- 1Institute of Pharmacology, Philipps-Universität Marburg, Marburg, Germany
- 2Department of Dermatology and Allergology, Philipps-Universität Marburg, Marburg, Germany
- 3Center for Dermatopathology, Freiburg, Germany
- 4Laboratory for Dermatohistology, Offenbach am Main, Germany
- 5Institute of Pathology, Philipps-Universität Marburg, Marburg, Germany
- 6Department of Pharmacology, Max-Planck-Institute for Heart and Lung Research, Bad Nauheim, Germany
- 7Department of Dermatology, University Hospital Basel, Basel, Switzerland
- 8Pathology Unit, University Hospital Basel, Basel, Switzerland
Introduction: We here thought to dissect the inflammatory signature in lesions of three skin disorders, which show a common adaptive immune response against autoantigens of the skin but are characterized by diverging clinical phenotypes. Pemphigus vulgaris (PV) and bullous pemphigoid (BP) are type-2-dependent, IgG autoantibody-driven blistering disorders of mucous membranes and skin, which target desmoglein (Dsg)3 and bullous pemphigoid (BP)180, respectively. In contrast, lichen planus (LP) is a common chronic inflammatory disease of the skin and mucous membranes with a pronounced dermal T cell infiltrate. We previously identified peripheral type 1 and 17 T cell responses against Dsg3 and BP180 in a cohort of LP patients strongly suggesting that the underlying inflammatory T cell signature may drive the evolving phenotype.
Methods: Paraffin-embedded skin biopsies from well-characterized patients with LP (n=31), BP (n=19), PV (n=9), and pemphigus foliaceus (PF) (n=2) were analysed. Areas with the most prominent inflammatory infiltrate were excised with punch biopsies and tissue microarrays (TMA) containing multiple biopsies were created. Using multicolor immunofluorescence, the inflammatory infiltrate was stained with antibodies against multiple cellular markers, i. e. CD3ϵ, CD4, CD15, TCR-δ, the cytokine IL-17A, and the transcription factors, T-bet and GATA-3.
Results: In LP, there was a higher number of CD4+ T cells expressing T-bet compared to GATA-3. In contrast, CD4+ T cells in PV and BP skin lesions more frequently expressed GATA-3 than T-bet. IL-17A+ cells and IL-17A+ T cells were found to a similar extent in all the three disorders. IL-17A+ granulocytes were more predominant in BP than in LP or PV. Of note, the majority of IL-17A+ cells in LP were neither T cells nor granulocytes.
Discussion: Our findings in inflammatory skin infiltrates clearly show a predominant type 1 signature in LP in contrast to a preponderance of type 2 T cells in PV and BP. In contrast to LP, granulocytes and to a much lesser extent CD3+ T cells were a cellular source of IL-17A in BP and PV. These data strongly suggest that different inflammatory cell signatures drive evolving clinically diverse phenotypes of LP, PV and BP despite common target antigens of the skin.
Introduction
Chronic inflammatory skin disorders associated with autoimmune responses remain poorly understood, due to a lack of knowledge about their specific target antigens, disease variability and co-morbidities. Immunologically, they can be grouped into disorders associated with T cell-mediated and with antibody-mediated autoimmune responses, both of which require the activation of autoreactive T cell subsets against target proteins expressed in the epidermis and dermis.
Lichen planus (LP) is one of the most common T cell-mediated autoimmune diseases of the skin and mucous membranes, and affects 1-2% of the general population (1–3). Clinically, it presents as reticular, purple papules and plaques of the skin and white papules and erosions of mucous membranes, and follows a chronic-relapsing course (4–6). LP mucosal and skin lesions are infiltrated by T cells which include CD8+ and CD4+ populations. Most relevant for the direct pathology of LP are pro-inflammatory CD8+ T cells, which are mainly located around the basal layer of the epidermis where they trigger apoptosis of epidermal keratinocytes (1, 2, 6–8). While the targeted autoantigens have not been fully identified, self-peptides of keratinocyte-derived proteins presented on HLA class I and II alleles may play a central role in disease pathogenesis (4, 7). Several type 1 and recently type 17 cytokines such as IFN-γ, TNF-α , IL-17A and IL-22 have been detected in LP lesions, as well as in patient sera, supporting the concept that LP is a highly inflammatory skin disorder (8, 9). These cytokines are thought to be mainly produced by CD8+ T cells, and to a lesser extent, by CD4+ T helper cell populations (10), which display a Th1 and Th17 phenotype. While their recruitment and activation mechanisms remain to be elucidated, pro-inflammatory cytokines produced by these populations play an important role in disease pathogenesis, and initial studies using small molecules or anti-cytokine monoclonal antibodies have shown promising results (11–14).
Autoantibody-mediated autoimmune diseases, such as pemphigus vulgaris (PV) and bullous pemphigoid (BP), share clinical similarities with mucosal LP. PV and BP are characterized by blisters and erosions of the skin and mucous membranes, which are caused by IgG autoantibodies against distinct adhesion proteins of desmosomes and hemidesmosomes. In PV and pemphigus foliaceus (PF), IgG autoantibodies are directed against components of desmosomes, namely desmoglein (Dsg) 3 and Dsg1. In BP, IgG targeting hemidesmosomal antigens, namely bullous pemphigoid antigen 1 (BP230) and bullous pemphigoid antigen 2 (BP180) induce the influx of innate immune cells in the skin leading to pronounced inflammation with pruritus and tense blisters.
The cellular infiltrate in BP and PV skin lesions is very heterogeneous and consists primarily of neutrophilic granulocytes (BP), eosinophils, CD4+ T cells and B lymphocytes, yet is less inflammatory than LP. Autoreactive T and B lymphocytes play a central role in the pathogenesis of both, PV and BP, and pro-inflammatory autoreactive Th2 cells are observed at a higher ratio in PV and BP than in LP (15).
A recent single-cell RNA-sequencing study in a small number of LP and BP patients showed that both diseases lack infiltrating myeloid derived populations, when compared to healthy controls (HC), atopic dermatitis or psoriasis lesions, yet display distinct T cell signatures (16). This correlates with our own recent findings which showed that patients with LP exhibit IFN-γ- and IL-17A-dominated peripheral blood T cell responses, while BP patients display a higher number of peripheral type 2 autoimmune T cell responses (15). In LP, BP180-specific peripheral Th17 cell responses correlated with a distinct Th17 cell infiltrate along the dermal-epidermal basement membrane zone in LP skin lesions. This observation strongly suggests that IL-17A is a major driver of disease pathogenesis. IL-17A polymorphism and increased serum levels of IL-17A are indeed associated with LP (17, 18), not only affecting local infiltration, but also the microbiome of oral LP patients (19). Therapeutic targeting of IL-17 or IL-23 (which is involved in Th17 development) by monoclonal antibodies has also shown positive effects in several case series (12, 14), suggesting that IL-17A producing CD4+ or CD8+ T cells play an important role in LP.
In light of the above observations, we hypothesized that LP, BP, and PV are inflammatory disorders which target identical autoantigens of the skin although with a different T cell signature. This led previous research to study whether functional differences of the evolving inflammatory skin infiltrate may eventually pave the way for the distinct clinical phenotypes that we see in LP, PV and BP in humans. A previous study has shown that mice with a transgenic T cell receptor for desmoglein 3 develop a type 1 inflammatory skin infiltrate resulting in a lichenoid phenotype with a dense band-like lymphocytic infiltrate resembling LP (20). These findings are in support of our initial hypothesis that LP is a type 1 variant of PV and BP, respectively. So far, thorough characterization of the inflammatory T cell responses in these disorders was mainly performed with peripheral blood lymphocytes. To characterize the immune signature of skin-infiltrating cells in more detail, we analyzed key transcription factors involved in type 1 and type 2 cell development, as well as IL-17A production by T cells and granulocytes in PV, BP and LP on tissue microarrays (TMA) of skin lesions using immunofluorescent antibody staining. Our findings link LP to a type 1-regulated inflammatory skin pathology while skin lesions from PV and BP patients show a more pronounced type 2 CD4+ T cell response.
Results
Dichotomy of type 1 CD4+ T cells in LP versus type 2 T cells in BP and PV
To characterize the immune signatures of the inflammatory CD4+ skin infiltrate in LP, BP, and pemphigus patients we collected paraffin-embedded skin biopsies. In total, the cohort comprised samples from 31 LP, 19 BP and 11 pemphigus patients. Within the pemphigus group, both PV (n=9) and pemphigus foliaceus (PF; n=2) were included. However, for statistical analyses, the PF samples were omitted due to the low sample number. All paraffin-embedded skin biopsies were re-embedded to generate tissue microarrays (TMAs) (Figure 1). The TMAs were then subjected to immunostaining with antibodies against distinct immune cell subsets.
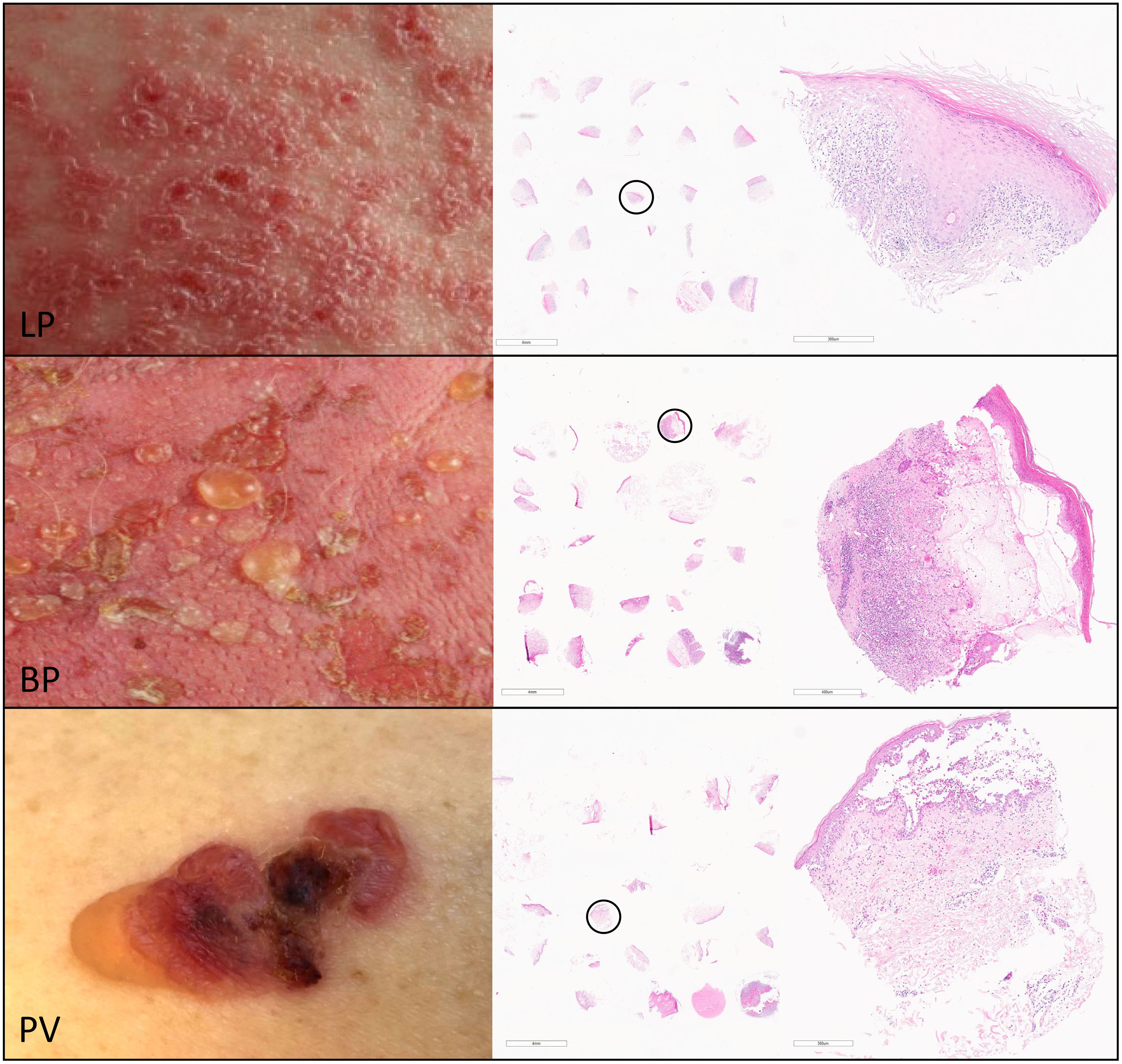
Figure 1 For the characterization of the inflammatory infiltrate of skin lesions in lichen planus (LP), bullous pemphigoid (BP) and pemphigus vulgaris (PV) we generated tissue micro arrays (TMAs) using punch biopsies from the inflammatory epidermal/dermal infiltrate of paraffin-embedded skin lesions from patients with LP (upper panel), BP (middle panel) and PV (lower panel). The right column shows representative hematoxylin-eosin staining of TMA sections from LP, BP and PV with a magnification of the circled punch biopsy.
It is known that CD4+ T helper (Th) cells play a pivotal role in inflammatory skin disorders. However, the predominant differentiation signatures of CD4+ T helper cells in the skin infiltrates in LP, BP and PV remain unclear. We therefore aimed at the visualization and quantification of Th1 and Th2 cells. To do so, we performed co-immunostaining for the Th cell marker CD4 together with T-bet and GATA-3, the master transcription factors for Th1 and Th2 cells, respectively (Figures 2A–D). In LP we found a significantly higher number of both CD4+/T-bet+ cells as well as CD4+/GATA-3+ cells compared to BP with a median of 44 CD4+/T-bet+ cells in LP and a median of 6.5 cells in BP (p < 0.0001) and a median of 19 CD4+/GATA-3+ cells in LP compared to a median of 13.5 cells in BP (p = 0.025). There was a significantly higher median of 44 CD4+/T-bet+ cells in LP compared to 5.2 cells in PV (p = 0.001) and no significant differences between BP and PV for both populations were observed (Figures 2E, F). Of note, Th1/Th2 cell ratios were highly significantly different between the three groups of LP, PV and BP patients: While LP skin lesions showed a preponderance of Th1 cells, PV and BP skin lesions predominantly contained Th2 cells (Figure 2G).
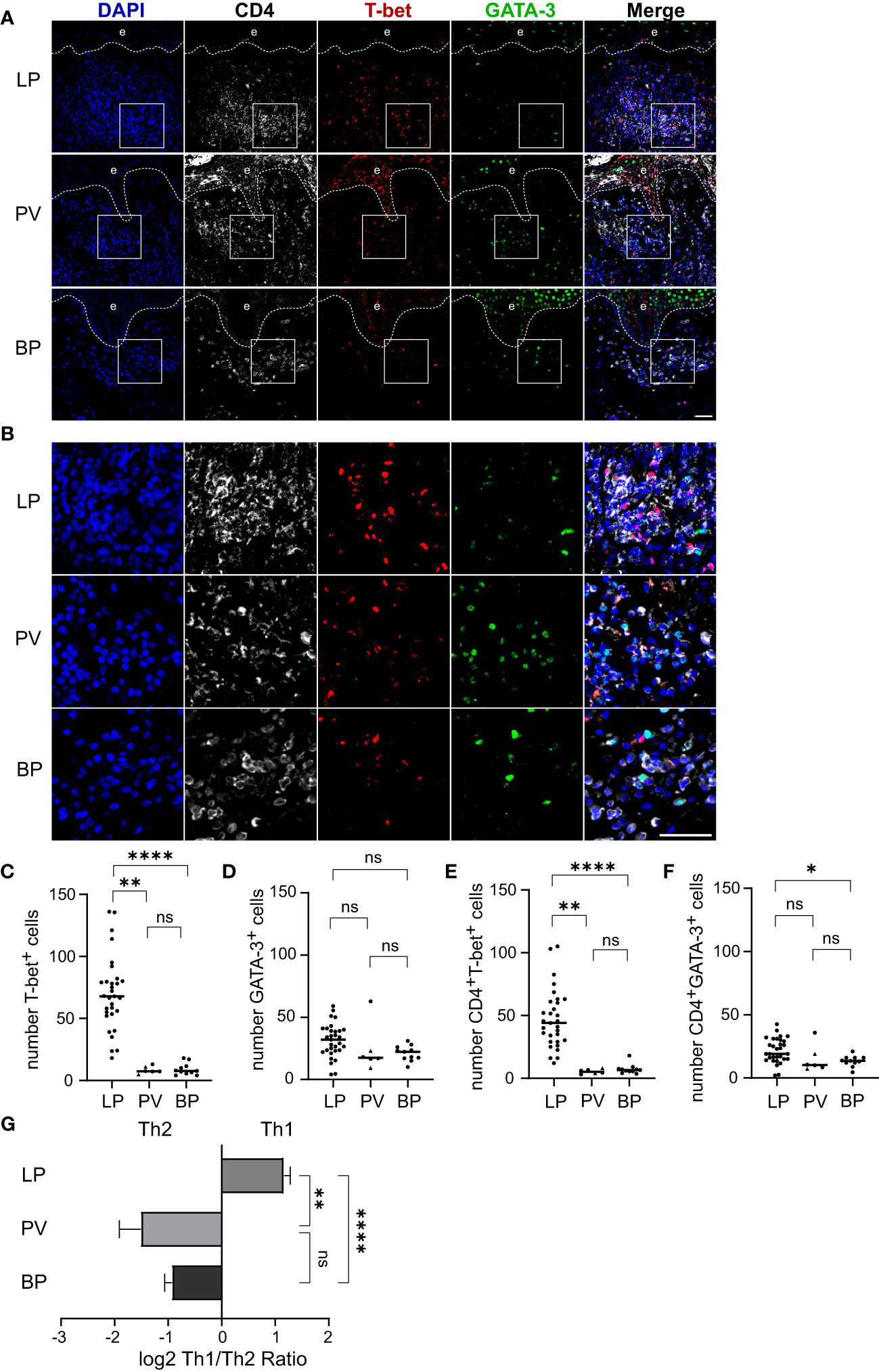
Figure 2 Th1/Th2 ratio in dermal immune cell infiltrates of LP, PV and BP patients. (A, B) Representative confocal images of immunostaining of tissue microarrays using anti-CD4 (white), T-bet (red) and GATA-3 (green) antibodies. Dashed lines in (A) indicate the border between epidermis (e) and dermis. Boxed areas in (A) are magnified in (B). Blue: 4′,6-diamidino-2-phenylindole (DAPI). Scale bars represent 40 µm. (C–F) Quantification of (C) T-bet-positive, (D) GATA-3-positive, (E) CD4-/T-bet-double-positive and (F) CD4-/GATA-3-double-positive cells per tissue section. Horizontal bars indicate median values and individual points represent a biopsy from a different patient with LP (n = 31), PV (n = 4) and BP (n = 11). Triangles in the PV-Group represent biopsies from patients with PF (n = 2), which were excluded from statistical analysis. (G) Quantification of the log2 transformed ratio between CD4-/T-bet-double-positive (Th1) and CD4-/GATA-3-double-positive (Th2) cells. Shown are mean values + S.E.M. Statistical significance was assessed using Kruskal-Wallis test with Dunn’s correction for multiple comparison. *p ≤ 0.05, **p ≤ 0.01, and ****p ≤ 0.0001. n.s., not significant.
Type 17 T cells in skin lesions from LP, BP and PV/PF patients
In addition to Th1 and Th2 cells, IL-17 producing T cells have been shown to be of major pathophysiological relevance in inflammatory skin diseases (21, 22). Whether T cell receptor (TCR)-α/β+ T cells or TCR-γ/δ+ T cells serve as IL-17A producers in the inflammatory skin infiltrate of LP, BP and/or PV is ill-defined. We therefore immunostained skin lesions for CD3ε (CD3 as a pan-T cell marker), TCR-δ chain (a marker for γδ T cells), and IL-17A (Figures 3A, B). As expected, the highest median of 312 CD3ε+ cells was found in LP skin lesions with significant differences compared to the median of 84 CD3ϵ+ cells in PV (p = 0.03) and 51 CD3ϵ+ cells in BP (p < 0.0001) (Figure 3C). In LP a median of 1.5% of the total T cell infiltrate were γδ T cells which was a significantly higher fraction compared to the median of 0% in both PV (p = 0.004) and BP (p = 0.02) (Figures 3D, E).
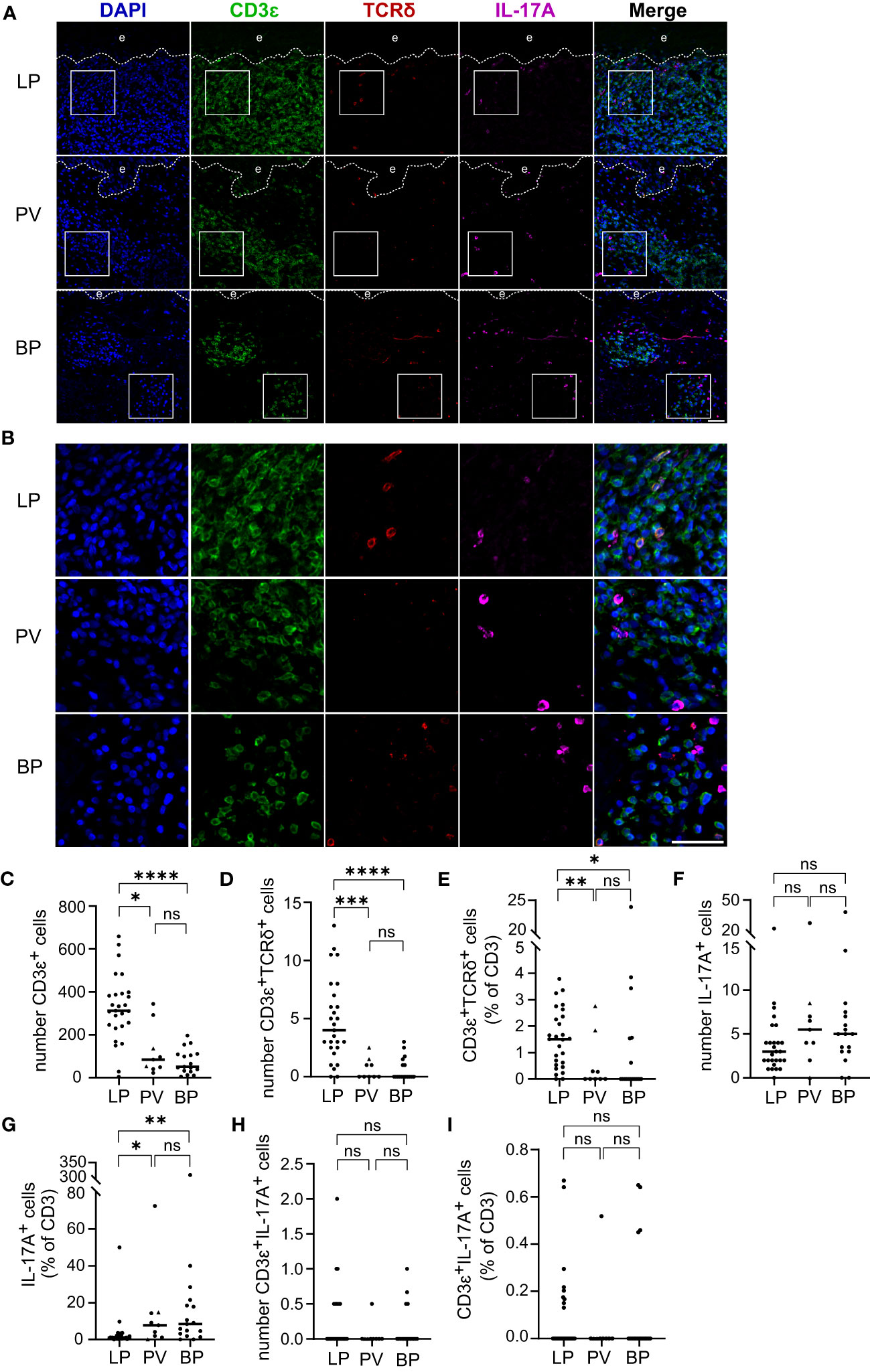
Figure 3 T cell infiltrate, γδ T cell proportion and IL-17A producing cells in LP, PV and BP patients. (A, B) Representative confocal images of immunostaining of tissue microarrays using anti-CD3ϵ (green), TCRδ (red) and IL-17A (purple) antibodies. Dashed lines in (A) indicate the border between epidermis (e) and dermis. Boxed areas in (A) are magnified in (B). Blue: 4′,6-diamidino-2-phenylindole (DAPI). Scale bars represent 40 µm. (C–E) Quantification of (C) CD3ϵ-positive cells per tissue section, (D) CD3ϵ-/TCRδ-double-positive cells per tissue section and (E) proportion of CD3ϵ-/TCRδ-double-positive cells as percentage of total CD3ϵ-positive cells. (F, G) Quantification of (F) IL-17A-positive cells per tissue section and (G) IL-17A-positive cells compared to the CD3ϵ-positive cells expressed as percentage. (H, I) Quantification of (H) CD3ϵ-/IL-17A-double-positive cells per tissue section and (I) fraction of CD3ϵ-/IL-17A-double-positive cells as percentage of total CD3ϵ-positive cells. Horizontal bars indicate median values and individual points represent a biopsy from a different patient with LP (n = 27), PV (n = 7) and BP (n = 17). Triangles in the PV-Group represent biopsies from patients with PF (n = 2), which were excluded from statistical analysis. Statistical significance was assessed using Kruskal-Wallis test with Dunn’s correction for multiple comparison. *p ≤ 0.05, **p ≤ 0.01, ***p ≤ 0.001, and ****p ≤ 0.0001. n.s., not significant.
In the analyzed skin disorders, IL-17A+ cells were observed in similar amounts, with a median of 3 IL-17A+ cells in LP, 5.5 in PV, and 5 in BP (Figure 3F). However, when normalized to the CD3ϵ+ infiltrate, there were significantly more IL-17A+ cells in PV (p = 0.02) and BP (p = 0.001) compared to LP (Figure 3G). Only a few CD3ϵ+/IL-17A+ cells were found, with no significant differences in both the absolute numbers and fraction of total T cells (Figures 3H, I). It is noteworthy that most IL-17A+ cells were negative for CD3ϵ, indicating that they were not T cells (compare Figures 3F, H), and none of the identified γδ T cells expressed IL-17A (data not shown). This prompted us to study inflammatory cells other than T cells as a potential source of IL-17A.
IL-17-producing granulocytes are predominant in BP
Our finding that the majority of IL-17A+ cells are no T cells prompted us to investigate the identity of the inflammatory cell populations other than T cells expressing IL-17A. Given that neutrophils, the most abundant of the granulocytes, are a common source of IL-17, we focused on CD15+ granulocytes in the skin infiltrates of LP, BP, and PV patients (Figures 4A, B). The total number of CD15+ granulocytes and CD15+/IL-17A+ cells were significantly greater in BP patients than in LP patients (Figures 4C, D). The median number of IL-17A+ granulocytes was 18.75 in BP and only 1 in LP (p < 0.0001). There was also a trend towards a higher median of granulocytes in PV compared to LP (Figures 4C, D). The medians of IL-17A+ cells that were negative for CD15 did not differ significantly between the three groups (Figure 4E). In LP, the majority of IL-17A+ cells were neither granulocytes nor T cells, whereas in BP and PV, most IL-17A+ cells were CD15+ granulocytes (Figures 4F, G).
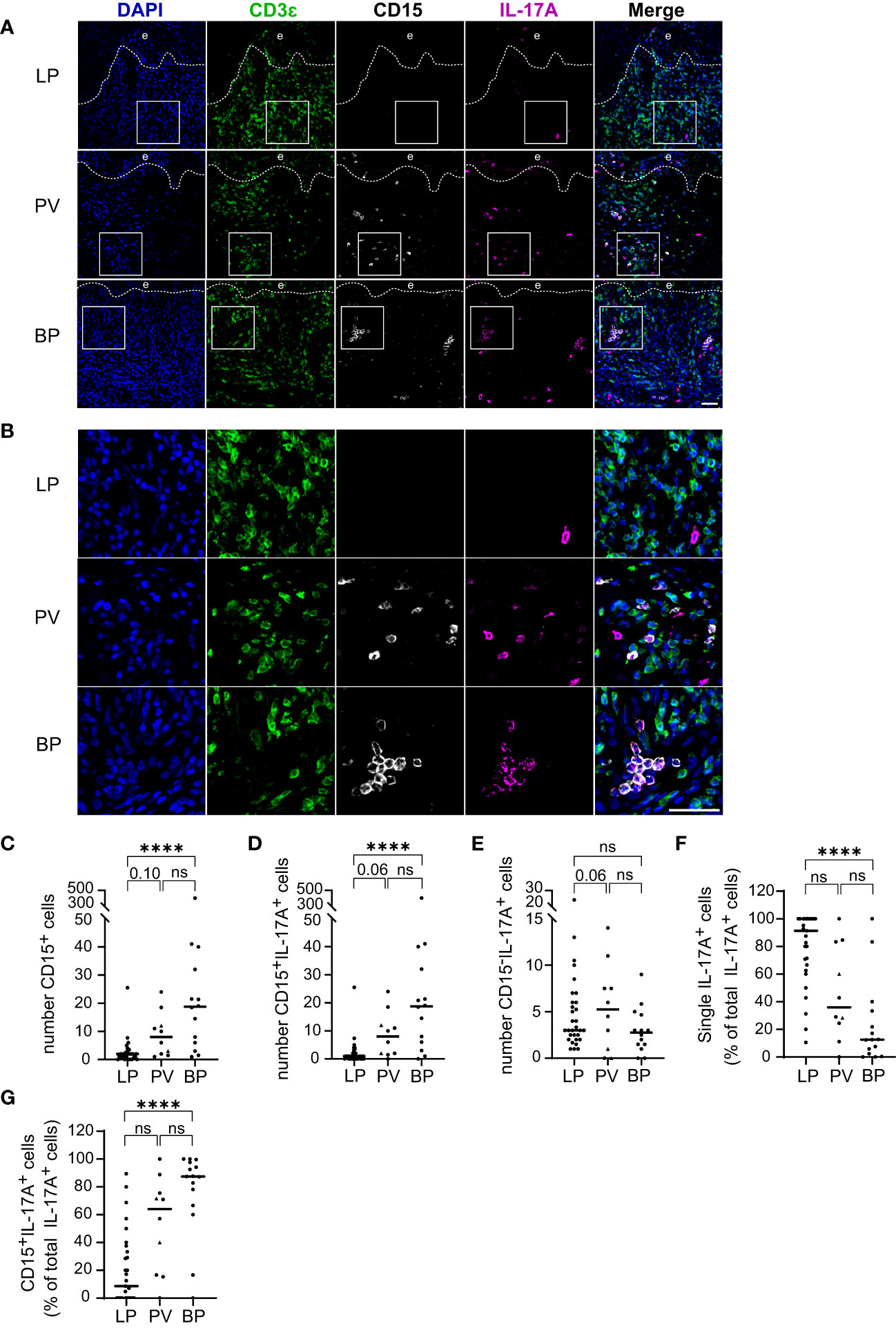
Figure 4 IL-17A producing Granulocytes in LP, PV and BP patients. (A, B) Representative confocal images of immunostaining of tissue microarrays using anti-CD3ϵ (green), CD15 (gray) and IL-17A (purple) antibodies. Dashed lines in (A) indicate the border between epidermis (e) and dermis. Boxed areas in (A) are magnified in (B). Blue: 4′,6-diamidino-2-phenylindole (DAPI). Scale bars represent 40 µm. (C–E) Quantification of (C) CD15-positive (D) CD15-/IL-17A-double-positive and (E) IL-17A-positive/CD15-negative cells per tissue section. (F, G) Quantification of the proportion of (F) single IL-17A-positive (CD3ϵ-negative/CD15-negative) cells and (G) CD15-/IL-17A-double-positive cells as percentage of total IL-17A-positive cells. Horizontal bars indicate median values and individual points represent a biopsy from a different patient with LP (n = 31), PV (n = 8) and BP (n = 14). Triangles in the PV-Group represent biopsies from patients with PF (n = 2), which were excluded from statistical analysis. Statistical significance was assessed using Kruskal-Wallis test with Dunn’s correction for multiple comparison. ****p ≤ 0.0001. n.s., not significant.
Monoclonal antibodies against IL-17 ameliorate skin lesions in lichen planus
To gain insight into the functional consequences of the distinct inflammatory signatures in LP, we studied the clinical effect of therapeutic cytokine inhibition in a patient with LP. As the majority of skin-derived IL-17A+ cells in LP neither belong to T cells nor to granulocytes, we sought to determine the functional impact of IL-17A+ cells on LP pathogenesis in general. In a 28-year-old female patient with extensive cutaneous LP, we took advantage of the availability of Secukinumab, an anti-IL-17A monoclonal antibody which is approved for the treatment of psoriasis vulgaris. The LP patient was not sufficiently responsive to topical steroids and was treated in a compassionate trial with Secukinumab at 300 mg s.c. weekly for four weeks and then 300 mg every four weeks. At week 12 of treatment with Secukinumab, the initially reddish erythematous plaques had completely disappeared leaving brownish hyperpigmentations (Figure 5).
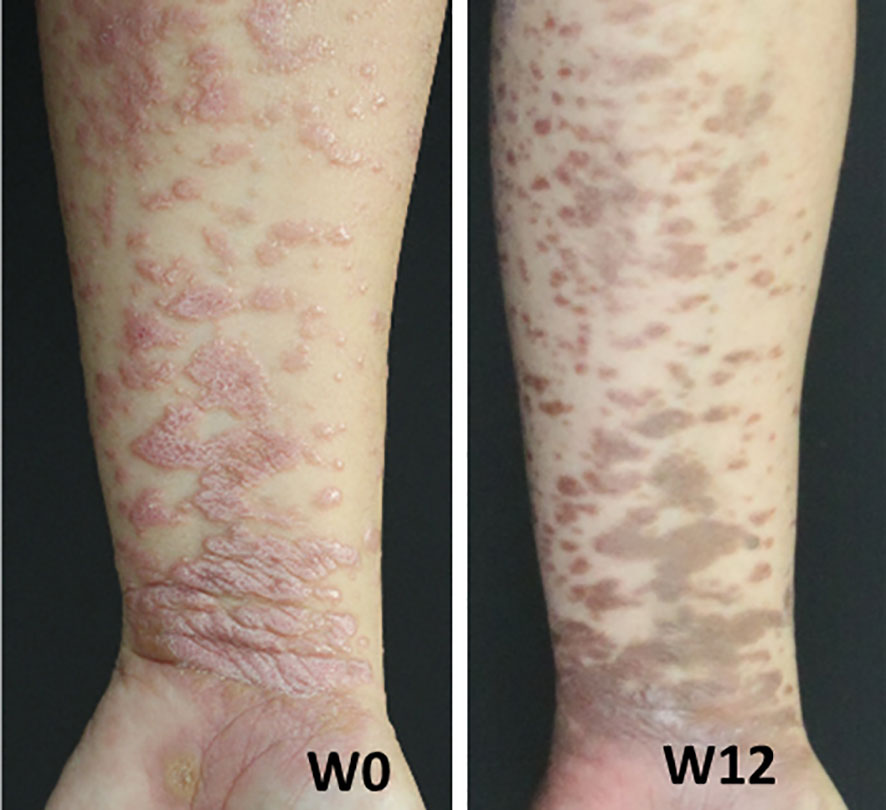
Figure 5 Lichen planus patient was treated with the anti-IL-17A monoclonal antibody Secukinumab at 300 mg administered weekly for 5 weeks starting at week 0, followed by monthly doses. Shown are images of the patient's left forearm at week 0 (W0) and week 12 (W12).
Discussion
The present study provides a comprehensive analysis of the inflammatory signature of skin lesions in three inflammatory skin disorders linked to T cell recognition of well-known autoantigens of the skin and mucous membranes. PV/PF and BP are IgG-driven autoimmune bullous skin disorders targeting the cutaneous autoantigens, Dsg3/Dsg1 and BP180, respectively. Previous studies by our group and others had identified mainly type 2 peripheral blood autoreactive T cells in both disorders (23–26). In contrast, we had identified type 1 and type 17 peripheral blood T cells reactive with Dsg3 and BP180 in patients with mucosal and cutaneous LP (15). As these studies have mainly focused on the peripheral blood, we here sought to investigate whether these peripheral signatures correspond to the signatures within the skin lesions that have remained largely unknown.
The major aim of the present study was therefore to study skin sections from a cohort of PV, BP and LP patients in order to investigate whether a similar polarized CD4+ T cellular signature could be identified in lesions of these inflammatory skin disorders. We generated TMAs allowing us to perform a comparative analysis of skin infiltrates with greater patient numbers and small inter-assay variability. Simultaneous staining of TMA containing several skin sections provides a practicable and suitable research instrument for immune profiling studies. Based on the ratio of CD4+/T-bet+ (Th1) and CD4+/GATA-3+ (Th2) cells, we clearly identified a type 1 inflammatory signature in LP skin lesions in contrast to a predominant type 2 inflammatory signature in BP and PV skin lesions.
It is well-known that LP lesions are infiltrated by large numbers of CD8+ and CD4+ T cells with a pro-inflammatory signature (4). Cytokines produced by these cells include IFN-γ, TNF-α, IL-6, IL-17, IL-22, which are not only observed in skin lesions but can also be detected in patients` sera (9, 27). While cytotoxic CD8+ T cells are presumably the main drivers of disease and target basal keratinocytes, CD4+ T cells produce the majority of proinflammatory cytokines. The spectrum of (auto)antigens which are recognized by these T cells and how they are recruited to LP skin lesions remains to be elucidated. Candidate target antigens of epidermal keratinocytes and components of the dermal-epidermal junction, such as Dsg3, BP180 and others including microbial peptides have been recently identified (19, 28). It is also possible that these cytokines play an important role in disease progression, as proinflammatory cytokines reduce wound healing, impact peripheral tolerance and enhance blister formation (10).
In contrast to LP, T cell infiltrates are much less pronounced in BP and PV skin lesions as confirmed in the present study and already strongly suggested by previous work (15, 29, 30). This is primarily linked to the distinct immunopathology associated with these diseases, as BP and PV are driven by autoantibodies whereas LP is mainly associated with effector T cells. While it is hard to identify autoreactive B cells in BP and PV skin lesions, the draining lymph nodes or the bone marrow, IgG autoantibodies can readily be detected in patients’ sera, indicating their systemic distribution (31).
Autoantibody-mediated acantholysis in PV is presumably largely independent from immune cell infiltration and can readily be achieved in vitro and in vivo in preclinical animal models (32), suggesting that inflammatory immune cells are not directly required for disease progression. In contrast, BP which is characterized by subepidermal loss of adhesion largely depends on complement fixing IgG autoantibodies which lead to an influx of innate immune cells such as neutrophils and macrophages, which secrete proteolytic enzymes eventually leading to blister formation. While BP and PV blisters display a mild to moderate inflammatory phenotype, innate inflammation is not a key driver of disease and presumably more likely a secondary inflammatory response to epidermal cell death, barrier disruption and exposure to infiltrating microbes (33). The T cell signature in BP and PV is therefore less proinflammatory than in LP, and CD8+ T cells are observed at a much lower frequency. A higher ratio of anti-inflammatory GATA-3+ Th2 cells, which are associated with wound healing, barrier homeostasis and B cell activation can also be observed in BP and PV lesions (12, 15). However, type 2 T cells can also induce proinflammatory responses, cause itch, recruit eosinophils, basophils and cause their activation (34). In our opinion, the present approach to directly identify polarized T cell responses, i.e. Th1 versus Th2 versus IL-17A producing T cells extends previous studies looking at cytokine expression in skin lesions from patients with LP, BP and PV (35, 36). In contrast to these previous studies, the present study allows to directly identify and quantitate defined T cell subsets in the skin infiltrate of these inflammatory skin disorders.
It is still a matter of debate as to whether type 2 cells play an anti- or pro-inflammatory role in BP and PV, but treatment with Dupilumab, an anti-IL-4Ra antibody, has shown positive results in a small cohort of BP patients with over 50% of patients showing complete clearance of disease (37). The T cell dichotomy between Th1 and Th2 cells observed in LP vs BP/PV might therefore have important clinical implications for targeted therapies that reduce disease pathogenesis.
In recent years, a distinct T cell population residing in human epithelia, characterized by the expression of IL-17 has received major attention, as these cells may possess pro-inflammatory function thereby promoting the pathology of several skin diseases and chronic inflammatory immune responses (22, 38). IL-17+ Th17 and Tc17 cells have been now identified as suitable therapeutic targets in psoriasis vulgaris and additional inflammatory skin disorders (Table 1). In the present study, IL-17A+ cells were found in all three skin disorders to a similar extent. While LP showed a few IL-17A+ T cells, a significant number of IL-17A+ cells were neither T cells nor granulocytes. Of note, γδ T cells, which were more common in LP than in BP or PV are potential IL-17A producers. Skin lesions from patients with BP contained to the greatest extent IL-17A-producing granulocytes. Moreover, PV skin lesions contained IL-17A+ T cells and granulocytes in addition to IL-17A+ cells of unknown origin.
Similarly to LP and BP, IL-17A+ T cells were present in skin lesions from PV patients which confirms previous findings (39). Still, their pro- or anti-inflammatory function remains to be elucidated. It is noteworthy that genetic deficiency of Dsg1, the autoantigen of PF, leads to a Th17 signature as do antibodies against Dsg1 (40). Th17 cells and related cytokines are expressed in PV/PF and BP skin lesions. Their direct impact on the immune pathogenesis, e.g. the local production of IgG autoantibodies, has not yet been demonstrated (41, 42). In contrast, the majority of IL-17+ inflammatory cells in LP skin lesions do not belong to the T cell or granulocyte compartment. This finding is novel and of potential pathophysiological importance since monoclonal antibodies against IL-17 have been shown to significantly improve LP skin lesions (15). Finally, the third cellular source of IL-17 are neutrophilic granulocytes, which are a major inflammatory cell subset in BP skin lesions. IL-17 dependent activation of IL-1ß associated inflammasome augments the innate autoinflammatory immune responses inherent to BP (43). Moreover, blockade of IL-17-induced neutrophil recruitment into BP skin leads to amelioration of skin symptoms (44).
In LP, serum levels of IL-17 were readily detectable and IL-17+ CD4+ and CD8+ T cells were observed in LP lesions (8, 17). A recent meta-analysis including more than 600 patients with oral LP (OLP) observed that increased serum levels of IL-17 are strongly associated with the clinical disease severity of LP (45). Under inflammatory conditions, IL-17 induced chemokines lead to the recruitment of pro-inflammatory effector cells in the skin and the proliferation of keratinocytes (22). Keratinocyte proliferation might further increase the pathological autoantigen pool in LP, leading to further disease progression. While the major producers of IL-17 include CD4+ Th17 cells, which express the transcription factor ROR-γt, other IL-17 immune cell populations have also been identified in inflamed skin (see Table 1). These include IL-17-producing CD8+ T cells, also known as Tc17 cells, γδ T cells, as well as IL-17+ neutrophilic granulocytes, all of which infiltrate the epidermis or the subepithelial layer. While the development of Th17 cells requires IL-23 signaling, which is produced by dendritic cells during T cell priming, it is unclear which factors trigger the production of IL-17 in other cell types (22). Several monoclonal antibodies against IL-17, as well as IL-23, have been developed and have been shown to improve inflammatory skin diseases such as psoriasis (22). Individual LP patients have already been treated with monoclonal antibodies against IL-17 and IL-23 (12, 14), which has resulted in a clinical as well as a histological improvement of disease. Shown here is a patient with extensive LP of the skin who showed an excellent therapeutic response, i.e. fast regression of skin lesions upon treatment with the IL-17A blocker Secukinumab. However, a larger cohort size and controlled setting as part of a clinical trial will be necessary to determine the efficacy of IL-17 blockade in LP and its clinical variants.
In summary, our findings support the concept that LP is a type 1 T cell-associated skin disorder with a type 17 component while PV and BP show type 2 cells with a strong autoantibody-driven pathogenesis. These findings are in line with the characterization of peripheral blood type 1/type 17 signatures in LP and a type 2 T cell response in PV. These immunological signatures may pave the way for the treatment of LP using antibodies targeting Th1-associated cytokines, as well as for PV and BP using antibodies targeting Th2-associated cytokines.
Material and methods
Human subjects
Formalin-fixed paraffin-embedded (FFPE) skin tissues from patients with LP (n=31), BP (n =19), PV (n=9) and PF (n=2) were included in the study. The cohorts were kindly provided by the Center for Dermatopathology in Freiburg (S. Hörster) and Dermatopathology (P. Kind) in Offenbach (Supplementary Table 1). The samples were processed, pseudonymized and included in the present study based on their diagnoses. The LP samples were included based on histologic criteria and the BP and PV samples were included based on histologic, direct immunofluorescence and immune serological criteria. The disease classification criteria were as follows:
1. Lichen planus: clinical suspicion (polygonal reddish papules on the skin) and histopathology showing a band-like subepidermal lymphocytic infiltrate in the upper dermis and sawtoothed rete ridges.
2. Bullous pemphigoid: clinical suspicion (tense blisters/erosions or polymorphic pruritic lesions of the skin); histopathology: subepidermal loss of adhesion and DIF: IgG and/or C3 at the dermal-epidermal junction or IIF: anti-basement membrane serum antibodies on monkey esophagus or ELISA: anti-BP180/BP230 serum IgG
3. Pemphigus vulgaris/foliaceus: clinical suspicion (flaccid blisters/erosions of the oral mucosa and/or skin); histopathology: intraepidermal loss of adhesion and DIF: IgG and/or C3 on the surface of epidermal keratinocytes or IIF: anti-epithelial serum antibodies on monkey esophagus or ELISA: anti-desmoglein 3/desmoglein 1 serum IgG.
This study was approved by the Ethics Committee of the Medical Faculty, Philipps University Marburg (165/19).
Tissue microarrays
To generate tissue microarrays (TMA), 2 mm punch biopsies were taken from FFPE tissues utilizing a Manual tissue arrayer (MTA-1) from Alphametrix Biotech, within regions of infiltrating cells. The excised tissues were combined and re-embedded in a newly arrayed recipient paraffin block, according to standard procedures of the Comprehensive Biomaterial Bank Marburg (CBBM).
Immunofluorescence staining
4 µm sections of TMAs were deparaffinized and rehydrated by two 5 min immersions in Xylene followed by 5 min in 99% ethanol and consecutive immersions in 95%-, 80%- and 70%-ethanol for 3 min each. After two 3 min washes in ddH2O heat-induced antigen retrieval was performed in 10 mM Citrate Buffer pH 6.0 for 10 min using a pressure cocker. After 45 min cool down and a following 1 min wash in ddH2O the sections were permeabilized by immersion in 0.2% Triton X-100 in PBS (PBST) for 10 min. Sections were blocked with 10% FCS in PBST for 1 h, primary antibodies were added in 2% FCS in PBST and incubated over night at 4°C in a humidified chamber. On the next day sections were washed three times with PBS for 10 min each followed by 2 h incubation with secondary antibodies in 1.5% FCS in PBST together with 4′,6-Diamidin-2-phenylindol (DAPI) in a humidified chamber at room temperature. After three consecutive washes in PBS the slides were mounted with Dako fluorescence mounting medium. The primary and secondary antibodies used as well as the dilutions can be found in Supplementary Table 2.
Image acquisition and cell counting
A Zeiss LSM 700 confocal microscope with a 20x objective was used to acquire 320 µm x 320 µm images of the immune cell infiltrate at the dermal-epidermal junction. The immunofluorescence images were analyzed using ImageJ (v 1.53c). Positively stained cells were manually counted when CD3ϵ, TCR-δ, CD4 or CD15 showed signals at the cell membrane around the DAPI+ nucleus. Since T-bet and GATA-3 are transcription factors positive cells were counted when the signal showed an overlap with DAPI. Cells were considered IL-17A positive when the signal was between the nucleus and the cell membrane.
Statistical analysis
Kruskal-Wallis test with Dunn’s correction for multiple comparison was used to test for statistical significance between groups. Level of significance is indicated by asterixis with *p ≤ 0.05, **p ≤ 0.01, ***p ≤ 0.001, and ****p ≤ 0.0001, whereas ns stands for not significant. The analysis was performed using GraphPad Prism Version 9.5.0.
Data availability statement
The raw data supporting the conclusions of this article will be made available by the authors, without undue reservation.
Ethics statement
The studies involving human participants were reviewed and approved by Ethics Committee of the Medical Faculty, Philipps University Marburg (165/19). Written informed consent for participation was not required for this study in accordance with the national legislation and the institutional requirements. Written informed consent was obtained from the individual(s) for the publication of any potentially identifiable images or data included in this article.
Author contributions
HJ: study concept and design HJ, SH, PK and TC: acquisition of samples JS: immunohistochemistry staining and analysis JS, TC and HJ: immunohistochemistry analysis TC and JS: drafting of the manuscript JS: statistical analysis CK: technical and material support MH and TW: study supervision All authors: acquisition, analysis and interpretation of data and critical revision of the manuscript for important intellectual content. All authors contributed to the article and approved the submitted version.
Funding
This study was supported by the Deutsche Forschungsgemeinschaft (FOR 2497 Pegasus; TP8 to MH). DD is supported by a Clinician Scientist Program of the Medical Faculty at the University of Marburg.
Acknowledgments
This paper is dedicated to the memory of Prof. Detlef Zillikens. Detlef was a world leader in autoimmune bullous disease research and largely contributed to much improved diagnostics and therapeutic options in pemphigus and the pemphigoids. He has encouraged many young scientists to pursue an academic career in this field some of whom have already become academic leaders. We are grateful to the patients of the study, to the medical doctors taking skin biopsies, and the technicians and the pathology unit of the Comprehensive Biobank Marburg (CBBM) for embedding the samples and creating the TMA.
Conflict of interest
MH is Coordinating PI of an ongoing phase 2 multicenter trial with guselkumab in lichen planus EutraCT 2021-000271-36 and has received an unrestricted grant from Janssen Cilag. TC and JM are investigators involved in an ongoing phase 2 multicenter trial with guselkumab in lichen planus EutraCT 2021-000271-36. MH has been Coordinating PI German study centers of a clinical phase 2 trial with secukinumab in lichen planus PRELUDE; Novartis Pharmaceuticals.
The remaining authors declare that the research was conducted in the absence of any commercial or financial relationships that could be constructed as a potential conflict of interest.
Publisher’s note
All claims expressed in this article are solely those of the authors and do not necessarily represent those of their affiliated organizations, or those of the publisher, the editors and the reviewers. Any product that may be evaluated in this article, or claim that may be made by its manufacturer, is not guaranteed or endorsed by the publisher.
Supplementary material
The Supplementary Material for this article can be found online at: https://www.frontiersin.org/articles/10.3389/fimmu.2023.1203776/full#supplementary-material
Supplementary Figure 1 | Secondary Antibody control, peritumoral skin control, positive T17 control. (A) Representative confocal images of immunostaining of peritumoral skin using only secondary antibodies anti-goat Alexa Fluor 488 (green), anti-mouse Alexa Fluor 555 (red) and anti-rabbit Alexa Fluor 647 (purple). (B) Representative confocal images of immunostaining of peritumoral skin using anti-CD3ϵ (green), anti-TCRδ (red) and anti-IL-17A (purple). (C) Representative confocal images of immunostaining of Psoriasis pustulosa using anti-CD3ϵ (green) and anti-IL-17A (purple) as a positive control for IL-17A producing T cells. Dashed lines indicate the border between epidermis (e) and dermis. Boxed areas in (B, C) are magnified in corresponding second row. Blue: 4′,6-diamidino-2-phenylindole (DAPI). Scale bars represent 40 µm.
Abbreviations
Dsg, desmoglein; BP180, bullous pemphigoid 180; HC, healthy controls; LP, lichen planus; PV, pemphigus vulgaris; BP, bullous pemphigoid; TMA, tissue microarray; Cd, cluster of differentiation; IL, Interleukin; IgG, Immunoglobulin G; Th cell, T helper cell, Tc cell, cytotoxic T cell; TCR, T cell receptor; DAPI, 4',6'-diamidino-2-phenylindole; IIF, indirect immunofluorescence; DIF, direct immunofluorescence; min, minutes; FCS, fetal calf serum; PBS, phosphate-buffered saline.
References
1. Kilpi A. Characterization of mononuclear cells of inflammatory infiltrates in oral tissues. a histochemical and immunohistochemical study of labial salivary glands in sjögren’s syndrome and of oral lesions in systemic lupus erythematosus and in lichen planus. Proc Finn Dent Soc (1988) 84 Suppl 3:1–93.
2. Matthews JB, Scully CM, Potts AJ. Oral lichen planus: an immunoperoxidase study using monoclonal antibodies to lymphocyte subsets. Br J Dermatol (1984) 111(5):587–95. doi: 10.1111/j.1365-2133.1984.tb06629.x
3. Zhou XJ, Sugerman PB, Savage NW, Walsh LJ, Seymour GJ. Intra-epithelial CD8+ T cells and basement membrane disruption in oral lichen planus. J Oral Pathol Med (2002) 31(1):23–7. doi: 10.1046/j.0904-2512.2001.10063.x
4. Sugerman PB, Savage NW, Walsh LJ, Zhao ZZ, Zhou XJ, Khan A, et al. The pathogenesis of oral lichen planus. Crit Rev Oral Biol Med (2002) 13(4):350–65. doi: 10.1177/154411130201300405
5. Le Cleach L, Chosidow O. Clinical practice. lichen planus. N Engl J Med (2012) 366(8):723–32. doi: 10.1056/NEJMcp1103641
6. Cooper SM, Dean D, Allen J, Kirtschig G, Wojnarowska F. Erosive lichen planus of the vulva: weak circulating basement membrane zone antibodies are present. Clin Exp Dermatol (2005) 30(5):551–6. doi: 10.1111/j.1365-2230.2005.01866.x
7. Gholizadeh N, Khoini Poorfar H, TaghaviZenouz A, Vatandoost M, Mehdipour M. Comparison of serum autoantibodies to desmogleins I, III in patients with oral lichen planus and healthy controls. Iran J Pathol (2015) 10(2):136–40.
8. Pouralibaba F, Babaloo Z, Pakdel F, Aghazadeh M. Serum level of interleukin 17 in patients with erosive and non erosive oral lichen planus. J Dent Res Dent Clin Dent Prospects (2013) 7(2):91–4. doi: 10.5681/joddd.2013.016
9. Lu R, Zhang J, Sun W, Du G, Zhou G. Inflammation-related cytokines in oral lichen planus: an overview. J Oral Pathol Med (2015) 44(1):1–14. doi: 10.1111/jop.12142
10. Wang H, Zhang D, Han Q, Zhao X, Zeng X, Xu Y, et al. Role of distinct CD4(+) T helper subset in pathogenesis of oral lichen planus. J Oral Pathol Med (2016) 45(6):385–93. doi: 10.1111/jop.12405
11. Alves de Medeiros AK, Speeckaert R, Desmet E, van Gele M, de Schepper S, Lambert J. JAK3 as an emerging target for topical treatment of inflammatory skin diseases. PLoS One (2016) 11(10):e0164080. doi: 10.1371/journal.pone.0164080
12. Solimani F, Pollmann R, Schmidt T, Schmidt A, Zheng X, Savai R, et al. Therapeutic targeting of Th17/Tc17 cells leads to clinical improvement of lichen planus. Front Immunol (2019) 10:1808. doi: 10.3389/fimmu.2019.01808
13. Damsky W, Wang A, Olamiju B, Peterson D, Galan A, King B. Treatment of severe lichen planus with the JAK inhibitor tofacitinib. J Allergy Clin Immunol (2020) 145(6):1708–10.e2. doi: 10.1016/j.jaci.2020.01.031
14. Ismail FF, Sinclair R. Clinical healing of erosive oral lichen planus with tildrakizumab implicates the interleukin-23/interleukin-17 pathway in the pathogenesis of lichen planus. Australas J Dermatol (2020) 61(2):e244–5. doi: 10.1111/ajd.13183
15. Schmidt T, Solimani F, Pollmann R, Stein R, Schmidt A, Stulberg I, et al. TH1/TH17 cell recognition of desmoglein 3 and bullous pemphigoid antigen 180 in patients with lichen planus. J Allergy Clin Immunol (2018) 142(2):669–72.e7. doi: 10.1016/j.jaci.2018.02.044
16. Liu Y, Wang H, Taylor M, Cook C, Martínez-Berdeja A, North JP, et al. Classification of human chronic inflammatory skin disease based on single-cell immune profiling. Sci Immunol (2022) 7(70):eabl9165. doi: 10.1126/sciimmunol.abl9165
17. Gueiros LA, Arão T, Souza T, Vieira CL, Gomez RS, Almeida OP, et al. IL17A polymorphism and elevated IL17A serum levels are associated with oral lichen planus. Oral Dis (2018) 24(3):377–83. doi: 10.1111/odi.12718
18. Żychowska M, Batycka-Baran A, Baran W. Increased serum level and high tissue immunoexpression of interleukin 17 in cutaneous lichen planus: a novel therapeutic target for recalcitrant cases? Dis Markers (2020) 2020:6521274. doi: 10.1155/2020/6521274
19. Wang K, Miao T, Lu W, He J, Cui B, Li J, et al. Analysis of oral microbial community and Th17-associated cytokines in saliva of patients with oral lichen planus. Microbiol Immunol (2015) 59(3):105–13. doi: 10.1111/1348-0421.12232
20. Takahashi H, Kouno M, Nagao K, Wada N, Hata T, Nishimoto S, et al. Desmoglein 3-specific CD4+ T cells induce pemphigus vulgaris and interface dermatitis in mice. J Clin Invest (2011) 121(9):3677–88. doi: 10.1172/JCI57379
21. Bernardini N, Skroza N, Tolino E, Mambrin A, Anzalone A, Balduzzi V, et al. IL-17 and its role in inflammatory, autoimmune, and oncological skin diseases: state of art. Int J Dermatol (2020) 59(4):406–11. doi: 10.1111/ijd.14695
22. Liu T, Li S, Ying S, Tang S, Ding Y, Li Y, et al. The IL-23/IL-17 pathway in inflammatory skin diseases: from bench to bedside. Front Immunol (2020) 11:594735. doi: 10.3389/fimmu.2020.594735
23. Veldman C, Höhne A, Dieckmann D, Schuler G, Hertl M. Type I regulatory T cells specific for desmoglein 3 are more frequently detected in healthy individuals than in patients with pemphigus vulgaris. J Immunol (2004) 172(10):6468–75. doi: 10.4049/jimmunol.172.10.6468
24. Hertl M, Eming R, Veldman C. T Cell control in autoimmune bullous skin disorders. J Clin Invest (2006) 116(5):1159–66. doi: 10.1172/JCI28547
25. Büdinger L, Borradori L, Yee C, Eming R, Ferencik S, Grosse-Wilde H, et al. Identification and characterization of autoreactive T cell responses to bullous pemphigoid antigen 2 in patients and healthy controls. J Clin Invest (1998) 102(12):2082–9. doi: 10.1172/JCI3335
26. Rizzo C, Fotino M, Zhang Y, Chow S, Spizuoco A, Sinha AA. Direct characterization of human T cells in pemphigus vulgaris reveals elevated autoantigen-specific Th2 activity in association with active disease. Clin Exp Dermatol (2005) 30(5):535–40. doi: 10.1111/j.1365-2230.2005.01836.x
27. Lauffer F, Jargosch M, Krause L, Garzorz-Stark N, Franz R, Roenneberg S, et al. Type I immune response induces keratinocyte necroptosis and is associated with interface dermatitis. J Invest Dermatol (2018) 138(8):1785–94. doi: 10.1016/j.jid.2018.02.034
28. Xie S, Ding L, Xiong Z, Zhu S. Implications of Th1 and Th17 cells in pathogenesis of oral lichen planus. J Huazhong Univ Sci Technolog Med Sci (2012) 32(3):451–7. doi: 10.1007/s11596-012-0078-7
29. Fang H, Li Q, Wang G. The role of T cells in pemphigus vulgaris and bullous pemphigoid. Autoimmun Rev (2020) 19(11):102661. doi: 10.1016/j.autrev.2020.102661
30. Hammers CM, Stanley JR. Mechanisms of disease: pemphigus and bullous pemphigoid. Annu Rev Pathol (2016) 11:175–97. doi: 10.1146/annurev-pathol-012615-044313
31. Sajda T, Sinha AA. Autoantibody signaling in pemphigus vulgaris: development of an integrated model. Front Immunol (2018) 9:692. doi: 10.3389/fimmu.2018.00692
32. Anhalt GJ, Labib RS, Voorhees JJ, Beals TF, Diaz LA. Induction of pemphigus in neonatal mice by passive transfer of IgG from patients with the disease. N Engl J Med (1982) 306(20):1189–96. doi: 10.1056/NEJM198205203062001
33. Gudjonsson JE, Kabashima K, Eyerich K. Mechanisms of skin autoimmunity: cellular and soluble immune components of the skin. J Allergy Clin Immunol (2020) 146(1):8–16. doi: 10.1016/j.jaci.2020.05.009
34. Ronchese F, Hilligan KL, Mayer JU. Dendritic cells and the skin environment. Curr Opin Immunol (2020) 64:56–62. doi: 10.1016/j.coi.2020.03.006
35. Rico MJ, Benning C, Weingart ES, Streilein RD, Hall RP. Characterization of skin cytokines in bullous pemphigoid and pemphigus vulgaris. Br J Dermatol (1999) 140(6):1079–86. doi: 10.1046/j.1365-2133.1999.02907.x
36. Khan A, Farah CS, Savage NW, Walsh LJ, Harbrow DJ, Sugerman PB. Th1 cytokines in oral lichen planus. J Oral Pathol Med (2003) 32(2):77–83. doi: 10.1034/j.1600-0714.2003.00077.x
37. Abdat R, Waldman RA, de Bedout V, Czernik A, Mcleod M, King B, et al. Dupilumab as a novel therapy for bullous pemphigoid: a multicenter case series. J Am Acad Dermatol (2020) 83(1):46–52. doi: 10.1016/j.jaad.2020.01.089
38. Miossec P, Kolls JK. Targeting IL-17 and TH17 cells in chronic inflammation. Nat Rev Drug Discovery (2012) 11(10):763–76. doi: 10.1038/nrd3794
39. Xue J, Su W, Chen Z, Ke Y, Du X, Zhou Q. Overexpression of interleukin-23 and interleukin-17 in the lesion of pemphigus vulgaris: a preliminary study. Mediators Inflammation (2014) 2014:463928. doi: 10.1155/2014/463928
40. Godsel LM, Roth-Carter QR, Koetsier JL, Tsoi LC, Huffine AL, Broussard JA, et al. Translational implications of Th17-skewed inflammation due to genetic deficiency of a cadherin stress sensor. J Clin Invest (2022) 132(3):13–15. doi: 10.1172/JCI144363
41. Arakawa M, Dainichi T, Ishii N, Hamada T, Karashima T, Nakama T, et al. Lesional Th17 cells and regulatory T cells in bullous pemphigoid. Exp Dermatol (2011) 20(12):1022–4. doi: 10.1111/j.1600-0625.2011.01378.x
42. Arakawa M, Dainichi T, Yasumoto S, Hashimoto T. Lesional Th17 cells in pemphigus vulgaris and pemphigus foliaceus. J Dermatol Sci (2009) 53(3):228–31. doi: 10.1016/j.jdermsci.2008.09.008
43. Le Jan S, Muller C, Plee J, Durlach A, Bernard P, Antonicelli F. IL-23/IL-17 axis activates IL-1β-Associated inflammasome in macrophages and generates an auto-inflammatory response in a subgroup of patients with bullous pemphigoid. Front Immunol (2019) 10:1972. doi: 10.3389/fimmu.2019.01972
44. Chakievska L, Holtsche MM, Künstner A, Goletz S, Petersen B-S, Thaci D, et al. IL-17A is functionally relevant and a potential therapeutic target in bullous pemphigoid. J Autoimmun (2019) 96:104–12. doi: 10.1016/j.jaut.2018.09.003
Keywords: lichen planus, bullous pemphigoid, pemphigus vulgaris, T cells, immunological signature, Th1/Th2, IL-17, blistering disorders
Citation: Schinner J, Cunha T, Mayer JU, Hörster S, Kind P, Didona D, Keber C, Hertl M, Worzfeld T and Juratli HA (2023) Skin-infiltrating T cells display distinct inflammatory signatures in lichen planus, bullous pemphigoid and pemphigus vulgaris. Front. Immunol. 14:1203776. doi: 10.3389/fimmu.2023.1203776
Received: 11 April 2023; Accepted: 30 May 2023;
Published: 20 June 2023.
Edited by:
Ralf J. Ludwig, University of Lübeck, GermanyCopyright © 2023 Schinner, Cunha, Mayer, Hörster, Kind, Didona, Keber, Hertl, Worzfeld and Juratli. This is an open-access article distributed under the terms of the Creative Commons Attribution License (CC BY). The use, distribution or reproduction in other forums is permitted, provided the original author(s) and the copyright owner(s) are credited and that the original publication in this journal is cited, in accordance with accepted academic practice. No use, distribution or reproduction is permitted which does not comply with these terms.
*Correspondence: Michael Hertl, bWljaGFlbC5oZXJ0bEB1bmktbWFyYnVyZy5kZQ==; Thomas Worzfeld, d29yemZlbGRAdW5pLW1hcmJ1cmcuZGU=
†These authors share first authorship