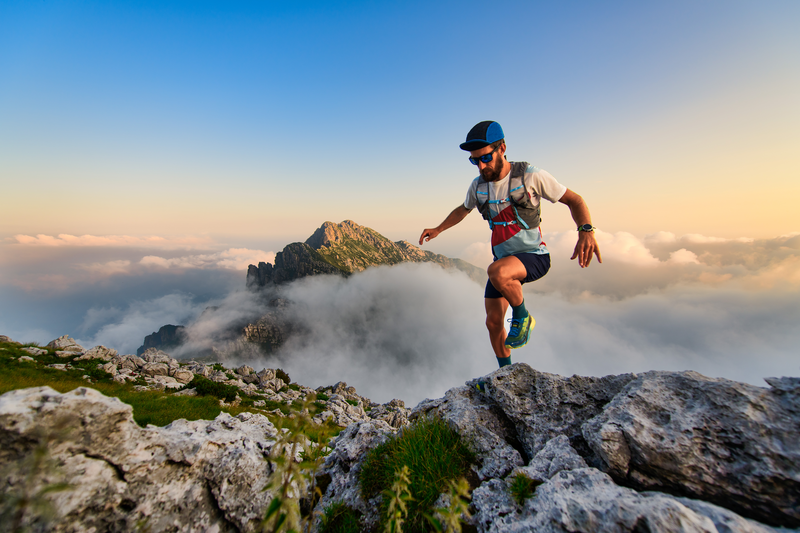
94% of researchers rate our articles as excellent or good
Learn more about the work of our research integrity team to safeguard the quality of each article we publish.
Find out more
ORIGINAL RESEARCH article
Front. Immunol. , 14 September 2023
Sec. Inflammation
Volume 14 - 2023 | https://doi.org/10.3389/fimmu.2023.1209097
Allergic airway inflammation (AAI) is a chronic respiratory disease that is considered a severe restriction in daily life and is accompanied by a constant risk of acute aggravation. It is characterized by IgE-dependent activation of mast cells, infiltration of eosinophils, and activated T-helper cell type 2 (Th2) lymphocytes into airway mucosa. Purinergic receptor signaling is known to play a crucial role in inducing and maintaining allergic airway inflammation. Previous studies in an ovalbumin (OVA)–alum mouse model demonstrated a contribution of the P2Y2 purinergic receptor subtype (P2RY2) in allergic airway inflammation. However, conflicting data concerning the mechanism by which P2RY2 triggers AAI has been reported. Thus, we aimed at elucidating the cell-type-specific role of P2RY2 signaling in house dust mite (HDM)-driven model of allergic airway inflammation. Thereupon, HDM-driven AAI was induced in conditional knockout mice, deficient or intact for P2ry2 in either alveolar epithelial cells, hematopoietic cells, myeloid cells, helper T cells, or dendritic cells. To analyze the functional role of P2RY2 in these mice models, flow cytometry of bronchoalveolar lavage fluid (BALF), cytokine measurement of BALF, invasive lung function measurement, HDM re-stimulation of mediastinal lymph node (MLN) cells, and lung histology were performed. Mice that were subjected to an HDM-based model of allergic airway inflammation resulted in reduced signs of acute airway inflammation including eosinophilia in BALF, peribronchial inflammation, Th2 cytokine production, and bronchial hyperresponsiveness in mice deficient for P2ry2 in alveolar epithelial cells, hematopoietic cells, myeloid cells, or dendritic cells. Furthermore, the migration of bone-marrow-derived dendritic cells and bone-marrow-derived monocytes, both deficient in P2ry2, towards ATP was impaired. Additionally, we found reduced levels of MCP-1/CCL2 and IL-8 homologues in the BALF of mice deficient in P2ry2 in myeloid cells and lower concentrations of IL-33 in the lung tissue of mice deficient in P2ry2 in alveolar epithelial cells. In summary, our results show that P2RY2 contributes to HDM-induced airway inflammation by mediating proinflammatory cytokine production in airway epithelial cells, monocytes, and dendritic cells and drives the recruitment of lung dendritic cells and monocytes.
Asthma is a chronic inflammatory disease (1) with an increasing prevalence affecting approximately 262 million in 2019, and it was responsible for 21.6 million disability-adjusted life years (DALYs) in the same year (2). Clinical symptoms are recurring breathlessness and wheezing accompanied by airway obstruction, bronchial hyperresponsiveness, and airway inflammation (3). Compelling evidence suggests asthma to be caused by a combination of genetic predispositions and exposure to environmental factors such as air pollution or allergens (4). The type 2 immune response causing an eosinophilic airway inflammation is initiated and maintained by airway epithelial cells along with dendritic cells (DCs) promoting innate lymphoid type 2 cell (ILC2) and Th2 cell activation (5). Activated ILC2s and Th2 cells secrete IL-4, IL-5, and IL-13, which mediates increased mucus secretion, airway smooth muscle contraction, allergen-specific IgE production, mast cell lung recruitment, and airway eosinophilia, all hallmarks of asthma pathogenesis (6).
Airway epithelial cells are the first point of contact of inhaled aeroallergens and secrete the DC maturation cytokines Thymic Stromal Lymphopoietin (TSLP) and Granulocyte-Macrophage Colony-Stimulating Factor (GM-CSF) (7). Pathogen-associated molecular pattern (PAMP) and damage-associated molecular patterns (DAMPs) bind their corresponding innate immune receptors driving allergic reaction by activating DCs (8, 9). In response to inflammatory, ischemic, and hypoxic conditions, nucleotides are released into the extracellular space. One of the earliest and most ubiquitous DAMPs is adenosine 5′-triphosphate (ATP), released at all inflammatory sites, where it activates nucleotide receptors that are called purinergic receptors (10).
Among the G-protein-coupled seven-transmembrane P2Y receptors is P2RY2, which has been implicated in the pathogenesis of various inflammatory disorders including allergic asthma by promoting inflammatory cell recruitment (11, 12). P2Y2 receptor is expressed in various epithelial cells (13–16), aortic smooth muscle (17, 18), and the brain (19, 20). Furthermore, P2Y2 receptor (P2RY2) expression has been shown in dendritic cells, eosinophils, B and T lymphocytes, macrophages, mast cells, monocytes, neutrophils, and NK cells (11, 21–27). P2RY2 is a well-known regulator of immune response and cell repair mechanisms (11, 28–31) that is activated by adenine and uracil nucleotides and triphosphates but not diphosphates (20, 32). In bronchial and intestinal epithelial, activation of P2Y2 receptor results in stimulation of chloride secretion and inhibition of NA+ transport (33–35). Hence, P2Y2 is a therapeutic target for cystic fibrosis (36). Moreover, it has been reported that P2Y2 receptor agonists enhance ciliary beat frequency and modulate mucin release in animals (33, 37, 38). Furthermore, the P2Y2-receptor-deficient mouse shows salt-resistant arterial hypertension (39). P2RY2 activation has been reported to be an initiator of inflammasome activation (40). We have previously shown that P2Y2 contributes to allergic lung inflammation by promoting migration of DCs and eosinophils (25). It has also been reported that activation of P2RY2 by eATP induces vascular inflammation and atherosclerosis (41).
As mentioned above, P2RY2 is predominantly expressed in immune cells (42), but its role in allergen-induced airway inflammation in a cell-type-specific manner has not yet been completely elucidated. In the current study, we aimed to identify the physiological and/or pathophysiological cell-type-specific role of P2RY2 in allergic airway inflammation. Here, we used conditional P2ry2 knockout expressing cre-recombinase in different cell types in an acute HDM-induced model of airway inflammation. As a result, the ablation of P2ry2 in epithelial cells, DCs, and myeloid cells alleviates AAI, whereas P2ry2 deficiency in CD4-positive T cells did not exert an attenuating effect on allergic lung inflammation.
Conditional P2ry2-floxed (P2ry2fl/fl) mice on a C57BL/6 background were generated by TaconicArtemis GmbH (Cologne, Germany). Two Cre recognition sequences (loxP sites) were inserted into intron 2 of the P2ry2 genomic sequence to flank exon 3. Exon 3 includes the entire coding region of P2RY2 gene. The insertion of the LoxP site into the P2ry2 gene facilitated Cre-mediated deletion of the entire P2ry2 coding region.
To generate conditional knockout mice, P2ry2fl/fl mice were mated with Cre+ mice of the lines C57BL/6-Tg(Scgb1a1-cre)1Tauc (Cct-cre) MGI:3610310 (43), C57BL/6-Tg(Vav1-icre)A2Kio/J (Vav-cre) MGI:2449949 (44), C57BL/6-Lyz2tm1(cre)Ifo (LysM-cre) MGI:1934631 (45), C57BL/6-Tg(Cd4-cre)1Cwi/BfluJ (Cd4-cre) MGI:5317293 (46), or C57BL/6-Tg(Itgax-cre)1-1Reiz/J (Cd11c-cre) MGI:3763248 (47) mice in the animal facilities of Freiburg University in order to receive cell-type-specific conditional knockout mice. All of these mice are on a C57BL/6 background and were maintained on this background.
In Cct-cre-positive mice, cre-recombinase is mainly expressed in the epithelial cells of the proximal conducting airways due to the transcriptional control by the major Clara cell secretory protein (CCSP) promoter (48). Vav-cre-positive mice show cre-recombinase activity in cells of the hematopoietic compartment and endothelial cells (49). In LysM-cre positive mice, cre-recombinase is mainly expressed in cells of the myeloid lineage (45) but also occurs in epithelial cells (50). Cd11c-cre+-positive mice exhibit cre-recombinase activity mainly in conventional dendritic cells (47), whereas Cd4-cre-positive mice show cre-recombinase activity exclusively in CD4+ cells (51).
Four to six female mice, 6–9 weeks, were considered in each group. All strains were bred and housed under specific pathogen-free (SPF) conditions. Mice experiments were performed in accordance with the local ethic committee (G12-096).
Epithelial cells, peripheral blood mononuclear cells (PBMCs), granulocytes, CD11c+ cells, CD4+ cells, bone marrow monocytes, and bone-marrow-derived macrophages from concerned strains were isolated, and P2Y2 receptor mRNA levels were measured by quantitative polymerase chain reaction (qPCR). Results were compared to cre-negative littermates (Supplementary Figure S1).
Anesthetized mice received three consecutive intratracheal (i.t.) administrations of 80 µL of Phosphate Buffered Saline (PBS) (Gibco, NY, USA) along with or without 100 µg house dust mite extract (HDM, Greer Laboratories, Lenoir, USA) at days 0, 7, and 14. Notably, the level of the endotoxin LPS in the commercial extract is 55,750 EU/vial (vial = 2.5 mL). The control group received PBS instead of HDM at days 0 and 7. All groups received HDM at day 14. After 72 h from the last HDM application, mice were either subjected to lung function measurement or sacrificed for bronchoalveolar lavage fluid (BALF) and mediastinal lymph node (MLN) isolation (25, 52–54). Subsequently, lungs were resected in Tissue-Tek optimal cutting temperature (OCT) compound (Sakura Finetek, CA, USA) for histological analysis. For BALF, the lungs were flushed three times with 1 mL of bronchoalveolar lavage buffer (PBS, 0.5 mM EDTA) each time. Usually, approximately 2 mL could be recovered. The three washes were pooled and then used for the flow cytometry and cytokine analysis. The bronchoalveolar lavage fluid obtained is centrifuged for 7 min at 8°C at 400g. The supernatant is then collected for cytokine measurements. Cell pellets were used for flow cytometry analysis. Cytokine concentrations in supernatants of BALF and MLN cells re-stimulated with HDM extracts (30 µg/mL) for 5 days at 37°C with 5% CO2 were determined using ELISA DuoSets (R&D Systems, USA) (55).
Anesthetized mice were intubated with an 18-gauge catheter, placed in a whole-body plethysmograph (EMMS, Bordon, UK) and mechanically ventilated (Minivent 485, Hugo-Sachs, March, Germany) at a rate of 200 strokes per minute with a volume of 200 µL. Airway resistance was recorded by eDacq software (EMMS, Bordon, UK) via a trans-pulmonary pressure transducer (TPP 200, EMMS, Bordon, UK) connected to an amplifier interface unit (AAC 091, EMMS, Bordon, UK), while mice were exposed to increasing doses (0 mg/mL, 3 mg/mL, 10 mg/mL, 30 mg/mL, and 100 mg/mL) of nebulized methacholine (Aristo Pharma GmbH, Germany).
Bone marrow (BM) were isolated from the tibia and femur of cre-positive and cre-negative mice by flushing the medullary cavity. After that, the cells were centrifuged and then resuspended in a red blood cell lysis buffer [ammonium chloride lysis buffer (150 mM NH4Cl, 10 mM KHCO3, 100 nM EDTA, dH2O, pH=7.4)] for 5 min on ice. Subsequently, 2×106 bone marrow cells were cultured in 2 mL Roswell Park Memorial Institute (RPMI) 1640 medium (Gibco, USA) containing 10% fetal calf serum (FCS, Sigma-Aldrich, Germany), 1% penicillin/streptomycin (Gibco, USA), and in 20 ng/mL of murine macrophage colony-stimulating factor (m-CSF, Miltenyi Biotec GmbH, Germany) in an Ultra-Low Attachment six-well plate (Corning Incorporated, USA) at 37°C with 5% CO2. On day 5, the non-adherent cells were collected and centrifuged at 400g at 4°C for 10 min to generate bone-marrow-derived monocytes (56, 57). The adherent cells were plated in complete medium to generate bone-marrow-derived macrophage.
Bone-marrow-derived dendritic cells (BMDCs) were generated with recombinant human Flt3 ligand (rh FLt3L/CD135) (ImmunoTools, Germany). In brief, bone marrow cells were isolated from the indicated experimental animals as described above and were grown at a concentration of 1×106 cells per well onto a 24-well plate in RPMI 1640 medium supplemented with 10% FCS, 1% penicillin/streptomycin, 50 μM 2-mercaptoethanol (Bio-Rad Laboratories, Germany), and 200 ng/mL rh FLt3 ligand. On day 6, the medium including 200 ng/mL rh FLt3 ligand was renewed. On day 9, the purity of bone-marrow-derived dendritic cells was >90% based on the proportion of the population expressing CD11c as determined by flow cytometry.
Migration assay was performed as described before (25, 58). Briefly, 1×105 cells/well in 100 µL medium was added to the upper compartment to each insert of a 24-well Transwell system with a pore size of 5 µm (Corning Incorporated, USA). To the lower compartment wells, ATP (Sigma-Aldrich, Germany) in different concentrations was added. Following 90 min of incubation at 37°C in a humidified atmosphere, cells from the upper compartment were removed, and migrated cells in the lower compartment were harvested and quantified by flow cytometry to determine the number of migrated cells. Only living F4/80− cells for monocytes and CD11c+ cells for DCs were considered for calculation of migratory index. Results are shown as chemotactic index defined as the number of F4/80− or CD11c+ cells in the lower compartments containing ATP divided by the number of F4/80− or CD11c+ cells in the chamber containing medium only. Experiments were performed in duplicate.
Resected lungs from P2ry2fl/fl Cct-cre+ mice and cre-negative littermates were filled up with 1 mL dispase (Corning Incorporated, USA) and digested in Dulbecco’s Modified Eagle Medium (DMEM) containing 2 µg/mL collagenase (Sigma-Aldrich, Germany) and 0.001% DNAse (Serva, Germany). Anti-mouse CD31− (Biolegend, CA, USA), anti-mouse CD45− (Biolegend, CA, USA), and anti-mouse EpCAM+ (Thermo Scientific, Germany) cells were sorted with FACS Aria III (BD Biosciences, Germany). Subsequent qPCR was performed to evaluate P2ry2 expression.
CD11c+ cells were isolated from the lung cell suspension with CD11c+ microbeads (Miltenyi Biotec, Germany), and subsequently, qPCR was performed.
Spleens from P2ry2fl/fl Cd4-cre+ and WT mice were excised and smashed through a 100-µm cell strainer (Corning Incorporated, USA). Cells were let rest for 10 min, and supernatants were collected. Subsequently, CD4+ cells were isolated from the cell suspension with CD4+ microbeads (Miltenyi Biotec, Germany).
A total of 500 µL of peripheral blood was taken from Vena cava of P2ry2fl/fl VaV-cre+ mice, P2ry2fl/fl LysM-cre+ mice, and cre negative littermates, supplemented with 50 µL 0.5M EDTA (Serva, Germany) and diluted with 500 µL of PBS. Cell separation was performed with polysaccharide separating solutions Pancoll (PAN-Biotech, Germany). After isolation, PBMCs and granulocytes were stimulated with LPS (300 ng/mL), and qPCR was performed to detect P2ry2 expression.
Flow cytometry analysis of the BALF was performed as described previously in (59, 60). Briefly, BALF was performed with 3 × 1 mL of Ca2+- and Mg2+-free PBS supplemented with 0.1 mM EDTA. Red blood cells were lysed using ammonium chloride lysis buffer (150 mM NH4Cl, 10 mM KHCO3, 100 nM EDTA, dH2O, pH=7.4). BALF cells were then counted under a microscope and washed. 0.25×106 to 1×106 cells/mL was used in each sample. First, BALF cells were incubated for 10 min with an unlabeled rat anti-mouse CD16/CD32 antibody (Mouse BD Fc Block, BD Biosciences, Germany) to avoid unspecific FcγRIII and FcγRII binding. Subsequently, cells were stained with FITC conjugated rat monoclonal anti-mouse Ly-6G (Gr-1) (clone 1A8-Ly6g, dilution 1:50, Thermo Scientific, Germany), PE-conjugated anti-mouse CD193 (CCR3) (dilution 1:50, eBioscience, USA), APC-conjugated Armenian hamster monoclonal anti-mouse CD11c (clone N418, dilution 1:400, Thermo Scientific, Germany), and PE-Cy7-conjugated rat monoclonal anti-mouse CD3− (clone 17A2, dilution 1:50, Thermo Scientific, Germany) and with PE-Cy7-conjugated rat monoclonal anti- B220 (clone RA3-6B2, dilution 1:100, Thermo Scientific, Germany) antibodies in FACS buffer (PBS containing 2.5% FCS, 5 mM EDTA and 0.01% sodium azide). Cells were incubated for 30 min at 4°C in the dark, washed twice with FACS buffer, and then resuspended in FACS buffer and analyzed by FACS. Flow cytometry was done immediately after the completion of the staining protocol. To establish proper gating strategy, isotype Igs were used as controls. A flow cytometer with appropriate lasers and filters for signal detection was used. It was performed using a FacsCalibur flow cytometer (BD Biosciences, USA) using Cellquest version 3.3 (BD Biosciences, USA). Data analysis was done using FlowJo software (TreeStar Inc., USA). For gating strategy, please see Supplementary Material and Methods. Approximately 1×105 was analyzed per sample. Murine cell frequencies are indicated in the plots in Supplementary Material and Methods.
For quantitative PCR, Takyon Mastermix (Eurogentec, Germany) was used. All measurements were executed with LightCyler 480 (Roche, Germany). An annealing temperature of 59°C has been chosen for all reactions. All primers and dual-labeled probes were designed as described before (61). All sequences are available upon request. The reference gene (RG) utilized was ß2m. To calculate the percent RG values, the following formula was used: %RG = 100 × 2(−Ct). The following formula was used for the calculation of the combined standard deviations of reference gene and gene of interest (GOI): SD = 100 × 2(−DCt) × ((ln2 × SDRG)2 + (ln2 × SDGOI)2)1/2 (62).
For the calculation of the statistical significance of differences between study groups and controls, one-way ANOVA was applied, followed by Bonferroni comparison test. Two-sided Student’s t-tests were performed when indicated. Analyses were performed using GraphPad Prism v8 (GraphPad Software, USA). Differences were considered significant at p < 0.05 and highly significant at p < 0.005.
To determine the protective role of P2ry2 in epithelial cells in allergic airway inflammation mouse model, we sensitized P2ry2fl/fl Cct-cre+ mice deficient for P2ry2 on airway epithelial cells and in control littermate. Subjecting P2ry2fl/fl Cct-cre+ mice to the HDM-induced model of airway inflammation resulted in an alleviated BALF eosinophilia (Figure 1A) and type 2 cytokine production of HDM re-stimulated MLN cells (Figure 1B). Histological analysis of H&E-stained lung sections showed decreased peribronchial inflammation in P2ry2fl/fl CCt-cre+ mice compared to P2ry2fl/fl mice treated with HDM (Figure 1C). To evaluate invasive lung function, airway resistance was recorded, and the results revealed a decrease in bronchial hyperreactivity (BHR) in response to increasing doses of nebulized methacholine compared to cre-negative littermates (Figure 1D). Furthermore, we found lower concentrations of IL-33 in the lung tissue homogenate (Figure 1E) and EpCAM+, CD31−, and CD45− lung cells at mRNA level (Figure 1F). Altogether, these results suggest that the epithelial cells deficient in P2ry2 show reduced HDM-induced airway inflammation in mice.
Figure 1 P2ry2 deficiency in P2ry2fl/fl CCt-cre+ mice decreases allergic airway inflammation. (A) BALF differential cell count. (B) Th2 cytokine concentrations in supernatants of HDM re-stimulated MLN cells. (C) Histology of H&E-stained lung sections obtained from P2ry2fl/fl CCt-cre+ mice and P2ry2fl/fl mice treated with HDM (magnification: 20× objective). (D) Methacholine-driven BHR represented by changes in airway resistance. (E) Level of IL-33 in the lung homogenate. (F) Relative IL-33 mRNA level in EpCAM+, CD31−, and CD45− lung cells. Graphs show mean ± SEM (n = 4–6). *p < 0.05, **p < 0.01, ***p < 0.001, P2ry2fl/fl CCt-cre+ HDM/HDM vs. P2ry2fl/fl HDM/HDM.
Next, we analyzed the effect of deletion of P2ry2 in hematopoietic cells using Vav-Cre transgenic mice expressing Cre recombinase under the control of the human vav 1 oncogene (VAV1) regulatory elements in all hematopoietic stem cell. Ablation of P2ry2 in hematopoietic cells in Vav-cre+ mice was accompanied by alleviated BALF eosinophils (Figure 2A) and a reduction in AAI marker cytokines (Figure 2B) after HDM challenge compared to cre-negative P2ry2fl/fl littermates. Histological analysis of the lung sections indicated lower peribronchial inflammation in P2ry2fl/fl Vav-cre+ mice compared to that of P2ry2fl/fl mice after HDM-challenge (Figure 2C). There was no difference in sVCAM1-levels measured in BALF supernatants (Figure 2D). Changes in airway resistance in response to increasing doses of inhaled methacholine was measured, and the results revealed a decreased BHR in P2ry2fl/fl Vav-cre+ mice (Figure 2E). Furthermore, we detected alleviated migration of BM dendritic cells from both Vav-cre+ and Cd11c-cre+ towards different concentrations of ATP compared to cre-negative littermates (Figure 3). These findings show that P2ry2-deficient mice in hematopoietic cells demonstrate an attenuated AAI phenotype.
Figure 2 P2ry2fl/fl Vav-cre+ mice deficient in P2ry2 show less AAI than cre− littermates. (A) BALF differential cell count. (B) Th2 cytokine concentrations in supernatants of HDM re-stimulated MLN cells. (C) Histology of H&E-stained lung sections (magnification: 20× objective) isolated from P2ry2fl/fl Vav-cre+ and P2ry2fl/fl mice after HDM-challenge. (D) Changes in airway resistance in response to increasing doses of inhaled methacholine. (E) Concentration of soluble vascular cell adhesion molecule 1 (sVCAM-1) in BALF supernatant. Graphs show mean ± SEM (n = 4–6). *p < 0.05, P2ry2fl/fl Vav-cre+ HDM/HDM vs. P2ry2fl/fl HDM/HDM.
Figure 3 Dendritic cell migration towards ATP is reduced. Migratory capacity of BM-derived dendritic cells from Cd11c-cre+ and Vav-cre+ mice compared to cre-negative littermates. Graphs show mean ± SEM (n = 3). ***p < 0.001.
To elucidate whether the AAI reduction in P2ry2fl/fl Vav-cre+ mice was conveyed by monocytes and macrophages, we performed experiments with P2ry2fl/fl LysM-cre+ mice missing P2ry2 in myeloid cells. Consistently, we noticed extenuated AAI indicated by reduced BALF eosinophilia (Figure 4A) and decreased production of Th2-cytokines by HDM re-stimulated MLN cells (Figure 4B). In line with these data, histological analysis of the lungs showed diminished peribronchial inflammation in P2ry2fl/fl LysM-cre+ mice compared to P2ry2fl/fl mice after HDM challenge (Figure 4C). By measuring airway resistance, we observed reduced BHR to increasing doses of inhaled methacholine in LysM-cre+ mice compared to P2ry2fl/fl mice (Figure 4D). Moreover, we observed shortened migration of BM-derived monocytes from LysM-cre+ mice towards various levels of ATP in in vitro experiments (Figure 4E). In addition, we detected reduced levels of chemokine (C–C motif) ligand 2 (CCL2) and the murine IL-8 homologues KC and MIP-2 in BALF supernatants (Figure 4F). In P2ry2fl/fl Cd11c-cre+ mice, we observed an alleviated AAI including BALF eosinophilia (Figure 5A) and production of Th2-cytokines by HDM re-stimulated MLN cells (Figure 5B). Histological analysis of the lung sections obtained from P2ry2fl/fl Cd11c-cre+ mice and P2ry2fl/fl mice challenged with HDM confirmed reduced inflammatory cell recruitment in the lungs of P2ry2fl/fl Cd11c-cre+ mice (Figure 5C). Measurement of airway resistance to increasing doses of inhaled methacholine revealed reduced BHR in P2ry2fl/fl Cd11c-cre+ mice in comparison to Cd11c-cre− littermates (Figure 5D). Taken together, these results indicate that P2ry2 ablation in myeloid cells reduces AAI.
Figure 4 Ablation of P2ry2 in P2ry2fl/fl LysM-cre+ diminishes AAI. (A) Total number of neutrophils, eosinophils, and lymphocytes. (B) Concentration of Th2 in supernatants of MLN cells after HDM re-stimulation. (C) Histological H&E staining from lungs taken from P2ry2fl/fl LysM-cre+ mice and P2ry2fl/fl mice after HDM challenge (magnification: 20× objective). (D) Changes in airway resistance in response to increasing doses of inhaled methacholine. (E) Chemotactic index of bone-marrow-derived monocytes compared to cre-negative littermates. (F) Levels of CCL2, KC, and MIP-2 in BALF supernatant. Graphs show mean ± SEM (n = 4–6). *p < 0.05, **p < 0.01, ***p < 0.001, P2ry2fl/fl LysM-cre+ HDM/HDM vs. P2ry2fl/fl HDM/HDM.
Figure 5 Recombinase activity in P2ry2fl/fl Cd11c-cre+ mitigates AAI. (A) Differential cell count of BALF. (B) IL-4, IL-5, and IL-13 concentrations in supernatants of HDM re-stimulated MLN cells. (C) Histology of H&E-stained lung sections obtained from P2ry2fl/fl Cd11c-cre+ mice and P2ry2fl/fl mice challenged with HDM (magnification: 20× objective). (D) BHR in response to increasing doses of inhaled methacholine, analyzed by recording the changes in airway resistance. Graphs show mean ± SEM (n = 4–6). *p < 0.05, P2ry2fl/fl Cd11c-cre+ HDM/HDM vs. P2ry2fl/fl HDM/HDM.
To investigate the role of P2ry2 in CD4+ cells, we created conditional knockout mice deficient in the P2RY2 receptor in T cells. P2ry2fl/fl Cd4-cre+ mice did not show different hallmarks of AAI compared to their Cd4-cre− littermates. We did not observe a reduction in BALF eosinophilia (Figure 6A) and production of Th2-cytokines by HDM re-stimulated MLN cells (Figure 6B). Consistently, histological screening of stained lung sections taken from P2ry2fl/fl Cd4-cre+ and P2ry2fl/fl mice after HDM challenge indicated no difference in inflammation between treatments in different mice groups (Figure 6C). As expected, measuring airway resistance also indicated no changes in BHR to increasing doses of inhaled methacholine (Figure 6D). Taken together, these data suggest that Cd4-cre+ P2ry2fl/fl mice deficient in P2ry2 in CD4-positive cells exhibit unchanged AAI compared to their cre-negative littermates.
Figure 6 P2ry2 deficiency in P2ry2fl/fl Cd4-cre+ does not affect degree of AAI. (A) Total count of neutrophils, eosinophils, and lymphocytes. (B) Concentration of Th2 determined in supernatants of MLN cells after HDM re-stimulation. (C) Histology of H&E-stained lung sections (magnification: 20× objective) taken from P2ry2fl/fl Cd4-cre+ mice and P2ry2fl/fl mice after HDM challenge. (D) Differences in airway resistance in response to increasing doses of inhaled nebulized methacholine. Graphs show mean ± SEM (n = 4–6). P2ry2fl/fl Cd4-cre+ HDM/HDM vs. P2ry2fl/fl HDM/HDM.
We have previously shown that P2ry2 deficiency is accompanied by an attenuated acute ovalbumin-induced airway inflammation (25). However, the OVA model begins with a systemic sensitization caused by injecting OVA coupled with aluminum hydroxide, which activates the NACHT, LRR, and PYD domains-containing protein 3 (NALP3) inflammasome and subsequently drives the activation of antigen presentation cell (APC) (63). Thus, this model has difficulty in simulating the human situation, in which sensitization occurs by pattern-recognition receptors (PRRs) ligand binding on the airway epithelium (64, 65). In this study, we used an HDM-based model of allergic airway inflammation, by administering house dust mite extract intratracheally to mice, which better imitates the human sensitization by taking the way through the airways including immediate innate immune response (66). Notably, the HDM-mouse model showed increased pulmonary ATP levels (67). Extracellular ATP accumulates in the lungs of asthmatic patients and animals in experimental models of allergic airway inflammation (52). In these settings, we previously observed that interference with ATP/type 2 purinergic receptor pathways attenuates allergic airway inflammation (25, 52, 53). We focused on investigating the cell-specific effects of P2RY2 signaling by applying conditional knockout animals. In these models, P2RY2 is inactivated in specific cell types such as alveolar epithelial cells, hematopoietic cells, myeloid cells, or dendritic cells, and CD4+ cells. We used the Cre-lox system, in which Cre recombinase recognizes the loci of recombination loxP that causes a deletion of the P2RY2 between the two loxP sites in a cell-specific manner. Other cell types and tissues exhibit an unmodified, functional P2RY2 expression. We identified for the first time that airway epithelial cells, monocytes, and dendritic cells express P2RY2 mediate acute airway inflammation in the HDM mouse model. We found that knockout of P2ry2 in these models resulted in reduced BALF eosinophilia, attenuated type 2 cytokine production of HDM re-stimulated MLN cells, decreased peribronchial inflammation, and reduced bronchial hyperreactivity in response to increasing doses of nebulized methacholine.
Clara cells represent a pool of non-ciliated secretory cells in the small airways and trachea, where they contribute to the control of inflammation and maintenance of the ciliated airway epithelial cell population (68). Activation of airway epithelial cells strongly depends on PAMPs, DAMPs, and their corresponding receptors (69). Former studies implicated autocrine ATP-induced P2RY2 signaling in mucociliary clearance (38) and IL-33 release by airway epithelial cells (70). To establish an HDM-induced model of airway inflammation, we applied HDM intratracheally to P2ry2fl/fl Cct-cre+ mice, which are deficient for P2ry2 in the epithelial cells of the proximal conducting airways. Importantly, we found that P2ry2fl/fl Cct-cre+ mice showed an attenuated BALF eosinophilia, type 2 cytokine production of HDM re-stimulated MLN cells, peribronchial inflammation, and BHR in response to increasing doses of nebulized methacholine compared to cre-negative littermates. This alleviated AAI phenotype observed in P2ry2fl/fl Cct-cre+ mice is in line with previous findings describing a contribution of P2RY2 signaling to IL-33 secretion by airway epithelial cells subsequently initiating a type 2 immune response (70). Consequently, P2ry2fl/fl Cct-cre+ mice exhibited diminished IL-33 levels in the lung homogenates and at mRNA level compared to cre-negative littermates. Remarkably, we have noticed that epithelial cells appeared small and disorganized in P2ry2fl/fl Cct-cre+ mice, which might be due to the effect of the deletion. To our knowledge, there is no description of this phenomenon yet, but it should be investigated further.
In addition to epithelial cells playing a crucial role in initiating inflammatory immune responses against allergens, cells of hematopoietic origin drive inflammation by exerting its specific effector functions (11). P2RY2 expression has been reported in monocytes, macrophages, dendritic cells, neutrophils, eosinophils, NK cells, and lymphocytes (11, 42), where it contributes to migration and mediator production (12, 25, 30). Consistently, the genetic ablation of P2ry2 in hematopoietic cells in P2ry2fl/fl Vav-cre+ mice results in an alleviated HDM-induced airway inflammation in terms of eosinophils in the BALF, production of type 2 cytokine of HDM re-stimulated MLN cells, peribronchial inflammation, and BHR compared to cre-negative littermates.
Dendritic cells (DCs) are essential for initiation and maintenance of Th2 response to inhaled allergens in asthma pathogenesis (71). DCs can derive from common dendritic cell progenitors (CDPs) subsequently infiltrate secondary lymphoid organs and tissue or differentiate from monocytes (72). Previously, our group reported an alleviated AAI phenotype in P2ry2 knockout mice applied to an OVA-driven model, which was associated with a reduced ATP-mediated chemotactic capacity of dendritic cells (25). Likewise, BMDCs derived from P2ry2fl/fl Vav-cre+ mice exhibited a reduced in vitro migration towards ATP compared to BMDCs from cre-negative littermates. Beside hematopoietic cells, P2ry2fl/fl VaV-cre+ mice exhibit P2ry2 deficiency in endothelial cells. Vanderstocken et al. demonstrated that P2ry2 knockout mice produce less vascular cell adhesion molecule 1 (VCAM-1) in an OVA-driven model of airway inflammation resulting in a mitigated eosinophil adhesion and infiltration (73). Nevertheless, we did not observe reduced sVCAM1 levels in the BALF of P2ry2fl/fl VaV-cre+ mice compared to cre-negative littermates. Thus, P2RY2 signaling is implicated in mediating its proinflammatory effects on cells of hematopoietic origin rather than endothelial cells in our HDM-induced model of airway inflammation.
Further confirmation came from P2ry2fl/fl Cd11c-cre+ and P2ry2fl/fl LysM-cre+ mice, which also exhibited an alleviated asthma phenotype including attenuated BALF eosinophilia, type 2 cytokine secretion by HDM re-stimulated MLN cells, peribronchial inflammation, and BHR similar to P2ry2fl/fl Vav-cre+ animals. In addition, BM-derived dendritic cells isolated from P2ry2fl/fl Cd11c-cre+ and BM-derived monocytes isolated from P2ry2fl/fl LysM-cre+ showed an attenuated in vitro migration in response to ATP compared to their cre-negative littermates. This is in line with previous reports demonstrating that P2RY2 contributes to allergen-induced airway inflammation by driving myeloid dendritic cell and monocytes lung recruitment (25, 29, 52, 74). Furthermore, P2RY2 on monocytes and macrophages is implicated in the production of the chemoattractant MCP-1/CCL2, which attracts dendritic cells, monocytes, and T cells towards the sides of inflammation (75–77). Concordantly, we found reduced MCP-1/CCL2 and murine IL-8 homologues KC and MIP-2 levels in the BALF of P2ry2fl/fl LysM-cre+ mice compared to cre-negative littermates. Beside MCP-1/CCL2, P2ry2fl/fl LysM-cre+ mice also exhibit diminished levels of the murine IL-8 homologues KC and MIP-2 in BALF corresponding with former observations that P2RY2 on monocytes is required for proinflammatory IL-8 secretion (78). Next, to investigate the role of P2ry2 in CD4+ cells, we generated conditional knockout mice lacking P2ry2 receptor in T cells. We found that P2ry2 in CD4+ cells is not essential for controlling CD4+ response during AAI in HDM mouse model.
Regarding the correlation of different types of involved cells, a remarkable array of defense mechanisms is implemented by the mammalian immune system to fight infections (79). Hematopoietic immune cells, such as myeloid cells that regulate innate immunity and lymphoid cells that make up adaptive immunity, are its primary constituents (79). There is structural immunity, which is the term used for the immune functions in the non-hematopoietic, structural cell populations of the body such as epithelial cells (79). It has been previously shown that the Th2 inflammatory responses are characterized by the recruitment and activation of mast cells, basophils, and eosinophils in airway and intestinal epithelia (80, 81). Furthermore, we have previously demonstrated that P2RY2 signal transduction in immune cells influences the pathogenesis of various inflammatory airway diseases (74). Thus, epithelial cells and myeloid cells might be involved in the inflammation and immune responses by different mediators directed in non-hematopoietic and hematopoietic pathways, respectively. In this context, purinergic receptors such as P2X and P2Y play a fundamental role in mediating lung inflammation associated with asthma and COPD.
This study proposes P2RY2 as a key player in HDM-induced airway inflammation. The P2RY2 signal transduction has a highly ambivalent effect in the body and can have a protective effect by defending against bacterial infections, promoting wound healing or the stimulation of mucociliary clearance, but at the same time, it can promote the pathogenesis of chronic inflammatory diseases. Therefore, P2RY2 antagonists are now also being considered as a therapeutic option, for example for the treatment of inflammatory respiratory diseases such as asthma or COPD (11). The studies by Müller et al. already provided the first evidence for the involvement of the P2Y2 receptor in allergic airway inflammation and its potential suitability as a target in the therapy of asthma bronchiales (25). The present work contributes to a better understanding of cells that mediate proinflammatory effects in allergic asthma via P2RY2 signaling. This provides new insights into the asthma pathogenesis and offers new possibilities regarding a cell-specific therapy of allergic asthma by P2RY2-targeted medication. In this respect, the accessibility of the airway epithelium, at the interface of the external and internal milieu, opens new avenues for the development of a new generation of potential therapies, such as RNA-based therapies, which could be administered via inhalation for rapid and targeted uptake by epithelial cells directly involved in driving airway inflammation. Epithelial miRNAs targeting P2RY2 are highly attractive for therapeutic intervention, since the airway epithelium is present and enables delivery of therapeutic drugs via an inhaled route. Non-epithelial cells in the airways, especially macrophages and other phagocytic cells, may also take up therapeutic oligonucleotides. However, studies using fluorescently conjugated antagomirs in mice have demonstrated efficient uptake specifically by the airway epithelium compared to other cellular populations (82). Using miRNA reporter systems to carefully target P2RY2 in epithelial cells will be an important future goal.
Taken together, our findings clearly demonstrate that P2RY2 promotes HDM-induced airway inflammation by mediating proinflammatory cytokine production in airway epithelial cells, monocytes, and dendritic cells. P2RY2 can also promote lung recruitment of dendritic cells and monocytes. In addition, P2RY2 on the epithelium favors IL-33 expression and subsequent Th2-like immune response in the airways. That points towards an involvement of the innate immune system in asthma pathogenesis. Our investigations concerning bone-marrow-derived cells from hematopoietic origin support our former findings demonstrating that P2RY2 is crucial for migration and maintenance of asthma by recruitment of dendritic cells. More unexpected was the apparent participation of monocytes/macrophages in asthma conducted possibly by production of MCP-1/CCL2, IL-8 homologues, and recruitment of monocytes into the lungs.
In conclusion, we previously described the potential role of P2RY2 in AAI, and in this study, we further identified the cell-type-specific role of P2RYR in AAI. Our results emphasize the importance of the P2Y2 receptor signaling specifically expressed by airway epithelial cells, monocytes, and dendritic cells as a potential therapeutic target in AAI. These findings point to the diversity and ambivalence of immune modulatory effects induced by P2RY2 signaling and underlines the necessity for further investigations.
The original contributions presented in the study are included in the article/Supplementary Material, further inquiries can be directed to the corresponding author/s.
Mice experiments were performed in accordance to the local ethic committee (G12-096) of Freiburg University. The study was conducted in accordance with the local legislation and institutional requirements.
DS and AE-G provided the main manuscript. AE-G prepared the revised manuscript and point-by-point response to reviewers’ comments. DS performed the mice experiments. DS and MI performed data analysis and statistical analysis. MI performed lung function analysis. ZK and AE-G generated BM-derived macrophage, monocytes, and dendritic cells. MK performed genotyping and breeding of animals. SM and SS assisted with in vitro experiments. MI planned and supervised the study. All authors contributed to the article and approved the submitted version.
This work was funded by University of Freiburg and Medical University of Vienna institutional funds.
We would like to thank the team of the animal facility at University of Freiburg and Medical University of Vienna for taking such good care of our animals. We are also grateful to Dr. Sanja Cicko (University of Freiburg) for her help during the course of the study.
The authors declare that the research was conducted in the absence of any commercial or financial relationships that could be construed as a potential conflict of interest.
All claims expressed in this article are solely those of the authors and do not necessarily represent those of their affiliated organizations, or those of the publisher, the editors and the reviewers. Any product that may be evaluated in this article, or claim that may be made by its manufacturer, is not guaranteed or endorsed by the publisher.
The Supplementary Material for this article can be found online at: https://www.frontiersin.org/articles/10.3389/fimmu.2023.1209097/full#supplementary-material
AAI, acute airway inflammation; ATP, adenosine triphosphate; BALF, broncho-alveolar lavage fluid; BHR, bronchial hyper-responsiveness; BM, bone marrow; BMDM, bone-marrow-derived macrophages; Cre, cyclization recombination or causes recombination; DALYs, disability-adjusted life years; DAMPs, damage-associated molecular patterns; DCs, dendritic cells; FACS, fluorescence-activated cell sorting; H&E, hematoxylin and eosin; HDM, house dust mite; i.p., intraperitoneal; i.t., intratracheally; IL, interleukin; LPS, lipopolysaccharide; MCP-1/CCL2, monocyte chemoattractant protein 1/chemokine (C–C motif) ligand 2; M-CSF, macrophage colony-stimulating factor; NALP3 NACHT, LRR and PYD domains-containing protein 3; MLN, mediastinal lymph node; P2R, purinergic type 2 receptor; PAMP, pathogen-associated molecular patterns; PBMC, peripheral blood mononuclear cell; qPCR, quantitative polymerase chain reaction; SPF, specific pathogen free; Th2 T-helper cell type 2.
1. Lv X, Tang W, Qin J, Wang W, Dong J, Wei Y. The crosslinks between ferroptosis and autophagy in asthma. Front Immunol (2023) 14:1140791. doi: 10.3389/fimmu.2023.1140791
2. Diseases GBD, Injuries C. Global burden of 369 diseases and injuries in 204 countries and territories, 1990-2019: a systematic analysis for the Global Burden of Disease Study 2019. Lancet (2020) 396:1204–22. doi: 10.1016/S0140-6736(20)30925-9
3. Cohn L, Elias JA, Chupp GL. Asthma: mechanisms of disease persistence and progression. Annu Rev Immunol (2004) 22:789–815. doi: 10.1146/annurev.immunol.22.012703.104716
4. Holgate ST, Davies DE, Powell RM, Howarth PH, Haitchi HM, Holloway JW. Local genetic and environmental factors in asthma disease pathogenesis: chronicity and persistence mechanisms. Eur Respir J (2007) 29:793–803. doi: 10.1183/09031936.00087506
5. Aron JL, Akbari O. Regulatory T cells and type 2 innate lymphoid cell-dependent asthma. Allergy (2017) 72:1148–55. doi: 10.1111/all.13139
6. Holgate ST. Innate and adaptive immune responses in asthma. Nat Med (2012) 18:673–83. doi: 10.1038/nm.2731
7. Hammad H, Lambrecht BN. Dendritic cells and epithelial cells: linking innate and adaptive immunity in asthma. Nat Rev Immunol (2008) 8:193–204. doi: 10.1038/nri2275
8. Ferreira I, Liberal J, Martins JD, Silva A, Neves BM, Cruz MT. Inflammasome in dendritic cells immunobiology: implications to diseases and therapeutic strategies. Curr Drug Targets (2017) 18:1003–18. doi: 10.2174/1389450117666160921144830
9. Murdoch JR, Lloyd CM. Chronic inflammation and asthma. Mutat Res (2010) 690:24–39. doi: 10.1016/j.mrfmmm.2009.09.005
10. Di Virgilio F, Sarti AC, Coutinho-Silva R. Purinergic signaling, DAMPs, and inflammation. Am J Physiol Cell Physiol (2020) 318:C832–5. doi: 10.1152/ajpcell.00053.2020
11. Idzko M, Ferrari D, Eltzschig HK. Nucleotide signalling during inflammation. Nature (2014) 509:310–7. doi: 10.1038/nature13085
12. Myrtek D, Idzko M. Chemotactic activity of extracellular nucleotideson human immune cells. Purinergic Signal (2007) 3:5–11. doi: 10.1007/s11302-006-9032-0
13. Choi JY, Shin JH, Kim JL, Jung SH, Son EJ, Song MH, et al. P2Y2 agonist induces mucin secretion via Ca2+- and inositol 1,4,5-triphosphate-dependent pathway in human middle ear epithelial cells. Hear Res (2005) 209:24–31. doi: 10.1016/j.heares.2005.05.012
14. Gayle S, Burnstock G. Immunolocalisation of P2X and P2Y nucleotide receptors in the rat nasal mucosa. Cell Tissue Res (2005) 319:27–36. doi: 10.1007/s00441-004-0979-2
15. Mori T, Miyashita T, Akiyama K, Inamoto R, Mori N. The expression of P2Y1, 2, 4, and 6 receptors in rat endolymphatic sac epithelia. Neuroreport (2009) 20:419–23. doi: 10.1097/WNR.0b013e328325a926
16. Wong AM, Chow AW, Au SC, Wong CC, Ko WH. Apical versus basolateral P2Y(6) receptor-mediated Cl(-) secretion in immortalized bronchial epithelia. Am J Respir Cell Mol Biol (2009) 40:733–45. doi: 10.1165/rcmb.2008-0020OC
17. Moore DJ, Chambers JK, Wahlin JP, Tan KB, Moore GB, Jenkins O, et al. Expression pattern of human P2Y receptor subtypes: a quantitative reverse transcription-polymerase chain reaction study. Biochim Biophys Acta (2001) 1521:107–19. doi: 10.1016/S0167-4781(01)00291-3
18. Tokuyama Y, Hara M, Jones EM, Fan Z, Bell GI. Cloning of rat and mouse P2Y purinoceptors. Biochem Biophys Res Commun (1995) 211:211–8. doi: 10.1006/bbrc.1995.1798
19. Kobayashi K, Fukuoka T, Yamanaka H, Dai Y, Obata K, Tokunaga A, et al. Neurons and glial cells differentially express P2Y receptor mRNAs in the rat dorsal root ganglion and spinal cord. J Comp Neurol (2006) 498:443–54. doi: 10.1002/cne.21066
20. Lustig KD, Shiau AK, Brake AJ, Julius D. Expression cloning of an ATP receptor from mouse neuroblastoma cells. Proc Natl Acad Sci U.S.A. (1993) 90:5113–7. doi: 10.1073/pnas.90.11.5113
21. Ferrari D, Idzko M, Dichmann S, Purlis D, Virchow C, Norgauer J, et al. P2 purinergic receptors of human eosinophils: characterization and coupling to oxygen radical production. FEBS Lett (2000) 486:217–24. doi: 10.1016/S0014-5793(00)02306-1
22. Gorini S, Callegari G, Romagnoli G, Mammi C, Mavilio D, Rosano G, et al. ATP secreted by endothelial cells blocks CX(3)CL 1-elicited natural killer cell chemotaxis and cytotoxicity via P2Y(1)(1) receptor activation. Blood (2010) 116:4492–500. doi: 10.1182/blood-2009-12-260828
23. Jasmer KJ, Woods LT, Forti KM, Martin AL, Camden JM, Colonna M, et al. P2Y(2) receptor antagonism resolves sialadenitis and improves salivary flow in a Sjogren’s syndrome mouse model. Arch Oral Biol (2021) 124:105067. doi: 10.1016/j.archoralbio.2021.105067
24. Jin J, Dasari VR, Sistare FD, Kunapuli SP. Distribution of P2Y receptor subtypes on haematopoietic cells. Br J Pharmacol (1998) 123:789–94. doi: 10.1038/sj.bjp.0701665
25. Muller T, Robaye B, Vieira RP, Ferrari D, Grimm M, Jakob T, et al. The purinergic receptor P2Y2 receptor mediates chemotaxis of dendritic cells and eosinophils in allergic lung inflammation. Allergy (2010) 65:1545–53. doi: 10.1111/j.1398-9995.2010.02426.x
26. Wang L, Jacobsen SE, Bengtsson A, Erlinge D. P2 receptor mRNA expression profiles in human lymphocytes, monocytes and CD34+ stem and progenitor cells. BMC Immunol (2004) 5:16. doi: 10.1186/1471-2172-5-16
27. Woods LT, Camden JM, Khalafalla MG, Petris MJ, Erb L, Ambrus JL Jr., et al. P2Y(2) R deletion ameliorates sialadenitis in IL-14alpha-transgenic mice. Oral Dis (2018) 24:761–71. doi: 10.1111/odi.12823
28. Chen Y, Corriden R, Inoue Y, Yip L, Hashiguchi N, Zinkernagel A, et al. ATP release guides neutrophil chemotaxis via P2Y2 and A3 receptors. Science (2006) 314:1792–5. doi: 10.1126/science.1132559
29. Elliott MR, Chekeni FB, Trampont PC, Lazarowski ER, Kadl A, Walk SF, et al. Nucleotides released by apoptotic cells act as a find-me signal to promote phagocytic clearance. Nature (2009) 461:282–6. doi: 10.1038/nature08296
30. Ferrari D, la Sala A, Panther E, Norgauer J, Di Virgilio F, Idzko M. Activation of human eosinophils via P2 receptors: novel findings and future perspectives. J Leukoc Biol (2006) 79:7–15. doi: 10.1189/jlb.0505286
31. Jacob F, Perez Novo C, Bachert C, Van Crombruggen K. Purinergic signaling in inflammatory cells: P2 receptor expression, functional effects, and modulation of inflammatory responses. Purinergic Signal (2013) 9:285–306. doi: 10.1007/s11302-013-9357-4
32. Nicholas RA, Watt WC, Lazarowski ER, Li Q, Harden K. Uridine nucleotide selectivity of three phospholipase C-activating P2 receptors: identification of a UDP-selective, a UTP-selective, and an ATP- and UTP-specific receptor. Mol Pharmacol (1996) 50:224–9.
33. Deterding R, Retsch-Bogart G, Milgram L, Gibson R, Daines C, Zeitlin PL, et al. Safety and tolerability of denufosol tetrasodium inhalation solution, a novel P2Y2 receptor agonist: results of a phase 1/phase 2 multicenter study in mild to moderate cystic fibrosis. Pediatr Pulmonol (2005) 39:339–48. doi: 10.1002/ppul.20192
34. Ghanem E, Robaye B, Leal T, Leipziger J, Van Driessche W, Beauwens R, et al. The role of epithelial P2Y2 and P2Y4 receptors in the regulation of intestinal chloride secretion. Br J Pharmacol (2005) 146:364–9. doi: 10.1038/sj.bjp.0706353
35. Yamamoto S, Ichishima K, Ehara T. Regulation of extracellular UTP-activated Cl- current by P2Y-PLC-PKC signaling and ATP hydrolysis in mouse ventricular myocytes. J Physiol Sci (2007) 57:85–94. doi: 10.2170/physiolsci.RP011406
36. Buscher R, Hoerning A, Patel HH, Zhang S, Arthur DB, Grasemann H, et al. P2Y2 receptor polymorphisms and haplotypes in cystic fibrosis and their impact on Ca2+ influx. Pharmacogenet Genomics (2006) 16:199–205. doi: 10.1097/01.fpc.0000189798.11468.6a
37. Davis CW, Lazarowski E. Coupling of airway ciliary activity and mucin secretion to mechanical stresses by purinergic signaling. Respir Physiol Neurobiol (2008) 163:208–13. doi: 10.1016/j.resp.2008.05.015
38. Kellerman D, Evans R, Mathews D, Shaffer C. Inhaled P2Y2 receptor agonists as a treatment for patients with Cystic Fibrosis lung disease. Adv Drug Delivery Rev (2002) 54:1463–74. doi: 10.1016/S0169-409X(02)00154-0
39. Rieg T, Bundey RA, Chen Y, Deschenes G, Junger W, Insel PA, et al. Mice lacking P2Y2 receptors have salt-resistant hypertension and facilitated renal Na+ and water reabsorption. FASEB J (2007) 21:3717–26. doi: 10.1096/fj.07-8807com
40. Jin H, Ko YS, Kim HJ. P2Y2R-mediated inflammasome activation is involved in tumor progression in breast cancer cells and in radiotherapy-resistant breast cancer. Int J Oncol (2018) 53:1953–66. doi: 10.3892/ijo.2018.4552
41. Stachon P, Geis S, Peikert A, Heidenreich A, Michel NA, Unal F, et al. Extracellular ATP induces vascular inflammation and atherosclerosis via purinergic receptor Y2 in mice. Arterioscler Thromb Vasc Biol (2016) 36:1577–86. doi: 10.1161/ATVBAHA.115.307397
42. Junger WG. Immune cell regulation by autocrine purinergic signalling. Nat Rev Immunol (2011) 11:201–12. doi: 10.1038/nri2938
43. Bertin G, Poujeol C, Rubera I, Poujeol P, Tauc M. In vivo Cre/loxP mediated recombination in mouse Clara cells. Transgenic Res (2005) 14:645–54. doi: 10.1007/s11248-005-7214-0
44. de Boer J, Williams A, Skavdis G, Harker N, Coles M, Tolaini M, et al. Transgenic mice with hematopoietic and lymphoid specific expression of Cre. Eur J Immunol (2003) 33:314–25. doi: 10.1002/immu.200310005
45. Clausen BE, Burkhardt C, Reith W, Renkawitz R, Forster I. Conditional gene targeting in macrophages and granulocytes using LysMcre mice. Transgenic Res (1999) 8:265–77. doi: 10.1023/A:1008942828960
46. Sawada S, Scarborough JD, Killeen N, Littman DR. A lineage-specific transcriptional silencer regulates CD4 gene expression during T lymphocyte development. Cell (1994) 77:917–29. doi: 10.1016/0092-8674(94)90140-6
47. Caton ML, Smith-Raska MR, Reizis B. Notch-RBP-J signaling controls the homeostasis of CD8- dendritic cells in the spleen. J Exp Med (2007) 204:1653–64. doi: 10.1084/jem.20062648
48. Simon DM, Arikan MC, Srisuma S, Bhattacharya S, Tsai LW, Ingenito EP, et al. Epithelial cell PPAR[gamma] contributes to normal lung maturation. FASEB J (2006) 20:1507–9. doi: 10.1096/fj.05-5410fje
49. Georgiades P, Ogilvy S, Duval H, Licence DR, Charnock-Jones DS, Smith SK, et al. VavCre transgenic mice: a tool for mutagenesis in hematopoietic and endothelial lineages. Genesis (2002) 34:251–6. doi: 10.1002/gene.10161
50. Miyake Y, Kaise H, Isono K, Koseki H, Kohno K, Tanaka M. Protective role of macrophages in noninflammatory lung injury caused by selective ablation of alveolar epithelial type II Cells. J Immunol (2007) 178:5001–9. doi: 10.4049/jimmunol.178.8.5001
51. Lee PP, Fitzpatrick DR, Beard C, Jessup HK, Lehar S, Makar KW, et al. A critical role for Dnmt1 and DNA methylation in T cell development, function, and survival. Immunity (2001) 15:763–74. doi: 10.1016/S1074-7613(01)00227-8
52. Idzko M, Hammad H, van Nimwegen M, Kool M, Willart MA, Muskens F, et al. Extracellular ATP triggers and maintains asthmatic airway inflammation by activating dendritic cells. Nat Med (2007) 13:913–9. doi: 10.1038/nm1617
53. Muller T, Vieira RP, Grimm M, Durk T, Cicko S, Zeiser R, et al. A potential role for P2X7R in allergic airway inflammation in mice and humans. Am J Respir Cell Mol Biol (2011) 44:456–64. doi: 10.1165/rcmb.2010-0129OC
54. Vieira RP, Muller T, Grimm M, von Gernler V, Vetter B, Durk T, et al. Purinergic receptor type 6 contributes to airway inflammation and remodeling in experimental allergic airway inflammation. Am J Respir Crit Care Med (2011) 184:215–23. doi: 10.1164/rccm.201011-1762OC
55. Hammad H, Chieppa M, Perros F, Willart MA, Germain RN, Lambrecht BN. House dust mite allergen induces asthma via Toll-like receptor 4 triggering of airway structural cells. Nat Med (2009) 15:410–6. doi: 10.1038/nm.1946
56. Francke A, Herold J, Weinert S, Strasser RH, Braun-Dullaeus RC. Generation of mature murine monocytes from heterogeneous bone marrow and description of their properties. J Histochem Cytochem (2011) 59:813–25. doi: 10.1369/0022155411416007
57. Wagner M, Koester H, Deffge C, Weinert S, Lauf J, Francke A, et al. Isolation and intravenous injection of murine bone marrow derived monocytes. J Vis Exp (2014) (94):52347. doi: 10.3791/52347
58. Muller T, Durk T, Blumenthal B, Herouy Y, Sorichter S, Grimm M, et al. Iloprost has potent anti-inflammatory properties on human monocyte-derived dendritic cells. Clin Exp Allergy (2010) 40:1214–21. doi: 10.1111/j.1365-2222.2010.03558.x
59. van Rijt LS, Kuipers H, Vos N, Hijdra D, Hoogsteden HC, Lambrecht BN. A rapid flow cytometric method for determining the cellular composition of bronchoalveolar lavage fluid cells in mouse models of asthma. J Immunol Methods (2004) 288:111–21. doi: 10.1016/j.jim.2004.03.004
60. Zech A, Wiesler B, Ayata CK, Schlaich T, Durk T, Hossfeld M, et al. P2rx4 deficiency in mice alleviates allergen-induced airway inflammation. Oncotarget (2016) 7:80288–97. doi: 10.18632/oncotarget.13375
61. Ayata CK, Ganal SC, Hockenjos B, Willim K, Vieira RP, Grimm M, et al. Purinergic P2Y(2) receptors promote neutrophil infiltration and hepatocyte death in mice with acute liver injury. Gastroenterology (2012) 143:1620–1629 e4. doi: 10.1053/j.gastro.2012.08.049
62. Muller PY, Janovjak H, Miserez AR, Dobbie Z. Processing of gene expression data generated by quantitative real-time RT-PCR. Biotechniques (2002) 32:1372–4, 1376, 1378-9.
63. Li H, Nookala S, Re F. Aluminum hydroxide adjuvants activate caspase-1 and induce IL-1beta and IL-18 release. J Immunol (2007) 178:5271–6. doi: 10.4049/jimmunol.178.8.5271
64. Haspeslagh E, Debeuf N, Hammad H, Lambrecht BN. Murine models of allergic asthma. Methods Mol Biol (2017) 1559:121–36. doi: 10.1007/978-1-4939-6786-5_10
65. Lambrecht BN, Hammad H. The airway epithelium in asthma. Nat Med (2012) 18:684–92. doi: 10.1038/nm.2737
66. Jacquet A. The role of innate immunity activation in house dust mite allergy. Trends Mol Med (2011) 17:604–11. doi: 10.1016/j.molmed.2011.05.014
67. Idzko M, Ayata CK, Muller T, Durk T, Grimm M, Baudiss K, et al. Attenuated allergic airway inflammation in Cd39 null mice. Allergy (2013) 68:472–80. doi: 10.1111/all.12119
68. Reynolds SD, Malkinson AM. Clara cell: progenitor for the bronchiolar epithelium. Int J Biochem Cell Biol (2010) 42:1–4. doi: 10.1016/j.biocel.2009.09.002
69. Papazian D, Hansen S, Wurtzen PA. Airway responses towards allergens - from the airway epithelium to T cells. Clin Exp Allergy (2015) 45:1268–87. doi: 10.1111/cea.12451
70. Kouzaki H, Iijima K, Kobayashi T, O’Grady SM, Kita H. The danger signal, extracellular ATP, is a sensor for an airborne allergen and triggers IL-33 release and innate Th2-type responses. J Immunol (2011) 186:4375–87. doi: 10.4049/jimmunol.1003020
71. Lambrecht BN, Hammad H. Biology of lung dendritic cells at the origin of asthma. Immunity (2009) 31:412–24. doi: 10.1016/j.immuni.2009.08.008
72. Kushwah R, Hu J. Complexity of dendritic cell subsets and their function in the host immune system. Immunology (2011) 133:409–19. doi: 10.1111/j.1365-2567.2011.03457.x
73. Vanderstocken G, Bondue B, Horckmans M, Di Pietrantonio L, Robaye B, Boeynaems JM, et al. P2Y2 receptor regulates VCAM-1 membrane and soluble forms and eosinophil accumulation during lung inflammation. J Immunol (2010) 185:3702–7. doi: 10.4049/jimmunol.0903908
74. Klambt V, Wohlfeil SA, Schwab L, Hulsdunker J, Ayata K, Apostolova P, et al. A novel function for P2Y2 in myeloid recipient-derived cells during graft-versus-host disease. J Immunol (2015) 195:5795–804. doi: 10.4049/jimmunol.1501357
75. Higgins KR, Kovacevic W, Stokes L. Nucleotides regulate secretion of the inflammatory chemokine CCL2 from human macrophages and monocytes. Mediators Inflammation (2014) 2014:293925. doi: 10.1155/2014/293925
76. Stokes L, Surprenant A. Purinergic P2Y2 receptors induce increased MCP-1/CCL2 synthesis and release from rat alveolar and peritoneal macrophages. J Immunol (2007) 179:6016–23. doi: 10.4049/jimmunol.179.9.6016
77. Xu LL, Warren MK, Rose WL, Gong W, Wang JM. Human recombinant monocyte chemotactic protein and other C-C chemokines bind and induce directional migration of dendritic cells in vitro. J Leukoc Biol (1996) 60:365–71. doi: 10.1002/jlb.60.3.365
78. Ben Yebdri F, Kukulski F, Tremblay A, Sevigny J. Concomitant activation of P2Y(2) and P2Y(6) receptors on monocytes is required for TLR1/2-induced neutrophil migration by regulating IL-8 secretion. Eur J Immunol (2009) 39:2885–94. doi: 10.1002/eji.200939347
79. Krausgruber T, Fortelny N, Fife-Gernedl V, Senekowitsch M, Schuster LC, Lercher A, et al. Structural cells are key regulators of organ-specific immune responses. Nature (2020) 583:296–302. doi: 10.1038/s41586-020-2424-4
80. Kim HY, DeKruyff RH, Umetsu DT. The many paths to asthma: phenotype shaped by innate and adaptive immunity. Nat Immunol (2010) 11:577–84. doi: 10.1038/ni.1892
81. Zhu J, Yamane H, Paul WE. Differentiation of effector CD4 T cell populations (*). Annu Rev Immunol (2010) 28:445–89. doi: 10.1146/annurev-immunol-030409-101212
Keywords: asthma, house dust mite, purinergic receptors, allergic airway inflammation, P2RY2
Citation: Schneble D, El-Gazzar A, Kargarpour Z, Kramer M, Metekol S, Stoshikj S and Idzko M (2023) Cell-type-specific role of P2Y2 receptor in HDM-driven model of allergic airway inflammation. Front. Immunol. 14:1209097. doi: 10.3389/fimmu.2023.1209097
Received: 20 April 2023; Accepted: 28 August 2023;
Published: 14 September 2023.
Edited by:
Neeloffer Mookherjee, University of Manitoba, CanadaReviewed by:
Kamil Grubczak, Medical University of Bialystok, PolandCopyright © 2023 Schneble, El-Gazzar, Kargarpour, Kramer, Metekol, Stoshikj and Idzko. This is an open-access article distributed under the terms of the Creative Commons Attribution License (CC BY). The use, distribution or reproduction in other forums is permitted, provided the original author(s) and the copyright owner(s) are credited and that the original publication in this journal is cited, in accordance with accepted academic practice. No use, distribution or reproduction is permitted which does not comply with these terms.
*Correspondence: Marco Idzko, bWFyY28uaWR6a29AbWVkdW5pd2llbi5hYy5hdA==
†These authors have contributed equally to this work and share first authorship
Disclaimer: All claims expressed in this article are solely those of the authors and do not necessarily represent those of their affiliated organizations, or those of the publisher, the editors and the reviewers. Any product that may be evaluated in this article or claim that may be made by its manufacturer is not guaranteed or endorsed by the publisher.
Research integrity at Frontiers
Learn more about the work of our research integrity team to safeguard the quality of each article we publish.