- 1Department of Medical Oncology, National Center for Cancer Care and Research, Hamad Medical Corporation, Doha, Qatar
- 2Translational Cancer Research Facility, Translational Research Institute, Academic Health System, Hamad Medical Corporation, Doha, Qatar
- 3Translational Research Institute (TRI), Academic Health System, Hamad Medical Corporation, Doha, Qatar
- 4Department of Human Genetics, Sidra Medical and Research Center, Doha, Qatar
- 5College of Health and Life Sciences, Hamad Bin Khalifa University, Doha, Qatar
- 6Department of Laboratory Medicine and Pathology, Hamad Medical Corporation, Doha, Qatar
- 7Diagnostic Genomic Division , Department of Laboratory Medicine and Pathology, Hamad Medical Corporation, Doha, Qatar
- 8Department of Radiation Oncology, National Center for Cancer Care and Research, Hamad Medical Corporation, Doha, Qatar
- 9Translational Research Institute and Dermatology Institute, Academic Health System, Hamad, Medical Corporation, Doha, Qatar
- 10Laboratory Animal Research Center, Qatar University, Doha, Qatar
- 11Clinical Pharmacy and Practice Department, College of Pharmacy, Qatar University (QU) Health, Qatar University, Doha, Qatar
Non-small cell lung cancer (NSCLC) is the leading cause of cancer-related morbidity and mortality worldwide. Immune checkpoint inhibitors (ICIs) including anti-PD-1 and anti-PD-L1 antibodies, have significantly changed the treatment outcomes with better overall survival, but only 15-40% of the patients respond to ICIs therapy. The search for predictive biomarkers of responses is warranted for better clinical outcomes. We aim here to identify pre-treatment soluble immune molecules as surrogate biomarkers for tissue PD-L1 (TPD-L1) status and as predictors of response to anti-PD-1/PD-L1 therapy in NSCLC patients. Sera from 31 metastatic NSCLC patients, eligible for anti-PD-1/PD-L1 or combined chemoimmunotherapy, were collected prior to treatment. Analysis of soluble biomarkers with TPD-L1 status showed significant up/down regulation of the immune inhibitory checkpoint markers (sSiglec7, sSiglec9, sULBP4 and sPD-L2) in patients with higher TPD-L1 (TPD-L1 >50%) expression. Moreover, correlation analysis showed significant positive linear correlation of soluble PD-L1 (sPD-L1) with higher TPD-L1 expression. Interestingly, only responders in the TPD-L1 >50% group showed significant down regulation of the immune inhibitory markers (sPD-L2, sTIMD4, sNectin2 and CEA). When responders vs. non-responders were compared, significant down regulation of other immune inhibitory biomarkers (sCD80, sTIMD4 and CEA) was recorded only in responding patients. In this, the optimal cut-off values of CD80 <91.7 pg/ml and CEA <1614 pg/ml were found to be significantly associated with better progression free survival (PFS). Indeed, multivariate analysis identified the cutoff-value of CEA <1614 pg/ml as an independent predictor of response in our patients. We identified here novel immune inhibitory/stimulatory soluble mediators as potential surrogate/predictive biomarkers for TPD-L1 status, treatment response and PFS in NSCLC patients treated with anti-PD-1/PD-L1 therapy.
Introduction
Lung Cancer is the second most common cancer and a leading cause of cancer-related deaths (a total of 18% of cancer deaths) worldwide. In 2020, 2.2 million new cancer cases and 1.8 million deaths were reported for lung cancer. The 5-year survival rate is poor, ranging between 10-20% in developed countries (1).
Non-small cell lung cancer (NSCLC) is the most common cancer type, accounting for approximately 85% of lung cancer cases (2). Treatment management includes surgical removal, adjuvant chemotherapy, radiotherapy, and molecular-targeted therapies for patients with driver mutations (3). However, in a cohort of metastatic NSCLC patients with wild type epidermal growth factor receptor (EGFR), anaplastic lymphoma kinase (ALK) gene and tumor tissue expressing programmed death ligand-1(TPD-L1), treatment mainly comprises of FDA approved immune checkpoint inhibitors (ICIs), anti-programmed death protein 1/programmed death ligand 1 (PD-1/PD-L1) (4). ICIs are mainly monoclonal antibodies that target immune checkpoints, PD-1, and PD-L1 and block their pathways to help unleash a robust anti-tumor response [5]. Although ICIs have been shown to improve the overall survival in NSCLC patients, limited response rates, ranging between 15-40%, have been documented (5). Several intrinsic and extrinsic factors circulating within the host tumor microenvironment such as regulatory T cells (T regs), myeloid derived suppressor cells (MDSCs), M2 macrophages, immune checkpoints, cytokines and chemokines, have been associated with manipulation of immune response to facilitate tumor progression (6). On the other hand, it is postulated that soluble forms of immune checkpoint T and Natural killer (NK) cell receptors/ligands such as soluble programmed death protein 1 (sPD-1), soluble programmed death ligand 1 (sPD-L1), soluble programmed death ligand 2 (sPD-L2), soluble T cell immunoglobulin domain and mucin domain 3 (sTIM3), soluble UL16 binding protein 1/4 (sULBP-1/4), soluble Natural killer group 2D receptor and ligands sNKG2DL may affect treatment dynamics, either in an immune inhibitory or immune stimulatory manner (7–9). Some of the immune modulatory mechanisms associated with soluble forms include their binding to the treatment active site to hinder treatment efficacy, activation of immune suppressive molecules, inhibition of Interleukin-2 (IL-2) production/T cell activation, T cell apoptosis, upregulation of Tumor necrosis factor-α (TNF-α)/Interferon-gamma (IFN-γ) and early activation of CD8+ T cells leading either to tumor immune escape or control (10–13). In anti-PD-1/PD-L1 treated NSCLC patients, a limited number of studies have associated soluble immune checkpoint markers with prognosis, response to treatment, and overall survival (14–19). The results from these studies indicate a potential role of soluble immune checkpoint mediators as biomarkers for patient stratification (responding vs. non-responding patients) and treatment dynamics. However, most studies have focused mainly on sPD-1 and sPD-L1, indicating a lack of data on other soluble T and NK immune checkpoint markers and their role in prognosis or prediction of response.
In addition to soluble T and NK markers, several studies have also reported on the role of tumor secreted antigens, such as Carcinoembryonic Antigen (CEA), Cytokeratin Fragment 19 (CYFRA21-1), and Carbohydrate Antigen 125 (CA-125), as biomarkers in some tumor types (20–22). These soluble antigens are expressed in various cancers, and some of them are widely used for clinical assessment and treatment monitoring in chemotherapyIn ICI-treated patients, limited number of studies have documented the role of circulating tumor antigens as dynamic biomarkers (23–27). However, the utility of these biomarkers in assessing immunotherapy efficacy in NSCLC patients is still poorly explored, indicating a significant knowledge gap on their role as potential predictive/prognostic biomarkers.
In addition to soluble biomarkers, tissue markers have also been reported as predictors of response. To date, TPD-L1, measured by the immunohistochemistry (IHC) technique, is the only predictive marker approved by FDA as a companion diagnostic for anti-PD-1 antibody treatment in advanced NSCLC. To date, several randomized controlled trials have associated various TPD-L1 tumor proportional scores (TPS) such as ≥1%, ≥5%, ≥10%, and ≥50% with clinical efficacy endpoints such as overall survival (OS), progression-free survival (PFS) and objective response rate (ORR) (28, 29). However, conflicting data regarding the utility of TPD-L1 TPS has been reported, with some trials reporting it as a powerful predictive marker for OS while others indicate limited value of this marker (30–33). In lieu of this, limited studies have investigated the linear relationship of TPD-L1 expression with soluble biomarkers and clinical response in ICI-treated NSCLC patients to understand the role of soluble mediators as surrogate markers for TPD-L1 (34–36). This is an essential area of research since finding non-invasive surrogate markers for tissue can have various advantages, such as ease of sampling, longitudinal monitoring, and limited heterogeneity.
Pre-treatment assessment of dynamic biomarkers is an essential timeline as it helps understand the correlation of baseline biomarkers with disease/treatment dynamics (37, 38). It is well documented that early markers of response can serve as powerful tools for patient stratification and prediction of response (39–41). For ICI-treatment in NSCLC patients, the significance of pre-treatment biomarkers is of utmost importance as this cohort of patients has limited treatment options, and early response prediction can facilitate better patient management.
We aimed here to identify pre-treatment soluble immune checkpoint and circulating tumor antigens as surrogate/predictive markers in TPD-L1 expressing patients and to determine the role of soluble markers as predictors of response in anti-PD-1/PD-L1 treated NSCLC patients.
Methods
Study population and data collection
This prospective study was conducted at the National Center for Cancer Care and Research (NCCCR), Hamad Medical Corporation (HMC), Doha, Qatar, from September 2020 to July 2022. A total of 31 metastatic advanced-stage NSCLC patients eligible for treatment with anti-PD-1 (Nivolumab, Pembrolizumab), anti-PD-L1 (Durvalumab) monotherapy or combined chemoimmunotherapy (Carboplatin + Pemetrexed + Pembrolizumab) were enrolled in the study. Demographics and clinical characteristics of all patients, including age, gender, ethnicity, smoking history, histology, stage, differentiation status, Eastern Cooperative Oncology Group performance status (ECOG PS), genetic aberrations, Tissue PD-L1 expression, metastasis sites, previous lines of radiotherapy/chemotherapy, imaging and clinical response were extracted from electronic health record system of HMC (CERNER®).
Written informed consent was obtained from all eligible participants per Declaration of Helsinki and good clinical practice guidelines. The study was approved by the Institutional Review Board of HMC (MOPH-HMC-020).
Sample collection
Blood sample (10 ml) was collected from eligible patients before anti-PD-1/anti-PD-L1 monotherapy or combined chemoimmunotherapy treatment in BD Vacutainer SST II Advance Serum tubes (Becton Dickenson, USA). The tubes were centrifuged at 1300 g for 10 minutes and the extracted serum was cryopreserved at -80°C until further analysis.
Measurement of soluble immune checkpoint mediators and circulating tumor biomarkers
According to manufacturers’ instruction, the level of soluble immune checkpoint T and NK cell mediators was detected using the Immuno-Oncology Checkpoint 14-Plex Human ProcartaPlex Panel 1, Panel 2, and Immuno-Oncology Checkpoint 9-Plex Human ProcartaPlex Panel 3 (ThermoFisher Scientific, USA). The 37 analytes tested included CD27, CD28, 4-1BB, GITR, HVEM, BTLA, CD80, CTLA-4, IDO, LAG-3, PD-1, PD-L1, PD-L2, TIM-3, MICA, MICB, Perforin, ULBP-1, ULBP-3, ULBP-4, Arginase, NT5E, Tactile, ECadherin, Nectin-2, PVR, Siglec-7, Siglec-9, B7-H6, B7-H3, IAP, BLAST-1,OX40, ICOS Ligand, TIMD-4, S100A8/A9, and VISTA.
The level of the circulating tumor biomarkers, CA-125, CA-15-3, CA-19-9, CEA, and CYFRA-21, was detected according to manufacturers’ instruction, using the customized MILLIPLEX Human Circulating Cancer Biomarker Panel 1 kit (Merck KGaA, Germany).
The concentration of serum immune checkpoint mediators and circulating tumor biomarkers was measured by Luminex Bio-Plex 200 system (BIO-RAD). Acquisition and data analysis were performed by Bio-plex Manager TM version 6.2 software. Analyte concentrations in patients were calculated against a seven-point standard curve using a five-parametric fit algorithm in xPONENT v4.0.3.
Measurement of PD-L1 expression in tumor tissue
TPD-L1 expression was performed in the CAP-accredited Department of Laboratory Medicine and Pathology (DLMP), HMC, Qatar, as part of routine diagnostic testing. TPD-L1 expression was assessed, as per manufacturers’ instructions, on formalin-fixed, paraffin-embedded (FFPE) tissue, by a qualitative immunohistochemical assay (DAKO PD-L1 IHC 22C3 pharmDx) using monoclonal mouse Anti-PD-L1, Clone 22C3 on Automated Autostainer Link 48 (Dako, USA). Briefly, following incubation with the primary monoclonal antibody to TPD-L1 or the Negative Control Reagent (NCR), specimens were incubated with a Linker antibody specific to the host species of the primary antibody, and then incubated with a ready-to-use visualization reagent, consisting of secondary antibody molecules and horseradish peroxidase molecules coupled to a dextran polymer backbone. The enzymatic conversion of the subsequently added chromogen resulted in the precipitation of a visible reaction product at the site of the antigen. The entire slide was evaluated by an independent pathologist using a light microscope objective of 10-40X. To ensure run quality control, the slides were examined in the order of hematoxylin and eosin (H&E), control cell line slide, positive control tissue slides, negative control tissue, patient tissue slide stained using the NCR, and patient tissue slide stained using the PD-L1 primary antibody slides. For TPD-L1 scoring, a minimum of 100 viable tumor cells, negative and positive controls, were tested for quality control and test validity. TPD-L1 protein expression was determined by using Tumor Proportion Score (TPS), which is the percentage of viable tumor cells showing partial or complete membrane staining. The specimen was considered PD-L1 weak positive if membrane staining of TPS≥ 1% but < 50% of the viable tumor cells was observed, high PD-L1 (strongly positive) if TPS≥ 50% of the viable tumor cells exhibited membrane staining at any intensity. The intensity was evaluated as follows: No staining scored as “0”, Weak staining as “1+”, Moderate staining as “2+”, Strong staining as “3+”. The specimen was considered PD-L1 positive if ≥1% of the viable tumor cells exhibited membrane staining at any intensity (regardless of degree intensity, 1+, 2+, 3+). Representative TPD-L1 negative, TPD-L1<50% and TPD-L1>50% IHC images (400 x magnifications) are shown in Figure 1A.
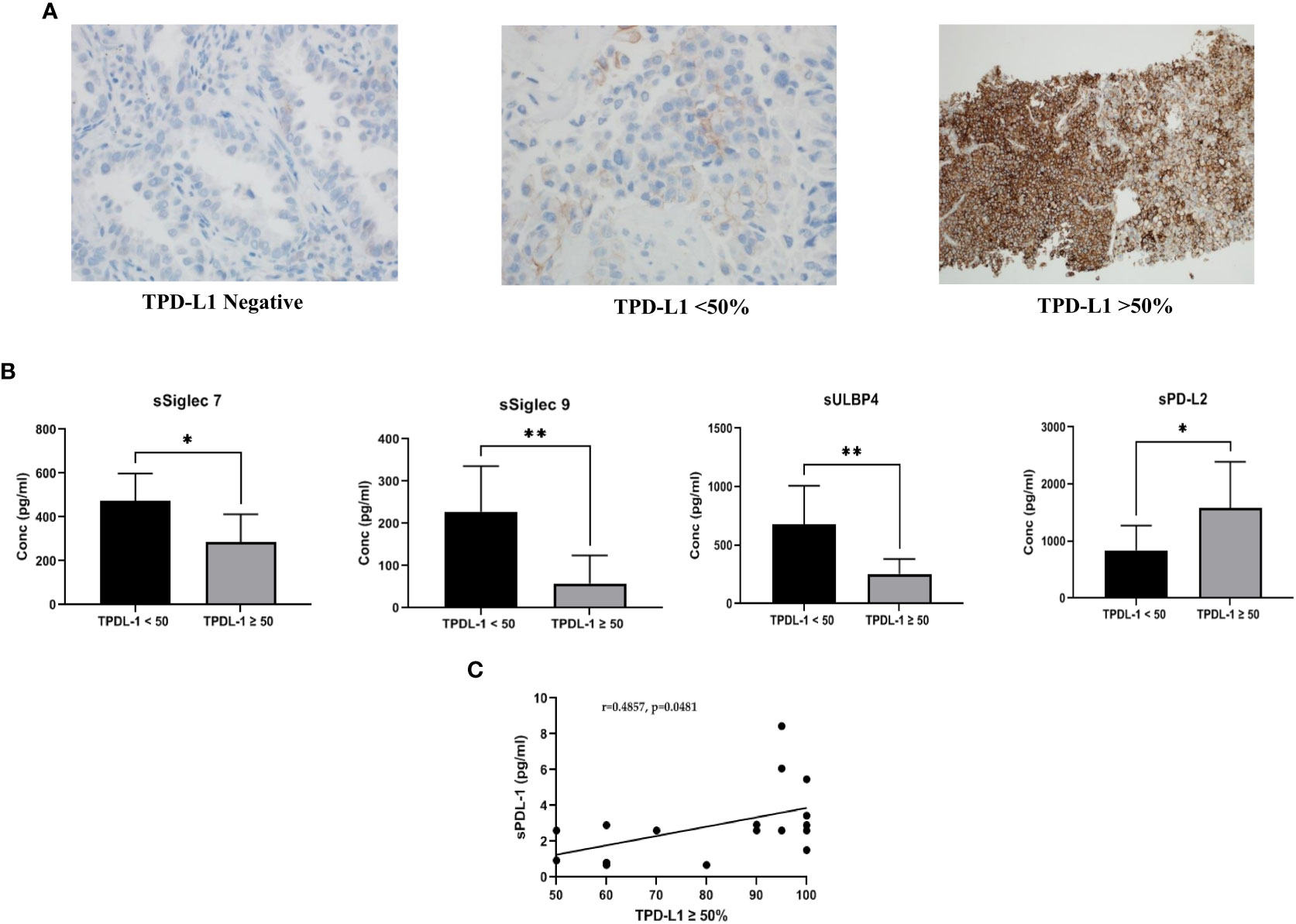
Figure 1 (A) Representative images of PD-L1 expression on tumor tissue: Tissue PD-L1 negative, tissue PD-L1<50% and tissue PD-L1>50% was observed by immunohistochemistry using DAKO PD-L1 IHC 22C3 pharmDx assay (B) Comparison of soluble immune biomarker expression between TPD-L1 low (<50%) and TPD-L1 high (>50%) groups: Significant down regulation of immune inhibitory checkpoint markers, sSiglec7 (p=0.011*), sSiglec9 (p=0.003**), sULBP4 (p=0.008**) and significant up regulation of sPD-L2 (p=0.015*) was observed in high TPD-L1 (>50%) expressing group (C) Pearson correlation showed significant moderate positive linear correlation (r =0.4857, p=0.048*) between the immune inhibitory marker, soluble PD-L1 and high TPD-L1(>50%) expressing group.
Next generation sequencing for determination of genetic aberrations
Next Generation Sequencing to detect genetic aberrations was performed in the CAP-accredited Department of Laboratory Medicine and Pathology (DLMP), HMC, Qatar, as part of routine diagnostic testing. The NGS Oncomine Focus Assay was performed for the samples. A total of 52 genes were tested to cover hotspots and copy number variations (CNVs) by DNA sequencing and most targeted gene fusions by RNA sequencing in a single workflow within the same NGS panel. The tumor area was collected from slides of a formalin-fixed paraffin-embedded (FFPE) specimen; this area was identified by the consultant pathologist from which genomic DNA/RNA was extracted and analyzed by using Next Generation Sequencing NGS – Ion S5 (Oncomine Focus Assay). The data generated were analyzed for alterations in the Hotspot genes and fusion drivers.
Clinical assessment of response
Response to treatment was assessed via PET-CT imaging data and clinical assessment per RECIST criteria. Progression-free survival (PFS) was defined as the period from blood sample collection (before the first dose of anti-PD-1/PD-L1/Combined chemoimmunotherapy) to the date of clinical and radiological disease progression or death by any cause observed within 6-8 months from the start of the treatment.
Statistical analysis
Statistical analysis was performed using GraphPad Prism version 9.3.2 (GraphPad Software, Inc., USA). Descriptive statistics including median (IQR), 95% CI and frequencies (%) were used for analysis of demographics and soluble biomarker concentrations. Mann-Whitney U test was used for analyzing differences in biomarkers expression levels in TPD-L1 groups, treatment response, and response in different treatment types. The correlation between TPD-L1 and soluble biomarkers was determined by Pearson correlation. Cut-off values of soluble biomarkers were estimated by receiver operating characteristic (ROC) curve. Association of cut-off values with demographic/clinical characteristics was performed by Fisher exact test. Survival curves were plotted using the Kaplan-Meier method and compared using the log-rank test. Univariate and multivariate analyses of Progression-free survival (PFS) were performed using the Cox Proportional Hazard regression model with hazards ratio (HR) and 95%CI. The results were considered statistically significant if p<0.05 was observed.
Results
Demographic and clinical characteristics
A total of 31 advanced-stage, metastatic NSCLC patients were enrolled in the study. The demographic and clinical characteristics of patients are shown in Table 1. Anti-PD-1 treatment was administered to 48% of the patients (Pembrolizumab 35%, Nivolumab 13%), while 10% of the patients were treated with anti-PD-L1 (Durvalumab). The remaining 42% of the patients were treated with combined chemoimmunotherapy (Pembrolizumab+Carboplatin+Pemetrexed). Response to treatment was observed in 48% (n=15) of the patients, while 52% of the patients (n=16) were categorized as non-responders (Table 1).
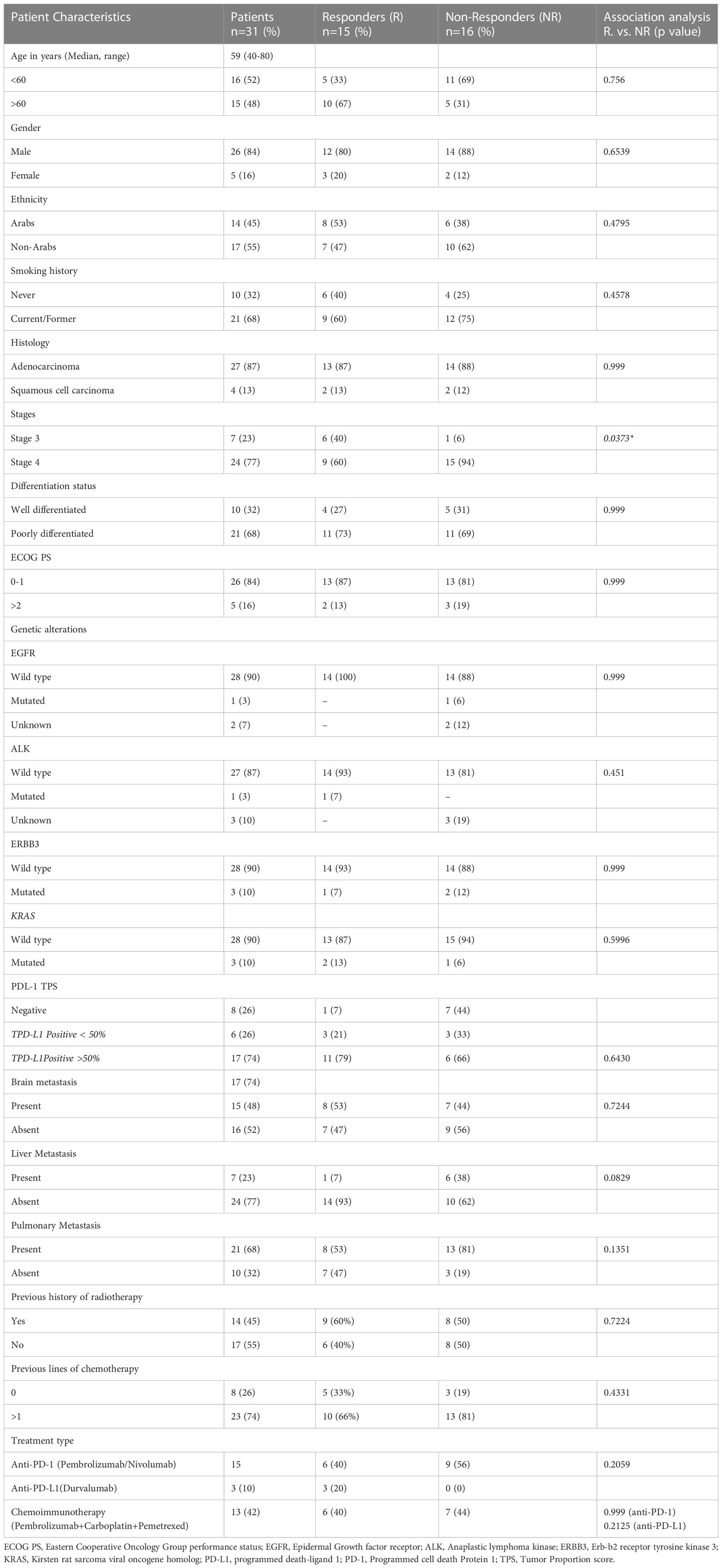
Table 1 Patient characteristics (all, responders and non-responders) and their association with treatment response.
Expression of soluble immune checkpoints/circulating tumor antigens and patients’ characteristics
The concentration of soluble immune checkpoints/circulating tumor antigens was successfully detected, and median + Interquartile (IQR) values of tested biomarkers are shown in Supplementary table 1.
Expression of TPD-L1 in enrolled patients
TPD-L1 expression was observed in 74% (n=23), while 26% (n=8) of the patients were found to be negative. For further analysis, TPD-L1 positive patients were stratified into two groups: TPD-L1<50% (n=6) and TPD-L1>50% (n=17). Representative images for TPD-L1 negative, TPD-L1<50% and TPD-L1>50% are shown in Figure 1A.
Soluble biomarkers and TPD-L1
Comparison of the expression level of soluble biomarkers between TPD-L1 negative vs. positive groups showed no significant change. However, comparison of TPD-L1<50% and >50% groups showed significant changes in various soluble markers. In the TPD-L1>50% group, significant downregulation of the immune inhibitory checkpoint markers, sSiglec7 (p=0.011*), sSiglec9 (p=0.003**), sULBP4 (p=0.008**) and significant up-regulation of sPD-L2 (p=0.015*) was observed (Figure 1B). The result indicates that high TPD-L1 expression could induce secretion of the soluble Natural Killer (NK) and T cell immune inhibitory checkpoint markers for immune regulation of anti-tumor response. The median (IQR) values of soluble biomarkers in TPDL-1<50% and TPD-L1>50% groups are given in supplementary Table 2.
Correlation between soluble immune checkpoint biomarkers and TPD-L1 >50% group
Pearson correlation analysis was performed to understand the linear relationship of TPD-L1 expression with up/down regulated soluble markers sSiglec7, sSiglec9, sULBP4, and sPD-L2. In addition to these markers, correlation analysis between TPD-L1 and sPD-L1 was also performed to determine if there is an existing relationship between the tissue and the secreted form of PD L1. No significant correlation between TPD-L1 >50% group and sSiglec7, sSiglec9, sULBP4, sPDL2 was noted. However, a moderate positive linear correlation (r =0.4857) was observed between the immune inhibitory marker, sPD-L1, and TPD-L1 >50%, with a significance value of p=0.048* (Figure 1C). This indicates that TPD-L1 expression levels are directly proportional to the concentration of sPD-L1 i.e., as TPD-L1 expression increases above 50%, the concentration of sPD-L1 also increases, making sPD-L1 a potential surrogate marker for longitudinal monitoring of TPD-L1.
Expression of soluble biomarkers in TPD-L1 >50% group and their role in treatment response
A comparison of the expression of soluble biomarkers with treatment response was performed in TPD-L1 groups. In TPD-L1 >50% group, comparison between responders (n=6) and non-responders (n=11) showed significant down regulation of immune inhibitory markers sPD-L2 (p=0.008**), sTIMD4 (p=0.040*), sNectin2 (p=0.012*) and CEA (p=0.024*) in responding patients (Figure 2). Our study results imply that in patients expressing TPD-L1 >50%, T cell immune checkpoint and circulating tumor antigens may play a role in immune modulation and tumor response. As such, these biomarkers may have utility as predictive biomarkers of response in this cohort. No significant expression of soluble biomarkers with treatment response was observed in TPD-L1 positive/negative groups and TPD-L1 <50% group (data not shown). The median (IQR) values of soluble biomarkers in responders vs. non-responders in the TPD-L1>50% group is given in Supplementary Table 2.
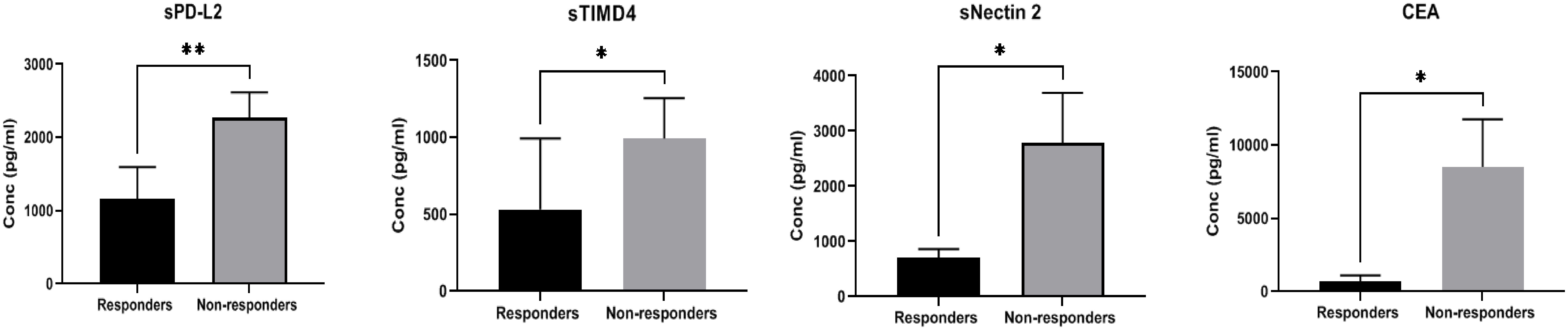
Figure 2 Comparison of soluble biomarker expression between responders (n=6) and non-responders (n=11) in high TPD-L1(>50%) group showed significant down regulation of immune inhibitory markers sPD-L2 (p=0.008**), sTIMD4 (p=0.040*), sNectin2 (p=0.012*) and CEA (p=0.024*) in responding patients.
Association of patient characteristics with treatment response
Based on imaging and clinical status as per RECIST criteria, the enrolled participants were stratified as responders (n=15) and non-responders (n=16). Association of treatment response with demographic/clinical characteristics showed significant association of disease stage 4 (p=0.037*) with non-responders. No other demographics/clinical characteristics were associated with treatment response (Table 1).
Soluble biomarkers and their association with treatment response in anti-PD-1/PD-L1 monotherapy and chemo-immunotherapy group
Treatment types utilized for patients included monotherapy with anti-PD1 (Nivolumab, Pembrolizumab), anti-PD-L1 (Durvalumab) and combination chemoimmunotherapy (Carboplatin +Pemetrexed+ Pembrolizumab). Due to the different treatment types, we stratified the patients into two groups. Group 1 comprised all patients who received anti-PD-1 and anti-PD-L1 monotherapy (anti-PD-1/PD-L1 monotherapy group: Nivolumab+Pembrolizumab+Durvalumab: n=18), whereas Group 2 included all patients who received combination chemoimmunotherapy (n=13).
The expression of soluble biomarkers was analyzed as follows a) responding patients in Group 1 (n=9) vs. Group 2 (n=6) and b) non-responding patients in Group 1 (n=9) vs. Group 2 (n=7). Interesting results were observed with both groups’ significant up/down-regulation of soluble biomarkers. In “responding” patients, the immune inhibitory checkpoint marker sPD-1, was significantly downregulated (p=0.012*) in Group 1 compared to Group 2. On the other hand, in “non-responding” patients, the immune suppressive biomarker S100A8/A9 (p=0.0084**) was significantly upregulated in Group 1 compared to Group 2. Our results clearly identify soluble biomarkers that can discriminate treatment response in different treatment groups and thus serve as predictive biomarkers (Figure 3A). Median (IQR) values of soluble biomarkers in responding and non-responding patients in Group 1 and Group 2 is given in Supplementary Table 3.
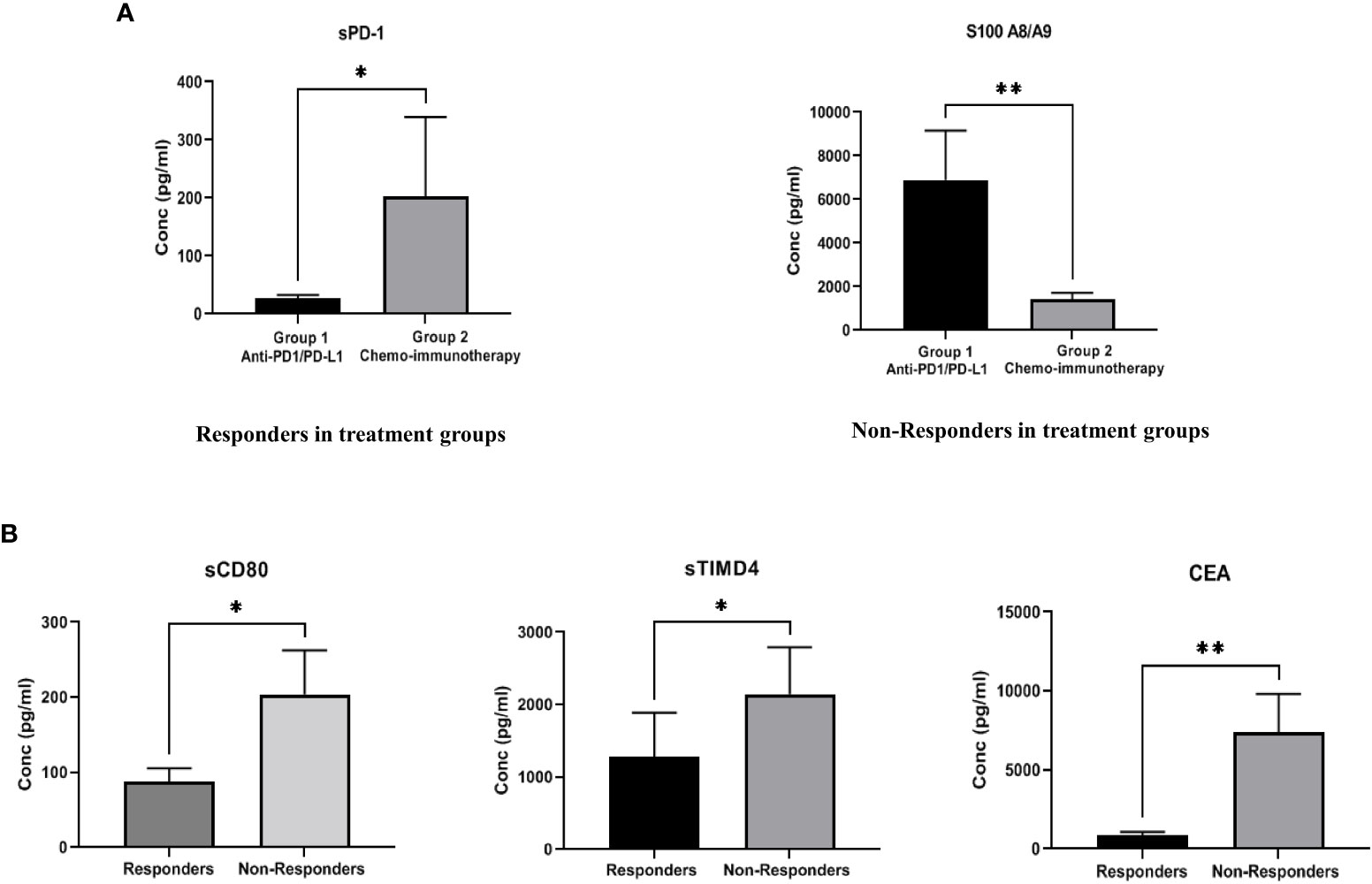
Figure 3 (A) Comparison of soluble biomarker expression between responders and non-responders in two treatment groups-Group 1 (anti-PD-1/PD-L1 monotherapy group), Group 2 (combination chemoimmunotherapy group). In “responding” patients, the immune inhibitory checkpoint marker sPD-1, was significantly down regulated (p=0.012*) in Group 1 as compared to Group 2. In “non-responding” patients, the immune suppressive biomarker S100A8/A9 (p=0.0084**) was significantly up regulated in Group 1 as compared to Group 2 (B) Comparison of soluble biomarker expression between all responders vs. all non-responders irrespective of treatment type. Significant down regulation of the immune inhibitory biomarkers sCD80 (p=0.023*), sTIMD4 (p=0.033*) and CEA (p=0.008**) in “responding” patients was observed.
Comparison of soluble biomarkers in responders and non-responders irrespective of treatment types
To identify generalized biomarkers of response in NSCLC patients treated with ICI, we compared the expression of soluble biomarkers in responders (n=15) vs. non-responders (n=16), irrespective of treatment groups. The results showed significant downregulation of the immune inhibitory biomarkers sCD80 (p=0.023*), sTIMD4 (p=0.033*), and CEA (p=0.008**) in “responding” patients indicating that these biomarkers may be playing a rather generalized but extensive role in immune modulation and treatment response to ICI therapy (Figure 3B). The median (IQR) values of soluble biomarkers between responders and non-responders, irrespective of treatment types, is given in supplementary Table 4.
Determination of optimal cut-off values of soluble biomarkers to discriminate responders from non-responders
The generalized soluble biomarkers that showed significant association with treatment response (irrespective of treatment types), including CD80, TIMD4, and CEA, were further analyzed by Receiver Operator Characteristic Curve (ROC) to determine their optimal cut-offs. It was found that the optimal cut-off value for soluble biomarkers to discriminate responders from non-responders were as follows: CD80 <91.7pg/ml (AUC: 0.7262, 95% CI: 0.535-0.917, sensitivity: 73%, specificity: 71%); TIMD4 <600pg/ml (AUC: 0.7250, 95% CI: 0.543 to 0.907, sensitivity: 75%, specificity: 66%); CEA <1614pg/ml (AUC: 0.778, 95% CI: 0.586-0.969, sensitivity: 67%, specificity: 83%) (Figure 4A). The cut-off values were further analyzed for their association with PFS in patients.
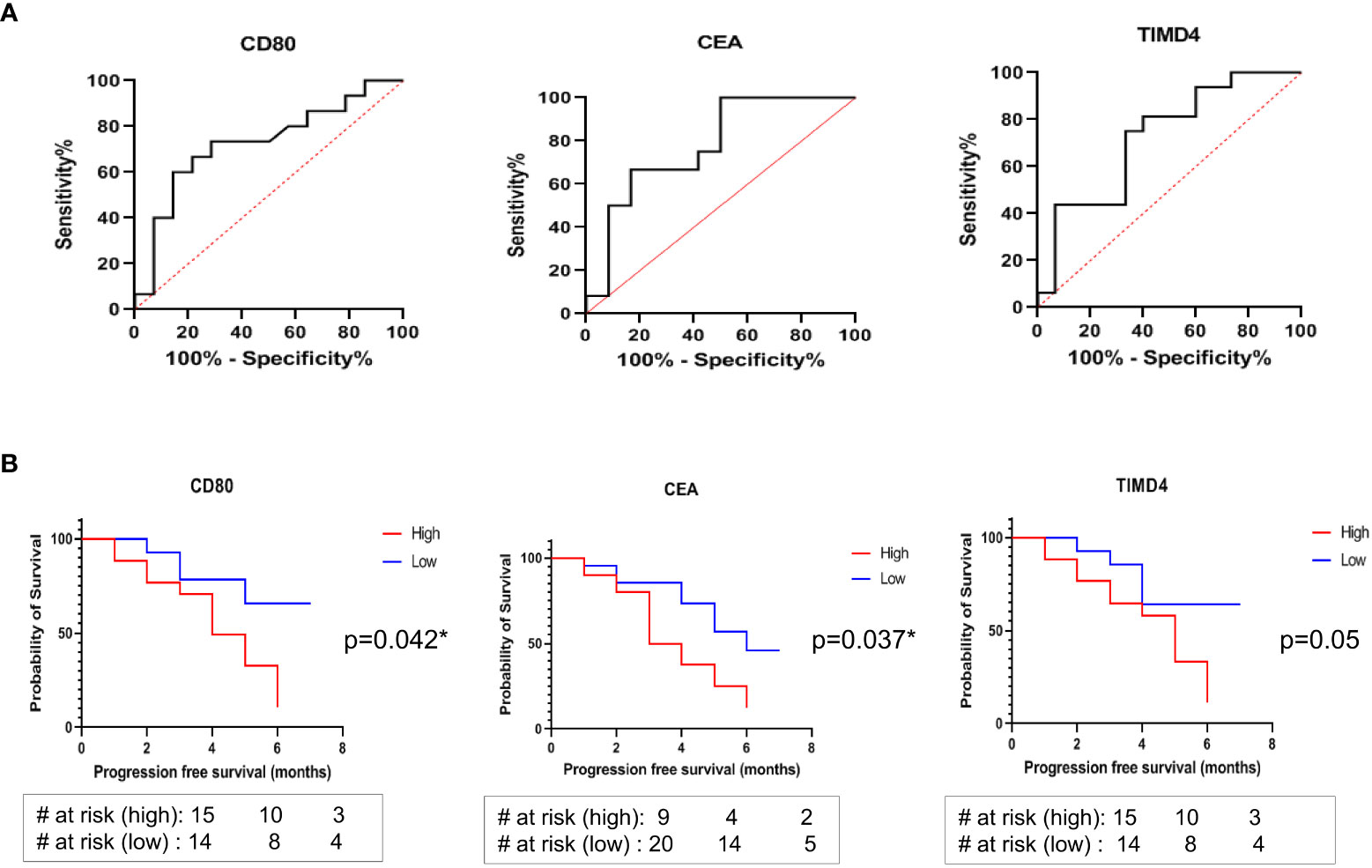
Figure 4 (A) ROC curves to discriminate responders from non-responders identified optimal cut-off values of soluble biomarkers: CD80 <91.7pg/ml, AUC: 0.7262, sensitivity: 73%, specificity: 71%; TIMD4 <600pg/ml, AUC: 0.7250, sensitivity: 75%, specificity: 66%; CEA <1614pg/ml AUC: 0.778, sensitivity: 67%, specificity: 83% (B) Kaplan Meier (log rank) analysis for association of cut-off values with progression free survival showed that patients having CD80 cut-off value of lower than 91.7 pg/ml (HR: 2.873, 95% CI: 1.078-7.658, p=0.042*) and CEA cut-off value of lower than 1614 pg/ml (HR: 2.566, 95% CI: 0.131-1.160, p=0.037*) were significantly associated with better progression free survival. No significant association of TIMD4 cut-off value with PFS was observed (HR: 2.699, 95% CI: 1.012-7.202, p=0.05).
Association of soluble immune checkpoint/circulating tumor antigens with progression free survival
The association of higher than cut-off and lower than cut-off values of the soluble biomarkers CD80, TIMD4, and CEA with PFS was determined using Kaplan Meier (log-rank) test. It was observed that patients having higher than cut-off values of CD80 and CEA had poor PFS (median survival of 4 months and 3.5 months, respectively). On the other hand, patients having CD80 cut-off value of lower than 91.7 pg/ml (HR: 2.873, 95% CI: 1.078-7.658, p=0.042*) and CEA cut-off value of lower than 1614 pg/ml (HR: 2.566, 95% CI: 0.131-1.160, p=0.037*) were significantly associated with better progression-free survival (Figure 4B). No significant association of TIMD4 cut-off value with PFS was observed (HR: 2.699, 95% CI: 1.012-7.202, p=0.05) (Figure 4B).
Cox proportional hazard regression analysis
To assess the impact of patient characteristics and soluble biomarkers as independent predictive factors of PFS, univariate and multivariate analysis by Cox Proportional Hazard Regression was performed. Multivariate analysis showed that age <60 years (HR 4.856 [95% CI: 1.244-23.10]; p=0.031) and CEA lower than the cut-off value of 1614 pg/ml (HR 0.1834 [95% CI: 0.04-0.65]; p=0.012) are independent predictors of better progression-free survival in patients (Table 2).
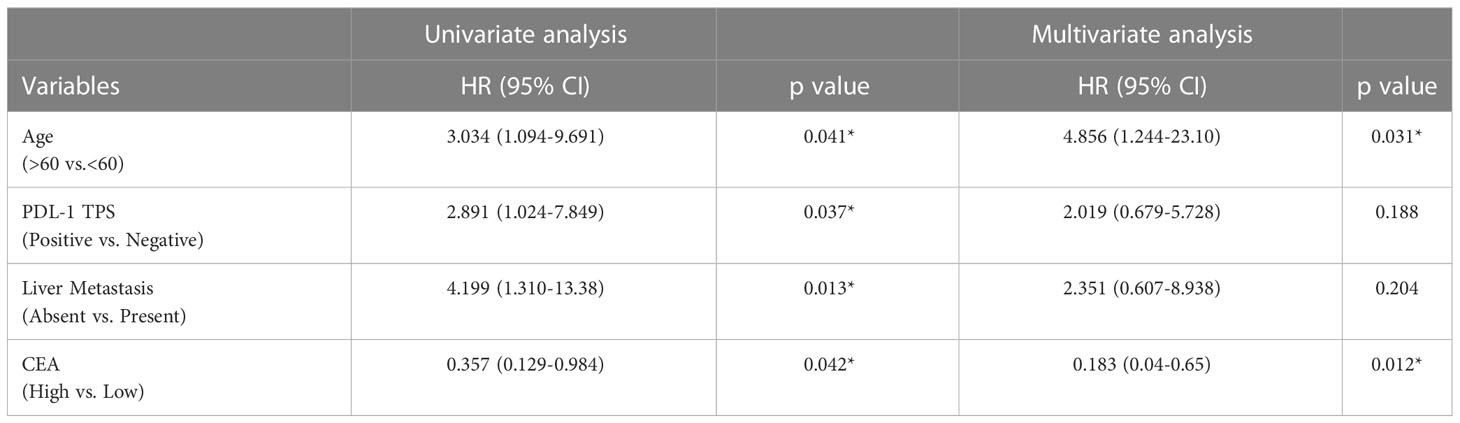
Table 2 Uni- and multivariate analysis of Progression free survival by Cox proportional Hazards model.
Discussion
We have identified in this study immune inhibitory/stimulatory soluble mediators as a potential surrogate/predictive biomarker for TPD-L1 status, treatment response, and progression-free survival in NSCLC patients treated with anti-PD-1/PD-L1. This a pilot study and the results showed a significant association of circulating tumor antigen, CEA, and several NK and T cell immune checkpoint markers with TPD-L1 expression and treatment response. To the best of our knowledge, this is the first study that extensively examines the role of NK/T cell immune checkpoint biomarkers/circulating tumor antigens with regards to TPD-L1 expression and treatment response in this cohort of patients.
We first aimed to identify and understand the role of various NK and T cell immune checkpoint serum markers as surrogate biomarkers/predictors of response with respect to TPD-L1 status. TPD-L1 is the only FDA approved companion diagnostic, predictive marker to assess the eligibility of NSCLC patients for ICI treatment (42). The ICI treatments for NSCLC include anti-PD-1, anti-PD-L1, or combined chemoimmunotherapy. Although TPD-L1 assessment is not a pre-requisite for all ICI treatments, several clinical trials have evaluated its role in predicting survival benefits for ICI-treated NSCLC patients (43). A large-scale meta-analysis on fifteen randomized controlled trials showed that patients with high TPD-L1 expression (>50%) exhibited improved overall response rates and subsequently benefitted from anti-PD-1/PD-L1 therapy (33). However, TPD-L1 expression could not predict survival benefits in patients on combined chemoimmunotherapy (33). This variability in predicting immunotherapy efficacy is possibly due to its inherent limitations, including inadequate tissue sampling, tumor heterogeneity, variable testing parameters, and evolutionary changes in TPD-L1 expression (induced by prior treatment lines), making its utility in clinical settings unclear. On the other hand, liquid biopsy, with its fundamental characteristics, such as noninvasiveness, incorporating tumor heterogeneity, ease of longitudinal monitoring via multiple sampling, and representation of systemic biomarker expression, could serve as an essential component to assess immunotherapy efficacy (44). Furthermore, its utility as a surrogate marker for TPD-L1 expression can help in longitudinal treatment monitoring. Our results showed that in patients with TPD-L1 >50% expression, significant downregulation of the soluble NK immune inhibitory markers Siglec-7 and-9, ULBP4 and significant upregulation of the soluble T cell immune inhibitory marker PD-L2 was observed. The role of these markers in immune regulation is well documented. Siglecs (Sialic acid-binding immunoglobulin-like lectins) are a family of receptors, present mainly on immune cells (45),. Siglec receptors recognize sialoglycan ligands on cell membranes and lead to eventual dephosphorylation of downstream immune pathways leading to inhibition of cellular activation (45). In tumors, the immune suppressive microenvironment helps facilitate this inhibition via aberrant expression of sialoglycan ligands on tumor cells and Siglec receptor overexpression on immune cells (46, 47). A strong receptor-ligand binding leads to immune inhibition and tumor escape (46, 47). Studies have shown that Siglec-7 and -9 are abundantly present in NK cells, and their interaction with sialoglycan ligands (on tumor cells) inhibits NK cell activation (48). Enhanced expression of siglec-7 and -9 in peripheral CD8+ T cells and tumor tissues have been observed in NSCLC, melanoma, and colon cancers (49, 50). Moreover, a study on NSCLC patients observed that high Siglec-9 expression on infiltrating CD8+ T cells was associated with increased expression of PD-L1, co-expression of inhibitory receptors PD-1, TIM-3, Lag3, and reduced production of inflammatory cytokines leading to an exhausted T cell phenotype and poor survival in patients (50–52). In lieu of this, our results show a different pattern. Serum-derived Siglec-7 and -9 were downregulated in patients exhibiting TPD-L1 >50% expression. Since we could not determine the expression of Siglecs in the tumor tissue, it is possible that Siglecs were overexpressed within the tumor tissue, subsequently leading to high PD-L1 expression. However, with their release into the circulation as soluble forms, other factors within the TME may have come into play for their downregulation and modulation. Down-regulation of Siglecs has been associated with augmentation of anti-tumor responses. In this, studies in mice deficient in Siglecs-E (the functional equivalent of human Siglec-9) showed increased in vivo killing of tumor cells and enhanced immunosurveillance (53). The same study showed that polymorphisms in human Siglec-9 contributed to its reduced binding to cancer cells, leading to improved survival in NSCLC patients (53). Therefore, we postulate that downregulation of soluble Siglecs in circulation in our cohort may indicate their role in the anti-tumor response. However, since no study on serum Siglecs and TPD-L1 has been reported, we could not corroborate our data with previous studies. Larger studies on this aspect could provide a better understanding of these Siglecs in TPD-L1 expression and immune regulation.
Another marker, UL16-binding protein 4 (ULBP4) was found to be significantly down regulated in patients expressing TPD-L1>50%. Mainly, NK cell−mediated cytotoxicity is regulated via the binding of NK group 2 member D (NKG2D) activating receptors with their ligands, such as the ULBP family (ULBP1-6) (54, 55). ULBP ligand expression is observed to be low in non-malignant cells (56, 57). However, in tumors, ULBP 1-6 ligands are aberrantly expressed, leading to modulation of anti-tumor responses (56, 57). Specifically, secreted forms of ULBP4 (generated via alternative splicing) have been reported to bind to NKG2D receptor, thus initiating its internalization for NK cell-acquired dysfunction and reduced NK cytotoxicity for tumor immune escape (58–60). Moreover, studies have reported that as ULBP4 ligand secretion increases, it induces the expansion of immune suppressive T cells, thus creating a favorable environment for tumor growth (61). On the other hand, studies on glioma and nasopharyngeal carcinoma have documented contrasting results, showing that upregulation of the cytokines TGF-β/IFN-γ and increased PD-L1 expression can lead to selective downregulation of ULBP3 and 4 to facilitate tumor escape (62–64). Our results agree with this notion showing that as PD-L1 expression increases, ULBP4 expression decreases, possibly playing its role in immune modulation. However, since the role of soluble ULBP4 with respect to PD-L1 expression in ICI-treated NSCLC patients has not been reported yet, we believe that our results could allow further studies to explore this aspect in detail.
In addition to NK markers, the T cell immune inhibitory checkpoint ligand PD-L2 was found to be upregulated in the TPD-L1 >50% group. PD-L2 that serves as second ligand for PD-1 and is involved in T cell regulation via decreased cytokine production and inhibition of T cell receptor (TCR)-mediated proliferation (65). Studies on lung and melanoma have shown that simultaneous expression of PD-L1 with PD-L2 is an important concept and could be one of the mechanisms utilized by tumor cells for immune evasion and tumor progression (66, 67). In fact, a study on ovarian cancer reported that blocking both PD-L1 and PD-L2 could help to overcome resistance to ICI treatment by unleashing the immune responses, thus indicating a clear role of both ligands in immune regulation (68). In our study, we observed simultaneous upregulation of sPD-L2 with TPD-L1 expression, indicating a possible synergistic effect for tumor response. Though tissue PD-L2 was not tested in our cohort, we assume that soluble PD-L2 (generated via splicing event of membrane-bound PD-L2) may indicate its presence within the tumor tissue. Also, as our result indicates concurrent up regulation of both markers (PD-L1 and PD-L2), we propose the utility of sPD-L2 as a surrogate marker for tissue PD-L1 and PD-L2. However, since limited studies on sPD-L2 are available in the literature, our assumption on the dualistic role of TPD-L1 and soluble PD-L2 in anti-tumor response needs further validation.
To understand if any linear relation exists between the up/down regulated soluble markers Siglec-7,-9, ULBP4 and PD-L2, Pearson correlation analysis was performed. We did not find any of these markers to correlate with TPD-L1. However, we did correlation analysis of sPD-L1 with TPD-L1 with the concept that since sPD-L1 is a spliced variant secreted by membrane-bound PD-L1, a linear relationship could exist between the two markers. Interestingly, correlation analysis between serum PD-L1 and TPD-L1 >50% showed a moderate positive relationship indicating that increased serum concentration of PD-L1 could be associated with increased PD-L1 expression in tissues. This is an important finding and allows the assumption that serum PD-L1 could be utilized as a surrogate marker for TPD-L1 status for longitudinal monitoring in patients on ICI treatment. Studies showing a significant positive correlation between the two markers have been reported, thus corroborating our observation (69, 70).
Furthermore, we aimed to identify specific biomarkers that could help stratify responding from non-responding patients in TPD-L1 >50% group. This is important as the identification of early biomarkers of response could help treatment management in this group. In responding patients with TPD-L1 >50% expression, the immune inhibitory markers sPD-L2, sTIMD4, sNectin2 and CEA were significantly downregulated. sPD-L2 is a spliced variant of membrane-bound PD-L2 that retains the ability to bind to its membrane-bound PD-1 receptor for immune regulation (71). Studies on the prognostic value of sPD-L2 in NSCLC are very limited. Only one study on 22 patients was carried out that evidenced better survival in patients with low pre-treatment sPD-L2 expression (18, 72). Moreover, co-expression of sPD-L2 with other soluble mediators such as PD-L1, CD137, TIM-3 BTLA-4 and CEA has been associated with favorable clinical response indicating a synergistic effect of these soluble mediators with each other to induce modulatory effects within the tumor microenvironment (18, 72). In our study, we observed downregulation of sPD-L2 with other soluble immune inhibitory markers such as sTIMD4, sNectin2, and CEA indicating the plausibility of a synergistic mechanism of soluble markers with each other thus enabling anti-tumor response in high tissue PD-L1 expressing patients. Further studies on these markers would enable a better understanding on this inference.
Besides sPD-L2, the NK associated ligand, sNectin2 was also found to be down regulated in high tissue PD-L1 responding patients. Nectin-2 is a immunoglobulin-like cell-to-cell adhesion protein that acts in a stimulatory or inhibitory manner Several studies on serum Nectin-2 have associated its overexpression with aggressiveness and metastasis in various cancers including colon, breast, esophageal and lung indicating its role as a prognostic and predictive biomarker in cancers (73–76). Moreover, blockade via anti-Nectin-2 monoclonal antibodies can induce antibody-dependent cellular cytotoxicity (ADCC) with robust anti-tumor response in breast and ovarian cancers, indicating its role in immune regulation (77, 78). Similar results were observed for Esophageal squamous cell carcinoma (ESCC) where knockdown of Nectin−2 in ESCC cell lines was associated with effective suppression of cell migration and invasion (75). Our results corroborate with these studies, and we postulate that high TPD-L1 could lead to immune-inflamed TME with downregulation of sNectin-2 as an anti-tumor response mechanism in responding patients of this cohort.
Our results also showed downregulation of the immune inhibitory marker TIMD4 (T Cell Immunoglobulin and Mucin Domain Containing 4) in TPD-L1 >50% group. TIMD4 is a cell-surface glycoprotein and in cancers including renal cell carcinoma, diffuse large B-cell lymphoma, pancreatic cancer, and glioma, expression of TIMD4 has been associated with enhanced apoptosis, reduced clonogenic ability of cancer cells, and better survival (79–82). In NSCLC, a comprehensive study documented the role of TIMD4 overexpression in the promotion of lung cancer cell proliferation and poor overall survival (83). Although the mechanism of TIMD4-mediated cancer progression remains unknown, the study showed that mutation in the TIMD4 RGD motif reduces cancer progression (83). We presuppose here (based on the mechanism of action of TIMD4) that high PD-L1 expression could have influenced the TME to induce downregulation of circulating TIMD4 as an active anti-tumor response mechanism in responding patients.
In addition to T and NK cell markers, we also found circulating tumor antigen CEA to be downregulated in the high TPD-L1 group. CEA is a serum glycoprotein and is a well-established prognostic and predictive tumor marker utilized for treatment monitoring in various cancers (84–86). In lung cancers, elevated CEA levels have been associated with tumor size, lymph node status, stage of disease, and treatment monitoring (87). Studies on ICI-treated NSCLC patients’ have associated high pre-treatment levels of CEA with worse PFS and OS (23, 25, 27). Moreover, a study on the correlation between CEA and PD-L1 has reported CEA as an independent prognostic indicator of worse OS in the PD-L1-positive group (88). On the other hand, a more specific role of CEA and immune modulation via PD-L1 has recently been documented (89–94). Several studies on T cell–bispecific antibody (CEA-TCB) targeting CEA and T cell receptor have shown interesting results in syngeneic tumor models, cell lines, in vivo humanized mice, and patients (89–94). CEA-TCB specifically induced T cell-mediated killing of CEA-expressing tumors by converting a non-inflamed PD-L1 negative tumor to a highly inflamed PD-L1 positive tumor (89–94). In our study, responding patients with high tissue PD-L1 showed down-regulation of CEA. Based on previous studies discussed above including low pre-treatment CEA associated with response and elevated PD-L1 expression inducing an immune hot/inflamed TME, we postulate that in our cohort high PD-L1 expression may have led to downregulation of CEA thus facilitating an efficient anti-tumor response.
The second aim of our study was to understand the role of soluble biomarkers as early predictors of response in NSCLC patients on ICI treatment. We stratified our analysis into various aspects, as discussed below. Firstly, we sought to identify early predictive biomarkers of response in patients on different therapeutic regimens (anti-PD-1/PD-L1 monotherapy group vs. chemoimmunotherapy group). In the anti-PD-1/PD-L1 monotherapy group, we identified two immune suppressive markers to be significantly associated with response. In responding patients, immune inhibitory checkpoint marker sPD-1 was found to be significantly downregulated. sPD-1 is a spliced variant of membranous PD-1 that retains its PD-L1 binding domain and can thus bind to membranous PD-L1 and PD-L2. This binding facilitates several immune modulatory effects, including early activation of CD8+ T cells, blocking of PD-L1 expression on tumor cells, and essentially reducing T cell inhibition (11, 95). On the other hand, some studies have documented its role in tumor immune escape via its ability to bind with membrane-bound PD-1 and in turn, compete with therapeutic anti-PD-1 monoclonal antibodies for their PD-1 binding site (95). The successful binding of sPD-1, instead of anti-PD-1 antibodies, leads to suboptimal efficacy/reduced bioavailability of therapeutic monoclonal antibodies (95). In ICI- treated NSCLC patients, the role of sPD-1 is still unclear and is described in a dynamic context (18, 19, 96). Mainly, dynamic increase in sPD-1 after anti-PD-1 treatment has been significantly associated with disease progression, indicating that as sPD-1 levels increase, it strengthens T cell inhibition and cancer immune evasion, thus resulting in poor outcome (18, 19, 96). Our result shows that in the anti-PD-1/PD-L1 group, low pre-treatment sPD-1 levels are associated with patients’ response to treatment. We postulate that low expression of sPD-1 may induce a weak affinity for membranous PD-1 thus allowing benefit to therapeutic anti-PD-1 antibodies to effectively bind and induce an active anti-tumor response. However, since we did not assess its modulation after treatment, we cannot comment on its dynamic role in immune regulation (as described in earlier studies). Our group is conducting a study on pre- and post-treatment sPD-1 levels which may give better insight into this aspect.
We also identified S100A8/A9 as a biomarker in non-responding patients on anti-PD-1/PD-L1 monotherapy. In tumors, pro-inflammatory S100A8/A9 production helps sustain MDSC accumulation for maintaining immune suppressive TME and facilitating tumor immune escape (97, 98). In lung cancers, S100A8/A9 overexpression has been implicated in the promotion of pre-metastatic niches, anchorage-independent invasion, and tumor cell proliferation (99, 100). Several studies on NSCLC have also associated overexpression of S100A8/A9 with poor survival and a high relapse rate (100–103). Moreover, the blockade of S100A8/A9 by anti-S100A8/A9 monoclonal antibodies demonstrated significant inhibition of lung metastasis in a mouse model (104). With respect to anti-PD-1 treatment, studies on head and neck, gastric, and melanoma have reported high levels of S100A8/A9 in non-responding patients indicating its role in ICI treatment resistance (105–108). However, studies on the role of S100A8/A9 in NSCLC patients treated with immune checkpoint inhibitors are limited. One single study, conducted on extracellular vesicle (EVs) proteins in 31 ICI-treated NSCLC patients, reported dynamic modulation of S100A8 with increased baseline associated with increased chemotaxis of myeloid cells (S100A8) while decreased expression (after treatment) was associated with inhibition of myeloid cell chemotaxis with induction of treatment response (109). Our result supports such a mechanism where the increased expression of S100A8/A9 may lead to increased chemotaxis of myeloid cells, and this resulted in immune suppression and resistance to the response. Additionally, results from other cancers (described above) corroborate with our study findings indicating the significance of S100A8/A9 as a novel predictive biomarker in ICI-treated NSCLC patients.
Having identified discriminatory markers in different treatment types, we intended to evaluate the predictive biomarkers of response irrespective of the treatment types used. This objective aimed to identify generalized biomarkers that could help to stratify responders vs. non-responders in patients on any type of ICI regimen. We observed downregulation of sCD80, CEA and sTIMD4 in responding patients. For TIMD4, the optimal value of <600 pg/ml was found to discriminate responders from non-responders with sensitivity and specificity of 75 to 66%, respectively. However, this optimal value could not be associated significantly with PFS. As discussed earlier, the mechanism of TIMD4 is still unclear. However, its low expression has been associated with better overall survival in NSCLC, indicating its potential as a prognostic/predictive biomarker (81, 83, 110). Since our results did not show its association with the response (as observed in previous studies), we hypothesize that synergistic expression of circulating immune modulatory molecules such as CD80, CEA, etc., with TIMD4 may be playing their role in influencing its association. Furthermore, it is possible that the role of TIMD4 as a predictive biomarker may be associated with its dynamic modulation in pre- and post-treatment samples.
In addition to sTIMD4, an optimal cut-off value of sCD80 level (<91.7 pg/ml) was found to be able to discriminate responders from non-responders and PFS. Briefly, soluble CD80 is generated via splicing of membranous CD80 (111). Though sCD80 lacks a transmembrane domain, it can still bind to CTLA-4, CD28 and activated T cells (111). Based on its ability to interact with both co-stimulatory (CD28) and co-inhibitory (CTLA-4) molecules, its role in immune modulation is contradictory. Its engagement with CD28 and PD-L1 is associated with T cell activation, while it’s binding with CTLA-4 can lead to co-inhibition of T cells leading to tumor immune escape and progression (112, 113). Moreover, sCD80 can compete with membrane-bound mCD80 on antigen-presenting cells thus reducing its co-stimulatory effects on T cells making the tumor invisible to the immune cells (114). Studies on prostate cancer, hematological malignancies, renal cell carcinoma, and NSCLC have associated low serum CD80 expression with progression-free survival while high levels are associated with enhanced invasiveness and poor prognosis (115–118). In this context, our results corroborate with previous findings. However, in our study, multivariate analysis did not identify sCD80 as an independent predictive biomarker in this cohort. This could be due to the inherent characteristic of this marker to form intricate, complex relationships with other checkpoints such as PD-L1, CD28, and CTLA4, making it a dynamic rather than an independent marker (119, 120). Larger comprehensive studies on sCD80 will help to provide a better understanding of this marker in ICI- treated NSCLC patients.
Our study identified CEA as a highly robust predictive biomarker in the ICI-treated NSCLC patient cohort. The optimal cut-off value of CEA <1614 pg/ml was associated with not only its ability to discriminate responders vs. non-responders but also with PFS and as an independent predictor of response. The role of CEA in its prognostic/predictive capacity has been documented for several cancers (23, 25, 27). However, limited studies have reported on this important tumor marker in ICI-treated NSCLC patients. Results from these studies showed high baseline CEA levels followed by a decrease of more than or equal to 20% within 4-6 weeks of immunotherapy treatment to be associated with response (23, 25, 27). Our study is the first to associate a specific cut-off, observed prior to treatment, to be associated with response prediction. As CEA is a routinely used marker in diagnostic settings, its utility in ICI treatment is complemented by this cut-off-value that could help in the early stratification of patients for efficient treatment management. Moreover, the mechanism of CEA in immune modulation (discussed earlier) further evidences its potential as a robust predictive biomarker in NSCLC patients treated with ICI.
The main limitation of this study is that we were unable to evaluate serum levels of immunosuppressive factors in a control group of individuals without NSCLC with approximately the same age and comorbidity profile as the patients. Since comorbidities such as atherosclerosis, inflammatory diseases, metabolic disorders, lifestyle and age are important factors of immune landscape change and can significantly influence the level of immunosuppressive mediators and cells in the blood, this could give a broader understanding of the immune mediators. However, due to the scope of study focusing only on patients and non-availability of healthy controls of same age and comorbidity profile as the patients, we were unable to assess this aspect.
Conclusions
Identifying soluble, non-invasive immune oncology and tumor antigens as biomarkers of response in ICI treated-NSCLC cohort is an emerging and exciting field that can help better understand immune regulatory mechanisms and their role in anti-tumor responses. This understanding can help to stratify responding patients from non-responding ones early in the treatment timeline thus aiding in robust treatment management. We were able to identify NK/T cell markers as biomarkers for TPD-L1 and CEA as robust predictive biomarkers of response in the ICI-treated NSCLC patient cohort. We have presented several novel early biomarkers concerning TPD-L1 expression and treatment response that have not been reported in previous studies, which is the main strength of this study. However, limitations of the study include a small sample size in a single-center study. We tried to overcome these limitations with robust analysis with recommendation that our study results serve as a foundation for large-scale studies for better patient stratification and management.
Data availability statement
The original contributions presented in the study are included in the article/Supplementary Material. Further inquiries can be directed to the corresponding author.
Ethics statement
The studies involving human participants were reviewed and approved by The Institutional Review Board, Medical Research Center, Hamad Medical Corporation, Doha, Qatar. The patients/participants provided their written informed consent to participate in this study.
Author contributions
Conceptualization, supervision, validation: AR, RM, AK, SD. Data curation, formal analysis, Software: AR, MI. Funding acquisition: AR, SD. Investigation, methodology, resources: AR, SA, AZ, SH, KP, AA-S, IA-B, WA, RA-A, SAS, SH. Project administration: AR, AP, SV, SH, MT. Visualization, roles/writing - original draft: AR. Writing - review & editing: AR, MM, VP, SU, SD, UA. All authors contributed to the article and approved the submitted version.
Funding
This research was funded by Academic Health System, Medical Research Center, Hamad Medical Corporation, Doha, Qatar, grant number MRC-01-20-507 and the Article Processing Charges was funded by Academic Health System, Medical Research Center, Hamad Medical Corporation, Doha, Qatar. The funders were not involved in the study design, collection, analysis, interpretation of data, the writing of this article or the decision to submit it for publication.
Acknowledgments
We would like to acknowledge all the enrolled patients for their participation.
Conflict of interest
Authors AR, RM, AK, AZ, AP, SV, SH, KP, AA-S, D-AE, VP, MM, IA-B, WA, RA-A, SAS, SH, SU, UA, SD were employed by Hamad Medical Corporation.
The remaining authors declare that the research was conducted in the absence of any commercial or financial relationships that could be construed as a potential conflict of interest.
Publisher’s note
All claims expressed in this article are solely those of the authors and do not necessarily represent those of their affiliated organizations, or those of the publisher, the editors and the reviewers. Any product that may be evaluated in this article, or claim that may be made by its manufacturer, is not guaranteed or endorsed by the publisher.
Supplementary material
The Supplementary Material for this article can be found online at: https://www.frontiersin.org/articles/10.3389/fimmu.2023.1157100/full#supplementary-material
Glossary
References
1. Sung H, Ferlay J, Siegel RL, Laversanne M, Soerjomataram I, Jemal A, et al. Global cancer statistics 2020: GLOBOCAN estimates of incidence and mortality worldwide for 36 cancers in 185 countries. CA Cancer J Clin (2021) 71(3):209–49. doi: 10.3322/caac.21660
2. Siegel RL, Miller KD, Jemal A. Cancer statistics, 2019. CA Cancer J Clin (2019) 69(1):7–34. doi: 10.3322/caac.21551
3. Daga A, Ansari A, Patel S, Mirza S, Rawal R, Umrania V. Current drugs and drug targets in non-small cell lung cancer: limitations and opportunities. Asian Pac J Cancer Prev (2015) 16(10):4147–56. doi: 10.7314/APJCP.2015.16.10.4147
4. Twomey JD, Zhang B. Cancer immunotherapy update: FDA-approved checkpoint inhibitors and companion diagnostics. AAPS J (2021) 23(2):39. doi: 10.1208/s12248-021-00574-0
5. Berghmans T, Durieux V, Hendriks LEL, Dingemans AM. Immunotherapy: from advanced NSCLC to early stages, an evolving concept. Front Med (Lausanne) (2020) 7:90. doi: 10.3389/fmed.2020.00090
6. Sharma P, Hu-Lieskovan S, Wargo JA, Ribas A. Primary, adaptive, and acquired resistance to cancer immunotherapy. Cell (2017) 168(4):707–23. doi: 10.1016/j.cell.2017.01.017
7. Pilla L, Maccalli C. Immune profiling of cancer patients treated with immunotherapy: advances and challenges. Biomedicines (2018) 6(3):76. doi: 10.3390/biomedicines6030076
8. Matsuo N, Azuma K, Hattori S, Ohtake J, Kawahara A, Ishii H, et al. Association between soluble immune mediators and tumor responses in patients with nonsmall cell lung cancer treated with anti-PD-1 inhibitor. Int J Cancer (2019) 144(5):1170–9. doi: 10.1002/ijc.31923
9. Raza A, Merhi M, Relecom A, Fernandes Q, Inchakalody V, Gul ARZ, et al. Evolving dynamic biomarkers for prediction of immune responses to checkpoint inhibitors in cancer. Adv Precis Med Oncol (2020). doi: 10.5772/intechopen.96494
10. Gu D, Ao X, Yang Y, Chen Z, Xu X. Soluble immune checkpoints in cancer: production, function and biological significance. J Immunother Cancer (2018) 6(1):132. doi: 10.1186/s40425-018-0449-0
11. He L, Zhang G, He Y, Zhu H, Zhang H, Feng Z. Blockade of B7-H1 with sPD-1 improves immunity against murine hepatocarcinoma. Anticancer Res (2005) 25(5):3309–13.
12. Kuipers H, Muskens F, Willart M, Hijdra D, van Assema FB, Coyle AJ, et al. Contribution of the PD-1 ligands/PD-1 signaling pathway to dendritic cell-mediated CD4+ T cell activation. Eur J Immunol (2006) 36(9):2472–82. doi: 10.1002/eji.200635978
13. Song MY, Park SH, Nam HJ, Choi DH, Sung YC. Enhancement of vaccine-induced primary and memory CD8(+) T-cell responses by soluble PD-1. J Immunother (2011) 34(3):297–306. doi: 10.1097/CJI.0b013e318210ed0e
14. Costantini A, Julie C, Dumenil C, Helias-Rodzewicz Z, Tisserand J, Dumoulin J, et al. Predictive role of plasmatic biomarkers in advanced non-small cell lung cancer treated by nivolumab. Oncoimmunology (2018) 7(8):e1452581. doi: 10.1183/13993003.congress-2018.OA3302
15. Okuma Y, Wakui H, Utsumi H, Sagawa Y, Hosomi Y, Kuwano K, et al. Soluble programmed cell death ligand 1 as a novel biomarker for nivolumab therapy for non-small-cell lung cancer. Clin Lung Cancer (2018) 19(5):410–7 e1. doi: 10.1016/j.cllc.2018.04.014
16. Bonomi M, Ahmed T, Addo S, Kooshki M, Palmieri D, Levine BJ, et al. Circulating immune biomarkers as predictors of the response to pembrolizumab and weekly low dose carboplatin and paclitaxel in NSCLC and poor PS: an interim analysis. Oncol Lett (2019) 17(1):1349–56. doi: 10.3892/ol.2018.9724
17. Tiako Meyo M, Jouinot A, Giroux-Leprieur E, Fabre E, Wislez M, Alifano M, et al. Predictive value of soluble PD-1, PD-L1, VEGFA, CD40 ligand and CD44 for nivolumab therapy in advanced non-small cell lung cancer: a case-control study. Cancers (Basel) (2020) 12(2):473. doi: 10.3390/cancers12020473
18. Zizzari IG, Di Filippo A, Scirocchi F, Di Pietro FR, Rahimi H, Ugolini A, et al. Soluble immune checkpoints, gut metabolites and performance status as parameters of response to nivolumab treatment in NSCLC patients. J Pers Med (2020) 10(4):208. doi: 10.3390/jpm10040208
19. Ohkuma R, Ieguchi K, Watanabe M, Takayanagi D, Goshima T, Onoue R, et al. Increased plasma soluble PD-1 concentration correlates with disease progression in patients with cancer treated with anti-PD-1 antibodies. Biomedicines (2021) 9(12):1929. doi: 10.3390/biomedicines9121929
20. Hao C, Zhang G, Zhang L. Serum CEA levels in 49 different types of cancer and noncancer diseases. Prog Mol Biol Transl Sci (2019) 162:213–27. doi: 10.1016/bs.pmbts.2018.12.011
21. Moriyama J, Oshima Y, Nanami T, Suzuki T, Yajima S, Shiratori F, et al. Prognostic impact of CEA/CA19-9 at the time of recurrence in patients with gastric cancer. Surg Today (2021) 51(10):1638–48. doi: 10.1007/s00595-021-02248-y
22. Zhang J, Wei Q, Dong D, Ren L. The role of TPS, CA125, CA15-3 and CEA in prediction of distant metastasis of breast cancer. Clin Chim Acta (2021) 523:19–25. doi: 10.1016/j.cca.2021.08.027
23. Dal Bello MG, Filiberti RA, Alama A, Orengo AM, Mussap M, Coco S, et al. The role of CEA, CYFRA21-1 and NSE in monitoring tumor response to nivolumab in advanced non-small cell lung cancer (NSCLC) patients. J Transl Med (2019) 17(1):74. doi: 10.1186/s12967-019-1828-0
24. Lang D, Haslinger W, Akbari K, Scala M, Hergan B, Asel C, et al. Serum tumor marker dynamics as predictive biomarkers in NSCLC chemo-immunotherapy and mono-immunotherapy maintenance: a registry-based descriptive study. Lung Cancer (Auckl) (2020) 11:113–21. doi: 10.2147/LCTT.S286228
25. Zhang Z, Yuan F, Chen R, Li Y, Ma J, Yan X, et al. Dynamics of serum tumor markers can serve as a prognostic biomarker for Chinese advanced non-small cell lung cancer patients treated with immune checkpoint inhibitors. Front Immunol (2020) 11:1173. doi: 10.3389/fimmu.2020.01173
26. Clevers MR, Kastelijn EA, Peters BJM, Kelder H, Schramel F. Evaluation of serum biomarker CEA and Ca-125 as immunotherapy response predictors in metastatic non-small cell lung cancer. Anticancer Res (2021) 41(2):869–76. doi: 10.21873/anticanres.14839
27. Muller M, Hoogendoorn R, Moritz RJG, van der Noort V, Lanfermeijer M, Korse CM, et al. Validation of a clinical blood-based decision aid to guide immunotherapy treatment in patients with non-small cell lung cancer. Tumour Biol (2021) 43(1):115–27. doi: 10.3233/TUB-219007
28. Postow MA, Callahan MK, Wolchok JD. Immune checkpoint blockade in cancer therapy. J Clin Oncol (2015) 33(17):1974–82. doi: 10.1200/JCO.2014.59.4358
29. Abdel-Rahman O. Correlation between PD-L1 expression and outcome of NSCLC patients treated with anti-PD-1/PD-L1 agents: a meta-analysis. Crit Rev Oncol Hematol (2016) 101:75–85. doi: 10.1016/j.critrevonc.2016.03.007
30. Borghaei H, Paz-Ares L, Horn L, Spigel DR, Steins M, Ready NE, et al. Nivolumab versus docetaxel in advanced nonsquamous non-Small-Cell lung cancer. N Engl J Med (2015) 373(17):1627–39. doi: 10.1056/NEJMoa1507643
31. Carbone DP, Reck M, Paz-Ares L, Creelan B, Horn L, Steins M, et al. First-line nivolumab in stage IV or recurrent non-Small-Cell lung cancer. N Engl J Med (2017) 376(25):2415–26. doi: 10.1056/NEJMoa1613493
32. Zhao Q, Xie R, Lin S, You X, Weng X. Anti-PD-1/PD-L1 antibody therapy for pretreated advanced or metastatic nonsmall cell lung carcinomas and the correlation between PD-L1 expression and treatment effectiveness: an update meta-analysis of randomized clinical trials. BioMed Res Int (2018) 2018:3820956. doi: 10.1155/2018/3820956
33. Xu Y, Wan B, Chen X, Zhan P, Zhao Y, Zhang T, et al. The association of PD-L1 expression with the efficacy of anti- PD-1/PD-L1 immunotherapy and survival of non-small cell lung cancer patients: a meta-analysis of randomized controlled trials. Trans Lung Cancer Res (2019) 8(4):413–28. doi: 10.21037/tlcr.2019.08.09
34. Li C, Li C, Zhi C, Liang W, Wang X, Chen X, et al. Clinical significance of PD-L1 expression in serum-derived exosomes in NSCLC patients. J Transl Med (2019) 17(1):355. doi: 10.1186/s12967-019-2101-2
35. Oh SY, Kim S, Keam B, Kim TM, Kim DW, Heo DS. Soluble PD-L1 is a predictive and prognostic biomarker in advanced cancer patients who receive immune checkpoint blockade treatment. Sci Rep (2021) 11(1):19712. doi: 10.1038/s41598-021-99311-y
36. Yang Q, Chen M, Gu J, Niu K, Zhao X, Zheng L, et al. Novel biomarkers of dynamic blood PD-L1 expression for immune checkpoint inhibitors in advanced non-Small-Cell lung cancer patients. Front Immunol (2021) 12:665133. doi: 10.3389/fimmu.2021.665133
37. Relecom A, Merhi M, Inchakalody V, Uddin S, Rinchai D, Bedognetti D, et al. Emerging dynamics pathways of response and resistance to PD-1 and CTLA-4 blockade: tackling uncertainty by confronting complexity. J Exp Clin Cancer Res (2021) 40(1):74. doi: 10.1186/s13046-021-01872-3
38. Dermime S, Merhi M, Merghoub T. Editorial: dynamic biomarkers of response to anti-immune checkpoint inhibitors in cancer. Front Immunol (2021) 12:781872. doi: 10.3389/fimmu.2021.781872
39. Zhang N, Liang R, Gensheimer MF, Guo M, Zhu H, Yu J, et al. Early response evaluation using primary tumor and nodal imaging features to predict progression-free survival of locally advanced non-small cell lung cancer. Theranostics (2020) 10(25):11707–18. doi: 10.7150/thno.50565
40. Oitaben A, Fonseca P, Villanueva MJ, Garcia-Benito C, Lopez-Lopez A, Garrido-Fernandez A, et al. Emerging blood-based biomarkers for predicting immunotherapy response in NSCLC. Cancers (Basel) (2022) 14(11):2626. doi: 10.3390/cancers14112626
41. Wang M, Zhai X, Li J, Guan J, Xu S, Li YY, et al. The role of cytokines in predicting the response and adverse events related to immune checkpoint inhibitors. Front Immunol (2021) 12. doi: 10.3389/fimmu.2021.670391
42. Wang Y, Tong Z, Zhang W, Zhang W, Buzdin A, Mu X, et al. FDA-Approved and emerging next generation predictive biomarkers for immune checkpoint inhibitors in cancer patients. Front Oncol (2021) 11:683419. doi: 10.3389/fonc.2021.683419
43. Paz-Ares L, Luft A, Vicente D, Tafreshi A, Gumus M, Mazieres J, et al. Pembrolizumab plus chemotherapy for squamous non-Small-Cell lung cancer. N Engl J Med (2018) 379(21):2040–51. doi: 10.1056/NEJMoa1810865
44. Rossi G, Russo A, Tagliamento M, Tuzi A, Nigro O, Vallome G, et al. Precision medicine for NSCLC in the era of immunotherapy: new biomarkers to select the most suitable treatment or the most suitable patient. Cancers (Basel) (2020) 12(5):1125. doi: 10.3390/cancers12051125
45. Duan S, Paulson JC. Siglecs as immune cell checkpoints in disease. Annu Rev Immunol (2020) 38:365–95. doi: 10.1146/annurev-immunol-102419-035900
46. Chen Z, Yu M, Guo L, Zhang B, Liu S, Zhang W, et al. Tumor derived SIGLEC family genes may play roles in tumor genesis, progression, and immune microenvironment regulation. Front Oncol (2020) 10:586820. doi: 10.3389/fonc.2020.586820
47. Bull C, den Brok MH, Adema GJ. Sweet escape: sialic acids in tumor immune evasion. Biochim Biophys Acta (2014) 1846(1):238–46. doi: 10.1016/j.bbcan.2014.07.005
48. Jandus C, Boligan KF, Chijioke O, Liu H, Dahlhaus M, Demoulins T, et al. Interactions between siglec-7/9 receptors and ligands influence NK cell-dependent tumor immunosurveillance. J Clin Invest (2014) 124(4):1810–20. doi: 10.1172/JCI65899
49. Haas Q, Boligan KF, Jandus C, Schneider C, Simillion C, Stanczak MA, et al. Siglec-9 regulates an effector memory CD8(+) T-cell subset that congregates in the melanoma tumor microenvironment. Cancer Immunol Res (2019) 7(5):707–18. doi: 10.1158/2326-6066.CIR-18-0505
50. Stanczak MA, Siddiqui SS, Trefny MP, Thommen DS, Boligan KF, von Gunten S, et al. Self-associated molecular patterns mediate cancer immune evasion by engaging siglecs on T cells. J Clin Invest (2018) 128(11):4912–23. doi: 10.1172/JCI120612
51. Wherry EJ, Kurachi M. Molecular and cellular insights into T cell exhaustion. Nat Rev Immunol (2015) 15(8):486–99. doi: 10.1038/nri3862
52. Thommen DS, Schreiner J, Muller P, Herzig P, Roller A, Belousov A, et al. Progression of lung cancer is associated with increased dysfunction of T cells defined by coexpression of multiple inhibitory receptors. Cancer Immunol Res (2015) 3(12):1344–55. doi: 10.1158/2326-6066.CIR-15-0097
53. Laubli H, Pearce OM, Schwarz F, Siddiqui SS, Deng L, Stanczak MA, et al. Engagement of myelomonocytic siglecs by tumor-associated ligands modulates the innate immune response to cancer. Proc Natl Acad Sci U S A (2014) 111(39):14211–6. doi: 10.1073/pnas.1409580111
54. Eagle RA, Trowsdale J. Promiscuity and the single receptor: NKG2D. Nat Rev Immunol (2007) 7(9):737–44. doi: 10.1038/nri2144
55. Cosman D, Mullberg J, Sutherland CL, Chin W, Armitage R, Fanslow W, et al. ULBPs, novel MHC class I-related molecules, bind to CMV glycoprotein UL16 and stimulate NK cytotoxicity through the NKG2D receptor. Immunity (2001) 14(2):123–33. doi: 10.1016/S1074-7613(01)00095-4
56. Kong Y, Cao W, Xi X, Ma C, Cui L, He W. The NKG2D ligand ULBP4 binds to TCRgamma9/delta2 and induces cytotoxicity to tumor cells through both TCRgammadelta and NKG2D. Blood (2009) 114(2):310–7. doi: 10.1182/blood-2008-12-196287
57. Conejo-Garcia JR, Benencia F, Courreges MC, Khang E, Zhang L, Mohamed-Hadley A, et al. Letal, a tumor-associated NKG2D immunoreceptor ligand, induces activation and expansion of effector immune cells. Cancer Biol Ther (2003) 2(4):446–51. doi: 10.4161/cbt.2.4.479
58. Zoller T, Wittenbrink M, Hoffmeister M, Steinle A. Cutting an NKG2D ligand short: cellular processing of the peculiar human NKG2D ligand ULBP4. Front Immunol (2018) 9:620. doi: 10.3389/fimmu.2018.00620
59. Cao W, Xi X, Wang Z, Dong L, Hao Z, Cui L, et al. Four novel ULBP splice variants are ligands for human NKG2D. Int Immunol (2008) 20(8):981–91. doi: 10.1093/intimm/dxn057
60. Arreygue-Garcia NA, Daneri-Navarro A, del Toro-Arreola A, Cid-Arregui A, Gonzalez-Ramella O, Jave-Suarez LF, et al. Augmented serum level of major histocompatibility complex class I-related chain a (MICA) protein and reduced NKG2D expression on NK and T cells in patients with cervical cancer and precursor lesions. BMC Cancer (2008) 8:16. doi: 10.1186/1471-2407-8-16
61. Groh V, Smythe K, Dai Z, Spies T. Fas-ligand-mediated paracrine T cell regulation by the receptor NKG2D in tumor immunity. Nat Immunol (2006) 7(7):755–62. doi: 10.1038/ni1350
62. Xu Y, Zhou L, Zong J, Ye Y, Chen G, Chen Y, et al. Decreased expression of the NKG2D ligand ULBP4 may be an indicator of poor prognosis in patients with nasopharyngeal carcinoma. Oncotarget (2017) 8(26):42007–19. doi: 10.18632/oncotarget.14917
63. Zhang X, Rao A, Sette P, Deibert C, Pomerantz A, Kim WJ, et al. IDH mutant gliomas escape natural killer cell immune surveillance by downregulation of NKG2D ligand expression. Neuro Oncol (2016) 18(10):1402–12. doi: 10.1093/neuonc/now061
64. Eisele G, Wischhusen J, Mittelbronn M, Meyermann R, Waldhauer I, Steinle A, et al. TGF-beta and metalloproteinases differentially suppress NKG2D ligand surface expression on malignant glioma cells. Brain (2006) 129(Pt 9):2416–25. doi: 10.1093/brain/awl205
65. Latchman Y, Wood CR, Chernova T, Chaudhary D, Borde M, Chernova I, et al. PD-L2 is a second ligand for PD-1 and inhibits T cell activation. Nat Immunol (2001) 2(3):261–8. doi: 10.1038/85330
66. Larsen TV, Hussmann D, Nielsen AL. PD-L1 and PD-L2 expression correlated genes in non-small-cell lung cancer. Cancer Commun (Lond) (2019) 39(1):30. doi: 10.1186/s40880-019-0376-6
67. Obeid JM, Erdag G, Smolkin ME, Deacon DH, Patterson JW, Chen L, et al. PD-L1, PD-L2 and PD-1 expression in metastatic melanoma: correlation with tumor-infiltrating immune cells and clinical outcome. Oncoimmunology (2016) 5(11):e1235107. doi: 10.1080/2162402X.2016.1235107
68. Miao YR, Thakkar KN, Qian J, Kariolis MS, Huang W, Nandagopal S, et al. Neutralization of PD-L2 is essential for overcoming immune checkpoint blockade resistance in ovarian cancer. Clin Cancer Res (2021) 27(15):4435–48. doi: 10.1158/1078-0432.CCR-20-0482
69. Shimada Y, Matsubayashi J, Kudo Y, Maehara S, Takeuchi S, Hagiwara M, et al. Serum-derived exosomal PD-L1 expression to predict anti-PD-1 response and in patients with non-small cell lung cancer. Sci Rep (2021) 11(1):7830. doi: 10.1038/s41598-021-87575-3
70. Murakami S, Shibaki R, Matsumoto Y, Yoshida T, Goto Y, Kanda S, et al. Association between serum level soluble programmed cell death ligand 1 and prognosis in patients with non-small cell lung cancer treated with anti-PD-1 antibody. Thorac Cancer (2020) 11(12):3585–95. doi: 10.1111/1759-7714.13721
71. He XH, Liu Y, Xu LH, Zeng YY. Cloning and identification of two novel splice variants of human PD-L2. Acta Biochim Biophys Sin (Shanghai) (2004) 36(4):284–9. doi: 10.1093/abbs/36.4.284
72. Gao J, Qiu X, Li X, Fan H, Zhang F, Lv T, et al. Expression profiles and clinical value of plasma exosomal Tim-3 and galectin-9 in non-small cell lung cancer. Biochem Biophys Res Commun (2018) 498(3):409–15. doi: 10.1016/j.bbrc.2018.02.114
73. Ak N, Serilmez M, Üçüncü MZ, Bademler S, Vatansever S. Nectin-2 and nectin-4 adhesion molecules in patients with breast cancer. Turkish J Oncol (2021) 36(2):165–70. doi: 10.5505/tjo.2021.2697
74. Erturk K, Karaman S, Dagoglu N, Serilmez M, Duranyildiz D, Tas F. Serum nectin-2 and nectin-4 are diagnostic in lung cancer: which is superior? Wien Klin Wochenschr (2019) 131(17-18):419–26. doi: 10.1007/s00508-019-01537-4
75. Li M, Qiao D, Pu J, Wang W, Zhu W, Liu H. Elevated nectin-2 expression is involved in esophageal squamous cell carcinoma by promoting cell migration and invasion. Oncol Lett (2018) 15(4):4731–6. doi: 10.3892/ol.2018.7953
76. Karabulut M, Gunaldi M, Alis H, Afsar CU, Karabulut S, Serilmez M, et al. Serum nectin-2 levels are diagnostic and prognostic in patients with colorectal carcinoma. Clin Transl Oncol (2016) 18(2):160–71. doi: 10.1007/s12094-015-1348-1
77. Sim YH, Um YJ, Park JY, Seo MD, Park SG. A novel antibody-drug conjugate targeting nectin-2 suppresses ovarian cancer progression in mouse xenograft models. Int J Mol Sci (2022) 23(20):12358. doi: 10.3390/ijms232012358
78. Oshima T, Sato S, Kato J, Ito Y, Watanabe T, Tsuji I, et al. Nectin-2 is a potential target for antibody therapy of breast and ovarian cancers. Mol Cancer (2013) 12:60. doi: 10.1186/1476-4598-12-60
79. Shi B, Chu J, Huang T, Wang X, Li Q, Gao Q, et al. The scavenger receptor MARCO expressed by tumor-associated macrophages are highly associated with poor pancreatic cancer prognosis. Front Oncol (2021) 11:771488. doi: 10.3389/fonc.2021.771488
80. Li Y, Zhang PY, Yang ZW, Ma F, Li FX. TIMD4 exhibits regulatory capability on the proliferation and apoptosis of diffuse large b-cell lymphoma cells via the wnt/beta-catenin pathway. J Gene Med (2020) 22(8):e3186. doi: 10.1002/jgm.3186
81. Yano H, Motoshima T, Ma C, Pan C, Yamada S, Nakayama T, et al. The significance of TIMD4 expression in clear cell renal cell carcinoma. Med Mol Morphol (2017) 50(4):220–6. doi: 10.1007/s00795-017-0164-9
82. Li W, Li X, Xu S, Ma X, Zhang Q. Expression of Tim4 in glioma and its regulatory role in LN-18 glioma cells. Med Sci Monit (2016) 22:77–82. doi: 10.12659/MSM.894963
83. Zhang Q, Wang H, Wu X, Liu B, Liu W, Wang R, et al. TIM-4 promotes the growth of non-small-cell lung cancer in a RGD motif-dependent manner. Br J Cancer (2015) 113(10):1484–92. doi: 10.1038/bjc.2015.323
84. Nakamura Y, Shida D, Tanabe T, Takamizawa Y, Imaizumi J, Ahiko Y, et al. Prognostic impact of preoperatively elevated and postoperatively normalized carcinoembryonic antigen levels following curative resection of stage I-III rectal cancer. Cancer Med (2020) 9(2):653–62. doi: 10.1002/cam4.2758
85. Zhao W, Li X, Wang W, Chen B, Wang L, Zhang N, et al. Association of preoperative serum levels of CEA and CA15-3 with molecular subtypes of breast cancer. Dis Markers (2021) 2021:5529106. doi: 10.1155/2021/5529106
86. Baqar AR, Wilkins S, Staples M, Angus Lee CH, Oliva K, McMurrick P. The role of preoperative CEA in the management of colorectal cancer: a cohort study from two cancer centres. Int J Surg (2019) 64:10–5. doi: 10.1016/j.ijsu.2019.02.014
87. Nasralla A, Lee J, Dang J, Turner S. Elevated preoperative CEA is associated with subclinical nodal involvement and worse survival in stage I non-small cell lung cancer: a systematic review and meta-analysis. J Cardiothorac Surg (2020) 15(1):318. doi: 10.1186/s13019-020-01353-2
88. Cui Y, Li X, Du B, Diao Y, Li Y. PD-L1 in lung adenocarcinoma: insights into the role of (18)F-FDG PET/CT. Cancer Manag Res (2020) 12:6385–95. doi: 10.2147/CMAR.S256871
89. Sam J, Colombetti S, Fauti T, Roller A, Biehl M, Fahrni L, et al. Combination of T-cell bispecific antibodies with PD-L1 checkpoint inhibition elicits superior anti-tumor activity. Front Oncol (2020) 10:575737. doi: 10.3389/fonc.2020.575737
90. Del Rivero J, Donahue RN, Marte JL, Gramza AW, Bilusic M, Rauckhorst M, et al. A case report of sequential use of a yeast-CEA therapeutic cancer vaccine and anti-PD-L1 inhibitor in metastatic medullary thyroid cancer. Front Endocrinol (Lausanne) (2020) 11:490. doi: 10.3389/fendo.2020.00490
91. Bacac M, Klein C, Umana P. CEA TCB: a novel head-to-tail 2:1 T cell bispecific antibody for treatment of CEA-positive solid tumors. Oncoimmunology (2016) 5(8):e1203498. doi: 10.1080/2162402X.2016.1203498
92. Bacac M, Fauti T, Sam J, Colombetti S, Weinzierl T, Ouaret D, et al. A novel carcinoembryonic antigen T-cell bispecific antibody (CEA TCB) for the treatment of solid tumors. Clin Cancer Res (2016) 22(13):3286–97. doi: 10.1158/1078-0432.CCR-15-1696
93. Osada T, Patel SP, Hammond SA, Osada K, Morse MA, Lyerly HK. CEA/CD3-bispecific T cell-engaging (BiTE) antibody-mediated T lymphocyte cytotoxicity maximized by inhibition of both PD1 and PD-L1. Cancer Immunol Immunother (2015) 64(6):677–88. doi: 10.1007/s00262-015-1671-y
94. Osada T, Hsu D, Hammond S, Hobeika A, Devi G, Clay TM, et al. Metastatic colorectal cancer cells from patients previously treated with chemotherapy are sensitive to T-cell killing mediated by CEA/CD3-bispecific T-cell-engaging BiTE antibody. Br J Cancer (2010) 102(1):124–33. doi: 10.1038/sj.bjc.6605364
95. Khan M, Zhao Z, Arooj S, Fu Y, Liao G. Soluble PD-1: predictive, prognostic, and therapeutic value for cancer immunotherapy. Front Immunol (2020) 11:587460. doi: 10.3389/fimmu.2020.587460
96. Sorensen SF, Demuth C, Weber B, Sorensen BS, Meldgaard P. Increase in soluble PD-1 is associated with prolonged survival in patients with advanced EGFR-mutated non-small cell lung cancer treated with erlotinib. Lung Cancer (2016) 100:77–84. doi: 10.1016/j.lungcan.2016.08.001
97. Srivastava MK, Andersson A, Zhu L, Harris-White M, Lee JM, Dubinett S, et al. Myeloid suppressor cells and immune modulation in lung cancer. Immunotherapy (2012) 4(3):291–304. doi: 10.2217/imt.11.178
98. Zwadlo G, Bruggen J, Gerhards G, Schlegel R, Sorg C. Two calcium-binding proteins associated with specific stages of myeloid cell differentiation are expressed by subsets of macrophages in inflammatory tissues. Clin Exp Immunol (1988) 72(3):510–5.
99. Sumardika IW, Chen Y, Tomonobu N, Kinoshita R, Ruma IMW, Sato H, et al. Neuroplastin-beta mediates S100A8/A9-induced lung cancer disseminative progression. Mol Carcinog (2019) 58(6):980–95. doi: 10.1002/mc.22987
100. Hiratsuka S, Watanabe A, Sakurai Y, Akashi-Takamura S, Ishibashi S, Miyake K, et al. The S100A8-serum amyloid A3-TLR4 paracrine cascade establishes a pre-metastatic phase. Nat Cell Biol (2008) 10(11):1349–55. doi: 10.1038/ncb1794
101. Perego M, Tyurin VA, Tyurina YY, Yellets J, Nacarelli T, Lin C, et al. Reactivation of dormant tumor cells by modified lipids derived from stress-activated neutrophils. Sci Transl Med (2020) 12(572):eabb5817. doi: 10.1126/scitranslmed.abb5817
102. Liu Y, Cui J, Tang YL, Huang L, Zhou CY, Xu JX. Prognostic roles of mRNA expression of S100 in non-Small-Cell lung cancer. BioMed Res Int (2018) 2018:9815806. doi: 10.1155/2018/9815806
103. Huang H, Huang Q, Tang T, Gu L, Du J, Li Z, et al. Clinical significance of calcium-binding protein S100A8 and S100A9 expression in non-small cell lung cancer. Thorac Cancer (2018) 9(7):800–4. doi: 10.1111/1759-7714.12649
104. Kinoshita R, Sato H, Yamauchi A, Takahashi Y, Inoue Y, Sumardika IW, et al. Newly developed anti-S100A8/A9 monoclonal antibody efficiently prevents lung tropic cancer metastasis. Int J Cancer (2019) 145(2):569–75. doi: 10.1002/ijc.31982
105. Zhou X, Fang D, Liu H, Ou X, Zhang C, Zhao Z, et al. PMN-MDSCs accumulation induced by CXCL1 promotes CD8(+) T cells exhaustion in gastric cancer. Cancer Lett (2022) 532:215598. doi: 10.1016/j.canlet.2022.215598
106. Rad Pour S, Pico de Coana Y, Demorentin XM, Melief J, Thimma M, Wolodarski M, et al. Predicting anti-PD-1 responders in malignant melanoma from the frequency of S100A9+ monocytes in the blood. J Immunother Cancer (2021) 9(5):e002171. doi: 10.1136/jitc-2020-002171
107. Kwak T, Wang F, Deng H, Condamine T, Kumar V, Perego M, et al. Distinct populations of immune-suppressive macrophages differentiate from monocytic myeloid-derived suppressor cells in cancer. Cell Rep (2020) 33(13):108571. doi: 10.1016/j.celrep.2020.108571
108. Wagner NB, Weide B, Gries M, Reith M, Tarnanidis K, Schuermans V, et al. Tumor microenvironment-derived S100A8/A9 is a novel prognostic biomarker for advanced melanoma patients and during immunotherapy with anti-PD-1 antibodies. J Immunother Cancer (2019) 7(1):343. doi: 10.1186/s40425-019-0828-1
109. Brocco D, Lanuti P, Pieragostino D, Cufaro MC, Simeone P, Bologna G, et al. Phenotypic and proteomic analysis identifies hallmarks of blood circulating extracellular vesicles in NSCLC responders to immune checkpoint inhibitors. Cancers (Basel) (2021) 13(4):585. doi: 10.3390/cancers13040585
110. Chen S, Wang Y, Liu W, Liang Y, Wang Y, Wu Z, et al. N-glycosylation at Asn291 stabilizes TIM-4 and promotes the metastasis of NSCLC. Front Oncol (2022) 12:730530. doi: 10.3389/fonc.2022.730530
111. Kakoulidou M, Giscombe R, Zhao X, Lefvert AK, Wang X. Human soluble CD80 is generated by alternative splicing, and recombinant soluble CD80 binds to CD28 and CD152 influencing T-cell activation. Scand J Immunol (2007) 66(5):529–37. doi: 10.1111/j.1365-3083.2007.02009.x
112. Haile ST, Dalal SP, Clements V, Tamada K, Ostrand-Rosenberg S. Soluble CD80 restores T cell activation and overcomes tumor cell programmed death ligand 1-mediated immune suppression. J Immunol (2013) 191(5):2829–36. doi: 10.4049/jimmunol.1202777
113. Butte MJ, Keir ME, Phamduy TB, Sharpe AH, Freeman GJ. Programmed death-1 ligand 1 interacts specifically with the B7-1 costimulatory molecule to inhibit T cell responses. Immunity (2007) 27(1):111–22. doi: 10.1016/j.immuni.2007.05.016
114. Zang X, Allison JP. The B7 family and cancer therapy: costimulation and coinhibition. Clin Cancer Res (2007) 13(18 Pt 1):5271–9. doi: 10.1158/1078-0432.CCR-07-1030
115. Wang Q, He Y, Li W, Xu X, Hu Q, Bian Z, et al. Soluble immune checkpoint-related proteins in blood are associated with invasion and progression in non-small cell lung cancer. Front Immunol (2022) 13:887916. doi: 10.3389/fimmu.2022.887916
116. Wang Q, Ye Y, Yu H, Lin SH, Tu H, Liang D, et al. Immune checkpoint-related serum proteins and genetic variants predict outcomes of localized prostate cancer, a cohort study. Cancer Immunol Immunother (2021) 70(3):701–12. doi: 10.1007/s00262-020-02718-1
117. Frigola X, Inman BA, Lohse CM, Krco CJ, Cheville JC, Thompson RH, et al. Identification of a soluble form of B7-H1 that retains immunosuppressive activity and is associated with aggressive renal cell carcinoma. Clin Cancer Res (2011) 17(7):1915–23. doi: 10.1158/1078-0432.CCR-10-0250
118. Hock BD, Starling GC, Patton WN, Salm N, Bond K, McArthur LT, et al. Identification of a circulating soluble form of CD80: levels in patients with hematological malignancies. Leuk Lymphoma (2004) 45(10):2111–8. doi: 10.1080/10428190410001712199
119. Sugiura D, Maruhashi T, Okazaki IM, Shimizu K, Maeda TK, Takemoto T, et al. Restriction of PD-1 function by cis-PD-L1/CD80 interactions is required for optimal T cell responses. Science (2019) 364(6440):558–66. doi: 10.1126/science.aav7062
Keywords: non-small cell lung cancer, anti-PD-1, anti-PD-L1, tissue PD-L1, predictive soluble biomarkers, CEA
Citation: Raza A, Mohsen R, Kanbour A, Zar Gul AR, Philip A, Vijayakumar S, Hydrose S, Prabhu KS, Al-Suwaidi AK, Inchakalody VP, Merhi M, Abo El-Ella DM, Tauro MA, Akbar S, Al-Bozom I, Abualainin W, Al-Abdulla R, Sirriya SA, Hassnad S, Uddin S, Mohamed Ibrahim MI, Al Homsi U and Demime S (2023) Serum immune mediators as novel predictors of response to anti-PD-1/PD-L1 therapy in non-small cell lung cancer patients with high tissue-PD-L1 expression. Front. Immunol. 14:1157100. doi: 10.3389/fimmu.2023.1157100
Received: 02 February 2023; Accepted: 13 April 2023;
Published: 15 May 2023.
Edited by:
Eyad Elkord, University of Salford, United KingdomReviewed by:
Vasyl Nagibin, National Academy of Sciences of Ukraine, UkraineJonghwa Won, ABL Bio/Sang Hoon Lee, Republic of Korea
Dorota Kwapisz, Early Phase Institute, Poland
Copyright © 2023 Raza, Mohsen, Kanbour, Zar Gul, Philip, Vijayakumar, Hydrose, Prabhu, Al-Suwaidi, Inchakalody, Merhi, Abo El-Ella, Tauro, Akbar, Al-Bozom, Abualainin, Al-Abdulla, Sirriya, Hassnad, Uddin, Mohamed Ibrahim, Al Homsi and Demime. This is an open-access article distributed under the terms of the Creative Commons Attribution License (CC BY). The use, distribution or reproduction in other forums is permitted, provided the original author(s) and the copyright owner(s) are credited and that the original publication in this journal is cited, in accordance with accepted academic practice. No use, distribution or reproduction is permitted which does not comply with these terms.
*Correspondence: Said Demime, sdermime@hamad.qa