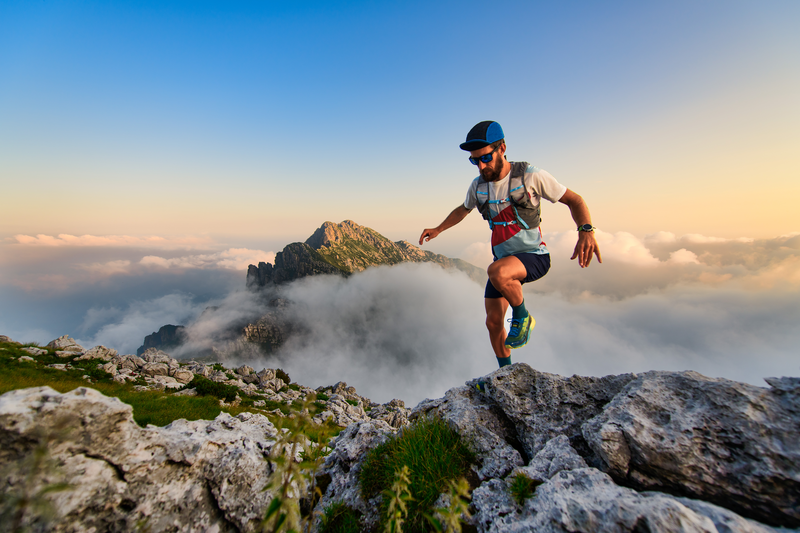
95% of researchers rate our articles as excellent or good
Learn more about the work of our research integrity team to safeguard the quality of each article we publish.
Find out more
ORIGINAL RESEARCH article
Front. Immunol. , 29 March 2023
Sec. Microbial Immunology
Volume 14 - 2023 | https://doi.org/10.3389/fimmu.2023.1155184
This article is part of the Research Topic Community Series in Immune Interactions with Pathogenic and Commensal Fungi: Volume II View all 7 articles
Introduction: The opportunistic filamentous fungus Aspergillus causes invasive pulmonary aspergillosis (IPA) that often turns into a fatal infection in immunocompromised hosts. However, the virulence capacity of different Aspergillus species and host inflammation induced by different species in IPA are not well understood.
Methods: In the present study, host inflammation, antimicrobial susceptibilities and virulence were compared among clinical Aspergillus strains isolated from IPA patients.
Results: A total of 46 strains were isolated from 45 patients with the invasive infection, of which 35 patients were diagnosed as IPA. Aspergillus flavus was the dominant etiological agent appearing in 25 cases (54.3%). We found that the CRP level and leukocyte counts (elevated neutrophilic granulocytes and monocytes, and reduced lymphocytes) were significantly different in IPA patients when compared with healthy individuals (P < 0.05). Antifungal susceptibilities of these Aspergillus isolates from IPA showed that 91%, 31%, 14%, and 14% were resistant to Fluconazole, Micafungin, Amphotericin B and Terbinafine, respectively. The survival rate of larvae infected by A. flavus was lower than larvae infected by A. niger or A. fumigatus (P < 0.05).
Discussion: Aspergillus flavus was the dominant clinical etiological agent. Given the prevalence of A. flavus in our local clinical settings, we may face greater challenges when treating IPA patients.
Aspergillus species are the most frequently reported filamentous fungi associated with human infections (1–3). The infections can be localized to the skin, nervous system, ear, eye, or can be systemically disseminated – all are referred to as invasive aspergillosis (IA) (4, 5). Invasive aspergillosis includes a family of closely-related severe life-threatening conditions occurring in patients with compromised immune systems with high fatality rates and the worst prognoses (6). Invasive pulmonary aspergillosis (IPA) is a common and severe disease of IA and diagnosis is challenging due to the non-specific nature of symptoms (7). The incidence of IPA today continues to increase with the introduction of advanced therapies involving bone marrow or solid organ transplantation to correct haematological malignancies and even in in immunocompetent patients with prolonged systemic steroids or deteriorating pulmonary functions (8, 9).
The majority of Aspergillus isolates reported so far can be classified into Aspergillus sections such as Fumigati, Circumdati, Flavi, Nidulantes, Nigri, Phialosimplex and Terrei. They are opportunistic pathogens in humans and animals (10–15). Given the fact that not all Aspergillus species possess the same biologic attributes and antifungal susceptibility patterns, identifying Aspergillus species at the species level is imperative for treatment initiation. Although Aspergillus has been studied by taxonomists for more than 200 years, challenges remain when using morphology-based methods for species identification, e.g., to discriminate among Aspergillus species in the Fumigati section (16, 17). To date, many non-morphological methods have been developed for Aspergillus identification by analyzing secondary metabolites, isozyme electrophoretic patterns, molecular sequencing and gene markers such as the Calmodulin (CaM) gene that could be appropriate identification markers for identification of Aspergillus (3, 5). With these molecular methods, subgenera and section of Aspergillus are continually being reframed and Aspergillus isolates at the same time are easily identified at the species level (18–22). The second advantage of molecular methods is to help with the identification of antifungal drug resistance. Drug-resistant Aspergillus has become a problem worldwide in recent years (23), which worsens the currently limited treatment options for invasive aspergillosis. Nevertheless, the early diagnosis of the Aspergillus pathogen at the species level and the rapid identification of drug resistance still fail to meet current clinical needs.
In recent years, Galleria mellonella (G. mellonella) larvae have been used as virulence study model mainly due to having the innate immune system that is structurally and functionally similar to that of mammals. It has been utilized as a host model to evaluate virulence of other fungal microbes such as Candida albicans (24), Candida auris (25), Conidiobolus coronatus (26), Beauveria bassiana (27), and Metarhiziun (28). When compared to other animal models, this model is cost-effective, easily operated, safe, and exempt from the usual requirements for ethical approval of vertebrate animal uses (29, 30).
In order to provide the more rational therapeutic strategies in clinical settings, we surveyed the prevalence of Aspergillus species in patients with IPA or other invasive fungal infections. In addition to profiling antifungal susceptibilities of these isolates, the degree of virulence and the host inflammation induced by these different strains were evaluated in the G. mellonella model.
A total of 46 fungal isolates were collected from 45 patients during June 2018 and April 2022 in Clinical Laboratory at Jining No. 1 People’s Hospital, Shandong (Jining, China). Fungal cultures were grown on Sabouraud Dextose Agar (SDA), yeast extract peptone dextrose agar (YPD), blood agar (BD) at 37 °C. Isolate stocks were stored in the Laboratory of Medical Mycology of Jining No. 1 People’s Hospital, Shandong, China. Among 45 patients, 35 patients were diagnosed as IPA according to diagnostic criteria of 2019 European Organization for the Research and Treatment of Cancer/Mycosis Study Group (EORTC/MSG) consensus (7). The serum samples collected from these 35 IPA patients and 38 healthy individuals were stored at -80°C. Routine blood tests for C-reactive protein (CRP) and counts of leukocyte including neutrophilic granulocytes, lymphocytes and monocytes were performed.
Genomic DNA was extracted from mature colonies grown on SDA plates according to the fungal DNA extraction instructions from the manufacturer (OMEGA) (21). The Calmodulin (CaM) gene was amplified using primer pairs (F, 5’-CCGAGTACAAGGARGCCTTC, R, 5’-CCGATRGAGGTCATRACGTGG) (31). The PCR conditions were set as follows: an initial denaturation step of 5 min at 94°C followed by 35 cycles of 30 s at 94°C, 50 s at 57°C and 1 min at 72°C, and a final elongation step of 7 min at 72°C. DNA sequencing was performed using an ABI PRISM® 3730XL DNA Analyser with a BigDyeTerminater Kit v. 3.1 (Invitrogen, USA) from the General Biology Company (Anhui, China). DNA sequencing was submitted to NCBI, and the accession numbers are listed in Table S1.
For phylogenetic reconstruction, newly generated sequences of CaM were initially subjected to BLAST search (BLASTn) in NCBI website (https://www.ncbi.nlm.nih.gov). Fungal species and their sequences from Visagie and Houbraken (3) were selected. The sequence alignments were conducted using MAFFT 7 (http://mafft.cbrc.jp/alignment/server/index.html), and manually edited in MEGA 7.0.21. Maximum Likelihood (ML) analysis was implemented on the CIPRES Science Gateway portal (https://www.phylo.org) using RAxML-HPC BlackBox 8.2.10. The GTR+GAMMA substitution model with 1000 bootstrap iterations was performed. Phylogenetic trees were viewed with FigTree v.1.3.1 and processed by Adobe Illustrator CS5.
35 strains from IPA patients were tested for antimicrobial susceptibilities. Conidial suspensions were diluted in order to obtain twice the final inoculum, which ranged from 0.4 × 104 to 5 × 104 cfu/mL, in a medium consisting of RPMI 1640 medium buffered at pH 7.0 with 0.165 M 3-(N-morpholine) propane-sulfonic acid (MOPS) (Gibco by Life Technologies). Candida parapsilosis (ATCC 22019) was used as a quality control.
Amphotericin B (Med Chem. Express Company, America), Micafungin sodium (Med Chem. Express Company, America), Voriconazole (Med Chem. Express Company, America), Itraconazole (Med Chem. Express Company, America), Terbinafine (Med Chem. Express Company, America) Furconazole (Med Chem. Express Company, America) were treated as clinical formulations and prepared according to the manufacturer’s guidelines and protocol CLSI M38-A3 in order to obtain working solutions. The concentrations ranged from 0.0313 to 16 ug/mL for Amphotericin B, from 0.015 to 8 ug/mL for Micafungin sodium, from 0.0313 to 16 ug/mL for Voriconazole, from 0.0313 to 16 ug/mL for Itraconazole, from 0.001 to 0.5 ug/mL for Terbinafine and from 0.25 to 128 ug/mL for fluconazole. Drugs were added into 96-well culture plates. While columns from 1 to 10 were filled with antifungal agents of the corresponding gradient, column 12 served as a negative control and column 11 as positive control without drug.
A total of 100 μL of the two-fold diluted conidial suspensions was inoculated into each well. Plates were incubated at 37°C for 46 to 50 h, and fungal growth in each well was assessed visually. The MIC values (minimal inhibitory concentration, MIC) of Itraconazole, Voriconazole and Terbinafine were determined by the turbidity reducing 80% or greater when compared with control wells without drug presence. For Fluconazole, the allowed turbidity reduced growth by 50% were compared to the control wells as well. For Micafungin, endpoints are often less clearly determined than those observed by amphotericin B. We also use endpoints of the minimum effective concentration (MEC) to improve reproducibility of MIC results. While the MIC is defined as the lowest concentration of drug that yields no growth, the MEC is the lowest concentration of drug that results in macroscopic changes in filamentous growth to microcolonies or granular growth when compared with growth control wells (12).
G. mellonella were purchased from Wax Moth Breeding, Tianjin, China and the larvae weighing around 250 mg were chosen for the infection experiment. Three strains CCJNMMM-E-0103, CCJNMMM-E-C0034 and CCJNMMM-E-C0119 from IPA patients respectively representing A. flavi, A. fumigati and A.nigri, were used to measure G. mellonella survival rates. Each 100 μL aliquot of conidia suspension at concentrations of 1×106 cfu/mL, 5×106 cfu/mL or 1×105 cfu/mL was injected into the last left proleg of each larva. For each tested concentration of each strain, 20 larvae were included and an additional 20 larvae injected with PBS were used as control group. Each treated group of larvae were then kept in Petri dishes at 37°C and surviving larvae were counted at 12, 24, 36 and 48 h post infection or PBS treatment. The larvae were considered dead when no movement was seen by visual inspection. The melanization of infected or PBS-treated G. mellonella larvae (set up according to the same infection schedules as for the survival test) was then evaluated by OD405 nm as previously reported, at 6, 12, 24 and 36 h, respectively (31).
The larvae infected with the strains of CCJNMMM-E-0103, CCJNMMM-E-C0034 and CCJNMMM-E-C0119 at 1×106 cfu/mL, 5×106 cfu/mL and 1×105 cfu/mL, respectively, were collected at 12 h for histopathological examination. Larvae were euthanized in 10% formalin that preserved and fixed the tissue anatomy at the same time. After embedded in paraffin, Periodic Acid-Schiff staining (PAS) was performed to analyze fungal structures in the tissues (25).
This study was approved by Jining No. 1 People’s Hospital, Shandong, China (2020-028). We also followed the available guidelines for strengthening the reporting of the Genetic Risk Prediction Studies. Written informed consent was obtained from all participants, or in the case of minors, from legal guardians.
A total of 46 clinical Aspergillus isolates were obtained from 45 patients, of which 35 patients were diagnosed as IPA. Based on CaM gene sequencing data, these 46 isolates were separated into five distinct clades in the phylogenic tree (Figure 1), which includes A. flavus accounting for 54.3%, A. fumigatus (19.6%), A. awamori (19.6%), A. terreus (4.3%) and A. tubingensis (2.2%). The characters of each clade on SDA, YPD, and BD plates after 5 days growth are shown in Figure 2. The results of blood routine examination in 35 IPA patients showed that the CRP level, numbers of total leukocytes, neutrophils, lymphocytes and monocytes are significantly higher in IPA than those of health controls (P <0.05) (Figure 3).
Figure 1 Phylogram of Diatrypaceae based on CaM gene sequence data. Phylogenetic analysis was performed using the maximum likelihood (ML). Bootstrapping phylogenetic trees support values above 70% are shown at the first and second positions, respectively. Thickened branches represent posterior probabilities above 0.95 from the BI (Bayesian inference). Ex-type strains are in bold, type species are denoted with the superscript “TS” and the disputable type species are denoted with the superscript “TSQ”. Strains from the current study are in blue. Red strain numbers indicated the strain from IPA.
Figure 2 The morphologic images of clinical isolated strains were visualized on SDA, YPD, and BD plates for 5 days. A1-A3, B1-B3, C1-C3, D1-D3 and E1-E3 present A. flavus, A. fumigatus, A. awamori, A. terreu, and A. tubingensis, respectively.
Figure 3 The blood routine examination. The blood routine test results from IPA and health people. (A, C) -reactive protein. (B) Leukocyte counts. (C) Neutrophilic granulocyte counts, (D) Lymphocyte counts and (E) monocytes count in blood samples. “*” present p < 0.05 and “***” present p < 0.001.
The MIC ranges of 35 strains from IPA patients were determined against several currently-used antifungal drugs in clinical setting, and the results are shown in Table 1. The lowest MIC value for Micafungin was 0.015 μg/mL, 0.006 μg/mL for Terbinafine, 0.0125 μg/mL for Itraconazole, 0.5μg/mL for Amphotericin B, 0.125 μg/mL for Voriconazole and 8 μg/mL for Fluconazole. However, there were 91% isolates (32/35) with MIC values greater than 128 μg/mL to Fluconazole, which is followed by 31% isolates (11/35) with MIC values above 8 μg/mL to Micafungin, 14% isolates (5/35) with MIC values greater than 0.5 μg/mL to Terbinafine or 16 μg/mL to Amphotericin B, respectively.
The survival rates of G. mellonella infected with each A. flavi, A. fumigati or A. nigri strain from IPA were compared and results are shown in Figure 3. We found that the survival rate of G. mellonella larvae infected with A. flavi, A. fumigati or A.nigri strain was dose-dependent. At the 24 h check point, the survival rates of G. mellonella larvae infected with A. flavi at 1×106 cfu/mL, 5×105 cfu/mL and 1×105 cfu/mL were 5%, 10% and 30%, respectively (P<0.05). When compared to 20%, 30% and 100% of A. nigri-infected larvae and 45%, 75% and 80% of A. fumigati-infected larvae, the survival rates of A. flavi-infected larvae are significantly lower at 24 h (P<0.05). The similar survival patterns among the three tested Aspergillus species were also observed at 36 h and 48 h check points (Figure 4).
Figure 4 The survival rates of G. mellonella infected by Aspergillus strains. (A) The survival rates of G. mellonella infected with A. flavi in 1×106 cfu/mL, 5×105 cfu/mL and 1×105 cfu/mL, respectively. (B) The survival rates of G mellonella infected with A.nigri in 1×106 cfu/mL, 5×105 cfu/mL and 1×105 cfu/mL, respectively. (C) The survival rates of G. mellonella infected with A. fumigati in 1×106 cfu/mL, 5×105 cfu/mL and 1×105 cfu/mL, respectively.
The melanization is a prominent immune response in many insects and arthropods that can be activated by the microbial infection (32). The degrees of melanization in G. mellonella larvae infected by A. flavi, A. fumigate and A.nigri isolates from IPA patients were analyzed at different time points as shown in Figure 5. At the concentrations of 1×106 cfu/mL and 5×105 cfu/mL infections, the melanization levels of the G. mellonella larvae infected by A. flavi were significantly higher than the controls at 6 h (P<0.05). By 12 h, the melanization levels of G. mellonella larvae infected by A.flavi with 1×106 cfu/mL, 5×105 cfu/mL and 1×105 cfu/mL were significantly higher than those infected by the same titer concentrations of A. fumigati or A. nigri (P<0.05). This higher melanization level induced by A. flavi at 1×106 cfu/mL or 5×105 cfu/mL (i.e., higher than A. fumigati and A. nigri) persisted at 24 h and 36 h (P<0.05). By contrast, the melanization levels of G. mellonella larvae infected by A. fumigati and A. nigri at 5×105 cfu/mL and 1×105 cfu/mL showed no significant difference at 24 h and 36 h (P >0.05) (Figure 5).
Figure 5 Melanization of G. mellonella larva. (A) and (B) represent the melanization of G. mellonella larva infected with A. flavi (E013), A. fumigati (C0034) and A. nigri (C0119) at 1×106 cfu/mL at 6 h and 12 h, respectively. (C) and (D) represent the melanization of G. mellonella larva infected with A. fumigati and A.nigri at 1×106 cfu/mL at 24 h and 36 h, respectively. (E) and (F) represent the melanization of G. mellonella larva infected with A. flavi (E013), A. fumigati (C0034) and A. nigri (C0119) at 5×105 cfu/mL at 6 h and 12 h, respectively. (G) and (H) represent the melanization of G. mellonella larva infected with A.fumigati and A.nigri at 5×105 cfu/mL at 24 h and 36 h, respectively. (I) and (J) represent the melanization of G. mellonella larva infected with A.flavi (E013), A.fumigati (C0034) and A.nigri (C0119) at 1×105 cfu/mL at 6 h and 12 h, respectively. (K) and (L) represent the melanization of G. mellonella larva infected with A.fumigati and A.nigri at 1×105 cfu/mL at 24 h and 36 h, respectively. “*” present p < 0.05, “**” present p < 0.01, “***” present p < 0.001, “****” present p < 0.0001 and “ns” present that there was no significant.
Histological examination of G. mellonella larvae infected by A. flavi, A. fumigate and A.nigri isolates from IPA patients was carried out to study the fungal load in the larval tissue. The results in Figure 6 show the larval tissues infected by 1×106 cfu/mL of A. flavi, A. fumigati and A.nigri at 12 h post infection. Under PAS staining, along with a large amount of conidia, the hyphae structures of A. flavi, A. fumigati or A. nigri were formed and easily distinguished from G. mellonella tissue, particular in A. flavi-infected larvae, the clustered hyphae form a hyphal ball in the subcuticular area of larvae. The length of hyphae for A. flavi, A. fumigate and A. nigri all continued to extend as time passed (data not shown).
Figure 6 Histopathology of infected G. mellonella. (A-D) represent the histopathology of G. mellonella infected by A.flavi, A.fumigati and A.nigri at 1×106 cfu/mL at 12 h, respectively.
Aspergillus spp. are ubiquitous in the environment, and as saprophytic fungi they will readily contaminate the environment and various food crops, thereby often directly affecting human health (33). In the present study, CaM gene sequencing was used to classify the 46 isolates from IA patients in Jining No. 1 People’s Hospital from Shandong province of China. To our surprise, our results showed that A. flavus was the most common etiological agent in our collection, followed by A. fumigatus, A. awamori, A. terreus, and A. tubingensis, which seems to differ from an earlier study showing that A. fumigatus and A. terreus were dominant in invasive aspergillosis (34). Indeed, Aspergillus strains isolated from our patients covered almost every sections described to be associated with invasive aspergillosis in a early study, including Fumigati, Flavi, Terrei, Usti, Nigri and Nidulantes (35).
IPA is the most severe type of pulmonary aspergillosis and evidence showed that immunocompromised hosts are particularly prone to IPA and cytokines play a pivotal role in the infection and progress (36). In the present study, we found that CRP levels and counts of total leukocytes, neutrophils, and monocytes in IPA patients were significantly higher than in patients in complete health (P<0.05), but lymphocytes were lower. CRP is a classical marker of inflammation and can be a predictor for the invasive aspergillosis (37). The elevation of CPR levels is similar to other reports in which inflammatory biomarkers were significantly increased in the patients with proven aspergillosis (38).
Aspergillus section Flavi includes 33 species and most of them are natural aflatoxin producers. In the present study, 25 clinical isolates (54.3%) were identified as A. flavus. As others have noted, A. flavus is the second leading cause of invasive aspergillosis after A. fumigatus (36). The cause of the observed dominance of Aspergillus section Flavi in our collection is not understood. Given the fact that our hospital serves patients mostly working on farms or elsewhere in the agriculture industry, they may be infected through contact with A. flavus-rich living environments such as the air and local common crops (wheat and peanuts). It has also been reported that section Flavi is often isolated from superficial infections such as keratitis, cutaneous infections and osteomyelitis after reported trauma (38). To our knowledge, the pathogenicity and antifungal susceptibility of A. flavus are not well studied compared to A. fumigatus. The antifungal susceptibility test in this study indicated that all A. flavus strains were susceptible to Terbinafine, Voriconazole and Itraconazole. A recent study has also shown that A. flavus strains were more susceptible to Voriconazole and Itraconazole than A. fumigatus (37). The absence of azole-resistant strains together with the greater prevalence of A. flavus in our local area may afford us some additional time to treat invasive aspergillosis by using azole drugs, but we should never lose sight of the fact that this time is borrowed, and is limited. However, we observed that 5 A. flavus isolates showed MICs > 8 µg/mL for Micafungin and >16 µg/mL for Amphotericin B. The differences in antifungal susceptibilities among these clinical isolates is not explored here, but may be related to genetic and specific treatment factors (39).
Fumigati section has been reclassified recently and at present contains 25 species (40). A. fumigatus is the most common human pathogen (36). In the present study, antifungal susceptibility tests indicated that all 9 A. fumigatus strains were still susceptible to Voriconazole and Itraconazole. These results are consistent with a previous study in which A. fumigatus strains had relatively low MICs to triazole drugs (41). Nigri section contains eight different species, among which A. acidus, A. awamori, A. brasiliensis, A. niger and A. tubingensis share morphological characteristics so similar (42) that a molecular technology is absolutely required to differentiate them at the species level. In the present study, A. awamori and A. tubingensis accounted for 19.6% and 2.2% in our collection. Our data also showed that A. tubingensis was the most common species among patients with type 2 diabetes mellitus (5). The antifungal susceptibility tests showed that A. awamori and A. tubingensis strains were more susceptible to Micafungin and Terbinafine, but resistant to Amphotericin B, Voriconazole, Itraconazole and Fluconazole. The resistances to Voriconazole and Itraconazole were stronger in A. tubingensis strains, suggesting a higher dose or alternative drug is required for effective treatment (5).
Our isolated A. flavus strain also presented a higher virulence than A. fumigatus and A. niger in the G. mellonella larvae model (43). As a species that produces a most popular aflatoxin (44), A. flaus-infected larvae showed a lower survival rate and more fungal structures in affected subcuticular areas. At the same time, a stronger host response (melanization level) was observed in A. flavus-infected larvae. However, whether this mycotoxin contributes to its virulence is unknown.
In summary, Aspergillus flavus was the dominant clinical etiological agent. The CRP level, the total numbers of leukocytes, neutrophilic granulocytes, and monocytes of IPA are significantly higher than those in healthy hosts – even though the reduced lymphocyte levels remain somewhat puzzling. We found that 91%, 31%, 14%, 14% of Aspergillus strains from IPA patients were resistant to Fluconazole, Micafungin, Amphotericin B and Terbinafine, respectively. The survival rates of larvae infected with A. flavus were much lower than those infected by A. niger or A. fumigatus (P < 0.05). Although the antimicrobial susceptibility and virulence data may not actually reflect in vivo effects, we believe this study provides some guidance for treatment choices in patients with IPA.
The datasets presented in this study can be found in online repositories. The names of the repository/repositories and accession number(s) can be found in the article/Supplementary Material.
All authors contributed to the article and approved the submitted version. BC performed the experiments and wrote the manuscript. GQ, ZY and NZ: Performed the experiments. DL Analyzed the data. DS: Wrote and revised the manuscript.
This work was supported in part by grants from the National Natural Science Foundation of China (NM82272358), the Key Research and Development Plan of Shandong Province (NM 2019GSF108191), the Natural Science Foundation of Shandong Province (NM ZR2020QH272 and 2022YXNS116), Doctoral Fund of Jining No.1 People’s Hospital (2019004) and Key Research and Development Plan of Jining (2022YXS116).
The authors declare that the research was conducted in the absence of any commercial or financial relationships that could be construed as a potential conflict of interest.
All claims expressed in this article are solely those of the authors and do not necessarily represent those of their affiliated organizations, or those of the publisher, the editors and the reviewers. Any product that may be evaluated in this article, or claim that may be made by its manufacturer, is not guaranteed or endorsed by the publisher.
The Supplementary Material for this article can be found online at: https://www.frontiersin.org/articles/10.3389/fimmu.2023.1155184/full#supplementary-material
1. Gianni C, Romano C. Clinical and histological aspects of toenail onychomycosis caused by aspergillus spp.: 34 cases treated with weekly intermittent terbinafine. Dermatology (2004) 209:104–10. doi: 10.1159/000079593
2. Balajee SA, Houbraken J, Verweij PE, Hong SB, Yaghuchi T, Varga J, et al. Aspergillus species identification in the clinical setting. Stud Mycol (2007) 59:39–46. doi: 10.3114/sim.2007.59.05
3. Visagie CM, Houbraken J. Updating the taxonomy of aspergillus in south africa. Stud Mycol (2020) 95:253–92. doi: 10.1016/j.simyco.2020.02.003
4. Shcab C, Yclb D, Cjlb D, Pskb D, Hywb D, Lthb D, et al. Invasive mold infections in acute leukemia patients undergoing allogeneic hematopoietic stem cell transplantation. J Microbiol Immunol Infect (2019) 52:973–82. doi: 10.1016/j.jmii.2018.09.006
5. Frias-De-Leon MG, Rosas-de Paz E, Arenas R, Atoche C, Duarte-Escalante E, Molina de Soschin D, et al. Identification of aspergillus tubingensis in a primary skin infection. J Mycol Med (2018) 28:274–8. doi: 10.1016/j.mycmed.2018.02.013
6. Jing R, Yang WH, Xiao M, Li Y, Hsueh PR. Species identification and antifungal susceptibility testing of aspergillus strains isolated from patients with otomycosis in northern china. J Microbiol Immunol Infect (2021) 55:282–90. doi: 10.1016/j.jmii.2021.03.011
7. Donnelly JP, Chen SC, Kauffman CA, Steinbach WJ, Baddley JW, Verweij PE, et al. Revision and update of the consensus definitions of invasive fungal disease from the european organization for research and treatment of cancer and the mycoses study group education and research consortium. Clin Infect Dis (2019) 71(6):1367–1376. doi: 10.1093/cid/ciz1008
8. Durieux MF, Melloul L, Jemel S, Roisin L, Marie-Laure D, Guillot J, et al. Galleria mellonella as a screening tool to study virulence factors of aspergillus fumigatus. Virulence (2021) 12:818–34. doi: 10.1080/21505594.2021.1893945
9. Naaraayan A, Kavian R, Lederman J, Basak P, Jesmajian S. Invasive pulmonary aspergillosis - case report and review of literature. J Community Hosp Intern Med Perspect (2015) 5(1):26322. doi: 10.3402/jchimp.v5.26322
10. Frisvad JC, Larsen TO. Extrolites of aspergillus fumigatus and other pathogenic species in aspergillus section fumigati. Front Microbiol (2016) 6:1485. doi: 10.3389/fmicb.2015.01485
11. Greiner K, PerOh D, Weig A, Rambold G. Phialosimplex salinarum, a new species of eurotiomycetes from a hypersaline habitat. Ima Fungus (2014) 5:161–72. doi: 10.5598/imafungus.2014.05.02.01
12. Subcommittee on Antifungal Susceptibility Testing of the ESCMID European Committee for Antimicrobial Susceptibility Testing. EUCAST technical note on the method for the determination of broth dilution minimum inhibitory concentrations of antifungal agents for conidia-forming moulds. Clin Microbiol Infect (2008) 14(10):982–4. doi: 10.1111/j.1469-0691.2008.02086.x
13. Sigler L, Sutton DA, Gibas CFC, Summerbell RC, Noel RK, Iwen PC. Phialosimplex, a new anamorphic genus associated with infections in dogs and having phylogenetic affinity to the. Trichocomaceae Med Mycol (2010) 48:335–45. doi: 10.3109/13693780903225805
14. Howard SJ. Multi-resistant aspergillosis due to cryptic species. Mycopathologia (2014) 178:435–9. doi: 10.1007/s11046-014-9774-0
15. Visagie CM, Varga J, Houbraken J, Meijer M, Kocsubé S, Yilmaz N, et al. Ochratoxin production and taxonomy of the yellow aspergilli (aspergillus section circumdati). Stud Mycol (2014) 78:1–61. doi: 10.1016/j.simyco.2014.07.001
16. Balajee SA, Marr KA. Phenotypic and genotypic identification of human pathogenic aspergill. Future Microbiol (2006) 1:435–45. doi: 10.2217/17460913.1.4.435
17. Alcazar-Fuoli L, Mellado E, Alastruey-Izquierdo A, Cuenca-Estrella M, Rodriguez-Tudela JL. Aspergillus section fumigati: antifungal susceptibility patterns and sequence-based identification. Antimicrob Agents Chemother (2008) 52:1244–51. doi: 10.1128/AAC.00942-07
18. Balajee SA, Weaver M, Imhof A, Gribskov J, Marr KA. Aspergillus fumigatus variant with decreased susceptibility to multiple antifungals. Antimicrob Agents Chemother (2004) 48:1197–203. doi: 10.1128/AAC.48.4.1197-1203.2004
19. Samson RA, Visagie CM, Houbraken J, Hong SB, Hubka V, Klaassen CH, et al. Phylogeny, identification and nomenclature of the genus aspergillus. Stud Mycol (2014) 78:141–73. doi: 10.1016/j.simyco.2014.07.004
20. Sanchez EKC, Almaguer CM, Duarte EE, Rojas FT, Frías-De-León MG, Reyes-Montes MDR. Aspergillus phylogenetic identification, diversity, and richness of aspergillus from homes in havana, cuba. Microorganism (2021) 9:115. doi: 10.3390/microorganisms9010115
21. Matsuzawa THY. Aspergillus takadae, a novel heterothallic species of aspergillus section fumigati isolated from soil in china. Mycoscience (2019) 60:354–60. doi: 10.1016/j.myc.2019.07.003
22. Hubka V, Barrs V, Dudova Z, Sklenar F, Kubátová A, Matsuzawa T, et al. Unravelling species boundaries in the aspergillus viridinutans complex (section fumigati): Opportunistic human and animal pathogens capable of interspecific hybridization. Persoonia (2018) 41:142–74. doi: 10.3767/persoonia.2018.41.08
23. Zhang YS, Ding G, Sun BD, Zhou YG, Zhao GZ, Chen AJ. Phylogeny of aspergillus section terrei with two new records from the china general microbiological culture collection centre. Phytotaxa (2018) 382:275–87. doi: 10.11646/phytotaxa.382.3.4
24. Howard SJ, Harrison E, Bowyer P, Varga J, Denning DW. Cryptic species and azole resistance in the aspergillus niger complex. Antimicrob Agents Chemother (2011) 55:4802–9. doi: 10.1128/AAC.00304-11
25. Kaskatepe B, Aslan Erdem S, Ozturk S, Safi Oz Z, Subasi E, Koyuncu M, et al. Antifungal and anti-virulent activity of origanum majorana l. essential oil on Candida albicans and in vivo toxicity in the galleria mellonella larval model. Molecules (2022) 27:663. doi: 10.3390/molecules27030663
26. Garcia-Bustos V, Ruiz-Saurí A, Ruiz-Gaitán A, Sigona-Giangreco IA, Cabañero-Navalon MD. Characterization of the differential pathogenicity of Candida auris in a galleria mellonella infection model. Microbiol Spectr (2021) 9:e0001321. doi: 10.1128/Spectrum.00013-21
27. Wrońska AK, Boguś MI. Harman and norharman, metabolites of the entomopathogenic fungus Conidiobolus coronatus (Entomophthorales), affect the serotonin levels and phagocytic activity of hemocytes, insect immunocompetent cells, in galleria mellonella (Lepidoptera). Cell Biosci (2019) . 9:29. doi: 10.1186/s13578-019-0291-1
28. Vertyporokh L, Hulas-Stasiak M, Wojda I. Host-pathogen interaction after infection of galleria mellonella with the filamentous fungus beauveria bassiana. Insect Sci (2020) 27:1079–89. doi: 10.1111/1744-7917.12706
29. Mukherjee K, Vilcinskas A. The entomopathogenic fungus metarhizium robertsii communicates with the insect host galleria mellonella during infection. Virulence (2017) 9:402–13. doi: 10.1080/21505594.2017.140519
30. Tsai C, Loh JMS, Proft T. Galleria mellonella infection models for the study of bacterial diseases and for antimicrobial drug testing. Virulence (2016) 7:214–29. doi: 10.1080/21505594.2015.1135289
31. Singkum P, Suwanmanee S, Pumeesat P, Luplertlop N. A powerful in vivo alternative model in scientific research: Galleria mellonella. Acta Microbiol Immunol Hung (2019) 66:31–55. doi: 10.1556/030.66.2019.001
32. Walsh TJ, Anaissie EJ, Denning DW, Herbrecht R, Kontoyiannis DP, Marr KA, et al. Treatment of aspergillosis: clinical practice guidelines of the infectious diseases society of america. Clin Infect Dis (2008) 46:327–60. doi: 10.1086/525258
33. Tang H. Regulation and function of the melanization reaction in drosophila. Fly (Austin) (2009) 3:105–11. doi: 10.4161/fly.3.1.7747
34. Norlia M, Jinap S, Nor-Khaizura MAR, Radu S, Samsudin NIP, Azri FA. Aspergillus section flavi and aflatoxins: occurrence, detection and identification in raw peanuts and peanut-based products along the supply chain. Front Microbiol (2019) 10:2602. doi: 10.3389/fmicb.2019.02602
35. Mayr A, Lass-FlRl C. Epidemiology and antifungal resistance in invasive aspergillosis according to primary disease - review of the literature. Eur J Med Res (2011) 16(4):153–7. doi: 10.1186/2047-783x-16-4-153
36. Samson RA, Hong SB, Frisvad JC. Old and new concepts of species differentiation in. aspergillus Med Mycol (2006) 44:S133–48. doi: 10.1080/13693780600913224
37. Donnelly JP, Chen SC, Kauffffman CA, Steinbach WJ, Baddley JW, Verweij PE. Revision and update of the consensus definitions of invasive fungal disease from the european organization for research and treatment of cancer and the mycoses study group education and research consortium. Clin Infect Dis (2020) 16:153–157. doi: 10.1093/cid/ciz1008
38. Ullmann AJ, Aguado JM, Arikan-Akdagli S, Denning DW, Groll AH, Lagrou K. Diagnosis and management of Aspergillus diseases: executive summary of the 2017 ESCMID-ECMM-ERS guideline. Clin Microbiol Infect (2018) 44:S133–S148. doi: 10.1016/j.cmi.2018.01.002
39. Chai L, Netea MG, Teerenstra S, Earnest A, Vonk AG, Schlamm HT. Early proinflammatory cytokines and c-reactive protein trends as predictors of outcome in invasive aspergillosis. J Infect Dis (2010) e1–e38. doi: 10.1086/656527
40. Frisvad JC, Hubka V, Ezekiel CN, Hong SB, Nováková A, Chen AJ, et al. Taxonomy of aspergillus section flavi and their production of aflatoxins, ochratoxins and other mycotoxins. Stud Mycol (2019) 93:1–63. doi: 10.1016/j.simyco.2018.06.001
41. Hedayati MT, Pasqualotto AC, Warn PA, Bowyer P, Denning DW. Aspergillus flavus: human pathogen, allergen and mycotoxin producer. Microbiology (2007) 153:1677–92. doi: 10.1099/mic.0.2007/007641-0
42. Tsang CC, Tang JYM, Ye H, Xing F, Lo SKF, Xiao C, et al. Rare/cryptic aspergillus species infections and importance of antifungal susceptibility testing. Mycoses Dis (2020) 63:1283–98. doi: 10.1111/myc.13158
43. Mohamadnia A, Salehi Z, Namvar Z, Tabarsi P, Pourabdollah-Toutkaboni M, Rezaie S, et al. Molecular identification, phylogenetic analysis and antifungal susceptibility patterns of aspergillusnidulans complex and aspergillusterreus complex isolated from clinical specimens. J Mycol Med (2020) 30:101004. doi: 10.1016/j.mycmed.2020.101004
Keywords: invasive pulmonary aspergillosis, inflammation, polyphasic taxonomy, antifungal susceptibility, virulence
Citation: Chen B, Qian G, Yang Z, Zhang N, Jiang Y, Li D, Li R and Shi D (2023) Virulence capacity of different Aspergillus species from invasive pulmonary aspergillosis. Front. Immunol. 14:1155184. doi: 10.3389/fimmu.2023.1155184
Received: 31 January 2023; Accepted: 13 March 2023;
Published: 29 March 2023.
Edited by:
Wanqing Liao, Shanghai Changzheng Hospital, ChinaReviewed by:
Maryam Roudbary, Iran University of Medical Sciences, IranCopyright © 2023 Chen, Qian, Yang, Zhang, Jiang, Li, Li and Shi. This is an open-access article distributed under the terms of the Creative Commons Attribution License (CC BY). The use, distribution or reproduction in other forums is permitted, provided the original author(s) and the copyright owner(s) are credited and that the original publication in this journal is cited, in accordance with accepted academic practice. No use, distribution or reproduction is permitted which does not comply with these terms.
*Correspondence: Dongmei Shi, c2hpZG9uZ21laTI4QDE2My5jb20=
Disclaimer: All claims expressed in this article are solely those of the authors and do not necessarily represent those of their affiliated organizations, or those of the publisher, the editors and the reviewers. Any product that may be evaluated in this article or claim that may be made by its manufacturer is not guaranteed or endorsed by the publisher.
Research integrity at Frontiers
Learn more about the work of our research integrity team to safeguard the quality of each article we publish.