- 1Department of Biochemical Engineering, Advanced Centre for Biochemical Engineering, University College London, London, United Kingdom
- 2CellTrials.org and Parent’s Guide to Cord Blood Foundation, a non-profit organization headquartered in Brookeville, Brookeville, MD, United States
- 3Department of Nephrology and Internal Intensive Care Medicine, Charité Universitätsmedizin Berlin, corporate member of Freie Universität Berlin, Humboldt-Universität zu Berlin, Berlin Institute of Health (BIH), Berlin, Germany
- 4Vascular Surgery Clinic, Charité Universitätsmedizin Berlin, Berlin, Germany
- 5Department of Immunology, Institute of Biomedical Sciences, University of São Paulo (USP), São Paulo, SP, Brazil
- 6Interunit Postgraduate Program on Bioinformatics, Institute of Mathematics and Statistics (IME), University of São Paulo (USP), São Paulo, SP, Brazil
- 7Department of Vascular Surgery, Universitätsklinikum Ruppin-Brandenburg, Medizinische Hochschule Brandenburg Theodor Fontane, Neuruppin, Germany
- 8Fakultät der Gesundheitswissenschaften Brandenburg, Gemeinsame Fakultät der Universität Potsdam, der Medizinischen Hochschule Brandenburg Theodor Fontane, und der Brandenburg Technischen Universität (BTU) Cottbus-Senftenberg, Potsdam, Germany
- 9Department of Biomedical Sciences, Mercer University School of Medicine, Savannah, GA, United States
- 10Advanced Cell Therapy (ACT) Laboratory, Yale School of Medicine, New Haven, CT, United States
- 11Department of Clinical and Toxicological Analyses, School of Pharmaceutical Sciences, University of São Paulo (USP), São Paulo, SP, Brazil
- 12Department of Pharmacy and Postgraduate Program of Health and Science, Federal University of Rio Grande do Norte, Natal, Brazil
- 13Department of Medicine, Division of Molecular Medicine, University of São Paulo School of Medicine, São Paulo, Brazil
- 14Laboratory of Medical Investigation 29, University of São Paulo School of Medicine, São Paulo, Brazil
- 15Berlin Institute of Health (BIH) Center for Regenerative Therapies (BCRT), Charité - Universitätsmedizin Berlin, Berlin, Germany
During the pandemic of severe respiratory distress syndrome coronavirus 2 (SARS-CoV2), many novel therapeutic modalities to treat Coronavirus 2019 induced disease (COVID-19) were explored. This study summarizes 195 clinical trials of advanced cell therapies targeting COVID-19 that were registered over the two years between January 2020 to December 2021. In addition, this work also analyzed the cell manufacturing and clinical delivery experience of 26 trials that published their outcomes by July 2022. Our demographic analysis found the highest number of cell therapy trials for COVID-19 was in United States, China, and Iran (N=53, 43, and 19, respectively), with the highest number per capita in Israel, Spain, Iran, Australia, and Sweden (N=0.641, 0.232, 0,223, 0.194, and 0.192 trials per million inhabitants). The leading cell types were multipotent mesenchymal stromal/stem cells (MSCs), natural killer (NK) cells, and mononuclear cells (MNCs), accounting for 72%, 9%, and 6% of the studies, respectively. There were 24 published clinical trials that reported on infusions of MSCs. A pooled analysis of these MSC studies found that MSCs provide a relative risk reduction for all-cause COVID-19 mortality of RR=0.63 (95% CI 0.46 to 0.85). This result corroborates previously published smaller meta-analyses, which suggested that MSC therapy demonstrated a clinical benefit for COVID-19 patients. The sources of the MSCs used in these studies and their manufacturing and clinical delivery methods were remarkably heterogeneous, with some predominance of perinatal tissue-derived products. Our results highlight the important role that cell therapy products may play as an adjunct therapy in the management of COVID-19 and its related complications, as well as the importance of controlling key manufacturing parameters to ensure comparability between studies. Thus, we support ongoing calls for a global registry of clinical studies with MSC products that could better link cell product manufacturing and delivery methods to clinical outcomes. Although advanced cell therapies may provide an important adjunct treatment for patients affected by COVID-19 in the near future, preventing pathology through vaccination still remains the best protection to date. We conducted a systematic review and meta-analysis of advanced cell therapy clinical trials as potential novel treatment for COVID-19 (resulting from SARS-CoV-2 coronavirus infection), including analysis of the global clinical trial landscape, published safety/efficacy outcomes (RR/OR), and details on cell product manufacturing and clinical delivery. This study had a 2-year observation interval from start of January 2020 to end of December 2021, including a follow-up period until end of July to identify published outcomes, which covers the most vivid period of clinical trial activity, and is also the longest observation period studied until today. In total, we identified 195 registered advanced cell therapy studies for COVID-19, employing 204 individual cell products. Leading registered trial activity was attributed to the USA, China, and Iran. Through the end of July 2022, 26 clinical trials were published, with 24 out of 26 articles employing intravenous infusions (IV) of mesenchymal stromal/stem cell (MSC) products. Most of the published trials were attributed to China and Iran. The cumulative results from the 24 published studies employing infusions of MSCs indicated an improved survival (RR=0.63 with 95% Confidence Interval 0.46 to 0.85). Our study is the most comprehensive systematic review and meta-analysis on cell therapy trials for COVID-19 conducted to date, clearly identifying the USA, China, and Iran as leading advanced cell therapy trial countries for COVID-19, with further strong contributions from Israel, Spain, Australia and Sweden. Although advanced cell therapies may provide an important adjunct treatment for patients affected by COVID-19 in the future, preventing pathology through vaccination remains the best protection.
1 Introduction
The outbreak of the novel severe respiratory distress syndrome coronavirus 2 (SARS-CoV2) and its adjunct symptomatic, Coronavirus 2019 induced disease (COVID-19), is one of the most significant world health events in recorded history (1). Early reports during the initial outbreak in Wuhan, China, found that up to 14% of patients presented with the severe form of COVID-19 and that mortality was as high as 3% (2–4). Subsequently, the virus became a global pandemic and new variants emerged (5–9). Major variants responsible for surges of virus infections include “Beta” (South Africa, May 2020), “Delta” (India, October 2020), and “Gamma” (Brazil, November 2020) (Figure 1A) (7). During the summer and fall seasons of 2022, the predominant circulating variants were sub-types of “Omicron”, first documented in November 2021 across multiple countries (10); E.g. the “Omicron” sublineage BQ.1 was designated as a Variant of Interest (VOI) by the European Center for Disease Prevention and Control (ECDC) as of 20th of October 2022 and it was expected that by mid-November to beginning of December 2022 more than 50% of SARS-CoV-2 infections were due to BQ.1/BQ.1.1 (11). This demonstrates the rapid dynamics in virus changes (9). By the end of December 2022, the worldwide death toll attributed directly to COVID-19 had surpassed 6.6 million individuals (5–7).
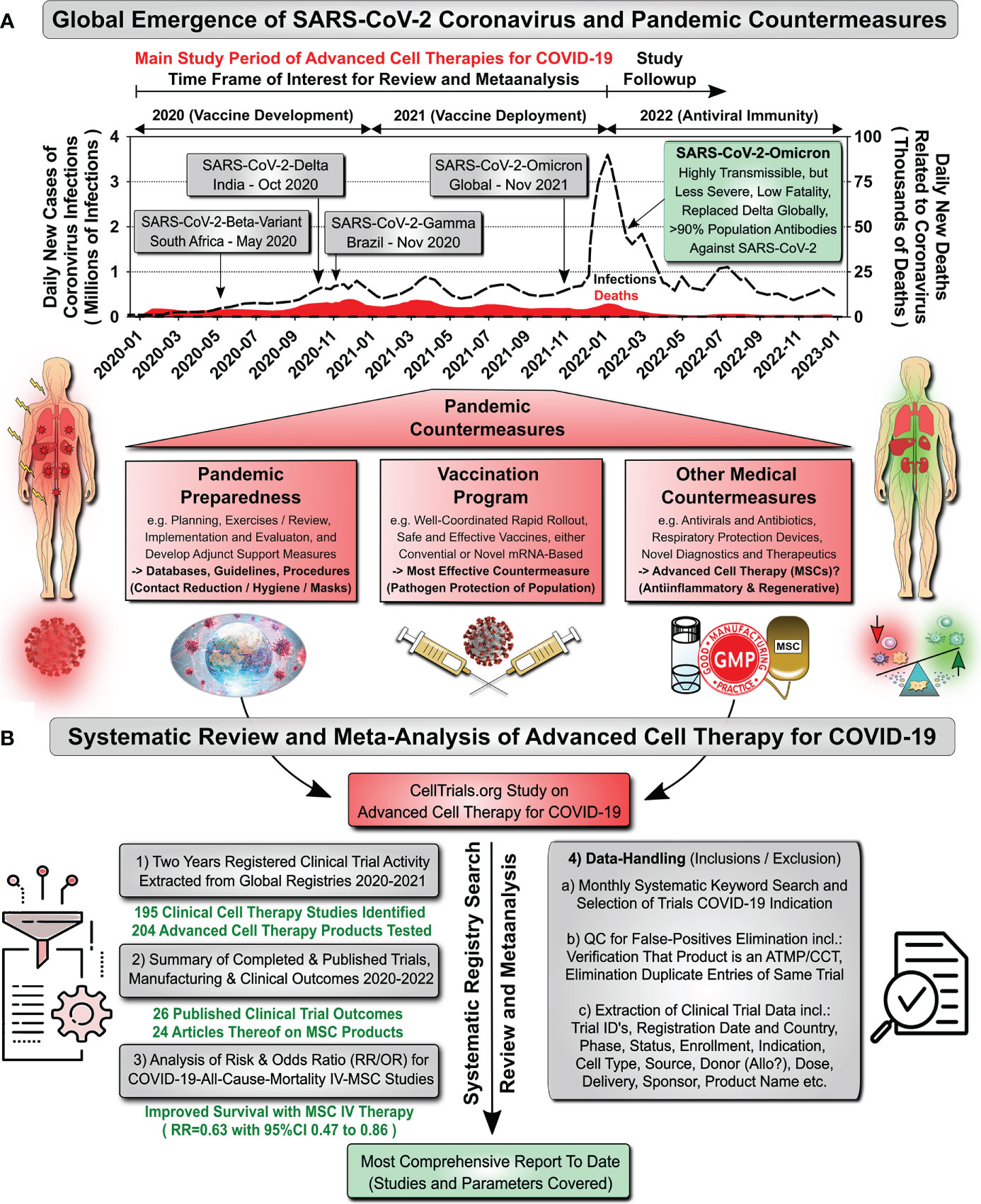
Figure 1 Study Design of Systematic Review & Meta-Analysis of Cell Therapy for COVID-19. (A) Global Emergence of SARS-CoV-2 Coronavirus and Pandemic Countermeasures: Data on daily new registered cases of coronavirus infections (Millions of Infections, dotted black curve) and daily new deaths related to coronavirus infection (Thousands of Deaths, red section/curve at bottom) were obtained from the COVID-Worldometer (6). Arrows indicate the emergence of major SARS-CoV-2 variants and interventional measures (2020 start of novel vaccine development programs; 2021 start of novel vaccine deployment, some countries already started in the late fall of 2020, e.g. the US started on the 14th of Dec, Israel on the 20th of Dec, and Germany on 26th of Dec 2020; and 2022 emergence of antiviral immunity in population). Our study period of advanced cellular therapy clinical trials for COVID-19 spans from January 2020 to end of December 2021, with follow-up search for publications until the end of July 2022. Pandemic countermeasures are indicated below the graph, depicting 1) Pandemic Preparedness (e.g. including databases, guidelines, and procedures), 2) Vaccination Programs (most effective countermeasures), and 3) Other Medical Countermeasures (e.g. advanced cell therapies, such as mesenchymal stromal/stem cells, MSCs, of unknown value). (B) Systematic Review and Meta-Analysis of Advanced Cell Therapies for COVID-19: Until today most information on advanced cell therapies for COVID-19 is still highly fragmented, in the sense that clinical trials and publications are being compiled separately. This prompted us to conduct this systematic review and meta-analysis to summarize and combine the current knowledge, covering: 1) Two years of registered clinical trial activity, 2) Summary of published clinical trial outcomes, cell product manufacturing and clinical delivery between 2020 and mid-2022, 3) Analysis of risk and odds ratios for COVID-19 all-cause-mortality considering intravenous (IV)-use of MSCs, and 4) A summary of the quality control routines, which were applied for the data-handling (Inclusion/Exclusion) in this study.
The COVID-19 pandemic created an ideal situation for the convergence of two research quests that had been progressing independently for decades. The first quest came from pulmonology, where researchers have sought to improve mortality from acute respiratory distress syndrome (ARDS) for decades, with mortality levels of 44% in clinical trials since the 1980’s (12). The second quest, dating back from the 1990s, was the scientific effort to demonstrate clinical efficacy for cell therapy products containing multipotent mesenchymal stromal/stem cells (MSCs) (2, 13–18). Preliminary evidence suggested that MSCs might be beneficial for pulmonary disorders (2, 18). This is supported by biodistribution studies which demonstrated that MSCs given intravenously (IV) rapidly localize to the lungs, where they may exert their beneficial properties (2, 15, 16, 18). It is well established that the immunomodulatory and regenerative properties of MSCs entail a plethora of distinct synergistic mechanisms of action (MoAs) that might help ameliorate pulmonary conditions (13, 18).
Between 2011 to 2019, the database CellTrials.org identified 16 clinical trials of MSCs for ARDS, and by April 2020, seven of these trials were completed, and five were published (19). Unfortunately, none of these publications demonstrated clinical efficacy of MSCs against ARDS. Similarly, a literature search based on published studies of MSCs for ARDS between 1990 to 2020 found nine such studies and confirmed that the improvement in mortality was not significant (20). In the past, poor outcomes considering ARDS mortality were often attributed to the high complexity of ARDS etiology and pathology (e.g. many small/difficult to target subgroups) and rapid disease progression (e.g. short time window for interventional treatment). Thus, any studies aiming to prove efficacy in ARDS typically require stringent inclusion/exclusion criteria and large trial cohorts to control for confounders. This has been challenging to achieve, given that patient enrollment only reaches sufficient numbers in larger specialized clinical centers, thus often requiring multi-center studies (12).
This led to the convergence of the quests mentioned above during the COVID-19 pandemic. For the first-time large cohorts were broadly available to effectively study novel therapeutic interventions. Pulmonologists noted very early that COVID-19 differs from classic presentations of ARDS (21), thus calling for an in-depth analysis of clinical trial outcomes. However, current knowledge on the outcome of cell therapy studies for both ARDS and COVID-19 is still fragmented (Figure 1B) (2, 18). Covering the most relevant 2-year interval (Jan 2020 to Dec 2021), we here report the outcomes of the available published clinical trials focusing on cell therapy of COVID-19 today. This also covers specific intricacies of product manufacturing and delivery to patients (2). Our analysis found that three quarters of cell therapies deployed against COVID-19 relied on MSCs. During 2020 alone more than 100 clinical trials were registered worldwide that employed MSCs to treat COVID-19 pneumonia and acute respiratory distress. Indeed, published outcomes from those trials that focus on MSC therapy for COVID-19 now appear to be sufficient to warrant a first comprehensive examination of the safety and efficacy profiles of MSCs for treating severe COVID-19.
2 Materials and methods
This study presents three types of data regarding cell-based therapies for COVID-19 (Figure 1B): (1) We have collected two years (from Jan 2020 to Dec 2021) of registered clinical trial activity extracted from worldwide registries; (2) We have gathered the published clinical outcomes and extracted any available information on manufacturing and clinical delivery of MSC products from the published studies to study the potential impact of cell product manufacturing and mode of delivery on clinical efficacy; (3) We have performed a relative risk ratio (RR) and odds ratio (OR) analysis for all-cause COVID-19 mortality for studies employing intravenous use of MSCs (most frequent).
2.1 Identification of registered clinical trials, keyword search, inclusion/exclusion criteria
The methodology for identifying and assembling the database of clinical trials was the same as described earlier by CellTrials.org (22, 23). Briefly, five main steps were performed monthly: (2.1.1) Keyword-based search (keywords shown below) for advanced cell therapy clinical trials worldwide, (2.1.2) Elimination of false positives, (2.1.3) Elimination of duplicate entries, (2.1.4) Gathering detailed data from included clinical trials, and (2.1.5) Extracting trials from the primary data-base where the indication for cell therapy use was COVID-19. The accuracy of the data search relies on the usage of multiple national registries of clinical trials (Table 1), including Australia and New Zealand, Brazil, China, Cuba, Germany, India, Iran, Japan, The Netherlands, Singapore, South Korea, Thailand, the United States of America (USA), the European Union (EU), and World Health Organization (WHO). The time frame for identifying advanced cell therapy trials for COVID-19 was between January 2020 until end of December 2021, containing the large majority of so far registered clinical trials (as detailed below). A tabular excel sheet summary of all 195 identified studies including 20 individual parameters can be found in Table S1.
2.1.1 Keyword search
The keywords used in the first step, broadly designed to capture all advanced cell therapies, included: “COVID-19”, “cell”, “cell therapy”, “cancer vaccine”, “CAR-T”, “chimeric antigen”, “DC”, “NK”, “TIL”, “tumor infiltrating”, “adoptive”, “regenerative”, “mesenchymal”, “adipose”, “bone marrow”, “cord blood”, and “umbilical”.
2.1.2 Elimination of false positives
A second curation step was needed to screen for studies that were performing advanced cell therapy and were not just a false hit on a keyword. We applied the definitions of Advanced Cell Therapy Medicinal Products (ATMPs) adopted by the European Medicines Agency (EMA), and Human Cellular Tissue Products (HCT/Ps) adopted by the US Food and Drug Administration (FDA) (24–26). This step was performed by having at least two scientists review each trial description.
2.1.3 Elimination of duplicate entries
To remove double postings of the same trial in more than one registry, scientific review was applied. If the trial was listed on the US registry ClinicalTrials.gov and another national registry within the same month, then the trial was assigned to ClinicalTrials.gov. If the trial appeared on a second registry months later, it stayed assigned to the month and registry where it first appeared.
2.1.4 Extraction of trial data
The dataset was built by recording the following parameters for each trial: registration date, clinical trial unique ID, secondary ID if any, country of registration, phase, status, cell type, cell source, route of administration, dosage if known, clinical indication, donor type (allogenic or autologous), target enrollment, age ranges of the patient population, type of sponsor (academia or industry), names of the sponsors, and product name if any. (1.5) Selection of trials with COVID-19 indication: The final step for this study was to extract the clinical trials of cell-based therapies where the indication for clinical use was COVID-19. On a monthly basis, we posted them online as an open-access community service. Since the early months of the COVID-19 pandemic, our living database of clinical trials has been listed as a resource on the evidence hub of the Center for Science in the Public Interest (CSPI) (27).
2.2 Published clinical trial outcomes, cell therapy manufacturing, and clinical cell delivery
The methodology for gathering information on safety and efficacy from clinical trials of cell-based therapies for COVID-19 has been previously described (23). Briefly, two complementary methods were employed. First, the PubMed registry was searched for publications using the keywords “COVID”, “cell” and “clinical trial”. Second, the search was refined by identifying publications containing the unique ID of each registered trial in our database. We only included publications reporting the outcomes of registered clinical trials but excluded case reports which could not be linked to registered trials. The collected parameters were as follows: connection between trial and publication, country where study was conducted, study design, study endpoints, target enrollment of trial, actual enrollment in paper, cell type(s), cell source(s), cell dose(s), route of administration, adverse events, survival of cell therapy patients and controls. The cut-off date for including publications from trials registered in 2020 and 2021 was the end of July 2022.
2.2.1 World map figures
Global distribution of cell therapy trials for the treatment of COVID-19 per country was displayed as heat map either for the absolute number of trials per country or per capita values. Maps were drawn using the “R” packages ‘maps’ (28) and ‘ggplot2’ (29). The corresponding analysis scripts are available at https://github.com/Starahoush/MSC-COVID19_metaanalysis.
2.2.2 Information on cell therapy manufacturing from published studies
Manufacturing and clinical delivery information for each MSC product were obtained upon close inspection of papers to extract information considering: cell sources, donors, cell isolation, cell expansion, cellular passages, medium formulation, storage (fresh or frozen) (30, 31), and quality control steps (e.g. did MSCs fulfill ISCT minimal criteria)? (17, 32, 33). This search often required checking additional sources when the cell product was supplied by a contract manufacturer or described in an earlier publication.
2.3 Risk ratio and odds ratio for COVID-19 all-cause mortality for published studies
Statistical analysis was carried out in “R” version 4.2.1 (34), the meta package version 6.0.0, and visualized via forest plots from the same package (35). The Risk Ratio (RR) and the Odds Ratio (OR) were calculated by using the Mantel-Haenszel test (36) and employed to analyze the effect of MSC therapy on the risk/odds of death following COVID-19 infection. The 95% confidence interval for both ratios and the combined statistics are reported for each study. The code used for this analysis was uploaded to https://github.com/Starahoush/MSC-COVID19_metaanalysis.
2.3.1 Handling of missing data
The RR and OR calculations require input studies to have two arms, one of the patients undergoing experimental treatment versus a second arm of control/placebo patients. However, during the COVID-19 pandemic, many clinical trials were conducted that only treated patients and did not have a control group. To incorporate their published outcomes, the following methodology was used to integrate single-arm studies, which lacked control data. First, the mean and median data of the controlled studies were obtained. Then, two assumptions were made about the studies without controls: First, it was assumed that the mean behavior of the missing controls was the same as that in the controlled studies, and second, it was assumed that the ratio of MSC patient number to control patient number was the same as the median for the controlled studies. With these two assumptions it was possible to incorporate the experimental results from single-arm studies to calculate RR and OR for all studies as a group. Of the 24 published MSC studies, 17 had complete information regarding the number of participants and events for both treatment and control groups (37–53); whereas seven studies were missing data for the control group (54–60). Also, three studies (43, 46, 49) were double-arm-zero-event, and we employed a treatment arm continuity correction (TACC) to incorporate them, since otherwise risk/odds ratios could not have been calculated (61, 62).
3 Results
3.1 2-Year registered global clinical trial landscape of advanced cell therapies for COVID-19
A comprehensive search for advanced cell therapy trials to target the clinical indication COVID-19 and related complications was conducted in 18 national and international registries (Table 1). The cell therapy products employed in these trials are referred to as advanced therapy medicinal products (ATMPs) or human cellular and tissue products (HCT/Ps) in the EU and US, respectively (2, 24, 26). Between January 2020 and December 2021, 195 advanced-cell-therapy-based clinical trials targeting COVID-19 and related complication were registered worldwide (Table S1). While we have released first smaller compilations in June 2020 and 2021 (63, 64), the current study covers the most relevant 2-year time window from January 2020 to the end of December 2021 (Figures 1A, 2A). The relevance of this time frame is depicted by the initial peak of monthly registered clinical trials in the first months of the pandemic, followed by dramatic decline and a long tail afterwards. The first clinical trial registrations appeared in China and USA in February 2020. Some February trials were registered retrospectively, but subsequent publications revealed that patients began receiving cell therapy for COVID-19 as early as January 2020 in China. The peak of registrations was April 2020. Noteworthy, registrations of cell therapy trials for COVID-19 had only one peak in spring 2020, although global COVID-19 cases went through four major surges during the 2-year timeframe (5–7). The peak in trial registrations subsided months before the roll out of vaccination programs (65).
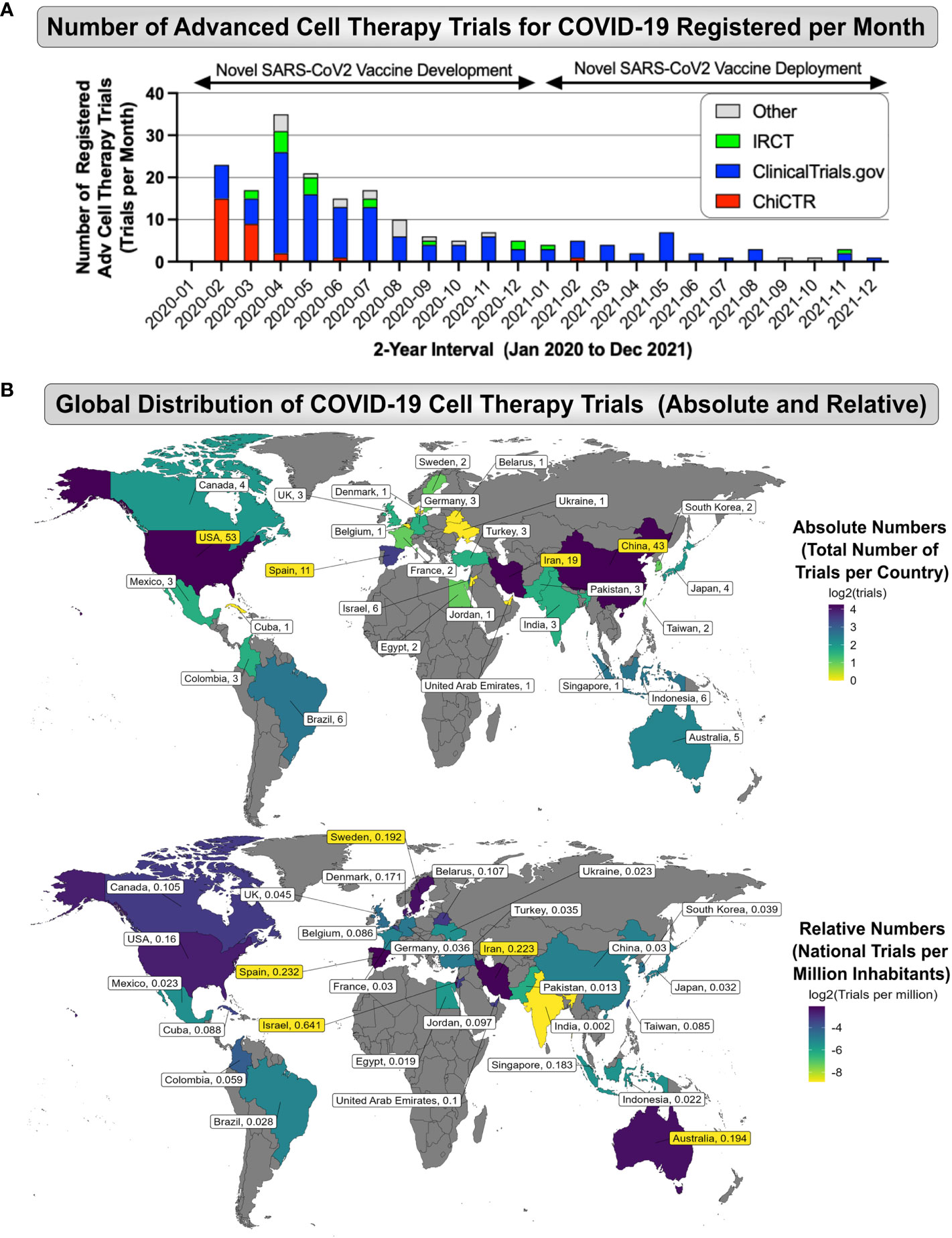
Figure 2 Global Landscape of Cell Therapy Trials for COVID-19. (A) Number of Advanced Cell Therapy Trials for COVID-19 Registered per Month: Data on the number of registered advanced cell therapy trials (Trials per Month; depicted is the 2-year interval of interest from the start of January 2020 to end of December 2021) were collected from available national and international clinical trial registries, e.g. the American registry (ClinicalTrials.gov; shown in blue), Chinese registry (ChiCTR, shown in red), Iranian registry (IRCT, shown in green), and other registries (shown in grey), depicting a peak in advanced cell therapy trial registrations between February to July of 2020, with a subsequent long tail of decline in cell therapy trial activity, which occurred at the same time the novel SARS-CoV-2 vaccines showed first success in early clinical trials and started to be deployed (e.g. vector or mRNA-based vaccines); and (B) Global Distribution of COVID-19 Advanced Cell Therapy Trials (Absolute and Relative No): The top panel depicts the Absolute Numbers (Total Number of Trials per Country) identifying the US (n=53), China (n=43), Iran (n=19), and Spain (n=11), as the most active countries considering the total trial number output, while the bottom panel depicts the Relative Trial Numbers (National Trials per Million Inhabitants) identifying Israel, Spain, Iran, Australia, and Sweden (N=0.641, 0.232, 0,223, 0.194, and 0.192 trials per million inhabitants) as the most prolific countries relative to their (smaller) national population size, again depicting Iran in place three as for the total trial output.
To date, information on advanced cell therapy trials for COVID-19 remains fragmented, although first valuable literature reviews and meta-analyses have been conducted, this is the first study that comprehensively connects trials to subsequent publications. We have listed a summary of prior compilations in descending order of the cut-off date of their conducted search (Table 2) (63, 64, 66–86). Reviews of COVID-19 clinical trials not strictly focused on cell therapy were excluded from the list. Importantly, the 195 trials identified in this article are more than double the number presented by previous authors, which demonstrates the outreach of our data search criteria. Our compilation of cell therapy trials for COVID-19 is so far the only one that offers worldwide trial data versus time for a 2-year time frame (Figure 2A). Our review also tracks contributions of different clinical trial registries over time, illustrated by the respective color coding, which indicates a dominance of contributions from the US (blue), Chinese (red), and Iranian (green) registries, while the contributions from other registries (grey) were smaller. This is partly because the most dominant US registry (clinical.trials.gov, 131 of the 195 registered trials) was used as the default template in our search. It must be noted, that in some countries that have a clinical trial registry, researchers are obligated to use their national registry, and cross posting their trial to ClinicalTrials.gov is optional, so that ClinicalTrials.gov should never be relied upon as a complete international record of clinical trials.
A global heatmap of the countries where clinical trials of cell therapy for COVID-19 were conducted, regardless of where they were registered, is shown in Figure 2B. Only one trial took place in more than one country. Among 30 participating countries, leaders were the US (n=53, 27%), China (n=43, 22%), Iran (n=19, 10%), and Spain (11, 6%), while other countries hosted <4% (Figure 2B top). The highest relative trial numbers per capita came from Israel, Spain, Iran, Australia, and Sweden (N=0.641, 0.232, 0,223, 0.194, 0.192 trials/million inhabitants, respectively) (Figure 2B bottom). Our compilation is the only one that identifies Iran as the 3rd absolute and relative leading contributor. Presumably, this is because most trials in Iran are only listed on the Iranian national registry, and not cross-posted to ClinicalTrials.gov. Noteworthy, the list of countries leading in cell therapy for COVID-19 through the end of 2021 (US, China, and Iran) does not match the lists of countries that reported the highest number of COVID-19 infections (US, India, France, Brazil) or the highest number of COVID-19 related deaths (US, Brazil, India, Russia) during that timeframe (6).
3.2 Types of cell products in registered clinical trials
Detailed information about the 195 advanced cell therapy trials for COVID-19 registered 2020-2021, including up to 20 individual parameters for each registered trial (listed in the Methods) are listed in Table S1 with representative plots of important parameters shown in Figure 3A and the top of 3B. While the terminology of our database uses “route of administration” and “cell storage”, in the discussion these topics are combined as “clinical delivery”.Among the 195 registered cell therapy trials for COVID-19, most (n=141, 72%) tested some type of MSC product (Figure 3A). The next most common cell types were natural killer (NK) cells and mononuclear cells (MNC), employed in 9% and 6% of the trials, respectively. Interestingly, n=7 of the registered trials used more than one cell type, including more than one MSC type, which is why the total number of cell products is n=204 in the chart (Figure 3A, left panel). Even with all cell types counted individually MSC-product-based trials still accounted for 147/204 (72%) of the registered cell therapy trials. The most common type of MSC source was perinatal tissue (PT)-derived PT-MSCs, accounting for 70 trials (34% of all cell types, or 48% of MSC products in trials) (Figure 3A right panel). Within this category we included umbilical cord (UC)-derived UC-MSCs in 58 trials, or other perinatal sources in 12 trials. This was followed by adipose tissue (AT)-derived AT-MSCs in 27 studies (13% of cell types in trials, or 18% of the MSC products), and bone marrow (BM)-derived BM-MSCs in 22 studies (11% of all cell types in trials, or 15% of the MSC products), and other types of MSC sources in 28 trials (14% of all cell types, and 19% of the MSC products).
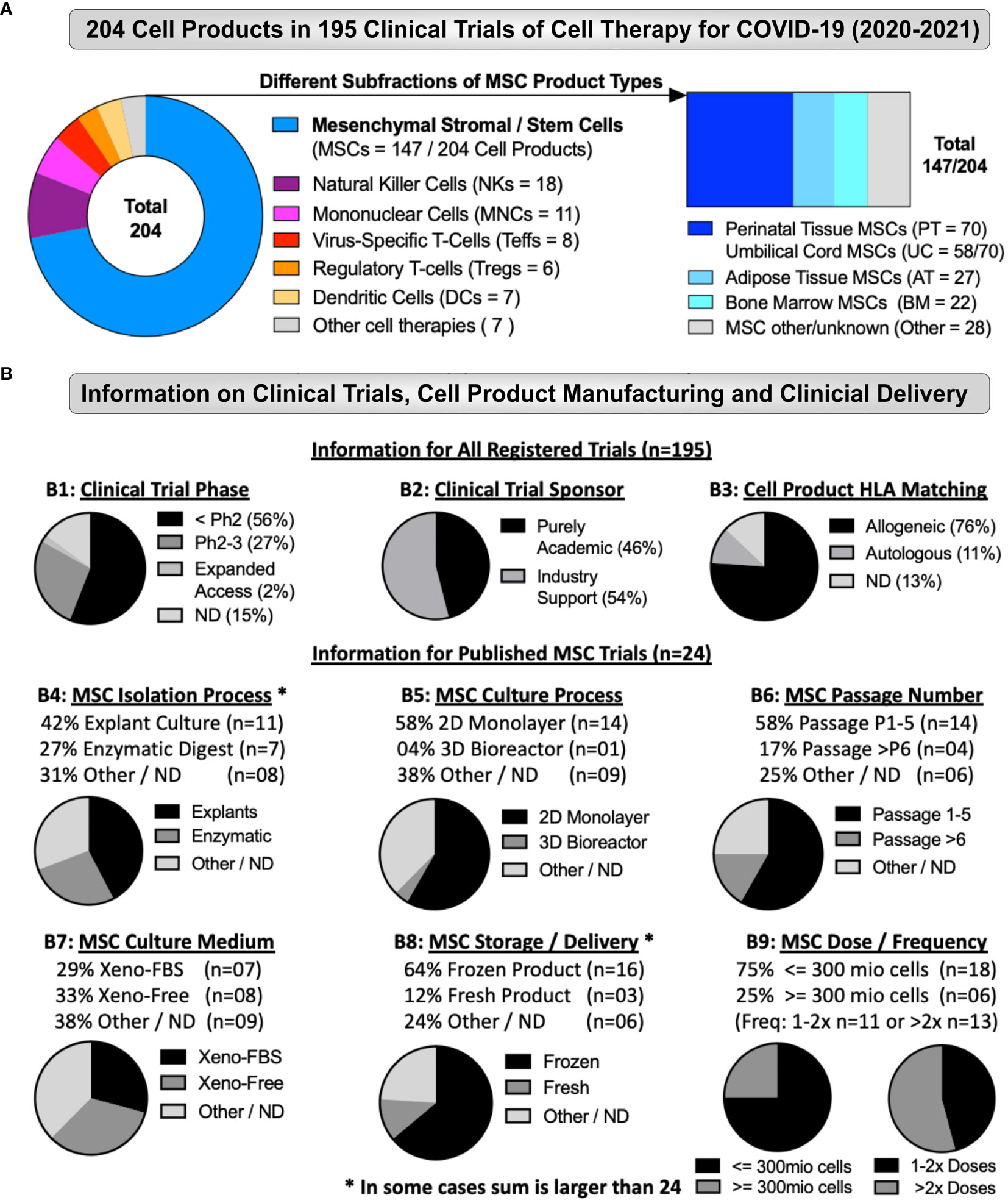
Figure 3 Cell Types and Manufacturing in Cell Therapy Trials for COVID-19. (A) Different Types of Cell Products Tested as Therapy for COVID-19 (2020-2021): The figure depicts the total number of advanced cell therapy products tested as treatment for COVID-19 in clinical trials registered between the start of January 2020 to end of December 2021 (The number of 204 products depicted here is higher than the 195 trials identified in total, due to the testing of multiple products in some studies). The products tested included several hematopoietic cell types (e.g. Natural Killer cells, virus-specific and regulatory T cells, but also mononuclear, dendritic cells, and others types of products), and in particular mesenchymal stromal/stem cells (MSCs), accounted for 147/204 products (left panel), including different subfractions of MSC product types (right panel; e.g. adipose tissue (AT-MSC; 27/147), bone marrow (BM-MSC; 22/147), and perinatal tissue (PT)-derived cells (70/147), with the latter being most abundant, which was mostly accounted for by the large number of umbilical cord (UC)-derived MSCs (58/147) products tested. (B) Clinical Trial Information, Cell Product Manufacturing and Clinical Delivery: (B1-3) Depicts information for all registered trials (n=195), including (B1) Clinical trial phase, (B2) Clinical trial sponsor, and (B3) Cell product HLA-matching, while (B4-9) Depicts information for the published MSC trials (n=24), including (B4) MSC isolation process, (B5) MSC culture process, (B6) MSC passage number, (B7) MSC culture media, (B8) MSC storage and clinical delivery, (B9) MSC dose and dosing frequency. Some displays in (B4-9) are marked with a star (*) to indicate that numbers can be greater than 24, as some published trials employed more than one method or product. Altogether, our analysis depicts a strong dominance in the data set for early stage trials (56%), employing allogeneic MSC products (76%), which were isolated by explant method (42%), cultured in 2D monolayer flasks (58%), expanded up to passage 5 (58%), and delivered intravenously (100%), mainly as cryostorage-derived freeze-thawed product (64%), and dosed below 3 million cells/kg (75%), in either one to two (46%), or even several more doses (54%), most likely to increase the total number of applied therapeutic cells over a given time frame, without increasing the individual therapeutic doses above a limit of 3 million cells/kg. Interestingly, some studies (17%) employed MSCs expanded above passage 5 up to passage 12. Many MSC products were applied thawed shortly upon retrieval from cryostorage as frozen cells (64%), which has previously been shown to compromise the therapeutic properties of clinical MSC products and may even have accounted for earlier trial failures (2, 14, 16–18, 30, 33, 87–91).
Most of the registered trials were early phase research, with at least 56% below phase 2 (Figure 3B1 and Table S1), which is probably an underestimate of early phase trials, since 15% of trials were of unknown phase. There were four phase 3 trials and four trials registered with US FDA under Expanded Access programs. The sponsors of the registered clinical trials were exclusively academic for 46% of the trials (Figure 3B2), but the remaining 54% of trials had industry support, typically from the company that manufactured the cell therapy product used in the trial. The large majority, 76% of all registered trials indicated an allogeneic product (Figure 3B3 and Table S1), while only 11% were autologous and 13% of trials did not report or define this aspect. The rationale behind the predominant use of (allogeneic) donor cells and off the shelf products is probably that the enrolled COVID-19 patients were often critically ill, and either unable to provide autologous cells (patient derived), or unable to wait for the autologous product to be manufactured.
3.3 Types of cell products in published outcomes of clinical trials
Our search to match clinical trials with reports of their outcomes identified 26 peer reviewed papers accepted for publication by the end of July 2022 (Table 3) (37–60, 92, 93). We also included a clinical trial of MSCs for ARDS that was originally registered in 2017 and published in 2021 (57). The study was included, since during the pandemic, the researchers pivoted to conduct a study of MSCs for ARDS induced by COVID-19 (38). Thus, the trial met our inclusion criteria as a published outcome of a registered clinical trial. As stated in the Methods, we excluded publications that could not be associated with registered trials, such as an extensive report on 210 patients that were treated under the approval from the Ministry of Health in Turkey (94), but not registered as a clinical trial. Our database of published trial outcomes includes two pairs of papers from two research groups in China, where in each case the group initially published a safety study (45, 53) and later published their data in a controlled trial (46, 49), respectively. The therapeutic modality reported across the published clinical trials was overwhelmingly allogeneic in all but one of the 26 published trials (96%). This confirms that those allogeneic products favored completion of trials with subsequent publication, while virtually no trials with autologous products were reported within the time frame of our database. Interestingly, many patients received cells from individual (HLA disparate) donors during each infusion in 13 of the 26 published studies (Table 4), while two studies used banks of pooled donors, but in 11 studies the donor selection is unknown. This frequent use of allogeneic products raises the issue of potential alloimmune-cross-reactivity from multiple infusions of HLA-mismatched cell products, which should be followed up in more detail in future studies. However, given the widely postulated hypoimmunogenic or immune-privileged status of MSCs, or better said the “immune-evasive nature of MSCs” (95), this critical aspect in clinical cell transplantation appeared to be of less concern in clinical trial design.
By our count, 18 of 26 published trials used cell products from a commercial entity (Table 4). Examples are proprietary cell product under development, or cells manufactured by a contract manufacturing organization (CMO), a biotech spin-off, or a cell therapy clinic. By comparison, eight studies used cells manufactured in the lab of an academic center, such as a university lab or a research hospital. This split between commercial facilities versus academic labs strongly impacts manufacturing data reporting. In the case of academic labs, manufacturing details are often available, but frequently buried in a supplement of the COVID-19 study, or in a previous paper. When authors used commercial facilities, they often did not describe cell manufacturing, simply citing that the cell products were approved for clinical use by their government. Some of the commercial entities that provided cells for COVID-19 trials have never described their cell manufacturing in any publication, so it is impossible for a reader to know how the cell product was produced and characterized. Given that MSCs were the dominant cell product in the registered cell therapy trials for COVID-19 (72% of all registered trials), it is not surprising that MSC products were employed in 24/26 (92%) of the published clinical trials, while the remaining two employed memory T-cells from convalescent donor plasma (92), or non-hematopoietic cells from peripheral blood (93). As indicated above (Figure 3A; Tables 3, S1) the sources of the MSCs in these studies were remarkably heterogeneous, with many additional variables during their manufacturing. The sources included MSCs from BM, from AT, and from various PT sources, such as from UC-derived Wharton’s Jelly, from Wharton’s Jelly plus selection for ACE2-negative cells, from the subepithelial layer of the UC after discarding the Wharton’s Jelly, from the fetal placenta, from the decidua (maternal side) of the placenta, from menstrual blood, but also MSCs derived from an embryonic cell line, and stromal cells isolated from heart tissue. The closest to a uniform group of cell types is the 11 trials that employed MSCs from Wharton’s Jelly alone without further selection.
We have summarized the cell product manufacturing for all 26 published trials in Table 4, while Figure 3B summarizes parameters for the 24 published studies on intravenous MSC therapy. Some trials employed more than one MSC product with different processing, such as fresh placenta MSCs and frozen UC MSCs (Table 3), so that for some parameters in 3B the total exceeds n=24. The 24 published studies that employed MSCs relied mainly on cell isolation by explants in nine trials (Figure 3B4 and Table 4), by enzymatic digestion in five trials, one trial combined MSCs isolated by each method, one trial alternated between MSCs isolated by each method, and the cell isolation method in the other eight MSC trials was unknown. The three studies that started with blood (either peripheral or menstrual blood), used centrifugation as their first step towards cell isolation. In the MSC trials, the cell expansion/culture process was monolayer in 14 trials (Figure 3B5 and Table 4), only one MSC trial employed a bioreactor, and not stated in nine studies. The number of passages in MSC products was reported for 18 trials and ranged from P3 to P12 with a median of P4 (Figure 3B6 and Table 4), while passages were unknown for six MSC trials. The medium used to grow MSCs for human clinical application contained fetal bovine serum (FBS) in seven of the products (Figure 3B7 and Table 4), while xeno-free medium was used in eight MSC products, and the medium formulation was unknown in nine products. Considering their storage and clinical delivery, 15 of the reported MSC trials used a previously frozen product readily derived from prior cryostorage (Figure 3B8 and Table 4). Two MSC trials used cells fresh from culture, one trial alternated between fresh or frozen MSC products, and in six trials the storage was not reported. The 24 published MSC trials all delivered MSCs by intravenous (IV) route of administration (Table 3). The cell dose was scaled by patient weight in 14 of the published clinical trials but set at a fixed dose in the remaining ten studies (Figure 3B9). For a patient weighing 70kg, the average cumulative MSC dose across all the trials was 225 million cells per patient, ranging from a minimum of 70 million to a maximum of 630 million cells per patient, thus typically 1-10 million cells/kg of patient body weight, which is the most commonly reported dose range in clinical trials involving IV delivery of MSCs (2, 16–18). Considering patient enrollment, despite the difficulty accruing patients for cell therapy trials during a pandemic with moving surges, we found that five of the 26 published trials managed to accrue more patients than the target enrollment listed in their trial registration (Table 5). The average target enrollment was 40 patients and the average achieved enrollment was 29 patients.
3.4 Published clinical trial outcomes: safety and efficacy based on RR/OR analysis
Two previous studies that connected advanced cell therapy clinical trials with their reported outcomes both found that only about 20% of these trials get published eventually (23, 96). To anticipate how many more publications of cell therapy for COVID-19 may be in preparation, we checked the status of all 195 of the 2020 and 2021 clinical trials, as of July 2022. We found that 27 (14%) of the trials had notifications that they had been cancelled, withdrawn, or terminated early. When an explanation was given for these premature endings, typical reasons stated were a lack of funding, or the inability to recruit patients. In addition to the 26 trials (13%) that have been published already, we found another 28 trials (14%) recorded as “completed”, which means that additional peer reviewed publications of cell therapy trials for COVID-19 can be anticipated (final publication rate 27%). Most of the 26 trials published so far were controlled studies: 11 were randomized controlled trials, seven trials included a control group without randomization, and eight trials had a single arm (Table 5).
We computed a meta-analysis of the survival benefit from IV MSC therapy for COVID-19. As explained above, the Relative Risk (RR) represents the ratio of the treated patients divided by the whole population (Figure 4A), while the Odds Ratio (OR) represents the ratio of the treated patients divided by the control group (Figure 4B). For the 24 published trials that employed IV MSCs, we used the survival data tabulated in Table 5 to assess the clinical efficacy of the treatment relative to controls, according to the statistical procedures described in the Methods. Initially we calculated RR and OR for all 24 studies, employing the missing data compensation described in the Methods. With this approach, there are 305 patients in the MSC treatment groups and 402 in the control groups, with 46 and 90 events (mortality), respectively. In the meta-analysis of these 24 studies, MSC therapy was associated with a diminished risk of all-cause mortality RR=0.63 [95% CI 0.46 to 0.85] (P < 0.01) or OR=0.51 [95% CI 0.33; 0.78] (P <0.01). We repeated our RR and OR calculation using only the 17 of 24 IV MSC studies that had a control arm (Figure S1). The existence of control arms means it is not necessary to perform any statistical procedures to correct for missing data. This group had a total of 237 patients in the MSC treatment group and 334 in the control group, with 35 and 73 events (mortality) reported, respectively. Here, MSC therapy was associated with a diminished risk of all-cause mortality RR=0.62 [95% CI 0.45 to 0.87], (P < 0.01) (Figure S1A) or OR=0.48 [95% CI 0.29 to 0.81] (P < 0.01) (Figure S1B). It is reassuring that the statistical results for this sub-group are almost indistinguishable from the RR and OR results of the full set of 24 studies (Table 6).
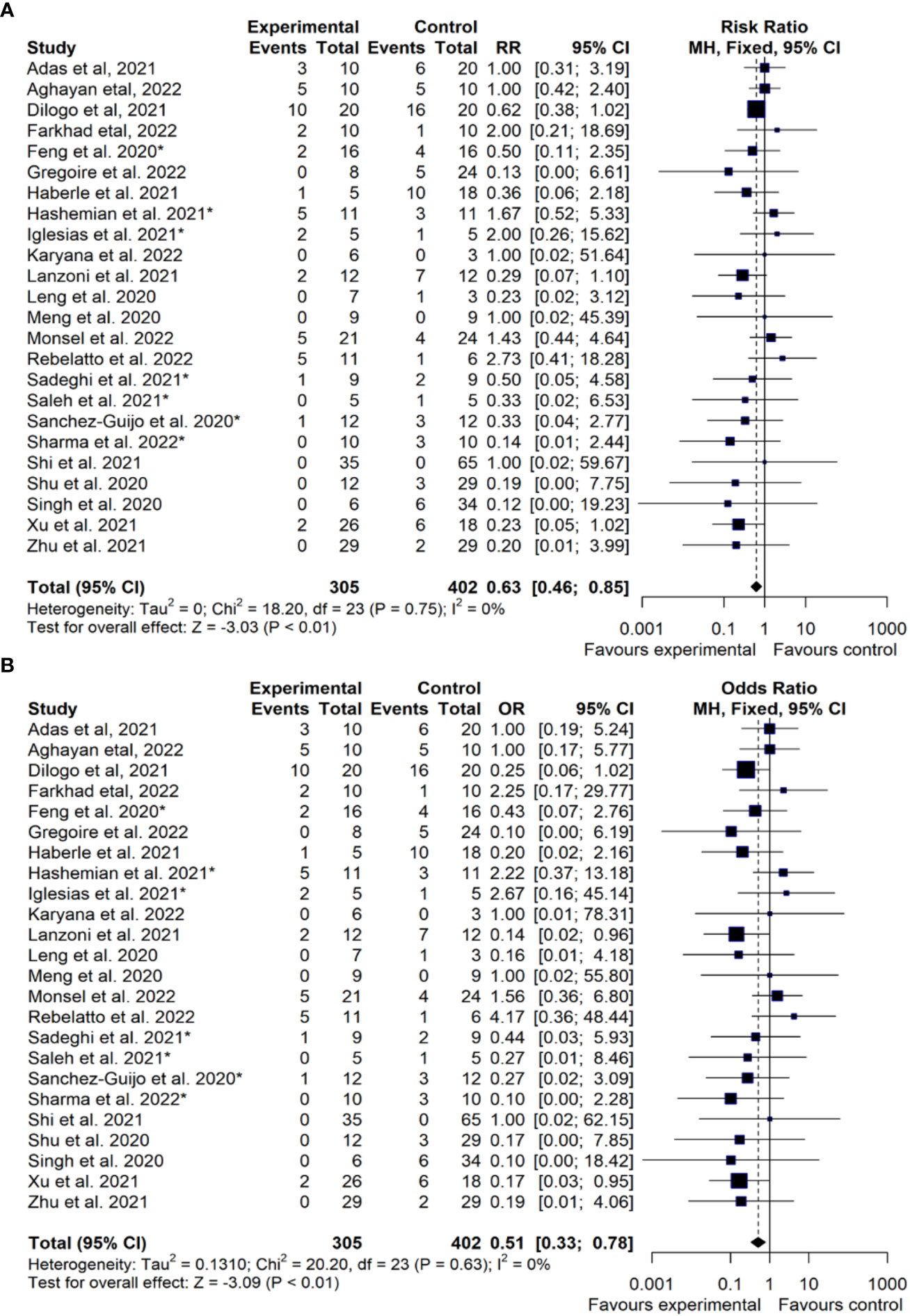
Figure 4 Treatment Efficacy of MSC Therapy for COVID-19 (RR/OR Analysis). Comparison of efficacy of mesenchymal stromal/stem cell (MSC) therapy (Experimental) vs. standard of care (Control), depicting calculations of: (A) Risk Ratio (RR) or (B) Odds Ratio (OR) for published cell therapy trials employing different types of MSC products (n=24 studies). This analysis includes MSC trials registered in the time period Jan-2020 to Dec-2021, with a follow-up period until the end of July 2022, to also detect trials published after the primary time window. The publications are sorted alphabetically according to the first author. CI, confidence interval; The asterisks (*) indicate the n=7 studies (54–60), where the values for missing controls were computed as indicated in more detail in the methods section. For the double-arm-zero-event studies (43, 46, 49) we employed a treatment arm continuity correction (TACC) to incorporate them, since otherwise RR/OR could not have been calculated (61, 62).
We have summarized the RR and OR meta-analyses for different sub-groups and compared them with previous reports that presented RR/OR survival benefit of cell therapy for COVID-19 (Table 6) (97–100). The first two previous meta-analyses in our table only used studies of IV MSC against controls, finding RR=0.54 for ten studies (97) and RR=0.50 for nine studies (98), respectively. While these two meta-analyses had very similar results, their statistical methods differed slightly. The first one included studies with no mortalities on either arm, whereas the second study excluded them. We have included studies with no mortalities by assigning them RR=1.0. A third previous meta-analysis found OR=0.24 for twelve studies (99), although we caution that their meta-analysis mixed different cell types in the statistics.
5 Discussion
To the best of our knowledge, this effort is the first report to date, that comprehensively links clinical trials of advanced cell therapy for COVID-19 with the published outcomes of those trials. This type of linkage requires that the starting database of clinical trials is as complete as possible but avoids/omits any redundancies. Thus, in the process of building the COVID-19 trials database at CellTrials.org, we have incorporated several crucial quality steps, e.g. inclusion of trials from all national registries, exclusion of false positives on keywords, and exclusion of double counting of the same trial. So far, none of the other existing trial compilations that we examined in Table 2 stated that they have employed such steps. We also must point out, that most academic studies of COVID-19 cell therapy trials ran their entire search at a single point in time and selected only for COVID-19 trials. In contrast, CellTrials.org has collected all advanced cell therapy trials monthly and then extracted the COVID-19 trials at the end of each month. In a typical month, CellTrials.org sorts through about 600 clinical trials that hit on keywords and finds that 10% qualify as advanced cell therapy trials.
The advanced cell therapy trials for COVID-19 have been conducted in 30 countries led by the US, China, Iran, and Spain, yet most resulting publications have come from China, Iran, and 11 other countries so far. The initial surge in clinical trials registered to apply cell-based therapy for treating COVID-19 peaked in April 2020 and subsided into an ongoing effort of a few new trials per month. It must be noted that many healthcare policies at both national and local levels influence the ability to launch trials and recruit COVID-19 patients for cell-based therapies. Despite ongoing outbreaks in the US, we have noted that multiple trials have been suspended because they cannot recruit enough patients at a single hospital. Thus, large research consortia with multi-institutional and multi-national collaboration are needed to tackle this shortcoming and more rapidly develop new treatment approaches for COVID-19. In China, the “Zero COVID” policy was so efficient at suppressing outbreaks for two years, that clinical trials had stopped because they could not accrue patients (54). Since this policy was changed in late 2022, new infections and probably also associated severe cases and deaths due COVID-19 are likely to have surged dramatically (101), with a need for effective treatments.
In many Western countries (e.g. Europe being subject to both national and EMA regulation, with considerable variability in regulation between different European nations) (2, 24), strict regulations on human cell therapy mean there were only a limited number of cell therapy products with established safety profiles that could be trialed. While we found that 56% of the trials were below phase 2, we found that 69% of the published outcomes were studies with a control arm. The fraction of the trials employing MSC was 72%, but 92% of the published outcomes are studies that relied on MSCs. The mechanisms of action by which ARDS and COVID-19 patients may benefit from MSC therapy have been exhaustively reviewed by the papers listed in Tables 2, 3 (37–53, 63, 64, 66–86, 97–100), such as relying on multiple synergistic effector mechanisms, and promoting/triggering beneficial immunomodulatory and regenerative pathways, as well as angiogenesis and antiapoptosis (18). Hence, we will not repeat that discussion here, but only refer to the most crucial key observations in the discussion further below. Most importantly, in this study, we have also endeavored to quantitatively calculate the safety and efficacy profile of MSC infusions as a novel treatment for COVID-19. However, efforts to treat these topics systematically appear to be fraught with difficulties.
On the issue of MSC safety, we found that all of the 24 published trials claimed that they had no severe adverse events related to the MSC infusion (37–60). Many of the studies gave anti-coagulant therapy as a prophylaxis (38, 42, 44, 56, 57, 60). Already in the first reports from the COVID-19 epicenter in Wuhan, and swiftly following global reports, severe coagulopathy was identified as one of the most evident complications arising from SARS-CoV2 infection and critical/severe COVID-19 (102–112). The increased incidence of thrombotic complications in these patients was verified in large population studies in Sweden (110, 111). Indeed, MSC-IV therapeutics carry a risk of thrombotic complications, due to their expression of the highly prothrombotic tissue factor (TF/CD142) (2, 16, 17). Hence, since the first months of the pandemic, we have identified prophylaxis against coagulation as a crucial factor for the safe application of IV MSC therapeutics in a COVID-19 setting (2, 14, 15). We were poised to highlight the safety aspect of IV MSC therapy for COVID-19, but we discovered that none of the published trials had conducted monitoring that could address this issue. For example, in one trial that treated patients suffering from severe COVID-19 in a hospital ICU, a patient that received IV MSC therapy died 13 days later following an arterial thrombosis (56). This event was considered unrelated to MSC therapy because it occurred outside their four-hour window of post-infusion monitoring.
This was common in the 24 publish trials: possible complications arising from MSC infusion were only monitored during or shortly after infusion. Yet, case studies of adverse events associated with MSC infusions found that elevated clotting markers (e.g. TAT and D-Dimer) typically peaked 9-12 hours post-MSC infusion (17), while pulmonary (but not arterial) embolism post MSC therapy could be detected days, weeks, and even months after treatment (17, 113, 114). This should caution us that the impact of the instant blood-mediated inflammatory reaction (IBMIR) post IV MSC therapy for COVID-19 may only become evident at later time points (2, 16, 17). Given that thromboembolism is a well-known side effect of either COVID-19 infection or MSC infusions, it is currently not possible to retrospectively assign that complication to one or the other group (17), when these studies were not constructed to discriminate the potential causes. Thus, we did not attempt to compute the rate of adverse events in the published trials because the few observed/reported events are largely anecdotal in nature and cannot be quantified at the current stage. Hopefully, future clinical trials will incorporate longer monitoring periods and will have large enough patient groups to statistically identify if any adverse events were increased in response to the experimental (MSC) treatment but not in response to the standard of care.
The primary theme of our analysis was to calculate the efficacy of MSC infusions as a therapy for COVID-19 based on the risk of mortality. The mortality data represent a quantitative set of facts that were extracted from each of the published clinical trials and are listed in Table 5. However, we must caution that not all these trials were designed with survival as an endpoint. For example, one study recruited convalescent patients to determine if MSC infusions would speed up the resolution of their lung lesions and consequently none of the patients died on either arm (49). The previous meta-analyses have also noted that published studies with intravenous use of MSC for COVID-19 have very heterogeneous patient populations, in terms of the severity of their COVID-19 illness as well as the forms of concomitant therapies that they received (97–100). Our statistical model has included more studies and more patients than previous meta-analyses of MSC efficacy for COVID-19 (97–100). We corroborate the conclusions of the previous meta-analyses, that intravenous MSC therapy appears to provide a benefit for the treatment of COVID-19 (97–100). Recently, earlier timing of MSC infusion has emerged as a new factor that may be associated with improved survival for patients receiving MSC therapy for COVID-19 (115). This was established in a single center study that gave an IV MSC dose 3 x 3 million cells/kg. We note that their cumulative dose is about three times higher than the average in our 24 studies of IV infused MSCs. We do find that most published studies (17 out of 24) spread out clinical MSC delivery over two to four doses.
Perinatal sources of MSCs (e.g. tissue of UC and/or placenta, collectively called PT-MSCs) (16, 17), were employed in 18 of 24 published trials (Table 3). Despite a great deal of preclinical and clinical research, there are still conflicting opinions on the biological characteristics of MSCs isolated from perinatal versus adult sources. Multiple earlier studies suggested that PT-MSCs may have superior immunomodulatory properties (116–123), higher proliferation (117, 124), and richer secretome (120, 125–127), compared to adult sources. In turn, some studies also reported that adult sources of MSCs have superior or similar immunomodulatory ability (126, 128, 129), and that adult MSCs have higher production rates of several vital molecular mediators [e.g. VEGF (126, 127), PLGF (126), IL-10 (130), and TGF-β1 (130)] when compared to perinatal cells. Intriguingly, when we performed meta-analysis for subgroups of our data, we found that the RR of the six studies with non-perinatal MSCs was more than factor two better than the RR of the 18 perinatal studies (Table 6). All six of the non-perinatal studies, which employed five different MSC products, reported good outcomes. In contrast, among the 18 perinatal studies there were five studies where mortality on the MSC treatment arm was higher than the control baseline (40, 47, 48, 55, 56). However, this RR comparison was not statistically significant, leading us to argue that more data is needed to confirm or refute this result.
Another focus of our study was to evaluate the diversity of manufacturing methods used to prepare MSC products and to highlight the importance of reporting manufacturing information to enable study comparability (Figure 3; Tables 3, 4). Indeed, diversity in cell product manufacturing parameters, cell dosing, and cell characterization for therapeutic use, but also the completeness of study descriptors, have all been identified as a potential confounder to interpretation of safety and efficacy outcomes in MSC studies and should be monitored/reported more thoroughly in future studies (2, 16, 17, 33, 131–133). Recent reviews (131, 132) have highlighted the frequent lack in reporting of MSC manufacturing and study descriptors as a considerable shortcoming to clinical trial reporting and subsequent study interpretation. This aspect is of such importance, that it has been taken up into: “A modified Delphi Study Protocol” for “Establishment of a Consensus Definition for Mesenchymal Stromal Cells (MSC) and Reporting Guidelines for Clinical Trials of MSC Therapy” (133). For example, one multi-center randomized control trial (RCT) that employed UC-MSCs to treat COVID-19 found that MSC therapy had no efficacy (47). However, the MSCs in that study were manufactured in a laboratory that alternated between cell isolation with explants vs. enzymatic digestion (which may impact cell yield and immunophenotype) (134, 135). Still, the research consortium did not publish any records of which patient received which MSC product (47). Consequently, the outcome of the entire multi-center RCT may be cast into doubt because it is unknown how manufacturing variability may have compromised the respective results. Another manufacturing issue, which has been largely overlooked in the discussion of cell therapy trials for COVID-19 so far, is the ability to scale up the production of successful therapies (31, 136–139). Most trials published so far have relied on 2D monolayer cell expansion methods in flasks, with only one study employing a hollow-fiber bioreactor (42). The monolayer methods are time-consuming, labor intensive, and have limited scalability (135, 140, 141). Given the potential combinatorial detrimental impact of both freeze-thawing and in vitro aging on cell potency (33, 87–91, 135), it is of importance to accurately evaluate the number of population doublings a cell product has accumulated during ex vivo culture expansion for COVID-19 therapy and other indications (2, 14, 16). Of course, it is also relevant to record if the cell product was given either fresh or as a freeze-thawed product derived from cryostorage, since this may impact substantially on the product performance (14, 30, 31, 33, 88–91, 142–144).
6 Conclusions and limitations
The International Society for Cell and Gene Therapy (ISCT) recently published an editorial calling for a global registry of clinical trials that employ MSCs for COVID-19 to harmonize the data on the limited number of patients and “To collect information on critical process parameters used to manufacture the MSCs” (145). We support that call to action. Our review of the manufacturing parameters in clinical trials giving cell-based therapy for COVID-19 has revealed a partial disconnect between clinical centers that treat patients versus laboratories that manufacture cell therapy products. Frequently, the clinicians running the trials have acquired cells and delivered them to patients without keeping any records about the cell production. This disconnect could be closed, if the clinical trials participated in a global registry that required completing standardized categories of information. In this study we have compiled two years of worldwide clinical trials testing cell-based therapies for COVID-19 and linked those trials to their published outcomes. This “end-to-end” survey of the research field has enabled us to learn new insights not published earlier. First, we found that global registrations of advanced cell-based therapies for COVID-19 were more numerous than previously reported, but that they experienced only one single early surge in trial registrations during a time frame, when global COVID-19 infections went through multiple surges. Our analysis also includes the contribution from registered clinical trials that are not listed on the national registries of the United States and China, with 53 and 43 trials, respectively. Hereby, we have learned that Iran (19 trials) is among the three leading nations running advanced cell therapy trials for COVID-19 and the 2nd in publishing trial outcomes. In turn, Israel, Spain, Iran, Australia, and Sweden are leading in relative contributions to COVID-19 cell therapy trials normalized to population size (N=0.641, 0.232, 0,223, 0.194, and 0.192 trials per million inhabitants).
Although 72% of the COVID-19 cell therapy trials employed tissue-derived MSCs, a significant fraction of clinical trials conducted immunotherapy with blood-derived cells. So far, most of the published trials describe infusions of MSCs, and 75% of those employed MSCs derived from perinatal tissue sources. Throughout these studies there is a strong theme of heterogeneity. The patient groups in the clinical trials are heterogeneous, as are the manufacturing methods used to prepare the MSCs. Most importantly, our statistical analysis shows that infusions of MSCs show a clinical benefit for COVID-19 patients. The risk ratio for all-cause mortality is RR=0.62 [95% CI 0.44 to 0.87] for the 17 MSC studies with control arms, and when we compensated for the missing controls and incorporated all 24 MSC studies the result is RR=0.63 [95% CI 0.46 to 0.85]. Early during the COVID-19 pandemic, it emerged that the respiratory distress caused by COVID-19 is a substantially different clinical entity compared to classic ARDS (21). Hence, we cannot assume that the efficacy results obtained here will similarly influence the long-standing quest to improve ARDS mortality. For this answer, we must await the outcomes of large placebo-controlled randomized trials of MSCs for ARDS, such as the REALIST trial in the UK, and the STAT trial in the US, respectively (146, 147). We close with the concern that there may never be enough data to fully explore the efficacy of cell-based therapy against ARDS from COVID-19. Owing to the evolution of the virus to less lethal variants and the rollout of vaccination, it has recently become difficult to accrue patients for clinical trials that treat severe symptoms of COVID-19 infection. The relative impact of MSC product source, MSC dosing, and the timing and type of MSC delivery, etc., may never be fully explored or known within the current setting of COVID-19.
From a public health perspective, the highest goal is to prevent the development of severe or critical COVID-19 through combined effective pandemic countermeasures (Figure 1A) (148). In this regard, vaccination is the most valuable tool available. Also, the standard-of-care for the treatment of severe and critical COVID-19 is continuously improving. Although we here found that infusions of MSCs confer a reduction in the risk for all-cause-mortality from COVID-19 in the studies published to date, more research is needed to clarify this point. Nonetheless, there will always be high-risk patients who develop severe or critical COVID-19, and for them the existence of adjunct treatment with advanced cell therapy may be beneficial. The target groups for whom this therapy may provide benefit include the elderly, immunocompromised individuals, cancer patients, and transplant patients (both stem cell transplants and solid organ transplants) as well as patients with kidney failure on dialysis (17, 149, 150). More research on the efficacy of advanced cell therapy for COVID-19 will reveal the degree to which these groups may benefit. Eventually, the cost and access to advanced cell therapy must also be anticipated, typically requiring advanced medical infrastructure. It is our hope that the testing of advanced therapies will be pursued in parallel to the improvement of standard care.
Data availability statement
The original contributions presented in the study are included in the article/Supplementary Material. Further inquiries can be directed to the corresponding authors.
Author contributions
PC and GM and FV conceived and designed this study. PC and AB and FV conducted primary data collection. PC and NA-A and IF and DF and RC and OC-M and GM and FV conducted the primary data analysis. PC and GM and FV wrote the first draft of the article. IH and RAC and OC-M. complemented and revised the manuscript and provided resources to support the study. All authors contributed to the article and approved the submitted version.
Funding
PC and FV were supported by donations to Parent’s Guide to Cord Blood® Foundation (non-profit). GM’s and RAC’s and NA-A’s contributions were made possible by the German Research Foundation (DFG; EXPAND-PD CA2816/1-1 to GM and RAC) and German Federal Ministry for Education and Research (BMBF) funding through the Berlin Institute of Healthy (BIH)-Center for Regenerative Therapies (BCRT) and the Berlin-Brandenburg School for Regenerative Therapies (BSRT, GSC203), respectively, and in part by the European Union’s Horizon 2020 Research and Innovation Program under grant agreements No 733006 (PACE) and 779293 (HIPGEN) and 754995 (EU-TRAIN). IH and NA-A were furthermore supported by the BIH and Stiftung Charité (Grant AdjTh-pAVK). We also like to thank the São Paulo Research Foundation (FAPESP grants 2018/18886-9, 2020/01688-0, and 2020/07069-0 to OC-M; and grant 2020/16246-2 to DF).
Acknowledgments
We acknowledge support from Frontiers Immunology and the DFG and the Open Access Publication Fund of the BIH and the Charité – Universitätsmedizin Berlin.
Conflict of interest
The authors declare that the research was conducted in the absence of any commercial or financial relationships that could be construed as a potential conflict of interest.
Publisher’s note
All claims expressed in this article are solely those of the authors and do not necessarily represent those of their affiliated organizations, or those of the publisher, the editors and the reviewers. Any product that may be evaluated in this article, or claim that may be made by its manufacturer, is not guaranteed or endorsed by the publisher.
Supplementary material
The Supplementary Material for this article can be found online at: https://www.frontiersin.org/articles/10.3389/fimmu.2023.1200180/full#supplementary-material
Supplementary Figure 1 | Treatment Efficacy of MSC Therapy for COVID-19 (RR/OR Analysis) controlled studies only (Analysis Supplemental to Part A).
References
1. Piret J, Boivin G. Pandemics throughout history. Front Microbiol (2021) 11. doi: 10.3389/fmicb.2020.631736
2. Moll G, Drzeniek N, Kamhieh-Milz J, Geissler S, Volk H-D, Reinke P. MSC therapies for COVID-19: importance of patient coagulopathy, thromboprophylaxis, cell product quality and mode of delivery for treatment safety and efficacy. Front Immunol (2020) 11:1091. doi: 10.3389/fimmu.2020.01091
3. Wölfel R, Corman VM, Guggemos W, Seilmaier M, Zange S, Müller MA, et al. Virological assessment of hospitalized patients with COVID-2019. Nature (2020) 581:465–9. doi: 10.1038/s41586-020-2196-x
4. Word-Health-Organization (WHO). Report of the WHO-China joint mission on coronavirus disease 2019 (COVID-19). Available at: https://www.who.int/publications-detail/report-of-the-who-china-joint-mission-on-coronavirus-disease-2019-(covid-19) (Accessed 2020-03-11).
5. COVID-19 Dashboard. Center for systems science and engineering (CSSE). Johns Hopkins University (JHU (2022). Available at: https://coronavirus.jhu.edu/map.html.
6. COVID Worldometer. Available at: https://www.worldometers.info/coronavirus/ (Accessed 2022-12-20).
7. World-Health-Organization (WHO). Tracking SARS-CoV-2 variants. Available at: https://www.who.int/activities/tracking-SARS-CoV-2-variants (Accessed 2022-12-20).
8. Prado C, Fonseca DLM, Singh Y, Filgueiras IS, Baiocchi GC, Plaça DR, et al. Integrative systems immunology uncovers molecular networks of the cell cycle that stratify COVID-19 severity. J Med Virol (2023) 95(2):e28450. doi: 10.1002/jmv.28450
9. Carabelli AM, Peacock TP, Thorne LG, Harvey WT, Hughes J, COVID-19 Genomics UK Consortium, et al. SARS-CoV-2 variant biology: immune escape, transmission and fitness. Nat Rev Microbiol (2023) 21(3):162–77 doi: 10.1038/s41579-022-00841-7
10. Mallapaty S, Badia JR, Adhikari NK, Friedrich JO, Fowler RA, Singh JM. Where did omicron come from? three key theories. Nature (2022) 602:26–8. doi: 10.1038/d41586-022-00215-2
11. European Centre for Disease Prevention and Control (ECDC). Spread of the SARS-CoV-2 omicron variant sub-lineage BQ.1 in the EU/EEA (2022). Available at: https://www.ecdc.europa.eu/en/publications-data/spread-sars-cov-2-omicron-variant-sub-lineage-bq1-eueea.
12. Phua J, Badia JR, Adhikari NK, Friedrich JO, Fowler RA, Singh JM, et al. Has mortality from acute respiratory distress syndrome decreased over time?: a systematic review. Am J Respir Crit Care Med (2009) 179(3):220–7. doi: 10.1164/rccm.200805-722OC
13. Pittenger MF, Discher DE, Péault BM, Phinney DG, Hare JM, Caplan AI, et al. Mesenchymal stem cell perspective: cell biology to clinical progress. NPJ Regen Med (2019) 4:22. doi: 10.1038/s41536-019-0083-6
14. Moll G, Hoogduijn MJ, Ankrum JA. Editorial: safety, efficacy and mechanisms of action of mesenchymal stem cell therapies. Front Immunol (2020) 11:243. doi: 10.3389/fimmu.2020.00243
15. Caplan H, Olson SD, Kumar A, George M, Prabhakara KS, Wenzel P, et al. Mesenchymal stromal cell therapeutic delivery: translational challenges to clinical application. Front Immunol (2019) 10:1645. doi: 10.3389/fimmu.2019.01645
16. Moll G, Ankrum JA, Kamhieh-Milz J, Bieback K, Ringdén O, Volk HD, et al. Intravascular mesenchymal Stromal/Stem cell therapy product diversification: time for new clinical guidelines. Trends Mol Med (2019) 25(2):149–63. doi: 10.1016/j.molmed.2018.12.006
17. Moll G, Ankrum JA, Olson SD, Nolta JA. Improved MSC minimal criteria to maximize patient safety: a call to embrace tissue factor and hemocompatibility assessment of MSC products. Stem Cells Trans Med (2022) 11:2–13. doi: 10.1093/stcltm/szab005
18. Ringdén O, Moll G, Gustafsson B, Sadeghi B. Mesenchymal stromal cells for enhancing hematopoietic engraftment and treatment of graft-versus-Host disease, hemorrhages and acute respiratory distress syndrome. Front Immunol (2022) 13:839844. doi: 10.3389/fimmu.2022.839844
19. Verter F. The role of MSC to treat coronavirus pneumonia and ARDS. part 1: is the emperor wearing clothes? Available at: https://celltrials.org/news/role-msc-treat-coronavirus-pneumonia-and-ards-part-1-is-emperor-wearing-clothes (Accessed 2020-04-25).
20. Qu W, Wang Z, Hare JM, Bu G, Mallea JM, Pascual JM, et al. Cell-based therapy to reduce mortality from COVID-19: systematic review and meta-analysis of human studies on acute respiratory distress syndrome. Stem Cells Trans Med (2020) 9(9):1007–22. doi: 10.1002/sctm.20-0146
21. Gattinoni L, Marini JJ. Isn’t it time to abandon ARDS? the COVID-19 lesson. Crit Care (2021) 25:326. doi: 10.1186/s13054-021-03748-6
22. Couto PS, Bersenev A, Verter F. The first decade of advanced cell therapy clinical trials using perinatal cells (2005-2015). Regen Med (2017) 12:953–68. doi: 10.2217/rme-2017-0066
23. Couto PS, Shatirishvili G, Bersenev A, Verter F. First decade of clinical trials and published studies with mesenchymal stromal cells from umbilical cord tissue. Regen Med (2019) 14:309–19. doi: 10.2217/rme-2018-0171
24. Goldsobel G, von Herrath C, Schlickeiser S, Brindle N, Stähler F, Reinke P, et al. RESTORE survey on the public perception of advanced therapies and ATMPs in Europe-why the European union should invest more! Front Med (Lausanne) (2021) 8:739987. doi: 10.3389/fmed.2021.739987
25. EMA regulation (EC) no 1394/2007 of the European parliament and of the council of 13 November 2007 on advanced therapy medicinal products and amending directive 2001/83/EC and regulation (EC) no 726/2004 (1). off J eur union. 2007 (2022). Available at: http://eur-lex.europa.eu/legal-content/EN/TXT/?uri=uriserv:OJ.L_.2007.324.01.0121.01.ENG&toc=OJ:L:2007:324:TOC.
26. FDA FDA Guidance Document. Regulatory considerations for human cells, tissues, and cellular and tissue-based products: minimal manipulation and homologous use (2020). Available at: https://www.fda.gov/regulatory-information/search-fda-guidance-documents/regulatory-considerations-human-cells-tissues-and-cellular-and-tissue-based-products-minimal.
27. Center for Science in the Public Interest. COVID-19 evidence hub (2020). Available at: https://www.cspinet.org/covid-19-evidence-hub (Accessed 2022-12-20).
28. Becker RA, Wilks AR, Minka TP, Deckmyn A. Maps: draw georaphical maps. r package version 3.4.1. Available at: https://CRAN.R-project.org/package=maps (Accessed 2022-10-30).
29. Wickham H. ggplot2: elegant graphics for data analysis. Springer-Verlag New York (2016). Available at: https://ggplot2.Pdyverse.org.
30. Moll G, Geißler S, Catar R, Ignatowicz L, Hoogduijn MJ, Strunk D, et al. Cryopreserved or fresh mesenchymal stromal cells: only a matter of taste or key to unleash the full clinical potential of MSC therapy? Adv Exp Med Biol (2016) 951:77–98. doi: 10.1007/978-3-319-45457-3_7
31. Cottle C, Porter AP, Lipat A, Turner-Lyles C, Nguyen J, Moll G, et al. Impact of cryopreservation and freeze-thawing on therapeutic properties of mesenchymal Stromal/Stem cells and other common cellular therapeutics. Curr Stem Cell Rep (2022) 8(2):72–92. doi: 10.1007/s40778-022-00212-1
32. Dominici M, Le Blanc K, Mueller I, Slaper-Cortenbach I, Marini F, Krause D, et al. Minimal criteria for defining multipotent mesenchymal stromal cells. the international society for cellular therapy position statement. Cytotherapy (2006) 8(4):315–7. doi: 10.1080/14653240600855905
33. Moll G, Le Blanc K. Engineering more efficient multipotent mesenchymal stromal (stem) cells for systemic delivery as cellular therapy. ISBT Sci Ser (2015) 10:357–65. doi: 10.1111/voxs.12133
34. R Core Team. R: A language and environment for statistical computing. Vienna: R Foundation for Statistical Computing. (2021). Available at: https://www.R-project.org.
35. Balduzzi S, Rücker G, Schwarzer G. How to perform a meta-analysis with r: a practical tutorial. Evid Based Ment Health (2019) 22:153–60. doi: 10.1136/ebmental-2019-300117
36. Mantel N, Haenszel W. Statistical aspects of the analysis of data from retrospective studies of disease. J Natl Cancer Inst (1959) 22(4):719–48.
37. Adas G, Cukurova Z, Yasar KK, Yilmaz R, Isiksacan N, Kasapoglu P, et al. The systematic effect of mesenchymal stem cell therapy in critical COVID-19 patients: a prospective double controlled trial. Cell Transplant (2021) 30:9636897211024942. doi: 10.1177/09636897211024942
38. Aghayan HR, Salimian F, Abedini A, Fattah Ghazi S, Yunesian M, Alavi-Moghadam S, et al. Human placenta-derived mesenchymal stem cells transplantation in patients with acute respiratory distress syndrome (ARDS) caused by COVID-19 (phase I clinical trial): safety profile assessment. Stem Cell Res Ther (2022) 13(1):365. doi: 10.1186/s13287-022-02953-6
39. Dilogo IH, Aditianingsih D, Sugiarto A, Burhan E, Damayanti T, Sitompul PA, et al. Umbilical cord mesenchymal stromal cells as critical COVID-19 adjuvant therapy: a randomized controlled trial. Stem Cells Trans Med (2021) 10(9):1279–87. doi: 10.1002/sctm.21-0046
40. Kaffash Farkhad N, Sedaghat A, Reihani H, Adhami Moghadam A, Bagheri Moghadam A, Khadem Ghaebi N, et al. Mesenchymal stromal cell therapy for COVID-19-induced ARDS patients: a successful phase 1, control-placebo group, clinical trial. Stem Cell Res Ther (2022) 13(1):283. doi: 10.1186/s13287-022-02920-1
41. Grégoire C, Layios N, Lambermont B, Lechanteur C, Briquet A, Bettonville V, et al. Bone marrow-derived mesenchymal stromal cell therapy in severe COVID-19: preliminary results of a phase I/II clinical trial. Front Immunol (2022) 13:932360. doi: 10.3389/fimmu.2022.932360
42. Häberle H, Magunia H, Lang P, Gloeckner H, Körner A, Koeppen M, et al. Mesenchymal stem cell therapy for severe COVID-19 ARDS. J Intensive Care Med (2021) 36(6):681–8. doi: 10.1177/0885066621997365
43. Karyana M, Djaharuddin I, Rif'ati L, Arif M, Choi MK, Angginy N, et al. Safety of DW-MSC infusion in patients with low clinical risk COVID-19 infection: a randomized, double-blind, placebo-controlled trial. Stem Cell Res Ther (2022) 13(1):134. doi: 10.1186/s13287-022-02812-4
44. Lanzoni G, Linetsky E, Correa D, Messinger Cayetano S, Alvarez RA, Kouroupis D, et al. Umbilical cord mesenchymal stem cells for COVID-19 acute respiratory distress syndrome: a double-blind, phase 1/2a, randomized controlled trial. Stem Cells Trans Med (2021) 10(5):660–73. doi: 10.1002/sctm.20-0472
45. Leng Z, Zhu R, Hou W, Feng Y, Yang Y, Han Q, et al. Transplantation of ACE2(-) mesenchymal stem cells improves the outcome of patients with COVID-19 pneumonia. Aging Dis (2020) 11(12):216–28. doi: 10.14336/AD.2020.0228
46. Meng F, Xu R, Wang S, Xu Z, Zhang C, Li Y, et al. Human umbilical cord-derived mesenchymal stem cell therapy in patients with COVID-19: a phase 1 clinical trial. Signal Transduct Target Ther (2020) 5(1):172. doi: 10.1038/s41392-020-00286-5
47. Monsel A, Hauw-Berlemont C, Mebarki M, Heming N, Mayaux J, Nguekap Tchoumba O, et al. Treatment of COVID-19-associated ARDS with mesenchymal stromal cells: a multicenter randomized double-blind trial. Crit Care (2022) 26(1):48. doi: 10.1186/s13054-022-03930-4
48. Rebelatto CLK, Senegaglia AC, Franck CL, Daga DR, Shigunov P, Stimamiglio MA, et al. Safety and long-term improvement of mesenchymal stromal cell infusion in critically COVID-19 patients: a randomized clinical trial. Stem Cell Res Ther (2022) 13(1):122. doi: 10.1186/s13287-022-02796-1
49. Shi L, Huang H, Lu X, Yan X, Jiang X, Xu R, et al. Effect of human umbilical cord-derived mesenchymal stem cells on lung damage in severe COVID-19 patients: a randomized, double-blind, placebo-controlled phase 2 trial. Signal Transduct Target Ther (2021) 6(1):58. doi: 10.1038/s41392-021-00488-5
50. Shu L, Niu C, Li R, Huang T, Wang Y, Huang M, et al. Treatment of severe COVID-19 with human umbilical cord mesenchymal stem cells. Stem Cell Res Ther (2020) 11(1):361. doi: 10.1186/s13287-020-01875-5
51. Singh S, Chakravarty T, Chen P, Akhmerov A, Falk J, Friedman O, et al. Allogeneic cardiosphere-derived cells (CAP-1002) in critically ill COVID-19 patients: compassionate-use case series. Basic Res Cardiol (2020) 115(4):36. doi: 10.1007/s00395-020-0795-1
52. Xu X, Jiang W, Chen L, Xu Z, Zhang Q, Zhu M, et al. Evaluation of the safety and efficacy of using human menstrual blood-derived mesenchymal stromal cells in treating severe and critically ill COVID-19 patients: an exploratory clinical trial. Clin Trans Med (2021) 11(2):e297. doi: 10.1002/ctm2.297
53. Zhu R, Yan T, Feng Y, Liu Y, Cao H, Peng G, et al. Mesenchymal stem cell treatment improves outcome of COVID-19 patients via multiple immunomodulatory mechanisms. Cell Res (2021) 31(12):1244–62. doi: 10.1038/s41422-021-00573-y
54. Feng Y, Huang J, Wu J, Xu Y, Chen B, Jiang L, et al. Safety and feasibility of umbilical cord mesenchymal stem cells in patients with COVID-19 pneumonia: a pilot study. Cell Prolif (2020) 53(12):e12947. doi: 10.1111/cpr.12947
55. Hashemian SR, Aliannejad R, Zarrabi M, Soleimani M, Vosough M, Hosseini SE, et al. Mesenchymal stem cells derived from perinatal tissues for treatment of critically ill COVID-19-induced ARDS patients: a case series. Stem Cell Res Ther (2021) 12(1):91. doi: 10.1186/s13287-021-02165-4
56. Iglesias M, Butrón P, Torre-Villalvazo I, Torre-Anaya EA, Sierra-Madero J, Rodriguez-Andoney JJ, et al. Mesenchymal stem cells for the compassionate treatment of severe acute respiratory distress syndrome due to COVID 19. Aging Dis (2021) 12(2):360–70. doi: 10.14336/AD.2020.1218
57. Sadeghi B, Roshandel E, Pirsalehi A, Kazemi S, Sankanian G, Majidi M, et al. Conquering the cytokine storm in COVID-19-induced ARDS using placenta-derived decidua stromal cells. J Cell Mol Med (2021) 25(22):10554–64. doi: 10.1111/jcmm.16986
58. Saleh M, Vaezi AA, Aliannejad R, Sohrabpour AA, Kiaei SZF, Shadnoush M, et al. Cell therapy in patients with COVID-19 using wharton’s jelly mesenchymal stem cells: a phase 1 clinical trial. Stem Cell Res Ther (2021) 12(1):410. doi: 10.1186/s13287-021-02483-7
59. Sánchez-Guijo F, García-Arranz M, López-Parra M, Monedero P, Mata-Martínez C, Santos A, et al. Adipose-derived mesenchymal stromal cells for the treatment of patients with severe SARS-CoV-2 pneumonia requiring mechanical ventilation. a proof of concept study. EClinicalMedicine (2020) 25:100454. doi: 10.1016/j.eclinm.2020.100454
60. Sharma A, Kulkarni R, Sane H, Awad N, Bopardikar A, Joshi A, et al. Phase 1 clinical trial for intravenous administration of mesenchymal stem cells derived from umbilical cord and placenta in patients with moderate COVID-19 virus pneumonia: results of stage 1 of the study. Am J Stem Cells (2022) 11(3):37–55.
61. Ren Y, Lin L, Lian Q, Zou H, Chu H. Real-world performance of meta-analysis methods for double-Zero-Event studies with dichotomous outcomes using the cochrane database of systematic reviews. J Gen Intern Med (2019) 34:960–8. doi: 10.1007/s11606-019-04925-8
62. Sweeting MJ, Sutton AJ, Lambert PC. What to add to nothing? use and avoidance of continuity corrections in meta-analysis of sparse data. Stat Med (2004) 23:1351–75. doi: 10.1002/sim.1761
63. Verter F, Couto PS. Development of COVID-19 therapies from birthing tissues and cord blood. Stem Cells Trans Med (2020) 9:S15–5. doi: 10.1002/sctm.12818
64. Verter F, Couto PS. Update: development of COVID-19 therapies from birthing tissues and cord blood. Stem Cells Trans Med (2021) 10:S9–9. doi: 10.1002/sct3.13002
65. Mathieu E, Ritchie H, Ortiz-Ospina E, Roser M, Hasell J, Appel C, et al. A global database of COVID-19 vaccinations. Nat Hum Behav (2021) 5(7):947–53. doi: 10.1038/s41562-021-01122-8
66. Grumet M, Sherman J, Dorf BS. Efficacy of MSC in patients with severe COVID-19: analysis of the literature and a case study. Stem Cells Trans Med (2022) 11:1103–12. doi: 10.1093/stcltm/szac067
67. Lu K, Geng ST, Tang S, Yang H, Xiong W, Xu F, et al. Clinical efficacy and mechanism of mesenchymal stromal cells in treatment of COVID-19. Stem Cell Res Ther (2022) 13(1):61. doi: 10.1186/s13287-022-02743-0
68. Zaki MM, Lesha E, Said K, Kiaee K, Robinson-McCarthy L, George H, et al. Cell therapy strategies for COVID-19: current approaches and potential applications. Sci Adv (2021). 7(33):eabg5995 doi: 10.1126/sciadv.abg5995
69. Khoury M, Ikonomou L, Dominici M, LeBlanc K, Levine BL, Weiss DJ, et al. The coronavirus pandemic: a pitfall or a fast track for validating cell therapy products? Stem Cells Dev (2021) 30(3):119–27. doi: 10.1089/scd.2020.0122
70. Li Z, Niu S, Guo B, Gao T, Wang L, Wang Y, et al. Stem cell therapy for COVID-19, ARDS and pulmonary fibrosis. Cell Prolif (2020) 53(12):e12939. doi: 10.1111/cpr.12939
71. Kim M, Knoepfler PS. Anticipated impact of stem cell and other cellular medicine clinical trials for COVID-19. Regen Med (2021) 16:525–33. doi: 10.2217/rme-2021-0025
72. Golchin A. Cell-based therapy for severe COVID-19 patients: clinical trials and cost-utility. Stem Cell Rev Rep (2021) 17:56–62. doi: 10.1007/s12015-020-10046-1
73. Choudhery MS, Harris DT. Stem cell therapy for COVID-19: possibilities and challenges. Cell Biol Int (2020) 44:2182–91. doi: 10.1002/cbin.11440
74. Shetty R, Murugeswari P, Chakrabarty K, Jayadev C, Matalia H, Ghosh A, et al. Stem cell therapy in coronavirus disease 2019: current evidence and future potential. Cytotherapy (2021) 23(6):471–82. doi: 10.1016/j.jcyt.2020.11.001
75. Market M, Angka L, Martel AB, Bastin D, Olanubi O, Tennakoon G, et al. Flattening the COVID-19 curve with natural killer cell based immunotherapies. Front Immunol (2020) 11:1512. doi: 10.3389/fimmu.2020.01512
76. Rada G, Corbalán J, Rojas P. Cell-based therapies for COVID-19: a living, systematic review. Medwave (2020) 20:e8079. doi: 10.1101/2020.04.24.20078667
77. Liao G, Zheng K, Lalu MM, Fergusson DA. & Allan, D.S. a scoping review of registered clinical trials of cellular therapy for COVID-19 and a framework for accelerated synthesis of trial evidence-FAST evidence. Transfus Med Rev (2020) 34:165–71. doi: 10.1016/j.tmrv.2020.06.001
78. Sahu KK, Siddiqui AD, Cerny J. Mesenchymal stem cells in COVID-19: a journey from bench to bedside. Lab Med (2021) 52:24–35. doi: 10.1093/labmed/lmaa049
79. Zumla A, Wang FS, Ippolito G, Petrosillo N, Agrati C, Azhar EI, et al. Reducing mortality and morbidity in patients with severe COVID-19 disease by advancing ongoing trials of mesenchymal stromal (stem) cell (MSC) therapy - achieving global consensus and visibility for cellular host-directed therapies. Int J Infect Dis (2020) 96:431–9. doi: 10.1016/j.ijid.2020.05.040
80. Thorlund K, Dron L, Park J, Hsu G, Forrest JI, Mills EJ. A real-time dashboard of clinical trials for COVID-19. Lancet Digit Health (2020) 2(6):e286–7. doi: 10.1016/S2589-7500(20)30086-8
81. Babaei F, Mirzababaei M, Nassiri-Asl M, Hosseinzadeh H. Review of registered clinical trials for the treatment of COVID-19. Drug Dev Res (2021) 82:474–93. doi: 10.1002/ddr.21762
82. Golchin A, Seyedjafari E, Ardeshirylajimi A. Mesenchymal stem cell therapy for COVID-19: present or future. Stem Cell Rev Rep (2020) 16:427–33. doi: 10.1007/s12015-020-09973-w
83. Khoury M, Cuenca J, Cruz FF, Figueroa FE, Rocco PRM, Weiss DJ, et al. Current status of cell-based therapies for respiratory virus infections: applicability to COVID-19. Eur Respir J (2020) 55(6):2000858. doi: 10.1183/13993003.00858-2020
84. Ji HL, Liu C, Zhao RZ. Stem cell therapy for COVID-19 and other respiratory diseases: global trends of clinical trials. World J Stem Cells (2020) 12:471–80. doi: 10.4252/wjsc.v12.i6.471
85. Lythgoe MP, Middleton P. Ongoing clinical trials for the management of the COVID-19 pandemic. Trends Pharmacol Sci (2020) 41:363–82. doi: 10.1016/j.tips.2020.03.006
86. Liu S, Peng D, Qiu H, Yang K, Fu Z, Zou L. Mesenchymal stem cells as a potential therapy for COVID-19. Stem Cell Res Ther (2020) 11(1):169. doi: 10.1186/s13287-020-01678-8
87. Moll G, Rasmusson-Duprez I, von Bahr L, Connolly-Andersen AM, Elgue G, Funke L, et al. Are therapeutic human mesenchymal stromal cells compatible with human blood? Stem Cells (2012) 30(7):1565–74. doi: 10.1002/stem.1111
88. Moll G, Alm JJ, Davies LC, von Bahr L, Heldring N, Stenbeck-Funke L, et al. Do cryopreserved mesenchymal stromal cells display impaired immunomodulatory and therapeutic properties? Stem Cells (2014) 32(9):2430–42. doi: 10.1002/stem.1729
89. Moll G, Hult A, von Bahr L, Alm JJ, Heldring N, Hamad OA, et al. Do ABO blood group antigens hamper the therapeutic efficacy of mesenchymal stromal cells? PloS One (2014) 9(1):e85040. doi: 10.1371/journal.pone.0085040
90. Moll G, Ignatowicz L, Catar R, Luecht C, Sadeghi B, Hamad O, et al. Different procoagulant activity of therapeutic mesenchymal stromal cells derived from bone marrow and placental decidua. Stem Cells Dev (2015) 24(19):2269–79. doi: 10.1089/scd.2015.0120
91. Giri J, Moll G. MSCs in space: mesenchymal stromal cell therapeutics as enabling technology for long-distance manned space travel. Curr Stem Cell Rep (2022) 8:1–13. doi: 10.1007/s40778-022-00207-y
92. Pérez-Martínez A, Mora-Rillo M, Ferreras C, Guerra-García P, Pascual-Miguel B, Mestre-Durán C, et al. Phase I dose-escalation single centre clinical trial to evaluate the safety of infusion of memory T cells as adoptive therapy in COVID-19 (RELEASE). eClinicalMedicine (2021) 39:101086. doi: 10.1016/j.eclinm.2021.101086
93. Ventura-Carmenate Y, Alkaabi FM, Castillo-Aleman YM, Villegas-Valverde CA, Ahmed YM, Sanna P, et al. Safety and efficacy of autologous non-hematopoietic enriched stem cell nebulization in COVID-19 patients: a randomized clinical trial, Abu Dhabi 2020. Transl Med Commun (2021) 6(1):25. doi: 10.1186/s41231-021-00101-5
94. N OE, Pekkoc-Uyanik KC, Alpaydin N, Gulay GR. & simsek, m. clinical experience on umbilical cord mesenchymal stem cell treatment in 210 severe and critical COVID-19 cases in Turkey. Stem Cell Rev Rep (2021) 17:1917–25. doi: 10.1007/s12015-021-10214-x
95. Ankrum JA, Ong JF, Karp JM. Mesenchymal stem cells: immune evasive, not immune privileged. Nat Biotechnol (2014) 32:252–60. doi: 10.1038/nbt.2816
96. Fung M, Yuan Y, Atkins H, Shi Q, Bubela T. Responsible translation of stem cell research: an assessment of clinical trial registration and publications. Stem Cell Rep (2017) 8:1190–201. doi: 10.1016/j.stemcr.2017.03.013
97. Qu W, Wang Z, Engelberg-Cook E, Yan D, Siddik AB, Bu G, et al. Efficacy and safety of MSC cell therapies for hospitalized patients with COVID-19: a systematic review and meta-analysis. Stem Cells Trans Med (2022) 11(7):688–703. doi: 10.1093/stcltm/szac032
98. Kirkham AM, Bailey AJM, Monaghan M, Shorr R, Lalu MM, Fergusson DA, et al. Updated living systematic review and meta-analysis of controlled trials of mesenchymal stromal cells to treat COVID-19: a framework for accelerated synthesis of trial evidence for rapid approval-FASTER approval. Stem Cells Trans Med (2022) 11:(7)675–87. doi: 10.1093/stcltm/szac038
99. Zhang M, Yan X, Shi M, Li R, Pi Z, Ren X, et al. Safety and efficiency of stem cell therapy for COVID-19: a systematic review and meta-analysis. Global Health Res Policy (2022) 7(1):19. doi: 10.1186/s41256-022-00251-5
100. Taufiq H, Shaik Fakiruddin K, Muzaffar U, Lim MN, Rusli S, Kamaluddin NR, et al. Systematic review and meta-analysis of mesenchymal stromal/stem cells as strategical means for the treatment of COVID-19. Ther Adv Respir Dis (2023) 17:17534666231158276. doi: 10.1177/17534666231158276
101. Dyer O. Covid-19: China stops counting cases as models predict a million or more deaths. BMJ (2023) 380:p2. doi: 10.1136/bmj.p2
102. Wu C, Chen X, Cai Y, Xia J, Zhou X, Xu S, et al. Risk factors associated with acute respiratory distress syndrome and death in patients with coronavirus disease 2019 pneumonia in wuhan, China. JAMA Intern Med (2020) 180(7):934–43. doi: 10.1001/jamainternmed.2020.0994.
103. Zhou F, Yu T, Du R, Fan G, Liu Y, Liu Z, et al. Clinical course and risk factors for mortality of adult inpatients with COVID-19 in wuhan, China: a retrospective cohort study. Lancet (2020) 395(10229):1054–62 doi: 10.1016/S0140-6736(20)30566-3
104. Arachchillage DR, Laffan M. Abnormal coagulation parameters are associated with poor prognosis in patients with novel coronavirus pneumonia. J Thromb Haemost (2020) 18(5):1233–4 doi: 10.1111/jth.14820
105. Tang N, Li D, Wang X, Sun Z. Abnormal coagulation parameters are associated with poor prognosis in patients with novel coronavirus pneumonia. J Thromb Haemostasis: JTH (2020) 18:844–7. doi: 10.1111/jth.14768
106. Wang T, Chen R, Liu C, Liang W, Guan W, Tang R, et al. Attention should be paid to venous thromboembolism prophylaxis in the management of COVID-19. Lancet Haematol (2020). 7(5):e362-e363 doi: 10.1016/S2352-3026(20)30109-5
107. Zhang Y, Xiao M, Zhang S, Xia P, Cao W, Jiang W, et al. Coagulopathy and antiphospholipid antibodies in patients with covid-19. N Engl J Med (2020), 382(17):e38. doi: 10.1056/NEJMc2007575
108. Klok FA, Kruip MJHA, van der Meer NJM, Arbous MS, Gommers DAMPJ, Kant KM, et al. Incidence of thrombotic complications in critically ill ICU patients with COVID-19. Thromb Res (2020). 191:145–7 doi: 10.1016/j.thromres.2020.04.013
109. Spyropoulos AC, Ageno W, Barnathan ES. Hospital-based use of thromboprophylaxis in patients with COVID-19. Lancet (2020). 395(10234):e75 doi: 10.1016/S0140-6736(20)30926-0
110. Magro C, Mulvey JJ, Berlin D, Nuovo G, Salvatore S, Harp J, et al. Complement associated microvascular injury and thrombosis in the pathogenesis of severe COVID-19 infection: a report of five cases. Transl Res (2020) 220:1–13. doi: 10.1016/j.trsl.2020.04.007
111. Oxley TJ, Mocco J, Majidi S, Kellner CP, Shoirah H, Singh IP, et al. Large-Vessel stroke as a presenting feature of covid-19 in the young. N Engl J Med (2020) 382(20):e60. doi: 10.1056/NEJMc2009787
112. Connors JM, Levy JH. COVID-19 and its implications for thrombosis and anticoagulation. Blood (2020) 135(23):2033–40. doi: 10.1182/blood.2020006000
113. Jung JW, Kwon M, Choi JC, Shin JW, Park IW, Choi BW, et al. Familial occurrence of pulmonary embolism after intravenous, adipose tissue-derived stem cell therapy. Yonsei Med J (2013) 54(5):1293–6. doi: 10.3349/ymj.2013.54.5.1293
114. Wu Z, Zhang S, Zhou L, Cai J, Tan J, Gao X, et al. Thromboembolism induced by umbilical cord mesenchymal stem cell infusion: a report of two cases and literature review. Transplant Proc (2017) 49(7):1656–8. doi: 10.1016/j.transproceed.2017.03.078
115. Yilmaz R, Çukurova Z, Hergünsel GO, Aşar S, Karaöz E, Işiksaçan N, et al. The importance of timing in the application of mesenchymal stem cells in critically ill patients with COVID-19 infection (Retrospective study). Anesth Crit Care (2022) 4:149–56. doi: 10.26502/acc.050
116. Ayatollahi M, Talaei-Khozani T, Razmkhah M. Growth suppression effect of human mesenchymal stem cells from bone marrow, adipose tissue, and wharton’s jelly of umbilical cord on PBMCs. Iran J Basic Med Sci (2016) 19(2):145–53.
117. Chen MY, Lie PC, Li ZL, Wei X. Endothelial differentiation of wharton’s jelly-derived mesenchymal stem cells in comparison with bone marrow-derived mesenchymal stem cells. Exp Hematol (2009) 37:629–40. doi: 10.1016/j.exphem.2009.02.003
118. Guan YT, Xie Y, Li DS, Zhu YY, Zhang XL, Feng YL, et al. Comparison of biological characteristics of mesenchymal stem cells derived from the human umbilical cord and decidua parietalis. Mol Med Rep (2019) 20(1):633–9. doi: 10.3892/mmr.2019.10286
119. Jin HJ, Bae YK, Kim M, Kwon SJ, Jeon HB, Choi SJ, et al. Comparative analysis of human mesenchymal stem cells from bone marrow, adipose tissue, and umbilical cord blood as sources of cell therapy. Int J Mol Sci (2013) 14(9):17986–8001. doi: 10.3390/ijms140917986
120. Kehl D, Generali M, Mallone A, Heller M, Uldry AC, Cheng P, et al. Proteomic analysis of human mesenchymal stromal cell secretomes: a systematic comparison of the angiogenic potential. NPJ Regener Med (2019) 4:8. doi: 10.1038/s41536-019-0070-y
121. Castro-Manrreza ME, Mayani H, Monroy-García A, Flores-Figueroa E, Chávez-Rueda K, Legorreta-Haquet V, et al. Human mesenchymal stromal cells from adult and neonatal sources: a comparative in vitro analysis of their immunosuppressive properties against T cells. Stem Cells Dev (2014) 23(11):1217–32. doi: 10.1089/scd.2013.0363
122. Najar M, Raicevic G, Boufker HI, Fayyad-Kazan H, De Bruyn C, Meuleman N, et al. Adipose-tissue-derived and wharton’s jelly-derived mesenchymal stromal cells suppress lymphocyte responses by secreting leukemia inhibitory factor. Tissue Eng Part A (2010) 16(11):3537–46. doi: 10.1089/ten.tea.2010.0159
123. Prasanna SJ, Gopalakrishnan D, Shankar SR, Vasandan AB. Pro-inflammatory cytokines, IFNgamma and TNFalpha, influence immune properties of human bone marrow and Wharton jelly mesenchymal stem cells differentially. PloS One (2010) 5:(2)e9016. doi: 10.1371/journal.pone.0009016
124. Baksh D, Yao R, Tuan RS. Comparison of proliferative and multilineage differentiation potential of human mesenchymal stem cells derived from umbilical cord and bone marrow. Stem Cells (2007) 25:1384–92. doi: 10.1634/stemcells.2006-0709
125. Dabrowski FA, Burdzinska A, Kulesza A, Sladowska A, Zolocinska A, Gala K, et al. Comparison of the paracrine activity of mesenchymal stem cells derived from human umbilical cord, amniotic membrane and adipose tissue. J Obstet Gynaecol Res (2017) 43(11):1758–68. doi: 10.1111/jog.13432
126. Petrenko Y, Vackova I, Kekulova K, Chudickova M, Koci Z, Turnovcova K, et al. A comparative analysis of multipotent mesenchymal stromal cells derived from different sources, with a focus on neuroregenerative potential. Sci Rep (2020) 10(1):4290. doi: 10.1038/s41598-020-61167-z
127. Wegmeyer H, Bröske AM, Leddin M, Kuentzer K, Nisslbeck AK, Hupfeld J, et al. Mesenchymal stromal cell characteristics vary depending on their origin. Stem Cells Dev (2013) 22(19):2606–18. doi: 10.1089/scd.2013.0016
128. Ribeiro A, Laranjeira P, Mendes S, Velada I, Leite C, Andrade P, et al. Mesenchymal stem cells from umbilical cord matrix, adipose tissue and bone marrow exhibit different capability to suppress peripheral blood b, natural killer and T cells. Stem Cell Res Ther (2013) 4(5):125. doi: 10.1186/scrt336
129. Yoo KH, Jang IK, Lee MW, Kim HE, Yang MS, Eom Y, et al. Comparison of immunomodulatory properties of mesenchymal stem cells derived from adult human tissues. Cell Immunol (2009) 259(2):150–6. doi: 10.1016/j.cellimm.2009.06.010
130. Heo JS, Choi Y, Kim HS, Kim HO. Comparison of molecular profiles of human mesenchymal stem cells derived from bone marrow, umbilical cord blood, placenta and adipose tissue. Int J Mol Med (2016) 37:115–25. doi: 10.3892/ijmm.2015.2413
131. Wiese DM, Wood CA, Braid LR. From vial to vein: crucial gaps in mesenchymal stromal cell clinical trial reporting. Front Cell Dev Biol (2022) 10. doi: 10.3389/fcell.2022.867426
132. Wilson AJ, Rand E, Webster AJ, Genever PG. Characterisation of mesenchymal stromal cells in clinical trial reports: analysis of published descriptors. Stem Cell Res Ther (2021) 12:360. doi: 10.1186/s13287-021-02435-1
133. Renesme L, Cobey KD, Le M, Lalu MM, Thebaud B. Establishment of a consensus definition for mesenchymal stromal cells (MSC) and reporting guidelines for clinical trials of MSC therapy: a modified Delphi study protocol. BMJ Open (2021) 11:e054740. doi: 10.1136/bmjopen-2021-054740
134. Taghizadeh RR, Cetrulo KJ, Cetrulo CL. Collagenase impacts the quantity and quality of native mesenchymal Stem/Stromal cells derived during processing of umbilical cord tissue. Cell Transplant (2018) 27:181–93. doi: 10.1177/0963689717744787
135. Andrzejewska A, Catar R, Schoon J, Qazi TH, Sass FA, Jacobi D, et al. Multi-parameter analysis of biobanked human bone marrow stromal cells shows little influence for donor age and mild comorbidities on phenotypic and functional properties. Front Immunol (2019) 10:2474. doi: 10.3389/fimmu.2019.02474
136. Teryek M, Doshi A, Sherman LS, et al. Clinical manufacturing of human mesenchymal stromal cells using a potency-driven paradigm. Curr Stem Cell Rep (2022) 8:61–71. doi: 10.1007/s40778-022-00208-x
137. Aijaz A, Vaninov N, Allen A, Barcia RN, Parekkadan B. Convergence of cell pharmacology and drug delivery. Stem Cells Trans Med (2019) 8(9):874–9. doi: 10.1002/sctm.19-0019
138. Levy O, Kuai R, Siren EMJ, Bhere D, Milton Y, Nissar N, et al. Shattering barriers toward clinically meaningful MSC therapies. Sci Adv (2020) 6(30):eaba6884. doi: 10.1126/sciadv.aba6884
139. Yin JQ, Zhu J, Ankrum JA. Manufacturing of primed mesenchymal stromal cells for therapy. Nat BioMed Eng (2019) 3:90–104. doi: 10.1038/s41551-018-0325-8
140. Aijaz A, Li M, Smith D, Khong D, LeBlon C, Fenton OS, et al. Biomanufacturing for clinically advanced cell therapies. Nat BioMed Eng (2018) 2(6):362–76. doi: 10.1038/s41551-018-0246-6
141. Silva Couto P, Rotondi MC, Bersenev A, Hewitt CJ, Nienow AW, Verter F, et al. Expansion of human mesenchymal stem/stromal cells (hMSCs) in bioreactors using microcarriers: lessons learnt and what the future holds. Biotechnol Adv (2020) 45:107636. doi: 10.1016/j.biotechadv.2020.107636
142. Hoogduijn MJ, de Witte SF, Luk F, van den Hout-van Vroonhoven MC, Ignatowicz L, Catar R, et al. Effects of freeze-thawing and intravenous infusion on mesenchymal stromal cell gene expression. Stem Cells Dev (2016) 25(8):586–97. doi: 10.1089/scd.2015.0329
143. Weiss ARR, Dahlke MH. Immunomodulation by mesenchymal stem cells (MSCs): mechanisms of action of living, apoptotic, and dead MSCs. Front Immunol (2019) 10. doi: 10.3389/fimmu.2019.01191
144. Weiss DJ, English K, Krasnodembskaya A, Isaza-Correa JM, Hawthorne IJ, Mahon BP, et al. The necrobiology of mesenchymal stromal cells affects therapeutic efficacy. Front Immunol (2019) 10:1228. doi: 10.3389/fimmu.2019.01228
145. Weiss DJ, Filiano A, Galipeau J, Khoury M, Krampera M, Lalu M, et al. An international society for cell and gene therapy mesenchymal stromal cells committee editorial on overcoming limitations in clinical trials of mesenchymal stromal cell therapy for coronavirus disease-19: time for a global registry. Cytotherapy (2022) 24(11):1071–3. doi: 10.1016/j.jcyt.2022.07.010
146. Repair of acute respiratory distress syndrome by stromal cell administration (REALIST). Available at: https://clinicaltrials.gov/ct2/show/NCT03042143 (Accessed 2023-01-10).
147. Mesenchymal stromal cells for acute respiratory distress syndrome (STAT). Available at: https://clinicaltrials.gov/ct2/show/NCT03818854 (Accessed 2023-01-10).
148. Davis HE, McCorkell L, Vogel JM, Topol EJ. Long COVID: major findings, mechanisms and recommendations. Nat Rev Microbiol (2023) 21(3):133–46. doi: 10.1038/s41579-022-00846-2
149. Stumpf J, et al. Humoral and cellular immunity to SARS-CoV-2 vaccination in renal transplant versus dialysis patients: a prospective, multicenter observational study using mRNA-1273 or BNT162b2 mRNA vaccine. Lancet Reg Health Eur (2021) 9:100178. doi: 10.1016/j.lanepe.2021.100178
Keywords: cell and gene therapy (CGT), advanced therapy medicinal products (ATMPs), mesenchymal stromal/stem cells (MSCs), severe respiratory distress syndrome coronavirus 2 (SARS-CoV2), coronavirus induced disease 2019 (COVID-19)
Citation: Couto PS, Al-Arawe N, Filgueiras IS, Fonseca DLM, Hinterseher I, Catar RA, Chinnadurai R, Bersenev A, Cabral-Marques O, Moll G and Verter F (2023) Systematic review and meta-analysis of cell therapy for COVID-19: global clinical trial landscape, published safety/efficacy outcomes, cell product manufacturing and clinical delivery. Front. Immunol. 14:1200180. doi: 10.3389/fimmu.2023.1200180
Received: 04 April 2023; Accepted: 24 May 2023;
Published: 21 June 2023.
Edited by:
Antoine Toubert, Université Paris Cité, FranceReviewed by:
Selim Kuci, University Hospital Frankfurt, GermanyLorena Braid, Simon Fraser University, Canada
Copyright © 2023 Couto, Al-Arawe, Filgueiras, Fonseca, Hinterseher, Catar, Chinnadurai, Bersenev, Cabral-Marques, Moll and Verter. This is an open-access article distributed under the terms of the Creative Commons Attribution License (CC BY). The use, distribution or reproduction in other forums is permitted, provided the original author(s) and the copyright owner(s) are credited and that the original publication in this journal is cited, in accordance with accepted academic practice. No use, distribution or reproduction is permitted which does not comply with these terms.
*Correspondence: Guido Moll, guido.moll@charite.de; Pedro S. Couto, pedro.couto.17@ucl.ac.uk; Frances Verter, fverter@his.com
†These authors have contributed equally to this work and share first authorship
‡These authors have contributed equally to this work and share senior authorship
§ORCID: Pedro S. Couto, orcid.org/0000-0002-7077-4453
Guido Moll, orcid.org/0000-0001-6173-5957
Frances Verter, orcid.org/0000-0001-5345-4514