- 1Department of Infectious Disease, Sichuan Provincial People’s Hospital, University of Electronic Science and Technology of China, Chengdu, China
- 2Chinese Academy of Sciences Sichuan Translational Medicine Research Hospital, Chengdu, China
- 3Clinical and Research Center for Infectious Diseases, Beijing Ditan Hospital, Beijing, China
Highly active antiretroviral therapy (ART) can effectively inhibit virus replication and restore immune function in most people living with human immunodeficiency virus (HIV). However, an important proportion of patients fail to achieve a satisfactory increase in CD4+ T cell counts. This state is called incomplete immune reconstitution or immunological nonresponse (INR). Patients with INR have an increased risk of clinical progression and higher rates of mortality. Despite widespread attention to INR, the precise mechanisms remain unclear. In this review, we will discuss the alterations in the quantity and quality of CD4+ T as well as multiple immunocytes, changes in soluble molecules and cytokines, and their relationship with INR, aimed to provide cellular and molecular insights into incomplete immune reconstitution.
1 Introduction
Highly active antiretroviral therapy (ART) significantly reduces human immunodeficiency virus (HIV) or acquired immune deficiency syndrome (AIDS) related morbidity and mortality (1). After ART initiation, the plasma viral load drops to an undetectable level and the immune function gradually recovers to an approximately normal level in most individuals (2). However, an important proportion of HIV/AIDS patients, about 8-42%, persistently maintain low CD4+ T cell counts despite continuous virological suppression after at least two years of ART (3). These patients are referred to as immunological non-responders (INRs) (4), and this state is called incomplete immune reconstitution, or immunological nonresponse (INR) (4, 5).
Currently, there is no consensus on the definition of INR. According to previous reports, CD4+ T cell counts and ART time were the best features to describe INR, and the most frequent criterion was CD4+ T cell count <350 cells/µL after ≥24 months of virological suppression (5, 6). Some researchers also defined INR with CD4+ T cell count less than 200, 250, 400, or 500 cells/µL (6). Persistent low CD4+ T cell levels in these patients lead to an increased incidence of AIDS and non-AIDS events, such as metabolic syndrome, cardiovascular disease, liver disease, neurocognitive impairment, and malignant tumors, which in turn increase the risk of mortality (7, 8).
The occurrence of INR in HIV/AIDS patients may be affected by multiple factors, mainly including decreased bone marrow hematopoiesis, insufficient thymus output, residual virus replication, co-infection during ART, intestinal flora translocation, abnormal immune activation, type of antiretroviral regimen, baseline CD4+ T cell levels, age, sex, and genetic characteristics (4, 9). However, the precise mechanisms underlying INR remain an extremely challenging issue. Several characteristics of immunocytes may provide insights into these mechanisms. Therefore, we conducted this review to outline the cellular and molecular alterations associated with INR from an immunological perspective.
2 Quantity and quality of T cells
2.1 Overview of T cells
T cells are the major components of the adaptive immune system. They are released from the thymus as mature naive T (TN, CD45RA+CCR7+CD27+CD28+) cells with a given epitope specificity after positive and negative selection (Figure 1) (10, 11). During pathogen infection or vaccination, TN cells get activated and differentiate into effector cells (TE), accompanied by the acquisition of effector function, altered tissue homing, and robust proliferation function to expand in number (12–14). Following antigen clearance, TE cells undergo a contraction phase and only a small portion develop into long-lived memory T cells (12). The gene expression, phenotypic and functional properties of memory T cell subsets suggest that these cells differentiation follows a linear progression along a continuum of major clusters, including stem-like memory (TSCM, CD45RA+CD45RO–CCR7+CD27+CD28+) cells, central memory (TCM, CD45RO+CCR7+CD27+CD28+) cells, transitional memory (TTM, CD45RO+CCR7–CD27+CD28+) cells, effector memory (TEM, CD45RO+CCR7–, CD27–CD28+ for CD4+ T and CD27+CD28– for CD8+ T) cells, and terminal effector (TTE, CD45RA+CCR7–CD27–CD28–) cells (10). Among them, the cells with lower differentiation degrees give rise to more differentiated progeny in response to antigen stimulation or potential homeostatic signaling (10, 15, 16). For instance, TSCM cells are able to self-renew and generate all memory subsets (15, 16), TCM cells have the potential to home to secondary lymphoid tissue and are capable of generating TEM cells in vitro (17), TTE cells are terminally differentiated cells with low proliferative and functional capacity (18, 19).
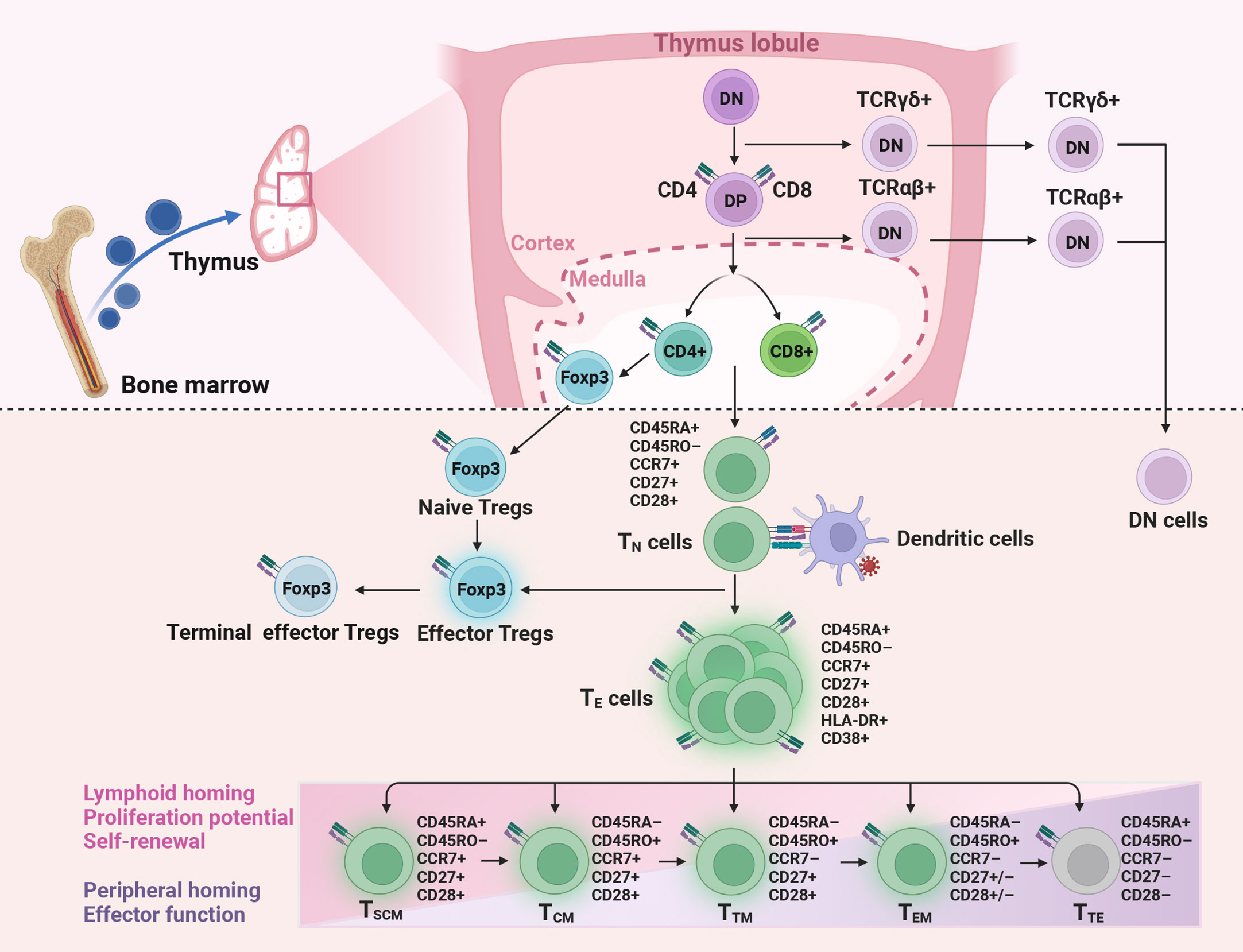
Figure 1 Production and differentiation of T cells. Naive CD4+ and CD8+ T (TN), naive regulatory T (Tregs) cells, and double-negative (DN) T cells are released from the thymus. Then CD4+ and CD8+ T cells get activated and differentiate into effector (TE) cells after encountering antigens. Only a small part of TE develops into memory T cells, including stem-like memory (TSCM), central memory (TCM), transitional memory (TTM), effector memory (TEM), and terminal effector (TTE) cells. In HIV immunological non-responders, the number of TN cells produced by the thymus is reduced, while the proportion of activated T cells is increased. DP, double-positive; TCRαβ, T cell receptor alpha and beta; TCRγδ, T cell receptor gamma and delta; Foxp3, factor forkhead box P3.
The life journey of a T cell includes quiescence, ignorance, anergy, exhaustion, senescence, and death, which is regulated by tolerance checkpoints to protect the body from hyperinflammation and autoimmunity (20). At the TN cells stage, quiescence and ignorance act as checkpoints to actively maintain tolerance (21, 22). At the effector stage, exhaustion and senescence can limit excessive inflammation and prevent immunopathology (20). However, during chronic infection or cancer, persistent antigenic stimulation or inflammation causes T cells to enter a progressive exhaustion state (23). Exhausted T cells are characterized by increasing loss in effector function coinciding with increased expression of immune checkpoint inhibitors, decreased secretion of cytokines, altered transcriptional as well as epigenetic programs, and poor memory recall as well as homeostatic self-renew (20).
2.2 CD4+ T cells
2.2.1 Decreased production
In HIV-INRs, the number of CD4+ TN cells and mature CD4+ T cell subsets were repeatedly observed to be significantly reduced (24–27). In general, the number of T cells is regulated by a dynamic balance between the production, destruction, and trafficking of lymphocytes between peripheral circulation and lymphoid organs (28). The thymus is crucial for the generation of T cells, and thymic function is usually assessed by T cell receptor excision circles (TRECs), recent thymus emigrants (RTEs), or TN cell counts. Previous studies have shown that the RTEs CD4+ T cells and the signal joint (sj)/β TREC ratios can predict disease progression in HIV-infected patients (29, 30). Moreover, the increase of TN cells, the proportion of RTEs in CD4+ T cells, the number of sj-TRECs, and the sj/β TREC ratios were significantly lower in INRs than in immunological responders (IRs) (31, 32). Our recent study also found that the CD4+ TN cells, RTEs CD4+ T cells, and TRECs were remarkably lower in INRs compared to complete responders and healthy subjects (33). These findings suggest that the thymus output of TN cells is significantly reduced in INRs, which play an important role in incomplete immune reconstitution.
In addition, HIV-infected individuals often have impaired mitochondrial function, resulting in diminished cellular metabolic activity and asymmetric mitochondrial distribution during cell division in which cells that received old mitochondria would be short-lived compared to cells that had newly synthesized mitochondria (34, 35). It is likely that INRs are incapable of generating memory long lived CD4+ T cells due to the asymmetric distribution of mitochondria in dividing cells (34). Moreover, mitochondrial dysfunction may also affect the regenerative potential of memory CD4+ T cells (36). And the low-productive proliferation of memory CD4+ T cells is linked to impaired immune restoration (36).
2.2.2 Increased destruction
In terms of T cells destruction, previous researchers found that a low nadir CD4+ T cell count was associated with high CD4+ T cell apoptosis (37), and the expression of apoptotic markers such as caspase-3, annexin-V, and proapoptotic proteins in INRs was significantly higher than that in IRs (38), indicating that apoptosis plays a major role in CD4+ T cell depletion (38, 39). In recent years, INRs and IRs were reported to have no statistical differences for RTEs CD4+ T cells in early and late apoptosis (40). However, INRs had a higher dead RTEs CD4+ T cells percentage driven by pyroptosis than IRs, and RTEs CD4+ T cells death by pyroptosis was significantly higher than by apoptosis in INRs (40). Pyroptosis is regulated by inflammasome-mediated caspase-1 activation and the release of interleukin-1β (IL-1β) and IL-18. The nucleotide-binding oligomerization domain (NOD)-like receptor (NLR) family (41), pyrin domain containing 3 (NLRP3) inflammasome is one of the prevalently studied among inflammasomes (41). According to a recent study, NLRP3 and IL-18 genes were significantly upregulated in INRs compared to IRs (42). Another study found that high-level expression of Caspase-1 and IL-18 were associated factors that affect the reconstruction of immune function (43). However, these two studies only showed the association of NLRP3, Caspase-1, and IL-18 with incomplete immune reconstitution. Zhang et al. identified that the NLRP3 inflammasome drives caspase-1 activation and pyroptosis in CD4+ T cells through a mechanism dependent on ROS production, suggesting that NLRP3-dependent pyroptosis plays an essential role in CD4+ T cell loss in chronically HIV-infected patients (44). Moreover, our recent study found that CD4+ T are prone to ferroptosis, which may be a novel way of increasing CD4+ T cell destruction (33). Collectively, these studies indicate the increase of CD4+ T cell destruction mediated by apoptosis, pyroptosis and ferroptosis may be an important cause of incomplete immune reconstitution.
2.2.3 Increased CD4+ T cell activation
Although HIV-INRs are characterized by significant decreases in the total number of CD4+ T cell counts (6, 45), the frequency of cycling CD4+ T cells is increased, and CD4+ T cells are more activated (Table 1) (25, 27, 56, 57). Using CD71 as a marker for cycling T cells, the researchers found that the significantly increased cycling cells in INRs included both CD4+CD45RA+ and CD4+CD45RA– subsets compared to IRs and healthy subjects (27), whereas IRs showed an increased frequency of CD4+CD71+CD45RA– subset with a significant decrease in CD4+CD71+CD45RA+ subset compared to healthy subjects (27). Lederman et al. found that proportions of cycling CD4+ TN cells were comparable among INRs, IRs, and healthy controls, proportions of cycling CD4+ TCM and TEM cells were significantly greater in INRs than in IRs and in healthy controls (25). In addition, cycling CD4+ T cells from healthy subjects and IRs can complete cell division in vitro, while cycling CD4+ T cells from INRs have mitochondrial dysfunction and are unable to complete cell division (58), suggesting that the function of cycling CD4+ T cells is impaired in INRs. Using CD38 and human leukocyte antigen (HLA)-DR to reflect T cell activation, Massanella et al. found that INRs exhibited a significantly increased frequency of HLA-DR expressing cells compared to IRs (46). Notably, INRs showed a significantly lower percentage of CD38+CD45RA+CD4+ T cells and a significantly higher percentage of CD38+CD45RA–CD4+ T cells (46), indicating a high level of activation in CD45RA–CD4+ T cells. In greater detail, the frequency of each subset in the CD45RA–CD4+ T cell compartment showed a significant increase in TTM, TEM, and TTE cells in INRs (47), while TCM cells showed similar levels in INRs, IRs, and healthy subjects (47). Except for the alterations in memory CD4+ T cells differentiation for INRs, their function may also be impaired. It’s reported that the metabolic activity of activated memory CD4+ T cells derived from INRs was reduced, which may lead to low regenerative potential (36). And the transition from TCM to TTM cells was found to be not completely normalized, and TCM cell death increased in vitro in INRs (47).
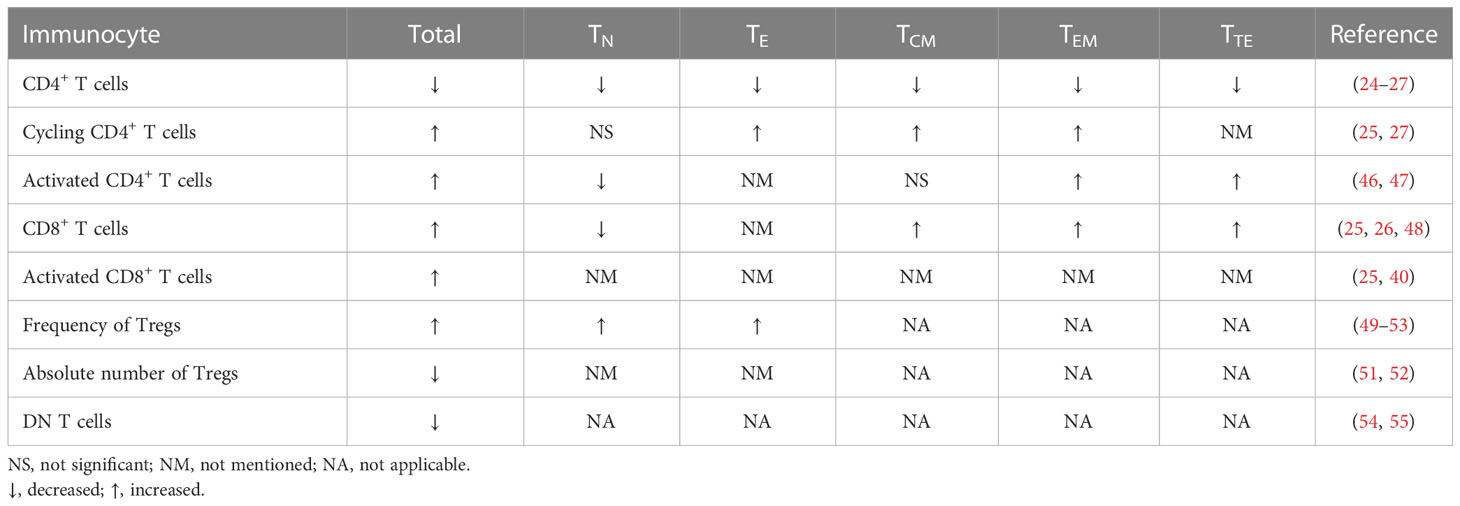
Table 1 Alterations in the quantity of immunocytes in HIV/AIDS patients with incomplete immune reconstitution.
2.2.4 Mechanisms of immune activation
The increased CD4+ T cell cycling and activation observed in INRs reflects extensive immune activation. It is firmly established that despite the effective suppression of HIV replication by ART, people living with HIV still present persistent chronic immune activation and systemic inflammation (59–61). This condition is driven by various factors, including persistent HIV viral reservoir (28), low residual viremia (62), co-infections (63, 64), microbial translocation and dysbiosis (65–67). As expected, the HIV reservoir was higher in INRs than IRs (68), and high levels of cell-associated RNA and proviral DNA were associated with lower CD4 counts (68, 69). Similar results were found by Scherpenisse et al. that the cell-associated HIV un-spliced RNA to multiply-spliced RNA ratio at 12 weeks of ART was negatively predicted CD4+ T cell counts at 48 and 96 weeks (70). However, the association between residual viremia and immune reconstitution is controversial. Some researchers found that very low-level viremia was not associated with INR (49, 71), and attempts to target residual viral replication in INRs have not yielded a decisive benefit in restoring CD4+ T cell counts (72, 73). Another study found that viral blips had no significant impact on immune reconstitution, whereas persistent detectable viremia and virological rebound to ≥5000 copies/mL were associated with arrested immune reconstitution (74). These findings suggest that the impact of low-level viremia on CD4+ T cell restoration may be related to the residual viral load levels. For intestinal microecology, plasma levels of bacterial ribosomal 16S RNA, an index of microbial translocation from the gastrointestinal tract, are correlated with the magnitude of immune restoration in HIV-infected patients on ART (75). Moreover, HIV infection alters the composition of intestinal flora and reduces its diversity, which is not normalized after the introduction of ART (76), and is closely associated with immune dysfunction (77). For example, a higher abundance of Fusobacterium (78), a reduced abundance of Ruminococcaceae (79), the relative abundances of unclassified Subdoligranulum sp. and Coprococcus comes (80), were demonstrated to be associated with poorer CD4+ T cell recovery following ART. To sum up, these studies indicate that hard-to-eliminate viral reservoirs, persistent detectable viremia, microbial translocation, intestinal flora imbalance, and co-infections contribute to excessive activation of T cells, which impair immune reconstitution. It is worth noting that the above factors are not the direct cause of incomplete immune reconstitution, and there may be individual differences in the primary causes of T cell overactivation.
2.2.5 Increased CD4+ T cell exhaustion
Persistent chronic immune activation and systemic inflammation contribute to the development of T cell exhaustion (81). In HIV infection, the expression of programmed death-1 (PD-1) on virus-specific T cells is a major marker of exhaustion (82). Other inhibitory receptors include T cell immunoglobulin and ITIM domain (TIGIT), cytotoxic T lymphocyte antigen-4 (CTLA-4), lymphocyte activation gene protein (LAG3), T cell immunoglobulin domain and mucin domain-containing protein 3 (TIM3), 2B4 (CD244) and CD160 (81). Among them, PD-1, TIGIT, LAG3, and TIM3 are common regulators of exhaustion on HIV-specific CD4+ and CD8+ T cells (83–86), CTLA-4 is more selectively upregulated on exhausted CD4+ T cells (87), and 2B4 as well as CD160 are characteristically upregulated on exhausted CD8+ T cells while low expression on exhausted CD4+ T cells (88). For CD4+ T cell exhaustion-related markers, researchers found that they were tightly correlated with the size of the T cell viral reservoir (89, 90), the decrease of CD4+ T cell counts, and disease progression (82, 87, 91). For example, in HIV elite controllers who can spontaneously control viral replication in the absence of ART and maintain a high CD4+ T cell count, the co-expression pattern of PD-1, TIGIT, and CTLA-4 was similar to healthy controls, and significantly lower than those of subjects receiving ART (85). Furthermore, the percentage of co-expression of inhibitory molecules on memory CD4+ T cells significantly negatively correlates to CD4 count and CD4/CD8 ratio (85). Cockerham LR et al. also found that PD-1 expression on CD4+ T cells was associated with CD4+ T cell activation and inversely with CD4+ T cell counts in patients on ART (69, 92). Similarly, patients with incomplete immune reconstitution despite successful ART express significantly higher levels of PD-1 than patients with normal recovery of CD4+ T cells (27, 93, 94). These studies indicate that inhibitory receptors mediated T cell suppression may be involved in the development of impaired immune reconstitution in HIV patients. However, the specific regulatory mechanism needs to be further studied.
Exhausted CD4+ T cells exhibit reduced proliferative capacity and helper functions, and a decreased production of cytokines such as IL-2 and interferon-gamma (IFN-γ) (81, 95, 96). Moreover, cell apoptosis is positively correlated with the level of PD-1 expression, indicating that exhausted CD4+ T cells from INRs are prone to apoptosis (27).
2.2.6 Increased CD4+ T cell senescence
Aside from T cell exhaustion, chronic viral infection and inflammation further induce immune senescence (27, 97, 98). The senescent T cell phenotype is marked by a lack of CD28 expression, a decrease in homing receptors (such as CD62L and CCR7), and an increase in the expression of the senescence marker, CD57 (99). In HIV-infected patients on ART, there was a significant negative correlation between the absolute count of the CD4+ TN cell subset and the expression of CD57 (26). Also, HIV-INRs displayed an increased frequency of CD57+ cells in total CD4+, CD4+CD45RA+, and CD4+CD45RA– cell subsets, and cycling as well as non-cycling CD4+ T cells compared to IRs (27). These results indicate that CD4+ T cells from INRs are generally more activated, exhausted, and senescent despite successful control of viral replication (26, 27). However, senescent cells exhibit telomere loss, mitochondrial compromise, cell cycle arrest, activation of pro-inflammatory secretory pathways, and limited proliferation in response to antigen stimulation (27, 99, 100). And the higher the expression of CD57 in CD4+ T cells, the lower their proliferative capacity (27).
2.3 Regulatory T cells
Regulatory T cells (Tregs) expressing the transcription factor forkhead box P3 (Foxp3) are naturally produced in the thymus as a functionally mature subpopulation of CD4+ T cells (nTregs) and can also be induced from TN cells (iTregs) after encountering of antigens in the periphery (101, 102). They can suppress the proliferation of TN cells, the differentiation from TN to TE cells, the effector activities of differentiated T cells, and can also suppress the functions of B cells, natural killer (NK) cells, NKT cells, as well as antigen-presenting cells (103–105). Thus, Tregs are indispensable for the maintenance of self-tolerance and immune homeostasis (103).
The role of Tregs in the pathogenesis of HIV infection has been extensively debated. They can play a beneficial role by inhibiting T cell activation and HIV replication in CD4+ T cells (106, 107), or play a harmful role by inhibiting HIV-specific CD4+ and CD8+ T cell responses (108, 109), aggravating lymphatic tissue fibrosis (110), and contributing for intestinal flora translocation (111). More importantly, dysregulation of homeostasis in Tregs may hamper immune reconstitution. According to several studies, HIV-INRs have a significantly higher percentage of Tregs within the CD4+ T cells (49–53), including total, naïve, effector, and terminal effector Tregs (49, 50), together with a drop in the absolute number of Tregs and a decrease in HIV-specific immunosuppressive functions (51, 52). A higher percentage of Tregs is associated with a reduced thymic output of CD4+ TN cells (52). Méndez-Lagares et al. also found a negative correlation between Tregs and CD4+ TN cells (51). Moreover, a recent study proposed that the failure of INRs to restore CD4+ T cells as a consequence of defective Treg survival and function, resulting in a phenotype of uncontrolled cycling, immune exhaustion, and increased cell death (58). As for the effect of baseline Tregs, one study found that Tregs percentage at baseline was a strong independent prognostic factor of immune recovery, and a 1% increase of initial Tregs percentage was associated with a 1.9% lower CD4+ T cell counts at month 24 (112). In contrast, another study found no effect of Tregs percentages at baseline was detected on CD4+ T cells recovery (113). These studies suggest that although the impact of baseline Tregs on immune reconstitution is controversial, a high percentage and functional defects of Tregs during antiretroviral therapy have a negative impact on immune reconstitution. Furthermore, it is necessary to validate the potential value of Tregs in HIV-INRs in larger sample cohorts because of the limited number of current studies and sample sizes.
2.4 CD8+ T cells
CD8+ T cells are a critical component of the cellular immune response to viral infections. In HIV-infected patients, CD8+ T cells play an important role in the control of HIV replication and the HIV reservoir (114–116). Additionally, for patients with CD4+ T cells above 500 cells/μL after long-term ART, high CD8+ T cell counts are associated with CD4 recovery (117). However, in incomplete immune reconstitution, the quantity and quality of CD8+ T cells are disordered. According to previous studies, the absolute numbers of CD8+ TN cells were significantly lower in INRs than that in IRs and healthy subjects, while circulating TCM, TEM, and TTE cells were higher in INRs (25). Méndez-Lagares et al. also found that the frequency of the CD8+ TN cell subset was significantly lower in INRs, and the CD8+ TEM cells showed a significant expansion in INRs when compared with the IRs (26). In fact, the total number of CD8+ T cells is consistently elevated even after long-term ART in HIV-infected patients (48). Additionally, when the low CD4+ T cells patients were included, the high CD8+ T counts were associated with a poor increase in CD4+ T cells during ART (48). These results can be attributed to the absence of CD4+ T cells help on the one hand (117), and the activation and exhaustion of CD8+ T cells on the other hand (118). As the study reported, CD8+ T cell counts were positively correlated with the viral reservoir (119–121), and the elevation of CD8+ T cells is associated with immune activation and increased immune anergy (48, 122). Elite controllers possessed a significantly lower level of activated HIV-specific CD8+ T cells than non-controllers (123), while INRs possessed a higher level of activated CD8+ T cells (CD38+, HLA-DR+) than IRs (25, 40). Hunt et al. found that for every 5% increase in the proportion of activated CD8+ T cells mean 35 fewer CD4+ T cells were gained (124). These studies indicate that the extensive expansion, activation, and exhaustion of CD8+ T cells further contribute to CD8 accumulation over disease progression, which plays a role in incomplete immune reconstitution.
The exhaustion of CD8+ T cells is a progressive condition that starts with an initial loss of proliferation, cytotoxic potential as well as decreased IL-2 production, and eventually loss of the ability to produce IFN-γ in more pronounced stages (83). Therefore, exhausted CD8+ T cells in HIV-infected patients lose their capacity to kill efficiently infected cells (83). Previous studies showed that PD-1 expression on virus-specific T cells is the primary marker of exhaustion (82). The expression of PD-1 on CD8+ T cells is significantly higher in HIV-INRs than IRs (93, 94), suggesting that INRs have more exhausted CD8+ T cells. Similarly, Li et al. found that the frequencies of PD-1+CD39+ CD8+ T cells are negatively correlated with CD4+ T cell counts and the CD4/CD8 ratio in ART naïve patients (125).
However, in contrast to T cell exhaustion, the concept of CD8+ T cell senescence induced by HIV remains controversial (118). Especially in INRs, the study found that CD8+ memory, TEM, and TTE cells showed lower markers of senescence than those of the IRs (26). One possible reason is that HIV inhibited the process of terminal differentiation and proliferation of CD8+ TEM cells, expanded the less-differentiated transitional memory and CD28–CD57–CD8+ T cells, therefore decreasing the proportion of CD28–CD8+ T cells that express CD57 (126).
2.5 Double-negative T cells
Double-negative (DN) T cells represent a small subpopulation of approximately 3-5% of T lymphocytes in peripheral blood (127, 128). They are CD3 positive, CD4 and CD8 negative, express either TCR alpha and beta (αβ) or TCR gamma and delta (γδ), but do not express NK T cell markers (129). Although lacking certain phenotypic classification, DN T cells can be divided into naïve and active cells according to the transcriptome landscape (130). It can also be classified according to different functions, such as DN Tregs that can secrete anti-inflammatory cytokines and exhibit remarkably potent immunosuppressive potential (129, 131), T helper (Th)-like DN T Cells that can secrete cytokines to exert Th function and exhibit either protective or pathologic functions in different infections (129, 132, 133), cytotoxic DN T cells that mediate the killing effect of malignant tumors (134, 135).
In SIV/HIV infection, studies have found that DN T cells are associated with disease progression (54, 133, 136). Milush et al. reported that DN T cells with Th-like helper functions may compensate for the very low levels of CD4+ T cells in SIV-infected sooty mangabeys that were free of clinical AIDS for a long time (133). However, Liang et al. demonstrated that the numbers of DN T cells in HIV-infected patients with CD4+ T cells <250 cells/μL were significantly lower than those with CD4+ T cells between 250-500 as well as >500 cells/μL (54). Another study found that HIV-INRs had a low level of DN T cells, and the number of these cells was positively correlated with CD4+ T cell counts but negatively correlated with immune activation (55). Moreover, the production of transforming growth factor (TGF)-β1 by DN T cells might participate in the downregulation of immune activation after long-term ART (55), suggesting that DN T cells may play a role in immune reconstitution by regulating the immune response. On the contrary, Wang et al. found that the frequency of DN T cells was comparable between INRs and IRs, and had no correlation with immune activation, while only CD73+ DN T frequency was positively correlated with CD4+ T cell counts (137). It is suggested that there may be other mechanisms in the participation of DN T cells in immune reconstitution.
To sum up, CD4+ T cells were decreased in quantity and altered in quality in HIV- INRs receiving antiviral therapy (Figure 2). Among them, the decrease in production and the increase in destruction of CD4+ T cells are the direct reasons for incomplete immune reconstitution. The abnormal quality is characterized by T cells’ overactivation, exhaustion, senescence, and susceptibility to death, resulting in weakened cell proliferation and differentiation, increased secretion of inflammatory molecules, and decreased secretion of anti-inflammatory cytokines. Furthermore, suboptimal CD4+ T recovery is associated with impaired homeostasis of other T cells such as CD8+ T cells, Tregs, and DN T cells. Although the abnormal quality of CD4+ T cells and impaired homeostasis of other T cells are not the direct cause of incomplete immune reconstitution, they may be involved in its pathological process. And the specific mechanism needs to be further studied.
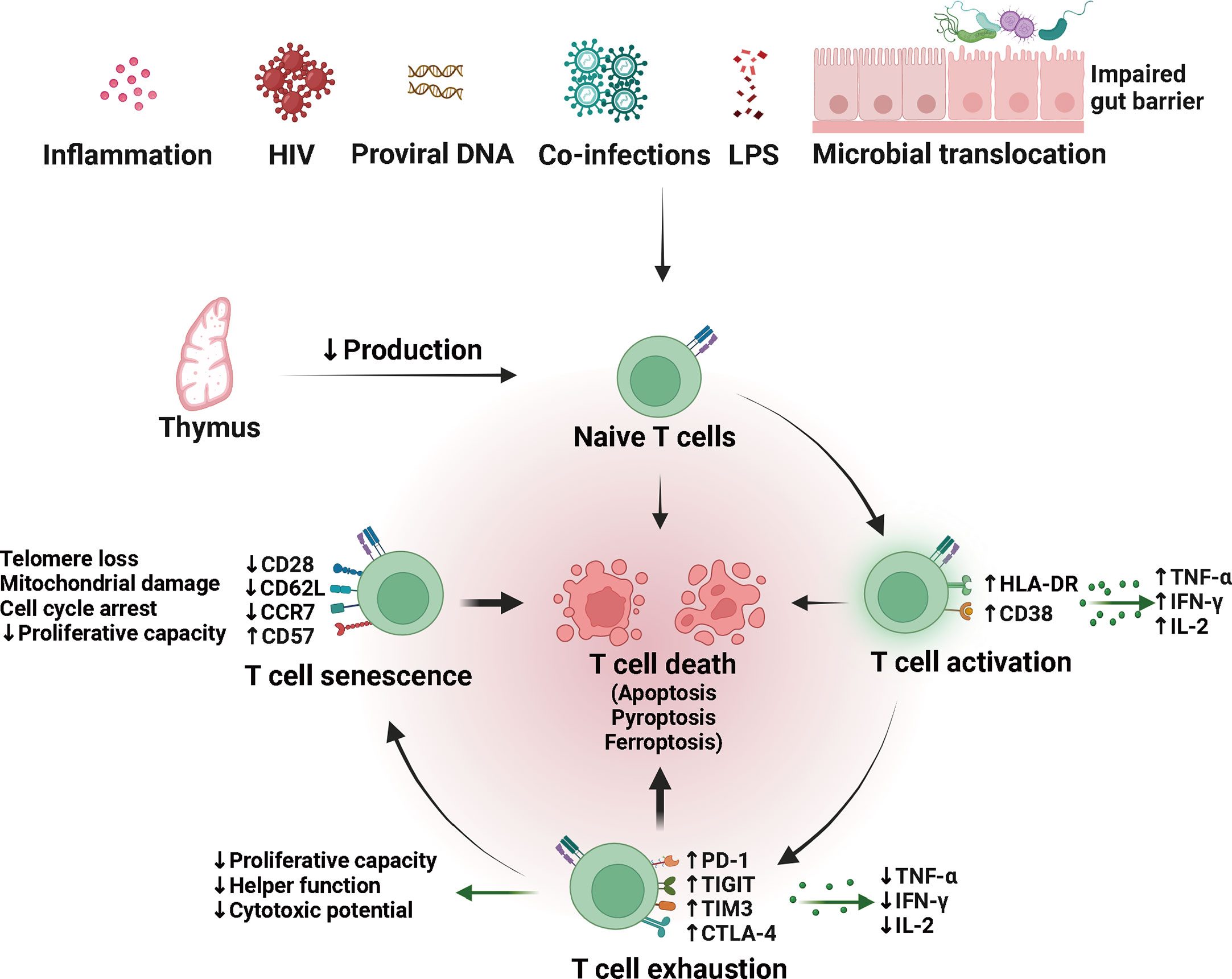
Figure 2 Quantity and quality alterations of T cells in HIV/AIDS patients with incomplete immune reconstitution. The naïve T (TN) cells produced by the thymus can be activated by multiple factors, including persistent viral reservoir, low residual viremia, co-infections, microbial translocation and dysbiosis. Difficult-to-remove risk factors may lead to persistent chronic immune activation, contributing to the development of T cell exhaustion and immune senescence. Exhausted and senescent T cells are qualitatively altered and prone to death, possibly through apoptosis, pyroptosis, and ferroptosis. LPS, lipopolysaccharide; HLA-DR, human leukocyte antigen DR; TNF-α, tumor necrosis factor-alpha; IFN-γ, interferon-gamma; IL-2, interleukin-2; PD-1, programmed death-1; TIGIT, T cell immunoglobulin and ITIM domain; TIM3, T cell immunoglobulin domain and mucin domain-containing protein 3; CTLA-4, cytotoxic T lymphocyte antigen-4.
3 Other immune cells
3.1 Natural killer cells
Human NK cells are broadly distributed innate lymphocytes (138, 139), including three common subpopulation groups: CD56brightCD16dim/− subpopulation which is typically viewed as immature precursors and primarily secretes cytokines, the larger CD56dimCD16bright subpopulation which is considered as mature subset with toxic effects, and the dysfunctional CD56–CD16bright subpopulation with low cytotoxic activity and cytokine production (138, 140). In HIV infection, NK cells play a negative regulation as well as protective function (141), and may be associated with immune reconstitution. Bayigga et al. found that INRs had a higher proportion of pro-inflammatory CD56brightCD16dim/− NK cells than IRs, while the largest CD56dim NK cell subset was comparable among INRs and IRs (142). Similarly, another study showed that INRs exhibit an accumulation of autoreactive CD56bright NK cells, possibly linked to decreased homeostatic control by Tregs, which contributes to incomplete immune reconstitution (143). However, Luo et al. reported that the absolute number, percentage, and subpopulation percentage of NK cells were similar between INRs and IRs, while the increased CD56dimCD16+ NK cell activation was predominantly in INRs and inversely correlated with the peripheral CD4+ T cell counts (144). Recently, the existence of a population of CD56dimCD16dim/− NK cells was detected and found to be significantly higher in INRs than IRs (145). In addition to increased proportion, the killing ability of CD56dim NK cells was also significantly increased in INRs, and significantly correlated with apoptosis of T lymphocytes (146). Although the above studies are not identical, they consistently indicate that NK cells play a negative role in immune reconstitution.
3.2 B cells
B cells are generated from stem cells in the bone marrow, and enter the periphery at an immature/transitional stage after forming a fully functional B-cell receptor, then develop into naïve B cells after further selection, accompanied by increased expression of CD21 (147, 148). Once a naïve B cell migrates into peripheral lymphoid tissues and encounters an antigen, its response can be divided into two ways: one that occurs without T-cell help, and one that occurs with T-cell help typically within the microenvironment of the germinal center (147). Affinity-matured B cells that exit the germinal center either serve as memory B cells or as long-lived plasma cells (148). In HIV infection, B cell-mediated immune response is sustained by HIV-specific memory B cells and plasma cells (148). A recent study found that the proportions of naïve B, memory B, and plasma cells are not associated with immune recovery, while the low frequency of CD21+ memory B cells is a risk factor for incomplete immune reconstitution (149). This may be related to the dysregulation of memory B cells and circulating T follicular helper cells (149, 150). In addition, another study found that the diversity of the B-cell receptor repertoire in HIV-INRs was decreased, and naïve B cells with low differentiation improve the immune reconstitution (151). These findings underscore the critical role of B cells in immune reconstitution after HIV infection.
3.3 Monocytes/macrophages and dendritic cells
Monocytes/macrophages act as first responders in innate immunity and then as mediators for adaptive immunity to help clear infections (152). In performing these functions, the macrophage inflammatory responses may also contribute to the pathogenesis. Stiksrud et al. found that HIV-infected individuals with suboptimal immune recovery exhibited more activated monocytes and dendritic cells (DCs) compared to individuals with adequate immune recovery (153). The persistent inflammation and activation of monocytes and other innate immune cells are likely associated with the persistent T cell activation and impaired effector functions in adults receiving antiretroviral therapy (154). Thus, it is suggested that monocytes/macrophages and DCs may be involved in the pathological process of changes in the quality of CD4+ T cells.
4 Soluble mediators and cytokines
4.1 Mechanisms of soluble biomarkers production
The immune response to HIV infection begins with infected CD4+ innate immunocytes and CD4+ T cells, where the pathogen-associated molecular patterns (PAMPs) in viral products are sensed by the pathogen-recognition receptors (PRRs) of the host cell to trigger the innate immune response (155). The PRRs include the Toll-like receptors (TLRs), NLRs, RIG-I-like receptors (RLRs), and the novel DNA sensor cyclic GMP/AMP synthase (cGAS) as well as IFN-inducible protein 16 (IFI16) (156–158). Among them, TLR is located on the surface of various immunocytes and intracellular organelle membranes. Its signal transduction pathways mainly include myeloid differentiation primary response gene 88 (MyD88)-dependent pathways and MyD88-independent pathways (Figure 3). TLR2/6 is dependent on MyD88, TLR3 is independent of MyD8, and TLR4 employs both signaling pathways (159). In MyD88-dependent pathways, TLRs combine with intracellular junction protein MyD88, then recruit the downstream TNF receptor-associated factor (TRAF)-6, and activate nuclear factor (NF)-κB and IFN regulatory factor (IRF)-7, induce the expression of pro-inflammatory factors and type I interferons (IFN-I) (159, 160). In MyD88- independent pathways, TLRs sense HIV products and recruit downstream TRAF3 and TRAF6, then activate NF-κB and IRF3 to trigger the production of inflammatory factors (161). NLRs represent a diverse family of PRRs expressed in the cytosol of various cell types, which can sense viral single-stranded RNA and signal to the activation of NF-κB and IRF3, and can also interact with caspase-1 upon activation to induce pyroptosis (162, 163). RLRs are another important class of PRRs that can sense the double-stranded RNA, then binds Cardif in the mitochondria to recruit I κB kinase (IKK), and activate NF-κB and IRF-3 to trigger IFN-I production to exert antiviral effect (159, 164). IFI16 and cGAS can sense and bind to cytosolic double-stranded DNA, then activate the stimulator of interferon genes (STING) signal pathways (157, 158).
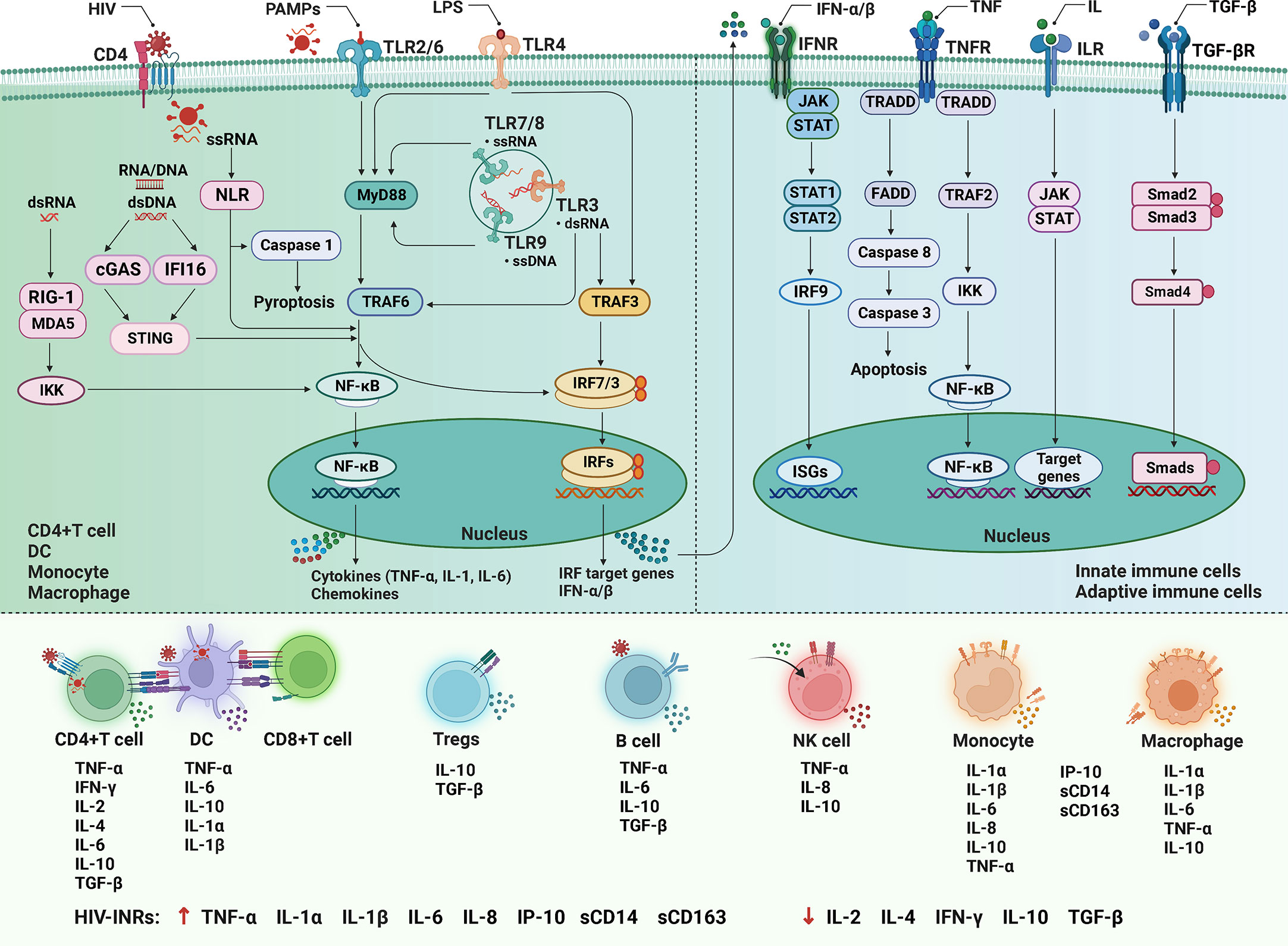
Figure 3 The cytokine signaling in HIV infection. After HIV infection, the pathogen-associated molecular patterns (PAMPs) are sensed by the pathogen-recognition receptors (PRRs) to trigger the innate immune response. Among them, the Toll-like receptors (TLRs) signal transduction pathways mainly include myeloid differentiation primary response gene 88 (MyD88) dependent and independent pathways. In the former, TLRs combine with MyD88 to recruit tumor necrosis factor (TNF) receptor-associated factor (TRAF)-6, and activate nuclear factor (NF)-κB and IFN regulatory factor (IRF)-7, induce the expression of pro-inflammatory factors and type I interferons (IFN-I); in the latter, TLRs sense HIV products and recruit TRAF3 and TRAF6, then activate NF-κB and IRF3. The nucleotide-binding oligomerization domain-like receptors (NLRs) can sense single-stranded RNA (ssRNA), and can also interact with caspase-1 to induce pyroptosis. The RIG-I-like receptors (RLRs) can sense the double-stranded RNA (dsRNA) to recruit I κB kinase (IKK) and activate NF-κB and IRF-3. The cyclic GMP/AMP synthase (cGAS) and IFN-inducible protein 16 (IFI16) can bind to double-stranded DNA (dsDNA), then activate the stimulator of interferon genes (STING) signal pathways. Initiation of the innate immune response produces a variety of soluble factors that recruit and activate innate immune cells. In addition, IFN-I, TNF, interleukin (ILs), and transforming growth factor (TGF)-β respectively bind to cell surface receptors and activate downstream signaling pathways to participate in immune regulation. LPS, lipopolysaccharide; MAD5, melanoma differentiation-associated protein 5; JAK-STAT, Janus kinase and signal transducer and activator of transcription proteins; ISGs, interferon-stimulated genes; TRADD, TNF receptor 1 (TNFR1)-associated death domain protein; FADD, Fas-associated protein with death domain; DC, dendritic cell; NK, natural killer cell; INRs, immunological non-responders.
Initiation of the innate immune response, in addition to antiviral defense, produces a variety of soluble factors, including IFN-I, inflammatory cytokines and chemokines. These soluble factors recruit and activate innate immune cells, including DCs, monocytes/macrophages and NK cells (155). In addition, IFN-I, TNF, ILs, and TGF-β respectively bind to cell surface receptors, activate downstream signaling pathways, and play several essential roles, such as inducing and regulating the development, differentiation, survival, and function of myeloid and lymphoid cells, activating and regulating adaptive immune response, and participating in inflammatory response (165).
4.2 T cell-related cytokines
Chronic infection and systemic inflammation lead to persistent activation of the immune system, and activated lymphocytes are consumed, which affects the secretion of cytokines. As reported in previous studies, HIV-INRs had a low level of certain cytokines than that in IRs, including IL-2, IL-4, IL-10, and IFN-γ (143, 146, 166). These cytokines have been proven to be closely correlated to the activation and proliferation of T lymphocytes (167–169). Thus, the decrease of cytokine production by lymphocytes in INRs eventually leads to T cell depletion (146). In theory, improving the regulation of T cell-related cytokines may be beneficial for INRs. For example, treatment with IL-2 in HIV-infected patients can significantly increase CD4+ T cell counts and enhance immune function (170).
IL-7 is also crucial in T cell homeostasis as it maintains T cell survival, induces proliferation, and promotes de novo production (171, 172). The IL-7 responsiveness is largely dependent on the presence or absence of the IL-7 receptor (IL-7R). But unlike the cytokines mentioned above, IL-7 is mainly produced by bone marrow and thymic stromal cells (173). In HIV-infected patients, the level of IL-7 is higher and the level of IL-7R is lower than that in healthy controls (174, 175), and there is a negative correlation between plasma IL-7 levels and CD4+ T cell counts (176). Consequently, INRs exhibit a higher stromal production of IL-7, a diminished expression of IL-7R and a reduced IL-7 mediated proliferation responsiveness compared to normal responders (177–180). Moreover, the reduction of naïve and thymic naïve CD4+ T cells in INRs is associated with increased serum IL-7 levels and decreased IL-7R expression (24). Down-regulation of IL-7R is related to T cell activation and is a main factor influencing the restoration of CD4+ T cells (181). Marziali et al. also found that the reduced expression of IL-7Rα was associated with persistent immune activation and the alteration of Treg frequencies, which in part explains the low level of CD4+ T cells observed in INRs (24). Another study found that the IL7RA polymorphisms seem to predict the CD4+ T cell recovery in HIV-infected patients on ART (182). These studies indicate that dysregulation of IL7/IL7R homeostasis plays an important role in incomplete immune reconstitution. Thus, IL-7-based therapy, combined with efficient ART, may be beneficial to HIV-INRs by promoting thymic output, inducing a sustained increase of TN and TCM cell counts, thereby enhancing T cell recovery (183–185).
4.3 Innate immunity activation markers
Soluble CD14 (sCD14) is part of innate immunity and plays an important role in the inflammatory response induced by lipopolysaccharide, an outer cell wall component of gram-negative bacteria. Plasma sCD14 forms a complex with lipopolysaccharide, then binds to LPS receptors on monocytes/macrophages, and activates the cells to produce pro-inflammatory cytokines (186). A series of studies have shown that HIV-infected patients have higher levels of sCD14 than healthy subjects (187–189), and plasma sCD14 are independently associated with disease progression (188, 190). Dunham et al. found that plasma sCD14 levels from INRs were significantly higher than that in HIV-negative subjects, while the difference between INRs and IRs was not significant (191). Moreover, the concentrations of sCD14 during ART were inversely associated with subsequent CD4+ T cell counts (191, 192), and were also correlated with blood inflammatory markers, shorter telomeres, and increased Treg levels (98, 191).
Soluble CD163 (sCD163) is another hallmark of monocyte/macrophage activation. It was higher in the plasma of chronic HIV-infected patients than in healthy subjects, and decreased after effective ART but did not return to HIV-seronegative levels (193). The high level of plasma sCD163 was correlated with gut mucosal disruption, positively correlated with the percentage of CD14+CD16+ monocytes and T cell activation markers (193, 194), and increased sCD163 may serve as a marker of immunosenescence (195). Moreover, Fischer-Smith et al. found a strong inverse correlation between CD163+/CD16+ monocyte and the number of CD4+ T cells below 450 cells/μL (196). Plasma sCD163 levels were also inversely correlated with CD4+ T cell percentage and CD4/CD8 ratio (194).
Interferon-γ-induced protein 10 (IP-10 or CXCL-10) is a chemokine involved in trafficking immune cells to inflammatory sites. It is produced by various cell types on stimulation, while monocytes are responsible for the greatest proportion of IP-10 expression (197). Plasma IP-10 levels are significantly higher in HIV-infected patients than that in healthy subjects (198–201), and inversely related to CD4+ T cell counts, regardless of those pre- or post-ART (200, 202). IP-10 levels are also associated with the time for CD4+ T cell counts to fall below 200 cells/μL during Fiebig stages III-V (203). In addition, elevated IP-10 levels are associated with immune activation and can promote the progression of inflammation (197, 204). Meanwhile, exposure to persistent IP-10 leads to a decrease in the number of CD4+ and CD8+ T cells capable of producing cytokines, a decrease in T cell proliferation, and can effectively impair NK cell function (198, 205).
Taken together, these studies suggest that monocyte/macrophage activation, marked by increased expression of sCD14, sCD163, and IP-10, is associated with T cell activation, immune senescence, and impaired immune cell function in HIV-infected patients, which is involved in the occurrence of incomplete immune reconstitution and may be an intervention measure for INRs.
4.4 Key pro-inflammatory cytokines
Immune activation and systemic inflammation are usually accompanied by the secretion of soluble inflammatory mediators, such as pro-inflammatory cytokine TNF-α, IL-1β, IL-6, and IL-8. Among them, plasma IL-6 levels were found to be elevated in HIV-INRs before ART as well as after virological suppression (206, 207), and were negatively correlated with CD4+ T cell counts (208). In addition, the intestinal microbiota of HIV/AIDS patients was disordered, and the number of intestinal flora was correlated with the number of CD4+ T cells and the levels of TNF-α and IL-6 (209). Shive et al. found that IL-6 can induce low-level cycling of TN cells, IL-1β can induce cell cycling and turnover of memory CD4+ T cells, and both cytokines can decrease T cell surface expression and RNA levels of IL-7 receptor (57). Moreover, the induction of CD4+ T cell turnover and diminished T cell responsiveness to IL-7 by IL-1β and IL-6 exposure may contribute to the lack of CD4+ T cell reconstitution in HIV-infected subjects on ART (57). These studies demonstrate that pro-inflammatory cytokines negatively affect the quantity of CD4+ T cells.
4.5 Anti-inflammatory cytokines
In addition to IL-10, TGF-β is another major anti-inflammatory cytokine that controls the development, differentiation, and function of Tregs (210). Younes et al. showed that the expression of genes for the TGF-β signaling pathway (TGIF1, SMAD1, SMAD7, LEFTY) was lower in HIV-INRs (58), and the production of TGF-β by Tregs was impaired in the setting of incomplete immune reconstitution (58). Another study also found that plasma TGF-β levels were significantly lower in the INRs when compared to plasma levels in the IRs (211). In addition, plasma levels of TGF-β were negatively correlated with T cell exhaustion and senescence phenotypes, and positively correlated with CD4+ T cell counts in INRs (211). These studies indicate that low levels of anti-inflammatory cytokines are associated with impaired function of Tregs and difficulty in controlling inflammation, which may participate in the occurrence of incomplete immune reconstitution in HIV-infected patients.
4.6 Other biomarkers associated with immune reconstitution
HIV infection induces widespread expression of IFN-I and IFN-stimulated genes (212, 213), and the abnormally elevated IFN-I signaling persists in some patients even under extensive ART (191, 214). Chronic exposure to IFN-I hampers the reversion of hyperimmune activation and immune recovery in INRs (191, 213). Therefore, targeting IFN-I mediated activation may provide a potential strategy to enhance T cell recovery (215, 216).
C-reactive protein (CRP) is a prophylactic acute-phase plasma protein and a non-specific marker of systemic inflammation that is stimulated by cytokines such as IL-6, IL-1, and TNF to be produced in the liver. The levels of CRP were significantly higher in INRs than that in IRs (217), and were inversely associated with CD4+ T cell counts (192). For hypersensitive CRP (hsCRP), there was no difference between INRs and IRs (26). However, the INRs showed significantly higher levels of hsCRP in comparison with healthy subjects (218), and INRs showed a tendency to have more subjects with hsCRP levels exceeding 2 μg/mL or 3 mg/dL when compared with IRs (26, 218).
Additionally, soluble TNF receptors, sTNF-RI, and sTNF-RII, were measured in plasma as biomarkers of TNF activity. Dunham et al. found that although the difference in sTNF-RI and sTNF-RII levels between INRs and IRs did not reach statistical significance, the levels of sTNF-RII in INRs were higher than those found in healthy subjects and were more comparable to those observed in viremic subjects (191).
5 Conclusions and perspectives
In summary, CD4+ T cell homeostasis alteration in HIV-infected subjects with incomplete immune reconstitution despite successful viral suppression during ART, including decreased quantity and altered quality. Additionally, suboptimal CD4+ T cell recovery is associated with impaired homeostasis of multiple immunocytes such as CD8+ T cells, Tregs, DN T cells, NK cells, B cells, monocytes/macrophages and DCs, as well as abnormal secretion of various soluble mediators and cytokines. While these data are impressive and informative, there is limited understanding of the primary causes of incomplete immune reconstitution and the causal relationship of immunocytes or soluble mediators to incomplete immune reconstitution. This will be a meaningful research direction that can help to identify INRs earlier and provide physicians with optimal strategies to improve CD4+ T cell recovery. When solving the above problems, it should be noted that different individuals may have different primary causes of incomplete immune reconstitution. Methodologically, immunology, genomics, transcriptomics, and single-cell sequencing methods are useful tools in this regard. Moreover, future more in-depth mechanistic and clinical studies are needed to develop immune-based interventions for incomplete immune reconstitution.
Author contributions
LY, FZ and XY were involved in the conception of the study. LY wrote the draft of the review. KX, QX, LT, TL, SW and RY revised the manuscript. All authors contributed to the article and approved the submitted version.
Funding
This work was supported by the Young Talent Fund of Sichuan Provincial People’s Hospital (2022QN46), and the Sichuan Science and Technology Program (2019YFS0368, 2020YFS0408).
Conflict of interest
The authors declare that the research was conducted in the absence of any commercial or financial relationships that could be construed as a potential conflict of interest.
Publisher’s note
All claims expressed in this article are solely those of the authors and do not necessarily represent those of their affiliated organizations, or those of the publisher, the editors and the reviewers. Any product that may be evaluated in this article, or claim that may be made by its manufacturer, is not guaranteed or endorsed by the publisher.
References
1. Maartens G, Celum C, Lewin SR. HIV Infection: epidemiology, pathogenesis, treatment, and prevention. Lancet (2014) 384(9939):258–71. doi: 10.1016/s0140-6736(14)60164-1
2. Guihot A, Bourgarit A, Carcelain G, Autran B. Immune reconstitution after a decade of combined antiretroviral therapies for human immunodeficiency virus. Trends Immunol (2011) 32(3):131–7. doi: 10.1016/j.it.2010.12.002
3. Wu Z, Yang C, Ma Y, Wang Y, Zhang Z, Liu Z, et al. The prevalence of HIV-infected patients with virological suppression but a CD4(+) T-cell count of ≤ 200 cells/mm(3) after highly active antiretroviral therapy initiation: a meta-analysis. AIDS Rev (2022) 24(1):16–23. doi: 10.24875/AIDSRev.21000011
4. Yang X, Su B, Zhang X, Liu Y, Wu H, Zhang T. Incomplete immune reconstitution in HIV/AIDS patients on antiretroviral therapy: challenges of immunological non-responders. J Leukoc Biol (2020) 107(4):597–612. doi: 10.1002/jlb.4mr1019-189r
5. Shuai Y, Peng H, Wang X, Pend X. A method for the definition of immunological non-response to antiretroviral therapy based on review analysis and supervised classification model. J Antivir Antiretrovir (2022) S24:005. doi: 10.35248/1948-5964-22.14.005
6. Rb-Silva R, Goios A, Kelly C, Teixeira P, João C, Horta A, et al. Definition of immunological nonresponse to antiretroviral therapy: a systematic review. J Acquir Immune Defic Syndr (2019) 82(5):452–61. doi: 10.1097/qai.0000000000002157
7. Engsig FN, Zangerle R, Katsarou O, Dabis F, Reiss P, Gill J, et al. Long-term mortality in HIV-positive individuals virally suppressed for >3 years with incomplete CD4 recovery. Clin Infect Dis (2014) 58(9):1312–21. doi: 10.1093/cid/ciu038
8. Pacheco YM, Jarrin I, Rosado I, Campins AA, Berenguer J, Iribarren JA, et al. Increased risk of non-AIDS-related events in HIV subjects with persistent low CD4 counts despite cART in the CoRIS cohort. Antiviral Res (2015) 117:69–74. doi: 10.1016/j.antiviral.2015.03.002
9. Xiao Q, Yu F, Yan L, Zhao H, Zhang F. Alterations in circulating markers in HIV/AIDS patients with poor immune reconstitution: novel insights from microbial translocation and innate immunity. Front Immunol (2022) 13:1026070. doi: 10.3389/fimmu.2022.1026070
10. Mahnke YD, Brodie TM, Sallusto F, Roederer M, Lugli E. The who's who of T-cell differentiation: human memory T-cell subsets. Eur J Immunol (2013) 43(11):2797–809. doi: 10.1002/eji.201343751
11. Germain RN. T-Cell development and the CD4-CD8 lineage decision. Nat Rev Immunol (2002) 2(5):309–22. doi: 10.1038/nri798
12. Williams MA, Bevan MJ. Effector and memory CTL differentiation. Annu Rev Immunol (2007) 25:171–92. doi: 10.1146/annurev.immunol.25.022106.141548
13. Kaech SM, Cui W. Transcriptional control of effector and memory CD8+ T cell differentiation. Nat Rev Immunol (2012) 12(11):749–61. doi: 10.1038/nri3307
14. Masopust D, Schenkel JM. The integration of T cell migration, differentiation and function. Nat Rev Immunol (2013) 13(5):309–20. doi: 10.1038/nri3442
15. Gattinoni L, Lugli E, Ji Y, Pos Z, Paulos CM, Quigley MF, et al. A human memory T cell subset with stem cell-like properties. Nat Med (2011) 17(10):1290–7. doi: 10.1038/nm.2446
16. Lugli E, Dominguez MH, Gattinoni L, Chattopadhyay PK, Bolton DL, Song K, et al. Superior T memory stem cell persistence supports long-lived T cell memory. J Clin Invest (2013) 123(2):594–9. doi: 10.1172/jci66327
17. Sallusto F, Lenig D, Förster R, Lipp M, Lanzavecchia A. Two subsets of memory T lymphocytes with distinct homing potentials and effector functions. Nature (1999) 401(6754):708–12. doi: 10.1038/44385
18. Lugli E, Goldman CK, Perera LP, Smedley J, Pung R, Yovandich JL, et al. Transient and persistent effects of IL-15 on lymphocyte homeostasis in nonhuman primates. Blood (2010) 116(17):3238–48. doi: 10.1182/blood-2010-03-275438
19. Geginat J, Lanzavecchia A, Sallusto F. Proliferation and differentiation potential of human CD8+ memory T-cell subsets in response to antigen or homeostatic cytokines. Blood (2003) 101(11):4260–6. doi: 10.1182/blood-2002-11-3577
20. ElTanbouly MA, Noelle RJ. Rethinking peripheral T cell tolerance: checkpoints across a T cell's journey. Nat Rev Immunol (2021) 21(4):257–67. doi: 10.1038/s41577-020-00454-2
21. Zhang S, Zhang X, Wang K, Xu X, Li M, Zhang J, et al. Newly generated CD4(+) T cells acquire metabolic quiescence after thymic egress. J Immunol (2018) 200(3):1064–77. doi: 10.4049/jimmunol.1700721
22. Parish IA, Heath WR. Too dangerous to ignore: self-tolerance and the control of ignorant autoreactive T cells. Immunol Cell Biol (2008) 86(2):146–52. doi: 10.1038/sj.icb.7100161
23. McLane LM, Abdel-Hakeem MS, Wherry EJ. CD8 T cell exhaustion during chronic viral infection and cancer. Annu Rev Immunol (2019) 37:457–95. doi: 10.1146/annurev-immunol-041015-055318
24. Marziali M, De Santis W, Carello R, Leti W, Esposito A, Isgrò A, et al. T-Cell homeostasis alteration in HIV-1 infected subjects with low CD4 T-cell count despite undetectable virus load during HAART. AIDS (2006) 20(16):2033–41. doi: 10.1097/01.aids.0000247588.69438.fd
25. Lederman MM, Calabrese L, Funderburg NT, Clagett B, Medvik K, Bonilla H, et al. Immunologic failure despite suppressive antiretroviral therapy is related to activation and turnover of memory CD4 cells. J Infect Dis (2011) 204(8):1217–26. doi: 10.1093/infdis/jir507
26. Méndez-Lagares G, García-Pergañeda A, del Mar del Pozo-Balado M, Genebat M, Ruiz-Mateos E, García García M, et al. Differential alterations of the CD4 and CD8 T cell subsets in HIV-infected patients on highly active antiretroviral therapy with low CD4 T cell restoration. J Antimicrob Chemother (2012) 67(5):1228–37. doi: 10.1093/jac/dkr594
27. Zhao J, Schank M, Wang L, Li Z, Nguyen LN, Dang X, et al. Mitochondrial functions are compromised in CD4 T cells from ART-controlled PLHIV. Front Immunol (2021) 12:658420. doi: 10.3389/fimmu.2021.658420
28. Corbeau P, Reynes J. Immune reconstitution under antiretroviral therapy: the new challenge in HIV-1 infection. Blood (2011) 117(21):5582–90. doi: 10.1182/blood-2010-12-322453
29. Zakhour R, Tran DQ, Degaffe G, Bell CS, Donnachie E, Zhang W, et al. Recent thymus emigrant CD4+ T cells predict HIV disease progression in patients with perinatally acquired HIV. Clin Infect Dis (2016) 62(8):1029–35. doi: 10.1093/cid/ciw030
30. Ferrando-Martinez S, De Pablo-Bernal RS, De Luna-Romero M, De Ory SJ, Genebat M, Pacheco YM, et al. Thymic function failure is associated with human immunodeficiency virus disease progression. Clin Infect Dis (2017) 64(9):1191–7. doi: 10.1093/cid/cix095
31. Li T, Wu N, Dai Y, Qiu Z, Han Y, Xie J, et al. Reduced thymic output is a major mechanism of immune reconstitution failure in HIV-infected patients after long-term antiretroviral therapy. Clin Infect Dis (2011) 53(9):944–51. doi: 10.1093/cid/cir552
32. Rb-Silva R, Nobrega C, Azevedo C, Athayde E, Canto-Gomes J, Ferreira I, et al. Thymic function as a predictor of immune recovery in chronically HIV-infected patients initiating antiretroviral therapy. Front Immunol (2019) 10:25. doi: 10.3389/fimmu.2019.00025
33. Xiao Q, Yan L, Han J, Yang S, Tang Y, Li Q, et al. Metabolism-dependent ferroptosis promotes mitochondrial dysfunction and inflammation in CD4(+) T lymphocytes in HIV-infected immune non-responders. EBioMedicine (2022) 86:104382. doi: 10.1016/j.ebiom.2022.104382
34. Younes SA. Mitochondrial exhaustion of memory CD4 T-cells in treated HIV-1 infection. Immunometabolism (2022) 4(2):e220013. doi: 10.20900/immunometab20220013
35. Ferrari B, Da Silva AC, Liu KH, Saidakova EV, Korolevskaya LB, Shmagel KV, et al. Gut-derived bacterial toxins impair memory CD4+ T cell mitochondrial function in HIV-1 infection. J Clin Invest (2022) 132(9):e149571. doi: 10.1172/jci149571
36. Vlasova VV, Saidakova EV, Korolevskaya LB, Shmagel NG, Chereshnev VA, Shmagel KV. Metabolic features of activated memory CD4(+) T-cells derived from HIV-infected immunological non-responders to highly active antiretroviral therapy. Dokl Biol Sci (2021) 501(1):206–9. doi: 10.1134/s0012496621060090
37. Negredo E, Massanella M, Puig J, Pérez-Alvarez N, Gallego-Escuredo JM, Villarroya J, et al. Nadir CD4 T cell count as predictor and high CD4 T cell intrinsic apoptosis as final mechanism of poor CD4 T cell recovery in virologically suppressed HIV-infected patients: clinical implications. Clin Infect Dis (2010) 50(9):1300–8. doi: 10.1086/651689
38. Molina-Pinelo S, Vallejo A, Díaz L, Soriano-Sarabia N, Ferrando-Martínez S, Resino S, et al. Premature immunosenescence in HIV-infected patients on highly active antiretroviral therapy with low-level CD4 T cell repopulation. J Antimicrob Chemother (2009) 64(3):579–88. doi: 10.1093/jac/dkp248
39. Hansjee N, Kaufmann GR, Strub C, Weber R, Battegay M, Erb P. Persistent apoptosis in HIV-1-infected individuals receiving potent antiretroviral therapy is associated with poor recovery of CD4 T lymphocytes. J Acquir Immune Defic Syndr (2004) 36(2):671–7. doi: 10.1097/00126334-200406010-00003
40. Carvalho-Silva WHV, Andrade-Santos JL, Souto FO, Coelho AVC, Crovella S, Guimarães RL. Immunological recovery failure in cART-treated HIV-positive patients is associated with reduced thymic output and RTE CD4+ T cell death by pyroptosis. J Leukoc Biol (2020) 107(1):85–94. doi: 10.1002/jlb.4a0919-235r
41. Broz P, Dixit VM. Inflammasomes: mechanism of assembly, regulation and signalling. Nat Rev Immunol (2016) 16(7):407–20. doi: 10.1038/nri.2016.58
42. Bandera A, Masetti M, Fabbiani M, Biasin M, Muscatello A, Squillace N, et al. The NLRP3 inflammasome is upregulated in HIV-infected antiretroviral therapy-treated individuals with defective immune recovery. Front Immunol (2018) 9:214. doi: 10.3389/fimmu.2018.00214
43. Lao X, Mei X, Zou J, Xiao Q, Ning Q, Xu X, et al. Pyroptosis associated with immune reconstruction failure in HIV-1- infected patients receiving antiretroviral therapy: a cross-sectional study. BMC Infect Dis (2022) 22(1):867. doi: 10.1186/s12879-022-07818-0
44. Zhang C, Song JW, Huang HH, Fan X, Huang L, Deng JN, et al. NLRP3 inflammasome induces CD4+ T cell loss in chronically HIV-1-infected patients. J Clin Invest (2021) 131(6):e138861. doi: 10.1172/jci138861
45. Robbins GK, Spritzler JG, Chan ES, Asmuth DM, Gandhi RT, Rodriguez BA, et al. Incomplete reconstitution of T cell subsets on combination antiretroviral therapy in the AIDS clinical trials group protocol 384. Clin Infect Dis (2009) 48(3):350–61. doi: 10.1086/595888
46. Massanella M, Negredo E, Pérez-Alvarez N, Puig J, Ruiz-Hernández R, Bofill M, et al. CD4 T-cell hyperactivation and susceptibility to cell death determine poor CD4 T-cell recovery during suppressive HAART. AIDS (2010) 24(7):959–68. doi: 10.1097/QAD.0b013e328337b957
47. Massanella M, Gómez-Mora E, Carrillo J, Curriu M, Ouchi D, Puig J, et al. Increased ex vivo cell death of central memory CD4 T cells in treated HIV infected individuals with unsatisfactory immune recovery. J Transl Med (2015) 13:230. doi: 10.1186/s12967-015-0601-2
48. Helleberg M, Kronborg G, Ullum H, Ryder LP, Obel N, Gerstoft J. Course and clinical significance of CD8+ T-cell counts in a large cohort of HIV-infected individuals. J Infect Dis (2015) 211(11):1726–34. doi: 10.1093/infdis/jiu669
49. Saison J, Ferry T, Demaret J, Maucort Boulch D, Venet F, Perpoint T, et al. Association between discordant immunological response to highly active anti-retroviral therapy, regulatory T cell percentage, immune cell activation and very low-level viraemia in HIV-infected patients. Clin Exp Immunol (2014) 176(3):401–9. doi: 10.1111/cei.12278
50. Piconi S, Trabattoni D, Gori A, Parisotto S, Magni C, Meraviglia P, et al. Immune activation, apoptosis, and treg activity are associated with persistently reduced CD4+ T-cell counts during antiretroviral therapy. AIDS (2010) 24(13):1991–2000. doi: 10.1097/QAD.0b013e32833c93ce
51. Méndez-Lagares G, Pozo-Balado MM, Genebat M, García Pergañeda A, Leal M, Pacheco YM. Severe immune dysregulation affects CD4+CD25(hi)foxp3+ regulatory T cells in HIV-infected patients with low-level CD4 T-cell repopulation despite suppressive highly active antiretroviral therapy. J Infect Dis (2012) 205(10):1501–9. doi: 10.1093/infdis/jis230
52. Suy F, Botelho-Nevers E, Gagneux-Brunon A, Frésard A, Paul S, Lambert C, et al. Immunologic nonresponders and T-regulatory cells in HIV-1 infection. AIDS (2013) 27(18):2968–71. doi: 10.1097/qad.0000000000000022
53. Horta A, Nobrega C, Amorim-Machado P, Coutinho-Teixeira V, Barreira-Silva P, Boavida S, et al. Poor immune reconstitution in HIV-infected patients associates with high percentage of regulatory CD4+ T cells. PloS One (2013) 8(2):e57336. doi: 10.1371/journal.pone.0057336
54. Liang Q, Jiao Y, Zhang T, Wang R, Li W, Zhang H, et al. Double negative (DN) [CD3+CD4-CD8-] T cells correlate with disease progression during HIV infection. Immunol Invest (2013) 42(5):431–7. doi: 10.3109/08820139.2013.805763
55. Lu X, Su B, Xia H, Zhang X, Liu Z, Ji Y, et al. Low double-negative CD3(+)CD4(-)CD8(-) T cells are associated with incomplete restoration of CD4(+) T cells and higher immune activation in HIV-1 immunological non-responders. Front Immunol (2016) 7:579. doi: 10.3389/fimmu.2016.00579
56. Marchetti G, Gori A, Casabianca A, Magnani M, Franzetti F, Clerici M, et al. Comparative analysis of T-cell turnover and homeostatic parameters in HIV-infected patients with discordant immune-virological responses to HAART. AIDS (2006) 20(13):1727–36. doi: 10.1097/01.aids.0000242819.72839.db
57. Shive CL, Mudd JC, Funderburg NT, Sieg SF, Kyi B, Bazdar DA, et al. Inflammatory cytokines drive CD4+ T-cell cycling and impaired responsiveness to interleukin 7: implications for immune failure in HIV disease. J Infect Dis (2014) 210(4):619–29. doi: 10.1093/infdis/jiu125
58. Younes SA, Talla A, Pereira Ribeiro S, Saidakova EV, Korolevskaya LB, Shmagel KV, et al. Cycling CD4+ T cells in HIV-infected immune nonresponders have mitochondrial dysfunction. J Clin Invest (2018) 128(11):5083–94. doi: 10.1172/jci120245
59. Lichtfuss GF, Cheng WJ, Farsakoglu Y, Paukovics G, Rajasuriar R, Velayudham P, et al. Virologically suppressed HIV patients show activation of NK cells and persistent innate immune activation. J Immunol (2012) 189(3):1491–9. doi: 10.4049/jimmunol.1200458
60. Zicari S, Sessa L, Cotugno N, Ruggiero A, Morrocchi E, Concato C, et al. Immune activation, inflammation, and non-AIDS co-morbidities in HIV-infected patients under long-term ART. Viruses (2019) 11(3):200. doi: 10.3390/v11030200
61. Lv T, Cao W, Li T. HIV-Related immune activation and inflammation: current understanding and strategies. J Immunol Res (2021) 2021:7316456. doi: 10.1155/2021/7316456
62. Martinez-Picado J, Deeks SG. Persistent HIV-1 replication during antiretroviral therapy. Curr Opin HIV AIDS (2016) 11(4):417–23. doi: 10.1097/coh.0000000000000287
63. Margolick JB, Bream JH, Nilles TL, Li H, Langan SJ, Deng S, et al. Relationship between T-cell responses to CMV, markers of inflammation, and frailty in HIV-uninfected and HIV-infected men in the multicenter AIDS cohort study. J Infect Dis (2018) 218(2):249–58. doi: 10.1093/infdis/jiy005
64. Gobran ST, Ancuta P, Shoukry NH. A tale of two viruses: immunological insights into HCV/HIV coinfection. Front Immunol (2021) 12:726419. doi: 10.3389/fimmu.2021.726419
65. Brenchley JM, Price DA, Schacker TW, Asher TE, Silvestri G, Rao S, et al. Microbial translocation is a cause of systemic immune activation in chronic HIV infection. Nat Med (2006) 12(12):1365–71. doi: 10.1038/nm1511
66. Somsouk M, Estes JD, Deleage C, Dunham RM, Albright R, Inadomi JM, et al. Gut epithelial barrier and systemic inflammation during chronic HIV infection. AIDS (2015) 29(1):43–51. doi: 10.1097/qad.0000000000000511
67. Gootenberg DB, Paer JM, Luevano JM, Kwon DS. HIV-Associated changes in the enteric microbial community: potential role in loss of homeostasis and development of systemic inflammation. Curr Opin Infect Dis (2017) 30(1):31–43. doi: 10.1097/qco.0000000000000341
68. Zhang LX, Song JW, Zhang C, Fan X, Huang HH, Xu RN, et al. Dynamics of HIV reservoir decay and naïve CD4 T-cell recovery between immune non-responders and complete responders on long-term antiretroviral treatment. Clin Immunol (2021) 229:108773. doi: 10.1016/j.clim.2021.108773
69. Hatano H, Jain V, Hunt PW, Lee TH, Sinclair E, Do TD, et al. Cell-based measures of viral persistence are associated with immune activation and programmed cell death protein 1 (PD-1)-expressing CD4+ T cells. J Infect Dis (2013) 208(1):50–6. doi: 10.1093/infdis/jis630
70. Scherpenisse M, Kootstra NA, Bakker M, Berkhout B, Pasternak AO. Cell-associated HIV-1 unspliced-to-multiply-spliced RNA ratio at 12 weeks of ART predicts immune reconstitution on therapy. mBio (2021) 12(2):e00099-21. doi: 10.1128/mBio.00099-21
71. Norris PJ, Zhang J, Worlock A, Nair SV, Anastos K, Minkoff HL, et al. Systemic cytokine levels do not predict CD4(+) T-cell recovery after suppressive combination antiretroviral therapy in chronic human immunodeficiency virus infection. Open Forum Infect Dis (2016) 3(1):ofw025. doi: 10.1093/ofid/ofw025
72. Massanella M, Negredo E, Puig J, Puertas MC, Buzón MJ, Pérez-Álvarez N, et al. Raltegravir intensification shows differing effects on CD8 and CD4 T cells in HIV-infected HAART-suppressed individuals with poor CD4 T-cell recovery. AIDS (2012) 26(18):2285–93. doi: 10.1097/QAD.0b013e328359f20f
73. Tincati C, Mondatore D, Bai F, d'Arminio Monforte A, Marchetti G. Do combination antiretroviral therapy regimens for HIV infection feature diverse T-cell phenotypes and inflammatory profiles? Open Forum Infect Dis (2020) 7(9):ofaa340. doi: 10.1093/ofid/ofaa340
74. Prendergast AJ, Szubert AJ, Pimundu G, Berejena C, Pala P, Shonhai A, et al. The impact of viraemia on inflammatory biomarkers and CD4+ cell subpopulations in HIV-infected children in sub-saharan Africa. AIDS (2021) 35(10):1537–48. doi: 10.1097/qad.0000000000002916
75. Jiang W, Lederman MM, Hunt P, Sieg SF, Haley K, Rodriguez B, et al. Plasma levels of bacterial DNA correlate with immune activation and the magnitude of immune restoration in persons with antiretroviral-treated HIV infection. J Infect Dis (2009) 199(8):1177–85. doi: 10.1086/597476
76. Ji Y, Zhang F, Zhang R, Shen Y, Liu L, Wang J, et al. Changes in intestinal microbiota in HIV-1-infected subjects following cART initiation: influence of CD4+ T cell count. Emerg Microbes Infect (2018) 7(1):113. doi: 10.1038/s41426-018-0117-y
77. Nowak P, Troseid M, Avershina E, Barqasho B, Neogi U, Holm K, et al. Gut microbiota diversity predicts immune status in HIV-1 infection. AIDS (2015) 29(18):2409–18. doi: 10.1097/qad.0000000000000869
78. Lee SC, Chua LL, Yap SH, Khang TF, Leng CY, Raja Azwa RI, et al. Enrichment of gut-derived fusobacterium is associated with suboptimal immune recovery in HIV-infected individuals. Sci Rep (2018) 8(1):14277. doi: 10.1038/s41598-018-32585-x
79. Lu D, Zhang JB, Wang YX, Geng ST, Zhang Z, Xu Y, et al. Association between CD4(+) T cell counts and gut microbiota and serum cytokines levels in HIV-infected immunological non-responders. BMC Infect Dis (2021) 21(1):742. doi: 10.1186/s12879-021-06491-z
80. Lu W, Feng Y, Jing F, Han Y, Lyu N, Liu F, et al. Association between gut microbiota and CD4 recovery in HIV-1 infected patients. Front Microbiol (2018) 9:1451. doi: 10.3389/fmicb.2018.01451
82. Day CL, Kaufmann DE, Kiepiela P, Brown JA, Moodley ES, Reddy S, et al. PD-1 expression on HIV-specific T cells is associated with T-cell exhaustion and disease progression. Nature (2006) 443(7109):350–4. doi: 10.1038/nature05115
83. Fenwick C, Joo V, Jacquier P, Noto A, Banga R, Perreau M, et al. T-Cell exhaustion in HIV infection. Immunol Rev (2019) 292(1):149–63. doi: 10.1111/imr.12823
84. Wang S, Zhang Q, Hui H, Agrawal K, Karris MAY, Rana TM. An atlas of immune cell exhaustion in HIV-infected individuals revealed by single-cell transcriptomics. Emerg Microbes Infect (2020) 9(1):2333–47. doi: 10.1080/22221751.2020.1826361
85. Noyan K, Nguyen S, Betts MR, Sönnerborg A, Buggert M. Human immunodeficiency virus type-1 elite controllers maintain low co-expression of inhibitory receptors on CD4+ T cells. Front Immunol (2018) 9:19. doi: 10.3389/fimmu.2018.00019
86. Chen H, Moussa M, Catalfamo M. The role of immunomodulatory receptors in the pathogenesis of HIV infection: a therapeutic opportunity for HIV cure? Front Immunol (2020) 11:1223. doi: 10.3389/fimmu.2020.01223
87. Kaufmann DE, Kavanagh DG, Pereyra F, Zaunders JJ, Mackey EW, Miura T, et al. Upregulation of CTLA-4 by HIV-specific CD4+ T cells correlates with disease progression and defines a reversible immune dysfunction. Nat Immunol (2007) 8(11):1246–54. doi: 10.1038/ni1515
88. Porichis F, Kwon DS, Zupkosky J, Tighe DP, McMullen A, Brockman MA, et al. Responsiveness of HIV-specific CD4 T cells to PD-1 blockade. Blood (2011) 118(4):965–74. doi: 10.1182/blood-2010-12-328070
89. Banga R, Procopio FA, Noto A, Pollakis G, Cavassini M, Ohmiti K, et al. PD-1(+) and follicular helper T cells are responsible for persistent HIV-1 transcription in treated aviremic individuals. Nat Med (2016) 22(7):754–61. doi: 10.1038/nm.4113
90. McGary CS, Deleage C, Harper J, Micci L, Ribeiro SP, Paganini S, et al. CTLA-4(+)PD-1(-) memory CD4(+) T cells critically contribute to viral persistence in antiretroviral therapy-suppressed, SIV-infected rhesus macaques. Immunity (2017) 47(4):776–88.e5. doi: 10.1016/j.immuni.2017.09.018
91. Tian X, Zhang A, Qiu C, Wang W, Yang Y, Qiu C, et al. The upregulation of LAG-3 on T cells defines a subpopulation with functional exhaustion and correlates with disease progression in HIV-infected subjects. J Immunol (2015) 194(8):3873–82. doi: 10.4049/jimmunol.1402176
92. Cockerham LR, Jain V, Sinclair E, Glidden DV, Hartogenesis W, Hatano H, et al. Programmed death-1 expression on CD4+ and CD8+ T cells in treated and untreated HIV disease. AIDS (2014) 28(12):1749–58. doi: 10.1097/qad.0000000000000314
93. Grabmeier-Pfistershammer K, Steinberger P, Rieger A, Leitner J, Kohrgruber N. Identification of PD-1 as a unique marker for failing immune reconstitution in HIV-1-infected patients on treatment. J Acquir Immune Defic Syndr (2011) 56(2):118–24. doi: 10.1097/QAI.0b013e3181fbab9f
94. Nakanjako D, Ssewanyana I, Mayanja-Kizza H, Kiragga A, Colebunders R, Manabe YC, et al. High T-cell immune activation and immune exhaustion among individuals with suboptimal CD4 recovery after 4 years of antiretroviral therapy in an African cohort. BMC Infect Dis (2011) 11:43. doi: 10.1186/1471-2334-11-43
95. Antoine P, Olislagers V, Huygens A, Lecomte S, Liesnard C, Donner C, et al. Functional exhaustion of CD4+ T lymphocytes during primary cytomegalovirus infection. J Immunol (2012) 189(5):2665–72. doi: 10.4049/jimmunol.1101165
96. Crawford A, Angelosanto JM, Kao C, Doering TA, Odorizzi PM, Barnett BE, et al. Molecular and transcriptional basis of CD4+ T cell dysfunction during chronic infection. Immunity (2014) 40(2):289–302. doi: 10.1016/j.immuni.2014.01.005
97. Ji Y, Dang X, Nguyen LNT, Nguyen LN, Zhao J, Cao D, et al. Topological DNA damage, telomere attrition and T cell senescence during chronic viral infections. Immun Ageing (2019) 16:12. doi: 10.1186/s12979-019-0153-z
98. Cobos Jiménez V, Wit FW, Joerink M, Maurer I, Harskamp AM, Schouten J, et al. T-Cell activation independently associates with immune senescence in HIV-infected recipients of long-term antiretroviral treatment. J Infect Dis (2016) 214(2):216–25. doi: 10.1093/infdis/jiw146
99. Deeks SG, Verdin E, McCune JM. Immunosenescence and HIV. Curr Opin Immunol (2012) 24(4):501–6. doi: 10.1016/j.coi.2012.05.004
100. Sokoya T, Steel HC, Nieuwoudt M, Rossouw TM. HIV As a cause of immune activation and immunosenescence. Mediators Inflammation (2017) 2017:6825493. doi: 10.1155/2017/6825493
101. Gratz IK, Rosenblum MD, Abbas AK. The life of regulatory T cells. Ann N Y Acad Sci (2013) 1283:8–12. doi: 10.1111/nyas.12011
102. Schumacher A, Zenclussen AC. Regulatory T cells: regulators of life. Am J Reprod Immunol (2014) 72(2):158–70. doi: 10.1111/aji.12238
103. Sakaguchi S, Yamaguchi T, Nomura T, Ono M. Regulatory T cells and immune tolerance. Cell (2008) 133(5):775–87. doi: 10.1016/j.cell.2008.05.009
104. Shevach EM. Mechanisms of foxp3+ T regulatory cell-mediated suppression. Immunity (2009) 30(5):636–45. doi: 10.1016/j.immuni.2009.04.010
105. Sakaguchi S, Miyara M, Costantino CM, Hafler DA. Foxp3+ regulatory T cells in the human immune system. Nat Rev Immunol (2010) 10(7):490–500. doi: 10.1038/nri2785
106. Card CM, McLaren PJ, Wachihi C, Kimani J, Plummer FA, Fowke KR. Decreased immune activation in resistance to HIV-1 infection is associated with an elevated frequency of CD4(+)CD25(+)foxp3(+) regulatory T cells. J Infect Dis (2009) 199(9):1318–22. doi: 10.1086/597801
107. Chevalier MF, Weiss L. The split personality of regulatory T cells in HIV infection. Blood (2013) 121(1):29–37. doi: 10.1182/blood-2012-07-409755
108. Weiss L, Donkova-Petrini V, Caccavelli L, Balbo M, Carbonneil C, Levy Y. Human immunodeficiency virus-driven expansion of CD4+CD25+ regulatory T cells, which suppress HIV-specific CD4 T-cell responses in HIV-infected patients. Blood (2004) 104(10):3249–56. doi: 10.1182/blood-2004-01-0365
109. Kinter A, McNally J, Riggin L, Jackson R, Roby G, Fauci AS. Suppression of HIV-specific T cell activity by lymph node CD25+ regulatory T cells from HIV-infected individuals. Proc Natl Acad Sci U.S.A. (2007) 104(9):3390–5. doi: 10.1073/pnas.0611423104
110. Estes JD, Wietgrefe S, Schacker T, Southern P, Beilman G, Reilly C, et al. Simian immunodeficiency virus-induced lymphatic tissue fibrosis is mediated by transforming growth factor beta 1-positive regulatory T cells and begins in early infection. J Infect Dis (2007) 195(4):551–61. doi: 10.1086/510852
111. Shaw JM, Hunt PW, Critchfield JW, McConnell DH, Garcia JC, Pollard RB, et al. Increased frequency of regulatory T cells accompanies increased immune activation in rectal mucosae of HIV-positive noncontrollers. J Virol (2011) 85(21):11422–34. doi: 10.1128/jvi.05608-11
112. Saison J, Maucort Boulch D, Chidiac C, Demaret J, Malcus C, Cotte L, et al. Increased regulatory T-cell percentage contributes to poor CD4(+) lymphocytes recovery: a 2-year prospective study after introduction of antiretroviral therapy. Open Forum Infect Dis (2015) 2(2):ofv063. doi: 10.1093/ofid/ofv063
113. Nobrega C, Horta A, Coutinho-Teixeira V, Martins-Ribeiro A, Baldaia A, Rb-Silva R, et al. Longitudinal evaluation of regulatory T-cell dynamics on HIV-infected individuals during the first 2 years of therapy. AIDS (2016) 30(8):1175–86. doi: 10.1097/qad.0000000000001074
114. Deng K, Pertea M, Rongvaux A, Wang L, Durand CM, Ghiaur G, et al. Broad CTL response is required to clear latent HIV-1 due to dominance of escape mutations. Nature (2015) 517(7534):381–5. doi: 10.1038/nature14053
115. Cartwright EK, Spicer L, Smith SA, Lee D, Fast R, Paganini S, et al. CD8(+) lymphocytes are required for maintaining viral suppression in SIV-infected macaques treated with short-term antiretroviral therapy. Immunity (2016) 45(3):656–68. doi: 10.1016/j.immuni.2016.08.018
116. Fan J, Liang H, Ji X, Wang S, Xue J, Li D, et al. CTL-mediated immunotherapy can suppress SHIV rebound in ART-free macaques. Nat Commun (2019) 10(1):2257. doi: 10.1038/s41467-019-09725-6
117. Zhang LX, Jiao YM, Zhang C, Song JW, Fan X, Xu RN, et al. HIV Reservoir decay and CD4 recovery associated with high CD8 counts in immune restored patients on long-term ART. Front Immunol (2020) 11:1541. doi: 10.3389/fimmu.2020.01541
118. Cao W, Mehraj V, Kaufmann DE, Li T, Routy JP. Elevation and persistence of CD8 T-cells in HIV infection: the achilles heel in the ART era. J Int AIDS Soc (2016) 19(1):20697. doi: 10.7448/ias.19.1.20697
119. Ananworanich J, Fletcher JL, Pinyakorn S, van Griensven F, Vandergeeten C, Schuetz A, et al. A novel acute HIV infection staging system based on 4th generation immunoassay. Retrovirology (2013) 10:56. doi: 10.1186/1742-4690-10-56
120. Jain V, Hartogensis W, Bacchetti P, Hunt PW, Hatano H, Sinclair E, et al. Antiretroviral therapy initiated within 6 months of HIV infection is associated with lower T-cell activation and smaller HIV reservoir size. J Infect Dis (2013) 208(8):1202–11. doi: 10.1093/infdis/jit311
121. Chen J, Xun J, Yang J, Ji Y, Liu L, Qi T, et al. Plasma indoleamine 2,3-dioxygenase activity is associated with the size of the human immunodeficiency virus reservoir in patients receiving antiretroviral therapy. Clin Infect Dis (2019) 68(8):1274–81. doi: 10.1093/cid/ciy676
122. Serrano-Villar S, Gutiérrez C, Vallejo A, Hernández-Novoa B, Díaz L, Abad Fernández M, et al. The CD4/CD8 ratio in HIV-infected subjects is independently associated with T-cell activation despite long-term viral suppression. J Infect (2013) 66(1):57–66. doi: 10.1016/j.jinf.2012.09.013
123. Owen RE, Heitman JW, Hirschkorn DF, Lanteri MC, Biswas HH, Martin JN, et al. HIV+ elite controllers have low HIV-specific T-cell activation yet maintain strong, polyfunctional T-cell responses. AIDS (2010) 24(8):1095–105. doi: 10.1097/QAD.0b013e3283377a1e
124. Hunt PW, Martin JN, Sinclair E, Bredt B, Hagos E, Lampiris H, et al. T Cell activation is associated with lower CD4+ T cell gains in human immunodeficiency virus-infected patients with sustained viral suppression during antiretroviral therapy. J Infect Dis (2003) 187(10):1534–43. doi: 10.1086/374786
125. Li J, Huang HH, Tu B, Zhou MJ, Hu W, Fu YL, et al. Reversal of the CD8(+) T-cell exhaustion induced by chronic HIV-1 infection through combined blockade of the adenosine and PD-1 pathways. Front Immunol (2021) 12:687296. doi: 10.3389/fimmu.2021.687296
126. Lee SA, Sinclair E, Hatano H, Hsue PY, Epling L, Hecht FM, et al. Impact of HIV on CD8+ T cell CD57 expression is distinct from that of CMV and aging. PloS One (2014) 9(2):e89444. doi: 10.1371/journal.pone.0089444
127. Fischer K, Voelkl S, Heymann J, Przybylski GK, Mondal K, Laumer M, et al. Isolation and characterization of human antigen-specific TCR alpha beta+ CD4(-)CD8- double-negative regulatory T cells. Blood (2005) 105(7):2828–35. doi: 10.1182/blood-2004-07-2583
128. Paul S, Shilpi, Lal G. Role of gamma-delta (γδ) T cells in autoimmunity. J Leukoc Biol (2015) 97(2):259–71. doi: 10.1189/jlb.3RU0914-443R
129. Wu Z, Zheng Y, Sheng J, Han Y, Yang Y, Pan H, et al. CD3(+)CD4(-)CD8(-) (double-negative) T cells in inflammation, immune disorders and cancer. Front Immunol (2022) 13:816005. doi: 10.3389/fimmu.2022.816005
130. Yang L, Zhu Y, Tian D, Wang S, Guo J, Sun G, et al. Transcriptome landscape of double negative T cells by single-cell RNA sequencing. J Autoimmun (2021) 121:102653. doi: 10.1016/j.jaut.2021.102653
131. Petitjean G, Chevalier MF, Tibaoui F, Didier C, Manea ME, Liovat AS, et al. Level of double negative T cells, which produce TGF-β and IL-10, predicts CD8 T-cell activation in primary HIV-1 infection. AIDS (2012) 26(2):139–48. doi: 10.1097/QAD.0b013e32834e1484
132. Vinton C, Klatt NR, Harris LD, Briant JA, Sanders-Beer BE, Herbert R, et al. CD4-like immunological function by CD4- T cells in multiple natural hosts of simian immunodeficiency virus. J Virol (2011) 85(17):8702–8. doi: 10.1128/jvi.00332-11
133. Milush JM, Mir KD, Sundaravaradan V, Gordon SN, Engram J, Cano CA, et al. Lack of clinical AIDS in SIV-infected sooty mangabeys with significant CD4+ T cell loss is associated with double-negative T cells. J Clin Invest (2011) 121(3):1102–10. doi: 10.1172/jci44876
134. Merims S, Li X, Joe B, Dokouhaki P, Han M, Childs RW, et al. Anti-leukemia effect of ex vivo expanded DNT cells from AML patients: a potential novel autologous T-cell adoptive immunotherapy. Leukemia (2011) 25(9):1415–22. doi: 10.1038/leu.2011.99
135. Fang L, Ly D, Wang SS, Lee JB, Kang H, Xu H, et al. Targeting late-stage non-small cell lung cancer with a combination of DNT cellular therapy and PD-1 checkpoint blockade. J Exp Clin Cancer Res (2019) 38(1):123. doi: 10.1186/s13046-019-1126-y
136. Sundaravaradan V, Mir KD, Sodora DL. Double-negative T cells during HIV/SIV infections: potential pinch hitters in the T-cell lineup. Curr Opin HIV AIDS (2012) 7(2):164–71. doi: 10.1097/COH.0b013e3283504a66
137. Wang X, Zhang L, Du J, Wei Y, Wang D, Song C, et al. Decreased CD73+ double-negative T cells and elevated level of soluble CD73 correlated with and predicted poor immune reconstitution in HIV-infected patients after antiretroviral therapy. Front Immunol (2022) 13:869286. doi: 10.3389/fimmu.2022.869286
138. Caligiuri MA. Human natural killer cells. Blood (2008) 112(3):461–9. doi: 10.1182/blood-2007-09-077438
139. Crinier A, Narni-Mancinelli E, Ugolini S, Vivier E. Snapshot: natural killer cells. Cell (2020) 180(6):1280–.e1. doi: 10.1016/j.cell.2020.02.029
140. Campos C, Pera A, Sanchez-Correa B, Alonso C, Lopez-Fernandez I, Morgado S, et al. Effect of age and CMV on NK cell subpopulations. Exp Gerontol (2014) 54:130–7. doi: 10.1016/j.exger.2014.01.008
141. Sun Y, Zhou J, Jiang Y. Negative regulation and protective function of natural killer cells in HIV infection: two sides of a coin. Front Immunol (2022) 13:842831. doi: 10.3389/fimmu.2022.842831
142. Bayigga L, Nabatanzi R, Sekiziyivu PN, Mayanja-Kizza H, Kamya MR, Kambugu A, et al. High CD56++CD16- natural killer (NK) cells among suboptimal immune responders after four years of suppressive antiretroviral therapy in an African adult HIV treatment cohort. BMC Immunol (2014) 15:2. doi: 10.1186/1471-2172-15-2
143. Giuliani E, Vassena L, Di Cesare S, Malagnino V, Desimio MG, Andreoni M, et al. NK cells of HIV-1-infected patients with poor CD4(+) T-cell reconstitution despite suppressive HAART show reduced IFN-γ production and high frequency of autoreactive CD56(bright) cells. Immunol Lett (2017) 190:185–93. doi: 10.1016/j.imlet.2017.08.014
144. Luo Z, Li Z, Martin L, Hu Z, Wu H, Wan Z, et al. Increased natural killer cell activation in HIV-infected immunologic non-responders correlates with CD4+ T cell recovery after antiretroviral therapy and viral suppression. PloS One (2017) 12(1):e0167640. doi: 10.1371/journal.pone.0167640
145. Zhang QY, Zhang X, Su B, Liu LF, Yang XD, Tang B, et al. Increased early activation of CD56dimCD16dim/- natural killer cells in immunological non-responders correlates with CD4+ T-cell recovery. Chin Med J (Engl) (2020) 133(24):2928–39. doi: 10.1097/cm9.0000000000001262
146. Qian F, Hu S, Zhu Y, Wang Y, Liu J, Qiao J, et al. CD56(dim) NK cell is an important factor in T cell depletion of cART-treated AIDS patients. Int J Gen Med (2022) 15:4575–83. doi: 10.2147/ijgm.S356771
147. Seifert M, Küppers R. Human memory b cells. Leukemia (2016) 30(12):2283–92. doi: 10.1038/leu.2016.226
148. Moir S, Fauci AS. B-cell responses to HIV infection. Immunol Rev (2017) 275(1):33–48. doi: 10.1111/imr.12502
149. Liu Y, Li Z, Lu X, Kuang YQ, Kong D, Zhang X, et al. Dysregulation of memory b cells and circulating T follicular helper cells is a predictor of poor immune recovery in HIV-infected patients on antiretroviral therapy. J Med Virol (2023) 95(2):e28559. doi: 10.1002/jmv.28559
150. Lu X, Zhang X, Cheung AKL, Moog C, Xia H, Li Z, et al. Abnormal shift in b memory cell profile is associated with the expansion of circulating T follicular helper cells via icos signaling during acute HIV-1 infection. Front Immunol (2022) 13:837921. doi: 10.3389/fimmu.2022.837921
151. Jia J, Zhao Y, Yang JQ, Lu DF, Zhang XL, Mao JH, et al. Naïve b cells with low differentiation improve the immune reconstitution of HIV-infected patients. iScience (2022) 25(12):105559. doi: 10.1016/j.isci.2022.105559
152. Merino KM, Allers C, Didier ES, Kuroda MJ. Role of monocyte/macrophages during HIV/SIV infection in adult and pediatric acquired immune deficiency syndrome. Front Immunol (2017) 8:1693. doi: 10.3389/fimmu.2017.01693
153. Stiksrud B, Aass HCD, Lorvik KB, Ueland T, Trøseid M, Dyrhol-Riise AM. Activated dendritic cells and monocytes in HIV immunological nonresponders: HIV-induced interferon-inducible protein-10 correlates with low future CD4+ recovery. AIDS (2019) 33(7):1117–29. doi: 10.1097/qad.0000000000002173
154. Nabatanzi R, Cose S, Joloba M, Jones SR, Nakanjako D. Effects of HIV infection and ART on phenotype and function of circulating monocytes, natural killer, and innate lymphoid cells. AIDS Res Ther (2018) 15(1):7. doi: 10.1186/s12981-018-0194-y
155. Altfeld M, Gale M Jr. Innate immunity against HIV-1 infection. Nat Immunol (2015) 16(6):554–62. doi: 10.1038/ni.3157
156. Rustagi A, Gale M Jr. Innate antiviral immune signaling, viral evasion and modulation by HIV-1. J Mol Biol (2014) 426(6):1161–77. doi: 10.1016/j.jmb.2013.12.003
157. Gao D, Wu J, Wu YT, Du F, Aroh C, Yan N, et al. Cyclic GMP-AMP synthase is an innate immune sensor of HIV and other retroviruses. Science (2013) 341(6148):903–6. doi: 10.1126/science.1240933
158. Jakobsen MR, Bak RO, Andersen A, Berg RK, Jensen SB, Tengchuan J, et al. IFI16 senses DNA forms of the lentiviral replication cycle and controls HIV-1 replication. Proc Natl Acad Sci U.S.A. (2013) 110(48):E4571–80. doi: 10.1073/pnas.1311669110
159. Shi Y, Su J, Chen R, Wei W, Yuan Z, Chen X, et al. The role of innate immunity in natural elite controllers of HIV-1 infection. Front Immunol (2022) 13:780922. doi: 10.3389/fimmu.2022.780922
160. Lin SC, Lo YC, Wu H. Helical assembly in the MyD88-IRAK4-IRAK2 complex in TLR/IL-1R signalling. Nature (2010) 465(7300):885–90. doi: 10.1038/nature09121
161. Kawai T, Akira S. The role of pattern-recognition receptors in innate immunity: update on toll-like receptors. Nat Immunol (2010) 11(5):373–84. doi: 10.1038/ni.1863
162. Ting JP, Duncan JA, Lei Y. How the noninflammasome NLRs function in the innate immune system. Science (2010) 327(5963):286–90. doi: 10.1126/science.1184004
163. Kufer TA, Sansonetti PJ. NLR functions beyond pathogen recognition. Nat Immunol (2011) 12(2):121–8. doi: 10.1038/ni.1985
164. Loo YM, Gale M Jr. Immune signaling by RIG-i-like receptors. Immunity (2011) 34(5):680–92. doi: 10.1016/j.immuni.2011.05.003
165. Yoshimura A, Ito M, Chikuma S, Akanuma T, Nakatsukasa H. Negative regulation of cytokine signaling in immunity. Cold Spring Harb Perspect Biol (2018) 10(7):a028571. doi: 10.1101/cshperspect.a028571
166. Erikstrup C, Kronborg G, Lohse N, Ostrowski SR, Gerstoft J, Ullum H. T-Cell dysfunction in HIV-1-infected patients with impaired recovery of CD4 cells despite suppression of viral replication. J Acquir Immune Defic Syndr (2010) 53(3):303–10. doi: 10.1097/QAI.0b013e3181ca3f7c
167. Huang W, August A. The signaling symphony: T cell receptor tunes cytokine-mediated T cell differentiation. J Leukoc Biol (2015) 97(3):477–85. doi: 10.1189/jlb.1RI0614-293R
168. Shaw DM, Merien F, Braakhuis A, Dulson D. T-Cells and their cytokine production: the anti-inflammatory and immunosuppressive effects of strenuous exercise. Cytokine (2018) 104:136–42. doi: 10.1016/j.cyto.2017.10.001
169. Matsuzaki G, Umemura M. Interleukin-17 family cytokines in protective immunity against infections: role of hematopoietic cell-derived and non-hematopoietic cell-derived interleukin-17s. Microbiol Immunol (2018) 62(1):1–13. doi: 10.1111/1348-0421.12560
170. Sabbatini F, Bandera A, Ferrario G, Trabattoni D, Marchetti G, Franzetti F, et al. Qualitative immune modulation by interleukin-2 (IL-2) adjuvant therapy in immunological non responder HIV-infected patients. PloS One (2010) 5(11):e14119. doi: 10.1371/journal.pone.0014119
171. Schluns KS, Kieper WC, Jameson SC, Lefrançois L. Interleukin-7 mediates the homeostasis of naïve and memory CD8 T cells. vivo. Nat Immunol (2000) 1(5):426–32. doi: 10.1038/80868
172. Lundström W, Fewkes NM, Mackall CL. IL-7 in human health and disease. Semin Immunol (2012) 24(3):218–24. doi: 10.1016/j.smim.2012.02.005
173. Isgrò A, Aiuti F, Mezzaroma I, Franchi F, Mazzone AM, Lebba F, et al. Interleukin 7 production by bone marrow-derived stromal cells in HIV-1-infected patients during highly active antiretroviral therapy. AIDS (2002) 16(16):2231–2. doi: 10.1097/00002030-200211080-00020
174. Llano A, Barretina J, Gutiérrez A, Blanco J, Cabrera C, Clotet B, et al. Interleukin-7 in plasma correlates with CD4 T-cell depletion and may be associated with emergence of syncytium-inducing variants in human immunodeficiency virus type 1-positive individuals. J Virol (2001) 75(21):10319–25. doi: 10.1128/jvi.75.21.10319-10325.2001
175. Rethi B, Fluur C, Atlas A, Krzyzowska M, Mowafi F, Grützmeier S, et al. Loss of IL-7ralpha is associated with CD4 T-cell depletion, high interleukin-7 levels and CD28 down-regulation in HIV infected patients. AIDS (2005) 19(18):2077–86. doi: 10.1097/01.aids.0000189848.75699.0f
176. Beq S, Delfraissy JF, Theze J. Interleukin-7 (IL-7): immune function, involvement in the pathogenesis of HIV infection and therapeutic potential. Eur Cytokine Netw (2004) 15(4):279–89.
177. Isgrò A, Leti W, De Santis W, Marziali M, Esposito A, Fimiani C, et al. Altered clonogenic capability and stromal cell function characterize bone marrow of HIV-infected subjects with low CD4+ T cell counts despite viral suppression during HAART. Clin Infect Dis (2008) 46(12):1902–10. doi: 10.1086/588480
178. Camargo JF, Kulkarni H, Agan BK, Gaitan AA, Beachy LA, Srinivas S, et al. Responsiveness of T cells to interleukin-7 is associated with higher CD4+ T cell counts in HIV-1-positive individuals with highly active antiretroviral therapy-induced viral load suppression. J Infect Dis (2009) 199(12):1872–82. doi: 10.1086/598858
179. Bellistrì GM, Casabianca A, Merlini E, Orlandi C, Ferrario G, Meroni L, et al. Increased bone marrow interleukin-7 (IL-7)/IL-7R levels but reduced IL-7 responsiveness in HIV-positive patients lacking CD4+ gain on antiviral therapy. PloS One (2010) 5(12):e15663. doi: 10.1371/journal.pone.0015663
180. Nguyen TP, Shukla S, Asaad R, Freeman ML, Lederman MM, Harding CV, et al. Responsiveness to IL-7 but not to IFN-α is diminished in CD4+ T cells from treated HIV infected patients who experience poor CD4+ T-cell recovery. AIDS (2016) 30(13):2033–42. doi: 10.1097/qad.0000000000001161
181. Benito JM, López M, Lozano S, González-Lahoz J, Soriano V. Down-regulation of interleukin-7 receptor (CD127) in HIV infection is associated with T cell activation and is a main factor influencing restoration of CD4(+) cells after antiretroviral therapy. J Infect Dis (2008) 198(10):1466–73. doi: 10.1086/592716
182. Guzmán-Fulgencio M, Berenguer J, Jiménez-Sousa MA, Micheloud D, García-Álvarez M, Bellón JM, et al. IL7RA polymorphisms predict the CD4+ recovery in HIV patients on cART. Eur J Clin Invest (2015) 45(11):1192–9. doi: 10.1111/eci.12539
183. Levy Y, Lacabaratz C, Weiss L, Viard JP, Goujard C, Lelièvre JD, et al. Enhanced T cell recovery in HIV-1-infected adults through IL-7 treatment. J Clin Invest (2009) 119(4):997–1007. doi: 10.1172/jci38052
184. Lévy Y, Sereti I, Tambussi G, Routy JP, Lelièvre JD, Delfraissy JF, et al. Effects of recombinant human interleukin 7 on T-cell recovery and thymic output in HIV-infected patients receiving antiretroviral therapy: results of a phase I/IIa randomized, placebo-controlled, multicenter study. Clin Infect Dis (2012) 55(2):291–300. doi: 10.1093/cid/cis383
185. Thiébaut R, Jarne A, Routy JP, Sereti I, Fischl M, Ive P, et al. Repeated cycles of recombinant human interleukin 7 in HIV-infected patients with low CD4 T-cell reconstitution on antiretroviral therapy: results of 2 phase II multicenter studies. Clin Infect Dis (2016) 62(9):1178–85. doi: 10.1093/cid/ciw065
186. Lødrup Carlsen KC, Granum B. Soluble CD14: role in atopic disease and recurrent infections, including otitis media. Curr Allergy Asthma Rep (2007) 7(6):436–43. doi: 10.1007/s11882-007-0067-2
187. Kroeze S, Wit FW, Rossouw TM, Steel HC, Kityo CM, Siwale M, et al. Plasma biomarkers of human immunodeficiency virus-related systemic inflammation and immune activation in sub-saharan Africa before and during suppressive antiretroviral therapy. J Infect Dis (2019) 220(6):1029–33. doi: 10.1093/infdis/jiz252
188. Sandler NG, Wand H, Roque A, Law M, Nason MC, Nixon DE, et al. Plasma levels of soluble CD14 independently predict mortality in HIV infection. J Infect Dis (2011) 203(6):780–90. doi: 10.1093/infdis/jiq118
189. Schnittman SR, Deitchman AN, Beck-Engeser G, Ahn H, York VA, Hartig H, et al. Abnormal levels of some biomarkers of immune activation despite very early treatment of human immunodeficiency virus. J Infect Dis (2021) 223(9):1621–30. doi: 10.1093/infdis/jiaa580
190. Krastinova E, Lecuroux C, Leroy C, Seng R, Cabie A, Rami A, et al. High soluble CD14 levels at primary HIV-1 infection predict more rapid disease progression. J Infect Dis (2015) 212(6):909–13. doi: 10.1093/infdis/jiv145
191. Dunham RM, Vujkovic-Cvijin I, Yukl SA, Broadhurst MJ, Loke P, Albright RG, et al. Discordance between peripheral and colonic markers of inflammation during suppressive ART. J Acquir Immune Defic Syndr (2014) 65(2):133–41. doi: 10.1097/01.qai.0000437172.08127.0b
192. Kroeze S, Rossouw TM, Steel HC, Wit FW, Kityo CM, Siwale M, et al. Plasma inflammatory biomarkers predict CD4+ T-cell recovery and viral rebound in HIV-1 infected africans on suppressive antiretroviral therapy. J Infect Dis (2021) 224(4):673–8. doi: 10.1093/infdis/jiaa787
193. Burdo TH, Lentz MR, Autissier P, Krishnan A, Halpern E, Letendre S, et al. Soluble CD163 made by monocyte/macrophages is a novel marker of HIV activity in early and chronic infection prior to and after anti-retroviral therapy. J Infect Dis (2011) 204(1):154–63. doi: 10.1093/infdis/jir214
194. Generoso M, Álvarez P, Kravietz A, Mwamzuka M, Marshed F, Ahmed A, et al. High soluble CD163 levels correlate with disease progression and inflammation in Kenyan children with perinatal HIV-infection. AIDS (2020) 34(1):33–8. doi: 10.1097/qad.0000000000002378
195. Srinivasa S, Fitch KV, Petrow E, Burdo TH, Williams KC, Lo J, et al. Soluble CD163 is associated with shortened telomere length in HIV-infected patients. J Acquir Immune Defic Syndr (2014) 67(4):414–8. doi: 10.1097/qai.0000000000000329
196. Fischer-Smith T, Tedaldi EM, Rappaport J. CD163/CD16 coexpression by circulating monocytes/macrophages in HIV: potential biomarkers for HIV infection and AIDS progression. AIDS Res Hum Retroviruses (2008) 24(3):417–21. doi: 10.1089/aid.2007.0193
197. Lei J, Yin X, Shang H, Jiang Y. IP-10 is highly involved in HIV infection. Cytokine (2019) 115:97–103. doi: 10.1016/j.cyto.2018.11.018
198. Ramirez LA, Arango TA, Thompson E, Naji M, Tebas P, Boyer JD. High IP-10 levels decrease T cell function in HIV-1-infected individuals on ART. J Leukoc Biol (2014) 96(6):1055–63. doi: 10.1189/jlb.3A0414-232RR
199. Wu X, Zhang LL, Yin LB, Fu YJ, Jiang YJ, Ding HB, et al. Deregulated MicroRNA-21 expression in monocytes from HIV-infected patients contributes to elevated IP-10 secretion in HIV infection. Front Immunol (2017) 8:1122. doi: 10.3389/fimmu.2017.01122
200. Mhandire K, Mlambo T, Zijenah LS, Duri K, Mateveke K, Tshabalala M, et al. Plasma IP-10 concentrations correlate positively with viraemia and inversely with CD4 counts in untreated HIV infection. Open AIDS J (2017) 11:24–31. doi: 10.2174/1874613601711010024
201. Valverde-Villegas JM, de Medeiros RM, Ellwanger JH, Santos BR, Melo MG, Almeida SEM, et al. High CXCL10/IP-10 levels are a hallmark in the clinical evolution of the HIV infection. Infect Genet Evol (2018) 57:51–8. doi: 10.1016/j.meegid.2017.11.002
202. Stiksrud B, Lorvik KB, Kvale D, Mollnes TE, Ueland PM, Trøseid M, et al. Plasma IP-10 is increased in immunological nonresponders and associated with activated regulatory T cells and persisting low CD4 counts. J Acquir Immune Defic Syndr (2016) 73(2):138–48. doi: 10.1097/qai.0000000000001080
203. Jiao Y, Zhang T, Wang R, Zhang H, Huang X, Yin J, et al. Plasma IP-10 is associated with rapid disease progression in early HIV-1 infection. Viral Immunol (2012) 25(4):333–7. doi: 10.1089/vim.2012.0011
204. Noel N, Boufassa F, Lécuroux C, Saez-Cirion A, Bourgeois C, Dunyach-Remy C, et al. Elevated IP10 levels are associated with immune activation and low CD4+ T-cell counts in HIV controller patients. AIDS (2014) 28(4):467–76. doi: 10.1097/qad.0000000000000174
205. Wang Z, Wu T, Ma M, Zhang Z, Fu Y, Liu J, et al. Elevated interferon-γ-induced protein 10 and its receptor CXCR3 impair NK cell function during HIV infection. J Leukoc Biol (2017) 102(1):163–70. doi: 10.1189/jlb.5A1016-444R
206. Rosado-Sánchez I, Jarrín I, Pozo-Balado MM, de Pablo-Bernal RS, Herrero-Fernández I, Alvarez-Ríos AI, et al. Higher levels of IL-6, CD4 turnover and treg frequency are already present before cART in HIV-infected subjects with later low CD4 recovery. Antiviral Res (2017) 142:76–82. doi: 10.1016/j.antiviral.2017.03.015
207. Theron AJ, Anderson R, Rossouw TM, Steel HC. The role of transforming growth factor beta-1 in the progression of HIV/AIDS and development of non-AIDS-defining fibrotic disorders. Front Immunol (2017) 8:1461. doi: 10.3389/fimmu.2017.01461
208. Hernández-Walias F, Ruiz-de-León MJ, Rosado-Sánchez I, Vázquez E, Leal M, Moreno S, et al. New signatures of poor CD4 cell recovery after suppressive antiretroviral therapy in HIV-1-infected individuals: involvement of miR-192, IL-6, sCD14 and miR-144. Sci Rep (2020) 10(1):2937. doi: 10.1038/s41598-020-60073-8
209. Lu J, Ma SS, Zhang WY, Duan JP. Changes in peripheral blood inflammatory factors (TNF-α and IL-6) and intestinal flora in AIDS and HIV-positive individuals. J Zhejiang Univ Sci B (2019) 20(10):793–802. doi: 10.1631/jzus.B1900075
210. Yamagiwa S, Gray JD, Hashimoto S, Horwitz DA. A role for TGF-beta in the generation and expansion of CD4+CD25+ regulatory T cells from human peripheral blood. J Immunol (2001) 166(12):7282–9. doi: 10.4049/jimmunol.166.12.7282
211. Shive CL, Freeman ML, Younes SA, Kowal CM, Canaday DH, Rodriguez B, et al. Markers of T cell exhaustion and senescence and their relationship to plasma TGF-β levels in treated HIV+ immune non-responders. Front Immunol (2021) 12:638010. doi: 10.3389/fimmu.2021.638010
212. Stacey AR, Norris PJ, Qin L, Haygreen EA, Taylor E, Heitman J, et al. Induction of a striking systemic cytokine cascade prior to peak viremia in acute human immunodeficiency virus type 1 infection, in contrast to more modest and delayed responses in acute hepatitis b and c virus infections. J Virol (2009) 83(8):3719–33. doi: 10.1128/jvi.01844-08
213. Hardy GA, Sieg S, Rodriguez B, Anthony D, Asaad R, Jiang W, et al. Interferon-α is the primary plasma type-I IFN in HIV-1 infection and correlates with immune activation and disease markers. PloS One (2013) 8(2):e56527. doi: 10.1371/journal.pone.0056527
214. Fernandez S, Tanaskovic S, Helbig K, Rajasuriar R, Kramski M, Murray JM, et al. CD4+ T-cell deficiency in HIV patients responding to antiretroviral therapy is associated with increased expression of interferon-stimulated genes in CD4+ T cells. J Infect Dis (2011) 204(12):1927–35. doi: 10.1093/infdis/jir659
215. Cheng L, Ma J, Li J, Li D, Li G, Li F, et al. Blocking type I interferon signaling enhances T cell recovery and reduces HIV-1 reservoirs. J Clin Invest (2017) 127(1):269–79. doi: 10.1172/jci90745
216. Zhen A, Rezek V, Youn C, Lam B, Chang N, Rick J, et al. Targeting type I interferon-mediated activation restores immune function in chronic HIV infection. J Clin Invest (2017) 127(1):260–8. doi: 10.1172/jci89488
217. Muthu S, Cecelia AJ, Pulimi S, Ameedha L, Srinivas CN, Solomon SS, et al. C-reactive protein in HIV-infected patients–could it be a marker of immunosuppression? Clin Chim Acta (2007) 376(1-2):246–7. doi: 10.1016/j.cca.2006.05.040
218. Ruiz-Briseño MDR, De Arcos-Jiménez JC, Ratkovich-González S, Sánchez-Reyes K, González-Hernández LA, Andrade-Villanueva JF, et al. Association of intestinal and systemic inflammatory biomarkers with immune reconstitution in HIV+ patients on ART. J Inflammation (Lond) (2020) 17:32. doi: 10.1186/s12950-020-00262-4
Keywords: human immunodeficiency virus, incomplete immune recovery, immunological nonresponders, immunocytes, soluble molecules
Citation: Yan L, Xu K, Xiao Q, Tuo L, Luo T, Wang S, Yang R, Zhang F and Yang X (2023) Cellular and molecular insights into incomplete immune recovery in HIV/AIDS patients. Front. Immunol. 14:1152951. doi: 10.3389/fimmu.2023.1152951
Received: 28 January 2023; Accepted: 18 April 2023;
Published: 02 May 2023.
Edited by:
Peter Hunt, University of California, San Francisco, United StatesReviewed by:
Souheil-Antoine Younes, Emory University, United StatesJulià Blanco, IrsiCaixa, Spain
Maura Manion, National Institute of Allergy and Infectious Diseases (NIH), United States
Scott Sieg, Case Western Reserve University, United States
Copyright © 2023 Yan, Xu, Xiao, Tuo, Luo, Wang, Yang, Zhang and Yang. This is an open-access article distributed under the terms of the Creative Commons Attribution License (CC BY). The use, distribution or reproduction in other forums is permitted, provided the original author(s) and the copyright owner(s) are credited and that the original publication in this journal is cited, in accordance with accepted academic practice. No use, distribution or reproduction is permitted which does not comply with these terms.
*Correspondence: Xingxiang Yang, 719525383@qq.com; Fujie Zhang, treatment@chinaaids.cn; Liting Yan, 112019000216@ccmu.edu.cn