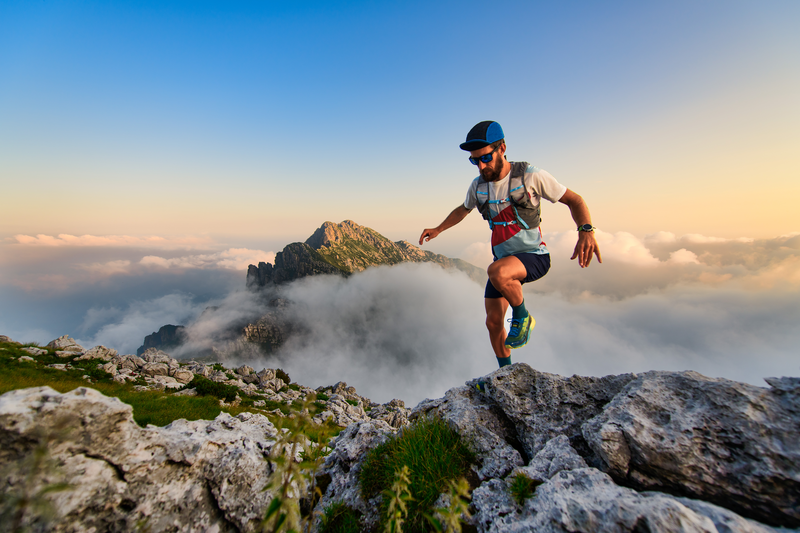
95% of researchers rate our articles as excellent or good
Learn more about the work of our research integrity team to safeguard the quality of each article we publish.
Find out more
REVIEW article
Front. Immunol. , 21 February 2023
Sec. Molecular Innate Immunity
Volume 14 - 2023 | https://doi.org/10.3389/fimmu.2023.1139450
This article is part of the Research Topic Emerging Molecular Signaling Pathways and Therapeutic Targets in the Genitourinary Immune Microenvironment View all 10 articles
Up to 50% of infertility is caused by the male side. Varicocele, orchitis, prostatitis, oligospermia, asthenospermia, and azoospermia are common causes of impaired male reproductive function and male infertility. In recent years, more and more studies have shown that microorganisms play an increasingly important role in the occurrence of these diseases. This review will discuss the microbiological changes associated with male infertility from the perspective of etiology, and how microorganisms affect the normal function of the male reproductive system through immune mechanisms. Linking male infertility with microbiome and immunomics can help us recognize the immune response under different disease states, providing more targeted immune target therapy for these diseases, and even the possibility of combined immunotherapy and microbial therapy for male infertility.
Infertility has been defined as the inability to conceive after at least 12 months of regular and unprotected sexual intercourse by WHO (1). It has brought substantial economic and psychological burden to the society (2). Nearly 15% of couples are affected by infertility, with male infertility contributing 50% among them (3). A review published in Lancet summarized some common factors that may cause male infertility, including varicocele, orchitis, prostatitis, oligospermia, asthenospermia, azoospermia and other male diseases (4).
The human microbiome is an ecosystem composed of many kinds of microorganisms. It is a relatively balanced state, not absolutely sterile, and mainly exists in external cavities such as the reproductive tract, oral cavity, and gastrointestinal tract. External bacteria, viruses, fungi, mycoplasma, chlamydia infection or opportunistic bacterial infection will cause the occurrence and development of a variety of diseases, male reproductive-diseases are also included (5). At present, more and more studies have been published on the relationship between microorganisms and male infertility. Male infertility caused by microbial infection is mostly achieved through immune response, and microorganisms induce the accumulation of immune cells and proinflammatory cytokines and chemokines. And the production of anti-sperm antibodies and biofilms also can damage germ cells and destroy the normal spermatogenic function (6).
At present, there is no systematic review on the immune mechanisms of several common diseases of male infertility (varicocele, orchitis, prostatitis, oligozoospermia, asthenospermia, azoospermia) caused by microbial infections. This review will fill this gap, aiming to provide new ideas and targets for the treatment of male infertility.
Varicocele is an important cause of male infertility. A large number of studies have shown that varicocele causes spermatogenesis and sperm dysfunction through oxidative stress. As for whether there are microbial colonization changes in the semen of patients with varicocele, recent studies have shown that the colonization of Ureaplasma urealyticum in patients with varicocele is significantly higher than that in healthy men. However, there is no direct evidence of the damage of Ureaplasma urealyticum to sperm, the oxidative stress caused by Ureaplasma urealyticum may have an adverse effect on semen quality (7). It is also possible that the co-infection of Chlamydia trachomatis, Ureaplasma urealyticum, and Mycoplasma hominis with the inflammation of the varicocele may lead to the loss of seminal ocilium, resulting in male infertility (8). Toxoplasma patients complicated with varicocele also can lead to abnormal sperm (9). In addition, varicocele may be associated with viral infection, but the correlation may be weak and more studies still needed to be explored. HSV was detected in 7% of 14 varicocele patients at one time, while CMV was detected in only two of 29 infertile men with varicocele in another study (10). Vicari et al. found that HBV and HCV were associated with varicocele. The sperm-related parameters of infertile men with HBV and varicocele were significantly lower than those of infertile men with only varicocele or HCV combined with varicocele. Therefore, HBV may aggravate the sperm damage of patients with varicocele (11).
Bacteria and viruses can interact with the host’s immune and inflammatory systems through their unique structure to elevate the polymorphonuclear leucocytes and granulocytes, resulting in oxidative stress and impaired male fertility (12). Recent studies have found that even though the concentration of white blood cells is normal in VC patients, the subsets of white blood cells are significantly changed, the proportion of CD8+ T cells and macrophages is significantly reduced, while the level of CD4+ Th cells is significantly increased, leading to the increase of cytokine levels in VC patients (13). Due to the increase of oxidative stress level in VC patients, it can mediate the change of immune cells in testis, further lead to irreversible damage of testis and affect normal spermatogenesis (14).
By binding to. specific receptors on the surface of target cells, cytokines control the intracellular response and expression of related genes, the details are shown in Table 1. In the earlier study of Adel et al., it was observed that the concentration of IL-6 was significantly increased in cases of varicocele without inflammation, but it was not found to be significant in cases of varicocele with inflammation, so IL-6 was negatively correlated with sperm concentration and sperm motility (26). Later, Nallella et al. found a significant increase in IL-6 in patients with varicocele (22). Sakamoto et al. also got the similar result, they found that the IL-6 level in the varicocele group was significantly higher than that in the control group (27). In recent years, it has been further found that TNF-α impairs testis by changing mitochondrial function, increasing NO production and promoting oxidative stress. A recent study by Moretti et al. showed a positive correlation between IL-6 levels and the level of malondialdehyde (MDA) in semen. The levels of malondialdehyde(MDA) in seminal plasma and semen were negatively correlated with sperm parameters, which further confirmed that IL-6 was involved in oxidative stress induced sperm damage (28). IL-1α and IL-1β are two forms of IL-1, which are regulators of testicular function, but increased expression of IL-1α and IL-1β in rats with varicocelectic veins, especially in the 11 - and 13-week-old groups, disrupted the balance of inflammation and immunity and had deleterious effects on testicular tissue (15). Moreover, IL-37 and IL-18 were also found to be upregulated in the seminal plasma of varicocele patients (15). These elevations lead to inflammatory response activation, leucocyte recruitment and ROS production, which are detrimental to normal testicular functions (29). Importantly, ROS can disrupt the blood–testis barrier, the sperm plasma membrane and DNA integrity and then affected male fertility (30). The production of NO and inflammatory factors can also promote the assembly of NLRP3 inflammasome complex through the differentiation of testis macrophages, and the increase of inflammasome can in turn promote the production of ROS and affect the normal spermatogenic function of testis (31). In addition, studies have demonstrated that down-regulation of inflammasome can reduce the apoptosis of spermogenesis related cells in testicular tissue of VC patients (32).
Another important immunological factor contributing to infertility in patients with varicocele is the presence of anti-sperm antibodies (ASAs). ASAs are found in 5%-15% of men with infertility (33). Animal experiments showed that the ASAs level of VC model rats was higher than that of normal rats (25). Similarly, studies in the population have further validated that. In varicocele patients, the autoimmune anti-sperm reaction is accompanied by a more significant decrease in the semen quality (33). ASAs can cause sperm agglutination and reduce sperm motility, resulting in male infertility, and even affect early implantation and pregnancy. In an earlier study, Golomb et al. observed that ASA was significantly present in the serum and seminal plasma of infertile men with varicocele, mainly IgA and IgM (34). Bozhedomov et al. showed an association between the grade of varicose veins and ASA level by direct current cytometry. Among ASA-positive patients, the more severe the varicose veins, the lower the sperm concentration, sperm density and sperm motility (33). For patients with varicose veins undergoing venous ligation, Djaladat et al. obtained that varicose vein surgery reduces ASA levels in some patients, but may also increase them in some patients, and this positive conversion does not adversely affect sperm parameters (35). A recent study found that the number of patients with active sperm autoimmunity was 2.8 times lower than that before surgery, and the average MAR-IgG level was 1.8 times lower in the group with improvement after surgery, but the presence of ASA reduced the efficacy of reproductive function recovery after varicocular surgery (36).
The pathogen interacts with the immune system of VC patients, resulting in increased levels of polymorphonuclear leucocytes, granulocytes and CD4+ Th, further increased levels of cytokines and inflammasome, and increased oxidative stress (12, 13). It changes the immune microenvironment in testis and affects male normal fertility. IL-6 is involved in the process of oxidative stress induced sperm damage, and can be considered as a biomarker (22, 26, 27). It is also very important to study the treatment of VC patients with ASAs, which affects the subsequent recovery of reproductive function (33–36).
In the early stage of SARS-induced orchitis, extensive destruction of germ cells, few or no sperm in seminiferous tubules, thickening of basement membranes and infiltration of white blood cells are shown, affecting male reproductive function (37). The recent epidemic of COVID-19 has a high degree of sequence similarity with SARS. Studies in respiratory system have shown that COVID-19 invades organs through ACE2 receptors, the testicular Ledig and Sertoli cells highly express ACE2 receptors, so that virus can also achieve its invasion into the testis through ACE2 receptors (38). The increase of immune cells in the testicular interstitium and proinflammatory cytokines IL-6, TNF-α and MCP-1 in the semen of patients with COVID-19 reduces the concentration of sperm and impairs spermatogenesis. The accumulation of inflammatory cells and their products caused by the virus can activate an autoimmune response leading to autoimmune orchitis, which damages spermatogenic epithelium. In addition, persistent fever may also be the cause of germ cell damage and degeneration in COVID-19 patients (39).
Mumps virus often causes patient orchitis and affects male fertility function. Mumps orchitis usually occurs about a week after the onset of mumps. It may start with systemic symptoms and later manifest as swelling and pain in the testicles. MuV induces immune response with TLR2 and RIG-I signaling, resulting in an increase in proinflammatory cytokines and chemokines (40). MuV can also inhibit antiviral IFN signaling through AXL and MER receptors, thereby promoting excess MuV replication in Leydig and Sertoli cells, and may even induce anti-sperm antibodies with potentially long-term adverse effects on patient fertility (6).
Chlamydia is also often considered as an important pathogen of orchitis through the route of sexual transmission, of which Chlamydia trachomatis and Neisseria gonorrhoeae are the most common. During the period of Chlamydial infection, testicular cells can be observed that DNA damage and the transcription of epigenetic mediated disorder lead to abnormal sperm epigenome, moreover also can increase the leukocyte infiltration, destroy the blood-testis barrier, reduce the number of sperm cells and the volume of seminiferous tubule, leading to low fertility and birth defects (41).
During the onset of autoimmune orchitis, the quantification and phenotype of infiltrating cells in the testis showed an increase in the number of T cell subsets, dendritic cells, and macrophages, including both Th and Treg cells. However, the active effect of Th cells exceeded that of accumulated Treg cells, and the presence of inflammation limited the ability of Treg cells to eliminate tissue damage. Treg cells usually prevent the induction of spontaneous organ-specific autoimmunity by persistent endogenous danger signals (42). Both CD4+ and CD8+ T cells increase in the initial inflammatory process, while CD4+ T cells decrease and CD8+ T numbers remain unchanged during the chronic maintenance of EAO. Therefore, CD4+ and CD8+ T cells play an advantage in the onset and chronic phase of EAO, respectively (43). For regulatory T cells, both CD4+Foxp3+ and CD8+Foxp3+ Treg cells were increased, and CD25+ cells were more common (43). In terms of another classification of T cells, both αβ and γδT cell subsets are increased in orchitis. αβT cells are the initiators of the autoaggressive response, and γδT cells play a regulatory role in infection-induced autoimmune orchitis (44).
Jing et al. found that cDC1 is necessary for the presence of T cells in the testis, but cDC2 does not have any significance for the maintenance of T cells, the depletion of T cells does not affect normal spermatogenesis, and cDC1-dependent T cells play an important role in chronic autoimmune orchitis (45).
Testicular macrophages are the major immune cells within the mammalian testis and are important for organogenesis, spermatogenesis, and androgen production, providing protection to the developing male germ cells while also allowing adequate response to inflammatory stimuli to produce proinflammatory immunity and anti-infection protection against invading pathogens. The ED1+ macrophage subsets are the main pathogenic subsets in the occurrence of EAO. HMGB1 can stimulate macrophages and phosphorylate p38 mitogen-activated protein kinase (MPPK) and p65 NF- κB, leading to increased TNF-α and IL-6 and causing testicular injury (46). While S100A9 by activating PI3K/Akt pathway to maintain the immunosuppressive function of macrophages (47). The same effect was observed in the macrophages of male genital tract infection caused by E. coli, which could not only increase the anti-inflammatory cytokines regulated by NFAT (calcium dependent nuclear factor), but also down-regulate the expression of proinflammatory factors in peritoneal macrophages (PM) inhibited by α-hemolysin. By LPS treated TM was not sensitive to the activation of NF- κB, and the secretion of proinflammatory factors was deficient. By this mechanism TM initiates an anti-infective response while maintaining testicular immune privilege and protecting spermatogenic cells (48).
Cytokines play an important role in the pathogenesis of orchitis. As shown in Table 2, some of the cytokines have changed in the orchitis model. Multiple studies have demonstrated the significant role of IL-6 in orchitis (50, 53, 54). Studies show that immune cells affect the normal immunosuppressive microenvironment of the testis by secreting cytokines such as TNF-α, IL-6, IL-12, IL-17 and IL-23, and proinflammatory cytokines can also play an indirect role by affecting the immunosuppressive effect of Treg cells (42). The same phenomenon was also observed in the study of Nicolas et al., who found that the expression of TNF, MCP-1, and IL-10 also increased as the increase of T cells (58). A large number of data on acute orchitis show that the up-regulation of IL-1β, IL-α, IL-6 and TNF-α can adversely affect germ cells, increasing germ cell expression and promoting germ cell apoptosis through the TNF-α/TNFR1, IL-6/IL-6R and Fas/FasL systems (59). Studies have shown that uropathogenic Escherichia Coli (UPEC) orchitis induces the activation of NLRP3 inflammasome through up-regulation of CaSR, promotes the secretion and maturation of IL-1β, and affects the synthesis of testosterone (56). IL-17A can promote the focal inflammatory cell infiltration in the testicular interstitium, resulting in the increased permeability of the blood-testis barrier and the loss of germ cells in the adjacent seminiferous tubules, thus affecting the spermatogenic function of the testis (52). In addition, high doses of IL-18 from immune cells in vivo can induce apoptosis of Leydig cells through the Fas pathway (60). The increase in chemokines such as CCL2, CCL3 and CCL4 can also be observed in EAO, inducing the attraction and extravasation of immune cells (59).Guazzone et al. also showed that the highest levels of CCL3 in the testis coincided with the onset of the disease (61).
Based on our data and the other aforementioned studies, it is reasonable to propose that Th1 cells are augmented at the onset of orchitis, inducing anti-infection responses. However, in the chronic phase, Th17 cells dominate the Th cell subsets and maintain the inflammation state in the testes by inhibiting Tregs and crowding out other effector T cells. Collectively, Th cells in orchitis are involved in the impairment of the structure and spermatogenesis of the testes (58). Immune cells in the testis affect the normal immunosuppressive microenvironment of the testis by secreting cytokines and chemokines. The TNF-α/TNFR1, IL-6/IL-6R and Fas/FasL systems promote the apoptosis of germ cells (42, 59).
According to the internationally recognized National Institutes of Health (NIH) classification, there are five types of prostatitis: acute prostatitis type I, chronic bacterial prostatitis type II, chronic prostatitis/chronic pelvic pain syndrome type III (CP/CPPS), and asymptomatic prostatitis type IV. Chronic pelvic pain syndrome type II is the most common type, accounting for 90-95% of all prostatitis diagnoses. The prevalence of the disease in the general population is 5-14.2%, and it is more common in people aged 35-45 years (62, 63).
The most common infections for acute bacterial prostatitis are coliforms and enterococci, which are similar to other common urogenital infections. Most of them can be cured in the acute phase, and fewer of them will develop non-chronic prostatitis, which is more complicated. Infection with viruses, fungi, mycoplasma and chlamydia may also be involved.
Corynebacterium was found in the urine and semen of both chronic prostatitis patients and healthy men, but it was evident that in prostatitis patients with severe leukoospermia, Corynebacterium was found in more species and in higher concentration, and group G Corynebacterium and Arthrobacter were more representative, which has a higher correlation with inflammatory prostatitis (64). P. acnes, a common skin microorganism, is also found in patients with prostatitis. It induces the secretion of cytokines and chemokines, and causes inflammation of the prostate and affecting sperm function through the host protein vimentin expressed in the prostate for bacterial erosion (65).
Patients with CP/CPPS had higher levels of Clostridium and Burkholderia in their urine microbiota (66, 67). In addition, the differences between CP/CPPS patients and controls were also different according to the time of urine collection. Nickel et al. found significant differences in overall species and genus composition in the initial urine flow, whereas no significant differences were observed at any level in urine samples from the middle and late stages of prostate massage. Therefore, the results of urinalysis are specific and time-sensitive, and do not represent the overall level (67). Recent studies on CP/CPPS showed that Achromobacter, Oligotrophomonas, and Brevibacterium were more common, and the diversity of the microbial community was also reduced in the patient group (68). The identification of various dominant microorganisms in CP/CPPS will be more conducive to the future development of microbiology in CP/CPPS and its application in treatment.
The gut microbe is also an important aspect of microbial research. Shoskes et al. showed that the diversity of the gut microbial community in patients with chronic pelvic pain syndrome was significantly lower than that in the control group, and the count of Prevotella in patients with CP/CPPS was significantly lower than that in the control group (69). In contrast, Konkol et al. found a significant increase in the gut microbial population but a decrease in the levels of Bacteroides homogenes, Lactobacilli and Lactobacilli in animal models with prostatitis (70). By sequencing amplified polymerase chain products, Mandar et al. found that patients with prostatitis had a higher diversity of species than healthy men, as well as a higher proportion of Proteobacteria and a lower proportion of Lactobacillus (71). Therefore gut microbiota can be considered as a biomarker of disease and as a target for future research and treatment.
Murphy et al. injected a specific strain of S. epidermidis from healthy men into mice with autoimmune prostatitis and found that a cell wall component of NPI (lipoteichoic acid) mediated CTLA4-like ligand on prostate antigen presenting cells, resulting in increased expression of PDL1 and PDL2, decreasing the pain response to pelvic tactile abnormalities (72).
Kogan’s latest study categorized chronic bacterial prostatitis into aerobes dominant, anaerobes dominant, and both aerobes and anaerobes dominant groups, and found that more severe clinical conditions were observed in patients with both aerobes and anaerobes and titers ≥103 CFU/ml. Relate aerobic - anaerobic conditions to the degree of clinical status (73).
The occurrence of prostatitis is partly caused by the infection of sexually transmitted pathogens, such as Trichomonas vaginalis, Mycoplasma, chlamydia, etc. Early studies by Skerk et al. detected Chlamydia trachomatis, Trichomonas vaginalis, and Ureaplasma urealyticumfrom from prostatic secretions or in urine samples collected after prostatic massage (74). Though the detection rate of Chlamydia trachomatis in subsequent studies is not high, we still believe that when considering the possibility of chronic prostattis, we should carry out routine detection of Chlamydia trachomatis also should be carried out to confirm the pathogen (75). Proof may be that IgA of Chlamydia trachomatis is closely related to sperm concentration and normal morphology, Chlamydia trachomatis can destroy germ cells through immune mediation and reduce male fertility (76). Ureaplasma microtium, Ureaplasma urealyticum, and Mycoplasma gendii are commonly detected in type IIa chronic prostatitis, and recent studies have also found that Ureaplasma urealyticum plays a role in promoting calcification formation and high white sperm count in chronic prostate infection (77). Jang et al. demonstrated that infection with Trichomonas vaginitis in rats can lead to prostatitis, mainly manifested as pathological changes, infiltration of mast cells and increased production of the chemokine CCL2 (78).
For prostatitis in HIV patients, except bacteria and viruses, the most common infection is fungal pathogens, such as Candida, Cryptococcus, Aspergillus, Blastobacter, Coccidioides and Histoplasmosis, which can lead to disseminated infection and prostatitis in immunocompromised men (79).
Unlike the testis, the prostate is generally considered a non-immune organ, but many immune cells can be found in it, such as NK cells, mast cells, lymphocytes and macrophages. In the study conducted by Vykhovanets et al., it was first found that the prostate of normal healthy Sprague-Dawley rats contained an unusually high proportion of NK and NKT cells (80). Subsequent studies confirmed that the level of CD4(+) NKT cells decreased significantly and CD45RC(+)CD49(+)αβTCR(+) T cells increased in elderly SPI and EPI patients compared with elderly NPI and young patients (81). Therefore, identification of the phenotype of NK cells and NKT cells and their proportional relationship with T cells will be helpful for the diagnosis and treatment of chronic prostatitis. NK and NKT cells can also kill cancer cells. Recently, more research has been conducted on the relationship between prostate cancer and NK cells, and how to use NK cells for the treatment of prostate cancer. CP/CPPS are mainly characterized by their pelvic pain, and studies by Done et al. and Roman et al. show that the release of mast cell mediators is a key factor in pain. It can be observed that there are degranulation products of mast cells in the prostate and urine of CP/CPPS patients, mainly the increase of tryptase-β and carboxypeptidase A3. The expression of the receptor PAR2 of tryptase is increased in patients, which regulates the phosphorylation of the kinase ERK1/2 and the influx of calcium ions through extracellular signaling, leading to the occurrence of pain. In addition, nerve growth factor also seems to play a role in the induction of pain, but there are no definitive results (82, 83). The study of the correlation of mast cells provides new ideas for treating CP/CPPS patients, which can reduce pain in patients by inhibiting the tryptase-PAR2 axis. However, the etiology of CP/CPPS is currently unclear, and most studies believe that autoimmunity is an important cause. In early studies of autoimmune prostatitis induced in non-obese diabetic (NOD) mice, PSBP (steroid-binding protein) was found to be an autoantigen recognized by the NOD immune system, and CD4+ T cells played an important role in EAP (84). In the mouse model of autoimmune prostatitis (EAP)established by Motrich et al., prostatitis and chronic pelvic pain were mainly induced by Th1-related immune responses after prostate autoantigen-induced autoimmunity, and the deficiency of Th1 or Th2 cytokines reduced or enhanced susceptibility to autoimmune prostatitis, respectively. The adaptive immune response mediated by Th1 and Th17 through the production of cytokines is equally significant in CP/CPPS (85).
Cytokine changes also play an important role in prostatitis. Common cytokines and their changes are shown in Table 3. In the study of Motrich et al., an increase of IFN-γ and IL-12 was observed in the prostate of autoimmune animals, with a decrease in IL-10. The mouse model lacking IFN-γ signaling transcription factor had no inflammatory response. IFN-γ can also stimulate IL-15 production in the prostate by paracrine, thereby inducing the proliferation of prostate T cells and participating in the inflammatory process of the prostate, which proves that IFN-γ is involved in the pathogenesis of CP/CPPS (89). IFN-γ and IF-17A expression were found to be increased in mice with chronic prostatitis (96). Through the detection of cytokines in CP/CPPS patients, Penna et al. found that IL-8 may be the most reliable and predictive marker for the diagnosis of prostatitis, and IL-8 was significantly increased in type IIIa patients. In addition, the level of IL-8 was positively correlated with the patient’s symptom score and prostate specific antigen level (97). In addition to the measurement of cytokines, Penna et al. found an increase of CCL1, CCL3 and CCL4, CCL17 and CCL22, CXCL8 in the seminal plasma in patients with CP/CPPS or benign prostate hyperplasia (97). Quick et al. focused on the CCL2 and CCL3 pathways associated with pelvic pain. The CCL2-CCR2 axis and CCL3 are important mediators of pelvic pain, and only anti-CCL2 antibody is effective in the treatment of autoimmune prostatitis (98).
In the process of activation of inflammatory innate immune system, in addition to cytokines playing an important role in the inflammatory process, inflammasome is also indispensable in promoting the formation and transformation of cytokines. Chronic bacterial prostatitis is through the upregulation of inflammasomes NLRP1 and NLRP3, and the increased expression of ASC and caspase-1, which together promote the conversion of downstream IL-1β and IL-18 precursors, promote the release of mature cytokines, and participate in the inflammatory and immune processes (99–101). Therefore, considering the role of inflammasome can provide a new target for the treatment of prostatitis.
The generation of chronic bacterial prostatitis is associated with a variety of bacterial infections. These bacteria escape the immune by forming biofilms and have high tolerance to antibiotics, which affects the treatment of bacterial prostatitis. Kanamaru et al. showed that the possibility of biofilm formation from patients with prostatitis was higher than that of acute cystitis and pyelonephritis, and the isolates from prostatitis had higher optical density values and more curli fimbriae, which further confirmed the connection between biofilm and prostatitis (102).In addition, the hemolysin produced by E. coli cooperates with the biofilm to lead to the persistence of E. coli in the prostate (103). In a recent study, it was found that patients with biofilm formation had higher NIH-CPSI scores and less improvement in symptoms than patients without biofilm formation, which had a negative impact on antibiotic treatment (104).
The types of pathogens infected by different types of prostatitis will be different, so the identification of dominant microorganisms can be used as biomarkers to provide new targets and ideas for future disease research and treatment. The study of abnormal proportions of NK and NKT cells in patients with prostatitis, and their association with T cells, will also contribute to the treatment of chronic prostatitis and prostate cancer. The formation of cytokines and inflammasome is involved in the inflammatory and immune processes of prostate. The formation of cytokines promotes the assembly of inflammasome complex, and the appearance of inflammasome further promotes the release of mature cytokines, promoting the development of prostatitis. In the treatment of prostatitis, bacterial biofilm formation can affect the role of antibiotics and immune system, so how to improve the treatment of patients with prostatitis accompanied by biofilm is also very important.
Clinically, unilateral and bilateral infections of the testis and epididymis often lead to azoospermia and severe oligozoospermia, while gonadal infections are mostly caused by bacterial infections. Yang et al. revealed that the microbiomes of asthenospermia and oligozoospermia were significantly different in terms of β diversity, with high relative abundances of Bacteroides, Anaerobic Cocci, Spermicococcus, Lactobacillus and Acinetobacter Roche seen in asthenospermia patients, and significantly higher relative abundances of Lactobacillus in oligozoospermia patients (105). However, other studies have linked oligoasthenozoospermia in part to an increase in Neisseria, Klebsiella, and Pseudomonas pathogens and a decrease in Lactobacillus probiotics, so whether the effects of Lactobacillus on male fertility are beneficial or harmful remains controversial (105). Mehta et al. studied the effects of Streptococcus faecalis on oligozoospermia and teratozoospermia in men. Compared with semen containing micrococcus or α-Streptococcus hemolyticus and normal uninfected semen, the incidence of oligozoospermia and teratozoospermia was significantly higher, and the mean sperm concentration and the mean percentage of normal spermatozoa in semen were significantly lower (106).
Human papillomavirus and herpesviridae frequently infect epithelial cells and are considered to be considerable risk factors for infertility. In an earlier study conducted by Lai et al., asthenospermia was found to be significantly more common in HPV-infected patients (75%) than in men without HPV infection (8%) (107). Nasseri et al. looked at HPV detection rates in patients with oligozoospermia and azoospermia from another perspective and found significant differences in sperm counts and sperm motility rates between positive and negative samples from patients with oligozoospermia. In addition, HPV16, 45 genotypes in the high-risk group and HPV6, 11, 42 genotypes in the low-risk group were more common (108). HSV was found in 279 semen samples collected by Kurscheidt et al., HSV-1 was significantly associated with a lower mean sperm count, and HSV-2 infection was significantly associated with hemospermia and lower mean semen quality (109).
Ureurealyticum can damage sperm structure and function by reducing motility and causing damage to the sperm membrane, especially the lipid bilayer. In the analysis of various types and subgroups of Ureaplasma, Yang et al. found that subgroups A and C of Ureaplasma microgenesis and subgroup 1 of Ureaplasma urealyticum were associated with oligozoospermia, and subgroup 2 of Ureaplasma urealyticum may have the ability to impair semen motility (110).
Chlamydia trachomatis is also a common sexually transmitted pathogen, previous studies have found reduced sperm concentration, normal sperm morphology, sperm volume, sperm motility, and sperm velocity in chlamydia-positive samples compared with negative samples, and a 14-fold risk of reduced semen volume when both couples were tested for Chlamydia trachomatis (111).
COVID-19 not only affects the respiratory system, but also has a certain impact on the reproductive function of patients. Thinning of spermatogenic tubules, interstitial edema, hyperemia, infiltration of inflammatory cells and inflammatory mediators can be observed in necropsy testis and epididymis specimens of patients with COVID-19. Compared with normal men, IL-6, TNF-α and MCP-1 in semen are increased, leading to low testosterone level and affecting normal spermatogenic function. 39.1% of the patients had oligozoospermia (39). The same phenomenon was observed in the study by Apaydin et al (112). In addition, after acute Zika virus infection, although there may be only early semen changes, which will return to normal values later, early pathological changes may also affect sperm function through testicular and epididymal pathological changes, thus affecting male fertility (113). Adeno-associated virus AAV was significantly higher in both oligoasthenospermia and oligozoospermia patients than in normal semen, but not in asthenospermia and azoospermia. AAV may cause oligoasthenospermia by infecting testes and interfering with normal sperm development (114).
In an earlier study of patients with azoospermia carried out by Mahmoud et al., it was observed that although T cells, B cells, macrophages and mast cells were all increased, only the increase in mast cells was significant. Due to the increase of tryptase, the number of mast cells were increased resulting in the testicles collagen fibers deposition obviously and affecting sperm production function (115). At the same time, the bacteria infection of the reproductive system may lead to leukopenia, which increases the number of anti-sperm antibodies by enhancing the function of T helper cells and B cells, and damages male fertility together with natural killer cells (116). In addition to the immune cell changes described above, proinflammatory factors are also changed in infertile men, especially in infertile men with genital tract infections. A recent study showed that for all cytokines measured in oligospermia, asthenospermia, and oligoasthenospermia compared with controls, only IL-5 levels were reduced, with significant reductions of 87%, 78%, and 87%, respectively (117).
In a meta-analysis of male infertility, ASA antibody positive patients had significantly lower sperm concentration and sperm motility than ASA negative controls (118). In addition to impairing sperm motility by activating the complement system, Dimitrov et al. also found that 15 out of 28 infertile men with asthenospermia were positive for anti-sperm CMI (cell-mediated immunity), thus ASA-mediated cellular immunity may be an important cause of oligozoospermia and asthenospermia (119). The immune complex also affects the male reproductive function. Murakami et al. found 4 disease-specific antigens in oligozoospermia patients and 5 disease-specific antigens in asthenospermia patients. The formation and deposition of immune complexes stimulate inflammation through the action of the complement system, leading to reproductive organ fibrosis and loss of related protein function, thus leading to spermatogenic dysfunction (120).
Genes in HLA loci that play key roles in antigen presentation and immune response are strongly associated with asthenospermia and oligozoospermia. HLA-A*11:01 is associated with multiple forms of male infertility; HLA-DQB1*03:02 and HLA-A*29:02 are associated with oligozoospermia, and HLA-A*29:02 can also interfere with sperm count with HPV, as well as risk genes associated with oligozoospermia, they are HLA-DQA1*05:01, HLA-C*03:03, and HLA-DQB1*03:01.HLA was associated with male infertility and HPV to further explore the multiple influencing factors of male infertility (121).
The β diversity of microflora in oligospermia patients was significant, and the infection of micrococcus or α-hemolytic streptococcus was more (105, 106). The infection of sexually transmitted pathogens and viruses also participated in the process of sperm damage. Abnormal levels of IL-5 and specific gene locus changes of HLA can be used as biomarkers for infertile men (117, 121).
There is growing evidence linking male infertility to the microbiome, and rapidly evolving microbial sequencing and analysis methods will help us understand more potential pathogens associated with male infertility in the future. In this review, we summarize the current microorganisms affecting male fertility and summarize their immunological mechanisms, providing certain ideas for the subsequent treatment of male infertility in Figure 1. There are still some difficulties that we have not yet overcome. Obtaining the microorganisms in the research site without being affected is one of the most complex difficulties. For example, the presence of pathogens in semen analysis is extremely easy to be affected by the surrounding structure and is not completely targeted. Therefore, the methods of microbial sampling from different parts need to be further studied. In addition, by linking male infertility with the microbiome and exploring the immune mechanism in the microbiome, we can not only better understand the immune response in vivo under different pathological conditions, including the changes in the number and types of immune cells, immune factors, and chemokines, etc., but also provide more targeted immune target therapies for pathological conditions. It is also possible to combine immunotherapy with microbiomes therapy, which may be more effective in treating male infertility.
JC prepared and wrote the manuscript; JYC oversaw the section, designed and finished the figure and tables; YF and QS commented on previous versions of the manuscript; KZ and CL revised the manuscript; HZ designed and revised the manuscript. All authors discussed the relevant literature and composed the sections of the review article. All authors contributed to the article and approved the submitted version.
This work was supported by National Key R&D Program of China (Grant numbers: 2018YFC1004300, 2018YFC1004304), the Fundamental Research Funds for the Central Universities (Grant numbers: YCJJ202201050), and National Nature Science Foundation of China (Grant numbers: 81871148).
The authors declare that the research was conducted in the absence of any commercial or financial relationships that could be construed as a potential conflict of interest.
All claims expressed in this article are solely those of the authors and do not necessarily represent those of their affiliated organizations, or those of the publisher, the editors and the reviewers. Any product that may be evaluated in this article, or claim that may be made by its manufacturer, is not guaranteed or endorsed by the publisher.
1. Zegers-Hochschild F, Adamson GD, de Mouzon J, Ishihara O, Mansour R, Nygren K, et al. International committee for monitoring assisted reproductive technology (ICMART) and the world health organization (WHO) revised glossary of ART terminology, 2009. Fertil Steril (2009) 92(5):1520–4. doi: 10.1016/j.fertnstert.2009.09.009
2. Lotti F, Maggi M. Sexual dysfunction and male infertility. Nat Rev Urol (2018) 15(5):287–307. doi: 10.1038/nrurol.2018.20
3. Chen J, Chen J, Fang Y, Shen Q, Zhao K, Liu C, et al. Diagnostic evaluation of the infertile male: A committee opinion. fertility and sterility. Emerging Molecular Signaling Pathways and Therapeutic Targets in the Genitourinary Immune Microenvironment (Frontiers in Immunology) (2015) 103(3):e18–25. doi: 10.1016/j.fertnstert.2014.12.103
4. Agarwal A, Baskaran S, Parekh N, Cho CL, Henkel R, Vij S, et al. Male Infertility. Lancet (London England) (2021) 397(10271):319–33. doi: 10.1016/S0140-6736(20)32667-2
5. Altmäe S, Franasiak JM, Mändar R. The seminal microbiome in health and disease. Nat Rev Urol (2019) 16(12):703–21. doi: 10.1038/s41585-019-0250-y
6. Wang F, Chen R, Jiang Q, Wu H, Gong M, Liu W, et al. Roles of sialic acid, AXL, and MER receptor tyrosine kinases in mumps virus infection of mouse sertoli and leydig cells. Front Microbiol (2020) 11:1292–. doi: 10.3389/fmicb.2020.01292
7. Peerayeh SN, Yazdi RS, Zeighami H. Association of ureaplasma urealyticum infection with varicocele-related infertility. J Infect Develop Countries (2008) 2(2):116–9. doi: 10.3855/T2.2.116
8. González-Jiménez MA, Villanueva-Díaz CA. Epididymal stereocilia in semen of infertile men: evidence of chronic epididymitis? Andrologia (2006) 38(1):26–30. doi: 10.1111/j.1439-0272.2006.00708.x
9. Colosi HA, Jalali-Zadeh B, Colosi IA, Simon LM, Costache CA. Influence of toxoplasma gondii infection on Male fertility: A pilot study on immunocompetent human volunteers. Iranian J Parasitol (2015) 10(3):402–9.
10. Naumenko V, Tyulenev Y, Kurilo L, Shileiko L, Sorokina T, Evdokimov V, et al. Detection and quantification of human herpes viruses types 4-6 in sperm samples of patients with fertility disorders and chronic inflammatory urogenital tract diseases. Andrology (2014) 2(5):687–94. doi: 10.1111/j.2047-2927.2014.00232.x
11. Vicari E, Arcoria D, Di Mauro C, Noto R, Noto Z, La Vignera S. Sperm output in patients with primary infertility and hepatitis b or c virus; negative influence of HBV infection during concomitant varicocele. Minerva medica (2006) 97(1):65–77.
12. Agarwal A, Rana M, Qiu E, AlBunni H, Bui AD, Henkel R. Role of oxidative stress, infection and inflammation in male infertility. Andrologia (2018) 50(11):e13126. doi: 10.1111/and.13126
13. Mongioì LM, Alamo A, Calogero AE, Compagnone M, Giacone F, Cannarella R, et al. Evaluation of seminal fluid leukocyte subpopulations in patients with varicocele. Int J Immunopathol Pharmacol (2020) 34:2058738420925719. doi: 10.1177/2058738420925719
14. Fang Y, Su Y, Xu J, Hu Z, Zhao K, Liu C, et al. Varicocele-mediated Male infertility: From the perspective of testicular immunity and inflammation. Front Immunol (2021) 12:729539. doi: 10.3389/fimmu.2021.729539
15. Sahin Z, Celik-Ozenci C, Akkoyunlu G, Korgun ET, Acar N, Erdogru T, et al. Increased expression of interleukin-1alpha and interleukin-1beta is associated with experimental varicocele. Fertil Steril (2006) 85 Suppl 1:1265–75. doi: 10.1016/j.fertnstert.2005.10.025
16. Habibi B, Seifi B, Mougahi SM, Ojaghi M, Sadeghipour HR. Increases in interleukin-6 and interferon-gamma levels is progressive in immature rats with varicocele. Irish J Med Sci (2015) 184(2):531–7. doi: 10.1007/s11845-014-1167-3
17. Zeinali M, Hadian Amree A, Khorramdelazad H, Karami H, Abedinzadeh M. Inflammatory and anti-inflammatory cytokines in the seminal plasma of infertile men suffering from varicocele. Andrologia (2017) 49(6):e12685. doi: 10.1111/and.12685
18. Micheli L, Collodel G, Cerretani D, Menchiari A, Noto D, Signorini C, et al. Relationships between ghrelin and obestatin with MDA, proinflammatory cytokines, GSH/GSSG ratio, catalase activity, and semen parameters in infertile patients with leukocytospermia and varicocele. Oxid Med Cell Longev (2019) 2019:7261842. doi: 10.1155/2019/7261842
19. Moretti E, Cosci I, Spreafico A, Serchi T, Cuppone AM, Collodel G. Semen characteristics and inflammatory mediators in infertile men with different clinical diagnoses. Int J Androl (2009) 32(6):637–46. doi: 10.1111/j.1365-2605.2008.00911.x
20. Lotti F, Corona G, Mancini M, Biagini C, Colpi GM, Innocenti SD, et al. The association between varicocele, premature ejaculation and prostatitis symptoms: possible mechanisms. J Sexual Med (2009) 6(10):2878–87. doi: 10.1111/j.1743-6109.2009.01417.x
21. Camargo M, Ibrahim E, Intasqui P, Belardin LB, Antoniassi MP, Lynne CM, et al. Seminal inflammasome activity in the adult varicocele. Hum Fertil (Cambridge England) (2022) 25(3):548–56. doi: 10.1080/14647273.2020.1870756
22. Nallella KP, Allamaneni SS, Pasqualotto FF, Sharma RK, Thomas AJ Jr., Agarwal A. Relationship of interleukin-6 with semen characteristics and oxidative stress in patients with varicocele. Urology (2004) 64(5):1010–3. doi: 10.1016/j.urology.2004.05.045
23. Wang H, Lv Y, Hu K, Feng T, Jin Y, Wang Y, et al. Seminal plasma leptin and spermatozoon apoptosis in patients with varicocele and leucocytospermia. Andrologia (2015) 47(6):655–61. doi: 10.1111/and.12313
24. Finelli R, Pallotti F, Cargnelutti F, Faja F, Carlini T, Rizzo F, et al. Sperm DNA damage and cytokines in varicocele: A case-control study. Andrologia (2021) 53(5):e14023. doi: 10.1111/and.14023
25. Zhang L, Zhao X, Wang F, Lin Q, Wang W. Effects of morinda officinalis polysaccharide on experimental varicocele rats. evidence-based complementary and alternative medicine. eCAM (2016) 2016:5365291. doi: 10.1155/2016/5365291
26. Zalata A, Hafez T, Van Hoecke MJ, Comhaire F. Evaluation of beta-endorphin and interleukin-6 in seminal plasma of patients with certain andrological diseases. Hum Reprod (Oxford England) (1995) 10(12):3161–5. doi: 10.1093/oxfordjournals.humrep.a135879
27. Sakamoto Y, Ishikawa T, Kondo Y, Yamaguchi K, Fujisawa M. The assessment of oxidative stress in infertile patients with varicocele. BJU Int (2008) 101(12):1547–52. doi: 10.1111/j.1464-410X.2008.07517.x
28. Moretti E, Cerretani D, Noto D, Signorini C, Iacoponi F, Collodel G. Relationship between semen IL-6, IL-33 and malondialdehyde generation in human seminal plasma and spermatozoa. Reprod Sci (Thousand Oaks Calif) (2021) 28(8):2136–43. doi: 10.1007/s43032-021-00493-7
29. Allen JD, Gow AJ. Nitrite, NO and hypoxic vasodilation. Br J Pharmacol (2009) 158(7):1653–4. doi: 10.1111/j.1476-5381.2009.00447.x
30. Barati E, Nikzad H, Karimian M. Oxidative stress and male infertility: current knowledge of pathophysiology and role of antioxidant therapy in disease management. Cell Mol Life Sci CMLS (2020) 77(1):93–113. doi: 10.1007/s00018-019-03253-8
31. Poli G, Fabi C, Sugoni C, Bellet MM, Costantini C, Luca G, et al. The role of NLRP3 inflammasome activation and oxidative stress in varicocele-mediated Male hypofertility. Int J Mol Sci (2022) 23(9):5233. doi: 10.3390/ijms23095233
32. Hajipour E, Mashayekhi FJ, Mosayebi G, Baazm M, Zendedel A. Resveratrol decreases apoptosis and NLRP3 complex expressions in experimental varicocele rat model. Iranian J Basic Med Sci (2018) 21(2):225–9. doi: 10.22038/IJBMS.2018.21943.5625
33. Bozhedomov VA, Lipatova NA, Rokhlikov IM, Alexeev RA, Ushakova IV, Sukhikh GT. Male Fertility and varicocoele: role of immune factors. Andrology (2014) 2(1):51–8. doi: 10.1111/j.2047-2927.2013.00160.x
34. Golomb J, Vardinon N, Homonnai ZT, Braf Z, Yust I. Demonstration of antispermatozoal antibodies in varicocele-related infertility with an enzyme-linked immunosorbent assay (ELISA). Fertil Steril (1986) 45(3):397–402. doi: 10.1016/S0015-0282(16)49224-1
35. Djaladat H, Mehrsai A, Rezazade M, Djaladat Y, Pourmand G. Varicocele and antisperm antibody: fact or fiction? South Med J (2006) 99(1):44–7. doi: 10.1097/01.smj.0000197036.08282.70
36. Bozhedomov VA, Lipatova NA, Alexeev RA, Alexandrova LM, Nikolaeva MA, Sukhikh GT. The role of the antisperm antibodies in male infertility assessment after microsurgical varicocelectomy. Andrology (2014) 2(6):847–55. doi: 10.1111/j.2047-2927.2014.00254.x
37. Xu J, Qi L, Chi X, Yang J, Wei X, Gong E, et al. Orchitis: a complication of severe acute respiratory syndrome (SARS). Biol Reproduct (2006) 74(2):410–6. doi: 10.1095/biolreprod.105.044776
38. Wang Z, Xu X. scRNA-seq profiling of human testes reveals the presence of the ACE2 receptor, a target for SARS-CoV-2 infection in spermatogonia, leydig and sertoli cells. Cells (2020) 9(4):920. doi: 10.3390/cells9040920
39. Li H, Xiao X, Zhang J, Zafar MI, Wu C, Long Y, et al. Impaired spermatogenesis in COVID-19 patients. E Clin Med (2020) 28:100604. doi: 10.1016/j.eclinm.2020.100604
40. Wu H, Shi L, Wang Q, Cheng L, Zhao X, Chen Q, et al. Mumps virus-induced innate immune responses in mouse sertoli and leydig cells. Sci Rep (2016) 6:19507. doi: 10.1038/srep19507
41. Bryan ER, Redgrove KA, Mooney AR, Mihalas BP, Sutherland JM, Carey AJ, et al. Chronic testicular chlamydia muridarum infection impairs mouse fertility and offspring development. Biol Reproduct (2020) 102(4):888–901. doi: 10.1093/biolre/ioz229
42. Jacobo P, Guazzone VA, Theas MS, Lustig L. Testicular autoimmunity. Autoimmun Rev (2011) 10(4):201–4. doi: 10.1016/j.autrev.2010.09.026
43. Jacobo P, Guazzone VA, Jarazo-Dietrich S, Theas MS, Lustig L. Differential changes in CD4+ and CD8+ effector and regulatory T lymphocyte subsets in the testis of rats undergoing autoimmune orchitis. J Reprod Immunol (2009) 81(1):44–54. doi: 10.1016/j.jri.2009.04.005
44. Mukasa A, Hiromatsu K, Matsuzaki G, O'Brien R, Born W, Nomoto K. Bacterial infection of the testis leading to autoaggressive immunity triggers apparently opposed responses of alpha beta and gamma delta T cells. J Immunol (Baltimore Md 1950) (1995) 155(4):2047–56. doi: 10.4049/jimmunol.155.4.2047
45. Jing Y, Cao M, Zhang B, Long X, Wang X. cDC1 dependent accumulation of memory T cells is required for chronic autoimmune inflammation in murine testis. Front Immunol (2021) 12:651860. doi: 10.3389/fimmu.2021.651860
46. Aslani F, Schuppe HC, Guazzone VA, Bhushan S, Wahle E, Lochnit G, et al. Targeting high mobility group box protein 1 ameliorates testicular inflammation in experimental autoimmune orchitis. Hum Reprod (Oxford England) (2015) 30(2):417–31. doi: 10.1093/humrep/deu320
47. Fan ZP, Peng ML, Chen YY, Xia YZ, Liu CY, Zhao K, et al. S100A9 activates the immunosuppressive switch through the PI3K/Akt pathway to maintain the immune suppression function of testicular macrophages. Front Immunol (2021) 12:743354. doi: 10.3389/fimmu.2021.743354
48. Bhushan S, Hossain H, Lu Y, Geisler A, Tchatalbachev S, Mikulski Z, et al. Uropathogenic e. coli induce different immune response in testicular and peritoneal macrophages: implications for testicular immune privilege. PloS One (2011) 6(12):e28452. doi: 10.1371/journal.pone.0028452
49. Chen B, Yu L, Wang J, Li C, Zhao K, Zhang H. Involvement of prokineticin 2 and prokineticin receptor 1 in lipopolysaccharide-induced testitis in rats. Inflammation (2016) 39(2):534–42. doi: 10.1007/s10753-015-0277-z
50. Rival C, Theas MS, Guazzone VA, Lustig L. Interleukin-6 and IL-6 receptor cell expression in testis of rats with autoimmune orchitis. J Reprod Immunol (2006) 70(1-2):43–58. doi: 10.1016/j.jri.2005.10.006
51. Suescun MO, Rival C, Theas MS, Calandra RS, Lustig L. Involvement of tumor necrosis factor-alpha in the pathogenesis of autoimmune orchitis in rats. Biol Reproduct (2003) 68(6):2114–21. doi: 10.1095/biolreprod.102.011189
52. Pérez CV, Pellizzari EH, Cigorraga SB, Galardo MN, Naito M, Lustig L, et al. IL17A impairs blood-testis barrier integrity and induces testicular inflammation. Cell Tissue Res (2014) 358(3):885–98. doi: 10.1007/s00441-014-1995-5
53. Wu P, Sun Z, Lv X, Pei X, Manthari RK, Wang J. Fluoride induces autoimmune orchitis involved with enhanced IL-17A secretion in mice testis. J Agric Food Chem (2019) 67(48):13333–43. doi: 10.1021/acs.jafc.9b05789
54. Ok F, Kaplan HM, Kizilgok B, Demir E. Protective effect of alpha-linolenic acid on lipopolysaccharide-induced orchitis in mice. Andrologia (2020) 52(9):e13667. doi: 10.1111/and.13667
55. Xu J, He C, Fang YW, Hu ZY, Peng ML, Chen YY, et al. Testicular exosomes disturb the immunosuppressive phenotype of testicular macrophages mediated by miR-155-5p in uropathogenic escherichia coli-induced orchitis. Asian J Androl (2022) 24:1–9. doi: 10.4103/aja202243
56. Su Y, Zhang Y, Hu Z, He L, Wang W, Xu J, et al. Prokineticin 2 via calcium-sensing receptor activated NLRP3 inflammasome pathway in the testicular macrophages of uropathogenic escherichia coli-induced orchitis. Front Immunol (2020) 11:570872. doi: 10.3389/fimmu.2020.570872
57. Li Y, Su Y, Zhou T, Hu Z, Wei J, Wang W, et al. Activation of the NLRP3 inflammasome pathway by prokineticin 2 in testicular macrophages of uropathogenic escherichia coli- induced orchitis. Front Immunol (2019) 10:1872. doi: 10.3389/fimmu.2019.01872
58. Nicolas N, Michel V, Bhushan S, Wahle E, Hayward S, Ludlow H, et al. Testicular activin and follistatin levels are elevated during the course of experimental autoimmune epididymo-orchitis in mice. Sci Rep (2017) 7:42391. doi: 10.1038/srep42391
59. Guazzone VA, Jacobo P, Theas MS, Lustig L. Cytokines and chemokines in testicular inflammation: A brief review. Microscopy Res Technique (2009) 72(8):620–8. doi: 10.1002/jemt.20704
60. Inoue T, Aoyama-Ishikawa M, Uemura M, Yamashita H, Koga Y, Terashima M, et al. Interleukin-18 levels and mouse leydig cell apoptosis during lipopolysaccharide-induced acute inflammatory conditions. J Reprod Immunol (2020) 141:103167. doi: 10.1016/j.jri.2020.103167
61. Guazzone VA, Jacobo P, Denduchis B, Lustig L. Expression of cell adhesion molecules, chemokines and chemokine receptors involved in leukocyte traffic in rats undergoing autoimmune orchitis. Reprod (Cambridge England) (2012) 143(5):651–62. doi: 10.1530/REP-11-0079
62. Bajic P, Dornbier RA, Doshi CP, Wolfe AJ, Farooq AV, Bresler L. Implications of the genitourinary microbiota in prostatic disease. Curr Urol Rep (2019) 20(7):34. doi: 10.1007/s11934-019-0904-6
63. Krieger JN, Thumbikat P. Bacterial prostatitis: Bacterial virulence, clinical outcomes, and new directions. Microbiol Spectr (2016) 4(1):4.1.01. doi: 10.1128/microbiolspec.UTI-0004-2012
64. Türk S, Korrovits P, Punab M, Mändar R. Coryneform bacteria in semen of chronic prostatitis patients. Int J Androl (2007) 30(2):123–8. doi: 10.1111/j.1365-2605.2006.00722.x
65. Mak TN, Fischer N, Laube B, Brinkmann V, Metruccio MM, Sfanos KS, et al. Propionibacterium acnes host cell tropism contributes to vimentin-mediated invasion and induction of inflammation. Cell Microbiol (2012) 14(11):1720–33. doi: 10.1111/j.1462-5822.2012.01833.x
66. Shoskes DA, Altemus J, Polackwich AS, Tucky B, Wang H, Eng C. The urinary microbiome differs significantly between patients with chronic Prostatitis/Chronic pelvic pain syndrome and controls as well as between patients with different clinical phenotypes. Urology (2016) 92:26–32. doi: 10.1016/j.urology.2016.02.043
67. Nickel JC, Stephens A, Landis JR, Chen J, Mullins C, van Bokhoven A, et al. Search for microorganisms in men with urologic chronic pelvic pain syndrome: A culture-independent analysis in the MAPP research network. J Urol (2015) 194(1):127–35. doi: 10.1016/j.juro.2015.01.037
68. Choi JB, Lee SJ, Kang SR, Lee SS, Choe HS. Analysis of bacterial community using pyrosequencing in semen from patients with chronic pelvic pain syndrome: a pilot study. Trans Androl Urol (2020) 9(2):398–404. doi: 10.21037/tau.2020.02.05
69. Shoskes DA, Wang H, Polackwich AS, Tucky B, Altemus J, Eng C. Analysis of gut microbiome reveals significant differences between men with chronic Prostatitis/Chronic pelvic pain syndrome and controls. J Urol (2016) 196(2):435–41. doi: 10.1016/j.juro.2016.02.2959
70. Konkol Y, Keskitalo A, Vuorikoski H, Pietilä S, Elo LL, Munukka E, et al. Chronic nonbacterial prostate inflammation in a rat model is associated with changes of gut microbiota that can be modified with a galactoglucomannan-rich hemicellulose extract in the diet. BJU Int (2019) 123(5):899–908. doi: 10.1111/bju.14553
71. Mändar R, Punab M, Korrovits P, Türk S, Ausmees K, Lapp E, et al. Seminal microbiome in men with and without prostatitis. Int J Urol Off J Japanese Urol Assoc (2017) 24(3):211–6. doi: 10.1111/iju.13286
72. Murphy SF, Schaeffer AJ, Done JD, Quick ML, Acar U, Thumbikat P. Commensal bacterial modulation of the host immune response to ameliorate pain in a murine model of chronic prostatitis. Pain (2017) 158(8):1517–27. doi: 10.1097/j.pain.0000000000000944
73. Kogan M, Naboka Y, Ferzauli A, Ibishev K, Gudima I, Ismailov R. Does the microbiota spectrum of prostate secretion affect the clinical status of patients with chronic bacterial prostatitis? Int J Urol Off J Japanese Urol Assoc (2021) 28(12):1254–9. doi: 10.1111/iju.14685
74. Skerk V, Schönwald S, Krhen I, Markovinović L, Beus A, Kuzmanović NS, et al. Aetiology of chronic prostatitis. Int J Antimicrob Agents (2002) 19(6):471–4. doi: 10.1016/S0924-8579(02)00087-0
75. Ouzounova-Raykova V, Ouzounova I, Mitov IG. May chlamydia trachomatis be an aetiological agent of chronic prostatic infection? Andrologia (2010) 42(3):176–81. doi: 10.1111/j.1439-0272.2009.00973.x
76. Mazzoli S, Cai T, Addonisio P, Bechi A, Mondaini N, Bartoletti R. Chlamydia trachomatis infection is related to poor semen quality in young prostatitis patients. Eur Urol (2010) 57(4):708–14. doi: 10.1016/j.eururo.2009.05.015
77. Magri V, Perletti G, Stamatiou K, Montanari E, Trinchieri A. Lithogenic potential of ureaplasma in chronic prostatitis. Urol Internationalis (2021) 105(3-4):328–33. doi: 10.1159/000511653
78. Jang KS, Han IH, Lee SJ, Yoo J, Kim YS, Sim S, et al. Experimental rat prostatitis caused by trichomonas vaginalis infection. Prostate (2019) 79(4):379–89. doi: 10.1002/pros.23744
79. Wise GJ, Shteynshlyuger A. How to diagnose and treat fungal infections in chronic prostatitis. Curr Urol Rep (2006) 7(4):320–8. doi: 10.1007/s11934-996-0012-2
80. Vykhovanets EV, Resnick MI, Marengo SR. The healthy rat prostate contains high levels of natural killer-like cells and unique subsets of CD4+ helper-inducer T cells: implications for prostatitis. J Urol (2005) 173(3):1004–10. doi: 10.1097/01.ju.0000149130.06055.f2
81. Vykhovanets EV, Resnick MI, Marengo SR. Intraprostatic lymphocyte profiles in aged wistar rats with estradiol induced prostate inflammation. J Urol (2006) 175(4):1534–40. doi: 10.1016/S0022-5347(05)00652-X
82. Roman K, Done JD, Schaeffer AJ, Murphy SF, Thumbikat P. Tryptase-PAR2 axis in experimental autoimmune prostatitis, a model for chronic pelvic pain syndrome. Pain (2014) 155(7):1328–38. doi: 10.1016/j.pain.2014.04.009
83. Done JD, Rudick CN, Quick ML, Schaeffer AJ, Thumbikat P. Role of mast cells in male chronic pelvic pain. J Urol (2012) 187(4):1473–82. doi: 10.1016/j.juro.2011.11.116
84. Rivero V, Carnaud C, Riera CM. Prostatein or steroid binding protein (PSBP) induces experimental autoimmune prostatitis (EAP) in NOD mice. Clin Immunol (Orlando Fla) (2002) 105(2):176–84. doi: 10.1006/clim.2002.5281
85. Motrich RD, Breser ML, Sánchez LR, Godoy GJ, Prinz I, Rivero VE. IL-17 is not essential for inflammation and chronic pelvic pain development in an experimental model of chronic prostatitis/chronic pelvic pain syndrome. Pain (2016) 157(3):585–97. doi: 10.1097/j.pain.0000000000000405
86. Hua X, Ge S, Zhang M, Mo F, Zhang L, Zhang J, et al. Pathogenic roles of CXCL10 in experimental autoimmune prostatitis by modulating macrophage chemotaxis and cytokine secretion. Front Immunol (2021) 12:706027. doi: 10.3389/fimmu.2021.706027
87. Hu C, Yang H, Zhao Y, Chen X, Dong Y, Li L, et al. The role of inflammatory cytokines and ERK1/2 signaling in chronic prostatitis/chronic pelvic pain syndrome with related mental health disorders. Sci Rep (2016) 6:28608. doi: 10.1038/srep28608
88. Zhang C, Chen J, Wang H, Chen J, Zheng MJ, Chen XG, et al. IL-17 exacerbates experimental autoimmune prostatitis via CXCL1/CXCL2-mediated neutrophil infiltration. Andrologia (2022) 54(8):e14455. doi: 10.1111/and.14455
89. Motrich RD, van Etten E, Baeke F, Riera CM, Mathieu C, Rivero VE. Crucial role of interferon-gamma in experimental autoimmune prostatitis. J Urol (2010) 183(3):1213–20. doi: 10.1016/j.juro.2009.11.008
90. Castiglione R, Salemi M, Vicari LO, Vicari E. Relationship of semen hyperviscosity with IL-6, TNF-α, IL-10 and ROS production in seminal plasma of infertile patients with prostatitis and prostato-vesiculitis. Andrologia (2014) 46(10):1148–55. doi: 10.1111/and.12207
91. Korrovits P, Ausmees K, Mändar R, Punab M. Seminal interleukin-6 and serum prostate-specific antigen as possible predictive biomarkers in asymptomatic inflammatory prostatitis. Urology (2011) 78(2):442–6. doi: 10.1016/j.urology.2011.02.013
92. Liu X, Fan S, Zheng M, Chen J, Zhang J, Li H. The mediation of interleukin-17 and chemokine ligand 2 in pelvic pain of experimental autoimmune prostatitis. Exp Ther Med (2017) 14(1):51–8. doi: 10.3892/etm.2017.4448
93. Motrich RD, Breser ML, Molina RI, Tissera A, Olmedo JJ, Rivero VE. Patients with chronic prostatitis/chronic pelvic pain syndrome show T helper type 1 (Th1) and Th17 self-reactive immune responses specific to prostate and seminal antigens and diminished semen quality. BJU Int (2020) 126(3):379–87. doi: 10.1111/bju.15117
94. Liu H, Zhu X, Cao X, Chi A, Dai J, Wang Z, et al. IL-1β-primed mesenchymal stromal cells exert enhanced therapeutic effects to alleviate chronic Prostatitis/Chronic pelvic pain syndrome through systemic immunity. Stem Cell Res Ther (2021) 12(1):514. doi: 10.1186/s13287-021-02579-0
95. Chen L, Bian Z, Chen J, Meng J, Zhang M, Liang C. Immunological alterations in patients with chronic prostatitis/chronic pelvic pain syndrome and experimental autoimmune prostatitis model: A systematic review and meta-analysis. Cytokine (2021) 141:155440. doi: 10.1016/j.cyto.2021.155440
96. Quick ML, Wong L, Mukherjee S, Done JD, Schaeffer AJ, Thumbikat P. Th1-Th17 cells contribute to the development of uropathogenic escherichia coli-induced chronic pelvic pain. PloS One (2013) 8(4):e60987. doi: 10.1371/journal.pone.0060987
97. Penna G, Mondaini N, Amuchastegui S, Degli Innocenti S, Carini M, Giubilei G, et al. Seminal plasma cytokines and chemokines in prostate inflammation: interleukin 8 as a predictive biomarker in chronic prostatitis/chronic pelvic pain syndrome and benign prostatic hyperplasia. Eur Urol (2007) 51(2):524–33; discussion 33. doi: 10.1016/j.eururo.2006.07.016
98. Quick ML, Mukherjee S, Rudick CN, Done JD, Schaeffer AJ, Thumbikat P. CCL2 and CCL3 are essential mediators of pelvic pain in experimental autoimmune prostatitis. Am J Physiol Regul Integr Comp Physiol (2012) 303(6):R580–9. doi: 10.1152/ajpregu.00240.2012
99. Chen CS, Chang PJ, Lin WY, Huang YC, Ho DR. Evidences of the inflammasome pathway in chronic prostatitis and chronic pelvic pain syndrome in an animal model. Prostate (2013) 73(4):391–7. doi: 10.1002/pros.22580
100. Kashyap M, Pore S, Wang Z, Gingrich J, Yoshimura N, Tyagi P. Inflammasomes are important mediators of prostatic inflammation associated with BPH. J Inflammation (London England) (2015) 12:37. doi: 10.1186/s12950-015-0082-3
101. Lu J, Su Y, Chen X, Chen Y, Luo P, Lin F, et al. Rapamycin−induced autophagy attenuates hormone−imbalance−induced chronic non−bacterial prostatitis in rats via the inhibition of NLRP3 inflammasome−mediated inflammation. Mol Med Rep (2019) 19(1):221–30. doi: 10.3892/mmr.2018.9683
102. Kanamaru S, Kurazono H, Terai A, Monden K, Kumon H, Mizunoe Y, et al. Increased biofilm formation in escherichia coli isolated from acute prostatitis. Int J Antimicrob Agents (2006) 28 Suppl 1:S21–5. doi: 10.1016/j.ijantimicag.2006.05.006
103. Soto SM, Smithson A, Martinez JA, Horcajada JP, Mensa J, Vila J. Biofilm formation in uropathogenic escherichia coli strains: relationship with prostatitis, urovirulence factors and antimicrobial resistance. J Urol (2007) 177(1):365–8. doi: 10.1016/j.juro.2006.08.081
104. Bartoletti R, Cai T, Nesi G, Albanese S, Meacci F, Mazzoli S, et al. The impact of biofilm-producing bacteria on chronic bacterial prostatitis treatment: results from a longitudinal cohort study. World J Urol (2014) 32(3):737–42. doi: 10.1007/s00345-013-1145-9
105. Yang H, Zhang J, Xue Z, Zhao C, Lei L, Wen Y, et al. Potential pathogenic bacteria in seminal microbiota of patients with different types of dysspermatism. Sci Rep (2020) 10(1):6876. doi: 10.1038/s41598-020-63787-x
106. Mehta RH, Sridhar H, Vijay Kumar BR, Anand Kumar TC. High incidence of oligozoospermia and teratozoospermia in human semen infected with the aerobic bacterium streptococcus faecalis. Reprod Biomed Online (2002) 5(1):17–21. doi: 10.1016/S1472-6483(10)61591-X
107. Lai YM, Lee JF, Huang HY, Soong YK, Yang FP, Pao CC. The effect of human papillomavirus infection on sperm cell motility. Fertil Steril (1997) 67(6):1152–5. doi: 10.1016/S0015-0282(97)81454-9
108. Nasseri S, Monavari SH, Keyvani H, Nikkhoo B, Vahabpour Roudsari R, Khazeni M. The prevalence of human papilloma virus (HPV) infection in the oligospermic and azoospermic men. Med J Islamic Republic Iran (2015) 29:272.
109. Kurscheidt FA, Damke E, Bento JC, Balani VA, Takeda KI, Piva S, et al. Effects of herpes simplex virus infections on seminal parameters in Male partners of infertile couples. Urology (2018) 113:52–8. doi: 10.1016/j.urology.2017.11.050
110. Yang T, Zou Y, Zhou W, Ruan Z, Kong Y, Zhou Y, et al. Clonal diversity of ureaplasma species and its relationship with oligozoospermia and semen quality in Chinese infertile males. Eur J Clin Microbiol Infect Dis Off Publ Eur Soc Clin Microbiol (2018) 37(10):1957–63. doi: 10.1007/s10096-018-3331-6
111. López-Hurtado M, Flores-Salazar VR, Gutierréz-Trujillo R, Guerra-Infante FM. Prevalence, concordance and reproductive sequelae after chlamydia trachomatis infection in Mexican infertile couples. Andrologia (2020) 52(10):e13772. doi: 10.1111/and.13772
112. Apaydin T, Sahin B, Dashdamirova S, Dincer Yazan C, Elbasan O, Ilgin C, et al. The association of free testosterone levels with coronavirus disease 2019. Andrology (2022) 10(6):1038–46. doi: 10.1111/andr.13152
113. Joguet G, Mansuy JM, Matusali G, Hamdi S, Walschaerts M, Pavili L, et al. Effect of acute zika virus infection on sperm and virus clearance in body fluids: a prospective observational study. Lancet Infect Dis (2017) 17(11):1200–8. doi: 10.1016/S1473-3099(17)30444-9
114. Erles K, Rohde V, Thaele M, Roth S, Edler L, Schlehofer JR. DNA Of adeno-associated virus (AAV) in testicular tissue and in abnormal semen samples. Hum Reprod (Oxford England) (2001) 16(11):2333–7. doi: 10.1093/humrep/16.11.2333
115. Hussein MR, Abou-Deif ES, Bedaiwy MA, Said TM, Mustafa MG, Nada E, et al. Phenotypic characterization of the immune and mast cell infiltrates in the human testis shows normal and abnormal spermatogenesis. Fertil Steril (2005) 83(5):1447–53. doi: 10.1016/j.fertnstert.2004.11.062
116. Seshadri S, Flanagan B, Vince G, Lewis-Jones DJ. Detection of subpopulations of leucocytes in different subgroups of semen sample qualities. Andrologia (2012) 44 Suppl 1:354–61. doi: 10.1111/j.1439-0272.2011.01189.x
117. Chyra-Jach D, Kaletka Z, Dobrakowski M, Machoń-Grecka A, Kasperczyk S, Bellanti F, et al. Levels of macro- and trace elements and select cytokines in the semen of infertile men. Biol Trace Element Res (2020) 197(2):431–9. doi: 10.1007/s12011-019-02022-9
118. Cui D, Han G, Shang Y, Liu C, Xia L, Li L, et al. Antisperm antibodies in infertile men and their effect on semen parameters: a systematic review and meta-analysis. Clinica Chimica Acta; Int J Clin Chem (2015) 444:29–36. doi: 10.1016/j.cca.2015.01.033
119. Dimitrov DG, Urbánek V, Zve˘rina J, Madar J, Nouza K, Kinský R. Correlation of asthenozoospermia with increased antisperm cell-mediated immunity in men from infertile couples. J Reprod Immunol (1994) 27(1):3–12. doi: 10.1016/0165-0378(94)90011-6
120. Murakami N, Kitajima M, Ohyama K, Aibara N, Taniguchi K, Wei M, et al. Comprehensive immune complexome analysis detects disease-specific immune complex antigens in seminal plasma and follicular fluids derived from infertile men and women. Clinica Chimica Acta; Int J Clin Chem (2019) 495:545–51. doi: 10.1016/j.cca.2019.05.031
Keywords: microbiology, immune, infertility, varicocele, orchitis, prostatitis, oligospermia
Citation: Chen J, Chen J, Fang Y, Shen Q, Zhao K, Liu C and Zhang H (2023) Microbiology and immune mechanisms associated with male infertility. Front. Immunol. 14:1139450. doi: 10.3389/fimmu.2023.1139450
Received: 07 January 2023; Accepted: 06 February 2023;
Published: 21 February 2023.
Edited by:
Yin Sun, University of Rochester Medical Center, United StatesReviewed by:
Kehua Jiang, Guizhou Provincial People’s Hospital, ChinaCopyright © 2023 Chen, Chen, Fang, Shen, Zhao, Liu and Zhang. This is an open-access article distributed under the terms of the Creative Commons Attribution License (CC BY). The use, distribution or reproduction in other forums is permitted, provided the original author(s) and the copyright owner(s) are credited and that the original publication in this journal is cited, in accordance with accepted academic practice. No use, distribution or reproduction is permitted which does not comply with these terms.
*Correspondence: Huiping Zhang, emhwbWVkQDEyNi5jb20=
Disclaimer: All claims expressed in this article are solely those of the authors and do not necessarily represent those of their affiliated organizations, or those of the publisher, the editors and the reviewers. Any product that may be evaluated in this article or claim that may be made by its manufacturer is not guaranteed or endorsed by the publisher.
Research integrity at Frontiers
Learn more about the work of our research integrity team to safeguard the quality of each article we publish.