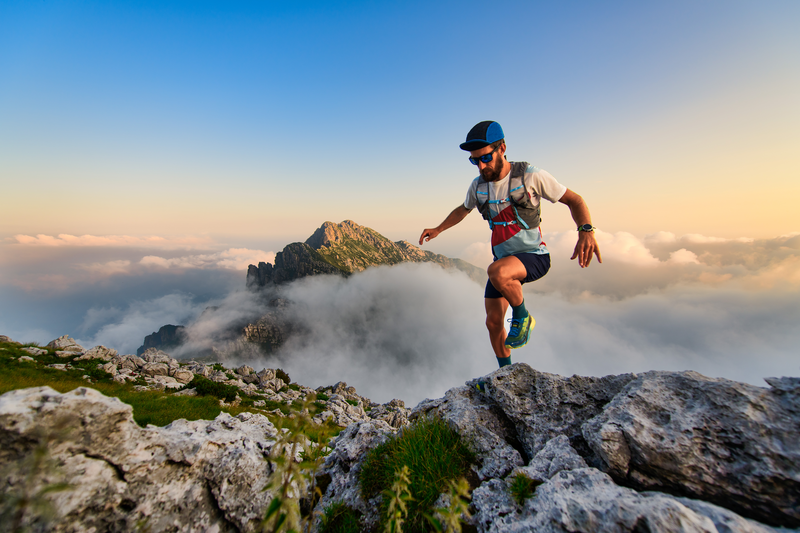
94% of researchers rate our articles as excellent or good
Learn more about the work of our research integrity team to safeguard the quality of each article we publish.
Find out more
REVIEW article
Front. Immunol. , 19 May 2023
Sec. Alloimmunity and Transplantation
Volume 14 - 2023 | https://doi.org/10.3389/fimmu.2023.1179195
Background: Joint allotransplantation (JA) within the field of vascularized composite allotransplantation (VCA) holds great potential for functional and non-prosthetic reconstruction of severely damaged joints. However, clinical use of JA remains limited due to the immune rejection associated with all forms of allotransplantation. In this study, we aim to provide a comprehensive overview of the current state of JA through a systematic review of clinical, animal, and immunological studies on this topic.
Methods: We conducted a systematic literature review in accordance with the PRISMA guidelines to identify relevant articles in PubMed, Cochrane Library, and Web of Science databases. The results were analyzed, and potential future prospects were discussed in detail.
Results: Our review included 14 articles describing relevant developments in JA. Currently, most JA-related research is being performed in small animal models, demonstrating graft survival and functional restoration with short-term immunosuppression. In human patients, only six knee allotransplantations have been performed to date, with all grafts ultimately failing and a maximum graft survival of 56 months.
Conclusion: Research on joint allotransplantation has been limited over the last 20 years due to the rarity of clinical applications, the complex nature of surgical procedures, and uncertain outcomes stemming from immune rejection. However, the key to overcoming these challenges lies in extending graft survival and minimizing immunosuppressive side effects. With the emergence of new immunosuppressive strategies, the feasibility and clinical potential of vascularized joint allotransplantation warrants further investigation.
Vascularized composite allotransplantation (VCA) is a relatively new field that offers functional restoration of severely damaged limbs. Joint allotransplantation (JA), a specialized subgroup within VCA, aims to replace joints such as the elbow or knee (1–4). Compared to other VCA procedures, JA provides a segmental transplantation that can potentially offer significant functional restoration of the affected joint (5–8). Although limited clinical experience suggests that knee transplantation should only be considered as a last resort for patients with extensive loss of cartilage and bone, deficient extensor mechanism, and soft tissue and skin defects, JA has the advantage of offering functional reconstruction in cases where modern prostheses have failed (9–13).
However, as with other VCA procedures, immune rejection is the main obstacle to wider clinical application of JA. Studies have shown that, while technically and anatomically feasible, long-term survival of allografts has been limited due to serious immune rejection (2, 3, 14, 15). In addition, the necessary life-long immunosuppression carries risks such as increased susceptibility to infection and malignancy, which need to be balanced against the benefits of functional joint reconstruction (16, 17).
Research and clinical application of JA have stagnated recently, potentially due to these considerations. In this study, we aim to systematically review the current literature on joint-specific allotransplantations, summarizing relevant clinical studies, animal models, immune processes, rejection, and functional aspects involved. We will also discuss the prospects of novel immunosuppressive strategies, as well as future challenges in JA.
We conducted a systematic literature review following the PRISMA guidelines (Preferred Reporting Items for Systematic Reviews and Meta-Analyses, PRISMA 2020) (18) using the search terms (((joint) AND (allotransplantation)) OR ((joint) AND (allograft)) OR ((knee) AND (allotransplantation)) OR ((elbow) AND (allotransplantation))) in the PubMed, Cochrane Library, and Web of Science databases. We included articles on clinical experience, anatomical studies, and joint allotransplantation-specific animal experiments, and applied the following exclusion criteria: (1) non-English articles, (2) inaccessible full text, (3) non-vascularized joint allotransplantation, (4) whole-limb or face VCA (vascularized composite allotransplantation), even if it includes joints, and (5) articles that do not contain original data or specific relevant outcomes regarding joint allotransplantation. Figure 1 shows the flow chart detailing the search strategy and inclusion of eligible articles.
Figure 1 Search strategy flow chart in accordance with the PRISMA 2020 guidelines for eligible articles.
Based on the inclusion and exclusion criteria, we included 14 studies in this review. Seven of them represent joint allotransplantation-specific papers on experimental animal research (Table 1), while the other seven studies included three articles reporting clinical cases, three anatomical cadaver studies, and one CT-scan-based article (Table 2).
The animal studies on JA mainly used small animal models in rats and rabbits, with just one proof of principle study in dogs in the 90s. The studies employed different immunosuppression regimes for different durations, which were compared. Table 1 provides a description of the findings of the experimental studies.
Currently, knee joint allotransplantation is the only joint allotransplantation that has been performed in humans. Six cases of knee allotransplantation have been reported in the literature between 1996 and 2004. The first five cases used anti-thymocyte globulin as an induction therapy, along with cyclosporin A, azathioprine, and methylprednisolone as immunosuppressive regimens. However, these five knee allografts were lost within three years. One graft was lost due to surgical site infection, while the other four grafts suffered graft rejection at different stages, including one due to noncompliance (4). The sixth patient received tacrolimus and mycophenolate mofetil and had an acceptable range of motion of the transplanted knee. He was ambulatory and full weight-bearing during long-term surveillance. However, at 50 months, knee function decreased from 0-0-90 degrees flexion to 0-10-40 degrees, and anterior instability developed. The knee graft was eventually lost due to late rejection vasculopathy at 56 months following transplantation (4).
In addition to clinical studies, anatomical human cadaveric studies focusing on the technical feasibility of elbow and temporomandibular joint allotransplantation have shown that both are technically and anatomically feasible. Table 2 summarizes the findings of these studies.
Animal models have been used to investigate JA, with small animals such as rats and rabbits being the primary models (Table 1). In rat models of elbow JA, successful outcomes require anastomosis of the brachial artery and median nerve neurorrhaphy, internal fixation of the joint, and soft tissue coverage with recipient musculature (20, 21, 24, 25). Similarly, knee allotransplantations in rabbits have been found to provide good skeletal stability during long-term observation (26). To improve graft viability without the need for long-term immunosuppression, Kremer et al. introduced surgical neoangiogenesis in rabbit knee allotransplantations using a superficial inferior epigastric fascial flap and a saphenous arteriovenous bundle to generate a neoangiogenic bone circulation (22, 23).
Despite their advantages, small animal models have limitations when compared to humans and large animals. These include differences in genome, immunological behavior, and biomechanical parameters such as weight load and range of motion (27–29). Hence, small animal models are typically used as the first step in any in vivo research. For example, chimerism has been induced in small animal models to overcome immunological barriers in VCA, but this has not been achieved in larger animals or humans (30, 31).
Large animal models can serve as an intermediate step before clinical application in humans. However, joint-specific allotransplantations have not been widely reported in this setting. Knee allotransplantations in dogs were reported over 20 years ago, but these were proof-of-principle reports lacking surgical details and radiological follow-up (19, 32). The focus then shifted towards heterotopic swine hind limb and porcine orthotopic forelimb models, which are currently widely used in VCA research (33–35). It appears to be more challenging to establish joint-specific large animal models for JA compared to whole limb allotransplantations. JA requires the precise dissection of structures surrounding the joint, such as ligaments, muscles, vessels, and regional nerves, in both the donor and recipient. For orthotopic replantation, internal fixation and joint remodeling are necessary, making it more demanding than whole limb allotransplantation, which only requires bone fixation and anastomosis on one end. In addition, ensuring long-term joint stability and eliminating potential external factors during follow-up observation is arguably more challenging in large animals than in small animals due to differences in biomechanical burden. Thus, future studies are needed to establish large animal models of JA with reliable and reproducible results.
Several studies have demonstrated that JA in humans is technically and anatomically feasible (Table 2). In addition to the knee, recent investigations in human cadavers have suggested that elbow allotransplantation could also be viable based on local vascular anatomy and existing surgical techniques (14, 15). Similarly, studies of the temporomandibular joint have suggested the feasibility of JA based on geometric analysis of maxillofacial CT-scans, and subsequent transplantations in cadavers appear to confirm these findings (9–11).
In 2021, the first composite vascularized elbow autograft was reported from a left to right upper extremity. The patient was able to complete daily living activities independently 4 years postoperatively. Although an autograft was used in this case, it further illustrates the technical feasibility and potential success of elbow joint transplantations (36).
However, despite the theoretical and technical viability of JA, its reported clinical applications lag far behind those of other VCA procedures (37). Graft rejection remains the main obstacle to clinical implementation of JA, as described by several studies (2, 3, 17). The limited clinical experience available thus far has shown rather sobering results. Hofmann et al. reported a total of six knee allotransplantations, and despite the application of immunosuppressants, all patients eventually lost the grafts due to immune rejections. Ultimately, four patients underwent above-the-knee amputations and two were subject to arthrodesis (3, 4). However, before the eventual immune rejection, joint function appeared to improve gradually during long-term follow-up.
The survival time of knee allotransplantations is currently limited, with the longest reported survival time being 56 months. This duration is much shorter than that of whole upper limb transplantations, which have been reported to survive for more than 10 years (3, 37–39). Rejection of the transplant is more likely to occur in JA due to their specific anatomical and surgical characteristics. JA is typically performed as a segmental, partial transplantation, where both the proximal and distal ends are connected to, and almost completely surrounded by, recipient tissue. This characteristic of JA resembles solid organ transplantation rather than other VCA, and theoretically renders it more vulnerable to immune recognition and rejection (39–43). Early detection of rejection in JA is challenging since the graft is usually embedded in recipient tissue (44, 45). Therefore, the diagnosis of rejection mainly relies on patient complaints, which typically lag behind the occurrence of rejection. In previous studies, it has been suggested that inclusion of a skin flap in the JA could improve graft monitoring and immunosuppressive administration. The limited success and shorter graft survival time of JA compared to whole upper limb transplantations may be explained by a combination of these factors (4, 38).
A joint allograft consists of multiple tissues, including articular capsule, bone, cartilage, ligament, synovia, and optionally some amount of skin and muscle. After transplantation, these tissues are targets of rejection reactions by both the innate and adaptive immune systems of the host. The initial inflammatory response – representing the ‘danger’ signal – will lead to direct and indirect graft antigen presentation – providing the ‘foreign’ signal – and finally to graft tissue damage via T cell-mediated cytotoxicity, antibody-dependent cell- and/or complement-mediated cytotoxicity, and other mechanisms (Figure 2). Mechanisms of rejection in VCA have been studied and discussed in detail, with a particular focus on the processes in the skin and soft tissues (40, 42, 46, 47). However, much less is known about the cartilage-specific rejection mechanisms, which are crucial in JA.
Figure 2 Components of joint allotransplantation and the immune response. The joint allograft comprises the articular capsule, bone, cartilage, ligaments, synovia, and possibly varying amounts of skin and muscle. Immune rejection begins with the recognition of donor major histocompatibility complex (MHC) molecules by donor antigen-presenting cells (APCs) in the direct pathway or by recipient APCs in the indirect pathway. These APCs subsequently present MHC to CD4 T helper (Th) cells, which activate immune cells such as cytotoxic T lymphocytes (CTLs), natural killer (NK) cells, macrophages, B cells, and the complement system. This immune response can induce rejection through mechanisms such as cell-mediated cytotoxicity, antibody-dependent cell-mediated cytotoxicity (ADCC), formation of the membrane attack complex (MAC), and the release of various cytokines and inflammatory mediators, eventually resulting in the death of the transplanted cells. Figure created with BioRender.com.
Bone typically represents the primary component in JA. It has been observed that the presence of vascularized bone creates a more tolerogenic environment than VCA grafts without bone (42). Previous bone VCA studies in large animal models have evaluated bone healing and remodeling and quantified bone characteristics. These studies have demonstrated that the development of a periosteal callus and new bone arising from the vascularized allotransplant led to the formation of a bridging callus, as opposed to cryopreserved allogenic bone (48). Despite these promising results, rejection of bone may still occur. Clinical studies have shown reduced or absent bone metabolism in late postoperative single photon emission computed tomography (SPECT) scans, with bone necrosis subsequently confirmed by bone biopsy in two patients with knee allotransplantations (49).
Allogeneic bone marrow transplantation offers distinct immunomodulatory benefits, enabling the formation of chimerism and even immunologic tolerance in transplantation (50, 51). This is particularly relevant for VCA, where bone marrow plays a critical role. The engraftment of the bone marrow component within the transplanted bone segment provides a native advantage in VCA, by facilitating a continuous supply of donor-derived hematopoietic progenitor cells, which in turn leads to enhanced and expedited reconstitution of transplanted tissues. Prior research has established that vascularized bone marrow plays a pivotal role in improving clinical outcomes in graft survival, as well as in promoting the formation of macrochimerism in primates (52). Furthermore, sustainable chimerism and immune tolerance have been successfully achieved in VCA mouse models via the engraftment of vascularized bone marrow (51). The JA approach may use the advantages of transplanted bone marrow, however the specific impact of this particular combination requires further investigation to fully realize its potential.
Cartilage serves an important functional role in JA. Studies have suggested that chondrocytes and cartilage possess immunosuppressive and immune privileged properties. Chondrocytes, for example, have been shown to express CD80/B7 inhibitors, chondromodulin I, and secrete indoleamine 2,3-dioxygenase (IDO) as well as exhibit mesenchymal lineage in vitro, suggesting they may be immuno-evasive (53–56). Moreover, the dense extracellular matrix (ECM) surrounding chondrocytes in vivo may sequester antigens and provide a physical barrier against immune detection (57–59). However, clinical studies in humans and animals do not entirely support the immune-privilege theory (58–61). Knee chondrocytes in a rat composite tissue allotransplantation model underwent apoptosis induced by acute rejection, and histopathology of human knee transplantation cases showed necrosis of articular cartilage (24). The mechanisms of cartilage rejection in JA are not well understood, although a possible explanation is that humoral signaling in the synovial fluid may play a role.
Fibrous tissues such as cruciate ligaments are typically included in knee transplantation to provide joint stability. They may, however, be attacked by the host immune system, leading to delayed remodeling and potentially impaired long-term stability compared to autografts. Clinical studies of fresh-frozen anterior cruciate ligament (ACL) allograft reconstruction have demonstrated excellent results, but also highlighted the risk of immune rejection (62–66).
In JA, skin is an optional component, but is often included due to its susceptibility to immune reactions. The skin contains twice as many T-cells as the same volume of blood as well as langerhans cells, skin-resident dendritic cells, which is usually the first tissue targeted by the host’s immune response in a vascularized composite allotransplantation (VCA) (67–70). As a result, several studies have suggested the inclusion of a skin component to monitor for early signs of JA rejection (71, 72).
Muscle is not essential in JA, but may be required in cases of extensive tissue loss. While muscles are less prone to lymphocytic infiltration and acute rejection due to their comparably low number of immune cells, they still carry a risk of atrophy and fibrosis, which may ultimately impact joint function (42, 73).
The primary objective of immunosuppression or immunoregulation in VCA, including JA, is to prevent immune rejection or, ideally, establish long-term immune tolerance, ultimately ensuring graft survival. In general, immunosuppressive treatment strategies used in VCA, including novel approaches, can be applied to JA (Figure 3). A standard treatment algorithm for VCA has not been clearly defined, and the common protocols used in clinics for immunosuppression and rejection management in VCA, usually derived from solid organ transplantation which can be classified in induction, maintenance, and rescue therapy (74–78).
Figure 3 Immunosuppressive strategies for joint allotransplantation (JA). Induction therapy includes total body irradiation (in experimental settings) and anti-thymocyte globulin (ATG). Surgical neoangiogenesis can be achieved through microsurgery. Traditional systemic administration of immunosuppressive drugs is one option. Site-specific delivery of immunosuppressive drugs to the transplant site can also be used. Costimulation blockade treatment is a newer approach that targets T cell activation and proliferation. Cellular therapy, including mesenchymal stem cells (MSCs) and hematopoietic cell transplantation, is another promising strategy that has shown potential in preclinical studies. Figure created with BioRender.com.
T-cell depleting agents such as antithymocyte immunoglobulin (ATG), basiliximab, and alemtuzumab are commonly used for immunosuppressive induction to prevent early acute rejection and graft loss (74–76). To date, ATG has been exclusively used for face transplant recipients, while all three agents are equally utilized in hand transplantation (74).
Triple therapy with calcineurin inhibitors tacrolimus, mycophenolate mofetil (MMF), and corticosteroids is typically used as maintenance immunosuppression in VCA (75, 76). Dual-steroid-free maintenance immunosuppression has also been reported and shows long-term allograft survival with infrequent and manageable complications in a series of VCA recipients (77).
Pulsed steroid therapy and increasing maintenance immunosuppression have successfully been used as rescue therapy. For steroid-resistant acute rejections, ATG and alemtuzumab are beneficial (78).
In the previously reported knee allotransplantation series, the first five cases used anti-thymocyte globulin as an induction therapy, along with cyclosporin A, azathioprine, and methylprednisolone as immunosuppressive regimens. In the sixth and last patient of the series, tacrolimus and MMF were used instead of cyclosporin A and azathioprine. This last patient showed better graft survival and functional outcomes compared to the first five patients (3). Considering the unique physiological and anatomical characteristics of JA, novel therapeutic approaches should also be discussed.
One promising treatment approach to reduce graft immunogenicity is surgical neoangiogenesis, which is distinct from whole limb transplantation. Joint allografts are interposed between recipient tissue on both ends, providing an opportunity to introduce recipient-specific blood supply and limit the size of the required donor pedicle. Previous studies have demonstrated that longer and thicker vascular pedicles from the donors are more likely to develop intimal hyperplasia, significant fibrotic perivascular tissue formation, and ischemia due to untreated episodes of acute rejection, leading to graft vasculopathy and poor survival of vascularized bone and joint allografts (4, 79). A saphenous arteriovenous (AV) bundle from the recipient has been used successfully to generate neoangiogenic bone circulation in JA (23, 48).
Another emerging approach to immunological control in VCA is site-specific delivery systems for immunosuppression. These systems have the potential to reduce the necessary systemic immunosuppression dosage, thereby alleviating its toxicity and side effects while maintaining sufficient drug levels within the graft to prevent rejection. The direct accessibility of the graft for these local interventions represents a native advantage in VCA (80). High-dose intra-graft administration of tacrolimus has been shown to prolong allograft survival, even when the drug is not detectable systemically (81). Similarly, site-specific application of tacrolimus-loaded hydrogel had a similar effect (82, 83). Other methods of site-specific immunosuppression delivery, such as implants and nanoparticles loaded with rapamycin, have also been employed successfully (84, 85). For JA, immunosuppression could potentially be delivered directly into the joint or surrounding tissue. However, at present, no such studies have been performed, and it remains to be seen whether the clinical outcomes of JA can be significantly improved by these methods.
Cellular therapies based on mesenchymal stem cells (MSCs), including bone marrow-derived MSCs and adipose-derived stem cells, have been explored as adjuvant therapy to traditional immunosuppression in allotransplantation. MSCs can increase the number of regulatory T-cells through various mechanisms, including TGF-B and indoleamine 2,3-dioxygenase, as well as decrease the expression of proinflammatory cytokines (TNF-α and IL-12) in circulation (52, 86, 87). Recent studies have demonstrated that after T cell-replete hematopoietic cell transplantation with induction therapy, VCAs rapidly become infiltrated with recipient T-cells in the absence of gross or histological evidence of rejection, resulting in cutaneous T-cell chimerism and suggesting that the infiltrating cells are rapidly tolerized (88). Because of their tissue composition, VCAs may be considered to carry an innate pool of MSCs. Whether grafted MSCs can exert a significant immunosuppressant effect in JA remains to be seen.
Costimulation blockade (CoB) has emerged as a promising approach for allotransplantation, including VCA (89). CTLA4-IgG1 or belatacept, a fusion protein of CTLA4 and IgG1, can effectively inhibit T- and B-lymphocyte activity and prevent immune rejection via CD28/B7 costimulation between T- and B-lymphocytes (90). A recent study in rats induced mixed chimerism and revealed intrinsic tolerogenic potential using CoB (31). Similar results have been demonstrated by other animal researches (91). Clinical case also showed belatacept can provide sufficient prophylaxis from rejection without chronic calcineurin inhibitors-associated side effects in VCA (92). However, no experimental studies have explored the impact of CoB in a JA model.
The question remains whether the benefits of functional joint reconstruction justify the potential costs of surgical failure and life-long immunosuppression side effects. These challenges considerably restrict the clinical application of JA, often leaving patients who do not qualify for an artificial joint replacement with few alternatives besides arthrodesis or amputation. However, the most recent JA clinical cases were performed over a decade ago, and ongoing clinical and preclinical trials for new immunosuppression therapies could help overcome the primary hurdles of rejection and immunosuppression-related toxicity.
Current research has demonstrated that induction therapy, such as total body irradiation and anti-thymocyte globulin combined with any of the treatments mentioned above, can prolong graft survival time and even result in chimerism and immune tolerance, particularly in small animal models. However, unlike life-threatening diseases, JA and other VCA pose long-term threats to patients. For young individuals and others, potential side effects that can result in death or disability are far more concerning than the possibility of functional restoration. Therefore, minimizing the side effects of immunosuppression is of paramount importance, given the significant potential for JA’s widespread clinical use. Promising prospects for local immunosuppression in the short term, as well as immunoregulation, exist for achieving this goal.
Functional reconstruction is the primary objective of JA and it is both theoretically and practically feasible to improve the quality of life through this procedure. Several studies have reported successful functional outcomes following JA, such as a study of hand transplantation which demonstrated a satisfying functional outcome over 10 postoperative years, and a knee transplantation case which achieved a range of motion almost equivalent to that of a healthy joint (3, 37, 39). However, restoring mobility and stability involves various structures, including muscles, nerves, and accessory structures around the joint, all of which are crucial to its static and dynamic function. The intrinsic function of a joint includes movement, weight-bearing, and buffering among other factors.
Achieving proper weight-bearing function after JA can be challenging, particularly as in VCA there are no direct comparisons due to the lack of leg transplant cases. Although previous studies have shown that an orthotopic load-bearing porcine forelimb VCA was successfully established within 14 days, patients who received knee transplants eventually lost their ability to fully or partially weight-bear due to complications such as fatigue fractures and infections (3, 33). Therefore, it is crucial to ensure good intraoperative bony fixation during the transplantation procedure to support weight-bearing ability. Moreover, preventing fractures in the long term is a significant concern as inflammation caused by rejection can weaken the bone and increase the risk of fractures. Hence, appropriate measures must be taken to prevent rejection and maintain the structural integrity of the transplanted joint to ensure optimal functional outcomes (93, 94). This includes adequate postoperative management, such as regular follow-up appointments to monitor bone density, physical therapy to strengthen muscles around the joint, and obviously appropriate immunosuppressive medication to prevent rejection.
Restoring motor function can be challenging and involves movement around one or more axes, depending on the specific joint. Joints act as a lever point around which the surrounding muscles generate movement (95). Each motor function typically involves the coordinated movement of entire muscle groups around the joint, rather than a single muscle (96). Reduced muscular excursion due to prolonged immobilization in patients who are candidates for JA makes restoring motor function a lengthy process that requires ongoing rehabilitation and physical training. Previous knee transplantation cases have shown improvement in the range of motion up to 1.5 years after transplantation (4).
Joints also act as buffers against movement and force, such as the meniscus in the knee joint, which plays a significant role in absorbing impact during activities like running and jumping. Preventing long-term collagen absorption and rejection in allografted menisci is challenging, and once the meniscus is affected by immune rejection, functional reconstruction of the knee becomes hard to maintain. Non-vascularized cartilage replacements including autografts and allografts have been used in the clinical setting to treat degenerative cartilage disorders. Previous studies have shown that the mean survival rate of osteochondral allografts in the patellofemoral joint was 87.9% at 5 years and 77.2% at 10 years (97). However, in JA, the cartilage, including the meniscus, is only part of a larger composite allograft and is therefore potentially more exposed to inflammation and immune rejection.
Thus, adequate immunosuppression is essential in JA to ensure long-term functional restoration and rehabilitation. Any inflammatory reaction in the graft from immune rejection will cause swelling and pain, leading to disturbances in motor function and eventually the failure of the desired functional reconstruction (98, 99). Therefore, careful management of immunosuppression is critical for maintaining long-term graft survival and improving the functional outcomes of JA.
As restoration of function is a primary goal of JA, it is crucial to have adequate assessment tools for accurate documentation of the functional reconstruction process. Kubiak et al. proposed three categories of functional assessment after VCA: surveys, observational rating of performance, and kinematic evaluations (100). These categories should be customized based on the specific joint being transplanted. In the clinical setting, several surveys and rating instruments, such as the Disabilities of the Arm, Shoulder and Hand questionnaire (DASH), are available for functional assessment. Additionally, kinematic evaluations provide objective measurements of motor function and strength (100–103). To evaluate functional parameters in animal JA models, passive range of motion, cantilever bending, and elasticity of cartilage can be assessed (48). Other investigations, including scintigraphy, sonography, angiography, electrophysiological examination, and CT-bone scans, can be used to verify the postoperative viability and perfusion of the transplanted joint (49). To objectively assess motor function in animal models, several methods such as axial compression test, Basso, Beattie and Bresnahan (BBB) locomotor scale method, and high-speed motion capture systems to measure three-dimensional kinematics of joints can be used (104–106).
While prosthetic replacements offer functional restoration without immune rejection, they also have their own problems and complications (107, 108). JA can be considered as a last salvage option for patients with advanced joint destruction. Although JA offers a promising alternative to arthrodesis and amputation, its clinical applications are insignificant compared to prostheses and function-sacrificing surgical procedures. The issue of immune rejection remains a significant obstacle, and several unsolved questions continue to hinder the widespread use of this reconstructive option. However, the clinical demand for functional reconstruction in young individuals and cases that do not qualify for prosthetic solutions warrants further investigations in the field of JA. In the future, a new generation of immunosuppressive treatment options, which prolong graft survival and alleviate systemic toxicity and long-term side effects, could address these concerns and promote the clinical application of JA. Despite the limited success in past clinical cases, these emerging new immunosuppressive strategies and the persisting clinical demand warrant further investigations in the field of JA.
Joint allotransplantation aims to restore joint motor function and improve the quality of life for recipients. Despite its potential benefits, research on joint-specific allotransplantation has been limited in the past two decades due to the complexity of the surgical procedures, limited clinical applications, and the risk of immune rejection, which can lead to uncertain outcomes.
To address these challenges, it is essential to prolong graft survival while minimizing the adverse effects of immunosuppressive drugs. Emerging immunosuppressive strategies offer new opportunities to improve transplantation outcomes, and further studies are needed to evaluate the feasibility and clinical potential of vascularized joint allotransplantation.
A deeper understanding of the immunological mechanisms involved in joint-specific transplantation may enable the optimization of transplantation outcomes and ultimately improve patient outcomes and quality of life. Therefore, advancing research in this field has the potential to contribute significantly to the advancement of transplantation medicine.
The study was conceived and designed by RO, while LZ was responsible for data summarization and manuscript drafting. The manuscript was then revised by IH, CZ, RR, MC, and RO, who also provided important reagents and approved the final version. All authors contributed to the article and approved the submitted version.
Open access funding by University Of Bern.
The authors declare that the research was conducted in the absence of any commercial or financial relationships that could be construed as a potential conflict of interest.
All claims expressed in this article are solely those of the authors and do not necessarily represent those of their affiliated organizations, or those of the publisher, the editors and the reviewers. Any product that may be evaluated in this article, or claim that may be made by its manufacturer, is not guaranteed or endorsed by the publisher.
1. Hofmann GO, Kirschner MH, Wagner FD, Brauns L, Gonschorek O, Bühren V. Allogeneic vascularized transplantation of human femoral diaphyses and total knee joints–first clinical experiences. Transplant Proc (1998) 30(6):2754–61. doi: 10.1016/s0041-1345(98)00803-3
2. Hofmann GO, Kirschner MH. Clinical experience in allogeneic vascularized bone and joint allografting. Microsurgery (2000) 20(8):375–83. doi: 10.1002/1098-2752(2000)20:8<375::aid-micr6>3.0.co;2-0
3. Diefenbeck M, Wagner F, Kirschner MH, Nerlich A, Mückley T, Hofmann GO. Outcome of allogeneic vascularized knee transplants. Transpl Int (2007) 20(5):410–8. doi: 10.1111/j.1432-2277.2007.00453.x
4. Diefenbeck M, Nerlich A, Schneeberger S, Wagner F, Hofmann GO. Allograft vasculopathy after allogeneic vascularized knee transplantation. Transpl Int (2011) 24(1):e1–5. doi: 10.1111/j.1432-2277.2010.01178.x
5. Gross AE, Silverstein EA, Falk J, Falk R, Langer F. The allotransplantation of partial joints in the treatment of osteoarthritis of the knee. Clin Orthopaedics Related Res (1975) 108):7–14. doi: 10.1097/00003086-197505000-00003
6. Janjić D, Ercegan G, Vukić D, Danka Z. [Degenerative arthritis of the knee joint after allotransplantation of the joint surface without immunosuppression]. Acta Chirurgica Iugoslavica (1990) 37 Suppl 1:35–9.
7. Somayaji HS, Tsaggerides P, Ware HE, Dowd GS. Knee arthrodesis–a review. Knee (2008) 15(4):247–54. doi: 10.1016/j.knee.2008.03.005
8. Park SE, Kim JY, Cho SW, Rhee SK, Kwon SY. Complications and revision rate compared by type of total elbow arthroplasty. J Shoulder Elbow Surg (2013) 22(8):1121–7. doi: 10.1016/j.jse.2013.03.003
9. Khavanin N, Davidson EH, Lee DY, Byrne P, Dorafshar AH. Anatomic considerations for temporomandibular joint vascularized composite allotransplantation. J Craniofac Surg (2018) 29(4):871–7. doi: 10.1097/SCS.0000000000004397
10. Khavanin N, Davidson EH, Smith RM, Macmillan A, Byrne P, Dorafshar AH. Considerations for temporomandibular joint procurement in vascularized composite allotransplantation. J Craniofac Surg (2018) 29(7):1742–6. doi: 10.1097/scs.0000000000004710
11. Nunez-Villaveiran T, Fahradyan V, Dalla Pozza E, Rezaei M, Drake RL, Jellema LM, et al. Full facial allotransplantation including the temporomandibular joints: a radiologic and anatomical cadaveric study. Plast Reconstr Surg (2020) 146(3):622–32. doi: 10.1097/prs.0000000000007069
12. Niedzielska I, Bak M, Niedzielski D, Okla H, Gabor J, Stanula A, et al. Temporomandibular joint prostheses: optimal materials for the optimal stomatognathic system performance-preliminary study. J Funct Biomater (2021) 12(1):7. doi: 10.3390/jfb12010007
13. Mercuri LG. Temporomandibular joint replacement devices - dark past to challenging future. Stomatol Dis Sci (2019) 3:3. doi: 10.20517/2573-0002.2018.25
14. Steinberger Z, Xu H, Kazmers NH, Thibaudeau S, Huffman RG, Levin LS. Elbow vascularized composite allotransplantation-surgical anatomy and technique. J Shoulder Elbow Surg (2017) 26(8):1325–34. doi: 10.1016/j.jse.2017.04.014
15. Pet MA, Lipira AB, Liu Y, Kao D, Ko JH. Vascularized composite allotransplantation of the elbow joint: a cadaveric study. Ann Plast Surg (2018) 80(4):438–47. doi: 10.1097/SAP.0000000000001292
16. Krezdorn N, Lian CG, Wells M, Wo L, Tasigiorgos S, Xu S, et al. Chronic rejection of human face allografts. Am J Transplant (2019) 19(4):1168–77. doi: 10.1111/ajt.15143
17. Lúcio MJ, Horta R. Hand transplantation-risks and benefits. J Handb Microsurg (2021) 13(4):207–15. doi: 10.1055/s-0040-1715427
18. Page MJ, McKenzie JE, Bossuyt PM, Boutron I, Hoffmann TC, Mulrow CD, et al. The PRISMA 2020 statement: an updated guideline for reporting systematic reviews. BMJ (Clinical Res ed) (2021) 372:n71. doi: 10.1136/bmj.n71
19. Rosso R, Schäfer D, Fricker R, Brennwald J, Jundt G, Spagnoli G, et al. Functional and morphological outcome of knee joint transplantation in dogs depends on control of rejection. Transplantation (1997) 63(12):1723–33. doi: 10.1097/00007890-199706270-00004
20. Vogelin E, Jones NF, Rao UN. Long-term viability of articular cartilage after microsurgical whole-joint transplantation and immunosuppression with rapamycin, mycophenolate mofetil, and tacrolimus. J Handb Surg Am (2002) 27(2):307–15. doi: 10.1053/jhsu.2002.32078
21. Larsen M, Friedrich PF, Bishop AT. A modified vascularized whole knee joint allotransplantation model in the rat. Microsurgery (2010) 30(7):557–64. doi: 10.1002/micr.20800
22. Kremer T, Giusti G, Friedrich PF, Willems W, Bishop AT, Giessler GA. Knee joint transplantation combined with surgical angiogenesis in rabbits–a new experimental model. Microsurgery (2012) 32(2):118–27. doi: 10.1002/micr.20946
23. Kremer T, Giessler GA, Friedrich PF, Willems WF, Giusti G, Bishop AT. Surgical angiogenesis with short-term immunosuppression maintains bone viability in rabbit allogenic knee joint transplantation. Plast Reconstr Surg (2013) 131(2):148e–57e. doi: 10.1097/PRS.0b013e3182789ad4
24. Shibuya N, Imai Y, Lee YS, Kochi T, Tachi M. Acute rejection of knee joint articular cartilage in a rat composite tissue allotransplantation model. J Bone Joint Surg Am (2014) 96(12):1033–9. doi: 10.2106/JBJS.M.00859
25. Tang J, Zhu H, Luo X, Li Q, Levin LS, Tintle SM. A vascularized elbow allotransplantation model in the rat. J Shoulder Elbow Surg (2015) 24(5):779–86. doi: 10.1016/j.jse.2015.01.006
26. Nolan LM, Bowen CV, Boyer MI. Skeletal fixation in the rabbit knee transplantation model. Microsurgery (1992) 13(5):291–2. doi: 10.1002/micr.1920130519
27. Hettiaratchy S, Melendy E, Randolph MA, Coburn RC, Neville DM Jr., Sachs DH, et al. Tolerance to composite tissue allografts across a major histocompatibility barrier in miniature swine. Transplantation (2004) 77(4):514–21. doi: 10.1097/01.tp.0000113806.52063.42
28. Cendales LC, Xu H, Bacher J, Eckhaus MA, Kleiner DE, Kirk AD. Composite tissue allotransplantation: development of a preclinical model in nonhuman primates. Transplantation (2005) 80(10):1447–54. doi: 10.1097/01.tp.0000183292.57349.27
29. Mundinger GS, Nam AJ, Hui-Chou HG, Stanwix MG, Jones LS, Drachenberg CB, et al. Nonhuman primate model of fibula vascularized composite tissue allotransplantation demonstrates donor-recipient bony union. Plast Reconstr Surg (2011) 128(6):1193–204. doi: 10.1097/PRS.0b013e318230c5d0
30. Brazio PS, Woodall J, Panda A, Brown EN, Ha JS, Azimzadeh AM, et al. Infused bone marrow fails to prevent vascularized composite allograft rejection in nonhuman primates. Am J Transplant (2015) 15(7):2011–2. doi: 10.1111/ajt.13268
31. Oh BC, Furtmuller GJ, Fryer ML, Guo Y, Messner F, Krapf J, et al. Vascularized composite allotransplantation combined with costimulation blockade induces mixed chimerism and reveals intrinsic tolerogenic potential. JCI Insight (2020) 5(7):e1285060. doi: 10.1172/jci.insight.128560
32. Schäfer D, Rosso R, Fricker R, Spagnoli G, Jundt G, Gerber H, et al. Functional outcome of transplanted knee joints in dogs. Arch Orthopaedic Trauma Surg (2000) 120(7-8):426–31. doi: 10.1007/s004029900115
33. Fries CA, Villamaria CY, Spencer JR, Rickard RF, Gorantla VS, Davis MR. A porcine orthotopic forelimb vascularized composite allotransplantation model: technical considerations and translational implications. Plast Reconstr Surg (2016) 138(3):461e–71e. doi: 10.1097/PRS.0000000000002451
34. Ibrahim Z, Cooney DS, Shores JT, Sacks JM, Wimmers EG, Bonawitz SC, et al. A modified heterotopic swine hind limb transplant model for translational vascularized composite allotransplantation (VCA) research. J Vis Exp (2013) 80:50475. doi: 10.3791/50475
35. Solla F, Pan H, Watrelot D, Leveneur O, Dubernard JM, Gazarian A. Composite tissue allotransplantation in newborns: a swine model. J Surg Res (2013) 179(1):e235–43. doi: 10.1016/j.jss.2012.01.030
36. Pathak N, Sides EE, Piper SL, Lee CK, Hansen SL, Terry MJ, et al. Composite vascularized autograft elbow transplant: a case report. JBJS Case Connector (2021) 11(3). doi: 10.2106/jbjs.cc.21.00220
37. Shores JT, Malek V, Lee WPA, Brandacher G. Outcomes after hand and upper extremity transplantation. J Mater Sci Mater Med (2017) 28(5):72. doi: 10.1007/s10856-017-5880-0
38. Park SH, Eun SC, Kwon ST. Hand transplantation: current status and immunologic obstacles. Exp Clin Transplant (2019) 17(1):97–104. doi: 10.6002/ect.2018.0163
39. Hautz T, Messner F, Weissenbacher A, Hackl H, Kumnig M, Ninkovic M, et al. Long-term outcome after hand and forearm transplantation - a retrospective study. Transpl Int (2020) 33(12):1762–78. doi: 10.1111/tri.13752
40. Sicard A, Kanitakis J, Dubois V, Morelon E, Thaunat O. Humoral alloreactivity in VCA recipients: should we learn from our experience? Transplantation (2020) 104(10):2003–10. doi: 10.1097/TP.0000000000003164
41. Kollar B, Pomahac B, Riella LV. Novel immunological and clinical insights in vascularized composite allotransplantation. Curr Opin Organ Transplant (2019) 24(1):42–8. doi: 10.1097/MOT.0000000000000592
42. Kaufman CL, Marvin MR, Chilton PM, Hoying JB, Williams SK, Tien H, et al. Immunobiology in VCA. Transpl Int (2016) 29(6):644–54. doi: 10.1111/tri.12764
43. Etra JW, Raimondi G, Brandacher G. Mechanisms of rejection in vascular composite allotransplantation. Curr Opin Organ Transplant (2018) 23(1):28–33. doi: 10.1097/mot.0000000000000490
44. Kanitakis J. The challenge of dermatopathological diagnosis of composite tissue allograft rejection: a review. J Cutaneous Pathol (2008) 35(8):738–44. doi: 10.1111/j.1600-0560.2007.00889.x
45. Issa F. Vascularized composite allograft-specific characteristics of immune responses. Transpl Int (2016) 29(6):672–81. doi: 10.1111/tri.12765
46. Kadono K, Gruszynski M, Azari K, Kupiec-Weglinski JW. Vascularized composite allotransplantation versus solid organ transplantation: innate-adaptive immune interphase. Curr Opin Organ Transplant (2019) 24(6):714–20. doi: 10.1097/MOT.0000000000000705
47. Kaufman CL, Cascalho M, Ozyurekoglu T, Jones CM, Ramirez A, Roberts T, et al. The role of b cell immunity in VCA graft rejection and acceptance. Hum Immunol (2019) 80(6):385–92. doi: 10.1016/j.humimm.2019.03.002
48. Houben RH, Kotsougiani D, Friedrich PF, Shin AY, Bishop AT. Outcomes of vascularized bone allotransplantation with surgically induced autogenous angiogenesis in a Large animal model: bone healing, remodeling, and material properties. J Reconstr Microsurg (2020) 36(2):82–92. doi: 10.1055/s-0039-1695052
49. Manthey N, Kirschner MH, Nerlich A, Hofmann GO, Tatsch K, Hahn K. 3-phase bone imaging and SPECT in the follow up of patients with allogenic vascularized knee joint transplants. Nuklearmedizin Nucl Med (2001) 40(6):187–92. doi: 10.1055/s-0038-1625759
50. Podesta MA, Remuzzi G, Casiraghi F. Mesenchymal stromal cells for transplant tolerance. Front Immunol (2019) 10:1287. doi: 10.3389/fimmu.2019.01287
51. Lin CH, Anggelia MR, Cheng HY, Wang AYL, Chuang WY, Lin CH, et al. The intragraft vascularized bone marrow component plays a critical role in tolerance induction after reconstructive transplantation. Cell Mol Immunol (2021) 18(2):363–73. doi: 10.1038/s41423-019-0325-y
52. Barth RN, Rodriguez ED, Mundinger GS, Nam AJ, Ha JS, Hui-Chou H, et al. Vascularized bone marrow-based immunosuppression inhibits rejection of vascularized composite allografts in nonhuman primates. Am J Transplant (2011) 11(7):1407–16. doi: 10.1111/j.1600-6143.2011.03551.x
53. Du WJ, Reppel L, Leger L, Schenowitz C, Huselstein C, Bensoussan D, et al. Mesenchymal stem cells derived from human bone marrow and adipose tissue maintain their immunosuppressive properties after chondrogenic differentiation: role of HLA-G. Stem Cells Dev (2016) 25(19):1454–69. doi: 10.1089/scd.2016.0022
54. Moskalewski S, Hyc A, Osiecka-Iwan A. Immune response by host after allogeneic chondrocyte transplant to the cartilage. Microsc Res Tech (2002) 58(1):3–13. doi: 10.1002/jemt.10110
55. Liu S, Yuan M, Hou K, Zhang L, Zheng X, Zhao B, et al. Immune characterization of mesenchymal stem cells in human umbilical cord wharton’s jelly and derived cartilage cells. Cell Immunol (2012) 278(1-2):35–44. doi: 10.1016/j.cellimm.2012.06.010
56. Klinger P, Surmann-Schmitt C, Brem M, Swoboda B, Distler JH, Carl HD, et al. Chondromodulin 1 stabilizes the chondrocyte phenotype and inhibits endochondral ossification of porcine cartilage repair tissue. Arthritis Rheumatol (2011) 63(9):2721–31. doi: 10.1002/art.30335
57. Smith B, Sigal IR, Grande DA. Immunology and cartilage regeneration. Immunol Res (2015) 63(1-3):181–6. doi: 10.1007/s12026-015-8720-7
58. Langer F, Gross AE. Immunogenicity of allograft articular cartilage. J Bone Joint Surg Am (1974) 56(2):297–304. doi: 10.2106/00004623-197456020-00007
59. Arzi B, DuRaine GD, Lee CA, Huey DJ, Borjesson DL, Murphy BG, et al. Cartilage immunoprivilege depends on donor source and lesion location. Acta Biomater (2015) 23:72–81. doi: 10.1016/j.actbio.2015.05.025
60. Cui P, Liu P, Li S, Ma R. De-epithelialized heterotopic tracheal allografts without immunosuppressants in dogs: long-term results for cartilage viability and structural integrity. Ann Otol Rhinol Laryngol (2021) 130(5):441–9. doi: 10.1177/0003489420957357
61. Kimura Y, Yamamoto Y, Sasaki S, Sasaki E, Sasaki T, Tsuda E, et al. Meniscus allograft transplantation obtained from adult patients undergoing total knee arthroplasty may be used for younger patients after lateral discoid meniscus meniscectomy. Arthrosc Sports Med Rehabil (2021) 3(6):e1679–e85. doi: 10.1016/j.asmr.2021.07.024
62. Busam ML, Rue JP, Bach BR Jr. Fresh-frozen allograft anterior cruciate ligament reconstruction. Clin Sports Med (2007) 26(4):607–23. doi: 10.1016/j.csm.2007.06.001
63. Sikka RS, Narvy SJ, Vangsness CT Jr. Anterior cruciate ligament allograft surgery: underreporting of graft source, graft processing, and donor age. Am J Sports Med (2011) 39(3):649–55. doi: 10.1177/0363546510382222
64. Nikolaou PK, Seaber AV, Glisson RR, Ribbeck BM, Bassett FH 3rd. Anterior cruciate ligament allograft transplantation. long-term function, histology, revascularization, and operative technique. Am J Sports Med (1986) 14(5):348–60. doi: 10.1177/036354658601400502
65. Scheffler SU, Schmidt T, Gangey I, Dustmann M, Unterhauser F, Weiler A. Fresh-frozen free-tendon allografts versus autografts in anterior cruciate ligament reconstruction: delayed remodeling and inferior mechanical function during long-term healing in sheep. Arthroscopy (2008) 24(4):448–58. doi: 10.1016/j.arthro.2007.10.011
66. Zeng C, Gao SG, Li H, Yang T, Luo W, Li YS, et al. Autograft versus allograft in anterior cruciate ligament reconstruction: a meta-analysis of randomized controlled trials and systematic review of overlapping systematic reviews. Arthroscopy (2016) 32(1):153–63 e18. doi: 10.1016/j.arthro.2015.07.027
67. Petruzzo P, Lanzetta M, Dubernard JM, Landin L, Cavadas P, Margreiter R, et al. The international registry on hand and composite tissue transplantation. Transplantation (2010) 90(12):1590–4. doi: 10.1097/TP.0b013e3181ff1472
68. Schneeberger S, Gorantla VS, Brandacher G, Zeevi A, Demetris AJ, Lunz JG, et al. Upper-extremity transplantation using a cell-based protocol to minimize immunosuppression. Ann Surgery (2013) 257(2):345–51. doi: 10.1097/SLA.0b013e31826d90bb
69. Chadha R, Leonard DA, Kurtz JM, Cetrulo CL Jr. The unique immunobiology of the skin: implications for tolerance of vascularized composite allografts. Curr Opin Organ Transplant (2014) 19(6):566–72. doi: 10.1097/MOT.0000000000000136
70. Clark RA, Chong B, Mirchandani N, Brinster NK, Yamanaka K, Dowgiert RK, et al. The vast majority of CLA+ T cells are resident in normal skin. J Immunol (Baltimore Md: 1950) (2006) 176(7):4431–9. doi: 10.4049/jimmunol.176.7.4431
71. Starzl R, Brandacher G, Lee WP, Carbonell J, Zhang W, Schnider J, et al. Review of the early diagnoses and assessment of rejection in vascularized composite allotransplantation. Clin Dev Immunol (2013) 2013:402980. doi: 10.1155/2013/402980
72. Robbins NL, Wordsworth MJ, Parida BK, Kaplan B, Gorantla VS, Weitzel EK, et al. Is skin the most allogenic tissue in vascularized composite allotransplantation and a valid monitor of the deeper tissues? Plast Reconstr Surg (2019) 143(4):880e–6e. doi: 10.1097/PRS.0000000000005436
73. Kuo YR, Sacks JM, Lee WP, Wu WS, Kueh NS, Yao SF, et al. Porcine heterotopic composite tissue allograft transplantation using a large animal model for preclinical studies. Chang Gung Med J (2006) 29(3):268–74.
74. Daneshgaran G, Stern CS, Garfein ES. Reporting practices on immunosuppression and rejection management in face transplantation: a systematic review. J Reconstr Microsurg (2019) 35(9):652–61. doi: 10.1055/s-0039-1691787
75. Fischer S, Lian CG, Kueckelhaus M, Strom TB, Edelman ER, Clark RA, et al. Acute rejection in vascularized composite allotransplantation. Curr Opin Organ Transplant (2014) 19(6):531–44. doi: 10.1097/MOT.0000000000000140
76. Matsunaga T, Azuma H, Tullius SG. Immunosuppression in vascularized composite allotransplant: the search for an effective and safe treatment continues. Transpl Int (2020) 33(10):1291–3. doi: 10.1111/tri.13629
77. Diaz-Siso JR, Fischer S, Sisk GC, Bueno E, Kueckelhaus M, Talbot S, et al. Initial experience of dual maintenance immunosuppression with steroid withdrawal in vascular composite tissue allotransplantation. Am J Transplant (2015) 15(5):1421–31. doi: 10.1111/ajt.13103
78. Kueckelhaus M, Fischer S, Seyda M, Bueno EM, Aycart MA, Alhefzi M, et al. Vascularized composite allotransplantation: current standards and novel approaches to prevent acute rejection and chronic allograft deterioration. Transpl Int (2016) 29(6):655–62. doi: 10.1111/tri.12652
79. Robbins NL, Wordsworth MJ, Parida BK, Kaplan B, Gorantla VS, Weitzel CEK, et al. A flow dynamic rationale for accelerated vascularized composite allotransplant rejection. Plast Reconstr Surg (2019) 143(3):637e–43e. doi: 10.1097/prs.0000000000005352
80. Gajanayake T, Olariu R, Leclère FM, Dhayani A, Yang Z, Bongoni AK, et al. A single localized dose of enzyme-responsive hydrogel improves long-term survival of a vascularized composite allograft. Sci Trans Med (2014) 6(249):249ra110. doi: 10.1126/scitranslmed.3008778
81. Olariu R, Denoyelle J, Leclere FM, Dzhonova DV, Gajanayake T, Banz Y, et al. Intra-graft injection of tacrolimus promotes survival of vascularized composite allotransplantation. J Surg Res (2017) 218:49–57. doi: 10.1016/j.jss.2017.05.046
82. Dzhonova DV, Olariu R, Leckenby J, Banz Y, Prost JC, Dhayani A, et al. Local injections of tacrolimus-loaded hydrogel reduce systemic immunosuppression-related toxicity in vascularized composite allotransplantation. Transplantation (2018) 102(10):1684–94. doi: 10.1097/tp.0000000000002283
83. Dzhonova D, Olariu R, Leckenby J, Dhayani A, Vemula PK, Prost JC, et al. Local release of tacrolimus from hydrogel-based drug delivery system is controlled by inflammatory enzymes in vivo and can be monitored non-invasively using in vivo imaging. PloS One (2018) 13(8):e0203409. doi: 10.1371/journal.pone.0203409
84. Sutter D, Dzhonova DV, Prost JC, Bovet C, Banz Y, Rahnfeld L, et al. Delivery of rapamycin using In situ forming implants promotes immunoregulation and vascularized composite allograft survival. Sci Rep (2019) 9(1):9269. doi: 10.1038/s41598-019-45759-y
85. Safi AF, Kauke M, Nelms L, Palmer WJ, Tchiloemba B, Kollar B, et al. Local immunosuppression in vascularized composite allotransplantation (VCA): a systematic review. J Plast Reconstr Aesthet Surg (2021) 74(2):327–35. doi: 10.1016/j.bjps.2020.10.003
86. Chen J, Wang Y, Hu H, Xiong Y, Wang S, Yang J. Adipose-derived cellular therapies prolong graft survival in an allogenic hind limb transplantation model. Stem Cell Res Ther (2021) 12(1):94. doi: 10.1186/s13287-021-02162-7
87. Vyas KS, Mohan AT, Morrison SD, Tran DC, Mardini S. Cell-based therapies in vascularized composite allotransplantation. J Reconstr Microsurg (2018) 34(8):642–50. doi: 10.1055/s-0038-1661336
88. Leonard DA, Powell HR, Defazio MW, Shanmugarajah K, Mastroianni M, Rosales IA, et al. Cutaneous leukocyte lineages in tolerant large animal and immunosuppressed clinical vascularized composite allograft recipients. Am J Transplant (2021) 21(2):582–92. doi: 10.1111/ajt.16230
89. Giannis D, Moris D, Cendales LC. Costimulation blockade in vascularized composite allotransplantation. Front Immunol (2020) 11:544186. doi: 10.3389/fimmu.2020.544186
90. Samy KP, Butler JR, Li P, Cooper DKC, Ekser B. The role of costimulation blockade in solid organ and islet xenotransplantation. J Immunol Res (2017) 2017:8415205. doi: 10.1155/2017/8415205
91. Atia A, Moris D, McRae M, Song M, Stempora L, Leopardi F, et al. Th17 cell inhibition in a costimulation blockade-based regimen for vascularized composite allotransplantation using a nonhuman primate model. Transpl Int (2020) 33(10):1294–301. doi: 10.1111/tri.13612
92. Cendales LC, Ruch DS, Cardones AR, Potter G, Dooley J, Dore D, et al. De novo belatacept in clinical vascularized composite allotransplantation. Am J Transplant (2018) 18(7):1804–9. doi: 10.1111/ajt.14910
93. Reynolds RJ, Walker PS, Buza J. Mechanisms of anterior-posterior stability of the knee joint under load-bearing. J Biomechanics (2017) 57:39–45. doi: 10.1016/j.jbiomech.2017.03.016
94. Bruno F, Arrigoni F, Palumbo P, Natella R, Splendiani A, Di Cesare E, et al. Weight-bearing MR imaging of knee, ankle and foot. Semin Musculoskeletal Radiol (2019) 23(6):594–602. doi: 10.1055/s-0039-1697940
95. Francis-Pester FW, Thomas R, Sforzin D, Ackland DC. The moment arms and leverage of the human finger muscles. J Biomechanics (2021) 116:110180. doi: 10.1016/j.jbiomech.2020.110180
96. Kimura A, Omura L, Yoshioka S, Fukashiro S. Identifying coordination between joint movements during a throwing task with multiple degrees of freedom. Hum Movement Sci (2021) 77:102799. doi: 10.1016/j.humov.2021.102799
97. Chahla J, Sweet MC, Okoroha KR, Nwachukwu BU, Hinckel B, Farr J, et al. Osteochondral allograft transplantation in the patellofemoral joint: a systematic review. Am J Sports Med (2019) 47(12):3009–18. doi: 10.1177/0363546518814236
98. McDougall JJ. Arthritis and pain. neurogenic origin of joint pain. Arthritis Res Ther (2006) 8(6):220. doi: 10.1186/ar2069
99. Salo P. The role of joint innervation in the pathogenesis of arthritis. Can J Surg J Canadien Chirurgie (1999) 42(2):91–100.
100. Kubiak CA, Etra JW, Brandacher G, Kemp SWP, Kung TA, Lee WPA, et al. Prosthetic rehabilitation and vascularized composite allotransplantation following upper limb loss. Plast Reconstr Surg (2019) 143(6):1688–701. doi: 10.1097/prs.0000000000005638
101. Karbach LE, Elfar J. Elbow instability: anatomy, biomechanics, diagnostic maneuvers, and testing. J Handb Surg Am (2017) 42(2):118–26. doi: 10.1016/j.jhsa.2016.11.025
102. Bernardon L, Gazarian A, Petruzzo P, Packham T, Guillot M, Guigal V, et al. Bilateral hand transplantation: functional benefits assessment in five patients with a mean follow-up of 7.6 years (range 4-13 years). J Plast Reconstr Aesthet Surg (2015) 68(9):1171–83. doi: 10.1016/j.bjps.2015.07.007
103. Salminger S, Sturma A, Roche AD, Hruby LA, Paternostro-Sluga T, Kumnig M, et al. Functional and psychosocial outcomes of hand transplantation compared with prosthetic fitting in below-elbow amputees: a multicenter cohort study. PloS One (2016) 11(9):e0162507. doi: 10.1371/journal.pone.0162507
104. Holowka NB, O’Neill MC, Thompson NE, Demes B. Chimpanzee ankle and foot joint kinematics: arboreal versus terrestrial locomotion. Am J Phys Anthropol (2017) 164(1):131–47. doi: 10.1002/ajpa.23262
105. Basso DM, Beattie MS, Bresnahan JC. A sensitive and reliable locomotor rating scale for open field testing in rats. J Neurotrauma (1995) 12(1):1–21. doi: 10.1089/neu.1995.12.1
106. Koffler J, Zhu W, Qu X, Platoshyn O, Dulin JN, Brock J, et al. Biomimetic 3D-printed scaffolds for spinal cord injury repair. Nat Med (2019) 25(2):263–9. doi: 10.1038/s41591-018-0296-z
107. Flesher SN, Downey JE, Weiss JM, Hughes CL, Herrera AJ, Tyler-Kabara EC, et al. A brain-computer interface that evokes tactile sensations improves robotic arm control. Sci (New York NY) (2021) 372(6544):831–6. doi: 10.1126/science.abd0380
Keywords: joint allotransplantation, knee allotransplantation, elbow allotransplantation, vascularized composite allotransplantation, functional reconstruction
Citation: Zhang L, Hoyos IA, Zubler C, Rieben R, Constantinescu M and Olariu R (2023) Challenges and opportunities in vascularized composite allotransplantation of joints: a systematic literature review. Front. Immunol. 14:1179195. doi: 10.3389/fimmu.2023.1179195
Received: 03 March 2023; Accepted: 08 May 2023;
Published: 19 May 2023.
Edited by:
Brian H. Johnstone, Ossium Health, Inc, United StatesReviewed by:
Byoung Chol Oh, Johns Hopkins University, United StatesCopyright © 2023 Zhang, Hoyos, Zubler, Rieben, Constantinescu and Olariu. This is an open-access article distributed under the terms of the Creative Commons Attribution License (CC BY). The use, distribution or reproduction in other forums is permitted, provided the original author(s) and the copyright owner(s) are credited and that the original publication in this journal is cited, in accordance with accepted academic practice. No use, distribution or reproduction is permitted which does not comply with these terms.
*Correspondence: Radu Olariu, cmFkdS5vbGFyaXVAaW5zZWwuY2g=
Disclaimer: All claims expressed in this article are solely those of the authors and do not necessarily represent those of their affiliated organizations, or those of the publisher, the editors and the reviewers. Any product that may be evaluated in this article or claim that may be made by its manufacturer is not guaranteed or endorsed by the publisher.
Research integrity at Frontiers
Learn more about the work of our research integrity team to safeguard the quality of each article we publish.