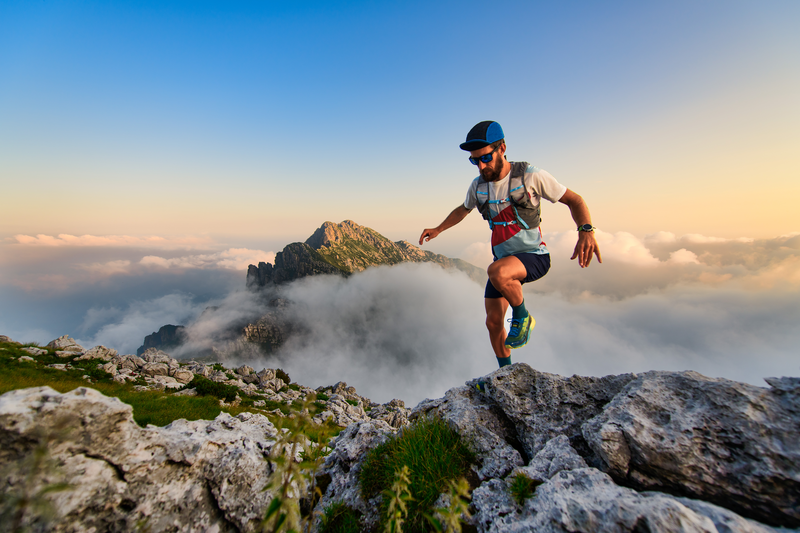
94% of researchers rate our articles as excellent or good
Learn more about the work of our research integrity team to safeguard the quality of each article we publish.
Find out more
MINI REVIEW article
Front. Immunol. , 09 May 2023
Sec. Autoimmune and Autoinflammatory Disorders: Autoinflammatory Disorders
Volume 14 - 2023 | https://doi.org/10.3389/fimmu.2023.1174789
This article is part of the Research Topic CD24 in the Regulation of Cellular Development and Disease View all 5 articles
CD24 is a small glycosylphosphatidylinositol (GPI)-anchored glycoprotein with broad expression in multiple cell types. Due to differential glycosylation, cell surface CD24 have been shown to interact with various receptors to mediate multiple physiological functions. Nearly 15 years ago, CD24 was shown to interact with Siglec G/10 to selectively inhibit inflammatory response to tissue injuries. Subsequent studies demonstrate that sialylated CD24 (SialoCD24) is a major endogenous ligand for CD33-family of Siglecs to protect the host against inflammatory and autoimmune diseases, metabolic disorders and most notably respiratory distress in COVID-19. The discoveries on CD24-Siglec interactions propelled active translational research to treat graft-vs-host diseases, cancer, COVID-19 and metabolic disorders. This mini-review provides a succinct summary on biological significance of CD24-Siglec pathway in regulation of inflammatory diseases with emphasis on clinical translation.
As a GPI-anchored glycoprotein, CD24 is expressed on multiple cell types in normal tissues, including hematopoietic and immature neuronal cells and tissue stem cells (1–3). While CD24 was first identified as a valuable marker for cellular development and differentiation, accumulating studies have revealed critical roles for CD24 in various pathological conditions, including autoimmune diseases (4–8), sepsis (9), metabolic disorders (10–12), graft vs host diseases (13, 14) and cancer (1, 15–20). Given the heterogeneity of in post-translational modifications of CD24, it is not surprising that CD24 may mediate different functions depending on its interacting partners. For example, fucosylated CD24 has been shown to be a ligand for P-selectin (21, 22), while sialylated CD24 have been shown to interact with Siglecs (9, 23). In this minireview, we will focus on CD24-Siglec interactions, with emphasis on potential translation of this pathway for patient care.
Sialic acid-binding immunoglobulin-type lectins (Siglecs) constitute a subfamily of type I lectin. The first member of Siglecs, or Siglec 1 was reported nearly 40 years ago (24, 25). Fifteen human Siglecs have been subsequently characterized (26, 27). Among them, a subgroup group called CD33 family have attracted most attention as potential negative regulators of immune response as they have one or more ITIM-like domains that are phosphorylated upon ligand engagement, leading recruitment of Src homology 2 domain containing protein tyrosine phosphatase (SHP)1/2 and potentially suppress NFkB activation (26, 27). While Siglecs have selectivity for different sialoglycans (28), the endogenous ligands for Siglecs have not been identified decades after first description of Siglecs. Lack of endogenous ligand made it difficult to discern the physiological function of Siglecs.
In 2009, Chen et al. reported CD24 as the major endogenous ligand for Siglec 10 and its mouse homologue Siglec G (23). The interaction was demonstrated by direct binding in vitro and co-precipitation in immune cells. Subsequent studies demonstrate requirement for sialylation in the interaction (9).
The biological significance of CD24-Siglec interaction was first revealed when Chen et al. (23) demonstrated that CD24-Siglec G/10 forms tri-molecular complex with HMGB1 and heat-shock proteins (HSPs), the prototypical danger-associated molecular patterns (DAMPs or danger signal). The concept of danger signal was first proposed to describe inflammatory stimuli released upon tissue injuries or cellular stress (29). Dendritic cells with mutations of either CD24 or Siglecg genes enhanced production of inflammatory cytokines to DAMPs such as HMGB1 and HSP70, but not to pathogen-associated molecular patterns (PAMPs), such as lipopolysaccharides and double-stranded RNA. Targeted mutations of CD24 or Siglecg in the mice fatally exacerbated inflammatory responses to acetaminophen-induced necrosis of hepatocytes without affecting inflammatory responses to PAMPs. Based on these data (23), the author proposed that CD24-Siglec 10 discriminate between PAMPs and DAMPs (23, 30) (Figure 1).
Figure 1 CD24-Siglec 10/G interaction selectively repress inflammatory response to tissue injuries. Sialylated CD24 interacts with Siglec 10 to repress inflammatory responses to danger (damage)-associated molecular patters (DAMPs) but not those to pathogen-associated molecular patterns (PAMPs). TLRs: toll-like receptors.
Pattern recognition is a pillar of contemporary immunology. As proposed by Janeway, innate immunity based on pattern recognition allowed the immune system to sense infections and launch innate immune response, leading to adaptive immune response (31–34). With the identification of pattern recognition receptors and their ability to recognize both DAMPs and PAMPs (35–39), it was difficult to explain how the pattern recognition allows self-nonself discrimination. The discovery that CD24-Siglec G interaction discriminates DAMPs from PAMPs provide a framework by which the self and non-self discrimination can be achieved by the innate immune system. Thus, while responses to PAMPs was unconstrained by the CD24-Siglec interaction, those to DAMPs was restrained by the interaction, allowing minimal inflammatory response to cell death under physiological conditions (30) (Figure 1).
Cellular stress and death are generally physiological and inflammatory response to DAMPs has been shown to beneficial for wound-healing (40, 41). However, massive cell death that occurs as part of cancer therapy can lead to undesirable consequences. Therefore, regulation of host response to cell death can be explored to enhance safety and efficacy of cancer therapy.
Due to graft vs leukemia (GVL) effect, bone marrow transplantation (BMT) is a curative therapy for refractory hematological malignancies (42, 43). Unfortunately, BMT often associates with immune destruction of host tissue by the immune cells in the graft, a pathological condition called graft vs host diseases (GVHD). Given the massive cell death associated with BMT, it was of interest to evaluate whether CD24-Siglec G/10 pathway contributes to pathogenesis of GVHD.
Toubai et al. reported that conditioning for BMT by irradiation reduced expression of Siglec G on the dendritic cells, leading to an increased expression of inflammatory cytokines and co-stimulatory molecules on the dendritic cells (13). Targeted mutations of either CD24 or Siglecg in the hematopoietic cells greatly exacerbated GVHD, while treatment with CD24Fc, a fusion protein consisting of extracellular domain of CD24 and IgG1 Fc, prevented the development of GvHD by targeting the Siglec G on the hematopoietic cells (13). Importantly, CD24Fc suppressed GVHD without negatively impact GVL and that CD24Fc can suppress DAMP-mediated T cell activation by interacting with Siglec G (14).
Based on the compelling preclinical data, OncoImmune, Inc. launched a randomized, prospective, multi-site, placebo-controlled phase 2a clinical trial to investigate safety of three dose levels of CD24Fc (or blinded placebo) plus standard GVHD prophylaxis with tacrolimus and methotrexate in matched unrelated donors. The trial enrolled 24 patients (CD24Fc, n=18; Placebo, n=6) with minimum follow up of one year. In this translational phase 2a trial, administration of CD24Fc was safe and tolerable. The grade 3-4 GVHD-free survival was 94% at 180 days post BMT in patients receiving treatment with CD24Fc versus 50% in pts receiving placebo (HR 0.1; 90% CI 0.0-0.7) (44). A dose expansion Phase IIb study has since been completed with data to be reported soon.
Immunotherapy has provided curative treatment for multiple cancer indications. In analogous to BMT, the efficacy of immunotherapy has been limited by immunotherapy-related adverse events (irAE). Given the massive death of cancer cells and normal cells in immunotherapy and conventional cancer therapy, it is of interest to investigate whether irAE is regulated by CD24-Siglec interactions. As the first-step to address this issue, Liu et al. tested the effect of CD24Fc on irAE and tumor responses (45). Using animal model of irAE (46), the authors demonstrated that CD24Fc ameliorate irAE caused by clinically used anti-CTLA-4 mAb, including multiple organ inflammation and animal survival. Surprisingly, CD24Fc not only preserve cancer therapeutic effect of anti-CTLA-4 and anti-PD-1 antibodies, but promoted tumor rejection in some cancer models, concurrent with reducing the frequency of regulatory T cells among CD4 cells and preventing exhaustion of CD8 T cells in the tumor microenvironment (45). Additional studies are needed to understand how CD24-Siglec pathway regulates tumor microenvironment.
Although early studies demonstrate that CD24-Siglec G/10 interaction controls inflammatory response to DAMPs but not PAMPs, host responses to pathogenic infections can be affected by this interaction because most infections cause cell death and thus may trigger inflammatory response to DAMPs. Moreover, many pathogens have been shown to disrupt CD24-Siglec G/10 interaction, either by down-regulation of Siglec G/10 (47) or by desialylation of CD24 (9). Preclinical studies have shown that CD24Fc protected non-human primates against acquired immunodeficiency syndrome (AIDS) caused by the simian immunodeficiency virus, including diarrhea and pneumonia (48, 49).
Based on the strong therapeutic effect in the non-human primate against AIDS as well as the safety and clinical activities of CD24Fc in healthy volunteers and BMT patients, OncoImmune, Inc. launched a randomized, double-blind, placebo-controlled, phase 3 study at 9 medical centers in the US testing safety and clinical efficacy of CD24Fc for hospitalized COVID-19 patients who needs oxygen support. The primary efficacy endpoint is time to clinical improvement from requiring oxygen support to independent of oxygen support during 28 days of study period. The data demonstrated that CD24Fc is well tolerated and significantly accelerates clinical improvement, by more than 60%, of hospitalized patients with COVID-19 who are receiving oxygen support (HR=1.61, 95% CI 1.16-2.23; P=0.0028) (50). Biomarker studies reveal that CD24Fc systematically repress inflammatory response in the COVID-19 patients (51). Taken together, the data demonstrated that targeting inflammation in response to tissue injuries may provide a therapeutic option for patients hospitalized with COVID-19.
Consistent with clinical activities of CD24Fc, HMGB1 is elevated in plasma of COVID-19 patients (52). More importantly, RNAseq analysis of lung tissue from healthy control and severe COVID-19 patients revealed selective reduction of SIGLEC10 mRNA without affecting expression of other SIGLECS (47). More recently, Shapira et al. reported in a non-randomized study that Exo-CD24, the CD24-containing exosomes, appeared to reduce inflammatory markers and cytokine/chemokine while accelerated recovery of hospitalized COVID-19 patients (53).
While the initial studies focused on the CD24-Siglec 10/Siglec G interaction, CD24 interacts with multiple Siglecs (12, 54). Given the promiscuous nature of CD24-Siglec interactions, genetic studies are necessary to understand contribution of different Siglecs in different disease models.
Metabolic disorders are among the most common threat to human health. While it is clear that chronic inflammation is a root cause, the host factors that regulate the chronic inflammation remain largely unidentified. A Phase I clinical study revealed unexpected activity of CD24Fc in reducing low-density lipoprotein levels in the plasma, which is consistent with broad down-regulation of inflammation-related genes (12). To confirm the rule for CD24 in metabolic disorder, Wang et al. compared CD24+/+ and CD24-/- littermate mice for their development of metabolic disorders, including obesity, dyslipidemia, insulin resistance, and nonalcoholic steatohepatitis (NASH) in response to high-fat feed diet and aging (12). The data demonstrated a critical role for CD24 in suppressing metabolic disorders. To identify CD24 receptor responsible for the metabolic disorders, the authors produced a panel of mouse strains with single or combined mutations of one or more Siglec genes, including those that encode CD22, CD33, Siglec E, Siglec F, Siglec G or Siglec H proteins. Among all genes tested, only Siglece mutation phenocopied that of CD24, suggesting that Siglec E is the functional receptor for CD24 in protection against metabolic disorders. This hypothesis is confirmed by direct and sialylation-dependent CD24-Siglec E interaction, requirement of CD24 in either the same (cis) or separate cells (trans) in constitutional activation of Siglec E. More importantly, in a Siglec E-dependent manner, CD24Fc effectively suppressed obesity, dyslipidemia, insulin resistance, and NASH in multiple mouse models.
As an endogenous ligand for Siglecs, CD24 has been implicated as a dominant suppressor of inflammatory responses in a number of pathological conditions. The biological significance of this pathway has been expanded into the realm of both sterile and non-sterile inflammations, and the concept has been confirmed in both preclinical study and clinical trials (Figure 2). In addition to inflammation, CD24 has been implicated in oncogenesis and tumor evasion of host immunity. While this review has focused on how this pathway maybe fortified to treat or prevent inflammatory diseases, the role for CD24 in cancer therapy has also attracted significant interest. Thus CD24-Siglec pathway has a vast potential for translation to patient cares.
Figure 2 Stimulating CD24-Siglec pathway for treatment of inflammatory and autoimmune diseases. The arrows indicate diseases in which either clinical (GVHD, COVID-19) or preclinical data (IrAE, AIDS, metabolic syndrome and autoimmune diseases) have been reported. GVHD: Graft vs host diseases; AIDS: acquired immunodeficiency syndrome; irAE: immunotherapy-related adverse events.
YL and PZ cowrote this mini review, which is in response to invitation for “CD24 in the Regulation of Cellular Development and Disease” (edited by Geraldine Cambridge, Sherri Christian). All authors contributed to the article and approved the submitted version.
We are grateful to our numerous collaborators for their valuable contributions to studies reviewed herein. We thank Dr. Libing Mu for her graphic design.
Both authors, YL and PZ, were co-founders of OncoImmune, Inc. OncoImmune, Inc. was acquired by Merck & Co., Inc., Rahway, NJ, USA. Both authors, YL and PZ are employees of OncoC4, Inc. and declare conflict of interest due to inventorship and equity ownership.
All claims expressed in this article are solely those of the authors and do not necessarily represent those of their affiliated organizations, or those of the publisher, the editors and the reviewers. Any product that may be evaluated in this article, or claim that may be made by its manufacturer, is not guaranteed or endorsed by the publisher.
1. Fang X, Zheng P, Tang J, Liu Y. CD24: from a to z. Cell Mol Immunol (2010) 7(2):100–3. doi: 10.1038/cmi.2009.119
2. Kay R, Takei F, Humphries RK. Expression cloning of a cDNA encoding M1/69-J11d heat-stable antigens. J Immunol (1990) 145(6):1952–9. doi: 10.4049/jimmunol.145.6.1952
3. Wenger RH, Ayane M, Bose R, Kohler G, Nielsen PJ. The genes for a mouse hematopoietic differentiation marker called the heat-stable antigen. Eur J Immunol (1991) 21(4):1039–46. doi: 10.1002/eji.1830210427
4. Bai XF, Liu JQ, Liu X, Guo Y, Cox K, Wen J, et al. The heat-stable antigen determines pathogenicity of self-reactive T cells in experimental autoimmune encephalomyelitis. J Clin Invest (2000) 105(9):1227–32. doi: 10.1172/JCI9012
5. Park JH, Kim JH, Jo KE, Na SW, Eisenhut M, Kronbichler A, et al. Field synopsis and re-analysis of systematic meta-analyses of genetic association studies in multiple sclerosis: a Bayesian approach. Mol Neurobiol (2018) 55(7):5672–88. doi: 10.1007/s12035-017-0773-2
6. Sanchez E, Abelson AK, Sabio JM, Gonzalez-Gay MA, Ortego-Centeno N, Jimenez-Alonso J, et al. Association of a CD24 gene polymorphism with susceptibility to systemic lupus erythematosus. Arthritis Rheumatol (2007) 56(9):3080–6. doi: 10.1002/art.22871
7. Wang L, Lin S, Rammohan KW, Liu Z, Liu JQ, Liu RH, et al. A dinucleotide deletion in CD24 confers protection against autoimmune diseases. PloS Genet (2007) 3(4):e49. doi: 10.1371/journal.pgen.0030049
8. Zhou Q, Rammohan K, Lin S, Robinson N, Li O, Liu X, et al. CD24 is a genetic modifier for risk and progression of multiple sclerosis. Proc Natl Acad Sci U S A (2003) 100(25):15041–6. doi: 10.1073/pnas.2533866100
9. Chen GY, Chen X, King S, Cavassani KA, Cheng J, Zheng X, et al. Amelioration of sepsis by inhibiting sialidase-mediated disruption of the CD24-SiglecG interaction. Nat Biotechnol (2011) 29(5):428–35. doi: 10.1038/nbt.1846
10. Fairbridge NA, Southall TM, Ayre DC, Komatsu Y, Raquet PI, Brown RJ, et al. Loss of CD24 in mice leads to metabolic dysfunctions and a reduction in white adipocyte tissue. PloS One (2015) 10(11):e0141966. doi: 10.1371/journal.pone.0141966
11. Shapira S, Kazanov D, Dankner R, Fishman S, Stern N, Arber N. High expression level of PPARgamma in CD24 knockout mice and gender-specific metabolic changes: a model of insulin-sensitive obesity. J Pers Med (2021) 11(1):939. doi: 10.3390/jpm11010050
12. Wang X, Liu M, Zhang J, Brown NK, Zhang P, Zhang Y, et al. CD24-siglec axis is an innate immune checkpoint against metaflammation and metabolic disorder. Cell Metab (2022) 34(8):1088–103 e6. doi: 10.1016/j.cmet.2022.07.005
13. Toubai T, Hou G, Mathewson N, Liu C, Wang Y, Oravecz-Wilson K, et al. Siglec-G-CD24 axis controls the severity of graft-versus-host disease in mice. Blood (2014) 123(22):3512–23. doi: 10.1182/blood-2013-12-545335
14. Toubai T, Rossi C, Oravecz-Wilson K, Zajac C, Liu C, Braun T, et al. Siglec-G represses DAMP-mediated effects on T cells. JCI Insight (2017) 2(14):e92293. doi: 10.1172/jci.insight.92293
15. Barkal AA, Brewer RE, Markovic M, Kowarsky M, Barkal SA, Zaro BW, et al. CD24 signalling through macrophage siglec-10 is a target for cancer immunotherapy. Nature (2019) 572(7769):392–6. doi: 10.1038/s41586-019-1456-0
16. Altevogt P, Sammar M, Huser L, Kristiansen G. Novel insights into the function of CD24: a driving force in cancer. Int J Cancer (2021) 148(3):546–59. doi: 10.1002/ijc.33249
17. Jackson D, Waibel R, Weber E, Bell J, Stahel RA. CD24, a signal-transducing molecule expressed on human b cells, is a major surface antigen on small cell lung carcinomas. Cancer Res (1992) 52(19):5264–70.
18. Zhang P, Zheng P, Liu Y. Amplification of the CD24 gene is an independent predictor for poor prognosis of breast cancer. Front Genet (2019) 10:560. doi: 10.3389/fgene.2019.00560
19. Liu W, Zhang Y, Wei S, Bae S, Yang WH, Smith GJ, et al. A CD24-p53 axis contributes to African American prostate cancer disparities. Prostate (2020) 80(8):609–18. doi: 10.1002/pros.23973
20. Wang L, Liu R, Ye P, Wong C, Chen GY, Zhou P, et al. Intracellular CD24 disrupts the ARF-NPM interaction and enables mutational and viral oncogene-mediated p53 inactivation. Nat Commun (2015) 6:5909. doi: 10.1038/ncomms6909
21. Aigner S, Sthoeger ZM, Fogel M, Weber E, Zarn J, Ruppert M, et al. CD24, a mucin-type glycoprotein, is a ligand for p-selectin on human tumor cells. Blood (1997) 89(9):3385–95. doi: 10.1182/blood.V89.9.3385
22. Sammar M, Aigner S, Hubbe M, Schirrmacher V, Schachner M, Vestweber D, et al. Heat-stable antigen (CD24) as ligand for mouse p-selectin. Int Immunol (1994) 6(7):1027–36. doi: 10.1093/intimm/6.7.1027
23. Chen GY, Tang J, Zheng P, Liu Y. CD24 and siglec-10 selectively repress tissue damage-induced immune responses. Science (2009) 323(5922):1722–5. doi: 10.1126/science.1168988
24. Crocker PR, Kelm S, Dubois C, Martin B, McWilliam AS, Shotton DM, et al. Purification and properties of sialoadhesin, a sialic acid-binding receptor of murine tissue macrophages. EMBO J (1991) 10(7):1661–9. doi: 10.1002/j.1460-2075.1991.tb07689.x
25. van den Berg TK, Breve JJ, Damoiseaux JG, Dopp EA, Kelm S, Crocker PR, et al. Sialoadhesin on macrophages: its identification as a lymphocyte adhesion molecule. J Exp Med (1992) 176(3):647–55. doi: 10.1084/jem.176.3.647
26. Crocker PR, Paulson JC, Varki A. Siglecs and their roles in the immune system. Nat Rev Immunol (2007) 7(4):255–66. doi: 10.1038/nri2056
27. Macauley MS, Crocker PR, Paulson JC. Siglec-mediated regulation of immune cell function in disease. Nat Rev Immunol (2014) 14(10):653–66. doi: 10.1038/nri3737
28. Duan S, Paulson JC. Siglecs as immune cell checkpoints in disease. Annu Rev Immunol (2020) 38:365–95. doi: 10.1146/annurev-immunol-102419-035900
29. Matzinger P. Tolerance, danger, and the extended family. Annu Rev Immunol (1994) 12:991–1045. doi: 10.1146/annurev.iy.12.040194.005015
30. Liu Y, Chen GY, Zheng P. CD24-siglec G/10 discriminates danger- from pathogen-associated molecular patterns. Trends Immunol (2009) 30(12):557–61. doi: 10.1016/j.it.2009.09.006
31. Janeway CA Jr. Approaching the asymptote? evolution and revolution in immunology. Cold Spring Harb Symp Quant Biol (1989) 54 Pt 1:1–13. doi: 10.1101/SQB.1989.054.01.003
32. Janeway CA Jr. The immune system evolved to discriminate infectious nonself from noninfectious self. Immunol Today (1992) 13(1):11–6. doi: 10.1016/0167-5699(92)90198-G
33. Liu Y, Janeway CA Jr. Microbial induction of co-stimulatory activity for CD4 T-cell growth. Int Immunol (1991) 3(4):323–32. doi: 10.1093/intimm/3.4.323
34. Liu Y, Janeway CA Jr. Cells that present both specific ligand and costimulatory activity are the most efficient inducers of clonal expansion of normal CD4 T cells. Proc Natl Acad Sci U S A (1992) 89(9):3845–9. doi: 10.1073/pnas.89.9.3845
35. Asea A, Rehli M, Kabingu E, Boch JA, Bare O, Auron PE, et al. Novel signal transduction pathway utilized by extracellular HSP70: role of toll-like receptor (TLR) 2 and TLR4. J Biol Chem (2002) 277(17):15028–34. doi: 10.1074/jbc.M200497200
36. Dybdahl B, Wahba A, Lien E, Flo TH, Waage A, Qureshi N, et al. Inflammatory response after open heart surgery: release of heat-shock protein 70 and signaling through toll-like receptor-4. Circulation (2002) 105(6):685–90. doi: 10.1161/hc0602.103617
37. Medzhitov R, Preston-Hurlburt P, Janeway CA Jr. A human homologue of the drosophila toll protein signals activation of adaptive immunity. Nature (1997) 388(6640):394–7. doi: 10.1038/41131
38. Millar DG, Garza KM, Odermatt B, Elford AR, Ono N, Li Z, et al. Hsp70 promotes antigen-presenting cell function and converts T-cell tolerance to autoimmunity in vivo. Nat Med (2003) 9(12):1469–76. doi: 10.1038/nm962
39. Urbonaviciute V, Furnrohr BG, Meister S, Munoz L, Heyder P, De Marchis F, et al. Induction of inflammatory and immune responses by HMGB1-nucleosome complexes: implications for the pathogenesis of SLE. J Exp Med (2008) 205(13):3007–18. doi: 10.1084/jem.20081165
40. Degryse B, Bonaldi T, Scaffidi P, Muller S, Resnati M, Sanvito F, et al. The high mobility group (HMG) boxes of the nuclear protein HMG1 induce chemotaxis and cytoskeleton reorganization in rat smooth muscle cells. J Cell Biol (2001) 152(6):1197–206. doi: 10.1083/jcb.152.6.1197
41. Tirone M, Tran NL, Ceriotti C, Gorzanelli A, Canepari M, Bottinelli R, et al. High mobility group box 1 orchestrates tissue regeneration via CXCR4. J Exp Med (2018) 215(1):303–18. doi: 10.1084/jem.20160217
42. Mielcarek M, Storer BE, Flowers ME, Storb R, Sandmaier BM, Martin PJ. Outcomes among patients with recurrent high-risk hematologic malignancies after allogeneic hematopoietic cell transplantation. Biol Blood Marrow Transplant (2007) 13(10):1160–8. doi: 10.1016/j.bbmt.2007.06.007
43. Thanarajasingam G, Kim HT, Cutler C, Ho VT, Koreth J, Alyea EP, et al. Outcome and prognostic factors for patients who relapse after allogeneic hematopoietic stem cell transplantation. Biol Blood Marrow Transplant (2013) 19(12):1713–8. doi: 10.1016/j.bbmt.2013.09.011
44. Magenau JM, Jaglowski S, Farag SS, Uberti J, Pawarode A, Riwes MM, et al. Mitigating damage response with CD24 fusion protein for prevention of acute graft-versus-host disease. Biol Blood Marrow Transplantation (2020) 26(3):S52–S3. doi: 10.1016/j.bbmt.2019.12.126
45. Liu M, Wang X, Du X, Zhang Y, Ai C, Hu-Lieskovan S, et al. CD24Fc ameliorates immune-related adverse events while preserving anti-tumor therapeutic effect. Signal Transduction Targeted Ther (2022) 7(1):224. doi: 10.1038/s41392-022-01030-x
46. Du X, Liu M, Su J, Zhang P, Tang F, Ye P, et al. Uncoupling therapeutic from immunotherapy-related adverse effects for safer and effective anti-CTLA-4 antibodies in CTLA4 humanized mice. Cell Res (2018) 28(4):433–47. doi: 10.1038/s41422-018-0012-z
47. Blanco-Melo D, Nilsson-Payant BE, Liu W-C, Uhl S, Hoagland D, Møller R, et al. Imbalanced host response to SARS-CoV-2 drives development of COVID-19. Cell (2020) 181(5):1036–45. e9. doi: 10.1016/j.cell.2020.04.026
48. Tian R-R, Zhang M-X, Liu M, Fang X, Li D, Zhang L, et al. CD24Fc protects against viral pneumonia in simian immunodeficiency virus-infected Chinese rhesus monkeys. Cell Mol Immunol (2020) 17(8):887–8. doi: 10.1038/s41423-020-0452-5
49. Tian R-R, Zhang M-X, Zhang L-T, Zhang P, Ma J-P, Liu M, et al. CD24 and fc fusion protein protects SIVmac239-infected Chinese rhesus macaque against progression to AIDS. Antiviral Res (2018) 157:9–17. doi: 10.1016/j.antiviral.2018.07.004
50. Welker J, Pulido JD, Catanzaro AT, Malvestutto CD, Li Z, Cohen JB, et al. Efficacy and safety of CD24Fc in hospitalised patients with COVID-19: a randomised, double-blind, placebo-controlled, phase 3 study. Lancet Infect Dis (2022) 22(5):611–21. doi: 10.1016/S1473-3099(22)00058-5
51. Song N-J, Allen C, Vilgelm AE, Riesenberg BP, Weller KP, Reynolds K, et al. Treatment with soluble CD24 attenuates COVID-19-associated systemic immunopathology. J Hematol Oncol (2022) 15:1–18. doi: 10.1186/s13045-021-01222-y
52. Chen R, Huang Y, Quan J, Liu J, Wang H, Billiar TR, et al. HMGB1 as a potential biomarker and therapeutic target for severe COVID-19. Heliyon (2020) 6(12):e05672. doi: 10.1016/j.heliyon.2020.e05672
53. Shapira S, Ben Shimon M, Hay-Levi M, Shenberg G, Choshen G, Bannon L, et al. A novel platform for attenuating immune hyperactivity using EXO-CD24 in COVID-19 and beyond. EMBO Mol Med (2022) 14(9):e15997. doi: 10.15252/emmm.202215997
Keywords: CD24, COVID-19, graft vs host diseases, immunotherapy-related adverse events, Siglecs
Citation: Liu Y and Zheng P (2023) CD24-Siglec interactions in inflammatory diseases. Front. Immunol. 14:1174789. doi: 10.3389/fimmu.2023.1174789
Received: 27 February 2023; Accepted: 13 April 2023;
Published: 09 May 2023.
Edited by:
Roberto Paganelli, Institute for Advanced Biologic Therapies, ItalyReviewed by:
Shoib Sarwar Siddiqui, University of Hertfordshire, United KingdomCopyright © 2023 Liu and Zheng. This is an open-access article distributed under the terms of the Creative Commons Attribution License (CC BY). The use, distribution or reproduction in other forums is permitted, provided the original author(s) and the copyright owner(s) are credited and that the original publication in this journal is cited, in accordance with accepted academic practice. No use, distribution or reproduction is permitted which does not comply with these terms.
*Correspondence: Yang Liu, eWFuZ2xAb25jb2M0LmNvbQ==
Disclaimer: All claims expressed in this article are solely those of the authors and do not necessarily represent those of their affiliated organizations, or those of the publisher, the editors and the reviewers. Any product that may be evaluated in this article or claim that may be made by its manufacturer is not guaranteed or endorsed by the publisher.
Research integrity at Frontiers
Learn more about the work of our research integrity team to safeguard the quality of each article we publish.