- Hepatic Surgery Center, Tongji Hospital, Tongji Medical College, Huazhong University of Science and Technology, Wuhan, Hubei, China
Cholangiocarcinoma (CCA) is a rare and aggressive type of malignant tumor. In the past few years, there has been an increase in the incidence of CCA. Surgery is the only effective treatment but is only suitable for a small percentage of patients. Comprehensive treatment is the normal therapy for terminal CCA patients, depending basically on gemcitabine and cisplatin combination chemotherapy. In the past decade, the emergence of next-generation sequencing technology can be used for the identification of important molecular features of CCA, and several studies have demonstrated that different CCA subtypes have unique genetic aberrations. Targeting fibroblast growth factor receptor (FGFR), isocitrate dehydrogenase (IDH) and epidermal growth factor receptor 2 (EGFR2) are emerging targeted therapies. In addition, researches have indicated that immunotherapy has a key function in CCA. There is ongoing research on programmed cell death protein 1 inhibitors (PD-1), chimeric antigen receptor T cells (CAR-T) and tumor-infiltrating leukocyte (TILs). Researches have shown that targeted therapy, immunotherapy, and conventional chemotherapy in CCA had certain mechanistic links, and the combination of those can greatly improve the prognosis of advanced CCA patients. This study aimed to review the research progress of targeted therapy and immunotherapy for CCA.
1. Introduction
Cholangiocarcinoma (CCA) is the second most common primary liver malignancy, accounting for 3% of digestive tract tumors. The CCA incidence rate ranges from 0.35 to 2 per 100,000 people every year in western countries. However, this incidence rate can be as high as 40 times in China, thereby leading to a major public health concern. CCA is divided into extrahepatic cholangiocarcinoma (eCCA) and intrahepatic cholangiocarcinoma (iCCA), with the former further divided into distal cholangiocarcinoma (dCCA) and perihilar cholangiocarcinoma (pCCA) (1). Although these subgroups differ significantly in prognosis, etiology, biology, and epidemiology (2), surgical treatment is found to be the optimal treatment for localized CCA. iCCA treatment can be performed with hepatectomy based on anatomical conditions, whereas eCCA may be treated with hepatopancreaticoduodenectomy. However, the surgical recurrence rate of CCA can be up to more than 50% despite receiving postoperative adjuvant chemotherapy (3).
Due to the lack of early clinical symptoms, about 75% of CCA patients are identified as metastatic or locally advanced disease at initial diagnosis. Based on the ABC-02 clinical study, cisplatin/gemcitabine (CisGem) is considered the first-line treatment for patients with advanced CCA. According to the ABC-06 study, the standard second-line treatment after CisGem is a fluorouracil plus oxaliplatin (FOLFOX) regimen, however, the objective response rate (ORR) was not statistically significant between the FOLFOX group and the control group (4). Besides, adding nab-paclitaxel (Abraxane) to CisGem did not result in a statistically significant improvement in OS in patients with newly diagnosed advanced CCA in the Phase 3 SWOG 1815 trial (NCT03768414). It is believed that the overall effect of systemic chemotherapy in CCA remains unsatisfactory.
With the enhanced understanding of the molecular pathways in CCA, targeted therapy has currently become one of the most innovative therapeutic approaches, and is primarily used to attack particular genes or proteins playing a crucial part in the carcinogenesis and progression of CCA. In a previous study (5), next-generation sequencing (NGS) was used for mapping CCA, 182 cancer-associated genes and 37 introns were identified from 14 cancer-rearranged genes, which demonstrated that biliary tract tumors share the same chromatin remodeling (ARID1A) and genomic aberrations (CDKN2B). The TME of CCA contains tumor-associated fibroblasts and inhibitory immune components, leading to T cell-mediated rejection, inhibition of anti-tumor immune response, and promotion of tumorigenesis, as well as possibly influencing the mechanism of chemotherapy (6). At present, immune checkpoint inhibitors (ICIs), chimeric antigen receptor T cells (CAR-T), tumor vaccines, and tumor-infiltrating leukocytes (TILs) are the major immunotherapy methods (7–9).
It was hypothesized that targeted therapy, immunotherapy, and conventional chemotherapy had synergistic effects in CCA. A combination of targeted therapy, immunotherapy, and conventional chemotherapy may significantly improve the prognosis of CCA. For example, MEK inhibitor monotherapy had limited efficacy in CCA; however, it can enhance the efficacy of PD-L1 inhibitors (10). In addition, the combination of VEGF inhibitors and ICIs have shown enhanced immune activation, increased tumor destruction, and improved efficacy in preclinical models (11). The present systemic review provided an overview of the studies based on the targeted therapy and immunotherapy for advanced CCA. Furthermore, this study also summarized the current status and potential mechanistic links between targeted therapy and immunotherapy (Figure 1).
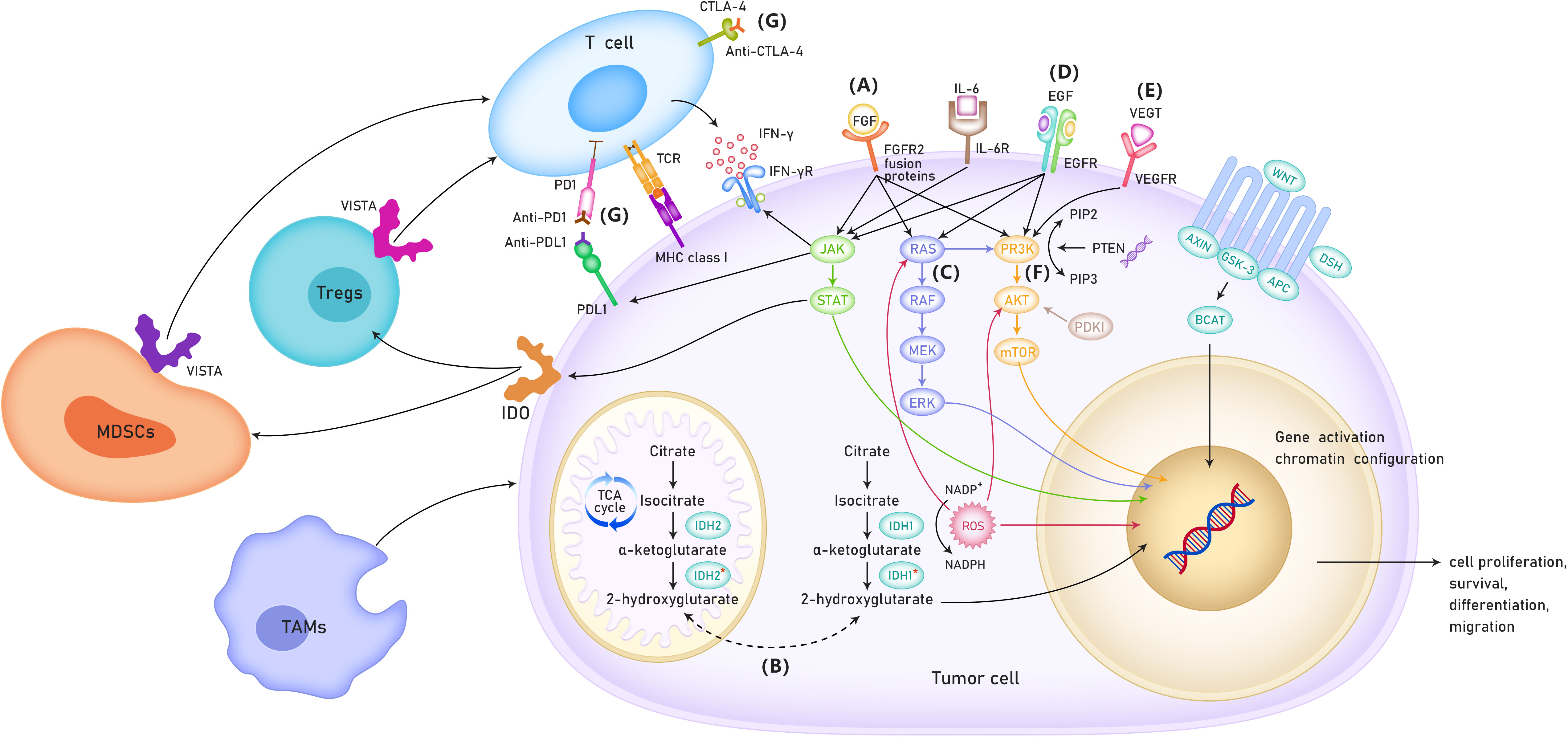
Figure 1 The possible mechanisms of targeted therapy and immunotherapy for cholangiocarcinoma. (A) Role of FGFR in the treatment of cholangio- carcinoma. (B) Role of IDH 1/2 mutation in the treatment of patients with cholangiocarcinoma. (C) RAS/RAF/MEK/ERK signaling pathway. (D) ErbB amplifi- cation. (E) VEGF inhibitors. (F) PI3K/AKT/mTOR signaling pathway. (G) Immune checkpoint inhibitors. TCA cycle, TriCarboxylic Acid cycle; VISTA, V-type immunoglobulin domain-containing suppressor of T cell activation; MDSCs, Myeloid-derived suppressor cells; TAMs,tumor-associated macro- phages; IDO, Indoleamine-2,3-Dioxygenase.
2. Targeted therapy
Molecular targets such as FGFR2 fusion, IDH1/2 mutation and HER2 amplification are proposed to be utilized for targeted therapies in CCA, but a majority of them are generally under clinical investigation (Table 1).
2.1. FGFR
The FGFR family of transmembrane receptors has five members (FGFR1–5). FGFR1–4 involve an endocellular tyrosine kinase domain, which triggers signaling via multiple pathways when activated, including PI3K/AKT/mTOR, RAS-Raf-MEK-ERK and JAK/STAT signaling pathways which are closely associated with cell proliferation, differentiation, migration, and angiogenesis (54). In addition to the point mutations and gene amplification, the most common genetic alterations of FGFRs in CCA are FGFR gene fusions/rearrangements, mainly in iCCA (55). Farther sequencing researches revealed that FGFR2 fusions were observed in about 14% of iCCA patients, and were generally mutually exclusive with IDH mutations (56).
In a sequencing study involving 115 CCA patients, the mutations in FGFR2 were the most common (6.1%) compared with those in FGFR1 (0.9%) and FGFR3–5 (0%). Although tyrosine kinase inhibitors (TKI) targeting FGFR2 have recently emerged, patients with FGFR2 fusions seem to be the unique group to respond to such inhibitors (12, 23). To date, FGFR2 fusion genes contain FGFR2-AHcyL1, FGFR2-BICC1, FGFR2-MGEA5, FGFR2-PPHLN1, and FRGR2-TACC3 (57). The fusion proteins lead to morphological changes in the cells followed by abnormal cell proliferation. Specific FGFR depressants, such as infigratinib, erdafitinib, pemigatinib, futibatinib, and Debio 1347, reversibly combine with cysteine residues in the P-loop region of the adenosine triphosphate (ATP) pocket (58). Specific FGFR inhibitors have demonstrated clinical efficacy in patients with advanced CCA who are FGFR-fusion-positive. Pemigatinib and infigratinib have been approved by the FDA for use in CCA patients with FGFR2 fusions after prior treatment with standard chemotherapy (59). Pemigatinib, a specific FGFR1/2/3 inhibitor, was the first drug to be authorized for the therapy of terminal CCA (60). In a multicenter phase II clinical study (FighT-202), the ORR was 35.5% within the FGFR2 fusion/rearrangement subgroup and in patients with other FGF/FGFR alterations (12). This study demonstrated the potential therapeutic value of pemigatinib for CCA patients with FGFR2 fusion/rearrangement.
An efficacy study of a second FGFR1/3 inhibitor, infigratinib, was presented at the 2018 ESMO Congress (14). In this study, advanced iCCA patients with FGFR2 gene fusions resistant to standard chemotherapy received oral infigratinib. The preliminary results from that study indicated that the ORR was 31.0% and the certain ORR (cORR) was 26.9%. Moreover, the most common grade 3/4 adverse events (AEs) were hypophosphatemia (14.1%), hyperphosphatemia (12.7%), and hyponatremia (11.3%). This study demonstrated that infigratinib could be used as a therapeutic agent for FGFR2-fused iCCA patients (14). Similarly, a phase II study of gemcitabine-resistant FGFR2 fusion/mutation/amplification in patients with terminal CCA demonstrated controlled toxicity and significant clinical activity with infigratinib (15). In another study, infigratinib was investigated as a first-line treatment in patients with FGFR2-positive advanced CCA in a phase III trial (PROOF 301) (17). Such patients were randomly divided in a 2:1 ratio to accept infigratinib or cisplatin plus gemcitabine. This ongoing study may provide evidence for the first-line FGFR2-targeted treatment in patients with advanced CCA (17).
Another targeted agent investigated for patients with FGFR-positive CCA was derazantinib (61). Apart form prohibiting FGFR, the medicine also blocks other kinases, such as KIT, VEGFR1 and DDR. Mazzaferro et al. evaluated derazantinib in the non-blind phase I/II tests. This study enrolled 29 iCCA patients who did not accept chemotherapy or were not sensitive to chemotherapy. Derazantinib administration resulted in an OS of 20.7% and a DCR of 82.8% (19). Moreover, derazantinib demonstrated significant antitumor activity and controlled toxicity.
A phase IIa study from Asia enrolled 22 CCA patients who received erdafitinib, which is an inhibitor of FGFR1/2/3/4. The ORR, mPFS, and mOS were 40.9%, 5.6 mo, and 40.2 mo, respectively (11). These results indicated that erdafitinib demonstrated significant efficacy and safety in the treatment of advanced CCA with FGFR mutations/fusions. A phase II, open-label, multicenter study indicated that futibatinib, a highly selective and irreversible FGFR1/2/3/4 inhibitor, significantly improved clinical outcomes for the advanced iCCA patients with FGFR2 gene fusion-rearrangement progressing after one or more previous lines of systemic therapy (27).
FGFR2 monotherapy was demonstrated to be more effective and less toxic than conventional chemotherapy and could be regarded as a second-line therapy for terminal CCA. Therefore, it is necessary to routinely evaluate the FGFR fusion/rearrangement in CCA patients. There are several treatments for the side effects of FGFR inhibitors, dietary modification(plant-derived food) and phosphate lowering therapies(phosphate binders and phosphaturic agents) can lower hyperphosphatemia, optimize nutrition and sleep are necessary for relieving fatigue, fluids intake and probiotics supplementcan improve diarrhea symptoms (62).
2.2. IDH 1/2 mutations
Among the three subtypes of IDH, IDH1 and IDH2 have significant carcinogenic effects. IDH 1/2 is a protease participated in DNA transcription and reestablish. Generally, this protease facilitates the transformation of isocitrate to α-ketoglutarate. Nevertheless, mutations in this gene may cause epigenetic alterations such as enhanced production of 2-hydroxyglutarate (a tumor metabolite), thereby resulting in DNA disruption and histone methylation.
The mutations in the IDH 1/2 gene exist in around 20–25% of iCCA patients and are virtually absent in patients with other CCA subtypes (63). A 2019 research indicated that IDH1 mutations were present in 13% of the 4214 iCCA patients and 0.8% of the 1123 eCCA patients (64). The IDH-1 mutations are more common than IDH-2 mutations and typically appear in non-hepatitis CCA patients (65).
In a phase III trial (ClarIDHy), ivosidenib, an IDH 1/2 inhibitor, was found to be more effective than a placebo in advanced CCA patients with IDH 1/2 mutations (29). The mPFS improved significantly in the ivosidenib (experimental) group (2.7 mo) compared with the placebo group (1.4 mo). The mOS was 10.3 mo in the ivosidenib group and 7.5 mo in the placebo group (p = 0.09). The most familiar grade 3/4 AE in the ivosidenib group was ascites (7%). Therefore, ivosidenib was authorized by the FDA as a updated treatment for chemotherapy-resistant iCCA patients with IDH 1/2 mutations (66).
2.3. RAS-RAF-MEK–ERK pathway
The RAS-RAF-MEK-ERK pathway is the primary signal transduction constituent of the mitogen-activated protein kinase (MAPK) cascade. The activated RAS initiates a downstream phosphorylation cascade, including RAF protein kinase, MEK1/2 kinase, and ERK1/2 kinase, which transmits signals from the intracellular membrane to the nucleus. The nuclear effectors regulate cell proliferation, differentiation, and apoptosis. Thus, the dysregulation of the RAS-Raf-MEK-ERK pathway is often the result of genetic alterations, which can lead to tumorigenesis.
KRAS mutations have been found in all the CCA subtypes but in different proportions and are associated with poorer prognosis (67). Effective RAS inhibitors have not been prescribed for decades (68), and therapies have centered on inhibiting the downstream components of RAS kinase. The most potent activators of this pathway are alterations in the BRAF gene, most commonly caused by a substitution of valine for glutamate (V600E). This mutation occurs at a lower rate (1–6%) in patients with CCA, especially iCCA (55). A recent study involving 54 iCCA patients with a V600E point mutation showed that this mutation was related to advanced TNM stage, chemoresistance, and worse life expectancy (69). However, as BRAF inhibitor monotherapy demonstrated limited efficacy in clinical use, combination therapy should be applied. A phase II trial evaluated the combination of the BRAF inhibitor dabrafenib and the MEK inhibitor trametinib in 178 tumor patients with V600E mutations, involving 33 patients with terminal CCA (31). The ORR was 41%, mPFS was 7.2 mo, and mOS was 11.3 mo. The patients experienced three grade 3/4 AEs, including elevated γ-glutamyltransferase (9%) and decreased white blood cell count (9%). Dabrafenib plus trametinib demonstrated promising efficacy with a favorable safety profile in BRAF V600E mutation-positive CCA patients.
In a phase II trial involving 28 advanced CCA patients, the MEK inhibitor selumetinib demonstrated promising clinical activity, with an ORR of 12%, mPFS of 3.7 mo, and mOS of 9.8 mo. The most common grade 3/4 AE was fatigue (4%) (32). Ulixertinib, the emerging ERK 1/2 depressor, has proved promising clinical effectivity in patients with MAPK-driven terminal tumors (35).
2.4. NTRK
NTRKs are a group of tyrosine kinases comprising of three portions (NTRK-1/2/3) that contribute to tumorigenesis in a large number of cancer patients through oncogenic fusion resulting in structural activation (70). Such fusions have been reported in CCA (36), with a chimeric NTRK gene spotted in 3.5% of the iCCA patients. The NTRK gene encodes the tropomyosin receptor kinase (TRK) receptor, which is activated by the chimeric gene for the promotion of differentiation, proliferation, and survival of cancer cells. Larotrectinib, a TRK receptor inhibitor, was currently approved by the FDA and EMA (37). In a phase II trial,55 patients with TRK fusion-positive advanced eCCA were administered larotrectinib. The ORR was found to be 75% (38).
2.5. HER
The four members of the epidermal growth factor receptor family (HER1/2/3/4) are tyrosine kinases receptors that are activated by diverse ligands to become homodimers or heterodimers. EGFR overexpression significantly enhanced the incidence of microvascular infiltration, lymph node metastasis, and perineural invasion (71). EGFR and HER2 overexpression are considered to be independent poor prognostic factors in CCA (72). The downstream pathways activated by the EGFR family members include RAS-RAF-MEK-ERK, PI3K/AKT/mTOR, and JAK/STAT (73). The expression of the EGFR pathway is prevalent in CCA, and a previous study demonstrated that it was expressed in 100% iCCA, 52.6% eCCA, and 38.5% GBC cases (74).
California Consortium conducted a phase II study of lapatinib, a dual HER2 and EGFR inhibitor, indicating lapatinib is well tolerated and effective in HCC patients but demonstrated minimal effect in CCA patients (42). Another phase II study evaluated the efficacy and safety of lapatinib in 25 advanced CCA patients (43). No somatic mutations in EGFR or HER2/neu were observed in enrolled patients. Lapatinib was well tolerated but failed to demonstrate significant efficacy as a monotherapy for CCA, indicating that targeting HER2 did not seem to be an effective approach for CCA. However, another study involving 14 GBC patients with HER2 overexpression or mutation as well as one eCCA patient demonstrated significant clinical activity with the targeted use of HER2 inhibitors (75).
Heterodimers containing HER2 are highly potent signal transducers. HER2 (ERBB2) mediates its signal transduction via MAPK and PI3K pathways. HER2 mutations are observed in approximately 5–15% of CCA cases, most commonly in GBC and eCCA (76). The potential molecular targets include HER2 alterations, HER2 amplification, and HER2 overexpression. The targeted therapy with anti-HER2 agents such as pertuzumab and trastuzumab has significantly improved the prognosis of CCA patients (44, 46).
MyPathway, multicenter phase IIa, prospective trial, evaluated the efficacy of pertuzumab + trastuzumab-targeted therapy in 39 advanced CCA patients with HER2 amplification and/or overexpression (44). The results demonstrated that the combination of pertuzumab and trastuzumab was effective and well tolerated. Another phase II SUMMIT trial evaluated the efficacy and safety of neratinib, an irreversible HER1/2/4 inhibitor, in 25 advanced CCA with HER2 mutations. The ORR was 12%, mPFS was 2.8 mo, and mOS was 5.4 mo. The most common treatment-related AEs were diarrhea (56%) and vomiting (48%) (45). In a retrospective study (46), the efficacy and safety of trastuzumab, lapatinib, and pertuzumab were analyzed in patients with HER2-positive advanced GBC/CCA. HER2 amplification or overexpression was observed in 8 patients in the GBC group, with stable disease (n = 3), partial response (PR) (n = 4), or complete response (n = 1). These results indicated that HER2 inhibition is a promising therapeutic strategy for GBC patients with HER2 gene amplification and deserves further exploration.
2.6. VEGF-TKI
Angiogenesis plays an important part in maintaining tumor survival, and the microvessel density (MVD) measurements indicate high vascularization in eCCA. High MVD is associated with higher lymph node diffusivity and postoperative local recurrence rates, thereby indicating poor prognosis of eCCA and other CCA subtypes. The vascular endothelial growth factor (VEGF) family and its receptors not only mediate tumor invasion by affecting angiogenesis and vascular permeability but also mediate tumorigenesis through the cell signal transduction pathway. The VEGF expression is correlated with tumor grade, invasion ability, and prognosis.
The VEGF inhibitors include VEGF monoclonal antibodies (mAbs) and multireceptor TKIs. Bevacizumab binds and neutralizes VEGF-A ligands and has little benefit as a monotherapy because of commutative activation mechanisms, that attenuate the antiangiogenic activity of VEGF inhibition. Nevertheless, TKIs can not only block multiple signaling pathways, including angiogenesis but are also directly involved in tumor growth.
Sorafenib, a multikinase inhibitor of VEGFR-2/3, PDGFR-β, b-RAF, and c-Raf, has demonstrated activity in preclinical models of CCA. In a phase II trial conducted by Bengala et al. (48) involving 46 advanced CCA patients, administration of single-agent sorafenib demonstrated less efficacy with manageable adverse reactions. Another phase II study evaluated the safety and efficiency of sunitinib as a second-line therapy for 56 advanced CCA patients with prior treatment with chemotherapy. The results from that study indicated that the median time to progression (mTTP) was 1.7 mo and the ORR and DCR were 8.9% and 50.0%, respectively. The most common grade 3/4 toxic side effects were neutropenia and thrombocytopenia (21.4%) (49).
2.7. PI3K/AKT/mTOR
The PI3K/AKT/mTOR signaling pathway plays an important part in cell proliferation and survival and participated in the development and progression of CCA. In a study (77) involving 88 CCAs (66% eCCA), activated mTOR was found to be a negative prognostic indicator (78). In addition, the upregulation of mTOR downstream effectors is common in CCA.
In a phase II trial (53) involving 39 advanced CCA patients who had received prior chemotherapy, the mTOR inhibitor everolimus demonstrated promising anti-tumor efficacy with tolerable toxic and side effects. Furthermore, the RADiChol study involving 27 advanced CCA patients indicated that everolimus as first-line monotherapy demonstrated promising clinical efficacy.
2.8. PARP
Poly ADP-ribose polymerase (PARP) depressants are used in the treatment of tumors with homologous repair defects (HRD) for targeting tumor cells with DNA damage repair (DDR) defects. The lack of a functional PARP enzyme results in DNA double-stranded breaks and consequent cell death. The alterations in several DDR genes may contribute to HRD, especially IDH1 and IDH2 mutations, most commonly observed in eCCA (79). Multiple phase II trials are underway for evaluating the PARP depressants in CCA, including monotherapy(NCT04042831, NCT03207347, NCT03212274) and combination with PD-1/PD-L1 inhibitors(NCT04895046, NCT04306367) or temozolomide(NCT04796454), demonstrating considerable efficacy.
Besides, Dr. Furuse et al. discussed another targeted drug, Nanvuranlat, an L-type amino acid transporter (LAT1) inhibitor for patients with pretreated advanced refractory CCA in ASCO GI 2023, showing that it has certain therapeutic effect.
3. Immunotherapy
Based on the immune microenvironment (TME) of CCA, the latest approaches to immunotherapy for CCA patients, including preclinical and clinical studies of immune checkpoint inhibitors (ICIs), cancer vaccines, and adoptive cell therapy are summarized in Table 2.
3.1. Immune checkpoint inhibitors
Studies have indicated PD-L1 expression in about half of the CCA patients, which is associated with poor prognosis (7). As studies have revealed that ICCs such as PD-1/PDL1 and CTLA-4 adjust antitumoral immune reactions, particular depressants against these targets have been exploited and used in anticancer therapy.
A multicenter phase II study involving 54 patients evaluated the efficacy and safety of nivolumab monotherapy in advanced CCA patients after the first- to third-line of treatment (7). The results revealed that the ORR was 22% and DCR 59%. In addition, the mPFS and mOS were 3.68 mo and 14.24 mo, respectively. They further indicated that the PD-L1 expression in tumors was positive in all the patients who responded to treatment and was associated with prolonged PFS. Similarly, a multicenter retrospective study was conducted for evaluating the clinical efficacy and safety of pembrolizumab in CisGem-refractory CCA patients (82). 51 advanced CCA patients who were PD-L1-positive after progression on first-line CisGem progression were enrolled and administered pembrolizumab. The ORR was 9.8%, mPFS was 2.1 mo, and OS was 6.9 mo. The grade 3/4 AEs occurred in only 4 patients (7.8%).
A phase I study evaluated the safety and tolerability of durvalumab (anti-PD-L1 antibody) and tremelimumab (anti-CTLA-4 antibody) in advanced CCA patients who had progressed on previous therapy (84). PR was existed in 2 patients in the durvalumab group and 7 patients in the durvalumab plus tremelimumab group. The mPFS and mOS were 8.1 mo and 10.1 mo, respectively. That study demonstrated that both durvalumab monotherapy and durvalumab plus tremelimumab combination therapy can be tolerated, and demonstrated good clinical efficacy.
Both germline mutations due to DNA mismatch repair (MMR) and somatic hypermethylation of the MLH1 promoter can lead to MMR defects (dMMR), which, in turn, can lead to MSI-H status in Lynch syndrome patients (89). The somatic mutation rates of MSI-H cancers are one to two orders of magnitude higher than those of microsatellite-stable cancers, with enhanced neoepitope production, intense lymphocytic infiltration, and a better prognosis, indicating that the PD-1/PD-L1 inhibitors can be used in the treatment of MSI-H cancers (90). MSI-H rarely occurs in patients with CCA and is more common in patients with eCCA. In a Japanese study (91), 13.2% of 38 eCCA patients had MSI-H. There have been several reports of partial responses in MSI-H CCA patients received with the PD-1 inhibitor pembrolizumab, including one study in eCCA patients that lasted more than 13 months (92).
In the KEYNOTE-158 trial involving 233 patients with 27 tumor types (endometrial, gastric, cholangiocarcinoma, and pancreatic cancer), including 22 patients with advanced CCA, pembrolizumab was used in pretreated MSI-H/dMMR cancer patients. The ORR was 34.3%, mPFS was 4.1 mo, and mOS was 23.5 mo. The grade 3–5 treatment-related AEs occurred in 34 patients (14.6%) (93). The studies have indicated that pembrolizumab had significant clinical benefits and manageable toxicity in previously treated advanced MSI-H/dMMR non-colorectal cancer patients. The FDA approved pembrolizumab as a second-line therapy for patients with advanced MSI-H/dMMR cancer (88).
3.2. Tumor-infiltrating leukocyte
The future role of immunotherapy in ICC is still unknown. A study involving 68 resectable ICC patients who underwent complete resection was conducted for evaluating the predictive value of TILs on the OS and PFS in patients. TIL was an independent negative predictor of poor OS and poor PFS. Low TIL was associated with high levels of CA125 and CA19-9 (94).
In a study conducted to analyze the tissue characteristics of tumor-invasive CD8+ T cells in ICC patients (95), blood and tissue samples were collected from 33 HCC patients. The results indicated that CD69+ CD103+ TRM-like CD8+ TILs represent significant tumor-specific immune responses. It is expected to be a potential therapeutic target in ICC patients.
3.3. Cancer vaccines
If the tumor cells are considered a “pathogen” with tumor-specific antigens, a vaccine injected with these antigens can stimulate the immune system and facilitate the differentiation of memory T cells, thereby increasing the anti-tumor immune anility. Several types of cancer vaccines, including single peptidyl, polypeptidyl, and dendritic cell (DC) vaccines, have been developed. However, a retrospective study involving advanced CCA patients demonstrated a PR of 6%, stable disease (SD) of 23%, and ORR of only 6% (8). It is speculated that weak anti-tumor responses of tumor vaccines are observed in CCA because of TME-regulated immunosuppression. Therefore, merely a fraction of T cells sensitive to the vaccine. Moreover, activatory T cells have trouble in tumor penetration as a result of the T-cell rejection mechanism described above. Therefore, the association of ICIs and tumor vaccines may represent the future of cancer vaccines.
3.4. Adoptive immunotherapy
Since the TME of CCA can inhibit T cell activity and evade the immune system, artificial injection of invasive cancer-specific T cells into the tumors may provide a novel approach for antitumor therapy. Fourth-generation anti-CD133CAR T cells demonstrated promising effectiveness against CD133-expressing CCA cells (96). Feng et al. showed an advanced CCA patient who was treated with EGFR and CD133-directed CAR-T cocktail of immunotherapy (9). A PR of 8.5 mo was achieved with EGFR-targeted CAR-T cells and 4.5 months with CART-133. A phase I trial of EGFR-specific CAR-T cells for the treatment of EGFR-positive terminal CCA demonstrated promising outcomes as well, with 58.8% achieving SD and 5.8% of patients achieving a CR (89). However, considering the toxicity and endothelial damage caused by CAR-T cells, further researches are necessary to demonstrate the safety and effectiveness of this treatment.
3.5. Oncolytic viruses
The oncolytic virus (OV) therapy infects and kills cancer cells through the natural or acquired ability of the viruses. In the past few years, in-depth research on viral gene function and molecular biology has meaningfully advanced the effectiveness and safety of OVs. The recruitment of T cells and the induction of tumor-reactive immunity form the basis of OV therapy. The researches have showed that OVs can enhance the immune system response after infecting the tumor cells. Currently, Talimogene laherparepvec is the merely OV authorized in the United States (97). Furthermore, the application of OV therapy in association with the inhibitors of the transforming growth factor-beta signal pathway could facilitate the effectiveness of immunotherapy. The objectives of the OV therapy include enhancing the alternative duplication power of OVs, selecting an efficient mode of OV infection, finding a equilibrium between the antiviral and the antitumor immunity, removing immunosuppressive TME, and increaseing the oncolytic effect. The oncolytic roles of a measles vaccine virus and three survitin-based conditionally replicating adenoviruses for CCA treatment were demonstrated in a preclinical study (98).
4. Combination therapy
4.1. Combination of targeted therapy and chemotherapy
In the past few years, several studies aim to evaluate the efficacy of the combination of targeted therapy and chemotherapy. However, these studies have shown contrasting results. ABC-04 study (33) enrolled 8 advanced CCA patients who received selumetinib in combination with CisGem as the first-line therapy. Among these patients, 3 had a PR and 5 had stable disease, with an mPFS of 6.4 mo. Subsequently, the BIL-MEK study involved 57 advanced CCA patients who were previously untreated. This study evaluated the efficacy of a sequential combination of selumetinib followed by CisGem. However, irrespective of the dosing regimen, this combination did not improve the tumor response at 10 weeks or prolong survival but instead increased toxicity (34).
A randomized phase III study comparing gemcitabine plus oxaliplatin (GEMOX) plus erlotinib versus GEMOX as the first-line therapy in advanced CCA patients (39). Distinct differences were not observed in the PFS and mOS between the two groups (p = 0.087; 0.611 respectively). The ORR of the GEMOX plus erlotinib group was higher than that of the GEMOX group (29.6% vs 15.8%; p = 0.005). The most common grade 3/4 AE was febrile neutropenia. GEMOX plus erlotinib demonstrated significant antitumor activity and could be used as a therapy modality in terminal CCA patients.
In a phase II research (40) involving 30 terminal CCA patients, cetuximab plus GEMOX was used as the first-line therapy. The results revealed that mPFS was 8.3 mo, OS was 12.7 mo, and ORR was 63% (complete response (CR) was 10%). Moreover, no grade 4 AEs occurred in that study. These results indicated that cetuximab plus GEMOX was well tolerated and demonstrated significant antitumor activity. Another randomized clinical trial (RCT) investigated the efficacy of cetuximab combined with chemotherapy as first-line therapy in advanced CCA patients, and the results demonstrated that the combination of GEMOX and cetuximab did not enhance the efficacy of chemotherapy when compared with GEMOX monotherapy in advanced CCA patients, although it was well tolerated (41).
In a recent phase II trial (51), the PI3K suppressant copanlisib was applied as second-line therapy in association with CisGem in advanced CCA patients. The results implied that mPFS and mOS were 6.2 mo and 13.7 mo, respectively. Some studies investigated the efficacy of the VEGF inhibitors in combination with GEMOX therapy for advanced CCA patients and showed little evidence of any benefit (47) (50). Presently, a phase III study of Fight-302 comparing pemigatinib with gemcitabine plus cisplatin (CisGem) as a first-line treatment for advanced CCA with FGFR2 gene rearrangement is in progress(NCT03656536). The primary endpoint of this study is PFS, and the secondary endpoints are ORR, OS, and DCR (13).
4.2. Combination of immunotherapy and chemotherapy
In a multicenter phase I study conducted in Japan, the safety and tolerability of the PD-1 ICI nivolumab alone or in combination with chemotherapy were evaluated in advanced CCA (80). Thirty patients were enrolled in each group, with advanced CCA patients who had progressed on previous gemcitabine therapy receiving nivolumab monotherapy and advanced CCA patients without prior treatment with chemotherapy receiving nivolumab plus Cis plus Gem. In the nivolumab group, mOS was 5.2 mo and mPFS was 1.4 mo. In the nivolumab plus CisGem group, mOS was 15.4 mo and mPFS was 4.2 mo. The most common side effects in the nivolumab group were decreased appetite (17%), fatigue (13%), and pruritus (13%). In the nivolumab plus CisGem group, the most common side effects were a decrease in neutrophil count (83%) and platelet count (83%). The results indicated that nivolumab demonstrated significant clinical efficacy and controllable toxic and side effects.
Topaz-1 was the first global phase III study evaluating first-line durvalumab plus CisGem in advanced CCA treatment (85). In this double-blind study, 685 advanced CCA patients who had no prior treatment were randomly assigned 1:1 to receive durvalumab plus CisGem (n = 341) or placebo plus CisGem (n = 344). The OS and PFS were significantly improved with durvalumab plus CisGem compared with placebo plus CisGem. The grade 3/4 AEs occurred in 62.7% of patients of the durvalumab group and 64.9% of patients of the placebo group, indicating that durvalumab plus CisGem may become a new first-line standard of care. At present, a phase III randomized KEYNOTE-966 study is in progress for evaluating the effect of pembrolizumab under the same conditions (NCT04003636).
A single-center phase II study involving 128 advanced ICC patients who had not received chemotherapy evaluated gemcitabine and cisplatin plus durvalumab with or without tremelimumab as the first-line treatment in patients with advanced biliary tract cancer. The results from that study indicated that the mOS was 18.1 mo in the gemcitabine plus durvalumab group and 20.7 mo in the gemcitabine plus durvalumab plus tremelimumab group. The combination of gemcitabine, durvalumab, and tremelimumab seems to be a better first-line treatment (99).
4.3. Combination of immunotherapy and targeted therapy
The MEK inhibitor monotherapy has limited efficacy in CCA, but it is considered to enhance the effect of PD-L1 inhibitors. Recently, a randomized, multicenter phase II study indicated that a association of atezolizumab (anti-PD-L1 inhibitor) and cobimetinib (MEK inhibitor) was effective in advanced CCA patients with prior first-line or second-line treatment. The mPFS was 3.65 mo in the combination group and 1.87 mo in the single drug group. PR was achieved in only one patient (3.3%) in the combination group and one patient (2.8%) in the single drug group. These results indicated that the combination of drugs could lead to greater clinical benefits. However, the combination group was characterized by more rashes, gastrointestinal events, elevated CPK, and thrombocytopenia (10). Therefore, additional phase III studies comparing ICIs with standard chemotherapy are needed.
4.4. Combination of immunotherapy, targeted therapy and chemotherapy
A phase 2 clinical study presented at the Annual Meeting of ESMO in 2020, evaluated the efficacy of a combination of PD-1 inhibitor (toripalimab), target drug (lenvatinib), and GEMOX as the first-line treatment of advanced and unresectable ICC, and obtained excellent results. The ORR was up to 80% and DCR was up to 93.3%, and the 6-month survival rate was as high as 90%. Most of the patients in that study had tumor shrinkage, indicating that more inoperable patients can become operable, which provides a novel idea for the conversion therapy of advanced ICC.
Based on data presented during the Gastrointestinal Cancers Symposium 2023, the Phase 2 IMbrave 151 trial (NCT04677504) found that in patients with advanced CCA, treatment with or without bevacizumab in combination with atrilizumab and CisGem had modest clinical benefits (86).
5. Conclusions and prospect
Research on the therapies for CCA is rapidly evolving. However, some important questions regarding the treatment for CCA still remain to be addressed. First, the efficacy of targeted therapies is largely limited by acquired resistance, such as the occurrence of secondary polyclonal mutations (20, 21). However, liquid biopsies can be applied for tracking the emergence of polyclonal mutations and guiding medication (100). Second, the use of a safe and effective combination of chemotherapy, targeted therapy, and immunotherapy still needs to be evaluated (12, 23). Third, the lack of specific predictive and reactive biomarkers has led to studies on genetic screening and microenvironmental testing. The comprehensive mechanisms governing CCA are complex. In addition to the above-mentioned drug action pathways, DNA damage repair (DDR), p53, and MDM2 oncoprotein have also been evaluated as therapeutic options in recent studies. Therefore, further larger clinical studies are necessary to validate the safety and effectiveness of different CCA treatment options. Targeted therapy and immunotherapy have demonstrated promising results, and these drugs are expected to be used as first-line therapies in the future.
Author contributions
EZ contributed to the conception of the study, JD contributed significantly to analysis and manuscript preparation, XL and ZZ performed the data analyses and wrote the manuscript, ZH and EZ helped perform the analysis with constructive discussions. All authors contributed to the article and approved the submitted version.
Funding
This study was supported by Tongji Hospital, Tongji Medical College, Huazhong University of Science and Technology.
Acknowledgments
Writing and editing of the manuscript were assisted by Z-YH and E-LZ.
Conflict of interest
The authors declare that the research was conducted in the absence of any commercial or financial relationships that could be construed as a potential conflict of interest.
Publisher’s note
All claims expressed in this article are solely those of the authors and do not necessarily represent those of their affiliated organizations, or those of the publisher, the editors and the reviewers. Any product that may be evaluated in this article, or claim that may be made by its manufacturer, is not guaranteed or endorsed by the publisher.
Abbreviations
FGFR, fibroblast growth factor receptor; ORR, object response rate; PFS, progression-free survival; cORR, certain objective response rate; mDOR, median duration of response; DCR, disease control rate; IDH1/2, Isocitrate dehydrogenase ½; PR, partial response; NTRK, neurotrophin receptor kinase; CBR, clinical benefit rate; TTP, time to progression; OS, overall survival; CART, chimeric antigen receptor T cell.
References
1. Rizzo A, Brandi G. BILCAP trial and adjuvant capecitabine in resectable biliary tract cancer: Reflections on a standard of care. Expert Rev Gastroenterol Hepatol (2021) 15(5):483–5. doi: 10.1080/17474124.2021.1864325
2. Rizvi S, Gores GJ. Emerging molecular therapeutic targets for cholangiocarcinoma. J Hepatol (2017) 67(3):632–44. doi: 10.1016/j.jhep.2017.03.026
3. Javle MM, Roychowdhury S, Kelley RK, Sadeghi S, Macarulla T, Waldschmidt DT, et al. Final results from a phase II study of infigratinib (BGJ398), an FGFR-selective tyrosine kinase inhibitor, in patients with previously treated advanced cholangiocarcinoma harboring an FGFR2 gene fusion or rearrangement. J Clin Oncol (2021) 39:265. doi: 10.1200/JCO.2021.39.3_suppl.265
4. Feng Y-H, Su W-C, Oh D-Y, Shen L, Kim KP, Liu X, et al. Updated analysis with longer follow up of a phase 2a study evaluating erdafitinib in Asian patients (pts) with advanced cholangiocarcinoma (CCA) and fibroblast growth factor receptor (FGFR) alterations. J Clin Oncol (2022) 40:430. doi: 10.1200/JCO.2022.40.4_suppl.430
5. Ross J, Wang K, Catenacci D, Siraj Chmielecki J, Ali M, Elvin J, et al. Comprehensive genomic profiling of biliary tract cancers to reveal tumor-specific differences and genomic alterations. J Clin Oncol (2015) 33(suppl; abstr 231). doi: 10.1200/jco.2015.33.3_suppl.231
6. Rizvi S, Khan SA, Hallemeier CL, Kelley RK, Gores GJ. Cholangiocarcinoma-evolving concepts and therapeutic strategies. Nat Rev Clin Oncol (2018) 15:95–111. doi: 10.1038/nrclinonc.2017.157
7. Xie Q, Wang L, Zheng S. Prognostic and clinicopathological significance of PD-L1 in patients with cholangiocarcinoma: A meta-analysis. Dis Markers (2020) 2020:1817931. doi: 10.1155/2020/1817931
8. Kobayashi M, Sakabe T, Abe H, Tanii M, Takahashi H, Chiba A, et al. DC-Vaccine study group at the Japan society of innovative cell therapy (J-SICT). dendritic cell-based immunotherapy targeting synthesized peptides for advanced biliary tract cancer. J Gastrointest Surg (2013) 17(9):1609–17. doi: 10.1007/s11605-013-2286-2
9. Feng KC, Guo YL, Liu Y, Dai HR, Wang Y, Lv HY, et al. Cocktail treatment with EGFR-specific and CD133-specific chimeric antigen receptor-modified T cells in a patient with advanced cholangiocarcinoma. J Hematol Oncol (2017) 10(1):4. doi: 10.1186/s13045-016-0378-7
10. Yarchoan M, Cope L, Anders RA, Noonan A, Goff LW, Goyal L, et al. Multicenter randomized phase II trial of atezolizumab with or without cobimetinib in biliary tract cancers. J Clin Invest (2021) 131(24):e152670. doi: 10.1172/JCI152670
11. Taylor MH, Schmidt EV, Dutcus C, Pinheiro EM, Funahashi Y, Lubiniecki G, et al. The LEAP program: lenvatinib plus pembrolizumab for the treatment of advanced solid tumors. Future Oncol (2021) 17(6):637–48. doi: 10.2217/fon-2020-0937
12. Abou-Alfa GK, Sahai V, Hollebecque A, Vaccaro G, Melisi D, Al-Rajabi R, et al. Pemigatinib for previously treated, locally advanced or metastatic cholangiocarcinoma: a multicentre, open-label, phase 2 study. Lancet Oncol (2020) 21(5):671–84. doi: 10.1016/S1470-2045(20)30109-1
13. Bekaii-Saab TS, Valle JW, Van Cutsem E, Rimassa L, Furuse J, Ioka T, et al. FIGHT-302: first-line pemigatinib vs gemcitabine plus cisplatin for advanced cholangiocarcinoma with FGFR2 rearrangements. Future Oncol (2020) 16(30):2385–99. doi: 10.2217/fon-2020-0429
14. Javle M, Kelley RK, Roychowdhury S, Weiss KH, Abou-Alfa GK, Macarulla T, et al. Updated results from a phase II study of infigratinib (BGJ398), a selective pan-FGFR kinase inhibitor, in patients with previously treated advanced cholangiocarcinoma containing FGFR2 fusions. Ann Oncol (2018) 29(8):viii720. doi: 10.1093/annonc/mdy424.030
15. Javle M, Lowery M, Shroff RT, Weiss KH, Springfeld C, Borad MJ, et al. Phase II study of BGJ398 in patients with FGFR-altered advanced cholangiocarcinoma. J Clin Oncol (2018) 36(3):276–82. doi: 10.1200/JCO.2017.75.5009
16. Javle M, Roychowdhury S, Kelley RK, Sadeghi S, Macarulla T, Weiss KH, et al. Infigratinib (BGJ398) in previously treated patients with advanced or metastatic cholangiocarcinoma with FGFR2 fusions or rearrangements: mature results from a multicentre, open-label, single-arm, phase 2 study. Lancet Gastroenterol Hepatol (2021) 6(10):803–15. doi: 10.1016/S2468-1253(21)00196-5
17. Makawita S, Abou-Alfa GK, Roychowdhury S, Sadeghi S, Borbath I, Goyal L, et al. Infigratinib in patients with advanced cholangiocarcinoma with FGFR2 gene fusions/translocations: the PROOF 301 trial. Future Oncol (2020) 16(30):2375–84. doi: 10.2217/fon-2020-0299
18. Mazzaferro V, El-Rayes BF, Cotsoglou C, Harris WP, Damjanov N, Masi G, et al. ARQ 087, an oral pan-fibroblast growth factor receptor (FGFR) inhibitor, in patients (pts) with advanced intrahepatic cholangiocarcinoma (iCCA) with FGFR2 genetic aberrations. J Clin Oncol (2017) 35:4017. doi: 10.1200/JCO.2017.35.15_suppl.4017
19. Mazzaferro V, El-Rayes BF, Droz Dit Busset M, Cotsoglou C, Harris WP, Damjanov N, et al. Derazantinib (ARQ 087) in advanced or inoperable FGFR2 gene fusion-positive intrahepatic cholangiocarcinoma. Br J Cancer (2019) 120(2):165–71. doi: 10.1038/s41416-018-0334-0
20. Busset MDD, Braun S, El-Rayes B, Harris W, Damjanov N, Masi G, et al. Efficacy of derazantinib (DZB) in patients (pts) with intrahepatic cholangiocarcinoma (iCCA) expressing FGFR2-fusion or FGFR2 mutations/amplifications. Ann Oncol (2019) 30:v276–7. doi: 10.1093/annonc/mdz247.048
21. Javle MM, Abou-Alfa GK, Macarulla T, Personeni N, Adeva J, Bergamo F, et al. Efficacy of derazantinib in intrahepatic cholangiocarcinoma patients with FGFR2 mutations or amplifications: Interim results from the phase 2 study FIDES-01. J Clin Oncol (2022) 40:427. doi: 10.1200/JCO.2022.40.4_suppl.427
22. Soria J-C, Strickler JH, Govindan R, Chai S, Chan N, Quiroga-Garcia V, et al. Safety and activity of the pan-fibroblast growth factor receptor (FGFR) inhibitor erdafitinib in phase 1 study patients (Pts) with molecularly selected advanced cholangiocarcinoma (CCA). J Clin Oncol (2017) 35:4074. doi: 10.1200/JCO.2017.35.15_suppl.4074
23. Bahleda R, Italiano A, Hierro C, Mita A, Cervantes A, Chan N, et al. Multicenter phase I study of erdafitinib (JNJ-42756493), oral pan-fibroblast growth factor receptor inhibitor, in patients with advanced or refractory solid tumors. Clin Cancer Res (2019) 25(16):4888–97. doi: 10.1158/1078-0432.CCR-18-3334
24. Goyal L, Bahleda R, Furuse J, Valle JW, Moehler MH, Oh D-Y, et al. FOENIX-101: A phase II trial of TAS-120 in patients with intrahepatic cholangiocarcinoma harboring FGFR2 gene rearrangements. J Clin Oncol (2019) 37:TPS468. doi: 10.1200/JCO.2019.37.4_suppl.TPS468
25. Borad MJ, Bridgewater JA, Morizane C, Shroff RT, Oh D-Y, Moehler MH, et al. A phase III study of futibatinib (TAS-120) versus gemcitabine-cisplatin (gem-cis) chemotherapy as first-line (1L) treatment for patients (pts) with advanced (adv) cholangiocarcinoma (CCA) harboring fibroblast growth factor receptor 2 (FGFR2) gene rearrangements (FOENIX-CCA3). J Clin Oncol (2020) 38:TPS600. doi: 10.1200/JCO.2020.38.4_suppl.TPS600
26. Rizzo A, Ricci AD, Brandi G. Futibatinib, an investigational agent for the treatment of intrahepatic cholangiocarcinoma: evidence to date and future perspectives. Expert Opin Investig Drugs (2021) 30(4):317–24. doi: 10.1080/13543784.2021.1837774
27. Goyal L, Meric-Bernstam F, Hollebecque A, Valle JW, Morizane C, Karasic TB, et al. FOENIX-CCA2 study investigators. futibatinib for FGFR2-rearranged intrahepatic cholangiocarcinoma. N Engl J Med (2023) 388(3):228–39. doi: 10.1056/NEJMoa2206834
28. Cleary JM, Voss MH, Meric-Bernstam F, Hierro C, Heist RS, Ishii N, et al. Safety and efficacy of the selective FGFR inhibitor debio 1347 in phase I study patients with FGFR genomically activated advanced biliary tract cancer (BTC). J Clin Oncol (2018) 36:447. doi: 10.1200/JCO.2018.36.4_suppl.447
29. Abou-Alfa GK, Macarulla T, Javle MM, Kelley RK, Lubner SJ, Adeva J, et al. Ivosidenib in IDH1-mutant, chemotherapy-refractory cholangiocarcinoma (ClarIDHy): a multicentre, randomised, double-blind, placebo-controlled, phase 3 study. Lancet Oncol (2020) 21(6):796–807. doi: 10.1016/S1470-2045(20)30157-1
30. Bekaii-Saab TS, Spira AI, Yaeger R, Buchschacher GL, McRee AJ, Sabari JK, et al. KRYSTAL-1: Updated activity and safety of adagrasib (MRTX849) in patients (Pts) with unresectable or metastatic pancreatic cancer (PDAC) and other gastrointestinal (GI) tumors harboring a KRASG12C mutation. J Clin Oncol (2022) 40:519. doi: 10.1200/JCO.2022.40.4_suppl.519
31. Wainberg ZA, Lassen UN, Elez E, Italiano A, Curigliano G, Braud F, et al. Efficacy and safety of dabrafenib (D) and trametinib (T) in patients (pts) with BRAF V600E-mutated biliary tract cancer (BTC): A cohort of the ROAR basket trial. J Clin Oncol (2019) 37:187. doi: 10.1200/JCO.2019.37.4_suppl.187
32. Bekaii-Saab T, Phelps MA, Li X, Saji M, Goff L, Kauh JS, et al. Multi-institutional phase II study of selumetinib in patients with metastatic biliary cancers. J Clin Oncol (2011) 29(17):2357–63. doi: 10.1200/JCO.2010.33.9473
33. Bridgewater J, Lopes A, Beare S, Duggan M, Lee D, Ricamara M, et al. A phase 1b study of selumetinib in combination with cisplatin and gemcitabine in advanced or metastatic biliary tract cancer: the ABC-04 study. BMC Cancer (2016) 16:153. doi: 10.1186/s12885-016-2174-8
34. Doherty M, Tam VC, McNamara MG, Hedley DW, Dhani NC, Chen EX, et al. Selumetinib (Sel) and cisplatin/gemcitabine (CisGem) for advanced biliary tract cancer (BTC): A randomized trial. J Clin Oncol (2018) 36:4084. doi: 10.1200/JCO.2018.36.15_suppl.4084
35. Sullivan RJ, Infante JR, Janku F, Wong DJL, Sosman JA, Keedy V, et al. First-in-Class ERK1/2 inhibitor ulixertinib (BVD-523) in patients with MAPK mutant advanced solid tumors: Results of a phase I dose-escalation and expansion study. Cancer Discovery (2018) 8(2):184–95. doi: 10.1158/2159-8290.CD-17-1119
36. Doebele RC, Drilon A, Paz-Ares L, Siena S, Shaw AT, Farago AF, et al. Entrectinib in patients with advanced or metastatic NTRK fusion-positive solid tumours: integrated analysis of three phase 1-2 trials. Lancet Oncol (2020) 21(2):271–82. doi: 10.1016/S1470-2045(19)30691-6
37. Hong DS, DuBois SG, Kummar S, Farago AF, Albert CM, Rohrberg KS, et al. Larotrectinib in patients with TRK fusion-positive solid tumours: a pooled analysis of three phase 1/2 clinical trials. Lancet Oncol (2020) 21(4):531–40. doi: 10.1016/S1470-2045(19)30856-3
38. Drilon A, Laetsch TW, Kummar S, DuBois SG, Lassen UN, Demetri GD, et al. Efficacy of larotrectinib in TRK fusion-positive cancers in adults and children. N Engl J Med (2018) 378(8):731–9. doi: 10.1056/NEJMoa1714448
39. Lee J, Park SH, Chang HM, Kim JS, Choi HJ, Lee MA, et al. Gemcitabine and oxaliplatin with or without erlotinib in advanced biliary-tract cancer: a multicentre, open-label, randomised, phase 3 study. Lancet Oncol (2012) 13(2):181–8. doi: 10.1016/S1470-2045(11)70301-1
40. Gruenberger B, Schueller J, Heubrandtner U, Wrba F, Tamandl D, Kaczirek K, et al. Cetuximab, gemcitabine, and oxaliplatin in patients with unresectable advanced or metastatic biliary tract cancer: a phase 2 study. Lancet Oncol (2010) 11(12):1142–8. doi: 10.1016/S1470-2045(10)70247-3
41. Malka D, Cervera P, Foulon S, Trarbach T, de la Fouchardière C, Boucher E, et al. BINGO investigators. gemcitabine and oxaliplatin with or without cetuximab in advanced biliary-tract cancer (BINGO): a randomised, open-label, non-comparative phase 2 trial. Lancet Oncol (2014) 15(8):819–28. doi: 10.1016/S1470-2045(14)70212-8
42. Ramanathan RK, Belani CP, Singh DA, Tanaka M, Lenz HJ, Yen Y, et al. A phase II study of lapatinib in patients with advanced biliary tree and hepatocellular cancer. Cancer Chemother Pharmacol (2009) 64(4):777–83. doi: 10.1007/s00280-009-0927-7
43. Peck J, Wei L, Zalupski M, O'Neil B, Villalona Calero M, Bekaii-Saab T. HER2/neu may not be an interesting target in biliary cancers: results of an early phase II study with lapatinib. Oncology (2012) 82(3):175–9. doi: 10.1159/000336488
44. Javle M, Borad MJ, Azad NS, Kurzrock R, Abou-Alfa GK, George B, et al. Pertuzumab and trastuzumab for HER2-positive, metastatic biliary tract cancer (MyPathway): a multicentre, open-label, phase 2a, multiple basket study. Lancet Oncol (2021) 22(9):1290–300. doi: 10.1016/S1470-2045(21)00336-3
45. Harding JJ, Piha-Paul SA, Shah RH, Murphy JJ, Cleary JM, Shapiro GI, et al. Antitumour activity of neratinib in patients with HER2-mutant advanced biliary tract cancers. Nat Commun (2023) 14(1):630. doi: 10.1038/s41467-023-36399-y
46. Javle M, Churi C, Kang HC, Shroff R, Janku F, Surapaneni R, et al. HER2/neu-directed therapy for biliary tract cancer. J Hematol Oncol (2015) 8:58. doi: 10.1186/s13045-015-0155-z
47. Zhu AX, Meyerhardt JA, Blaszkowsky LS, Kambadakone AR, Muzikansky A, Zheng H, et al. Efficacy and safety of gemcitabine, oxaliplatin, and bevacizumab in advanced biliary-tract cancers and correlation of changes in 18-fluorodeoxyglucose PET with clinical outcome: a phase 2 study. Lancet Oncol (2010) 11(1):48–54. doi: 10.1016/S1470-2045(09)70333-X
48. Bengala C, Bertolini F, Malavasi N, Boni C, Aitini E, Dealis C, et al. Sorafenib in patients with advanced biliary tract carcinoma: a phase II trial. Br J Cancer (2010) 102(1):68–72. doi: 10.1038/sj.bjc.6605458
49. Yi JH, Thongprasert S, Lee J, Doval DC, Park SH, Park JO, et al. A phase II study of sunitinib as a second-line treatment in advanced biliary tract carcinoma: a multicentre, multinational study. Eur J Cancer (2012) 48(2):196–201. doi: 10.1016/j.ejca.2011.11.017
50. Valle JW, Vogel A, Denlinger CS, He AR, Bai LY, Orlova R, et al. Addition of ramucirumab or merestinib to standard first-line chemotherapy for locally advanced or metastatic biliary tract cancer: a randomised, double-blind, multicentre, phase 2 study. Lancet Oncol (2021) 22(10):1468–82. doi: 10.1016/S1470-2045(21)00409-5
51. Tan ES, Cao B, Kim J, Al-Toubah TE, Mehta R, Centeno BA, et al. Phase 2 study of copanlisib in combination with gemcitabine and cisplatin in advanced biliary tract cancers. Cancer (2021) 127(8):1293–300. doi: 10.1002/cncr.33364
52. Buzzoni R, Pusceddu S, Bajetta E, De Braud F, Platania M, Iannacone C, et al. Activity and safety of RAD001 (everolimus) in patients affected by biliary tract cancer progressing after prior chemotherapy: a phase II ITMO study. Ann Oncol (2014) 25(8):1597–603. doi: 10.1093/annonc/mdu175
53. Lau DK, Tay RY, Yeung YH, Chionh F, Mooi J, Murone C, et al. Phase II study of everolimus (RAD001) monotherapy as first-line treatment in advanced biliary tract cancer with biomarker exploration: the RADiChol study. Br J Cancer (2018) 118(7):966–71. doi: 10.1038/s41416-018-0021-1
54. Alzahrani AS. PI3K/Akt/mTOR inhibitors in cancer: At the bench and bedside. Semin Cancer Biol (2019) 59:125–32. doi: 10.1016/j.semcancer.2019.07.009
55. Lowery MA, Ptashkin R, Jordan E, Berger MF, Zehir A, Capanu M, et al. Comprehensive molecular profiling of intrahepatic and extrahepatic cholangiocarcinomas: Potential targets for intervention. Clin Cancer Res (2018) 24(17):4154–61. doi: 10.1158/1078-0432.CCR-18-0078
56. Javle M, Bekaii-Saab T, Jain A, Wang Y, Kelley RK, Wang K, et al. Biliary cancer: Utility of next-generation sequencing for clinical management. Cancer (2016) 122(24):3838–47. doi: 10.1002/cncr.30254
57. Sia D, Losic B, Moeini A, Cabellos L, Hao K, Revill K, et al. Massive parallel sequencing uncovers actionable FGFR2-PPHLN1 fusion and ARAF mutations in intrahepatic cholangiocarcinoma. Nat Commun (2015) 6:6087. doi: 10.1038/ncomms7087
58. Simbolo M, Fassan M, Ruzzenente A, Mafficini A, Wood LD, Corbo V, et al. Multigene mutational profiling of cholangiocarcinomas identifies actionable molecular subgroups. Oncotarget (2014) 5(9):2839–52. doi: 10.18632/oncotarget.1943
59. Kang C. Infigratinib: First approval. Drugs (2021) 81(11):1355–60. doi: 10.1007/s40265-021-01567-1
60. Rizzo A, Brandi G. A foreword on biliary tract cancers: emerging treatments, drug targets, and fundamental knowledge gaps. Expert Opin Investig Drugs (2021) 30(4):279. doi: 10.1080/13543784.2021.1901192
61. Rizzo A. Novel approaches for the management of biliary tract cancer: today and tomorrow. Expert Opin Investig Drugs (2021) 30(4):295–7. doi: 10.1080/13543784.2021.1896247
62. Kommalapati A, Tella SH, Borad M, Javle M, Mahipal A. FGFR inhibitors in oncology: Insight on the management of toxicities in clinical practice. Cancers (Basel) (2021) 13(12):2968. doi: 10.3390/cancers13122968
63. Goyal L, Govindan A, Sheth RA, Nardi V, Blaszkowsky LS, Faris JE, et al. Prognosis and clinicopathologic features of patients with advanced stage isocitrate dehydrogenase (IDH) mutant and IDH wild-type intrahepatic cholangiocarcinoma. Oncologist (2015) 20(9):1019–27. doi: 10.1634/theoncologist.2015-0210
64. Boscoe AN, Rolland C, Kelley RK. Frequency and prognostic significance of isocitrate dehydrogenase 1 mutations in cholangiocarcinoma: a systematic literature review. J Gastrointest Oncol (2019) 10(4):751–65. doi: 10.21037/jgo.2019.03.10
65. Fujimoto A, Furuta M, Shiraishi Y, Gotoh K, Kawakami Y, Arihiro K, et al. Whole-genome mutational landscape of liver cancers displaying biliary phenotype reveals hepatitis impact and molecular diversity. Nat Commun (2015) 6:6120. doi: 10.1038/ncomms7120
66. Zhu AX, Macarulla T, Javle MM, Kelley RK, Lubner SJ, Adeva J, et al. Final overall survival efficacy results of ivosidenib for patients with advanced cholangiocarcinoma with IDH1 mutation: The phase 3 randomized clinical ClarIDHy trial. JAMA Oncol (2021) 7(11):1669–77. doi: 10.1001/jamaoncol.2021.3836
67. Jusakul A, Cutcutache I, Yong CH, Lim JQ, Huang MN, Padmanabhan N, et al. Whole-genome and epigenomic landscapes of etiologically distinct subtypes of cholangiocarcinoma. Cancer Discovery (2017) 7(10):1116–35. doi: 10.1158/2159-8290.CD-17-0368
68. Moore AR, Rosenberg SC, McCormick F, Malek S. RAS-targeted therapies: is the undruggable drugged? Nat Rev Drug Discovery (2020) 19(8):533–52. doi: 10.1038/s41573-020-0068-6
69. Robertson S, Hyder O, Dodson R, Nayar SK, Poling J, Beierl K, et al. The frequency of KRAS and BRAF mutations in intrahepatic cholangiocarcinomas and their correlation with clinical outcome. Hum Pathol (2013) 44(12):2768–73. doi: 10.1016/j.humpath.2013.07.026
70. Cocco E, Scaltriti M, Drilon A. NTRK fusion-positive cancers and TRK inhibitor therapy. Nat Rev Clin Oncol (2018) 15(12):731–47. doi: 10.1038/s41571-018-0113-0
71. Yoshikawa D, Ojima H, Iwasaki M, Hiraoka N, Kosuge T, Kasai S, et al. Clinicopathological and prognostic significance of EGFR, VEGF, and HER2 expression in cholangiocarcinoma. Br J Cancer (2008) 98(2):418–25. doi: 10.1038/sj.bjc.6604129
72. Vivaldi C, Fornaro L, Ugolini C, Niccoli C, Musettini G, Pecora I, et al. HER2 overexpression as a poor prognostic determinant in resected biliary tract cancer. Oncologist (2020) 25(10):886–93. doi: 10.1634/theoncologist.2019-0922
73. Wieduwilt MJ, Moasser MM. The epidermal growth factor receptor family: biology driving targeted therapeutics. Cell Mol Life Sci (2008) 65(10):1566–84. doi: 10.1007/s00018-008-7440-8
74. Pignochino Y, Sarotto I, Peraldo-Neia C, Penachioni JY, Cavalloni G, Migliardi G, et al. Targeting EGFR/HER2 pathways enhances the antiproliferative effect of gemcitabine in biliary tract and gallbladder carcinomas. BMC Cancer (2010) 10:631. doi: 10.1186/1471-2407-10-631
75. Mondaca S, Razavi P, Xu C, Offin M, Myers M, Scaltriti M, et al. Genomic characterization of ERBB2-driven biliary cancer and a case of response to ado-trastuzumab emtansine. JCO Precis Oncol (2019) 3:PO.19.00223. doi: 10.1200/PO.19.00223
76. Galdy S, Lamarca A, McNamara MG, Hubner RA, Cella CA, Fazio N, et al. HER2/HER3 pathway in biliary tract malignancies; systematic review and meta-analysis: a potential therapeutic target? Cancer Metastasis Rev (2017) 36(1):141–57. doi: 10.1007/s10555-016-9645-x
77. Riener MO, Bawohl M, Clavien PA, Jochum W. Rare PIK3CA hotspot mutations in carcinomas of the biliary tract. Genes Chromosomes Cancer (2008) 47(5):363–7. doi: 10.1002/gcc.20540
78. Herberger B, Puhalla H, Lehnert M, Wrba F, Novak S, Brandstetter A, et al. Activated mammalian target of rapamycin is an adverse prognostic factor in patients with biliary tract adenocarcinoma. Clin Cancer Res (2007) 13(16):4795–9. doi: 10.1158/1078-0432.CCR-07-0738
79. Ahn DH, Bekaii-Saab T. Biliary tract cancer and genomic alterations in homologous recombinant deficiency: exploiting synthetic lethality with PARP inhibitors. Chin Clin Oncol (2020) 9(1):6. doi: 10.21037/cco.2020.02.02
80. Ueno M, Ikeda M, Morizane C, Kobayashi S, Ohno I, Kondo S, et al. Nivolumab alone or in combination with cisplatin plus gemcitabine in Japanese patients with unresectable or recurrent biliary tract cancer: a non-randomised, multicentre, open-label, phase 1 study. Lancet Gastroenterol Hepatol (2019) 4(8):611–21. doi: 10.1016/S2468-1253(19)30086-X
81. Piha-Paul SA, Oh DY, Ueno M, Malka D, Chung HC, Nagrial A, et al. Efficacy and safety of pembrolizumab for the treatment of advanced biliary cancer: Results from the KEYNOTE-158 and KEYNOTE-028 studies. Int J Cancer (2020) 147(8):2190–8. doi: 10.1002/ijc.33013
82. Lee SH, Lee HS, Lee SH, Woo SM, Kim DU, Bang S. Efficacy and safety of pembrolizumab for Gemcitabine/Cisplatin-refractory biliary tract cancer: A multicenter retrospective study. J Clin Med (2020) 9(6):1769. doi: 10.3390/jcm9061769
83. Kang J, Jeong JH, Hwang HS, Lee SS, Park DH, Oh DW, et al. Efficacy and safety of pembrolizumab in patients with refractory advanced biliary tract cancer: Tumor proportion score as a potential biomarker for response. Cancer Res Treat (2020) 52(2):594–603. doi: 10.4143/crt.2019.493
84. Ioka T, Ueno M, Oh D-Y, Fujiwara Y, Chen J-S, Doki Y, et al. Evaluation of safety and tolerability of durvalumab (D) with or without tremelimumab (T) in patients (pts) with biliary tract cancer (BTC). J Clin Oncol (2019) 37(4_Suppl l):387. doi: 10.1200/JCO.2019.37.4_suppl.387
85. Oh D-Y, He AR, Qin S, Chen L-T, Okusaka T, Vogel A, et al. A phase 3 randomized, double-blind, placebo-controlled study of durvalumab in combination with gemcitabine plus cisplatin (GemCis) in patients (pts) with advanced biliary tract cancer (BTC): TOPAZ-1. J Clin Oncol (2022) 40:378. doi: 10.1200/JCO.2022.40.4_suppl.378
86. Hack SP, Verret W, Mulla S, Liu B, Wang Y, Macarulla T, et al. IMbrave 151: a randomized phase II trial of atezolizumab combined with bevacizumab and chemotherapy in patients with advanced biliary tract cancer. Ther Adv Med Oncol (2021) 13:17588359211036544. doi: 10.1177/17588359211036544
87. Marabelle A, Le DT, Ascierto PA, Di Giacomo AM, De Jesus-Acosta A, Delord J-P, et al. Efficacy of pembrolizumab in patients with noncolorectal high microsatellite Instability/Mismatch repair–deficient cancer: Results from the phase II KEYNOTE-158 study. J Clin Oncol Off J Am Soc Clin Oncol (2020) 38:1–10. doi: 10.1200/JCO.19.02105
88. Marcus L, Lemery SJ, Keegan P, Pazdur R. FDA Approval summary: Pembrolizumab for the treatment of microsatellite instability-high solid tumors. Clin Cancer Res (2019) 25(13):3753–8. doi: 10.1158/1078-0432.CCR-18-4070
89. Guo Y, Feng K, Liu Y, Wu Z, Dai H, Yang Q, et al. Phase I study of chimeric antigen receptor-modified T cells in patients with EGFR-positive advanced biliary tract cancers. Clin Cancer Res (2018) 24(6):1277–86. doi: 10.1158/1078-0432.CCR-17-0432
90. Dudley JC, Lin MT, Le DT, Eshleman JR. Microsatellite instability as a biomarker for PD-1 blockade. Clin Cancer Res (2016) 22(4):813–20. doi: 10.1158/1078-0432.CCR-15-1678
91. Winkelmann R, Schneider M, Hartmann S, Schnitzbauer AA, Zeuzem S, Peveling-Oberhag J, et al. Microsatellite instability occurs rarely in patients with cholangiocarcinoma: A retrospective study from a German tertiary care hospital. Int J Mol Sci (2018) 19(5):1421. doi: 10.3390/ijms19051421
92. Ikeda Y, Ono M, Ohmori G, Ameda S, Yamada M, Abe T, et al. Successful pembrolizumab treatment of microsatellite instability-high intrahepatic cholangiocarcinoma: A case report. Clin Case Rep (2021) 9(4):2259–63. doi: 10.1002/ccr3.4008
93. Hoadley KA, Yau C, Hinoue T, Wolf DM, Lazar AJ, Drill E, et al. Cell-of-Origin patterns dominate the molecular classification of 10,000 tumors from 33 types of cancer. Cell (2018) 173(2):291–304.e6. doi: 10.1016/j.cell.2018.03.022
94. Qiang Z, Dai K, He Y, Yang Z, Tao L, Zhang H, et al. The prognostic value of stromal tumor-infiltrating lymphocytes in intrahepatic cholangiocarcinoma: a population-based study. Scand J Gastroenterol (2022) 57(8):965–71. doi: 10.1080/00365521.2022.2055972
95. Kim HD, Jeong S, Park S, Lee YJ, Ju YS, Kim D, et al. Implication of CD69+ CD103+ tissue-resident-like CD8+ T cells as a potential immunotherapeutic target for cholangiocarcinoma. Liver Int (2021) 41(4):764–76. doi: 10.1111/liv.14814
96. Sangsuwannukul T, Supimon K, Sujjitjoon J, Phanthaphol N, Chieochansin T, Poungvarin N, et al. Anti-tumour effect of the fourth-generation chimeric antigen receptor T cells targeting CD133 against cholangiocarcinoma cells. Int Immunopharmacol (2020) 89(Pt B):107069. doi: 10.1016/j.intimp.2020.107069
97. Hamid O, Ismail R, Puzanov I. Intratumoral immunotherapy-update 2019. Oncologist (2020) 25(3):e423–38. doi: 10.1634/theoncologist.2019-0438
98. Lange S, Lampe J, Bossow S, Zimmermann M, Neubert W, Bitzer M, et al. A novel armed oncolytic measles vaccine virus for the treatment of cholangiocarcinoma. Hum Gene Ther (2013) 24(5):554–64. doi: 10.1089/hum.2012.136
99. Oh DY, Lee KH, Lee DW, Yoon J, Kim TY, Bang JH, et al. Gemcitabine and cisplatin plus durvalumab with or without tremelimumab in chemotherapy-naive patients with advanced biliary tract cancer: an open-label, single-centre, phase 2 study. Lancet Gastroenterol Hepatol (2022) 7(6):522–32. doi: 10.1016/S2468-1253(22)00043-7
Keywords: cholangiocarcinoma, targeted therapy, immunotherapy, systemic therapy, PD-1
Citation: Du J, Lv X, Zhang Z, Huang Z and Zhang E (2023) Revisiting targeted therapy and immunotherapy for advanced cholangiocarcinoma. Front. Immunol. 14:1142690. doi: 10.3389/fimmu.2023.1142690
Received: 12 January 2023; Accepted: 20 February 2023;
Published: 01 March 2023.
Edited by:
Jiang Chen, Zhejiang University, ChinaReviewed by:
Anuhya Kommalapati, Mayo Clinic, United StatesLingxi Jiang, Shanghai Jiao Tong University, China
Copyright © 2023 Du, Lv, Zhang, Huang and Zhang. This is an open-access article distributed under the terms of the Creative Commons Attribution License (CC BY). The use, distribution or reproduction in other forums is permitted, provided the original author(s) and the copyright owner(s) are credited and that the original publication in this journal is cited, in accordance with accepted academic practice. No use, distribution or reproduction is permitted which does not comply with these terms.
*Correspondence: Erlei Zhang, YmFpeXUxOTg2MTEwNEAxNjMuY29t; Zhiyong Huang, aHVhbmd6eUB0amgudGptdS5lZHUuY24=