- 1Laboratorio de Genómica del Cáncer, Instituto Nacional de Medicina Genómica (INMEGEN), Mexico City, Mexico
- 2Programa de Doctorado, Posgrado en Ciencias Biológicas, Unidad de Posgrado, Universidad Nacional Autónoma de México (UNAM), Mexico City, Mexico
- 3Laboratorio de Epigenética, Instituto Nacional de Medicina Genómica (INMEGEN), Mexico City, Mexico
Breast cancer is the most frequently diagnosed malignancy and the leading cause of cancer-related death in women worldwide. Breast cancer development and progression are mainly associated with tumor-intrinsic alterations in diverse genes and signaling pathways and with tumor-extrinsic dysregulations linked to the tumor immune microenvironment. Significantly, abnormal expression of lncRNAs affects the tumor immune microenvironment characteristics and modulates the behavior of different cancer types, including breast cancer. In this review, we provide the current advances about the role of lncRNAs as tumor-intrinsic and tumor-extrinsic modulators of the antitumoral immune response and the immune microenvironment in breast cancer, as well as lncRNAs which are potential biomarkers of tumor immune microenvironment and clinicopathological characteristics in patients, suggesting that lncRNAs are potential targets for immunotherapy in breast cancer.
1 Introduction
Breast cancer (BC) is the most frequently diagnosed malignancy and the leading cause of cancer-related death in women worldwide (1, 2). BC is a multifactorial and heterogeneous disease that includes well-defined histological types and protein markers, such as estrogen receptor (ER), progesterone receptor (PR), human epidermal growth factor receptor 2 (HER2), and Ki-67 (1, 3–5). According to the PAM50 gene signature, BC is classified into Luminal A (LA), Luminal B (LB), HER2-enriched, and Basal-like (BL) subtypes. Remarkably, luminal BCs represent around 60 to 70% of diagnosed cases and are frequently associated with improved prognosis, in contrast to non-luminal subtypes (1, 6, 7). Understanding the alterations in specific genes and disrupted signaling pathways involved in BC is essential to unravel the underlying mechanisms of development and progression. In this regard, accumulated evidence has shown recurrent alterations in diverse genes (i.e., TP53, ESR1, PIK3CA, PTEN, CDH1, GATA3, CCND1, FGFR1/2, ERBB2, CDKN2A/2B, MYC and BRCA1/2) as well as dysregulations in various signaling pathways (i.e., hormone receptors, DNA damage repair, PI3K/AKT/mTOR, MAPK/ERK, TGF-β, NFκB, WNT/β-Catenin, Notch, Hippo, and SHH), which are associated with cell survival, proliferation, epithelial-mesenchymal transition (EMT), therapy resistance, immune evasion and tumor immune microenvironment (TIME) alterations in BC (1, 8–19).
The TIME consists of dynamic niches where cancer cells coexist and interact with diverse lymphoid (i.e., natural killer (NK) cells, B cells, CD4+ T cells, CD8+ T cells, regulatory T cells, and T follicular helper cells) and myeloid immune cell populations (i.e., dendritic cells (DCs), mast cells, myeloid-derived suppressor cells (MDSCs), M0, M1, M2 macrophages, and neutrophils), as well as with soluble factors secreted by these cells (i.e., cytokines and chemokines) in the extracellular matrix. Notably, the cancer genotype and phenotype have a crucial role in the TIME’s composition and functionality, varying depending on the cancer type and clinical stage. In this context, TIME is essential at primary, pre-metastatic, and metastatic sites (20–22). Therefore, the immune context in cancer is associated with prognosis and therapeutic efficacy in patients (23, 24). Previous articles have reviewed the cancer-immunity cycle and the cancer-immune set point for a better understanding of cancer immunobiology (25, 26). Remarkably, several studies have characterized and analyzed the composition and functionality of tumor-infiltrating immune cells (TIICs) across different BC subtypes, evidencing significant associations with prognosis in patients (27–34). Immunotherapies for BC treatment based on CAR T cells, CAR NK cells, immune-checkpoint (IC) inhibitors, cytokine modulation, chemotherapy drugs to induce immunogenic cell death, and personalized vaccines related to tumor-associated antigens are being tested in clinical trials (35). Despite these advances, there is still a lack of knowledge to understand BC immunobiology fully. A fascinating research field focused on long non-coding RNAs (lncRNAs) is being explored in this context.
LncRNAs are non-protein-coding transcripts of more than 200 nucleotides in length and are classified according to their location and orientation relative to protein-coding genes into sense, anti-sense, intronic, intergenic, and bidirectional (36, 37). Notably, lncRNAs are frequently transcribed by RNA polymerase II (Pol II) and III (Pol III). Pol II-transcribed lncRNAs are spliced, bear 7-methyl guanosine caps at the 5’ end, and have polyadenylated tails at the 3’ end. In contrast, Pol III-transcribed lncRNAs lack caps and poly-A tails. Remarkably, the expression of lncRNAs is lower compared to protein-coding genes. However, lncRNAs exhibit higher tissue and cell specificity, highlighting their regulatory roles (36, 37).
LncRNAs may act in the nucleus or cytoplasm cell fraction, exhibiting a wide range of functions. In a non-pathological context, lncRNAs have essential roles in diverse biological processes, such as regulation of gene expression, chromatin modification, genomic imprinting, and transcriptional and translational processing (36, 37). These functions are mainly achieved due to lncRNA may interact with diverse DNA elements (i.e., exons, introns, and promoters), RNA species (i.e., mRNAs, miRNAs, and other lncRNAs), proteins (i.e., related to epigenetic, transcriptional, translational processes and extracellular vesicles). Previous findings have demonstrated that dysregulation of lncRNAs is associated with cancer biology, evidencing that these molecules are critical modulators of cancer signaling pathways and may act as oncogenes and tumor suppressors, showing versatile and complex roles associated with diverse hallmarks of cancer (38–43). Notably, various reports have evidenced that functional mechanisms and dysregulations associated with various lncRNAs (i.e., SPRY4-IT1, DANCR, PVT1, TUSC8, ATV1, LINC00617, PICART1, APOC1P1-3, SERM, and SERT) are promoters of cell proliferation, invasion, migration, apoptosis, stemness, and drug resistance in BC (44). Particularly, recent investigations have demonstrated the importance of lncRNAs in BC immunobiology, showing that lncRNAs are essential players in the antitumoral immune response, immune evasion mechanisms, and composition and functionality of the TIME. Abnormal expression of lncRNAs has been shown to affect the immune phenotypes across different cancer types, including BC (45–48). In this review, we provide the current advances about the role of lncRNAs as tumor-intrinsic and tumor-extrinsic modulators of the antitumoral immune response and TIME in BC, as well as lncRNAs which are potential biomarkers of the TIME and clinicopathological characteristics in BC patients.
2 LncRNAs as tumor-intrinsic modulators of the antitumoral immune response in BC
Previous research demonstrated that metabolic changes, loss of antigenicity, upregulation of immune inhibitory factors, and alterations in the TIME are important tumor-intrinsic mechanisms of immune evasion and immunotherapy resistance across different cancer types (23, 49, 50). In addition, the dysregulation of oncogenic pathways, such as WNT/β-catenin, MYC, LKB1, PTEN, and TP53, have a crucial role in the promotion and suppression of local antitumor immune response, depending on the cell context and cancer type (8). Prior findings evidenced that BRCA1, BRCA2, and TP53 mutations are associated with high mutational burden, neoantigen load, tumor-infiltrating lymphocyte density, high cytolytic activity, and improved prognosis in BC. Interestingly, the crosstalk between BRCA1/BRCA2 alterations with NFkB, NOTCH, and PTEN signaling pathways hampers the immune response in BC (51–59). Remarkably, recent studies have evidenced that lncRNAs are critical regulators of cancer immunobiology (45–48). In this regard, the role of diverse lncRNAs as tumor-intrinsic modulators of BC immunobiology has been explored, identifying lncRNAs that function as promoters and suppressors of the antitumoral immune response.
2.1 Tumor-intrinsic lncRNAs as promoters of the antitumoral immune response in BC
Recent findings have evidenced the regulatory roles of lncRNAs as tumor-intrinsic promoters of the antitumoral immune response in BC. Salama et al. identified that triple-negative breast cancers (TNBC) exhibit a high expression of PD-L1 and a low expression of XIST. Notably, the XIST knockdown promotes an increased expression of PD-L1 in TNBC cells (60). In addition, Zhao et al. evidenced that the XIST knockdown promotes macrophage polarization from M1 to M2, supporting the proliferation and migration of BC cell lines (61). Another research demonstrated that the XIST loss upregulates the c-Met/MSN signaling pathway in TNBC, promoting brain metastasis. Specifically, the XIST loss promotes the microglia reprogramming from M1 to M2 macrophages by exosomal miR-503 releasing, STAT3, and NFκB pathways. Furthermore, BL and TNBC patients have a low expression of XIST, which is associated with poor metastasis-free survival (62). In addition, Hamed et al. showed that the oleuropein compound promotes the expression of XIST and the inhibition of miR194-5p/PD-L1 in TNBC, suggesting the feasibility of modulating the BC immunobiology by targeting lncRNAs and IC inhibitors (63). Overall, XIST is a positive regulator of the antitumoral immune response by preventing PD-L1 expression and M2 macrophage-related phenotypes in BC (Figure 1).
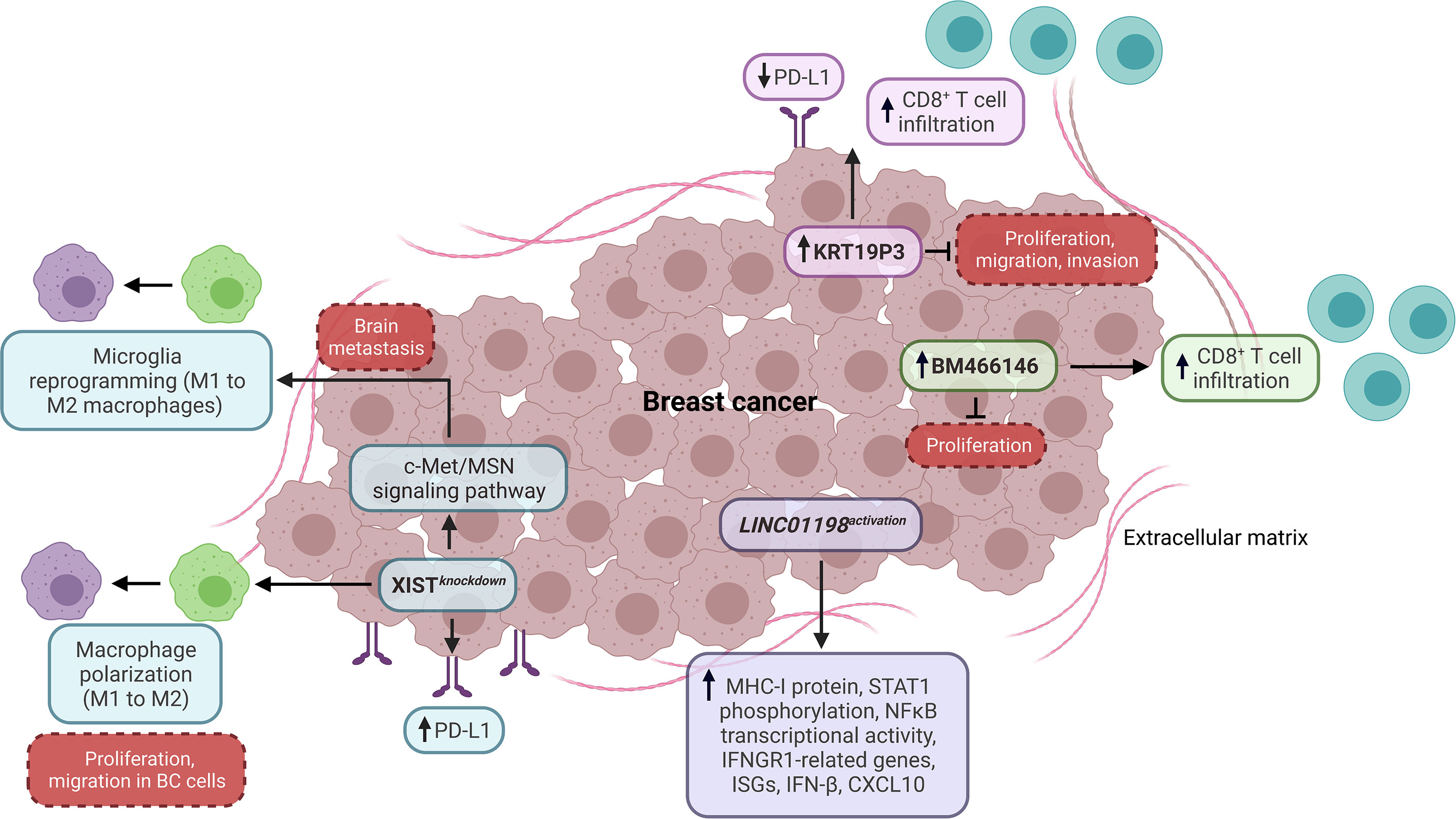
Figure 1 Impact of lncRNAs as tumor-intrinsic promoters of the immune response in BC. LncRNAs, including XIST, KRT19P3, BM466146, and LINC01198, function as tumor-intrinsic promoters of the immune response in BC, preventing PD-L1 expression, macrophage polarization, and promoting CD8+ T cell infiltration, as well as the expression of genes and proteins associated with antitumoral immune response. Dashed boxes in red denote cancer-related phenotypes. ISGs, interferon-stimulated genes.
Another study showed that the high expression of KRT19P3 is related to the low expression of PD-L1 and high infiltration of CD8+ T cells in BC, indicating that this lncRNA might have an essential role in the T cell function through the PD-L1 modulation. Also, KRT19P3 decreases proliferation, migration, and invasion in vitro (64) (Figure 1). Otherwise, Beltran-Anaya et al. found that LncKLHDC7B is enriched in TNBC immunomodulatory subtype samples with high immunophenoscore values. The silencing of this lncRNA promotes cell migration and invasion while decreasing apoptosis in vitro. In addition, the low expression of LncKLHDC7B is associated with recurrence, metastatic events, and reduced survival in TNBC patients (65). In other research, Zhang et al. found that the expression of lncRNA BM466146 positively and negatively correlates with the infiltration level of CD8+ T cells and the Ki-67 index in BC patients, respectively. Particularly, BM466146 could upregulate the CXCL13 expression to recruit CD8+ T cells to the BC immune microenvironment. Also, the overexpression of BM466146 reduces the proliferation in vitro, while the high expression of this lncRNA is associated with increased overall survival (OS) in BC patients (66). In addition, an investigation based on an innovative CRISPR activation screening strategy showed that LINC01198 is suppressed in diverse cancer types, including BC. Additional analyses demonstrated that IFNGR1-related genes, MHC-I protein expression, and STAT1 phosphorylation increase when LINC01198 is activated in BC cells, while its inhibition decreases the expression of type I IFN pathway-related genes. Specifically, the activation of LINC01198 promotes the expression of CXCL10, IFN-β, type I IFN receptors, interferon-stimulated genes (ISGs), and the transcriptional activity of NFκB (related to p65 component) in vitro (Figure 1). Equally important, the high expression of LINC01198 is associated with a high score of CD8, IL-2, IL-8, and IL-12 immune signatures and improved OS in BC patients, indicating that LINC01198 is a promoter of IFN-related antitumoral immune response (67).
2.2 Tumor-intrinsic lncRNAs as suppressors of the antitumoral immune response in BC
Different studies have shown the regulatory roles of lncRNAs as tumor-intrinsic suppressors of the antitumoral immune response in BC. In this context, Salama et al. demonstrated that the TSIX knockdown (a negative regulator of XIST) promotes a reduced expression of PD-L1 in TNBC cells. Moreover, TSIX is highly expressed in TNBC patients with high expression of PD-L1 (60). In another research, Samir et al. exhibited that the increased expression of MALAT1 and miR-182-5p positively modulates the PD-L1 expression through a negative and positive regulation of XIST and TSIX expression, respectively, promoting an immunosuppressive phenotype in TNBC (68). Recent findings showed that MALAT1 knockdown promotes the expression of stress-induced ligands (MICA and MICB) and the repression of immune checkpoints (PD-L1 and B7-H4) in TNBC cells. Also, the MALAT1 knockdown boosts the NK cells-mediated killing and CD8+ T cells-mediated cytotoxic activity via miR-34a and miR-17-5p, respectively, indicating that this lncRNA hampers the innate and adaptive immune response in TNBC (69). In another report, Xiping et al. found that MALAT1 knockdown decreases the expression of MYC oncogene and CD47 (a protein that binds to SIRPα and blocks the antigen uptake mediated by macrophages and DCs) in HER2+ and TNBC cells. In addition, the MALAT1 expression promotes proliferation and invasion in vitro, supporting the role of this lncRNA in the immune evasion of BC (70). A study showed that the methoxylated quercetin glycoside compound diminishes the MALAT1 expression, altering the immunogenic profile in BC (71). In addition, Wang et al. demonstrated that TINCR is a promoter of immune evasion in BC. Specifically, TINCR acts as a molecular sponge for miR-199a-5p and upregulates the USP20 stability through a ceRNA regulatory mechanism, promoting the upregulation of PD-L1 protein by inhibiting its ubiquitination. Additional analyses revealed that TINCR transcription is promoted through the activation of STAT1 signaling by IFNγ stimulation. Moreover, the TINCR knockdown reduces tumor growth, cell proliferation, migration, and invasion in BC (72).
On the other hand, a comprehensive investigation found that GATA3-AS1 expression enhances the PD-L1 protein levels and promotes cell proliferation and migration of TNBC cells. Particularly, GATA3-AS1 promotes the deubiquitination of PD-L1 protein through the upregulation of COPS5. Besides, the upregulation of GATA3-AS1 is related to a reduced percentage of CD8+ T cells in TNBC, and the high expression of this lncRNA is associated with reduced OS. In contrast, the high level of PD-L1 protein correlates with poor prognosis, large tumor size, and clinical stage in TNBC patients (73) (Figure 2). Another study demonstrated that HEIH is highly expressed in TNBC. At the same time, silencing this lncRNA reduces the expression of miR-939-5p, NOS2, decreases NO production and inhibits cell viability and migration in vitro. Moreover, the HEIH silencing increases the expression of MICA and MICB while decreasing the expression of PD-L1, IL-10, and TNFα, suggesting that HEIH significantly promotes immunosuppressive phenotypes in TNBC (74).
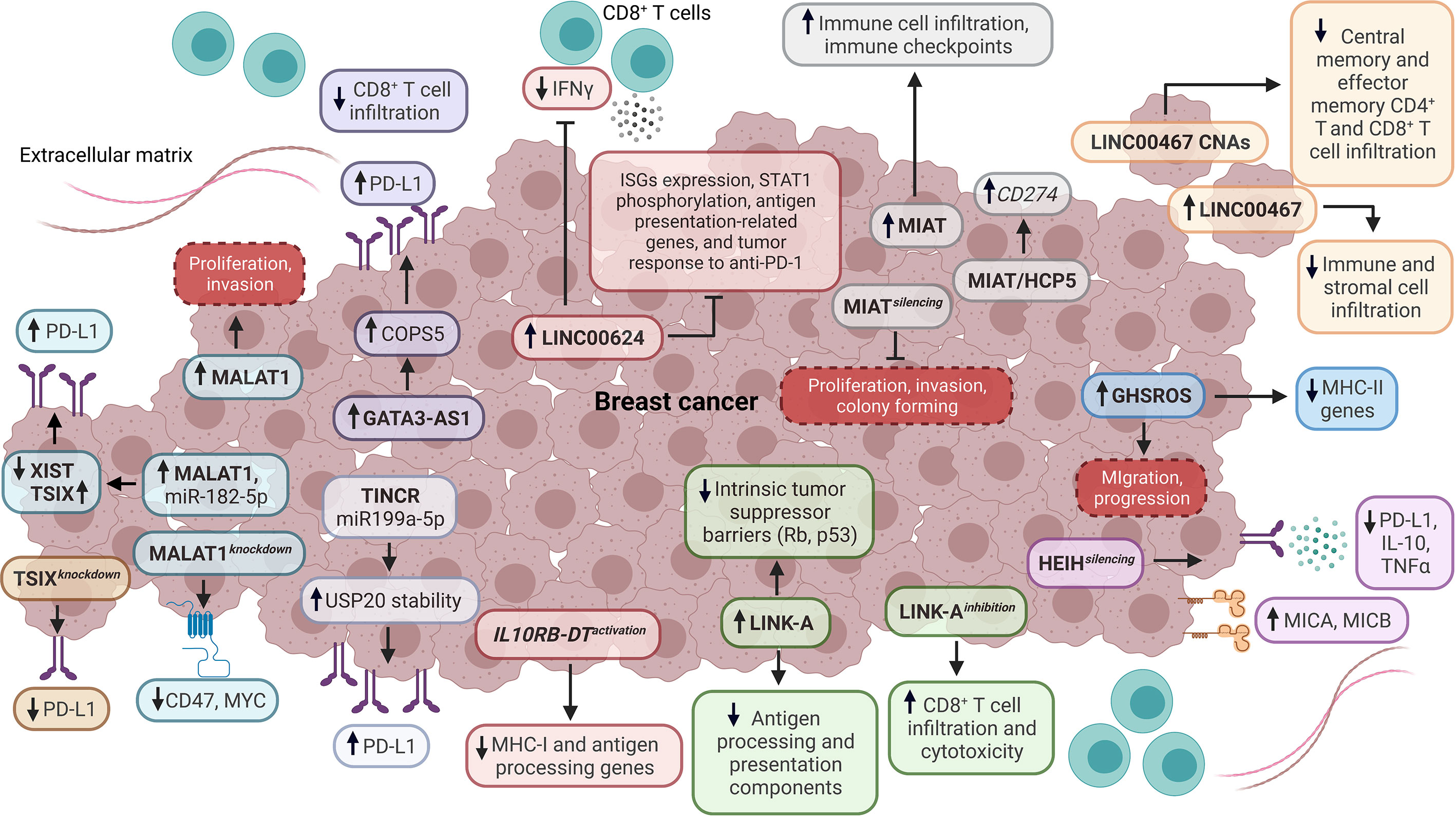
Figure 2 Impact of lncRNAs as tumor-intrinsic suppressors of the immune response in BC. Diverse lncRNAs, such as TSIX, TINCR, MALAT1, GATA3-AS1, LINC00624, IL10RB-DT, LINK-A, HEIH, GHSROS, LINC00467, MIAT, and HCP5 function as tumor-intrinsic suppressors of immune response in BC, dysregulating the expression of antigen processing and presentation components, interferon-stimulated genes (ISGs), immune checkpoints, cytokines, stress-induced ligands, STAT1 phosphorylation, tumor suppressor barriers, oncogenes, immunotherapy response, infiltration and functionality of tumor-infiltrating immune cells. Dashed boxes in red denote cancer-related phenotypes. CNAs, copy number amplifications.
Hu et al. evidenced that LINK-A is upregulated in TNBC and negatively correlates with antigen-presenting cells and CD8+ T cell levels in BL BC. Particularly, LINK-A downregulates antigen processing and presentation components (i.e., TPSN, TAP1, TAP2, and CALR) and intrinsic tumor suppressor barriers (Rb and p53), which indicates that this lncRNA promotes tumor immune evasion (Figure 2). Likewise, TNBC patients who are responders to Pembrolizumab have a low expression of LINK-A and high infiltration of CD8+ T cells, in contrast to non-responders. Remarkably, LINK-A inhibition improves the CD8+/CD3+ T cell infiltration and cytotoxicity, indicating that this lncRNA might be a potential immunosuppressive biomarker and therapeutic target in TNBC patients (75). Wang et al. characterized the function of IL10RB-DT through a CRISPR activation screening. They found that the activation of this lncRNA inhibits the transcription of MHC-I and antigen-processing genes in BC cells. Equally important, the IL10RB-DT expression is associated with poor survival in BC patients (67). A study about the LINC00624 expression showed that this lncRNA negatively correlates with type I IFN and antigen processing and presentation pathways in vitro. Also, the overexpression of LINC00624 inhibits the ISGs expression and STAT1 phosphorylation in vitro. Additionally, IFNα induces the LINC00624 expression, suggesting that this lncRNA is an ISG that is a negative feedback modulator of the IFN signaling pathway (76) (Figure 2). Further analyses demonstrated that ADAR1 interacts with LINC00624, promoting A-to-I substitutions in this lncRNA in vitro, which increase after IFNα treatment. Interestingly, the function of the edited LINC00624 depends on ADAR1 to inhibit the IFN response and to promote Lapatinib and anti-HER2 treatment resistance in HER2+ BC cells. Also, tumor cells overexpressing LINC00624 co-cultured with CD8+ T cells inhibit IFNγ production in vivo. In contrast, the antigen presentation-related genes, ISGs, and tumor response to anti-PD-1 treatment are inhibited by LINC00624 in vivo (76) (Figure 2). This lncRNA is highly expressed in HER2+ BC patients with a non-pathological complete response, and the high expression of LINC00624 is associated with poor disease-free survival (DFS) (76).
Recent research evidenced that the lncRNA MIAT is co-expressed with different genes related to immune cells’ regulation, activation, and adhesion. BC patients with high expression of MIAT exhibit a high infiltration of CD8+ T cells, resting memory CD4+ T cells, activated memory CD4+ T cells, gamma-delta T cells, and M1 macrophages. In contrast, the infiltration of plasma cells, activated NK cells, monocytes, M2 macrophages, and activated mast cells are reduced (77). Furthermore, the MIAT expression positively correlates with IC genes like PDCD1, CD274, and CTLA-4, which are critical players in suppressing the antitumoral response mediated by T cells (Figure 2). Moreover, the high expression of MIAT is associated with clinical stage and lymph node metastasis in serum samples derived from BC patients. The high expression of MIAT is associated with reduced OS in BL BC. In contrast, the high expression of this lncRNA is associated with reduced post-progression survival in LA, LB, and HER2+ BC patients, which indicates a subtype-specific prognostic role of MIAT (77). Additional research confirmed that this lncRNA positively correlates with IC gene expression and its prognostic role associated with OS. Also, MIAT silencing reduces proliferation, colony forming, and invasion, while increasing TNBC cell apoptosis in vivo, indicating that MIAT is a promoter of immunosuppressive phenotypes in BC (78). A similar behavior was detected for lncRNA HCP5 in BC. Additional analyses showed that MIAT and HCP5 upregulate the expression of CD274 through a ceRNA mechanism, which involves miR-150-5p sponging in human cancer (78) (Figure 2). Interestingly, Wu et al. found that a ceRNA network composed of BTN3A1-has-miR-20b-5p-HCP5 could have a role in the interaction between BC cells and T cells in vitro (79). In this regard, different studies have indicated that ceRNA networks are composed of mRNAs-miRNAs-lncRNAs and are potential modulators of the TIME in BC (80–83).
Another study evidenced that the overexpression of lncRNA GHSROS induces the downregulation of MHC-II genes (HLA-DRA, HLA-DPB1, HLA-DPA1, and HLA-DRB3) in TNBC cells. In addition, the overexpression of GHSROS is associated with the downregulation of immune-related pathways, including antigen processing and presentation signaling. The expression of GHSROS promotes cell migration in vitro and progression in vivo, suggesting that this lncRNA is involved in BC immune evasion (84) (Figure 2). In another study, Bo et al. identified a high expression of LINC00467 in metastatic BC and circulating tumor cells. Functional analyses demonstrated that LINC00467 silencing decreases migration and invasion in vitro. The high expression of LINC00467 is associated with poor distant metastasis-free survival and relapse-free survival (RFS) in patients across different BC subtypes. Also, the expression of LINC00467 negatively correlates with immune and stromal infiltration in BC. Significantly, the copy number amplifications of LINC00467 are related to low infiltration of central and effector memory CD4+ and CD8+ T cells (Figure 2) and are also associated with poor disease-specific survival and progression-free survival in BC patients (85).
2.3 LncRNAs related to IL-6 in BC
IL-6 is a pleiotropic cytokine that is crucial in the immune response in non-pathological and pathological conditions. IL-6 can antagonize or promote tumor progression depending on the cell context (86–89). Recent studies indicate that some lncRNAs are related to IL-6 in BC. A study showed that lncRNA BCAR4 is recruited to PTCH1, MUC5AC, TGF-β1, and IL-6 promoters to induce their expression in response to CCL21 in BC cells. Also, the BCAR4 expression promotes migration and invasion in vitro (90). Moreover, DeVaux et al. identified that BHLHE40‐AS1 promotes migration and invasion in ductal infiltrating BC through a low expression of IL-6 and STAT3 phosphorylation (91). Similarly, Nyati et al. found that lncRNA AU021063 expression is promoted by IL-6/Arid5a signaling. Additional analyses showed that AU021063 induces invasion and metastasis of BC in vitro and in vivo via upregulation of Trib3 and activation of the Mek/Erk signaling pathway (92).
3 LncRNAs as tumor-extrinsic regulators of the TIME in BC
Different studies have demonstrated that diverse lncRNAs function as tumor-extrinsic factors specifically expressed by diverse immune cell populations to regulate their functionality, which is essential in the TIME and prognosis in BC. Despite recent advances, the role of lncRNAs as extrinsic regulators of the TIME in BC has been only reported in cytotoxic T lymphocytes, regulatory T cells, and tumor-associated macrophages.
3.1 Cytotoxic T lymphocytes
The cytotoxic T lymphocytes (CTLs) are a subpopulation of CD8+ T cells that are the main effectors of the antitumoral immune response (24, 93). Remarkably, the CTLs eliminate cancer cells through perforin and granzyme mechanisms. The functionality of CTLs is mainly suppressed in cancer by the induction of anergy states and exhaustion phenotypes (93, 94). Therefore, CTLs are frequently associated with improved prognosis in different cancer types (24). Recent studies have evidenced that lncRNAs are crucial regulators of CTLs in the TIME of BC. Although the role of NKILA in non-neoplastic and neoplastic conditions has been recently reviewed (95), different studies have identified critical roles of this lncRNA in CTLs from the TIME of BC. In this regard, Huang et al. found that NKILA is highly expressed in tumor-specific CTLs and Th1 cells, enhancing their sensibility to activation-induced cell death (AICD) compared to Treg and Th2 cells in BC. Specifically, NKILA suppresses the IκBα phosphorylation, p65 nuclear translocation, and transcription of NFκB-target anti-apoptotic genes in CTLs (96). Additional studies have corroborated the role of NKILA as a negative regulator of NFκB in immune cells from BC (97, 98) (Figure 3). Notably, the transcription of NKILA is regulated by calmodulin-induced histone acetylation and STAT1 signaling, and the high levels of NKILAhi tumor-specific CTLs are associated with poor survival in BC patients (96). In contrast, Liu et al. found that the low expression of NKILA is associated with distal metastasis, lymph node status, advanced clinical stage, tumor size, and poor DFS in BC patients (97). Similarly, Wu et al. demonstrated that NKILA silencing promotes TGFβ-induced EMT in vivo and the low expression of this lncRNA is associated with poor DFS in BC patients (98) (Figure 3). Therefore, NKILA could exert a context-dependent role as a regulator of NFκB signaling and metastasis, suggesting the potential of this lncRNA as a therapeutic target to modulate the function of tumor-specific T cells in BC. In addition, Yu et al. showed that lncRNA expression and CTLs predict the OS and immunotherapy response in cancer patients stratified by immune groups (99).
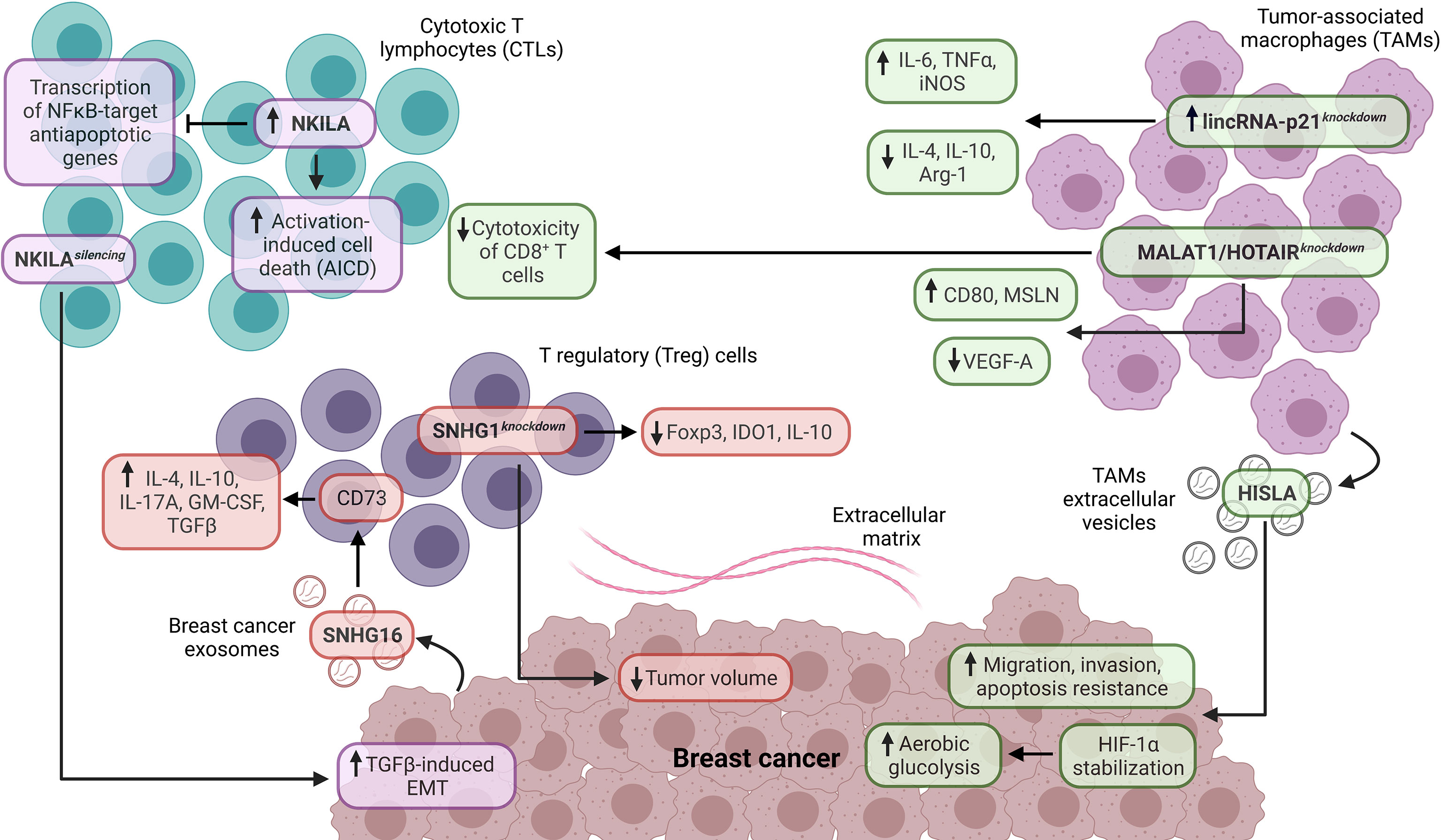
Figure 3 Role of lncRNAs as tumor-extrinsic regulators of the TIME in BC. In the TIME of BC, lncRNAs are critical modulators of the functionality in immune cell populations and BC. In this regard, NKILA is associated with cytotoxic T lymphocytes (CTLs), while SNHG1 is related to regulatory T (Treg) cells. In contrast, lincRNA-p21, HOTAIR, and MALAT1 are related to tumor-associated macrophages (TAMs). In addition, the lncRNAs, such as SNHG16, HISLA, MALAT1, and HOTAIR, are expressed by specific cell populations and have a crucial impact on the functionality of target cell populations in the TIME of BC. EMT, epithelial-mesenchymal transition.
3.2 Regulatory T cells
The regulatory T (Treg) cells are a specialized subpopulation of CD4+ T cells characterized by the expression of CD25 and FOXP3. In a non-pathological context, Treg cells suppress the immune response for homeostasis maintaining, self-tolerance, and preventing autoimmune diseases (100). In cancer, Treg cells often promote immunosuppression by expressing IL-10, TGFβ, and CTLA-4. Therefore, Treg cells are frequently associated with poor prognosis in different cancer types (24, 101). Recent research indicates that lncRNAs are important regulators of Treg cells in the TIME of BC. Moallemi-Rad et al. evaluated the Treg cell-related lncRNAs expression from BC in this regard. In particular, RMRP and MAFTRR expression is positively associated with nuclear grade, tubule formation, and tumor size. Conversely, the expression of FLICR differs according to the HER2 levels in BC (102).
SNHG1 and SNHG16 belong to the SNHG lncRNA family, which has a critical oncogenic role in different cancer types (103). Recent findings have evidenced their role as tumor-extrinsic regulators in the TIME of BC. Pei et al. demonstrated that lncRNA SNHG1 is highly expressed on CD4+ T cells from the peripheral blood of BC patients, in contrast to CD4+ T cells from normal donors. Moreover, the SNHG1 knockdown decreases IDO1, Foxp3, and IL-10 expression, essential Treg cell differentiation promoters. In addition, the SNHG1 knockdown reduces the tumor volume in murine models with BC xenografts (104). In another study, Ni et al. identified that lncRNA SNHG16, delivered from BC exosomes, promotes the activation of the TGF-β1/SMAD5 signaling pathway and miR-16-5p downregulation to induce the upregulation of CD73 in γδ1 Treg cells. The γδ1 Treg cells constitute a high proportion of TIICs in TNBC, ER+PR+, and HER2+ BCs, and the CD73 expression is higher in γδ1 Treg cells derived from BC. Remarkably, CD73+ γδ1 Treg cells exhibit high expression of IL-4, IL-10, IL-17A, GM-CSF, and TGFβ, which are critical immunosuppressive molecules, indicating that CD73+ γδ1 Treg cells have an immunosuppressive role in the TIME of BC (105) (Figure 3).
3.3 Tumor-associated macrophages
Tumor-associated macrophages (TAMs) are a crucial cell component in the TIME (106). According to their functions, TAMs are separated into M1 and M2 macrophages. M1 macrophages have pro-inflammatory and antitumoral functions mediated by the secretion of IL-1β, IL-6, IL-12, TNFα, and reactive oxygen species. Moreover, M1 macrophages are key promoters of Th1-type response and are associated with improved prognosis in different cancer types (21, 24, 106). Conversely, M2 macrophages have anti-inflammatory and pro-tumoral functions mediated by the expression of IL-10, TGFβ, PGE2, PD-L1, and PD-L2, promoting immunosuppression. Therefore, M2 macrophages are strongly associated with poor prognosis in cancer patients (21, 24, 106). Interestingly, recent research indicates that lncRNAs are critical regulators of TAMs in the TIME of BC. Zhou et al. evidenced that lincRNA-p21 is upregulated in TAMs from BC murine models. Moreover, the lincRNA-p21 knockdown in TAMs promotes the production of pro-inflammatory molecules (IL-6 and TNFα), iNOS, and decreases the production of anti-inflammatory molecules (IL-4 and IL-10) and Arg-1, indicating that this lncRNA has an essential role in the TAM polarization in vivo (Figure 3). In addition, the lincRNA-p21 knockdown promotes the interaction of MDM2/p53 to activate the NFκB and STAT3 signaling pathways. Interestingly, the lincRNA-p21 knockdown decreases the BC progression and improves survival in vivo (107). On the other hand, Chen et al. found that the extracellular vesicle-packaged lncRNA HISLA from TAMs is delivered to TNBC cells, stabilizing HIF-1α (through the inhibition of PHD2/HIF-1α interaction) and enhancing tumoral aerobic glycolysis, suggesting a metabolic reprogramming of BC mediated by cell-cell communication. Also, HISLA promotes migration, invasion, and apoptosis resistance in BC cells (Figure 3). The high expression of this lncRNA is associated with lymph node metastasis and poor DFS in BC patients (108). Another investigation analyzed the relationship between lncRNAs and their immunomodulatory role in TAMs derived from BC. In particular, MALAT1 and HOTAIR are upregulated in TAMs derived from TNBC, HER2+, and hormonal BCs. In these TAMs, the MALAT1 and HOTAIR silencing promotes the upregulation of CD80 and MSLN and the downregulation of VEGF-A. Furthermore, this study showed a cytotoxicity decrease in CD8+ T cells against TNBC cells treated with anti-PD-L1 inhibitor and cultured under conditioned media derived from TAMs with MALAT1 and HOTAIR silencing, indicating the role of both lncRNAs as tumor-extrinsic negative modulators of the antitumoral immune response in BC (109) (Figure 3).
4 LncRNAs as potential biomarkers of the TIME and clinicopathological characteristics in BC
In recent years, several Next-Generation Sequencing (NGS) approaches (i.e. bulk RNA-seq, single cell RNA-seq, whole-genome sequencing and whole-exome sequencing) and bioinformatic tools (i.e., Polysolver, NetMHCpan, CIBERSORTx and MiXCR) have been incorporated for interrogating cancer immunobiology, creating the area known as cancer immunogenomics and immunotranscriptomics (110–114), which includes comprehensive pan-cancer analyses focused on neoantigens prediction (115, 116), MHC class I and class II genes (117, 118), compositional and functional characterization of TIMEs (119, 120), cytolytic activity estimation (121), BCR/TCR repertoires (122) and immunotherapies monitoring (110, 119, 123). Remarkably, large-scale bioinformatics pan-cancer studies have focused on the characterization of lncRNAs as immune-related oncogenic biomarkers and as modifiers of TIMEs, highlighting the potential clinical application of lncRNAs as immunotherapy targets (124, 125). Remarkably, recent findings based on identifying lncRNAs as biomarkers of the TIME and clinicopathological characteristics in BC have gained particular interest. This section highlights relevant studies, primarily based on NGS data mining and bioinformatic approaches, which have explored the role of diverse lncRNAs as individual biomarkers and prognostic models/signatures in the BC immunobiology context.
4.1 Individual lncRNAs
Recent advances in BC patients have revealed that individual lncRNAs are promising biomarkers of the TIME and clinicopathological characteristics. A study found that the expression of lncRNA ST7-AS1 is associated with various signaling pathways, including MYC, KRAS, IL6-JAK-STAT3, and apoptosis signaling pathways. In addition, the expression of ST7-AS1 differentially correlates with elevated levels of diverse lymphoid and myeloid cell populations. The high expression of ST7-AS1 is associated with improved OS, progression-free survival, and disease-specific survival (DSS) in BC patients (126).
Yi et al. observed that the expression of SLC26A4-AS1 is associated with the infiltration of diverse immune cell populations. Notably, high expression of this lncRNA is associated with improved OS and DSS in BC patients (127). A study by Zhao et al. revealed that the expression of lncRNA DRAIC is inversely correlated with the infiltration of DCs and neutrophils. High expression of DRAIC is associated with advanced tumor stage, positive lymph node status, and unfavorable OS and DSS in ER+ BC patients (128). Another research demonstrated that elevated expression of lncRNA TCL6 is associated with various immune-related pathways in BC. TCL6 expression positively correlates with infiltration of neutrophils, DCs, B cells, CD4+ T, and CD8+ T cells, as well as the expression of IC genes, such as PD-1, PD-L1, PD-L2, and CTLA-4. The low and high expression of TCL6 is associated with poor and improved OS, respectively, in LB BC (129). Similarly, a recent study demonstrated that high and low expression of LINC00426 is consistently associated with increased and poor OS in LB BC, respectively. Moreover, the LINC00426 expression correlates with the infiltration level of diverse immune cell populations, IC, and cytolytic activity-related genes, evidencing that this lncRNA is an immune phenotype-related biomarker in LB BC (130).
Liu et al. reported that lncRNA OSTN-AS1 positively correlates with B and T cell signaling pathways in BC, involving genes like PDCD1, CTLA-4, CD79A, and CD79B. High expression of OSTN-AS1 is related to diverse immune functions encompassing cytokines, chemokines, NK cells, B cells, T cells, and others. The high expression of OSTN-AS1 is associated with a favorable prognosis in TNBC patients (131). In another study, De Santiago et al. found that LINC00944 is upregulated in TNBC cells due to ADAR1 loss. This lncRNA is related to immune signaling pathways, such as interferon-gamma response, inflammatory response, IL2-STAT5, and TNFα-NFκB. Also, the LINC00944 expression positively correlates with T cell-associated gene markers (CD3D, CD3E, CD3G, SH2D1A, and TRAT1) in BC patients. Reduced expression of LINC00944 is associated with diminished T-cell infiltration, while the high expression of this lncRNA is related to the upregulation of anti-apoptotic genes. The high expression of LINC00944 is associated with improved RFS in TNBC patients (132). Similarly, a study identified that lncRNAs RP3-460G2.2, RP11-1008C21.1, and RP5-899E9.1 are correlated with the infiltration of diverse immune cell populations and are strongly associated with macrophage gene markers (CD68 and MSR1) in BC (133).
An investigation revealed a negative correlation between LINC00472 expression and IFNγ, IFNα, and TNFα pathways while exhibiting a positive correlation with p53, ER, and PR pathways in ER+, ER- BCs, and TNBC, implying an association with immunosuppression. Conversely, the opposite correlation was detected for lnc-HLA-DRB1-5 in ER+, ER- BCs, and TNBC (134). Notably, recent research has focused on evaluating the expression and roles of immune-related lncRNAs in different BC subtypes. Mathias et al. highlighted the high expression of LINC01871 in BL BC, which participates in interferon-gamma response, allograft rejection, interferon alpha, inflammatory response, IL6-JAK-STAT3, and IL2-STAT5 signaling. Additionally, the upregulation of LINC01871 was associated with improved OS and progression-free interval (PFI) in BL BC (135).
Similarly, XXYLT1-AS2 exhibits high expression in HER2-enriched BC and correlates with improved PFI in this subtype. This lncRNA positively correlates with allograft rejection, interferon-gamma response, inflammatory response, IL-2, and IL-6 signaling, while negatively correlates with EMT, hypoxia, and myogenesis. Conversely, MEG3 is highly expressed in LA BC and positively correlated with TNFα-NFκB, inflammatory response, allograft rejection, interferon-gamma response, and IL2-STAT5 signaling (135). Furthermore, the lncRNA EBLN3P is highly expressed in LB BC and is associated with improved OS. The expression of EBLN3P negatively correlates with TNFα-NFκB and allograft rejection signaling (135).
Recent findings highlighted LINC01087 as a potential promoter of the antitumoral immune response with high expression in luminal BCs. Specifically, LINC01087 demonstrated a relationship with the NFκB signaling pathway in LA and LB BC. Moreover, this lncRNA is also related to chemoattractants, chemokine, and pattern recognition receptors signaling pathways in LA BC. Elevated expression of LINC01087 downregulates oncogenic network components related to proliferation and adhesion, including the WNT/β-catenin pathway. Remarkably, the high expression of LINC01087 was correlated with improved OS and RFS in LA and LB BC patients (136). On the other hand, a recent study analyzed the expression of immune-related lncRNAs in BC, revealing differences based on hormone status. Notably, the low expression of immune-related lncRNAs ENST0000615051, lnc-DDX31, and LINC02381 was detected in ER+ BC, while reduced expression of lnc-DDX31 was observed in PR+ BC (137). Equally important, an investigation found epigenetically dysregulated lncRNAs associated with immune pathways related to inflammation, cytokines, chemokines, and T cells, depending on the BC subtype. In this context, LINC01983, UCA1, RP11-221J22.1 and RP11-221J22.2 were specific to luminal BCs, while RP1-140K8.5, AC005162.1, AC020916.2 SLC26A4-AS1, and CTC-303L1.2 were specific to BL subtype (138). These findings point out the potential of specific lncRNAs as valuable biomarkers for assessing the TIME and predicting clinical outcomes in BC patients.
4.2 LncRNA prognostic models/signatures
Recent studies have explored the combined roles of diverse immune-related lncRNAs in models/signatures, which are prognostic predictors and markers of immune landscapes in BC, indicating their potential usefulness in clinical settings (139–143). Liu et al. showed that a nomogram, based on seven immune-related lncRNAs, age, clinical stage, ER status, and BC subtype, is a predictor of OS in BC. Also, the study exhibited that low-risk patients have an enrichment of immune pathways associated with inflammation and a correlation between the infiltration of B cells, T cells, and macrophages with the risk score. In contrast, high-risk patients show a high mutational burden (144). Similarly, a signature based on five immune-related lncRNAs predicts the OS and negatively correlates with the infiltration of B cells, T cells, DCs, neutrophils, and macrophages (145). In this context, different immune-related lncRNA models/signatures are predictors of survival and metastatic status. Notably, the lncRNA models/signatures can stratify BC patients based on their risk score, associated with the enrichment of various immune-related pathways, the abundance of diverse immune cell populations, and the expression of different IC genes (Table 1).
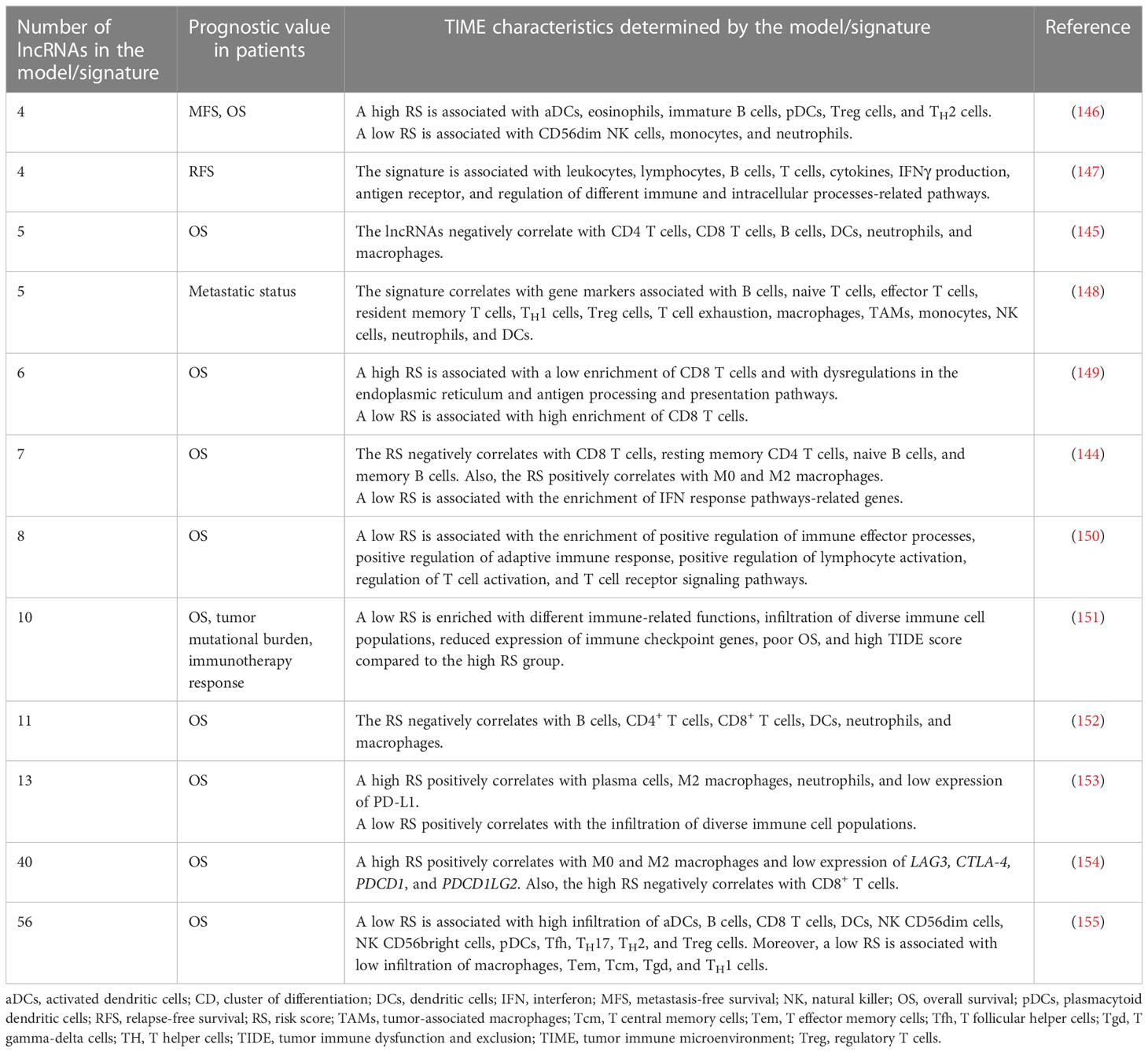
Table 1 Immune-related lncRNA models/signatures are prognostic predictors and markers of the TIME characteristics in BC.
Interestingly, different studies have demonstrated a relationship between tumor immune response and ferroptosis, necroptosis, pyroptosis, autophagy, and genomic instability processes (156–161). In this regard, various studies have developed prognostic lncRNA models/signatures related to these processes, which predict the TIME characteristics and immunotherapy response in BC patients (Table 2). Additional lncRNA prognostic models/signatures focused on other biological processes, such as lipid metabolism, hypoxia, glycolysis, EMT, stemness, RNA-binding proteins, endoplasmic reticulum stress, cuproptosis, lactate, oxidative stress, androgen receptor signaling pathway, mitochondrial function, aging and angiogenesis have also demonstrated to be predictors of immune landscapes characteristics in BC (176–190). However, further studies are mandatory to explore the relationship between these processes with BC immunobiology.
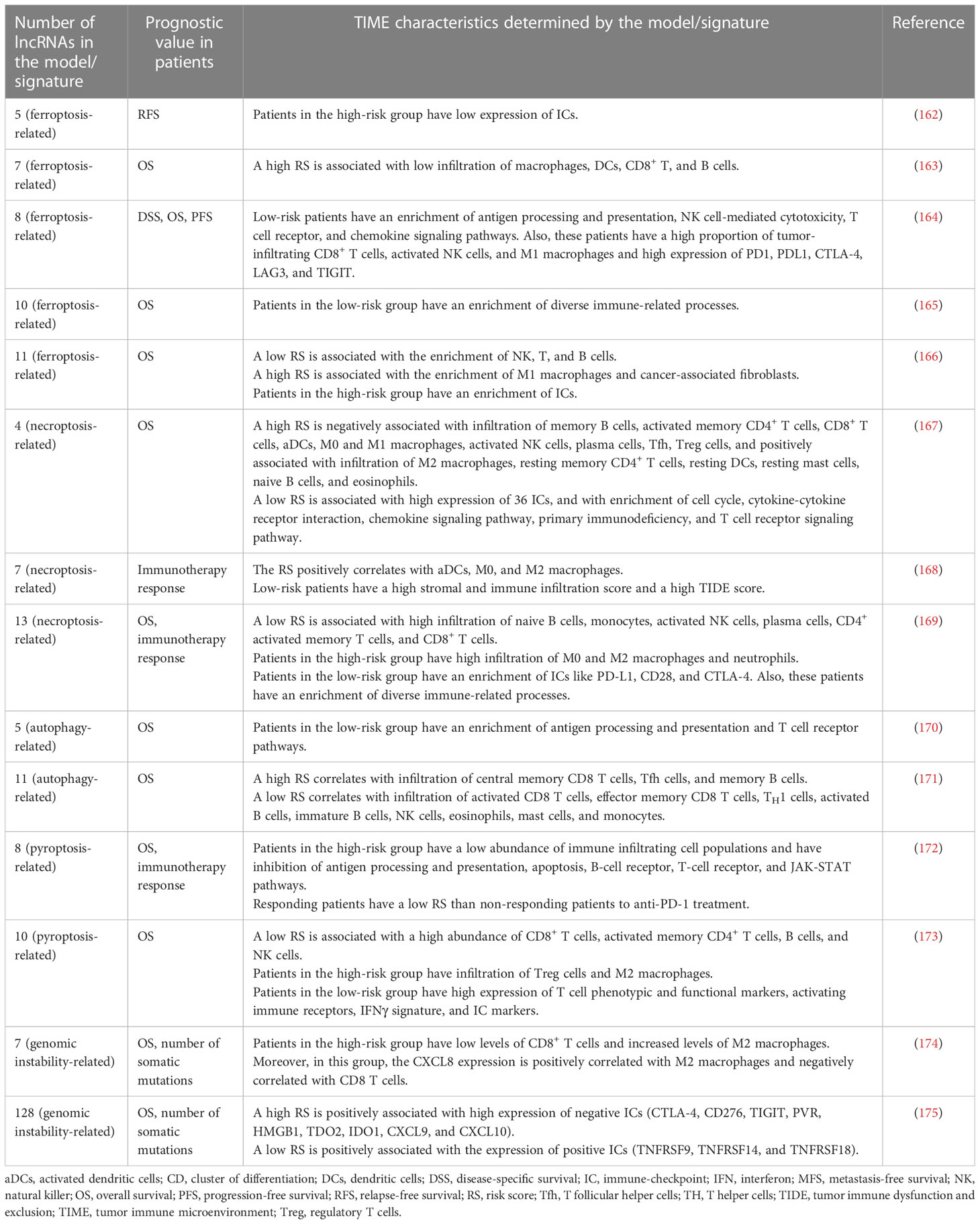
Table 2 LncRNA models/signatures related to ferroptosis, necroptosis, pyroptosis, autophagy, and genomic instability are prognostic predictors and markers of the TIME characteristics in BC.
5 Potential limitations and advantages of lncRNAs for BC immunotherapy
Diverse studies have demonstrated the importance of lncRNAs in cancer biology (38–42). As discussed in this review, lncRNAs are critical players in diverse BC-intrinsic and extrinsic immune-related processes. Also, lncRNAs are potential biomarkers of patients’ TIME and clinicopathological characteristics. Previous studies have supported the potential usefulness of strategies for targeting lncRNAs in a cancer context. These approaches are mainly focused on post-transcriptional targeting [i.e., RNA-mediated interference (RNAi), Morpholino oligomers, and anti-sense oligonucleotides (ASOs)], modulation of lncRNA-expressing loci via CRISPR/Cas9-based genome editing, transcriptional upregulation through targeting natural anti-sense RNAs, steric inhibition of lncRNA function, and lncRNA tertiary structure disrupting-based strategies (43, 44, 191–193). Notably, a comprehensive study used ASOs to target LINC00624 in HER2+ BC, resulting in the inhibition of proliferation in vitro and increasing the expression of innate immune response-related genes in xenograft tumor models, supporting the role of LINC00624 as an inhibitor of the antitumoral immune response (76). In addition, a CRISPR activation screening strategy was recently used to determine the mechanistic role of LINC01198 and IL10RB-DT in BC cells, concluding an association with promoting and suppressing the antitumoral immune response, respectively (67). These findings highlight lncRNAs as attractive targets for BC immunotherapy. However, some issues must be addressed before incorporating lncRNAs in clinical settings. Firstly, most of the functional studies focused on lncRNAs, in the context of BC immunobiology, are based on targeting strategies, like CRISPR-Cas9, RNAi and ASOs, and routine functional assays in vitro and vivo (i.e., co-cultures, proliferation, migration and invasion assays). Despite these advances, there is still a gap in our understanding of the exact mechanistic role of lncRNAs; therefore, the incorporation of comprehensive functional approaches and complementary strategies to fully dissect the crosstalk of lncRNAs in signaling pathways, lncRNA tertiary structure, and lncRNAs interactions with diverse RNA species, DNA elements, chromatin, and proteins are mandatory to completely understand the versatile and complex mechanistic roles of lncRNAs in BC immunobiology. Secondly, bioinformatic studies focused on lncRNAs must be validated using experimental methodologies like flow cytometry and multiplex immunofluorescence. Thirdly, we still lack information about the tumor extrinsic roles of diverse lncRNAs in the remaining BC TIME cell components, such as B cells, MDSCs, and neutrophils. Additionally, clinical trials by FDA and EMA are mandatory for validating immune-related prognostic biomarkers and immunotherapy strategies based on lncRNAs in BC, considering the current hurdles associated with non-coding RNA therapeutics, such as on-target specificity, unwanted off-target effects, and delivery systems (43, 44, 192, 194).
Despite these challenges, lncRNAs are promising molecules for BC immunotherapy because different molecules like XIST, LINC001198, TINCR, LINK-A, and HEIH function as promoters or suppressors of the antitumoral immune response at intrinsic and extrinsic levels, demonstrated by in vivo and in vitro studies. Also, investigations based on NGS data mining from public repositories and bioinformatic analyses have elucidated the role of diverse lncRNAs like DRAIC, OSTN-AS1, LINC00944, and LINC01871 as biomarkers of the TIME and clinicopathological features in BC, highlighting lncRNAs as potential immunotherapy targets. In addition, lncRNA targeting strategies may be combined with current and approved immunotherapies based on protein and cellular targets (i.e., IC inhibitors, cytokine modulation, and immune cell-based therapies) to increase the therapeutic options, improve the response to immunotherapies and consider personalized treatments for BC patients. Also, lncRNAs like GATA3-AS1, LINC00624, TCL6, LINC00426, and MIAT have BC subtype-specific expression that can be useful for proper designing and specific implementation for patients’ stratification strategies and immunotherapies based on lncRNAs in BC in the next coming years.
6 Conclusion and perspectives
The dysregulation of lncRNAs has a crucial role in tumorigenesis and cancer progression. Mainly, various studies have reported the relevance of different lncRNAs in alterations of processes associated with cancer immunobiology. In this review, we provided the current advances in the role of lncRNAs as modulators of antitumoral immune response and the TIME in BC, as well as their role as potential biomarkers of the TIME and clinicopathological characteristics in BC patients. The pivotal role of lncRNAs in regulating antigen processing and presentation, ICs expression, infiltration, and functionality of immune cell populations, and their association with diverse prognosis parameters, highlights that lncRNAs are potential biomarkers of immune phenotypes and immunotherapy targets for BC. Limitations in our knowledge of lncRNAs in BC immunobiology are associated with the complexity of thoroughly dissecting their exact mechanistic roles and interactions. Therefore, future lncRNA research based on comprehensive functional strategies, bioinformatics approaches, and clinical trials is mandatory to fully understand the versatile and complex mechanistic and clinical roles of diverse lncRNAs in BC immunobiology. Taken together, the advances in lncRNAs have opened a novel and exciting area to dissect BC immunobiology and its potential therapeutic significance in the next coming years.
Author contributions
Conceptualization, investigation, resources, original draft, figures and tables preparation: MAF-M. Writing and editing: MAF-M. Review and comments: KIV-S. Supervision: AH-M. All authors contributed to the article and approved the submitted version.
Funding
This research was funded by the Consejo Nacional de Ciencia y Tecnología (CONACYT) 708515 grant (scholarship).
Acknowledgments
We thank to Programa de Doctorado en Ciencias Biológicas, UNAM. The authors thank the reviewers for their helpful comments on the manuscript.
Conflict of interest
The authors declare that the research was conducted in the absence of any commercial or financial relationships that could be construed as a potential conflict of interest.
Publisher’s note
All claims expressed in this article are solely those of the authors and do not necessarily represent those of their affiliated organizations, or those of the publisher, the editors and the reviewers. Any product that may be evaluated in this article, or claim that may be made by its manufacturer, is not guaranteed or endorsed by the publisher.
References
1. Harbeck N, Penault-Llorca F, Cortes J, Gnant M, Houssami N, Poortmans P, et al. Breast cancer. Nat Rev Dis Prim (2019) 5(1):66. doi: 10.1038/s41572-019-0111-2
2. Sung H, Ferlay J, Siegel RL, Laversanne M, Soerjomataram I, Jemal A, et al. Global cancer statistics 2020: GLOBOCAN estimates of incidence and mortality worldwide for 36 cancers in 185 countries. CA Cancer J Clin (2021) 71(3):209–49. doi: 10.3322/caac.21660
3. Bydoun M, Marcato P, Dellaire G. Breast cancer genomics. In: Cancer genomics: from bench to personalized medicine. Elsevier Inc (2013). p. 213–32. doi: 10.1016/B978-0-12-396967-5.00013-X
4. Goldhirsch A, Winer EP, Coates AS, Gelber RD, Piccart-Gebhart M, Thürlimann B, et al. Personalizing the treatment of women with early breast cancer: highlights of the st gallen international expert consensus on the primary therapy of early breast cancer 2013. Ann Oncol (2013) 24(9):2206–23. doi: 10.1093/annonc/mdt303
5. Akram M, Iqbal M, Daniyal M, Khan AU. Awareness and current knowledge of breast cancer. Biol Res (2017) 50(1):1–23. doi: 10.1186/s40659-017-0140-9
6. Perou CM, Sørile T, Eisen MB, Van De Rijn M, Jeffrey SS, Ress CA, et al. Molecular portraits of human breast tumours. Nature (2000) 406(6797):747–52. doi: 10.1038/35021093
7. Bernard PS, Parker JS, Mullins M, Cheung MCU, Leung S, Voduc D, et al. Supervised risk predictor of breast cancer based on intrinsic subtypes. J Clin Oncol (2009) 27(8):1160–7. doi: 10.1200/JCO.2008.18.1370
8. Spranger S, Gajewski TF. Impact of oncogenic pathways on evasion of antitumour immune responses. Nat Rev Cancer (2018) 18(3):139–47. doi: 10.1038/nrc.2017.117
9. Yousefnia S, Seyed Forootan F, Seyed Forootan S, Nasr Esfahani MH, Gure AO, Ghaedi K. Mechanistic pathways of malignancy in breast cancer stem cells. Front Oncol (2020) 10:452(April). doi: 10.3389/fonc.2020.00452
10. Ortega MA, Fraile-Martínez O, Asúnsolo Á, Buján J, García-Honduvilla N, Coca S. Signal transduction pathways in breast cancer: the important role of PI3K/Akt/mTOR. J Oncol (2020) 2020:1–11. doi: 10.1155/2020/9258396
11. Tufail M, Cui J, Wu C. Breast cancer: molecular mechanisms of underlying resistance and therapeutic approaches. Am J Cancer Res (2022) 12(7):2920–49. doi: 2156-6976/ajcr0143265
12. Shen L, Shi Q, Wang W. Double agents: genes with both oncogenic and tumor-suppressor functions. Oncogenesis (2018) 7(3):1–14. doi: 10.1038/s41389-018-0034-x
13. Kontomanolis EN, Koutras A, Syllaios A, Schizas D, Mastoraki A, Garmpis N, et al. Role of oncogenes and tumor-suppressor genes in carcinogenesis: a review. Anticancer Res (2020) 40(11):6009–15. doi: 10.21873/anticanres.14622
14. Chen L, Liu S, Tao Y. Regulating tumor suppressor genes: post-translational modifications. Signal Transduct Target Ther (2020) 5(1):1–25. doi: 10.1038/s41392-020-0196-9
15. Datta N, Chakraborty S, Basu M, Ghosh MK. Tumor suppressors having oncogenic functions: the double agents. Cells (2021) 10(1):1–26. doi: 10.3390/cells10010046
16. Nik-Zainal S, Davies H, Staaf J, Ramakrishna M, Glodzik D, Zou X, et al. Landscape of somatic mutations in 560 breast cancer whole-genome sequences. Nature (2016) 534(7605):47–54. doi: 10.1038/nature17676
17. Angus L, Smid M, Wilting SM, van Riet J, Van Hoeck A, Nguyen L, et al. The genomic landscape of metastatic breast cancer highlights changes in mutation and signature frequencies. Nat Genet (2019) 51(10):1450–8. doi: 10.1038/s41588-019-0507-7
18. Condorelli R, Mosele F, Verret B, Bachelot T, Bedard PL, Cortes J, et al. Genomic alterations in breast cancer: level of evidence for actionability according to ESMO scale for clinical actionability of molecular targets (ESCAT). Ann Oncol (2019) 30(3):365–73. doi: 10.1093/annonc/mdz036
19. Zhou S, Liu S, Zhao L, Sun HX. A comprehensive survey of genomic mutations in breast cancer reveals recurrent neoantigens as potential therapeutic targets. Front Oncol (2022) 12:786438(March). doi: 10.3389/fonc.2022.786438
20. Nagarsheth N, Wicha MS, Zou W. Chemokines in the cancer microenvironment and their relevance in cancer immunotherapy. Nat Rev Immunol (2017) 17:1–14. doi: 10.1038/nri.2017.49
21. Binnewies M, Roberts EW, Kersten K, Chan V, Fearon DF, Merad M, et al. Understanding the tumor immune microenvironment (TIME) for effective therapy. Nat Med (2018) 24(5):541–50. doi: 10.1038/s41591-018-0014-x
22. Wang H, Pan J, Barsky L, Jacob JC, Zheng Y, Gao C, et al. Characteristics of pre-metastatic niche: the landscape of molecular and cellular pathways. Mol BioMed (2021) 2(1):1–32. doi: 10.1186/s43556-020-00022-z
23. Pitt JM, Vétizou M, Daillère R, Roberti MP, Yamazaki T, Routy B, et al. Resistance mechanisms to immune-checkpoint blockade in cancer: tumor-intrinsic and -extrinsic factors. Immunity (2016) 44(6):1255–69. doi: 10.1016/j.immuni.2016.06.001
24. Bruni D, Angell HK, Galon J. The immune contexture and immunoscore in cancer prognosis and therapeutic efficacy. Nat Rev Cancer (2020) 20(11):662–80. doi: 10.1038/s41568-020-0285-7
25. Chen DS, Mellman I. Oncology meets immunology: the cancer-immunity cycle. Nature (2013) step 3):1–10. doi: 10.1016/j.immuni.2013.07.012
26. Chen DS, Mellman I. Elements of cancer immunity and the cancer-immune set point. Nature (2017) 541(7637):321–30. doi: 10.1038/nature21349
27. Savas P, Salgado R, Denkert C, Sotiriou C, Darcy PK, Smyth MJ, et al. Clinical relevance of host immunity in breast cancer: from TILs to the clinic. Nat Rev Clin Oncol (2016) 13(4):228–41. doi: 10.1038/nrclinonc.2015.215
28. Bense RD, Sotiriou C, Piccart-gebhart MJ, Haanen JBAG, Vugt MATM, Van, Vries EGE, et al. Relevance of tumor-infiltrating immune cell composition and functionality for disease outcome in breast cancer. J Natl Cancer Inst (2017) 109:1–9. doi: 10.1093/jnci/djw192.
29. Glajcar A, Szpor J, Hodorowicz-zaniewska D, Tyrak KE, Oko K. The composition of T cell infiltrates varies in primary invasive breast cancer of different molecular subtypes as well as according to tumor size and nodal status. Virchows Arch (2019) 475:13–23. doi: 10.1007/s00428-019-02568-y
30. Degnim AC, Hoskin TL, Arshad M, Frost MH, Winham SJ, Brahmbhatt RA, et al. Alterations in the immune cell composition in premalignant breast tissue that precede breast cancer development. Clin Cancer Res (2017) 23(14):3945–52. doi: 10.1158/1078-0432.CCR-16-2026
31. Graeser M, Feuerhake F, Gluz O, Volk V, Hauptmann M, Jozwiak K, et al. Immune cell composition and functional marker dynamics from multiplexed immunohistochemistry to predict response to neoadjuvant chemotherapy in the WSG-ADAPT-TN trial. J Immunother Cancer (2021) 9(5):1–11. doi: 10.1136/jitc-2020-002198
32. Hanamura T, Kitano S, Kagamu H, Yamashita M, Terao M, Tsuda B, et al. Immunological profiles of the breast cancer microenvironment represented by tumor-infiltrating lymphocytes and PD-L1 expression. Sci Rep (2022) 12(1):1–10. doi: 10.1038/s41598-022-11578-x
33. Coppola L, Smaldone G, D’aiuto M, D’aiuto G, Mossetti G, Rinaldo M, et al. Identification of immune cell components in breast tissues by a multiparametric flow cytometry approach. Cancers (Basel) (2022) 14(16):1–17. doi: 10.3390/cancers14163869
34. Zhang SC, Hu ZQ, Long JH, Zhu GM, Wang Y, Jia Y, et al. Clinical implications of tumor-infiltrating immune cells in breast cancer. J Cancer (2019) 10(24):6175–84. doi: 10.7150/jca.35901
35. Salemme V, Centonze G, Cavallo F, Defilippi P, Conti L. The crosstalk between tumor cells and the immune microenvironment in breast cancer: implications for immunotherapy. Front Oncol (2021) 11:610303(March). doi: 10.3389/fonc.2021.610303
36. Zhu JJ, Fu HJ, Wu YG, Zheng XF. Function of lncRNAs and approaches to lncRNA-protein interactions. Sci China Life Sci (2013) 56(10):876–85. doi: 10.1007/s11427-013-4553-6
37. Chen LL. Linking long noncoding RNA localization and function. Trends Biochem Sci (2016) 41(9):761–72. doi: 10.1016/j.tibs.2016.07.003
38. Qiu MT, Hu JW, Yin R, Xu L. Long noncoding RNA: an emerging paradigm of cancer research. Tumor Biol (2013) 34(2):613–20. doi: 10.1007/s13277-013-0658-6
39. Bhan A, Soleimani M, Mandal SS. Long noncoding RNA and cancer: a new paradigm. Cancer Res (2017) 77(15):1–18. doi: 10.1158/0008-5472.CAN-16-2634
40. Bolha L, Ravnik-Glavač M, Glavač D. Long noncoding RNAs as biomarkers in cancer. Dis Markers (2017) 2017:1–14. doi: 10.1155/2017/7243968
41. Schmitt AM, Chang HY. Long noncoding RNAs: At the intersection of cancer and chromatin biology. Cold Spring Harb Perspect Med (2017), 1-16. doi: 10.1101/cshperspect.a026492
42. Zhao S, Zhang X, Chen S, Zhang S. Long noncoding RNAs: fine-tuners hidden in the cancer signaling network. Cell Death Discovery (2021) 7(1):1–10. doi: 10.1038/s41420-021-00678-8
43. Arun G, Diermeier SD, Spector DL. Therapeutic targeting of long non-coding RNAs in cancer. Trends Mol Med (2018) 24(3):257–77. doi: 10.1016/j.molmed.2018.01.001
44. Jin H, Du W, Huang W, Yan J, Tang Q, Chen Y, et al. lncRNA and breast cancer: progress from identifying mechanisms to challenges and opportunities of clinical treatment. Mol Ther - Nucleic Acids (2021) 25(September):613–37. doi: 10.1016/j.omtn.2021.08.005
45. Denaro N, Merlano MC, Lo Nigro C. Long noncoding RNAs as regulators of cancer immunity. Mol Oncol (2019) 13(1):61–73. doi: 10.1002/1878-0261.12413
46. Luo Y, Yang J, Yu J, Liu X, Yu C, Hu J, et al. Long non-coding RNAs: emerging roles in the immunosuppressive tumor microenvironment. Front Oncol (2020) 10:48(January). doi: 10.3389/fonc.2020.00048
47. Wu M, Fu P, Qu L, Liu J, Lin A. Long noncoding RNAs, new critical regulators in cancer immunity. Front Oncol (2020) 10:550987(October). doi: 10.3389/fonc.2020.550987
48. Zhang L, Xu X, Su X. Noncoding RNAs in cancer immunity: functions, regulatory mechanisms, and clinical application. Mol Cancer (2020) 19(1):1–12. doi: 10.1186/s12943-020-01154-0
49. Kim SK, Cho SW. The evasion mechanisms of cancer immunity and drug intervention in the tumor microenvironment. Front Pharmacol (2022) 13:868695(May). doi: 10.3389/fphar.2022.868695
50. Spranger S, Gajewski TF. Mechanisms of tumor cell-intrinsic immune evasion. Annu Rev Cancer Biol (2018) 2:213–28. doi: 10.1146/annurev-cancerbio-030617-050606
51. Van Verschuer VMT, Hooning MJ, Van Baare-Georgieva RD, Hollestelle A, Timmermans AM, Koppert LB, et al. Tumor-associated inflammation as a potential prognostic tool in BRCA1/2-associated breast cancer. Hum Pathol (2015) 46(2):182–90. doi: 10.1016/j.humpath.2014.10.020
52. Kraya AA, Maxwell KN, Wubbenhorst B, Wenz BM, Pluta J, Rech AJ, et al. Genomic signatures predict the immunogenicity of BRCA-deficient breast cancer. Clin Cancer Res (2019) 25(14):4363–74. doi: 10.1158/1078-0432.CCR-18-0468
53. Wen WX, Leong CO. Association of BRCA1- and BRCA2-deficiency with mutation burden, expression of PD-L1/ PD-1, immune infiltrates, and T cell-inflamed signature in breast cancer. PloS One (2019) 14(4):1–16. doi: 10.1371/journal.pone.0215381
54. Solinas C, Marcoux D, Garaud S, Vitória JR, Van den Eynden G, de Wind A, et al. BRCA gene mutations do not shape the extent and organization of tumor infiltrating lymphocytes in triple negative breast cancer. Cancer Lett (2019) 450(December 2018):88–97. doi: 10.1016/j.canlet.2019.02.027
55. Tung N, Garber JE, Hacker MR, Torous V, Freeman GJ, Poles E, et al. Prevalence and predictors of androgen receptor and programmed death-ligand 1 in BRCA1-associated and sporadic triple-negative breast cancer. NPJ Breast Cancer (2016) 2(1):1–7. doi: 10.1038/npjbcancer.2016.2
56. Qi F, Qin WX, Zang YS. Molecular mechanism of triple-negative breast cancer-associated BRCA1 and the identification of signaling pathways. Oncol Lett (2019) 17(3):2905–14. doi: 10.3892/ol.2019.9884
57. Behring M, Vazquez AI, Cui X, Irvin MR, Ojesina AI, Agarwal S, et al. Gain of function in somatic TP53 mutations is associated with immune-rich breast tumors and changes in tumor-associated macrophages. Mol Genet Genomic Med (2019) 7(12):1–9. doi: 10.1002/mgg3.1001
58. Li L, Li M, Wang X. Cancer type-dependent correlations between TP53 mutations and antitumor immunity. DNA Repair (Amst) (2020) 88(January):1–9. doi: 10.1016/j.dnarep.2020.102785
59. Liu Z, Jiang Z, Gao Y, Wang L, Chen C, Wang X. TP53 mutations promote immunogenic activity in breast cancer. J Oncol (2019) 2019:1–19. doi: 10.1155/2019/5952836
60. Salama EA, Adbeltawab RE, El Tayebi HM. XIST and TSIX: novel cancer immune biomarkers in PD-L1-Overexpressing breast cancer patients. Front Oncol (2020) 9:1459(January). doi: 10.3389/fonc.2019.01459
61. Zhao Y, Yu Z, Ma R, Zhang Y, Zhao L, Yan Y, et al. lncRNA-Xist/miR-101-3p/KLF6/C/EBPα axis promotes TAM polarization to regulate cancer cell proliferation and migration. Mol Ther Nucleic Acids (2021) 23:536–51. doi: 10.1016/j.omtn.2020.12.005
62. Xing F, Liu Y, Wu SY, Wu K, Sharma S, Mo YY, et al. Loss of XIST in breast cancer activates MSN-c-Met and reprograms microglia via exosomal miRNA to promote brain metastasis. Cancer Res (2018) 78(15):4316–30. doi: 10.1158/0008-5472.CAN-18-1102
63. Hamed MM, Handoussa H, Hussein NH, Eissa RA, Abdel-Aal LK, El Tayebi HM. Oleuropin controls miR-194/XIST/PD-L1 loop in triple negative breast cancer: new role of nutri-epigenetics in immune-oncology. Life Sci (2021) 277:1–12. doi: 10.1016/j.lfs.2021.119353
64. Fan Y, Dong X, Li M, Liu P, Zheng J, Li H. LncRNA KRT19P3 is involved in breast cancer cell proliferation, migration and invasion. Front Oncol (2022) 11:799082(January). doi: 10.3389/fonc.2021.799082
65. Beltrán-Anaya FO, Romero-Córdoba S, Rebollar-Vega R, Arrieta O, Bautista-Piña V, Dominguez-Reyes C, et al. Expression of long non-coding RNA ENSG00000226738 (LncKLHDC7B) is enriched in the immunomodulatory triple-negative breast cancer subtype and its alteration promotes cell migration, invasion, and resistance to cell death. Mol Oncol (2019) 13(4):909–27. doi: 10.1002/1878-0261.12446
66. Zhang Y, Dong X, Wang Y, Wang L, Han G, Jin L, et al. Overexpression of LncRNA BM466146 predicts better prognosis of breast cancer. Front Oncol (2021) 10:628757(January). doi: 10.3389/fonc.2020.628757
67. Wang Y, Zhao Y, Guo W, Yadav GS, Bhaskarla C, Wang Z, et al. Genome-wide gain-of-function screening characterized lncRNA regulators for tumor immune response. Sci Adv (2022) 8(49):1–17. doi: 10.1126/sciadv.add0005
68. Samir A, Tawab RA, Eltayebi HM. Long non-coding RNAs XIST and MALAT1 hijack the PD-L1 regulatory signaling pathway in breast cancer subtypes. Oncol Lett (2021) 22(593):1–12. doi: 10.3892/ol.2021.12854
69. Mekky RY, Ragab MF, Manie T, Attia AA, Youness RA. MALAT-1: immunomodulatory lncRNA hampering the innate and the adaptive immune arms in triple negative breast cancer. Transl Oncol (2023) 31(March):1–9. doi: 10.1016/j.tranon.2023.101653
70. Wang Q, Li G, Ma X, Liu L, Liu J, Yin Y, et al. LncRNA TINCR impairs the efficacy of immunotherapy against breast cancer by recruiting DNMT1 and downregulating MiR-199a-5p via the STAT1–TINCR-USP20-PD-L1 axis. Cell Death Dis (2023) 14(2):1–12. doi: 10.1038/s41419-023-05609-2
71. Xiping Z, Bo C, Shifeng Y, Feijiang Y, Hongjian Y, Qihui C, et al. Roles of MALAT1 in development and migration of triple negative and her-2 positive breast cancer. Oncotarget (2018) 9(2):2255–67. doi: 10.18632/oncotarget.23370
72. Abdel−Latif M, Riad A, Soliman RA, Elkhouly AM, Nafae H, Gad MZ, et al. MALAT−1/p53/miR−155/miR−146a ceRNA circuit tuned by methoxylated quercitin glycoside alters immunogenic and oncogenic profiles of breast cancer. Mol Cell Biochem (2022) 477):1281–93. doi: 10.1007/s11010-022-04378-4
73. Zhang M, Wang N, Song P, Fu Y, Ren Y, Li Z, et al. LncRNA GATA3-AS1 facilitates tumour progression and immune escape in triple-negative breast cancer through destabilization of GATA3 but stabilization of PD-L1. Cell Prolif (2020) 53(9):1–13. doi: 10.1111/cpr.12855
74. Nafea H, Youness RA, Abou-Aisha K, Gad MZ. LncRNA HEIH/miR-939-5p interplay modulates triple-negative breast cancer progression through NOS2-induced nitric oxide production. J Cell Physiol (2021) 236:5362–72. doi: 10.1002/jcp.30234
75. Hu Q, Ye Y, Chan LC, Li Y, Liang K, Lin A, et al. Oncogenic lncRNA downregulates cancer cell antigen presentation and intrinsic tumor suppression. Nat Immunol (2019) 20(7):835–51. doi: 10.1038/s41590-019-0400-7
76. Zhang Q, Xiu B, Zhang L, Chen M, Chi W, Li L, et al. Immunosuppressive lncRNA LINC00624 promotes tumor progression and therapy resistance through ADAR1 stabilization. J Immunother Cancer (2022) 10(10):1–15. doi: 10.1136/jitc-2022-004666
77. Ye T, Feng J, Cui M, Yang J, Wan X, Xie D, et al. lncRNA MIAT services as a noninvasive biomarker for diagnosis and correlated with immune infiltrates in breast cancer. Int J Womens Health (2021) 13:991–1004. doi: 10.2147/IJWH.S312714
78. Xu S, Wang Q, Kang Y, Liu J, Yin Y, Liu L, et al. Long noncoding RNAs control the modulation of immune checkpoint molecules in cancer. Cancer Immunol Res (2020) 8(7):937–51. doi: 10.1158/2326-6066.CIR-19-0696
79. Wu J, Li M, Zhang Y, Cai Y, Zhao G. Molecular mechanism of activated T cells in breast cancer. Onco Targets Ther (2018) 11:5015–24. doi: 10.2147/OTT.S173018
80. Wang Y, Zhu M, Guo F, Song Y, Fan X, Qin G. Identification of tumor microenvironment-related prognostic biomarkers in luminal breast cancer. Front Genet (2020) 11. doi: 10.3389/fgene.2020.555865
81. Liu S, Song A, Zhou X, Huo Z, Yao S, Yang B, et al. ceRNA network development and tumour-infiltrating immune cell analysis of metastatic breast cancer to bone. J Bone Oncol (2020) 24:1–16. doi: 10.1016/j.jbo.2020.100304
82. Peng Z, Su P, Yang Y, Yao X, Zhang Y, Jin F, et al. Identification of CTLA-4 associated with tumor microenvironment and competing interactions in triple negative breast cancer by co-expression network analysis. J Cancer (2020) 11:6365–75. doi: 10.7150/jca.46301
83. Wang Z, Yang X, Shen J, Xu J, Pan M, Liu J, et al. Gene expression patterns associated with tumor-infiltrating CD4+ and CD8+ T cells in invasive breast carcinomas. Hum Immunol (2021) 82:279–87. doi: 10.1016/j.humimm.2021.02.001
84. Thomas PB, Seim I, Jeffery PL, Gahete MD, Maugham M, Crisp GJ, et al. The long non-coding RNA GHSROS facilitates breast cancer cell migration and orthotopic xenograft tumour growth. Int J Oncol (2019) 55:1223–36. doi: 10.3892/ijo.2019.4891
85. Bo H, Zhang W, Zhong X, Chen J, Liu Y, Cheong K, et al. LINC00467, driven by copy number amplification and DNA demethylation, is associated with oxidative lipid metabolism and immune infiltration in breast cancer. Oxid Med Cell Longev (2021) 2021:1–27. doi: 10.1155/2021/4586319
86. Chen J, Wei Y, Yang W, Huang Q, Chen Y, Zeng K, et al. IL-6: the link between inflammation, immunity and breast cancer. Front Oncol (2022) 12:903800. doi: 10.3389/fonc.2022.903800
87. Kumari N, Dwarakanath BS, Das A, Bhatt AN. Role of interleukin-6 in cancer progression and therapeutic resistance. Tumor Biol (2016) 37(9):11553–72. doi: 10.1007/s13277-016-5098-7
88. Rašková M, Lacina L, Kejík Z, Venhauerová A, Skaličková M, Kolář M, et al. The role of IL-6 in cancer cell invasiveness and metastasis–overview and therapeutic opportunities. Cells (2022) 11(22):1–23. doi: 10.3390/cells11223698
89. Fisher DT, Appenheimer MM, Evans SS. The two faces of IL-6 in the tumor microenvironment. Semin Immunol (2014) 26(1):38–47. doi: 10.1016/j.smim.2014.01.008
90. Xing Z, Lin A, Li C, Liang K, Wang S, Liu Y, et al. LncRNA directs cooperative epigenetic regulation downstream of chemokine signals. Cell (2014) 159:1110–25. doi: 10.1016/j.cell.2014.10.013
91. DeVaux RS, Ropri AS, Grimm SL, Hall PA, Herrera EO, Chittur SV, et al. Long noncoding RNA BHLHE40-AS1 promotes early breast cancer progression through modulating IL-6/STAT3 signaling. J Cell Biochem (2020) 121(7):3465–78. doi: 10.1002/jcb.29621
92. Nyati KK, Hashimoto S, Singh SK, Tekguc M, Metwally H, Liu YC, et al. The novel long noncoding RNA AU021063, induced by IL-6/Arid5a signaling, exacerbates breast cancer invasion and metastasis by stabilizing Trib3 and activating the Mek/Erk pathway. Cancer Lett (2021) 520:295–306. doi: 10.1016/j.canlet.2021.08.004
93. McLane LM, Abdel-Hakeem MS, Wherry EJ. CD8 T cell exhaustion during chronic viral infection and cancer. Annu Rev Immunol (2019) 37:457–95. doi: 10.1146/annurev-immunol-041015-055318
94. Prado-Garcia H, Romero-Garcia S. The role of exhaustion in tumor-induced T-cell dysfunction in cancer. Cancer Immunol A Transl Med Context Second Ed (2020), 117–32. doi: 10.1007/978-3-030-30845-2_8
95. Hussen BM, Azimi T, Hidayat HJ, Taheri M, Ghafouri-Fard S. NF-KappaB interacting LncRNA: review of its roles in neoplastic and non-neoplastic conditions. BioMed Pharmacother (2021) 139:1–8. doi: 10.1016/j.biopha.2021.111604
96. Huang D, Chen J, Yang L, Ouyang Q, Li J, Lao L, et al. NKILA lncRNA promotes tumor immune evasion by sensitizing T cells to activation-induced cell death. Nat Immunol (2018) 19(10):1112–25. doi: 10.1038/s41590-018-0207-y
97. Liu B, Sun L, Liu Q, Gong C, Yao Y, Lv X, et al. A cytoplasmic NF-κB interacting long noncoding RNA blocks IκB phosphorylation and suppresses breast cancer metastasis. Cancer Cell (2015) 27(3):370–81. doi: 10.1016/j.ccell.2015.02.004
98. Wu W, Chen F, Cui X, Yang L, Chen J, Zhao J, et al. LncRNA NKILA suppresses TGF-β-induced epithelial–mesenchymal transition by blocking NF-κB signaling in breast cancer. Int J Cancer (2018) 143:2213–24. doi: 10.1002/ijc.31605
99. Yu Y, Zhang W, Li A, Chen Y, Ou Q, He Z, et al. Association of long noncoding RNA biomarkers with clinical immune subtype and prediction of immunotherapy response in patients with cancer. JAMA Netw Open (2020) 3(4):1–12. doi: 10.1001/jamanetworkopen.2020.2149
100. Sakaguchi S, Mikami N, Wing JB, Tanaka A, Ichiyama K, Ohkura N. Regulatory T cells and human disease. Annu Rev Immunol (2020) 38:541–66. doi: 10.1146/annurev-immunol-042718-041717
101. Speiser DE, Ho PC, Verdeil G. Regulatory circuits of T cell function in cancer. Nat Rev Immunol (2016) 16(10):599–611. doi: 10.1038/nri.2016.80
102. Moallemi-Rad L, Ghorbani A, Dadyar M, Hussen BM, Rasul MF, Eslami S, et al. Expression of treg-associated lncRNAs in breast cancer. Pathol Res Pract (2022) 241:1–7. doi: 10.1016/j.prp.2022.154270
103. Zimta AA, Tigu AB, Braicu C, Stefan C, Ionescu C, Berindan-Neagoe I. An emerging class of long non-coding RNA with oncogenic role arises from the snoRNA host genes. Front Oncol (2020) 10:389(April). doi: 10.3389/fonc.2020.00389
104. Pei X, Wang X, Li H. LncRNA SNHG1 regulates the differentiation of treg cells and affects the immune escape of breast cancer via regulating miR-448/IDO. Int J Biol Macromol (2018) 118:24–30. doi: 10.1016/j.ijbiomac.2018.06.033
105. Ni C, Fang QQ, Chen WZ, Jiang JX, Jiang Z, Ye J, et al. Breast cancer-derived exosomes transmit lncRNA SNHG16 to induce CD73+γδ1 treg cells. Signal Transduct Target Ther (2020) 5(1):1–14. doi: 10.1038/s41392-020-0129-7
106. Mantovani A, Marchesi F, Malesci A, Laghi L, Allavena P. Tumour-associated macrophages as treatment targets in oncology. Nat Rev Clin Oncol (2017) 14(7):399–416. doi: 10.1038/nrclinonc.2016.217
107. Zhou L, Tian Y, Guo F, Yu B, Li J, Xu H, et al. LincRNA-p21 knockdown reversed tumor-associated macrophages function by promoting MDM2 to antagonize* p53 activation and alleviate breast cancer development. Cancer Immunol Immunother (2020) 69(5):835–46. doi: 10.1007/s00262-020-02511-0
108. Chen F, Chen J, Yang L, Liu J, Zhang X, Zhang Y, et al. Extracellular vesicle-packaged HIF-1α-stabilizing lncRNA from tumour-associated macrophages regulates aerobic glycolysis of breast cancer cells. Nat Cell Biol (2019) 21(4):498–510. doi: 10.1038/s41556-019-0299-0
109. Amer HT, Eissa RA, El Tayebi HM. A cutting-edge immunomodulatory interlinkage between HOTAIR and MALAT1 in tumor-associated macrophages in breast cancer: a personalized immunotherapeutic approach. Front Mol Biosci (2022) 9:1032517. doi: 10.3389/fmolb.2022.1032517
110. Liu XS, Mardis ER. Applications of immunogenomics to cancer. Cell (2017) 168(4):600–12. doi: 10.1016/j.cell.2017.01.014
111. Finotello F, Rieder D, Hackl H, Trajanoski Z. Next-generation computational tools for interrogating cancer immunity. Nat Rev Genet (2019) 20(12):724–46. doi: 10.1038/s41576-019-0166-7
112. Mardis ER. The impact of next-generation sequencing on cancer genomics: from discovery to clinic. Cold Spring Harb Perspect Biol (2019) 11(9):1–14. doi: 10.1101/cshperspect.a036269
113. Hammerbacher J, Snyder A. Informatics for cancer immunotherapy. Ann Oncol (2017) 28(January):xii56–73. doi: 10.1093/annonc/mdx682
114. Finotello F, Trajanoski Z. Quantifying tumor-infiltrating immune cells from transcriptomics data. Cancer Immunol Immunother (2018) 67:1–10. doi: 10.1007/s00262-018-2150-z
115. Turajlic S, Litchfield K, Xu H, Rosenthal R, McGranahan N, Reading JL, et al. Insertion-and-deletion-derived tumour-specific neoantigens and the immunogenic phenotype: a pan-cancer analysis. Lancet Oncol (2017) 18(8):1009–21. doi: 10.1016/j.cell.2017.01.014
116. Hashimoto S, Noguchi E, Bando H, Miyadera H, Morii W, Nakamura T, et al. Neoantigen prediction in human breast cancer using RNA sequencing data. Cancer Sci (2021) 112(1):465–75. doi: 10.1111/cas.14720
117. Marty R, Kaabinejadian S, Rossell D, Slifker MJ, van de Haar J, Engin HB, et al. MHC-I genotype restricts the oncogenic mutational landscape. Cell (2017) 171(6):1–28. doi: 10.1016/j.cell.2017.09.050
118. Schaafsma E, Fugle CM, Wang X, Cheng C. Pan-cancer association of HLA gene expression with cancer prognosis and immunotherapy efficacy. Br J Cancer (2021) 125(3):422–32. doi: 10.1038/s41416-021-01400-2
119. Charoentong P, Angelova M, Finotello F, Mayer C, Efremova M, Rieder D, et al. Pan-cancer immunogenomic analyses reveal genotype-immunophenotype relationships and predictors of response to checkpoint blockade. Cell Rep (2017) 18(1):248–62. doi: 10.1016/j.celrep.2016.12.019
120. Thorsson V, Gibbs DL, Brown SD, Wolf D, Bortone DS, Ou Yang TH, et al. The immune landscape of cancer. Immunity (2018) 48(4):812–830.e14. doi: 10.1016/j.immuni.2018.03.023
121. Rooney MS, Shukla SA, Wu CJ, Getz G, Hacohen N. Molecular and genetic properties of tumors associated with local immune cytolytic activity. Cell (2015) 160(1–2):48–61. doi: 10.1016/j.cell.2014.12.033
122. Li B, Li T, Pignon JC, Wang B, Wang J, Shukla SA, et al. Landscape of tumor-infiltrating T cell repertoire of human cancers. Nat Genet (2016) 48(7):725–32. doi: 10.1038/ng.3581
123. Zhang Y, Zhang Z. The history and advances in cancer immunotherapy: understanding the characteristics of tumor-infiltrating immune cells and their therapeutic implications. Cell Mol Immunol (2020) 17(8):807–21. doi: 10.1038/s41423-020-0488-6
124. Li Y, Jiang T, Zhou W, Li J, Li X, Wang Q, et al. Pan-cancer characterization of immune-related lncRNAs identifies potential oncogenic biomarkers. Nat Commun (2020) 11(1):1–13. doi: 10.1038/s41467-020-14802-2
125. Zhang Z, Yan C, Li K, Bao S, Li L, Chen L, et al. Pan-cancer characterization of lncRNA modifiers of immune microenvironment reveals clinically distinct de novo tumor subtypes. NPJ Genomic Med (2021) 6(1):1–11. doi: 10.1038/s41525-021-00215-7
126. Zhang Z, Zhang H, Li D, Zhou X, Wang J, Zhang Q. LncRNA ST7-AS1 is a potential novel biomarker and correlated with immune infiltrates for breast cancer. Front Mol Biosci (2021) 8:604261(April). doi: 10.3389/fmolb.2021.604261
127. Yi W, Shen H, Sun D, Xu Y, Feng Y, Li D, et al. Low expression of long noncoding RNA SLC26A4 antisense RNA 1 is an independent prognostic biomarker and correlate of immune infiltrates in breast cancer. Med Sci Monit (2021) 27:1–14. doi: 10.12659/MSM.934522
128. Zhao D, Dong JT. Upregulation of long non-coding RNA DRAIC correlates with adverse features of breast cancer. Non-coding RNA (2018) 4(39):1–9. doi: 10.3390/ncrna4040039
129. Zhang Y, Li Z, Chen M, Chen H, Zhong Q, Liang L, et al. lncRNA TCL6 correlates with immune cell infiltration and indicates worse survival in breast cancer. Breast Cancer (2020) 27(4):573–85. doi: 10.1007/s12282-020-01048-5
130. Fonseca-Montaño MA, Cisneros-Villanueva M, Coales I, Hidalgo-Miranda A. LINC00426 is a potential immune phenotype-related biomarker and an overall survival predictor in PAM50 luminal b breast cancer. Front Genet (2023) 2:1034569(May). doi: 10.3389/fgene.2023.1034569
131. Liu Z, Mi M, Li X, Zheng X, Wu G, Zhang L. lncRNA OSTN-AS1 may represent a novel immune-related prognostic marker for triple-negative breast cancer based on integrated analysis of a ceRNA network. Front Genet (2019) 10:850. doi: 10.3389/fgene.2019.00850
132. de Santiago PR, Blanco A, Morales F, Marcelain K, Harismendy O, Sjöberg Herrera M, et al. Immune-related IncRNA LINC00944 responds to variations in ADAR1 levels and it is associated with breast cancer prognosis. Life Sci (2021) 268:1–11. doi: 10.1016/j.lfs.2020.118956
133. Bradford JR, Cox A, Bernard P, Camp NJ. Consensus analysis of whole transcriptome profiles from two breast cancer patient cohorts reveals long non-coding RNAs associated with intrinsic subtype and the tumour microenvironment. PloS One (2016) 11(9):1–18. doi: 10.1371/journal.pone.0163238
134. Liu D. Identification of a prognostic LncRNA signature for ER-positive, ER-negative and triple-negative breast cancers. Breast Cancer Res Treat (2020) 183:95–105. doi: 10.1007/s10549-020-05770-8
135. Mathias C, Muzzi JCD, Antunes BB, Gradia DF, Castro MAA, Carvalho de Oliveira J. Unraveling immune-related lncRNAs in breast cancer molecular subtypes. Front Oncol (2021) 11:692170(May). doi: 10.3389/fonc.2021.692170
136. De Palma FDE, Del Monaco V, Pol JG, Kremer M, D’Argenio V, Stoll G, et al. The abundance of the long intergenic non-coding RNA 01087 differentiates between luminal and triple-negative breast cancers and predicts patient outcome. Pharmacol Res (2020) 161:1–17. doi: 10.1016/j.phrs.2020.105249
137. Ghafouri-Fard S, Asadi M, Sohrabi B, Arsang-Jang S, Mehravaran E, Taheri M, et al. Down-regulation of a panel of immune-related lncRNAs in breast cancer. Pathol Res Pract (2021) 224:1–9. doi: 10.1016/j.prp.2021.153534
138. Zhao H, Liu X, Yu L, Lin S, Zhang C, Xu H, et al. Comprehensive landscape of epigenetic-dysregulated lncRNAs reveals a profound role of enhancers in carcinogenesis in BC subtypes. Mol Ther Nucleic Acids (2021) 23:667–81. doi: 10.1016/j.omtn.2020.12.024
139. Sui Y, Ju C, Shao B. A lymph node metastasis-related protein-coding genes combining with long noncoding RNA signature for breast cancer survival prediction. J Cell Physiol (2019) 23:1–10. doi: 10.1002/jcp.28600
140. Li H, Liu H, Hao Q, Liu X, Yao Y, Cao M. Oncogenic signaling pathway-related long non-coding RNAs for predicting prognosis and immunotherapy response in breast cancer. Front Immunol (2022) 13:891175(August). doi: 10.3389/fimmu.2022.891175
141. Shen S, Chen X, Hu X, Huo J, Luo L, Zhou X. Predicting the immune landscape of invasive breast carcinoma based on the novel signature of immune-related lncRNA. Cancer Med (2021) 10:6561–75. doi: 10.1002/cam4.4189
142. Liu B, Zhu N, Huo H, Long J, Ji X, Li J, et al. A 5-pathway signature predicts prognosis based on immune-derived lncRNAs in patients with breast cancer. J Oncol (2022) 2022:1–17. doi: 10.1155/2022/2906049
143. Li S, Sun X, Li J, Zheng A, Cao Y, Guo Y, et al. A novel prognostic signature of immune-related long noncoding RNA pairs for tumor-infiltrating immune cells and drug susceptibility in breast cancer. DNA Cell Biol (2022) 41(2):103–15. doi: 10.1089/dna.2021.0489
144. Liu Z, Mi M, Li X, Zheng X, Wu G, Zhang L. A lncRNA prognostic signature associated with immune infiltration and tumour mutation burden in breast cancer. J Cell Mol Med (2020) 24(21):1–13. doi: 10.1111/jcmm.15762
145. Xiao R, Yang M, Tan Y, Ding R, Li D. Identification of five immune-related lncRNAs predicting survival and tumor microenvironment characteristics in breast cancer. Comput Math Methods Med (2021) 2021:1–12. doi: 10.1155/2021/6676692
146. Li YX, Wang SM, Li CQ. Four-lncRNA immune prognostic signature for triple-negative breast cancer. Math Biosci Eng (2021) 18(4):3939–56. doi: 10.3934/mbe.2021197
147. Lai J, Chen B, Zhang G, Li X, Mok H, Liao N. Molecular characterization of breast cancer: a potential novel immune-related lncRNAs signature. J Transl Med (2020) 18(416):1–10. doi: 10.1186/s12967-020-02578-4
148. Li Q, Yang H, Wang P, Liu X, Lv K, Ye M. XGBoost-based and tumor-immune characterized gene signature for the prediction of metastatic status in breast cancer. J Transl Med (2022) 20(1):1–12. doi: 10.1186/s12967-022-03369-9
149. Li Z, Li Y, Wang X, Yang Q. Identification of a six-Immune-Related long non-coding RNA signature for predicting survival and immune infiltrating status in breast cancer. Front Genet (2020) 11:680(July). doi: 10.3389/fgene.2020.00680
150. Ma W, Zhao F, Yu X, Guan S, Suo H, Tao Z, et al. Immune-related lncRNAs as predictors of survival in breast cancer: a prognostic signature. J Transl Med (2020) 18(442):1–13. doi: 10.1186/s12967-020-02522-6
151. Jiang T, Wang Y, Chen X, Xia W, Xue S, Gu L, et al. Neutrophil extracellular traps (NETs)-related lncRNAs signature for predicting prognosis and the immune microenvironment in breast cancer. Front Cell Dev Biol (2023) 11:1117637(February). doi: 10.3389/fcell.2023.1117637
152. Shen Y, Peng X, Shen C. Identification and validation of immune-related lncRNA prognostic signature for breast cancer. Genomics (2020) 112:2640–6. doi: 10.1016/j.ygeno.2020.02.015
153. Yang W, Qiu Z, Zhang J, Zhi X, Yang L, Qiu M, et al. Correlation between immune cell infiltration and PD-L1 expression and immune-related lncRNA determination in triple-negative breast cancer. Front Genet (2022) 13:878658(March). doi: 10.3389/fgene.2022.878658
154. Zhu JY, Lyu AQ, Wang ZT, Chan WY, Qin T, Miu KK, et al. Machine learning-devised immune-related lncRNA signature panel predicts the prognosis and immune landscape in breast cancer novel IRLP signature in BRCA. J Immunol Res (2022) 2022:1–16. doi: 10.1155/2022/3704
155. Huang Z, Xiao C, Zhang F, Zhou Z, Yu L, Ye C, et al. A novel framework to predict breast cancer prognosis using immune-associated LncRNAs. Front Genet (2021) 11:634195. doi: 10.3389/fgene.2020.634195
156. Dang Q, Sun Z, Wang Y, Wang L, Liu Z, Han X. Ferroptosis: a double-edged sword mediating immune tolerance of cancer. Cell Death Dis (2022) 13(11):1–16. doi: 10.1038/s41419-022-05384-6
157. Shi L, Liu Y, Li M, Luo Z. Emerging roles of ferroptosis in the tumor immune landscape: from danger signals to anti-tumor immunity. FEBS J (2022) 289(13):3655–65. doi: 10.1111/febs.16034
158. Anusha A, Kumar S, Kaushik S, Jyoti A. Necroptosis in immuno-oncology and cancer immunotherapy. J Pharm Sci Res (2017) 9(5):662–6. doi: 10.1097/mou.0000000000000337
159. Liu W, Peng J, Xiao M, Cai Y, Peng B, Zhang W, et al. The implication of pyroptosis in cancer immunology: current advances and prospects. Genes Dis (2022), 1–12. doi: 10.1016/j.gendis.2022.04.019
160. Xia H, Green DR, Zou W. Autophagy in tumour immunity and therapy. Nat Rev Cancer (2021) 21(5):281–97. doi: 10.1038/s41568-021-00344-2
161. Chen M, Linstra R, van Vugt MATM. Genomic instability, inflammatory signaling and response to cancer immunotherapy. Biochim Biophys Acta - Rev Cancer (2022) 1877(1):1–15. doi: 10.1016/j.bbcan.2021.188661
162. Wang Y, Xu Y, Zhang Y. A novel ferroptosis-related long noncoding RNA signature for relapse free survival prediction in patients with breast cancer. Med (United States) (2022) 101(31):1–10. doi: 10.1097/MD.0000000000029573
163. Wei T, Zhu N, Jiang W, Xing XL. Development and validation of ferroptosis- and immune-related lncRNAs signatures for breast infiltrating duct and lobular carcinoma Front oncol. (2022) 12(April):1–11. doi: 10.3389/fonc.2022.844642
164. Zhang K, Ping L, Du T, Liang G, Huang Y, Li Z, et al. A ferroptosis-related lncRNAs signature predicts prognosis and immune microenvironment for breast cancer. Front Mol Biosci (2021) 8:678877(June). doi: 10.3389/fmolb.2021.678877
165. Shen S, Yang D, Yang Y, Chen Y, Xiong J, Hu X. A novel prognostic ferroptosis-related lncRNA signature associated with immune landscape in invasive breast cancer. Dis Markers (2022) 2022:1–25. doi: 10.1155/2022/9168556
166. Jia CL, Yang F, Li R. Prognostic model construction and immune microenvironment analysis of breast cancer based on ferroptosis-related lncRNAs. Int J Gen Med (2021) 14:9817–31. doi: 10.2147/IJGM.S342783
167. Zhang Y, Yue Q, Cao F, Li YQ, Wei Y. Necroptosis-related lncRNA signatures determine prognosis in breast cancer patients. Sci Rep (2022) 12(1):1–14. doi: 10.1038/s41598-022-15209-3
168. Chen F, Yang J, Fang M, Wu Y, Su D, Sheng Y. Necroptosis-related lncRNA to establish novel prognostic signature and predict the immunotherapy response in breast cancer. J Clin Lab Anal (2022) 36(4):1–11. doi: 10.1002/jcla.24302
169. Tao S, Tao K, Cai X. Necroptosis-associated lncRNA prognostic model and clustering analysis: prognosis prediction and tumor-infiltrating lymphocytes in breast cancer. J Oncol (2022) 2022:1–18. doi: 10.1155/2022/7099930
170. Wu Q, Li Q, Zhu W, Zhang X, Li H. Identification of autophagy-related long non-coding RNA prognostic signature for breast cancer. J Cell Mol Med (2021) 25:4088–98. doi: 10.1111/jcmm.16378
171. Luo Z, Nong B, Ma Y, Fang D. Autophagy related long non-coding RNA and breast cancer prognosis analysis and prognostic risk model establishment. Ann Transl Med (2022) 10(2):58–8. doi: 10.21037/atm-21-6251
172. Liu L, Chen C, Tu G, Peng Y, Shen M, Xu Y, et al. Pyroptosis-related lncRNAs for predicting the prognosis and identifying immune microenvironment infiltration in breast cancer lung metastasis. Front Cell Dev Biol (2022) 10:821727. doi: 10.3389/fcell.2022.821727
173. Yang X, Weng X, Yang Y, Jiang ZN. Pyroptosis-related lncRNAs predict the prognosis and immune response in patients with breast cancer. Front Genet (2022) 12:792106(March). doi: 10.3389/fgene.2021.792106
174. Lv Z, Wang Q, Liu X, Du Z, Liang W, Liu T, et al. Genetic instability-related lncRNAs predict prognosis and influence the immune microenvironment in breast cancer. Front Genet (2022) 13:926984(September). doi: 10.3389/fgene.2022.926984
175. Jiao Y, Li S, Wang X, Yi M, Wei H, Rong S, et al. A genomic instability-related lncRNA model for predicting prognosis and immune checkpoint inhibitor efficacy in breast cancer. Front Immunol (2022) 13:929846(August). doi: 10.3389/fimmu.2022.929846
176. Shi GJ, Zhou Q, Zhu Q, Wang L, Jiang GQ. A novel prognostic model associated with the overall survival in patients with breast cancer based on lipid metabolism-related long noncoding RNAs. J Clin Lab Anal (2022) 36(6):1–9. doi: 10.1002/jcla.24384
177. Gu P, Zhang L, Wang R, Ding W, Wang W, Liu Y, et al. Development and validation of a novel hypoxia-related long noncoding RNA model with regard to prognosis and immune features in breast cancer. Front Cell Dev Biol (2021) 9:796729(December). doi: 10.3389/fcell.2021.796729
178. Jiang ZR, Yang LH, Jin LZ, Yi LM, Bing PP, Zhou J, et al. Identification of novel cuproptosis-related lncRNA signatures to predict the prognosis and immune microenvironment of breast cancer patients. Front Oncol (2022) 12:988680(September). doi: 10.3389/fonc.2022.988680
179. Li J, Zhang Y, Li C, Wu H, Feng C, Wang W, et al. A lactate-related LncRNA model for predicting prognosis, immune landscape and therapeutic response in breast cancer. Front Genet (2022) 13:956246(October). doi: 10.3389/fgene.2022.956246
180. Liu Z, Ren C, Cai J, Yin B, Yuan J, Ding R, et al. A novel aging-related prognostic lncRNA signature correlated with immune cell infiltration and response to immunotherapy in breast cancer. Molecules (2023) 28(8):1–22. doi: 10.3390/molecules28083283
181. Wang Y, Gao S, Xu Y, Tang Z, Liu S. A mitochondrial function-related LncRNA signature predicts prognosis and immune microenvironment for breast cancer. Sci Rep (2023) 13(1):1–17. doi: 10.1038/s41598-023-30927-y
182. Gao S, Wang Y, Xu Y, Liu S. An angiogenesis-related lncRNA signature is associated with prognosis and tumor immune microenvironment in breast cancer. J Pers Med (2023) 13(3):1–23. doi: 10.3390/jpm13030513
183. Zhao J, Ma H, Feng R, Li D, Liu B, Yu Y, et al. A novel oxidative stress-related lncRNA signature that predicts the prognosis and tumor immune microenvironment of breast cancer. J Oncol (2022) 2022:1–22. doi: 10.1155/2022/9766954
184. Huang G, Cao H, Liu G, Chen J. Role of androgen receptor signaling pathway-related lncRNAs in the prognosis and immune infiltration of breast cancer. Sci Rep (2022) 12(1):1–17. doi: 10.1038/s41598-022-25231-0
185. Zou J, Gu Y, Zhu Q, Li X, Qin L. Identifying glycolysis-related LncRNAs for predicting prognosis in breast cancer patients. Cancer Biomarkers (2022) 34(3):393–401. doi: 10.3233/CBM-210446
186. Guo J, Yi X, Ji Z, Yao M, Yang Y, Song W, et al. Development of a prognostic model based on the identification of EMT-related lncRNAs in triple-negative breast cancer. J Oncol (2021) 2021:1–18. doi: 10.1155/2021/9219961
187. Qian D, Qian C, Ye B, Xu M, Wu D, Li J, et al. Development and validation of a novel stemness-Index-Related long noncoding RNA signature for breast cancer based on weighted gene Co-expression network analysis. Front Genet (2022) 13:760514(February). doi: 10.3389/fgene.2022.760514
188. Xu S, Xie J, Zhou Y, Liu H, Wang Y, Li Z. Integrated analysis of RNA binding protein-related lncRNA prognostic signature for breast cancer patients. Genes (Basel) (2022) 13(2):1–18. doi: 10.3390/genes13020345
189. Cai J, Ji Z, Wu J, Chen L, Zheng D, Chen Y, et al. Development and validation of a novel endoplasmic reticulum stress-related lncRNA prognostic signature and candidate drugs in breast cancer. Front Genet (2022) 13:949314. doi: 10.3389/fgene.2022.949314
190. Zhang L, Zhang Y, Bao J, Gao W, Wang D, Pan H. Cuproptosis combined with lncRNAs predicts the prognosis and immune microenvironment of breast cancer. Comput Math Methods Med (2022) 2022:1–32. doi: 10.1155/2022/5422698
191. Chen BQ, Dragomir MP, Yang C, Li Q, Horst D, Calin GA. Targeting non-coding RNAs to overcome cancer therapy resistance. Signal Transduct Target Ther (2022) 7(1):1–20. doi: 10.1038/s41392-022-00975-3
192. Winkle M, El-Daly SM, Fabbri M, Calin GA. Noncoding RNA therapeutics-challenges and potential solutions. Nat Rev Drug Discovery (2021) 20(8):629–51. doi: 10.1038/s41573-021-00219-z
193. Jiang M-C, Ni J-J, Cui W-Y, Wei Z. Emerging roles of lncRNA in cancer and therapeutic opportunities. Am J Cancer Res (2019) 9(7):1354–66. doi: 2156-6976/ajcr0097340
Keywords: breast cancer, immune cells, tumor immune microenvironment, lncRNAs, immune response, biomarker, prognosis
Citation: Fonseca-Montaño MA, Vázquez-Santillán KI and Hidalgo-Miranda A (2023) The current advances of lncRNAs in breast cancer immunobiology research. Front. Immunol. 14:1194300. doi: 10.3389/fimmu.2023.1194300
Received: 26 March 2023; Accepted: 24 May 2023;
Published: 05 June 2023.
Edited by:
Eyad Elkord, University of Salford, United KingdomCopyright © 2023 Fonseca-Montaño, Vázquez-Santillán and Hidalgo-Miranda. This is an open-access article distributed under the terms of the Creative Commons Attribution License (CC BY). The use, distribution or reproduction in other forums is permitted, provided the original author(s) and the copyright owner(s) are credited and that the original publication in this journal is cited, in accordance with accepted academic practice. No use, distribution or reproduction is permitted which does not comply with these terms.
*Correspondence: Marco Antonio Fonseca-Montaño, mfonseca.mntn@gmail.com; Alfredo Hidalgo-Miranda, ahidalgo@inmegen.gob.mx