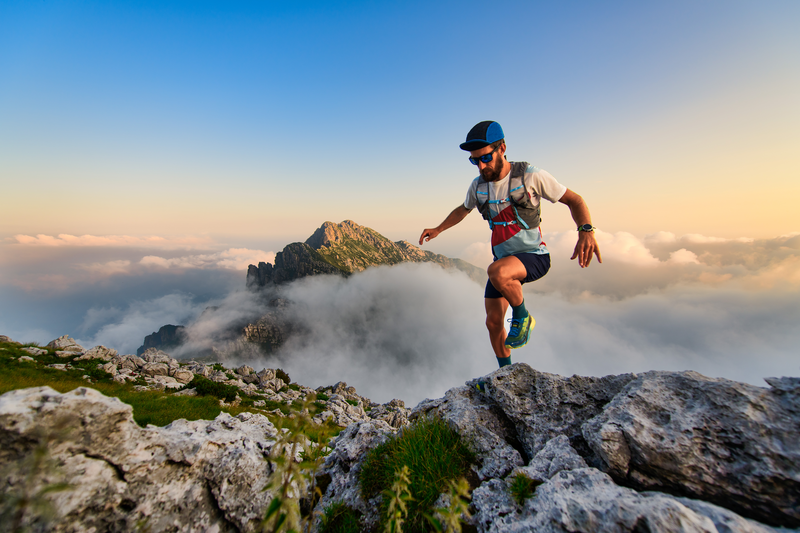
94% of researchers rate our articles as excellent or good
Learn more about the work of our research integrity team to safeguard the quality of each article we publish.
Find out more
REVIEW article
Front. Immunol. , 02 May 2023
Sec. NK and Innate Lymphoid Cell Biology
Volume 14 - 2023 | https://doi.org/10.3389/fimmu.2023.1175147
This article is part of the Research Topic Therapeutic Potential of Innate and Innate-like Effector Lymphocytes in Autoimmune and Inflammatory Diseases View all 14 articles
The innate system constitutes a first-line defence mechanism against pathogens. 80% of the blood supply entering the human liver arrives from the splanchnic circulation through the portal vein, so it is constantly exposed to immunologically active substances and pathogens from the gastrointestinal tract. Rapid neutralization of pathogens and toxins is an essential function of the liver, but so too is avoidance of harmful and unnecessary immune reactions. This delicate balance of reactivity and tolerance is orchestrated by a diverse repertoire of hepatic immune cells. In particular, the human liver is enriched in many innate immune cell subsets, including Kupffer cells (KCs), innate lymphoid cells (ILCs) like Natural Killer (NK) cells and ILC-like unconventional T cells – namely Natural Killer T cells (NKT), γδ T cells and Mucosal-associated Invariant T cells (MAIT). These cells reside in the liver in a memory-effector state, so they respond quickly to trigger appropriate responses. The contribution of aberrant innate immunity to inflammatory liver diseases is now being better understood. In particular, we are beginning to understand how specific innate immune subsets trigger chronic liver inflammation, which ultimately results in hepatic fibrosis. In this review, we consider the roles of specific innate immune cell subsets in early inflammation in human liver disease.
Understanding the liver´s architecture and the niches formed by the different hepatic immune cells is equally important to deciphering their immune roles. The liver is subdivided into hepatic lobules, which consist of a portal triad (hepatic artery, portal vein and bile duct), hepatocytes arranged in linear cords between a capillary network (sinusoids) and a central vein (Figure 1). The blood flows from the portal triad to the central vein. The vascular system connecting the portal triad to the central vein is mainly constituted by liver sinusoidal endothelial cells (LSECs). Large fenestrae allow the exchange of macromolecules and components from the sinusoids with hepatocytes (1, 2). Interestingly, hepatocytes have different functions based on their zoning. Close to the portal triad, hepatocytes are the first to interact with gut-derived antigens whereas hepatocytes in proximity to the central vein are associated with detoxification (3). The gradual change in blood nutrients, oxygen and antigen load is correlated with significant changes in hepatocytes´ gene expression signature (3, 4). Immune cells could also perform different functions according to their position within the liver. The distribution of innate cells in the liver is based on different chemokines, adhesion molecules and surface receptors (5). KCs are located adherent in the sinusoids and emit extensions into the Disse space. KCs along with LSECs constitute part of the reticuloendothelial system, which clears debris and harmful compounds in the blood. 65% of intrahepatic lymphocytes consist of NK cells, NKT cells, MAIT cells and γδ T cells (6–8) (Figures 2A, B). NK cells are in close proximity to KCs in both mouse and human models, suggesting a physical co-dependence (9, 10). NKT cells are constantly surveilling the liver sinusoids and stop when they detect inflammatory signals (9). CXCR6 was identified as a receptor to regulate mouse intrahepatic NKT cell frequencies and its ligand CXCL16 is overexpressed in macrophages and endothelium near injury areas (10). Human γδ T cells were identified in portal sections and in association with biliary epithelium (11). Human MAIT cells are reported to reside predominantly around bile ducts (12). However, the distribution and frequency of innate cells during inflammation are drastically changed with the recruitment of immune cells to the site of inflammation (9).
Figure 1 Diagrammatic representation of the liver architecture. The classical hexagonal lobule constitutes the anatomic unit of the liver. The lobule´s parenchyma is mainly formed by hepatocytes that are distributed along the sinusoids. The portal triad, formed by the hepatic artery (HA), the portal vein (PV) and the biliary duct (BD), carries the blood supply towards the centroid of the lobule where it is collected by the central vein (CV). Within the sinusoids, Kupffer cells (K) and Natural Killer cells (NK) are located in close proximity to the endothelium (beige). Other ILC-like cells such as iNKT cells and T2NKT cells are constantly surveying the sinusoids. Closer to the triad, especially near the BDs, there is a high frequency of MAIT cells and γδ T cells.
Figure 2 (A) Diagram tree of the approximate frequency of liver-resident cells and a FACS-based gating strategy to identify each cell type. The liver is mainly constituted by parenchyma (hepatocytes) and ILCs. Among ILCs, Kupffer cells and NK cells are the most abundant immune cells. The liver is also characteristic for having a niche of unconventional T cells, namely iNKT cells, T2NKT cells, γδ T cells and MAIT cells. (B) The main types of antigen recognition by unconventional T cells through their T-cell receptors (TCRs), Kupffer cells and NK cells. Kupffer cells and NK cells are activated through pattern recognition receptors. Additionally, NK cells have receptors that can sense healthy and stressed or dead cells.
KCs are liver-resident macrophages that constitute 15% of the total human non-parenchymal liver cell count (13). They represent the primary barrier against pathogens and toxic compounds coming from portal circulation (14). KCs are antigen-presenting cells (APC) and play a crucial role in inducing liver tolerance through cell-to-cell contact, cytokines and other mechanisms such as dioxygenase-dependent sequestration of tryptophan (15). Under physiological conditions, KCs are the major reservoir of macrophages in the liver and can self-renew independently from the bone marrow (16). Upon activation, KCs secrete chemokine ligand 2 (CCL2) which promotes the infiltration of human circulating monocyte-derived macrophages. Increased frequency of CCR2+ monocytes participates in liver fibrosis in mouse models (17, 18) and is indicative of pathology in human acetaminophen-induced acute liver injury (19). However, it is not yet clear whether liver-resident and circulating macrophages are two distinguished populations with different functions. The majority of pathogens coming from portal circulation are trapped in the liver by KCs phagocytosis. KCs cooperate with other non-parenchymal liver cells to clear potential infections (20). KCs can also sense damage-associated molecular patterns (DAMPs) expressed in hepatocytes that induce the secretion of a variety of cytokines and chemokines to efficiently restore homeostasis (20). When liver diseases compromise KCs function, aggravation of the diseases can be foreseen due to secondary infections (21).
MAIT cells are an abundant subset of hepatic T lymphocytes. They constitute up to 30-40% of human hepatic CD8+ T cells (6, 7). Their roles in pathogen defense and tissue repair have been previously reported (22–24). MAIT cells have an invariant T cell receptor (TCR) that recognizes the nonpolymorphic class Ib major histocompatibility (MHC) class I-related protein (MR1) when loaded with antigens. MAIT cells recognize riboflavin derivatives which are necessary for metabolism of many bacteria. These cells are considered an evolutionary system to defend hosts from pathogens since mammals do not produce these metabolites. Under inflammatory conditions, hepatocytes present the riboflavin derivative 5-A-RU to MAIT cells and also secrete IL-7 which is known to shape MAIT cells towards a pro-inflammatory state (7, 25). Upon activation, MAIT cells secrete large amounts of pro-inflammatory and pro-fibrogenic cytokines such as IFN-γ, TNF-α and IL-17 (26). Studies in humans demonstrated that triggering MAIT cells in the absence of co-stimulation with cytokines induces wound repair and tissue regeneration (24). These studies suggest that under physiological conditions, MAIT cells probably contribute to tissue repair and regeneration since there is a constant influx of 5-A-RU present in human sera (27) but promote inflammation under acute inflammation. The high sensitivity for cytokines indicates that MAIT cells might be one of the first contributors to early inflammatory responses.
γδ T cells are non-conventional subset of T lymphocytes with a limited non-MHC-restricted TCR repertoire. They constitute around 1-10% of human circulating T cells (28). They can recognize a wide variety of antigens and can be activated via pathogen-associated molecular patterns (PAMPs), DAMPs or cytokines alone. Upon activation, cells can execute cytotoxic as well as effector functions. Moreover, γδ T cells also play a role in tissue homeostasis (29). In humans, the stratification of γδ T cells is based on the Vδ gene segments used to produce their TCR. Vδ1+ T cells are abundant in the epithelium (30) and protect tissues via recognition of non-classical MHCs such as CD1a, CD1c and CD1d (31). Vδ2+ T cells are the most abundant subtype in circulation and can clear infections in periphery organs (28, 32). They recognize phosphoantigens, which are non-peptide low molecular weight antigens. Vδ2+ T cells respond rapidly in a Th1-like fashion to high amounts of self-phosphoantigens (for example in tumor cells) or microbial phosphoantigens (33, 34). The butyrophilin 3A (BTN3A) family can trigger activation of Vδ2+ T cells upon stimulation with phosphoantigens (35). The heterodimer BTNL3/BTNL8 expressed in APC was reported to mediate the TCR-dependent activation of Vδ2+ T cells by binding of the intracellular domain of BTNL3 with phosphoantigens (36). Interestingly, the expression of BTNL8 was not detectable in human PBMC but it was highly expressed in regulatory T cells after polyclonal stimulation (37). This suggests further investigation into the role of the butyrophilin family in the development of hepatitis and potential role in influencing Vδ2+ T cells. Vδ3+ T cells are a heterogeneous group of T lymphocytes enriched in the liver and also in some diseases such as leukaemia or chronic viral infection (38). They recognize antigens presented by CD1d molecules and respond by producing cytokines and killing of CD1d+ cells (38). Recent evidences suggest that γδ T cells may be involved in liver diseases as previously shown in other autoimmune diseases (28), especially due to the rapid and large secretion of IL-17 (39).
NKT cells are a rare subset of T lymphocytes comprising less than 1% of human peripheral blood T lymphocytes but enriched in the liver (8, 40). NKT cells are known to express NK cell markers like CD56, CD16 and CD161, and produce granzyme (40, 41). Their restricted TCR repertoire recognizes antigenic lipids presented by the MHC class I-like molecule CD1d (42, 43). Based on their TCR, NKT cells have been divided into two subsets. Type I NKT, or invariant (i)NKT cells, are the most studied group because they are enriched in mouse liver and have a semi-invariant TCR. The prototype ligand for iNKT cells is α-galactosylceramide (α-GalCer) (44). Type II NKT cells (T2NKT) consist of a subset with more diverse TCR. The major ligand recognized by T2NKT cells is sulfatide, which is a glycolipid enriched in the myelin of the central nervous system, pancreas, kidney and liver (45). It is difficult to study T2NKT cells because there is a lack of tools to identify and characterize them. Recently, we proposed a novel strategy to isolate and characterize T2NKT cells in humans but the low number of cells in blood is still a limitation (40). The role of iNKT cells and T2NKT cells in liver diseases have been mainly studied using transgenic mice models of CD1d-knockouts or TCRVα14-knockouts, which lack iNKT cells. These studies suggest that, in general, iNKT cells play a pro-inflammatory phenotype whereas T2NKT cells suppress inflammation through direct and indirect inhibition of inflammatory cells, including iNKT cells (46–49). We described a novel subpopulation of T2NKT cells that expresses regulatory T cell markers such as FoxP3 and CD25 (40). FoxP3+ T2NKT cells were found both in the periphery and in the liver and may explain some of the regulatory functions reported previously.
NK cells are a major component of the liver’s innate immune cell compartment. They account for almost 50% of human intrahepatic lymphocytes (50). Human hepatic NK cells are classified into three different subsets based upon their transcriptional, phenotypical and functional features (50). Liver-resident NK cells are CD56bright CD69+ CXCR6+ CCR5+ and highly cytotoxic (51–54). These cells are long-lived tissue-resident subsets (55). Interestingly, a subset of liver-resident CXCR6+ NK cells was described as having a memory-like responsiveness against - vesicular stomatitis virus (VSV), human immunodeficiency virus (HIV) and influenza (56). Memory-like NK cells produce higher amounts of IFN-γ after rechallenge with the virus. The third NK cell subset is transient circulating NK cells, which are CD56dim CD69- CXCR6- CCR5- and show less cytotoxic activity. They can secrete high amounts of pro-inflammatory cytokines such as TNF-α and GM-CSF (57–59). The regulation of NK cell activity consist on a balance between activating and inhibitory receptors displayed on their surface (60). NK cells survey the liver and induce apoptosis in infected or aberrant cells via different mechanisms such as FasL or TRAIL (61, 62). Under inflammatory conditions, NK cells kill hepatic stellate cells (HSCs) to resolve inflammation and limit liver fibrosis via granzyme-induced apoptosis and IFN-γ secretion (62, 63). NK cells are fundamental for the proper protection of the liver and aberrant functions have been reported in several liver diseases. Over the past decade, studies on NK cells suggest very heterogeneous populations with distinctive transcriptomes and cellular interactions (64).
Liver inflammation is the first step to resolving and healing from different hepatocellular stress. When not effective, inflammation can become pathogenic. Hepatitis is a hallmark of liver disease (65) (Figure 3). It is important to identify which cells are precursors of early liver inflammation to avoid unnecessary harm. A recent report highlights the importance of the inflammasome in early inflammation (66). KCs express a variety of pathogen recognition receptors (PRRs) to cover a wide range of dangers. Some of these dangers overactivate the inflammasome, which triggers pyroptosis, a form of cell death accompanied by cell membrane rupture and release of pro-inflammatory IL-1β and IL-18 (67). These cytokines are responsible for the recruitment and activation of innate immune cells (68, 69). The direct cytotoxic and effector functions of innate immune cells can restore homeostasis. However, innate immune cells can also have early involvement in disease processes when the danger is not resolved (e.g. chronic viral infection) or because of repeated insults (e.g. alcohol or drug abuse) (Supplementary Table 1) . Innate immune cells can also recruit other immune cells from the liver and peripheral circulation. Overall, innate immune cells are suggested to be the precursors of the inflammatory niche because of their optimal location, preactivated state, enrichment in the liver and strong effector functions.
Hepatotropic viruses such as hepatitis A, B, C, D and E (HAV, HBV, HCV, HDV and HEV) possess mechanisms to escape from the hosts´ antiviral immunity. When the viruses replicate, often the innate immunity detects viral components, hence triggering an acute inflammatory response resulting in the killing of infected hepatocytes. Since the infection is not properly resolved, viruses remain in a latent state and replicate opportunistically. This progressively leads to chronic liver inflammation (70). In particular, HBV and HCV are the main causes of chronic liver disease and are estimated to affect 257 million (data from WHO 2015) and 115 million people (71), respectively. Together they represent the most common cause of liver cirrhosis, liver cancer and viral hepatitis-related deaths (72).
HBV is directly mutagenic and induces low-grade inflammation progressing into HCC (73). HBV-infected hepatocytes release PAMPs such as glycoproteins, secreted HBsAg or free viral nucleic acids that are recognized by the innate immune system. Human KCs release pro-inflammatory cytokines to orchestrate an antiviral response which also arrests hepatocyte replication, hence viral replication (74). Studies in mice demonstrated the antiviral roles of NK cells and NKT cells (75, 76). HBV patients present higher levels of NK cells in blood compared to HBV-negative controls (77, 78) and are deemed as the major contributors to HBV clearance (79). A positive correlation was found between NK cell activation levels and HBV clearance (79). NK and NKT cell numbers from peripheral blood correlated to the frequency of HBcAg-specific cytotoxic T lymphocytes (CTLs) (80). However, infiltration of circulating NK cells can contribute to liver injury (81). NK cells from HBV patients produced higher levels of TNF-α and induced in vitro expression of TRAIL in hepatocytes (82). This study showed that infiltrated circulating NK cells could induce apoptosis of non-infected hepatocytes via TRAIL (82). Additional studies in mice and patients show that NK cells could also exacerbate liver injury via TNF-α, Fas/FasL and NKG2D/NKG2DL pathways (83, 84). NKT cells and KCs secrete induced nitric oxide synthase (iNOS) as a viral eradication mechanism (85, 86). Moreover, the frequency of NKT cells was increased to normal values with virus clearance (80). These results suggest that circulating NK cells and NKT cells are recruited in the liver causing a reduction in their frequencies in blood. In contrast, peripheral MAIT cells were significantly decreased in HBV-related liver failure patients compared with chronic HBV patients (87). The study suggested that MAIT cells are recruited in the liver and promote a strong inflammatory response damaging the liver. MAIT cells were also reduced in patients with middle/late-stage compared with early-stage liver failure (87). Similar to NK cells and NKT cells, patients that showed disease improvement had an increment in the frequency of MAIT cells (87). In another two studies exploring changes in peripheral γδ T cells in HBV patients, γδ T cells were less abundant in liver failure patients and correlated with disease severity (88). Activation of γδ T cells with PMA/Ionomycin induced the greatest amount of pro-inflammatory TNF-α and IL-17 in liver failure patients (89). However, another study indicated that γδ2 T cells exhibited impaired proliferation and chemotaxis (90). The same study showed in vitro that γδ2 T cells inhibit Th17 T cells through cell-to-cell contact and produce high amounts of IFN-γ (90). These results suggest that NK cells and NKT cells are the first-line of defense against HBV infection. Failing to clear the infection, MAIT cells and γδ T cells contribute to chronic inflammation. IFN-α therapy is effective in 20-30% of chronic HBV patients (91). The low response rates may be attributed to the wide spectrum of different clinical conditions. Based on the current understanding of the role of NK cells in HBV clearance, IFN-α is likely to improve the cytotoxic function of liver-resident NK cells by targeting HSC cells and reduce fibrosis (92). It is necessary to investigate whether IFN-α therapy response is subjected to the frequency of circulating NK cell infiltration.
HCV-induced inflammation is partly triggered by non-structural proteins of the virus (93) but the major contributor to HCV-hepatitis are the inflammatory immune cells. In vitro studies show that HCV-infected hepatocytes produce several pro-inflammatory cytokines including IL-6, IL-8, MIP-1α and MIP-1β as a response to IL-1β secreted by HSCs (94) or IL-1β and TNF-α by KCs (95). Similar to HBV infection, human circulating MAIT cells were generally reported to be depleted with markers of exhaustion and hyperactivation (96–98). Additional studies suggest that hepatic MAIT cells are major contributors to hepatitis and fibrosis given the nature of the cells. Repetitive IL-12 stimulation or IL-7 secretion by hepatocytes was a sufficient stimulus to induce secretion of the pro-inflammatory cytokines IFN-γ, TNF-α and IL-17 (7, 26). Intrahepatic γδ T cells were shown to be cytotoxic against human hepatocytes in culture (99). We have recently identified a subset of CD8+ γδ T cells that were more abundant in baseline peripheral blood of melanoma patients that had hepatitis after ICI therapy versus non-hepatitis cohort. ICI therapy might induce γδ T cells cytotoxic activity against hepatocytes as observed in HCV infection. NK cells were shown to be compromised in HCV patients allowing the virus to replicate (78, 100). IFN-α therapy induced activation of NK cells and further improved the clearance of the virus (101). NKT cells were also reported to play a role in HCV resolution and progression. The frequency of activated CD38+ or CD69+ iNKT cells strongly correlated with alanine transaminase levels (102). Increased levels of activated iNKT cells were observed during acute inflammation and chronic HCV infection without apparent functional differences (102). The frequency of activated iNKT cells declined spontaneously in resolving patients (102). These data suggest that HCV infection could be mainly managed by NK and NKT cells. Viral clearance also involves other ILC-like cells such as MAIT cells and γδ T cells. Under inflammatory conditions, host hepatocytes switch to an antiviral state to prevent further viral replication. If the infection is not properly resolved, we propose a model where NK cells and MAIT cells have an exhausted phenotype while iNKT cells and γδ T cells promote pathogenesis by targeting infected hepatocytes.
The liver is vital for the detoxification of substances that are harmful to the body. Liver detoxification consists mainly of converting ingested drugs into water-soluble metabolites via xenobiotic biotransforming enzymes (103). This allows drugs to be efficiently secreted through urine. However, in an attempt to solubilize drugs, some compounds are converted into their active form. Acetaminophen, also known as paracetamol, leads to reactive metabolites causing apoptosis and necrosis of hepatocytes (104). In the case of alcohol, free radicals and acetaldehyde are harmful by-products that can lead to significant liver damage over time. Drugs and alcohol can also damage the intestine barrier leading to more bacteria translocation to the bloodstream (105, 106). The influx of gut microbiota and its metabolites activate the immune system through PAMPs and DAMPs (107–110). KCs were reported to be major contributors to the development of alcohol-related liver disease (ALD). Intestine permeability is directly associated with KC activation (111, 112). Exposing mice to LPS and alcohol-derived reactive oxygen species (ROS) has shown to induce TNF-α secretion by KCs (113, 114). In a paracrine manner, IL-1ß secretion by KCs had a significant effect on the pathological progression of ALD (115). A rat model of ALD with depletion of KCs resulted in impaired progression of the pathology suggesting a key role of KCs (116). NK cells were less frequent in alcoholic patients (117) and were less cytotoxic compared to healthy individuals (118). A reduced expression of the activating receptor NKG2D and production of IFN-γ in mice suggests that NK cells cannot efficiently kill activated HSCs (119). Chronic ethanol feeding in mice increased CD1d by enterocytes (120). Similarly, patients affected by alcohol misuse also show increased expression of CD1d in the small intestine (120). An in vitro study showed that CD1d increased the loading of αGalCer following increasing concentrations of ethanol and thus, could increase stimulation of iNKT cells (121). Many studies in mice suggest that iNKT cells have a pathogenic role in the development of ALD. It was reported that iNKT cells crosstalk with KCs through IL-1β, promote inflammation and recruit neutrophils (122, 123). CD1d blocking antibodies could partially prevent liver injury (123). Intestinal iNKT cells were observed to migrate to the liver and, collectively with liver iNKT cells, showed a chronic activated phenotype with downregulation of TCR, increased apoptosis and FasL expression (120). In vitro experiments from the same study confirmed that iNKT cells could kill hepatocytes via Fas-FasL mechanism (120). Activation of T2NKT cells by sulfatide inhibited iNKT cell hepatic damage (124, 125). In a concanavalin A-induced hepatitis mouse model, injection of lysophosphatidylcholine (LPC) activated T2NKT cells and prevented liver injury by iNKT cells (125). Another study described the crosstalk of T2NKT cells with plasmacytoid dendritic cells and recruitment of anergic iNKT cells to the mouse liver via IL-12 and MIP-2 (126). As mentioned above, our group recently identified a novel population of human FoxP3+ T2NKT cells that might exert immunoregulatory functions in this scenario (40). Alcoholic-related cirrhosis and severe alcoholic hepatitis patients had a dramatic depletion and hyperactivated circulating MAIT cells (127, 128). Dysfunctional MAIT cells could explain the susceptibility to infection of these patients (127, 128). In another study, MAIT cells had an exhausted phenotype and partially recovered with patient´s alcohol abstinence (129). MAIT cells may contribute to the pathogenesis of ALD via IL-17 secretion (129). Surprisingly, only a few reports have described the role of γδ T cells in ALD. In a mouse study following binge ethanol drinking, γδ T cells were described to produce higher amounts of IL-17A than non-binge ethanol-drinking mice (130). The activation of γδ T cells was IL-1ß-dependent, possibly by KCs (130). However, under acute-on-chronic ethanol consumption, γδ T cells did not produce further IL-17A. Instead, CD4+ T cells were the major contributors. This suggests that KCs could play a predominant role in the development of ALD. KCs orchestrate an inflammatory response that involves pro-inflammatory iNKT cells and γδ T cells. Alcohol could directly affect MAIT cells and NK cells causing depletion and impaired functions such as the inactivation of HSCs by NK cells, and tissue repair by MAIT cells.
Non-alcoholic fatty liver disease (NAFLD), characterized by an excessive accumulation of fat in hepatocytes, is the most common indication for liver transplant in Western countries and the leading cause of liver transplantation in women (131, 132). It is estimated that 23-25% of the global population have NAFLD to some degree (133). Etiologically, it is suggested that the adipose tissue from patients with NAFLD predisposition release free fatty acids (FFA) and pro-inflammatory mediators into the circulation (134, 135). As a result, an inflammatory response is triggered in the liver. Lipotoxicity, mitochondrial dysfunction and endoplasmic reticulum stress are key inducers of the inflammatory cascade (136). Higher frequencies of KCs were observed in liver biopsies of non-alcoholic steatohepatitis (NASH) patients (137). Depletion of KCs in rats exposed to a high-fat diet (HFD) prevented the development of steatosis (138). In vitro experiments showed that TNF-α was responsible for the increased accumulation and the reduced oxidation of fatty acids in hepatocytes (139). Immunohistological stainings revealed a complex crown-like structure consisting of KCs surrounding dying steatotic hepatocytes. Cholesterol crystals are accumulated in the center of these structures (140). Interestingly, previous exposure of KCs to cholesterol crystals showed to precondition the cells towards a pro-inflammatory innate memory-like state (141). Similar observations were taken from macrophages cultured with oxidized low-density lipoproteins (142). Likewise to the effect of alcohol, NK cells of obese individuals had lower NKG2D expression (143) and impaired cytotoxicity (144, 145). Another study showed that there were no differences between NK cells from healthy individuals and NAFLD, while higher expression of NKG2D in NK cells was found in NASH patients (146). Data from mice and humans suggest that iNKT cells have a dual role in NAFLD. More specifically, it is hypothesized that iNKT cells have a protective role during early stages of simple steatosis. In different mouse models of hepatosteatosis, like ob/ob mice, animals fed with HFD or a choline-deficient diet, iNKT cells were apoptotic and showed decreased intrahepatic frequency (147–149). Adoptive transfer of hepatic mononuclear cells but not CD1d-/- mononuclear cells regulated hepatic steatosis via IL-10 (150). However, in other instances, opposite results were reported. Mice fed with HFD developed adipose tissue inflammation and glucose intolerance (151). This was significantly exacerbated by αGalCer-dependent activation of iNKT cells (151). In the liver, iNKT cells could be directly activated via hepatic CD1d molecules, exacerbate steatosis and decrease insulin sensitivity by promoting a pro-inflammatory cytokine environment (152). This could suggest that iNKT cells play a protective role during early stages of simple steatosis but exacerbate the disease in chronic steatosis. It would also be interesting to study the potential effect of iNKT cell migration from tissues like the intestines as discussed earlier. T2NKT cells might also play dual roles. In HFD mice, T2NKT cells initiate inflammation in the liver and adipose tissue and promote obesity and insulin resistance (153). However, adoptive transfer of T2NKT cells in HFD obese mice induced prolonged weight loss and glucose tolerance (154). The heterogeneity and impact of fat in intrahepatic T2NKT cell populations remains unclear. The frequency of human NKT cells is decreased in steatosis (155) but increased accordingly to the progression of NAFLD, especially IFN-γ+ and IL-4+ cells (156–158). NASH patients had a 4-5 fold relative increase in liver NKT cells (158). CD1d expression was reported to be increased in liver immunohistochemical samples of NAFLD and correlated with disease progression (156). Taken together, NKT cells are reduced in the early stages of simple steatosis. A pro-inflammatory response is protective against obesity. In advanced NAFLD, NKT cells are increased and pathogenic. Circulating MAIT cell frequency was reported to decrease while the number of intrahepatic MAIT cells was increased in NAFLD patients’ livers and it tended to be greater with disease progression (159). MAIT cells from NAFLD patients had increased secretion of IL-4 and reduced expression of IFN-γ and TNF-α (159). The current knowledge about the role of γδ T cells in NAFLD is mostly based on mice models. γδ T cells can recognize molecules presented by CD1d and its differentiation is dependent on hepatocyte CD1d (160). γδ T cells are high producers of IL-17A in steatohepatitis (161), a key cytokine known to induce fibrosis and ROS production (162, 163). In HFD mice, IL-17+ γδ T cells are elevated (164). Additionally, adoptive transfer and gene knockout experiments in HFD mice demonstrated that γδ T cells exacerbate steatohepatitis and liver damage (160, 161). In humans, NAFLD patients showed decreased frequencies of Vδ2+ T cells, but elevated frequencies of Vδ2- T cells compared to healthy controls (143). Overall, the progression of NAFLD to NASH is a process derived from the increased cellular oxidative stress that leads to the activation of inflammatory pathways (165). Accumulation of ROS induces the expression of TNF-α which can trigger necrotic cell death (166). In line with these results, NK cells were suppressed by ROS (167). KCs develop an apparent pro-inflammatory immune memory state by contact with cholesterol crystals. γδ T cells promote pathogenesis through IL-17 secretion, while NKT cells and MAIT cells exacerbate steatosis by secretion of Th2 cytokines which also contributes to fibrosis (168).
The three main autoimmune liver diseases are autoimmune hepatitis (AIH), primary biliary cirrhosis (PBC) and primary sclerosing cholangitis (PSC). AIH affects portal tracts and liver lobules by lymphoplasmacytic infiltrates while PSC and PBC mainly affect bile ducts. The etiologies of these diseases are yet unknown, but several studies suggest a common immune-mediated liver injury. The dysregulation of immune regulatory networks causes the activation and expansion of autoreactive T cells and B cells (169, 170). The innate system plays an important role in the regulation of the adaptive system. In AIH, an increased frequency of cytotoxic circulating NK cells in the liver was observed in an experimental mouse model of AIH (171). In humans, the frequency of circulating CD56bright NK cells was higher in untreated AIH, while the frequency of circulating CD56dim NK cells was reported to be reduced in active AIH patients or while in remission (171, 172). Our knowledge about NKT cells in liver autoimmunity is mainly based on mouse models. In AIH, concanavalin-induced hepatitis is the preferred model. iNKT cells were reported to upregulate FasL expression to mediate cytotoxicity against hepatocytes (173). Activation of iNKT cells via α-GalCer exacerbates the disease and is suggested to be carried out via IL-4 and TNF-α secretion (174, 175). Inflammation was also promoted via the secretion of IL-17 (176). MAIT cells were reported to be depleted and exhausted in the periphery in patients (177). Chronic stimulation of MAIT cells due to an increased influx of bacteria antigens and chronic inflammation may lead to MAIT cell function impairment. Induction of the exhausted state by repetitive stimulation with IL-12 and IL-18 showed that MAIT cells reduced IFN-γ production but maintained expression of the proinflammatory cytokine IL-17 (177). The frequency of circulating γδ T cells was increased in patients with AIH, PSC and PBC (8). Vδ1+ T cells, known to produce high levels of IFN-γ and granzyme B, were especially incremented in patients with AIH (178). Another study showed that γδ T cells with low expression of TOX were enriched in AIH patients and had prediction potential (179). TOX deficiency was suggested to promote the expression of IL-17A in γδ T cells (179). In general, IL-17 secretion was reported in iNKT cells, MAIT cells and γδ T cells. Although the clinical profile of the distinctive autoimmune liver diseases is different, current studies support common immunological pathways. Taking for instance the role of circulating NK cells, the frequency of these cells was reported to be increased and a higher expression of cytotoxic molecules such as perforin was found in PBC and PSC patients compared to healthy individuals (180, 181).
Liver transplantation represents a major hepatic injury. One of the unavoidable injuries is caused by oxygen deprivation. After liver resection, blood flow is restricted for a period of time and the organ becomes hypoxic. This leads to different forms of cell death like apoptosis, ferroptosis, pyroptosis and necrosis (182). After reperfusion, innate immune cells from the recipient migrate to the liver and induce inflammation or tolerance (183). The degree of ischemia-reperfusion injury (I/R) is correlated to the risk of liver rejection (184, 185). I/R injury increased the expression of monocyte chemoattractant protein-1 (MCP-1) and it was associated with poorer graft function (186). This observation was correlated with the increased recruitment of monocytes 2 hours after reperfusion (186). The role of NK cells is dependent on activating and inhibitory receptors expressed in hepatocytes as well as cytokines secreted by neighbour cells. In I/R injury, components of the inflammasome in KCs like NLRP3 and AIM2 are hyper-activated (187, 188). Inflammasome-derived IL-18 secretion can induce FasL (189) and IFN-γ production in NK cells (190). IFN-γ was reported to induce expression of Fas receptor in hepatocytes and neutralization of IFN-γ secretion by NK cells could protect mice from tissue damage (191). Due to the increased demand for livers and the increasing prevalence of NAFLD, the debate of using steatotic livers for transplant is on the table (192). Steatosis is deemed to cause oxidative stress in the liver, which worsens the graft´s condition with I/R injury. In a retrospective, exploratory study, steatotic livers showing signs of I/R had a significantly worse one-year survival rate, while the survival rate was not conditioned in healthy livers´ by I/R injury (193). In this study, γδ T cells were suggested to exacerbate liver rejection in steatotic livers (193). NKT cells were reported to promote I/R injury. After reperfusion, NKT cells rapidly expand in the liver and produce IFN-γ (194, 195). Depletion of NKT cells with antibodies or both NKT cells and NK cells significantly reduced I/R injury (196). The role of MAIT cells in liver I/R injury remains to be elucidated. In focal cerebral ischemia, MAIT cells were reported to play a pro-inflammatory role (197).
Cancer immunotherapies, especially immune checkpoint inhibitor (ICI) therapy, have opened new clinical perspectives for cancer patients and is fast becoming one of the main pillars of cancer treatment. ICI therapy uses monoclonal antibodies blocking T cell receptors that are used by cancer cells to evade the immune system. Immune-related adverse events (irAEs) are the result of immune activation derived from ICI therapy. The incidence of ICI-derived hepatitis is approximately 1-3% for programmed cell death 1 (PD1) inhibitors and 3-9% in cytotoxic T-lymphocyte-associated protein 4 (CTLA4) inhibitors (198). The combination of α-PD1/CTLA4 increases the rate of hepatitis (198). CTLA4 plays an important role in downregulating the immune response. The expression of CTLA4 is upregulated in T cells after activation and competes with the costimulatory receptor CD28 to bind to its ligand CD80/CD86 on APC (199). PD-1 is expressed on T cells and B cells and it promotes self-tolerance. Upon binding to its ligand PD-L1, it drives T cell apoptosis or regulatory phenotype. Thus, ICI therapy can arguably impair liver immunotolerance. In acute liver injury, α-PD1 therapy improved the bacterial clearance function of KCs (200). A study treating melanoma patients with α-PD1 showed that NK cell frequency in blood was not affected while NKT frequency was significantly increased (201). Another study observed no changes in either the number or function of MAIT cells in melanoma patients treated with α-PD1 therapy (202). γδ T cells showed no apparent functional changes upon PD-1 blockade in vitro (203). The frequency of γδ T cells in melanoma patients treated with a combination of α-PD1/CTLA4 remained unchanged (204). Overall, these data suggest that innate immune cells are not drastically affected by ICI therapies, with the exception of KCs and NKT cells. Immune-suppressive KCs expresses PD-1 to suppress T lymphocytes in acute liver injury (200). α-PD-1 therapy has shown to invigorate bacteria clearance, but it also suggests that KCs may have impaired tolerogenic function to self-antigens reactive T cells. NKT cells also responded to α-PD-1 therapy and exert increased anti-tumor functions by secretion of IFN- γ secretion of inflammatory cytokines (205).
The innate immune system is also involved in immune homeostasis and healthy tissue turnover. This is accomplished via three steps consisting of early inflammation, amplification of the inflammatory signal and resolution. Liver fibrosis is a consequence of inflammation and inefficient resolution. Liver biopsy is the gold standard for diagnosing cirrhotic liver disease, yet it is estimated to miss 10-30% of cases (169). Additionally, biopsy is not ideal because of invasiveness, pain, hypertension and bleeding (206). An optimal approach would be to identify early inflammation before fibrosis development. This could improve patient’s treatment and prognosis. Blood markers bring promising perspectives to detect liver damage and abnormal functions (207). The current scoring system for diagnosis and prognosis of fibrosis includes serum proteins (albumin), bilirubin, liver enzymes (aminotransferases, alkaline phosphatase, γ-glutamyl transferase) and direct markers of extracellular matrix turnover (type IV collagen, matrix metalloproteinases). However, there is room for improvement regarding specificity (etiology) and sensitivity (disease stages) (206). The immune system has emerged as an interesting diagnostic and therapeutic target in liver inflammation. Innate immune cells are the frontline defenders in the liver and participate in the initiation, amplification and resolution of inflammation. Identifying immune changes in innate immune cell´s surface expression markers and frequencies can bring future perspective to the diagnosis of low-grade inflammation and also novel therapies. As discussed in this review, depletion of innate immune cells in mice models with hepatitis was able to attenuate several liver diseases. Noteworthy, the close relationship between innate immune cells with DAMPs and cytokines signaling suggests taking into consideration all three factors for the future of liver immunomonitoring and therapies.
JYZ performed literature search and wrote the manuscript. The author confirms being the sole contributor of this work and has approved it for publication.
We are very grateful to Dieter Pirner from the Department of Surgery, University Hospital Regensburg, for his contribution to the design of the illustrations.
The author declares that the research was conducted in the absence of any commercial or financial relationships that could be construed as a potential conflict of interest.
All claims expressed in this article are solely those of the authors and do not necessarily represent those of their affiliated organizations, or those of the publisher, the editors and the reviewers. Any product that may be evaluated in this article, or claim that may be made by its manufacturer, is not guaranteed or endorsed by the publisher.
The Supplementary Material for this article can be found online at: https://www.frontiersin.org/articles/10.3389/fimmu.2023.1175147/full#supplementary-material
Supplementary Table 1 | Innate immune cells in liver inflammation.
1. Wisse E, Braet F, Luo D, deZanger R, Jans D, Crabbé E, et al. Structure and function of sinusoidal lining cells in the liver. Toxicol Pathol (1996) 24:100–11. doi: 10.1177/019262339602400114
2. Wake K, Sato T. “The sinusoid” in the liver: lessons learned from the original definition by Charles Sedgwick Minot (1900). Anat Rec (Hoboken) (2015) 298:2071–80. doi: 10.1002/ar.23263
3. Ben-Moshe S, Itzkovitz S. Spatial heterogeneity in the mammalian liver. Nat Rev Gastroenterol Hepatol (2019) 16:395–410. doi: 10.1038/s41575-019-0134-x
4. McEnerney L, Duncan K, Bang B-R, Elmasry S, Li M, Miki T, et al. Dual modulation of human hepatic zonation via canonical and non-canonical wnt pathways. Exp Mol Med (2017) 49:e413. doi: 10.1038/emm.2017.226
5. Cheng ML, Nakib D, Perciani CT, MacParland SA. The immune niche of the liver. Clin Sci (Lond) (2021) 135:2445–66. doi: 10.1042/CS20190654
6. Dusseaux M, Martin E, Serriari N, Péguillet I, Premel V, Louis D, et al. Human MAIT cells are xenobiotic-resistant, tissue-targeted, CD161hi IL-17-secreting T cells. Blood (2011) 117:1250–9. doi: 10.1182/blood-2010-08-303339
7. Tang X-Z, Jo J, Tan AT, Sandalova E, Chia A, Tan KC, et al. IL-7 licenses activation of human liver intrasinusoidal mucosal-associated invariant T cells. J Immunol (2013) 190:3142–52. doi: 10.4049/jimmunol.1203218
8. Doherty DG. Immunity, tolerance and autoimmunity in the liver: A comprehensive review. J Autoimmun (2016) 66:60–75. doi: 10.1016/j.jaut.2015.08.020
9. Freitas-Lopes MA, Mafra K, David BA, Carvalho-Gontijo R, Menezes GB. Differential location and distribution of hepatic immune cells. Cells (2017) 6: 48. doi: 10.3390/cells6040048
10. Wehr A, Baeck C, Heymann F, Niemietz PM, Hammerich L, Martin C, et al. Chemokine receptor CXCR6-dependent hepatic NK T cell accumulation promotes inflammation and liver fibrosis. J Immunol (2013) 190:5226–36. doi: 10.4049/jimmunol.1202909
11. Hammerich L, Tacke F. Role of gamma-delta T cells in liver inflammation and fibrosis. World J Gastrointest Pathophysiol (2014) 5:107–13. doi: 10.4291/wjgp.v5.i2.107
12. Jeffery HC, van Wilgenburg B, Kurioka A, Parekh K, Stirling K, Roberts S, et al. Biliary epithelium and liver b cells exposed to bacteria activate intrahepatic MAIT cells through MR1. J Hepatol (2016) 64:1118–27. doi: 10.1016/j.jhep.2015.12.017
13. Sitia G, Iannacone M, Aiolfi R, Isogawa M, van Rooijen N, Scozzesi C, et al. Kupffer cells hasten resolution of liver immunopathology in mouse models of viral hepatitis. PloS Pathog (2011) 7:e1002061. doi: 10.1371/journal.ppat.1002061
14. Dou L, Shi X, He X, Gao Y. Macrophage phenotype and function in liver disorder. Front Immunol (2019) 10:3112. doi: 10.3389/fimmu.2019.03112
15. Ju C, Tacke F. Hepatic macrophages in homeostasis and liver diseases: from pathogenesis to novel therapeutic strategies. Cell Mol Immunol (2016) 13:316–27. doi: 10.1038/cmi.2015.104
16. Tran S, Baba I, Poupel L, Dussaud S, Moreau M, Gélineau A, et al. Impaired kupffer cell self-renewal alters the liver response to lipid overload during non-alcoholic steatohepatitis. Immunity (2020) 53:627–640.e5. doi: 10.1016/j.immuni.2020.06.003
17. Heymann F, Hammerich L, Storch D, Bartneck M, Huss S, Rüsseler V, et al. Hepatic macrophage migration and differentiation critical for liver fibrosis is mediated by the chemokine receptor c-c motif chemokine receptor 8 in mice. Hepatology (2012) 55:898–909. doi: 10.1002/hep.24764
18. Karlmark KR, Weiskirchen R, Zimmermann HW, Gassler N, Ginhoux F, Weber C, et al. Hepatic recruitment of the inflammatory Gr1+ monocyte subset upon liver injury promotes hepatic fibrosis. Hepatology (2009) 50:261–74. doi: 10.1002/hep.22950
19. Mossanen JC, Krenkel O, Ergen C, Govaere O, Liepelt A, Puengel T, et al. Chemokine (C-c motif) receptor 2-positive monocytes aggravate the early phase of acetaminophen-induced acute liver injury. Hepatology (2016) 64:1667–82. doi: 10.1002/hep.28682
20. Krenkel O, Tacke F. Liver macrophages in tissue homeostasis and disease. Nat Rev Immunol (2017) 17:306–21. doi: 10.1038/nri.2017.11
21. Dixon LJ, Barnes M, Tang H, Pritchard MT, Nagy LE. Kupffer cells in the liver. Compr Physiol (2013) 3:785–97. doi: 10.1002/cphy.c120026
22. Cowley SC. MAIT cells and pathogen defense. Cell Mol Life Sci (2014) 71:4831–40. doi: 10.1007/s00018-014-1708-y
23. Gao B, Ma J, Xiang X. MAIT cells: a novel therapeutic target for alcoholic liver disease? Gut (2018) 67:784–6. doi: 10.1136/gutjnl-2017-315284
24. Lamichhane R, Munro F, Harrop TWR, deLa Harpe SM, Dearden PK, Vernall AJ, et al. Human liver-derived MAIT cells differ from blood MAIT cells in their metabolism and response to TCR-independent activation. Eur J Immunol (2021) 51:879–92. doi: 10.1002/eji.202048830
25. Gracey E, Qaiyum Z, Almaghlouth I, Lawson D, Karki S, Avvaru N, et al. IL-7 primes IL-17 in mucosal-associated invariant T (MAIT) cells, which contribute to the Th17-axis in ankylosing spondylitis. Ann Rheum Dis (2016) 75:2124–32. doi: 10.1136/annrheumdis-2015-208902
26. Mehta H, Lett MJ, Klenerman P, Filipowicz Sinnreich M. MAIT cells in liver inflammation and fibrosis. Semin Immunopathol (2022) 44:429–44. doi: 10.1007/s00281-022-00949-1
27. Lett MJ, Mehta H, Keogh A, Jaeger T, Jacquet M, Powell K, et al. Stimulatory MAIT cell antigens reach the circulation and are efficiently metabolised and presented by human liver cells. Gut (2022) 71:2526–38. doi: 10.1136/gutjnl-2021-324478
28. Shiromizu CM, Jancic CC. γδ T lymphocytes: An effector cell in autoimmunity and infection. Front Immunol (2018) 9:2389. doi: 10.3389/fimmu.2018.02389
29. Toulon A, Breton L, Taylor KR, Tenenhaus M, Bhavsar D, Lanigan C, et al. A role for human skin-resident T cells in wound healing. J Exp Med (2009) 206:743–50. doi: 10.1084/jem.20081787
30. Deusch K, Moebius U, Zum Meyer Büschenfelde KH, Meuer SC. T Lymphocyte control of autoreactivity: analysis with human T cell clones and limiting dilution culture. Eur J Immunol (1986) 16:1433–8. doi: 10.1002/eji.1830161119
31. Luoma AM, Castro CD, Adams EJ. γδ T cell surveillance via CD1 molecules. Trends Immunol (2014) 35:613–21. doi: 10.1016/j.it.2014.09.003
32. Davey MS, Willcox CR, Hunter S, Kasatskaya SA, Remmerswaal EBM, Salim M, et al. The human Vδ2+ T-cell compartment comprises distinct innate-like Vγ9+ and adaptive Vγ9- subsets. Nat Commun (2018) 9:1760. doi: 10.1038/s41467-018-04076-0
33. Park JH, Lee HK. Function of γδ T cells in tumor immunology and their application to cancer therapy. Exp Mol Med (2021) 53:318–27. doi: 10.1038/s12276-021-00576-0
34. Feurle J, Espinosa E, Eckstein S, Pont F, Kunzmann V, Fournié J-J, et al. Escherichia coli produces phosphoantigens activating human gamma delta T cells. J Biol Chem (2002) 277:148–54. doi: 10.1074/jbc.M106443200
35. Blazquez J-L, Benyamine A, Pasero C, Olive D. New insights into the regulation of γδ T cells by BTN3A and other BTN/BTNL in tumor immunity. Front Immunol (2018) 9:1601. doi: 10.3389/fimmu.2018.01601
36. Harly C, Guillaume Y, Nedellec S, Peigné C-M, Mönkkönen H, Mönkkönen J, et al. Key implication of CD277/butyrophilin-3 (BTN3A) in cellular stress sensing by a major human γδ T-cell subset. Blood (2012) 120:2269–79. doi: 10.1182/blood-2012-05-430470
37. Riquelme P, Haarer J, Kammler A, Walter L, Tomiuk S, Ahrens N, et al. TIGIT+ iTregs elicited by human regulatory macrophages control T cell immunity. Nat Commun (2018) 9:2858. doi: 10.1038/s41467-018-05167-8
38. Mangan BA, Dunne MR, O’Reilly VP, Dunne PJ, Exley MA, O’Shea D, et al. Cutting edge: CD1d restriction and Th1/Th2/Th17 cytokine secretion by human Vδ3 T cells. J Immunol (2013) 191:30–4. doi: 10.4049/jimmunol.1300121
39. Papotto PH, Ribot JC, Silva-Santos B. IL-17+ γδ T cells as kick-starters of inflammation. Nat Immunol (2017) 18:604–11. doi: 10.1038/ni.3726
40. Yang Zhou J, Werner JM, Glehr G, Geissler EK, Hutchinson JA, Kronenberg K. Identification and isolation of type II NKT cell subsets in human blood and liver. Front Immunol (2022) 13:898473. doi: 10.3389/fimmu.2022.898473
41. Bayatipoor H, Mehdizadeh S, Jafarpour R, Shojaei Z, Pashangzadeh S, Motallebnezhad M. Role of NKT cells in cancer immunotherapy-from bench to bed. Med Oncol (2022) 40:29. doi: 10.1007/s12032-022-01888-5
42. Dasgupta S, Kumar V. Type II NKT cells: a distinct CD1d-restricted immune regulatory NKT cell subset. Immunogenetics (2016) 68:665–76. doi: 10.1007/s00251-016-0930-1
43. Borg NA, Wun KS, Kjer-Nielsen L, Wilce MCJ, Pellicci DG, Koh R, et al. CD1d-lipid-antigen recognition by the semi-invariant NKT T-cell receptor. Nature (2007) 448:44–9. doi: 10.1038/nature05907
44. Bricard G, Venkataswamy MM, Yu KOA, Im JS, Ndonye RM, Howell AR, et al. A-galactosylceramide analogs with weak agonist activity for human iNKT cells define new candidate anti-inflammatory agents. PloS One (2010) 5:e14374. doi: 10.1371/journal.pone.0014374
45. Takahashi T, Suzuki T. Role of sulfatide in normal and pathological cells and tissues. J Lipid Res (2012) 53:1437–50. doi: 10.1194/jlr.R026682
46. Renukaradhya GJ, Khan MA, Vieira M, Du W, Gervay-Hague J, Brutkiewicz RR. Type I NKT cells protect (and type II NKT cells suppress) the host’s innate antitumor immune response to a b-cell lymphoma. Blood (2008) 111:5637–45. doi: 10.1182/blood-2007-05-092866
47. Terabe M, Berzofsky JA. The immunoregulatory role of type I and type II NKT cells in cancer and other diseases. Cancer Immunol Immunother (2014) 63:199–213. doi: 10.1007/s00262-013-1509-4
48. Rhost S, Sedimbi S, Kadri N, Cardell SL. Immunomodulatory type II natural killer T lymphocytes in health and disease. Scand J Immunol (2012) 76:246–55. doi: 10.1111/j.1365-3083.2012.02750.x
49. Sedimbi SK, Hägglöf T, Garimella MG, Wang S, Duhlin A, Coelho A, et al. Combined proinflammatory cytokine and cognate activation of invariant natural killer T cells enhances anti-DNA antibody responses. Proc Natl Acad Sci U S A (2020) 117:9054–63. doi: 10.1073/pnas.1920463117
50. Mikulak J, Bruni E, Oriolo F, Di Vito C, Mavilio D. Hepatic natural killer cells: Organ-specific sentinels of liver immune homeostasis and physiopathology. Front Immunol (2019) 10:946. doi: 10.3389/fimmu.2019.00946
51. Cai L, Zhang Z, Zhou L, Wang H, Fu J, Zhang S, et al. Functional impairment in circulating and intrahepatic NK cells and relative mechanism in hepatocellular carcinoma patients. Clin Immunol (2008) 129:428–37. doi: 10.1016/j.clim.2008.08.012
52. Marquardt N, Béziat V, Nyström S, Hengst J, Ivarsson MA, Kekäläinen E, et al. Cutting edge: identification and characterization of human intrahepatic CD49a+ NK cells. J Immunol (2015) 194:2467–71. doi: 10.4049/jimmunol.1402756
53. Hudspeth K, Donadon M, Cimino M, Pontarini E, Tentorio P, Preti M, et al. Human liver-resident CD56(bright)/CD16(neg) NK cells are retained within hepatic sinusoids via the engagement of CCR5 and CXCR6 pathways. J Autoimmun (2016) 66:40–50. doi: 10.1016/j.jaut.2015.08.011
54. Stegmann KA, Robertson F, Hansi N, Gill U, Pallant C, Christophides T, et al. CXCR6 marks a novel subset of T-bet(lo)Eomes(hi) natural killer cells residing in human liver. Sci Rep (2016) 6:26157. doi: 10.1038/srep26157
55. Cuff AO, Robertson FP, Stegmann KA, Pallett LJ, Maini MK, Davidson BR, et al. Eomeshi NK cells in human liver are long-lived and do not recirculate but can be replenished from the circulation. J Immunol (2016) 197:4283–91. doi: 10.4049/jimmunol.1601424
56. Peng H, Wisse E, Tian Z. Liver natural killer cells: subsets and roles in liver immunity. Cell Mol Immunol (2016) 13:328–36. doi: 10.1038/cmi.2015.96
57. Tang L, Peng H, Zhou J, Chen Y, Wei H, Sun R, et al. Differential phenotypic and functional properties of liver-resident NK cells and mucosal ILC1s. J Autoimmun (2016) 67:29–35. doi: 10.1016/j.jaut.2015.09.004
58. Daussy C, Faure F, Mayol K, Viel S, Gasteiger G, Charrier E, et al. T-Bet and eomes instruct the development of two distinct natural killer cell lineages in the liver and in the bone marrow. J Exp Med (2014) 211:563–77. doi: 10.1084/jem.20131560
59. Sojka DK, Plougastel-Douglas B, Yang L, Pak-Wittel MA, Artyomov MN, Ivanova Y, et al. Tissue-resident natural killer (NK) cells are cell lineages distinct from thymic and conventional splenic NK cells. Elife (2014) 3:e01659. doi: 10.7554/eLife.01659
60. Kumar S. Natural killer cell cytotoxicity and its regulation by inhibitory receptors. Immunology (2018) 154:383–93. doi: 10.1111/imm.12921
61. Backes CS, Friedmann KS, Mang S, Knörck A, Hoth M, Kummerow C. Natural killer cells induce distinct modes of cancer cell death: Discrimination, quantification, and modulation of apoptosis, necrosis, and mixed forms. J Biol Chem (2018) 293:16348–63. doi: 10.1074/jbc.RA118.004549
62. Zhang Y, Wu Y, Shen W, Wang B, Yuan X. Crosstalk between NK cells and hepatic stellate cells in liver fibrosis (Review). Mol Med Rep (2022) 25:208. doi: 10.3892/mmr.2022.12724
63. Shi J, Zhao J, Zhang X, Cheng Y, Hu J, Li Y, et al. Activated hepatic stellate cells impair NK cell anti-fibrosis capacity through a TGF-β-dependent emperipolesis in HBV cirrhotic patients. Sci Rep (2017) 7:44544. doi: 10.1038/srep44544
64. Subedi N, Verhagen LP, Bosman EM, van Roessel I, Tel J. Understanding natural killer cell biology from a single cell perspective. Cell Immunol (2022) 373:104497. doi: 10.1016/j.cellimm.2022.104497
65. Guillot A, Tacke F. Liver macrophages: Old dogmas and new insights. Hepatol Commun (2019) 3:730–43. doi: 10.1002/hep4.1356
66. Zhang H, Zahid A, Ismail H, Tang Y, Jin T, Tao J. An overview of disease models for NLRP3 inflammasome over-activation. Expert Opin Drug Discovery (2021) 16:429–46. doi: 10.1080/17460441.2021.1844179
67. Zheng D, Liwinski T, Elinav E. Inflammasome activation and regulation: toward a better understanding of complex mechanisms. Cell Discovery (2020) 6:36. doi: 10.1038/s41421-020-0167-x
68. Kimura K, Sekiguchi S, Hayashi S, Hayashi Y, Hishima T, Nagaki M, et al. Role of interleukin-18 in intrahepatic inflammatory cell recruitment in acute liver injury. J Leukoc Biol (2011) 89:433–42. doi: 10.1189/jlb.0710412
69. Barbier L, Ferhat M, Salamé E, Robin A, Herbelin A, Gombert J-M, et al. Interleukin-1 family cytokines: Keystones in liver inflammatory diseases. Front Immunol (2019) 10:2014. doi: 10.3389/fimmu.2019.02014
70. Diaz O, Vidalain P-O, Ramière C, Lotteau V, Perrin-Cocon L. What role for cellular metabolism in the control of hepatitis viruses? Front Immunol (2022) 13:1033314. doi: 10.3389/fimmu.2022.1033314
71. Platt L, Easterbrook P, Gower E, McDonald B, Sabin K, McGowan C, et al. Prevalence and burden of HCV co-infection in people living with HIV: a global systematic review and meta-analysis. Lancet Infect Dis (2016) 16:797–808. doi: 10.1016/S1473-3099(15)00485-5
72. McGlynn KA, Petrick JL, El-Serag HB. Epidemiology of hepatocellular carcinoma. Hepatology (2021) 73 Suppl 1:4–13. doi: 10.1002/hep.31288
73. Zaki MYW, Fathi AM, Samir S, Eldafashi N, William KY, Nazmy MH, et al. Innate and adaptive immunopathogeneses in viral hepatitis; crucial determinants of hepatocellular carcinoma. Cancers (Basel) (2022) 14:1255. doi: 10.3390/cancers14051255
74. Hösel M, Quasdorff M, Ringelhan M, Kashkar H, Debey-Pascher S, Sprinzl MF, et al. Hepatitis b virus activates signal transducer and activator of transcription 3 supporting hepatocyte survival and virus replication. Cell Mol Gastroenterol Hepatol (2017) 4:339–63. doi: 10.1016/j.jcmgh.2017.07.003
75. Kakimi K, Guidotti LG, Koezuka Y, Chisari FV. Natural killer T cell activation inhibits hepatitis b virus replication in vivo. J Exp Med (2000) 192:921–30. doi: 10.1084/jem.192.7.921
76. Li F, Wei H, Wei H, Gao Y, Xu L, Yin W, et al. Blocking the natural killer cell inhibitory receptor NKG2A increases activity of human natural killer cells and clears hepatitis b virus infection in mice. Gastroenterology (2013) 144:392–401. doi: 10.1053/j.gastro.2012.10.039
77. Arababadi MK, Pourfathollah AA, Jafarzadeh A, Hassanshahi G, Mohit M, Hajghani M, et al. Evaluation of CCR5 expression on NK cells in Iranian patients with occult hepatitis b infection. Lab Med (2010) 41:735–8. doi: 10.1309/LMAUISL84Q4SRSBT
78. Lunemann S, Malone DFG, Hengst J, Port K, Grabowski J, Deterding K, et al. Compromised function of natural killer cells in acute and chronic viral hepatitis. J Infect Dis (2014) 209:1362–73. doi: 10.1093/infdis/jit561
79. Zhao J, Li Y, Jin L, Zhang S, Fan R, Sun Y, et al. Natural killer cells are characterized by the concomitantly increased interferon-γ and cytotoxicity in acute resolved hepatitis b patients. PloS One (2012) 7:e49135. doi: 10.1371/journal.pone.0049135
80. Li J, Han Y, Jin K, Wan Y, Wang S, Liu B, et al. Dynamic changes of cytotoxic T lymphocytes (CTLs), natural killer (NK) cells, and natural killer T (NKT) cells in patients with acute hepatitis b infection. Virol J (2011) 8:199. doi: 10.1186/1743-422X-8-199
81. Zheng Q, Zhu YY, Chen J, Ye YB, Li JY, Liu YR, et al. Activated natural killer cells accelerate liver damage in patients with chronic hepatitis b virus infection. Clin Exp Immunol (2015) 180:499–508. doi: 10.1111/cei.12597
82. Dunn C, Brunetto M, Reynolds G, Christophides T, Kennedy PT, Lampertico P, et al. Cytokines induced during chronic hepatitis b virus infection promote a pathway for NK cell-mediated liver damage. J Exp Med (2007) 204:667–80. doi: 10.1084/jem.20061287
83. Zou Y, Chen T, Han M, Wang H, Yan W, Song G, et al. Increased killing of liver NK cells by Fas/Fas ligand and NKG2D/NKG2D ligand contributes to hepatocyte necrosis in virus-induced liver failure. J Immunol (2010) 184:466–75. doi: 10.4049/jimmunol.0900687
84. Wang Y, Wang W, Shen C, Wang Y, Jiao M, Yu W, et al. NKG2D modulates aggravation of liver inflammation by activating NK cells in HBV infection. Sci Rep (2017) 7:88. doi: 10.1038/s41598-017-00221-9
85. Guidotti LG, McClary H, Loudis JM, Chisari FV. Nitric oxide inhibits hepatitis b virus replication in the livers of transgenic mice. J Exp Med (2000) 191:1247–52. doi: 10.1084/jem.191.7.1247
86. Duwaerts CC, Sun EP, Cheng C-W, van Rooijen N, Gregory SH. Cross-activating invariant NKT cells and kupffer cells suppress cholestatic liver injury in a mouse model of biliary obstruction. PloS One (2013) 8:e79702. doi: 10.1371/journal.pone.0079702
87. Xue H, Li H, Ju L-L, Han X-D, Cheng T-C, Luo X, et al. Mucosal-associated invariant T cells in hepatitis b virus-related liver failure. World J Gastroenterol (2020) 26:4703–17. doi: 10.3748/wjg.v26.i31.4703
88. Chen M, Zhang D, Zhen W, Shi Q, Liu Y, Ling N, et al. Characteristics of circulating T cell receptor gamma-delta T cells from individuals chronically infected with hepatitis b virus (HBV): an association between V(delta)2 subtype and chronic HBV infection. J Infect Dis (2008) 198:1643–50. doi: 10.1086/593065
89. Chen M, Hu P, Ling N, Peng H, Lei Y, Hu H, et al. Enhanced functions of peripheral γδ T cells in chronic hepatitis b infection during interferon α treatment in vivo and in vitro. PloS One (2015) 10:e0120086. doi: 10.1371/journal.pone.0120086
90. Wu X, Zhang J-Y, Huang A, Li Y-Y, Zhang S, Wei J, et al. Decreased Vδ2 γδ T cells associated with liver damage by regulation of Th17 response in patients with chronic hepatitis b. J Infect Dis (2013) 208:1294–304. doi: 10.1093/infdis/jit312
91. Brunetto MR, Bonino F. Interferon therapy of chronic hepatitis b. Intervirology (2014) 57:163–70. doi: 10.1159/000360941
92. Fisicaro P, Rossi M, Vecchi A, Acerbi G, Barili V, Laccabue D, et al. The good and the bad of natural killer cells in virus control: Perspective for anti-HBV therapy. Int J Mol Sci (2019) 20:5080. doi: 10.3390/ijms20205080
93. Núñez O, Fernández-Martínez A, Majano PL, Apolinario A, Gómez-Gonzalo M, Benedicto I, et al. Increased intrahepatic cyclooxygenase 2, matrix metalloproteinase 2, and matrix metalloproteinase 9 expression is associated with progressive liver disease in chronic hepatitis c virus infection: role of viral core and NS5A proteins. Gut (2004) 53:1665–72. doi: 10.1136/gut.2003.038364
94. Nishitsuji H, Funami K, Shimizu Y, Ujino S, Sugiyama K, Seya T, et al. Hepatitis c virus infection induces inflammatory cytokines and chemokines mediated by the cross talk between hepatocytes and stellate cells. J Virol (2013) 87:8169–78. doi: 10.1128/JVI.00974-13
95. Hosomura N, Kono H, Tsuchiya M, Ishii K, Ogiku M, Matsuda M, et al. HCV-related proteins activate kupffer cells isolated from human liver tissues. Dig Dis Sci (2011) 56:1057–64. doi: 10.1007/s10620-010-1395-y
96. van Wilgenburg B, Scherwitzl I, Hutchinson EC, Leng T, Kurioka A, Kulicke C, et al. MAIT cells are activated during human viral infections. Nat Commun (2016) 7:11653. doi: 10.1038/ncomms11653
97. Hengst J, Strunz B, Deterding K, Ljunggren H-G, Leeansyah E, Manns MP, et al. Nonreversible MAIT cell-dysfunction in chronic hepatitis c virus infection despite successful interferon-free therapy. Eur J Immunol (2016) 46:2204–10. doi: 10.1002/eji.201646447
98. Bolte FJ, Rehermann B. Mucosal-associated invariant T cells in chronic inflammatory liver disease. Semin Liver Dis (2018) 38:60–5. doi: 10.1055/s-0037-1621709
99. Tseng CT, Miskovsky E, Houghton M, Klimpel GR. Characterization of liver T-cell receptor gammadelta T cells obtained from individuals chronically infected with hepatitis c virus (HCV): evidence for these T cells playing a role in the liver pathology associated with HCV infections. Hepatology (2001) 33:1312–20. doi: 10.1053/jhep.2001.24269
100. Nattermann J, Feldmann G, Ahlenstiel G, Langhans B, Sauerbruch T, Spengler U. Surface expression and cytolytic function of natural killer cell receptors is altered in chronic hepatitis c. Gut (2006) 55:869–77. doi: 10.1136/gut.2005.076463
101. Stegmann KA, Björkström NK, Ciesek S, Lunemann S, Jaroszewicz J, Wiegand J, et al. Interferon α-stimulated natural killer cells from patients with acute hepatitis c virus (HCV) infection recognize HCV-infected and uninfected hepatoma cells via DNAX accessory molecule-1. J Infect Dis (2012) 205:1351–62. doi: 10.1093/infdis/jis210
102. Senff T, Menne C, Cosmovici C, Lewis-Ximenez LL, Aneja J, Broering R, et al. Peripheral blood iNKT cell activation correlates with liver damage during acute hepatitis c. JCI Insight (2022) 7:e155432. doi: 10.1172/jci.insight.155432
103. Grant DM. Detoxification pathways in the liver. J Inherit Metab Dis (1991) 14:421–30. doi: 10.1007/BF01797915
104. Francis P, Navarro VJ. StatPearls: Drug induced hepatotoxicity. In: . Treasure Island (FL (2022).
105. Kinashi Y, Hase K. Partners in leaky gut syndrome: Intestinal dysbiosis and autoimmunity. Front Immunol (2021) 12:673708. doi: 10.3389/fimmu.2021.673708
106. Twardowska A, Makaro A, Binienda A, Fichna J, Salaga M. Preventing bacterial translocation in patients with leaky gut syndrome: Nutrition and pharmacological treatment options. Int J Mol Sci (2022) 23:3204. doi: 10.3390/ijms23063204
107. Szabo G, Bala S. Alcoholic liver disease and the gut-liver axis. World J Gastroenterol (2010) 16:1321–9. doi: 10.3748/wjg.v16.i11.1321
108. Mihm S. Danger-associated molecular patterns (DAMPs): Molecular triggers for sterile inflammation in the liver. Int J Mol Sci (2018) 19:3104. doi: 10.3390/ijms19103104
109. Kubes P, Mehal WZ. Sterile inflammation in the liver. Gastroenterology (2012) 143:1158–72. doi: 10.1053/j.gastro.2012.09.008
110. Schlechte J, Skalosky I, Geuking MB, McDonald B. Long-distance relationships - regulation of systemic host defense against infections by the gut microbiota. Mucosal Immunol (2022) 15:809–18. doi: 10.1038/s41385-022-00539-2
111. Choi SE, Jeong W-I. Innate lymphoid cells: New culprits of alcohol-associated steatohepatitis. Cell Mol Gastroenterol Hepatol (2023) 15:279–80. doi: 10.1016/j.jcmgh.2022.10.012
112. Enomoto N, Ikejima K, Bradford BU, Rivera CA, Kono H, Goto M, et al. Role of kupffer cells and gut-derived endotoxins in alcoholic liver injury. J Gastroenterol Hepatol (2000) 15 Suppl:D20–5. doi: 10.1046/j.1440-1746.2000.02179.x
113. Kono H, Rusyn I, Yin M, Gäbele E, Yamashina S, Dikalova A, et al. NADPH oxidase-derived free radicals are key oxidants in alcohol-induced liver disease. J Clin Invest (2000) 106:867–72. doi: 10.1172/JCI9020
114. Nagy LE, Ding W-X, Cresci G, Saikia P, Shah VH. Linking pathogenic mechanisms of alcoholic liver disease with clinical phenotypes. Gastroenterology (2016) 150:1756–68. doi: 10.1053/j.gastro.2016.02.035
115. Zhang L, Bansal MB. Role of kupffer cells in driving hepatic inflammation and fibrosis in HIV infection. Front Immunol (2020) 11:1086. doi: 10.3389/fimmu.2020.01086
116. Koop DR, Klopfenstein B, Iimuro Y, Thurman RG. Gadolinium chloride blocks alcohol-dependent liver toxicity in rats treated chronically with intragastric alcohol despite the induction of CYP2E1. Mol Pharmacol (1997) 51:944–50. doi: 10.1124/mol.51.6.944
117. Pan H, Sun R, Jaruga B, Hong F, Kim W-H, Gao B. Chronic ethanol consumption inhibits hepatic natural killer cell activity and accelerates murine cytomegalovirus-induced hepatitis. Alcohol Clin Exp Res (2006) 30:1615–23. doi: 10.1111/j.1530-0277.2006.00194.x
118. Perney P, Portalès P, Corbeau P, Roques V, Blanc F, Clot J. Specific alteration of peripheral cytotoxic cell perforin expression in alcoholic patients: a possible role in alcohol-related diseases. Alcohol Clin Exp Res (2003) 27:1825–30. doi: 10.1097/01.ALC.0000093742.22787.30
119. Jeong W-I, Park O, Radaeva S, Gao B. STAT1 inhibits liver fibrosis in mice by inhibiting stellate cell proliferation and stimulating NK cell cytotoxicity. Hepatology (2006) 44:1441–51. doi: 10.1002/hep.21419
120. Lee K-C, Chen P, Maricic I, Inamine T, Hu J, Gong S, et al. Intestinal iNKT cells migrate to liver and contribute to hepatocyte apoptosis during alcoholic liver disease. Am J Physiol Gastrointest Liver Physiol (2019) 316:G585–97. doi: 10.1152/ajpgi.00269.2018
121. Buschard K, Hansen AK, Jensen K, Lindenbergh-Kortleve DJ, deRuiter LF, Krohn TC, et al. Alcohol facilitates CD1d loading, subsequent activation of NKT cells, and reduces the incidence of diabetes in NOD mice. PloS One (2011) 6:e17931. doi: 10.1371/journal.pone.0017931
122. Cui K, Yan G, Xu C, Chen Y, Wang J, Zhou R, et al. Invariant NKT cells promote alcohol-induced steatohepatitis through interleukin-1β in mice. J Hepatol (2015) 62:1311–8. doi: 10.1016/j.jhep.2014.12.027
123. Mathews S, Feng D, Maricic I, Ju C, Kumar V, Gao B. Invariant natural killer T cells contribute to chronic-plus-binge ethanol-mediated liver injury by promoting hepatic neutrophil infiltration. Cell Mol Immunol (2016) 13:206–16. doi: 10.1038/cmi.2015.06
124. Maricic I, Sheng H, Marrero I, Seki E, Kisseleva T, Chaturvedi S, et al. Inhibition of type I natural killer T cells by retinoids or following sulfatide-mediated activation of type II natural killer T cells attenuates alcoholic liver disease in mice. Hepatology (2015) 61:1357–69. doi: 10.1002/hep.27632
125. Maricic I, Girardi E, Zajonc DM, Kumar V. Recognition of lysophosphatidylcholine by type II NKT cells and protection from an inflammatory liver disease. J Immunol (2014) 193:4580–9. doi: 10.4049/jimmunol.1400699
126. Halder RC, Aguilera C, Maricic I, Kumar V. Type II NKT cell-mediated anergy induction in type I NKT cells prevents inflammatory liver disease. J Clin Invest (2007) 117:2302–12. doi: 10.1172/JCI31602
127. Riva A, Patel V, Kurioka A, Jeffery HC, Wright G, Tarff S, et al. Mucosa-associated invariant T cells link intestinal immunity with antibacterial immune defects in alcoholic liver disease. Gut (2018) 67:918–30. doi: 10.1136/gutjnl-2017-314458
128. Zhang Y, Fan Y, He W, Han Y, Bao H, Yang R, et al. Persistent deficiency of mucosa-associated invariant T (MAIT) cells during alcohol-related liver disease. Cell Biosci (2021) 11:148. doi: 10.1186/s13578-021-00664-8
129. Li W, Lin EL, Liangpunsakul S, Lan J, Chalasani S, Rane S, et al. Alcohol abstinence does not fully reverse abnormalities of mucosal-associated invariant T cells in the blood of patients with alcoholic hepatitis. Clin Transl Gastroenterol (2019) 10:e00052. doi: 10.14309/ctg.0000000000000052
130. Lee J-H, Shim Y-R, Seo W, Kim M-H, Choi W-M, Kim H-H, et al. Mitochondrial double-stranded RNA in exosome promotes interleukin-17 production through toll-like receptor 3 in alcohol-associated liver injury. Hepatology (2020) 72:609–25. doi: 10.1002/hep.31041
131. Marcellin P, Kutala BK. Liver diseases: A major, neglected global public health problem requiring urgent actions and large-scale screening. Liver Int (2018) 38 Suppl 1:2–6. doi: 10.1111/liv.13682
132. Noureddin M, Vipani A, Bresee C, Todo T, Kim IK, Alkhouri N, et al. NASH leading cause of liver transplant in women: Updated analysis of indications for liver transplant and ethnic and gender variances. Am J Gastroenterol (2018) 113:1649–59. doi: 10.1038/s41395-018-0088-6
133. Lazarus JV, Mark HE, Villota-Rivas M, Palayew A, Carrieri P, Colombo M, et al. The global NAFLD policy review and preparedness index: Are countries ready to address this silent public health challenge? J Hepatol (2022) 76:771–80. doi: 10.1016/j.jhep.2021.10.025
134. Nati M, Chung K-J, Chavakis T. The role of innate immune cells in nonalcoholic fatty liver disease. J Innate Immun (2022) 14:31–41. doi: 10.1159/000518407
135. Rodrigues RM, Guan Y, Gao B. Targeting adipose tissue to tackle NASH: SPARCL1 as an emerging player. J Clin Invest (2021) 13:e153640. doi: 10.1172/JCI153640
136. Neuschwander-Tetri BA. Therapeutic landscape for NAFLD in 2020. Gastroenterology (2020) 158:1984–1998.e3. doi: 10.1053/j.gastro.2020.01.051
137. Park J-W, Jeong G, Kim SJ, Kim MK, Park SM. Predictors reflecting the pathological severity of non-alcoholic fatty liver disease: comprehensive study of clinical and immunohistochemical findings in younger Asian patients. J Gastroenterol Hepatol (2007) 22:491–7. doi: 10.1111/j.1440-1746.2006.04758.x
138. Huang W, Metlakunta A, Dedousis N, Zhang P, Sipula I, Dube JJ, et al. Depletion of liver kupffer cells prevents the development of diet-induced hepatic steatosis and insulin resistance. Diabetes (2010) 59:347–57. doi: 10.2337/db09-0016
139. Diehl KL, Vorac J, Hofmann K, Meiser P, Unterweger I, Kuerschner L, et al. Kupffer cells sense free fatty acids and regulate hepatic lipid metabolism in high-fat diet and inflammation. Cells (2020) 9:2258. doi: 10.3390/cells9102258
140. Ioannou GN, Landis CS, Jin G-Y, Haigh WG, Farrell GC, Kuver R, et al. Cholesterol crystals in hepatocyte lipid droplets are strongly associated with human nonalcoholic steatohepatitis. Hepatol Commun (2019) 3:776–91. doi: 10.1002/hep4.1348
141. Leroux A, Ferrere G, Godie V, Cailleux F, Renoud M-L, Gaudin F, et al. Toxic lipids stored by kupffer cells correlates with their pro-inflammatory phenotype at an early stage of steatohepatitis. J Hepatol (2012) 57:141–9. doi: 10.1016/j.jhep.2012.02.028
142. Tercan H, Riksen NP, Joosten LAB, Netea MG, Bekkering S. Trained immunity: Long-term adaptation in innate immune responses. Arterioscler Thromb Vasc Biol (2021) 41:55–61. doi: 10.1161/ATVBAHA.120.314212
143. Diedrich T, Kummer S, Galante A, Drolz A, Schlicker V, Lohse AW, et al. Characterization of the immune cell landscape of patients with NAFLD. PloS One (2020) 15:e0230307. doi: 10.1371/journal.pone.0230307
144. O’Shea D, Hogan AE. Dysregulation of natural killer cells in obesity. Cancers (Basel) (2019) 11:573. doi: 10.3390/cancers11040573
145. Michelet X, Dyck L, Hogan A, Loftus RM, Duquette D, Wei K, et al. Metabolic reprogramming of natural killer cells in obesity limits antitumor responses. Nat Immunol (2018) 19:1330–40. doi: 10.1038/s41590-018-0251-7
146. Stiglund N, Strand K, Cornillet M, Stål P, Thorell A, Zimmer CL, et al. Retained NK cell phenotype and functionality in non-alcoholic fatty liver disease. Front Immunol (2019) 10:1255. doi: 10.3389/fimmu.2019.01255
147. Li Z, Soloski MJ, Diehl AM. Dietary factors alter hepatic innate immune system in mice with nonalcoholic fatty liver disease. Hepatology (2005) 42:880–5. doi: 10.1002/hep.20826
148. Kremer M, Thomas E, Milton RJ, Perry AW, van Rooijen N, Wheeler MD, et al. Kupffer cell and interleukin-12-dependent loss of natural killer T cells in hepatosteatosis. Hepatology (2010) 51:130–41. doi: 10.1002/hep.23292
149. Guebre-Xabier M, Yang S, Lin HZ, Schwenk R, Krzych U, Diehl AM. Altered hepatic lymphocyte subpopulations in obesity-related murine fatty livers: potential mechanism for sensitization to liver damage. Hepatology (2000) 31:633–40. doi: 10.1002/hep.510310313
150. Kim HM, Lee BR, Lee ES, Kwon MH, Huh JH, Kwon B-E, et al. iNKT cells prevent obesity-induced hepatic steatosis in mice in a c-c chemokine receptor 7-dependent manner. Int J Obes (Lond) (2018) 42:270–9. doi: 10.1038/ijo.2017.200
151. Ohmura K, Ishimori N, Ohmura Y, Tokuhara S, Nozawa A, Horii S, et al. Natural killer T cells are involved in adipose tissues inflammation and glucose intolerance in diet-induced obese mice. Arterioscler Thromb Vasc Biol (2010) 30:193–9. doi: 10.1161/ATVBAHA.109.198614
152. Wu L, Parekh VV, Gabriel CL, Bracy DP, Marks-Shulman PA, Tamboli RA, et al. Activation of invariant natural killer T cells by lipid excess promotes tissue inflammation, insulin resistance, and hepatic steatosis in obese mice. Proc Natl Acad Sci U S A (2012) 109:E1143–52. doi: 10.1073/pnas.1200498109
153. Satoh M, Andoh Y, Clingan CS, Ogura H, Fujii S, Eshima K, et al. Type II NKT cells stimulate diet-induced obesity by mediating adipose tissue inflammation, steatohepatitis and insulin resistance. PloS One (2012) 7:e30568. doi: 10.1371/journal.pone.0030568
154. Hams E, Locksley RM, McKenzie ANJ, Fallon PG. Cutting edge: IL-25 elicits innate lymphoid type 2 and type II NKT cells that regulate obesity in mice. J Immunol (2013) 191:5349–53. doi: 10.4049/jimmunol.1301176
155. Xu C-F, Yu C-H, Li Y-M, Xu L, Du J, Shen Z. Association of the frequency of peripheral natural killer T cells with nonalcoholic fatty liver disease. World J Gastroenterol (2007) 13:4504–8. doi: 10.3748/wjg.v13.i33.4504
156. Tajiri K, Shimizu Y, Tsuneyama K, Sugiyama T. Role of liver-infiltrating CD3+CD56+ natural killer T cells in the pathogenesis of nonalcoholic fatty liver disease. Eur J Gastroenterol Hepatol (2009) 21:673–80. doi: 10.1097/MEG.0b013e32831bc3d6
157. Adler M, Taylor S, Okebugwu K, Yee H, Fielding C, Fielding G, et al. Intrahepatic natural killer T cell populations are increased in human hepatic steatosis. World J Gastroenterol (2011) 17:1725–31. doi: 10.3748/wjg.v17.i13.1725
158. Syn W-K, Oo YH, Pereira TA, Karaca GF, Jung Y, Omenetti A, et al. Accumulation of natural killer T cells in progressive nonalcoholic fatty liver disease. Hepatology (2010) 51:1998–2007. doi: 10.1002/hep.23599
159. Li Y, Huang B, Jiang X, Chen W, Zhang J, Wei Y, et al. Mucosal-associated invariant T cells improve nonalcoholic fatty liver disease through regulating macrophage polarization. Front Immunol (2018) 9:1994. doi: 10.3389/fimmu.2018.01994
160. Li F, Hao X, Chen Y, Bai L, Gao X, Lian Z, et al. The microbiota maintain homeostasis of liver-resident γδT-17 cells in a lipid antigen/CD1d-dependent manner. Nat Commun (2017) 7:13839. doi: 10.1038/ncomms13839
161. Torres-Hernandez A, Wang W, Nikiforov Y, Tejada K, Torres L, Kalabin A, et al. γδ T cells promote steatohepatitis by orchestrating innate and adaptive immune programming. Hepatology (2020) 71:477–94. doi: 10.1002/hep.30952
162. Xu X, Zhang S, Song X, Hu Q, Pan W. IL-17 enhances oxidative stress in hepatocytes through Nrf2/keap1 signal pathway activation. Int J Clin Exp Pathol (2018) 11:3318–23.
163. Tan Z, Jiang R, Wang X, Wang Y, Lu L, Liu Q, et al. RORγt+IL-17+ neutrophils play a critical role in hepatic ischemia-reperfusion injury. J Mol Cell Biol (2013) 5:143–6. doi: 10.1093/jmcb/mjs065
164. Mehta P, Nuotio-Antar AM, Smith CW. γδ T cells promote inflammation and insulin resistance during high fat diet-induced obesity in mice. J Leukoc Biol (2015) 97:121–34. doi: 10.1189/jlb.3A0414-211RR
165. Kumar S, Duan Q, Wu R, Harris EN, Su Q. Pathophysiological communication between hepatocytes and non-parenchymal cells in liver injury from NAFLD to liver fibrosis. Adv Drug Delivery Rev (2021) 176:113869. doi: 10.1016/j.addr.2021.113869
166. Morgan MJ, Liu Z. Reactive oxygen species in TNFalpha-induced signaling and cell death. Mol Cells (2010) 30:1–12. doi: 10.1007/s10059-010-0105-0
167. Whiteside TL. NK cells in the tumor microenvironment and thioredoxin activity. J Clin Invest (2020) 130:5115–7. doi: 10.1172/JCI141460
168. Barron L, Wynn TA. Fibrosis is regulated by Th2 and Th17 responses and by dynamic interactions between fibroblasts and macrophages. Am J Physiol Gastrointest Liver Physiol (2011) 300:G723–8. doi: 10.1152/ajpgi.00414.2010
169. Oo YH, Adams DH. Regulatory T cells and autoimmune hepatitis: what happens in the liver stays in the liver. J Hepatol (2014) 61:973–5. doi: 10.1016/j.jhep.2014.08.005
170. Taylor SA, Assis DN, Mack CL. The contribution of b cells in autoimmune liver diseases. Semin Liver Dis (2019) 39:422–31. doi: 10.1055/s-0039-1688751
171. Xiao F, Ai G, Yan W, Wan X, Luo X, Ning Q. Intrahepatic recruitment of cytotoxic NK cells contributes to autoimmune hepatitis progression. Cell Immunol (2018) 327:13–20. doi: 10.1016/j.cellimm.2017.12.008
172. Jeffery HC, Braitch MK, Bagnall C, Hodson J, Jeffery LE, Wawman RE, et al. Changes in natural killer cells and exhausted memory regulatory T cells with corticosteroid therapy in acute autoimmune hepatitis. Hepatol Commun (2018) 2:421–36. doi: 10.1002/hep4.1163
173. Guan J, Wang G, Yang Q, Chen C, Deng J, Gu X, et al. Natural killer T cells in various mouse models of hepatitis. BioMed Res Int (2021) 2021:1782765. doi: 10.1155/2021/1782765
174. Biburger M, Tiegs G. Activation-induced NKT cell hyporesponsiveness protects from alpha-galactosylceramide hepatitis and is independent of active transregulatory factors. J Leukoc Biol (2008) 84:264–79. doi: 10.1189/jlb.0607352
175. Wang H, Feng D, Park O, Yin S, Gao B. Invariant NKT cell activation induces neutrophil accumulation and hepatitis: opposite regulation by IL-4 and IFN-γ. Hepatology (2013) 58:1474–85. doi: 10.1002/hep.26471
176. Santodomingo-Garzon T, Swain MG. Role of NKT cells in autoimmune liver disease. Autoimmun Rev (2011) 10:793–800. doi: 10.1016/j.autrev.2011.06.003
177. Böttcher K, Rombouts K, Saffioti F, Roccarina D, Rosselli M, Hall A, et al. MAIT cells are chronically activated in patients with autoimmune liver disease and promote profibrogenic hepatic stellate cell activation. Hepatology (2018) 68:172–86. doi: 10.1002/hep.29782
178. Ferri S, Longhi MS, de MC, Lalanne C, Muratori P, Granito A, et al. A multifaceted imbalance of T cells with regulatory function characterizes type 1 autoimmune hepatitis. Hepatology (2010) 52:999–1007. doi: 10.1002/hep.23792
179. He Q, Lu Y, Tian W, Jiang R, Yu W, Liu Y, et al. TOX deficiency facilitates the differentiation of IL-17A-producing γδ T cells to drive autoimmune hepatitis. Cell Mol Immunol (2022) 19:1102–16. doi: 10.1038/s41423-022-00912-y
180. Panasiuk A, Prokopowicz D, Zak J, Panasiuk B, Wysocka J. Lymphocyte subpopulations in peripheral blood in primary sclerosing cholangitis. Hepatogastroenterology (2004) 51:1289–91.
181. Chang C-H, Chen Y-C, Yu Y-H, Tao M-H, Leung PSC, Ansari AA, et al. Innate immunity drives xenobiotic-induced murine autoimmune cholangitis. Clin Exp Immunol (2014) 177:373–80. doi: 10.1111/cei.12298
182. Liu H, Man K. New insights in mechanisms and therapeutics for short- and long-term impacts of hepatic ischemia reperfusion injury post liver transplantation. Int J Mol Sci (2021) 22:8210. doi: 10.3390/ijms22158210
183. Lu L, Zhou H, Ni M, Wang X, Busuttil R, Kupiec-Weglinski J, et al. Innate immune regulations and liver ischemia-reperfusion injury. Transplantation (2016) 100:2601–10. doi: 10.1097/TP.0000000000001411
184. Ito T, Naini BV, Markovic D, Aziz A, Younan S, Lu M, et al. Ischemia-reperfusion injury and its relationship with early allograft dysfunction in liver transplant patients. Am J Transplant (2021) 21:614–25. doi: 10.1111/ajt.16219
185. Zhai Y, Petrowsky H, Hong JC, Busuttil RW, Kupiec-Weglinski JW. Ischaemia-reperfusion injury in liver transplantation–from bench to bedside. Nat Rev Gastroenterol Hepatol (2013) 10:79–89. doi: 10.1038/nrgastro.2012.225
186. Robertson F, Male V, Wright G, Fuller B, Davidson B. Recruitment of inflammatory monocytes after liver transplantation and correlation with clinical outcome. Lancet (2017) 389:S84. doi: 10.1016/S0140-6736(17)30480-4
187. Kim H-Y, Kim S-J, Lee S-M. Activation of NLRP3 and AIM2 inflammasomes in kupffer cells in hepatic ischemia/reperfusion. FEBS J (2015) 282:259–70. doi: 10.1111/febs.13123
188. Wu T, Zhang C, Shao T, Chen J, Chen D. The role of NLRP3 inflammasome activation pathway of hepatic macrophages in liver ischemia-reperfusion injury. Front Immunol (2022) 13:905423. doi: 10.3389/fimmu.2022.905423
189. Tsutsui H, Matsui K, Kawada N, Hyodo Y, Hayashi N, Okamura H, et al. IL-18 accounts for both TNF-alpha- and fas ligand-mediated hepatotoxic pathways in endotoxin-induced liver injury in mice. J Immunol (1997) 159:3961–7. doi: 10.4049/jimmunol.159.8.3961
190. Okamura H, Kashiwamura S, Tsutsui H, Yoshimoto T, Nakanishi K. Regulation of interferon-gamma production by IL-12 and IL-18. Curr Opin Immunol (1998) 10:259–64. doi: 10.1016/s0952-7915(98)80163-5
191. Beldi G, Banz Y, Kroemer A, Sun X, Wu Y, Graubardt N, et al. Deletion of CD39 on natural killer cells attenuates hepatic ischemia/reperfusion injury in mice. Hepatology (2010) 51:1702–11. doi: 10.1002/hep.23510
192. Linares I, Hamar M, Selzner N, Selzner M. Steatosis in liver transplantation: Current limitations and future strategies. Transplantation (2019) 103:78–90. doi: 10.1097/TP.0000000000002466
193. Eggenhofer E, Groell A, Junger H, Kasi A, Kroemer A, Geissler EK, et al. Steatotic livers are more susceptible to ischemia reperfusion damage after transplantation and show increased γδ T cell infiltration. Int J Mol Sci (2021) 22:2036. doi: 10.3390/ijms22042036
194. Shimamura K, Kawamura H, Nagura T, Kato T, Naito T, Kameyama H, et al. Association of NKT cells and granulocytes with liver injury after reperfusion of the portal vein. Cell Immunol (2005) 234:31–8. doi: 10.1016/j.cellimm.2005.04.022
195. Lappas CM, Day Y-J, Marshall MA, Engelhard VH, Linden J. Adenosine A2A receptor activation reduces hepatic ischemia reperfusion injury by inhibiting CD1d-dependent NKT cell activation. J Exp Med (2006) 203:2639–48. doi: 10.1084/jem.20061097
196. Kuboki S, Sakai N, Tschöp J, Edwards MJ, Lentsch AB, Caldwell CC. Distinct contributions of CD4+ T cell subsets in hepatic ischemia/reperfusion injury. Am J Physiol Gastrointest Liver Physiol (2009) 296:G1054–9. doi: 10.1152/ajpgi.90464.2008
197. Nakajima S, Tanaka R, Yamashiro K, Chiba A, Noto D, Inaba T, et al. Mucosal-associated invariant T cells are involved in acute ischemic stroke by regulating neuroinflammation. J Am Heart Assoc (2021) 10:e018803. doi: 10.1161/JAHA.120.018803
198. Llewellyn HP, Arat S, Gao J, Wen J, Xia S, Kalabat D, et al. T Cells and monocyte-derived myeloid cells mediate immunotherapy-related hepatitis in a mouse model. J Hepatol (2021) 75:1083–95. doi: 10.1016/j.jhep.2021.06.037
199. Sansom DM. CD28, CTLA-4 and their ligands: who does what and to whom? Immunology (2000) 101:169–77. doi: 10.1046/j.1365-2567.2000.00121.x
200. Triantafyllou E, Gudd CL, Mawhin M-A, Husbyn HC, Trovato FM, Siggins MK, et al. PD-1 blockade improves kupffer cell bacterial clearance in acute liver injury. J Clin Invest (2021) 131:e140196. doi: 10.1172/JCI140196
201. Hakanen H, Hernberg M, Mäkelä S, Yadav B, Brück O, Juteau S, et al. Anti-PD1 therapy increases peripheral blood NKT cells and chemokines in metastatic melanoma patients. Ann Oncol (2018) 29:x3. doi: 10.1093/annonc/mdy493.007
202. Jarvis E-M, Collings S, Authier-Hall A, Dasyam N, Luey B, Nacey J, et al. Mucosal-associated invariant T (MAIT) cell dysfunction and PD-1 expression in prostate cancer: Implications for immunotherapy. Front Immunol (2021) 12:748741. doi: 10.3389/fimmu.2021.748741
203. Tomogane M, Sano Y, Shimizu D, Shimizu T, Miyashita M, Toda Y, et al. Human Vγ9Vδ2 T cells exert anti-tumor activity independently of PD-L1 expression in tumor cells. Biochem Biophys Res Commun (2021) 573:132–9. doi: 10.1016/j.bbrc.2021.08.005
204. Gubin MM, Esaulova E, Ward JP, Malkova ON, Runci D, Wong P, et al. High-dimensional analysis delineates myeloid and lymphoid compartment remodeling during successful immune-checkpoint cancer therapy. Cell (2018) 175:1014–1030.e19. doi: 10.1016/j.cell.2018.09.030
205. Jovanovic M, Gajovic N, Jurisevic M, Sekulic S, Arsenijevic N, Jocic M, et al. Anti-PD-1 therapy activates tumoricidic properties of NKT cells and contributes to the overall deceleration of tumor progression in a model of murine mammary carcinoma. VSP (2022) 79:764–73. doi: 10.2298/VSP210126039J
206. Nallagangula KS, Nagaraj SK, Venkataswamy L, Chandrappa M. Liver fibrosis: a compilation on the biomarkers status and their significance during disease progression. Future Sci OA (2018) 4:FSO250. doi: 10.4155/fsoa-2017-0083
Keywords: Inflammation, Innate immnuity, Hepatitis (general), NK cells, MAIT cell, Gd T cell, NKT (natural killer T) cell, kupffer cell (KC)
Citation: Yang Zhou J (2023) Innate immunity and early liver inflammation. Front. Immunol. 14:1175147. doi: 10.3389/fimmu.2023.1175147
Received: 27 February 2023; Accepted: 30 March 2023;
Published: 02 May 2023.
Edited by:
Luc Van Kaer, Vanderbilt University Medical Center, United StatesReviewed by:
Vipin Kumar, University of California, San Diego, United StatesCopyright © 2023 Yang Zhou. This is an open-access article distributed under the terms of the Creative Commons Attribution License (CC BY). The use, distribution or reproduction in other forums is permitted, provided the original author(s) and the copyright owner(s) are credited and that the original publication in this journal is cited, in accordance with accepted academic practice. No use, distribution or reproduction is permitted which does not comply with these terms.
*Correspondence: Jordi Yang Zhou, Sm9yZGkuWWFuZy1aaG91QGtsaW5pay51bmktcmVnZW5zYnVyZy5kZQ==
Disclaimer: All claims expressed in this article are solely those of the authors and do not necessarily represent those of their affiliated organizations, or those of the publisher, the editors and the reviewers. Any product that may be evaluated in this article or claim that may be made by its manufacturer is not guaranteed or endorsed by the publisher.
Research integrity at Frontiers
Learn more about the work of our research integrity team to safeguard the quality of each article we publish.