNA-seq analysis to identify 316 differentially expressed genes in PBMCs among INR patients (Table 1) (38). A total of 170 genes were down-regulated and 46 were up-regulated in INR compared to IR, and their significant function was related to the type I interferon (IFN) signaling pathway, the mitotic cell cycle process, and the immune response (38). Interestingly, the authors emphasized the function of IFI27, with expression that correlated negatively with CD4+ T-cell counts, and obtained a good score as a biomarker to distinguish INR from IR patients, as shown in another study (78). Likewise, Younes et al. found up-regulation of proapoptotic genes IFI27 andIFIT2 and down-regulation of PGC1α (the master regulator of mitochondrial biogenesis) in cycling CD4+ T-cells of INR compared with IR (38). Cycling memory CD4+ T-cells are characterized by Ki67 and CD71+ expression (markers of cell cycle activation), and their frequency increases despite if total CD4+ T-cell number is lower in INR than IR, showing a disruption of CD4+ T-cell homeostasis (39). Of note, the down-regulation of PGC1α promotes decreased OXPHOS and up-regulation of glycolysis (Table 1) (39). Other studies also correlate INR phenotype with mitochondrial dysfunction and activation of apoptosis or senescence in CD4+ T-cells. Ghneim et al. classified INR in two subgroups, INR-A and INR-B, based on transcriptomic profile, despite the fact that all these patients showed similar CD4+ nadir and count, inflammatory and gut barrier dysfunction markers, age, and years on ART. Concretely, INR-B and IR showed similar transcriptomic profiles, whereas 3000 differentially expressed genes, most of them down-regulated, were found in INR-A. These downregulated genes are involved in cell cycling and metabolism, and for this reason, the authors named this group senescent-INR. Specifically, IRF3 activation, reactive oxygen species (ROS) production regulated by FOXO, TGFβ signaling, inhibition of NF-κB activation, oxidative phosphorylation pathways (NDUFs and COXs) and down-regulation of proliferation by inhibition of MYC targets were associated with senescent-INR. In contrast, the transcriptomic profile of INR-B showed up-regulation of pro-inflammatory factors such as NFKB1, NFKB2, and RELB, the inflammasome complex (IL1B and IL18), for which INR-B called Inflammatory-INR (40). Recently, single-cell RNA-seq analysis of PBMCs from two IR and two INR differentially detected 1445 genes in INRs and 1384 in IRs. Specifically, in mucosal-associated invariant T (MAIT) cells, the up-regulation of proapoptotic genes (IFIT2 and SAMD9) and down-regulation of the expression of regulators of T-cell function (KLRB1 and JUN) and mitochondrial biogenesis (MT-ND1, MT-ATP6 and RPS26) were observed. In cytotoxic CD4+ T-cells, 440 DEGs related to the IFN-γ pathway, leukocyte adhesion, and the regulation of hemopoiesis. In contrast, in CD8+ effector T cells were identified DEGs related to antigen processing and presentation and the IFN-γ pathway (41). All these findings suggest that the transcriptional regulation of the senescence/apoptosis program, mitochondrial function, and glycolysis activation are associated with the lack of CD4+ T-cell reconstitution. More work on differential gene expression has been performed to understand poor CD4+ T-cell recovery. For example, Woelk et al. found that 40 genes with significantly different expression levels between INR and IR groups had a 10% predictive power to distinguish PLHIV who would experience the ‘good’ or ‘poor’ CD4+ T-cell recovery (Table 1) (42).
4 Proteomics: the means to execute the INR blueprint
Proteomics provides a better understanding of the structure and function of the organism than genomics or transcriptomics. This analysis identifies and quantifies the proteome in a specific cell type or tissue at a precise developmental or cellular stage (79). Several translational modifications and diverse patterns of protein expression in different organs and tissues add complexity when interpreting proteomics results (80) as do internal and external stimuli such as nutritional status, exercise, or diseases that affect proteomic dynamics (81). High-throughput methods to identify proteins include protein array and mass spectrometry (MS) techniques, but because of the higher accuracy and sensitivity of MS measurements and the advanced software used for data analysis, MS is the preferred technique among proteomics approaches (82). The use of proteomics has allowed for the identification and monitoring of biomarkers related to different aspects of HIV infection, including CD4+ T-cell reconstitution failure (83). Recent studies provide a comprehensive map of protein interactions associated with INR, which, in turn, facilitates the development of new diagnostic and treatment opportunities (Figure 1B).
In 2016, a study used LC−MS/MS on CD4+ T-cells from healthy controls (HIV-negative controls) and found an altered T-cell proteome profile in both IR and INR. Azzam et al. identified some deregulated signaling pathways and biological processes, such as translation, cellular stress, eIF2-alpha, mTOR and actin cytoskeleton pathways, in INR. A total of 153 proteins were found to vary significantly between the INR and IR groups, and 99 proteins were significantly altered between the INR and healthy control groups (Table 1). These findings showed that the cell cycle and apoptosis were altered in immune recovery failure during ART (43). Moreover, a recent study analyzing the baseline proteome of B cells from patients with acute HIV infection showed approximately 2500 significant proteins differentiated among groups. Specifically, the TGF-β, NF-κB and CD35 proteins were highly altered in INR compared to IR and healthy controls (Table 1) (44). The enormous number of altered proteins found in this study suggests that dysregulation of B cells in HIV patients may serve as a predictor of poor CD4+ T-cell recovery.
The applicability of high-throughput methods related to INR studies is quite new; hence, in this review, some interesting studies that have employed low-throughput methods, such as ELISA or western blotting, to identify proteins that are potential biomarkers in INR are included. For example, CD5 levels were found to be lower in INR than in IR, which was associated with a lower capacity to respond to immune activation as this protein is a specific antigen of the immune system (84). This study also demonstrated that a combination of low levels of TRANCE and CD5 with high levels of CDCP1, CXCL11, CT5 and SLAMF1 may be used to discriminate INRs from IRs.
Otherwise, the chronic infection and systemic inflammation characteristics of PLHIV result in persistent activation of the immune system, affecting cytokine secretion. Accordingly, it has been shown that IL-2, IL-4, IL-10, IL-17, and IFN-γ levels are significantly lower in INR than in IR (15, 85). These cytokines are strongly involved in T-cell activation and proliferation, and their decrease might lead to their depletion (43, 44, 83). Interestingly, Meyer et al. showed that IL-17 levels and CD4+ T-cell counts were negatively correlated with high levels of I-FABP and REG3α in INR individuals compared with IR. I-FABP and REG3α have been described as enterocyte damage markers; hence, mucosal immune dysfunction is a factor that may contribute to the INR condition (86). In addition, previous findings have shown that chronic immune activation and ongoing HIV replication may contribute to the persistence of T-cell dysfunction in patients with poor CD4+ T-cell recovery (15, 85). To explore the immunological alterations present before ART initiation, Rosado-Sánchez et al. proposed that elevated IL-6 cytokine levels characterize immune damage in subjects with low CD4+ T-cell recovery before ART (87). Therefore, these unfavorable immunologic characteristics may be key to helping clinicians personalize treatment strategies and improve long-term immune recovery outcomes. Other important cytokines that play a key role in immune function in the INR phenotype are adipokines, which are secreted by adipose tissue and produce both proinflammatory and anti-inflammatory effects (88). Because low APLNR and RBP4 concentrations were found to be associated with poor immune recovery, these adipokines were proposed as potential biomarkers of the INR phenotype (89).
5 Metabolomics: the real-time processes occurring in INR
Metabolomics is defined as the comprehensive, qualitative, and quantitative study of low-molecular-weight molecules in an organism or biological sample. Unlike genomic and proteomics methods, metabolomics measures endogenous metabolism (metabolomics) as well as perturbations of metabolism caused by environmental factors and pathophysiological processes, offering a snapshot of the physiology of the cell most closely related to the phenotype (90, 91). In general, two types of experimental workflows can be applied in metabolomics: untargeted studies, which are used to identify a wide range of metabolites (profiling) to generate a hypothesis; and targeted studies, which focus on a few specific metabolites when a hypothesis is already postulated. The two most frequently used analytical platforms are liquid (LC) and gas (GC) chromatography coupled to mass spectrometry (MS) and nuclear magnetic resonance (NMR) spectroscopy (Figure 1B) (92).
In 2016, Scarpellini B et al. used targeted MS/MS and postulated that the number of ether lipids, as measured by the total acyl-alkyl-containing phosphatidylcholines to total phosphatidylcholines (AGPS) ratio, is up-regulated after one year of follow-up in INRs when a significant decline in sphingosine‐1‐phosphate phosphatase 1 activity (SGPP1, SYNE2 locus) and β-oxidation is also observed (Table 1) (45). On the one hand, sphingosine-1-phosphate (S1P) is involved in lymphocyte release into circulation; on the other hand, ether lipids dynamically participate in signal transduction pathways (93, 94). Finally, the authors proposed a combination of five different metabolites and ratios to identify rapid progressors and INR at baseline, with 88.89% sensitivity, 92.31% specificity, 88.89% positive predictive value, and 92.31% negative predictive value (45).
In 2018, NMR analysis revealed high baseline (pre-ART) ratios of non-HDL lipoprotein particles, high levels of VLDL particles, and high concentrations of glucose related to the INR phenotype (46). The positive correlation between medium VLDL particles and ratios of ‘total/HDL particles’ and ‘LDL/HDL particles’ supports the negative link between the ‘LDL/HDL particle’ ratios and CD4+ T-cell recovery already reported (Table 1) (95). Moreover, the association between plasma glucose levels and low CD4+ T-cell counts corroborates previous data, indicating increased glycolytic metabolism in CD4+ T-cells because activated immune cells consume glucose at an extremely high rate in PLHIV (96). Of interest, using the same study cohort, the authors evaluated lipoprotein profiles at months 12 and 36 after initiation of ART (47). Both large and small HDL-P concentrations were significantly increased during ART in INR, suggesting that the proportion of small particles and large particles is involved in an atherogenic environment related to cardiovascular risk. Additionally, this follow-up study confirmed the negative correlation between CD4+ T-cell counts and medium HDL-P concentrations and corroborated the immunomodulatory role of HDL particles as well as their controversial function in cardiovascular diseases (Table 1) (47).
More recently, an untargeted metabolomics experimental design revealed that acylcarnitines, which are fatty acid metabolites synthesized by the combination of carnitine and acyl-CoA (activated fatty acid) and transported into mitochondria, are elevated in INR (48, 97). The authors suggested that an increase in acylcarnitine levels may be related to impaired mitochondrial translocation activity via promotion of β-oxidation, which might induce apoptosis in CD4+ T-cells and lead to elevated oxidative stress or membrane disruption (48). Furthermore, regression model combining myristoylcarnitine (MC), palmitoylcarnitine (PC), stearoylcarnitine (SC) and oleylcarnitine (OC) obtained the best discriminatory power to distinguish INRs from IRs with high sensitivity and specificity rates (Table 1) (48). In another study, 127 significant metabolites were associated with INR. The citrate and aconitate levels increased, whereas the precursors of NAD (nicotinamide), fumarate, and malate significantly decreased in INR samples compared to IR. Moreover, Ferrari et al. detected alterations in amino acid metabolism, sphingomyelin and phospholipids in the INR group. All these data suggest mitochondrial dysfunction and a reduction in TCA cycle activity. Also, the gut-derived bacterial solutes p-cresol sulfate (PCS) and indoxyl sulfate (IS) were found to increase and be negatively correlated with CD4+ T-cell counts. Of note, CD4+ T-cells in the presence of PCS and IS stop the cell cycle and down-regulate COXIV and mTFA (mitochondrial proteins), producing inhibition of proliferation and cell death (49). Moreover, Nyström et al. identified more than 200 metabolites associated with HIV recovery using the LC−MS technique (50). They postulated that high levels of cysteine correlate positively with CD4+ T-cell numbers and are associated with serine and glycine metabolism, which are important for maintaining redox balance in CD4+ T cells (Table 1) (50, 98). Overall, the low levels of cysteine in the INR group might be associated with poor CD4+ T-cell recovery.
Of interest, glycomics is the subset of metabolomics that aims to identify the structure and function of the complete set of glycans (the glycome) that play a crucial role in modulation of the innate and adaptive immune systems, inflammation, and pathological processes (99). In the study of Malo et al. (51), glycoprotein concentrations in the same study cohort were determined by H-NMR via protein bond N-acetylglucosamine and N-acetylgalactosamine (GlycA) signals and the N-acetylneuraminic acid signal (GlycB) associated with the sugar–protein bond concentration and aggregation state shapes (height and width) (Table 1). Additionally, the combination of glycoprotein Groups A and B plus H/W ratios could predict the immunological recovery condition at baseline (51).
6 Conclusions
The findings from different omic studies collected in the present review suggest that four main pathways are involved in controlling CD4+T-cell fates. Cell cycle, apoptosis and senescence, mitochondrial function, and OXPHOS/Glycolysis are the pathways associated with the poor CD4+ T-cell recovery of the INR phenotype. Specifically, several transcriptomic and proteomic analyses claimed that high levels of IFI27 and TGF-β, inhibition of NF-κB and Myc target genes, and actin cytoskeleton down-regulation are associated with low CD4+ T-cell count by activation of apoptosis and senescence programs. In addition, the down-regulation of PGC1α, MT-ND1, MT-ATP6, and RPS26, the activation of the PI3K/AKT/mTOR pathway, the high presence of GLUT1 in CD4+ T-cell, and alteration in amino acid metabolism, sphingomyelin, and phospholipid levels indicate that TCA activity is reduced by activation of glycolysis instead of OXPHOS producing mitochondrial dysfunction. Mitochondrial dysfunction causes cell exhaustion that reduces the capacity for proliferation and differentiation and the immune response to pathogens, which would explain the poor recovery of the immune cells. Although this review identified several factors and pathways associated with the INR phenotype, it also shows that more integrative studies are required to better understand the causes of poor CD4+ T-cell recovery. Indeed, this is one of the great challenges of omics application, i.e., the management and integration of all these data for a correct biological interpretation.
7 Limitations and future perspectives
There are no universal criteria for the immunological nonresponse definition. The high disparity in INR criteria makes it difficult to compare data from different studies; thus, standard terms and criteria used by both HIV clinical staff and the research community will be of great interest. Additionally, demographic data such as sex differences, age, or other confounding variables is important for interpreting and integrating different omics data, as phenotype is critically conditioned by environmental influence. Metabolomics (metabolome), which is directly related to phenotype, reflects genetic (genome) and protein (proteome) activity and provides a real-time view of dynamic changes because metabolomics quantifies hundreds of small molecules. Hence, the accuracy of the biological and molecular pathways defined by knowledge of environmental factors is crucial for understanding immunological non-response. Moreover, omics produces a large amount of data that helps investigators identify possible targets, but validation studies are also required to assess the biological function related to developing specific INR therapies. Finally, metabolomics has continually grown, and it has been applied to different sample types where bacterial metabolites may be present. In fact, as suggested through the present review, INR condition seems to be highly related to gut dysbiosis. Nonetheless, microbiome effects on the host metabolome in INR were not included in the present review, as we consider that the microbiome/host metabolism provides a framework for investigation and deserves a more specific review.
Author contributions
SE, MF-P, SC, AR and JP participated in the conception and design of the review. CV, EN, JM, BV, SM and FV provided scientific discussion. SE, MF-P, SC and AR wrote the manuscript. SF-A, EN, JM, SM, FV and JP revised the manuscript. All authors read and approved the final version of the manuscript.
Funding
This research was funded by the Fondo de Investigacion Sanitaria [PI19/01337 and PI20/00326]-ISCIII-FEDER (co-funded by the European Regional Development Fund/European Social Fund; “A way to make Europe”/”Investing in your future”); the Programa de Suport als Grups de Recerca AGAUR (2021SGR01404) and the CIBER -Consorcio Centro de Investigación Biomédica en Red- (CB21/13/00020, CB21/13/00063, CB21/13/00086, CB21/13/00094), Instituto de Salud Carlos III, Ministerio de Ciencia e Innovación and Unión Europea – NextGenerationEU. FV is supported by grants from the Programa de Intensificacioín de Investigadores (INT20/00031)-ISCIII and by “Premi a la Trajectòria Investigadora dels Hospitals de l’ICS 2018”. AR is supported by a grant from IISPV through the project “2019/IISPV/05” (Boosting Young Talent), by GeSIDA through the “III Premio para Joívenes Investigadores 2019” and by the Instituto de Salud Carlos III (ISCIII) under grant agreement “CP19/00146” through the Miguel Servet Program. SE is supported by the Instituto de Salud Carlos III (ISCIII) under grant agreement “FI21/00210” through the programme “Contratos Predoctorales de Formación en Investigación en Salud”.
Conflict of interest
The authors declare that the research was conducted in the absence of any commercial or financial relationships that could be construed as a potential conflict of interest.
Publisher’s note
All claims expressed in this article are solely those of the authors and do not necessarily represent those of their affiliated organizations, or those of the publisher, the editors and the reviewers. Any product that may be evaluated in this article, or claim that may be made by its manufacturer, is not guaranteed or endorsed by the publisher.
References
1. Fanales-Belasio E, Raimondo M, Suligoi B, Buttò S. HIV virology and pathogenetic mechanisms of infection: a brief overview. Ann Ist Super Sanita (2010) 46:5–14. doi: 10.4415/ANN_10_01_02
2. Yang X, Su B, Zhang X, Liu Y, Wu H, Zhang T. Incomplete immune reconstitution in HIV/AIDS patients on antiretroviral therapy: Challenges of immunological non-responders. J Leukoc Biol (2020) 107:597–612. doi: 10.1002/JLB.4MR1019-189R
3. Portilla-Tamarit J, Reus S, Portilla I, Fuster Ruiz-de-Apodaca MJ, Portilla J. Impact of advanced HIV disease on quality of life and mortality in the era of combined antiretroviral treatment. J Clin Med (2021) 10:716. doi: 10.3390/jcm10040716
4. Calmy A, Ford N, Meintjes G. The persistent challenge of advanced HIV disease and AIDS in the era of antiretroviral therapy. Clin Infect Dis Off Publ Infect Dis Soc Am (2018) 66:S103–SS105. doi: 10.1093/cid/cix1138
5. Bono V, Augello M, Tincati C, Marchetti G. Failure of CD4+ T-cell Recovery upon Virally-Effective cART: an Enduring Gap in the Understanding of HIV+ Immunological non-Responders. New Microbiol (2022) 45:155–72.
6. AIDSinfo | UNAIDS. Available at: https://aidsinfo.unaids.org/ (Accessed May 24, 2023).
7. Marchetti G, Gazzola L, Trabattoni D, Bai F, Ancona G, Ferraris L, et al. Skewed T-cell maturation and function in HIV-infected patients failing CD4+ recovery upon long-term virologically suppressive HAART. AIDS Lond Engl (2010) 24:1455–60. doi: 10.1097/QAD.0b013e328339cf40
8. Franceschi S, Maso LD, Rickenbach M, Polesel J, Hirschel B, Cavassini M, et al. Kaposi sarcoma incidence in the Swiss HIV Cohort Study before and after highly active antiretroviral therapy. Br J Cancer (2008) 99:800–4. doi: 10.1038/sj.bjc.6604520
9. Clifford GM, Franceschi S. Cancer risk in HIV-infected persons: influence of CD4(+) count. Future Oncol Lond Engl (2009) 5:669–78. doi: 10.2217/fon.09.28
10. Lichtenstein KA, Armon C, Buchacz K, Chmiel JS, Buckner K, Tedaldi EM, et al. Low CD4+ T cell count is a risk factor for cardiovascular disease events in the HIV outpatient study. Clin Infect Dis Off Publ Infect Dis Soc Am (2010) 51:435–47. doi: 10.1086/655144
11. Shete A, Dhayarkar S, Sangale S, Medhe U, Panchal N, Rahane G, et al. Incomplete functional T-cell reconstitution in immunological non-responders at one year after initiation of antiretroviral therapy possibly predisposes them to infectious diseases. Int J Infect Dis IJID Off Publ Int Soc Infect Dis (2019) 81:114–22. doi: 10.1016/j.ijid.2019.01.017
12. Shenoy N, Ramapuram JT, Shenoy A, Ahmed J, Srikant N. Incidence of opportunistic infections among HIV-positive adults on highly active antiretroviral therapy in a teaching hospital, India: prospective study. J Int Assoc Provid AIDS Care (2017) 16:309–11. doi: 10.1177/2325957416686192
13. Wandeler G, Gsponer T, Bihl F, Bernasconi E, Cavassini M, Kovari H, et al. Hepatitis B virus infection is associated with impaired immunological recovery during antiretroviral therapy in the Swiss HIV cohort study. J Infect Dis (2013) 208:1454–8. doi: 10.1093/infdis/jit351
14. Potter M, Odueyungbo A, Yang H, Saeed S, Klein MB, Canadian Co-infection Cohort Study Investigators. Impact of hepatitis C viral replication on CD4+ T-lymphocyte progression in HIV-HCV coinfection before and after antiretroviral therapy. AIDS Lond Engl (2010) 24:1857–65. doi: 10.1097/QAD.0b013e32833adbb5
15. Erikstrup C, Kronborg G, Lohse N, Ostrowski SR, Gerstoft J, Ullum H. T-cell dysfunction in HIV-1-infected patients with impaired recovery of CD4 cells despite suppression of viral replication. J Acquir Immune Defic Syndr 1999 (2010) 53:303–10. doi: 10.1097/QAI.0b013e3181ca3f7c
16. Bernardes SS, Borges IK, Lima JE, Milanez P de AO, Conchon-Costa I, Felipe I, et al. Involvement of regulatory T cells in HIV immunopathogenesis. Curr HIV Res (2010) 8:340–6. doi: 10.2174/157016210791208613
17. Hunt PW. Th17, gut, and HIV: therapeutic implications. Curr Opin HIV AIDS (2010) 5:189–93. doi: 10.1097/COH.0b013e32833647d9
18. Piconi S, Trabattoni D, Gori A, Parisotto S, Magni C, Meraviglia P, et al. Immune activation, apoptosis, and Treg activity are associated with persistently reduced CD4+ T-cell counts during antiretroviral therapy. AIDS Lond Engl (2010) 24:1991–2000. doi: 10.1097/QAD.0b013e32833c93ce
19. What Are Omics Sciences? SpringerLink. Available at: https://link.springer.com/chapter/10.1007/978-3-319-43033-1_1 (Accessed May 23, 2023).
20. Hu Z-Z, Huang H, Wu CH, Jung M, Dritschilo A, Riegel AT, et al. Omics-based molecular target and biomarker identification. Methods Mol Biol Clifton NJ (2011) 719:547–71. doi: 10.1007/978-1-61779-027-0_26
21. Almouzni G, Altucci L, Amati B, Ashley N, Baulcombe D, Beaujean N, et al. Relationship between genome and epigenome–challenges and requirements for future research. BMC Genomics (2014) 15:487. doi: 10.1186/1471-2164-15-487
22. Yong YK, Shankar EM, Solomon A, Spelman T, Fairley CK, Elliott JH, et al. Polymorphisms in the CD14 and TLR4 genes independently predict CD4+ T-cell recovery in HIV-infected individuals on antiretroviral therapy. AIDS Lond Engl (2016) 30:2159–68. doi: 10.1097/QAD.0000000000001179
23. Marelli S, Williamson JC, Protasio AV, Naamati A, Greenwood EJ, Deane JE, et al. Antagonism of PP2A is an independent and conserved function of HIV-1 Vif and causes cell cycle arrest. eLife (2020) 9:e53036. doi: 10.7554/eLife.53036
24. Celerino da Silva R, Alves NMP, Gondim Silva ML, Agrelli A, Coelho AVC, Guimarães RL, et al. Brief report: polymorphisms in TNF-α/TNFR1 pathway genes are associated with CD4+ T-cell recovery in HIV-1-infected individuals on antiretroviral therapy. J Acquir Immune Defic Syndr 1999 (2021) 88:322–7. doi: 10.1097/QAI.0000000000002761
25. Singh S, Sharma A, Arora SK. High producer haplotype (CAG) of -863C/A, -308G/A and -238G/A polymorphisms in the promoter region of TNF-α gene associate with enhanced apoptosis of lymphocytes in HIV-1 subtype C infected individuals from North India. PloS One (2014) 9:e98020. doi: 10.1371/journal.pone.0098020
26. An P, Duggal P, Wang LH, O’Brien SJ, Donfield S, Goedert JJ, et al. Polymorphisms of CUL5 are associated with CD4+ T cell loss in HIV-1 infected individuals. PloS Genet (2007) 3:e19. doi: 10.1371/journal.pgen.0030019
27. Klein J, Sato A. The HLA system. First Two Parts N Engl J Med (2000) 343:702–9. doi: 10.1056/NEJM200009073431006
28. El-Beeli M, Al-Mahrooqi SH, Youssef RM, Zadjali F, Balkhair A, Al-Balushi MS, et al. HLA-A68 and HLA-B15 alleles correlate with poor immune response among AIDS patients on combined antiretroviral therapy. Hum Immunol (2016) 77:490–7. doi: 10.1016/j.humimm.2016.04.009
29. Pereira LMS, França EDS, Costa IB, Jorge EVO, Mattos PJ de SM, Freire ABC, et al. HLA-B*13, B*35 and B*39 alleles are closely associated with the lack of response to ART in HIV infection: A cohort study in a population of Northern Brazil. Front Immunol (2022) 13:829126. doi: 10.3389/fimmu.2022.829126
30. Soria A, Guerini FR, Bandera A, Bolognesi E, Uglietti A, Fusco C, et al. KIR-HLA genotypes in HIV-infected patients lacking immunological recovery despite effective antiretroviral therapy. PloS One (2011) 6:e27349. doi: 10.1371/journal.pone.0027349
31. Carvalho-Silva WHV, Andrade-Santos JL, Guedes MCDS, Crovella S, Guimarães RL. CCR5 genotype and pre-treatment CD4+ T-cell count influence immunological recovery of HIV-positive patients during antiretroviral therapy. Gene (2020) 741:144568. doi: 10.1016/j.gene.2020.144568
32. García M, Jiménez-Sousa MA, Blanco J, Restrepo C, Pacheco YM, BroChado-Kith Ó, et al. CD4 recovery is associated with genetic variation in IFNγ and IL19 genes. Antiviral Res (2019) 170:104577. doi: 10.1016/j.antiviral.2019.104577
33. Yeregui E, Viladés C, Domingo P, Ceausu A, Pacheco YM, Veloso S, et al. High circulating SDF-1and MCP-1 levels and genetic variations in CXCL12, CCL2 and CCR5: Prognostic signature of immune recovery status in treated HIV-positive patients. EBioMedicine (2020) 62:103077. doi: 10.1016/j.ebiom.2020.103077
34. Restrepo C, Gutierrez-Rivas M, Pacheco YM, García M, Blanco J, Medrano LM, et al. Genetic variation in CCR2 and CXCL12 genes impacts on CD4 restoration in patients initiating cART with advanced immunesupression. PloS One (2019) 14:e0214421. doi: 10.1371/journal.pone.0214421
35. Masson JJR, Cherry CL, Murphy NM, Sada-Ovalle I, Hussain T, Palchaudhuri R, et al. Polymorphism rs1385129 within Glut1 gene SLC2A1 is linked to poor CD4+ T cell recovery in antiretroviral-treated HIV+ Individuals. Front Immunol (2018) 9:900. doi: 10.3389/fimmu.2018.00900
36. Fu Y, Liu Y, Liu Z, Liu L, Yuan L, An X, et al. The Impact of microRNA Regulation on Immune Recovery in HIV-1-Infected Patients Treated during Acute Infection: A Pilot Study. BioMed Res Int (2020) 2020:5782927. doi: 10.1155/2020/5782927
37. Lv J-N, Li J-Q, Cui Y-B, Ren Y-Y, Fu Y-J, Jiang Y-J, et al. Plasma microRNA signature panel predicts the immune response after antiretroviral therapy in HIV-infected patients. Front Immunol (2021) 12:753044. doi: 10.3389/fimmu.2021.753044
38. Liu X, Lin L, Lu L, Li X, Han Y, Qiu Z, et al. Comparative transcriptional analysis identified characteristic genes and patterns in HIV-infected immunological non-responders. Front Immunol (2022) 13:807890. doi: 10.3389/fimmu.2022.807890
39. Younes S-A, Talla A, Pereira Ribeiro S, Saidakova EV, Korolevskaya LB, Shmagel KV, et al. Cycling CD4+ T cells in HIV-infected immune nonresponders have mitochondrial dysfunction. J Clin Invest (2023) 128:5083–94. doi: 10.1172/JCI120245
40. Ghneim K, Ashish-Arunkumar S, Ribeiro S, Fourati S, Jeffery A, Filali-Mouhim A, et al. Microbiome and Metabolome driven differentiation of TGF-β producing Tregs leads to Senescence and HIV latency. bioRxiv, (2020) 12. http://dx.doi.org/10.2139/ssrn.3822732.
41. Li H, Tang Y, Wang Y, Li Y, Yang Y, Liao K, et al. Single-cell sequencing resolves the landscape of immune cells and regulatory mechanisms in HIV-infected immune non-responders. Cell Death Dis (2022) 13:849. doi: 10.1038/s41419-022-05225-6
42. Woelk CH, Beliakova-Bethell N, Goicoechea M, Zhao Y, Du P, Rought SE, et al. Gene expression before HAART initiation predicts HIV-infected individuals at risk of poor CD4+ T-cell recovery. AIDS Lond Engl (2010) 24:217–22. doi: 10.1097/QAD.0b013e328334f1f0
43. Azzam S, Schlatzer D, Maxwell S, Li X, Bazdar D, Chen Y, et al. Proteome and protein network analyses of memory T cells find altered translation and cell stress signaling in treated human immunodeficiency virus patients exhibiting poor CD4 recovery. Open Forum Infect Dis (2016) 3:ofw037. doi: 10.1093/ofid/ofw037
44. Liu Y, Li Z, Lu X, Kuang Y-Q, Kong D, Zhang X, et al. Dysregulation of memory B cells and circulating T follicular helper cells is a predictor of poor immune recovery in HIV-infected patients on antiretroviral therapy. J Med Virol (2023) 95:e28559. doi: 10.1002/jmv.28559
45. Scarpellini B, Zanoni M, Sucupira MCA, Truong H-HM, Janini LMR, Segurado IDC, et al. Plasma metabolomics biosignature according to HIV stage of infection, pace of disease progression, viremia level and immunological response to treatment. PloS One (2016) 11:e0161920. doi: 10.1371/journal.pone.0161920
46. Rodríguez-Gallego E, Gómez J, Pacheco YM, Peraire J, Viladés C, Beltrán-Debón R, et al. A baseline metabolomic signature is associated with immunological CD4+ T-cell recovery after 36 months of antiretroviral therapy in HIV-infected patients. AIDS Lond Engl (2018) 32:565–73. doi: 10.1097/QAD.0000000000001730
47. Masip J, Jorba R, López-Dupla M, Domingo P, Pacheco YM, García-Pardo G, et al. Lipoprotein profile in immunological non-responders PLHIV after antiretroviral therapy initiation. Int J Mol Sci (2022) 23:8071. doi: 10.3390/ijms23158071
48. Qian S, Chen X, Wu T, Sun Y, Li X, Fu Y, et al. The accumulation of plasma acylcarnitines are associated with poor immune recovery in HIV-infected individuals. BMC Infect Dis (2021) 21:808. doi: 10.1186/s12879-021-06525-6
49. Ferrari B, Da Silva AC, Liu KH, Saidakova EV, Korolevskaya LB, Shmagel KV, et al. Gut-derived bacterial toxins impair memory CD4+ T cell mitochondrial function in HIV-1 infection. J Clin Invest (2022) 132:e149571. doi: 10.1172/JCI149571
50. Nyström S, Govender M, Yap SH, Kamarulzaman A, Rajasuriar R, Larsson M. HIV-infected individuals on ART with impaired immune recovery have altered plasma metabolite profiles. Open Forum Infect Dis (2021) 8:ofab288. doi: 10.1093/ofid/ofab288
51. Malo A-I, Rull A, Girona J, Domingo P, Fuertes-Martín R, Amigó N, et al. Glycoprotein profile assessed by 1H-NMR as a global inflammation marker in patients with HIV infection. A Prospective Study J Clin Med (2020) 9:1344. doi: 10.3390/jcm9051344
52. Rauch A, Nolan D, Furrer H, McKinnon E, John M, Mallal S, et al. HLA-Bw4 homozygosity is associated with an impaired CD4 T cell recovery after initiation of antiretroviral therapy. Clin Infect Dis Off Publ Infect Dis Soc Am (2008) 46:1921–5. doi: 10.1086/588479
53. Flores-Villanueva PO, Yunis EJ, Delgado JC, Vittinghoff E, Buchbinder S, Leung JY, et al. Control of HIV-1 viremia and protection from AIDS are associated with HLA-Bw4 homozygosity. Proc Natl Acad Sci U.S.A. (2001) 98:5140–5. doi: 10.1073/pnas.071548198
54. Jost S, Altfeld M. Control of human viral infections by natural killer cells. Annu Rev Immunol (2013) 31:163–94. doi: 10.1146/annurev-immunol-032712-100001
55. Murakami T, Yamamoto N. Roles of chemokines and chemokine receptors in HIV-1 infection. Int J Hematol (2000) 72:412–7.
56. Flanagan CA. Receptor conformation and constitutive activity in CCR5 chemokine receptor function and HIV infection. Adv Pharmacol San Diego Calif (2014) 70:215–63. doi: 10.1016/B978-0-12-417197-8.00008-0
57. Samson M, Libert F, Doranz BJ, Rucker J, Liesnard C, Farber CM, et al. Resistance to HIV-1 infection in caucasian individuals bearing mutant alleles of the CCR-5 chemokine receptor gene. Nature (1996) 382:722–5. doi: 10.1038/382722a0
58. Camargo JF, Quinones MP, Mummidi S, Srinivas S, Gaitan AA, Begum K, et al. CCR5 expression levels influence NFAT translocation, IL-2 production, and subsequent signaling events during T lymphocyte activation. J Immunol Baltim Md 1950 (2009) 182:171–82. doi: 10.4049/jimmunol.182.1.171
59. Peraire J, Viladés C, Pacheco YM, López-Dupla M, Domingo P, Gutiérrez M, et al. Evaluation of the pharmacogenetics of immune recovery in treated HIV-infected patients. Expert Opin Drug Metab Toxicol (2014) 10:81–101. doi: 10.1517/17425255.2014.854330
60. Brumme ZL, Dong WWY, Chan KJ, Hogg RS, Montaner JSG, O’Shaughnessy MV, et al. Influence of polymorphisms within the CX3CR1 and MDR-1 genes on initial antiretroviral therapy response. AIDS Lond Engl (2003) 17:201–8. doi: 10.1097/00002030-200301240-00010
61. Vijay K. Toll-like receptors in immunity and inflammatory diseases: Past, present, and future. Int Immunopharmacol (2018) 59:391–412. doi: 10.1016/j.intimp.2018.03.002
62. Joshi A, Punke EB, Mehmetoglu-Gurbuz T, Peralta DP, Garg H. TLR9 polymorphism correlates with immune activation, CD4 decline and plasma IP10 levels in HIV patients. BMC Infect Dis (2019) 19:56. doi: 10.1186/s12879-019-3697-9
63. Macintyre AN, Gerriets VA, Nichols AG, Michalek RD, Rudolph MC, Deoliveira D, et al. The glucose transporter Glut1 is selectively essential for CD4 T cell activation and effector function. Cell Metab (2014) 20:61–72. doi: 10.1016/j.cmet.2014.05.004
64. Silva A, Gírio A, Cebola I, Santos CI, Antunes F, Barata JT. Intracellular reactive oxygen species are essential for PI3K/Akt/mTOR-dependent IL-7-mediated viability of T-cell acute lymphoblastic leukemia cells. Leukemia (2011) 25:960–7. doi: 10.1038/leu.2011.56
65. Torroni A, Huoponen K, Francalacci P, Petrozzi M, Morelli L, Scozzari R, et al. Classification of European mtDNAs from an analysis of three European populations. Genetics (1996) 144:1835–50. doi: 10.1093/genetics/144.4.1835
66. Hendrickson SL, Hutcheson HB, Ruiz-Pesini E, Poole JC, Lautenberger J, Sezgin E, et al. Mitochondrial DNA haplogroups influence AIDS progression. AIDS Lond Engl (2008) 22:2429–39. doi: 10.1097/QAD.0b013e32831940bb
67. Guzmán-Fulgencio M, Berenguer J, Micheloud D, Fernández-Rodríguez A, García-Álvarez M, Jiménez-Sousa MA, et al. European mitochondrial haplogroups are associated with CD4+ T cell recovery in HIV-infected patients on combination antiretroviral therapy. J Antimicrob Chemother (2013) 68:2349–57. doi: 10.1093/jac/dkt206
68. Greenblatt R, Bacchetti P, Boylan R, Kober K, Springer G, Anastos K, et al. Genetic and clinical predictors of CD4 lymphocyte recovery during suppressive antiretroviral therapy: Whole exome sequencing and antiretroviral therapy response phenotypes. PloS One (2019) 14:e0219201. doi: 10.1371/journal.pone.0219201
69. Zapalska-Sozoniuk M, Chrobak L, Kowalczyk K, Kankofer M. Is it useful to use several “omics” for obtaining valuable results? Mol Biol Rep (2019) 46:3597–606. doi: 10.1007/s11033-019-04793-9
70. Rashid F, Zaongo SD, Song F, Chen Y. The diverse roles of miRNAs in HIV pathogenesis: Current understanding and future perspectives. Front Immunol (2022) 13:1091543. doi: 10.3389/fimmu.2022.1091543
71. Letafati A, Najafi S, Mottahedi M, Karimzadeh M, Shahini A, Garousi S, et al. MicroRNA let-7 and viral infections: focus on mechanisms of action. Cell Mol Biol Lett (2022) 27:14. doi: 10.1186/s11658-022-00317-9
72. Noh S-J, Miller SH, Lee YT, Goh S-H, Marincola FM, Stroncek DF, et al. Let-7 microRNAs are developmentally regulated in circulating human erythroid cells. J Transl Med (2009) 7:98. doi: 10.1186/1479-5876-7-98
73. Shahbaz S, Bozorgmehr N, Koleva P, Namdar A, Jovel J, Fava RA, et al. CD71+VISTA+ erythroid cells promote the development and function of regulatory T cells through TGF-β. PloS Biol (2018) 16:e2006649. doi: 10.1371/journal.pbio.2006649
74. Trebak M, Kinet J-P. Calcium signalling in T cells. Nat Rev Immunol (2019) 19:154–69. doi: 10.1038/s41577-018-0110-7
75. Xu T, Huang C, Chen Z, Li J. MicroRNA-323-3p: a new biomarker and potential therapeutic target for rheumatoid arthritis. Rheumatol Int (2014) 34:721–2. doi: 10.1007/s00296-013-2767-3
76. Bandera A, Masetti M, Fabbiani M, Biasin M, Muscatello A, Squillace N, et al. The NLRP3 inflammasome is upregulated in HIV-infected antiretroviral therapy-treated individuals with defective immune recovery. Front Immunol (2018) 9:214. doi: 10.3389/fimmu.2018.00214
77. Broz P, Dixit VM. Inflammasomes: mechanism of assembly, regulation and signalling. Nat Rev Immunol (2016) 16:407–20. doi: 10.1038/nri.2016.58
78. Watanabe D, Uehira T, Suzuki S, Matsumoto E, Ueji T, Hirota K, et al. Clinical characteristics of HIV-1-infected patients with high levels of plasma interferon-γ: a multicenter observational study. BMC Infect Dis (2019) 19:11. doi: 10.1186/s12879-018-3643-2
79. Cui M, Cheng C, Zhang L. High-throughput proteomics: a methodological mini-review. Lab Investig J Tech Methods Pathol (2022) 102:1170–81. doi: 10.1038/s41374-022-00830-7
80. Uzozie AC, Aebersold R. Advancing translational research and precision medicine with targeted proteomics. J Proteomics (2018) 189:1–10. doi: 10.1016/j.jprot.2018.02.021
81. Grabowska K, Harwood E, Ciborowski P. HIV and proteomics: what we have learned from high throughput studies. Proteomics Clin Appl (2021) 15:e2000040. doi: 10.1002/prca.202000040
82. Rozanova S, Barkovits K, Nikolov M, Schmidt C, Urlaub H, Marcus K. Quantitative mass spectrometry-based proteomics: an overview. Methods Mol Biol Clifton NJ (2021) 2228:85–116. doi: 10.1007/978-1-0716-1024-4_8
83. Donnelly MR, Ciborowski P. Proteomics, biomarkers, and HIV-1: A current perspective. Proteomics Clin Appl (2016) 10:110–25. doi: 10.1002/prca.201500002
84. Wan L-Y, Huang H-H, Zhen C, Chen S-Y, Song B, Cao W-J, et al. Distinct inflammation-related proteins associated with T cell immune recovery during chronic HIV-1 infection. Emerg Microbes Infect (2023) 12:2150566. doi: 10.1080/22221751.2022.2150566
85. Qian F, Hu S, Zhu Y, Wang Y, Liu J, Qiao J, et al. CD56dim NK cell is an important factor in T cell depletion of cART-treated AIDS patients. Int J Gen Med (2022) 15:4575–83. doi: 10.2147/IJGM.S356771
86. Meyer-Myklestad MH, Medhus AW, Lorvik KB, Seljeflot I, Hansen SH, Holm K, et al. Human immunodeficiency virus-infected immunological nonresponders have colon-restricted gut mucosal immune dysfunction. J Infect Dis (2022) 225:661–74. doi: 10.1093/infdis/jiaa714
87. Rosado-Sánchez I, Jarrín I, Pozo-Balado MM, de Pablo-Bernal RS, Herrero-Fernández I, Alvarez-Ríos AI, et al. Higher levels of IL-6, CD4 turnover and Treg frequency are already present before cART in HIV-infected subjects with later low CD4 recovery. Antiviral Res (2017) 142:76–82. doi: 10.1016/j.antiviral.2017.03.015
88. Francisco V, Pino J, Gonzalez-Gay MA, Mera A, Lago F, Gómez R, et al. Adipokines and inflammation: is it a question of weight? Br J Pharmacol (2018) 175:1569–79. doi: 10.1111/bph.14181
89. Yeregui E, Masip J, Viladés C, Domingo P, Pacheco YM, Blanco J, et al. Adipokines as new biomarkers of immune recovery: apelin receptor, RBP4 and ZAG are related to CD4+ T-cell reconstitution in PLHIV on suppressive antiretroviral therapy. Int J Mol Sci (2022) 23:2202. doi: 10.3390/ijms23042202
91. Clish CB. Metabolomics: an emerging but powerful tool for precision medicine. Cold Spring Harb Mol Case Stud (2015) 1:a000588. doi: 10.1101/mcs.a000588
92. Chen Y, Li E-M, Xu L-Y. Guide to metabolomics analysis: A bioinformatics workflow. Metabolites (2022) 12:357. doi: 10.3390/metabo12040357
93. Simmons S, Ishii M. Sphingosine-1-phosphate: a master regulator of lymphocyte egress and immunity. Arch Immunol Ther Exp (Warsz) (2014) 62:103–15. doi: 10.1007/s00005-013-0264-8
94. Paltauf F. Ether lipids in biomembranes. Chem Phys Lipids (1994) 74:101–39. doi: 10.1016/0009-3084(94)90054-x
95. Azzoni L, Foulkes AS, Firnhaber C, Yin X, Crowther NJ, Glencross D, et al. Metabolic and anthropometric parameters contribute to ART-mediated CD4+ T cell recovery in HIV-1-infected individuals: an observational study. J Int AIDS Soc (2011) 14:37. doi: 10.1186/1758-2652-14-37
96. Palmer CS, Ostrowski M, Gouillou M, Tsai L, Yu D, Zhou J, et al. Increased glucose metabolic activity is associated with CD4+ T-cell activation and depletion during chronic HIV infection. AIDS Lond Engl (2014) 28:297–309. doi: 10.1097/QAD.0000000000000128
97. Dambrova M, Makrecka-Kuka M, Kuka J, Vilskersts R, Nordberg D, Attwood MM, et al. Acylcarnitines: nomenclature, biomarkers, therapeutic potential, drug targets, and clinical trials. Pharmacol Rev (2022) 74:506–51. doi: 10.1124/pharmrev.121.000408
98. Watson WH, Ritzenthaler JD, Peyrani P, Wiemken TL, Furmanek S, Reyes Vega AM, et al. Plasma cysteine/cystine and glutathione/glutathione disulfide redox potentials in HIV and COPD patients. Free Radic Biol Med (2019) 143:55–61. doi: 10.1016/j.freeradbiomed.2019.07.031
Keywords: genomics, immunological non-response, metabolomics, proteomics, transcriptomics, PLHIV
Citation: Espineira S, Flores-Piñas M, Chafino S, Viladés C, Negredo E, Fernández-Arroyo S, Mallolas J, Villar B, Moreno S, Vidal F, Rull A and Peraire J (2023) Multi-omics in HIV: searching insights to understand immunological non-response in PLHIV. Front. Immunol. 14:1228795. doi: 10.3389/fimmu.2023.1228795
Received: 25 May 2023; Accepted: 25 July 2023;
Published: 15 August 2023.
Edited by:
Scott Sieg, Case Western Reserve University, United StatesReviewed by:
Carey Shive, United States Department of Veterans Affairs, United StatesJoseph Mudd, Tulane University, United States
Copyright © 2023 Espineira, Flores-Piñas, Chafino, Viladés, Negredo, Fernández-Arroyo, Mallolas, Villar, Moreno, Vidal, Rull and Peraire. This is an open-access article distributed under the terms of the Creative Commons Attribution License (CC BY). The use, distribution or reproduction in other forums is permitted, provided the original author(s) and the copyright owner(s) are credited and that the original publication in this journal is cited, in accordance with accepted academic practice. No use, distribution or reproduction is permitted which does not comply with these terms.
*Correspondence: Silvia Chafino, silvia.chafino@ciberinfec.es; Anna Rull, anna.rull@iispv.cat
†These authors have contributed equally to this work and share first authorship
‡These authors have contributed equally to this work and share senior authorship