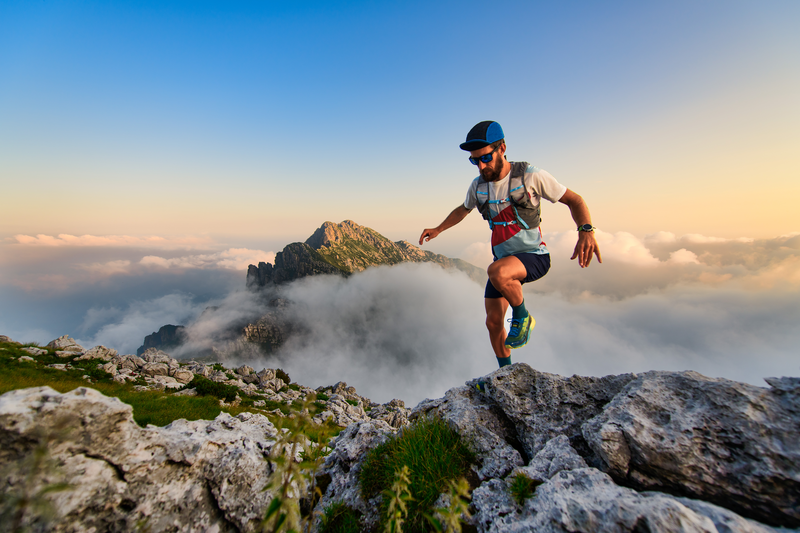
94% of researchers rate our articles as excellent or good
Learn more about the work of our research integrity team to safeguard the quality of each article we publish.
Find out more
PERSPECTIVE article
Front. Immunol. , 08 June 2023
Sec. Cancer Immunity and Immunotherapy
Volume 14 - 2023 | https://doi.org/10.3389/fimmu.2023.1197053
This article is part of the Research Topic Cell network in antitumor immunity of pediatric and adult solid tumors View all 15 articles
DNAM-1 is a major NK cell activating receptor and, together with NKG2D and NCRs, by binding specific ligands, strongly contributes to mediating the killing of tumor or virus-infected cells. DNAM-1 specifically recognizes PVR and Nectin-2 ligands that are expressed on some virus-infected cells and on a broad spectrum of tumor cells of both hematological and solid malignancies. So far, while NK cells engineered for different antigen chimeric receptors (CARs) or chimeric NKG2D receptor have been extensively tested in preclinical and clinical studies, the use of DNAM-1 chimeric receptor-engineered NK cells has been proposed only in our recent proof-of-concept study and deserves further development. The aim of this perspective study is to describe the rationale for using this novel tool as a new anti-cancer immunotherapy.
NK cells are cytotoxic lymphocytes belonging to innate immunity that, by a complex array of activating and inhibitory receptors, are tolerant versus healthy cells and can recognize and kill virus-infected and transformed cells through the release of cytolytic granules and cytotoxic cytokines (1). The peculiar ability to elicit a potent response against target cells is due to the expression by NK cells of a repertoire of activating receptors such as NKG2D, the accessory molecule DNAX (DNAM-1, CD226), and natural cytotoxicity receptors (NCRs) including NKp30, NKp44, and NKp46 (2, 3). Of note, ligands for NKG2D and DNAM-1 are poorly expressed in normal cells [proteinatlas.org, Genotype-Tissue Expression (GTEx) from The Cancer Genome Atlas (TCGA) database and (4)] and highly expressed in virus-infected and transformed cells (5, 6). Furthermore, NK cells, through the expression of FcγRIIIA (CD16) receptor, are responsible for the antibody-dependent cellular cytotoxicity (ADCC) (7), which is a crucial function in the clinical context of all immunotherapies involving monoclonal antibodies (mAb) (8).
In addition to their cytotoxic function, NK cells play a crucial role in regulating the maturation and activation state of other immune cells, through sophisticated cross-talks and biological mechanisms that further support their use in immunotherapy (9).
In contrast, it is noteworthy that NK cells in cancer patients show impaired functions accompanied by a poor ability to infiltrate the tumor microenvironment (TME), as tumor cells adopt different various immune evasion mechanisms (10–17). Therefore, the adoptive transfer of ex vivo expanded and activated allogeneic NK cells for immunotherapy turns out to be a strategic clinical adoption to help cancer patients to fight tumor cells, thus attracting increasing interest in the past decade (18).
Primary allogeneic and alloreactive NK cells, from healthy donors with a favorable immunoglobulin-like receptor (KIR)-human leukocyte antigen (HLA) mismatch (19), can be harvested from several sources such as peripheral blood (20), umbilical cord blood (21) or be derived by induced pluripotent stem cells (iPSC) (22, 23). Compared with the therapeutic use of T cells, that of allogeneic NK cells has several advantages: this has progressively stimulated the improvement of previously limited ex vivo amplification methods of NK cells and designs for the expression of various chimeric antigen receptors (CARs) and NKG2D chimeric receptor (24, 25) suitable for clinical use (ClinilTrial.gov and Supplementary Table S1).
In this context, one should consider that T and NK cells are often dysfunctional in cancer patients, limiting the use of autologous cells for engineered manipulation (26). Noticeably, NK cells display greater antitumor effects in allogeneic settings than in autologous ones (20, 27). However, the use of allogeneic T or CAR-T cells presents limitations related to severe haploidentical mismatch conditions necessary to reduce the risk of graft-versus-host disease (GvHD) and cytokine release syndrome (28–30). In contrast, allogeneic NK cells do not cause GvHD (31–33) and display a low risk of proliferation in transfused patients and, thus a major safety, as compared with infused T cells. Finally, the high availability of allogeneic NK cells, their low cost compared to CAR-T cells, and the possibility of cryopreserving them for further administration allowing the treatment of many patients from a single NK cell donor, entitles their clinical use for several types of cancers (34, 35).
So far, the successful use of NK cells engineered for several CARs and for NKG2D chimeric receptor in the hematological and solid tumor settings has been widely reported (ClinicalTrial.gov Supplementary Table S1). Based on the success of CD19-targeted CAR-T cells (36), approved by U.S. Food and Drug Administration (FDA), the first CAR-NK cells were engineered with chimeric anti-CD19 single chain fragment variable (scFv) for the cure of hematologic malignancies (21). Currently, the use of CAR- or NKG2D chimeric receptor-engineered NK cells has been extended to different type of cancers; however, the number of clinical trials evaluating their efficacy against solid tumors is far lower than against hematologic malignancies (14 versus 29, as reported in Supplementary Table S1). This represents a clinical gap that needs to be filled. CAR-T or CAR-NK cells have generally shown greater efficacy in hematologic malignancies than in solid tumors, mainly for the following reasons: (i) firstly, the accessibility of CAR-T or CAR-NK cells to tumor cells is significantly different between solid and hematological tumors, depending on cell morphology (absence or presence of cell-cell adhesions) and body distribution; (ii) secondly, solid tumor cells are less sensitive to cytotoxic lymphocytes, as the immune suppression mechanisms occurring in TME constitute a barrier to lymphocyte infiltration. Therefore, in order to improve the efficacy of the adoptive transfer of CAR-NK cells for immunotherapy of solid tumors, the search for more specific tumor target molecules, accompanied by mechanisms that overcome the barriers of TME, still needs to be extensively explored (37).
Aiming to fill this gap, recently we have provided promising in vitro results on the efficacy of never before explored DNAM-1-chimeric receptor-engineered NK cells against neuroblastoma (NB) (38). This proof-of-concept study is prompting us at optimizing the DNAM-1-based chimeric construct with the aim of developing highly efficient DNAM-1 chimeric receptor-engineered NK cells to be employed in preclinical studies and prospective clinical trials primarily directed against solid tumors.
Human DNAX accessory molecule-1 (DNAM-1, CD226) is constitutively expressed in T, NK cells, and some myeloid cells. It is a type I transmembrane glycoprotein containing a leader sequence of 18 amino acid (aa), two extracellular Ig-like C2-set domains of 230 aa, a transmembrane domain of 28 aa and a cytoplasmic region of 60 aa. Together with other activating receptors, such as NKG2D and NCRs (39), DNAM-1 triggers powerful activating signals that promote NK cell-mediated cytotoxicity and cytokine secretion (40, 41). DNAM-1 mediates activation signals through the engagement with two ligands such as PVR (poliovirus receptor, CD155) and Nectin-2 (poliovirus receptor-related 2 protein, PVRL2, also known as CD112) (5). Furthermore, through cis-binding to the integrin LFA-1 upon the engagement of LFA-1 with ICAM-1 (42), DNAM-1 undergoes phosphorylation at conserved amino acid residues in its cytoplasmic domain such as tyrosine 322 [Y322 in human and Y319 in mouse, (42)] and serine 326 (40) via Src family kinase Fyn and protein kinase C, respectively (43). The coordinated expression of DNAM-1 and LFA-1 is also crucial for NK cell education (44).
Adequate expression of DNAM-1 enables NK cells to recognize and kill hematopoietic malignancies such as acute myeloid leukemia (AML) (45), multiple myeloma (MM) (39), and solid tumor cells such as melanoma (46) and NB (47), thus contributing to a favorable prognosis (45, 48). In contrast, DNAM-1 expression is impaired in AML cancer patients and its loss has been correlated with the tumor severity (49).
Both PVR and Nectin-2 ligands are closely linked to tumorigenesis. Indeed, in addition to being expressed in virus-infected cells (43), these ligands are overexpressed in several hematological and solid tumors (5, 50–52). Noticeably, these ligands, in particular PVR, are potential prognostic markers in AML (53, 54), MM (55), hepatocellular carcinoma (56), and bladder urothelial carcinoma (BLCA) (57). As we have previously reported, PVR expression is directly under the control of p53 at promoter level (47), whilst the transcriptional regulation of Nectin-2 remains more widely to be explored (58). Furthermore, PVR and Nectin-2 are both upregulated by Toll-like receptors agonists in dendritic cells (59, 60) and by DNA-damage response in multiple myeloma cells (61) or in Ag-activated T lymphocytes (62). In addition, PVR is upregulated by IFN-γ in NB cell lines (63) and epigenetic modulations in malignant lymphocytes (64), while it is downregulated by the human immunodeficiency virus type 1 Nef and Vpu proteins (65) and the human cytomegalovirus UL141 protein (66).
The activating signal mediated by DNAM-1 following the engagement of the ligands PVR or Nectin-2 is counteracted by the competing binding of inhibitory receptors such as TIGIT (T-cell immunoglobulin and ITIM domain) (67), TACTILE (T cell activation, increased late expression, also known as CD96) (68) and PVRIG (69) for the same ligands. In particular, PVR is recognized by TIGIT and TACTILE (70, 71), while Nectin-2 is recognized by TIGIT and PVRIG (69, 70). For this reason, TIGIT, TACTILE and PVRIG have been considered targets for checkpoint blockade immunotherapy (72). Of note, the high expression levels of PVR, typical of various tumor types, revealed its hypothetical proto-oncogenic role, leading researchers to develop therapeutic strategies that directly target PVR (73).
Adoptive transfer of activated NK cells expressing higher and more stable levels of DNAM-1, might be a useful clinical approach to help cancer patients to fight tumor cells. The DNAM-1 chimeric receptor could confer a dual advantage to NK cells: (i) specific recognition of ligands such as PVR and Nectin-2, which are highly expressed in tumor cells, but importantly absent or poorly expressed in normal cells, and (ii) its overexpression, which should result in a favorable molecular imbalance with respect to the normal expression of competing receptors (TIGIT, TACTILE, PVRIG), leading to its increased binding to PVR and Nectin-2. In addition, its function could be strategically improved by in-frame expression of costimulatory molecules that support cytotoxic activity and overcome TME immune escape mechanisms. We previously reported a proof-of-concept study on the activity of DNAM-1-chimeric receptor-engineered NK cells obtained by transient transfection of primary human NK cells for a DNAM-1-chimeric receptor (38). Specifically, we compared four different constructs, including the full-length DNAM-1 receptor, and three different DNAM-1-based chimeric receptors providing the expression of DNAM-1 in frame with costimulatory molecules such as 2B4 and CD3ζ, and we showed that the DNAM-1-CD3ζ construct, which recapitulates a first generation of DNAM-1 chimeric receptor, yielded the best results in terms of expression of DNAM-1 chimeric receptor and NK cell functions. Furthermore, DNAM-1-CD3ζ engineered NK cells were particularly more effective to recognize and kill two NB cell lines, LAN-5 and SMS-KCNR, treated with Nutlin-3a, an MDM2 targeting drug with immunomodulatory effects on the upregulation of ligands for NK cell-activating receptors, including PVR and Nectin-2 (47). Therefore, the combined use of DNAM-1-CD3ζ engineered NK cells with Nutlin-3a in tumors that retain p53-wt, such as most forms of NB, with the exception of some cases of relapse (74), may represent a novel therapeutic approach for solid tumors.
The widely reported high expression of both PVR and Nectin-2 in solid tumor cells and very low expression in normal cells [protein.atlas.gov and GTEx from TCGA database], was the main reason for choosing to engineer NK cells with a DNAM-1 chimeric receptor. In order to further explore the expression of both PVR and Nectin-2 in solid tumors, and to prospectively propose the adoptive transfer of DNAM-1 chimeric receptor-engineered NK cells also in adult solid malignancies, we performed an in-silico bioinformatic analysis by using GEPIA2 (www.gepia2.cancer-pku.cn, Figure 1). Specifically, we queried this online tool providing data concerning gene expression and tumor stage/grade, to compare the expression of selected genes between tumor and normal tissues, based on TCGA. Interestingly, we found that the expression profile of both PVR and Nectin-2 resulted higher in several tumor samples than in paired normal tissues across a broad spectrum of solid tumors. In particular, the expression of PVR was significantly higher in colon adenocarcinoma (COAD), esophageal carcinoma (ESCA), head and neck squamous cell carcinoma (HNSC), pancreatic adenocarcinoma (PAAD), rectum adenocarcinoma (READ), stomach adenocarcinoma (STAD) and thymoma (THYM), while that of Nectin-2 was significantly higher in bladder urothelial carcinoma (BLCA), breast invasive carcinoma (BRCA), COAD, lymphoid neoplasm diffuse large B-cell lymphoma (DLBC), glioblastoma multiforme (GBM), brain lower grade glioma (LGG), ovarian serous cystadenocarcinoma (OV), PAAD, READ, STAD, THYM and uterine corpus endometrial carcinoma (UCEC) (Figure 1A). In addition, the higher expression of PVR or Nectin-2 correlated with the advanced stage of different forms of solid tumors. In particular, PVR higher expression correlated with the advanced stage of adrenocortical carcinoma (ACC), BLCA, liver hepatocellular carcinoma (LIHC), lung adenocarcinoma (LUAD), lung squamous cell carcinoma (LUSC) (Figure 1B), while that of Nectin-2 correlated with the advanced stage of ACC, BLCA, HNSC, testicular germ cell tumors (TGCT), skin cutaneous melanoma (SKCM) and UCEC (Figure 1C). These data indicate that the high expression of PVR and Nectin-2 in tumor cells compared to normal cells affects several solid tumors, supporting the hypothesis of a wide prospective clinical use of DNAM-1 chimeric receptor-engineered NK cells.
Figure 1 In-silico bioinformatics analysis of PVR and NECTIN2 gene expression by GEPIA2 web-tool based on The Cancer Genome Atlas (TCGA) database. (A) Dot plot profiling of PVR (top) and Nectin-2 (down) differential expression levels in 33 cancer types, derived from TCGA database, compared to the normal, derived from TCGA or Genotype-Tissue Expression (GTEx). Each dot represents a distinct tumor (red) or normal sample (green) while each column represents a different tumor type (tumor labels and sample sizes are reported in Supplementary Table 2). The transcript per million (TPM) value, shown in ordinate, is used to display the relative gene expression. Tumor labels are indicated in red when there is a significant difference between tumor (T) versus normal (N) tissues. Data were analyzed by ANOVA test. |log2FC| > 1 and FDR < 0.05 were considered as differentially expressed. (B, C) Violin plots showing the expression level of PVR (B) and Nectin-2 (C) among different pathologic stages (S) of indicated solid tumors. F-value indicates the statistical value of the F test; Pr (> F) indicates p value. A p value of < 0.05 was considered statistically significant.
Furthermore, we used the R2 Genomics Analysis and Visualization Platform (https://hgserver1.amc.nl/cgi-bin/r2/main.cgi?open_page=login) to investigate the prognostic value of PVR and Nectin-2 ligands in a variety of tumor types. We found that higher expression of PVR significantly correlated with lower patient overall survival in ACC, BLCA, COAD, ESCA, HNSC, kidney renal clear cell carcinoma (KIRC), kidney renal papillary cell carcinoma (KIRP), LUAD, LUSC, mesothelioma (MESO), OV, prostate adenocarcinoma (PRAD), SKCM, STAD and uveal melanoma (UVM) (Supplementary Figure 1A). By contrast, the lower expression of PVR significantly correlated with lower patient survival in BRCA, PAAD, READ and THYM (Supplementary Figure 1B), in agreement with published data from a cohort of patients with a pediatric form of solid tumor such as NB (75). Similarly, the higher expression of Nectin-2 correlated with lower patient overall survival in KIRC, KIRP, GBM, HNSC, LIHC, LUAD, LUSC, MESO, OV, READ, SKCM, UCEC and uterine carcinosarcoma (UCS) (Supplementary Figure 2A). By contrast, the lower expression of Nectin-2 correlated with lower patient overall survival in BRCA, COAD, ESCA, PRAD, STAD and UVM (Supplementary Figure 2B). These data suggest that the expression levels of both PVR and Nectin-2 can correlate differently with patient overall survival, depending on the kind of solid tumors.
With a view to finding an optimized off-the-shelf product for cellular immunotherapeutic approaches, we foresee that DNAM-1 chimeric receptor engineered-NK cells have several strengths that should be taken into account. NK cells engineered for a chimeric form of an activating receptor such as DNAM-1 are likely to specifically target tumor cells which express high levels of PVR and Nectin-2 (Figure 1), while should be tolerant of normal cells expressing low levels of PVR and Nectin-2 [protein.atlas.org, GTEx from TCGA database and (4)]. This represents an advantage over many types of single-chain antibody-based CAR-engineered lymphocytes designed to target proteins expressed not only by tumor cells but also, at high physiological levels, by various normal cells such as CD19 and B220 (B lymphocytes and follicular dendritic cells), disialoganglioside or GD2 (neurons, skin melanocytes and peripheral nerves), human epidermal growth factor receptor 2 or HER2 (many tissues), prostate-specific membrane antigen or PSMA (kidneys, small intestine and salivary glands), etc. This non-selective tumor specificity is often the cause of high toxicity and adverse effects due to the cytotoxic reaction mediated by CAR-lymphocytes against normal tissues. So far, with a restricted expression in normal tissues and overexpression in many types of solid tumors, B7-H3 resulted a more promising therapeutic target compared to the others (76). DNAM-1 ligands PVR and Nectin-2 have been described to be absent or very scarcely expressed in normal tissue [proteinatlas.org and (73, 77)], so their targeting should hypothetically not be toxic; however, the differential expression of DNAM-1 ligands in cancer versus normal cells does not exclude a possible toxicity mediated by DNAM-1 chimeric receptor-engineered NK cells, which should be carefully explored by preclinical studies.
For a hypothetic good manufacturing practice (GMP) production and clinical use of DNAM-1 chimeric receptor-engineered NK cells, primary NK cells should be isolated through leukapheresis by the blood of a HLA-matched unrelated healthy donor, ex vivo expanded and activated, engineered for the expression of DNAM-1 chimeric receptor, expanded to be infused in cancer patients or be cryopreserved for future use (Figure 2). Different modes of administration should be considered, depending on the type and location of the tumor in the body, such as intravenous or local injection. DNAM-1 chimeric receptor, expressed at stable and high levels, should strongly compete for the binding of PVR and Nectin-2 with the agonist receptors TIGIT, TACTILE and PVRIG, thus favoring activating cytotoxic signals over inhibitory ones. The high expression of PVR and Nectin-2 in tumor cells could make them strongly susceptible to DNAM-1 chimeric receptor-engineered NK cell-mediated recognition and killing. Within days after the injection of DNAM-1 chimeric receptor-engineered NK cells, tumor cell death could occur at the tumor site and lead the patient to an objective clinical response, depending on the aggressiveness and size of primary or secondary tumor masses. To avoid recurrence, the number of administrations of DNAM-1 chimeric receptor-engineered NK cells should be carefully planned, depending on the characteristics of the tumor, such as location, extent, stage, or presence of metastasis. To enhance the anticancer efficiency, the use of DNAM-1 chimeric receptor-engineered NK cells could be combined with that of current anticancer cytotoxic drugs (78, 79), activating cytokines or mAbs recognizing immune checkpoint molecules (80). Ideally, the administration of DNAM-1 chimeric receptor-engineered NK cells should be also considered after surgical removal of solid tumor masses to avoid the risk of developing the minimal residual disease (MRD).
Figure 2 Clinical perspective of the GMP manufacturing and clinical use of DNAM-1 chimeric receptor-engineered NK cells. After leukapheresis of a healthy HLA-related donor, mature alloreactive NK cells can be isolated to be firstly ex vivo expanded and activated and then engineered for the expression of DNAM-1 chimeric receptor. Large quantities of DNAM-1 chimeric receptor-engineered NK cells can be obtained to be infused in cancer patient or cryopreserved for future use. The high expression of PVR and Nectin-2 specifically in tumor cells should facilitate their recognition mainly by DNAM-1 chimeric receptor compared to competing receptors (TIGIT, TACTILE and PVRIG), thus promoting tumor cell death. The figure was created with Biorender (https://biorender.com/).
The adoptive transfer of DNAM-1 chimeric receptor-engineered NK cells is expected to represent an innovative strategic clinical tool to help cancer patients in fighting solid tumors. Therefore, the development of preclinical and clinical studies aimed at obtaining stable, nontoxic, highly antitumor cytotoxic DNAM-1 chimeric receptor-engineered NK cells, in high quantities for cryopreservation and immediate future use, applicable to a broad spectrum of solid tumors, deserves further exploration.
The original contributions presented in the study are included in the article/Supplementary Materials, further inquiries can be directed to the corresponding author/s.
All authors listed have made a substantial, direct, and intellectual contribution to the work and approved it for publication.
This study was funded by grants from Ministero dell’Università e della Ricerca, PRIN 2020 (BeiR20Prin, CUP: E85F22000060006 to RB).
The authors declare that the research was conducted in the absence of any commercial or financial relationships that could be construed as a potential conflict of interest.
All claims expressed in this article are solely those of the authors and do not necessarily represent those of their affiliated organizations, or those of the publisher, the editors and the reviewers. Any product that may be evaluated in this article, or claim that may be made by its manufacturer, is not guaranteed or endorsed by the publisher.
The Supplementary Material for this article can be found online at: https://www.frontiersin.org/articles/10.3389/fimmu.2023.1197053/full#supplementary-material
Supplementary Figure 1 | Overall survival probability of patients with the indicated solid tumor type (tumor labelling is explained in Supplementary Table 2) in each graph carrying high (blue line) or low (red line) PVR gene expression. High PVR gene expression can correlate with a worse (A) or favorable overall survival (B). Statistically significant p values are indicated.
Supplementary Figure 2 | Overall survival probability of patients with the indicated solid tumor type (tumor labelling is explained in Supplementary Table 2 in each graph carrying high (blue line) or low (red line) NECTIN2 gene expression. High NECTIN2 gene expression can correlate with a worse (A) or favorable overall survival (B). Statistically significant p values are indicated.
1. Lanier LL. Up on the tightrope: natural killer cell activation and inhibition. Nat Immunol (2008) 9:495–502. doi: 10.1038/ni1581
2. Lanier LL. NK cell receptors. Annu Rev Immunol (1998) 16:359–93. doi: 10.1146/annurev.immunol.16.1.359
3. Biassoni R, Cantoni C, Pende D, Sivori S, Parolini S, Vitale M, et al. Human natural killer cell receptors and co-receptors. Immunol Rev (2001) 181:203–14. doi: 10.1034/j.1600-065x.2001.1810117.x
4. Uhlen M, Fagerberg L, Hallstrom BM, Lindskog C, Oksvold P, Mardinoglu A, et al. Proteomics. tissue-based map of the human proteome. Science (2015) 347:1260419. doi: 10.1126/science.1260419
5. Bottino C, Castriconi R, Pende D, Rivera P, Nanni M, Carnemolla B, et al. Identification of PVR (CD155) and nectin-2 (CD112) as cell surface ligands for the human DNAM-1 (CD226) activating molecule. J Exp Med (2003) 198:557–67. doi: 10.1084/jem.20030788
6. Xiong P, Sang HW, Zhu M. Critical roles of co-activation receptor DNAX accessory molecule-1 in natural killer cell immunity. Immunology (2015) 146:369–78. doi: 10.1111/imm.12516
7. Wang W, Erbe AK, Hank JA, Morris ZS, Sondel PM. NK cell-mediated antibody-dependent cellular cytotoxicity in cancer immunotherapy. Front Immunol (2015) 6:368. doi: 10.3389/fimmu.2015.00368
8. Li F, Liu S. Focusing on NK cells and ADCC: a promising immunotherapy approach in targeted therapy for HER2-positive breast cancer. Front Immunol (2022) 13:1083462. doi: 10.3389/fimmu.2022.1083462
9. Lucarini V, Melaiu O, Tempora P, D’Amico S, Locatelli F, Fruci D. Dendritic cells: behind the scenes of T-cell infiltration into the tumor microenvironment. Cancers (Basel) (2021) 13(3):433. doi: 10.3390/cancers13030433
10. Melaiu O, Lucarini V, Cifaldi L, Fruci D. Influence of the tumor microenvironment on NK cell function in solid tumors. Front Immunol (2019) 10:3038. doi: 10.3389/fimmu.2019.03038
11. Cozar B, Greppi M, Carpentier S, Narni-Mancinelli E, Chiossone L, Vivier E. Tumor-infiltrating natural killer cells. Cancer Discovery (2021) 11:34–44. doi: 10.1158/2159-8290.CD-20-0655
12. Demaria O, Cornen S, Daeron M, Morel Y, Medzhitov R, Vivier E. Harnessing innate immunity in cancer therapy. Nature (2019) 574:45–56. doi: 10.1038/s41586-019-1593-5
13. Lavin Y, Kobayashi S, Leader A, Amir ED, Elefant N, Bigenwald C, et al. Innate immune landscape in early lung adenocarcinoma by paired single-cell analyses. Cell (2017) 169:750–765 e717. doi: 10.1016/j.cell.2017.04.014
14. Tumino N, Nava Lauson CB, Tiberti S, Besi F, Martini S, Fiore PF, et al. The tumor microenvironment drives NK cell metabolic dysfunction leading to impaired antitumor activity. Int J Cancer (2023) 152:1698–706. doi: 10.1002/ijc.34389
15. Gemelli M, Noonan DM, Carlini V, Pelosi G, Barberis M, Ricotta R, et al. Overcoming resistance to checkpoint inhibitors: natural killer cells in non-small cell lung cancer. Front Oncol (2022) 12:886440. doi: 10.3389/fonc.2022.886440
16. Albini A, Noonan DM. Decidual-like NK cell polarization: from cancer killing to cancer nurturing. Cancer Discov (2021) 11:28–33. doi: 10.1158/2159-8290.CD-20-0796
17. Bruno A, Mortara L, Baci D, Noonan DM, Albini A. Myeloid derived suppressor cells interactions with natural killer cells and pro-angiogenic activities: roles in tumor progression. Front Immunol (2019) 10:771. doi: 10.3389/fimmu.2019.00771
18. Davis ZB, Felices M, Verneris MR, Miller JS. Natural killer cell adoptive transfer therapy: exploiting the first line of defense against cancer. Cancer J (2015) 21:486–91. doi: 10.1097/PPO.0000000000000156
19. Velardi A, Ruggeri L. Alessandro; moretta; moretta, l. NK cells: a lesson from mismatched hematopoietic transplantation. Trends Immunol (2002) 23:438–444. doi: 10.1016/s1471-4906(02)02284-6
20. Veluchamy JP, Kok N, van der Vliet HJ, Verheul HMW, de Gruijl TD, Spanholtz J. The rise of allogeneic natural killer cells as a platform for cancer immunotherapy: recent innovations and future developments. Front Immunol (2017) 8:631. doi: 10.3389/fimmu.2017.00631
21. Liu E, Marin D, Banerjee P, Macapinlac HA, Thompson P, Basar R, et al. Use of CAR-transduced natural killer cells in CD19-positive lymphoid tumors. N Engl J Med (2020) 382:545–53. doi: 10.1056/NEJMoa1910607
22. Goldenson BH, Hor P, Kaufman DS. iPSC-derived natural killer cell therapies - expansion and targeting. Front Immunol (2022) 13:841107. doi: 10.3389/fimmu.2022.841107
23. Maddineni S, Silberstein JL, Sunwoo JB. Emerging NK cell therapies for cancer and the promise of next generation engineering of iPSC-derived NK cells. J Immunother Cancer (2022) 10(5):e004693. doi: 10.1136/jitc-2022-004693
24. Lapteva N, Szmania SM, van Rhee F, Rooney CM. Clinical grade purification and expansion of natural killer cells. Crit Rev Oncog (2014) 19:121–32. doi: 10.1615/critrevoncog.2014010931
25. Kundu S, Gurney M, O’Dwyer M. Generating natural killer cells for adoptive transfer: expanding horizons. Cytotherapy (2021) 23:559–66. doi: 10.1016/j.jcyt.2020.12.002
26. Zhang W, Zhao Z, Li F. Natural killer cell dysfunction in cancer and new strategies to utilize NK cell potential for cancer immunotherapy. Mol Immunol (2022) 144:58–70. doi: 10.1016/j.molimm.2022.02.015
27. Liang S, Xu K, Niu L, Wang X, Liang Y, Zhang M, et al. Comparison of autogeneic and allogeneic natural killer cells immunotherapy on the clinical outcome of recurrent breast cancer. Onco Targets Ther (2017) 10:4273–81. doi: 10.2147/OTT.S139986
28. Sanber K, Savani B, Jain T. Graft-versus-host disease risk after chimeric antigen receptor T-cell therapy: the diametric opposition of T cells. Br J Haematol (2021) 195:660–8. doi: 10.1111/bjh.17544
29. Lu H, Zhao X, Li Z, Hu Y, Wang H. From CAR-T cells to CAR-NK cells: a developing immunotherapy method for hematological malignancies. Front Oncol (2021) 11:720501. doi: 10.3389/fonc.2021.720501
30. Kalos M, June CH. Adoptive T cell transfer for cancer immunotherapy in the era of synthetic biology. Immunity (2013) 39:49–60. doi: 10.1016/j.immuni.2013.07.002
31. Asai O, Longo DL, Tian ZG, Hornung RL, Taub DD, Ruscetti FW, et al. Suppression of graft-versus-host disease and amplification of graft-versus-tumor effects by activated natural killer cells after allogeneic bone marrow transplantation. J Clin Invest (1998) 101:1835–42. doi: 10.1172/JCI1268
32. Gill S, Olson JA, Negrin RS. Natural killer cells in allogeneic transplantation: effect on engraftment, graft- versus-tumor, and graft-versus-host responses. Biol Blood Marrow Transplant (2009) 15:765–76. doi: 10.1016/j.bbmt.2009.01.019
33. Geller MA, Miller JS. Use of allogeneic NK cells for cancer immunotherapy. Immunotherapy (2011) 3:1445–59. doi: 10.2217/imt.11.131
34. Heipertz EL, Zynda ER, Stav-Noraas TE, Hungler AD, Boucher SE, Kaur N, et al. Current perspectives on “Off-The-Shelf” allogeneic NK and CAR-NK cell therapies. Front Immunol (2021) 12:732135. doi: 10.3389/fimmu.2021.732135
35. Kennedy PR, Felices M, Miller JS. Challenges to the broad application of allogeneic natural killer cell immunotherapy of cancer. Stem Cell Res Ther (2022) 13:165. doi: 10.1186/s13287-022-02769-4
36. Davila ML, Brentjens RJ. CD19-targeted CAR T cells as novel cancer immunotherapy for relapsed or refractory b-cell acute lymphoblastic leukemia. Clin Adv Hematol Oncol (2016) 14:802–8.
37. Maalej KM, Merhi M, Inchakalody VP, Mestiri S, Alam M, Maccalli C, et al. CAR-cell therapy in the era of solid tumor treatment: current challenges and emerging therapeutic advances. Mol Cancer (2023) 22:20. doi: 10.1186/s12943-023-01723-z
38. Focaccetti C, Benvenuto M, Pighi C, Vitelli A, Napolitano F, Cotugno N, et al. DNAM-1-chimeric receptor-engineered NK cells, combined with nutlin-3a, more effectively fight neuroblastoma cells in vitro: a proof-of-concept study. Front Immunol (2022) 13:886319. doi: 10.3389/fimmu.2022.886319
39. El-Sherbiny YM, Meade JL, Holmes TD, McGonagle D, Mackie SL, Morgan AW, et al. The requirement for DNAM-1, NKG2D, and NKp46 in the natural killer cell-mediated killing of myeloma cells. Cancer Res (2007) 67:8444–9. doi: 10.1158/0008-5472.CAN-06-4230
40. Shibuya A, Campbell D, Hannum C, Yssel H, Franz-Bacon K, McClanahan T, et al. DNAM-1, a novel adhesion molecule involved in the cytolytic function of T lymphocytes. Immunity (1996) 4:573–81. doi: 10.1016/s1074-7613(00)70060-4
41. Tahara-Hanaoka S, Shibuya K, Onoda Y, Zhang H, Yamazaki S, Miyamoto A, et al. Functional characterization of DNAM-1 (CD226) interaction with its ligands PVR(CD155) and nectin-2 (PRR-2/CD112). Int Immunol (2004) 16:533–8. doi: 10.1093/intimm/dxh059
42. Shibuya K, Lanier LL, Phillips JH, Ochs HD, Shimizu K, Nakayama E, et al. Physical and functional association of LFA-1 with DNAM-1 adhesion molecule. Immunity (1999) 11:615–23. doi: 10.1016/s1074-7613(00)80136-3
43. Cifaldi L, Doria M, Cotugno N, Zicari S, Cancrini C, Palma P, et al. DNAM-1 activating receptor and its ligands: how do viruses affect the NK cell-mediated immune surveillance during the various phases of infection? Int J Mol Sci (2019) 20(15):3715. doi: 10.3390/ijms20153715
44. Enqvist M, Ask EH, Forslund E, Carlsten M, Abrahamsen G, Beziat V, et al. Coordinated expression of DNAM-1 and LFA-1 in educated NK cells. J Immunol (2015) 194:4518–27. doi: 10.4049/jimmunol.1401972
45. Chashchina A, Marklin M, Hinterleitner C, Salih HR, Heitmann JS, Klimovich B. DNAM-1/CD226 is functionally expressed on acute myeloid leukemia (AML) cells and is associated with favorable prognosis. Sci Rep (2021) 11:18012. doi: 10.1038/s41598-021-97400-6
46. Lakshmikanth T, Burke S, Ali TH, Kimpfler S, Ursini F, Ruggeri L, et al. NCRs and DNAM-1 mediate NK cell recognition and lysis of human and mouse melanoma cell lines in vitro and in vivo. J Clin Invest (2009) 119:1251–63. doi: 10.1172/JCI36022
47. Veneziani I, Infante P, Ferretti E, Melaiu O, Battistelli C, Lucarini V, et al. Nutlin-3a enhances natural killer cell-mediated killing of neuroblastoma by restoring p53-dependent expression of ligands for NKG2D and DNAM-1 receptors. Cancer Immunol Res (2021) 9:170–83. doi: 10.1158/2326-6066.CIR-20-0313
48. Guillamon CF, Martinez-Sanchez MV, Gimeno L, Mrowiec A, Martinez-Garcia J, Server-Pastor G, et al. NK cell education in tumor immune surveillance: DNAM-1/KIR receptor ratios as predictive biomarkers for solid tumor outcome. Cancer Immunol Res (2018) 6:1537–47. doi: 10.1158/2326-6066.CIR-18-0022
49. Sanchez-Correa B, Gayoso I, Bergua JM, Casado JG, Morgado S, Solana R, et al. Decreased expression of DNAM-1 on NK cells from acute myeloid leukemia patients. Immunol Cell Biol (2012) 90:109–15. doi: 10.1038/icb.2011.15
50. Sloan KE, Eustace BK, Stewart JK, Zehetmeier C, Torella C, Simeone M, et al. CD155/PVR plays a key role in cell motility during tumor cell invasion and migration. BMC Cancer (2004) 4:73. doi: 10.1186/1471-2407-4-73
51. Gao J, Zheng Q, Xin N, Wang W, Zhao C. CD155, an onco-immunologic molecule in human tumors. Cancer Sci (2017) 108:1934–8. doi: 10.1111/cas.13324
52. Casado JG, Pawelec G, Morgado S, Sanchez-Correa B, Delgado E, Gayoso I, et al. Expression of adhesion molecules and ligands for activating and costimulatory receptors involved in cell-mediated cytotoxicity in a large panel of human melanoma cell lines. Cancer Immunol Immunother (2009) 58:1517–26. doi: 10.1007/s00262-009-0682-y
53. Stamm H, Klingler F, Grossjohann EM, Muschhammer J, Vettorazzi E, Heuser M, et al. Immune checkpoints PVR and PVRL2 are prognostic markers in AML and their blockade represents a new therapeutic option. Oncogene (2018) 37:5269–80. doi: 10.1038/s41388-018-0288-y
54. Hattori N, Kawaguchi Y, Sasaki Y, Shimada S, Murai S, Abe M, et al. Monitoring TIGIT/DNAM-1 and PVR/PVRL2 immune checkpoint expression levels in allogeneic stem cell transplantation for acute myeloid leukemia. Biol Blood Marrow Transplant (2019) 25:861–7. doi: 10.1016/j.bbmt.2019.01.013
55. Lee BH, Kim JH, Kang KW, Lee SR, Park Y, Sung HJ, et al. PVR (CD155) expression as a potential prognostic marker in multiple myeloma. Biomedicines (2022) 10(5):1099. doi: 10.3390/biomedicines10051099
56. Liu WF, Quan B, Li M, Zhang F, Hu KS, Yin X. PVR-a prognostic biomarker correlated with immune cell infiltration in hepatocellular carcinoma. Diagnostics (Basel) (2022) 12(12):2953. doi: 10.3390/diagnostics12122953
57. Luo C, Ye W, Hu J, Othmane B, Li H, Chen J, et al. Poliovirus receptor (CD155)-related risk signature predicts the prognosis of bladder cancer. Front Oncol (2021) 11:660273. doi: 10.3389/fonc.2021.660273
58. Molfetta R, Zingoni A, Santoni A, Paolini R. Post-translational mechanisms regulating NK cell activating receptors and their ligands in cancer: potential targets for therapeutic intervention. Front Immunol (2019) 10:2557. doi: 10.3389/fimmu.2019.02557
59. Kamran N, Takai Y, Miyoshi J, Biswas SK, Wong JS, Gasser S. Toll-like receptor ligands induce expression of the costimulatory molecule CD155 on antigen-presenting cells. PloS One (2013) 8:e54406. doi: 10.1371/journal.pone.0054406
60. Pende D, Castriconi R, Romagnani P, Spaggiari GM, Marcenaro S, Dondero A, et al. Expression of the DNAM-1 ligands, nectin-2 (CD112) and poliovirus receptor (CD155), on dendritic cells: relevance for natural killer-dendritic cell interaction. Blood (2006) 107:2030–6. doi: 10.1182/blood-2005-07-2696
61. Soriani A, Zingoni A, Cerboni C, Iannitto ML, Ricciardi MR, Di Gialleonardo V, et al. ATM-ATR-dependent up-regulation of DNAM-1 and NKG2D ligands on multiple myeloma cells by therapeutic agents results in enhanced NK-cell susceptibility and is associated with a senescent phenotype. Blood (2009) 113:3503–11. doi: 10.1182/blood-2008-08-173914
62. Ardolino M, Zingoni A, Cerboni C, Cecere F, Soriani A, Iannitto ML, et al. DNAM-1 ligand expression on Ag-stimulated T lymphocytes is mediated by ROS-dependent activation of DNA-damage response: relevance for NK-T cell interaction. Blood (2011) 117:4778–86. doi: 10.1182/blood-2010-08-300954
63. Marrella A, Dondero A, Aiello M, Casu B, Olive D, Regis S, et al. Cell-laden hydrogel as a clinical-relevant 3D model for analyzing neuroblastoma growth, immunophenotype, and susceptibility to therapies. Front Immunol (2019) 10:1876. doi: 10.3389/fimmu.2019.01876
64. Wang W, Gao L, Wang X, Kang H, Li Y, Wang L, et al. Modulation of the poliovirus receptor expression in malignant lymphocytes by epigenetic alterations. J Immunother (2011) 34:353–61. doi: 10.1097/CJI.0b013e3182188017
65. Matusali G, Potesta M, Santoni A, Cerboni C, Doria M. The human immunodeficiency virus type 1 nef and vpu proteins downregulate the natural killer cell-activating ligand PVR. J Virol (2012) 86:4496–504. doi: 10.1128/JVI.05788-11
66. Tomasec P, Wang EC, Davison AJ, Vojtesek B, Armstrong M, Griffin C, et al. Downregulation of natural killer cell-activating ligand CD155 by human cytomegalovirus UL141. Nat Immunol (2005) 6:181–8. doi: 10.1038/ni1156
67. Zhang Q, Bi J, Zheng X, Chen Y, Wang H, Wu W, et al. Blockade of the checkpoint receptor TIGIT prevents NK cell exhaustion and elicits potent anti-tumor immunity. Nat Immunol (2018) 19:723–32. doi: 10.1038/s41590-018-0132-0
68. Georgiev H, Ravens I, Papadogianni G, Bernhardt G. Coming of age: CD96 emerges as modulator of immune responses. Front Immunol (2018) 9:1072. doi: 10.3389/fimmu.2018.01072
69. Zhu Y, Paniccia A, Schulick AC, Chen W, Koenig MR, Byers JT, et al. Identification of CD112R as a novel checkpoint for human T cells. J Exp Med (2016) 213:167–76. doi: 10.1084/jem.20150785
70. Stanietsky N, Simic H, Arapovic J, Toporik A, Levy O, Novik A, et al. The interaction of TIGIT with PVR and PVRL2 inhibits human NK cell cytotoxicity. Proc Natl Acad Sci U.S.A. (2009) 106:17858–63. doi: 10.1073/pnas.0903474106
71. Fuchs A, Cella M, Giurisato E, Shaw AS, Colonna M. Cutting edge: CD96 (tactile) promotes NK cell-target cell adhesion by interacting with the poliovirus receptor (CD155). J Immunol (2004) 172:3994–8. doi: 10.4049/jimmunol.172.7.3994
72. Sanchez-Correa B, Valhondo I, Hassouneh F, Lopez-Sejas N, Pera A, Bergua JM, et al. DNAM-1 and the TIGIT/PVRIG/TACTILE axis: novel immune checkpoints for natural killer cell-based cancer immunotherapy. Cancers (Basel) (2019) 11(6):877. doi: 10.3390/cancers11060877
73. Kucan Brlic P, Lenac Rovis T, Cinamon G, Tsukerman P, Mandelboim O, Jonjic S. Targeting PVR (CD155) and its receptors in anti-tumor therapy. Cell Mol Immunol (2019) 16:40–52. doi: 10.1038/s41423-018-0168-y
74. Carr-Wilkinson J, O’Toole K, Wood KM, Challen CC, Baker AG, Board JR, et al. High frequency of p53/MDM2/p14ARF pathway abnormalities in relapsed neuroblastoma. Clin Cancer Res (2010) 16:1108–18. doi: 10.1158/1078-0432.CCR-09-1865
75. Dondero A, Morini M, Cangelosi D, Mazzocco K, Serra M, Spaggiari GM, et al. Multiparametric flow cytometry highlights B7-H3 as a novel diagnostic/therapeutic target in GD2neg/low neuroblastoma variants. J Immunother Cancer (2021) 9(4):e002293. doi: 10.1136/jitc-2020-002293
76. Li G, Wang H, Wu H, Chen J. B7-H3-targeted CAR-T cell therapy for solid tumors. Int Rev Immunol (2022) 41:625–37. doi: 10.1080/08830185.2022.2102619
77. Oshima T, Sato S, Kato J, Ito Y, Watanabe T, Tsuji I, et al. Nectin-2 is a potential target for antibody therapy of breast and ovarian cancers. Mol Cancer (2013) 12:60. doi: 10.1186/1476-4598-12-60
78. Cifaldi L, Locatelli F, Marasco E, Moretta L, Pistoia V. Boosting natural killer cell-based immunotherapy with anticancer drugs: a perspective. Trends Mol Med (2017) 23:1156–75. doi: 10.1016/j.molmed.2017.10.002
79. Miyazato K, Hayakawa Y. Pharmacological targeting of natural killer cells for cancer immunotherapy. Cancer Sci (2020) 111:1869–75. doi: 10.1111/cas.14418
Keywords: CAR-NK cells, solid tumors, DNAM-1, NK cell-based immunotherapy, NK cell engineering
Citation: Cifaldi L, Melaiu O, Giovannoni R, Benvenuto M, Focaccetti C, Nardozi D, Barillari G and Bei R (2023) DNAM-1 chimeric receptor-engineered NK cells: a new frontier for CAR-NK cell-based immunotherapy. Front. Immunol. 14:1197053. doi: 10.3389/fimmu.2023.1197053
Received: 30 March 2023; Accepted: 26 May 2023;
Published: 08 June 2023.
Edited by:
John Maher, King’s College London, United KingdomReviewed by:
Roberta Castriconi, University of Genoa, ItalyCopyright © 2023 Cifaldi, Melaiu, Giovannoni, Benvenuto, Focaccetti, Nardozi, Barillari and Bei. This is an open-access article distributed under the terms of the Creative Commons Attribution License (CC BY). The use, distribution or reproduction in other forums is permitted, provided the original author(s) and the copyright owner(s) are credited and that the original publication in this journal is cited, in accordance with accepted academic practice. No use, distribution or reproduction is permitted which does not comply with these terms.
*Correspondence: Loredana Cifaldi, Y2lmYWxkaUBtZWQudW5pcm9tYTIuaXQ=
Disclaimer: All claims expressed in this article are solely those of the authors and do not necessarily represent those of their affiliated organizations, or those of the publisher, the editors and the reviewers. Any product that may be evaluated in this article or claim that may be made by its manufacturer is not guaranteed or endorsed by the publisher.
Research integrity at Frontiers
Learn more about the work of our research integrity team to safeguard the quality of each article we publish.