- 1Department of Hygiene, Microbiology and Public Health, Institute of Virology, Innsbruck Medical University, Innsbruck, Austria
- 2Department of Hygiene, Microbiology and Public Health, Institute of Hygiene and Medical Microbiology, Innsbruck Medical University, Innsbruck, Austria
Introduction: The rapid evolution of SARS-CoV-2 has posed a challenge to long-lasting immunity against the novel virus. Apart from neutralizing function, binding antibodies induced by vaccination or infection play an important role in containing the infection.
Methods: To determine the proportion of wild-type (WT)–generated antibodies recognizant of more recent variants, plasma samples from either SARS-CoV-2 WT-infected (n = 336) or double-mRNA (Comirnaty)–vaccinated individuals (n = 354, age and sex matched to the convalescent group) were analyzed for binding antibody capacity against the S1 protein of the BA.1 omicron variant.
Results: Overall, 38.59% (95% CI, 37.01– 40.20) of WT-generated antibodies recognized Omicron BA.1 S1 protein [28.83% (95% CI, 26.73–30.91) after infection and 43.46% (95% CI, 41.61–45.31) after vaccination; p < 0.001]. Although the proportion of WT-generated binding and neutralizing antibodies also binding to BA.1 is substantially reduced, the avidity of the remaining antibodies against the Omicron variant was non-inferior to that of the ancestral virus: Omicron: 39.7% (95% CI: 38.1–41.3) as compared to the avidity to WT: 27.0% (95% CI, 25.5–28.4), respectively (p < 0.001). Furthermore, we noticed a modestly yet statistically significant higher avidity toward the Omicron epitopes among the vaccinated group (42.2%; 95% CI, 40.51–43.94) as compared to the convalescent counterparts (36.4%; 95% CI, 33.42–38.76) (p = 0.003), even after adjusting for antibody concentration.
Discussion: Our results suggest that an aspect of functional immunity against the novel strain was considerably retained after WT contact, speculatively counteracting the impact of immune evasion toward neutralization of the strain. Higher antibody levels and cross-binding capacity among vaccinated individuals suggest an advantage of repeated exposure in generating robust immunity.
1 Introduction
Since the emergence of the SARS-CoV-2 pandemic and the subsequent introduction of vaccines, the question of individual immunity against the novel virus has been a central part of the discussion of mitigating the disease worldwide. Owing to genetic instability common to most RNA viruses, SARS-CoV-2 has been mutating at a constant pace since its emergence in December 2019.
Its high mutagenicity resulting in multiple emerging variants of concern (VOCs) has posed a challenge to vaccine- and/or infection-induced long-term immunity. In particular, the appearance of the Omicron strain represented a turn in pre-established SARS-CoV-2 immune defense, as it presented as the most antigenically distinct variant since the emergence of VOCs with the majority of the mutations affecting the S1 subdomain of the spike protein, enabling various degrees of immune escape and a potential reduction in long term protection against reinfection (1–9).
Although a number of previous studies clearly showed a greatly reduced neutralizing capacity of antibodies induced by earlier variants or vaccination (1, 3–5, 9–13), data on avidity of persisting antibodies are scarce. Antibody avidity refers to the binding strength to the target antigen and is a crucial feature of functionality. Although both binding and neutralizing antibodies have been described to wane over time (13–19), avidity has been shown to increase with the duration after contact through B cell maturation at germinal centers (14–16), thereby possibly offsetting the waning effect to an extent.
With this study, we seek to characterize concentration and binding affinity of wild-type (WT)–induced antibodies against the Omicron subvariant BA.1 (OM). For this purpose, we used plasma samples obtained from WT convalescents with no history of vaccination and age/sex-matched vaccinees who received two doses of Comirnaty (BioNTech/Pfitzer) and had no previous history of infection. Blood samples were obtained approximately 7–8 months after antigen contact in all participants adding to the comparability of the convalescent and vaccinated groups.
2 Methods
2.1 Study population and sample collection
2.1.1 Convalescent group
The post-infection cohort is composed of the seropositive adult participants of the Ischgl-2 seroprevalence study (20). Seropositivity in this cohort was determined during the so-called “first infection wave” in March/April 2020. Blood samples for this study were taken in November 2020 (7–8 months after pathogen contact). Infection was ascertained by anti-S and anti-N seropositivity, and none of the included participants reported a second infection at the time of sampling.
2.1.2 Vaccination group
This cohort consists of a subset of the ShieldVacc-2 study participants (21, 22), age- and sex-matched to the post-infection cohort. Participants in this group had received their second dose of Comirnaty (BioNTech/Pfitzer) SARS-CoV-2 mRNA vaccine in March/April 2021. Blood samples were taken in November 2021 (7–8 months after antigen contact). Only participants vaccinated with two doses of Comirnaty given 4 weeks apart and no history of SARS-CoV-2 infection at the time of sampling were included in the present analysis. In addition to questionnaire-based information, we excluded previous infection using an anti-N antibody assay.
2.2 Serological testing
2.2.1 Anti–spike-1 IgG
2.2.1.1 Wild type
Anti–SARS-CoV-2-QuantiVac-ELISA (IgG) (EUROIMMUN, Lübeck, Germany, CE-marked) was used to measure anti-S immunoglobulin G (IgG) antibodies targeting WT S1 protein (WT anti-S1) according to the manufacturer in a fully automated manner (Euroimmun Analyzer I, Lübeck Germany). In brief, plasma controls, calibrators, and diluted samples were incubated in wells precoated with S1 domain of SARS-CoV-2. Peroxidase-conjugated anti-human IgGs were used for the detection of specific antibodies that remain bound after three wash steps. The concentration [relative units per milliliter (RU/mL)] was calculated using six standards of known concentration provided by the manufacturer. A sample is considered to be positive if the concentration exceeds 11 RU/mL.
2.2.1.2 Omicron (BA.1)
Anti–SARS-CoV-2-Omikron-ELISA (IgG) (EUROIMMUN, Lübeck, Germany, CE-marked) was used to measure in the same manner as the WT anti-S IgG antibody test above with the only exception that the plates are coated with Omicron BA.1 S1 subunit (OM). Samples are considered positive above the cutoff at 11 RU/mL.
2.2.2 Avidity testing
2.2.2.1 Wild type
Plasma was diluted 1:101 in a sample buffer and transferred into two microtiter wells each, which were precoated with WT S1 protein. One well was incubated with urea at 5.5 M concentrations for 10 min, whereas the other was left untreated (PBS treated as control). Antibody avidity was calculated as ratio of urea treated and untreated sample optical density in percentages. Samples exceeding the linear range limit of 2.5 were retested in appropriate dilution of 401. The test was conducted in a fully automated manner on Immunomat (Virion/Serion, Würzburg Germany).
2.2.2.2 Omicron (BA.1)
Avidity testing for Omicron was conducted in the same manner as the WT avidity testing, except with wells precoated with OM BA.1 S1 protein.
2.2.2.3 Ascertaining the validity of the method
To present accurately comparable avidity results, we conducted internal validation of our chosen urea assay, using 17 paired samples of convalescent subjects taken 6 months apart (Supplementary Figure 1). Test runs both for WT and for OM avidity at 5.5 M urea concentration showed that there was a significant increase in urea resistant fraction of antibodies over time (baseline to follow-up sample). In addition, a test run using sample triplets (Supplementary Table 1) confirmed on the intra-assay validity of the method chosen as the mean coefficient of variation lay below 10% [mean (median): 7.6% (8.7%)].
2.2.3 Neutralization assay
Pseudovirus neutralization assays based on a replication defective vesicular stomatitis virus (VSV) were performed as earlier described (23). Briefly, VSVΔG virus expressing Green Florescent Protein (GFP) as marker gene was pseudotyped with a C-terminally truncated spike of either Wuhan (WT) or BA.1 Omicron variant. Heat-inactivated plasma samples were serially four-fold diluted starting at a 1:16 dilution and mixed with virus, to result in ~100–200 spots in control wells without serum. After 1 h of pre-incubation, plasma/virus mixes were used to infect sub-confluent 293T cells overexpressing Angiontensin converting enzyme 2 (ACE2), which were seeded one day in 96-well plates. Approximately 16 h after infection plates were analyzed in an ImmunoSpot S6 Ultra-V reader and FluoroSpot software (CTL Europe GmbH, Bonn, Germany), and infected cells were counted. Continuous 50% neutralization titers were calculated using a non-linear regression (GraphPad Prism 9.0.1, GraphPad Software, Inc., La Jolla, CA, USA). Titers > 16 were considered positive. Titers < 1 were set to 1.
2.3 Ethical statement
The study was approved by the Ethics Committee of the Medical University of Innsbruck (EC numbers 1330/2020 and 1168/2021), and the participants gave their informed consent to be included in the research.
2.4 Statistical analysis
Descriptive statistics were used for characterizing demographic data and antibody levels {mean [standard deviation (SD)] and geometric mean (GM) [95% confidence interval (CI)]}. We estimated Spearman’s correlation between WT- and OM-binding anti-S1 antibody levels as well as binding affinities to WT versus OM S1 protein. Non-parametric paired Wilcoxon test or non-parametric unpaired Kruskal–Wallis test was used to assess significant differences between immune parameters and predicting independent variables. We used multivariable linear regression model to characterize antibody concentrations as well as avidity values across multiple independent variables like age, sex, number of days after contact, and mode of antibody acquisition. Using hierarchical linear regression approach, we determined potential independent roles of variables, in particular mode of antibody acquisition, in predicting the outcome after accounting for the rest of the covariables. Statistical analysis was performed using SPSS (Version 25.0. IBM Corp., Armonk, NY, USA) and Graphpad Prism 9.3.0 (Graphpad Software Inc., La Jolla, CA, USA). A p-value of <0.05 is defined as statistically significant.
3 Results
3.1 Baseline information
Samples consisted of a total of 690 [n = 354 double mRNA–vaccinated (Comirnaty, BioNTech/Pfitzer), age- and sex-matched to n = 336 WT-infected] individuals. Groups did not differ significantly in terms of age and sex; median age of participants was 46 for either group, and both groups had a slightly higher proportion of male participants (51.8% in the infected cohort and 52.8% in the vaccinated cohort). Mean number of days between potential pathogen/vaccine contacts before sampling was 222.65 (SD, 10.68) and 219.63 (SD, 1.6), respectively. Median anti-S1 IgG antibody levels were significantly higher among the vaccinated group (Table 1).
3.2 Overall cross binding capacity
Approximately 7–8 months after antigen contact, 75.36% (n = 520/690) of individuals tested positive for anti-WT S1 antibodies and 49.6% (n = 342/690) subjects tested positive for OM specific anti-S1 antibodies (Table 1, Figures 1A, B). In individuals, who tested positive for anti-WT S1 antibodies, we found that a mean 38.59% (CI, 37.01–40.20) of WT-elicited antibodies—either through vaccination or infection—also bind to OM S1 epitopes with significant differences between modes of acquisition and age group above/below 50 years. Neither sex nor having symptomatic infection/experiencing systemic reactivity after vaccination predicted binding capacity (Table 2).
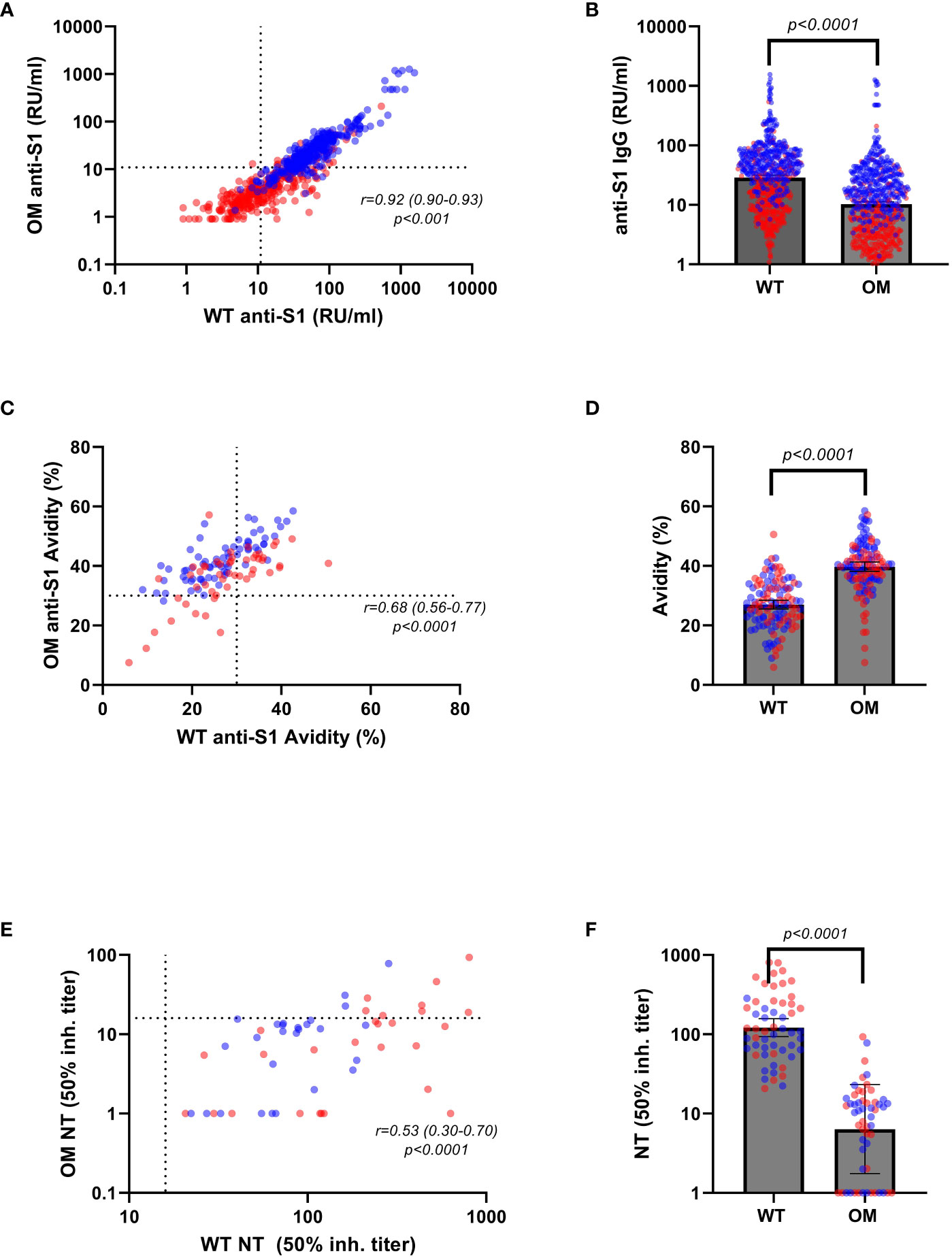
Figure 1 Anti-S1 IgG antibody levels, avidity indices, and neutralizing titers by variants. Serum levels of Omicron (OM) and wild-type (WT) antibodies (RU/mL) in convalescent (red) and vaccinated (blue) individuals correlated (A) and compared (B) by variants. WT and OM anti-S1 IgG avidity (%) in convalescent (red) and vaccinated (blue) individuals correlated (C) and compared (D) by variants. WT and OM neutralizing antibody titers in convalescent (red) and vaccinated (blue) correlated (E) and compared (F) by variants. Spearman’s correlation coefficients were calculated to assess correlations between binding antibody levels and avidity indices toward different variants (A, C, E). Absolute differences in antibody concentration and avidity index in respect to variants were calculated using non-parametric Wilcoxon test (B, D, F). Statistical significance was assumed at a p-value ≤ 0.05. RU, relative units.
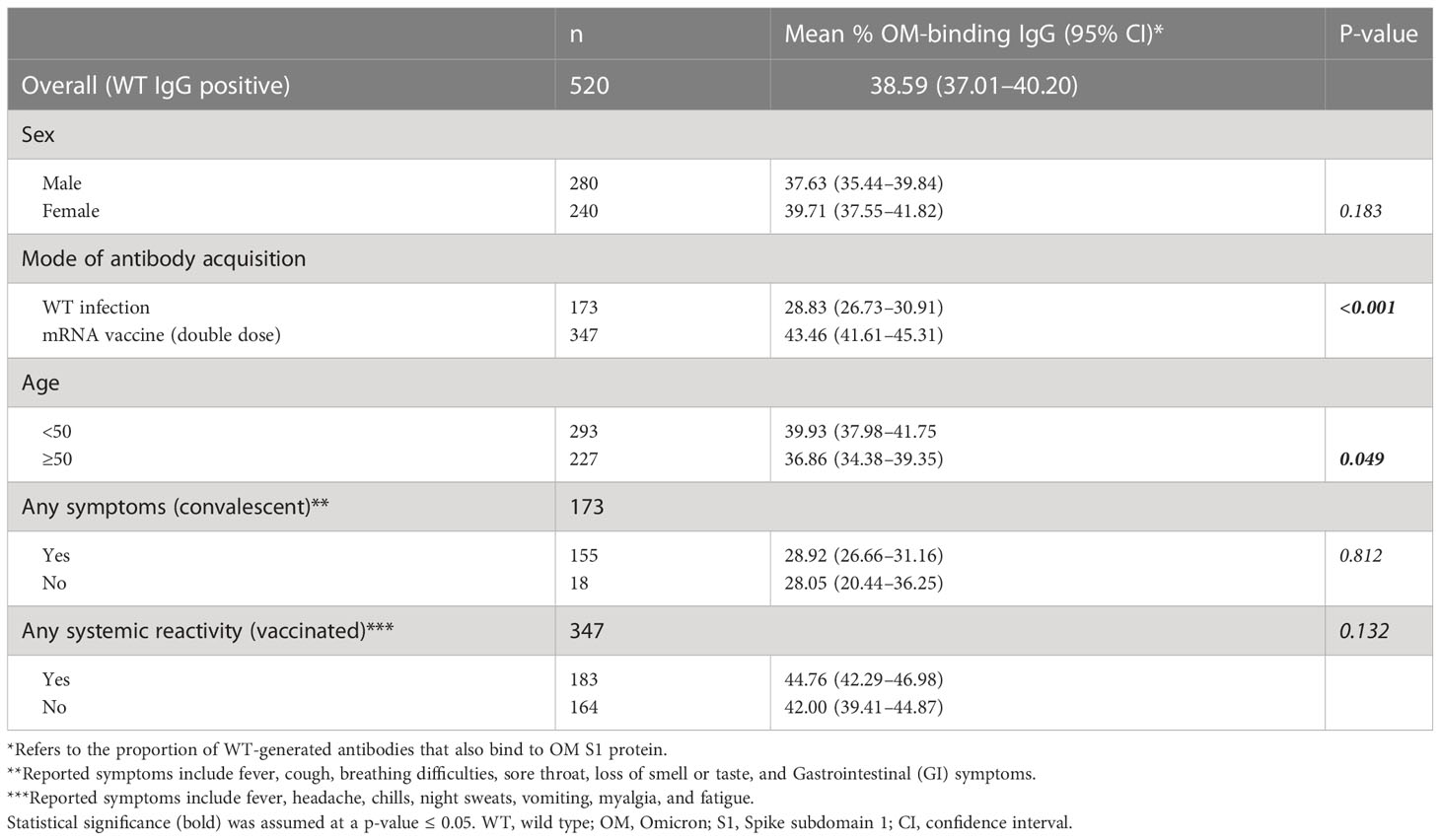
Table 2 Cross-binding capacity of wild-type generated antibodies toward OM S1 epitopes among WT S1–positive samples.
3.3 Binding affinity
For the analysis of binding affinity, we included all the samples that had tested positive for anti-OM IgG (n = 51) from the convalescent group (Table 1), as well as 69 samples from the vaccinated group. Selected samples from the vaccinated cohort were OM IgG antibody concentration-matched to the convalescent samples to prevent any influence of antibody level on avidity determination and guarantee comparability of the avidity maturation progress, because the antibody concentrations have been shown to affect avidity outcomes (24).
Contrary to our findings of higher antibody concentrations toward the WT S1 protein [GM (95% CI): 28.83 (26.36–31.84) vs. 10.24 (9.31–11.33); p ≤ 0.001], mean binding affinity was shown to be greater toward the OM epitopes than to the WT epitopes [mean (95% CI): 39.70 (38.04–41.23) vs. 26.97 (25.46–28.34); p < 0.001] (Table 3, Figures 1C, D). This finding remained significant in a multivariable regression analysis that adjusted for age, sex, and days after contact (Supplementary Tables 2, 3).
3.4 Neutralization testing
For a subcohort of 55 (randomly selected from the avidity dataset) samples [n = 28 (50.9%) convalescent], we evaluated neutralizing capacity toward WT and OM BA.1 strain (Figures 1E, F). Expectedly, we found that neutralization capacity from WT to OM was greatly reduced, with only 11 (20%) samples testing positive (cutoff ≥ 16) for OM neutralization titer, as compared to 100% (n=55) of samples that showed positive neutralization titers for WT epitope. When compared by Omicron neutralization status, positive samples showed statistically significantly higher binding antibody titers against WT (GM 140.81 vs. 66.96; p < 0.001) and Omicron (GM 44.94 vs. 27.48; p = 0.004), as well as higher avidity levels toward both WT (mean 34.14 vs. 26.55; p = 0.006) and OM (mean 43.17 vs. 39.34; p = 0.202) (Table 4).
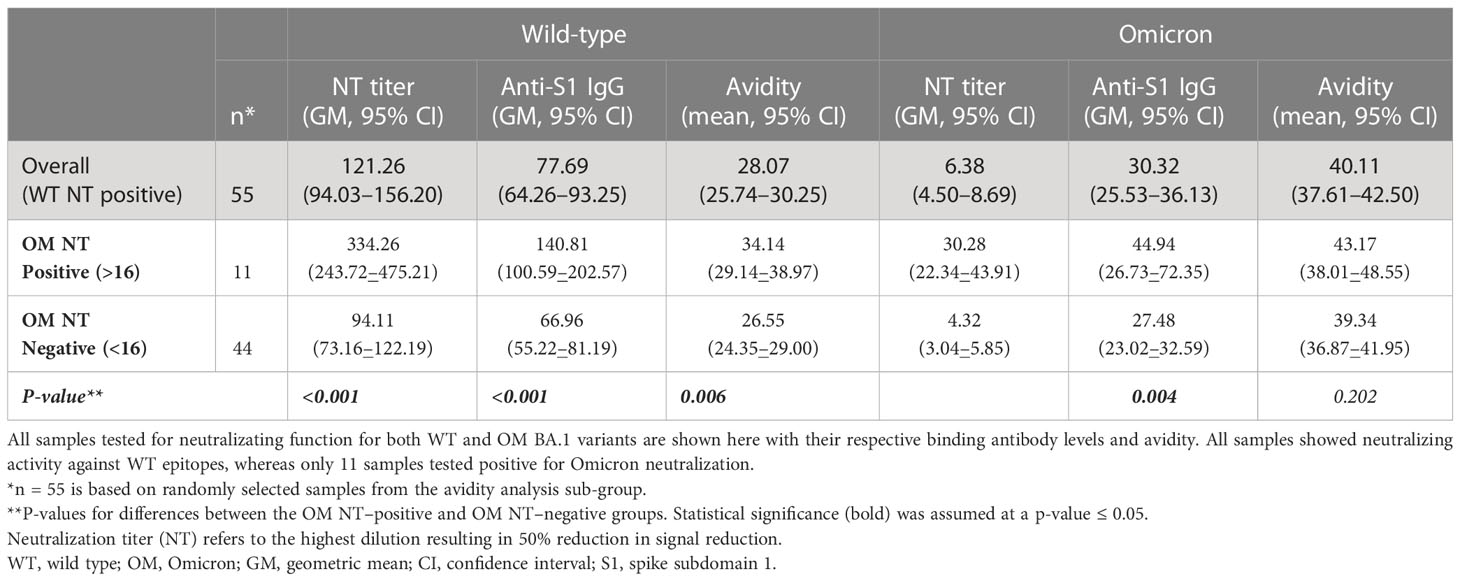
Table 4 Characterizing binding antibody concentration and avidity for wild-type and Omicron variant across neutralization status.
3.5 Analysis across mode of antibody acquisition (convalescent versus vaccinated)
Of 520 WT positive tested samples, 173 (33.3%) were convalescent samples, whereas 347 (66.7%) were vaccinated. When tested for OM BA.1, of the 342 positive samples, 51 (14.9%) were from convalescent and 291 (85.1%) from vaccinated individuals (Table 1). Antibody concentration for both WT and OM subtypes were significantly higher among vaccinated subjects as compared to the convalescent group (Table 1, Figures 2A, C, D) . Likewise, the proportion of WT-generated antibodies also binding to OM S1 was higher among vaccinated subjects (Table 2).
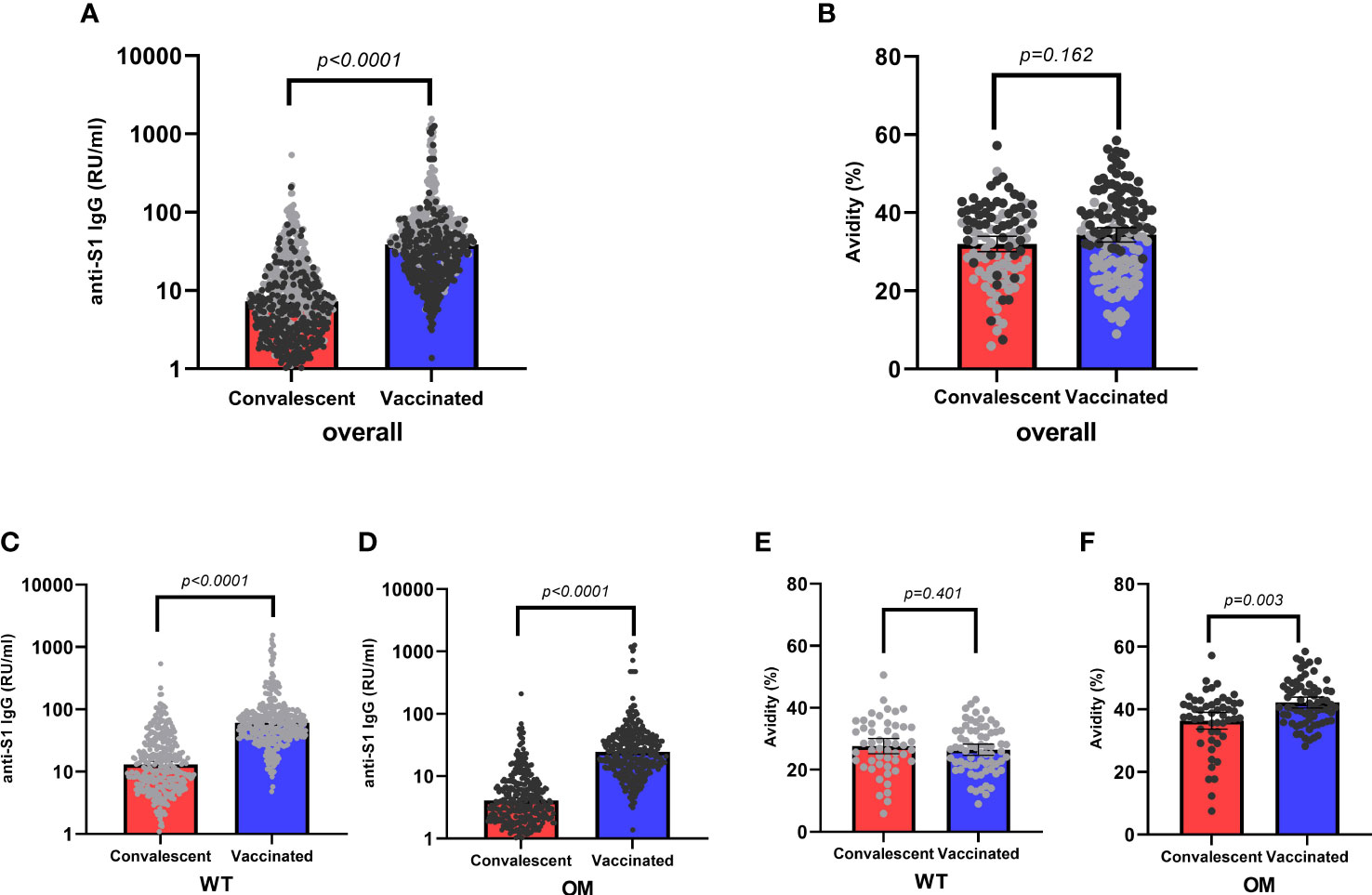
Figure 2 Anti-S1 IgG antibody levels and avidity indices by mode of acquisition. Overall antibody levels (RU/mL) (A) and avidity (%) (B) toward wild-type (WT, gray) and Omicron epitopes (OM, black) by mode of acquisition (convalescent, red; vaccinated, blue). WT (C) antibody levels and OM (D) antibodies by mode of acquisition. Avidity indices of WT S1 IgG (E) and avidity indices of OM S1 IgG (F) by mode of acquisition. Kruskal–Wallis test was used to assess absolute differences in antibody concentration and avidity toward different variants in respect to different modes of acquisition. Statistical significance was assumed at a p-value ≤ 0.05. RU, relative units.
Avidity, on the other hand, was only modestly higher among the vaccinated and only statistically significant for avidity to OM S1 (Table 3, Figures 2B, E, F). After accounting for differences in age, sex, and antibody concentration in a hierarchical regression analysis model, vaccination remained an independent predictor of higher avidity to the OM S1 (Supplementary Table 4).
4 Discussion
Because the Omicron variant carries a decent number of mutations on the spike protein with respect to the original strain, it is expected that not the entirety of binding antibodies generated after WT contact would recognize the S1 domain of the Omicron variant. Our results showing lower binding and neutralizing antibody titers toward the OM epitopes in our main analysis reflect these findings and extend on the work of Carreno et al. (12), showing a reduced binding capacity from WT infection–generated antibodies toward Omicron receptor binding domain (RBD).
Although lower titers toward the mutated antigen were expected, what did come as a surprise was the fact that antibody avidity to the Omicron variant was found to be not only non-inferior to WT avidity. In fact, binding affinity was indeed slightly higher toward OM antigen across all tested cohorts Whether or not any mutation-associated structural change of epitopes contributes to this observation cannot be determined on the basis of the data obtained in this study and may warrant further studies scrutinizing the molecular aspects of this interaction. However, considering the fact that the contact of these cohorts was limited to WT antigen only, this finding is worthy of note, especially considering the findings of other studies (12, 25) showing that pre-existing non-neutralizing antibodies and antibody avidity seem to have a protective role against severe disease course and may, among others, also play a role in the attenuated severity of the Omicron era (26, 27). Similar data involving all other relevant VOCs may shed light on this presumption if the higher binding affinity correlates across variants.
Given that sample acquisition took place 7–8 months after antigen contact, a reduced percentage of individuals testing positive even for WT protein can be anticipated. Our results of an overall 75% of WT positive individuals fits well into research showing considerable IgG antibody waning after an initial peak within the first 3 months after antigen contact. Data on antibody dynamics have further shown initially high IgG levels (especially after vaccination) to decay rapidly, eventually plateauing into stable levels, persisting up to 1 year and beyond (19, 28–32).
4.1 Mode of acquisition
Structural changes following mutation may impact binding capacity and functionality, in our study resulting in a reduced yet substantial preservation of binding capacity toward the mutated variant both in the convalescent and vaccinated groups. Although a solid correlation could be shown between OM and WT anti-S (S1) antibody titers across both groups, vaccinees presented with higher titers overall. Similar results have been found by Carreno et al. (12), who found, in their study, that one-third of convalescents tested positive for antibodies against the mutated antigen and titers were more than 7.5-fold reduced as compared to the ancestral protein. In double mRNA (Comirnaty)–vaccinated individuals, reduction was 2.5-fold. Compared to our study, higher positivity rates among convalescents as found by these authors may arise from testing different epitopes as well as earlier sampling, as convalescent and double vaccinated samples were taken no more than 3 months after contact.
Antibodies generated following vaccination also seem to have slightly superior binding affinity as compared to the post-convalescent ones at the same concentrations. Considering that antibody titers impact avidity results (24), our approach of concentration-matched avidity testing allows for better comparability of the binding affinity. Because avidity maturation is a time-dependent process, differences in the time-period between antigen contact and blood sampling between the vaccinated and convalescent groups might impact results. However, with a median of 225 days after infection in the convalescent cohort and 219 days after vaccination in the vaccinated group, this difference is highly unlikely to affect outcomes. Study groups also differed in age, yet multivariable analysis revealed no confounding impact of said age differences between study cohorts on avidity results, a finding supported by a previous study from our group that showed no difference in avidity maturation across age (33). We consider higher avidity in vaccinated individuals likely to reflect a possible benefit of repeated exposure to the antigen (28, 34, 35); a perception also in line with our finding that vaccination was shown to be a predictor of avidity maturation toward the new variant, independent of antibody titer.
4.2 The role of binding antibodies
Although neutralizing antibodies are considered the protective class, binding antibodies have been shown to correlate with protection against SARS-CoV-2 (36–38), highlighting their importance in immune response against SARS-CoV-2. In particular, Fc effector functions like antibody-dependent cell-mediated cytotoxicity and antibody-dependent cell-mediated phagocytosis seem to play a vital role (12, 38–40). Bahnan et al. (39) found significant protective properties of non-neutralizing antibodies conferred by enhanced phagocytosis, and Bates et al. show that neutralization breadth across variants was also linked to Fc effector functions, adding to the significance of characterizing antibodies beyond neutralization function (40). Furthermore, (binding) antibody retention against mutated variants can be argued to harbor protective potential even at low concentrations, because it signifies the presence of memory B cells whose activity can be ramped up by new contact.
Our characterization of neutralizing function showed a greatly reduced neutralizing capacity from WT to Omicron variants as was expected from previous reports showing the great immune evasion potential of the Omicron strain (1, 3–5, 8–13). Because neither vaccination nor past infection could be shown to confer sterilizing immunity, serum-neutralizing activity cannot solely be regarded as the key immune function (25, 41–43). Thus, looking at other parts of immune function such as binding affinity can provide insights crucial for understanding immunity against SARS-CoV-2 and preventing (severe) disease.
4.3 Strengths and limitations
Although we took considerable measures to rule out prior infection in the vaccinated cohort by both assessing participants’ history of SARS-CoV-2, as well as testing for anti-N antibodies, there remains a residual risk of past infections that might affect results. Another limitation of our research may be the fact that the Enzyme-Linked Immunosorbent Assay (ELISA) assay was coated with a particular Omicron subvariant (BA.1) limiting the generalizability of our finding to other Omicron subvariants. However, antigenic mapping studies revealed Omicron subvariants to be antigenically distinct to previous VOCs (44–46), and individuals responding to the BA.1 subvariant have been shown to exhibit more comparable humoral immune response among other Omicron subvariants as opposed to more antigenically distinct pre-Omicron variants and vice versa (44, 47). This relation between Omicron subvariants is also reflected in clinical data, showing a similar disease severity among Omicron subvariants (26).
Our study focuses on humoral aspects of immunity. Because attenuated course of SARS-CoV-2 following an infection is primarily due to pathogen-specific T-cell response, it is indeed a limitation that we lack data on cellular immunity, which might have helped better characterize our finding on the role of higher antibody avidity toward the Omicron variant. We encourage further inquiry into potential confounding or a synergistic effect of these two aspects of immunity on SARS-CoV-2 disease outcome.
One major strength of this study is its large sample size and the comparability of age and sex distribution across cohorts, ruling out possible bias that may arise from biological or demographic differences. The large sample size enabled the selection of a meaningful comparison group of titer-matched samples for avidity testing across mode of antibody acquisition. This helped circumvent the genuine impact of antibody titer on avidity outcomes. A further strength of the study is that the ELISA assays used are validated according to the European standards and approved for diagnostic testing. In contrast to previous studies presenting data collected at various points in time after contact, samples in our study were taken uniformly at the approximately same time after antigen contact, providing better grounds for genuine characterization and comparison.
5 Conclusion
Although there is significant antigenic drift between the SARS-CoV-2 WT and Omicron BA.1 variants, our study showed that a non-negligible proportion of binding antibodies elicited after WT contact (both WT infection and double mRNA vaccination) was able to recognize the Omicron BA.1 variant, with a non-inferior binding affinity to the mutated epitopes 7–8 months after contact. Considering the importance of highly functional binding antibodies in combatting viral infections, this can be regarded as a relevant part of immunity. However, our results may not warrant a presumption of attenuated severity of the Omicron era of the SARS-CoV-2 pandemic without similar data examining other VOCs with distinct disease course.
Data availability statement
The raw data supporting the conclusions of this article will be made available by the authors, without undue reservation.
Ethics statement
The studies involving human participants were reviewed and approved by Ethikkommission der Medizinischen Universität Innsbruck. The patients/participants provided their written informed consent to participate in this study.
Author contributions
Conceptualization: WB. Data acquisition: WB, TH, DB, and BF. Laboratory: WB, VF, MH, AR, and MS. Data analysis and Interpretation: WB and TH. Writing—original draft: WB and TH. Writing—revision and editing: WB, TH, BF, JK, DL, HB, DB, AR, VF, MH, MS, and RW. All authors contributed to the article and approved the submitted version.
Funding
The study was funded by the Virology Diagnostic Laboratory (project number D155140-010-010) of the Medical University of Innsbruck.
Acknowledgments
We thank Bianca Neurauter, Eva Hochmuth, Luiza Hoch, and the whole study organization team, as well as the entire diagnostic team, particularly Albert Falch, for their great effort and assistance.
Conflict of interest
DB declares to hold shares of Pfizer.
The remaining authors declare that the research was conducted in the absence of any commercial or financial relationships that could be construed as a potential conflict of interest.
Publisher’s note
All claims expressed in this article are solely those of the authors and do not necessarily represent those of their affiliated organizations, or those of the publisher, the editors and the reviewers. Any product that may be evaluated in this article, or claim that may be made by its manufacturer, is not guaranteed or endorsed by the publisher.
Supplementary material
The Supplementary Material for this article can be found online at: https://www.frontiersin.org/articles/10.3389/fimmu.2023.1196988/full#supplementary-material
Supplementary Figure 1 | Course of anti-S IgG avidity over a period of 6 months using samples from wild-type convalescent individuals. A &B: rise in IgG antibody avidity towards wild-type S1. C & D: rise in IgG antibody avidity towards Omicron BA.1. S1. i1 samples were taken approximately 4-6 weeks post pathogen contact and i2 samples exactly 6 months after the baseline (i1) sampling. WTAV= wild-type avidity, OMAV=Omicron (BA1) avidity i1=Ischgl 1, i2= Ischgl 2, S1= Spike protein 1.
References
1. Cao Y, Wang J, Jian F, Xiao T, Song W, Yisimayi A, et al. Omicron escapes the majority of existing SARS-CoV-2 neutralizing antibodies. Nature (2022) 602(7898):657–63. doi: 10.1038/s41586-021-04385-3
2. He X, Hong W, Pan X, Lu G, Wei X. SARS-CoV-2 Omicron variant: characteristics and prevention. MedComm (2021) 2(4):838–45. doi: 10.1002/mco2.110
3. Liu L, Iketani S, Guo Y, Chan JF-W, Wang M, Liu L, et al. Striking antibody evasion manifested by the Omicron variant of SARS-CoV-2. Nature (2022) 602(7898):676–81. doi: 10.1038/s41586-021-04388-0
4. Planas D, Saunders N, Maes P, Guivel-Benhassine F, Planchais C, Buchrieser J, et al. Considerable escape of SARS-CoV-2 Omicron to antibody neutralization. Nature (2022) 602(7898):671–5. doi: 10.1038/s41586-021-04389-z
5. van der Straten K, Guerra D, van Gils MJ, Bontjer I, Caniels TG, van Willigen HD, et al. Antigenic cartography using sera from sequence-confirmed SARS-CoV-2 variants of concern infections reveals antigenic divergence of Omicron. Immunity (2022) 55(9):1725–1731.e4. doi: 10.1016/j.immuni.2022.07.018
6. CoVariants: 21K (Omicron) (2023). Available at: https://covariants.org/variants/21K.Omicron.
7. GISAID - hCov19 variants (2023). Available at: https://gisaid.org/hcov19-variants/.
8. Pulliam JRC, van Schalkwyk C, Govender N, Gottberg A, von Cohen C, Groome MJ, et al. Increased risk of SARS-CoV-2 reinfection associated with emergence of Omicron in south Africa. Science (2022) 376(6593):eabn4947. doi: 10.1126/science.abn4947
9. Rössler A, Riepler L, Bante D, von Laer D, Kimpel J. SARS-CoV-2 Omicron variant neutralization in serum from vaccinated and convalescent persons. N Engl J Med (2022) 386(7):698–700. doi: 10.1056/NEJMc2119236
10. Cameroni E, Bowen JE, Rosen LE, Saliba C, Zepeda SK, Culap K, et al. Broadly neutralizing antibodies overcome SARS-CoV-2 Omicron antigenic shift. Nature (2022) 602(7898):664–70. doi: 10.1038/s41586-021-04386-2
11. Cele S, Jackson L, Khoury DS, Khan K, Moyo-Gwete T, Tegally H, et al. Omicron extensively but incompletely escapes pfizer BNT162b2 neutralization. Nature (2022) 602(7898):654–6. doi: 10.1038/s41586-021-04387-1
12. Carreño JM, Alshammary H, Tcheou J, Singh G, Raskin AJ, Kawabata H, et al. Activity of convalescent and vaccine serum against SARS-CoV-2 Omicron. Nature (2022) 602(7898):682–8. doi: 10.1038/s41586-022-04399-5
13. Evans JP, Zeng C, Carlin C, Lozanski G, Saif LJ, Oltz EM, et al. Neutralizing antibody responses elicited by SARS-CoV-2 mRNA vaccination wane over time and are boosted by breakthrough infection. Sci Transl Med (2022) 14(637):eabn8057. doi: 10.1126/scitranslmed.abn8057
14. Garcia L, Woudenberg T, Rosado J, Dyer AH, Donnadieu F, Planas D, et al. Kinetics of the SARS-CoV-2 antibody avidity response following infection and vaccination. Viruses (2022) 14(7). doi: 10.3390/v14071491
15. Monroe JM, Haralambieva IH, Warner ND, Grill DE, Quach HQ, Kennedy RB. Longitudinal antibody titer, avidity, and neutralizing responses after SARS-CoV-2 infection. Heliyon (2022) 8(11):e11676. doi: 10.1016/j.heliyon.2022.e11676
16. Tauzin A, Gendron-Lepage G, Nayrac M, Anand SP, Bourassa C, Medjahed H, et al. Evolution of anti-RBD IgG avidity following SARS-CoV-2 infection. Viruses (2022) 14(3). doi: 10.3390/v14030532
17. Pegu A, O'Connell SE, Schmidt SD, O'Dell S, Talana CA, Lai L, et al. Durability of mRNA-1273 vaccine-induced antibodies against SARS-CoV-2 variants. Science (2021) 373(6561):1372–7. doi: 10.1126/science.abj4176
18. Israel A, Shenhar Y, Green I, Merzon E, Golan-Cohen A, Schäffer AA, et al. Large-Scale study of antibody titer decay following BNT162b2 mRNA vaccine or SARS-CoV-2 infection. Vaccines (Basel) (2021) 10(1). doi: 10.3390/vaccines10010064
19. Gaebler C, Wang Z, Lorenzi JC, Muecksch F, Finkin S, Tokuyama M, et al. Evolution of antibody immunity to SARS-CoV-2. Nature (2021) 591(7851):639–44. doi: 10.1038/s41586-021-03207-w
20. Borena W, Bánki Z, Bates K, Winner H, Riepler L, Rössler A, et al. Persistence of immunity to SARS-CoV-2 over time in the ski resort ischgl. EBioMedicine (2021) 70:103534. doi: 10.1016/j.ebiom.2021.103534
21. Seekircher L, Bánki Z, Kimpel J, Rössler A, Schäfer H, Falkensammer B, et al. Immune response after two doses of the BNT162b2 COVID-19 vaccine and risk of SARS-CoV-2 breakthrough infection in Tyrol, Austria: an open-label, observational phase 4 trial. (2023). doi: 10.1016/S2666-5247(23)00107-6
22. Bánki Z, Seekircher L, Falkensammer B, Bante D, Schäfer H, Harthaller T, et al. Six-month follow-up of immune responses after a rapid mass vaccination against SARS-CoV-2 with BNT162b2 in the district of Schwaz/Austria. Viruses (2022) 14(8). doi: 10.3390/v14081642
23. Riepler L, Rössler A, Falch A, Volland A, Borena W, Laer von D, et al. Comparison of four SARS-CoV-2 neutralization assays. Vaccines (Basel) (2020) 9(1). doi: 10.3390/vaccines9010013
24. Nurmi V, Hedman L, Perdomo MF, Weseslindtner L, Hedman K. Comparison of approaches for IgG avidity calculation and a new highly sensitive and specific method with broad dynamic range. Int J Infect Dis (2021) 110:479–87. doi: 10.1016/j.ijid.2021.05.047
25. Manuylov V, Burgasova O, Borisova O, Smetanina S, Vasina D, Grigoriev I, et al. Avidity of IgG to SARS-CoV-2 RBD as a prognostic factor for the severity of COVID-19 reinfection. Viruses (2022) 14(3). doi: 10.3390/v14030617
26. Wolter N, Jassat W, Walaza S, Welch R, Moultrie H, Groome MJ, et al. Clinical severity of SARS-CoV-2 Omicron BA.4 and BA.5 lineages compared to BA.1 and delta in south Africa. Nat Commun (2022) 13(1):5860. doi: 10.1038/s41467-022-33614-0
27. Menni C, Valdes AM, Polidori L, Antonelli M, Penamakuri S, Nogal A, et al. Symptom prevalence, duration, and risk of hospital admission in individuals infected with SARS-CoV-2 during periods of Omicron and delta variant dominance: a prospective observational study from the ZOE COVID study. Lancet (2022) 399(10335):1618–24. doi: 10.1016/S0140-6736(22)00327-0
28. Lagousi T, Routsias J, Mavrouli M, Papadatou I, Geropeppa M, Spoulou V. Comparative characterization of human antibody response induced by BNT162b2 vaccination vs. SARS-CoV-2 wild-type infection. Vaccines (Basel) (2022) 10(8). doi: 10.3390/vaccines10081210
29. Zuiani A, Wesemann DR. Antibody dynamics and durability in coronavirus disease-19. Clinics Lab Med (2022) 42(1):85–96. doi: 10.1016/j.cll.2021.10.004
30. Guo L, Wang G, Wang Y, Zhang Q, Ren L, Gu X, et al. SARS-CoV-2-specific antibody and T-cell responses 1 year after infection in people recovered from COVID-19: a longitudinal cohort study. Lancet Microbe (2022) 3(5):e348–56. doi: 10.1016/S2666-5247(22)00036-2
31. Keshavarz B, Richards NE, Workman LJ, Patel J, Muehling LM, Canderan G, et al. Trajectory of IgG to SARS-CoV-2 after vaccination with BNT162b2 or mRNA-1273 in an employee cohort and comparison with natural infection. Front Immunol (2022) 13:850987. doi: 10.3389/fimmu.2022.850987
32. Townsend JP, Hassler HB, Sah P, Galvani AP, Dornburg A. The durability of natural infection and vaccine-induced immunity against future infection by SARS-CoV-2. Proc Natl Acad Sci U.S.A. (2022) 119(31):e2204336119. doi: 10.1073/pnas.2204336119
33. Pichler D, Baumgartner M, Kimpel J, Rössler A, Riepler L, Bates K, et al. Marked increase in avidity of SARS-CoV-2 antibodies 7-8 months after infection is not diminished in old age. J Infect Dis (2021) 224(5):764–70. doi: 10.1093/infdis/jiab300
34. Dapporto F, Marchi S, Leonardi M, Piu P, Lovreglio P, Decaro N, et al. Antibody avidity and neutralizing response against SARS-CoV-2 Omicron variant after infection or vaccination. J Immunol Res (2022) 2022:4813199. doi: 10.1155/2022/4813199
35. Nakagama Y, Candray K, Kaku N, Komase Y, Rodriguez-Funes M-V, Dominguez R, et al. Antibody avidity maturation, following recovery from infection or the booster vaccination, grants breadth in SARS-CoV-2 neutralizing capacity. J Infect Dis (2022). doi: 10.1093/infdis/jiac492
36. Earle KA, Ambrosino DM, Fiore-Gartland A, Goldblatt D, Gilbert PB, Siber GR, et al. Evidence for antibody as a protective correlate for COVID-19 vaccines. Vaccine (2021) 39(32):4423–8. doi: 10.1016/j.vaccine.2021.05.063
37. Gilbert PB, Montefiori DC, McDermott AB, Fong Y, Benkeser D, Deng W, et al. Immune correlates analysis of the mRNA-1273 COVID-19 vaccine efficacy clinical trial. Science (2022) 375(6576):43–50. doi: 10.1126/science.abm3425
38. Goldblatt D, Alter G, Crotty S, Plotkin SA. Correlates of protection against SARS-CoV-2 infection and COVID-19 disease. Immunol Rev (2022) 310(1):6–26. doi: 10.1111/imr.13091
39. Bahnan W, Wrighton S, Sundwall M, Bläckberg A, Larsson O, Höglund U, et al. Spike-dependent opsonization indicates both dose-dependent inhibition of phagocytosis and that non-neutralizing antibodies can confer protection to SARS-CoV-2. Front Immunol (2021) 12:808932. doi: 10.3389/fimmu.2021.808932
40. Bates TA, Lu P, Kang YJ, Schoen D, Thornton M, McBride SK, et al. BNT162b2-induced neutralizing and non-neutralizing antibody functions against SARS-CoV-2 diminish with age. Cell Rep (2022) 41(4):111544. doi: 10.1016/j.celrep.2022.111544
41. Beukenhorst AL, Koch CM, Hadjichrysanthou C, Alter G, Wolf F, de Anderson RM, et al. SARS-CoV-2 elicits non-sterilizing immunity and evades vaccine-induced immunity: implications for future vaccination strategies. Eur J Epidemiol (2023) 38(3):237–42. doi: 10.1007/s10654-023-00965-x
42. Focosi D, Maggi F, Casadevall A. Mucosal vaccines, sterilizing immunity, and the future of SARS-CoV-2 virulence. Viruses (2022) 14(2). doi: 10.3390/v14020187
43. Wahl I, Wardemann H. Sterilizing immunity: understanding COVID-19. Immunity (2022) 55(12):2231–5. doi: 10.1016/j.immuni.2022.10.017
44. Xia H, Yeung J, Kalveram B, Bills CJ, Chen JY-C, Kurhade C, et al. Cross-neutralization and viral fitness of SARS-CoV-2 Omicron sublineages. Emerging Microbes Infections (2023) 12(1):e2161422. doi: 10.1080/22221751.2022.2161422
45. Wilks SH, Mühlemann B, Shen X, Türeli S, LeGresley EB, Netzl A, et al. Mapping SARS-CoV-2 antigenic relationships and serological responses. bioRxiv (2022):2022.01.28.477987. doi: 10.1101/2022.01.28.477987
46. Rössler A, Netzl A, Knabl L, Bante D, Wilks SH, Borena W, et al. Characterizing SARS-CoV-2 neutralization profiles after bivalent boosting using antigenic cartography. medRxiv (Preprint) (2023) 2023.05.02.23289412; doi: 10.1101/2023.05.02.23289412
Keywords: COVID-19, binding antibodies, avidity, mutation, humoral immunity, convalescent, S1 domain, ancestral strain
Citation: Harthaller T, Falkensammer B, Bante D, Huber M, Schmitt M, Benainouna H, Rössler A, Fleischer V, von Laer D, Kimpel J, Würzner R and Borena W (2023) Retained avidity despite reduced cross-binding and cross-neutralizing antibody levels to Omicron after SARS-COV-2 wild-type infection or mRNA double vaccination. Front. Immunol. 14:1196988. doi: 10.3389/fimmu.2023.1196988
Received: 30 March 2023; Accepted: 29 June 2023;
Published: 21 July 2023.
Edited by:
Nimesh Gupta, National Institute of Immunology (NII), IndiaReviewed by:
Tesfaye Gelanew, Armauer Hansen Research Institute (AHRI), EthiopiaTom Tipton, University of Oxford, United Kingdom
Copyright © 2023 Harthaller, Falkensammer, Bante, Huber, Schmitt, Benainouna, Rössler, Fleischer, von Laer, Kimpel, Würzner and Borena. This is an open-access article distributed under the terms of the Creative Commons Attribution License (CC BY). The use, distribution or reproduction in other forums is permitted, provided the original author(s) and the copyright owner(s) are credited and that the original publication in this journal is cited, in accordance with accepted academic practice. No use, distribution or reproduction is permitted which does not comply with these terms.
*Correspondence: Wegene Borena, wegene.borena@i-med.ac.at