- 1Oncology Department, The First Affiliated Hospital of Shandong First Medical University & Shandong Provincial Qianfoshan Hospital, Jinan, China
- 2Shandong Provincial Key Laboratory for Rheumatic Disease and Translational Medicine and Shandong Lung Cancer Institute, Jinan, China
The treatment outcome of breast cancer is closely related to estrogen receptor (ER), progesterone receptor (PR), and human epidermal growth factor receptor 2 (HER2) expression. Triple-negative breast cancer (TNBC) lacking ER, PR, and HER2 expression has limited treatment options and a poor prognosis. Tumor-infiltrating lymphocytes (TILs) play a role in promoting or resisting tumors by affecting the tumor microenvironment and are known as key regulators in breast cancer progression. However, treatments for TNBC (e.g., surgery, chemotherapy and radiotherapy) have non-satisfaction’s curative effect so far. This article reviews the role of different types of TILs in TNBC and the research progress of adoptive cell therapy, aiming to provide new therapeutic approaches for TNBC.
1 Introduction
Breast cancer is the most common cancer in women worldwide. The International Agency for Research on Cancer estimates that the incidence of breast cancer will increase by more than 40% and the mortality rate will increase by more than 50% by 2040 (1). Breast cancer can be characterized into four subtypes: luminal A, luminal B, human epidermal growth factor receptor 2 (HER2)-enriched, and triple-negative breast cancer (TNBC) subtypes (2). Among them, TNBC with negative immunohistochemical results for estrogen receptor (ER), progesterone receptor (PR), and HER2 in the breast cancer tissue (3) is a highly heterogenous disease with an extremely poor prognosis. TNBC accounts for 15–20% of breast cancers (4). Because of its unique phenotype, TNBC is mainly treated by cytotoxic chemotherapy.
Increasingly more studies in recent years have shown that tumor-infiltrating lymphocytes (TILs) are associated with the progression of TNBC. Among patients with metastatic breast cancer, those with high-level TILs tend to have better therapeutic outcomes (5). TILs are mainly composed of T cells, B cells, and natural killer (NK) cells and react with tumor cells and non-TILs of breast cancer patients in various ways, promoting or resisting tumors and affecting the prognosis of the patients (6). Hence, further research on the role of TILs in TNBC helps provide new approaches for the treatment of TNBC.
2 TIL subsets interact and coordinate to build a more rigorous anti-tumor immunity against the tumor microenvironment
With the participation of the extracellular matrix, cellular components, such as tumor cells, vascular endothelial cells, pericytes, immune cells, bone marrow-derived cells, and tumor-associated fibroblasts form a relatively dynamic environment, the TME, through the release or induction of cell-signaling proteins (e.g., cytokines and chemokines). The interaction between tumor cells and the TME has a fundamental impact on cancer initiation, progression, and therapeutic efficacy (7). The rapid proliferation of tumor cells creates a hypoxic environment in the body, upregulates the expression of hypoxia-inducible factors, accelerates the recruitment of tumor cells and other related cell components to the TME, and ultimately promotes the development and metastasis of cancer cells (8). For example, hypoxia affects the release of exosomes, thereby promoting the secretion of various pro-angiogenic factors (e.g., vascular endothelial growth factor (VEGF), angiopoietin 1, and matrix metalloproteinase 9) in human umbilical vein endothelial cells (9, 10), stimulating the proliferation and migration of cancer cells and regulating tumor angiogenesis. VEGF inhibits the effect of antigen-presenting cells and effector T cells or activates immunosuppressive cells such as T regulatory cells and myeloid-derived suppressor cells and enhances the invasion effect of tumor-associated macrophages, thereby jointly suppressing the immune responses of the body (11). Nevertheless, a high TIL density in patients with TNBC is usually correlated with longer survival (12). Among the TIL subsets, T cells account for up to 75% of TILs (13). As the lymphocytes with the largest proportion in invasive breast cancer, CD8+ T cells are indirectly recruited by NK cells, which are only 5% of TILs, to form an important line of defense for anti-tumor immunity (14–16). B cells serve as antigen-presenting cells for T cells, eliciting localized T-cell responses in tumors (6) (Figure 1).
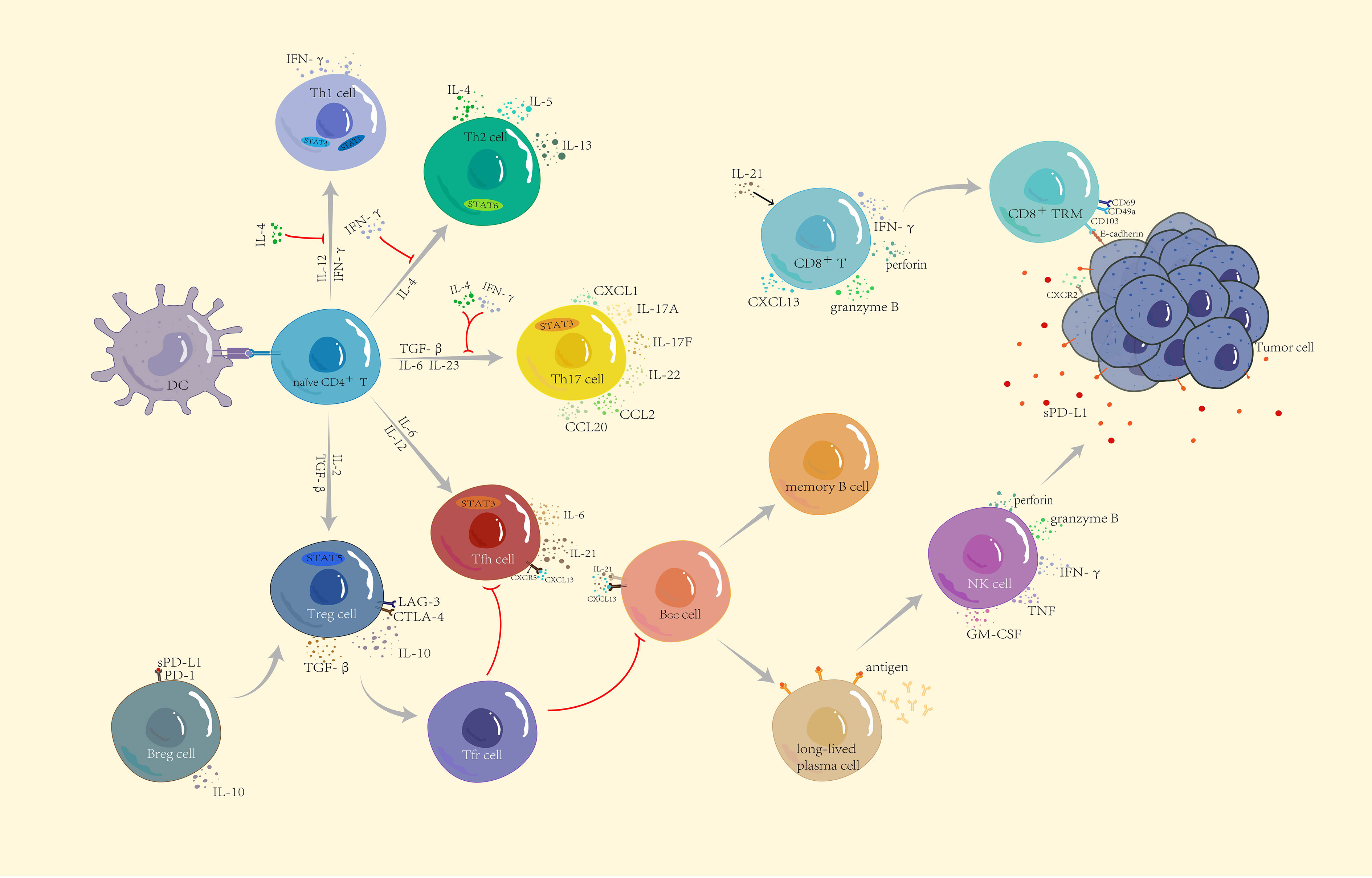
Figure 1 Functional subsets of CD4+ T cells and the role of other lymphocytes in immunity. Naïve CD4+ T cells differentiate into five subtypes: Th1, Th2, Th17, Treg, and Tfh, through the corresponding STAT pathway and cytokine induction. IFN-γ and IL-4 produced by anti-tumor Th1 cells and tumor-promoting Th2 cells not only restrict each other to maintain balance but also jointly inhibit the differentiation of Th17 cells. Th17 cells have a dual role—according to the different TMEs to secrete CCL2 and CCL20 to enhance immunosuppression or secrete CXCL1 to directly act on the surface of tumor cells to promote tumor progression. CXCL13 secreted by CD8+ T cells not only recruits Tfh but also participates in B cell differentiation together with IL-21. Differentiated and mature long-lived plasma cells release corresponding antibodies and upregulate NK cells to kill tumor cells. CD8+ T cells differentiate into tissue-resident memory CD8+ T cells to recognize E-cadherin on the surface of tumor cells and inhibit tumor growth. Breg and Treg cells are both tumor-promoting cells. Breg cells release IL-10 and recognize soluble PD-L1, which jointly induce Treg cell differentiation, suppress immune responses, and build a microenvironment which is conducive to tumor growth.
2.1 As a good prognostic marker, TILs provide a basis for subsequent diagnosis and treatment of TNBC
2.2.1 T cells cooperate with each other to push the TME to the peak of tumor growth inhibition
T cell–mediated immunity plays a leading role in the anti-tumor process. After positive selection, T cells differentiate into CD4+ T and CD8+ T cells. Naïve CD4+ T cells differentiate into five subtypes: T helper (Th)1, Th2, Th17, T regulatory (Treg), and T follicular helper (Tfh) cells. Th1-secreted interferon gamma (IFN-γ) not only induces the activation of signal transducer and activator of transcription (STAT)1 and STAT4 to promote the differentiation of more Th1 cells but also recruits CD8+ T cells, NK cells, and other immune cells to enhance anti-tumor immunity, while Th2 cells with tumor-promoting effects mainly depend on the induction of IL-4 for differentiation (17). Importantly, IFN-γ inhibits the differentiation of Th2 cells, and interleukin (IL)-4 inhibits the differentiation of Th1 cells, thereby mutually restricting each other to maintain balance in the tumor. IFN-γ and IL-4 inhibit the development of Th17 cells (18). In the absence of these two cytokines (IFN-γ and IL-4), naïve CD4+ T cells are polarized into Th17 cells under the co-induction of TGF-β, IL-6, and IL-23 and subsequently secrete IL-17A, IL-17F, and IL-22 and trigger the inflammatory response in the body; moreover, Th17 cells induce the production of chemokines, such as C-C motif ligand 2 (CCL2) and CCL20 to promote macrophages and activate T cells to infiltrate the TME (19, 20). In addition, Th17, as a novel prognostic marker, was directly associated with improved overall survival of patients with non-inflammatory TNBC (21). However, a previous study showed that Th17 cells produced C-X-C motif chemokine ligand 1 (CXCL1) during breast cancer progression, which enhanced cancer cell invasion (22). As another subtype of CD4+ T cells, Tfh cells exist in secondary lymphoid tissues and recognize CXCL13 released by CD8+ T cells through regulating C-X-C chemokine receptor type 5 (CXCR5) to mediate Tfh cell recruitment. Tfh cells also secrete IL-21 to stimulate the differentiation of CD8+ T cells to participate in cytokine production and play cytotoxicity. As the main helper cells of tumor-infiltrating B cells, Tfh cells participate in germinal center formation and B cell differentiation, and control the humoral immune response by interacting with T follicular regulatory cells that differentiate from Treg cells. When the equilibrium shifts to the side with Tfh cells, they activate humoral immunity to actively kill tumors (23, 24).
Treg cells are a T cell subtype that promotes immune escape of tumor cells. Because of a relatively high immunogenicity, TNBC has higher Treg cell infiltration. A study by Bai et al. showed that targeting Annexin A1 downregulated CD25, C-C chemokine receptor 8 (CXCR8), and programmed cell death protein 1 (PD-1) expression and reduced the function of Treg cells, thereby enhancing anti-tumor immunity in TNBC (25). However, some scholars believe that Treg cells have a positive impact on the prognosis of patients with breast cancer. Cai et al. showed that Foxp3+ and Foxp3− Treg cells were found in CD4+CD25+ TILs in 17 patients with TNBC (12). Foxp3+ circulating T cells expressed higher levels of cytotoxic T lymphocyte antigen 4, lymphocyte activation gene 3, and transforming growth factor-beta (TGF-β) after T-cell receptor (TCR) stimulation, while Foxp3− circulating T cells produced high levels of IL-10, enhancing the immune effect of CD8+ TILs (26).
CD8+ T cells recognize specific antigenic peptides on the surface of tumor cells and release large amounts of IFN-γ, granzyme B, and perforin to destroy tumor cells. Surprisingly, the higher expression of CD8+ T cell is significantly correlated with better survival in TNBC (27). CD103 is composed of the integrin subunit αE and integrin β7. CD8+ T cells co-expressing CD103, CD69, and CD49a are called tissue-resident memory T cells (28). As an E-cadherin receptor, CD103 further interacts with E-cadherin to promote the activation and migration of T cells. Shields et al. showed that E-cadherin enhanced the expression of CD103 in melanoma. Under the combined actions of mature B and T lymphocytes, exogenous expression of E-cadherin delayed tumor growth, reduced tumor metastasis, and improved the survival of patients with melanoma (29). In contrast, E-cadherin deficiency promoted tumor growth and metastasis. However, the expression and anti-tumor activity of E-cadherin on CD103 in TNBC have not been verified, but some studies have preliminarily shown that CD103 and E-cadherin exist in TNBC as good prognostic markers (30, 31).
2.2.2 Are B cells “friend” or “foe”?
Humoral immunity has increasingly shown a crucial role in the TME in recent years. B cells are effector cells that are mainly responsible for humoral immunity. They are activated by antigen stimulation and co-stimulatory signals. Under the action of CXCL13, the interaction between follicular dendritic and Tfh cells and B cells triggers the germinal center response, which induces the differentiation of activated B cells into memory B cells and long-lived plasma cells (32). When antigens are presented to B cells, B cells recognize and secrete the corresponding antibodies, which stimulate NK cells to naturally kill target tumor cells.
Studies have shown that TNBC is rich in highly activated B cells. By enhancing the expression of CXCL13, CXCR4, CCL19, IL-17, IL-22, and other cytokines or chemokines, B cells are recruited and accumulate in the tertiary lymphoid structure, and anti-tumor dense aggregates are formed (33, 34). The density of B lymphocytes expressing immunoglobulin (Ig)G, and particularly IgG1, are elevated in tumors and induce antigen-driven reactions with a high affinity. This improves the sensitivity of the humoral immunity of the body, which was shown to relate to positive therapeutic outcomes of TNBC. Vito et al. showed that upregulation of the B cell antigen receptor signaling pathway inhibited the expression of genes encoding nitric oxide synthase 2, arginase 2, IL-1 receptor type 1, and related factors, destroying the inhibitory TME formed by high-density myeloid-derived suppressor cells (MDSCs) in breast cancer, blocking the immune suppression of T cells by MDSCs, and promoting anti-tumor responses of immune cells in the TME (35).
Similar to T cells, regulatory B (Breg) cells are a subset of B cells that suppress immune responses in the TME. They secrete a large amount of IL-10 to negatively regulate T cell immunity. In addition, Breg cells recognize soluble programmed death-ligand 1 (PD-L1), promote B cell differentiation into Breg cells, and stimulate naïve CD4+ T cells to differentiate into Treg cell subtypes. A study by Li et al. showed that the percentages of Breg cells and PD-1+ Breg cells were the highest among TNBC patients with different breast cancer subtypes, indicating that the prognosis of TNBC was worse than that of the other breast cancer subtypes (36).
2.2.3 NK cells with memory-like functions are expected to become a powerful weapon against anti-tumor immunity
As the first line of defense against tumors, NK cells are roughly divided into two types according to the expression of surface proteins, CD56highCD16dim and CD56dimCD16high. NK cells exhibit two different roles, in which CD56dimCD16high NK cells play a cytotoxic role, and release perforin and granzyme B after binding to target cells to mediate apoptosis, while CD56highCD16dim NK cells release IFN-γ, tumor necrosis factor, granulocyte-macrophage colony-stimulating factor, and other cytokines to induce other immune cells to attack the target tumor cells. NK cells exert different immune functions as tumor growth progresses. However, long-term exposure to the TME also leads to the inhibition of certain NK cell functions, ultimately promoting the immune escape of tumors (37). Recent studies have found that Socs3highCD11b-CD27- immature NK cells, which exist only in TNBC, can activate Wnt signaling to achieve tumorigenic effects (38). By targeting PD-L1 with high-affinity NK cells, which is artificially engineered, derived from NK-92, high concentrations of granzyme and perforin granules were released while preserving NK cell receptor expression, which inhibited the growth of MDA-MB-231 cells in TNBC with high expression of PD-L1 (39).
Most studies of tumor immunity focus on adaptive immunity mediated by T or B cells, while only a few studies focus on NK cells in the innate immune system. This is because the turnover rate of NK cells is relatively fast in the human body, and because the memory function of NK cells in the human body remains uncertain. A recent study by Nikzad et al. showed that human liver-resident NK cells displayed long-term antigen-specific memory responses after immunization or viral infection and were not induced by T cells or B cells (40). In addition, the killing ability of memory-like NK cells mediated by IL-12, IL-15, and IL-18 against tumors was significantly enhanced in vivo and in vitro (41). There are various indications that we are expected to differentiate tumor-associated memory NK cells and maximize their strong killing ability and become a powerful weapon for anti-tumor immunity.
3 Adoptive cell therapy brings hope to patients with TNBC
Generally speaking, ACT artificially cultivates lymphocytes that recognize tumors with a high affinity for attacking target tumor cells in vivo. Thus, ACT is highly individualized and is divided into four types according to the different mechanisms of action: ACT with chimeric antigen receptor (CAR)-engineered T (CAR-T) cells, ACT with CAR-engineered NK (CAR-NK) cells, ACT with TILs, and ACT with TCR-engineered T (TCR-T) cells (Figure 2).
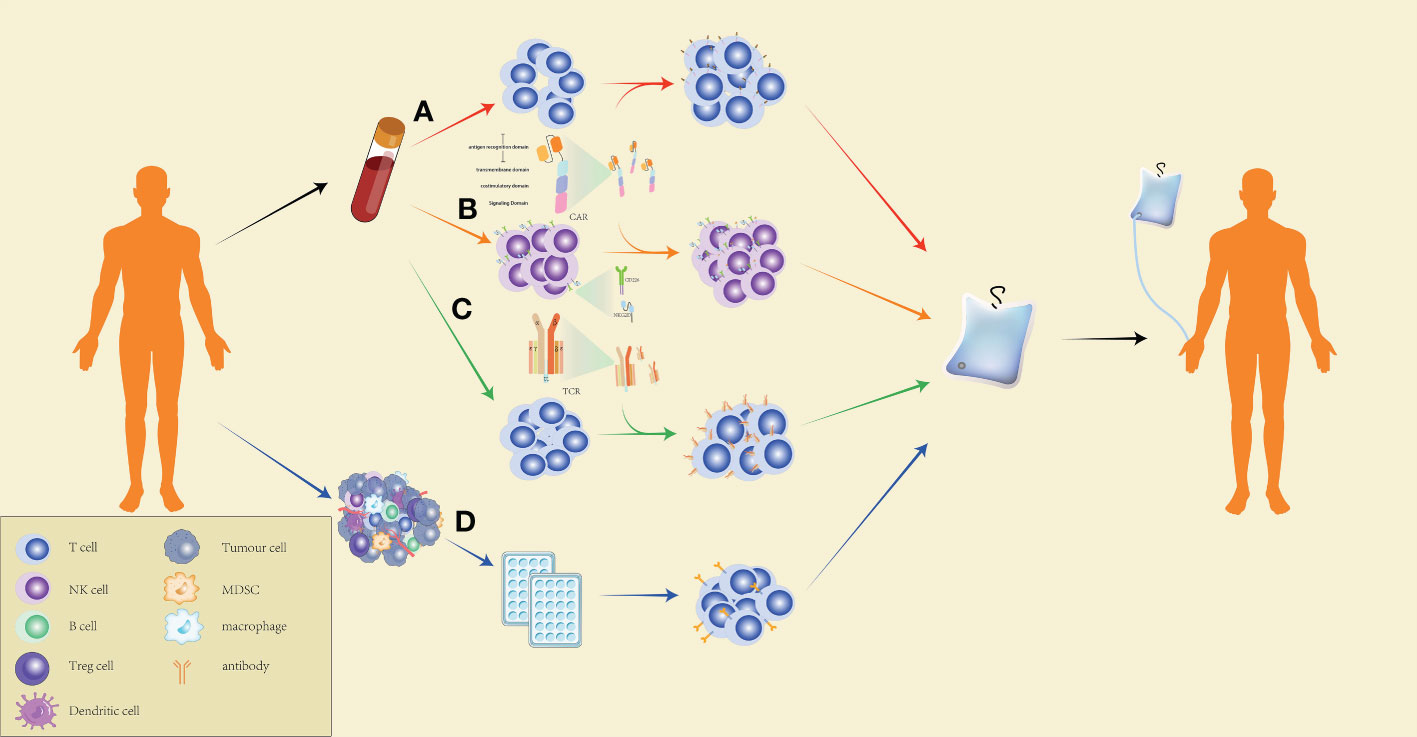
Figure 2 Production process of ACT. (A) Preparation of CAR-T cells for CAR-T-cell therapy: peripheral blood mononuclear cells of the patients are extracted by leukapheresis, washed to remove impurities, and specific T cells are isolated using magnetic-bead separation. After activation, viral or non-viral vectors are used to transduce T cells for CAR expression on the cell surface. The CAR-T cells are then expanded to the target dose, and the effectiveness and safety of the cells are checked. CAR-T cells that pass quality control are reinfused into the patients. (B) The preparation of CAR-NK cells for ACT is similar to the preparation of CAR-T cells. However, CAR-NK cells exert a more powerful and specific tumor targeting capacity by expressing NKG2D, CD266, and other NK cell surface receptors. (C) Preparation of TCR-T cells for ACT: T cells are extracted from the peripheral blood of the patients, followed by delivering specific TCR genes to the T cells through a viral or non-viral delivery system, expanding the TCR-T cells in large quantities, and conducting quality control for the cell culture. The qualified TCR-T cultured cells are reinfused into the patients. (D) Preparation of TILs for ACT: Tumor tissues collected from the patients are cut into many small pieces and cultured in a 24-well plate for two to three weeks with medium containing a high concentration of IL-2, followed by selecting TILs which are capable of secreting IFN-γ and culturing them to a therapeutic dose before infusing the cells into patients.
3.1 ACT with CAR-T cell technology is the most mature
ACT with CAR-T cells (CAR-T-cell therapy) is an emerging cell therapy. Through genetic engineering, CARs are introduced into the surface of T cells extracted from the patient’s blood. The cells are further cultivated to a certain number and subsequently infused back into the patient’s body, where the modified T cells are now equipped to identify camouflaged tumor cells. CARs are composed of antigen recognition domain, transmembrane domain, costimulatory domain, and signaling domain. After continuous improvement, TRUCKs were raised in the fourth generation of CAR-T-cell therapy. By introducing the nuclear factor of the activated T cell (NFAT)-reactive expression cassette, which can activate cytokines such as IL-12, into CAR-T, and then innate immune cells are attracted to eliminate tumors, bringing hope for solid tumor treatment (42, 43). To 2023, eight CAR-T cell products have been approved for marketing (Table 1), indicating that its industrial chain has been approached mature. In addition, CAR-T-cell therapy constantly optimizes production processes and turns its attention to automated production processes that are less costly and less polluting. The successes of CAR-T-cell therapy in clinical aspects also provide new strategies for the production of other ACTs (54, 55).
However, CAR-T-cell therapy also has disadvantages, such as stimulating an excessive release of immune cytokines after cell infusion and causing the accumulation of a large number of activated macrophages, resulting in serious clinical diseases (56). To reduce the occurrence of such events, numerous improved CAR-T-cells have been established. Due to the high expression of epidermal growth factor receptor (EGFR) in TNBC, Lin et al. designed a CAR-T-cell therapy model targeting EGFR and applied it to TNBC cells. The results showed that the granzyme-perforin-poly adenosine diphosphate (ADP) -ribose polymerase (PARP), factor-associated suicide (Fas) -Fas-associated death domain (FADD) -caspase, and IFN-γ signaling pathways were activated in TNBC cells and had a durable and potent inhibitory effect while ensuring safety (57). Similarly, high expression of mesothelin targets were positively correlated with TNBC progression and differentially expressed in normal and tumor cells. Wang et al. used mesothelin as the potential target of a CAR-T-cell therapy model and combined mesothelin with natural killer group 2, member D (NKG2D) protein to construct a dual-target mesothelin in the VHH-NKG2D CAR-T-cell model, characterized by a high activation level and secretion of many cytokines that killed TNBC cells in vitro (58).
There are more complex TMEs in solid tumors, which secrete signaling factors to inhibit the anti-tumor effects of CAR-T cells. Based on this, Stüber et al. blocked TGF-β signaling with SD-208, a TGF-β receptor 1 inhibitor, to effectively prevent the exhaustion of receptor tyrosine kinase-like orphan receptor 1-CAR-T cells in TNBC (59).
3.2 CAR-NK-cell therapy has demonstrated a high level of safety
The construction of CAR-NK cells continues the research and development ideas of CAR-T cells. NK cells are mainly derived from peripheral blood, umbilical cord blood, stem cells, and NK92 cell lines. CAR-NK cell models are obtained by using viral or non-viral vectors to transduce CARs into NK cells (60).
Hu (61) used tissue factor (TF) as a target for CAR-NK-cell therapy and developed TF-CAR-NK cells containing a human FVII light-chain recognition domain, CD28 transmembrane domain, 4-1BB co-stimulatory domain, and CD3ζ signaling region. TF-CAR-NK cells directly killed TNBC cells, and this effect was enhanced when combined with second-generation TF-targeted immunoconjugates. Jan et al. used low-dose chemotherapy drugs to downregulate the expression of DNA-methyltransferase 1 and to stimulate the demethylation of the promoter of the transporter associated with antigen processing 1 gene, thus promoting the expression of human leukocyte antigen G (HLA-G) on the surface of tumor cells, facilitating the killing ability of HLA-G-CAR-NK cells and causing extensive tumor ablation (62).
Due to the short survival time of NK cells in the body and the reduced cytotoxicity, with the same therapeutic effect there would be fewer side effects than encountered during CAR-T-cell therapy, such as cytokine release syndrome. Therefore, ACT with CAR-NK cells has shown good safety and therapy responses with great potential for further development (63).
3.3 The affinity of TCR-T cells is significantly correlated with the major histocompatibility complex
TCR-T cell technology use lentivirus as a carrier to transduce specific TCRs that are then expressed on the surface of T cells of the patients. Although both ACT with TCR-T cells and ACT with CAR-T cells are referred to as TCR redirection technologies, they have significantly different mechanisms of antigen recognition. ACT with TCR-T cells has an absolute dependence on major histocompatibility complex (MHC). CAR-T cells directly bind to tumor surface antigens, while TCR-T cells must be presented and recognized by the α-β chain heterodimer through MHC. This antigen-recognition property means that TCR-T cells are not limited in the selection of target cell antigens and thus are more suitable for the treatment of solid tumors (64).
Nevertheless, TCR-T cells have a low affinity for antigens than do CAR-T cells (65). Improving the affinity of TCR-T cells to antigens has become an urgent need in anticancer therapy. However, the blind pursuit of a high affinity can also bring many harmful side effects in the anti-tumor treatment process. The affinity between the TCRs and peptide-MHC is low, and the combination of the two fails to induce a strong immune response. To solve these problems, Zhao et al. designed a capture project to prolong the interaction duration between T cells and peptide-MHC and to improve the responsiveness of T cells to ligand signals while ensuring a moderate affinity level (66).
The results of a clinical trial of Kimmtrak-related ACT with TCR-T cells showed that the 1-year overall survival rate of patients who received ACT with TCR-T cells increased to 73%, and the relative risk of death was reduced by 49% compared with patients treated with other drugs (67). As a result, Kimmtrak was approved by the U.S. Food and Drug Administration and became the first approved ACT in the world using TCR-T cells for the treatment of refractory solid tumors. In this context, relevant clinical trials for TNBC, such as ACT with TCR-T cells targeting KK-LC-1 (NCT05483491) and MAGE-A1 combined with atezolizumab (NCT04639245), are in the enrollment stage.
3.4 ACT with TILs more accurately identifies targeted tumor cells
ACT with TILs was first demonstrated and applied to the treatment of melanoma by Rosenberg et al., with satisfactory results (68). Unlike CARs, the TILs of this ACT were mainly derived from the tumor tissues of the patients, and TILs capable of secreting IFN-γ in the tissue were extracted and selected for cell culture, which were finally injected into the patients (69). Compared with ACT with CAR-T cells and ACT with TCR-T cells, ACT with TILs has certain advantages and is more suitable for the treatment of solid tumors. T cells that recognize many surface antigens of cancer cells are key for ACT with TILs to attack tumors. In a previous study, 10 out of 13 volunteers who had received other therapeutic regimens but still had metastatic melanoma were infused with TIL products. Among the 10 patients, 50% achieved clinical efficacy, in which 2 patients were in complete remission and 3 patients achieved partial remission. Notably, neoantigen-specific T cell populations, such as RBM12, ENTPD4, VARS, and RAD51AP1-002, emerged and persisted in the peripheral blood of all 10 patients after TIL infusion (70).
The efficacy of ACT with TILs has also been demonstrated in other solid tumors. Standard treatments (e.g., chemotherapy and radiotherapy) are not effective because of the extremely low immunogenicity of metastatic breast cancer (especially TNBC), while ACT with TILs effectively solves this problem. Zacharakis et al. selected 42 patients with metastatic breast cancer who were injected simultaneously with short-term cultured TILs and pembrolizumab. One patient with TNBC had complete regression of liver, lymph node, and mediastinal metastases after 66 months of treatment, and the disease was controlled thereafter by only requiring surgery (71). To date, ACT with TIL therapy for TNBC is now in the stage of recruiting volunteers (NCT04842812 and NCT04111510), fully showing the tremendous potential of this therapy.
4 Summary and outlook
As the subtype of breast cancer with the worst prognosis, TNBC has unsatisfactory curative effects after radiotherapy and chemotherapy, and therefore, new treatment options are needed. As the special soldiers in the TME, TILs play an important role in the process of identifying and killing target tumor cells, which has led to the development of immunotherapies. Among those immunotherapies, ACT is a new star and has achieved good results in recent years. The outstanding achievements of CAR-T-cell therapy in hematological tumors and the promising effects of ACT with TCR-T cells in solid tumors have prompted the search for more suitable targets or combination programs for applying ACT to solid tumors. Although issues remain, such as an unknown therapeutic safety, long training period, and expensive cost, with the continuous improvement and development of the technology, ACT that meets expectations will likely be designed in the future, bringing hope to patients with TNBC.
Author contributions
RL wrote articles and designed diagrams. LC contributed to the revision of the manuscript. Both authors contributed to the article and approved the submitted version.
Funding
Shandong Provincial Natural Science Foundation (ZR2020MH203).
Conflict of interest
The authors declare that the research was conducted in the absence of any commercial or financial relationships that could be construed as a potential conflict of interest.
Publisher’s note
All claims expressed in this article are solely those of the authors and do not necessarily represent those of their affiliated organizations, or those of the publisher, the editors and the reviewers. Any product that may be evaluated in this article, or claim that may be made by its manufacturer, is not guaranteed or endorsed by the publisher.
References
1. Arnold M, Morgan E, Rumgay H, Mafra A, Singh D, Laversanne M, et al. Current and future burden of breast cancer: global statistics for 2020 and 2040. Breast (2022) 66:15–23. doi: 10.1016/j.breast.2022.08.010
2. Goldhirsch A, Wood WC, Coates AS, Gelber RD, Thurlimann B, Senn HJ, et al. Strategies for subtypes–dealing with the diversity of breast cancer: highlights of the st. gallen international expert consensus on the primary therapy of early breast cancer 2011. Ann Oncol (2011) 22(8):1736–47. doi: 10.1093/annonc/mdr304
3. Su GH, Xiao Y, Jiang L, Zheng RC, Wang H, Chen Y, et al. Radiomics features for assessing tumor-infiltrating lymphocytes correlate with molecular traits of triple-negative breast cancer. J Transl Med (2022) 20(1):471. doi: 10.1186/s12967-022-03688-x
4. Bauer KR, Brown M, Cress RD, Parise CA, Caggiano V. Descriptive analysis of estrogen receptor (ER)-negative, progesterone receptor (PR)-negative, and HER2-negative invasive breast cancer, the so-called triple-negative phenotype: a population-based study from the California cancer registry. Cancer (2007) 109(9):1721–8. doi: 10.1002/cncr.22618
5. Dieci MV, Radosevic-Robin N, Fineberg S, van den Eynden G, Ternes N, Penault-Llorca F, et al. Update on tumor-infiltrating lymphocytes (TILs) in breast cancer, including recommendations to assess TILs in residual disease after neoadjuvant therapy and in carcinoma in situ: a report of the international immuno-oncology biomarker working group on breast cancer. Semin Cancer Biol (2018) 52(Pt 2):16–25. doi: 10.1016/j.semcancer.2017.10.003
6. Nelson MA, Ngamcherdtrakul W, Luoh SW, Yantasee W. Prognostic and therapeutic role of tumor-infiltrating lymphocyte subtypes in breast cancer. Cancer Metastasis Rev (2021) 40(2):519–36. doi: 10.1007/s10555-021-09968-0
7. Jorge NAN, Cruz JGV, Pretti MAM, Bonamino MH, Possik PA, Boroni M. Poor clinical outcome in metastatic melanoma is associated with a microRNA-modulated immunosuppressive tumor microenvironment. J Transl Med (2020) 18(1):56. doi: 10.1186/s12967-020-02235-w
8. Chaturvedi P, Gilkes DM, Takano N, Semenza GL. Hypoxia-inducible factor-dependent signaling between triple-negative breast cancer cells and mesenchymal stem cells promotes macrophage recruitment. Proc Natl Acad Sci USA (2014) 111(20):E2120–9. doi: 10.1073/pnas.1406655111
9. Danza K, Pilato B, Lacalamita R, Addati T, Giotta F, Bruno A, et al. Angiogenetic axis angiopoietins/Tie2 and VEGF in familial breast cancer. Europ J Hum Genet (2013) 21(8):824–30. doi: 10.1038/ejhg.2012.273
10. Casali BC, Gozzer LT, Baptista MP, Altei WF, Selistre-de-Araújo HS. The effects of αvβ3 integrin blockage in breast tumor and endothelial cells under hypoxia in vitro. Int J Mol Sci (2022) 23(3):1745. doi: 10.3390/ijms23031745
11. Rahma OE, Hodi FS. The intersection between tumor angiogenesis and immune suppression. Clin Cancer Res (2019) 25(18):5449–57. doi: 10.1158/1078-0432.CCR-18-1543
12. Cai B, Ma P, Ding P, Sun DW, Bu Q, Zhang J. Composition and plasticity of triple-negative breast carcinoma-infiltrating regulatory T cells. APMIS (2020) 128(3):260–9. doi: 10.1111/apm.13022
13. Ahn SG, Jeong J, Hong S, Jung WH. Current issues and clinical evidence in tumor-infiltrating lymphocytes in breast cancer. J Pathol Transl Med (2015) 49(5):355–63. doi: 10.4132/jptm.2015.07.29
14. Mattiuz R, Brousse C, Ambrosini M, Cancel JC, Bessou G, Mussard J, et al. Type 1 conventional dendritic cells and interferons are required for spontaneous CD4(+) and CD8(+) T-cell protective responses to breast cancer. Clin Transl Immunol (2021) 10(7):e1305. doi: 10.1002/cti2.1305
15. Juliá EP, Mordoh J, Levy EM. Cetuximab and IL-15 promote NK and dendritic cell activation In vitro in triple negative breast cancer. Cells (2020) 9(7):1573. doi: 10.3390/cells9071573
16. Böttcher JP, Bonavita E, Chakravarty P, Blees H, Cabeza-Cabrerizo M, Sammicheli S, et al. NK cells stimulate recruitment of cDC1 into the tumor microenvironment promoting cancer immune control. Cell (2018) 172(5):1022–37.e14. doi: 10.1016/j.cell.2018.01.004
17. Basu A, Ramamoorthi G, Albert G, Gallen C, Beyer A, Snyder C, et al. Differentiation and regulation of T(H) cells: a balancing act for cancer immunotherapy. Front Immunol (2021) 12:669474. doi: 10.3389/fimmu.2021.669474
18. Harrington LE, Hatton RD, Mangan PR, Turner H, Murphy TL, Murphy KM, et al. Interleukin 17-producing CD4+ effector T cells develop via a lineage distinct from the T helper type 1 and 2 lineages. Nat Immunol (2005) 6(11):1123–32. doi: 10.1038/ni1254
19. Volpe E, Servant N, Zollinger R, Bogiatzi SI, Hupe P, Barillot E, et al. A critical function for transforming growth factor-beta, interleukin 23 and proinflammatory cytokines in driving and modulating human T(H)-17 responses. Nat Immunol (2008) 9(6):650–7. doi: 10.1038/ni.1613
20. Martin-Orozco N, Muranski P, Chung Y, Yang XO, Yamazaki T, Lu S, et al. T Helper 17 cells promote cytotoxic T cell activation in tumor immunity. Immunity (2009) 31(5):787–98. doi: 10.1016/j.immuni.2009.09.014
21. Faucheux L, Grandclaudon M, Perrot-Dockès M, Sirven P, Berger F, Hamy AS, et al. A multivariate Th17 metagene for prognostic stratification in T cell non-inflamed triple negative breast cancer. #N/A (2019) 8(9):e1624130. doi: 10.1080/2162402x.2019.1624130
22. Ma K, Yang L, Shen R, Kong B, Chen W, Liang J, et al. Th17 cells regulate the production of CXCL1 in breast cancer. Int Immunopharmacol (2018) 56:320–9. doi: 10.1016/j.intimp.2018.01.026
23. Niogret J, Berger H, Rebe C, Mary R, Ballot E, Truntzer C, et al. Follicular helper-T cells restore CD8(+)-dependent antitumor immunity and anti-PD-L1/PD-1 efficacy. J Immunother Cancer (2021) 9(6):e002157. doi: 10.1136/jitc-2020-002157
24. Noël G, Fontsa ML, Garaud S, De Silva P, de Wind A, Van den Eynden GG, et al. Functional Th1-oriented T follicular helper cells that infiltrate human breast cancer promote effective adaptive immunity. J Clin Invest (2021) 131(19):e139905. doi: 10.1172/jci139905
25. Bai F, Zhang P, Fu Y, Chen H, Zhang M, Huang Q, et al. Targeting ANXA1 abrogates treg-mediated immune suppression in triple-negative breast cancer. J Immunother Cancer (2020) 8(1):e000169. doi: 10.1136/jitc-2019-000169
26. Qiao J, Liu Z, Dong C, Luan Y, Zhang A, Moore C, et al. Targeting tumors with IL-10 prevents dendritic cell-mediated CD8(+) T cell apoptosis. Cancer Cell (2019) 35(6):901–15.e4. doi: 10.1016/j.ccell.2019.05.005
27. Oshi M, Asaoka M, Tokumaru Y, Yan L, Matsuyama R, Ishikawa T, et al. CD8 T cell score as a prognostic biomarker for triple negative breast cancer. Int J Mol Sci (2020) 21(18):6968. doi: 10.3390/ijms21186968
28. Ganesan AP, Clarke J, Wood O, Garrido-Martin EM, Chee SJ, Mellows T, et al. Tissue-resident memory features are linked to the magnitude of cytotoxic T cell responses in human lung cancer. Nat Immunol (2017) 18(8):940–50. doi: 10.1038/ni.3775
29. Shields BD, Koss B, Taylor EM, Storey AJ, West KL, Byrum SD, et al. Loss of e-cadherin inhibits CD103 antitumor activity and reduces checkpoint blockade responsiveness in melanoma. Cancer Res (2019) 79(6):1113–23. doi: 10.1158/0008-5472.Can-18-1722
30. Park MH, Kwon SY, Choi JE, Gong G, Bae YK. Intratumoral CD103-positive tumour-infiltrating lymphocytes are associated with favourable prognosis in patients with triple-negative breast cancer. Histopathology (2020) 77(4):560–9. doi: 10.1111/his.14126
31. Kashiwagi S, Yashiro M, Takashima T, Nomura S, Noda S, Kawajiri H, et al. Significance of e-cadherin expression in triple-negative breast cancer. Br J Cancer (2010) 103(2):249–55. doi: 10.1038/sj.bjc.6605735
32. Gu-Trantien C, Migliori E, Buisseret L, de Wind A, Brohée S, Garaud S, et al. CXCL13-producing TFH cells link immune suppression and adaptive memory in human breast cancer. JCI Insight (2017) 2(11):e91487. doi: 10.1172/jci.insight.91487
33. Garaud S, Buisseret L, Solinas C, Gu-Trantien C, de Wind A, Van den Eynden G, et al. Tumor infiltrating b-cells signal functional humoral immune responses in breast cancer. JCI Insight (2019) 5(18):e129641. doi: 10.1172/jci.insight.129641
34. Harris RJ, Cheung A, Ng JCF, Laddach R, Chenoweth AM, Crescioli S, et al. Tumor-infiltrating b lymphocyte profiling identifies IgG-biased, clonally expanded prognostic phenotypes in triple-negative breast cancer. Cancer Res (2021) 81(16):4290–304. doi: 10.1158/0008-5472.Can-20-3773
35. Vito A, Salem O, El-Sayes N, MacFawn IP, Portillo AL, Milne K, et al. Immune checkpoint blockade in triple negative breast cancer influenced by b cells through myeloid-derived suppressor cells. Commun Biol (2021) 4(1):859. doi: 10.1038/s42003-021-02375-9
36. Li X, Du H, Zhan S, Liu W, Wang Z, Lan J, et al. The interaction between the soluble programmed death ligand-1 (sPD-L1) and PD-1(+) regulator b cells mediates immunosuppression in triple-negative breast cancer. Front Immunol (2022) 13:830606. doi: 10.3389/fimmu.2022.830606
37. Wagner JA, Rosario M, Romee R, Berrien-Elliott MM, Schneider SE, Leong JW, et al. CD56bright NK cells exhibit potent antitumor responses following IL-15 priming. J Clin Invest (2017) 127(11):4042–58. doi: 10.1172/jci90387
38. Thacker G, Henry S, Nandi A, Debnath R, Singh S, Nayak A, et al. Immature natural killer cells promote progression of triple-negative breast cancer. Sci Transl Med (2023) 15(686):eabl4414. doi: 10.1126/scitranslmed.abl4414
39. Fabian KP, Padget MR, Donahue RN, Solocinski K, Robbins Y, Allen CT, et al. PD-L1 targeting high-affinity NK (t-haNK) cells induce direct antitumor effects and target suppressive MDSC populations. J Immunother Cancer (2020) 8(1):e000450. doi: 10.1136/jitc-2019-000450
40. Nikzad R, Angelo LS, Aviles-Padilla K, Le DT, Singh VK, Bimler L, et al. Human natural killer cells mediate adaptive immunity to viral antigens. Sci Immunol (2019) 4(35):eaat8116. doi: 10.1126/sciimmunol.aat8116
41. Marin ND, Krasnick BA, Becker-Hapak M, Conant L, Goedegebuure SP, Berrien-Elliott MM, et al. Memory-like differentiation enhances NK cell responses to melanoma. Clin Cancer Res (2021) 27(17):4859–69. doi: 10.1158/1078-0432.Ccr-21-0851
42. Asmamaw Dejenie T, Tiruneh GMM, Dessie Terefe G, Tadele Admasu F, Wale Tesega W, Chekol Abebe E. Current updates on generations, approvals, and clinical trials of CAR T-cell therapy. Hum Vaccin Immunother (2022) 18(6):2114254. doi: 10.1080/21645515.2022.2114254
43. Chmielewski M, Abken H. TRUCKs: the fourth generation of CARs. Expert Opin Biol Ther (2015) 15(8):1145–54. doi: 10.1517/14712598.2015.1046430
44. Maude SL, Laetsch TW, Buechner J, Rives S, Boyer M, Bittencourt H, et al. Tisagenlecleucel in children and young adults with b-cell lymphoblastic leukemia. N Engl J Med (2018) 378(5):439–48. doi: 10.1056/NEJMoa1709866
45. Schuster SJ, Tam CS, Borchmann P, Worel N, McGuirk JP, Holte H, et al. Long-term clinical outcomes of tisagenlecleucel in patients with relapsed or refractory aggressive b-cell lymphomas (JULIET): a multicentre, open-label, single-arm, phase 2 study. Lancet Oncol (2021) 22(10):1403–15. doi: 10.1016/s1470-2045(21)00375-2
46. Fowler NH, Dickinson M, Dreyling M, Martinez-Lopez J, Kolstad A, Butler J, et al. Tisagenlecleucel in adult relapsed or refractory follicular lymphoma: the phase 2 ELARA trial. Nat Med (2022) 28(2):325–32. doi: 10.1038/s41591-021-01622-0
47. Locke FL, Ghobadi A, Jacobson CA, Miklos DB, Lekakis LJ, Oluwole OO, et al. Long-term safety and activity of axicabtagene ciloleucel in refractory large b-cell lymphoma (ZUMA-1): a single-arm, multicentre, phase 1-2 trial. Lancet Oncol (2019) 20(1):31–42. doi: 10.1016/s1470-2045(18)30864-7
48. Wang M, Munoz J, Goy A, Locke FL, Jacobson CA, Hill BT, et al. KTE-X19 CAR T-cell therapy in relapsed or refractory mantle-cell lymphoma. New Engl J Med (2020) 382(14):1331–42. doi: 10.1056/NEJMoa1914347
49. Abramson JS, Palomba ML, Gordon LI, Lunning MA, Wang M, Arnason J, et al. Lisocabtagene maraleucel for patients with relapsed or refractory large b-cell lymphomas (TRANSCEND NHL 001): a multicentre seamless design study. Lancet (2020) 396(10254):839–52. doi: 10.1016/s0140-6736(20)31366-0
50. Munshi NC, Anderson LD Jr., Shah N, Madduri D, Berdeja J, Lonial S, et al. Idecabtagene vicleucel in relapsed and refractory multiple myeloma. New Engl J Med (2021) 384(8):705–16. doi: 10.1056/NEJMoa2024850
51. Berdeja JG, Madduri D, Usmani SZ, Jakubowiak A, Agha M, Cohen AD, et al. Ciltacabtagene autoleucel, a b-cell maturation antigen-directed chimeric antigen receptor T-cell therapy in patients with relapsed or refractory multiple myeloma (CARTITUDE-1): a phase 1b/2 open-label study. Lancet (2021) 398(10297):314–24. doi: 10.1016/s0140-6736(21)00933-8
52. Ying Z, Yang H, Guo Y, Li W, Zou D, Zhou D, et al. Relmacabtagene autoleucel (relma-cel) CD19 CAR-T therapy for adults with heavily pretreated relapsed/refractory large b-cell lymphoma in China. Cancer Med (2021) 10(3):999–1011. doi: 10.1002/cam4.3686
53. Fosunkite. instructions for axicabtagene ciloleucel injection (2021). Available at: https://www.fosunkitebio.com/images/SMS.pdf (Accessed January 27, 2023).
54. Hiltensperger M, Krackhardt AM. Current and future concepts for the generation and application of genetically engineered CAR-T and TCR-T cells. Front Immunol (2023) 14:1121030. doi: 10.3389/fimmu.2023.1121030
55. Lock D, Monjezi R, Brandes C, Bates S, Lennartz S, Teppert K, et al. Automated, scaled, transposon-based production of CAR T cells. J Immunother Cancer (2022) 10(9):e005189. doi: 10.1136/jitc-2022-005189
56. Yan Z, Zhang H, Cao J, Zhang C, Liu H, Huang H, et al. Characteristics and risk factors of cytokine release syndrome in chimeric antigen receptor T cell treatment. Front Immunol (2021) 12:611366. doi: 10.3389/fimmu.2021.611366
57. Xia L, Zheng ZZ, Liu JY, Chen YJ, Ding JC, Xia NS, et al. EGFR-targeted CAR-T cells are potent and specific in suppressing triple-negative breast cancer both in vitro and in vivo. Clin Transl Immunol (2020) 9(5):e01135. doi: 10.1002/cti2.1135
58. Qi W. Mesoclin and NKG2D dual-target enhanced CAR-T for triple-negative breast cancer of research. University of Chinese Academy of Sciences (Shenzhen Institute of Advanced Technology, Chinese Academy of Sciences) (2022). doi: 10.27822/d.cnki.gszxj.2022.000090
59. Stüber T, Monjezi R, Wallstabe L, Kühnemundt J, Nietzer SL, Dandekar G, et al. Inhibition of TGF-β-receptor signaling augments the antitumor function of ROR1-specific CAR T-cells against triple-negative breast cancer. J Immunother Cancer (2020) 8(1):e000676. doi: 10.1136/jitc-2020-000676
60. Bahmanyar M, Vakil MK, Al-Awsi GRL, Kouhpayeh SA, Mansoori Y, Mansoori B, et al. Anticancer traits of chimeric antigen receptors (CARs)-natural killer (NK) cells as novel approaches for melanoma treatment. BMC Cancer (2022) 22(1):1220. doi: 10.1186/s12885-022-10320-0
61. Hu Z. Tissue factor as a new target for CAR-NK cell immunotherapy of triple-negative breast cancer. Sci Rep (2020) 10(1):2815. doi: 10.1038/s41598-020-59736-3
62. Jan CI, Huang SW, Canoll P, Bruce JN, Lin YC, Pan CM, et al. Targeting human leukocyte antigen G with chimeric antigen receptors of natural killer cells convert immunosuppression to ablate solid tumors. J Immunother Cancer (2021) 9(10):e003050. doi: 10.1136/jitc-2021-003050
63. Li Y, Hermanson DL, Moriarity BS, Kaufman DS. Human iPSC-derived natural killer cells engineered with chimeric antigen receptors enhance anti-tumor activity. Cell Stem Cell (2018) 23(2):181–92.e5. doi: 10.1016/j.stem.2018.06.002
64. Wang H, Song X, Shen L, Wang X, Xu C. Exploiting T cell signaling to optimize engineered T cell therapies. Trends Cancer (2022) 8(2):123–34. doi: 10.1016/j.trecan.2021.10.007
65. Wachsmann TLA, Wouters AK, Remst DFG, Hagedoorn RS, Meeuwsen MH, van Diest E, et al. Comparing CAR and TCR engineered T cell performance as a function of tumor cell exposure. Oncoimmunology (2022) 11(1):2033528. doi: 10.1080/2162402x.2022.2033528
66. Zhao X, Kolawole EM, Chan W, Feng Y, Yang X, Gee MH, et al. Tuning T cell receptor sensitivity through catch bond engineering. Science (2022) 376(6589):eabl5282. doi: 10.1126/science.abl5282
67. Nathan P, Hassel JC, Rutkowski P, Baurain JF, Butler MO, Schlaak M, et al. Overall survival benefit with tebentafusp in metastatic uveal melanoma. N Engl J Med (2021) 385(13):1196–206. doi: 10.1056/NEJMoa2103485
68. Rosenberg SA, Packard BS, Aebersold PM, Solomon D, Topalian SL, Toy ST, et al. Use of tumor-infiltrating lymphocytes and interleukin-2 in the immunotherapy of patients with metastatic melanoma. a preliminary report. N Engl J Med (1988) 319(25):1676–80. doi: 10.1056/nejm198812223192527
69. Hopewell EL, Cox C, Pilon-Thomas S, Kelley LL. Tumor-infiltrating lymphocytes: streamlining a complex manufacturing process. Cytotherapy (2019) 21(3):307–14. doi: 10.1016/j.jcyt.2018.11.004
70. van den Berg JH, Heemskerk B, van Rooij N, Gomez-Eerland R, Michels S, van Zon M, et al. Tumor infiltrating lymphocytes (TIL) therapy in metastatic melanoma: boosting of neoantigen-specific T cell reactivity and long-term follow-up. J Immunother Cancer (2020) 8(2):e000848. doi: 10.1136/jitc-2020-000848
Keywords: triple-negative breast cancer (TNBC), tumor-infiltrating lymphocytes (TILs), adoptive cell therapy (ACT), breast cancer, solid tumor
Citation: Li R and Cao L (2023) The role of tumor-infiltrating lymphocytes in triple-negative breast cancer and the research progress of adoptive cell therapy. Front. Immunol. 14:1194020. doi: 10.3389/fimmu.2023.1194020
Received: 26 March 2023; Accepted: 09 May 2023;
Published: 18 May 2023.
Edited by:
Mohammad Hojjat-Farsangi, Karolinska Institutet (KI), SwedenReviewed by:
Hualin Chen, Peking Union Medical College Hospital (CAMS), ChinaCihui Yan, Tianjin Medical University Cancer Institute and Hospital, China
Weiqing Jing, Northwestern University, United States
Copyright © 2023 Li and Cao. This is an open-access article distributed under the terms of the Creative Commons Attribution License (CC BY). The use, distribution or reproduction in other forums is permitted, provided the original author(s) and the copyright owner(s) are credited and that the original publication in this journal is cited, in accordance with accepted academic practice. No use, distribution or reproduction is permitted which does not comply with these terms.
*Correspondence: Lili Cao, Y2xsQHNkdS5lZHUuY24=