- 1Fujian Provincial Key Laboratory of Agroecological Processing and Safety Monitoring (School of Life Sciences, Fujian Agriculture and Forestry University), Fuzhou, China
- 2Key Laboratory of Crop Ecology and Molecular Physiology (Fujian Agriculture and Forestry University), Fujian Province University, Fuzhou, China
- 3State Key Laboratory of Microbial Technology, Shandong University, Qingdao, China
- 4Centre for Research, Centre for Materials Engineering and Regenerative Medicine, Bharath Institute of Higher Education and Research, Selaiyur, Chennai, Tamil Nadu, India
Bacterial AraC is a transcription factor family that initiates transcription by recruiting RNA polymerase to the promoter and directly regulating various bacterial phenotypes. It also directly regulates various bacterial phenotypes. However, how this transcription factor regulates bacterial virulence and affects host immunity is still largely unknown. In this study, deleting the orf02889 (AraC-like transcription factor) gene in virulent Aeromonas hydrophila LP-2 affected several important phenotypes, such as increasing biofilm formation and siderophore production abilities. Moreover, Δorf02889 also significantly decreased the virulence of A. hydrophila and has promising attenuated vaccine potential. To better understand the effects of orf02889 on biological functions, a data independent acquisition (DIA)-based quantitative proteomics method was performed to compare the differentially expressed proteins between Δorf02889 and the wild-type strain in extracellular fractions. The following bioinformatics analysis suggested that ORF02889 may regulate various metabolic pathways, such as quorum sensing and ATP binding cassette (ABC) transporter metabolism. Moreover, 10 selected genes from the top 10 decreasing abundances in proteomics data were deleted, and their virulence to zebrafish was evaluated, respectively. The results showed that ΔcorC, Δorf00906, and Δorf04042 significantly reduced bacterial virulence. Finally, the following chromatin immunoprecipitation and polymerase chain reaction (ChIP-PCR) assay validated that the promoter of corC was directly regulated by ORF02889. Overall, these results provide insight into the biological function of ORF02889 and demonstrate its inherent regulatory mechanism for the virulence of A. hydrophila.
Introduction
AraC is a regulator of the arabinose operon, belonging to the AraC-type transcriptional superfamily of proteins (1). It usually binds to specific promoter sites as monomers or dimers to control the expression of multiple genes and plays a variety of important roles in various bacterial physiological functions, such as carbon metabolism, stress response, and pathogenicity (2, 3). For example, the deletion of the AraC-like transcriptional regulator yqhc gene in Erwinia amylovora EaUMG3 significantly reduced the expression of several virulence factors (VFs) such as siderophore production and amylovoran production-related genes (4). For another example, the Staphylococcus aureus Rbf regulator that belongs to the AraC family negatively regulates the icaADBC inhibitor SarR and indirectly inhibits the ica operon activator SarX to form RBf-Sarr-Sarx, thus promoting its expression to control the biofilm phenotype of S. aureus (5). All available evidence suggests that AraC is crucial in regulating basic biological processes, whereas the instinct regulatory mechanism is still largely unknown.
Aeromonas hydrophila is a pathogen that can be transmitted from humans to animals and fish, and is one of the main pathogenic bacteria responsible for bacterial fish diseases. It can cause various illnesses in fish, including gastroenteritis, septicemia, and necrotizing fasciitis (6, 7). The VFs of A. hydrophila can be divided into two categories: structural molecules such as lipopolysaccharides (LPS), outer membrane proteins (OMPs), S-layer proteins, and flagella, and virulence-related extracellular secretions, many of which are proteases that are biosynthesized in the cytoplasm and then secreted outside of the cell to infect hosts and cause diseases (8, 9). Previous research has shown that ExsA, an AraC-like protein of the A. hydrophila AH3 strain, is a negative regulator of the lateral flagella system and plays an important role in the regulation of the type III secretion system (T3SS) (10). However, few studies have analyzed how the transcription factor AraC regulates the pathogenicity of bacteria in the extracellular fraction level, especially for A. hydrophila.
ORF02889 protein is an AraC-like transcriptional regulator in the virulent A. hydrophila LP-2 strain that was isolated from diseased silver carp, and its genome was re-sequenced in our library. In this study, the orf02889 gene was deleted, its rescued strains in A. hydrophila LP-2 were constructed, and their physiological functions were evaluated. The results showed that the Δorf02889 gene significantly affected multiple biological functions, especially for reducing the bacterial virulence in zebrafish. To better understand the regulatory mechanism of ORF02889 on bacterial virulence, a quantitative proteomics method was used to compare extracellular differentially expressed proteins (DEPs) between Δorf02889 and wild-type (WT) strains. Subsequent bioinformatics analysis revealed that ORF02889 might regulate various intracellular metabolic pathways. Moreover, the following bacterial experiments also displayed that several selected proteins from proteomics results significantly affected pathogenic virulence. Finally, ChIP-PCR assay showed that several virulence-related genes corC were directly regulated by ORF02889. In general, these results provide insight into the biological functions of orf02889 and demonstrate its regulatory mechanism for A. hydrophila virulence.
Materials and methods
Bacterial strains and plasmids
In this study, we used the virulent A. hydrophila LP-2 strain, which was isolated from a diseased silver carp, and whose genome was re-sequenced in our laboratory. We also used Escherichia coli DH5α (GenBank ID: GCA_000982435.1), MC1061 (GenBank ID: LN877770.1), and S17 strains (GenBank ID: GCA_000340255.1), as well as the pRE112 suicide (GenBank ID: JN788332.1) and pBBR1-MCS1 plasmids (GenBank ID: MH238456.1). All of these strains and plasmids were maintained in our laboratory. All strains were cultured using Luria-Bertani (LB) medium (composition: 10 g/L peptone, 10 g/L sodium chloride, and 5 g/L yeast powder). Chrome azurol S (CAS) agar medium was prepared as previously described (11).
Construction of the gene deletion mutant and rescued strain
The gene deletion strain was constructed based on the principle of homologous recombination as previously described (12). Briefly, the recombinant plasmid containing the target gene’s upper and downstream homologous arms was constructed on the pRE112 suicide plasmid using overlapping PCR technology and introduced into E. coli S17. Then, the recombinant suicide plasmid was introduced into the WT strain of A. hydrophila through bacterial conjugation. The sucrose lethal gene was used to screen mutant strains. Finally, the deletion strain was verified by PCR and DNA sequencing validation.
The gene-rescued strain was constructed on the broad-host vector pBBR1-MCS1 as previously described (13). After restriction enzyme digestion, the recovery fragment was ligated to the rescue pBBR1-MCS1 plasmid, and then transformed into E. coli DH5α competent cells. The single clones were selected from the LB medium plate for bacterial liquid PCR verification, and then the correct recombinant plasmid was extracted and transferred into the previous deletion strain.
Swimming and swarming ability assays
The swimming and swarming agar plates were prepared using LB medium containing 0.3% and 0.6% agar powder, respectively (14). A single clone of bacterial strains was stabbed and point inoculated on the swimming and swarming agar plates and incubated at 30°C for 6 h and 12 h, respectively. Finally, the swimming and swarming circles’ diameters were measured for three independent repeats.
Siderophore production assay
Overnight-cultured bacteria were transferred to fresh LB medium at 1% (v/v) and cultured to OD600 (optical density at 600 nm) = 1.0 at 200 rpm, 30°C. Then, 5 μl of bacteria solution was spotted onto the CAS agar plate and incubated at 30°C for 16 h. The diameter of the orange halo was observed and measured and was independently repeated three times.
Detection of biofilm formation by crystal violet staining
Bacterial solutions cultured to OD600 = 1.0 were transferred at 1:20 (v/v) to 96-well microplates with 200 μl in each well. Each sample was technically replicated across four wells per plate and then incubated at 30°C for 24 h (15). After removing the culture medium, 200 μl of 0.1% (w/v) crystal violet solution was added to each well. After 20 min of staining, the solution was removed. Each well was added with 200 μl of 95% ethanol solution and incubated at room temperature for 10 min. The absorbance values under OD 595-nm wavelength were detected by SpectraMax® i3 (Molecular Devices Co., Ltd., Shanghai, China). Each experiment was performed at least three times as biological duplicates.
The bacterial challenge of mutant strains
The bacterial challenge of mutant strains in zebrafish was performed as previously described (16, 17). Briefly, bacterial strains were cultured overnight and then transferred to fresh LB medium at 1:100 (v/v) for further culture to reach OD600 = 1.0. Then, the bacterial cells from 1 ml of medium were collected by centrifugation, washed with phosphoric acid buffer salt solution (PBS) buffer solution, and then re-suspended in 1 ml of PBS buffer. The bacterial suspension was diluted to a concentration of 1.2 × 104 CFU/ml. Zebrafish fasting for 1 day was randomly divided into 30 fish in each group, and then were intraperitonially injected with the above bacteria concentration at the dose of 10 μl per tail with the injection of the same dose of PBS as the negative control. Challenged fish were monitored for 7 days, and the number of deaths was recorded every day.
qPCR assay
The quantitative polymerase chain reaction (qPCR) method was used to detect the mRNA expression level of related genes as previously described (18). Briefly, the livers of zebrafish infected with A. hydrophila were extracted after intraperitoneal injection of bacterial strains for 3 days. Total RNA from zebrafish liver was extracted by the Trizol-chloroform (TaKaRa Inc., Japan) method. The total RNA was then reverse transcribed into cDNA following the instructions in the Prime Script RT Kit (TransGen Biotech Co., Ltd., Beijing, China). Finally, a real-time PCR detection system (Bio-Rad Inc., CA, USA) was used to detect the expression of related genes by real-time qPCR, and 16S rRNA was used as the internal reference gene. The primers used are shown in Supplementary Table S1.
Extraction of extracellular proteins
Bacterial strains were transferred to 50 ml of LB medium and cultured at 30°C with shaking at 200 rpm until OD600 reached 1.0. The supernatant was collected by centrifugation at 10,000 rpm for 10 min at 4°C, and then passed through a 0.22-μm membrane filter. The proteins in the obtained filtrate were precipitated by adding 8% precooled tricarboxylic acid (TCA) (Aladdin Co., Ltd., Shanghai, China) and leaving it overnight at 4°C. After centrifugation at 10,000 rpm for 30 min at 4°C, the proteins were precipitated again from the supernatant by adding 1 ml of precooled acetone and washed three times with acetone. The pellets were dissolved in lysis buffer [6 M urea, 2 M thiourea, and 0.1 M Tris–HCl (pH 8.5) solution with protease inhibitor]. The protein concentration was determined by the Bradford method and stored at −80°C until use.
Liquid chromatograph mass spectrometer (LC-MS/MS)
Approximately 50 μg of each group of protein samples were taken into an ultrafiltration tube, reduced with 50 mM DTT (dithiothreitol) at 56°C for 40 min. Then, 25 mM IAA (iodoacetamide) was used for alkylation in the dark for 30 min. Then, the protein samples were transferred to an ultrafiltration tube (10 kDa, Millipore, Boston, Massachusetts, USA) and digested by trypsin by using the FASP (filter-aided proteome preparation) method at a ratio of 1:20 (w/v) at 37°C overnight for enzymatic hydrolysis (19). The treated peptide sample was desalted with a C18 column (Waters, Inc., Milford, MA, USA). The desalted polypeptide samples were separated at a flow rate of 60 nl/min by using an EASY-nano-LC chromatography system (Thermo Scientific Inc., Waltham, MA, USA) (20). The separation gradient was as follows: 0–18 min, liquid B (acetonitrile containing 0.1% formic acid) rose linearly from 6% to 12%; 18–77 min, liquid B increased linearly from 12% to 20%; 77–109 min, liquid B rose linearly from 20% to 32%, then rose to 90% within 1 min and remained constant for 120 min. Peptides were analyzed using an Orbitrap Fusion Lumos Tribrid mass spectrometer (Thermo Scientific Inc., Waltham, MA, USA). Specific parameters were set as follows: ion source spray voltage, 2.1 kV; cycle time, 3 s; ion transfer tube temperature, 300°C; scanning range, 300–1,400 m/z; resolution, 120 K; AGC target, 5e5; maximum intensity threshold (IT), 50 ms. The ion trap method was used by MS/MS, the AGC target is 5e3, the maximum IT is 35 ms, and the collision energy is 30%. Data-dependent acquisition (DDA) data from mass spectrum acquisition were imported into Spectronout Pulsar X (biognosys, Schlieren, Switzerland) to establish a DDA database. The database was built using the default optimal parameter “BGS factory setting”. All raw files were searched by Maxquant software (version 1.6.17.0) for database search and data analysis. The mass spectrometry proteomics raw datasets have been deposited to the ProteomeXchange Consortium (http://proteomecentral.proteomexchange.org) via the iProX partner repository with the dataset identifier PXD040986.
Bioinformatics analysis
Based on the mass spectrum data, proteins with a p-value less than 0.05 and a difference multiple greater than 2.0 or less than 0.5 were set as differential proteins. GO (Gene Ontology) and KEGG (Kyoto Encyclopedia of Genes and Genomes) enrichment analysis of differential proteins were performed using STRING online software (https://cn.string-db.org/) and visualized by Cytoscape software version 3.9.1 or GOplot and R software. The predicted VFs of DEPs were analyzed with the virulence factors database (VFDB) and visualized with a circular heat map using the TBtool software (21, 22).
ChIP-PCR assay
The interaction between transcription factor orf02889 and candidate genes was verified by ChIP-PCR as previously described (23). Briefly, Δorf02889-C and Δorf02889-EV strains were transferred to 50 ml of LB medium, incubated at 200 rpm overnight, and then centrifuged at 5,000 g at 4°C for 10 min. The obtained sample was washed twice with precooled PBS. Formaldehyde solution with a final concentration of 1% was added to the sample for cross-linking for 5 min. Then, glycine with a final concentration of 0.176 M was added to stop the cross-linking. After centrifugation, an appropriate amount of PBS was added for ultrasonic crushing. Then, the supernatant was centrifuged, and the appropriate amount of nickel magnetic beads was added for incubation at 4°C for 2 h. The samples were washed three times with a washing buffer, and then 400 μl of 500 mM imidazole was added to wash and collect. The eluent was added with 40 μl of 10% SDS and 2 μl of 10 mg/ml protease K and incubated at 37°C overnight. The next day, the DNA was extracted using phenol-chloroform. Finally, the purified DNA was verified by PCR using the primers listed in Supplementary Table S1.
Results
Δorf02889 decreases bacterial motility in A. hydrophila
To better characterize the physiological phenotypes of ORF02889, the orf02889 gene was deleted in A. hydrophila LP-2, and its rescued strain (Δorf02889-C) with Δorf02889 carrying empty vector (Δorf02889-EV) as negative control were constructed in this study (Supplementary Figure S1). The swimming and swarming abilities of mutants were assessed on 0.3% and 0.6% semi-solid LB agar plates, respectively, as the locomotion ability of bacteria is closely related to the pathogenicity of pathogenic bacteria. As shown in Figure 1A, when compared to the A. hydrophila WT strain, the swimming and swarming circles in the Δorf02889 strain were significantly reduced. In contrast, these phenotypes were recovered in the rescued strain (Figure 1B), indicating that the deletion of the orf02889 gene affects the locomotion abilities of A. hydrophila.
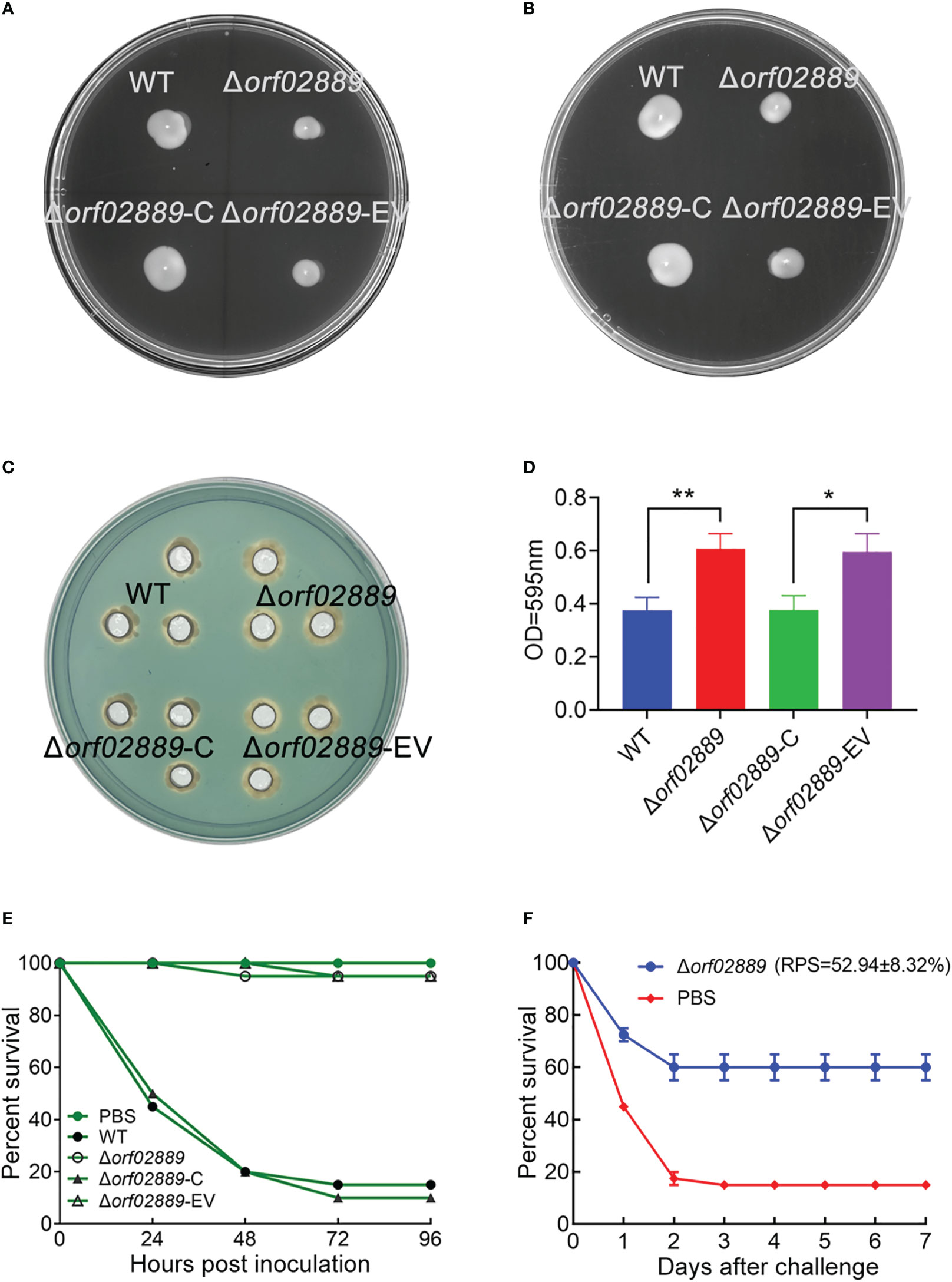
Figure 1 Determination of Δorf02889 physiological phenotypes. (A–D) The swimming and swarming motility, siderophore production (CAS assay), and biofilm formation of WT and mutant strains. (E) Survival rates of zebrafish after challenged with Δorf02889. (F) Zebrafish inoculated with Δorf02889 and PBS were challenged with A. hydrophila LP-2 for 7 days. Data represent the mean ± SEM of n ≥ 2 independent replicates. *P < 0.05; **P < 0.01.
Δorf02889 increases siderophore biosynthesis in A. hydrophila
The production of siderophore in A. hydrophila was observed using CAS dye solution. As shown in Figure 1C, the Δorf02889 strain produced significantly larger bright orange halos than the LP-2 WT strain, and phenotypic recovery was also observed in rescued strains. This indicates that orf02889 may negatively regulate the production of siderophore in A. hydrophila.
Effect of Δorf02889 on the biofilm formation of A. hydrophila
The biofilm formation ability of the Δorf02889 strain was detected by the crystal violet staining method. As shown in Figure 1D, the deletion of the orf02889 gene significantly increased the biofilm formation ability of A. hydrophila when compared to the WT strain. Meanwhile, the biofilm formation ability of the rescued strain was recovered, indicating the important regulatory role of this AraC family regulator on the bacterial biofilm formation in A. hydrophila.
Δorf02889 reduces A. hydrophila virulence in zebrafish
The effects of Δorf02889 on bacterial virulence and immune system-related genes in zebrafish were evaluated in this study. The survival rate of zebrafish on A. hydrophila LP-2 infection was 15%, whereas the deletion of orf02889 caused the survival rate to increase to 95%, indicating that it is a strongly attenuated strain when compared to the results of the PBS injection group as negative control. The behaviors of its rescued strain Δorf02889-C and control strain Δorf02889-EV were similar to those of the WT and Δorf02889 strains (Figure 1E). Moreover, the overall survival rate of orf02889 was 60%, and the relative percent survival (RPS) was 52.94 ± 8.32% (Figure 1F). It suggested that orf02889 can be employed as a candidate protein for developing the A. hydrophila vaccine.
Δorf02889 stimulates host immunity
The effect of the Δorf02889 strain on the zebrafish immune system was also determined. Zebrafish were intraperitoneally injected with WT and Δorf02889 mutant strains, respectively, and the transcription levels of genes responsible for pro- and anti-inflammatory cytokines, chemokines, anti-bacterial peptides/enzymes, and antioxidants in zebrafish liver were detected by qPCR after 3 days of injection. As shown in Table 1, the deletion of orf02889 significantly evoked the transcription levels of several pro- and anti-inflammatory cytokines (il-1β, il-6, and il-10), chemokines (ccl-34α.4, and cxcl-8α), anti-bacterial peptides/enzymes (defβl-1 and lyz-c), and antioxidants (cat, sod-1, gstp-1, and txndr-1), downregulated the mRNA levels of tnf-α and tnf-β1, but did not affect the expression of cxcl-18b and prdx-4 in the host. These results indicate that the A. hydrophila Δorf02889 attenuated strain could stimulate many host immune-related genes.
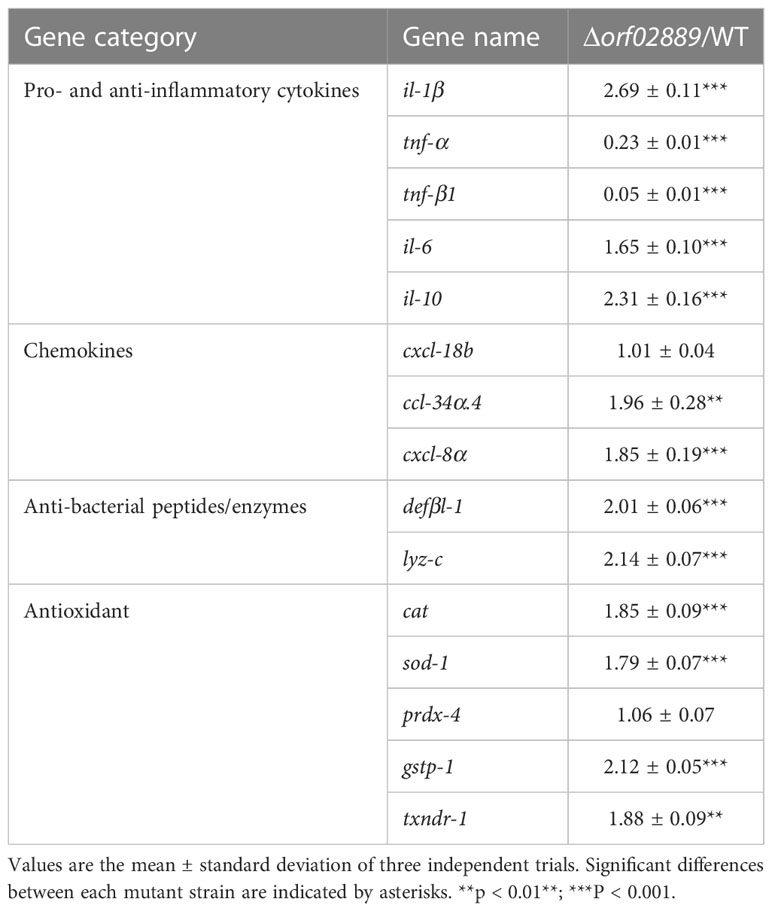
Table 1 The zebrafish immune response after vaccination with Δorf02889 and WT strain by qPCR, respectively.
The differential expressions between LP-2 and the Δorf02889 strain in extracellular protein samples
Extracellular proteins can directly interact with the host immune system, inducing the body to produce immune protection due to their high immunogenicity. In this study, extracellular protein samples were extracted from A. hydrophila LP-2 WT and Δorf02889 mutant strains, with three biological repeats for each strain (Figure 2A). The protein samples in each group were quantitated by LC-MS/MS to compare the protein expression differences between WT and Δorf02889. As shown in Figure 2B, correlation analysis for each biological replicate found that all the correlation coefficients R were greater than 0.75, indicating that the proteomic data were accurate and reasonable and could be used for further analysis. The further volcano map showed that a total of 2,886 extracellular proteins were identified with 256 DEPs, namely, 112 increasing and 144 decreasing abundance proteins (Figure 2C).
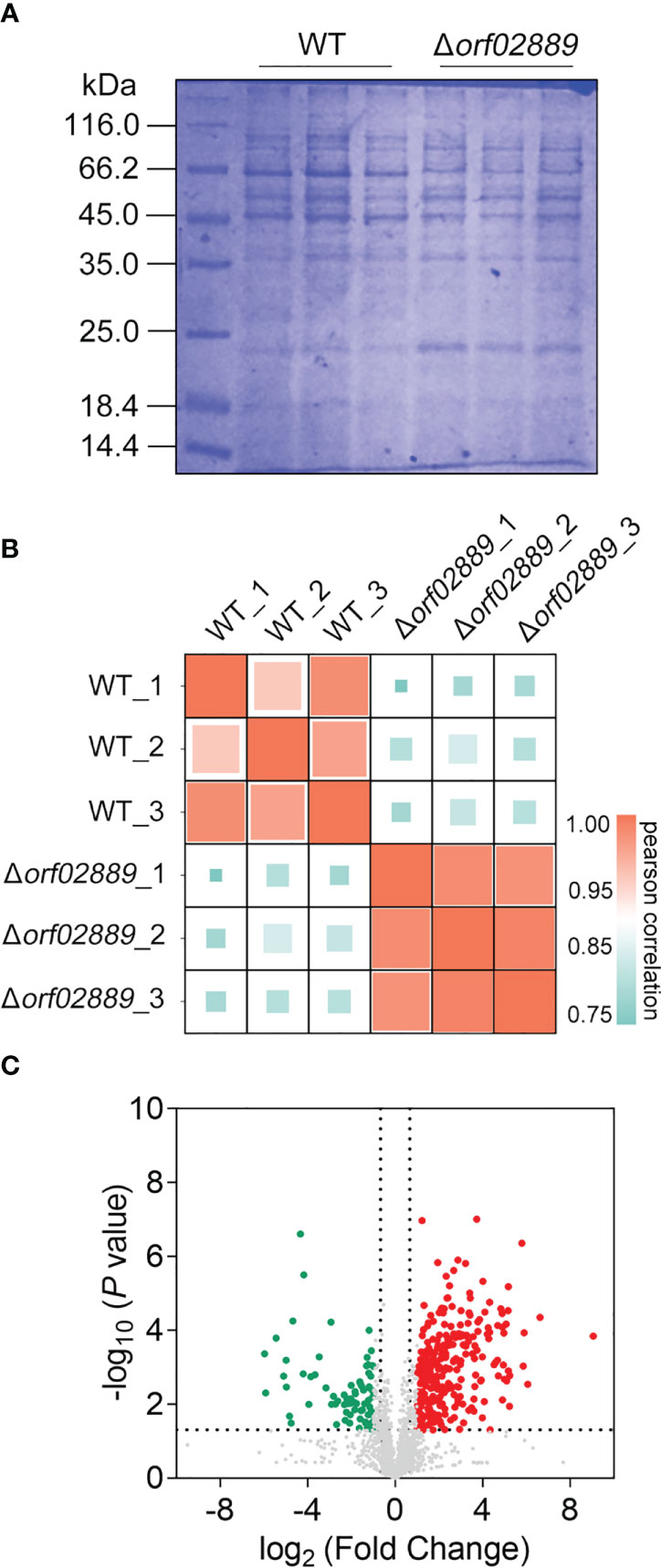
Figure 2 Quantitative proteomic data analysis. (A) Coomassie bright blue SDS-PAGE of extracellular proteins extracted from A. hydrophila LP-2 and Δorf02889; (B) Pearson correlation assay of protein intensities for three biological replicates from each group. (C) Volcano plot showing the abundance ratios of significant DEPs. Red points represent differentially expressed upregulated proteins, green points represent downregulated proteins, and gray points indicate non-differentially expressed proteins.
Bioinformatics analysis
The STRING online software was used for GO enrichment analysis and Cytoscape software for visualization of all the DEPs. The results showed that nine pathways of altered extracellular proteins were enriched in the cell components (CC) category, namely, cell outer membrane, external encapsulating structure, pore complex, cell envelope, envelope, membrane protein complex, OM-bounded periplasmic space, periplasmic space, and extracellular region. In the molecular function (MF) category, two GO terms of altered proteins, namely, porin activity and tetrapyrrole binding, were enriched (Figure 3).
KEGG enrichment and virulence factor analysis
The STRING online website was used to enrich and analyze the KEGG metabolic pathways of DEPs. It was found that three KEGG pathways in A. hydrophila were enriched after orf02889 deletion, namely, ABC transporters, cationic antimicrobial peptide (CAMP) resistance, and quorum sensing (Figure 4). Among them, at least 39 DEPs were involved in ABC transporters, which is the most enriched KEGG pathway in this study.
The amino acid sequences of DEPs were further submitted to search against the VFDB to identify the well-known VFs in this study. As shown in Figure 5, at least 20 VFs were identified, namely, 14 increased proteins and 6 decreased proteins, after orf02889 was deleted. Interestingly, the protein abundances of these VFs were fluctuant, indicating that the virulence behavior should be a comprehensive complicated regulatory mechanism.
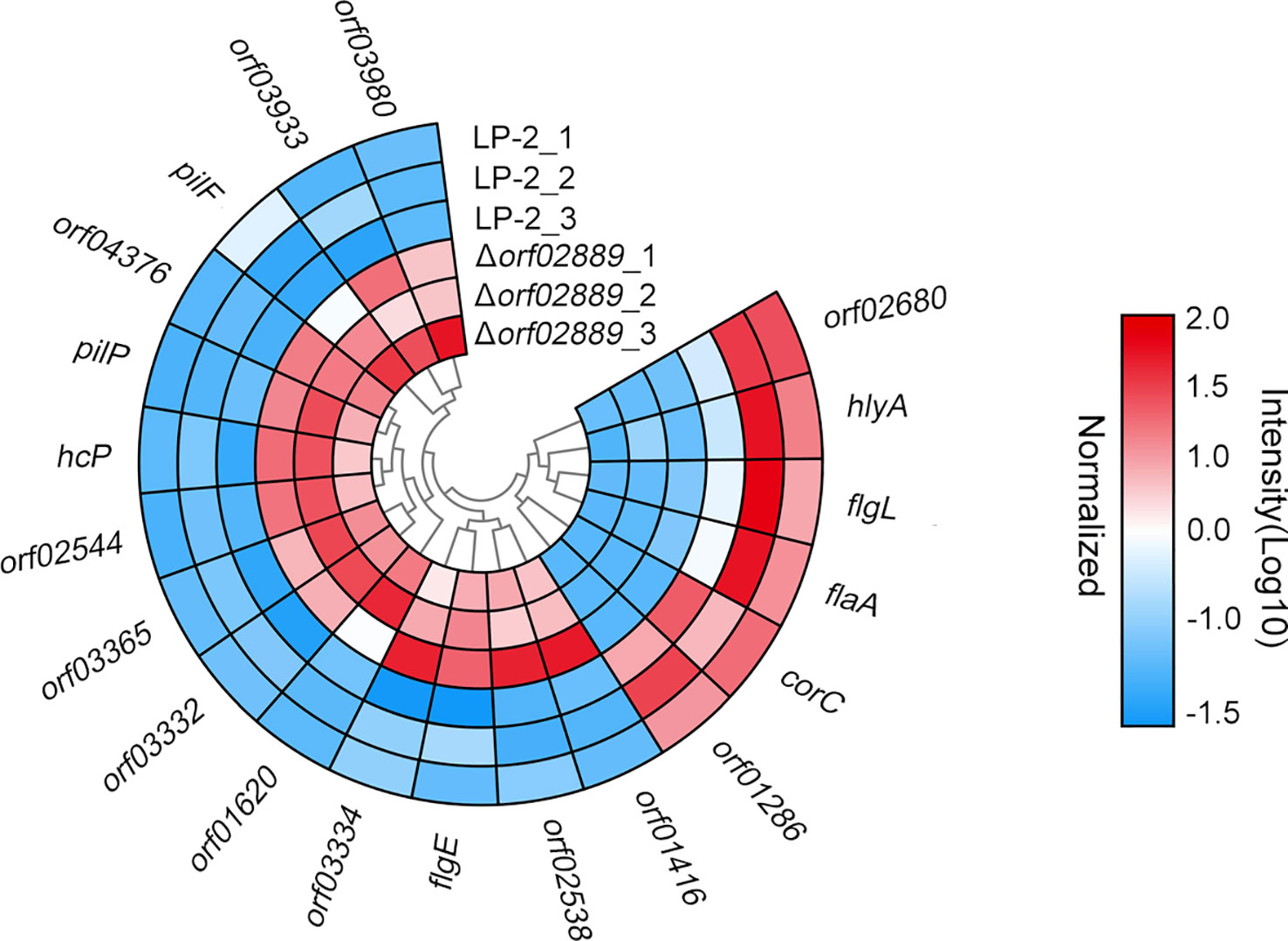
Figure 5 Circular heat map displays the expression of predicted virulence factors in the current proteomics result. The heat map displays the normalized MS intensity of altered proteins in three biological repeats for each group (log10 scale).
The effects of selected genes regulated by ORF02889 on bacterial virulence
To better understand the role of the transcription regulator orf02889 in the virulence of A. hydrophila LP-2, we first selected 10 proteins [ORF00560(CorC), ORF00906, ORF01286, ORF02228(CcoP), ORF03485, ORF03490, ORF03989, ORF04007, ORF04042, and ORF04270] with the highest downregulated abundances from the proteomic data for gene knockout and further functional verification. These protein-coded genes were successfully knocked out in A. hydrophila LP-2 (Supplementary Figure S2), and their effects on the virulence of A. hydrophila were evaluated by intraperitoneal injection of mutants into zebrafish. The results showed that the cumulative mortality rate of ΔcorC, Δorf04042, and Δorf00906 fell by 70%, 36.7%, and 30% when compared to A. hydrophila LP-2 with a mortality rate of 93.33%, respectively, indicating a significant reduction in virulence of these mutants. Besides these, the mortality rate of the remaining gene deletion strains was similar to or a little lower than that of A. hydrophila LP-2 (Figure 6). These results suggest that CorC, ORF00906, and ORF04042 may be novel A. hydrophila virulence effectors and may play an important role during bacterial invasions.
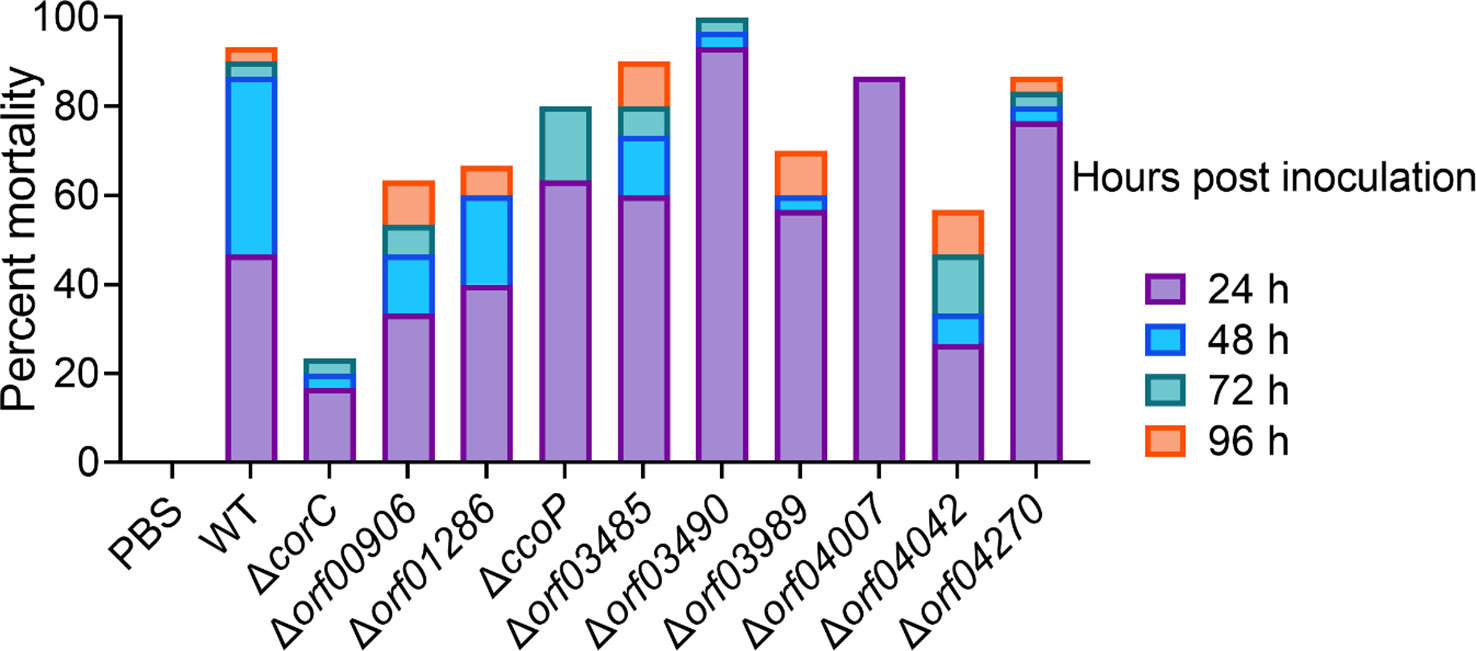
Figure 6 Cumulative zebrafish mortality in 96 h after a challenge by associated gene deletion strains.
orf02889 directly regulates the transcription of corC
The ChIP-PCR method was used to detect the binding ability of four candidate genes with orf02889; the virulence of these related mutants was attenuated in zebrafish infection. They are magnesium and cobalt efflux protein CorC (ORF 00560), acetyl-coenzyme A synthetase (ORF00906), diguanylate cyclase/phosphodiesterase (ORF01286), and ferrichrome-iron receptor (ORF04042). As shown in Figure 7, all candidate genes (corC, orf00906, orf01286, and orf4042) and their related predicted promoter regions (PcorC, Porf00906, Porf01286, and Porf4042) can be detected in the Δorf02889-C input sample (positive control). All immunoprecipitated (IP) DNA fragments of candidate genes cannot be amplified in the Δorf02889-C sample as expected using the designed primer pair for target gene amplification. Meanwhile, Porf00906, Porf01286, and Porf4042 were not amplified, but PcorC can be detected in IP samples. These results indicate that orf02889 may affect the A. hydrophila virulence by directly regulating the expression of the corC gene.
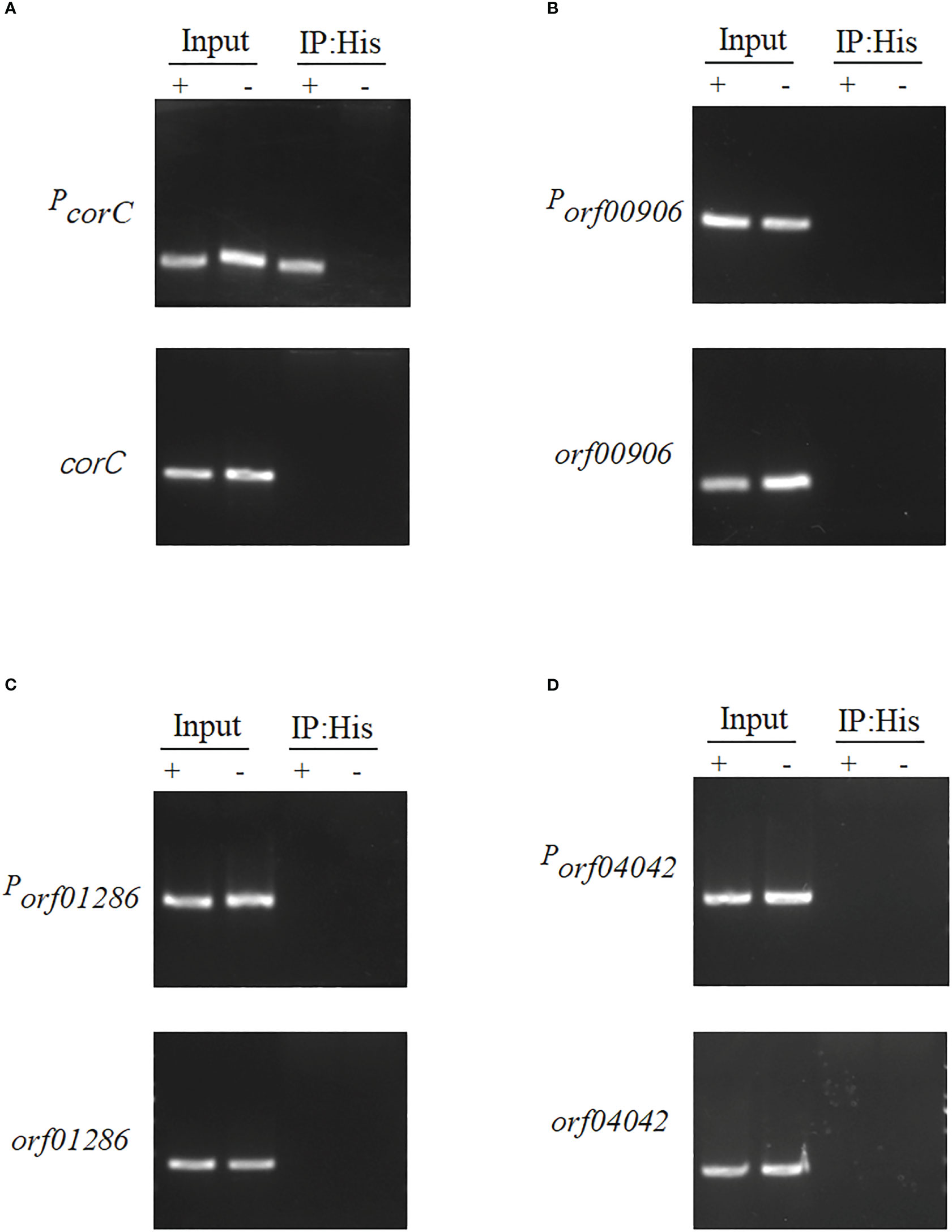
Figure 7 The binding assay of orf02889 with the promoters of four candidate genes. (A–E) ChIP-PCR analysis of the binding of orf02889 and the promoter fragments of corC, orf0906, orf01286, and orf04042. The A. hydrophila chromatin was immunoprecipitated without or with anti-His antibody (Input or IP: His) for PCR with the target primer pairs, respectively. The promoter fragments of candidate genes are marked as Pgene name; the orf02889 complementary strain was marked as “+”; Δorf02889 carrying empty vector was marked as “-”.
Discussion
Numerous studies have demonstrated that AraC-like transcription factors play a crucial role in regulating various physiological functions of bacteria. They are mainly involved in the metabolism of carbon sources, responses to environmental stress, and pathogenesis. For example, the S. aureus Rsp protein, which belongs to the AraC/XylS transcription regulatory factor (AFTRs) family, can promote its own expression by directly binding to the promoter regions of hla and psm (pore toxin) to positively regulate S. aureus virulence determinants, indicating its important role in the regulation of bacterial virulence (24).
ORF02889 is a member of the bacterial transcription factor AraC family in virulent A. hydrophila LP-2. Our current physiological phenotype assays of orf02889 mutants showed that this transcription factor played important roles in various bacterial biological functions. For example, it reduced motility ability while increasing biofilm formation and siderophore biosynthesis capabilities. Subsequent quantitative proteomics studies have shown that Δorf02889 strains affect the ABC transport system. Reduced motility in bacteria can increase the interaction time between the bacterium and its substratum, thereby promoting biofilm formation (25). However, ferriferous carriers are necessary not only for surface transport, but also for bacterial biofilm formation. In addition, Carol et al. found that the surface motility of bacteria is prevented under high-iron conditions, but biofilm formation is promoted by inducing the formation of more structured and thicker biofilms than those formed under low iron levels. It has also been shown that Sinorhizobium meliloti has control mechanisms that inversely regulate swarming and biofilm formation, and facilitate bacterial invasion of the host (26).
Moreover, the deletion of orf02889 significantly decreased the virulence of A. hydrophila LP-2 in zebrafish, and it may be a promising attenuated vaccine against A. hydrophila infection. This is because the injection of the mutant strain can provoke the host immune response, and the RPS of the immunized zebrafish was higher than 50% when challenged with the A. hydrophila LP-2 strain.
To better understand how ORF02889 regulates bacterial virulence, we first used quantitative MS to characterize the effect of Δorf02889 on the exoproteome of A. hydrophila cells. The results showed that 380 extracellular proteins were differentially expressed in Δorf02889 strains, among which 295 proteins were upregulated and 85 were downregulated. Subsequent bioinformatics analysis indicated that orf02889 might be involved in the regulation of the quorum sensing and ABC transporters’ mechanisms. Previous research has reported that AraC family transcription factors such as Pseudomonas aeruginosa VqsM and Yersinia pestis YbtA directly regulate quorum sensing and ABC transport systems, which is consistent with our current results (27, 28).
In this study, at least 20 altered proteins are predicted as VFs based on the homology comparison in the VFDB. For example, Vibrio cholerae hemolysin HlyA can influence the invasion ability of the bacteria by regulating the hemolytic activity of the bacteria, thus participating in the pathogenic process of the bacteria (29). In this study, the abundance of HlyA was decreased in Δorf02889 strains, which indicates that ORF02889 may affect A. hydrophila virulence by regulating hlyA. However, of these altered proteins, only six predicted VFs were downregulated in Δorf02889 strains, suggesting that these proteins may be more important in contributing to ORF02889-mediated A. hydrophila virulence. To further investigate the virulence regulatory mechanism of this AraC-like transcription factor, we selected 10 altered proteins that have top decreasing abundances in our exoproteomic data for further functional validation, including the above two predicted VFs, ORF01286 and CorC. ORF01286 is a diguanylate cyclase/phosphodiesterase containing a GGDEF domain that synthesizes bacterial second messenger bis-(3′–5′)-cyclic dimeric guanosine monophosphate (c-di-GMP) controls and plays an important role in the regulation of bacterial flagella movement and virulence (30, 31); CorC is a magnesium and cobalt efflux protein that plays an important role in Mg2+ and Co2+ homeostasis in various bacterial species (32). Although previous research documented that CorA but not CorC in Salmonella enterica serovar Typhimurium is important for the invasion of Caco-2 epithelial cells, the deletion mutation of Francisella tularensis FTL_0883, a homolog of CorC, attenuated F. tularensis subsp. holarctica live vaccine strain (LVS) replication within macrophages in vitro, suggesting that the role of CorC on virulence has species specificity (33, 34).
Moreover, we deleted the selected 10 genes, respectively, and evaluated their effects on bacterial virulence. Our results displayed that at least three mutants (ΔcorC, Δorf00906, and Δorf04042) significantly reduced bacterial virulence during the zebrafish infection when compared with the WT strain, especially for ΔcorC, which increased the zebrafish survival rate to 76.67%. The following ChIP-PCR analysis further confirmed that orf02889 can directly bind to the promoter of corC, but orf00906, orf01286, and orf04042 did not. Therefore, our results indicate that the AraC-like transcription factor orf02889 may regulate the expression of several VFs, such as CorC, and play an important role in A. hydrophila virulence.
Data availability statement
The datasets presented in this study can be found in online repositories. The names of the repository/repositories and accession number(s) can be found at http://www.proteomexchange.org/,PXD040986.
Ethics statement
The animal study was reviewed and approved by the Fujian Agriculture and Forestry University Animal Care and Use Committee (Certification Number: CNFJAC0027).
Author contributions
XL conceived and supervised the project. LZ constructed strains, performed the experimental work, and drafted the manuscript. LS and ML contributed to quantitative proteomics and data analysis. RS and LG improved the manuscript. All authors contributed to the article and approved the submitted version.
Funding
This work was sponsored by grants from NSFC projects (Nos. 32171435 and 316701293) and Key Projects of the Natural Science Foundation of Fujian Province (No. 2020J02023).
Acknowledgments
We acknowledge the support of the program for Innovative Research Team in Fujian Agricultural and Forestry University (No.712018009) and the Fujian–Taiwan Joint Innovative Center for Germplasm Resources and Cultivation of Crop (FJ 2011 Program, No.2015-75, China). We also thank the help from Central Laboratory, Fujian Medical University Union Hospital, China.
Conflict of interest
The authors declare that the research was conducted in the absence of any commercial or financial relationships that could be construed as a potential conflict of interest.
Publisher’s note
All claims expressed in this article are solely those of the authors and do not necessarily represent those of their affiliated organizations, or those of the publisher, the editors and the reviewers. Any product that may be evaluated in this article, or claim that may be made by its manufacturer, is not guaranteed or endorsed by the publisher.
Supplementary material
The Supplementary Material for this article can be found online at: https://www.frontiersin.org/articles/10.3389/fimmu.2023.1191209/full#supplementary-material
References
1. Schleif R. AraC protein, regulation of the l-arabinose operon in Escherichia coli, and the light switch mechanism of AraC action. FEMS Microbiol Rev (2010) 34(5):779–96. doi: 10.1111/j.1574-6976.2010.00226.x
2. Martin RG, Rosner JL. The AraC transcriptional activators. Curr Opini Microbiol (2001) 4(2):132–7. doi: 10.1016/S1369-5274(00)00178-8
3. Kotecka K, Kawalek A, Kobylecki K, Bartosik AA. The AraC-type transcriptional regulator GliR (Pa3027) activates genes of glycerolipid metabolism in Pseudomonas aeruginosa. Int J Mol Sci (2021) 22(10):5066. doi: 10.3390/ijms22105066
4. Sahebi M, Tarighi S, Taheri P. The AraC-like transcriptional regulator YqhC is involved in pathogenicity of Erwinia amylovora. J Appl Microbiol (2022) 132(2):1319–29. doi: 10.1111/jam.15286
5. Li TM, He L, Song Y, Villaruz AE, Joo HS, Liu Q, et al. AraC-type regulator rsp adapts Staphylococcus aureus gene expression to acute infection. Infect Immun (2016) 84(3):723–34. doi: 10.1128/IAI.01088-15
7. Golas I, Szmyt M, Glinska-Lewczuk K. Water as a source of indoor air contamination with potentially pathogenic Aeromonas hydrophila in aquaculture. Int J Env Res Pub He (2022) 19(4):2379. doi: 10.3390/ijerph19042379
8. Barger PC, Liles MR, Beck BH, Newton JC. Differential production and secretion of potentially toxigenic extracellular proteins from hypervirulent Aeromonas hydrophila under biofilm and planktonic culture. BMC Microbiol (2021) 21(1):8. doi: 10.1186/s12866-020-02065-2
9. Beaz-Hidalgo R, Figueras MJ. Aeromonas spp. whole genomes and virulence factors implicated in fish disease. J Fish Dis (2013) 36(4):371–88. doi: 10.1111/jfd.12025
10. Zhao YH, Shaw JG. Cross-talk between the Aeromonas hydrophila type III secretion system and lateral flagella system. Front Microbiol (2016) 7:1434. doi: 10.3389/fmicb.2016.01434
11. Schwyn B, Neilands JB. Universal chemical assay for the detection and determination of siderophores. Anal Biochem (1987) 160(1):47–56. doi: 10.1016/0003-2697(87)90612-9
12. Liu ZQ, Zhang LS, Song QL, Song HH, Xu YQ, Lu JL, et al. Quantitative proteomics reveal the inherent antibiotic resistance mechanism against norfloxacin resistance in Aeromonas hydrophila. J Proteome Res (2023) 22(4):1193–200. doi: 10.1021/acs.jproteome.2c00663
13. Zhang LS, Yao ZJ, Tang HM, Song QL, Song HH, Yao JD, et al. The lysine acetylation modification in the porin Aha1 of Aeromonas hydrophila regulates the uptake of multidrug antibiotics. Mol Cell Proteomics (2022) 21(9):100248. doi: 10.1016/j.mcpro.2022.100248
14. Song QL, Zhang LS, Wang GB, Song HH, Zhang SZY, Yao JD, et al. Proteomics analysis reveals that CirA in Aeromonas hydrophila is involved in nutrient uptake. Environ Microbiol (2023) 2023:1–13. doi: 10.1111/1462-2920.16336
15. Zhao XL, Chen ZG, Yang TC, Jiang M, Wang J, Cheng ZX, et al. Glutamine promotes antibiotic uptake to kill multidrug-resistant uropathogenic bacteria. Sci Transl Med (2021) 13:eabj0716. doi: 10.1126/scitranslmed.abj0716
16. Wang YQ, Wang XY, Ali F, Li ZQ, Fu YY, Yang XJ, et al. Comparative extracellular proteomics of Aeromonas hydrophila reveals iron-regulated secreted proteins as potential vaccine candidates. Front Immunol (2019) 10:256. doi: 10.3389/fimmu.2019.00256
17. Zhang S, Wang J, Jiang M, Xu D, Peng B, Peng XX, et al. Reduced redox-dependent mechanism and glucose-mediated reversal in gentamicin-resistant Vibrio alginolyticus. Environ Microbiol (2019) 21(12):4724–39. doi: 10.1111/1462-2920.14811
18. Jiang M, Su YB, Ye JZ, Li H, Kuang SF, Wu JH. Ampicillin-controlled glucose metabolism manipulates the transition from tolerance to resistance in bacteria. Sci Adv (2023) 9(10):eade8582. doi: 10.1126/sciadv.ade8582
19. Tanca A, Biosa G, Pagnozzi D, Addis MF, Uzzau S. Comparison of detergent-based sample preparation workflows for LTQ-orbitrap analysis of the Escherichia coli proteome. Proteomics (2013) 13(17):2597–607. doi: 10.1002/pmic.201200478
20. Wang GB, Wang YQ, Zhang LS, Cai QL, Lin YX, Lin L, et al. Proteomics analysis reveals the effect of Aeromonas hydrophila sirtuin CobB on biological functions. J Proteomics (2020) 225:103848. doi: 10.1016/j.jprot.2020.103848
21. Liu B, Zheng DD, Zhou SY, Chen LH, Yang J. VFDB 2022: a general classification scheme for bacterial virulence factors. Nucleic Acids Res (2022) 50(D1):D912–7. doi: 10.1093/nar/gkab1107
22. Chen CJ, Chen H, He YH, Xia R. TBtools, a toolkit for biologists integrating various biological data handling tools with a user-friendly interface. BioRxiv (2018). doi: 10.1101/289660
23. Fu YY, Zhang LS, Wang GB, Lin YX, Ramanathan S, Yang GD, et al. The lysr-type transcriptional regulator YeeY plays important roles in the regulatory of furazolidone resistance in Aeromonas hydrophila. Front Microbiol (2020) 11:577376. doi: 10.3389/fmicb.2020.577376
24. Liu BH, Sun BL. Rsp promotes the transcription of virulence factors in an agr-independent manner in Staphylococcus aureus. Emerg Microbes Infect (2020) 9(1):796–812. doi: 10.1080/22221751.2020.1752116
25. Caiazza NC, Merritt JH, Brothers KM, O’Toole GA. Inverse regulation of biofilm formation and swarming motility by Pseudomonas aeruginosa PA14. J Bacteriol (2007) 189(9):3603–12. doi: 10.1128/JB.01685-06
26. Amaya-Gómez CV, Hirsch AM, Soto MJ. Biofilm formation assessment in Sinorhizobium meliloti reveals interlinked control with surface motility. BMC Microbiol (2015) 15:1–14. doi: 10.1186/s12866-015-0390-z
27. Liang HH, Deng X, Li XF, Ye Y, Wu M. Molecular mechanisms of master regulator VqsM mediating quorum-sensing and antibiotic resistance in Pseudomonas aeruginosa. Nucleic Acids Res (2014) 42(16):10307–20. doi: 10.1093/nar/gku586
28. Fetherston JD, Bertolino VJ, Perry RD. YbtP and YbtQ: two ABC transporters required for iron uptake in Yersinia pestis. Mol Microbiol (1999) 32(2):289–99. doi: 10.1046/j.1365-2958.1999.01348.x
29. Wang GL, Fan C, Wang H, Jia CY, Li XT, Yang JR, et al. Type VI secretion system-associated FHA domain protein TagH regulates the hemolytic activity and virulence of Vibrio cholerae. Gut Microbes (2022) 14(1):2055440. doi: 10.1080/19490976.2022.2055440
30. Feirer N, Xu J, Allen KD, Koestler BJ, Bruger EL, Waters CM, et al. A pterin-dependent signaling pathway regulates a dual-function diguanylate cyclase-phosphodiesterase controlling surface attachment in Agrobacterium tumefaciens. mBio (2015) 6(4):e00156–15. doi: 10.1128/mBio.00156-15
31. Rick T, Kreiling V, Höing A, Fiedler S, Glatter T, Steinchen W, et al. GGDEF domain as spatial on-switch for a phosphodiesterase by interaction with landmark protein HubP. NPJ Biofilms Microbi (2022) 8(1):35. doi: 10.1038/s41522-022-00297-w
32. Wu YH, Fang C, Zhou P, Wang CS, Xu XW. Complete genome sequence of a heavy metal resistant bacterium Maribacter cobaltidurans B1T, isolated from the deep-sea sediment of the south Atlantic ocean. Mar Genomics (2018) 39:19–21. doi: 10.1016/j.margen.2017.12.005
33. Papp-Wallace KM, Maguire ME. Regulation of CorA Mg2+ channel function affects the virulence of Salmonella enterica serovar typhimurium. J Bacteriol (2008) 190(19):6509–16. doi: 10.1128/JB.00144-08
Keywords: AraC-like transcription factor, quantitative proteomics, Aeromonas hydrophila, virulence, ORF02889
Citation: Zhang L, Sun L, Srinivasan R, Lin M, Gong L and Lin X (2023) Unveiling a Virulence-Regulating Mechanism in Aeromonas hydrophila: a Quantitative Exoproteomic Analysis of an AraC-Like Protein. Front. Immunol. 14:1191209. doi: 10.3389/fimmu.2023.1191209
Received: 21 March 2023; Accepted: 17 April 2023;
Published: 09 May 2023.
Edited by:
Ming Jiang, Guangdong Academy of Agricultural Sciences, ChinaReviewed by:
Selvaraj Anthonymuthu, University of California, Irvine, United StatesRajamanikandan S., Karpagam Academy of Higher Education, India
Copyright © 2023 Zhang, Sun, Srinivasan, Lin, Gong and Lin. This is an open-access article distributed under the terms of the Creative Commons Attribution License (CC BY). The use, distribution or reproduction in other forums is permitted, provided the original author(s) and the copyright owner(s) are credited and that the original publication in this journal is cited, in accordance with accepted academic practice. No use, distribution or reproduction is permitted which does not comply with these terms.
*Correspondence: Xiangmin Lin, xiangmin@fafu.edu.cn