- Institute of Biology, University of Szczecin, Szczecin, Poland
Acute liver failure (ALF) is a rare and severe disease, which, despite continuous advances in medicine, is still characterized by high mortality (65-85%). Very often, a liver transplant is the only effective treatment for ALF. Despite the implementation of prophylactic vaccinations in the world, the viral background of ALF is still a problem and leads to many deaths. Depending on the cause of ALF, it is sometimes possible to reverse this condition with appropriate therapies, which is why the search for effective antiviral agents seems to be a very desirable direction of research. Defensins, which are our natural antimicrobial peptides, have a very high potential to be used as therapeutic agents for infectious liver diseases. Previous studies on the expression of human defensins have shown that increased expression of human α and β-defensins in HCV and HBV infections is associated with a better response to treatment. Unfortunately, conducting clinical trials for ALF is very difficult due to the severity of the disease and the low incidence, therefore animal models are important for the development of new therapeutic strategies. One of the best animal models that has real reference to research on acute liver failure (ALF) is rabbit hemorrhagic disease in rabbits caused by the Lagovirus europaeus virus. So far, there have been no studies on the potential of defensins in rabbits infected with Lagovirus europaeus virus.
1 Introduction
Acute liver failure (ALF) is a serious consequence of sudden damage to liver cells that can develop over days or weeks and eventually lead to death. Although it is a relatively rare clinical entity, available data indicate an incidence of 1-6 cases per million people per year, accounting for 6% of liver-related deaths and 7% of orthotopic liver transplants in the United States (1). The distinguishing feature of ALF from acute chronic liver failure (ACLF) or decompensated cirrhosis is the absence of pre-existing liver disease. The etiology of ALF is diverse and may include paracetamol toxicity, drug-induced liver injury associated with prescription drugs, herbs and dietary supplements, hepatic ischemia, and autoimmune or viral liver diseases (2). It is estimated that 20-45% of ALF cases have an undetermined cause (2, 3). Despite the high mortality rate, reaching up to 80%, the current state of knowledge about ALF, the availability of treatment methods, including liver transplantation, has improved the prognosis of patients. Currently, liver transplantation is the main treatment method for ALF and remains the only method that enables recovery of patients who have not been cured spontaneously. It is estimated that ALF patients after liver transplantation have a one-year survival rate in the range of 65-70% (4). The incidence of ALF due to viral infection has decreased significantly in Europe, with only 19% of all ALF cases being viral. Despite this, viral hepatitis is still the most common cause of acute liver failure in Asia and Africa in all age groups and in children in Asia and South America (5). For this reason, the search for therapeutic methods of ALF based on antiviral activity is an important and promising direction of research.
Defensins are short antimicrobial peptides (AMPs) present in almost all organisms that are integral to innate immunity. The current state of knowledge about defensins shows that they are characterized by a broad spectrum of antimicrobial activity against bacterial, viral and fungal pathogens, but also exhibit multifaceted immunomodulatory functions. These features of defensins made them a subject of research for the development of therapeutic preparations for infectious diseases (6). Literature data indicate an important role of defensins in ALF-associated virus infections (7). As a result, the development of therapies based on these peptides may prove to be an important research direction that can significantly improve patient survival. However, in the case of clinical entities such as ALF, which are difficult to study clinically due to the rarity of occurrence and high mortality, studies based on animal models are crucial. They enable, above all, a better understanding of pathophysiology, but also the development of potential therapeutic methods (8). One of the proposed animal models of ALF is infection of rabbits (Oryctolagus cuniculus) with Lagovirus (L.) europaeus virus, which causes severe Rabbit Hemorrhagic Disease (RHD) (9).
In this review, we summarize the key information regarding ALF induced by viral infections, and also shed light on the use of defensins as potential therapeutic agents. Considering the usefulness of the rabbit model of L. europaeus infection in research on ALF, we also review the state of knowledge about defensins in rabbits. In this way, we outline the need for further research to use the data obtained in the future to develop therapeutic strategies for the treatment of ALF in humans.
2 Viral origin of acute liver failure
Acute liver failure (ALF) is a form of severe liver dysfunction in a patient with no previous history of liver disease. ALF is characterized by severe acute liver injury with encephalopathy in a short time. Defining the root cause of ALF is ambiguous. In developing countries, viral infections (hepatitis A, B and E) are the main cause. In turn, in developed countries, in the United States and most Western European countries, drug-induced damage is considered to be the dominant cause of ALF. This difference results from definitely better sanitary conditions in these countries, as well as the availability of preventive vaccinations (10, 11). In addition to the two most common causes of ALF mentioned above, there are a number of others. Other causes include hypoxia-induced liver damage, acute Budd-Chiari syndrome, veno-occlusive disease, Wilson’s disease, mushroom ingestion, sepsis, autoimmune hepatitis, acute fatty liver of pregnancy, HELLP syndrome (hemolysis, elevated liver enzymes, low platelet count). blood), heatstroke and malignancies (12). The viral background of ALF mainly concerns viral hepatitis infection. The most common virus that causes ALF is HBV (Hepatitis B Virus). ALF due to HBV infection may be the result of acute hepatitis, but also secondary or spontaneous reactivation of HBV in chronic carriers. Several theories have been proposed to explain how ALF is induced in the course of HBV infection (13). Studies have shown (14) that core promoter mutations with a phenotype that increases viral replication are registered in patients with more severe course of the disease and in chronic inflammation. Through increased viral replication, a stronger immune system response and prolonged infection may occur, which may be an important factor leading to ALF (15). An important factor mediating liver damage in HBV infection is the immune response of T lymphocytes (16). However, Farci et al. (17) demonstrated that the onset of ALF in HBV-infected patients was characterized by an overwhelming B-cell response and massive production of IgG and IgM by hepatic lobule-infiltrating plasma cells in the absence of significant T-cell activity. This suggests that B-cell-generated immunity is an important factor in the pathogenesis of ALF in infection HBV and distinguishes this condition from acute hepatitis B (17). An important aspect considered in understanding the substrate of ALF in HBV infection is also the activity of the apoptosis and necroptosis pathway. Gong et al. (18) demonstrated increased activity of these pathways in hepatic failure compared to normal liver by applying bioinformatics strategies, suggesting that these pathways may be central to the pathogenesis of HBV-ALF. HBV infection may be accompanied by co-infection or superinfection with Hepatitis D Virus (HDV). This is because HDV assembly and release depends on the presence of HBV as HDV does not produce its own proteins (19). It is indicated that in the presence of HDV the rate of ALF-HBV approaches 20% in the case of co-infection, and the occurrence of ALF-HBV in the case of superinfection during chronic HBV is also possible (20).
Estimates indicate that HAV (Hepatitis A Virus) accounts for 3% of ALF (15). However, recent studies have shown that HAV is the most common cause of pediatric acute liver failure (PALF) in low- and middle-income countries (21). The prognosis for patients with ALF due to HAV infection is good, with a spontaneous resolution rate of approximately 70%, while the remaining 30% require liver transplantation or death (22). The causality of the development of HAV-ALF is not clear and fully explained. It has been suggested that both the viral agent and host characteristics may influence the likelihood of developing severe disease. Fujiwara et al. (23) reported that nucleotide variations in the central part of the untranslated region (NTR) at the 5’ end of HAV may influence the severity of hepatitis A. On the other hand, age and the presence of concomitant chronic liver disease are important factors that increase the risk of severe infection (24). Vento et al. (25) also reported that in patients with chronic HCV (Hepatitis C Virus) infection, HAV superinfection was associated with an increased risk of acute course and death. There are also indications of a role for immune system components in liver injury, as CD8+ specific T cells have been demonstrated in the liver and blood of patients with acute HAV infection. It has been suggested that CD8+ T cells may lyse infected hepatocytes, but a correlation between the severity of the disease and the level of response mediated by these cells has not been demonstrated so far, therefore the unambiguous role of these cells requires further research (26). Studies in a mouse model have shown that the observed liver damage may result from mitochondrial apoptosis in liver cells as a result of MAVS and IRF3/7 signaling. In addition, classically activated CD8+ T cells, NKT cells, and pro-inflammatory Treg cells were associated with the severity of HAV infection (26–28).
Another important factor of ALF is HEV (Hepatitis E Virus), as it is indicated to be a common cause in Africa and Asia, and accounts for 0.4% of ALF cases in the USA (29). In turn, data from Germany indicate that 10-15% of ALF cases were associated with HEV infection (30). HEV infection is usually self-limiting in healthy patients, but in some cases it can develop into an acute form of the disease, which is observed in pregnant women (31). Additional factors that may affect the development of ALF-HEV are coexisting liver diseases and age (32). Undoubtedly, a very important aspect determining the course of HEV infection is the level of the host’s immune response (33). As the mother’s immune status changes during pregnancy to suit the fetus, this may be a key mechanism for the acute course of infection in pregnant women (34). Comparing women with ALF-HEV with a group of women without ALF, significant differences in TLR expression, inflammatory cytokine production and immune cell compartment were recorded (33). Srivastava et al. (34) also showed that antiviral cellular and humoral responses are related to the severity of HEV infection. In patients with a severe course, an increase in B lymphocytes specific for the HEV antigen, producing IgG antibodies, was recorded. This suggests that progressive liver damage may be mediated by antibodies. In addition, the observed infiltration of CD4+ and CD8+ T cells into the liver in patients with acute HEV infection and acute liver failure in pregnant women may indicate the involvement of these cells in the development of ALF by aggravating inflammatory damage to the liver tissue due to the activation of monocytes and macrophages releasing high levels of pro-inflammatory TNF-α (35).
Data on HCV (Hepatitis C Virus) infection with ALF show only isolated cases of this association. In a study by Rao et al. (36) only three cases out of 2,999 ALF or ALI (Acute Liver Infection) were considered to be related to HCV infection, and all patients had comorbidities. Progressive liver damage in the case of other viral hepatitis is the result of a strong immune reaction of the body. However, HCV eludes immune clearance by suppressing interferon signaling mediators, modulation of complement expression, and neutralizing antibodies, which may explain the rarity of ALF-HCV (37). In addition to viral hepatitis, herpes viruses can also cause ALF, but these cases are relatively less frequently reported. It is indicated that hepatitis caused by Epstein-Barr virus (EBV) infection can lead to ALF with a high mortality rate (38, 39). Thus, EBV is estimated to be responsible for 1.1% of ALF cases in children (40). The mechanism of liver injury in ALF-EBV is not entirely clear, but it is undeniable that liver injury in EBV infection is immunologically mediated by T-cell responses against EBV-infected B cells and CD8+ T-cells infected (41). In the study of Nakajima et al. (42) liver biopsy showed that ALF was due to monoclonal expansion and massive infiltration of EBV-infected CD8+ T cells. Similar observations were recorded by other authors (43, 44) in the case reports of children’s ALF after EBV infection, which confirms the involvement of immune elements in the pathogenesis of ALF-EBV. Herpes simplex virus (HSV)-1 and HSV-2 have also been reported to cause ALF (45, 46). Although rare, ALF-HSV has been reported in neonates, immunocompromised patients and pregnant women. Data indicate that HSV accounts for 0.8% of ALF cases and 1-2% of viral hepatitis (47). Available case reports report that human herpesvirus 6 (HHV-6) infection may also be associated with ALF (48, 49). There are speculations that HHV-6 may influence immunological events which, by disturbing liver homeostasis, lead to the occurrence of ALF (48). There are also isolated reports indicating infection with parvovirus B19 (50), cytomegalovirus (51) and Dengue virus (52) as the cause of ALF.
3 Rabbit model of acute liver failure
Acute hepatic failure is associated with a rapid deterioration of the patient’s liver function and is associated with high mortality, which makes it a rather problematic entity for clinical trials. For this reason, research based on various models is a very important direction. Most ALF models are artificial systems, which include surgical, pharmacological or immunogenic models. Immunogenic models include various viral hepatitis models (8). One of them is Lagovirus europaeus infection, which causes Rabbit Hemorrhagic Disease (RHD). Terblanche and Hickman (53) described the main criteria that an animal model of acute liver failure should meet: reversibility - treatment will result in the survival of some animals; repeatability – the obtained results can be replicated; the death of the animal occurs as a result of liver failure; therapeutic window; the size of the animal to allow the collection of adequate amounts of blood and tissue for testing; minimal risk to testing personnel. According to Tunon et al. (9) infection with L. europaeus virus fulfills all these criteria and is therefore a valid model for ALF. In addition to the changes observed in the liver following infection with L. europaeus being consistent with those manifested in humans during ALF, the similarities recorded also apply to physiological, histological and biochemical features (Table 1).
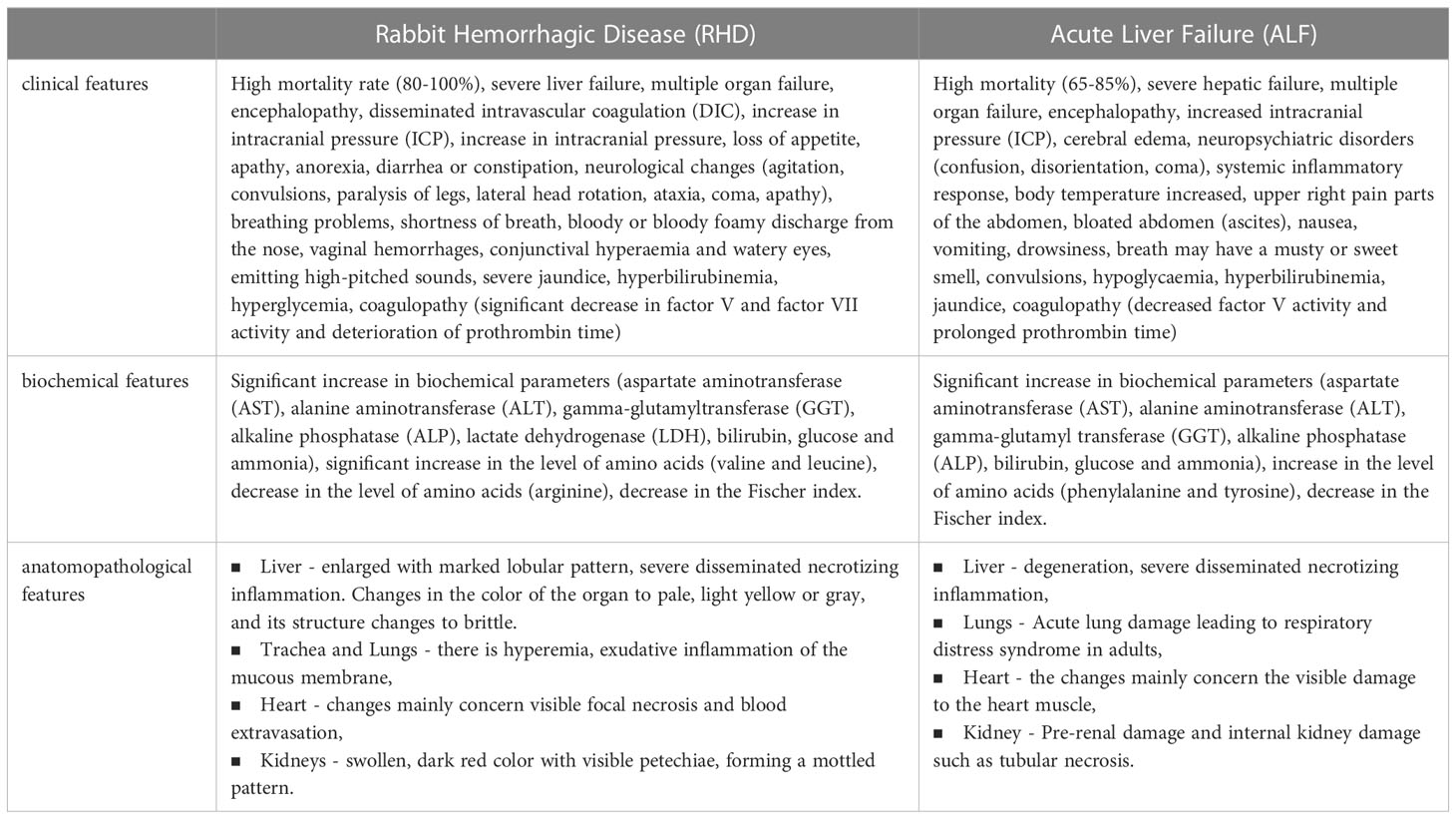
Table 1 Table showing the similarities between rabbit hemorrhagic disease (RHD) and acute liver failure (ALF) (2, 9, 10, 13, 53–55).
Available studies using L. europaeus infection as a model for ALF focus on the therapeutic approach. San-Miquel et al. (56) reported that treatment with N-acetylcysteine (NAC) showed hepatoprotective effects in hepatic failure mediated by modulation of the intrinsic apoptosis pathway. Promising results were also obtained by Tunon et al. (57) while analyzing the effect of melatonin on hepatocyte apoptosis.
It was shown that the anti-apoptotic effect of melatonin was dose-dependent and contributed to reduced expression of the pro-apoptotic Bax protein and cytosolic release of cytochrome c, as well as increased expression of the anti-apoptotic proteins Bcl-2 and Bcl-xL and inhibition of the activity of caspase-9, which is a key protein initiating apoptosis. In another study by Crespo et al. (58) showed that melatonin prevented liver damage by inducing significant inhibition of alanine aminotransferase (ALT), aspartate aminotransferase (AST) and lactate dehydrogenase (LDH) and partially abolishing hypoglycemia. In addition, melatonin inhibited oxidative stress and increased the activity of antioxidant defense mechanisms. The therapeutic potential of melatonin was also confirmed by San-Miquel et al. (59). Melatonin was also shown to induce a reduction in autophagy associated with L. europaeus infection and inhibited viral replication. In another study by Crespo et al. (60) reported that melatonin, by modulating molecular clock signaling, also inhibited the mitophagy pathway, prevented the activation of the NLRP3 inflammasome, and influenced the innate immune response associated with Toll-like receptor 4 (TLR4) and TLR3, retinoic acid-inducible gene (RIG-1), mitochondrial antiviral signaling (MAVS), natural killer 2D groups (NKG2D) and interferon α (IFN-α), stimulator of interferon (STING), GZMA and perforin (PER1) genes, induced by L. europaeus infection.
4 Characteristics of defensins
Defensins are a family of cationic peptides of size (2-5kDa), which together with cathelicidins are included in the category of antimicrobial peptides (AMPs). Defensins are produced by the innate immune system and have a broad spectrum of antibacterial, antiviral and antifungal activity (6). They are characterized by a mainly β-sheet structure, which is stabilized by three disulfide bonds. Their diverse structure and organization of disulfide bonds make it possible to divide them into three groups: α-, β- and θ-defensins. Importantly, not all defensins are expressed in every mammal. For example, α-defensins are not expressed in mouse neutrophils, while θ-defensins are only expressed in primates, while β-defensins are widely expressed in mammals (61). Defensins are found in tissues involved in the host’s immune response and are present in leukocyte granules (6). They perform a wide range of regulatory functions in both innate and acquired immunity. In addition to inducing the production of cytokines and chemokines, they also exhibit chemotactic activity against T cells, monocytes and immature dendritic cells (62). Defensins act in innate immunity by depolarizing the cell membrane, opsonizing immune cells, stimulating cytokines and limiting replication by inhibiting nuclear enzymes (62).
The mechanisms of antiviral action of defensins also focus on direct defensin-virus interaction. They interact with the lipid bilayers of enveloped viruses, and some are also capable of binding to glycoproteins and glycolipids. They can also engage in protein-protein or protein-DNA interactions, and defensin oligomerization and conformational stability can also affect binding. Moreover, the antiviral activity of these peptides is relatively dependent on the type of defensin and virus that we are considering in a given case (63). Defensins also have an inhibitory effect on non-enveloped viruses. Studies have shown (64) that the formation of multimeric forms by defensins can affect virion aggregation. This is because, in addition to the strong binding due to oligomerization, neutralizing the charge of the viral capsid can affect inter-virion repulsion. Such aggregation of viral particles may reduce the level of infectivity by impeding cell binding or cause viral aggregates to enter fewer host cells (64). Other mechanisms of antiviral action of defensins also include inhibition of viral shedding and blocking viral entry to the nucleus of the genome. Some defensins have been shown to inhibit protein kinase C (PKC) signaling, a pathway used by many viruses for fusion, transcription, integration and assembly (65). Moreover, defensins can also activate intracellular antiviral mechanisms related to the CCR6 chemokine receptor and inducing the expression of apolipoprotein B mRNA-editing enzyme, catalytic polypeptide-like 3G (APOBEC3G) (63). A summary of the antiviral effects of defensins is shown in Figure 1.
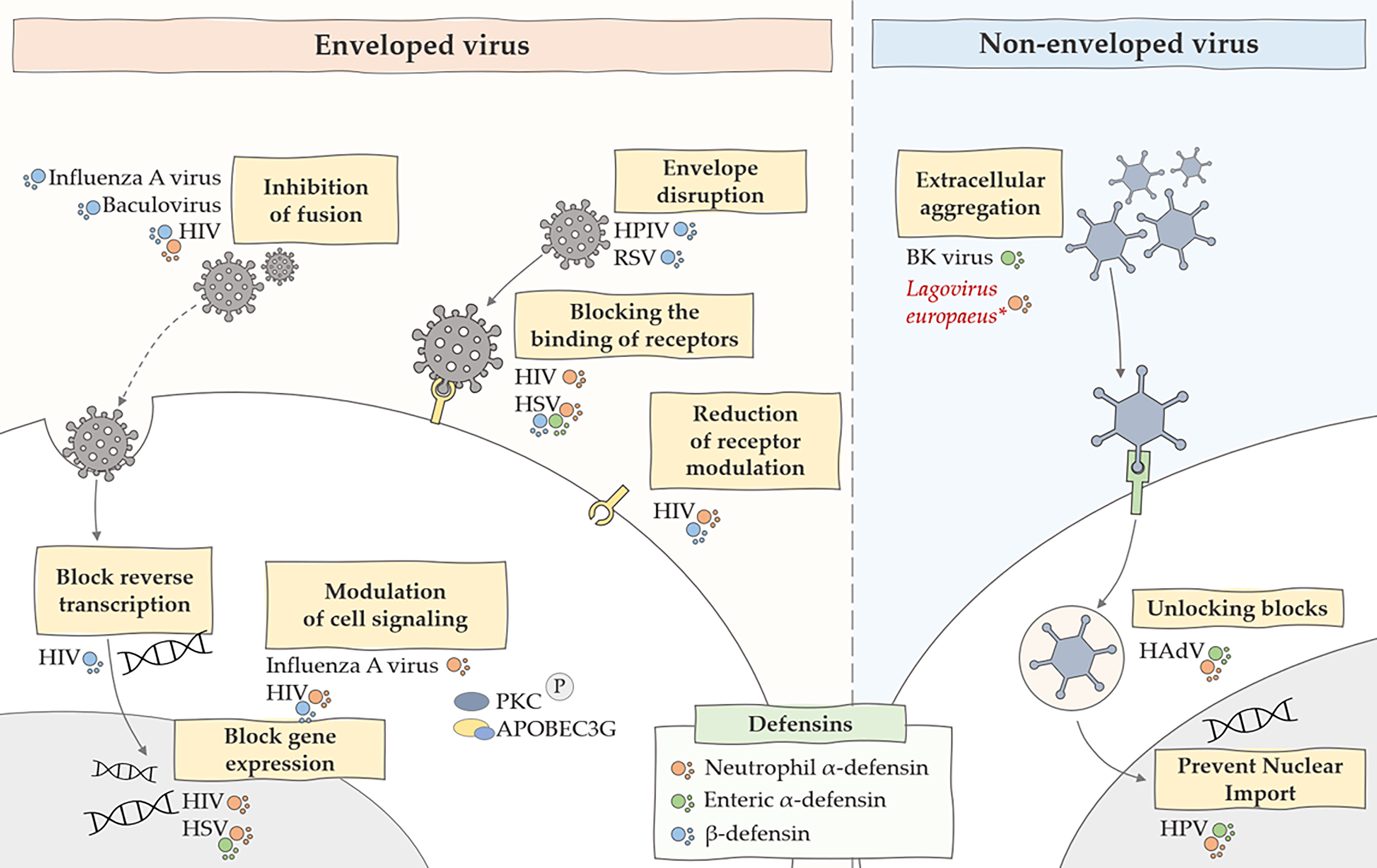
Figure 1 Summary of the main antiviral mechanisms of defensins. The figure shows the inhibitory effect of defensins on many stages of viral infections caused by enveloped and non-enveloped viruses. HIV, human immunodeficiency virus; HSV, herpes simplex virus; HPV, human papillomavirus, HPIV, human parainfluenza viruses, RSV, respiratory syncytial virus; *, predicted effect of rabbit α-defensin on inhibition of Lagovirus europaeus infection.
5 Defensins in rabbits
The rabbit is a special organism in the context of defensins. The first mammalian defensin, then called a bactericidal cationic protein, was isolated for the first time from rabbit lung macrophages in 1980 by Lehler et al. (66, 67) in 1985, the same research team isolated peptides from human neutrophils that were homologous to those of rabbits, and then referred to these peptides as “defensins” (68, 69). Currently, six defensins have been described in rabbits (70, 71): NP-1 (otherwise corticostatin-3, antiadrenocorticotropin peptide III, macrophage antibiotic peptide MCP-1), NP-2 (otherwise corticostatin-4, defensin alpha 4, antiadrenocorticotropin peptide IV, macrophage antibiotic peptide MCP-2), NP-3A (otherwise corticostatin-1, antiadrenocorticotropin peptide I, corticostatin-1, microbicidal peptide NP-3A, neutrophil antibiotic peptide NP-3A), NP-3B (otherwise corticostatin-2, antiadrenocorticotropin peptide II, microbicidal peptide NP-3B, neutrophil antibiotic peptide NP-3B), NP-4 (otherwise neutrophil antibiotic peptide NP-4, microbicidal peptide NP-4) and NP-5 (otherwise neutrophil antibiotic peptide NP-5, microbicidal peptide NP-5, corticostatin-6). Defensins are reported to account for at least 15% of the protein content of rabbit neutrophils (72). White et al. (73) demonstrated that rabbit defensins have high sequence and structural similarity to human defensins. Selested et al. (69) determined that rabbit defensins contain 32-34 amino acid residues and are rich in cysteine and arginine, each containing three intramolecular disulfide bonds. The occurrence of defensins in the rabbit body is not fully understood. So far, the presence of defensins (Figure 2) in the following sites has been confirmed: NP-1 and NP-2 (peritoneal, lungs, tears), NP-3A (peritoneum), NP-3B (tears), NP-4 (liver, peritoneal) and NP-5 (bone marrow, spleen, thymus, peritoneum, liver, tears) (69, 74–78).
In vitro studies have shown that defensin NP-1 has a broad spectrum of antimicrobial activity against bacteria (79, 80), fungi (81), and viruses (82, 83). Hristova et al. (70) studied the interaction of rabbit defensins derived from neutrophils with large unilamellar vesicles (LUVs) made of a mixture of lipids mimicking the lipid composition of Escherichia coli membranes. As a result, it was shown that the defensins NP-1, NP-2, NP-3A, NP-3B and the natural mixture of NP-1-5 induced membrane permeability most likely through transient damage, while NP-4 and NP-5 did not affect membrane permeability. membranes, but had a synergistic effect when used with other defensins. The antiviral functions of defensins were for example analyzed by Sinha et al. (83) in their studies. It was recorded that NP-1 defensin inactivated HSV, prevented virus entry and viral cell-to-cell spread. In turn, Zhou et al. (77) also demonstrated the up-regulation of tear defensin NP-1 and NP-2 during re-epithelialization of an experimental corneal wound. In the study, defensin levels were correlated with the course of wound healing, suggesting an important role for these peptides in protecting the cornea from microbes, as well as a possible involvement in modulating the healing process. Literature data suggest that NP-4 and NP-5 defensins express the weakest antimicrobial properties. However, the effects of NP-5 defensin increase when pathogens are metabolically active (84). Moreover, the antifungal effect of NP-5 defensin was not demonstrated, but its addition in submicromolar concentrations to the solution enhanced the activity of NP-1, NP-2 and NP-3A defensin (85). A summary of the antimicrobial properties of rabbit defensins is provided in Table 2.
6 Defensins – potential therapeutic agent for ALF
Despite significant progress in the development of the pharmaceutical industry and the development of new synthetic and semi-synthetic drugs, drug resistance remains an important problem in the treatment of infectious diseases and is a serious challenge today. For this reason, the search for natural substances with potential therapeutic use is currently a desirable direction of research. Defensins are antimicrobial peptides that are characterized by a wide spectrum of activity, which is why their therapeutic use can bring promising results. Currently, literature data on the role and therapeutic potential of defensins in liver diseases are scarce. Recently, Warner et al. (96) conducted a study on the effects of human β-defensin (HBD-2) on Alcohol-Associated Liver Disease (ALD) in mice. As a result, systematic administration of HBD-2 was shown to attenuate liver damage. In addition, HBD-2 showed immunomodulatory effects by up-regulating cytokines and affecting the abundance of regulatory T cells to create a less pro-inflammatory environment in the liver.
In turn, Zhong et al. (97) reported that knock-out of α-defensins resulted in greater liver damage in a mouse model of alcoholic steatohepatitis, while administration of synthetic human α-defensins 5 showed a therapeutic effect in this study. Mani et al. (98) showed that the level of human β-defensin 1 was significantly elevated in patients with acute or chronic hepatic failure, indicating a strong predictive value as a marker of mortality. There are also reports indicating increased expression of α-defensin 4 in infections with hepatitis viruses, which, as described earlier, are associated with ALF. Zhou et al. (99) showed that the expression of the gene encoding α-defensin 4 (DEFA4) is significantly elevated in patients with acute chronic liver failure (ACLF) associated with HBV. Thus, the involvement of this defensin in the host antiviral response to HBV infection has been suggested. In addition, the authors indicated that the analysis of the expression of this gene may serve as a potential prognostic marker for the detection of ACLF-HBV. Moreover, in another study, β-defensin 3 was upregulated by HBV and prevented intrauterine transmission of HBV to infants (100). Another study showed (101) that DEFA4 expression was elevated in the peripheral blood of HCV-infected liver transplant patients. DEFA4 gene expression analysis was also analyzed in HEV infection (102). As a result, an increased level of expression of α-defensins was recorded in HEV patients, regardless of pregnancy and the clinical picture of the disease, suggesting a protective role of these peptides. The protective role of defensins in HCV infection was also confirmed by Ling et al. (7) in a study on the expression of β-defensin 1 in patients with HCV infection and liver cancer. As a result, it was reported that expression of β-defensin 1 was reduced in HCV-infected liver, and treatment with interferon and ribavirin resulted in increased expression of this defensin. Moreover, the increased upregulation of this expression was associated with a better response to treatment, suggesting a protective role of β-defensin 1 in HCV progression and its predictive value for assessing the effectiveness of interferon and ribavirin treatment (7). The antiviral role of human α and β-defensins and synthetic avian α-defensins was also confirmed by Mattar et al. (103) in an in vitro study in tissue culture. The results showed that the defensins used showed strong anti-HCV activity, suggesting that they are potent antiviral agents. The antiviral potential of the defensin BmKDfsin4 derived from the scorpion Mesobuthus martensii Karsch was analyzed in a study by Zeng et al. (104). As a result, BmKDfsin4 was shown to significantly inhibit HBV replication in vitro by reducing the production of HBsAg viral core protein. The available data suggest that defensins should be considered as potential therapeutic agents in the treatment of viral infections, and thus of viral ALF.
However, despite the many advantages of using defensins as therapeutic agents, there are also some limitations. These challenges are related primarily to the rapid turnover in the human body, high cost of production or non-specific hemolytic activity on human cells and their immunogenicity. So, more research is required to address these issues (6). Some authors suggest that the use of drugs based on antimicrobial peptides as adjuvants or in combination with other antiviral drugs with different mechanisms of action may prove helpful, which in turn may help to reduce the side effects of treatment and reduce the problem of drug resistance (105).
7 Conclusions
Despite the implementation of preventive vaccination in many regions of the world, the viral background of ALF remains a big problem and leads to many deaths (65-85%), therefore the search for effective antiviral drugs seems to be a desirable direction of research. Defensins, being natural antimicrobial peptides, have considerable potential for use as therapeutic agents in infectious liver diseases. Previous studies on the role of human α and β defensins in liver diseases caused by viral infection confirmed the significant role of defensins in the course of the disease. In patients infected with HCV and HBV, a significantly increased expression of defensins is observed, which translates into greater effectiveness of treatment of patients and confirms that defensins are powerful antiviral agents with high therapeutic and prognostic potential in liver diseases. In the case of ALF, conducting clinical trials is quite problematic due to the low incidence and severe course of the disease, due to this fact, studies on the role and therapeutic potential of defensins in liver diseases are scarce, therefore the use of animal models is important for the development of new therapeutic strategies. While searching for an appropriate animal model for ALF, it was observed that the course of rabbit hemorrhagic disease in rabbits is very similar to the course of Acute liver failure. Therefore, experimental infection with L. europaeus virus in rabbits is a good and effective model of ALF. For this reason, the role of defensins in infections should be analyzed, and the obtained results may be an important contribution to the development of new therapeutic strategies in infectious liver diseases. So far, there have been no studies on the therapeutic use of defensins in the course of rabbit hemorrhagic disease.
Author contributions
All authors listed have made a substantial, direct, and intellectual contribution to the work and approved it for publication.
Conflict of interest
The authors declare that the research was conducted in the absence of any commercial or financial relationships that could be construed as a potential conflict of interest.
Publisher’s note
All claims expressed in this article are solely those of the authors and do not necessarily represent those of their affiliated organizations, or those of the publisher, the editors and the reviewers. Any product that may be evaluated in this article, or claim that may be made by its manufacturer, is not guaranteed or endorsed by the publisher.
References
1. Akamatsu N, Sugawara Y, Kokudo N. Acute liver failure and liver transplantation. Intractable Rare Dis Res (2013) 2:77–87. doi: 10.5582/IRDR.2013.V2.3.77
2. Stravitz RT, Lee WM. Acute liver failure. Lancet (2019) 394:869–81. doi: 10.1016/S0140-6736(19)31894-X
3. Donnelly MC, Davidson JS, Martin K, Baird A, Hayes PC, Simpson KJ. Acute liver failure in Scotland: changes in aetiology and outcomes over time (the Scottish look-back study). Aliment Pharmacol Ther (2017) 45:833–43. doi: 10.1111/APT.13943
4. Lee WM, Squires RH, Nyberg SL, Doo E, Hoofnagle JH. Acute liver failure: summary of a workshop. Hepatology (2008) 47:1401. doi: 10.1002/HEP.22177
5. Patterson J, Hussey HS, Abdullahi LH, Silal S, Goddard L, Setshedi M, et al. The global epidemiology of viral-induced acute liver failure: a systematic review protocol. BMJ Open (2019) 9:e029819. doi: 10.1136/BMJOPEN-2019-029819
6. Solanki SS, Singh P, Kashyap P, Sansi MS, Ali SA. Promising role of defensins peptides as therapeutics to combat against viral infection. Microb Pathog (2021) 155:104930. doi: 10.1016/J.MICPATH.2021.104930
7. Ling YM, Chen JY, Guo L, Wang CY, Tan WT, Wen Q, et al. β-defensin 1 expression in HCV infected liver/liver cancer: an important role in protecting HCV progression and liver cancer development. Sci Rep (2017) 7:1–14. doi: 10.1038/s41598-017-13332-0
8. Hefler J, Marfil-Garza BA, Pawlick RL, Freed DH, Karvellas CJ, Bigam DL, et al. Preclinical models of acute liver failure: a comprehensive review. PeerJ (2021) 9:e12579. doi: 10.7717/PEERJ.12579
9. Tuñón MJ, Alvarez M, Culebras JM, González-Gallego J. An overview of animal models for investigating the pathogenesis and therapeutic strategies in acute hepatic failure. World J Gastroenterol (2009) 15:3086–98. doi: 10.3748/WJG.15.3086
10. Bernal W, Wendon J. Acute liver failure. N Engl J Med (2013) 369:2525–34. doi: 10.1056/NEJMRA1208937
11. Fredricks DN. Viruses associated with unexplained acute liver failure: next generation reveals the last generation. Clin Infect Dis (2017) 65:1486–8. doi: 10.1093/CID/CIX597
12. Axley P, Ahmed Z, Arora S, Haas A, Kuo YF, Kamath PS, et al. NASH is the most rapidly growing etiology for acute-on-Chronic liver failure-related hospitalization and disease burden in the united states: a population-based study. Liver Transplant (2019) 25:695–705. doi: 10.1002/LT.25443
14. Baumert TF, Yang C, Schürmann P, Köck J, Ziegler C, Grüllich C, et al. Hepatitis b virus mutations associated with fulminant hepatitis induce apoptosis in primary tupaia hepatocytes. Hepatology (2005) 41:247–56. doi: 10.1002/HEP.20553
15. Manka P, Verheyen J, Gerken G, Canbay A. Liver failure due to acute viral hepatitis (A-e). Visc Med (2016) 32:80–5. doi: 10.1159/000444915
16. Thimme R, Wieland S, Steiger C, Ghrayeb J, Reimann KA, Purcell RH, et al. CD8 + T cells mediate viral clearance and disease pathogenesis during acute hepatitis b virus infection. J Virol (2003) 77:68–76. doi: 10.1128/JVI.77.1.68-76.2003/ASSET/DA13A643-A96C-4E42-AB41-A5A2AC80F92C/ASSETS/GRAPHIC/JV0131592004.JPEG
17. Farci P, Diaz G, Chen Z, Govindarajan S, Tice A, Agulto L, et al. B cell gene signature with massive intrahepatic production of antibodies to hepatitis b core antigen in hepatitis b virus-associated acute liver failure. Proc Natl Acad Sci U.S.A. (2010) 107:8766–71. doi: 10.1073/PNAS.1003854107/SUPPL_FILE/PNAS.201003854SI.PDF
18. Gong J, Chen Y, Cao J, Wang Y, Chen J, Li D, et al. The immune landscape of hepatitis b virus-related acute liver failure by integration analysis. J Immunol Res (2022) 2022:6764379. doi: 10.1155/2022/6764379
19. Muhammad H, Tehreem A, Hammami MB, Ting P-S, Idilman R, Gurakar A. Hepatitis d virus and liver transplantation: indications and outcomes. World J Hepatol (2021) 13:291–9. doi: 10.4254/wjh.v13.i3.291
20. Shukla NB, Poles MA. Hepatitis b virus infection: co-infection with hepatitis c virus, hepatitis d virus, and human immunodeficiency virus. Clin Liver Dis (2004) 8:445–60. doi: 10.1016/J.CLD.2004.02.005
21. Keles E, Hassan-Kadle MA, Osman MM, Eker HH, Abusoglu Z, Baydili KN, et al. Clinical characteristics of acute liver failure associated with hepatitis a infection in children in Mogadishu, Somalia: a hospital-based retrospective study. BMC Infect Dis (2021) 21:1–7. doi: 10.1186/S12879-021-06594-7/TABLES/1
22. Taylor RM, Davern T, Munoz S, Han SH, McGuire B, Larson AM, et al. Fulminant hepatitis a virus infection in the united states: incidence, prognosis, and outcomes. Hepatology (2006) 44:1589–97. doi: 10.1002/HEP.21439
23. Fujiwara K, Yokosuka O, Ehata T, Saisho H, Saotome N, Suzuki K, et al. Association between severity of type a hepatitis and nucleotide variations in the 5` non-translated region of hepatitis a virus RNA: strains from fulminant hepatitis have fewer nucleotide substitutions. Gut (2002) 51:82. doi: 10.1136/GUT.51.1.82
24. Muraoka H. [Clinical and epidemiological study on factors of serious development of viral hepatitis type a] - PubMed. Nihon Shokakibyo Gakkai Zasshi (1990) 87:1383–91.
25. Vento S, Garofano T, Renzini C, Cainelli F, Casali F, Ghironzi G, et al. Fulminant hepatitis associated with hepatitis a virus superinfection in patients with chronic hepatitis c. N Engl J Med (1998) 338:286–90. doi: 10.1056/NEJM199801293380503
26. Walker CM. Adaptive immune responses in hepatitis a virus and hepatitis e virus infections. Cold Spring Harb Perspect Med (2019) 9:a033472. doi: 10.1101/CSHPERSPECT.A033472
27. Hirai-Yuki A, Hensley L, McGivern DR, González-López O, Das A, Feng H, et al. MAVS-dependent host species range and pathogenicity of human hepatitis a virus. Sci (1979) (2016) 353:1541–5. doi: 10.1126/SCIENCE.AAF8325/SUPPL_FILE/HIRAI-YUKI.SM.PDF
28. Hirai-Yuki A, Whitmire JK, Joyce M, Tyrrell DL, Lemon SM. Murine models of hepatitis a virus infection. Cold Spring Harb Perspect Med (2019) 9:a031674. doi: 10.1101/CSHPERSPECT.A031674
29. Shalimar, Kedia S, Gunjan D, Sonika U, Mahapatra SJ, Nayak B, et al. Acute liver failure due to hepatitis e virus infection is associated with better survival than other etiologies in Indian patients. Dig Dis Sci (2017) 62:1058–66. doi: 10.1007/S10620-017-4461-X/TABLES/4
30. Manka P, Bechmann LP, Coombes JD, Thodou V, Schlattjan M, Kahraman A, et al. Hepatitis e virus infection as a possible cause of acute liver failure in Europe. Clin Gastroenterol Hepatol (2015) 13:1836–1842.e2. doi: 10.1016/J.CGH.2015.04.014
31. Sehgal R, Patra S, David P, Vyas A, Khanam A, Hissar S, et al. Impaired monocyte-macrophage functions and defective toll-like receptor signaling in hepatitis e virus-infected pregnant women with acute liver failure. Hepatology (2015) 62:1683–96. doi: 10.1002/HEP.28143/SUPPINFO
32. Crossan CL, Simpson KJ, Craig DG, Bellamy C, Davidson J, Dalton HR, et al. Hepatitis e virus in patients with acute severe liver injury. World J Hepatol (2014) 6:426–34. doi: 10.4254/wjh.v6.i6.426
33. Li Y, Qu C, Yu P, Ou X, Pan Q, Wang W. The interplay between host innate immunity and hepatitis e virus. Viruses 2019 Vol 11 Page 541 (2019) 11:541. doi: 10.3390/V11060541
34. Srivastava R, Aggarwal R, Sachdeva S, Alam MI, Jameel S, Naik S. Adaptive immune responses during acute uncomplicated and fulminant hepatitis e. J Gastroenterol Hepatol (2011) 26:306–11. doi: 10.1111/J.1440-1746.2010.06356.X
35. Wu J, Ling B, Guo N, Zhai G, Li M, Guo Y. Immunological manifestations of hepatitis e-associated acute and chronic liver failure and its regulatory mechanisms. Front Med (Lausanne) (2021) 8:725993/BIBTEX. doi: 10.3389/FMED.2021.725993/BIBTEX
36. Rao A, Rule JA, Cerro-Chiang G, Stravitz RT, McGuire BM, Lee G, et al. Role of hepatitis c infection in acute liver Injury/Acute liver failure in north America. Dig Dis Sci (2022) 68(1):304–11. doi: 10.1007/S10620-022-07524-6
37. Kwon YC, Ray RB, Ray R. Hepatitis c virus infection: establishment of chronicity and liver disease progression. EXCLI J (2014) 13:977.
38. Srivastava P, Sharma R, Kharwas P. Acute liver failure in a child due to Epstein Barr virus and response to antivirals. Pediatr Oncall (2020) 17:124–5. doi: 10.7199/PED.ONCALL.2020.41
39. Mellinger JL, Rossaro L, Naugler WE, Nadig SN, Appelman H, Lee WM, et al. Epstein-Barr Virus (EBV) related acute liver failure: a case series from the US acute liver failure study group. Dig Dis Sci (2014) 59:1630–7. doi: 10.1007/S10620-014-3029-2/TABLES/3
40. Narkewicz MR, Olio DD, Karpen SJ, Murray KF, Schwarz K, Yazigi N, et al. Pattern of diagnostic evaluation for the causes of pediatric acute liver failure: an opportunity for quality improvement. J Pediatr (2009) 155:801–806.e1. doi: 10.1016/J.JPEDS.2009.06.005
41. Hara S, Hoshino Y, Naitou T, Nagano K, Iwai M, Suzuki K, et al. Association of virus infected-T cell in severe hepatitis caused by primary Epstein-Barr virus infection. J Clin Virol (2006) 35:250–6. doi: 10.1016/J.JCV.2005.07.009
42. Nakajima K, Hiejima E, Nihira H, Kato K, Honda Y, Izawa K, et al. Case report: a case of Epstein-Barr virus-associated acute liver failure requiring hematopoietic cell transplantation after emergent liver transplantation. Front Immunol (2022) 13:825806. doi: 10.3389/FIMMU.2022.825806
43. Nakazawa A, Nakano N, Fukuda A, Sakamoto S, Imadome KI, Kudo T, et al. Use of serial assessment of disease severity and liver biopsy for indication for liver transplantation in pediatric Epstein-Barr virus–induced fulminant hepatic failure. Liver Transplant (2015) 21:362–8. doi: 10.1002/LT.24052
44. Park HW, Lee SG, Hwang SK, Kim KH, Park YH. Epstein–Barr Virus associated fulminant hepatitis requiring liver transplantation (case report). HPB Off J Int Hepato Pancreato Biliary Assoc (2013) 13:174–244.
45. Ahmed A, Granillo A, Burns E, Glassner K, Naseem N, Force C, et al. Herpes simplex virus-2 hepatitis: a case report and review of the literature. Case Rep Med (2020) 2020:8613840. doi: 10.1155/2020/8613840
46. Then EO, Gayam V, Are VS, Sunkara T, Gaduputi V. Herpes simplex virus hepatitis: a brief review of an oft-overlooked pathology. Cureus (2019) 11(3):e4313. doi: 10.7759/CUREUS.4313
47. Chávez SM, Poniachik JM, Urzua ÁM, Roblero JP, Cattaneo MJ, Jimenez AP, et al. Acute liver failure due to herpes simplex virus: diagnostic clues and potential role of plasmapheresis: a case report. Medicine (2021) 100:e27139. doi: 10.1097/MD.0000000000027139
48. Szewc AM, Taylor S, Cage GD, Jacobsen J, Bulut OP, De Mello DE. Acute liver failure in an adolescent Male induced by human herpesvirus 6 (HHV-6): a case report with literature review. Lab Med (2018) 49:165–74. doi: 10.1093/LABMED/LMX088
49. Chevret L, Boutolleau D, Halimi-Idri N, Branchereau S, Baujard C, Fabre M, et al. Human herpesvirus-6 infection: a prospective study evaluating HHV-6 DNA levels in liver from children with acute liver failure. J Med Virol (2008) 80:1051–7. doi: 10.1002/JMV.21143
50. Amado Leon LA, Rocha Alves AD, Cubel Garcia R de CN, Melgaço JG, de Paula VS, Pinto MA. Parvovirus B19 infection in a fatal case of acute liver failure. Pediatr Infect Dis J (2017) 36:E355–8. doi: 10.1097/INF.0000000000001731
51. Hu J, Zhao H, Lou D, Gao H, Yang M, Zhang X, et al. Human cytomegalovirus and Epstein-Barr virus infections, risk factors, and their influence on the liver function of patients with acute-on-chronic liver failure. BMC Infect Dis (2018) 18:1–8. doi: 10.1186/S12879-018-3488-8/TABLES/5
52. Jhamb R, Kashyap B, Ranga GS, Kumar A. Dengue fever presenting as acute liver failure–a case report. Asian Pac J Trop Med (2011) 4:323–4. doi: 10.1016/S1995-7645(11)60095-3
53. Terblanche J, Hickman R. Animal models of fulminant hepatic failure. Dig Dis Sci (1991) 36:770–4. doi: 10.1007/BF01311235
54. Abrantes J, van der Loo W, Le Pendu J, Esteves PJ. Rabbit haemorrhagic disease (RHD) and rabbit haemorrhagic disease virus (RHDV): a review. Vet Res (2012) 43:1–19. doi: 10.1186/1297-9716-43-12/FIGURES/4
55. Bębnowska D, Niedźwiedzka-Rystwej P. Characteristics of a new variant of rabbit haemorrhagic disease virus – RHDV2. Acta Biologica (2019) 26:83–97. doi: 10.18276/AB.2019.26-08
56. San-Miguel B, Alvarez M, Culebras JM, González-Gallego J, Tuñón MJ. N-acetyl-cysteine protects liver from apoptotic death in an animal model of fulminant hepatic failure. Apoptosis (2006) 11:1945–57. doi: 10.1007/S10495-006-0090-0/METRICS
57. Tuñõn MJ, Miguel BS, Crespo I, Jorquera F, Santamaría E, Alvarez M, et al. Melatonin attenuates apoptotic liver damage in fulminant hepatic failure induced by the rabbit hemorrhagic disease virus. J Pineal Res (2011) 50:38–45. doi: 10.1111/J.1600-079X.2010.00807.X
58. Crespo I, Miguel BS, Laliena A, Álvarez M, Culebras JM, González-Gallego J, et al. Melatonin prevents the decreased activity of antioxidant enzymes and activates nuclear erythroid 2-related factor 2 signaling in an animal model of fulminant hepatic failure of viral origin. J Pineal Res (2010) 49:193–200. doi: 10.1111/J.1600-079X.2010.00787.X
59. San-Miguel B, Crespo I, Vallejo D, Álvarez M, Prieto J, González-Gallego J, et al. Melatonin modulates the autophagic response in acute liver failure induced by the rabbit hemorrhagic disease virus. J Pineal Res (2014) 56:313–21. doi: 10.1111/JPI.12124
60. Crespo I, Fernández-Palanca P, San-Miguel B, Álvarez M, González-Gallego J, Tuñón MJ. Melatonin modulates mitophagy, innate immunity and circadian clocks in a model of viral-induced fulminant hepatic failure. J Cell Mol Med (2020) 24:7625–36. doi: 10.1111/JCMM.15398
61. Wilson SS, Wiens ME, Holly MK, Smith JG. Defensins at the mucosal surface: latest insights into defensin-virus interactions. J Virol (2016) 90:5216–8. doi: 10.1128/JVI.00904-15/ASSET/6A103AC2-112A-46EB-84C9-EDF14453DF45/ASSETS/GRAPHIC/ZJV9991816800001.JPEG
62. Ding J, Chou YY, Chang TL. Defensins in viral infections. J Innate Immun (2009) 1:413–20. doi: 10.1159/000226256
63. Wilson SS, Wiens ME, Smith JG. Antiviral mechanisms of human defensins. J Mol Biol (2013) 425:4965–80. doi: 10.1016/J.JMB.2013.09.038
64. Dugan AS, Maginnis MS, Jordan JA, Gasparovic ML, Manley K, Page R, et al. Human alpha-defensins inhibit BK virus infection by aggregating virions and blocking binding to host cells. J Biol Chem (2008) 283:31125–32. doi: 10.1074/JBC.M805902200
65. Chang TL, Vargas J, DelPortillo A, Klotman ME. Dual role of alpha-defensin-1 in anti-HIV-1 innate immunity. J Clin Invest (2005) 115:765–73. doi: 10.1172/JCI21948
66. Patterson-Delafield J, Martinez RJ, Lehrer RI. Microbicidal cationic proteins in rabbit alveolar macrophages: a potential host defense mechanism. Infect Immun (1980) 30:180–92. doi: 10.1128/IAI.30.1.180-192.1980
67. Patterson-Delafield J, Szklarek D, Martinez RJ, Lehrer RI. Microbicidal cationic proteins of rabbit alveolar macrophages: amino acid composition and functional attributes. Infect Immun (1981) 31:723–31. doi: 10.1128/iai.31.2.723-731.1981
68. Ganz T, Selsted ME, Szklarek D, Harwig SS, Daher K, Bainton DF, et al. Defensins. natural peptide antibiotics of human neutrophils. J Clin Invest (1985) 76:1427–35. doi: 10.1172/JCI112120
69. Selsted ME, Harwig SSL, Ganz T, Schilling JW, Lehrer RI. Primary structures of three human neutrophil defensins. J Clin Invest (1985) 76:1436. doi: 10.1172/JCI112121
70. Hristova K, Selsted ME, White SH. Critical role of lipid composition in membrane permeabilization by rabbit neutrophil defensins. J Biol Chem (1997) 272:24224–33. doi: 10.1074/JBC.272.39.24224
71. Linzmeier R, Michaelson D, Liu L, Ganz T. The structure of neutrophil defensin genes. FEBS Lett (1993) 326:299. doi: 10.1016/0014-5793(93)81813-F
72. Michaelson D, Rayner J, Couto M, Ganz T. Cationic defensins arise from charge-neutralized propeptides: a mechanism for avoiding leukocyte autocytotoxicity? J Leukoc Biol (1992) 51:634–9. doi: 10.1002/JLB.51.6.634
73. White SH, Wimley WC, Selsted ME. Structure, function, and membrane integration of defensins. Curr Opin Struct Biol (1995) 5:521–7. doi: 10.1016/0959-440X(95)80038-7
74. Selstedls ME, Browny DM, Delanged RJ, Lehrerl RI. Primary structures of MCP-1 and MCP-2, natural peptide antibiotics of rabbit lung macrophages. J Biol Chem (1983) 258:14485–9. doi: 10.1016/S0021-9258(17)43888-9
75. Wołącewicz M, Hrynkiewicz R, Niedźwiedzka-Rystwej P. Preliminary studies on defensins expression in liver of rabbits experimentally infected with lagovirus europaeus GI.1 and GI.1a. Nantes, France: World Rabbit Science Association 12th World Rabbit Congress (2021).
76. Hrynkiewicz R, Bębnowska D, Niedźwiedzka-Rystwej P. Initial investigation on NP-5 (corticostatin-6) defensin expression in liver of healthy and lagovirus europaeus infected rabbits. Białystok, Poland: XVII Congress of the Polish Society of Experimental and Clinical Immunology (2021).
77. Zhou L, Beuerman RW, Huang L, Barathi A, Yong HF, Li SFY, et al. Proteomic analysis of rabbit tear fluid: defensin levels after an experimental corneal wound are correlated to wound closure. Proteomics (2007) 7:3194–206. doi: 10.1002/PMIC.200700137
78. Sadro LC, Tremblay A, Solomon S, Palfree RGE. Differential expression of Corticostatins/Defensins: higher levels of CS-4 (NP-2) transcripts compared with CS-6 (NP-5) in rabbit lung. Biochem Biophys Res Commun (1993) 190:1009–16. doi: 10.1006/BBRC.1993.1149
79. Lehrer RI, Selsted ME, Szklarek D, Fleischmann J. Antibacterial activity of microbicidal cationic proteins 1 and 2, natural peptide antibiotics of rabbit lung macrophages. Infect Immun (1983) 42:10–4. doi: 10.1128/IAI.42.1.10-14.1983
80. Miyakawa Y, Ratnakar P, Rao AG, Costello ML, Mathieu-Costello O, Lehrer RI, et al. In vitro Activity of the antimicrobial peptides human and rabbit defensins and porcine leukocyte protegrin against mycobacterium tuberculosis. Infect Immun (1996) 64:926–32. doi: 10.1128/IAI.64.3.926-932.1996
81. Lehrer RI, Szklarek D, Ganz T, Selsted ME. Correlation of binding of rabbit granulocyte peptides to candida albicans with candidacidal activity. Infect Immun (1985) 49:207–11. doi: 10.1128/IAI.49.1.207-211.1985
82. Lehrer RI, Daher K, Ganz T, Selsted ME. Direct inactivation of viruses by MCP-1 and MCP-2, natural peptide antibiotics from rabbit leukocytes. J Virol (1985) 54:467–72. doi: 10.1128/JVI.54.2.467-472.1985
83. Sinha S, Cheshenko N, Lehrer RI, Herold BC. NP-1, a rabbit alpha-defensin, prevents the entry and intercellular spread of herpes simplex virus type 2. Antimicrob Agents Chemother (2003) 47:494–500. doi: 10.1128/AAC.47.2.494-500.2003
84. Hristova K, Selsted ME, White SH. Interactions of monomeric rabbit neutrophil defensins with bilayers: comparison with dimeric human defensin HNP-2†. Biochemistry (1996) 35:11888–94. doi: 10.1021/BI961100D
85. Zhang L, Hancock REW. Peptide antibiotics. In: Hughes D, Andersson DI, editors. Antibiotic development and resistance. CRC Press (2001). p. 209–32. doi: 10.1201/B12547-16
86. Lehrer RI, Lichtenstein AK, Ganz T. Defensins: antimicrobial and cytotoxic peptides of mammalian cells. Annu Rev Immunol (1993) 11:105–28. doi: 10.1146/ANNUREV.IY.11.040193.000541
87. Selsted ME, Szklarek D, Ganz T, Lehrer RI. Activity of rabbit leukocyte peptides against candida albicans. Infect Immun (1985) 49:202–6. doi: 10.1128/IAI.49.1.202-206.1985
88. Yamashita T, Saito K. Purification, primary structure, and biological activity of guinea pig neutrophil cationic peptides. Infect Immun (1989) 57:2405–9. doi: 10.1128/IAI.57.8.2405-2409.1989
89. Eisenhauer PB, Harwig SSL, Szklarek D, Ganz T, Selsted ME, Lehrer RI. Purification and antimicrobial properties of three defensins from rat neutrophils. Infect Immun (1989) 57:2021. doi: 10.1128/IAI.57.7.2021-2027.1989
90. Selsted ME, Szklarek D, Lehrer RI. Purification and antibacterial activity of antimicrobial peptides of rabbit granulocytes. Infect Immun (1984) 45:150–4. doi: 10.1128/IAI.45.1.150-154.1984
91. Miyasaki KT, Bodeau AL, Selsted ME, Ganz T, Lehrer RI. Killing of oral, gram-negative, facultative bacteria by the rabbit defensin, NP-1. Oral Microbiol Immunol (1990) 5:315–9. doi: 10.1111/J.1399-302X.1990.TB00432.X
92. Fields PI, Groisman EA, Heffron F. A salmonella locus that controls resistance to microbicidal proteins from phagocytic cells. Sci (1979) (1989) 243:1059–62. doi: 10.1126/SCIENCE.2646710
93. Borenstein LA, Selsted ME, Lehrer RI, Miller JN. Antimicrobial activity of rabbit leukocyte defensins against treponema pallidum subsp. pallidum. Infect Immun (1991) 59:1359–67. doi: 10.1128/IAI.59.4.1359-1367.1991
94. Levitz SM, Selsted ME, Ganz T, Lehrer RI, Diamond RD, Levitz SM, et al. In vitro Killing of spores and hyphae of aspergillus fumigatus and rhizopus oryzae by rabbit neutrophil cationic peptides and bronchoalveolar macrophages. J Infect Dis (1986) 154:483–9. doi: 10.1093/INFDIS/154.3.483
95. Alcouloumre MS, Ghannoum MA, Ibrahim AS, Selsted ME, Edwards JE. Fungicidal properties of defensin NP-1 and activity against cryptococcus neoformans. vitro. Antimicrob Agents Chemother (1993) 37:2628–32. doi: 10.1128/AAC.37.12.2628
96. Warner JB, Larsen IS, Hardesty JE, Song YL, Warner DR, McClain CJ, et al. Human beta defensin 2 ameliorated alcohol-associated liver disease in mice. Front Physiol (2022) 12:812882/BIBTEX. doi: 10.3389/FPHYS.2021.812882/BIBTEX
97. Zhong W, Wei X, Hao L, Du LT, Yue R, Sun X, et al. Paneth cell dysfunction mediates alcohol-related steatohepatitis through promoting bacterial translocation in mice: role of zinc deficiency. Hepatology (2020) 71:1575–91. doi: 10.1002/HEP.30945
98. Mani I, Alexopoulou A, Vasilieva L, Hadziyannis E, Agiasotelli D, Bei M, et al. Human beta-defensin-1 is a highly predictive marker of mortality in patients with acute-on-chronic liver failure. Liver Int (2019) 39:299–306. doi: 10.1111/LIV.13977
99. Zhou Q, Ding W, Jiang L, Xin J, Wu T, Shi D, et al. Comparative transcriptome analysis of peripheral blood mononuclear cells in hepatitis b-related acute-on-chronic liver failure. Sci Rep 2016 6:1 (2016) 6:1–9. doi: 10.1038/srep20759
100. Bai X, Tian T, Wang P, Yang X, Wang Z, Dong M. Potential roles of placental human beta-defensin-3 and apolipoprotein b mRNA-editing enzyme catalytic polypeptide 3G in prevention of intrauterine transmission of hepatitis b virus. J Med Virol (2015) 87:375–9. doi: 10.1002/JMV.24072
101. Muffak-Granero K, Bueno P, Olmedo C, Comino AM, Hassan L, Garcia-Alcalde F, et al. Study of gene expression profile in liver transplant recipients with hepatitis c virus. Transplant Proc (2008) 40:2971–4. doi: 10.1016/J.TRANSPROCEED.2008.09.003
102. Ramdasi AY, Arankalle VA. The expression patterns of immune response genes in the peripheral blood mononuclear cells of pregnant women presenting with subclinical or clinical HEV infection are different and trimester-dependent: a whole transcriptome analysis. PloS One (2020) 15(2):e0228068. doi: 10.1371/JOURNAL.PONE.0228068
103. Mattar EH, Almehdar HA, Uversky VN, Redwan EM. Virucidal activity of human α- and β-defensins against hepatitis c virus genotype 4. Mol Biosyst (2016) 12:2785–97. doi: 10.1039/C6MB00283H
104. Zeng Z, Zhang Q, Hong W, Xie Y, Liu Y, Li W, et al. Scorpion defensin BmKDfsin4 inhibits hepatitis b virus replication in vitro. Toxins (2016) 8:124. doi: 10.3390/TOXINS8050124
Keywords: acute liver failure (ALF), defensin, animal model, Lagovirus europaeus, rabbit hemorrhagic disease, potential therapeutic agent, antimicrobial peptides (AMPs), rabbit defensins
Citation: Hrynkiewicz R and Niedźwiedzka-Rystwej P (2023) Etiology of viral induced acute liver failure and defensins as potential therapeutic agents in ALF treatment. Front. Immunol. 14:1153528. doi: 10.3389/fimmu.2023.1153528
Received: 29 January 2023; Accepted: 12 April 2023;
Published: 21 April 2023.
Edited by:
Simon John Clark, University of Tübingen, GermanyReviewed by:
Joanna Wessely-Szponder, University of Life Sciences of Lublin, PolandTomasz Maślanka, University of Warmia and Mazury, Poland
Copyright © 2023 Hrynkiewicz and Niedźwiedzka-Rystwej. This is an open-access article distributed under the terms of the Creative Commons Attribution License (CC BY). The use, distribution or reproduction in other forums is permitted, provided the original author(s) and the copyright owner(s) are credited and that the original publication in this journal is cited, in accordance with accepted academic practice. No use, distribution or reproduction is permitted which does not comply with these terms.
*Correspondence: Rafał Hrynkiewicz, cmFmYWwuaHJ5bmtpZXdpY3pAdXN6LmVkdS5wbA==; Paulina Niedźwiedzka-Rystwej, cGF1bGluYS5uaWVkendpZWR6a2EtcnlzdHdlakB1c3ouZWR1LnBs