- 1Division of Dermatology, Department of Medicine, Faculty of Medicine, Ramathibodi Hospital, Mahidol University, Bangkok, Thailand
- 2Division of Allergy, Immunology, and Rheumatology, Department of Medicine, Faculty of Medicine, Ramathibodi Hospital, Bangkok, Thailand
- 3Immunology Laboratory, Department of Pathology, Faculty of Medicine, Ramathibodi Hospital, Mahidol University, Bangkok, Thailand
Background: By depleting circulating B lymphocytes, rituximab time-dependently suppresses coronavirus disease 2019 (COVID-19) vaccines’ humoral immunogenicity for a prolonged period. The optimal time to vaccinate rituximab-exposed immune-mediated dermatologic disease (IMDD) patients is currently unclear.
Objective: To estimate the vaccination timeframe that equalized the occurrence of humoral immunogenicity outcomes between rituximab-exposed and rituximab-naïve IMDD patients.
Methods: This retrospective cohort study recruited rituximab-exposed and age-matched rituximab-naïve subjects tested for severe acute respiratory syndrome coronavirus 2 (SARS-CoV-2)-specific immunity post-vaccination. Baseline clinical and immunological data (i.e., immunoglobulin levels, lymphocyte immunophenotyping) and SARS-CoV-2-specific immunity levels were extracted. The outcomes compared were the percentages of subjects who produced neutralizing antibodies (seroconversion rates, SR) and SARS-CoV-2-specific IgG levels among seroconverters. The outcomes were first analyzed using multiple regressions adjusted for the effects of corticosteroid use, steroid-spearing agents, and pre-vaccination immunological status (i.e., IgM levels, the percentages of the total, naïve, and memory B lymphocytes) to identify rituximab-related immunogenicity outcomes. The rituximab-related outcome differences with a 95% confidence interval (CI) between groups were calculated, starting by including every subject and then narrowing down to those with longer rituximab-to-vaccination intervals (≥3, ≥6, ≥9, ≥12 months). The desirable cut-off performances were <25% outcome inferiority observed among rituximab-exposed subgroups compared to rituximab-naïve subjects, and the positive likelihood ratio (LR+) for the corresponding outcomes ≥2.
Findings: Forty-five rituximab-exposed and 90 rituximab-naive subjects were included. The regression analysis demonstrated a negative association between rituximab exposure status and SR but not with SARS-CoV-2-specific IgG levels. Nine-month rituximab-to-vaccination cut-off fulfilled our prespecified diagnostic performance (SR difference between rituximab-exposed and rituximab-naïve group [95%CI]: -2.6 [-23.3, 18.1], LR+: 2.6) and coincided with the repopulation of naïve B lymphocytes in these patients.
Conclusions: Nine months of rituximab-to-vaccination interval maximize the immunological benefits of COVID-19 vaccines while avoiding unnecessary delay in vaccination and rituximab treatment for IMDD patients.
1 Introduction
During the pre-vaccination period, the coronavirus disease 2019 (COVID-19) pandemic substantially burdened the world medically and socioeconomically, with the major determinant of patients’ survival relying solely on supportive measures (1). By inducing certain inflammatory cascades (2), COVID-19 vaccination induces the production of pathogen-specific neutralizing antibodies (3), which plays a significant role in decelerating the disease spreading and reducing the cases of COVID-19-related organ failure, hospitalization and mortality, allowing a gradual resume of pre-pandemic lifestyle in many parts of the world (4, 5). Nonetheless, emerging COVID-19 variants and the spike in disease incidence associated with waning immunity emphasize the importance of maintaining high-level immunity to prevent the COVID-19 pandemic from rehappening (6, 7). Achieving this goal can be challenging for patients who require rituximab treatment, as numerous studies demonstrated markedly decreased humoral immunogenicity of COVID-19 vaccines in these patients, possibly resulting in an increased susceptibility to infection than the general population (8–10). In addition, given their comorbidities and immunosuppressed status, managing severe COVID-19 in these patients can be extremely challenging both in the diagnosis (11) and treatment aspects (1, 12), making it necessary to maximize protection via adequate passive immunity (13–15). With the increasing indications of rituximab treatment in dermatological conditions (16–19), immune-mediated dermatologic disease (IMDD) patients are among those whose immune responses to COVID-19 vaccines can be affected.
Our previous study showed that rituximab use was associated with non-seroconversion following COVID-19 vaccination among autoimmune bullous disease patients (20). Avoiding vaccination while rituximab is taking effect, whereby completing vaccination 2-4 weeks before rituximab treatment, is suggested by international practice guidelines (21, 22). However, this recommendation may not be practicable for some IMDD patients, such as patients with severe pemphigus, as their disease circumstance may benefit from urgent rituximab treatment (23, 24). For IMDD patients who need to be vaccinated after drug administration, the optimal time for COVID-19 vaccination is still being determined. Therefore, this study aimed to determine the earliest rituximab-to-vaccination interval cut-off for successful vaccination among rituximab-treated IMDD patients by comparing the COVID-19 vaccine’s humoral immunogenicity outcomes measured from rituximab-exposed subjects with various rituximab-to-vaccination intervals to those of rituximab-naïve individuals.
2 Materials and methods
2.1 Study design and patient selection
We employed a cohort study design to estimate the vaccination timeframe that equalized the immunogenicity outcomes between rituximab-exposed and rituximab-naïve IMDD patients. This retrospective pilot cohort study screened participants of previous prospective cohort studies conducted in our institutions for eligibility. These studies evaluated either or both humoral and cellular immune responses to various types of COVID-19 vaccines in IMDD patients 28 days post-vaccination (20, 25). Adult (≥ 18 years old) patients diagnosed with IMDD or cutaneous manifestations of connective tissue diseases (e.g., systemic lupus erythematosus with cutaneous lupus erythematosus, rheumatoid arthritis with cutaneous vasculitis, dermatomyositis, systemic sclerosis, mixed connective tissue disease) tested for SARS-CoV-2-specific humoral immunity levels after vaccination were included in this study. Subjects receiving at least one course of rituximab per any standard protocols before vaccination were included as an exposed group (26). Subjects who had never received rituximab were recruited as the unexposed group. A ratio between the number of rituximab-exposed to rituximab-naive subjects was set at 1:2. The two groups were matched by age groups (18-<40, 40-<60, ≥60 years old). The subjects were excluded if they received rituximab between the first dose of the vaccine and the date of the vaccine’s immunogenicity assessment. This study was in full compliance with the declaration of Helsinki and was approved by the Human Research Ethics Committee, Faculty of Medicine Ramathibodi Hospital, Mahidol University (MURA 2022/686, Thai Clinical Trails Registry No. 20221213001), which granted a waiver of consent.
2.2 Data sources and extraction
Data were extracted from the hospital’s electronic medical record and case record forms of the original cohort studies and transferred to new case record forms in a deidentified manner. Clinical information collected includes age, sex, the diagnosis of immune-mediated dermatologic diseases, other comorbidities, immunosuppressive drugs used listed in medical records of every clinic visit, baseline immunological assessment such as immunoglobulin levels, peripheral blood immunophenotyping before vaccination (if available). Treatment protocols and the date of the last dose received were documented for rituximab-exposed patients. COVID-19 vaccines-related data extracted comprises the types of COVID-19 vaccines received, the date of vaccination, the magnitude of humoral immune responses to COVID-19 vaccines in the forms of SARS-CoV-2-specific binding IgG or neutralizing antibody (NAb) levels, and the presence or absence of SARS-CoV-2-specific cellular immunity was demonstrated either by interferon-γ release assay or enzyme-linked immune absorbent spot test.
2.3 Operational definition of humoral immunogenicity outcomes
The primary outcome was the ability to produce the SARS-CoV-2-specific NAb (i.e., seroconversion probability). Seroconversion probability was calculated as the proportion of those who tested positive for NAb within the group and defined as seroconversion rates (SR). NAb was measured by the surrogate viral neutralization test (sVNT) (SARS-CoV-2-NeutraLISA, Euroimmun, Lübeck, Germany), which quantifies the capacity of the patient-produced NAb in inhibiting the in-vitro interaction between human angiotensin-converting enzyme 2 receptors and SARS-CoV-2 S1 receptor binding protein (RBD). Results were reported as the percentages of reactions inhibited ranging from 0-100% (positive threshold: 35%). The secondary outcome was the magnitude of humoral immune responses to COVID-19 vaccines in seroconverters represented by SARS-CoV-2 S1 RBD IgG level measured by chemiluminescent microparticle immunoassay (SARS-CoV-2 IgG II SEMI QUANT assay, Abbott, Chicago, US), which was more suitable than sVNT for this purpose because its limit of detection was broader than sVNT.
2.4 Statistical analysis
STATA 17.0 (StataCorp LLC, TX, US) was used for analysis with p < 0.05 as a statistically significant threshold. Categorical variables were summarized as percentages and continuous variables as means with standard deviations or medians with interquartile ranges (IQR) depending on data distribution. Subjects with missing outcome data were excluded prior to analysis.
We defined the rituximab-to-vaccination interval cut-offs for successful vaccination as the time when the SR of rituximab-exposed subjects was comparable to those of rituximab-naïve subjects. To identify this timeframe, we divided the analyses into two steps. First, the primary and secondary outcomes were compared between rituximab-exposed and rituximab-naïve groups using chi-squared and rank-sum tests. The immunogenicity outcomes that showed a statistically significant difference between groups subsequently underwent regression analyses adjusted for corticosteroid use, steroid-spearing agents, and other possible confounders found during univariate analysis to ensure that the differences in the occurrence of outcomes were not attributable to non-rituximab immunosuppressants. Outcomes which retained significant associations with rituximab exposure status were used for rituximab-to-vaccination interval cut-off analysis. Second, the mean or proportional differences and their 95% confidence interval (CI) in the rituximab-related immunogenicity outcomes between rituximab-exposed and rituximab-naïve groups were calculated. The desirable diagnostic performances of the cut-offs were set as follows; the immunogenicity outcomes observed among rituximab-exposed subgroups were less than 25% inferior to those of rituximab-naïve subjects, and the positive likelihood ratio for predicting the immunogenicity outcomes at least two. The comparisons were started by including every subject and then narrowed down to rituximab-exposed subgroups with longer rituximab-to-vaccination intervals, starting at ≥3 months and increasing the cut-offs sequentially at three-month intervals until reaching ≥12 months.
3 Results
3.1 Clinical characteristics of study participants
Forty-seven IMDD patients with a history of rituximab exposure before vaccination were identified from the database; 45 were included as two subjects received rituximab again before the immunogenicity assessment date. Ninety rituximab-naïve subjects were included in the unexposed group (Table 1). Post-vaccination SARS-CoV-2-specific S1 RBD IgG and sVNT were measured in all subjects, while SARS-CoV-2-specific cell-mediated immunity was evaluated in 61%. All rituximab-exposed subjects received rheumatoid arthritis treatment protocol (two intravenous infusions of rituximab 1,000 mg two weeks apart); the intervals between rituximab and vaccination ranged from 80 to 931 days (median [IQR]: 365 [151, 783]). Approximately 60% of the study subjects were patients with autoimmune bullous dermatoses; the rest were diagnosed with connective tissue diseases. Subjects in both groups used similar immunosuppressive therapies. The types of COVID-19 vaccines were equally distributed between groups, with the majority of subjects receiving a viral vector vaccine (i.e., ChadOx1-s recombinant). Baseline immunoglobulin level measurements and lymphocyte immunophenotyping performed in 93.3% of subjects showed more rituximab-exposed subjects with depleted B lymphocytes and hypoIgM than rituximab-naïve subjects. There was no significant difference in the overall number of peripheral blood T lymphocyte and B lymphocyte subsets between rituximab-exposed and rituximab naïve groups.
3.2 Immunogenicity outcomes among study participants
Overall, the primary outcome (i.e., seroconversion) was found less frequently among rituximab-exposed subjects than in the rituximab-naïve group (Table 1). For the secondary immunogenicity outcomes, anti-SARS-CoV-2 S1 RBD IgG levels measured from seroconverted subjects did not significantly differ between the two groups, suggesting that rituximab-exposed subjects can mount the humoral immune response to COVID-19 vaccines of similar strength to rituximab-naïve subjects once they were able to produce NAb. In 11 (24.4%) rituximab-exposed and 18 (20.0%) rituximab-naïve subjects, the attenuated humoral immune responses to COVID-19 manifested as the production of SARS-CoV-2 binding antibodies without viral neutralizing capacity (i.e., tested positive for SARS-CoV-2-specific S1 RBD IgG but negative for neutralizing antibody by sVNT). The proportion of subjects who mounted cell-mediated immunity was also similar between the two groups. Multiple logistic regression analysis demonstrated an inverse correlation between rituximab exposure status and seroconversion (correlation coefficient [95%CI]: -1.53 [-2.57, -0.51], p=0.004) after adjusted for the effects of corticosteroid dosages, the use of steroid-sparing agents, and pre-vaccination immunological status (i.e., IgM levels, the percentages of the total, naïve, and memory B lymphocytes). The analysis also showed a positive association between seroconversion and the percentage of naïve B lymphocytes (correlation coefficient [95%CI]: 0.04 [0.01, 0.08], p=0.009) but not with the percentages of total and memory B lymphocytes. Regression analyses adjusted for the same covariates did not find an association between the anti-SARS-CoV-2 S1 RBD IgG levels and rituximab exposure status. These findings concluded that SR but not anti-SARS-CoV-2 S1 RBD IgG levels as rituximab-related immunogenicity outcomes. SR was therefore used as the outcome variable during cut-off analysis.
3.3 Rituximab-to-vaccination intervals: cut-off analysis
Immunogenicity outcomes among rituximab-exposed subjects were illustrated by their rituximab-to-vaccination intervals in Figure 1, which showed the clustering of seroconverters towards the longer rituximab-to-vaccine intervals. Most non-seroconverters completed COVID-19 vaccination within the first six-month post-rituximab administration. The first patient who was able to produce NAb was vaccinated at approximately six months after rituximab administration; the number of seroconverters gradually increased after about nine months had passed from rituximab treatment, although some patients still could not produce NAb despite being vaccinated two-year post-rituximab. Subgroup analyses determining rituximab-to-vaccination intervals cut-offs for successful vaccination demonstrated that the cut-offs of at least six months raised the likelihood ratio for seroconversion to above two (Table 2). Additionally, nine months or above were required for the outcome differences between rituximab-exposed and rituximab-naïve groups to decrease below 25%. The earliest rituximab-to-vaccination cut-off, which fulfilled our prespecified desirable diagnostic performance, is nine months. Examining B lymphocyte subpopulations in subjects with different rituximab-to-vaccination intervals revealed naïve cell-predominant populations among subjects with longer rituximab-to-vaccination intervals (Figure 2). The median [IQR] percentages of pre-vaccination naïve B lymphocytes in subjects vaccinated nine months before and after rituximab treatment were 0.8 [0-3.9] and 81.9 [68.1-87.5], respectively (p<0.001).
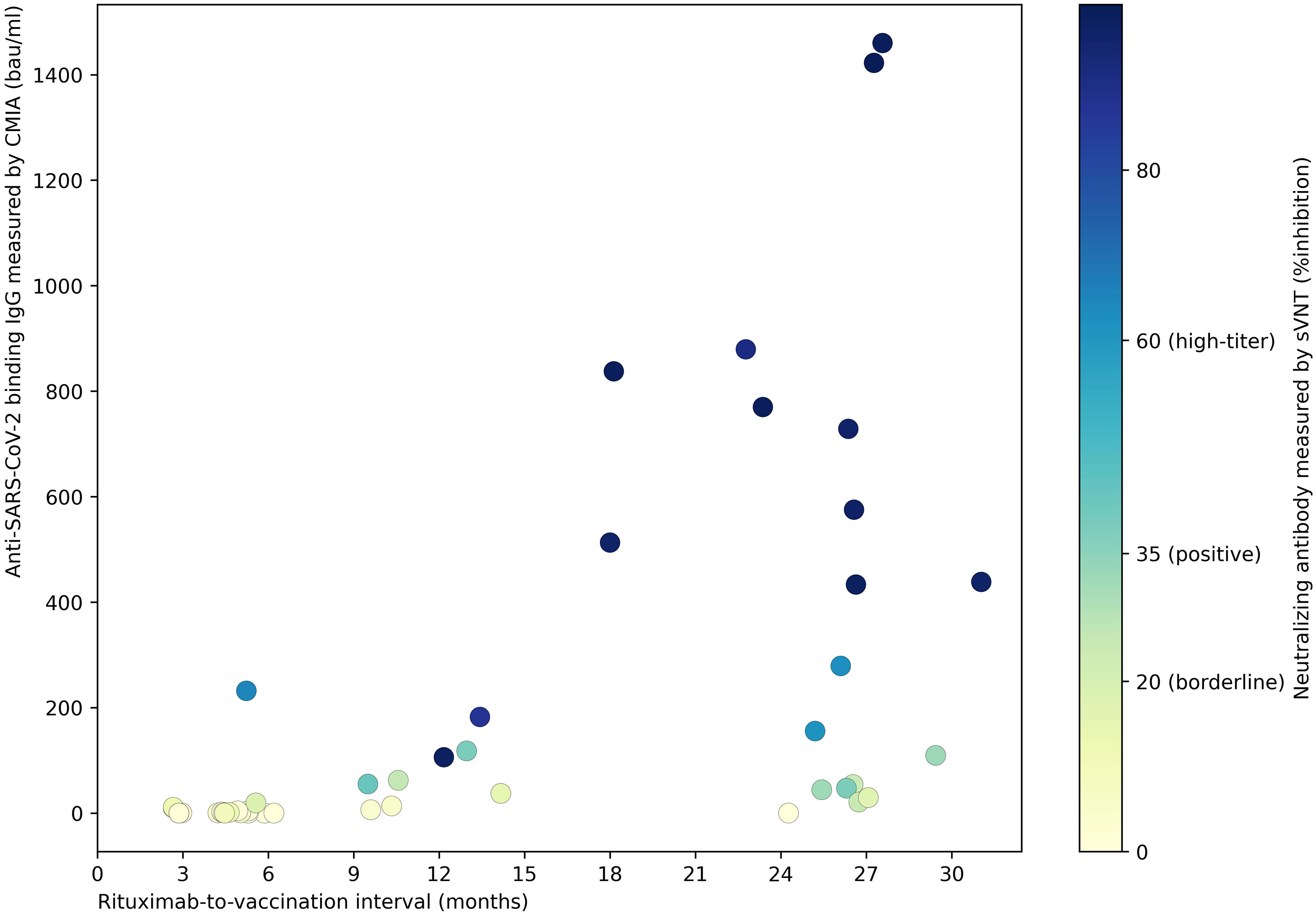
Figure 1 The strength of humoral immune responses† to COVID-19 vaccines among rituximab-exposed IMDD patients with different rituximab-to-vaccination intervals. Post-vaccination SARS-CoV-2-specific binding and neutralizing antibody levels were demonstrated. NAb production was observed in IMDD patients vaccinated as early as six months post-rituximab administration, with a stronger humoral immune response as rituximab-to-vaccination intervals were extended. Some patients could not produce NAb despite being vaccinated two-year post-rituximab. †CMIA (SARS-CoV-2 IgG II SEMI QUANT assay, Abbott, Chicago, US) measured the serum concentration of antibodies bound to SARS-CoV-2 S1 receptor binding protein. sVNT (SARS-CoV-2-NeutraLISA, Euroimmun, Lübeck, Germany) provides an in-vitro evaluation of the ability of anti-SARS-CoV-2 S1 IgG in the patient’s serum to prevent the interaction between SARS-CoV-2 S1 and human angiotensin-converting enzyme 2. bau, international binding antibody unit; CMIA, chemiluminescent microparticle immunoassay; IMDD, immune-mediated dermatologic diseases; NAb, neutralizing antibody; SARS-CoV-2; severe acute respiratory syndrome 2; sVNT, surrogate viral neutralization test.
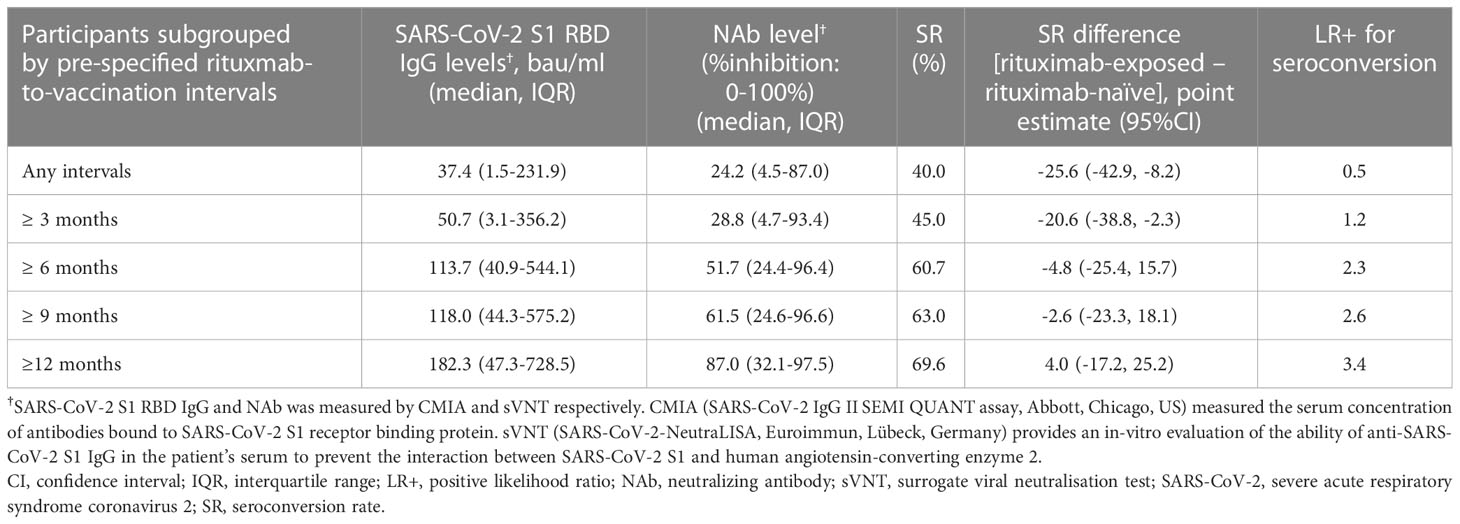
Table 2 SARS-CoV-2-specific humoral immunity levels achieved by rituximab users with different ranges of rituximab-to-vaccination intervals.
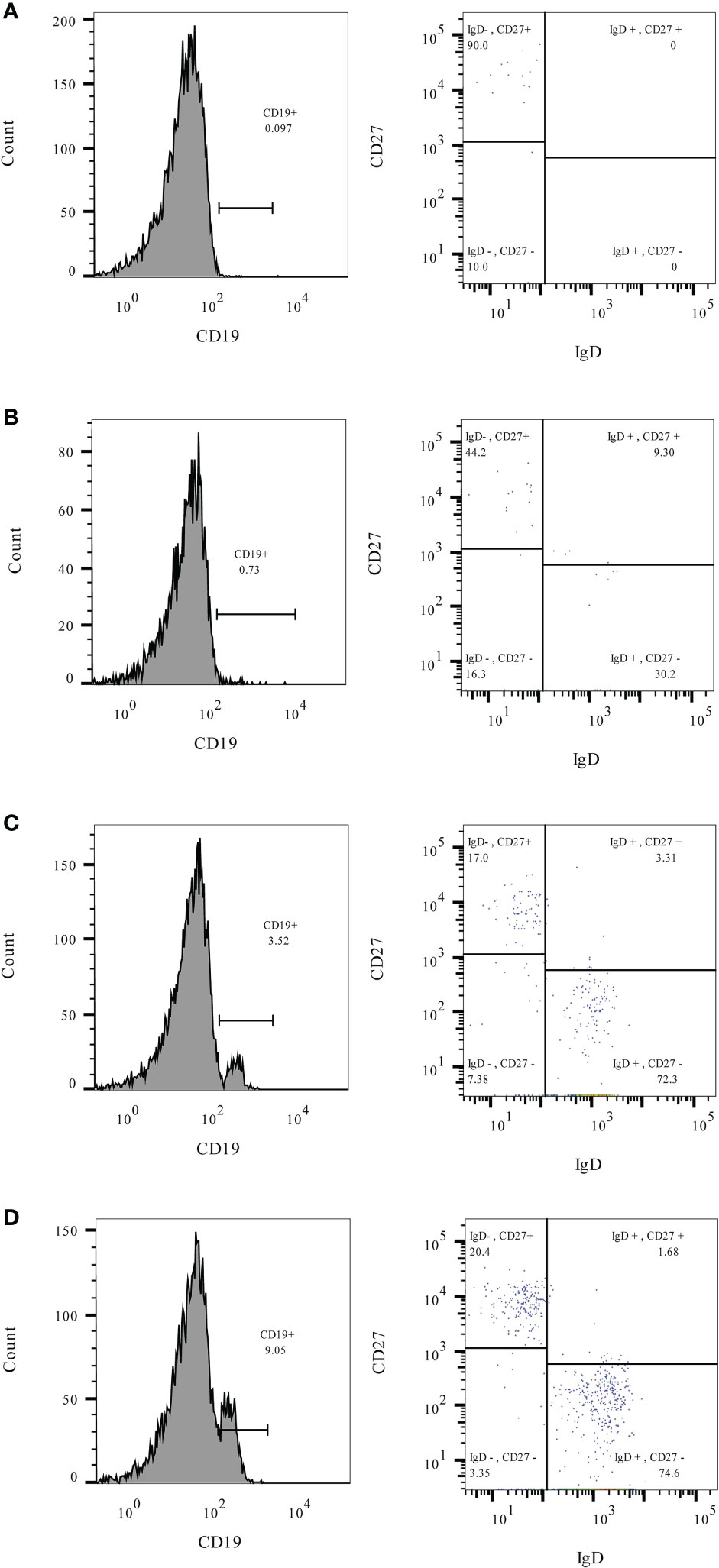
Figure 2 The percentages of total (CD19+), naïve (CD19+/IgD+/CD27-), and memory (CD19+/IgD-/CD27+) B lymphocytes measured before COVID-19 vaccination in rituximab-exposed subjects with different rituximab-to-vaccination intervals. Examples of pre-vaccination B lymphocyte immunophenotyping† were demonstrated for rituximab-exposed subjects with rituximab-to-vaccination intervals of 3-6 months (A), 6-9 months (B), 9-12 months (C), and more than 12 months (D). Naïve B lymphocytes predominated the B lymphocyte population of subjects with rituximab-to-vaccination intervals longer than nine months. †Leukocytes were prepared for flow cytometry from 25µL of fresh heparinized blood using Lyse/Stain/Wash method. Fluorescent-conjugated antibodies used for staining include C45-PE (Cat. No. 368510 Biolegend, SD, CA), CD19-PerCP-Cy5.5 (Cat. No. 45-0199-42, Thermo Fisher Scientific, WLM, MA), IgD-FITC (Cat. No. 11-9868-42, Thermo Fisher Scientific, WLM, MA), and CD27-PE-Cy7 (Cat. No. 25-0271-82, Thermo Fisher Scientific, WLM, MA). Lymphocytes were identified by size (forward scatter) and granularity (side scatter) and confirmed with surface expression of CD19 and CD45. CD19+ B lymphocytes were gated as naïve or memory subsets based on their IgD and CD27 expression patterns.
4 Discussion
The negative impact of rituximab on vaccines’ immunogenicity has been demonstrated for many vaccines before the arrival of COVID-19 vaccines (27–29). Despite the differences in dosage and timing of drug administration, most patients who required repeated courses of rituximab treatment, including those with IMDD, have struggled to mount humoral immunity following COVID-19 vaccines (8, 9, 20, 30–32). Attempts to rectify the situation by using more-immunogenic vaccine platforms (e.g., mRNA and viral vector technologies), lowering rituximab dose, or giving additional vaccine doses have not shown promising benefits (32–36). Previous COVID-19 vaccine immunogenicity studies involving patients with systemic autoimmune diseases, multiple sclerosis, and lymphoma suggested that the key to successful COVID-19 vaccination among rituximab users is to extend the interval between rituximab treatment and vaccination (37–39). In keeping with the previous studies, we also found a positive correlation between the longer rituximab-to-vaccination intervals and the seroconversion probability of rituximab-exposed IMDD patients.
Current evidence has placed the preferential vaccination time frame for rituximab users at ≥ 12 months after the rituximab treatment (37, 40); delaying rituximab administration to match this timeframe may not be in the patient’s best interest for IMDD patients who may require annual or biannual maintenance therapy to control the disease activity or prevent relapse (41). The critical question is, “what would be the appropriate timing to vaccinate IMDD patients after rituximab treatment?” Experts suggest waiting at least five months after the last rituximab administration (21, 22). Our study, corresponding to others, found that vaccinating at less than six months post-rituximab is extremely low yielded since the humoral immune response to COVID-19 vaccines was virtually blocked by rituximab during this period (42–45). IMDD patients who required rituximab every six months should be considered for alternative COVID-19 preventive measures, for instance, passive immunization with Tixagevimab/Cilgavimab injection (i.e., human monoclonal antibodies against the surface spike protein of SARS-CoV-2). For patients with an allowable gap of rituximab treatment longer than six months, we proposed a vaccination timeframe of at least nine months post-rituximab to balance the vaccine immunogenicity against the delay in vaccination. This cut-off was supported by two other clinical studies in rheumatic disease patients; one of them also found the reappearance of circulating naïve B lymphocytes at this time, similar to our finding (38, 46). Interestingly, based on our calculated positive likelihood ratios for predicting seroconversion, extending rituximab-to-vaccination time beyond 12 months did not substantially escalate the seroconversion rate among rituximab-exposed IMDD patients as the positive likelihood ratios remained less than five.
Although a low number of circulating CD19+ B lymphocytes has been proposed as a marker of vaccine non-responders (47, 48), a few studies have argued against this idea as they found that B-cell depleted patients with as few as 10 circulating B lymphocytes per microliter (0.4% of lymphocytes) can seroconvert (49, 50). Our regression analysis suggests the percentage of pre-vaccination naïve B lymphocytes as a better marker for successful vaccination than the total B lymphocytes since its association with seroconversion probability is independent not only of the percentages of total B lymphocytes but also of the rituximab exposure status, although its use falls short on the lack of standard normal value. Further analysis of other B lymphocytes subpopulations with a good correlation with vaccine responses (e.g., HLA-DR-hi, CXCR-hi, CD95-low, CD21-low B lymphocytes) (51) and its interactions with other rituximab-affected components of the immune system may be required to comprehensively analyze the key elements that drive the immune response to vaccination for IMDD patients. As predicting vaccination response via immunophenotyping is a work in progress, the use of rituximab-to-vaccination interval to determine an appropriate vaccination timeframe for rituximab-exposed IMDD patients is a more pragmatic solution for the status quo.
5 Conclusion
In conclusion, this study proposed a nine-month rituximab-to-vaccination interval as a time point that maximizes the immunological benefits of COVID-19 vaccines while avoiding unnecessary delay in vaccination and rituximab treatment for IMDD patients. A larger study may be warranted to confirm our finding as this is a relatively small single-centered study.
Data availability statement
The raw data supporting the conclusions of this article will be made available by the authors, without undue reservation.
Ethics statement
The study involving de-identified data of human participants was reviewed and approved by the Human Research Ethics Committee, Faculty of Medicine Ramathibodi Hospital, Mahidol University, which granted a waiver of consent.
Author contributions
All authors conceptualized and planed the study methodology. CS-a, YR, SC, TS, CS and PO-C collected the data. CS-a and YR performed data curation and conducted formal analysis. CS-a created data visualisation and wrote the original draft of the manuscript. KC, PR, PS, PO-C, and PP supervised the project administration. All authors contributed to the article and approved the submitted version.
Funding
Division of Dermatology, Department of Internal Medicine, Faculty of Medicine Ramathibodi Hospital; Mahidol University’s Specific League funds, and Mahidol University’s post-graduate program research funds.
Acknowledgments
We would like to thank Assist. Prof. Kunlawat Thadanipon from the Department of Clinical Epidemiology and Biostatistics, Faculty of Medicine Ramathibodi Hospital, Mahidol University, for the statistical consultation for the data analysis.
Conflict of interest
The authors declare that the research was conducted in the absence of any commercial or financial relationships that could be construed as a potential conflict of interest.
Publisher’s note
All claims expressed in this article are solely those of the authors and do not necessarily represent those of their affiliated organizations, or those of the publisher, the editors and the reviewers. Any product that may be evaluated in this article, or claim that may be made by its manufacturer, is not guaranteed or endorsed by the publisher.
References
1. Pelosi P, Tonelli R, Torregiani C, Baratella E, Confalonieri M, Battaglini D, et al. Different methods to improve the monitoring of noninvasive respiratory support of patients with severe Pneumonia/ARDS due to COVID-19: An update. J Clin Med (2022) 11(6):1704. doi: 10.3390/jcm11061704
2. Zhang W, Li D, Xu B, Xu L, Lyu Q, Liu X, et al. Serum peptidome profiles immune response of COVID-19 vaccine administration. Front Immunol (2022) 13. doi: 10.3389/fimmu.2022.956369
3. Rudi E, Martin AP, Zurita E, Ledesma GLMM, Bottero D, Malito J, et al. Immunological study of COVID-19 vaccine candidate based on recombinant spike trimer protein from different SARS-CoV-2 variants of concern. Front Immunol (2022) 13:1020159. doi: 10.3389/fimmu.2022.1020159
4. Watson OJ, Barnsley G, Toor J, Hogan AB, Winskill P, Ghani AC. Global impact of the first year of COVID-19 vaccination: A mathematical modelling study. Lancet Infect Dis (2022) 22(9):1293–302. doi: 10.1016/S1473-3099(22)00320-6
5. Khoury DS, Cromer D, Reynaldi A, Schlub TE, Wheatley AK, Juno JA, et al. Neutralizing antibody levels are highly predictive of immune protection from symptomatic SARS-CoV-2 infection. Nat Med (2021) 27(7):1205–11. doi: 10.1038/s41591-021-01377-8
6. Hernandez-Suarez C, Murillo-Zamora E. Waning immunity to SARS-CoV-2 following vaccination or infection. Front Med (Lausanne). (2022) 9:972083. doi: 10.3389/fmed.2022.972083
7. Collatuzzo G, Visci G, Violante FS, Porru S, Spiteri G, Monaco MGL, et al. Determinants of anti-s immune response at 6 months after COVID-19 vaccination in a multicentric European cohort of healthcare workers - ORCHESTRA project. Front Immunol (2022) 13:986085. doi: 10.3389/fimmu.2022.986085
8. Moor MB, Suter-Riniker F, Horn MP, Aeberli D, Amsler J, Möller B, et al. Humoral and cellular responses to mRNA vaccines against SARS-CoV-2 in patients with a history of CD20 b-cell-depleting therapy (RituxiVac): An investigator-initiated, single-centre, open-label study. Lancet Rheumatol (2021) 3(11):e789–e97. doi: 10.1016/S2665-9913(21)00251-4
9. Rotterdam J, Thiaucourt M, Weiss C, Schwaab J, Reiter A, Kreil S, et al. Definition of factors associated with negative antibody response after COVID-19 vaccination in patients with hematological diseases. Ann Hematol (2022) 101(8):1825–34. doi: 10.1007/s00277-022-04866-z
10. Seree-Aphinan C, Suchonwanit P, Rattanakaemakorn P, Pomsoong C, Ratanapokasatit Y, Setthaudom C, et al. Risk-benefit profiles associated with receiving moderna COVID-19 (mRNA-1273) vaccine as an additional pre-booster dose in immune-mediated dermatologic disease patients with low SARS-CoV-2-specific immunity following the primary series: A prospective cohort study. J Eur Acad Dermatol Venereol (2023). doi: 10.1111/jdv.18890
11. Orlandi M, Landini N, Sambataro G, Nardi C, Tofani L, Bruni C, et al. The role of chest CT in deciphering interstitial lung involvement: systemic sclerosis versus COVID-19. Rheumatol (Oxford). (2022) 61(4):1600–9. doi: 10.1093/rheumatology/keab615
12. Vetrugno L, Castaldo N, Fantin A, Deana C, Cortegiani A, Longhini F, et al. Ventilatory associated barotrauma in COVID-19 patients: A multicenter observational case control study (COVI-MIX-study). Pulmonology (2022). doi: 10.1016/j.pulmoe.2022.11.002
13. Levavi H, Lancman G, Gabrilove J. Impact of rituximab on COVID-19 outcomes. Ann Hematol (2021) 100(11):2805–12. doi: 10.1007/s00277-021-04662-1
14. Furlan A, Forner G, Cipriani L, Vian E, Rigoli R, Gherlinzoni F, et al. COVID-19 in b cell-depleted patients after rituximab: A diagnostic and therapeutic challenge. Front Immunol (2021) 12:763412. doi: 10.3389/fimmu.2021.763412
15. Sparks JA, Wallace ZS, Seet AM, Gianfrancesco MA, Izadi Z, Hyrich KL, et al. Associations of baseline use of biologic or targeted synthetic DMARDs with COVID-19 severity in rheumatoid arthritis: Results from the COVID-19 global rheumatology alliance physician registry. Ann Rheum Dis (2021) 80(9):1137–46. doi: 10.1136/annrheumdis-2021-220418
16. Lowe CD, Brahe CA, Green B, Lam TK, Meyerle JH. Bullous systemic lupus erythematosus successfully treated with rituximab. Cutis. (2019) 103(6):E5–e7.
17. Coffey CM, Richter MD, Crowson CS, Koster MJ, Warrington KJ, Ytterberg SR, et al. Rituximab therapy for systemic rheumatoid vasculitis: Indications, outcomes, and adverse events. J Rheumatol (2020) 47(4):518–23. doi: 10.3899/jrheum.181397
18. Werth VP, Joly P, Mimouni D, Maverakis E, Caux F, Lehane P, et al. Rituximab versus mycophenolate mofetil in patients with pemphigus vulgaris. N Engl J Med (2021) 384(24):2295–305. doi: 10.1056/NEJMoa2028564
19. Smith V, Pizzorni C, Riccieri V, Decuman S, Brusselle G, DEP M, et al. Stabilization of microcirculation in patients with early systemic sclerosis with diffuse skin involvement following rituximab treatment: An open-label study. J Rheumatol (2016) 43(5):995–6. doi: 10.3899/jrheum.151018
20. Chanprapaph K, Seree-aphinan C, Rattanakaemakorn P, Pomsoong C, Ratanapokasatit Y, Setthaudom C, et al. A real-world prospective cohort study of immunogenicity and reactogenicity of ChAdOx1-s[recombinant] among patients with immune-mediated dermatologic diseases. Br J Dermatol (2023) 118(2):268–77. doi: 10.1093/bjd/ljac045
21. Landewe RBM, Kroon FPB, Alunno A, Najm A, Bijlsma JW, Burmester GR, et al. EULAR recommendations for the management and vaccination of people with rheumatic and musculoskeletal diseases in the context of SARS-CoV-2: the November 2021 update. Ann Rheum Dis (2022) 81(12):1628–39. doi: 10.1136/annrheumdis-2021-222006
22. Curtis JR, Johnson SR, Anthony DD, Arasaratnam RJ, Baden LR, Bass AR, et al. American College of rheumatology guidance for COVID-19 vaccination in patients with rheumatic and musculoskeletal diseases: Version 4. Arthritis Rheumatol (2022) 74(5):e21–36. doi: 10.1002/art.42109
23. Zhao W, Wang J, Zhu H, Pan M. Comparison of guidelines for management of pemphigus: A review of systemic corticosteroids, rituximab, and other immunosuppressive therapies. Clin Rev Allergy Immunol (2021) 61(3):351–62. doi: 10.1007/s12016-021-08882-1
24. Kanokrungsee S, Anuntrangsee T, Tankunakorn J, Srisuwanwattana P, Suchonwanit P, Chanprapaph K. Rituximab therapy for treatment of pemphigus in southeast asians. Drug Des Devel Ther (2021) 15:1677–90. doi: 10.2147/DDDT.S306046
25. Seree-Aphinan C, Chanprapaph K, Rattanakaemakorn P, Setthaudom C, Suangtamai T, Pomsoong C, et al. Inactivated COVID-19 vaccine induces a low humoral immune response in a subset of dermatological patients receiving immunosuppressants. Front Med (Lausanne). (2021) 8:769845. doi: 10.3389/fmed.2021.769845
26. Kanwar AJ, Vinay K. Rituximab in pemphigus. Indian J Dermatol Venereol Leprol. (2012) 78(6):671–6. doi: 10.4103/0378-6323.102354
27. Rehnberg M, Brisslert M, Amu S, Zendjanchi K, Håwi G, Bokarewa MI. Vaccination response to protein and carbohydrate antigens in patients with rheumatoid arthritis after rituximab treatment. Arthritis Res Ther (2010) 12(3):R111. doi: 10.1186/ar3047
28. Oren S, Mandelboim M, Braun-Moscovici Y, Paran D, Ablin J, Litinsky I, et al. Vaccination against influenza in patients with rheumatoid arthritis: the effect of rituximab on the humoral response. Ann Rheum Dis (2008) 67(7):937–41. doi: 10.1136/ard.2007.077461
29. de Araujo-Neto JM, Guimaraes GS, Fernandes FF, Soares MA. Hepatitis b surface antibody (Anti-HBs) kinetics during rituximab chemotherapy and performance of hepatitis b vaccine before immunosuppression: Two prospective studies. Viruses-Basel. (2022) 14(8):1780. doi: 10.3390/v14081780
30. Sormani MP, Inglese M, Schiavetti I, Carmisciano L, Laroni A, Lapucci C, et al. Effect of SARS-CoV-2 mRNA vaccination in MS patients treated with disease modifying therapies. EBioMedicine. (2021) 72:103581. doi: 10.1016/j.ebiom.2021.103581
31. Mauro D, Ciancio A, Di Vico C, Passariello L, Rozza G, Pasquale MD, et al. Serological response to BNT162b2 anti-SARS-CoV-2 vaccination in patients with inflammatory rheumatic diseases: Results from the RHEUVAX cohort. Front Immunol (2022) 13:901055. doi: 10.3389/fimmu.2022.901055
32. Takai S, Nishida H, Ito H, Fukuhara H, Nawano T, Narisawa T, et al. Immunogenicity and safety of two doses of SARS-CoV-2 mRNA vaccine in kidney transplant recipients with low-dose rituximab. Int J Urology. (2022) 29(11):1279–86. doi: 10.1111/iju.14978
33. Simon D, Tascilar K, Fagni F, Schmidt K, Kroeneke G, Kleyer A, et al. Efficacy and safety of SARS-CoV-2 revaccination in non-responders with immune-mediated inflammatory disease. Ann Rheumatic Diseases. (2022) 81(7):1023–7. doi: 10.1136/annrheumdis-2021-221554
34. Isnardi CA, Cerda OL, Landi M, Cruces L, Schneeberger EE, Montoro CC, et al. Immune response to SARS-CoV-2 third vaccine in patients with rheumatoid arthritis who had no seroconversion after primary 2-dose regimen with inactivated or vector-based vaccines. J Rheumatol (2022) 49(12):1385–9. doi: 10.3899/jrheum.220469
35. Furer V, Eviatar T, Freund T, Peleg H, Paran D, Levartovsky D, et al. Immunogenicity induced by two and three doses of the BNT162b2 mRNA vaccine in patients with autoimmune inflammatory rheumatic diseases and immunocompetent controls: a longitudinal multicentre study. Ann Rheum Dis (2022) 81(11):1594–602. doi: 10.1136/ard-2022-222550
36. Aikawa NE, Kupa LVK, Medeiros-Ribeiro AC, Saad CGS, Yuki EFN, Pasoto SG, et al. Increment of immunogenicity after third dose of a homologous inactivated SARS-CoV-2 vaccine in a large population of patients with autoimmune rheumatic diseases. Ann Rheum Dis (2022) 81(7):1036–43. doi: 10.1136/annrheumdis-2021-222096
37. Liebers N, Speer C, Benning L, Bruch PM, Kraemer I, Meissner J, et al. Humoral and cellular responses after COVID-19 vaccination in anti-CD20-treated lymphoma patients. Blood. (2022) 139(1):142–7. doi: 10.1182/blood.2021013445
38. Troldborg A, Thomsen MK, Bartels LE, Andersen JB, Vils SR, Mistegaard CE, et al. Time since rituximab treatment is essential for developing a humoral response to COVID-19 mRNA vaccines in patients with rheumatic diseases. J Rheumatol (2022) 49(6):644–9. doi: 10.3899/jrheum.211152
39. Woopen C, Dunsche M, Haase R, Raposo C, Pedotti R, Akguen K, et al. Timing of SARS-CoV-2 vaccination matters in people with multiple sclerosis on pulsed anti-CD20 treatment. Neurology-Neuroimmunology Neuroinflamm (2022) 9(6):e200031. doi: 10.1212/NXI.0000000000200031
40. Svensson M, Dahlin U, Kimby E. Better response with conjugate vaccine than with polysaccaride vaccine 12 months after rituximab treatment in lymphoma patients. Br J Haematol (2012) 156(3):407–9. doi: 10.1111/j.1365-2141.2011.08867.x
41. Joly P, Horvath B, Patsatsi A, Uzun S, Bech R, Beissert S, et al. Updated S2K guidelines on the management of pemphigus vulgaris and foliaceus initiated by the european academy of dermatology and venereology (EADV). J Eur Acad Dermatol Venereology. (2020) 34(9):1900–13. doi: 10.1111/jdv.16752
42. Yri OE, Torfoss D, Hungnes O, Tierens A, Waalen K, Nordøy T, et al. Rituximab blocks protective serologic response to influenza a (H1N1) 2009 vaccination in lymphoma patients during or within 6 months after treatment. Blood. (2011) 118(26):6769–71. doi: 10.1182/blood-2011-08-372649
43. Schumacher F, Mrdenovic N, Scheicht D, Pons-Kuehnemann J, Scheibelhut C, Strunk J. Humoral immunogenicity of COVID-19 vaccines in patients with inflammatory rheumatic diseases under treatment with rituximab: A case-control study (COVID-19VacRTX). Rheumatology. (2022) 61(10):3912–8. doi: 10.1093/rheumatology/keac036
44. Konig M, Lorentzen AR, Torgauten HM, Tran TT, Schikora-Rustad S, Vaage EB, et al. Humoral immunity to SARS-CoV-2 mRNA vaccination in multiple sclerosis: The relevance of time since last rituximab infusion and first experience from sporadic revaccinations. J Neurol Neurosurg Psychiatry (2021) 94(1):19–22. doi: 10.1136/jnnp-2021-327612
45. van der Togt CJT, Ten Cate DF, den Broeder N, Rahamat-Langendoen J, van den Bemt BJF, den Broeder AA. Humoral response to coronavirus disease-19 vaccines is dependent on dosage and timing of rituximab in patients with rheumatoid arthritis. Rheumatology (2022) 61(SI2):SI175–SI9. doi: 10.1093/rheumatology/keac206
46. Benucci M, Damiani A, Infantino M, Manfredi M, Grossi V, Lari B, et al. Correspondence on "SARS-CoV-2 vaccination in rituximab-treated patients: Evidence for impaired humoral but inducible cellular immune response"by bonelli et al. Ann Rheumatic Dis (2021) 80(10):e166. doi: 10.1136/annrheumdis-2021-220829
47. Connolly CM, Koenig D, Ravi SN, Azar A, Kant S, Dalal M, et al. Correspondence on "SARS-CoV-2 vaccination in rituximab-treated patients: Evidence for impaired humoral but inducible cellular immune response" by bonelli et al. Ann Rheumatic Dis (2021) 80(10):e164. doi: 10.1136/annrheumdis-2021-220972
48. Jinich S, Schultz K, Jannat-Khah D, Spiera R. B cell reconstitution is strongly associated with COVID-19 vaccine responsiveness in rheumatic disease patients who received treatment with rituximab. Arthritis Rheumatol (2022) 74(5):776–82. doi: 10.1002/art.42034
49. Mrak D, Tobudic S, Koblischke M, Graninger M, Radner H, Sieghart D, et al. SARS-CoV-2 vaccination in rituximab-treated patients: B cells promote humoral immune responses in the presence of T-cell-mediated immunity. Ann Rheum Dis (2021) 80(10):1345–50. doi: 10.1136/annrheumdis-2021-220781
50. Stefanski A-L, Rincon-Arevalo H, Schrezenmeier E, Karberg K, Szelinski F, Ritter J, et al. B cell numbers predict humoral and cellular response upon SARS-CoV-2 vaccination among patients treated with rituximab. Arthritis Rheumatol (2022) 74(6):934–47. doi: 10.1002/art.42060
Keywords: rituximab, anti-CD20 antibody, vaccines, COVID-19 vaccines, immunogenicity, immune-mediated dermatologic diseases, autoimmune diseases
Citation: Seree-aphinan C, Ratanapokasatit Y, Suchonwanit P, Rattanakaemakorn P, O-Charoen P, Pisitkun P, Suangtamai T, Setthaudom C, Chirasuthat S and Chanprapaph K (2023) Optimal time for COVID-19 vaccination in rituximab-treated dermatologic patients. Front. Immunol. 14:1138765. doi: 10.3389/fimmu.2023.1138765
Received: 06 January 2023; Accepted: 23 February 2023;
Published: 15 March 2023.
Edited by:
Mattia Bellan, University of Eastern Piedmont, ItalyReviewed by:
Liliana Trotta, Ospedale di Cattinara, ItalyRiccardo Pozzan, University of Trieste, Italy
Clodoveo Ferri, University of Modena and Reggio Emilia, Italy
Copyright © 2023 Seree-aphinan, Ratanapokasatit, Suchonwanit, Rattanakaemakorn, O-Charoen, Pisitkun, Suangtamai, Setthaudom, Chirasuthat and Chanprapaph. This is an open-access article distributed under the terms of the Creative Commons Attribution License (CC BY). The use, distribution or reproduction in other forums is permitted, provided the original author(s) and the copyright owner(s) are credited and that the original publication in this journal is cited, in accordance with accepted academic practice. No use, distribution or reproduction is permitted which does not comply with these terms.
*Correspondence: Kumutnart Chanprapaph, a3VtdXRuYXJ0cEBob3RtYWlsLmNvbQ==
†These authors share first authorship