- Department of Hematology Oncology Children’s Hospital of Chongqing Medical University, National Clinical Research Center for Child Health and Disorders, Ministry of Education Key Laboratory of Child Development and Disorders, China International Science and Technology Cooperation base of Child development and Critical Disorders, Children’s Hospital of Chongqing Medical University, Chongqing Key Laboratory of Child Infection and Immunity, Children’s Hospital of Chongqing Medical University, Chongqing, China
Objective: To investigate similarities and differences in immune reconstitution after allogeneic hematopoietic stem cell transplantation (allo-HSCT) in children with Wiskott-Aldrich syndrome (WAS) and chronic granulomatous disease (CGD).
Method: We retrospectively analyzed the lymphocyte subpopulations and the serum level of various immune-related protein or peptide on Days 15, 30, 100, 180 and 360 post-transplantation in 70 children with WAS and 48 children with CGD who underwent allo-HSCT at the Transplantation Center of the Department of Hematology-Oncology, Children’s Hospital of Chongqing Medical University from January 2007 to December 2020, and we analyzed the differences in the immune reconstitution process between the two groups.
Results: ① The WAS group had higher lymphocyte subpopulation counts than the CGD group. ② Among children aged 1-3 years who underwent transplantation, the WAS group had higher lymphocyte subpopulation counts than the CGD group. ③ Further comparisons were performed between children with non-umbilical cord blood transplantation (non-UCBT) and children with umbilical cord blood transplantation (UCBT) in the WAS group. On Day 15 and 30 post-transplantation, the non-UCBT group had higher B-cell counts than the UCBT group. On the remaining time points post-transplantation, the UCBT group had higher lymphocyte subpopulation counts than the non-UCBT group. ④ Comparisons were performed between children with non-UCBT in the WAS group and in the CGD group, the lymphocyte subpopulation counts were higher in the WAS group compared to the CGD group. ⑤ On Day 100 post-transplantation, the CGD group had higher C3 levels than the WAS group. On Day 360 post-transplantation, the CGD group had higher IgA and C4 levels than the WAS group.
Conclusion: ① The rate of immunity recovery was faster in children within the WAS group compared to those children within the CGD group, which may be attributed to the difference of percentage undergoing UCBT and primary diseases. ② In the WAS group, the non-UCBT group had higher B-cell counts than the UCBT group at Day 15 and 30 post-transplantation, however, the UCBT group had higher B-cell counts than the non-UCBT group at Day 100 and 180 post-transplantation, suggesting that cord blood has strong B-cell reconstitution potentiality after transplantation.
1 Introduction
Allogeneic hematopoietic stem cell transplantation (allo-HSCT) is a treatment that transplants hematopoietic stem cells from a healthy donor into the patient and reestablishes hematopoiesis and immunity in the recipient through these stem cells with normal hematopoietic function. Currently, allo-HSCT can treat not only hematologic diseases but also certain immunodeficiency diseases and inherited metabolic disorders. The effectiveness of transplantation depends largely on the hematopoietic and immune reconstitution ability of the donor’s hematopoietic stem cells in the recipient, suggesting that post-transplantation hematopoietic and immune reconstitution is fundamental to the success of allo-HSCT. Delayed immune reconstitution may lead to an increased risk of infection-related death, which is a significant barrier to successful recovery from allo-HSCT (1).
Wiskott-Aldrich syndrome (WAS) and chronic granulomatous disease (CGD) are two different types of primary immunodeficiency diseases (PIDs). WAS, a syndromic combined immunodeficiency disease marked by an impaired lymphocyte lineage, is an X-linked invisible genetic disorder that usually affects males and is carried by females. WAS is prompted by mutations in WAS genes encoding the WAS protein (WASp), in which WASp is expressed only in the hematopoietic system and plays an important role in hematopoietic cell differentiation and migration, cell signaling, immune synapse formation, and lymphocyte apoptosis. As many as 300 mutations in WAS genes are known, resulting in varying degrees of defective WASp, and this can contribute to a variety of abnormal immune cell functions and lead to a variety of clinical phenotypes (2, 3). WAS features an increased incidence of congenital thrombocytopenia, eczema, recurrent infections, autoimmune diseases, and malignancies (4). Without radical treatment, most patients suffer from recurrent infections and long-term immunodeficiency, which affects the quality of life and life expectancy. CGD, an X-linked or autosomal recessive sickness, was brought on by mutations in the gene encoding a subunit of the NADPH oxidase complex, resulting in an inherited defect in phagocytosis (5). Patients with CGD are prone to recurrent bacterial and fungal infections, excessive inflammatory responses, and immune dysregulation due to defective oxygen radical production by phagocytes, which cannot effectively clear infections.
Currently, allo-HSCT offers the potential to cure PIDs such as WAS and CGD in the long term, along with the prophylactic use of antibacterial and antifungal drugs and improved symptomatic control of infections, autoimmune diseases and inflammatory complications. Since the first successful allo-HSCT was reported in 1968 for the treatment of children with WAS (6), allo-HSCT has gradually developed into a major treatment tool for patients with PIDs (7, 8). The level of immune reconstitution after transplantation is an important factor affecting the prognosis, and nonspecific immune reconstitution is mostly rapid within weeks, while specific immune reconstitution takes 1 year or longer (9, 10), during which time children are highly susceptible to life-threatening serious infections such as bacterial, fungal, and viral infections. It can be seen that robust and timely immune reconstitution is essential for immune protection against opportunistic infections. At present, there are no studies on the differences in immune recovery after transplantation in children with different immunodeficiency diseases, and there is limited published information on immune reconstitution after allo-HSCT in patients with WAS and CGD. Therefore, our study summarized and analyzed the immune reconstitution characteristics of 70 children with WAS and 48 children with CGD within 1 year after undergoing allo-HSCT and summarized transplant-related information in both groups to explore the differences in immune reconstitution in children with both diseases.
2 Methods
2.1 Cases
A total of 118 children with two PIDs who underwent allo-HSCT at the Transplantation Center of the Department of Hematology-Oncology, Children’s Hospital of Chongqing Medical University from January 2007 to December 2020 were included in the study. Inclusion criteria: (1) having a documented immune reconstitution process on Day 100 posttransplantation or after Day 100 posttransplantation; (2) receiving nondepleted T cells as a conditioning regimen; and (3) undergoing hematopoietic reconstitution. Exclusion criteria: (1) children with failed transplants, failure to achieve hematopoietic reconstitution, or death within 3 months posttransplantation and (2) no record of an immune reconstitution process on or after Day 100 posttransplantation. In the WAS group, the median time to engraftment of neutrophils was Day 11 (5–36 days) posttransplantation, and the median time to engraftment of platelets was Day 20 (5–83 days) posttransplantation. In the CGD group, the median time to engraftment of neutrophils was Day 11 (9–17 days) posttransplantation, and the median time to engraftment of platelets was Day 11 (7–35 days) posttransplantation.
2.2 Lymphocyte classification and immunoglobulin monitoring
Changes in lymphocyte subsets, including CD3+ T cells, CD4+ T cells, CD8+ T cells, NK cells (CD56+) and B cells (CD19+), were monitored on Days 15, 30, 100, 180 and 360 after allo-HSCT using flow cytometry. Absolute counts of lymphocyte subpopulations in our center are referenced to previous study (11).
Immunofluorescence detection of immunoglobulin and peptide levels: The levels of various types of immunoglobulins and peptides, including IgM, IgA, IgG, C3, and C4, were detected on Days 15, 30, 100, 180, and 360 after allo-HSCT using an immunoturbidimetric assay.
2.3 Statistical processing
SPSS 26.0 was used for statistical processing. The Mann-Whitney U test was used for continuous variables, the chi-square test or Fisher exact test was used for categorical variables, and Student’s t test was used for numerical variables in the comparison of the clinical characteristics of the children. When analyzing the absolute counts of lymphocyte subpopulations in children with both diseases at different time points, data that did not satisfy the normal distribution were analyzed using the Mann-Whitney nonparametric test, and statistical descriptions were performed using the median and interquartile range (Q25 to Q75). Plots were processed using GraphPad Prism 9. P<0.05 (two-sided) was considered a statistically significant difference.
3 Results
3.1 Basic information and transplant characteristics of children
The basic characteristics of the 118 children are shown in Table 1. Among them, children with WAS and CGD were statistically different in terms of the age at transplant, nucleated cell infusion volume, CD34+ cell infusion volume, graft type, and ATG use. The transplantation age of children in the WAS group was apparently younger compared with that in the CGD group; the nucleated cell infusion volume and CD34+ cell infusion volume in the CGD group had been greater compared with that in the WAS group; the proportion of UCBT in the WAS group was apparently higher compared with that in the CGD group; and the proportion of patients receiving ATG pretreatment in the CGD group used to be considerably greater compared with that in the WAS group. There were also differences in infection between the WAS and CGD groups before transplantation: the number of CMV infections in the WAS group was higher than that in the CGD group, and the numbers of EBV and fungal infections in the CGD group were higher than those in the WAS group.The median time to last immunoglobulin infusion after transplantation was 238.50 days (179.75days-329.75days) for children in the WAS group compared to 309.00 days (255.25days-417.75days) for children in the CGD group. The time for last immunoglobulin infusion was significantly less in the WAS group than that in the CGD group after transplantation. All children in the WAS and CGD groups were male, 1 child in the WAS group was transplanted with CB+BM, and the rest were transplanted with a single graft. As of December 31, 2022, one child in the WAS group died of respiratory and circulatory failure due to severe pulmonary infection in the 11th month after allo-HSCT (2013/7/2), and the remaining 117 children survived. The median follow-up time was 78.1 months (24.4-159.3 months) for the surviving children in the WAS group (69 children) and 52.3 months (19.5-128.0 months) for the surviving children in the CGD group (48 children).
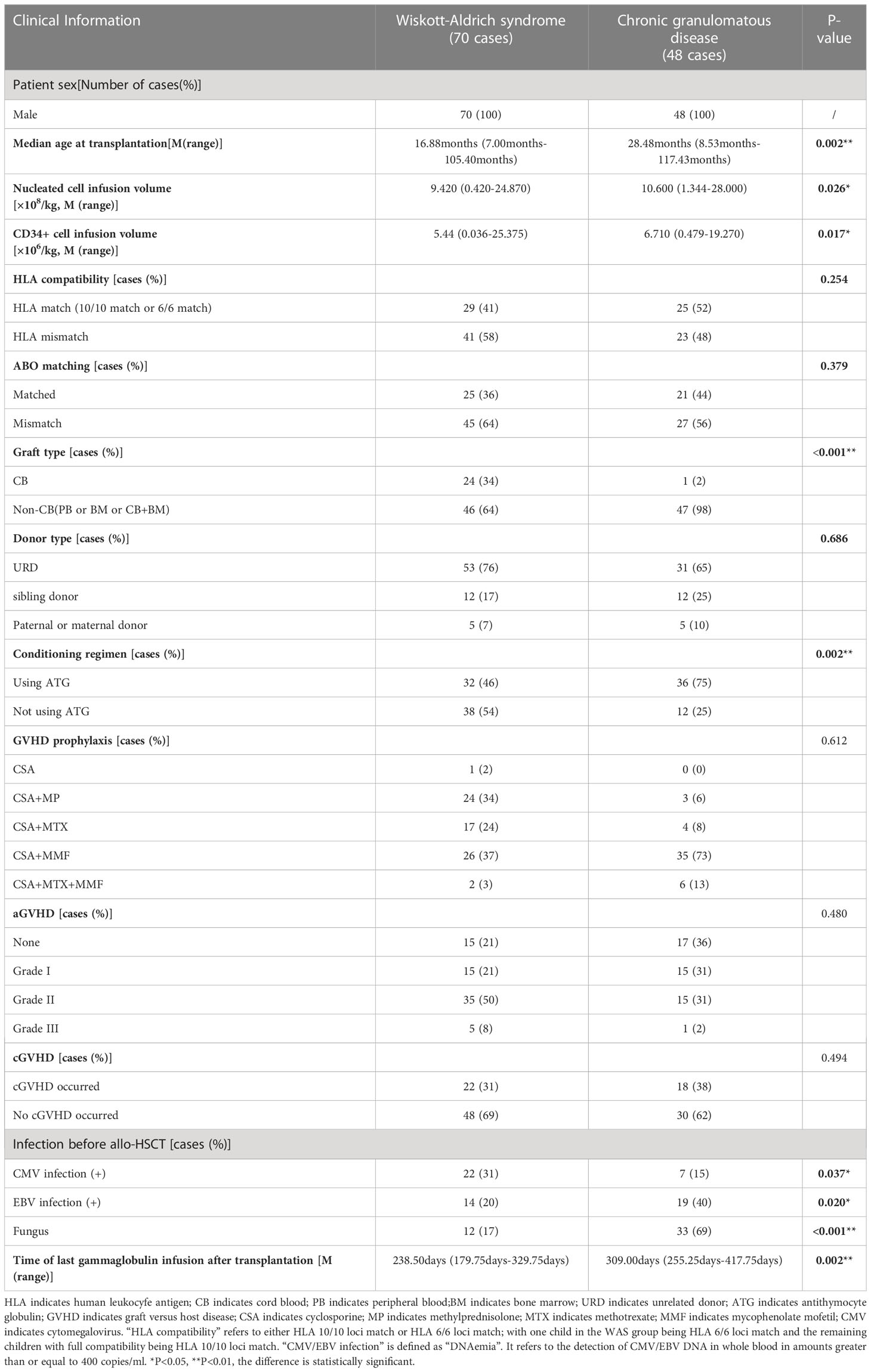
Table 1 Basic characteristics and transplantion characteristics of 118 children in the WAS and CGD groups.
3.2 Reconstruction of lymphocyte subsets in the WAS and CGD groups
On Day 15 after allo-HSCT, the WAS group had significantly higher NK cell counts than the CGD group [233.47 (59.69-395.78) ×106cells/L vs. 129.25 (28.41-217.53) ×106cells/L, P=0.022]. On Days 30, 100 and 180 after allo-HSCT, the WAS group had notably higher CD4+ T-cell counts than the CGD group [305.47 (117.81-427.50) ×106cells/L vs. 134.77 (62.74-244.97) ×106cells/L, P<0.001], [312.80 (127.00-546.95) ×106cells/L vs. 148.64 (111.06-282.32) ×106cells/L, P=0.005], [397.93 (228.15-631.74) ×106cells/L vs. 179.85(120.61-366.11)×106cells/L, P=0.004]. On Days 100 and 180 after allo-HSCT, the WAS group had considerably higher B-cell counts than the CGD group [32.28 (12.24-231.74) ×106cells/L vs. 18.43 (8.39-55.82) ×106cells/L, P=0.027], [132.05 (43.04-418.20) ×106cells/L vs. 37.32 (14.96-132.36) ×106cells/L, P=0.002] shown in Table 2 and Figures 1A–E.
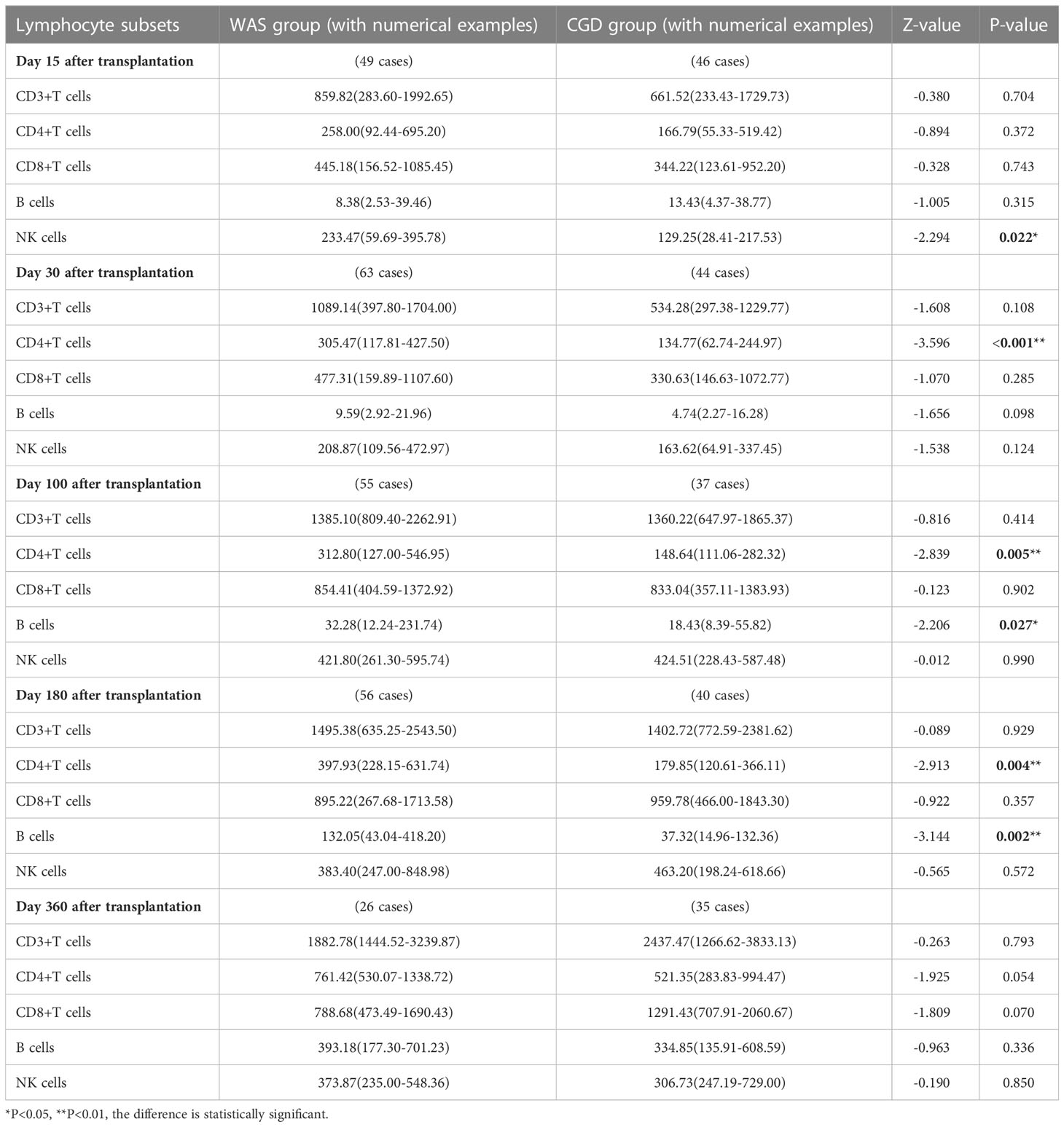
Table 2 Changes in lymphocyte subpopulation counts after allo-HSCT in WAS and CGD groups [×106cells/L, P50 (P25, P75)].
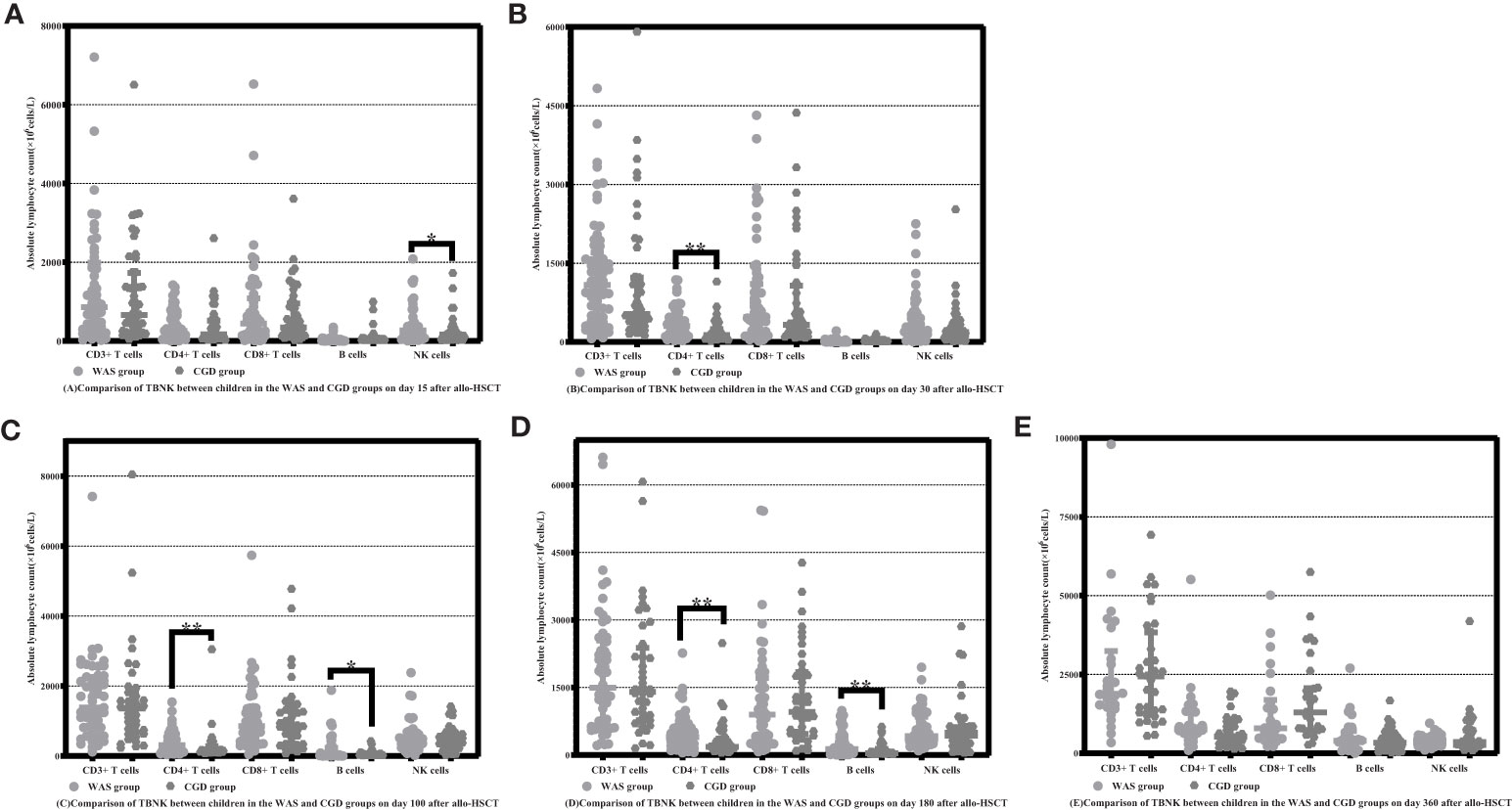
Figure 1 Comparison of changes in the number of lymphocyte subpopulations in children in the WAS and CGD groups at different time points after allo-HSCT. The time points included day 15, day 30, day 100, day 180, and day 360 after transplantation in the WAS and CGD groups, and the lymphocyte categories included CD3+ T cells, CD4+ T cells, CD8+ T cells, B cells, and NK cells. (A–E) Comparison of lymphocyte subsets count in WAS group and CGD group on Days 15, 30, 100, 180 and 360 after allo-HSCT. *P<0.05, **P<0.01. Horizontal bars represent median with interquartile range (A–E).
3.3 The reconstitution of lymphocyte subsets and analysis of graft types in children aged 1-3 years in the WAS and CGD groups
We selected the age group with the largest number of transplants in WAS and CGD groups, i.e., children aged 1-3 years who underwent allo-HSCT, and we analyzed the reconstruction of lymphocyte subpopulations in these children at different time points after allo-HSCT. The results are as follows.
On Day 15 posttransplantation, NK cell counts in the WAS group were considerably higher than those in the CGD group among children aged 1-3 years who underwent transplants [265.60 (61.98-375.97) ×106cells/L vs. 113.46 (26.85-223.29) ×106cells/L, P=0.050]. On Days 30 and 180 posttransplantation, the WAS group had notably higher CD4+ T-cell counts than the CGD group among children aged 1-3 years who underwent transplants [325.80 (143.29-465.00) ×106cells/L vs. 133.93 (63.94-241.75) ×106cells/L, P=0.011], [308.20 (201.21-526.40) ×106cells/L vs. 174.39 (129.96-282.96) ×106cells/L, P=0.025]. On Day 180 posttransplantation, B-cell counts in the WAS group were consistently higher than those in the CGD group among children aged 1-3 years who underwent transplants [131.20 (95.70-266.64) ×106cells/L vs. 28.02 (13.10-104.23) ×106cells/L, P=0.003]. On Day 360 posttransplantation, the CGD group had notably higher CD8+ T-cell counts than the WAS group among children aged 1-3 years who underwent transplants [1278.28 (674.62-3540.85)×106cells/L vs. 578.54 (454.63-803.18)×106cells/L, P=0.021].
In addition, the study compared the graft types of children in the WAS and CGD groups who underwent allo-HSCT at the age of 1-3 years. The results showed that there were no significant differences in graft type between children in the WAS and CGD groups who underwent allo-HSCT at the age of 1-3 years (P=0.100) shown in Tables 3, 4, Figures 2A–E.
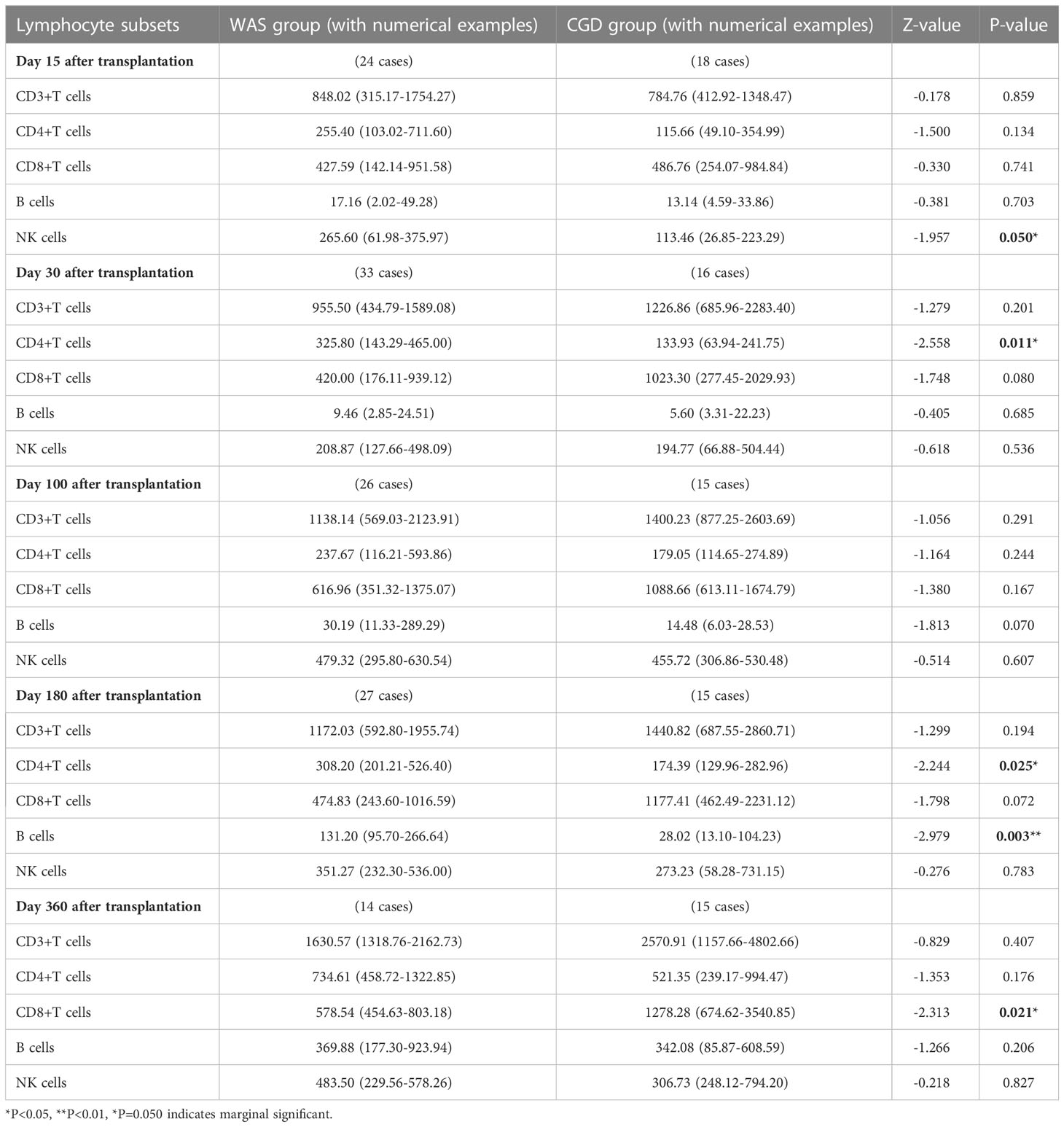
Table 3 Changes in lymphocyte subsets after allo-HSCT in children aged 1-3 years in the WAS and CGD groups[×106cells/L, P50 (P25, P75)].

Table 4 Types of grafts in children in the WAS and CGD groups who received allo-HSCT at the age of 1-3 years.
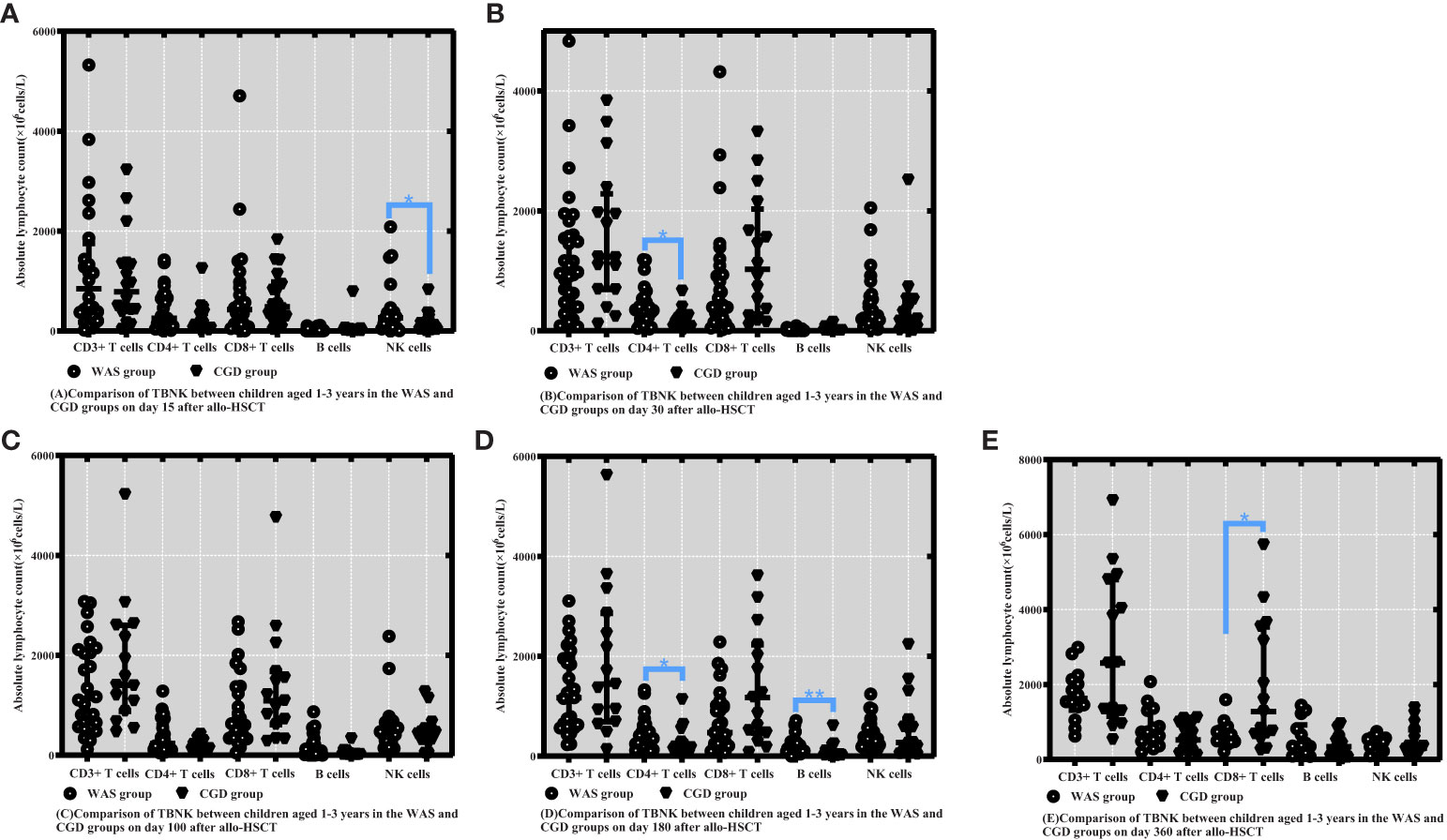
Figure 2 Comparison of changes in lymphocyte subpopulation values at different time points after allo-HSCT in children aged 1-3 years in the WAS and CGD groups. The time points included day 15, day 30, day 100, day 180, and day 360 after transplantation, and the lymphocyte categories included CD3+ T cells, CD4+ T cells, CD8+ T cells, B cells, and NK cells. (A–E) Comparison of lymphocyte subsets count between children aged 1-3 years in the WAS and CGD groups on Days 15, 30, 100, 180 and 360 after allo-HSCT. *P<0.05, **P<0.01. Horizontal bars represent median with interquartile range (A-E).
3.4 Reconstruction of lymphocyte subsets after umbilical cord blood transplantation and non-UCBT in the WAS group
On Days 15 and 30 posttransplantation, children who underwent non-UCBT had significantly higher B-cell counts than children who underwent UCBT in the WAS group [15.55 (5.69-60.66) ×106cells/L vs. 0.48 (0.00-1.41) ×106cells/L, P<0.001], [14.63 (8.48-25.46) ×106cells/L vs. 1.24 (0.20-2.23) ×106cells/L, P<0.001]. On Days 100 and 180 posttransplantation, children who underwent UCBT had apparently higher B-cell counts than children who underwent non-UCBT in the WAS group [250.80 (125.50-552.30) ×106cells/L vs. 24.99 (11.18-89.16) ×106cells/L, P=0.001], [445.600 (131.20-712.71) ×106cells/L vs. 96.60 (39.24-191.95) ×106cells/L, P<0.001]. On Day 30 posttransplantation, children who underwent UCBT had notably higher CD3+ T-cell counts than children who underwent non-UCBT in the WAS group [1427.40 (961.69-2080.18) ×106cells/L vs. 914.92 (282.17-1614.18) ×106cells/L, P=0.026]. On Days 30, 100 and 180 posttransplantation, children who underwent UCBT had obviously higher CD4+ T-cell counts than children who underwent non-UCBT in the WAS group [507.04 (218.31-705.45) ×106cells/L vs. 244.39 (97.21-401.69) ×106cells/L, P=0.003], [466.82 (289.30-734.57) ×106cells/L vs. 260.25 (118.72-479.53) ×106cells/L, P=0.038], [571.29 (384.52-832.00) ×106cells/L vs. 308.20 (168.48-488.70) ×106cells/L, P=0.002]. On Day 360 posttransplantation, children who underwent UCBT had markedly higher NK cell counts than children who underwent non-UCBT in the WAS group [668.62 (515.46-901.64) ×106cells/L vs. 346.13 (229.56-488.45) ×106cells/L, P=0.007] shown in Table 5 and Figures 3A–E.
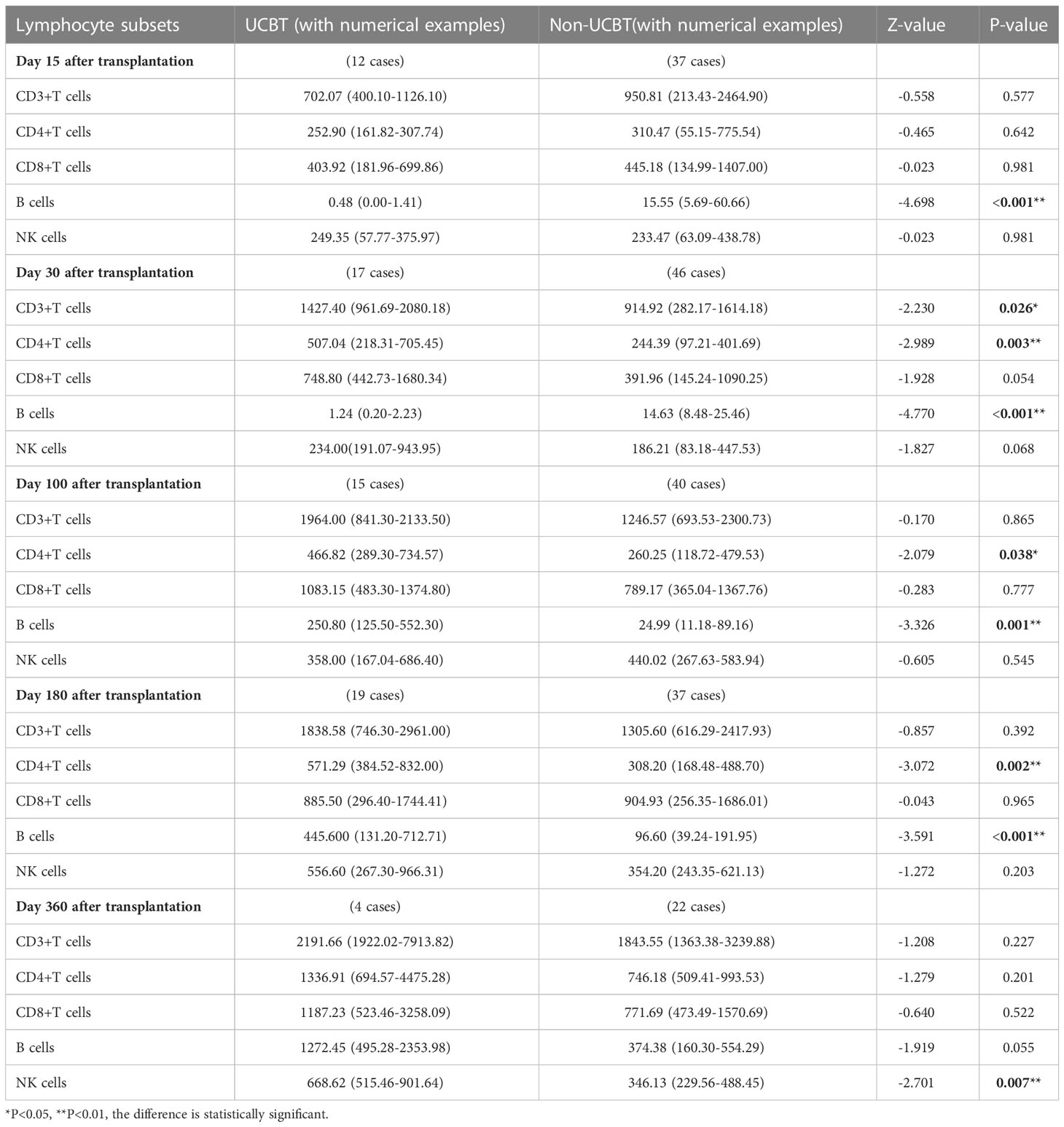
Table 5 Changes in lymphocyte subpopulation counts after UCBT and non-UCBT in children in the WAS group[×106cells/L, P50 (P25, P75)].
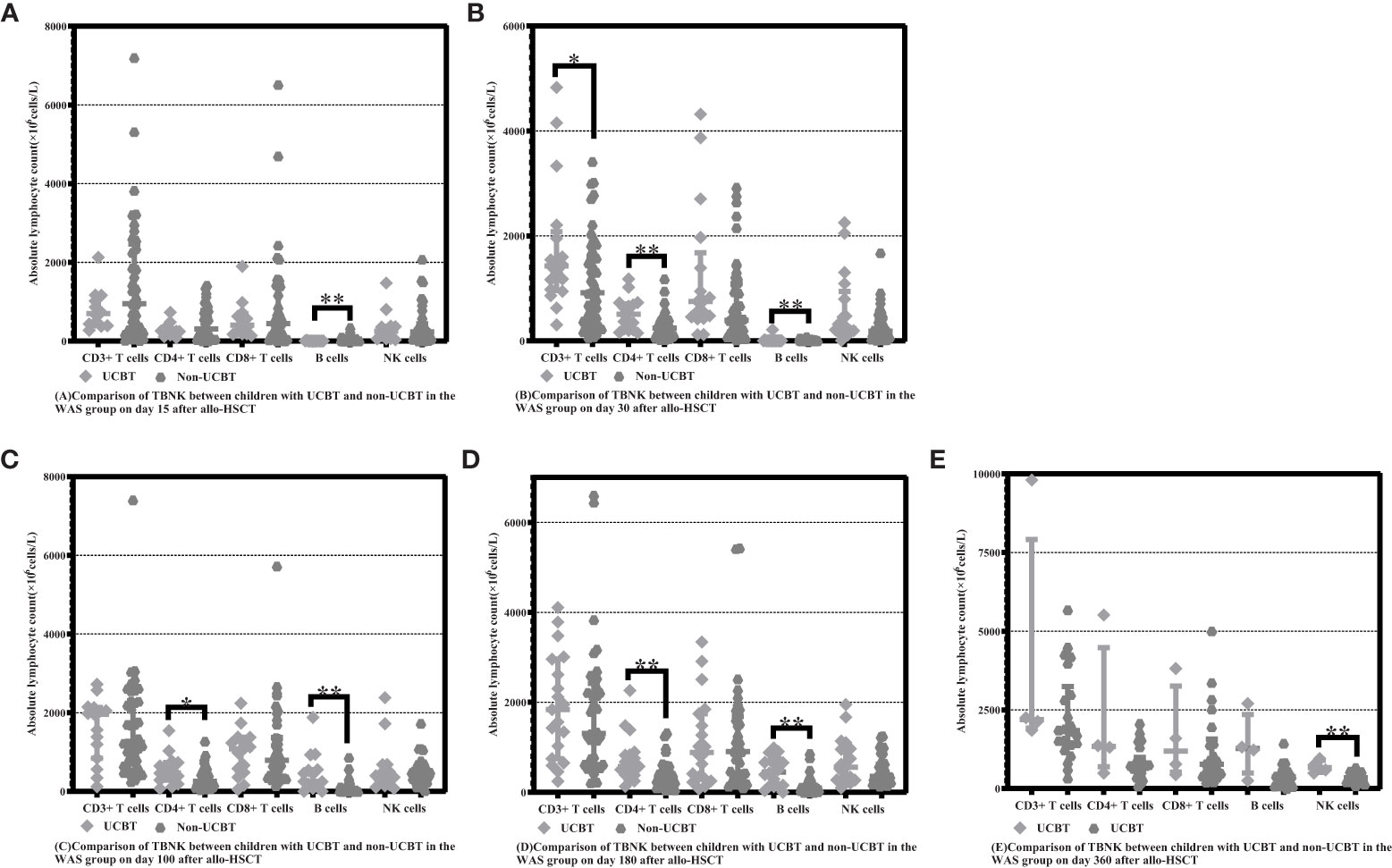
Figure 3 Comparison of changes in lymphocyte subpopulation values at different time points after allo-HSCT in children with UCBT and non-UCBT in the WAS group. The time points included day 15, day 30, day 100, day 180, and day 360 after transplantation, and the lymphocyte categories included CD3+ T cells, CD4+ T cells, CD8+ T cells, B cells, and NK cells. (A–E) Comparison of lymphocyte subsets count between children with UCBT and non- UCBT in WAS group on Days 15, 30, 100, 180 and 360 after allo-HSCT. *P<0.05, **P<0.01. Horizontal bars represent median with interquartile range (A–E).
3.5 Reconstruction of lymphocyte subsets after non-UCBT in the WAS and CGD groups
On Day 15 posttransplantation, NK cell counts were probably higher in the non-cord-blood-transplanted children with WAS compared to the non-cord-blood-transplanted children with CGD [233.47 (63.09-438.78) ×106cells/L vs. 129.69 (28.02-222.26) ×106cells/L, P=0.043]. On Days 30 and 100 posttransplantation, CD4+ T-cell counts were significantly higher in the non-cord-blood-transplanted children with WAS compared to the non-cord-blood-transplanted children with CGD [244.39 (97.21-401.69) ×106cells/L vs. 139.22 (63.26-253.12) ×106cells/L, P=0.024], [260.25 (118.72-479.53) ×106cells/L vs. 148.64 (111.06-282.32) ×106cells/L, P=0.049]. On Day 30 posttransplantation, B-cell counts were notably higher in the non-cord-blood-transplanted children with WAS compared to the non-cord-blood-transplanted children with CGD [14.63 (8.48-25.46) ×106cells/L vs. 4.51 (2.21-11.52) ×106cells/L, P<0.001] shown in Table 6 and Figures 4A–E.
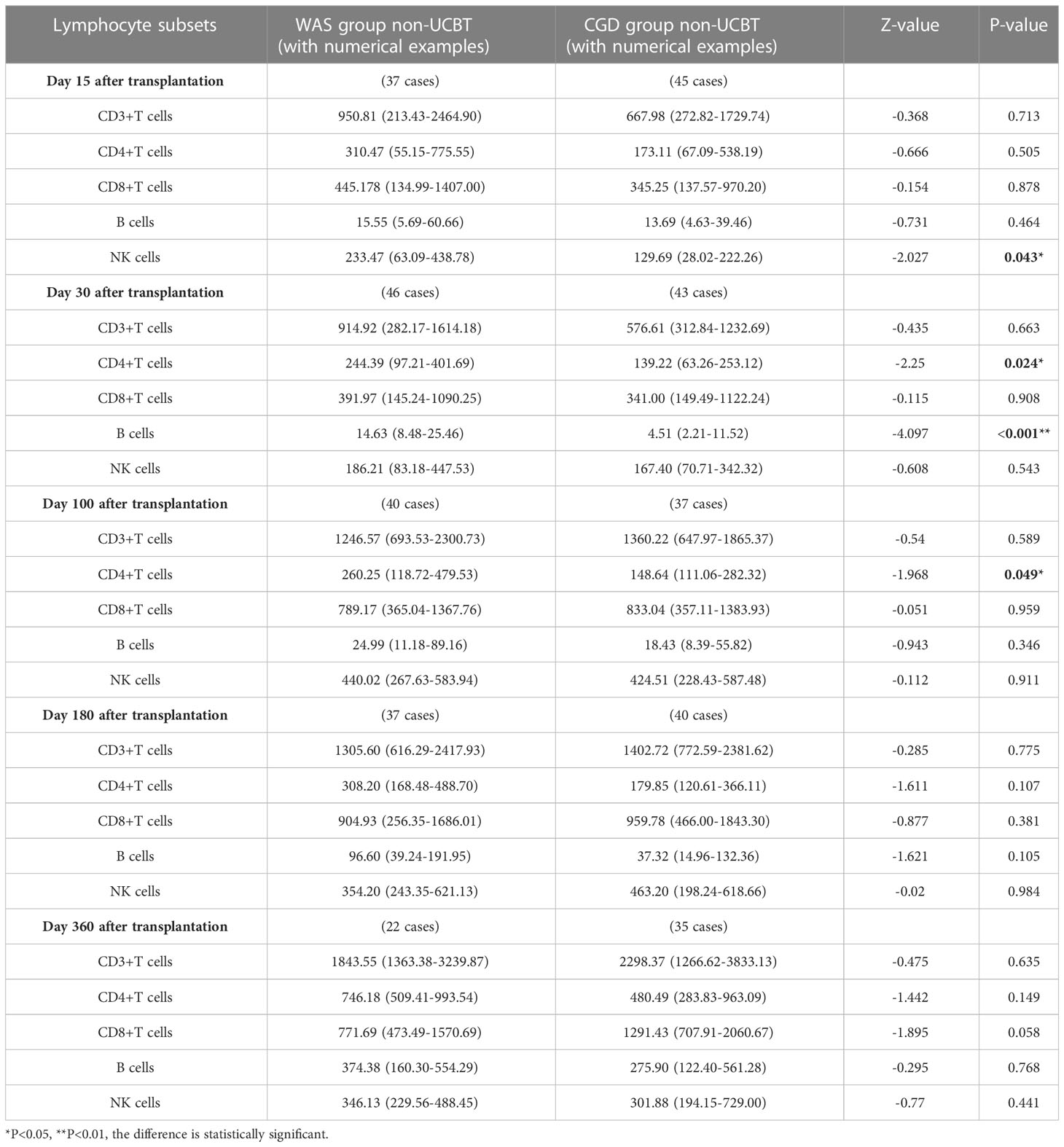
Table 6 Changes of lymphocyte subsets after non-UCBT in WAS and CGD groups[×106cells/L, P50 (P25, P75)].
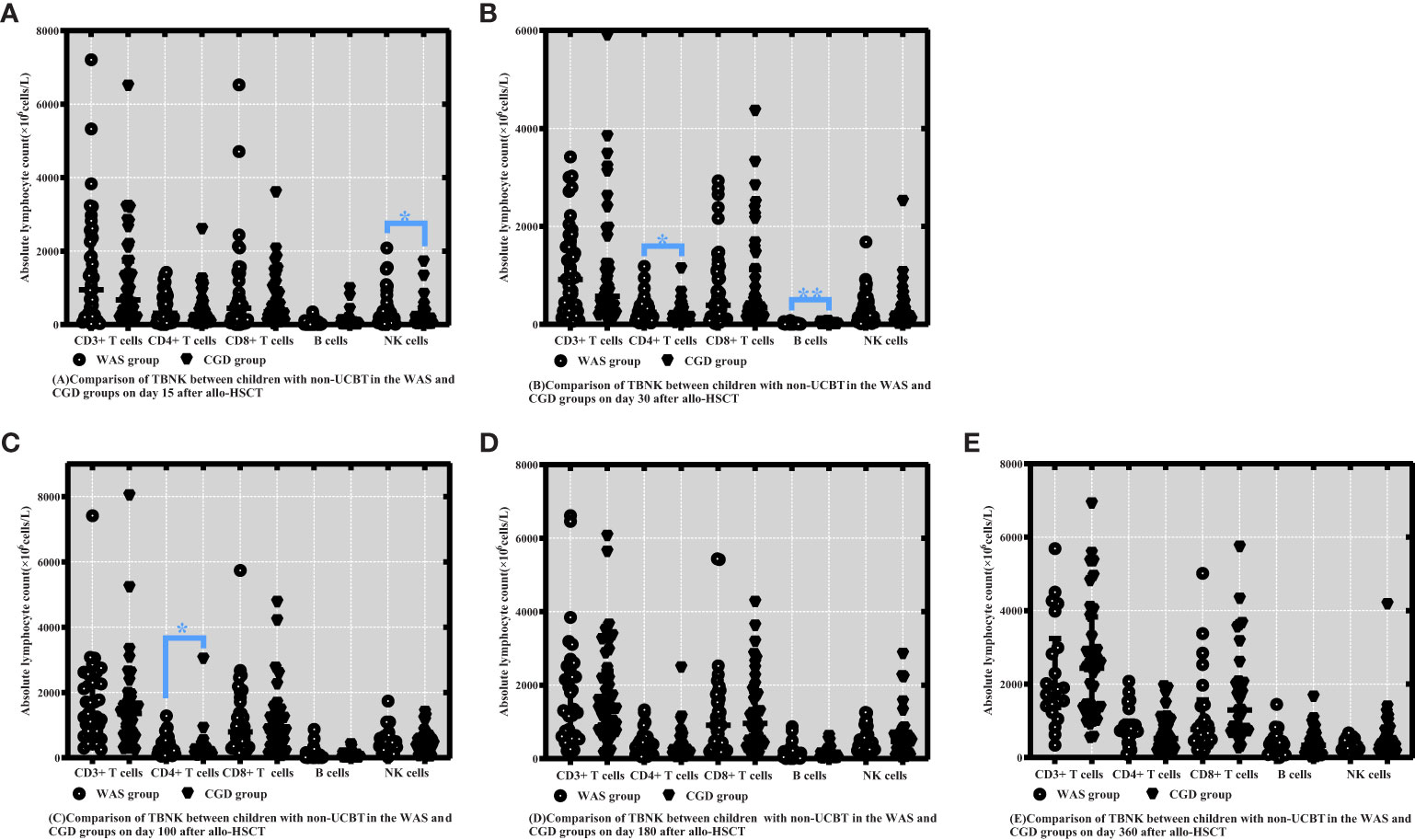
Figure 4 Comparison of changes in lymphocyte subpopulation values at different time points after allo-HSCT in children with non-UCBT in the WAS and CGD groups. The time points included day 15, day 30, day 100, day 180, and day 360 after transplantation, and the lymphocyte categories included CD3+ T cells, CD4+ T cells, CD8+ T cells, B cells, and NK cells. (A–E) Comparison of lymphocyte subsets count between children with non-UCBT in WAS and CGD groups on Days 15, 30, 100, 180 and 360 after allo-HSCT. *P<0.05, **P<0.01. Horizontal bars represent median with interquartile range (A-E).
3.6 Immunoglobulin reconstitution in the WAS and CGD groups
On Day 100 after allo-HSCT, the CGD group had higher C3 levels than the WAS group [0.9000 (0.8075-1.0750) ×g/L vs. 0.7650 (0.6900-0.9825) ×g/L, P=0.002]. On Day 360 after allo-HSCT, the CGD group had higher IgA and C4 levels than the WAS group [0.4930 (0.3595-0.76975) ×g/L vs. 0.4030 (0.2740-0.5320) ×g/L, P=0.033], [0.2000 (0.1475-0.2200) ×g/L vs. 0.150 (0.130-0.180) ×g/L, P=0.004]. There was no statistically significant change in immunoglobulin levels between the both groups on Days 15, 30 and 180 after allo-HSCT (P > 0.05). Among the patients who underwent allo-HSCT in our center, due to the influence of regular infusions of gamma globulin (IVIG), the IgG levels of the WAS and CGD groups of patients at various time points posttransplantation are not of comparative value shown in Table 7 and Figures 5A–F.
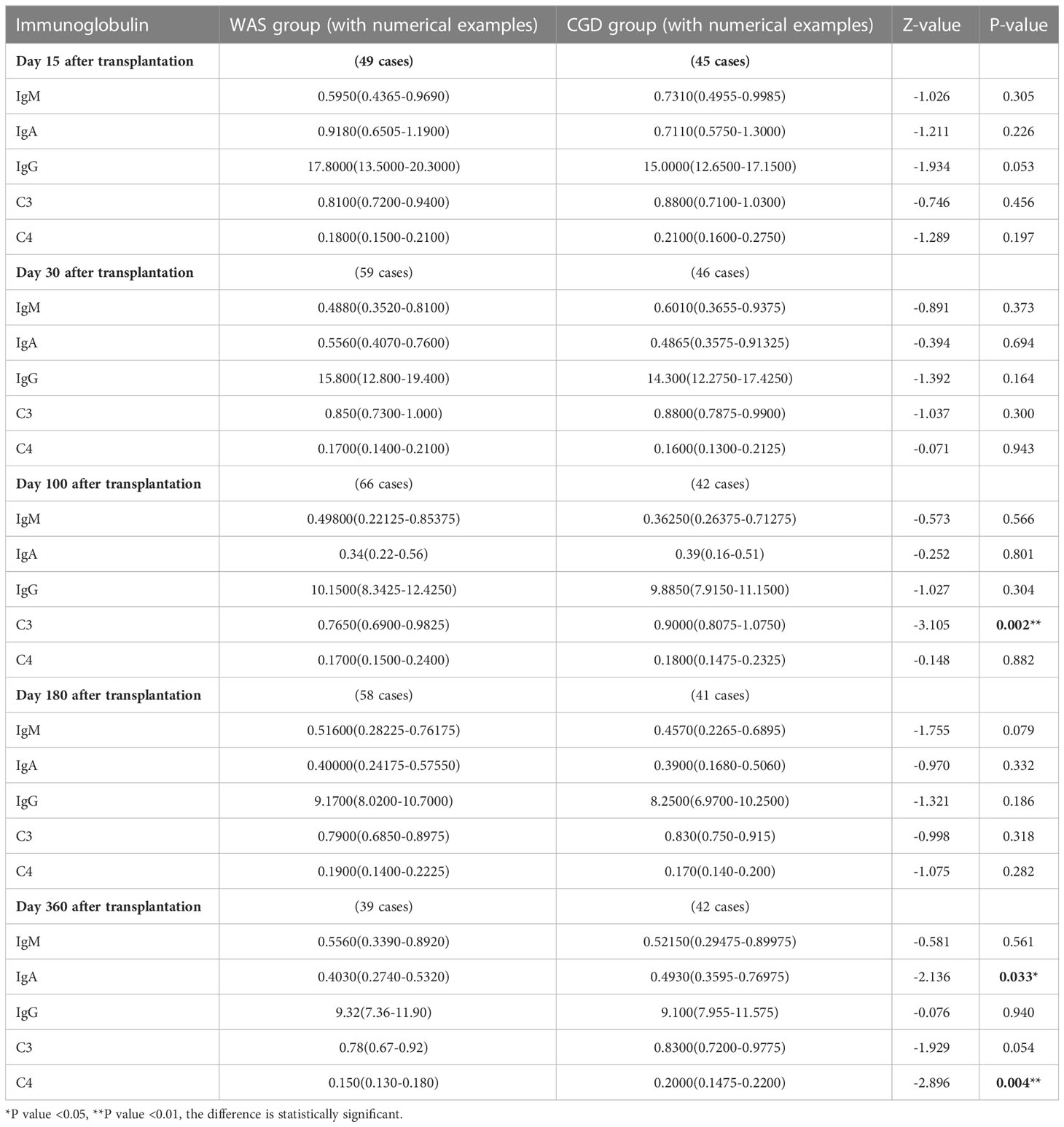
Table 7 Changes in immunoglobulin levels after allo-HSCT in the WAS and CGD groups [g/L, P50 (P25, P75)].
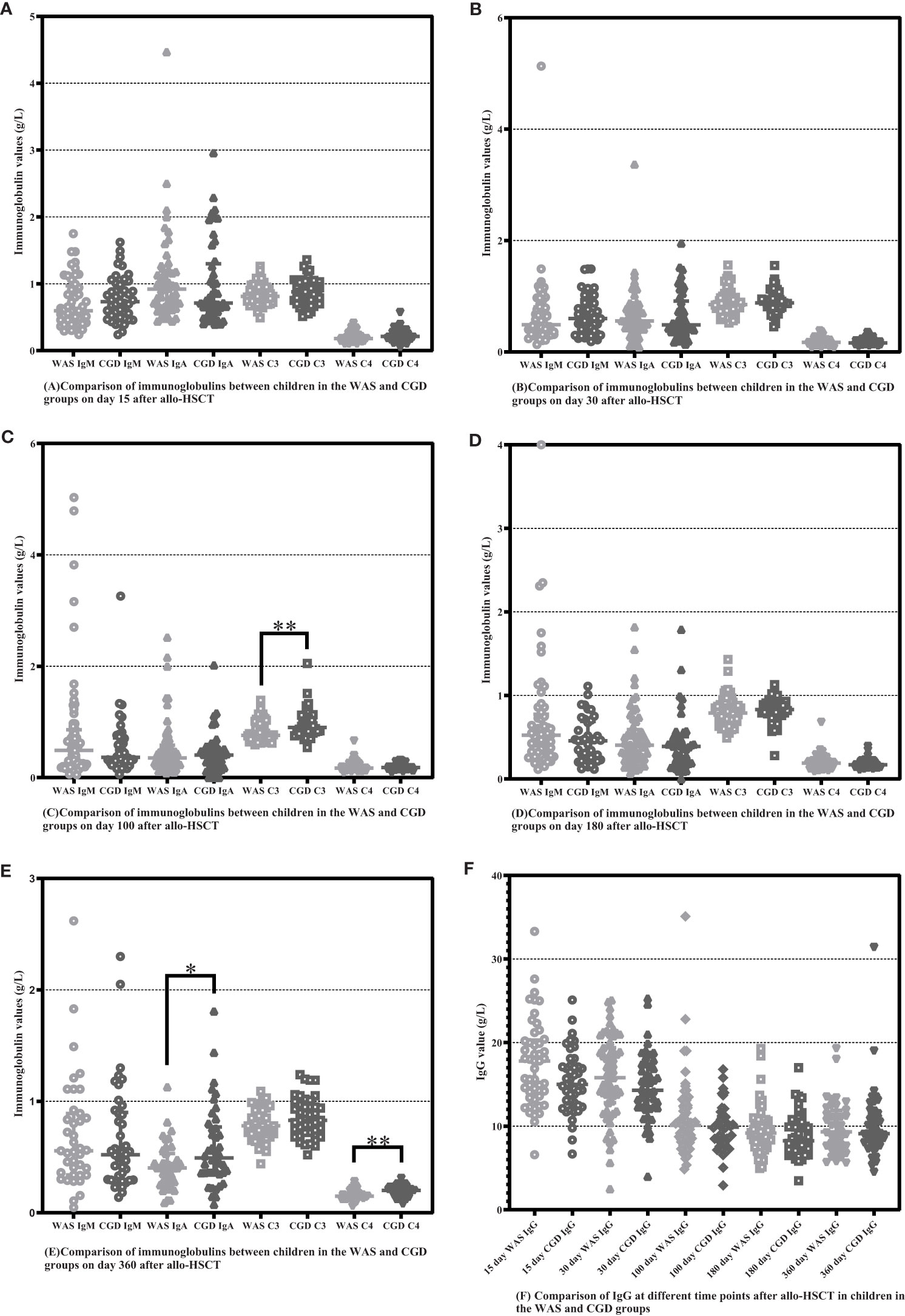
Figure 5 Comparison of immunoglobulins between WAS and CGD groups at different time points after allo-HSCT. The time points included day 15, day 30, day 100, day 180, and day 360 after transplantation, and various immunoglobulins include IgM, IgA, IgG, C3 and C4. (A–F) Comparison of immunoglobulins between WAS and CGD groups on Days 15, 30, 100, 180 and 360 after allo-HSCT. *P < 0.05, **P < 0.01. Horizontal bars represent median with interquartile range (A-F).
3.7 Infection and GVHD after allo-HSCT in the WAS and CGD groups
In this paper, children in the WAS and CGD groups were divided into different age groups (<1 year, 1-3 years, 3-5 years, and >5 years), and we compared the numbers of posttransplantation infections and occurrences of aGVHD in children in different age groups (shown in Table 8). In children younger than 1 year of age, the numbers of posttransplantation EBV and fungal infections were higher in the CGD group than in the WAS group. In children 1-3 years of age, the numbers of posttransplantation EBV and CMV infections were higher in the CGD group than in the WAS group. In children older than 5 years, the number of posttransplantation fungal infections was higher in the CGD group than in the WAS group. When comparing the incidence of aGVHD in children of different ages in the two groups, the results showed no statistical difference in the incidence of aGVHD in children of all ages in the WAS and CGD groups (P > 0.05). In Table 1, we also found that the incidence of cGVHD after transplantation in children with WAS and CGD was also not statistically different.
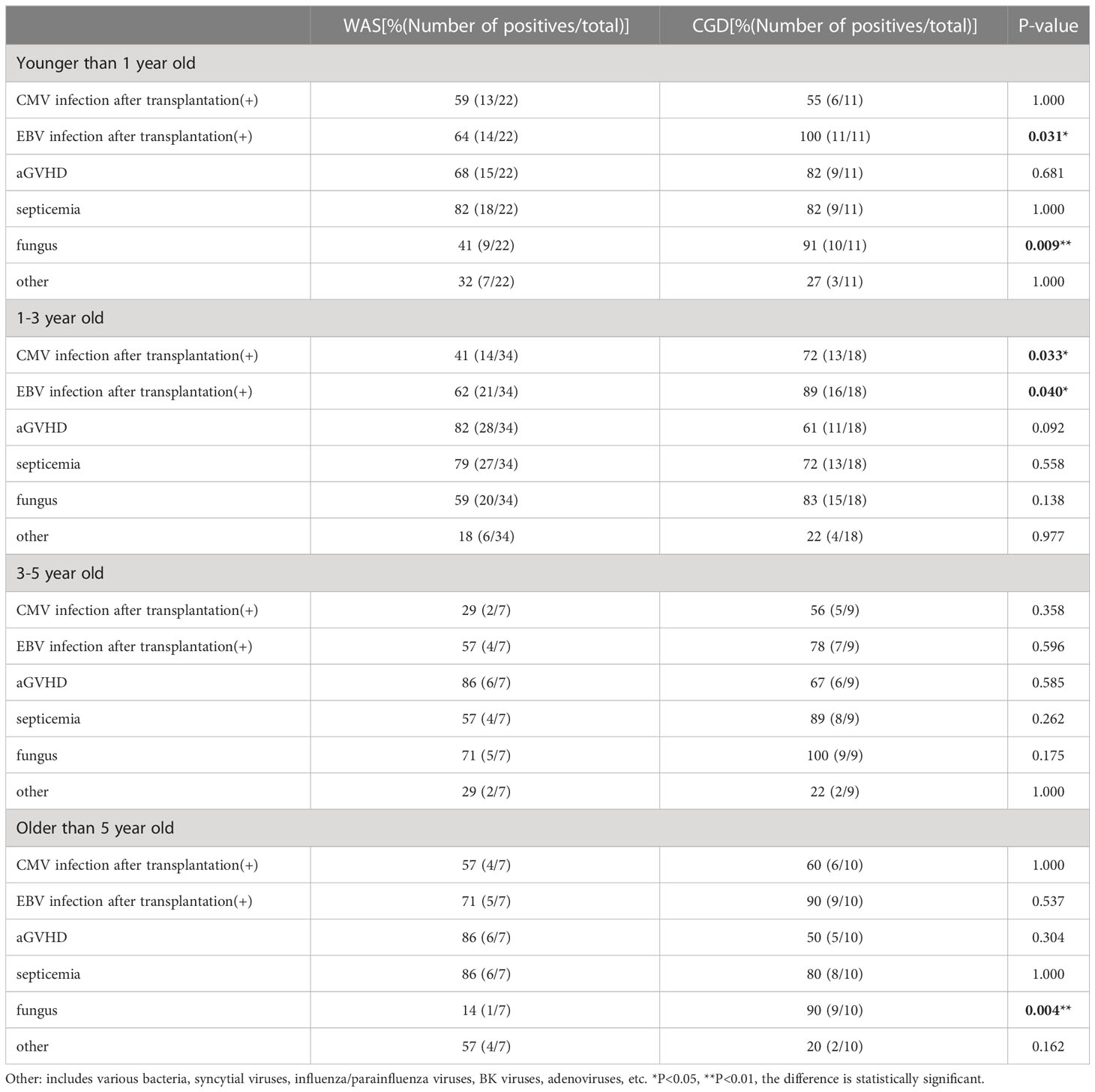
Table 8 Incidence of post-transplant infection and aGVHD in children of different ages in the WAS and CGD groups.
4 Discussion
The effect of allo-HSCT depends primarily on the hematopoietic and immune reconstitution capacity of the donor’s hematopoietic stem cells in the recipient, which suggests that posttransplantation hematopoietic and immune reconstitution is the basis of successful allo-HSCT. It was in this study that we compared for the first time the immune reconstitution data of WAS and CGD within 1 year after allo-HSCT. We first analyzed the reconstitution of lymphocyte subsets after transplantation in children with WAS and CGD by flow cytometry and found that the reconstitution of lymphocyte subsets posttransplantation was different in both groups. At Day 15 after allo-HSCT, the WAS group had significantly higher NK cell counts compared to the CGD group. At Days 30, 100, and 180 posttransplantation, the WAS group had notably higher CD4+ T-cell counts compared to the CGD group. At Days 100 and 180 posttransplantation, the WAS group had considerably higher B-cell counts compared to the CGD group. In general, immune reconstitution in the WAS group was faster than that in the CGD group after allo-HSCT.
WAS group has faster immune reconstitution than CGD group after allo-HSCT, which may be attributed to different factors inherent in the different primary diseases or to some modifiable factors (e.g., graft type). First, we included factors such as age at transplantation, graft type, HLA match, conditioning regimen, graft MNC content, and graft CD34+ cell count as independent variables, and the absolute counts of lymphocyte subpopulations on days 15, 30, 100, 180, and 360 after transplantation were selected as the dependent variables for multiple linear regression analysis, respectively. The results showed that donor type, HLA match, age at transplantation, posttransplantion CMV and EBV infection, graft type, conditioning regimen, aGVHD and blood group could affect the absolute counts of lymphocyte subpopulations.
Then, to investigate the reasons for the faster immune reconstitution in the WAS group than in the CGD group, we compared the basic clinical characteristics of the two groups of children (Table 1), including the age at transplantation, graft mononuclear cell (MNC) content and CD34+ cell count, human leukocyte antigen (HLA) matching, ABO blood group, graft type, donor type, conditioning regimen, graft versus host disease (GVHD) prophylaxis regimen, and GVHD occurrence. The graft type, graft MNC content, CD34+ cell count, and conditioning regimen were significantly different between the WAS and CGD groups. It is noteworthy that in this study, there were more umbilical cord blood transplantations (UCBTs) in the WAS group and only one UCBT in the CGD group, which led to differences in graft type between the two groups. More children with WAS underwent UCBT, while cord blood (CB) contained fewer cells, which led to differences in graft MNC content and CD34+ cell counts between children with WAS and children with CGD. The lower number of cells in CB, and therefore children who underwent UCBT did not use ATG during pretreatment, led to differences in the use of ATG during the conditioning regimen in the two groups of children. Consequently, differences in graft type between the WAS and CGD groups of children in this study resulted in differences in graft MNC content and CD34+ cell count as well as conditioning regimen. In the present paper, we focused on the effect of two variables (transplantation age and graft type) on immune recovery in those with WAS and CGD.
In this paper, the median age of children who received transplants in the WAS group was significantly younger than that of children in the CGD group, so does the age at transplantation contribute to the difference in immune reconstitution between the two groups of children? Patient age at transplantation has been recognized as a prime determinant of the speed and quality of immune reconstitution since the start of this research (12). It was shown that patients younger than 8 years who underwent allo-HSCT exhibited higher absolute lymphocyte counts at Day 30 posttransplantation and better CD4+ T-cell recovery at Day 100 posttransplantation (13). Another previous study reported that younger recipient age was associated with higher total and memory B-cell counts after transplantation, pointing to a potential role of nonhematopoietic/microenvironmental factors (14). We divided the WAS and CGD groups into different age groups (<1 year, 1-3 years, 3-5 years, >5 years); selected the age group with the largest number of children, i.e., children who underwent transplantation at 1-3 years; and compared their posttransplantation lymphocyte subpopulation reconstitution (Table 3). The results showed a higher level of lymphocyte subpopulation reconstitution in children who underwent transplants at 1-3 years in the WAS group compared to children who underwent transplants at 1-3 years in the CGD group. The finding was generally consistent with the findings of immune reconstitution in children of all ages in the WAS and CGD groups. Considering the effect of graft type, a comparison was also made between the graft types of children in the age group 1-3 years (Table 4), and it was observed that there was no significant difference between the graft types of children in the WAS and CGD groups within this age group. Therefore, in children with WAS and CGD who received allo-HSCT at the age of 1-3 years, we have not found an effect of age on immune reconstitution in both groups.
We next investigated the effect of graft type on immune reconstitution in WAS and CGD groups. As more patients in the WAS group underwent UCBT, we divided the patients in the WAS group into two groups according to UCBT and non-UCBT and compared the differences in lymphocyte subpopulation levels between the two groups after transplantation (Table 5). We found children who underwent non-UCBT had higher B-cell counts than children who underwent UCBT in the WAS group at Days 15 and 30 after allo-HSCT, but children who underwent UCBT had higher B-cell counts than children who underwent non-UCBT in the WAS group on Days 100 and 180 after allo-HSCT. This phenomenon seems to indicate that CB has strong B-cell reconstitution potential after allo-HSCT. Previous studies suggested that B-cell recovery after UCBT was faster than after sibling peripheral blood transplantation because of better B-lymphocyte reconstitution in vitro and in vivo in CB containing a higher proportion of progenitor cells (15–18). Several articles report an advantage of CB over bone marrow (BM) or peripheral blood stem cells in terms of B-cell recovery time and B-cell differentiation (15, 19, 20). A previous study concluded that the total number of B cells, non-transformed memory cells, and transformed memory B cells was higher in the CB compared to the BM or peripheral blood (15). Children with WAS have a strong B-cell reconstitution potential after UCBT, which can also be explained by the higher number of B lymphocyte progenitor cells in CB compared to BM (16). Reconstruction of CD4+ T cells in recipients receiving different graft sources varies considerably (19, 21). Early CD4+ T-cell reconstitution is significantly affected by the components of the conditioning regimen and is extremely late when T-cell depletion (e.g., ATG) is used in the conditioning regimen (22). An investigation noted that the non-use of ATG in conditioning regimens in patients with UCBT was associated with rapid CD4+ T-cell reconstitution (23). In this study, children underwent UCBT in the WAS group had higher CD4+ T-cell counts than children underwent non-UCBT, considering that none of the children underwent UCBT in the WAS group used ATG in the conditioning regimen (0%, 0/24), whereas children underwent non-UCBT in the WAS group partially used ATG in the conditioning regimen (63%, 29/46). Therefore, we considered that the T-cell counts of children with UCBT in the WAS group in this study were significantly higher than those of children with non-UCBT, which may be highly associated with the use of ATG. A higher proportion of children in the WAS group received UCBT compared to the CGD group; thus, UCBT may be partly responsible for the faster immune reconstitution in children with WAS after transplantation than in children with CGD.
In addition to the effect of UCBT, we additionally did a comparison of the counts of lymphocyte subpopulations in non-cord-blood-transplanted children in the WAS and CGD groups (Table 6). Coincidentally, lymphocyte counts were also higher in the non-cord-blood-transplanted children in the WAS group compared to the non-cord-blood-transplanted children in the CGD group. Therefore, UCBT cannot fully explain the faster immune reconstitution in children in the WAS group than in children in the CGD group. Previously, we found that the effect of age at transplantation on immune reconstitution was relatively small in both groups, and that children with non-UCBT in the WAS group still had a faster rate of immune reconstitution than children with non-UCBT in the CGD group when the effect of UCBT was not considered, therefore, we hypothesize that there may be an effect of the primary disease itself in addition to the effect of CB.
Immunoglobulins and peptides were used as a crude proxy for B-cell counts and function, and the present study also analyzed IgM, IgA, IgG, C3, and C4 serum levels after allo-HSCT in WAS and CGD groups of children (Table 7). Past studies have shown that immunoglobulin levels seem to recover simultaneously with B-cell reconstitution, where the recovery of Ig subclasses usually occurs in a unique order (15, 24). In this article, kids with CGD had higher IgA values than those with WAS at day 360 posttransplantation. However, B-cell counts were higher in children with WAS than in those with CGD at days 100 and 180 post-transplantation, with no statistical difference between the two groups at day 360 post-transplantation, indicating that B-cell reconstitution levels were inconsistent with immunoglobulin recovery in children in the WAS and CGD groups. This may be because the number of B cells is not synchronized with the recovery of secretory function, but this speculation needs to be confirmed by further studies. The results of this study also showed that children with CGD had higher C3 values than those with WAS at day 100 posttransplantation, and children with CGD had higher C4 values than those with WAS at day 360 posttransplantation. Complement is one of the important mechanisms by which antigenic antibodies exert their immune effects. In children with CGD, where the number of B cells recovered is low, more complement is beneficial to balance the immune deficiency caused by insufficient number of B cells, so the higher level of C3 and C4 in children with CGD may be a feedback effect from insufficient number of B cells. Regarding IgG reconstitution, most children in our center received regular gammaglobulin infusions during the first months posttransplantation, which may have led to similar levels of IgG reconstitution in the both groups of children.
In the above analysis, it was demonstrated that the difference in age of transplantation had a relatively small effect on immune reconstitution in children aged 1-3 years, whereas differences in graft type may have a partial effect on immune reconstitution in children with WAS and CGD, and in addition, we speculate that it was associated with the fact that WAS and CGD are two different primary diseases.
We also analyzed the posttransplantation infections in children with WAS and CGD in different age groups (<1 year, 1-3 years, 3-5 years, >5 years) (Table 8), and results showed that among children under 1 year old who underwent transplantation, the CGD group had a higher number of posttransplantation EBV infections and fungal infections compared to the WAS group. Among children 1-3 years old who underwent transplantation, the CGD group had a higher number of posttransplantation EBV infections and CMV infections than the WAS group. The number of posttransplantation fungal infections was also higher in the CGD group than in the WAS group among children over 5 years of age who received transplants. When comparing the pre and post-transplantation infections in the two groups, we found that before transplantation, the number of CMV infections in children with WAS was higher than that in children with CGD, while the number of EBV and fungal infections was lower than that in children with CGD. After transplantation, the number of CMV, EBV and fungal infections was significantly lower in children with WAS than in those with CGD. This phenomenon suggests that faster immune reconstitution in children with WAS may reduce the incidence of fungal and viral infections after transplantation.
In addition to analyzing the impact of differences in immune reconstitution on posttransplantion infection in the two groups of children, we also evaluated the impact of immune reconstitution on the duration of posttransplantion immunoglobulin infusion (Table 1). The analysis showed that the time to final immunoglobulin infusion was significantly less in children in the WAS group than in those in the CGD group after transplantation. This result confirms that the faster immune reconstitution in children with WAS may shorten the time to immunoglobulin infusion in children with WAS. The Chinese Expert Consensus on Vaccination for Children in Special Health Status recommends that after 1 year posttransplantation, immune function is normal and all types of inactivated vaccines can be administered (25). Children are in a special immune state after transplantation, and it takes 1-2 years for the immune function to be reestablished; therefore, most children in our center are not vaccinated within one year after transplantation.
Overall, this study pioneered a study on the differences in immune reconstitution posttransplantation in patients with WAS and CGD. We found that immune reconstitution is faster in children with WAS than in children with CGD, and this difference may be related to the fact that the two diseases are different primary diseases and to graft types. Our retrospective study has limitations regarding the irregularity, sample size, and patient follow-up time of immunosurveillance via flow cytometry or immunoturbidimetry after transplantation. In the future, we need to keep applying the existing standardized immune reconstitution monitoring methods and explore more patterns for immune reconstitution in children with different primary diseases undergoing allo-HSCT, which can guide physicians in using antimicrobial drugs and screening for pathogens and the development of strategies to enhance immune reconstitution, which will significantly improve the efficacy of allo-HSCT in children with immunodeficiency diseases.
Data availability statement
The raw data supporting the conclusions of this article will be made available by the authors, without undue reservation.
Ethics statement
The studies involving human participants were reviewed and approved by the Children’s Hospital of Chongqing Medical University Research Ethics Committee. Written informed consent to participate in this study was provided by the participants’ legal guardian/next of kin.
Author contributions
All authors contributed to the study conception and design. Data collection were performed by YZ and LJ. Data analysis were performed by YZ. The first draft of the manuscript was written by YZ and all authors commented on previous versions of the manuscript. All authors read and approved the final manuscript.
Funding
This study was supported by funds from the Chongqing Medical University Future Medical Youth Innovation Team Development Support Program (Grant No. W0132), the Chongqing Medical Scientific Research Project (Joint Project of Chongqing Health Commission and Science and Technology Bureau) (Grant No. 2021MSXM112), and the National Natural Science Foundation of China Youth Science Fund Project (Grant No. 81601753).
Acknowledgments
We would like to thank the medical staff of the Department of Hematology and Oncology, Children’s Hospital of Chongqing Medical University for their support in data collection for this study.
Conflict of interest
The authors declare that the research was conducted in the absence of any commercial or financial relationships that could be construed as a potential conflict of interest.
Publisher’s note
All claims expressed in this article are solely those of the authors and do not necessarily represent those of their affiliated organizations, or those of the publisher, the editors and the reviewers. Any product that may be evaluated in this article, or claim that may be made by its manufacturer, is not guaranteed or endorsed by the publisher.
References
1. Majhail NS, Rizzo JD, Lee SJ, Aljurf M, Atsuta Y, Bonfim C, et al. Recommended screening and preventive practices for long-term survivors after hematopoietic cell transplantation. Biol Blood Marrow Transplant. (2012) 18(3):348–71. doi: 10.1016/j.bbmt.2011.12.519
2. Burns S, Cory GO, Vainchenker W, Thrasher AJ. Mechanisms of WASp-mediated hematologic and immunologic disease. Blood (2004) 104(12):3454–62. doi: 10.1182/blood-2004-04-1678
3. Jin Y, Mazza C, Christie JR, Giliani S, Fiorini M, Mella P, et al. Mutations of the wiskott-Aldrich syndrome protein (WASP): hotspots, effect on transcription, and translation and phenotype/genotype correlation. Blood (2004) 104(13):4010–9. doi: 10.1182/blood-2003-05-1592
4. Massaad MJ, Ramesh N, Geha RS. Wiskott-Aldrich syndrome: a comprehensive review. Ann N Y Acad Sci (2013) 1285(1):26–43. doi: 10.1111/nyas.12049
5. Segal BH, Leto TL, Gallin JI, Malech HL, Holland SM. Genetic, biochemical, and clinical features of chronic granulomatous disease. Med (Baltimore). (2000) 79(3):170–200. doi: 10.1097/00005792-200005000-00004
6. Bach FH, Albertini RJ, Joo P, Anderson JL, Bortin MM. Bone-marrow transplantation in a patient with the wiskott-Aldrich syndrome. Lancet (1968) 2(7583):1364–6. doi: 10.1016/S0140-6736(68)92672-X
7. Burroughs LM, Petrovic A, Brazauskas R, Liu X, Griffith LM, Ochs HD, et al. Excellent outcomes following hematopoietic cell transplantation for wiskott-Aldrich syndrome: a PIDTC report. Blood (2020) 135(23):2094–105. doi: 10.1182/blood.2019002939
8. Chiesa R, Wang J, Blok HJ, Hazelaar S, Neven B, Moshous D, et al. Hematopoietic cell transplantation in chronic granulomatous disease: a study of 712 children and adults. Blood (2020) 136(10):1201–11. doi: 10.1182/blood.2020005590
9. Storek J, Geddes M, Khan F, Huard B, Helg C, Chalandon Y, et al. Reconstitution of the immune system after hematopoietic stem cell transplantation in humans. Semin Immunopathol (2008) 30(4):425–37. doi: 10.1007/s00281-008-0132-5
10. Bosch M, Khan FM, Storek J. Immune reconstitution after hematopoietic cell transplantation. Curr Opin Hematol (2012) 19(4):324–35. doi: 10.1097/MOH.0b013e328353bc7d
11. Ding Y, Zhou L, Xia Y, Wang W, Wang Y, Li L, et al. Reference values for peripheral blood lymphocyte subsets of healthy children in China. J Allergy Clin Immunol (2018) 142(3):970–3. doi: 10.1016/j.jaci.2018.04.022
12. Small TN, Papadopoulos EB, Boulad F, Black P, Castro-Malaspina H, Childs BH, et al. Comparison of immune reconstitution after unrelated and related T-cell-depleted bone marrow transplantation: effect of patient age and donor leukocyte infusions. Blood (1999) 93(2):467–80. doi: 10.1182/blood.V93.2.467
13. Belinovski AR, Pelegrina PD, Lima ACM, Dumke CCK, Rodrigues AM, Loth G, et al. Immune reconstitution after allogenic stem cell transplantation: An observational study in pediatric patients. Hematol Transfus Cell Ther (2023) 45(2):235–244. doi: 10.1016/j.htct.2022.05.008
14. van der Maas NG, von Asmuth EGJ, Berghuis D, van Schouwenburg PA, Putter H, van der Burg M, et al. Modeling influencing factors in b-cell reconstitution after hematopoietic stem cell transplantation in children. Front Immunol (2021) 12:684147. doi: 10.3389/fimmu.2021.684147
15. Abdel-Azim H, Elshoury A, Mahadeo KM, Parkman R, Kapoor N. Humoral immune reconstitution kinetics after allogeneic hematopoietic stem cell transplantation in children: a maturation block of IgM memory b cells may lead to impaired antibody immune reconstitution. Biol Blood Marrow Transplant. (2017) 23(9):1437–46. doi: 10.1016/j.bbmt.2017.05.005
16. Arakawa-Hoyt J, Dao MA, Thiemann F, Hao QL, Ertl DC, Weinberg KI, et al. The number and generative capacity of human b lymphocyte progenitors, measured in vitro and in vivo, is higher in umbilical cord blood than in adult or pediatric bone marrow. Bone Marrow Transplant. (1999) 24(11):1167–76. doi: 10.1038/sj.bmt.1702048
17. Jacobson CA, Turki AT, McDonough SM, Stevenson KE, Kim HT, Kao G, et al. Immune reconstitution after double umbilical cord blood stem cell transplantation: comparison with unrelated peripheral blood stem cell transplantation. Biol Blood Marrow Transplant. (2012) 18(4):565–74. doi: 10.1016/j.bbmt.2011.08.018
18. Elmariah H, Brunstein CG, Bejanyan N. Immune reconstitution after haploidentical donor and umbilical cord blood allogeneic hematopoietic cell transplantation. Life (Basel). (2021) 11(2):102. doi: 10.3390/life11020102
19. Bartelink IH, Belitser SV, Knibbe CA, Danhof M, de Pagter AJ, Egberts TC, et al. Immune reconstitution kinetics as an early predictor for mortality using various hematopoietic stem cell sources in children. Biol Blood Marrow Transplant. (2013) 19(2):305–13. doi: 10.1016/j.bbmt.2012.10.010
20. Rénard C, Barlogis V, Mialou V, Galambrun C, Bernoux D, Goutagny MP, et al. Lymphocyte subset reconstitution after unrelated cord blood or bone marrow transplantation in children. Br J Haematol (2011) 152(3):322–30. doi: 10.1111/j.1365-2141.2010.08409.x
21. Fedele R, Martino M, Garreffa C, Messina G, Console G, Princi D, et al. The impact of early CD4+ lymphocyte recovery on the outcome of patients who undergo allogeneic bone marrow or peripheral blood stem cell transplantation. Blood Transfus. (2012) 10(2):174–80. doi: 10.2450/2012.0034-11
22. Admiraal R, van Kesteren C, Jol-van der Zijde CM, Lankester AC, Bierings MB, Egberts TC, et al. Association between anti-thymocyte globulin exposure and CD4+ immune reconstitution in paediatric haemopoietic cell transplantation: a multicentre, retrospective pharmacodynamic cohort analysis. Lancet Haematol (2015) 2(5):e194–203. doi: 10.1016/S2352-3026(15)00045-9
23. Politikos I, Lavery JA, Hilden P, Cho C, Borrill T, Maloy MA, et al. Robust CD4+ T-cell recovery in adults transplanted with cord blood and no antithymocyte globulin. Blood Adv (2020) 14;4(1):191–202. doi: 10.1182/bloodadvances.2019000836
24. Bosch M, Dhadda M, Hoegh-Petersen M, Liu Y, Hagel LM, Podgorny P, et al. Immune reconstitution after anti-thymocyte globulin-conditioned hematopoietic cell transplantation. Cytotherapy (2012) 14(10):1258–75. doi: 10.3109/14653249.2012.715243
Keywords: immune reconstruction, lymphocyte reconstruction, allogeneic hematopoietic stem cell transplantation, Wiskott-Aldrich syndrome, chronic granulomatous disease
Citation: Zhou Y, Zhang L, Meng Y, Lei X, Jia L, Guan X, Yu J and Dou Y (2023) Differential analysis of immune reconstitution after allogeneic hematopoietic stem cell transplantation in children with Wiskott-Aldrich syndrome and chronic granulomatous disease. Front. Immunol. 14:1202772. doi: 10.3389/fimmu.2023.1202772
Received: 09 April 2023; Accepted: 30 May 2023;
Published: 14 June 2023.
Edited by:
Joerg Halter, University of Basel, SwitzerlandReviewed by:
Pamela P. Lee, The University of Hong Kong, Hong Kong SAR, ChinaSheng-Li Xue, The First Affiliated Hospital of Soochow University, China
Copyright © 2023 Zhou, Zhang, Meng, Lei, Jia, Guan, Yu and Dou. This is an open-access article distributed under the terms of the Creative Commons Attribution License (CC BY). The use, distribution or reproduction in other forums is permitted, provided the original author(s) and the copyright owner(s) are credited and that the original publication in this journal is cited, in accordance with accepted academic practice. No use, distribution or reproduction is permitted which does not comply with these terms.
*Correspondence: Ying Dou, ZG91eWluZzUyM0BhbGl5dW4uY29t