- 1Department of Allergy and Immunology, IIS-Fundación Jiménez Díaz, Universidad Autónoma de Madrid (UAM), Madrid, Spain
- 2Allergy Department, Hospital Infantil Universitario Niño Jesús, Fundación Hospital Niño Jesús (HNJ), Instituto de Investigación del Hospital de La Princesa (IIS-P), Madrid, Spain
- 3Department of Pediatric Onco-Hematology and Cell and Gene Therapy, Bambino Gesù Children’s Hospital, Istituti di Ricovero e Cura a Carattere Scientifico (IRCCS), Rome, Italy
- 4Department of Veterinary Medicine, University of Perugia, Perugia, Italy
- 5Centro de Biotecnología y Genómica de Plantas, Universidad Politécnica de Madrid-Instituto Nacional de Investigación y Tecnología Agraria y Alimentaria (UPM-INIA), Universidad Politécnica de Madrid, Madrid, Spain
- 6Department of Allergy. Fundación Jiménez Díaz University Hospital, Universidad Autónoma de Madrid (UAM), Madrid, Spain
- 7Allergy Unit, Allergo-Anaesthesia Unit, Cruz Roja Central Hospital, Villanueva de la Cañada, Madrid, Spain
- 8Faculty of Medicine and Biomedicine, Universidad Alfonso X el Sabio (UAX), Madrid, Spain
Introduction: Anaphylaxis is among the most severe manifestations of allergic disorders, but its molecular basis remains largely unknown and reliable diagnostic markers are not currently available. MicroRNAs (miRNAs) regulate several pathophysiological processes and have been proposed as non-invasive biomarkers. Therefore, this study aims to evaluate their involvement in anaphylactic reaction and their value as biomarkers.
Methods: Acute (anaphylaxis) and baseline (control) serum samples from 67 patients with anaphylaxis were studied. Among them, 35 were adults with drug-induced anaphylaxis, 13 adults with food-induced anaphylaxis and 19 children with food-induced anaphylaxis. The circulating serum miRNAs profile was characterized by next-generation sequencing (NGS). For this purpose, acute and baseline samples from 5 adults with drug-induced anaphylaxis were used. RNA was extracted, retrotranscribed, sequenced and the readings obtained were mapped to the human database miRBase_20. In addition, a system biology analysis (SBA) was performed with its target genes and revealed pathways related to anaphylactic mediators signaling. Moreover, functional and molecular endothelial permeability assays were conducted with miR-375-3p-transfected cells in response to cAMP.
Results: A total of 334 miRNAs were identified, of which 21 were significant differentially expressed between both phases. Extracellular vesicles (EVs) were characterized by Western blot, electron microscopy and NanoSight. A decrease of miR-375-3p levels was determined by qPCR in both serum and EVs of patients with anaphylaxis (****p<.0001). Precisely, the decrease of miR-375-3p correlated with the increase of two inflammatory cytokines: monocyte chemoattractant protein-1 (MCP-1) and granulocyte macrophage colony-stimulating factor (GM-CSF). On the other hand, functional and molecular data obtained showed that miR-375-3p partially blocked the endothelial barrier maintenance and stabilization by disassembly of cell-cell junctions exhibiting low Rac1-Cdc42 levels.
Discussion: These findings demonstrate a differential serum profile of circulating miRNAs in patients with anaphylaxis and exhibit the miR-375-3p modulation in serum and EVs during drug- and food-mediated anaphylactic reactions. Furthermore, the in silico and in vitro studies show a negative role for miR-375-3p/Rac1-Cdc42 in the endothelial barrier stability.
Introduction
Anaphylaxis is a potentially life-threatening hypersensitivity reaction and one of the most severe manifestation of allergic disorders (1). The major triggers of this pathological event include drugs, foods and Hymenoptera venoms. Specifically, drugs are the main allergens provoking anaphylaxis in adults, while foods are the most common cause of reaction in children (2, 3).
The diagnosis of anaphylaxis is based on clinical symptoms, but sometimes remains elusive as some manifestations are common to many other pathologies (4), and clinical criteria are not fully consistent across different expert consensus (5). Currently, the main biomarker used in clinical practice to complement diagnosis is serum tryptase. However, this molecule is not increased in many cases, with poor reliability in mild cases where it is most needed to aid in the differential diagnosis process (6). Therefore, it of utmost relevance to find novel and reliable molecular markers that allows us to enlarge the diagnosis of this pathological event.
Mechanistically, anaphylaxis is mainly produced by IgE-mediated activation of reaction’s effector cells, mast cells and basophils (7). However, other IgE-independent molecular processes and cells are involved in this pathological event (8). Overall, there is a release of mediators that gives rise to signs and symptoms of anaphylaxis (9). Among them, the cutaneous ones are the most frequent, although the respiratory and cardiovascular systems mark the development of the most severe cases (10). In turn, vessels´ endothelium is the main component affected during anaphylaxis. This structure, composed of an extensive monolayer of endothelial cells (ECs), forms a physical barrier between blood and tissues allowing selective transport of molecules (11). Mediators released by effector cells act on ECs destabilizing the monolayer and leading to increased endothelial permeability and fluid extravasation through mechanisms that mainly regulates disruption of cell junctions and cytoskeletal disability (12).
MicroRNAs (miRNAs) are small non-coding RNA molecules (~22 nucleotides) that can be found circulating in serum and other body fluids associated with protein complexes or within extracellular vesicles (EVs) (13). In addition, due to their stability and their ability to mirror the pathophysiological state of the patient, they have been proposed as promising non-invasive biomarkers (14). miRNA transcripts are processed, resulting in a mature duplex miRNA which strands separate giving rise to the RNA-induced silencing complex (RISC) that regulates the translation of messenger RNAs (mRNAs) (15). miRNAs modulate many physiological functions (13, 16). Specifically, those transported by EVs may mediate communication between different tissues (13). Therefore, miRNAs levels changes are relevant in a wide range of allergic diseases (16). Precisely, our group reported the increase of miR-21-3p and miR-487b-3p levels during the acute phase of anaphylaxis in a study including 19 children with food-induced reactions (17). Moreover, elevated values of miR-451a have also been described in 16 adults with food- and Hymenoptera venom-mediated anaphylaxis (18). However, miRNAs levels have never been characterized in adults with drug-mediated reactions.
Therefore, this study aims to determine the serum circulating miRNA profile in adults with drug-anaphylactic mediated reactions, to evaluate their capacity as diagnostic biomarkers and to investigate the possible involvement of these molecules in the underlying molecular basis of the reaction.
Materials and methods
Study population
Initial population included 204 paired sera from 102 patients presenting anaphylaxis and recruited from three Spanish hospitals (Fundación Jiménez Díaz University Hospital, Cruz Roja Central Hospital and Niño Jesús University Children’s Hospital). However, 35 of them were discarded due to the hemolysis of their samples.
Anaphylaxis diagnosis was confirmed by an allergist in agreement with the definition of anaphylaxis established by the “National Institute of Allergy and Infectious Disease and Food Allergy and Anaphylaxis Network” (19). In addition, from each patient were recorder their gender, age, the trigger of the reaction and if they were treated before or after obtaining the acute sample. Furthermore, their clinical signs and symptoms were also collected and, based on these, the severity of the reaction was determined according to the criteria established by Brown (20).
The study was approved by the Ethics Committee (CEIm FJD, PIC057-19), authors adhered to the declaration of Helsinki and all patients were included after giving informed consent by the donors or their relatives. Inclusion criteria were acceptance to participate in the study and an objective diagnosis of the reaction. Exclusion criteria were the presence of a blood-borne disease and/or any psychic or psychological diseases that would prevent acceptance for the study.
Sample collection
Serum samples were collected from each patient under two conditions: during acute phase (anaphylaxis) and at basal phase (control), at least 14 days after the reaction. Considering the heterogeneity of participants, the acute sample data was normalized by calculating the increase or decrease ratio upon baseline individual sample values (ratio acute/basal).
All samples were obtained from patients who developed anaphylaxis accidentally. If the allergic reaction occurred accidentally, acute phases were collected in emergency departments. On the other hand, if the hypersensitivity reaction was provoked by a controlled challenge test, sera were obtained in allergy units.
For serum processing, tubes with a separator gel (BD Vacutainer) were used. Once the peripheral blood was obtained, it was centrifuged at 1,200 g for 10 minutes at 4°C. Afterwards, the serum was aliquoted in 0.5 ml and stored at -80°C until its use in each of the experiments.
Profile of serum circulating miRNAs
The serum circulating miRNAs profile was determined by Next Generation Sequencing (NGS) at Qiagen Genomic Services, as previously described by Nuñez-Borque et al. (17, 21). For the analysis, acute and baseline samples from 5 adult patients with drug anaphylaxis were tested in the same batch.
RNA was extracted from 200 μl of serum using the miRNeasy Serum/Plasma Kit (Qiagen). Moreover, 52 exogenous sequences were included to confirm the correct isolation of miRNAs. In turn, libraries were performed with the QIAseq miRNA Library Kit (Qiagen). For this purpose, RNA was retrotranscribed to copy DNA (cDNA), amplified by PCR (22 cycles) and purified from samples. Libraries were carried out by a gel-free system through the addition of Unique Molecular Identifiers (UMIs) and were specific for small RNA species ranging from 15-40 nucleotides. Quality controls were determined using the Bioanalyzer 2100 or the TapeStation 4200 (Agilent Technologies). In turn, miRNAs data were normalized to equimolar ratios depending on the quality of the library, the quality of the inserts and the concentration measurements. Finally, libraries were quantified by quantitative PCR (qPCR) and sequenced on an Illumina NextSeq500 instrument. Raw data files (FASTQ) were generated for each sample using the bcl2fastq program (Illumina Inc.) and Cutadapt (1.11) software was utilized to remove adapter sequences and collapse the reads by UMIs. Readings were mapped against the miRbase_20 database using the Bowtie2 program (2.2.2) and no errors or more than 1 mismatch were allowed.
Serum miRNAs extraction, retrotranscription and quantification
To the validation step, paired samples from each patient were always analyzed simultaneously. Serum miRNAs were extracted using the miRNeasy Serum/Plasma Advanced kit (Qiagen). For each sample, 300 μl of serum were used and added 90 μl of lysis buffer and 1 μl of UniSp2/4/5 mix (miRCURY LNA RNA Spike-in kit, Qiagen), synthetic miRNAs that serve as a quality control of the technique. Following this, samples were incubated for 3 minutes at room temperature, 30 μl of precipitation buffer were added, and a new 3-minute incubation at room temperature was performed. Tubes were centrifuged at 12,000 g for 3 minutes, supernatants were mixed with an equal volume of isopropanol and transferred to a specific column where RNA was retained. Subsequently, three washes were performed and 15 μl of RNAase-free water was added to the column, which was incubated for 1 minute at room temperature and centrifuged for 1 minute to elute the isolated RNA.
Total RNA was retrotranscribed using the miRCURY LNA RT kit (Qiagen). For this purpose, 2 μl of the reaction-specific buffer, 4.5 μl of RNAase-free water, 1 μl of the enzyme mixture, 2 μl of the RNA previously obtained in the extraction and 0.5 μl of UniSp6, a synthetic miRNA used as a quality control of the reverse transcription, were included for each sample. Once the mix was done, samples were placed in the PTC-100 Thermal Cycler (MJ research, Inc) where retrotranscription was carried out.
Finally, miRNAs quantification (miRCURY LNA SYBR Green PCR kit, Qiagen) was performed by quantitative PCR (qPCR) with specific primers (Qiagen) in the LightCycle96 Real Time PCR System (Roche Life Science). Samples from each patient were always measured at the same time and in the same batch. UniSp2, UniSp4, UniSp5 and UniSp6 were used as exogenous controls of the extraction and reverse transcription, while miR-451a and miR-23a-3p were measured as endogenous controls of sample hemolysis (22, 23). Moreover, miR-30e-5p was chosen as endogenous control for the normalization of the target miRNAs, as suggested by the NGS according to the levels of all molecules identified. Data obtained were analyzed using the 2-ΔΔCT method (24).
Purification of extracellular vesicles
The purification of EVs from serum was performed using the miRCURY Exosome isolation Kit – Serum and Plasma (Qiagen) according to Colletti et al. (25). For this purpose, 500 μl of serum were centrifuged at 12,000 g for 20 minutes at 10°C. Subsequently, 200 μl of the precipitate buffer were added to the supernatant and the mix was incubated for 3 hours at 4°C. After this time, samples were centrifuged at 1,500 g for 30 minutes and supernatants were removed. Finally, EVs were collected adding 270 μl of the resuspension buffer.
Suitable isolation and characterization of EVs was determined by Western blot (WB), nanoparticle tracking analysis (NTA) and electron microscopy (EM) as previously described (26) and following MISEV2018 guidelines (27). Subsequently, the extraction, retrotranscription and quantification of miRNAs contained in EVs were carried out as previously described in the section “Serum miRNAs extraction, retrotranscription and quantification”. Paired samples from each patient were always analyzed simultaneously.
Western blot
Three bona fide markers (CD9, CD63 and TSG101) of EVs were characterized by WB. Proteins were separated by electrophoresis on sodium dodecyl sulfate polyacrylamide gels (SDS-PAGE) at 12.5% using the PowerPac Basic Power Supply section (BioRad). Subsequently, proteins were transferred to a nitrocellulose membrane (BioRad) using the Trans-Blot Turbo Transfer system (BioRad). Non-specific membrane junctions were blocked by applying a solution of phosphate buffered saline (PBS) with 0.1% Tween (Sigma) and 5% milk. After this, the membrane was incubated overnight with the corresponding primary antibodies diluted 1:500 in all cases: anti-CD9 (Invitrogen), anti-CD63 (Invitrogen) and anti-TSG101 (Abcam). For signal detection, the membrane was incubated for 1 hour with a rabbit anti-mouse (RAM) peroxidase-conjugated secondary antibody (Jackson Laboratory) diluted at 1:5,000 in 0.05% PBS-Tween solution with 3% milk. Lastly, proteins were detected in the Amersham Imager 600 system (GE Healthcare) using the chemiluminescent ECL Prime Western Blotting Detection Reagent (Thermo Scientific).
Electron microscopy
For the EVs visualization by EM, 5 μl of the previously purified particles were fixed for 24 hours at 4°C using 50 μl of PBS with 2% paraformaldehyde (PFA, Sigma). After this, EVs were adsorbed on copper/carbon grids (Fedelco) for 3 minutes, washed with water for 1 minute and counterstained with 1% uranyl acetate for 30 seconds. Then, samples were observed using a JEOL 1010 transmission EM (JEOL) and employing a voltage of 100 kV. The analysis and image processing were carried out with the Soft Imaging Viewer software.
Nanoparticle tracking analysis
NTA was performed at the Spanish National Cancer Research Center (CNIO, Madrid) with the NS500 nanoparticle characterization system (NanoSight) equipped with a blue laser (405 nm) and diluting samples 1:500.
System biology analysis
Systems Biology Analysis (SBA) of miR-375-3p was performed in silico using all its target genes with more than 50 target score (269 genes) in the miRDB database (http://mirdb.org/) (28). All genes were mapped against their corresponding gene ontology (GO) in the Ingenuity Pathway Knowledge Base using the Ingenuity Pathway Analysis (IPA) software (Qiagen). This program provided different functional categories: “ingenuity canonical pathways”, “upstream regulators”, “disease and disorders”, “network” and “tox lists”. In addition, it evaluated the significance using a one-tailed Fisher’s test. Among the different functional categories obtained, “disease and disorders” and “ingenuity canonical pathways” were the only one considered.
Quantification of serum cytokines
Serum cytokine levels were determined using the multiplex assay RayPlex Human Cytokine Storm Array 1 Kit (RayBiotech). For this purpose, acute and basal samples from 40 patients with anaphylaxis were studied. Dilution of the samples and standards was performed according to the manufacture indications. A panel of 25 mediators involved in the inflammatory process was tested: basic Fibroblast growth factor (bFGF), Eotaxin, Granulocyte colony-stimulating factor (G-CSF), Granulocyte macrophage colony-stimulating factor (GM-CSF), Interferon-γ (IFNγ), Interleucin-10 (IL-10), IL-12p70, IL-13, IL-15, IL-17A, IL-1β, IL-1RA, IL-2, IL-4, IL-5, IL-6, IL-7, IL-8, Monocyte chemoattractant protein-1 (MCP-1), Macrophage inflammatory protein-1α (MIP-1α), MIP-1β, Platelet-derived growth factor-BB (PDGF-BB), RANTES, Tumor necrosis factor-α (TNFα), Vascular endothelial growth factor (VEGF). Mean fluorescent intensities of the samples and standards were determined in a paired manner using a BD FACSCanto II flow cytometer (Becton Dickinson).
Transfection of miRNA
Human dermal microvascular ECs (HMVEC-D) were acquired from Lonza (CC-2543) and maintained in EGM-2MV BulletKit medium, as previously described (29). First, HMVEC-D were seeded and allowed to grow for 24 hours to reach 70-80% confluence. Next day, transfection was conducted incubating cells with Opti-MEM medium (Thermo Scientific) and using Transit-X2 reagent and mimics (50 mM) of the miR-375-3p (Qiagen). In addition, fluorescently labeled mimic scrambles (50 mM) were applied as controls of the technique (Qiagen). Subsequently, after 72 hours, molecular and functional studies were performed in HMVEC-D. In turn, the correct development of the transfection was confirmed by qPCR and confocal microscopy.
miRNA extraction from endothelial cells
Extraction of miRNAs from HMVEC-D was carried out using the MasterPure Complete DNA & RNA Purification Kit (Lucigen). Cells were lysed by adding 300 μl of lysis buffer and 1 μl of Proteinase K. The lysate obtained was collected and incubated at 65°C for 15 minutes. Then, samples were incubated at 4°C for 5 minutes and 150 μl of precipitation buffer were added. Subsequently, each sample was centrifuged at 10,000 g for 10 minutes and supernatants were collected. An equal volume of isopropanol was added, and mixtures were centrifuged again. Next, to remove the remaining DNA, the precipitate was resuspended in 200 μl of the DNA nuclease buffer and 5 μl of DNA nuclease I. Consecutively, samples were incubated at 37°C for 30 minutes and 200 μl of the lysis and precipitation buffers were added. Then, samples were incubated at 4°C for 5 minutes and centrifuged at 10,000 g for 10 minutes at 4°C. After this, an equal volume of isopropanol was added, and samples were centrifuged again. Finally, the RNA extracted was resuspended in 20 μl of trisaminomethane buffer (TE) and 1 μl of RNAse inhibitor. Finally, reverse transcription and miRNAs quantification were performed as previously described in the section “Serum miRNAs extraction, retrotranscription and quantification”.
Protein extraction and quantification from endothelial cells
Cells were seeded and transfected in P6 plates (Corning) as previously described in the “Transfection of miRNA” section. 30 µM cyclic AMP (cAMP) was used to stabilize the endothelial barrier during 30 minutes. Once the incubation time was over, proteins were extracted by adding 100 μl of lysis buffer supplemented with 5 mM protease inhibitor (Sigma), 1 mM phenylmethylsulfonyl fluoride (PMSF, Sigma) and 5 mM dithiothreitol (DTT, BioRad). Cells were detached using a scraper and the lysates obtained were kept in agitation for 15 minutes at 4°C. After this, they were centrifuged for 15 minutes at 12,000 rpm at 4°C and the supernatants, which contained the proteins, were stored at -20°C until use. Rac1-CDC42 levels were analyzed by WB following the protocol previously described in the “Western Blot” section. Membranes were incubated overnight with primary antibody anti-Rac1-Cdc42 (Cell Signaling) diluted 1:500. For signal detection, the membrane was incubated for one hour with a goat anti-rabbit (GAR) peroxidase-conjugated secondary antibody (Jackson Laboratory) diluted at 1:5,000 in 0.05% PBS-Tween soluction with 3% milk. Likewise, an anti-β-actin antibody (Santa Cruz) was used to detect β-actin as a housekeeping protein diluted at 1:500 while the RAM secondary antibody was diluted 1:5,000. Bands intensity was quantified using the ImageJ software and normalization to the amount of total protein loaded was calculated using the ratio of the Rac1-Cdc42/β-actin signal.
Endothelial permeability assay
The endothelial barrier integrity was assessed by measuring the trans-endothelial electrical resistance (TEER) value of the monolayer through an EndOhm chamber (WPI) using 24-well Transwell (TWs) cell culture inserts (26, 29). For determining the effect of miR-375-3p in a cAMP-stabilized endothelial system, HMVEC-Ds were transfected on TW. Four different HMVEC-D systems were registered and analyzed: non transfected, incubated with Transit-X2 alone, transfected with the scramble, as control, and transfected with the miR-375-3p mimic (50 mM). All these systems were evaluated in presence of cAMP and TEER measures were obtained at different times (10, 30, 45, 60, 90, 120 and 180 minutes).
Confocal microscopy
For confocal microscopy HMVEC-D were seeded in P8 chambers (IBIDI). Cells were fixed with 4% PFA for 15 minutes and permeabilized with 0.1% Triton (Sigma) for 5 minutes at 4°C. After this, non-specific junctions were blocked by adding a PBS solution with 2% BSA for 20 minutes. Cells were incubated overnight with an anti-VE-cadherin antibody (Cell Signaling) diluted 1:400 in a solution of PBS with 2% BSA. The next day, cells were incubated for 1 hour with a donkey anti-rabbit Alexa Fluor 647-conjugated secondary antibody (Invitrogen). Then, actin filaments were red stained by incubating HMVEC-D for 30 minutes with Texas-Red-X phalloidin reagent (Invitrogen) at a concentration of 1:40 in a solution of PBS with 2% BSA. Subsequently, a second 10-minutes incubation was carried out with a solution of DAPI (Sigma) at a concentration of 1:1,000 in distilled water to stain the nuclei blue. Lastly, the slides were assembled using the FlourSave Reagent (Calbiochem) and visualized on the LSM 700 inverted confocal microscope (Carl Zeiss). Transfection was confirmed by visualization of the fluorescent scramble (green) inside ECs.
Statistical analysis
Data obtained from NGS were normalized and analyzed with the statistic software R 3.5.3. The principal component analysis (PCA) and the heat map were carried out on the ClustVis website (https://biit.cs.ut.ee/clustvis/) (30). Graphical representation and statistical evaluation were performed by using the Graph Pad Prism 8 software (La Jolla, CA, USA).
Qualitative variables were described as frequency and percentage. However, in quantitative variables, data distribution was always first assessed by normality tests. When data presented a normal distribution, parametric analyses were used, and the graphs were represented by the mean ± standard error of the mean (SEM). On the other hand, when the data did not follow a normal distribution, non-parametric tests were used, and the graphs showed the median ± interquartile range (IQR). Differences were considered significant at level of p<.05.
NGS analysis were performed with the Prostar package (http://live.prostar-proteomics.org/) distributed by Bioconductor and implemented in R (R Core Team, 2019). Abundance data were transformed (log2) using R 3.5.3 software to obtain a symmetrical distribution. Moreover, all miRNAs not detected in at least two patients were discarded. The matrices were normalized with the Cyclic Loess method to reduce systemic variances (31). Finally, Student’s paired t-test was used to determine the significant differences between both conditions (acute vs basal) (32).
For the validation stage and measurements of miRNAs within EVs, data did not follow a normal distribution, so the two-tailed Wilcoxon matched-pairs non-parametric test was used to determine the significant differences between acute and basal phases. In turn, for the characterization of EVs purified from serum, non-parametric tests were used and both size and concentration were analysed with the Wilcoxon matched-pairs test. On the other hand, the study of serum cytokines was carried out using the Wilcoxon matched-pairs test in all cases. In turn, comparison of their levels with those of miR-375-3p was performed using Spearman’s non-parametric correlation test. Moreover, data obtained from in vitro assays followed a normal distribution and, consequently, analyses were performed using the paired t-test or 2 way ANOVA.
Results
Characteristic of the patients´ cohorts
The studied cohort included 67 patients with anaphylaxis recruited from different Spanish hospitals (Supplemental Figure 1). Their clinical characteristics and those of their reactions are shown in Table 1. This population aged between 4 and 76 years old and more than half were females. The most frequent symptoms were cutaneous and respiratory, followed by gastrointestinal, mucosal, cardiovascular and central nervous system manifestations. Overall, two thirds of the reactions were classified as moderate (Grade 2), while the remaining ones were categorized as severe (Grade 3). Outstandingly, the frequency of Grade 3 cases was lower in children with food-induced reactions compared to any of the two adult populations (11% vs 43% and 46%, respectively). Finally, even though patient health was always prioritized, a percentage of acute samples were obtained immediately before treatment administration. In these subjects, the most used medications were epinephrine, histamine receptor 1 antagonists and corticosteroids.
Identification of circulating serum miRNA profile in patients with drug-induced anaphylaxis
The profile of circulating miRNAs was determined by NGS in an exploratory study carried out in samples from patients with drug anaphylaxis. For this purpose, acute and baseline serum samples were used. The similarity among the biological replicates was verified by the PCA, which exhibited a clear separation between the two stages (Figure 1A). In turn, the correct performance of NGS was confirmed through the analysis of different quality controls (Supplementary Figure 2).
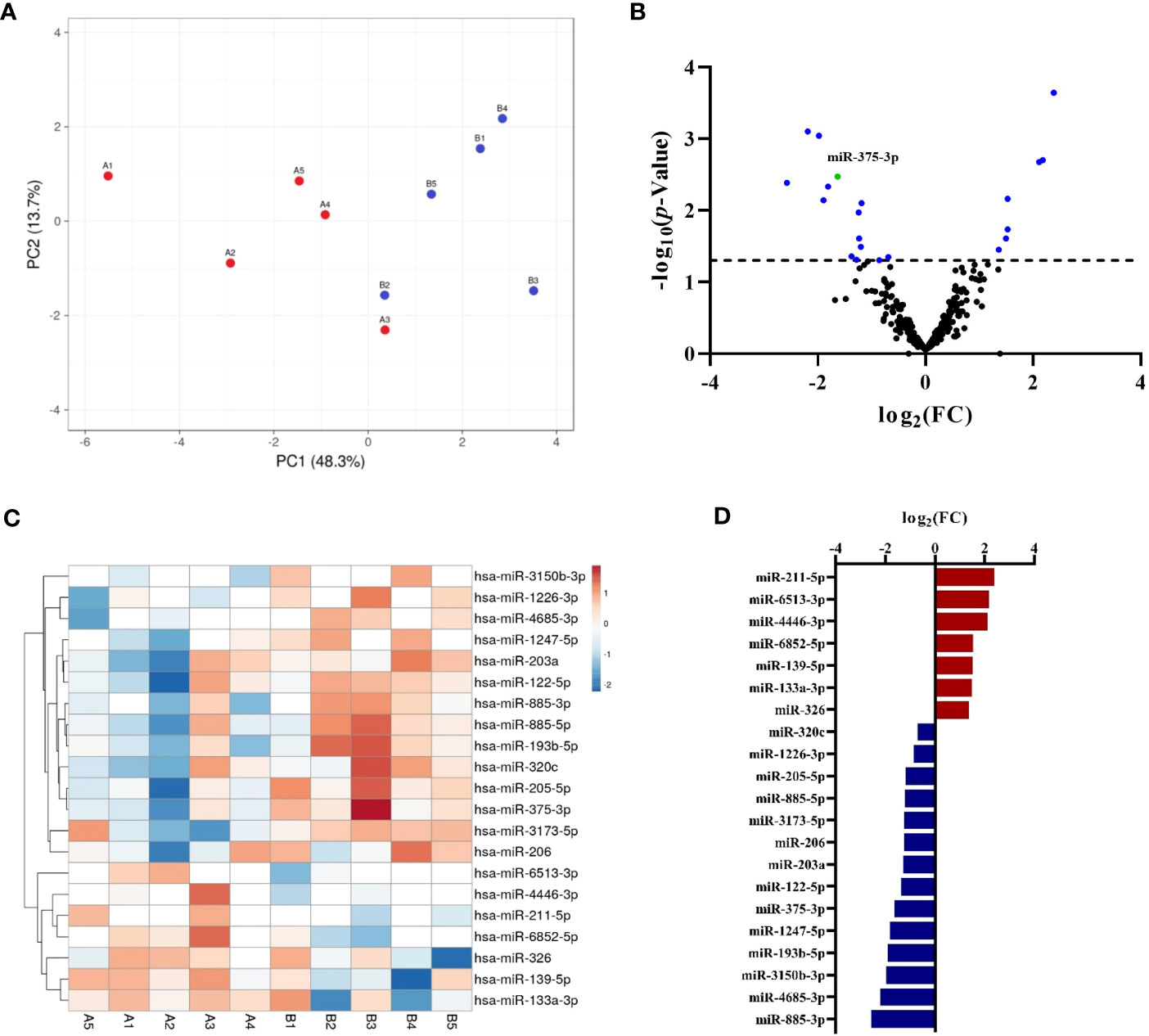
Figure 1 Characterization by NGS of the circulating serum miRNA profile in adults with drug-induced anaphylaxis. (A) The PCA showed similarity among the biological replicates and a separation between the acute (A, red points) and basal (B, blue points) conditions. (B) Volcano plot exhibits the distribution of total miRNAs identified by NGS. Those statistically significant (p<.05) are indicated in blue, whereas miR-375-3p is shown in green. (C) Heat map graphically represents the levels of the statistically significant miRNAs in each sample (A: acute phase, B: basal phase). The units represent the transformed (log2) and normalized (cyclic Loess method) abundance data obtained by NGS. Red color indicates an increase during the acute phase, whereas blue indicates a decrease. Missing data are represented in white. (D) Graphical distribution according to their fold change (FC) of the 14 increased (red) and 7 decreased (blue) miRNAs during the acute phase compared to baseline.
A total of 334 miRNAs were identified (Supplementary Table 1) and after statistical analysis only 21 of them showed significant differences between acute and basal phases (Figure 1B; Supplementary Table 2). Each sample´s signals dispersion of significant miRNAs is depicted in Figure 1C. Among them, 7 were increased during the acute phase, while the remaining 14 were decreased (Figure 1D). Interestingly, more than half of these identified miRNAs have been previously described in allergy, although they have never been associated with anaphylaxis (Supplementary Table 3).
Circulating serum levels of miR-375-3p decrease during the acute phase of anaphylaxis compared to baseline
To evaluate miRNAs capacity as diagnostic biomarkers we extended analysis to 134 samples obtained from 67 patients with anaphylaxis (34 adults with drug-induced reactions and 33 [14 adults and 19 children] with food-induced reactions). 15 patients were discarded from the study because acute and/or basal serum sample presented hemolysis (≥7 cycles) (Figure 2A). Therefore, the final cohort included 52 patients with anaphylaxis: 23 adults with drug-induced reactions and 29 (10 adults and 19 children) with food-induced reactions. In all these cases, UniSp2, UniSp4 and UniSp5 were detected approximately 5-7 cycles apart, confirming the correct development of the extraction (Supplementary Figure 3A). Furthermore, UniSp6 was amplified around cycle 18 demonstrating that reverse transcription had been successfully carried out (Supplementary Figure 3B).
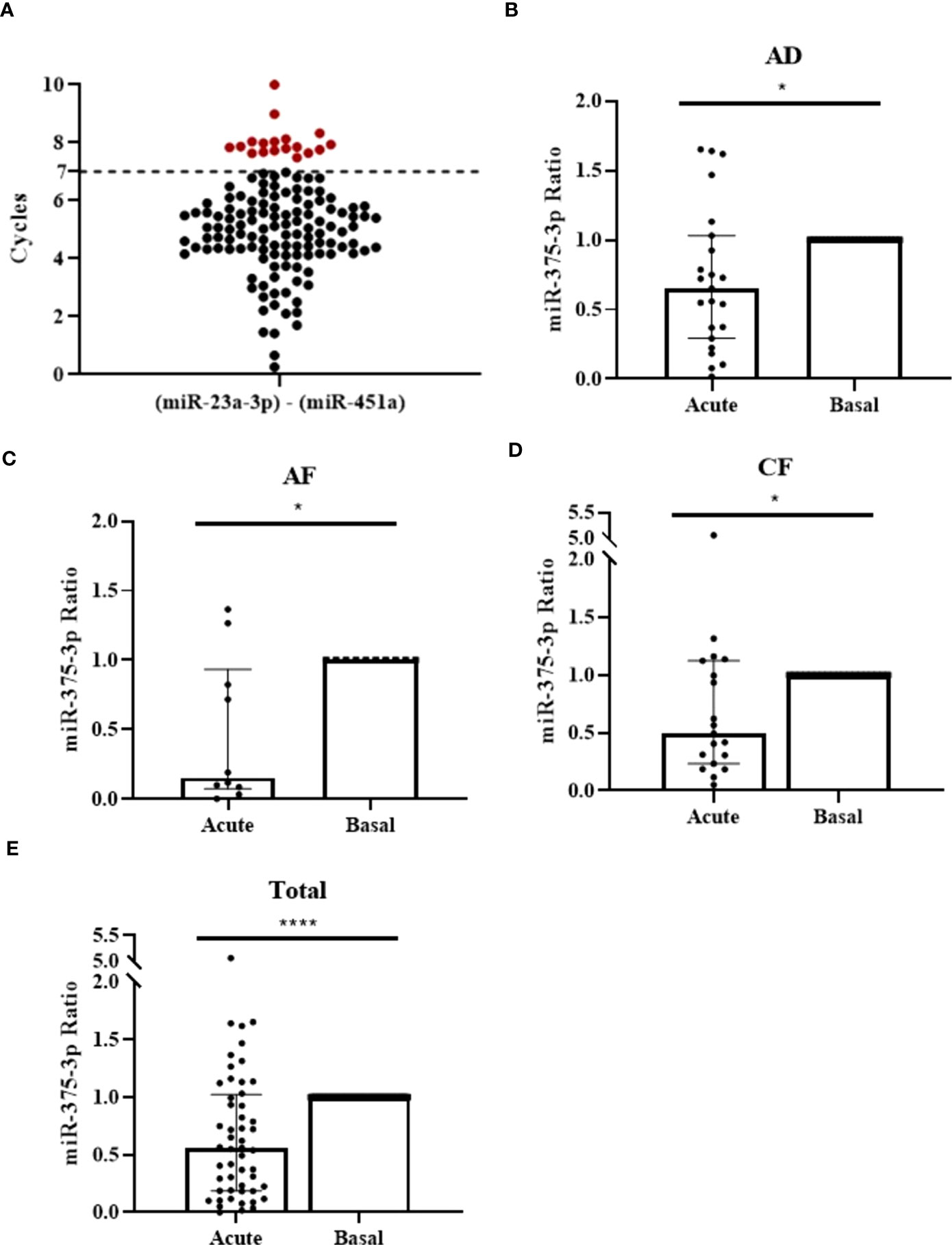
Figure 2 Serum miR-375-3p levels decrease during the reaction in patients with anaphylaxis. (A) Measurement of serum hemolysis by the coefficient between the two endogenous controls used: miR-23a-3p and miR-451a. 18 samples exceeded the established threshold (≥7 cycles) and were excluded from the study (red points). (B) Serum levels of miR-375-3p decrease in adults with drug-induced anaphylaxis during the acute phase of the reaction compared to baseline (*p=.0196; n: 23). Median ± IQR (C) Serum levels of miR-375-3p decrease in adults with food-induced anaphylaxis (*p=.0273; n: 10), (D) children with food-induced anaphylaxis (*p=.0181; n: 19) and (E) total patients (****p<.0001; n: 52) during the acute phase of the reaction compared to baseline. Median ± IQR.
After sample quality control, we choose 6 NGS identified miRNAs (miR-211-5p, miR-139-5p, miR-133a-3p, miR-375-3p, miR-193b-5p, miR-885-3p) to validate their serum level. Statistically significant differences were found exclusively for miR-375-3p, which is decreased during the acute phase of anaphylaxis compared to baseline in adults with drug-induced reactions, as observed in NGS data (FC: -1,63) (Figure 2B and Supplementary Figures 3C–E). To enlarge its value as a plausible anaphylactic biomarker, we extended the study of miR-375-3p into two cohorts of adults and children food-induced reactions, showing a decrease which was consistent with the results observed in samples from drug-induced reactions (Figures 2C, D). Overall, the global analysis of circulating serum levels of miR-375-3p showed a significant reduction during acute phase when compared to baseline in anaphylaxis (Figure 2E).
Levels of miR-375-3p within extracellular vesicles decrease during the acute phase of anaphylaxis compared to baseline
As a great portion of circulating miRNAs is contained in EVs (13), we investigated vesicle-associated miR-375-3p levels in anaphylactic human serum samples. We characterized circulating EVs from acute and baseline stages by different techniques. First, we confirmed vesicles purification by CD9, CD63 and TSG101 immunodetection, all of them considered bona fide protein markers of EVs (Supplementary Figure 4A). Transmission em showed heterogeneous size particles consistent previous EVs reports (27) (Supplementary Figure 4B). Next, we performed NTA in 9 paired samples (3 adults with drug-induced reactions and 6 (3 adults and 3 children) with food-induced reactions. (Supplementary Figure 4C). We did not observe differences in size or concentration when acute and basal vesicles were compared (Figures 3A, B).
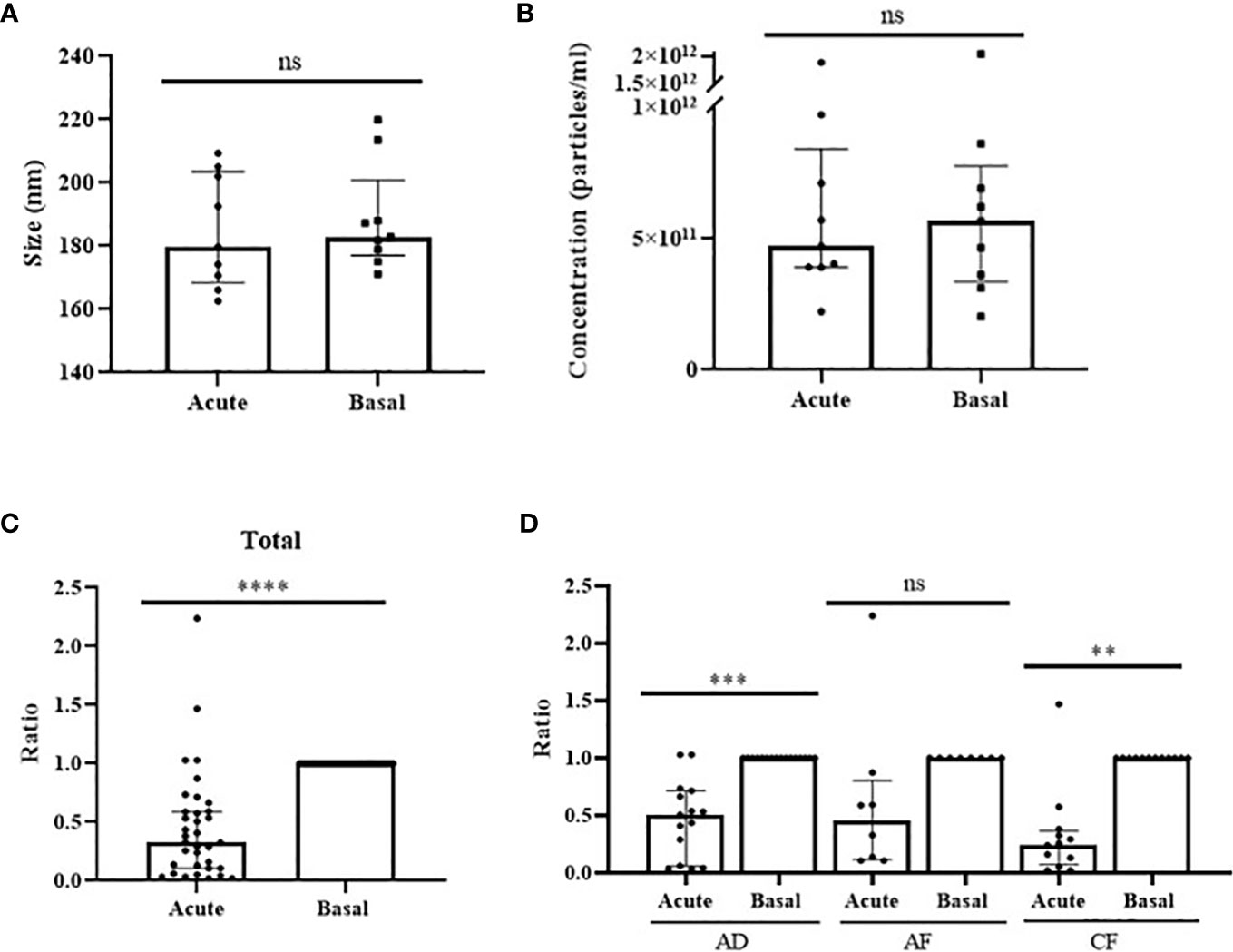
Figure 3 Levels of miR-375-3p within EVs decrease during anaphylaxis. Comparison of particles (A) size (p=.6523) and (B) concentration (p>.9999) between EVs obtained from acute and basal phase serum. ns: non-significant. Median ± IQR. (C) Levels of miR-375-3p (ratio acute/basal) decrease within EVs during the acute phase of anaphylaxis compared to baseline (****p<.0001; n: 35). Median ± IQR. (D) Comparison of changes in vesicular values of miR-375-3p among the three populations studied: adults with drug-induced anaphylaxis (***p=.0003; n: 15), adults with food-induced anaphylaxis (p=.1875; n: 8) and children with food-induced anaphylaxis (**p<.0015; n: 12). ns: non-significant. Median ± IQR.
Next, the hemolysis level of paired samples of 53 patients with anaphylaxis (19 adults with drug-induced reactions and 34 [19 adults and 15 children] with food-induced reactions) was evaluated. The coefficient between miR-23a-3p and miR-451a excluded 23 samples from 18 subjects that were discarded (Supplementary Figure 4D). Consequently, the determination of the miRNAs cargo was performed in 35 patients with anaphylaxis (15 adults with drug-induced reactions and 20 [8 adults and 12 children] with food-induced reactions). The correct extraction and reverse transcription of the miRNAs contained in EVs was confirmed using exogenous controls (Supplementary Figures 4E, F). Afterwards, the determination of vesicular miR-375-3p levels revealed a decrease during the acute phase of anaphylaxis compared to baseline, similarly as it was observed in serum (Figure 3C). Specifically, the independent analysis accordingly to trigger and age showed significant differences in the different cohorts of patients, confirming the drop of this miRNA in the acute phase of anaphylaxis (Figure 3D).
System biology analysis for miR-375-3p and its targets
miR-375-3p serum and EVs-associated reduced levels during anaphylactic reactions could have downstream consequences and participate in the underlying molecular mechanisms of anaphylaxis. Therefore, we conducted an in silico SBA on miR-375-3p 269 target genes. The analysis pointed to several diseases and disorders related to anaphylaxis pathophysiology, such as gastrointestinal and dermatological diseases. In addition, different signaling pathways regulated by miR-375-3p were found to be closely related to the pathological reaction as Phosphoinositide 3-kinases/Protein kinase B (PI3K/Akt), G-Protein coupled receptor (GPCR) and cAMP (Table 2).
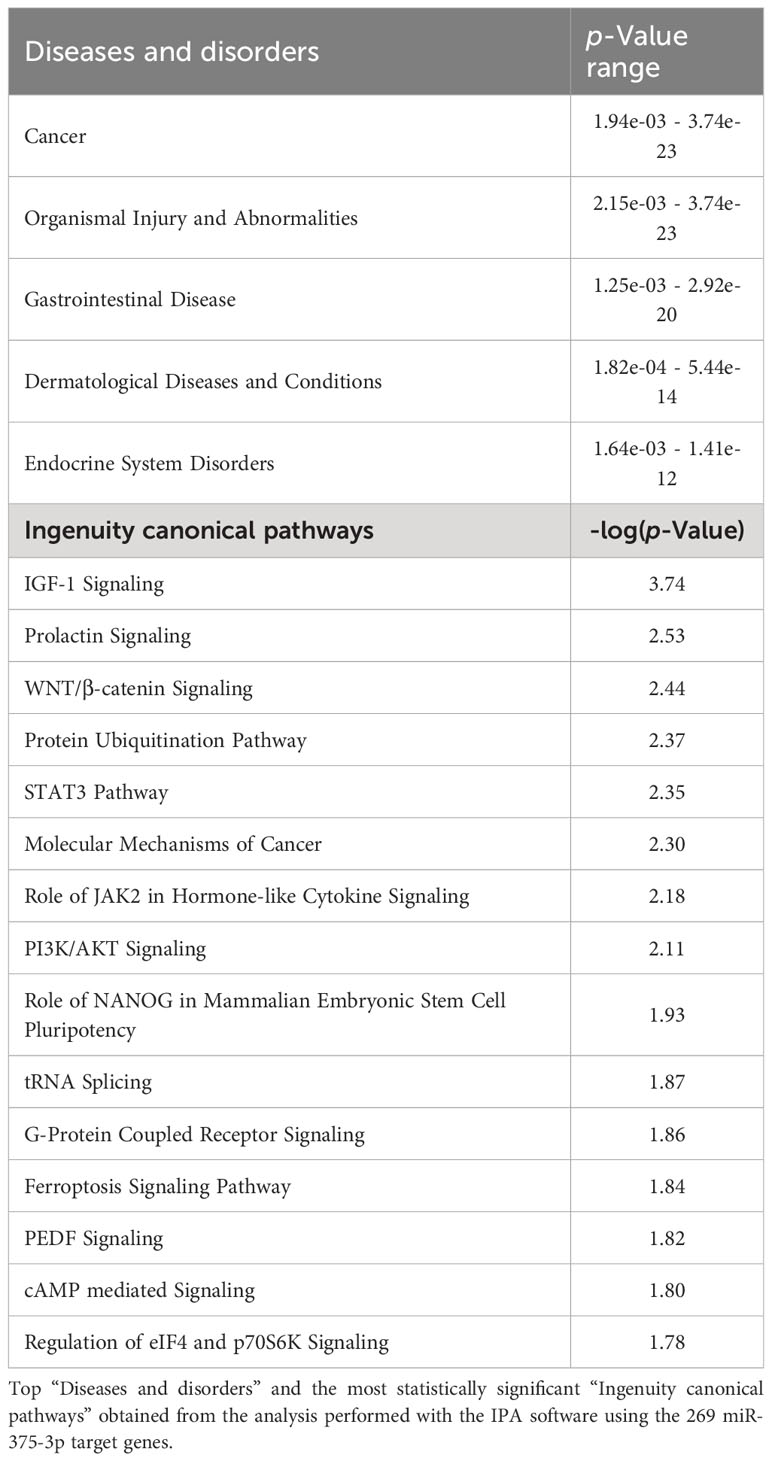
Table 2 In silico System Biology Analysis exhibit a possible involvement of miR-375-3p in the molecular mechanisms underlying anaphylaxis.
Precisely, various of these mechanisms were associated with the inflammatory process, thus we evaluated a panel of inflammatory cytokines to compare their levels with those of miR-375-3p. The analysis performed in serum of patients with anaphylaxis revealed an increase of several of these molecules during the acute phase of the reaction (Figure 4A). Furthermore, increased GM-CSF and MCP-1 proteins correlated with decreased serum levels of miR-375-3p, although no association was observed with the other cytokines studied (Figure 4B). In turn, no relation was found between miR-375-3p levels within EVs and the molecules assessed by the multiplex assay (Figure 4C).
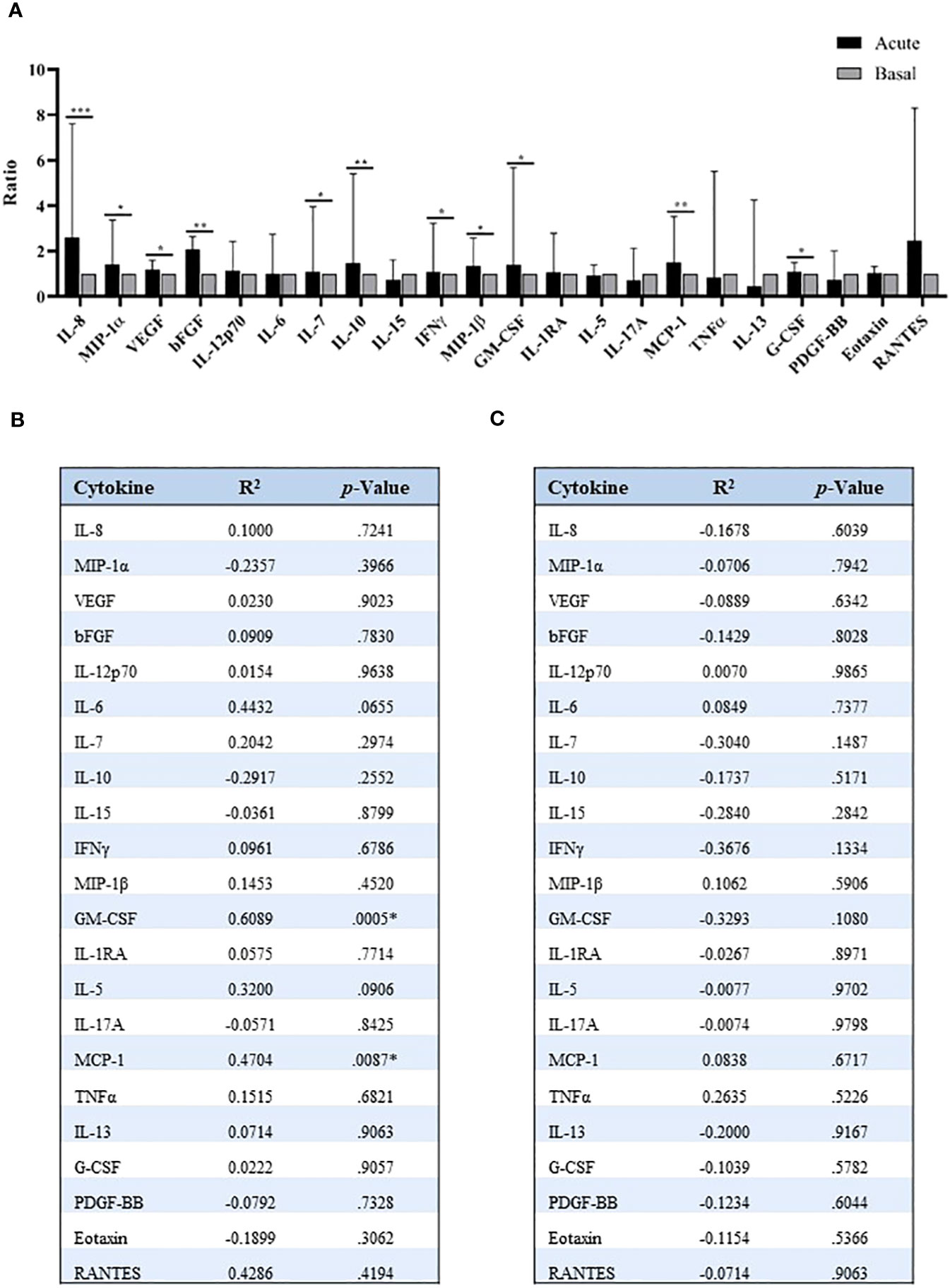
Figure 4 Quantification of serum inflammatory cytokines in patients with anaphylaxis. (A) Comparison of acute and basal levels of the 25 inflammatory cytokines identified by multiplex assay: IL-8 (***p=.0007; n: 18), MIP-1α (*p=.0361; n: 19), VEGF (*p=.0322; n: 40), bFGF (**p=.0093; n: 12), IL-12p70 (p=.2312; n: 16), IL-6 (p=.3270; n: 22), IL-7 (*p=.0394; n: 33), IL-10 (*p=.0290; n: 21), IL-15 (p=.9881; n: 23), IFNγ (*p=.0422; n: 26), MIP-1β (*p=.0173; n: 37), GM-CSF (*p=.0105; n: 34), IL-1RA (p=.1166; n: 35), IL-5 (p=.8620; n: 35), IL-17A (p=.3529; n: 19), MCP-1 (**p=.0014; n: 37), TNFα (p=.8501; n: 12), IL-13 (p=.9453; n: 8), G-CSF (*p=.0159; n: 40), PDGF-BB (p=.7317; n: 27), Eotaxin (p=.1744; n: 40), RANTES (p=.2500; n: 8). IL-1β, IL-2 and IL-4 plots are not shown due to their null detection in most samples. Median ± IQR. Correlation between (B) serum and (C) vesicular levels of miR-375-3p, and cytokines values. R2: Pearson correlation coefficient; bFGF: basic Fibroblast growth factor; G-CSF: granulocyte colony-stimulating factor; GM-CSF: granulocyte macrophage colony-stimulating factor; IFNγ: interferon-γ; IL: interleukin; MCP-1: monocyte chemoattractant protein-1; MIP: macrophage inflammatory protein; PDGF-BB: platelet-derived growth factor-BB; TNFα: tumor necrosis factor-α; VEGF: vascular endothelial growth factor. * Significant difference between groups (p<.05).
miR-375-3p partially blocks the cAMP stabilizing effect in the endothelial barrier
Increased vascular permeability is a process tightly related to anaphylaxis that involves endothelial barrier rupture. Among the mediators and molecular mechanisms affecting barrier stability, cAMP is crucial for integrity maintenance (11). To evaluate miR-375-3p role in this canonical pathway, we performed in vitro endothelial assays. For this purpose, HMVEC-Ds were transfected with an unlabeled mimic to overexpress miR-375-3p. First, the correct performance of the technique was confirmed by measuring the intracellular levels of miR-375-3p and by immunofluorescence images. A high number of miR-375-3p copies was observed by qPCR in those transfected mimic cells (Figure 5A), and its corresponding fluorescent labeled scramble was correctly visualized inside cells (Figure 5B). Subsequently, we evaluated the functional role of this miRNA on the endothelial barrier integrity. First, the stabilizing effect of cAMP was confirmed by increased values of the cellular monolayer´s resistance in non-transfected ECs (Figure 5C). However, cells transfected with miR-375-3p failed to response at the strengthening role exerted by this mediator (Figure 5D). Precisely, significant differences in endothelial resistance levels at 30 and 45 minutes were observed in cells transfected with miR-375-3p when compared to control systems (medium, TransIT-X2 and scramble) (Figures 5E, F). Next, we tested whether the functional change induced by miR-375-3p was also detectable morphologically via staining of VE-cadherin together with actin filaments (Figure 5G). Both in non-transfected ECs and scramble cells, VE-cadherin was localized at cellular membranes and actin was adjacently distributed forming the typical cobblestones cell structure. Contrary, separated cell borders were visualized in miR-375-3p ECs showing VE-cadherin disruption. In addition, miR-375-3p ECs exhibited diminished Rac1-Cdc42 protein levels (Figure 5H).
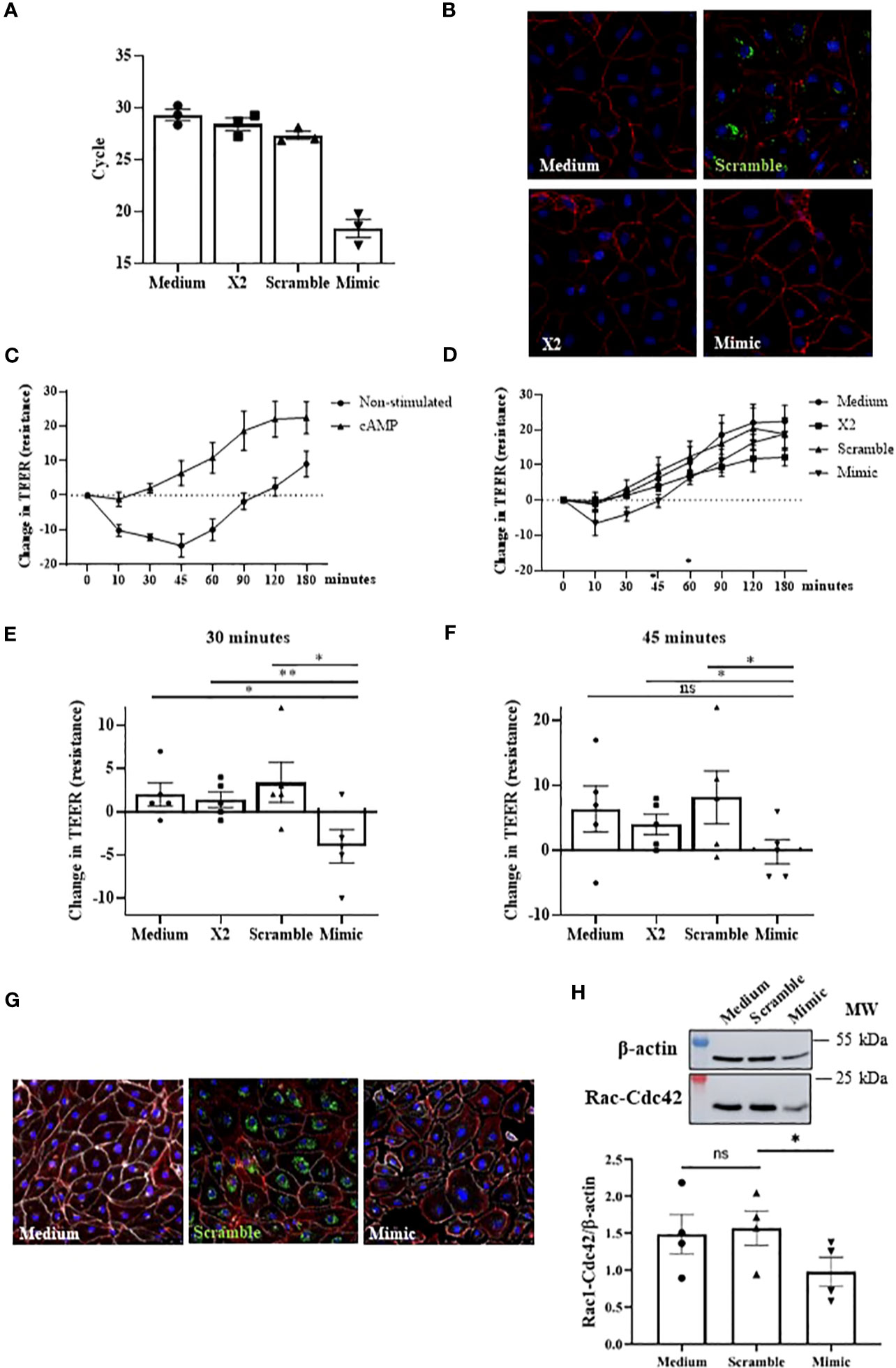
Figure 5 The miR-375-3p partially blocks the stabilizing cAMP response in HMVEC-D. (A) Determination of intracellular miRNA levels by qPCR after cells transfection (50mM). (B) Immunofluorescence images of transfected HMVEC-D. In red (Texas Red) are visualized the F-actin filaments, in blue (DAPI) the nuclei and in green (fluorescent scramble control) the transfected miRNA. (C) Graphic shows the stabilizing effect of cAMP in non-transfected cells (n: 5). (D) Changes in TEER measurements in HMVEC-D transfected with miR-375-3p and stimulated with cAMP (n: 5). Significant differences were observed at (E) 30 and (F) 45 minutes. 30’ miR-375-3p vs medium (*p=.0144), vs X2 (**p=.0090) and vs scramble (*p=.0215); 45’ miR-375-3p vs medium (p=.0514), vs X2 (*p=.0167) and vs scramble (*p=.0388). X2: transfection vehicle; ns: non-significant. Mean ± SEM. (G) Integrity of endothelial barrier in transfected HMVEC-D evaluated by immunofluorescence performed in 3 independent experiments. Panels show a representative picture of every condition. In red (Texas Red) are visualized the F-actin filaments, in white (Alexa Fluor 647) VE-cadherin, in blue (DAPI) the nuclei and in green (fluorescent scramble control) the transfected miRNA. (H) The bottom graphic represents the quantification of Rac1-Cdc42 protein levels in transfected HMVEC-D and the upper panel shows a representative experiment of four performed (*p=.0445).
Discussion
During the last decades, the relevance of miRNAs in allergy has been increasing due to the potential benefits of their use as diagnostic biomarkers, as well as their role regulating a wide variety of molecular processes (33). Accordingly, we have profiled serum circulating miRNAs in drug-mediated anaphylactic reactions and identified decreased serum and EVs-associated levels of miR-375-3p in different groups of patients with anaphylaxis. Furthermore, we have evaluated in silico miR-375-3p potential involvement in the reaction and observed a negative role for miR-375-3p in the stability of the endothelial barrier.
Recent studies determined a profile of circulating serum miRNAs in children with food-induced reactions and adults with food- and Hymenoptera venom-mediated anaphylaxis (17, 18). However, no miRNA has been characterized in anaphylactic reactions mediated by drug, even though these compounds are the main elicitors of anaphylaxis in adults (3). Therefore, as NGS offers new possibilities for molecular diagnosis (34), we applied this technology to analyze circulating miRNAs levels in a scarce number of paired serum samples. A total of 21 miRNAs were found statistically different between acute and basal phases, being more than half of them previously described in other allergic diseases. Six of those identified molecules were further studied in a larger and independent cohort of samples from drug mediated anaphylactic reactions using qPCR. The observations showed that only miR-375-3p exhibited statistically significant differences, decreasing in the acute phase of anaphylaxis. Precisely, miR-375-3p reduction has been also described in other allergic diseases such as allergic rhinitis and eosinophilic esophagitis, where it has been proposed as a non-invasive biomarker (35, 36). Additionally, the validation of low levels of miR-375-3p in two extra cohorts of food-mediated anaphylactic reactions patients reinforces its interest. Therefore, this molecule is postulated as a possible molecular marker for the acute phase of anaphylaxis. Moreover, extending the analysis of the miR-375-3p to new samples in future studies would allow the evaluation of its role as a preventive or prognostic biomarker, increasing its clinical relevance. In addition, the use of a set panel including specific proteins, other miRNAs or even metabolites would be a best tool in the clinical practice diagnosing or predicting the risk of reactions.
The systemic nature of anaphylaxis demonstrates the existence of different anaphylactic microenvironments connected through molecular pathways (9). Precisely, secreted miRNAs can mediate communication between different tissues modulating the gene expression of the target cells. In turn, those miRNAs located in EVs are relevant in molecular terms due to the EVs properties as carriers of signaling (13). Therefore, we determined miR-375-3p levels within anaphylactic reaction patient’s serum circulating EVs. The analysis performed demonstrated a significant decrease of this miRNA during the acute phase, as occurred in serum. Respectively, these results demonstrate not only miR-375-3p relevance as a biomarker but also as a molecular player. To our knowledge, this is the first time that miRNA levels have been measured in EVs from patients with anaphylaxis. Therefore, we inquired about EVs-associated miR-375-3p potential role in the underlying mechanisms of the reaction.
Accordingly, we performed SBA to evaluate plausible biological and molecular functions of miR-375-3p in anaphylaxis. The analysis highlighted gastrointestinal and dermatological diseases among the main disorders related to miR-375-3p gene targets. Relevantly, the information obtained associates with anaphylaxis pathophysiology since both systems are altered during this pathological event. Precisely, the skin is the main organ affected during the reaction (80-90%), while the gastrointestinal system is impaired in 45% of cases (37). Canonical pathways analysis exhibited miR-375-3p involvement in relevant molecular mechanisms previously described for anaphylaxis. For instance, this miRNA regulates PI3K/Akt signaling, which is crucial for the degranulation of mast cells and the release of inflammatory mediators (38). Furthermore, this miRNA also modulates Rac signaling, a Ras GTPase that regulates the actin cytoskeleton and controls mast cell degranulation, among other functions (39, 40). Moreover, the miR-375-3p controls Janus kinase 2 (JAK2) signaling which has been linked to atopic dermatitis (41). These receptors are associated with kinases that activate different transcription factors and are involved in both allergic inflammation and Th2 response (42, 43). Precisely, JAK blockade in mast cells prevents immediate hypersensitivity and anaphylactic reactions (44). On the other hand, in silico analysis revealed that these miRNAs regulated STAT3 signaling, a transcription factor required for mast cell degranulation (45). In addition, miR-375-3p also modulates GPCRs, a family of receptors activated in anaphylaxis and crucial in the response to vasoactive mediators on the endothelial barrier, modulating vascular permeability and vasodilation (11).
Increased vascular leakage is one of the main alterations underlying anaphylaxis, particularly in severe cases (46). Many vasoactive and proinflammatory molecules are involved in this process causing barrier breakdown (47). EVs purified from anaphylactic patients serum induce an increase in vascular permeability (26). In cancer, miR-375-3p containing EVs are internalized and cause tight junctions (TJs) disruption, altering endothelial barrier integrity through claudin inhibition (48). In addition, other studies in this area of research have shown that miR-375 modulates ECs proliferation, chemotaxis, angiogenesis and inflammation (49, 50). Here, in-silico analysis pointed out different miR-375-3p targets pathways that largely converge in the regulation of TJs (51). In particular, the plausible interaction stablished between miR-375 and cAMP signaling attracted our attention because of its central role in the regulation of the endothelial barrier (47). In vitro, miR-375-3p transfected cells revealed that its overexpression partially avoids the barrier strengthening induced by cAMP. These monolayers exhibited barrier breakdown accompanied by typical hallmarks such as intercellular gap formation and fragmentation of VE-cadherin staining. Under these conditions, Rac1-Cdc42 protein levels were decreased suggesting the upstream effect of miR-375-3p contributing to the loss of the endothelial barrier properties. In fact, RAC1 target sites has been previously pointed out for miR−375 (52). Therefore, our results show that modulation of miR-375-3p levels and its downstream effects could exert a role in the vascular pathophysiology of anaphylaxis.
Consequently, SBA demonstrated a clear relationship of miR-375-3p with assorted molecular mechanisms related to the pathophysiology of anaphylaxis. However, further studies are needed to confirm the role of this miRNA in each of the signaling pathways studied, as miR-375-3p could have pathological or protective functions. Clarifying these aspects would allow the development of new therapies by increasing or reducing its levels through different pharmacological strategies, such as the use of mimics or sponges, respectively (53).
Anaphylaxis is a systemic reaction where mediators released by effector cells trigger a severe inflammatory scenario (9). In turn, miR-375-3p has been widely implicated in the inflammation subjacent to other pathologies (54). Accordingly, several of the signaling pathways identified in the in-silico analysis revealed a possible involvement of this miRNA in the inflammation underlying the anaphylactic reaction. Therefore, we evaluated a panel of inflammatory cytokines to see if their levels correlated with the decrease in miR-375-3p. The results obtained exhibited an increase during the acute phase of several cytokines involved in the inflammatory process, which had been previously described in anaphylaxis (9). In addition, data showed a correlation between the decrease in miR-375-3p levels and the increase in two of the molecules evaluated in the multiplex assay, MCP-1 and GM-CSF. These evidences postulate an association between miR-375-3p and monocytes/macrophages, crucial cells in the alternative pathways of anaphylaxis, especially in IgG-mediated reactions (55, 56). Precisely, miR-375 has been shown to exert an immunoregulatory effect through macrophages (54). Therefore, changes in the levels of this miRNA could be due to the activation of these cells or could be modulating the response of monocytes and macrophages during the anaphylactic reaction.
The recruitment and study of anaphylactic serum samples states several limitations and strengths. First, to mention that the volume of sera harvested in the acute phases was insufficient to use all the patients in each of the approaches carried out in this study. Furthermore, the heterogeneity of the anaphylactic reactions hinders the selection of a representative set of samples for the discovery analysis. However, in this study, 5 well characterized individuals with a specific age range and trigger representing drug anaphylactic reactions, including moderate and severe cases, were chosen for NGS. In the case of miR-375-3p, the results exhibit reproducibility in other cohort of patients with drug-induced anaphylaxis and in adults and children with food anaphylactic reactions. Additionally, the difficulty of obtaining high-quality samples in anaphylaxis hinders obtaining an optimal miRNAs analysis. Red blood cells hemolysis highly influences the release of determined miRNAs (57). Therefore, a strength of our study is the use of abundant controls ensuring to our analysis the elucidation of specific miRNA, free of other cellular contaminants. Specifically, the discarding of hemolyzed samples is essential to avoid unspecific miRNAs detections. In our study, this fact substantially improves the quality of the results, but on the contrary it importantly reduced the number of accurate samples from patients to study. In addition, another limitation is the absence of healthy controls. Accordingly, we consider that it is very complicated to define this group in an acute pathological situation, such as anaphylaxis. Therefore, to overcome this obstacle and to reduce patient heterogeneity, all analyses were performed on a paired manner comparing the acute phase to the baseline phase of the patients, which was used as a control.
In summary, we have identified for the first time a differential profile of circulating serum miRNAs in adults with drug-induced anaphylactic reactions. We have assessed serum and EV-associated miR-375-3p levels in different cohorts of patients, postulating miR-375-3p as a promising molecular marker in anaphylaxis. We have evaluated the possible role of miR-375-3p in the molecular mechanisms of the reaction and studied its functional effect in endothelial barrier stabilization. These results will be validated in larger cohorts to solidify miR-375-3p´s value as a clinical biomarker. In conclusion, and even considering that our findings are exploratory in nature, our results present a clinical potential for the development of new diagnostic and therapeutic strategies.
Data availability statement
The data presented in the study are deposited in the GEO-NCBI repository, accession number GSE245653.
Ethics statement
The study was approved by the Ethics Committee (CEIm FJD, PIC057-19), authors adhered to the declaration of Helsinki and all patients were included after giving informed consent by the donors or their parents. The studies were conducted in accordance with the local legislation and institutional requirements. The human samples used in this study were acquired from primarily isolated as part of your previous study for which ethical approval was obtained.
Author contributions
EN-B, SF-B and AB-M performed the experimental work with participations of LP-G, VDP, AG, MC, LP and JT-A. VE coordinated the work. PRR, ADG, MI-S, JC-H and JJL provided the clinical support and the recruitment of human serum samples. VE, EN-B and ADG designed the experiments and interpreted the results with participations of SF-B and AB-M. VE and EN-B wrote the manuscript. All the authors reviewed and approved the manuscript.
Funding
The author(s) declare financial support was received for the research, authorship, and/or publication of this article. This research was supported by grants from the Instituto de Salud Carlos III (PI18/00348, PI21/00158) and FEDER Thematic Networks and Cooperative Research Centres RETICS ARADyAL (RD16/0006/0003, RD16/0006/0013, RD16/0006/0026, RD16/0006/0033). This work was also sustained by the SEAIC (19_A08) and Alfonso X el Sabio University Foundations. EN-B was granted by funding from the Community of Madrid included in the project FOODAL (FOODAL-CM_P2018/BAAA-4574) and SF-B was granted by funding from the Instituto de Salud Carlos III (FI22/00046). MC and VDP were supported by Fondazione Umberto Veronesi.
Conflict of interest
The authors declare that the research was conducted in the absence of any commercial or financial relationships that could be construed as a potential conflict of interest.
Publisher’s note
All claims expressed in this article are solely those of the authors and do not necessarily represent those of their affiliated organizations, or those of the publisher, the editors and the reviewers. Any product that may be evaluated in this article, or claim that may be made by its manufacturer, is not guaranteed or endorsed by the publisher.
Supplementary material
The Supplementary Material for this article can be found online at: https://www.frontiersin.org/articles/10.3389/fimmu.2023.1209874/full#supplementary-material
Glossary
References
1. Cardona V, Ansotegui IJ, Ebisawa M, El-Gamal Y, Fernandez Rivas M, Fineman S, et al. World allergy organization anaphylaxis guidance 2020. World Allergy Organ J (2020) 13:100472. doi: 10.1016/j.waojou.2020.100472
2. Castells M. Diagnosis and management of anaphylaxis in precision medicine. J Allergy Clin Immunol (2017) 140:321–33. doi: 10.1016/j.jaci.2017.06.012
3. Braganza SC, Acworth JP, Mckinnon DRL, Peake JE, Brown AFT. Paediatric emergency department anaphylaxis: different patterns from adults. Arch Dis Child (2006) 91:159–63. doi: 10.1136/adc.2004.069914
4. Atanaskovic-Markovic M, Gomes E, Cernadas JR, du Toit G, Kidon M, Kuyucu S, et al. Diagnosis and management of drug-induced anaphylaxis in children: An EAACI position paper. Pediatr Allergy Immunol Off Publ Eur Soc Pediatr Allergy Immunol (2019) 30:269–76. doi: 10.1111/pai.13034
5. Turner PJ, Worm M, Ansotegui IJ, El-Gamal Y, Rivas MF, Fineman S, et al. Time to revisit the definition and clinical criteria for anaphylaxis? World Allergy Organ J (2019) 12:100066. doi: 10.1016/j.waojou.2019.100066
6. Sala-Cunill A, Cardona V, Labrador-Horrillo M, Luengo O, Esteso O, Garriga T, et al. Usefulness and limitations of sequential serum tryptase for the diagnosis of anaphylaxis in 102 patients. Int Arch Allergy Immunol (2013) 160:192–9. doi: 10.1159/000339749
7. Reber LL, Hernandez JD, Galli SJ. The pathophysiology of anaphylaxis. J Allergy Clin Immunol (2017) 140:335–48. doi: 10.1016/j.jaci.2017.06.003
8. Muñoz-Cano R, Picado C, Valero A, Bartra J. Mechanisms of anaphylaxis beyond igE. J Investig Allergol Clin Immunol (2016) 26:73–82. doi: 10.18176/jiaci.0046
9. Fernández-Bravo S, Palacio-Garcia L, Requena-Robledo N, Yuste-Montalvo A, Nuñez-Borque E, Esteban V. Anaphylaxis: mediators, biomarkers and microenvironments. J Investig Allergol Clin Immunol (2022) 32(6):419–39. doi: 10.18176/jiaci.0854
10. Simons FER. Anaphylaxis. J Allergy Clin Immunol (2010) 125:S161–181. doi: 10.1016/j.jaci.2009.12.981
11. Nuñez-Borque E, Fernandez-Bravo S, Yuste-Montalvo A, Esteban V. Pathophysiological, cellular, and molecular events of the vascular system in anaphylaxis. Front Immunol (2022) 13:836222. doi: 10.3389/fimmu.2022.836222
12. Nguyen SMT, Rupprecht CP, Haque A, Pattanaik D, Yusin J, Krishnaswamy G. Mechanisms governing anaphylaxis: inflammatory cells, mediators, endothelial gap junctions and beyond. Int J Mol Sci (2021) 22:7785. doi: 10.3390/ijms22157785
13. Mori MA, Ludwig RG, Garcia-Martin R, Brandão BB, Kahn CR. Extracellular miRNAs: from biomarkers to mediators of physiology and disease. Cell Metab (2019) 30:656–73. doi: 10.1016/j.cmet.2019.07.011
14. Backes C, Meese E, Keller A. Specific miRNA disease biomarkers in blood, serum and plasma: challenges and prospects. Mol Diagn Ther (2016) 20:509–18. doi: 10.1007/s40291-016-0221-4
15. Ha M, Kim VN. Regulation of microRNA biogenesis. Nat Rev Mol Cell Biol (2014) 15:509–24. doi: 10.1038/nrm3838
16. Weidner J, Bartel S, Kılıç A, Zissler UM, Renz H, Schwarze J, et al. Spotlight on microRNAs in allergy and asthma. Allergy (2021) 76:1661–78. doi: 10.1111/all.14646
17. Nuñez-Borque E, Fernandez-Bravo S, Rodriguez Del Rio P, Alwashali EM, Lopez-Dominguez D, Gutierrez-Blazquez MD, et al. Increased miR-21-3p and miR-487b-3p serum levels during anaphylactic reaction in food allergic children. Pediatr Allergy Immunol Off Publ Eur Soc Pediatr Allergy Immunol (2021) 32:1296–306. doi: 10.1111/pai.13518
18. Francuzik W, Pažur K, Dalke M, Dölle-Bierke S, Babina M, Worm M. Serological profiling reveals hsa-miR-451a as a possible biomarker of anaphylaxis. JCI Insight (2022) 7:e156669. doi: 10.1172/jci.insight.156669
19. Sampson HA, Muñoz-Furlong A, Campbell RL, Adkinson NF, Bock SA, Branum A, et al. Second symposium on the definition and management of anaphylaxis: summary report–Second National Institute of Allergy and Infectious Disease/Food Allergy and Anaphylaxis Network symposium. J Allergy Clin Immunol (2006) 117:391–7. doi: 10.1016/j.jaci.2005.12.1303
20. Brown SGA. Clinical features and severity grading of anaphylaxis. J Allergy Clin Immunol (2004) 114:371–6. doi: 10.1016/j.jaci.2004.04.029
21. Nuñez-Borque E, Palacio-Garcia L, Fernández-Bravo S, Esteban V. Study of microRNAs expression in food allergy. Methods Mol Biol Clifton NJ (2024) 2717:337–50. doi: 10.1007/978-1-0716-3453-0_23
22. Takada Y, Shibuta T, Hatano M, Sato K, Koga M, Ishibashi A, et al. Pre-Analytical Modification of Serum miRNAs: Diagnostic Reliability of Serum miRNAs in Hemolytic Diseases. J Clin Med (2021) 10:5045. doi: 10.3390/jcm10215045
23. LaBelle J, Bowser M, Brown A, Farnam L, Kho A, Li J, et al. Commercially available blocking oligonucleotides effectively suppress unwanted hemolysis-related miRNAs in a large whole-blood RNA cohort. J Mol Diagn JMD (2021) 23:671–82. doi: 10.1016/j.jmoldx.2021.03.006
24. Rao X, Huang X, Zhou Z, Lin X. An improvement of the 2ˆ(-delta delta CT) method for quantitative real-time polymerase chain reaction data analysis. Biostat Bioinforma Biomath (2013) 3:71–85.
25. Colletti M, Tomao L, Galardi A, Paolini A, Di Paolo V, De Stefanis C, et al. Neuroblastoma-secreted exosomes carrying miR-375 promote osteogenic differentiation of bone-marrow mesenchymal stromal cells. J Extracell Vesicles (2020) 9:1774144. doi: 10.1080/20013078.2020.1774144
26. Nuñez-Borque E, Fernandez-Bravo S, Pastor-Vargas C, Alvarez-Llamas G, Gutierrez-Blazquez MD, Alwashali E, et al. Proteomic profile of extracellular vesicles in anaphylaxis and their role in vascular permeability. Allergy (2021) 76:2276–9. doi: 10.1111/all.14792
27. Théry C, Witwer KW, Aikawa E, Alcaraz MJ, Anderson JD, Andriantsitohaina R, et al. Minimal information for studies of extracellular vesicles 2018 (MISEV2018): a position statement of the International Society for Extracellular Vesicles and update of the MISEV2014 guidelines. J Extracell Vesicles (2018) 7:1535750. doi: 10.1080/20013078.2018.1535750
28. Chen Y, Wang X. miRDB: an online database for prediction of functional microRNA targets. Nucleic Acids Res (2020) 48:D127–31. doi: 10.1093/nar/gkz757
29. Ballesteros-Martinez C, Mendez-Barbero N, Montalvo-Yuste A, Jensen BM, Gomez-Cardenosa A, Klitfod L, et al. Endothelial regulator of calcineurin 1 promotes barrier integrity and modulates histamine-induced barrier dysfunction in anaphylaxis. Front Immunol (2017) 8:1323. doi: 10.3389/fimmu.2017.01323
30. Metsalu T, Vilo J. ClustVis: a web tool for visualizing clustering of multivariate data using Principal Component Analysis and heatmap. Nucleic Acids Res (2015) 43:W566–570. doi: 10.1093/nar/gkv468
31. Ballman KV, Grill DE, Oberg AL, Therneau TM. Faster cyclic loess: normalizing RNA arrays via linear models. Bioinforma Oxf Engl (2004) 20:2778–86. doi: 10.1093/bioinformatics/bth327
32. Elo LL, Filen S, Lahesmaa R, Aittokallio T. Reproducibility-optimized test statistic for ranking genes in microarray studies. IEEE/ACM Trans Comput Biol Bioinform (2008) 5:423–31. doi: 10.1109/tcbb.2007.1078
33. Lu TX, Rothenberg ME. MicroRNA. J Allergy Clin Immunol (2018) 141:1202–7. doi: 10.1016/j.jaci.2017.08.034
34. Zhong Y, Xu F, Wu J, Schubert J, Li MM. Application of next generation sequencing in laboratory medicine. Ann Lab Med (2021) 41:25–43. doi: 10.3343/alm.2021.41.1.25
35. Wang T, Chen D, Wang P, Xu Z, Li Y. miR-375 prevents nasal mucosa cells from apoptosis and ameliorates allergic rhinitis via inhibiting JAK2/STAT3 pathway. BioMed Pharmacother Biomed Pharmacother (2018) 103:621–7. doi: 10.1016/j.biopha.2018.04.050
36. Lu TX, Sherrill JD, Wen T, Plassard AJ, Besse JA, Abonia JP, et al. MicroRNA signature in patients with eosinophilic esophagitis, reversibility with glucocorticoids, and assessment as disease biomarkers. J Allergy Clin Immunol (2012) 129:1064–1075.e9. doi: 10.1016/j.jaci.2012.01.060
37. Simons FER, Ardusso LRF, Bilò MB, El-Gamal YM, Ledford DK, Ring J, et al. World allergy organization guidelines for the assessment and management of anaphylaxis. World Allergy Organ J (2011) 4:13–37. doi: 10.1097/WOX.0b013e318211496c
38. Kim M-S, Rådinger M, Gilfillan AM. The multiple roles of phosphoinositide 3-kinase in mast cell biology. Trends Immunol (2008) 29:493–501. doi: 10.1016/j.it.2008.07.004
39. Hong-Geller E, Holowka D, Siraganian RP, Baird B, Cerione RA. Activated Cdc42/Rac reconstitutes Fcepsilon RI-mediated Ca2+ mobilization and degranulation in mutant RBL mast cells. Proc Natl Acad Sci USA (2001) 98:1154–9. doi: 10.1073/pnas.98.3.1154
40. Price LS, Norman JC, Ridley AJ, Koffer A. The small GTPases Rac and Rho as regulators of secretion in mast cells. Curr Biol CB (1995) 5:68–73. doi: 10.1016/S0960-9822(95)00018-2
41. Fridman JS, Scherle PA, Collins R, Burn T, Neilan CL, Hertel D, et al. Preclinical evaluation of local JAK1 and JAK2 inhibition in cutaneous inflammation. J Invest Dermatol (2011) 131:1838–44. doi: 10.1038/jid.2011.140
42. Cotter DG, Schairer D, Eichenfield L. Emerging therapies for atopic dermatitis: JAK inhibitors. J Am Acad Dermatol (2018) 78:S53–62. doi: 10.1016/j.jaad.2017.12.019
43. Ashino S, Takeda K, Li H, Taylor V, Joetham A, Pine PR, et al. Janus kinase 1/3 signaling pathways are key initiators of TH2 differentiation and lung allergic responses. J Allergy Clin Immunol (2014) 133:1162–74. doi: 10.1016/j.jaci.2013.10.036
44. Malaviya R, Zhu D, Dibirdik I, Uckun FM. Targeting Janus kinase 3 in mast cells prevents immediate hypersensitivity reactions and anaphylaxis. J Biol Chem (1999) 274:27028–38. doi: 10.1074/jbc.274.38.27028
45. Siegel AM, Stone KD, Cruse G, Lawrence MG, Olivera A, Jung M, et al. Diminished allergic disease in patients with STAT3 mutations reveals a role for STAT3 signaling in mast cell degranulation. J Allergy Clin Immunol (2013) 132:1388–96. doi: 10.1016/j.jaci.2013.08.045
46. Nuñez-Borque E, Betancor D, Pastor-Vargas C, Fernández-Bravo S, Martin-Blazquez A, Casado-Navarro N, et al. Personalized diagnostic approach and indirect quantification of extravasation in human anaphylaxis. Allergy (2023) 78(1):202–13. doi: 10.1111/all.15443
47. Wettschureck N, Strilic B, Offermanns S. Passing the vascular barrier: endothelial signaling processes controlling extravasation. Physiol Rev (2019) 99:1467–525. doi: 10.1152/physrev.00037.2018
48. Mao S, Zheng S, Lu Z, Wang X, Wang Y, Zhang G, et al. Exosomal miR-375-3p breaks vascular barrier and promotes small cell lung cancer metastasis by targeting claudin-1. Transl Lung Cancer Res (2021) 10:3155–72. doi: 10.21037/tlcr-21-356
49. McCoy MG, Jamaiyar A, Sausen G, Cheng HS, Pérez-Cremades D, Zhuang R, et al. MicroRNA-375 repression of Kruppel-like factor 5 improves angiogenesis in diabetic critical limb ischemia. Angiogenesis (2023) 26:107–27. doi: 10.1007/s10456-022-09856-3
50. An Y, Liu Z, Ding H, Lv Q, Fan H, Hou S, et al. MiR-375-3p regulates rat pulmonary microvascular endothelial cell activity by targeting Notch1 during hypoxia. J Int Med Res (2020) 48:300060520926851. doi: 10.1177/0300060520926851
51. Cong X, Kong W. Endothelial tight junctions and their regulatory signaling pathways in vascular homeostasis and disease. Cell Signal (2020) 66:109485. doi: 10.1016/j.cellsig.2019.109485
52. Liang Z, Li J, Zhao L, Deng Y. miR-375 affects the hedgehog signaling pathway by downregulating RAC1 to inhibit hepatic stellate cell viability and epithelial-mesenchymal transition. Mol Med Rep (2021) 23:182. doi: 10.3892/mmr.2020.11821
53. Ho PTB, Clark IM, Le LTT. MicroRNA-based diagnosis and therapy. Int J Mol Sci (2022) 23:7167. doi: 10.3390/ijms23137167
54. Liu Y, Wang Q, Wen J, Wu Y, Man C. MiR-375: A novel multifunctional regulator. Life Sci (2021) 275:119323. doi: 10.1016/j.lfs.2021.119323
55. Finkelman FD. Anaphylaxis: lessons from mouse models. J Allergy Clin Immunol (2007) 120:506–15. doi: 10.1016/j.jaci.2007.07.033
56. Escribese MM, Rosace D, Chivato T, Fernández TD, Corbí AL, Barber D. Alternative anaphylactic routes: the potential role of macrophages. Front Immunol (2017) 8:515. doi: 10.3389/fimmu.2017.00515
Keywords: anaphylaxis, biomarker, endothelial permeability, extracellular vesicles, miRNA
Citation: Nuñez-Borque E, Fernandez-Bravo S, Rodríguez Del Rio P, Palacio-García L, Di Giannatale A, Di Paolo V, Galardi A, Colletti M, Pascucci L, Tome-Amat J, Cuesta-Herranz J, Ibañez-Sandin MD, Laguna JJ, Benito-Martin A and Esteban V (2023) Novel mediator in anaphylaxis: decreased levels of miR-375-3p in serum and within extracellular vesicles of patients. Front. Immunol. 14:1209874. doi: 10.3389/fimmu.2023.1209874
Received: 02 June 2023; Accepted: 13 October 2023;
Published: 30 October 2023.
Edited by:
Lucia La Sala, MultiMedica Holding SpA (IRCCS), ItalyReviewed by:
Peisong Gao, Johns Hopkins University, United StatesElisa Pesce, National Institute of Molecular Genetics (INGM), Italy
Valentina Carlini, MultiMedica (IRCCS), Italy
Copyright © 2023 Nuñez-Borque, Fernandez-Bravo, Rodríguez Del Rio, Palacio-García, Di Giannatale, Di Paolo, Galardi, Colletti, Pascucci, Tome-Amat, Cuesta-Herranz, Ibañez-Sandin, Laguna, Benito-Martin and Esteban. This is an open-access article distributed under the terms of the Creative Commons Attribution License (CC BY). The use, distribution or reproduction in other forums is permitted, provided the original author(s) and the copyright owner(s) are credited and that the original publication in this journal is cited, in accordance with accepted academic practice. No use, distribution or reproduction is permitted which does not comply with these terms.
*Correspondence: Vanesa Esteban, VkVzdGViYW5AZmpkLmVz
†These authors have contributed equally to this work and share senior authorship