- 1Animal Bioscience and Biotechnology Laboratory, United States Department of Agriculture, Beltsville Agricultural Research Center, Beltsville, MD, United States
- 2AVT Natural North America, Santa Clara, CA, United States
Both in vitro and in vivo studies were conducted to evaluate the beneficial effects of green tea extract (GT), cinnamon oil (CO), and pomegranate extract (PO) on avian coccidiosis. In experiment (EXP) 1, an in vitro culture system was used to investigate the individual effects of GT, CO, and PO on the proinflammatory cytokine response and integrity of tight junction (TJ) in chicken intestinal epithelial cells (IEC), on the differentiation of quail muscle cells and primary chicken embryonic muscle cells, and anticoccidial and antibacterial activities against Eimeria tenella sporozoites and Clostridium perfringens bacteria, respectively. In EXP 2 and 3, in vivo trials were carried out to study the dose-dependent effect of blended phytochemicals (GT, CO, PO) on coccidiosis in broiler chickens infected with E. maxima. For EXP 2, one hundred male broiler chickens (0-day-old) were allocated into the following five treatment groups: Control group for non-infected chickens (NC), Basal diet group for E. maxima-infected chickens (PC), PC group supplemented with phytochemicals at 50 (Phy 50), 100 (Phy 100), and 200 (Phy 200) mg/kg feed diets for E. maxima-infected chickens. For EXP 3, one hundred twenty male broiler chickens (0-day-old) were allocated into the following six treatment groups: NC, PC, PC supplemented with phytochemicals at 10 (Phy 10), 20 (Phy 20), 30 (Phy 30), and 100 (Phy 100) mg/kg feed for E. maxima-infected chickens. Body weights (BW) were measured on days 0, 7, 14, 20, and 22, and jejunum samples were used to measure cytokine, TJ protein, and antioxidant enzyme responses at 8 days post-infection (dpi). Fecal samples for oocyst enumeration were collected from 6 to 8 dpi. In vitro, CO and PO reduced LPS-induced IL-1β and IL-8 in IEC, respectively, and GT enhanced the gene expression of occludin in IEC. PO at 1.0 and 5.0 mg/mL exerted antimicrobial effect against E. tenella sporozoites and C. perfringens bacteria, respectively. In vivo, chickens fed a diet supplemented with phytochemicals showed enhanced BW, reduced oocyst shedding, and decreased proinflammatory cytokines following E. maxima challenge. In conclusion, the combination of GT, CO, and PO in the diet of broiler chickens infected with E. maxima induced enhanced host disease resistance including innate immunity and gut health, which contributed to improved growth and reduced disease responses. These findings provide scientific support for the development of a novel phytogenic feed additive formula that enhances the growth and intestinal health of broiler chickens infected with coccidiosis.
1 Introduction
With the increasing governmental restriction of growth-promoting antibiotics in commercial poultry production (1, 2), there is a timely need to develop research-based novel feed additives to enhance gut health and mitigate negative disease impacts to increase animal resilience in agriculture. Today, there are wide ranges of nutraceuticals available in the feed industry for agriculture animal production including acidifiers, postbiotics, prebiotics, probiotics, phytochemicals, enzymes, minerals, etc (2, 3). For avian coccidiosis, which is caused by several distinct species of Eimeria protozoan parasites with an estimated global economic cost of $ 14 billion (4) there has been much development on using plant-derived phytochemicals. These phytochemicals aim to mitigate the negative impacts of avian coccidiosis caused by several distinct species of Eimeria (5).
Plant-derived phytochemicals have been effectively used as natural bioactive compounds in agricultural animal production to improve animal health and productivity (2, 5). The main bioactive compounds of the phytochemicals also referred to as phytobiotics or phytogenics, are composed of polyphenols. Their composition and concentration are diverse and vary according to the plant, parts of the plant, geographical origin, harvesting season, environmental factors, storage conditions, and processing techniques (5). Dietary supplementation with selected phytochemicals, either as a single compound, or a mixture of phytochemicals, in young poultry, exerted beneficial effects on growth performance, intestinal immune response, intestinal barrier integrity, and altered intestinal microbiome of broiler chickens challenged with Eimeria spp (2). Not all phytochemicals show beneficial effects and there is a need to investigate the relationship between dose and health effects for each phytochemical since some showed toxicity at high dose ranges in vitro and in vivo which indicates the importance of understanding many contributing factors that will affect the efficacy of phytochemical feed additives in agricultural animal production (6).
In our previous study (Unpublished), 22 types of phytochemicals were evaluated with the novel in vitro screening assays based on immunity and cell growth-promoting activities of macrophages, intestinal cells, and muscle cells. Among them, green tea (GT), cinnamon oil (CO), and pomegranate extract (PO) showed various beneficial activities without any cell toxicity, so we further evaluated these phytochemicals as novel phytogenic feed additives for their health-promoting effects on broiler infected with Eimeria spp. The main objectives of the present study were: [1] in vitro evaluation of effects of the phytochemicals on the host innate immune response in chicken macrophages and the barrier integrity in chicken intestinal epithelial cells (IEC), on myogenic differentiation of quail muscle cells (QMC) and growth effect on primary chicken embryonic muscle cells (PMC), and antimicrobial ability against Eimeria maxima and Clostridium perfringens, and [2] the in vivo effects of dietary supplementation with the selected phytochemical mixture on growth, intestinal immunity, epithelial integrity and antioxidant enzymes in young broiler chickens infected with E. maxima.
2 Materials and methods
All animal care procedures were approved by the Beltsville Agricultural Research Center Institutional Animal Care and Use Committee (# 20-014). Supplementary Figure 1 depicts the schematic outline of the experimental design for these studies. All the phytochemicals utilized in this study were produced and provided by collaborating scientists from the AVT Natural. GT, CO, and PO were dissolved in water, sunflower oil, and DMSO, respectively, for in vitro studies. The control group, which received zero concentration of phytochemicals, was treated with only each diluent, serving as a negative control group. For the in vivo study, the blend of phytochemicals was formulated in a ratio of 1:1:1 (GT : CO:PO) as granulated form.
2.1 Experiment 1
2.1.1 Intestinal epithelial cells culture
IEC (2 × 105/mL, HD11; Micromol) were seeded in 24-well plates and maintained in the Dulbecco’s modified Eagle medium (DMEM)/F-12 (Hyclone, Logan, UT) supplemented with 10% heat-inactivated fetal bovine serum (FBS, Hyclone) and 1% penicillin (10,000 unit/mL)/streptomycin (10 mg/mL, Gibco, Grand Island, NY). The IEC was incubated at 41°C in a humidified atmosphere with 5% CO2 and 95% air. After 24 h, lipopolysaccharide (LPS; Sigma-Aldrich Inc, St. Louis, MO) at a concentration of 1.0 µg/mL and each phytochemical (CO, GT, and PO) at concentrations of 0.0, 0.5, 1.0, and 10.0 µg/mL were administrated to each well in the 24-well plates. After incubation for 18 h, lysis buffer (Qiagen, Germantown, MD) and 2-mercaptoethanol (Sigma-Aldrich) were used to harvest all cells. Total RNA was isolated from the IEC using the RNeasy Isolation Kit (Qiagen) in a QIAcube (Qiagen) for performing quantitative real-time polymerase chain reaction (qRT-PCR) analysis. All experiments were replicated independently six times.
2.1.2 Quail muscle cell culture
QMCs (2 × 105/mL) were seeded in 24-well plates as per methods described previously (7). The QMCs were maintained in Medium 199 (Hyclone) containing 10% FBS and 1% penicillin/streptomycin until cells reached 70% confluence. Media in 12 wells were replaced by Medium 199 containing 0.5% FBS with 1% penicillin/streptomycin to induce cell differentiation, and in the remaining 12 wells of the same plate, media were replaced by a basic Medium 199 containing 10% FBS to maintain cell proliferation. Each phytochemical (CO, GT, and PO) at concentrations of 0.0, 0.5, 1.0, and 10.0 µg/mL was administrated to each well in the 24-well plates. After incubation at 41°C in a humidified atmosphere with 5% CO2 for 18 h, all cells were collected in lysis buffer and 2-mercaptoethanol. RNA from QMC was isolated using the RNeasy Isolation Kit in the QIAcube for performing qRT-PCR analysis. All experiments were replicated more than six times independently.
2.1.3 Primary chicken embryonic muscle cell culture
Fertilized eggs for the embryonic muscle cell culture were obtained from Moyer’s hatchery (Quakertown, PA). The PMC culture was modified based on the method described by (8). In brief, the eggs were incubated using an automated incubator (GQF 1500 professional, Savannah, GA) set at a temperature of 41°C and a humidity level of 70%. The pectoralis major region of the embryos was extracted at 13 days; it was minced and digested with 0.05% trypsin-EDTA (Sigma-Aldrich) at 37°C for 20 min. The PMCs were washed 2–3 times with Hanks balanced salt solution (Sigma-Aldrich) and seeded (2 × 105/mL) in 24-well plates. The PMCs were maintained in DMEM (Hyclone) containing 10% FBS and 1% penicillin/streptomycin until visual confirmation of 70% confluence was attained. Culture media in 12 wells were replaced as DMEM containing 2% FBS with 1% penicillin/streptomycin to induce cell differentiation, and in the remaining 12 wells of the same plate, media were replaced by basic DMEM containing 10% FBS to maintain cell proliferation. Each phytochemical (CO, GT, and PO) at concentrations of 0.0, 0.5, 1.0, and 10.0 µg/mL was added to each well in the 24-well plates. After incubation at 41°C in a humidified atmosphere with 5% CO2 for 18 h, all cells were collected in lysis buffer and 2-mercaptoethanol. Total RNA from the PMC was extracted using the RNeasy Isolation Kit in the QIAcube or performing qRT-PCR analysis. RNA was eluted in 30 μL RNase-free water. All experiments were replicated independently more than six times.
2.1.4 cDNA synthesis
The quantity of RNA was evaluated using the NanoDrop spectrophotometer (Thermo Scientific, Waltham, MA) according to the absorbance at 260 nm wavelength. RNA purity was accepted based on the OD260/OD280 ratio of 1.8 and 2.0. 1 ug of total RNA was then reverse-transcribed to cDNA using the QuantiTect reverse transcription kit (Qiagen). The cDNA samples were aliquoted into cryotubes and were stored at - 20°C.
2.1.5 Analysis of cytokines, tight junction proteins, and markers of muscle cell growth by qRT-PCR
The stored cDNA samples were used to measure the gene expression of cytokines (IL-1β and IL-8) and tight junction (TJ) proteins (occluding and ZO-1) on IEC and growth markers (Pax7 and MyoG) on QMCs and PMCs. qRT-PCR was performed using Applied Biosystems QuantStudio 3 Real-Time PCR Systems (Life Technologies, Carlsbad, CA) and SYBR Green qPCR Master Mix (PowerTrack, Applied Biosystems, Foster City, CA). Oligonucleotide primer sequences and product size used for qRT-PCR are listed in Table 1. A melting curve was obtained at the end of each run to verify the presence of a single amplification product without primer dimers. Standard curves were generated using serial, 5-fold dilutions of cDNA. The fold changes in each transcript were normalized to glyceraldehyde-3-phosphate dehydrogenase and are relative to the transcript expression in the unstimulated control group (normalized to 1) using the comparative ΔΔ Ct method as previously described (9).
2.1.6 Anticoccidial assay against E. tenella
The anticoccidial effect of GT, CO, and PO on the sporozoite of E. tenella was tested using the sporozoite killing assay as described by (10). Briefly, sporulated oocysts of E. tenella were prepared and the oocyst shells were disrupted with 0.5-mm glass beads for 10 s using the Mini-Beadbeater (BioSpec Products, Bartlesville, OK). The released sporocysts were washed in chilled Hanks’ balanced salt solution (Hyclone, Logan, UT) and treated with excystation media (0.25% trypsin and 0.014 M taurocholic acid, pH 7.4) for 1 h to induce the release of sporozoites. The sporozoites (2.5 × 105) in each well of 96-well plate were treated with three different novel phytochemicals (GT, CO, and PO). Two different phytochemical doses (0.1 and 1.0 mg/mL) were tested against freshly prepared live sporozoites and incubated at 41°C for 3 hr. AO/PI staining solution (Nexcelom Bioscience LLC, Lawrence, MA) was then added to each mixture at a 1:1 ratio and live sporozoites were counted with a cell counting chamber (Cellometer, Nexcelom Bioscience LLC).
2.1.7 Antibacterial assay against C. perfringens
Clostridium perfringens (1 × 109 CFU/mL) were treated with four phytochemicals in brain-heart infusion broth (BD Difco, Sparks, MD). Three concentrations of the phytochemicals (0.5, 1.0, and 5.0 mg/mL) were added to the broth and it was incubated at 41°C for 18 hr under anaerobic conditions. After incubation, 100 µL of the cell suspension was spread on differential reinforced Clostridial agar (BD Difco) plates and the plates were incubated at 41°C for 18 hr under anaerobic conditions. Colonies of growing bacteria on the plates were counted to determine the survival ratios (%) of C. perfringens within each treatment group.
2.2 Experiment 2
2.2.1 Chickens and experimental design
A total of one hundred newly hatched male broiler chickens (Ross 708) at zero-day old (Longenecker’s hatchery, Elizabethtown, PA) were weighed and allocated to five dietary treatments in a randomized complete block design. Each treatment group had five cages with four chickens per cage (0.65 × 0.75 m2). The dietary treatments (Supplementary Table 1) included basal diet for non-infected chickens (NC), basal diet for E. maxima infected chickens (PC), PC supplemented with a blended phytochemical mixture (Phy) at 50 (Phy 50), 100 (Phy 100), and 200 (Phy 200) mg/kg feed. Based on experiment (EXP) 1, the Phy was manufactured and provided by AVT natural products. During the experimental period, the chickens were provided with ad libitum access to experimental feed and water. The experimental design used for the study is illustrated in Supplementary Figure 1.
2.2.2 Determination of body weight
The body weights (BW) of chickens were measured on days 0, 7, 14, 20, and 22 for calculating average daily gain (ADG). Dead chickens were removed and weighed to perform adjustments for the growth data.
2.2.3 Oral administration of E. maxima
All chickens, except for the NC group, were orally inoculated with E. maxima (10,000 sporulated oocysts/chicken, Beltsville strain 41A) on day 14 as per previously described methods (1). A DNA genotyping test was performed to identify the purity of the infected E. maxima (11).
2.2.4 Collection of jejunal samples
One chicken of nearly average BW per each cage was euthanized by cervical dislocation on day 22, and a small section (2 cm) of the distal jejunum without digest was collected and stored in RNAlater® (Invitrogen, Carlsbad, CA) at -20°C until subsequent analysis.
2.2.5 Fecal oocyst shedding
From days 20 to 22 (6 to 8 days post-infection: dpi), fecal samples were collected, and the number of oocysts was counted as per protocols described previously (12) using the McMaster chamber according to the formula below:
Total oocysts/chicken = [counted oocyst × dilution factor × (fecal sample volume/counting chamber volume)]/number of chickens per cage.
2.2.6 Isolation of RNA and reverse transcription from jejunal samples
Total RNA was isolated from the jejunal samples that were stored in the RNAlater® (Invitrogen). Total RNA was extracted using TRIzol reagent (Invitrogen) followed by DNase digestion as described (13). The methods of RNA quantity, RNA purity, and cDNA production in this study were carried out as described previously (14). The cDNA samples were aliquoted into cryotubes and were stored at - 20°C.
2.2.7 Gene expression analysis by qRT-PCR from the extracted RNA
The oligonucleotide primer sequences used for qRT-PCR analysis are shown in Table 1. The expression of various cytokines (IL-1β, IL-8, IFN-γ, and TNFSF15), TJ proteins (claudin, JAM-2, occludin, and ZO-1), antioxidant enzymes (CAT, HMOX, and SOD-1) was evaluated in the jejunal samples. The gene expression analysis by qRT-PCR from the extracted RNA was carried out as described previously (15).
2.3 Experiment 3
All the methods in EXP 3, except for treatment groups and the number of jejunal samples, were conducted the same as in EXP 2.
2.3.1 Chickens and experimental design
A total of 120 newly hatched male broiler chickens (Ross 708) at zero-day old were used for EXP 3. The chickens were allocated to six dietary treatments in a randomized complete block design. Each treatment group had five cages with four chickens per cage. The dietary treatments included a basal diet without infection (NC), a basal diet with E. maxima infection (PC), PC with a blended phytochemical mixture (Phy) at 10 (Phy 10), 20 (Phy 20), 30 (Phy 30), and 100 (Phy 100) mg/kg feed. The experimental design used for the study is illustrated in Supplementary Figure 1.
2.3.2 Collection of jejunal samples
Eight chickens of nearly average BW per treatment were euthanized by cervical dislocation on day 22, and a small section (2 cm) of the distal jejunum without digest was collected and stored in RNAlater® (Invitrogen) at -20°C until subsequent analysis.
2.3.3 Statistical analysis
Each response in in-vitro was evaluated using the Proc GLM in SAS software version 9.4 (SAS Inc., Cary, NC). Data from the animal trial were analyzed using a mixed model (PROC MIXED) in SAS. Linear or quadratic effects were analyzed through orthogonal polynomial contrasts which were generated using the PROC IML procedure for non-equally spaced doses. NC was excluded in the analysis of the linear and quadratic effects after infection. If a significant value in treatment groups, linear, and quadratic existed, the mean values between treatments were compared in a pairwise manner through the PDIFF option in SAS. The results are reported as least squares mean values ± standard error of the mean. Probability values less than 0.05 were considered significantly different.
3 Results
3.1 Experiment 1:in-vitro study
3.1.1 Proinflammatory cytokine in chicken intestinal epithelial cells
Administration of LPS without any phytochemicals increased (P < 0.01) IL-1β expression level in IEC more than 7-fold compared to untreated control (Figure 1). According to the concentrations of CO administration, the IL-1β expression level was linearly reduced (P < 0.001) by the administration of CO with LPS (Figure 1A). In the case of GT, only 10.0 µg/mL dose reduced (P < 0.05) LPS-induced IL-1β expression level (Figure 1B). Administration of PO did not affect IL-1β expression levels compared to that of the LPS group without administration of PO (Figure 1C).
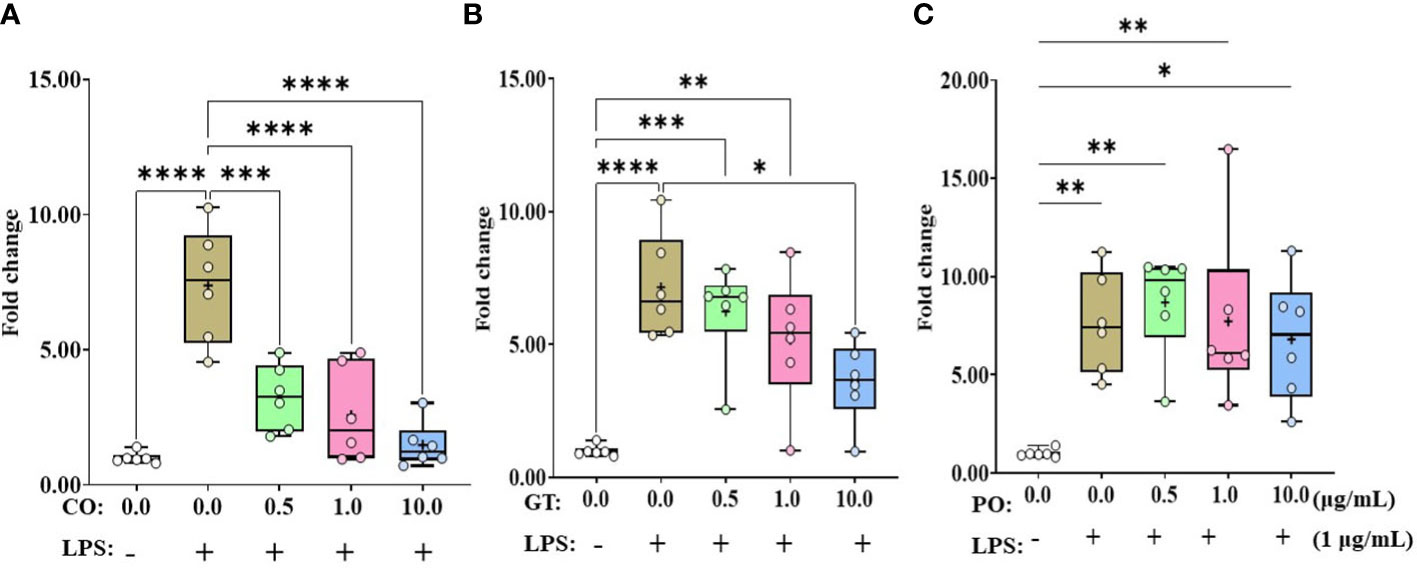
Figure 1 Gene expression of IL-1β in phytochemical-induced chicken intestinal epithelial cells (IECs). The IECs were treated with three different concentrations (0.5, 1.0, and 10.0 mg/mL) of each phytochemical [cinnamon oil (CO, A), green tea extract (GT, B), and pomegranate (PO, C)] for 18 h with LPS (1μg/mL). The data represent the average of six independent experiments. P < 0.05 (*), P < 0.01 (**), P < 0.001 (***), and P < 0.0001 (****) were considered statistically significant compared to the control (0.0 mg/mL phytochemicals). The fold changes in each transcript were normalized to glyceraldehyde-3-phosphate dehydrogenase and are relative to the transcript expression in unstimulated control group (normalized to 1) using the comparative ΔΔ Ct method.
IL-8 expression levels in IEC were elevated by more than 17-fold when administrating LPS without any phytochemicals (Figure 2). Administration of PO at 10 µg/mL suppressed (P < 0.05) the LPS-induced IL-8 expression level (Figure 2C). However, IL-8 expression levels in LPS groups were not affected (P > 0.05) by CO and GT treatment (Figures 2A, B).
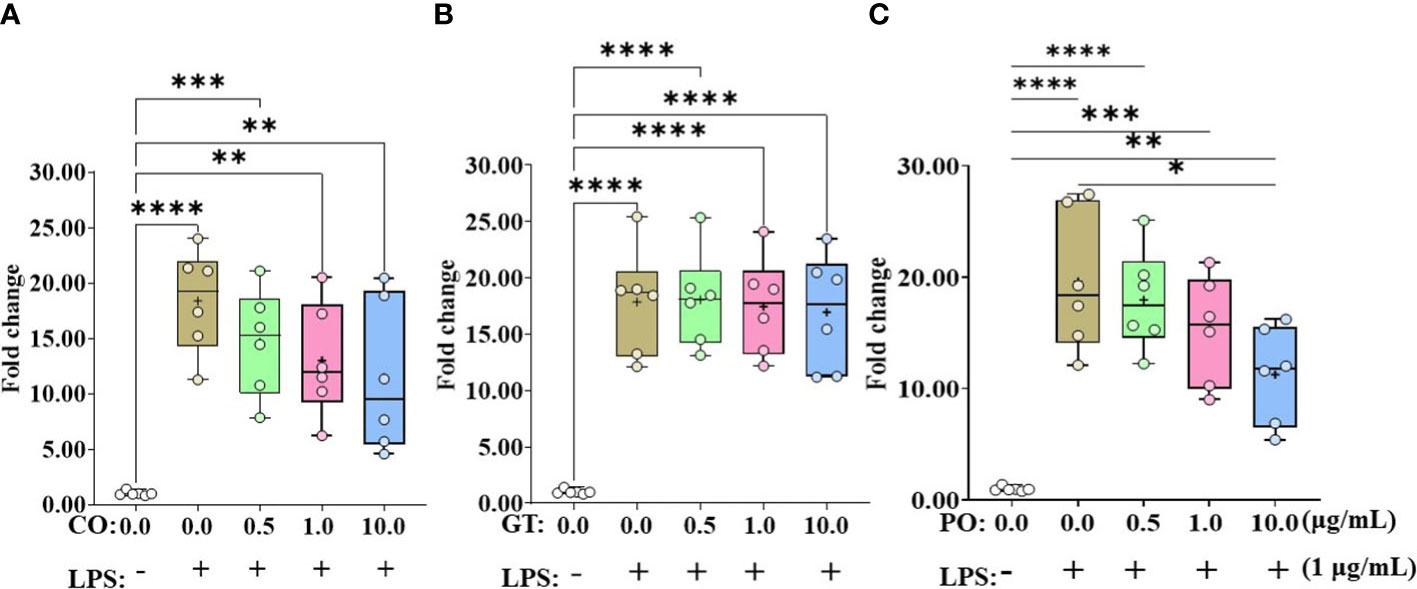
Figure 2 Gene expression of IL-8 in phytochemical-induced chicken intestinal epithelial cells. Cells were treated with three different concentrations (0.5, 1.0, and 10.0 mg/mL) of each phytochemical [cinnamon oil (CO, A), green tea extract (GT, B), and pomegranate (PO, C)] for 18 h with LPS (1μg/mL). The data represent the average of six independent experiments. P < 0.05 (*), P < 0.01 (**), P < 0.001 (***), and P < 0.0001 (****) were considered statistically significant compared to the control (0.0 mg/mL phytochemicals). The fold changes in each transcript were normalized to glyceraldehyde-3-phosphate dehydrogenase and are relative to the transcript expression in unstimulated control group (normalized to 1) using the comparative ΔΔ Ct method.
3.1.2 Tight junction proteins in chicken intestinal epithelial cells
Occludin expression level was (P < 0.005) increased by 10 µg GT treatment compared to the untreated group (Table 2) while administration of CO and PO did not change the occludin expression level. ZO-1 expression level was not changed by the administration of CO, GT, and PO.
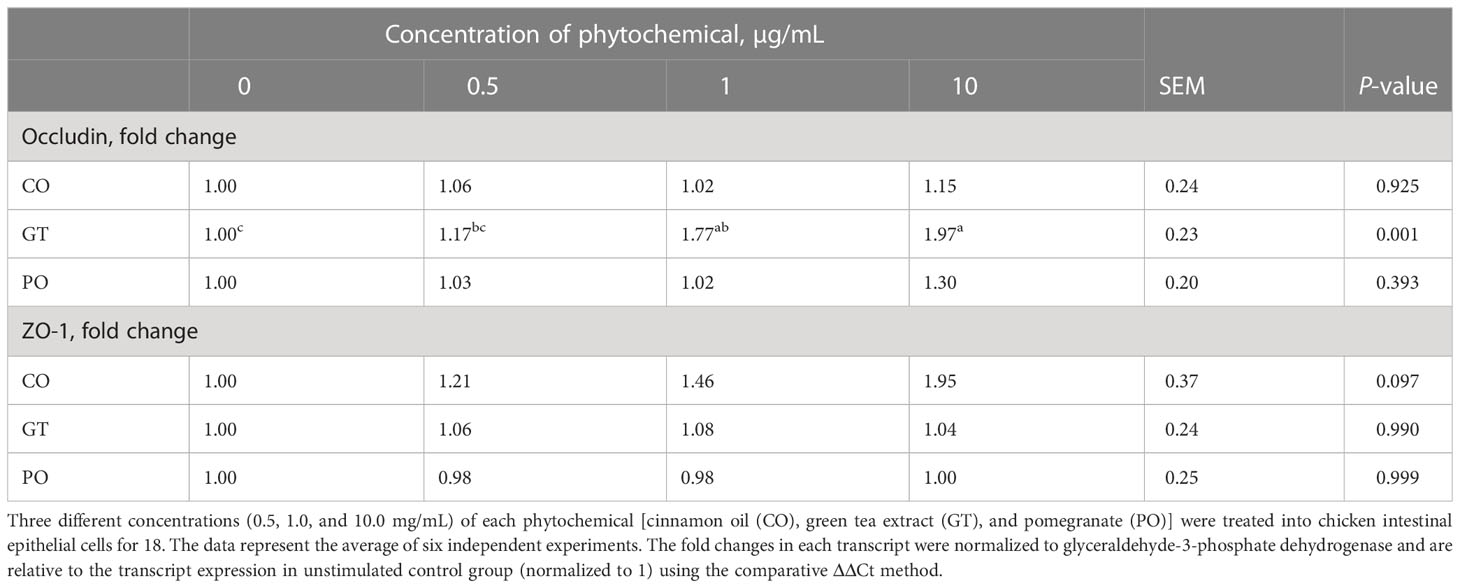
Table 2 Alteration of mucin and tight junction proteins in chicken intestinal epithelial cells by phytochemicals.
3.1.3 Proliferation and differentiation of quail muscle cells and primary chicken embryonic muscle cells
In QMC culture (Table 3), reducing the concentration of 10% FBS up to 0.5% showed (P < 0.05) higher Pax7 expression levels by more than 2-fold regardless of phytochemical administration compared to that of 10% FBS. 0.5% FBS showed (P < 0.05) greater MyoG expression levels compared to that of 10% FBS. When 0.5% FBS was used, treatment with 10 µg/ml PO increased the MyoG expression level from 5 to 8-fold (P < 0.05). Other phytochemicals such as CO, and GT did not affect MyoG expression levels. In PMC culture (Table 4), unlike QMC, most Pax7 and MyoG expression levels were not changed in the presence of 2% FBS and phytochemical administration. However, treatment with 10 µg GT increased (P < 0.05) Pax7 expression level compared to that of 10% FBS.
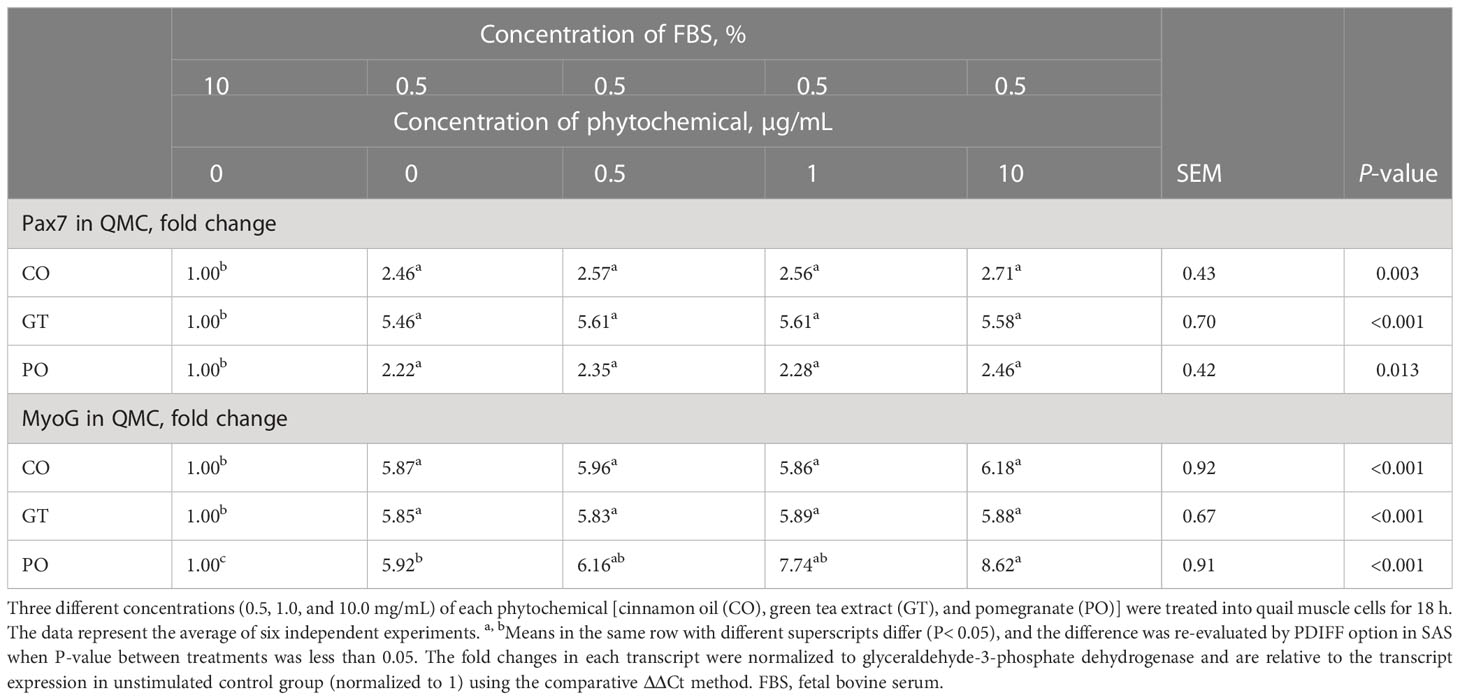
Table 3 Proliferation and differentiation of quail muscle cells by fetal bovine serum concentration and phytochemicals.
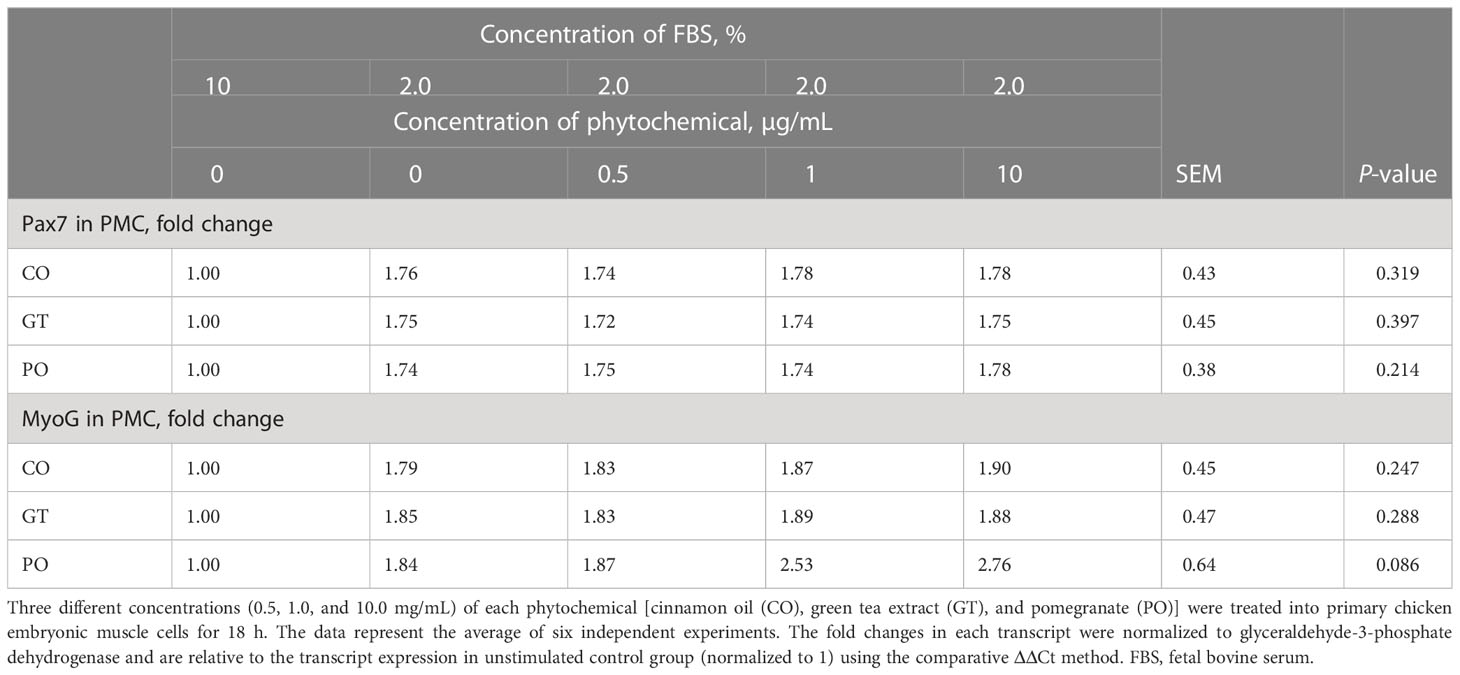
Table 4 Proliferation and differentiation of primary chicken embryonic muscle cells by fetal bovine serum concentration and phytochemicals.
3.1.4 Anticoccidial with C. perfringens
Treatment with CO (Figure 3A) and GT (Figure 3B), regardless of their doses (0.1 and 1.0 mg/mL), did not affect (P > 0.05) E. tenella sporozoite viability whereas administration of PO (Figure 3C) decreased (P < 0.0001) the viability of E. tenella sporozoites by 83% at 0.1 mg/mL, and 90% at 1.0 mg/mL. C. perfringens bacterial counts were not changed (P > 0.05) by treating with CO (Figure 4A) at three different dose levels (0.5, 1.0, and 5.0 mg/mL). GT at the dose of 5.0 mg/mL decreased (P < 0.001) the viability of C. perfringens by 63.3% compared to the control group. However, GT at 0.5 and 1.0 mg/mL also numerically decreased (P > 0.05) the viability of C. perfringens without statistical differences (Figure 4B). In PO groups, there were no differences up to 1.0 mg/mL, whereas PO at 5.0 mg/mL decreased the viability of C. perfringens compared to 0.0 and 0.5 mg/mL of PO (Figure 4C).
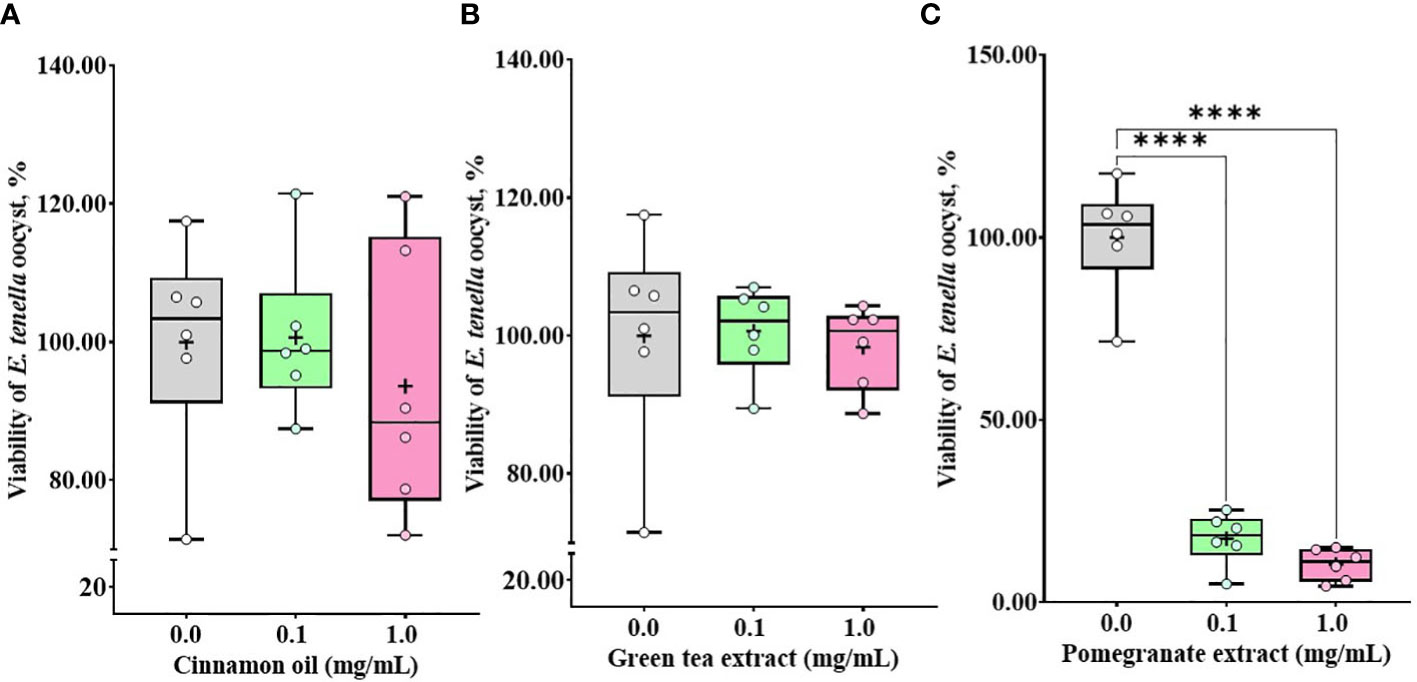
Figure 3 Viability of E. tenella sporozoites against phytochemical administration. Antiparasitic activities against E. tenella sporozoites were determined by counting viable sporozoites after 3 h incubation with cinnamon oil (A), green tea extract (B), and pomegranate extract (C). The data represent the average of six independent experiments. P < 0.0001 (****) was considered statistically significant compared to the control (0.0 mg/mL phytochemicals). 100% value of the control is 2.23 × 107 ± 2.0 × 106 of E. tenella sporozoites. Pomegranate administration reduced the viability of sporozoites by less than 20% in the experimental range.
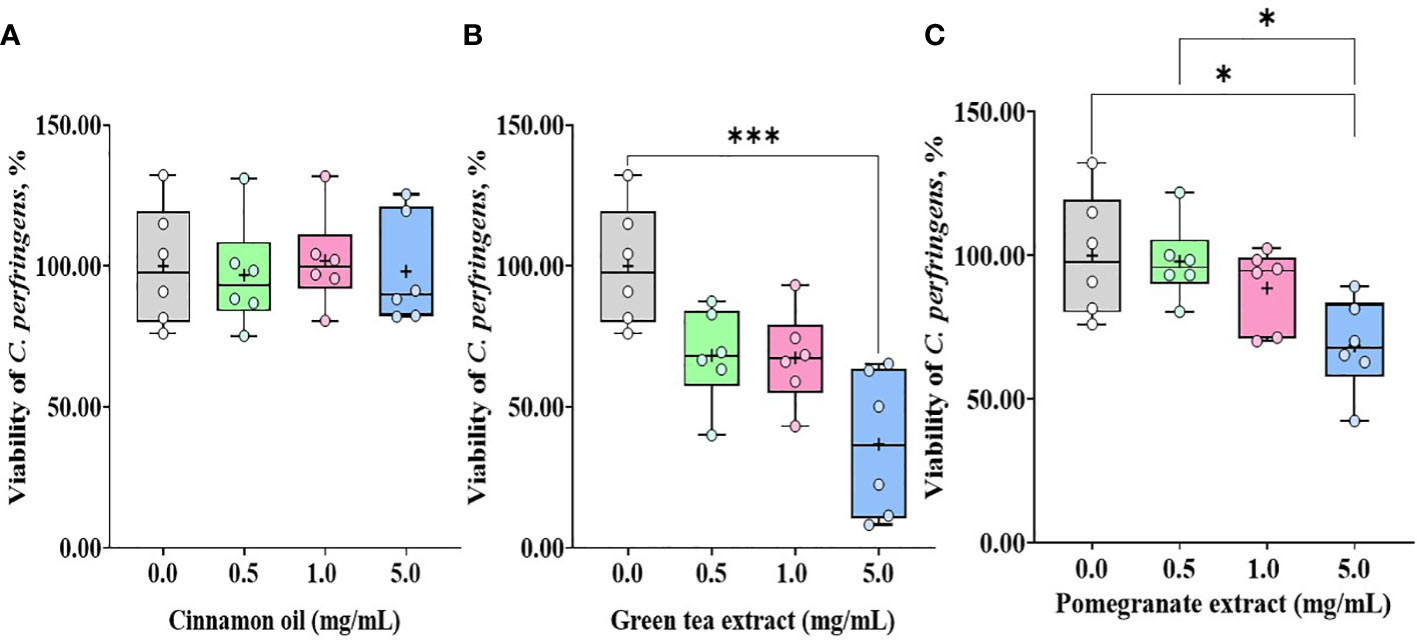
Figure 4 Viability of C.m perfringens against phytochemical administration. Antibacterial activities against C. perfringens were determined by counting colonies after 18 h incubation with cinnamon oil (A), green tea extract (B), and pomegranate extract (C). The data represent the average of six independent experiments. P < 0.05 (*) and P < 0.001 (***) were considered statistically significant compared to the control (0.0 mg/mL phytochemicals). 100% value of the control is 1 × 109 ± 1.1 × 108 CFU of C. perfringens. Pomegranate and tea extract at 5.0 mg/mL reduced the viability of C. perfringens by less than 40%.
3.2 Experiment 2: in vivo studies
3.2.1 Body weight and average daily gain
Dietary Phy supplementation did not change the BW of chickens until d 7 (Table 5). Dietary supplementation with Phy at 200 mg/kg feed decreased (P < 0.05) BW on d 14 before infection compared to others. E. maxima infection, regardless of phytochemical supplementations, reduced the BW on d 20 and 22 compared to that of the NC group. Phy supplementation at 100 mg/kg feed increased (P < 0.05) the BW on d 20 (6 dpi) of chickens infected with E. maxima compared to that of the PC group. On d 22 (8 dpi), the dietary Phy supplementation showed significant linear (P = 0.002) and quadratic effects (P < 0.001) on the final BW. In treatment groups, dietary supplementation with Phy at 50 and 100 mg/kg feed increased the final BW compared to that of the PC group.
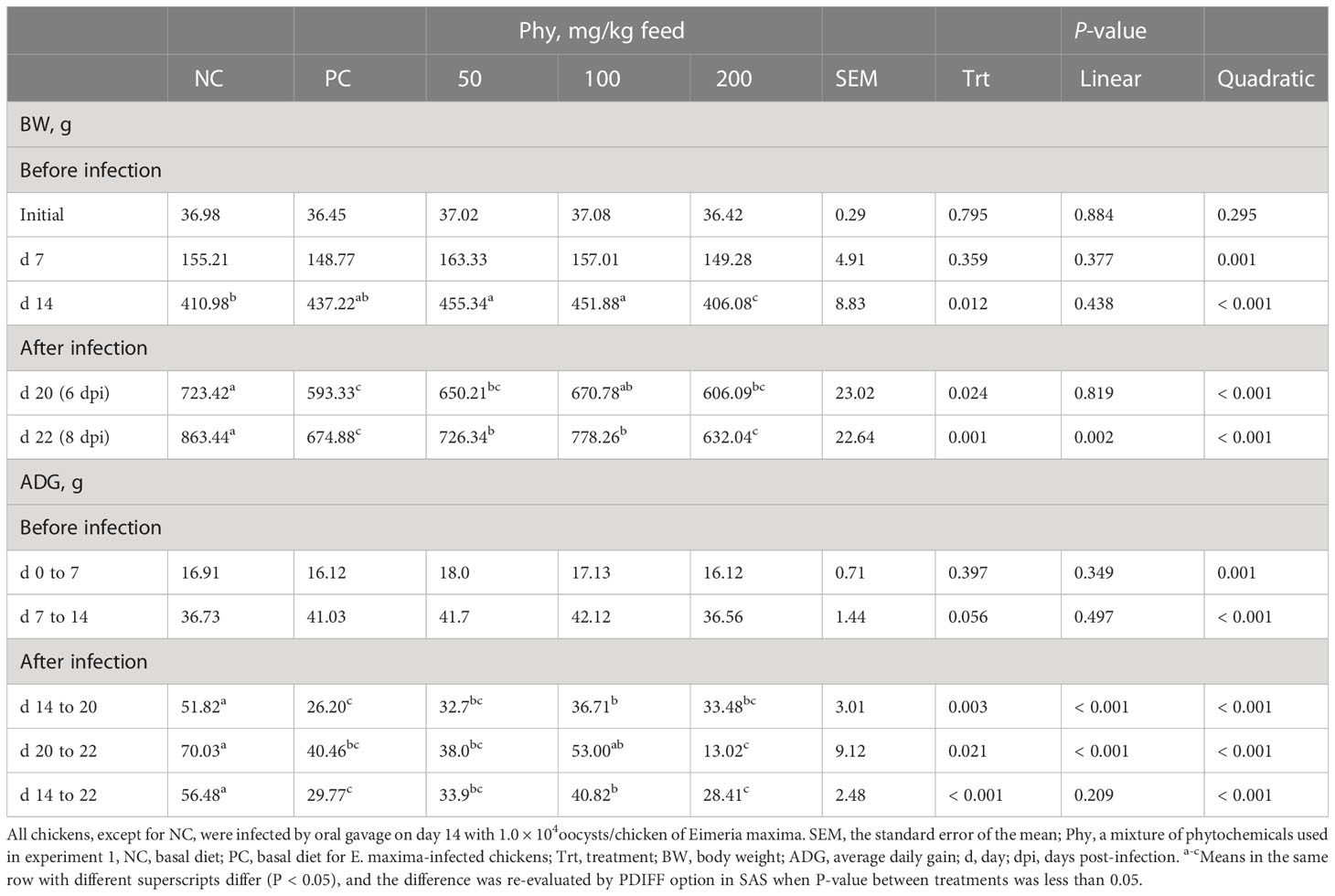
Table 5 Body weight and average daily gain chickens fed a diet supplemented with a phytochemical mixture (Phy) in experiment 2.
Like the BW change according to dietary Phy supplementation, ADG was also affected by the Phy supplementation. Among treatment groups from d 0 to 14, dietary Phy supplementation did not change the ADG of chickens even though the quadratic effect regarding the ADG (P < 0.001) significantly appeared in this period. Infection groups with E. maxima decreased the ADG of chickens during the whole infection period (d 14 to 22) compared to that of the NC group. During two different periods during E. maxima infection which are d 14 to 20 and d 20 to 22, dietary supplementation with the Phy showed (P < 0.001) linear and quadratic effects on ADG of chicken dose-dependently. Chickens supplemented with the Phy at 100 mg/kg feed showed (P < 0.05) greater ADG between infected groups on d 14 to 20, whereas dietary supplementation with Phy at 200 mg/kg feed lowered the ADG of chickens compared to that of the phytochemical group at 100 mg/kg feed from day 20 to 22. During the entire infection period post-E. maxima, from day 14 to 22, dietary supplementation with the Phy induced (P < 0.001) quadratic effects on ADG of chicken in a dose-dependent manner, and the mean of ADG was the highest with 100 mg/kg Phy feed, whereas PC and Phy at 200 mg showed the lowest ADG.
3.2.2 Jejunal cytokines, tight junction proteins, and antioxidant enzymes
E. maxima infection increased (P < 0.05) IL-1β, TNFSF-15, and IFN-γ expression levels in the jejunum of chickens compared to that of NC, while dietary Phy 100 supplementation decreased (P < 0.05) these proinflammatory cytokine levels compared to that of PC (Figure 5). Jejunal tight junction (TJ) proteins (Figure 6) and antioxidant enzymes (Supplementary Figure 2) in chickens were not changed by E. maxima infection and dietary Phy 100 supplementation.
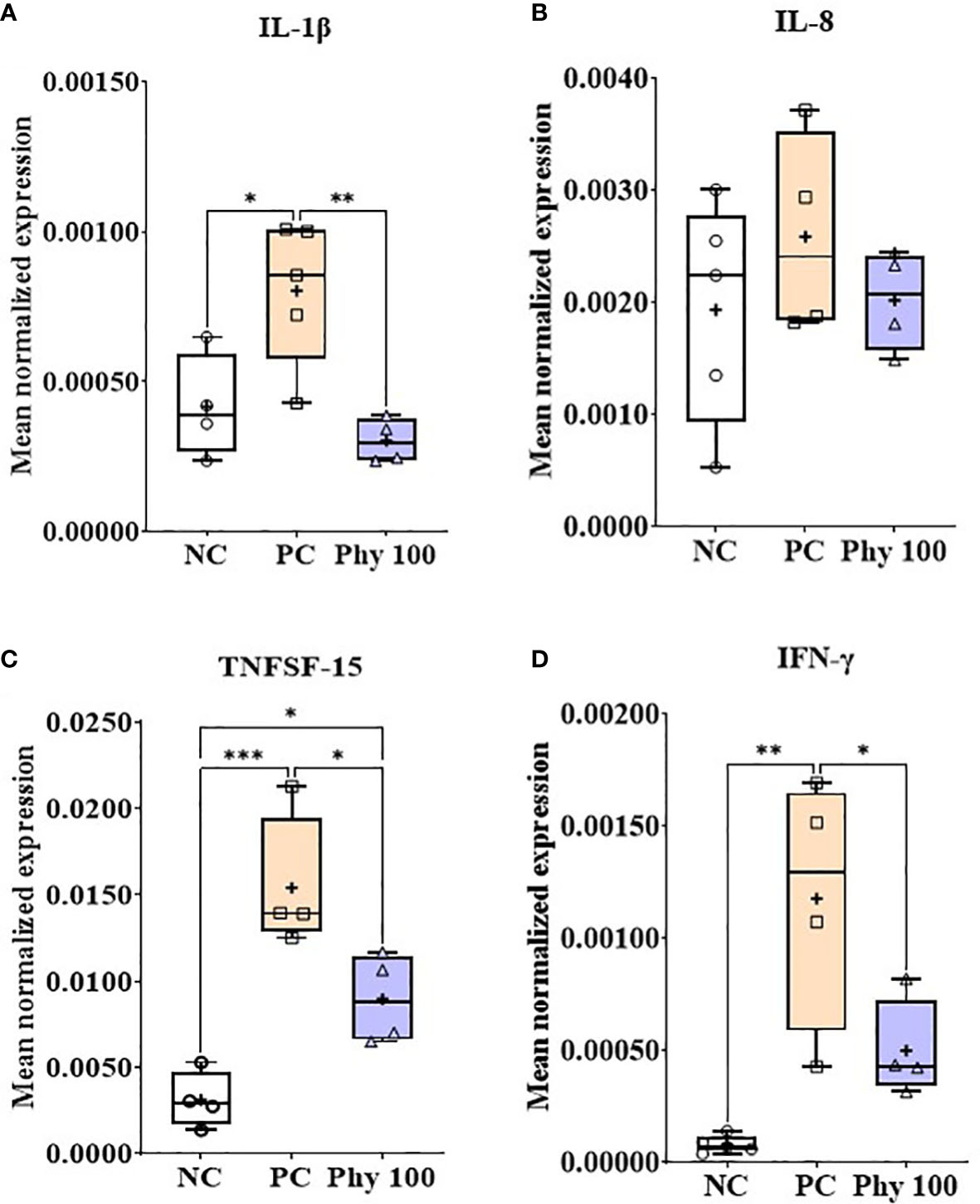
Figure 5 Transcripts of proinflammatory cytokines (A; IL-1β, B: IL-8, C: TNFSF-15 and D: IFN-γ) in jejunum of chickens fed diet supplemented with phytochemical mixture at 100 mg/kg feed during infection with E. maxima in experiment 2. NC, basal diet; PC, basal diet for infected chickens; Phy 100, phytochemical at 100 mg/kg feed; IL, interleukin; TNFSF, tumor necrosis factor superfamily; IFN, interferon. All chickens, except for NC, were infected by oral gavage on day 14 with 1.0 × 104 oocysts/chicken of E. maxima. P < 0.05 (*), P < 0.01 (**), and P < 0.001 (***) were considered statistically significant. The data were collected from jejunal tissues of 5 chickens per treatment on d 22 (8 days post-infection). Transcript levels of the cytokines were measured using quantitative RT-PCR and normalized to GAPDH transcript levels.
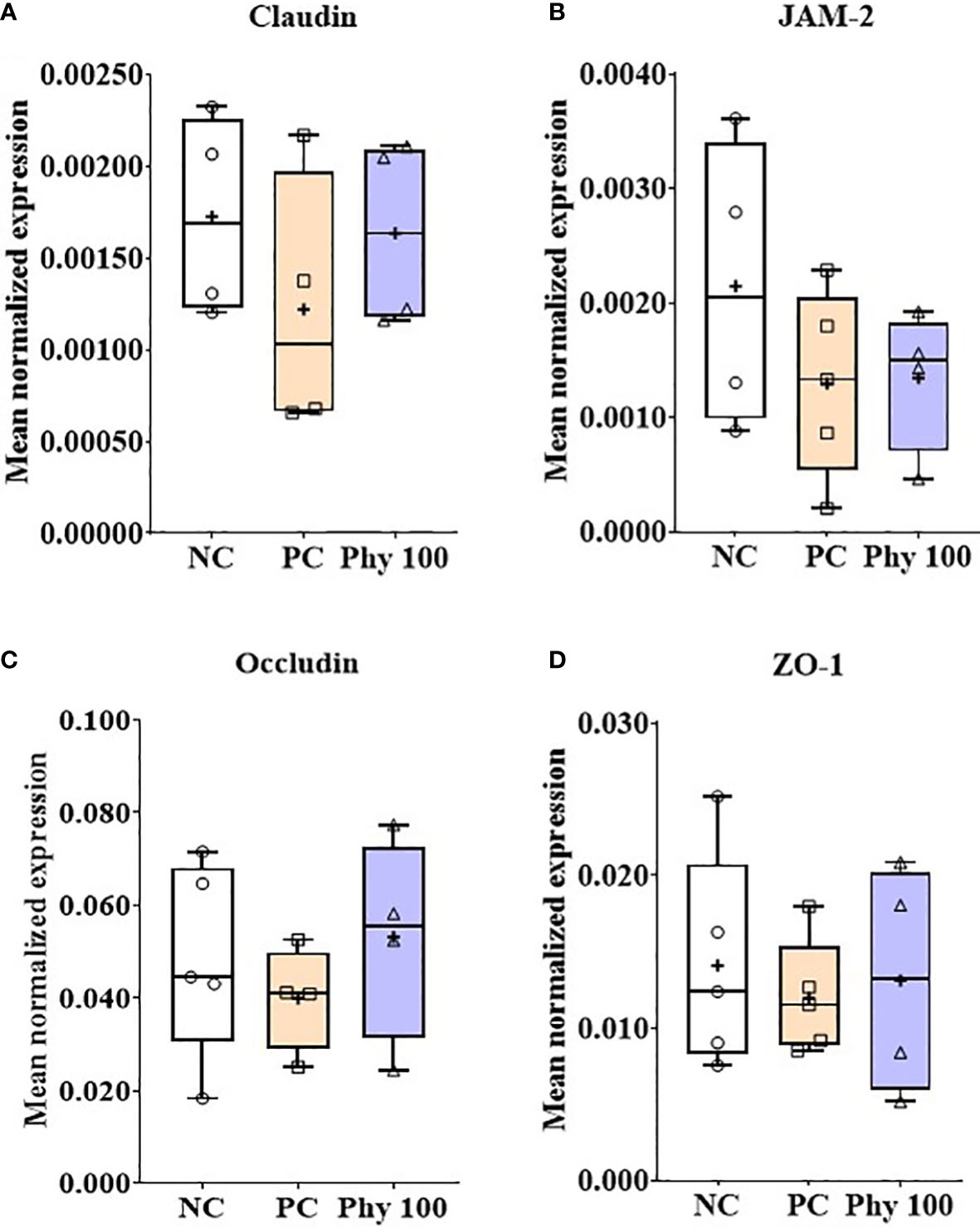
Figure 6 Transcripts of tight junction proteins (A; Claudin, B: JAM-2, C: Occludin and D: ZO-1) in jejunum of chickens fed diet supplemented with phytochemical mixture at 100 mg/kg feed during infection with E. maxima in experiment 2. NC, basal diet; PC, basal diet for infected chickens; Phy 100, phytochemical at 100 mg/kg feed; JAM, junctional adhesion molecule; ZO, zonula occludins. All chickens, except for NC, were infected by oral gavage on day 14 with 1.0 × 104 oocysts/chicken of E. maxima. The data were collected from jejunal tissues of 5 chickens per treatment on d 22 (8 days post-infection). Transcript levels of the cytokines were measured using quantitative RT-PCR and normalized to GAPDH transcript levels.
3.2.3 Oocyst shedding
Dietary supplementation of Phy decreased (P < 0.05) fecal oocyst numbers of the E. maxima-infected chickens in a dose-dependent manner (Figure 7A). Especially, the Phy 200-fed chickens significantly reduced (P < 0.05) the fecal oocyst number compared to that of PC chickens from 6 to 8 dpi.
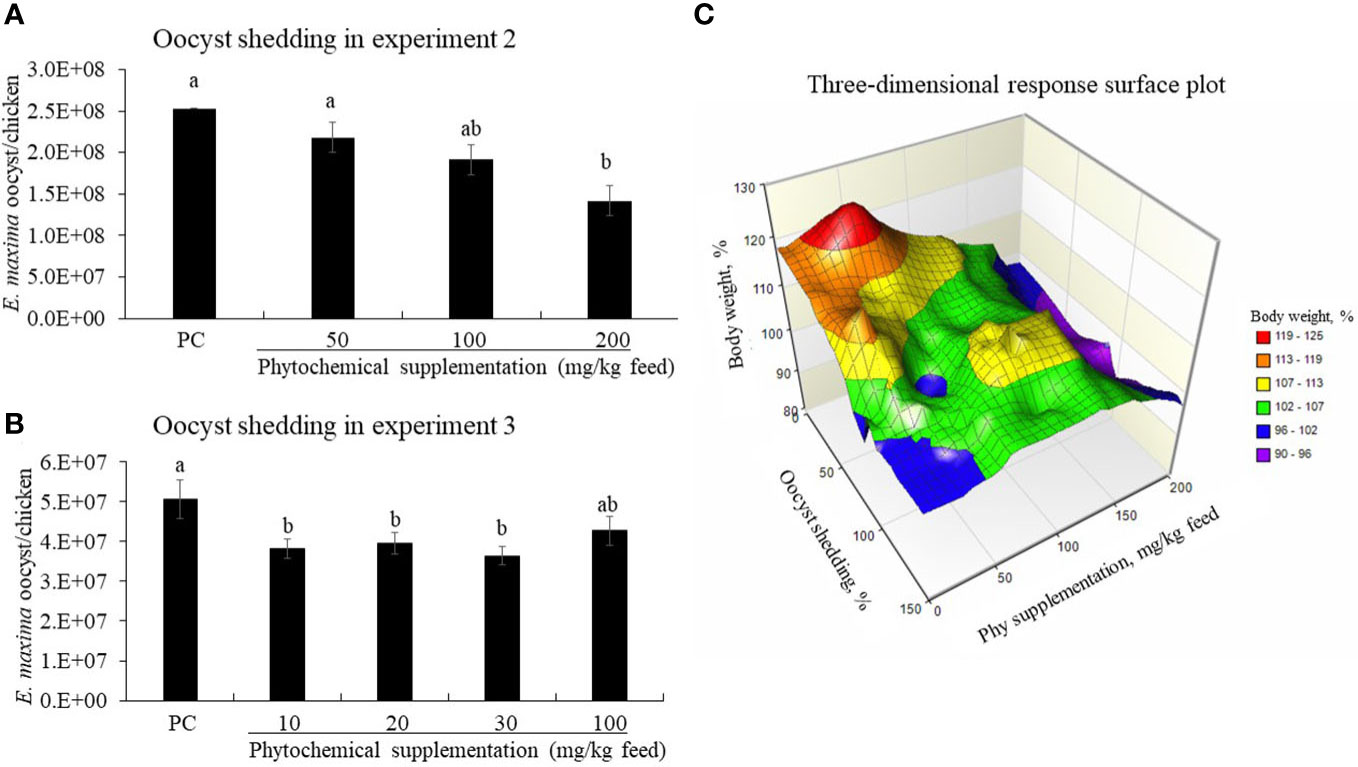
Figure 7 Fecal oocyst shedding (A, B) and three-dimensional response plot (C) on final body weight (BW) of chicken fed diet supplemented with phytochemical mixture. The oocyst shedding was measured from fecal samples which were collected on a tray installed under the cage from 6 to 8 days post-infection. NC group, basal diet without infection, was excluded in oocyst results because there was no oocyst. In the three-dimensional plot, the average values of PC on final BW and oocyst number were considered as 100%. The surface was drawn with each value of BWs, oocyst numbers, and Phy concentrations without statistic calculation.
3.3 Experiment 3: in vivo studies
3.3.1 Body weight and average daily gain
Before infection with E. maxima, BW or ADG of chickens were not changed by dietary Phy supplementation (Table 6). However, E. maxima infection without any Phy reduced (P < 0.05) the BW of chickens compared to that of NC. Among infection groups, chickens fed Phy at 20 mg/kg feed had a bigger BW on d 20 compared to that of the PC group. On d 22, three supplementations (20, 30, and 100 mg/kg feed) of Phy increased (P < 0.05) the BW of chicken compared to that of NC. Like BW, the E. maxima infection also decreased the ADG of chickens on d 14 to 20 compared to that of NC. Three concentrations (20, 30, and 100 mg/kg feed) of the Phy increased (P < 0.05) the ADG of chicken on d 14 to 20 compared to that of NC.
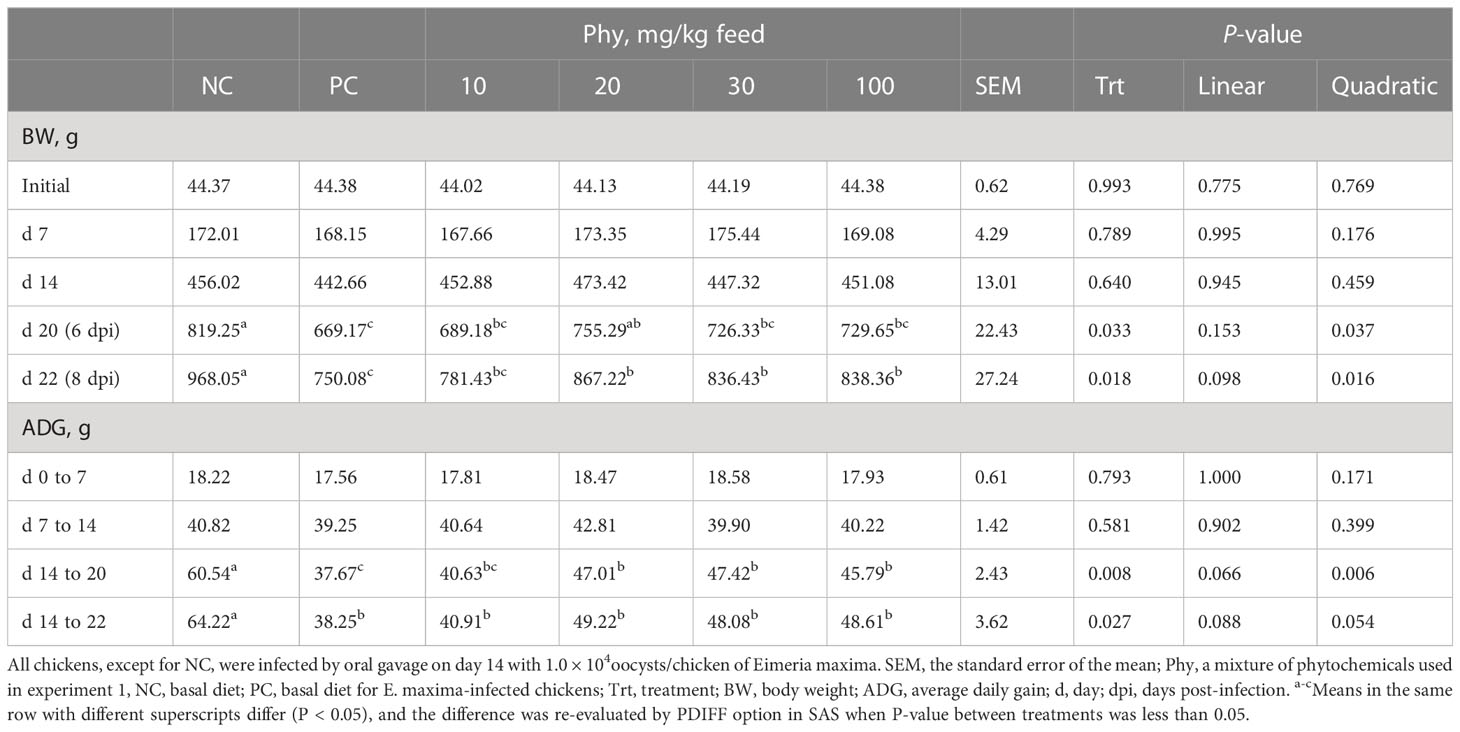
Table 6 Body weight and average daily gain chickens fed a diet supplemented with phytochemical mixture (Phy) in experiment 3.
3.3.2 Jejunal cytokines, tight junction proteins, and antioxidant enzymes
E. maxima infection increased (P < 0.05) IL-1β, TNFSF-15, and IFN-γ expression levels in the jejunum of chickens compared to that of the NC group whereas chickens supplemented with Phy 20 had (P < 0.05) the lower IL-1β and TNFSF-15 levels compared to that of PC (Figure 8). The infection also decreased (P < 0.05) JAM-2 and occludin expression levels compared to that of the NC and Phy 20 recovered (P <0.05) these levels as much as that of the NC group (Figure 9). Jejunal antioxidant enzymes (Supplementary Figure 3) in chickens were not changed by E. maxima infection and dietary supplementation with Phy 20.
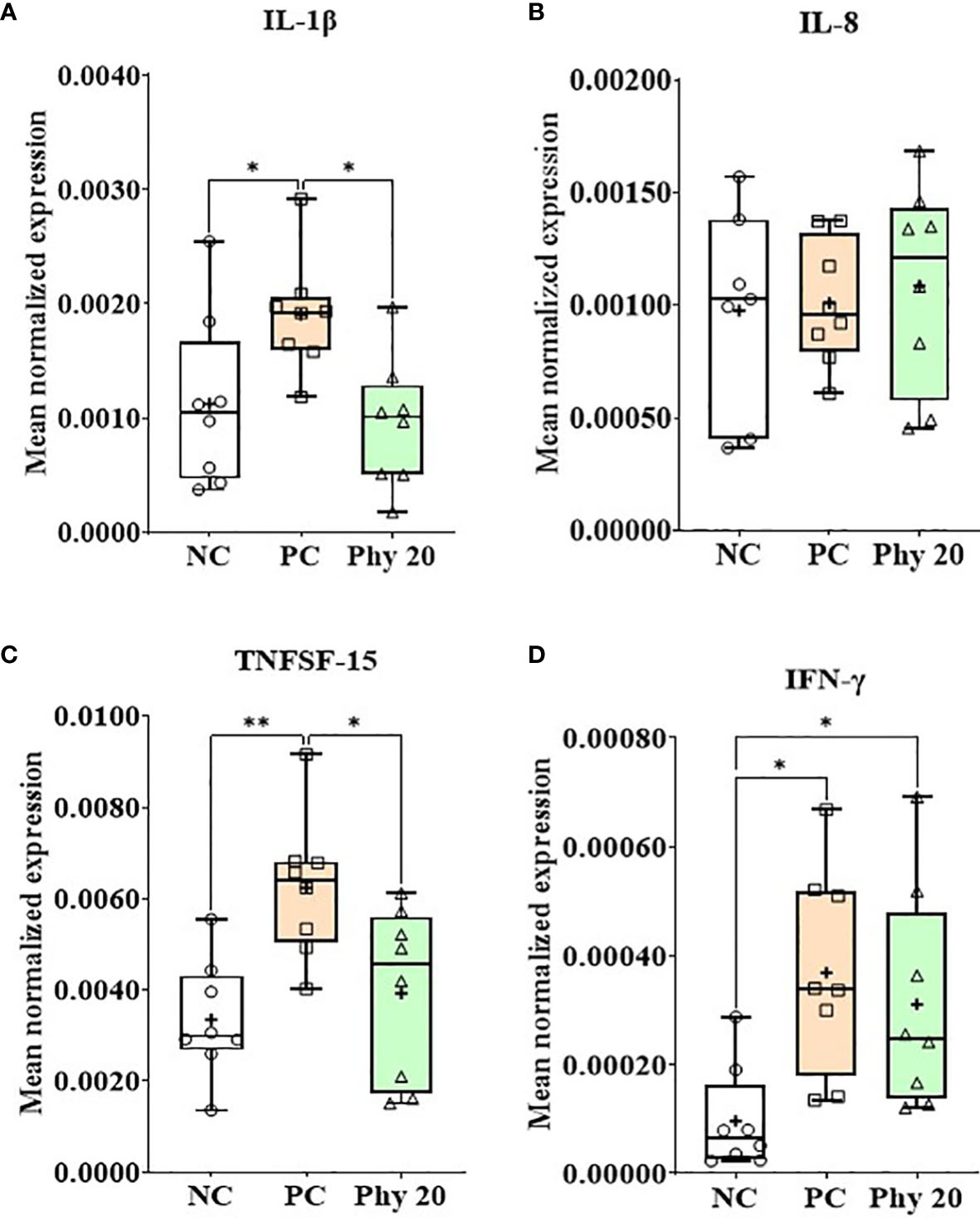
Figure 8 Transcripts of proinflammatory cytokines (A; IL-1β, B: IL-8, C: TNFSF-15 and D: IFN-γ) in jejunum of chickens fed diet supplemented with phytochemical during infection with E. maxima in experiment 3. NC, basal diet; PC, basal diet for infected chickens; Phy 20, phytochemical mixture at 20 mg/kg feed; IL, interleukin; TNFSF, tumor necrosis factor superfamily; IFN, interferon. All chickens, except for NC, were infected by oral gavage on day 14 with 1.0 × 104 oocysts/chicken of E. maxima. P < 0.05 (*) and P < 0.01 (**) were considered statistically significant. The data were collected from jejunal tissues of 5 chickens per treatment on d 22 (8 days post-infection). Transcript levels of the cytokines were measured using quantitative RT-PCR and normalized to GAPDH transcript levels.
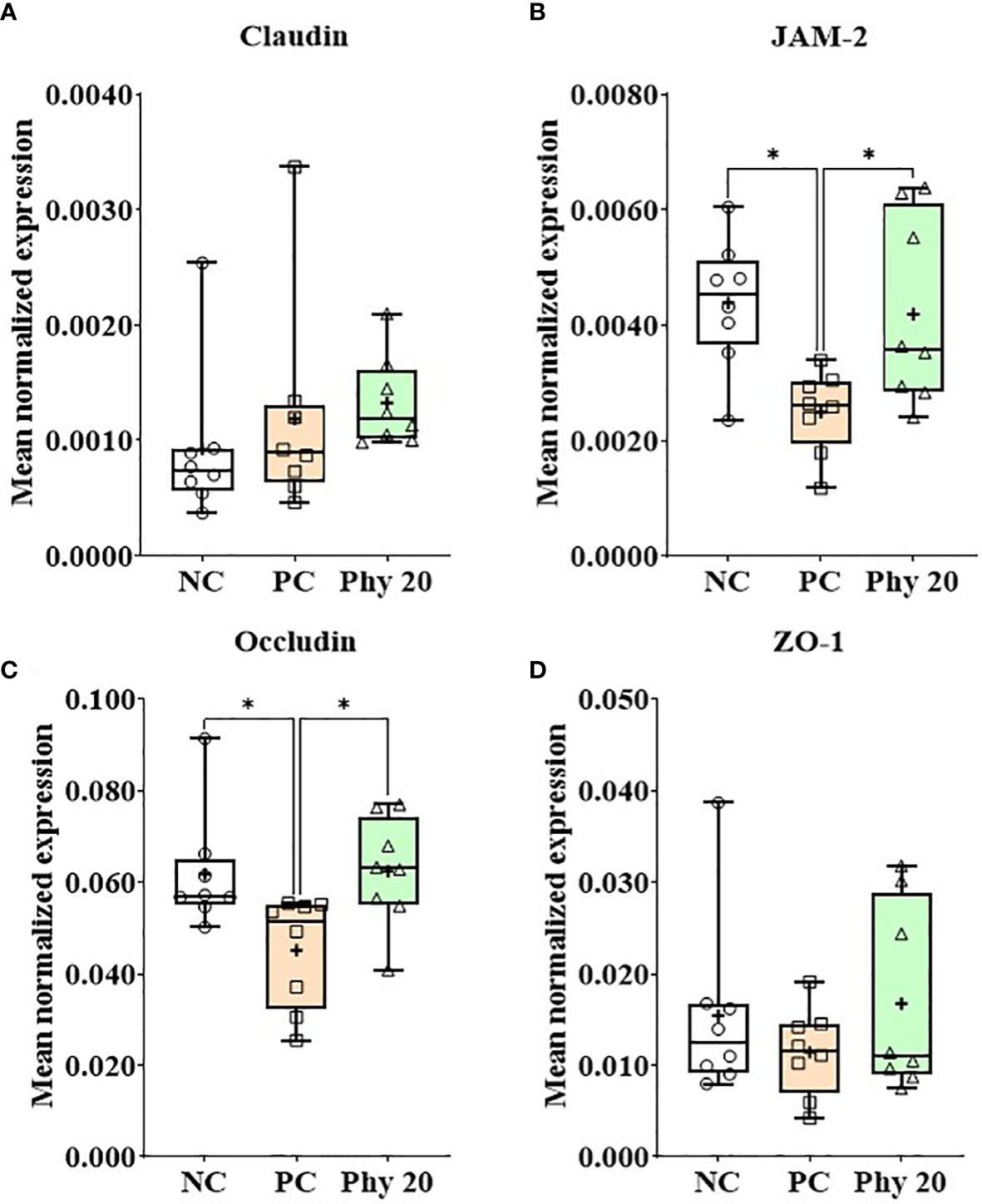
Figure 9 Transcripts of tight junction proteins (A; Claudin, B: JAM-2, C: Occludin and D: ZO-1) in jejunum of chickens fed diet supplemented with phytochemicals during infection with E. maxima in experiment 3. NC, basal diet; PC, basal diet for infected chickens; Phy 20, phytochemical mixture at 20 mg/kg feed; ZAM, junctional adhesion molecule; ZO, zonula occludins. All chickens, except for NC, were infected by oral gavage on day 14 with 1.0 × 104 oocysts/chicken of E. maxima. P < 0.05 (*) was considered statistically significant. The data were collected from jejunal tissues of 5 chickens per treatment on d 22 (8 days post-infection). Transcript levels of the cytokines were measured using quantitative RT-PCR and normalized to GAPDH transcript levels.
3.3.3 Oocyst shedding and three-dimensional response surface plot
In EXP 3, chickens fed diets supplemented with Phy 10, Phy 20, and Phy 30 showed reduced oocyst numbers compared to that of PC and the decrease was dependent on the concentration of Phy (Figure 7B). According to the three-dimensional response surface plot that was combined with EXP 2 and 3 on the final body weight interaction between oocyst number and phytochemical supplementation, 50 mg of Phy supplementation increased the final body weight by 125% and lowered the oocyst number to less than 20% (Figure 7C).
4 Discussions
Phytochemicals promote human and animal health as demonstrated by extensive scientific literature and medical practices on traditional medicine which has focused on the disease-healing responses of the host (humans) to medicinal plants (5). Considerable studies from many laboratories including ours (5) for over 10 years have provided research data showing the beneficial effects of various phytochemicals in animals, especially in poultry. In this study, we took a novel approach to select beneficial phytochemicals that affect chicken physiological responses using in vitro screening system using different chicken cell lines to select phytochemicals showing desirable physiological effects on immune cells, muscle cells, and intestinal cells in chickens based on their unique biomarkers associated with cell function. In addition, we have evaluated their direct effects on parasites on select phytochemicals. Based on the effects of phytochemicals on different cell lines, we then designed novel formula that effectively mitigates coccidiosis, and special phytochemical combinations were designed to enhance host resilience during disease stress by positively affecting host physiological responses including immunity, muscle cell function, and gut health. The results of our study showed that these approaches could be successfully used to find a unique practical formulation impacting host-mediated responses involving muscle, gut, and immunity which can mitigate coccidiosis without antibiotics
Today, there are wide ranges of nutraceuticals available in the feed industry to control economically important infections in animal agriculture (3). Especially, commercial phytochemical products to reduce disease effects against coccidiosis, a major intestinal disease caused by several different species of Eimeria which is estimated to cause an annual economic loss of more than $14 billion worldwide (4, 12) have been reported (5). However, with an increased understanding of the mechanisms of action of many phytochemicals (3), it is becoming evident that we need to better understand the underlying mechanisms of host-pathogen interactions and the effects of various phytochemicals on host physiological responses to economically important diseases. Eventually, combinatory effects of various phytochemical feed additives that work best to reduce the harmful host response in various disease situations could effectively reduce the economic cost of animal production in the post-antibiotic era.
Phytochemicals derived from plants are natural bioactive compounds that can be incorporated into animal feed to improve productivity and are also referred to as phytobiotics or phytogenics (2). The main bioactive compounds of the phytochemicals are composed of polyphenols, and their composition and concentration vary according to the plant, parts of the plant, geographical origin, harvesting season, environmental factors, storage conditions, and processing techniques (5). The In-vitro model has the advantage that can predict more reliable and standardized results before performing the studies in-vivo (16). Notably, high concentrations of phytochemicals emerge to have cytotoxicity effects which can affect normal or healthy cells as well as cancer cells (17), therefore collecting preliminary data through an in vitro model may be helped to minimalize the risk to animal health in animal studies.
We have also evaluated the direct antimicrobial effects of CO, GT, and PO against E. tenella sporozoite and C. perfringens bacteria. In general, the dose ranges that showed direct cytotoxic effects against these pathogens are significantly higher than the doses mediating beneficial host physiological responses and are not practical for commercial application. The PO reduced sporozoite of E. tenella up to 17% at 0.1 mg/mL and 10% at 10 mg/mL. Furthermore, GO and PO showed antibacterial activity against C. perfringens but only at high concentrations of phytochemicals (5.0 mg/mL). Pomegranate is well known in general as having strong antioxidant and anti-inflammatory activities (18) and inhibiting apicomplexan parasite growth (19, 20) as well as gram-positive and negative bacteria growth (21). Juneja et al. (22) reported that green tea leaf extracts can reduce the potential risk of C. perfringens spore germination. Among active compounds in phytochemicals, polyphenols mainly play a pivotal role in antiparasitic and antibacterial activities including anthocyanidins, catechins [epicatechin (EC), epicatechin-3-gallate (ECG), epigallocatechin (EGC), gallocatechin, epigallocatechin-3-gallate (EGCG), catechin gallate], cinnamic aldehyde, gallic acid, tannins (gallotannins and ellagitannins), and theaflavins [theaflavin (TF), TF-3-O-gallate(TF3G), TF-3′-O-gallate (TF3′G), TF-3,3′-O-digallate (TFdG)] (23–28). CO did not show any antimicrobial activities even though they are known as having similar bioactive constituents (polyphenols) as green tea extract and pomegranate. This discrepancy may be contributed by the variety of botanic components, as well as the variability of the animal conditions, the presence of a pathogen, and methods of producing phytochemicals (6). LPS treatment of chicken epithelial cells dramatically increased proinflammatory cytokines such as IL-1β and IL-8 (29, 30). The IL-1β mediates the inflammatory responses for a wide range and the IL-8 is responsible for recruiting neutrophils (heterophils) to the inflamed site (31). Administration of CO and GT suppress LPS-induced IL-1β in chicken epithelial cells and PO administration reduced LPS-induced IL-8. Plant-derived phytochemicals showed anti-inflammatory effects altering the cytokine levels in the intestine in chicken and pigs following treatment with various pathogens such as LPS (32), Eimeria spp (33), Salmonella spp (31), and E. coli (34).
The optimum regulation of intestinal permeability through intricate mechanisms of physiological and immunologic events on the intestinal epithelial barrier is an inevitable requirement to allow efficient digestion, absorption, and passage of nutrients as well as restrict the entry of larger molecules, such as antigens (35). The paracellular pathway for intestinal permeability is manipulated by TJs, adherens junctions, and desmosomes (36, 37). Among them, TJs are composed of multiprotein complexes such as membrane proteins (claudin and occludin), and scaffold proteins (ZO-I and II) (38). In the current study, GT improved occludin expression levels in IEC by 1.97-fold. Park et al. (28) and Lagha and Grenier (39), reported that green tea extract improved occludin levels similarly to our observation by around 2-fold. They also revealed that these beneficial results were due to EGCG and TF3′G from green tea extract. Sundstrom et al. (40) and Tash et al. (41) demonstrated that the altered occludin level regulates TJ barriers in response to phosphorylation, cytokines, and growth factors. However, the ZO-1 level was not changed by these phytochemical administrations. Unlike our result, ZO-1 is generally known as a TJ protein that mediated interactions with occludin and F-actin. Further studies will be necessary to better understand the molecular mechanisms involved in the phytochemical regulation of TJ proteins.
In the current study, the gene expression levels of MyoG and Pax7 were measured to investigate how phytochemicals affect muscle growth related to the growth of chickens. Lower FBS concentration [0.5% FBS in QMC (13) and 2% FBS in PMC (15)] to induce myogenic differentiation improved MyoG expression levels in QMC and PMC by more than 5-fold and 2-fold, respectively. In this study, only PO at a 10 mg dose showed that MyoG in QMC was improved by around 45% compared to the non-treated group when 0.5% FBS was used. Unlike our expectation, Pax7 was also increased in the groups that induced cell differentiation by lowering the FBS concentration. According to Rudnicki et al. (42), satellite cells are precursor or stem cells of skeletal muscles as mononuclear cells, divided into four stages (quiescence, proliferation, renewal self, and differentiation) of growth. Every stage expresses its myogenic regulatory factors (Pax7, MyoD, Myf5, myogenin, and MRF4). When muscle production occurs, satellite cells, which were normally quiescence (Pax7+MyoD-), enter the cell cycle again, increasing the expression of MyoD and Myf5 (Pax7+MyoD+). For the differentiation of these satellite cells, Pax7 is reduced and myogenin (Pax7-MyoD+Myogenin+) is increased to produce a new nuclear or myotube (43). Therefore, the current result of QMC indicates preferential cell differentiation because the MyoG level was more than 5-fold compared to that (more than 2-fold) of Pax7. PMC cells were in a cell proliferation phase based on the ratio of Pax to MyoG which was almost 1:1. To induce differentiation of PMC, it may be possible to extend incubation time or lower FBS concentration further. Although most of the data came from studies in mice, polyphenols showed a positive effect on muscle development (44).
In this study (EXP 1), each phytochemical showed beneficial effects on host inflammatory response, TJ proteins, and/or muscle growth factors. Therefore, a mixture of CO, GT, and PO provides beneficial effects against coccidiosis in poultry. The effects of selected phytochemical mixture were evaluated for its efficacy on growth performance and intestinal health of young broiler chickens infected with E. maxima (EXP 2 and 3) in commercial broiler chickens. In previous studies, dietary supplementation of young broiler chickens with a single phytochemical has shown a beneficial effect on growth, intestinal immune responses, and intestinal epithelial integrity of chicken infected with Eimeria spp (5, 45–47). However, a single phytochemical feed additive sometimes failed to exert beneficial effects on host, and in some cases, its effects were harmful (48–51). Richards et al. (52) and Rizeq et al. (53) suggested that a combination of phytochemical feed additives may promote a synergy effect. As shown in our study (EXP 2), BWs on d 14 (before infection) of chickens fed dietary Phy 50 and 100 were improved by 7% and 6%, respectively, compared to that of chickens fed basal diet (NC and PC). However, chickens fed dietary Phy 200 showed decreased BW by 4.2% than that of NC and PC. Phytochemicals may have a biphasic dose-response relationship, showing a beneficial effect at lower doses but may have adverse effects at higher doses. This could be due to a variety of reasons, such as saturation of absorption, metabolism, or elimination pathways, as well as the. activation of different cellular mechanisms that can lead to toxicity or cellular damage (5). Infection (PC) with E. maxima dramatically reduced the BW at 6 dpi by 22% compared to that of NC while Phy 100 enhanced the BW of PC by 13%. The effect of Phy supplementation was long-lasting since Phy-treated chickens showed a greater BW at 8 dpi by 7.5% in Phy 50 and 15% in Phy 100 when compared to that of PC.
In EXP 3, the effect of the phytochemical supplementation was evaluated in the lower concentration of Phy (10 to 100 mg/kg feed) than in EXP 2. All phytochemical-supplemented groups did not show a negative effect of BW on d 7 and 14 (before infection) compared to that of NC and PC. However, unlike our expectation, Phy 100 did not have any difference compared to that of NC or PC. E. maxima infection in EXP 3 also reduced the BW at 6 dpi by 22% like EXP 2 and Phy 20 improved the BW by 13% compared to that of the PC group. Especially, in BW at 8 dpi, all Phy groups except 10 mg improved the BW of chickens by 16% in 20 mg, 12% in 30 mg, and 12% in 100 mg when compared to that of the PC group. There was no mortality by Eimeria infection during both EXP 2 and 3. In this study, we chose the infecting dose of Eimeria to induce a mild infection to avoid severe coccidiosis and to show the beneficial effects of phytonutrients. In this study, the infection decreased growth by approximately 22%, which we believe to be an appropriate level of infection based on our extensive previous experience (1, 13–15).
Distal jejunal samples were collected at 8 dpi (EXP 2 and 3) to analyze proinflammatory cytokines, TJ proteins, and antioxidant enzymes. Among them, samples of Phy 100 in EXP 2 and Phy 20 in EXP 3 were selected for these analyses because each group showed the best growth in each EXP. Infection with E. maxima induced all cytokines except IL-8 in both EXPs at 8 dpi. Both Phy 100 and Phy 20 treatment groups showed mitigated E. maxima-induced inflammatory responses by suppressing proinflammatory cytokines (IL-1β and TNFSF-15) in the jejunum of chickens. In EXP 2, Phy 100 group did not show any changes in TJ protein expression after E maxima infection, although the infection with E. maxima increased intestinal permeability by decreasing the levels of JAM-2 and occludin and then the Phy 20 recovered these proteins in EXP 3.
In the case of antioxidant enzymes, no phytochemical-supplemented groups show any beneficial effect in both studies. When measuring host immune responses and barrier integrity in the intestine to evaluate the growth-promoting effects of feed additives in Eimeria infections, it is very important to choose the optimum time points for sample collection since host-parasite interaction changes with the life cycle of Eimeria. In chickens, infection with Eimeria spp reaches the peak response between 4 and 6 dpi even though the magnitude of infection will be determined by the infecting dose and genetics of the host (54). In this study, the sample collection was done at 8 dpi based on our established animal protocol. Fecal oocyst numbers gradually decreased as the concentrations of Phy increased although Phy 200 showed a negative effect on BW in EXP 2. In EXP 3, a lower concentration (10 to 30 mg/kg feed) of Phy reduced the oocyst number in fecal samples than that of PC.
In the current study, high concentrations of phytochemicals may have exerted toxic effects against parasites and bacteria as we have seen in the in vitro antimicrobial assays, but it is not clear what effects these high-dose treatments had on host response in animal trials, especially with respect to body weight and parasite fecundity after coccidiosis challenge. Thus, it is important to consider the effect of phytochemical doses on their physiological (nutritional) effects to maintain optimal health. In the three-dimensional plot, the average values of PC on final body weight and oocyst number were considered 100%. The surface was completed with each value of BW, oocyst number, and Phy concentration without statistic calculation, thus the figure shows a simple tendency. Therefore, Phy at around 50 mg increased BW by around more than 20% and reduced the oocyst number by less than 20%.
In conclusion, this is the first study demonstrating the development of an effective phytochemical feed additive formula based on the in vitro evaluation of different physiological host functions using chicken cell lines to mitigate coccidiosis. This paper showed that the combination of CO, GT, and PO exerted beneficial effects on mitigating coccidiosis response by impacting host immunity, muscle growth, and gut integrity. Although these phytochemicals showed antiparasitic and antibacterial activities against E. tenella and C. perfringens, respectively, at higher phytochemical doses, their beneficial effects in coccidiosis trials were due to phytochemical-induced mitigation of host disease responses including reduced inflammation, enhance gut function and facilitated muscle growth. Their combinatory effects on promoting gut health significantly reduced parasite-induced gut injury after coccidiosis infection. These findings will provide a scientific rationale to develop a science-based antibiotic-independent strategy to mitigate coccidiosis response in poultry production to reduce the economic cost associated with commercial broiler production.
Data availability statement
The original contributions presented in the study are included in the article/Supplementary Material. Further inquiries can be directed to the corresponding author.
Ethics statement
The animal study was reviewed and approved by The Beltsville Agricultural Research Center Institutional Animal Care and Use Committee.
Author contributions
IP and HL designed the research. HL supervised the research. IP, and HN conducted the in vitro research. IP, YL, and SW conducted the in vivo research. IP analyzed data and drafted the manuscript. HL supervised the research and edited the manuscript. IP, HN, SW, YL, EW, SR, and HL had responsibility for the content. All authors contributed to the article and approved the submitted version.
Funding
Funding was partly supported by ARS CRIS 8042-32000-115-00D and partly by USDA/NIFA SAS award 2020-69012-31823.
Conflict of interest
Authors EW and SR were employed by the company AVT natural.
The remaining authors declare that the research was conducted in the absence of any commercial or financial relationships that could be construed as a potential conflict of interest.
Publisher’s note
All claims expressed in this article are solely those of the authors and do not necessarily represent those of their affiliated organizations, or those of the publisher, the editors and the reviewers. Any product that may be evaluated in this article, or claim that may be made by its manufacturer, is not guaranteed or endorsed by the publisher.
Supplementary material
The Supplementary Material for this article can be found online at: https://www.frontiersin.org/articles/10.3389/fimmu.2023.1145367/full#supplementary-material
References
1. Park I, Oh S, Goo D, Celi P, Lillehoj HS. Effect of dietary sophorolipids on growth performance and gastrointestinal functionality of broiler chickens infected with eimeria maxima. Poult Sci (2022) 101:101944. doi: 10.1016/j.psj.2022.101944
2. Gadde U, Kim WH, Oh ST, Lillehoj HS. Alternatives to antibiotics for maximizing growth performance and feed efficiency in poultry: a review. Anim Heal Res Rev (2017) 18:26–45. doi: 10.1017/S1466252316000207
3. Gadde U, Oh ST, Lee YS, Davis E, Zimmerman N, Rehberger T, et al. The effects of direct-fed microbial supplementation, as an alternative to antibiotics, on growth performance, intestinal immune status, and epithelial barrier gene expression in broiler chickens. Probiotics Antimicrob Proteins (2017) 9:397–405. doi: 10.1007/s12602-017-9275-9
4. Blake DP, Knox J, Dehaeck B, Huntington B, Rathinam T, Ravipati V, et al. Re-calculating the cost of coccidiosis in chickens. Vet Res (2020) 51:1–14. doi: 10.1186/s13567-020-00837-2
5. Lillehoj H, Liu Y, Calsamiglia S, Fernandez-Miyakawa ME, Chi F, Cravens RL, et al. Phytochemicals as antibiotic alternatives to promote growth and enhance host health. Vet Res (2018) 49:1–18. doi: 10.1186/s13567-018-0562-6
6. Abdelli N, Solà-Oriol D, Pérez JF. Phytogenic feed additives in poultry: achievements, prospective and challenges. Animals (2021) 11:3471. doi: 10.3390/ANI11123471
7. Shin S, Choi YM, Suh Y, Lee K. Delta-like 1 homolog (DLK1) inhibits proliferation and myotube formation of avian QM7 myoblasts. Comp Biochem Physiol B Biochem Mol Biol (2015) 179:37–43. doi: 10.1016/j.cbpb.2014.09.006
8. Hassan A, Ahn J, Suh Y, Choi YM, Chen P, Lee K. Selenium promotes adipogenic determination and differentiation of chicken embryonic fibroblasts with regulation of genes involved in fatty acid uptake, triacylglycerol synthesis and lipolysis. J Nutr Biochem (2014) 25:858–67. doi: 10.1016/j.jnutbio.2014.03.018
9. Kim WH, Jeong J, Park AR, Yim D, Kim S, Chang HH, et al. Downregulation of chicken interleukin-17 receptor a during Eimeria infection. Infect Immun (2014) 82:3845–54. doi: 10.1128/IAI.02141-14
10. Kim WH, Lillehoj HS, Min W. Evaluation of the immunomodulatory activity of the chicken NK-lysin-derived peptide cNK-2. Sci Rep (2017) 7:1–11. doi: 10.1038/srep45099
11. Haug A, Thebo P, Mattsson JG. A simplified protocol for molecular identification of Eimeria species in field samples. Vet Parasitol (2007) 146:35–45. doi: 10.1016/j.vetpar.2006.12.015
12. Lee Y, Park I, Wickramasuriya SS, Ben AJ, ME K, Lillehoj HS. Co-Administration of chicken IL-7 or NK-lysin peptide 2 enhances the efficacy of eimeria elongation factor-1α vaccination against Eimeria maxima infection in broiler chickens. Poult Sci (2022) 101:102013. doi: 10.1016/j.psj.2022.102013
13. Park I, Nam H, Goo D, Wickramasuriya SS, Zimmerman N, Smith AH, et al. Gut microbiota-derived indole-3-carboxylate influences mucosal integrity and immunity through the activation of the aryl hydrocarbon receptors and nutrient transporters in broiler chickens challenged with eimeria maxima. Front Immunol (2022) 13:867754. doi: 10.3389/fimmu.2022.867754
14. Wickramasuriya SS, Park I, Lee Y, Kim WH, Przybyszewski C, Gay CG, et al. Oral delivery of Bacillus subtilis expressing chicken NK-2 peptide protects against Eimeria acervulina infection in broiler chickens. Front Vet Sci (2021) 8:684818. doi: 10.3389/fvets.2021.684818
15. Park I, Goo D, Nam H, Wickramasuriya SS, Lee K, Zimmerman NP, et al. Effects of dietary maltol on innate immunity, gut health, and growth performance of broiler chickens challenged with eimeria maxima. Front Vet Sci (2021) 8:667425. doi: 10.3389/fvets.2021.667425
16. Grover M, Behl T, Sehgal A, Singh S, Sharma N, Virmani T, et al. In vitro phytochemical screening, cytotoxicity studies of Curcuma longa extracts with isolation and characterisation of their isolated compounds. Molecules (2021) 26:1–17. doi: 10.3390/molecules26247509
17. Shokrzadeh M, Azadbakht M, Ahangar N, Naderi H, Saeedi Saravi SS. Comparison of the cytotoxic effects of Juniperus sabina and Zataria multiflora extracts with Taxus baccata extract and cisplatin on normal and cancer cell lines. Pharmacogn Mag (2010) 6:102–5. doi: 10.4103/0973-1296.62894
18. Wunderlich F, Al-Quraishy S, Steinbrenner H, Sies H, Dkhil MA. Towards identifying novel anti-Eimeria agents: trace elements, vitamins, and plant-based natural products. Parasitol Res (2014) 113:3547–56. doi: 10.1007/s00436-014-4101-8
19. Dell’Agli M, Galli GV, Corbett Y, Taramelli D, Lucantoni L, Habluetzel A, et al. Antiplasmodial activity of Punica granatum l. fruit rind. J Ethnopharmacol (2009) 125:279–85. doi: 10.1016/j.jep.2009.06.025
20. Iqbal S, Tanveer S, Maqbool N. Avian coccidiosis-a critical review. Mun Ent Zool (2022) 17:1155–75.
21. Haghayeghi K, Shetty K, Labbé R. Inhibition of foodborne pathogens by pomegranate juice. J Med Food (2013) 16:467–70. doi: 10.1089/jmf.2012.0233
22. Juneja VK, Bari ML, Inatsu Y, Kawamoto S, Friedman M. Control of Clostridium perfringens spores by green tea leaf extracts during cooling of cooked ground beef, chicken, and pork. J Food Prot (2007) 70:1429–33. doi: 10.4315/0362-028X-70.6.1429
23. Adhami VM, Mukhtar H. Polyphenols from green tea and pomegranate for prevention of prostate cancer. Free Radic Res (2006) 40:1095–104. doi: 10.1080/10715760600796498
24. Madrigal-Carballo S, Rodriguez G, Krueger CG, Dreher M, Reed JD. Pomegranate (Punica granatum) supplements: authenticity, antioxidant and polyphenol composition. J Funct Foods (2009) 1:324–9. doi: 10.1016/j.jff.2009.02.005
25. Ribeiro-Santos R, Andrade M, Madella D, Martinazzo AP, de Aquino Garcia Moura L, de Melo NR, et al. Revisiting an ancient spice with medicinal purposes: cinnamon. Trends Food Sci Technol (2017) 62:154–69. doi: 10.1016/j.tifs.2017.02.011
26. Rahmani AH, Alsahli MA, Almatroodi SA. Active constituents of pomegranates (Punica granatum) as potential candidates in the management of health through modulation of biological activities. Pharmacogn J (2017) 9:689–95. doi: 10.5530/pj.2017.5.109
27. Takemoto M, Takemoto H. Synthesis of theaflavins and their functions. Molecules (2018) 23:1–18. doi: 10.3390/molecules23040918
28. Park HY, Kunitake Y, Hirasaki N, Tanaka M, Matsui T. Theaflavins enhance intestinal barrier of caco-2 cell monolayers through the expression of AMP-activated protein kinase-mediated occludin, claudin-1, and ZO-1. Biosci Biotechnol Biochem (2015) 79:130–7. doi: 10.1080/09168451.2014.951027
29. Silva ACO, Faria MR, Fontes A, Campos MS, Cavalcanti BN. Interleukin-1 beta and interleukin-8 in healthy and inflamed dental pulps. J Appl Oral Sci (2009) 17:527–32. doi: 10.1590/S1678-77572009000500031
30. Li J, Moran T, Swanson E, Julian C, Harris J, Bonen DK, et al. Regulation of IL-8 and IL-1β expression in crohn’s disease associated NOD2/CARD15 mutations. Hum Mol Genet (2004) 13:1715–25. doi: 10.1093/hmg/ddh182
31. Kogut MH. Dynamics of a protective avian inflammatory response: the role of an IL-8-like cytokine in the recruitment of heterophils to the site of organ invasion by salmonella enteritidis. Comp Immunol Microbiol Infect Dis (2002) 25:159–72. doi: 10.1016/S0147-9571(01)00035-2
32. Lu H, Adedokun SA, Adeola L, Ajuwon KM. Anti-inflammatory effects of non-antibiotic alternatives in coccidia challenged broiler chickens. J Poult Sci (2014) 51:14–21. doi: 10.2141/jpsa.0120176
33. Oh S, Gadde UD, Bravo D, Lillehoj EP, Lillehoj HS. Growth-promoting and antioxidant effects of magnolia bark extract in chickens uninfected or co-infected with clostridium perfringens and Eimeria maxima as an experimental model of necrotic enteritis. Curr Dev Nutr (2018) 2:1–10. doi: 10.1093/cdn/nzy009
34. Yuan M, Peng LY, Wei Q, Li JH, Song K, Chen S, et al. Schizandrin attenuates lung lesions induced by avian pathogenic Escherichia coli in chickens. Microb Pathog (2020) 142:104059. doi: 10.1016/j.micpath.2020.104059
35. Perrier C, Corthésy B. Gut permeability and food allergies. Clin Exp Allergy (2011) 41:20–8. doi: 10.1111/j.1365-2222.2010.03639.x
36. Schoultz I, Keita ÅV. The intestinal barrier and current techniques for the assessment of gut permeability. Cells (2020) 9:1–30. doi: 10.3390/cells9081909
37. Ghosh SS, Wang J, Yannie PJ, Ghosh S. Intestinal barrier dysfunction, LPS translocation, and disease development. J Endocr Soc (2020) 4:1–15. doi: 10.1210/jendso/bvz039
38. Heinemann U. Structural features of tight-junction proteins. Int J Mol Sci (2019) 20(23):6020. doi: 10.3390/ijms20236020
39. Lagha AB, Grenier D. Tea polyphenols protect gingival keratinocytes against TNF-α-induced tight junction barrier dysfunction and attenuate the inflammatory response of monocytes/macrophages. Cytokine (2019) 115:64–75. doi: 10.1016/j.cyto.2018.12.009
40. Sundstrom JM, Tash BR, Murakami T, Flanagan JM, Bewley MC, Stanley BA, et al. Identification and analysis of occludin phosphosites: a combined mass spectrometry and bioinformatics approach. J Proteome Res (2009) 8:808–17. doi: 10.1021/pr7007913
41. Tash BR, Bewley MC, Russo M, Keil JM, Griffin KA, Sundstrom JM, et al. The occludin and ZO-1 complex, defined by small angle X-ray scattering and NMR, has implications for modulating tight junction permeability. Proc Natl Acad Sci U.S.A. (2012) 109:10855–60. doi: 10.1073/pnas.1121390109
42. Rudnicki MA, Le Grand F, McKinnell I, Kuang S. The molecular regulation of muscle stem cell function. Cold Spring Harb Symp Quant Biol (2008) 73:323–31. doi: 10.1101/sqb.2008.73.064
43. Lim CH, Kim CK. The effect of two-week resistance and endurance training on skeletal muscle repair and regeneration after ischemia-reperfusion in rat. Exerc Sci (2017) 26:49–60. doi: 10.15857/ksep.2017.26.1.49
44. Wang Y, Liu Q, Quan H, Kang SG, Huang K, Tong T. Nutraceuticals in the prevention and treatment of the muscle atrophy. Nutrients (2021) 13:1914. doi: 10.3390/nu13061914
45. Lee SH, Lillehoj HS, Jang SI, Lee KW, Park MS, Bravo D, et al. Cinnamaldehyde enhances in vitro parameters of immunity and reduces in vivo infection against avian coccidiosis. Br J Nutr (2011) 106:862–9. doi: 10.1017/S0007114511001073
46. Lee YS, Lee SH, Gadde UD, Oh ST, Lee SJ, Lillehoj HS. Allium hookeri supplementation improves intestinal immune response against necrotic enteritis in young broiler chickens. Poult Sci (2018) 97:1899–908. doi: 10.3382/ps/pey031
47. Kim DK, Lillehoj HS, Lee SH, Jang SI, Lillehoj EP, Bravo D. Dietary Curcuma longa enhances resistance against Eimeria maxima and Eimeria tenella infections in chickens. Poult Sci (2013) 92:2635–43. doi: 10.3382/ps.2013-03095
48. Moraes PO, Cardinal KM, Gouvêa FL, Schroeder B, Ceron MS, Lunedo R, et al. Comparison between a commercial blend of functional oils and monensin on the performance and microbiota of coccidiosis-challenged broilers. Poult Sci (2019) 98:5456–64. doi: 10.3382/ps/pez345
49. Yadav S, Teng PY, Souza dos Santos T, Gould RL, Craig SW, Lorraine Fuller A, et al. The effects of different doses of curcumin compound on growth performance, antioxidant status, and gut health of broiler chickens challenged with Eimeria species. Poult Sci (2020) 99:5936–45. doi: 10.1016/j.psj.2020.08.046
50. Moraes PO, Andretta I, Cardinal KM, Ceron M, Vilella L, Borille R, et al. Effect of functional oils on the immune response of broilers challenged with Eimeria spp. Animal (2019) 13:2190–8. doi: 10.1017/S1751731119000600
51. Oelschlager ML, Rasheed MSA, Smith BN, Rincker MJ, Dilger RN. Effects of Yucca schidigera-derived saponin supplementation during a mixed Eimeria challenge in broilers. Poult Sci (2019) 98:3212–22. doi: 10.3382/ps/pez051
52. Richards LA, Glassmire AE, Ochsenrider KM, Smilanich AM, Dodson CD, Jeffrey CS, et al. Phytochemical diversity and synergistic effects on herbivores. Phytochem Rev (2016) 15:1153–66. doi: 10.1007/s11101-016-9479-8
53. Rizeq B, Gupta I, Ilesanmi J, AlSafran M, Rahman MDM, Ouhtit A. The power of phytochemicals combination in cancer chemoprevention. J Cancer (2020) 11:4521–33. doi: 10.7150/jca.34374
54. Teng PY, Yadav S, Castro FL de S, Tompkins YH, Fuller AL, Kim WK. Graded Eimeria challenge linearly regulated growth performance, dynamic change of gastrointestinal permeability, apparent ileal digestibility, intestinal morphology, and tight junctions of broiler chickens. Poult Sci (2020) 99:4203–16. doi: 10.1016/j.psj.2020.04.031
Keywords: broiler chicken, coccidiosis, Eimeria maxima, growth, gut health, intestinal immunity, phytochemicals, alternatives to antibiotics
Citation: Park I, Nam H, Wickramasuriya SS, Lee Y, Wall EH, Ravichandran S and Lillehoj HS (2023) Host-mediated beneficial effects of phytochemicals for prevention of avian coccidiosis. Front. Immunol. 14:1145367. doi: 10.3389/fimmu.2023.1145367
Received: 18 January 2023; Accepted: 22 May 2023;
Published: 02 June 2023.
Edited by:
Guiju Sun, Southeast University, ChinaReviewed by:
Shawky Mohamed Aboelhadid, Beni-Suef University, EgyptKyung-Woo Lee, Konkuk University, Republic of Korea
Copyright © 2023 Park, Nam, Wickramasuriya, Lee, Wall, Ravichandran and Lillehoj. This is an open-access article distributed under the terms of the Creative Commons Attribution License (CC BY). The use, distribution or reproduction in other forums is permitted, provided the original author(s) and the copyright owner(s) are credited and that the original publication in this journal is cited, in accordance with accepted academic practice. No use, distribution or reproduction is permitted which does not comply with these terms.
*Correspondence: Hyun S. Lillehoj, aHl1bi5saWxsZWhvakB1c2RhLmdvdg==
†Present address: Emma H. Wall, Nutreco Exploration, Nutreco, Amersfoort, Netherlands