- 1Department of Pathology, Xianyang Central Hospital, Xianyang, China
- 2Department of Pathology, Division of Experimental Diagnostic, KingMed Medical Laboratory (Xi’an) Co., Ltd., Xi’an, China
- 3Department of Pathology, Xi’an Central Hospital, Xi’an, China
- 4Department of Thoracic Surgery, Tangdu Hospital, Fourth Military Medical University, Xi’an, China
The utilisation of neoadjuvant immunotherapy has demonstrated promising preliminary clinical outcomes for early-stage resectable non-small-cell lung cancer (NSCLC). Nevertheless, it is imperative to develop novel neoadjuvant combination therapy regimens incorporating immunotherapy to further enhance the proportion of patients who derive benefit. Recent studies have revealed that stereotactic body radiotherapy (SBRT) not only induces direct tumour cell death but also stimulates local and systemic antitumour immune responses. Numerous clinical trials have incorporated SBRT into immunotherapy for advanced NSCLC, revealing that this combination therapy effectively inhibits local tumour growth while simultaneously activating systemic antitumour immune responses. Consequently, the integration of SBRT with neoadjuvant immunotherapy has emerged as a promising strategy for treating resectable NSCLC, as it can enhance the systemic immune response to eradicate micrometastases and recurrent foci post-resection. This review aims to elucidate the potential mechanism of combination of SBRT and immunotherapy followed by surgery and identify optimal clinical treatment strategies. Initially, we delineate the interplay between SBRT and the local tumour immune microenvironment, as well as the systemic antitumour immune response. We subsequently introduce the preclinical foundation and preliminary clinical trials of neoadjuvant SBRT combined with immunotherapy for treating resectable NSCLC. Finally, we discussed the optimal dosage, schedule, and biomarkers for neoadjuvant combination therapy in its clinical application. In conclusion, the elucidation of potential mechanism of neoadjuvant SBRT combined immunotherapy not only offers a theoretical basis for ongoing clinical trials but also contributes to determining the most efficacious therapy scheme for future clinical application.
1 Introduction
Stereotactic body radiation therapy (SBRT) has demonstrated promising outcomes in various solid tumours, particularly in early-stage non-small-cell lung cancer (NSCLC) (1–4). Compared to traditional radiotherapy, SBRT can significantly improve patient prognosis while posing a low risk of toxicity by precisely targeting local tumours and delivering high-dose, hypofractionated therapy (5–8). It is noteworthy that SBRT has a significant advantage over conventional radiotherapy due to its potent immune-activating effect (9). Conventional radiotherapy was previously believed to have immunosuppressive effects, as evidenced by bone marrow myelosuppression and reduced peripheral blood count during treatment (10). This notion was further supported by the use of whole-body irradiation as a myeloablative conditioning before haematopoietic stem cell transplantation (11). However, unlike conventional radiotherapy, the advent of SBRT enables patients to receive higher doses of precise radiotherapy in fewer fractions. The advantage enables SBRT to minimise the potential persistent immunosuppressive effects on the host when compared to conventional radiotherapy (12). In fact, researchers are increasingly recognizing the potent immunomodulatory effects of SBRT, which can convert refractory “cold” tumours into immunotherapy-responsive “hot” tumours (13). For instance, the incorporation of SBRT with immunotherapy in advanced NSCLC patients not only prolonged survival but also significantly increased cytotoxic T cell infiltration within the tumour microenvironment (TME) (14). Given the promising results of combing SBRT and immunotherapy, it is worthwhile to explore whether this approach can be applied to early-stage NSCLC for improved local tumour control and prevention of postoperative recurrence and metastasis.
Clinically, neoadjuvant immunotherapy has demonstrated promising potential in the treatment of early operable NSCLC (15, 16). Unlike traditional neoadjuvant chemotherapy, neoadjuvant immunotherapy not only promotes local tumour control but also activates the systemic antitumour immune response, which is considered a crucial factor in preventing postoperative recurrence and metastasis (17). Numerous clinical trials have confirmed that combining neoadjuvant immunotherapy with chemotherapy can significantly improve the pathological remission rate in patients (16, 18). For example, the CheckMate-816 trial demonstrated that neoadjuvant nivolumab combined with chemotherapy not only significantly increased both pathological complete response (pCR) (24% vs. 2.2%) and event-free survival (EFS) (31.6 months vs. 20.8 months), without increasing the risk of adverse events, compared to neoadjuvant chemotherapy alone (19). Based in these promising clinical trials, neoadjuvant immunotherapy combined with chemotherapy has been approved as the first-line treatment for early operable NSCLC (19). Given this success, it is worthwhile to investigate whether SBRT can also be combined with immunotherapy as neoadjuvant therapy for early operable NSCLC. Indeed, there are ongoing preclinical and clinical trials exploring the potential synergies between SBRT and immunotherapy in the neoadjuvant setting (20–22). However, before conducting further clinical trials and applications, it is important to fully understand the mechanism of interaction between SBRT and antitumour immune response, as well as determine the optimal dosage and scheduling for combination therapy.
Herein, we present an overview of the current status and potential mechanism of neoadjuvant SBRT in combination with immunotherapy, followed by surgery, for the treatment of NSCLC. We also discuss the optimal therapy schedule and predictive biomarkers for clinical application. Furthermore, we highlight future research directions and challenges that require further investigation.
2 The interplay between SBRT and antitumour immune response
Several studies have suggested that SBRT can promote the antitumour immune response through various pathways beyond its direct DNA damage to tumour cells (23–25). Previous studies have demonstrated that SBRT can induce the presentation of antigens by promoting the release of major histocompatibility complex 1 (MHC-1) and immunogenic cell death (ICD) of tumour cells. Additionally, it can directly stimulate dendritic cell (DC) maturation and CD8+ cytotoxic T lymphocyte infiltration in the TME (12, 26, 27). Notably, conventional radiotherapy has been demonstrated to mobilise several immunosuppressive cells, including regulatory T cells (Tregs), M2 macrophages, and bone marrow-derived suppressor cells (28). However, studies on SBRT-related immunosuppressive modification are scarce. A recent study compared the effects of SBRT (40 Gy/3 fractions) with conventional radiotherapy (62 Gy/20 fractions or 66-69 Gy/30 fractions) on the tumour immune microenvironment. The results showed that conventional radiotherapy has a negative impact on systemic immunity, resulting in an increase in neutrophils/lymphocytes and a decrease in total lymphocyte count. In contrast, SBRT increased B cell, central memory T cell, and effector CD8+ T cell infiltration in the TME, as well as increased CD8/Treg ratio (29). In summary, SBRT could activate the immune system through multiple pathways and create an ideal TME for subsequent immunotherapy (Figure 1).
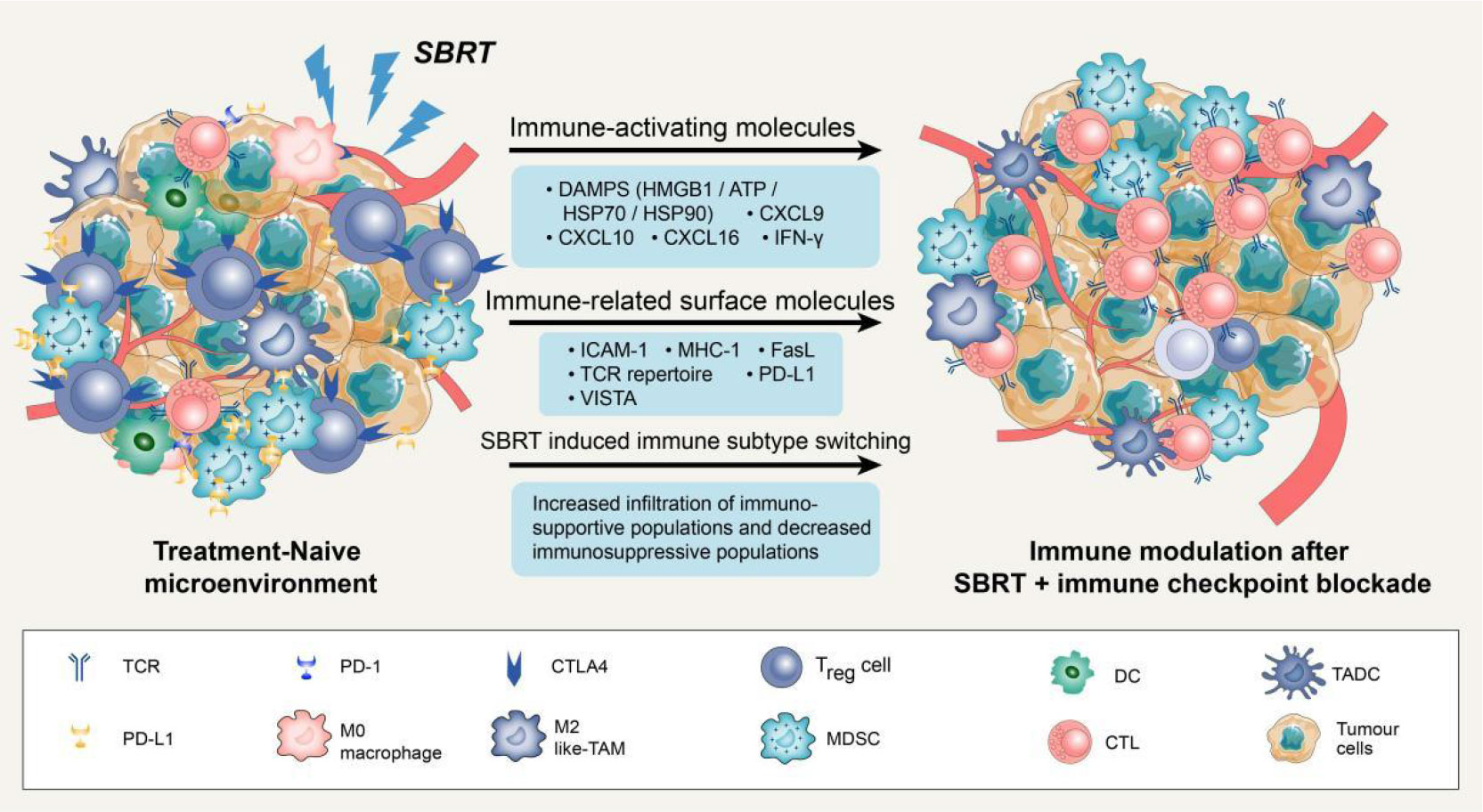
Figure 1 Potential mechanisms of synergy with SBRT and immune checkpoint blockade. Stereotactic body radiation therapy (SBRT) can transfer treatment-naive tumour microenvironment (TME) into “hot” tumour by producing immune-activating molecules and immune-related surface molecules, as well as by directly regulating immune cells composition. Compared with treatment-naive TME, the synergy with SBRT and immune checkpoint blockade could promote the infiltration of immune cells via up-regulation of adhesion molecules (ICAM-1) or chemokine (CXCL9/10/16). Notably, SBRT can contribute to adaptive immune resistance via IFN-γ mediated up-regulation of PD-L1 and VISTA expression on tumour cells. Blockade of these checkpoints by inhibitors permits the activation of T cells in tumour-associated draining lymph node and TME.
When tumour cells are exposed to lethal stimuli such as radiation or chemotherapy, a cascade of signaling molecules known as damage-associated molecular patterns (DAMPs) is released (30). These DAMPs include calreticulin, which is exposed to the cell surface, high mobility group box 1 (HMGB1), which is secreted by tumour cells as well as ATP molecules and heat shock proteins (HSP70 and HSP90) (31). Studies have shown that DAMPs induced by ICD could promote cytotoxic T lymphocyte infiltration by facilitating dendritic cell maturation and antigen presentation (32). Moreover, SBRT can stimulate the release of various chemokines such as C-X-C motif chemokine ligand-9/10/16 and interferons (IFNs), which play a crucial role in recruiting activated T cells to infiltrate the TME (33, 34). It has also been reported that SBRT can trigger the exposure and release of numerous tumour-associated antigens, which can be taken up by DCs, transported to lymph nodes, and presented as antigens (35, 36). Overall, these SBRT-induced factors are critical for the activation of local and systemic antitumour immune responses.
SBRT can also enhance the immunogenicity and antigenicity of tumour cells by regulating the expression of cell surface molecules and receptors. For instance, in a dose-dependent manner, SBRT can up-regulate cell surface markers such as intercellular adhesion molecule 1 (ICAM-1), MHC-1, and death receptor Fas (27, 37). It is widely recognized that MHC-1 is an essential co-stimulatory molecule for activating CD8+ T cells (38). ICAM-1 is the key adhesion molecule that facilitates immune cell adhesion and migration into the TME (39). The up-regulation of these surface molecules could enhance T cell-mediated antitumour immune response and increase the sensitivity of cytotoxic T lymphocytes to recognize and eliminate tumour cells (40). Notably, SBRT could also increase immune checkpoint expression on the surface of tumour cells. For example, the analysis of paired lung cancer samples following SBRT revealed an increase in the diversity of the T cell receptor repertoire and programmed cell death ligand 1 (PD-L1) expression, while no significant increase of CD8+ T cell and IFN expression was observed within tumour tissues (33). In addition to PD-L1, SBRT can also significantly up-regulate V-domain immunoglobulin suppressor of T cell activation (VISTA) expression in CD8+ T cells (29). It is worth noting that not all immune checkpoints are elevated following SBRT. Studies have reported that SBRT can significantly increase the frequency of Ki67+ programmed cell death protein 1 (PD-1)+ T cells and natural killer cells in advanced tumours without a significant increase in immune checkpoints such as T cell immunoglobulin and mucin domain-containing protein 3 (TIM-3) and Lymphocyte activation gene-3 (LAG-3) (41). In conclusion, SBRT-induced up-regulation of certain immune checkpoints might render patients more sensitive to subsequent immune checkpoint inhibitors, resulting in higher response rates and prolonging overall survival (OS).
Previous studies have demonstrated that SBRT can also transfer immunosuppressive microenvironments into “hot” tumors by directly regulating the immune cell composition (42). Reprogramming of the TME after SBRT is primarily induced by the production of chemokines and cytokines to recruit specific immune cell subsets. In mouse tumours, a single high-dose radiotherapy increased the influx of CD8+ T cells and simultaneously decreased Treg cell invasion (43). This change may attributed to the release of chemokine and vascular morphological (44). The enhanced homing of immune cells creates an ideal microenvironment for subsequent immunotherapy to effectively elicit antitumour response. A phase 2 clinical trial was conducted to evaluate the efficacy of combing pembrolizumab (a PD-1 inhibitor) with SBRT in NSCLC patients and further studied the reprogramming of TME. Results demonstrated a significant increase in the overall response rate (4.87-fold vs. 2.56-fold) and CD103+cytotoxic T cell infiltration after 6 weeks of SBRT plus pembrolizumab therapy, as compared to pembrolizumab monotherapy (14).
In addition to its direct tumour-killing effect, SBRT can induce tumour shrinkage in non-irradiated and distant metastatic tumours through the abscopal effect (45). The current understanding is that local immune activation triggered by SBRT can initiated a systemic immune response that produces cytokines and circulating CD8+ T cells. These molecules can then act on distant non-irradiated sites and effectively inhibit metastatic tumour progression (46). While this phenomenon is rare in SBRT monotherapy, combining it with immunotherapy is expected to increase its incidence.
3 The preclinical foundation of neoadjuvant SBRT combined with immunotherapy
It is widely acknowledged that neoadjuvant immunotherapy has the potential to not only control local tumours but also inhibit postoperative recurrence and metastasis through systemic immunity (47). While the effects of SBRT on the local TME have been studied, the impact of combining it with immunotherapy on systemic immunity remains unclear. The activation of systemic antitumour immune response is believed to be the mechanism underlying the radiotherapy-induced abscopal effect (48, 49). In a study with mice bearing breast cancer, combining radiotherapy with immunotherapy resulted in significant tumour shrinkage at both irradiated and non-irradiated sites. Notably, the abscopal effect was abolished in T cell-deficient mice (nude mice), indicating that T cells are essential for radiotherapy-induced distal tumour suppression (50).
Tumour-draining lymph nodes (TDLNs) are acknowledged as the primary sites for initiating antitumour immune responses, where immune cells differentiate into progenitor cells upon binding to antigens presented by DCs. These progenitor cells then differentiate and migrate into the TME, contributing to systemic immunity (51). Recently, Huang et al. proposed a novel concept suggesting that the antitumour effects of immune checkpoint inhibitors primarily occur in TDLNs rather than TME. The research found that injecting PD-L1 inhibitors into TDLNs significantly inhibited tumour growth, whereas injecting PD-L1 inhibitors directly into tumours had no effect. Furthermore, surgical removal of TDLNs abrogated the antitumour effects of PD-L1 inhibitors. Further mechanistic studies demonstrated that immune checkpoint inhibitor therapy initially promotes the amplification of T cell factor 1 (TCF-1)+ thymocyte selection-associated HMGB (TOX)- CD8+ T cells), which are tumour-specific memory cells in TDLNs, These cells subsequently migrated to the TME and peripheral immunity where they differentiate into effector T cells (52). This novel concept highlights the importance of TDLNs and systemic immunity in the antitumour response to immunotherapy (Figure 2).
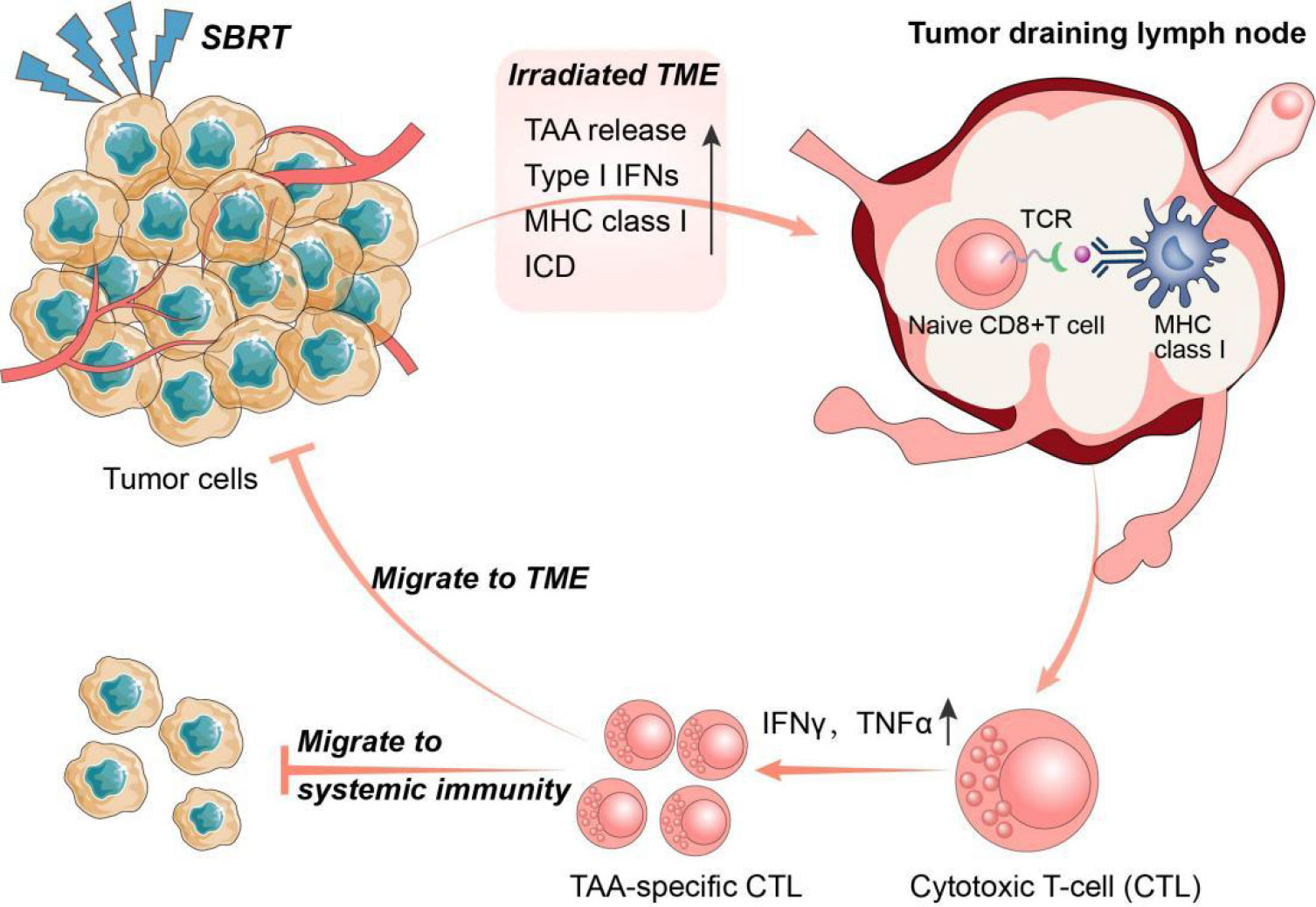
Figure 2 The interplay between SBRT and antitumour immune response. Stereotactic body radiation therapy (SBRT) could promote the activation of anti-tumour immune system through multiple pathways. In turn, activated anti-tumour immune responses also play a key role in the radio-induced abscopal effect. Specifically, SBRT can initiate antigen presentation by promoting the release of tumor-associated antigens (TAA) and major histocompatibility complex I (MHC I), and induce immunogenic cell death (ICD) in tumour cells. Tumour draining lymph nodes (TDLNs) are the main sites of anti-tumour immunity initiation where immune cells develop into progenitor cells after binding to antigens presented by dendritic cells (DCs). The progenitor cells subsequently differentiate into TAA-specific cytotoxic T lymphocytes (CTLs) and migrate into the tumour microenvironment (TME) and systemic immunity.
According to research, SBRT has demonstrated a greater potential than conventional radiotherapy in activating immune cells in TDLNs, leading to a more robust systemic immune response capable of eliminating potential metastases. Lee et al. were among the first to report that high-dose radiotherapy (15-25 Gy×1) could enhance the activation of immune cells in TDLNs of advanced tumours, resulting in activated CD8+ T cells that not only targeted primary tumours but also eliminated distant metastases in some cases. Moreover, the incorporation of immunotherapy into high-dose radiotherapy resulted in enhanced tumour eradication and systemic antitumour immune response (53). Additionally, Walker et al. demonstrated that the combination of high-dose radiotherapy with bempegaldesleukin (a CD122-preferential interleukin-2 pathway agonist) not only impedes the growth of irradiated tumours but also activated tumour-specific CD8+ T cells in systemic immunity, leading to the elimination of non-irradiated metastases (54). Additionally, in a preclinical model featuring disseminated metastasis (4T1 and mouse oral carcinoma 2), researchers discovered that the addition immune checkpoint inhibitors to radiotherapy plus bempegaldesin significantly prolonged the survival of mice by preventing distant metastasis. The effect was achieved by generating immune memory cells in TDLNs (55). Collectively, these preclinical studies suggest that combining SBRT with immunotherapy may enhance the incidence of the abscopal effect by promoting immune cell activation in TDLNs, generating a systemic immune response.
The dose and fraction of SBRT have also been demonstrated to impact the activation of TDLN-mediated systemic immune response. However, there are conflicting data and divergent opinions on the superiority of low-dose SBRT and single high-dose SBRT. Lee et al. discovered that low-dose SBRT (5 Gy×4 over 2 weeks) was significantly less effective in inducing a systemic antitumour immune response than a single dose of SBRT (20 Gy), possibly due to the gradual elimination of effector T cells and subsequent early relapse (53). In contrast, other reports have demonstrated that low-dose SBRT can elicit more favourable local and systemic immune responses and synergize with immunotherapy. For instance, Dewan et al. found that in a bilateral preclinical model of breast cancer, low-dose radiotherapy (8 Gy×3 or 6 Gy×5) combined with immunotherapy was more effective than administering a single high dose of 20 Gy. This combination not only slowed down tumour growth at the irradiation site but also significantly inhibiting lung metastasis and prolonging the survival of mice (56). Furthermore, Schaue et al. demonstrated in a mouse model loaded with B16-OVA melanoma cells that low-dose SBRT (7.5 Gy×2 and 5 Gy×3) was generally superior to a single dose of radiotherapy (15 Gy) in inducing peripheral tumour-specific immune responses (57).
A noteworthy finding from Savage et al. in a preclinical model of lung cancer was the efficacy of a new radiotherapy regimen (22 Gy followed by 0.5 Gy × 4 days) in increasing the infiltration of Granzyme B+CD8+ T cells within TME, while simultaneously reducing immune suppression caused by Tregs and M2 macrophages when compared to standard SBRT. Further immunoassay of secondary lymphoid organs indicated a significant increase in Granzyme B+CD8+ T cells and IFN-γ+CD8+ T cells in TDLNs of mice treated with the new radiotherapy regimen. These promising preclinical results offer a potential new radiotherapy regimen for clinical application to enhance its immunogenic potential (58). Overall, the above preclinical studies provide a foundation for the use of neoadjuvant SBRT combined with immunotherapy in early NSCLC to prevent distant metastasis.
4 Advances in neoadjuvant SBRT combined with immunotherapy for NSCLC
The combination of SBRT and immunotherapy has exhibited substantial potential in triggering a systematic antitumour immune response, as evidenced by preclinical studies. Clinically, Shaverdian et al. conducted a prospective analysis and demonstrated that advanced NSCLC patients who received radiotherapy prior to pembrolizumab treatment had significantly longer progression-free survival and overall survival compared to those without previous radiotherapy (59). As for neoadjuvant therapy, preliminarily clinical trials have shown that neoadjuvant SBRT combined with immunotherapy can prolong the survival of patients in several tumours by inducing a systemic antitumour immune response. For instance, in a study of 30 patients with locally advanced oral cavity squamous cell carcinoma, the addition of SBRT to neoadjuvant nivolumab therapy resulted in significant rates of major pathological response (MPR)(60.0%) and pCR(33.3%) in the neoadjuvant therapy group. Additionally, the group receiving combined treatment had an improved 24-month disease-free survival rate (70.4%) and OS rates (76.4%) (60). In another prospective study of locally advanced head and neck squamous cell carcinoma, the combination of neoadjuvant nivolumab and SBRT (40 Gy×5 or 24 Gy×3) significantly improved pathological responses in patients, with 86% achieving MPR and 67% achieving pCR (60).
Several phase II clinical trials have been initiated in resectable early NSCLC to investigate the feasibility, toxicity, and optimal schedule of neoadjuvant SBRT combined with immunotherapy based on increasing preclinical and clinical evidence (Table 1). For instance, a multicentre phase II trial (NCT04245514) evaluated the safety and efficacy of adding immunotherapy to neoadjuvant radiotherapy in patients with resectable stage III NSCLC. Durvalumab was administered in combination with SBRT (5 Gy×5 and 8 Gy×3) or conventional radiotherapy (2 Gy×20) to observe 12-month EFS after surgery, with recurrence-free survival and OS as secondary outcomes. Notably, the timing of SBRT initiation in neoadjuvant therapy remains inconsistent across current clinical trials. For instance, in one clinical trial (NCT05319574) for operable stage IB to III NSCLC, SBRT (8 Gy×3) was initiated 1-7 days before the first cycle of immunochemotherapy, whereas another trial (NCT05500092) started SBRT (8 Gy×3) therapy at the end of the first immunotherapy cycle (three cycles of neoadjuvant nivolumab plus chemotherapy). Additionally, in the clinical trial (NCT03110978) conducted by chang et al., nivolumab was administered either 36 hours before or after the initial fraction of SBRT. Significantly, the recent report on this trial demonstrated that combination therapy effectively enhances the 4-year EFS rate to 77%, compared to only 53% (95% CI 42–67%) achieved with SBRT monotherapy (61). The variation in therapy schedules among these clinical trials facilitates the exploration of optimal combination therapy strategies and underscores the need for a thorough understanding of the underlying mechanisms of neoadjuvant combination therapy.
5 Future challenges and directions for neoadjuvant combination therapy
Further research is needed to determine the optimal dosage, fraction, and schedule of radiotherapy in neoadjuvant combination therapy to enhance the systemic antitumour immune response, which remains a challenging task (62).
Limited research has been conducted on the impact of varying doses and fractions of radiotherapy on local and systemic immune responses in NSCLC patients. A recent clinical trial investigated the safety and efficacy of pembrolizumab combined with SBRT (50 Gy in four fractions) or conventional radiotherapy (45 Gy in 15 fractions) for treating lung and liver metastases in metastatic NSCLC. The results revealed that the group receiving pembrolizumab plus SBRT had an objective response rate of 38%, while only 10% was observed in the group receiving pembrolizumab plus conventional radiotherapy (63). This clinical trial demonstrated the superiority of SBRT over conventional radiotherapy in antitumour metastasis, no differences were observed between different SBRT regimens. Therefore, a convincing preclinical study is required to address this issue. However, the challenge with current preclinical research is the disparity between its findings and applicability in clinical practice (64). The inconsistency maybe attributed to the absence of preclinical models that can accurately replicate the immune microenvironment of patients. Current preclinical models primarily employ murine-derived cell lines in normal mice, and their use of murine-derived immune system and immune checkpoint inhibitors further undermines the reliability of preclinical research outcomes (56). Therefore, the development of a humanised mouse model, in which human immune cells are transplanted into mice with severe combined immunodeficiency, is anticipated to offer a solution to this challenge (65).
In addition to investigating the effects of radiotherapy dosage and fraction on the immune system, determining the optimal schedule is also critical in neoadjuvant therapy. To this end, Dewan et al. conducted a preclinical study using a bilateral breast cancer model to investigate the effect of combination therapy when altering the timing of immunotherapy relative to radiotherapy. The study aimed to investigate the impact of initiating immunotherapy 2 days before, on that same day as, or 2 days after SBRT (8 Gy×3) completion on tumour growth. Results showed that initiating immunotherapy 2 days before or on the same day as radiotherapy ended inhibited tumour growth at both irradiated and non-irradiated sites. However, delaying immunotherapy until 2 days after the completion of radiotherapy reduced therapeutic efficacy, resulting in complete regression of only one of the six primary tumours and reduced growth inhibition in the non-irradiated sites. This indicates that the timing of immunotherapy vs. radiotherapy might influence the efficacy of the combination therapy (56). Similarly, Dovedi et al. investigated the optimal schedule for combining immunotherapy with SBRT (10 Gy×5) by varying the timing of treatment. The results showed that adding immunotherapy at the beginning or end of SBRT did not significantly affect the OS in mice. However, initiating immunotherapy one week after the end of SBRT was entirely ineffective in improving OS, similar to radiotherapy alone. Furthermore, they analysed the dynamics of CD4+ T cells and CD8+ T cells in the TME to explore the underlying mechanisms of the optimal combination schedule. Results indicated a significant increase in PD-1+CD4+ and PD-1+CD8+ T cell proportions within the tumour one day after SBRT completion, but a significant decrease in PD-1+CD8+ T cells seven days post-radiotherapy (66). Collectively, these preclinical studies suggest that SBRT leads to an acute increase in tumour-specific CD8+ T cells. Adding immunotherapy after the completion of the radiotherapy cycle might result in a significant decrease in the treatment’s efficacy due to the anergy of these cells.
Identification of predictive biomarkers for neoadjuvant combination therapy is crucial in determining the population that will benefit and dynamically evaluating therapy efficacy (67). Nevertheless, the biomarkers of neoadjuvant SBRT in combination with immunotherapy remain unknown as classical immunotherapy biomarkers such as PD-L1 and tumour mutation burden fail to dynamically reflect the changes in the TME and systemic antitumour immune response (68). Recent studies have focused on the kinetics of specific immune cell subsets in systemic immunity and their correlation with efficacy (69). For example, Huang et al. reported that TCF-1+TOX-CD8+ T cells in TDLNs are bona fide memory T cells that can migrate and differentiate into systemic immunity after immune checkpoint inhibitor therapy (52). Therefore, the kinetics of CD8+ T cell subsets in peripheral immunity after neoadjuvant immunotherapy might be closely associated with efficacy. In addition, Kamphorst et al. reported that increased Ki67+PD-1+CD8+ T cells could be detected in the peripheral blood of approximately 70% of patients with lung cancer 4 weeks after receiving immunotherapy. These cells are considered to be tumour-specific T cells, and their kinetics are correlated with positive clinical outcomes (70). In summary, specific immune subpopulations in systemic immunity might serve as potential biomarkers for dynamically monitoring immune responses in patients with NSCLC undergoing neoadjuvant combination therapy.
6 Discussion
Preliminary preclinical findings demonstrate the significant potential of SBRT in combination with immunotherapy in neoadjuvant setting for resectable NSCLC. Furthermore, several ongoing clinical trials are investigating the feasibility and toxicity of this novel neoadjuvant combination therapy; however, it will take some time for data to confirm its clinical efficacy. In addition, the determination of optimal dosage and fractions, identification of predictive biomarkers, and establishment of an optimal schedule for combination therapy are all crucial factors that impact the efficacy of neoadjuvant therapy. Therefore, further preclinical and clinical studies are imperative to address these challenges prior to widespread implementation it in clinical practice.
Author contributions
YS, XM and DH contribute to the literature research, figures and drafted the manuscript. BD and TQ provided supervision and editing the final manuscript. All authors have agreed to the published version of the manuscript.
Conflict of interest
XM was employed by KingMed Medical Laboratory Xi’an Co., Ltd.
The remaining authors declare that the research was conducted in the absence of any commercial or financial relationships that could be construed as a potential conflict of interest.
Publisher’s note
All claims expressed in this article are solely those of the authors and do not necessarily represent those of their affiliated organizations, or those of the publisher, the editors and the reviewers. Any product that may be evaluated in this article, or claim that may be made by its manufacturer, is not guaranteed or endorsed by the publisher.
References
1. Bezjak A, Paulus R, Gaspar L, Timmerman R, Straube W, Ryan W, et al. Safety and efficacy of a five-fraction stereotactic body radiotherapy schedule for centrally located non-small-cell lung cancer: NRG oncology/RTOG 0813 trial. J Clin Oncol: Off J Am Soc Clin Oncol (2019) 37(15):1316–25. doi: 10.1200/JCO.18.00622
2. Collen C, Christian N, Schallier D, Meysman M, Duchateau M, Storme G, et al. Phase II study of stereotactic body radiotherapy to primary tumour and metastatic locations in oligometastatic nonsmall-cell lung cancer patients. Ann Oncol: Off J Eur Soc Med Oncol (2014) 25(10):1954–9. doi: 10.1093/annonc/mdu370
3. Iyengar P, Westover K, Timmerman RD. Stereotactic ablative radiotherapy (SABR) for non-small cell lung cancer. Semin Respir Crit Care Med (2013) 34:845–54. doi: 10.1055/s-0033-1358554
4. Videtic G, Donington J, Giuliani M, Heinzerling J, Karas T, Kelsey C, et al. Stereotactic body radiation therapy for early-stage non-small cell lung cancer: executive summary of an ASTRO evidence-based guideline. Pract Radiat Oncol (2017) 7(5):295–301. doi: 10.1016/j.prro.2017.04.014
5. Nyman J, Hallqvist A, Lund J, Brustugun O, Bergman B, Bergström P, et al. SPACE - A randomized study of SBRT vs conventional fractionated radiotherapy in medically inoperable stage I NSCLC. Radiother Oncol: J Eur Soc Ther Radiol Oncol (2016) 121(1):1–8. doi: 10.1016/j.radonc.2016.08.015
6. Jeppesen S, Schytte T, Jensen H, Brink C, Hansen O. Stereotactic body radiation therapy versus conventional radiation therapy in patients with early stage non-small cell lung cancer: an updated retrospective study on local failure and survival rates. Acta Oncol (Stockholm Sweden). (2013) 52(7):1552–8. doi: 10.3109/0284186X.2013.813635
7. Li C, Wang L, Wu Q, Zhao J, Yi F, Xu J, et al. A meta-analysis comparing stereotactic body radiotherapy vs conventional radiotherapy in inoperable stage I non-small cell lung cancer. Medicine (2020) 99(34):e21715. doi: 10.1097/MD.0000000000021715
8. von Reibnitz D, Shaikh F, Wu A, Treharne G, Dick-Godfrey R, Foster A, et al. Stereotactic body radiation therapy (SBRT) improves local control and overall survival compared to conventionally fractionated radiation for stage I non-small cell lung cancer (NSCLC). Acta Oncol (Stockholm Sweden) (2018) 57(11):1567–73. doi: 10.1080/0284186X.2018.1481292
9. Timmerman R, Herman J, Cho L. Emergence of stereotactic body radiation therapy and its impact on current and future clinical practice. J Clin Oncol: Off J Am Soc Clin Oncol (2014) 32(26):2847–54. doi: 10.1200/JCO.2014.55.4675
10. Mac Manus M, Lamborn K, Khan W, Varghese A, Graef L, Knox S. Radiotherapy-associated neutropenia and thrombocytopenia: analysis of risk factors and development of a predictive model. Blood (1997) 89(7):2303–10. doi: 10.1182/blood.V89.7.2303
11. Tse E, Kwong Y. The diagnosis and management of NK/T-cell lymphomas. J Hematol Oncol (2017) 10(1):85. doi: 10.1186/s13045-017-0452-9
12. Mills B, Qiu H, Drage M, Chen C, Mathew J, Garrett-Larsen J, et al. Modulation of the human pancreatic ductal adenocarcinoma immune microenvironment by stereotactic body radiotherapy. Clin Cancer Res: an Off J Am Assoc Cancer Res (2022) 28(1):150–62. doi: 10.1158/1078-0432.CCR-21-2495
13. Swamy K. Vascular normalisation and immunotherapy: spawning a virtuous cycle. Front Oncol (2022) 12:1002957. doi: 10.3389/fonc.2022.1002957
14. van der Woude L, Gorris M, Wortel I, Creemers J, Verrijp K, Monkhorst K, et al. Tumour microenvironment shows an immunological abscopal effect in patients with NSCLC treated with pembrolizumab-radiotherapy combination. J Immunother Cancer (2022) 10(10):e005248. doi: 10.1136/jitc-2022-005248
15. Cascone T, Leung C, Weissferdt A, Pataer A, Carter B, Godoy M, et al. Neoadjuvant chemotherapy plus nivolumab with or without ipilimumab in operable non-small cell lung cancer: the phase 2 platform NEOSTAR trial. Nat Med (2023) 29(3):593–604. doi: 10.1038/s41591-022-02189-0
16. Forde P, Chaft J, Smith K, Anagnostou V, Cottrell T, Hellmann M, et al. Neoadjuvant PD-1 blockade in resectable lung cancer. N Engl J Med (2018) 378(21):1976–86. doi: 10.1056/NEJMoa1716078
17. Hwang M, Canzoniero J, Rosner S, Zhang G, White J, Belcaid Z, et al. Peripheral blood immune cell dynamics reflect antitumour immune responses and predict clinical response to immunotherapy. J Immunother Cancer (2022) 10(6):e004688. doi: 10.1136/jitc-2022-004688
18. Rothschild S, Zippelius A, Eboulet E, Savic Prince S, Betticher D, Bettini A, et al. SAKK 16/14: durvalumab in addition to neoadjuvant chemotherapy in patients with stage IIIA(N2) non-small-cell lung cancer-A multicenter single-arm phase II trial. J Clin Oncol: Off J Am Soc Clin Oncol (2021) 39(26):2872–80. doi: 10.1200/JCO.21.00276
19. Forde P, Spicer J, Lu S, Provencio M, Mitsudomi T, Awad M, et al. Neoadjuvant nivolumab plus chemotherapy in resectable lung cancer. N Engl J Med (2022) 386(21):1973–85. doi: 10.1056/NEJMoa2202170
20. Leidner R, Crittenden M, Young K, Xiao H, Wu Y, Couey M, et al. Neoadjuvant immunoradiotherapy results in high rate of complete pathological response and clinical to pathological downstaging in locally advanced head and neck squamous cell carcinoma. J Immunother Cancer (2021) 9(5):e002485. doi: 10.1136/jitc-2021-002485
21. Daro-Faye M, Kassouf W, Souhami L, Marcq G, Cury F, Niazi T, et al. Combined radiotherapy and immunotherapy in urothelial bladder cancer: harnessing the full potential of the anti-tumour immune response. World J Urol (2021) 39(5):1331–43. doi: 10.1007/s00345-020-03440-4
22. Wang Z, Qiang Y, Shen Q, Zhu X, Song Y. Neoadjuvant programmed cell death protein 1 blockade combined with stereotactic body radiation therapy for stage III(N2) non-small cell lung cancer: a case series. Front Oncol (2022) 12:779251. doi: 10.3389/fonc.2022.779251
23. Rodríguez Plá M, Dualde Beltrán D, Ferrer Albiach E. Immune checkpoints inhibitors and SRS/SBRT synergy in metastatic non-small-cell lung cancer and melanoma: a systematic review. Int J Mol Sci (2021) 22(21):11621. doi: 10.3390/ijms222111621
24. Azghadi S, Daly M. Radiation and immunotherapy combinations in non-small cell lung cancer. Cancer Treat Res Commun (2021) 26:100298. doi: 10.1016/j.ctarc.2020.100298
25. Simone C, Burri S, Heinzerling J. Novel radiotherapy approaches for lung cancer: combining radiation therapy with targeted and immunotherapies. Trans Lung Cancer Res (2015) 4(5):545–52. doi: 10.3978/j.issn.2218-6751.2015.10.05
26. Tubin S, Yan W, Mourad W, Fossati P, Khan M. The future of radiation-induced abscopal response: beyond conventional radiotherapy approaches. Future Oncol (London England) (2020) 16(16):1137–51. doi: 10.2217/fon-2020-0063
27. Muraro E, Furlan C, Avanzo M, Martorelli D, Comaro E, Rizzo A, et al. Local high-dose radiotherapy induces systemic immunomodulating effects of potential therapeutic relevance in oligometastatic breast cancer. Front Immunol (2017) 8:1476. doi: 10.3389/fimmu.2017.01476
28. Vaupel P, Multhoff G. Adenosine can thwart antitumor immune responses elicited by radiotherapy: Therapeutic strategies alleviating protumor ADO activities. Strahlenther Onkol (2016) 192(5):279–87. doi: 10.1007/s00066-016-0948-1
29. Palermo B, Bottero M, Panetta M, Faiella A, Sperduti I, Masi S, et al. Stereotactic ablative radiation therapy in 3 fractions induces a favorable systemic immune cell profiling in prostate cancer patients. Oncoimmunology (2023) 12(1):2174721. doi: 10.1080/2162402X.2023.2174721
30. Zhu M, Yang M, Zhang J, Yin Y, Fan X, Zhang Y, et al. Immunogenic cell death induction by ionizing radiation. Front Immunol (2021) 12:705361. doi: 10.3389/fimmu.2021.705361
31. Ahmed A, Tait S. Targeting immunogenic cell death in cancer. Mol Oncol (2020) 14(12):2994–3006. doi: 10.1002/1878-0261.12851
32. Li Z, Lai X, Fu S, Ren L, Cai H, Zhang H, et al. Immunogenic cell death activates the tumour immune microenvironment to boost the immunotherapy efficiency. Adv Sci (Weinheim Baden-Wurttemberg Germany) (2022) 9(22):e2201734. doi: 10.1002/advs.202201734
33. Zhou P, Chen D, Zhu B, Chen W, Xie Q, Wang Y, et al. Stereotactic body radiotherapy is effective in modifying the tumour genome and tumour immune microenvironment in non-small cell lung cancer or lung metastatic carcinoma. Front Immunol (2020) 11:594212. doi: 10.3389/fimmu.2020.594212
34. Rao A, Liu Y, von Eyben R, Hsu C, Hu C, Rosati L, et al. Multiplex proximity ligation assay to identify potential prognostic biomarkers for improved survival in locally advanced pancreatic cancer patients treated with stereotactic body radiation therapy. Int J Radiat Oncol Biol Phys (2018) 100(2):486–9. doi: 10.1016/j.ijrobp.2017.10.001
35. Choi C, Jeong M, Park Y, Son C, Lee H, Koh E. Combination treatment of stereotactic body radiation therapy and immature dendritic cell vaccination for augmentation of local and systemic effects. Cancer Res Treat (2019) 51(2):464–73. doi: 10.4143/crt.2018.186
36. Singh A, Winslow T, Kermany M, Goritz V, Heit L, Miller A, et al. A pilot study of stereotactic body radiation therapy combined with cytoreductive nephrectomy for metastatic renal cell carcinoma. Clin Cancer Res (2017) 23(17):5055–65. doi: 10.1158/1078-0432.CCR-16-2946
37. Guo L, Ding G, Xu W, Lu Y, Ge H, Jiang Y, et al. Prognostic biological factors of radiation pneumonitis after stereotactic body radiation therapy combined with pulmonary perfusion imaging. Exp Ther Med (2019) 17(1):244–50. doi: 10.3892/etm.2018.6936
38. Friedrich M, Neri P, Kehl N, Michel J, Steiger S, Kilian M, et al. The pre-existing T cell landscape determines the response to bispecific T cell engagers in multiple myeloma patients. Cancer Cell (2023) 41(4):711–25.e6. doi: 10.1016/j.ccell.2023.02.008
39. Lee H, Guo Y, Ross J, Schoen S, Degertekin F, Arvanitis C. Spatially targeted brain cancer immunotherapy with closed-loop controlled focused ultrasound and immune checkpoint blockade. Sci Adv (2022) 8(46):eadd2288. doi: 10.1126/sciadv.add2288
40. Garnett C, Palena C, Chakraborty M, Tsang K, Schlom J, Hodge J. Sublethal irradiation of human tumour cells modulates phenotype resulting in enhanced killing by cytotoxic T lymphocytes. Cancer Res (2004) 64(21):7985–94. doi: 10.1158/0008-5472.CAN-04-1525
41. Guo Y, Shen R, Wang F, Wang Y, Xia P, Wu R, et al. Carbon ion irradiation induces DNA damage in melanoma and optimizes the tumour microenvironment based on the cGAS-STING pathway. J Cancer Res Clin Oncol (2023) 149(9):6315–28. doi: 10.1007/s00432-023-04577-6
42. Mireştean C, Iancu R, Iancu D. Immunotherapy and radiotherapy as an antitumoral long-range weapon-A partnership with unsolved challenges: dose, fractionation, volumes, therapeutic sequence. Curr Oncol (Toronto Ont) (2022) 29(10):7388–95. doi: 10.3390/curroncol29100580
43. Ji D, Song C, Li Y, Xia J, Wu Y, Jia J, et al. Combination of radiotherapy and suppression of Tregs enhances abscopal antitumour effect and inhibits metastasis in rectal cancer. J Immunother Cancer (2020) 8(2):e000826. doi: 10.1136/jitc-2020-000826
44. Frey B, Rückert M, Deloch L, Rühle P, Derer A, Fietkau R, et al. Immunomodulation by ionizing radiation-impact for design of radio-immunotherapies and for treatment of inflammatory diseases. Immunol Rev (2017) 280(1):231–48. doi: 10.1111/imr.12572
45. Ashrafizadeh M, Farhood B, Eleojo Musa A, Taeb S, Rezaeyan A, Najafi M. Abscopal effect in radioimmunotherapy. Int Immunopharmacol (2020) 85:106663. doi: 10.1016/j.intimp.2020.106663
46. Kimura T, Fujiwara T, Kameoka T, Adachi Y, Kariya S. The current role of stereotactic body radiation therapy (SBRT) in hepatocellular carcinoma (HCC). Cancers (2022) 14(18):4383. doi: 10.3390/cancers14184383
47. Cloughesy T, Mochizuki A, Orpilla J, Hugo W, Lee A, Davidson T, et al. Neoadjuvant anti-PD-1 immunotherapy promotes a survival benefit with intratumoral and systemic immune responses in recurrent glioblastoma. Nat Med (2019) 25(3):477–86. doi: 10.1038/s41591-018-0337-7
48. Liu Y, Dong Y, Kong L, Shi F, Zhu H, Yu J. Abscopal effect of radiotherapy combined with immune checkpoint inhibitors. J Hematol Oncol (2018) 11(1):104. doi: 10.1186/s13045-018-0647-8
49. Ngwa W, Irabor O, Schoenfeld J, Hesser J, Demaria S, Formenti S. Using immunotherapy to boost the abscopal effect. Nat Rev Cancer (2018) 18(5):313–22. doi: 10.1038/nrc.2018.6
50. Demaria S, Ng B, Devitt M, Babb J, Kawashima N, Liebes L, et al. Ionizing radiation inhibition of distant untreated tumors (abscopal effect) is immune mediated. Int J Radiat Oncol Biol Phys (2004) 58(3):862–70. doi: 10.1016/j.ijrobp.2003.09.012
51. Dammeijer F, van Gulijk M, Mulder E, Lukkes M, Klaase L, van den Bosch T, et al. The PD-1/PD-L1-checkpoint restrains T cell immunity in tumor-draining lymph nodes. Cancer Cell (2020) 38(5):685–700.e8. doi: 10.1016/j.ccell.2020.09.001
52. Huang Q, Wu X, Wang Z, Chen X, Wang L, Lu Y, et al. The primordial differentiation of tumor-specific memory CD8 T cells as bona fide responders to PD-1/PD-L1 blockade in draining lymph nodes. Cell (2022) 185(22):4049–66.e25. doi: 10.1016/j.cell.2022.09.020
53. Lee Y, Auh S, Wang Y, Burnette B, Wang Y, Meng Y, et al. Therapeutic effects of ablative radiation on local tumour require CD8+ T cells: changing strategies for cancer treatment. Blood (2009) 114(3):589–95. doi: 10.1182/blood-2009-02-206870
54. Walker J, Rolig A, Charych D, Hoch U, Kasiewicz M, Rose D, et al. NKTR-214 immunotherapy synergizes with radiotherapy to stimulate systemic CD8 T cell responses capable of curing multi-focal cancer. J Immunother Cancer (2020) 8(1):e000464. doi: 10.1136/jitc-2019-000464
55. Pieper A, Rakhmilevich A, Spiegelman D, Patel R, Birstler J, Jin W, et al. Combination of radiation therapy, bempegaldesleukin, and checkpoint blockade eradicates advanced solid tumors and metastases in mice. J Immunother Cancer (2021) 9(6):e002715. doi: 10.1136/jitc-2021-002715
56. Dewan M, Galloway A, Kawashima N, Dewyngaert J, Babb J, Formenti S, et al. Fractionated but not single-dose radiotherapy induces an immune-mediated abscopal effect when combined with anti-CTLA-4 antibody. Clin Cancer Res: an Off J Am Assoc Cancer Res (2009) 15(17):5379–88. doi: 10.1158/1078-0432.CCR-09-0265
57. Schaue D, Ratikan J, Iwamoto K, McBride W. Maximizing tumour immunity with fractionated radiation. Int J Radiat Oncol Biol Phys (2012) 83(4):1306–10. doi: 10.1016/j.ijrobp.2011.09.049
58. Savage T, Pandey S, Guha C. Postablation modulation after single high-dose radiation therapy improves tumour control via enhanced immunomodulation. Clin Cancer Res: an Off J Am Assoc Cancer Res (2020) 26(4):910–21. doi: 10.1158/1078-0432.CCR-18-3518
59. Shaverdian N, Lisberg A, Bornazyan K, Veruttipong D, Goldman J, Formenti S, et al. Previous radiotherapy and the clinical activity and toxicity of pembrolizumab in the treatment of non-small-cell lung cancer: a secondary analysis of the KEYNOTE-001 phase 1 trial. Lancet Oncol (2017) 18(7):895–903. doi: 10.1016/S1470-2045(17)30380-7
60. Shen P, Qiao B, Jin N, Wang S. Neoadjuvant immunoradiotherapy in patients with locally advanced oral cavity squamous cell carcinoma: a retrospective study. Investigational New Drugs (2022) 40(6):1282–9. doi: 10.1007/s10637-022-01293-9
61. Chang J, Lin S, Dong W, Liao Z, Gandhi S, Gay C, et al. Stereotactic ablative radiotherapy with or without immunotherapy for early-stage or isolated lung parenchymal recurrent node-negative non-small-cell lung cancer: an open-label, randomised, phase 2 trial. Lancet (London England) (2023) S0140-6736(23)01406-X. doi: 10.1016/S0140-6736(23)01384-3
62. Moran A, Azghadi S, Maverakis E, Christensen S, Dyer B. Combined immune checkpoint blockade and stereotactic ablative radiotherapy can stimulate response to immunotherapy in metastatic melanoma: a case report. Cureus (2019) 11(2):e4038. doi: 10.7759/cureus.4038
63. Welsh J, Menon H, Chen D, Verma V, Tang C, Altan M, et al. Pembrolizumab with or without radiation therapy for metastatic non-small cell lung cancer: a randomized phase I/II trial. J Immunother Cancer (2020) 8(2):e001001. doi: 10.1136/jitc-2020-001001
64. McBride S, Sherman E, Tsai C, Baxi S, Aghalar J, Eng J, et al. Randomized phase II trial of nivolumab with stereotactic body radiotherapy versus nivolumab alone in metastatic head and neck squamous cell carcinoma. J Clin Oncol: Off J Am Soc Clin Oncol (2021) 39(1):30–7. doi: 10.1200/JCO.20.00290
65. Guo W, Zhang C, Qiao T, Zhao J, Shi C. Strategies for the construction of mouse models with humanized immune system and evaluation of tumour immune checkpoint inhibitor therapy. Front Oncol (2021) 11:673199. doi: 10.3389/fonc.2021.673199
66. Dovedi S, Adlard A, Lipowska-Bhalla G, McKenna C, Jones S, Cheadle E, et al. Acquired resistance to fractionated radiotherapy can be overcome by concurrent PD-L1 blockade. Cancer Res (2014) 74(19):5458–68. doi: 10.1158/0008-5472.CAN-14-1258
67. Yamauchi T, Hoki T, Oba T, Jain V, Chen H, Attwood K, et al. T-cell CX3CR1 expression as a dynamic blood-based biomarker of response to immune checkpoint inhibitors. Nat Commun (2021) 12(1):1402. doi: 10.1038/s41467-021-21619-0
68. Deng H, Zhao Y, Cai X, Chen H, Cheng B, Zhong R, et al. PD-L1 expression and tumour mutation burden as pathological response biomarkers of neoadjuvant immunotherapy for early-stage non-small cell lung cancer: A systematic review and meta-analysis. Crit Rev Oncol/Hematol (2022) 170:103582. doi: 10.1016/j.critrevonc.2022.103582
69. Hiam-Galvez K, Allen B, Spitzer M. Systemic immunity in cancer. Nat Rev Cancer (2021) 21(6):345–59. doi: 10.1038/s41568-021-00347-z
Keywords: non-small cell cancer (NSCLC), stereotactic body radiation therapy (SBRT), neoadjuvant therapy, immunotherapy, biomarker
Citation: Shi Y, Ma X, He D, Dong B and Qiao T (2023) Neoadjuvant SBRT combined with immunotherapy in NSCLC: from mechanisms to therapy. Front. Immunol. 14:1213222. doi: 10.3389/fimmu.2023.1213222
Received: 27 April 2023; Accepted: 24 July 2023;
Published: 03 August 2023.
Edited by:
Gulderen Yanikkaya Demirel, Yeditepe University, TürkiyeReviewed by:
Fiori Alite, Geisinger Commonwealth School of Medicine, United StatesCharles B. Simone, Memorial Sloan Kettering Cancer Center, United States
Copyright © 2023 Shi, Ma, He, Dong and Qiao. This is an open-access article distributed under the terms of the Creative Commons Attribution License (CC BY). The use, distribution or reproduction in other forums is permitted, provided the original author(s) and the copyright owner(s) are credited and that the original publication in this journal is cited, in accordance with accepted academic practice. No use, distribution or reproduction is permitted which does not comply with these terms.
*Correspondence: Tianyun Qiao, MTU2NjcwOTc4MTNAMTYzLmNvbQ==; Bingwei Dong, cnhyZmRid0AxMjYuY29t
†These authors have contributed equally to this work