- 1Department of Pathology, University of Texas Southwestern Medical Center, Dallas, TX, United States
- 2Simmons Comprehensive Cancer Center, Dallas, TX, United States
The adenosine pathway is an exciting new target in the field of cancer immunotherapy. CD73 is the main producer of extracellular adenosine. Non-small cell lung cancer (NSCLC) has one of the highest CD73 expression signatures among all cancer types and the presence of common oncogenic drivers of NSCLC, such as mutant epidermal growth factor receptor (EGFR) and KRAS, correlate with increased CD73 expression. Current immune checkpoint blockade (ICB) therapies only benefit a subset of patients, and it has proved challenging to understand which patients might respond even with the current understanding of predictive biomarkers. The adenosine pathway is well known to disrupt cytotoxic function of T cells, which is currently the main target of most clinical agents. Data thus far suggests that combining ICB therapies already in the clinic with adenosine pathway inhibitors provides promise for the treatment of lung cancer. However, antigen loss or lack of good antigens limits efficacy of ICB; simultaneous activation of other cytotoxic immune cells such as natural killer (NK) cells can be explored in these tumors. Clinical trials harnessing both T and NK cell activating treatments are still in their early stages with results expected in the coming years. In this review we provide an overview of new literature on the adenosine pathway and specifically CD73. CD73 is thought of mainly for its role as an immune modulator, however recent studies have demonstrated the tumor cell intrinsic properties of CD73 are potentially as important as its role in immune suppression. We also highlight the current understanding of this pathway in lung cancer, outline ongoing studies examining therapies in combination with adenosine pathway targeting, and discuss future prospects.
1 Introduction
The field of cancer immunotherapy has rapidly evolved over the last decade and numerous agents have received FDA approval (1). The search for new immune modulating agents is a major current focus of the broader cancer research community. Recently antibodies and small molecules targeting the adenosine pathway have gained traction as therapeutic agents for a multitude of cancer types. Numerous pre-clinical studies and clinical trials have demonstrated that the adenosine pathway is a promising therapeutic target (2–6). This is especially true in the field of lung cancer (2, 5, 7, 8). There are two key pieces of the adenosine pathway that are actively being explored as therapeutic targets including the production of adenosine itself and the receptors to which this metabolite binds (2, 3, 6). When ATP is released from the cell, a series of enzymatic events occurs on the cell surface through CD39 which converts ATP to AMP and CD73 which converts AMP to adenosine (9). There is also a non-canonical pathway leading to AMP production, but both pathways eventually converge to CD73 activity (10). CD73 is encoded by the gene NT5E and plays a role in numerous tumor cell intrinsic and extrinsic functions (11). Until recently, CD73 was studied and viewed mainly for its role as an ectonucleotidase involved in immune suppression, but recent studies have elucidated far more functions related to this molecule (12–14).
Adenosine is a nucleoside and is necessary for cellular functions, providing the building blocks for RNA (15). Under normal physiological conditions, extracellular adenosine levels within tissues are in the low nM concentration, however under conditions of cellular stress and cancer formation concentrations of adenosine can reach up to the 100uM range (16, 17). This has been observed within the tumor microenvironment; making targeting adenosine production a promising therapeutic strategy (15). Importantly, adenosine signaling plays a key homeostatic role throughout the body including maintaining cardiac function (18), neuronal signaling (19), and renal function (20). Regulation of adenosine levels both intracellularly and extracellularly are tightly controlled through both canonical and non canonical pathways (21). However, when there is increased expression of andenosine pathway producing enzymes, which is seen across cancer types, this equilibrium is disturbed resulting in an immune suppressive environment.
The field of lung cancer treatment has made immense strides with the addition of immune checkpoint blockade therapies to the standard of care (22–24). However, therapy resistance remains a major problem and discovering additional treatments is vital. NSCLC and a major subtype of NSCLC, lung adenocarcinoma (LUAD), has been researched most extensively in terms of the adenosine pathway (25–27). This has led to clinical trials testing adenosine pathway targeting in a subset of LUAD, EGFR mutant tumors (5, 8, 28). Additionally in NSCLC, Durvalumab, a PD-L1 antibody, is currently being tested in combination with Oleclumab, a CD73 blocking antibody, or Monalizumab, an antibody blocking inhibitory NK cell receptor NKG2A, with promising phase II results leading to the initiation of a phase III trial (2). However, in other lung cancer subtypes such as lung squamous carcinomas (LUSC), large cell neuroendocrine cancers (LCNEC), un-transdifferentiated thoracic tumors (UT), carcinoid like tumors of the lung or small cell lung cancer (SCLC) this pathway has not been thoroughly studied.
This review will outline the current status of targeting the adenosine pathway and its specific outlook in the field of lung cancer. We highlight both cell intrinsic and extrinsic properties of CD73 and the link to immune cell regulation (Figure 1). We provide the current understanding of this pathway in both pre-clinical models and clinical trials across lung cancer subtypes. Finally, we will outline prospects of targeting the adenosine pathway in combination with other treatment options that are already being tested in pre-clinical and clinical models.
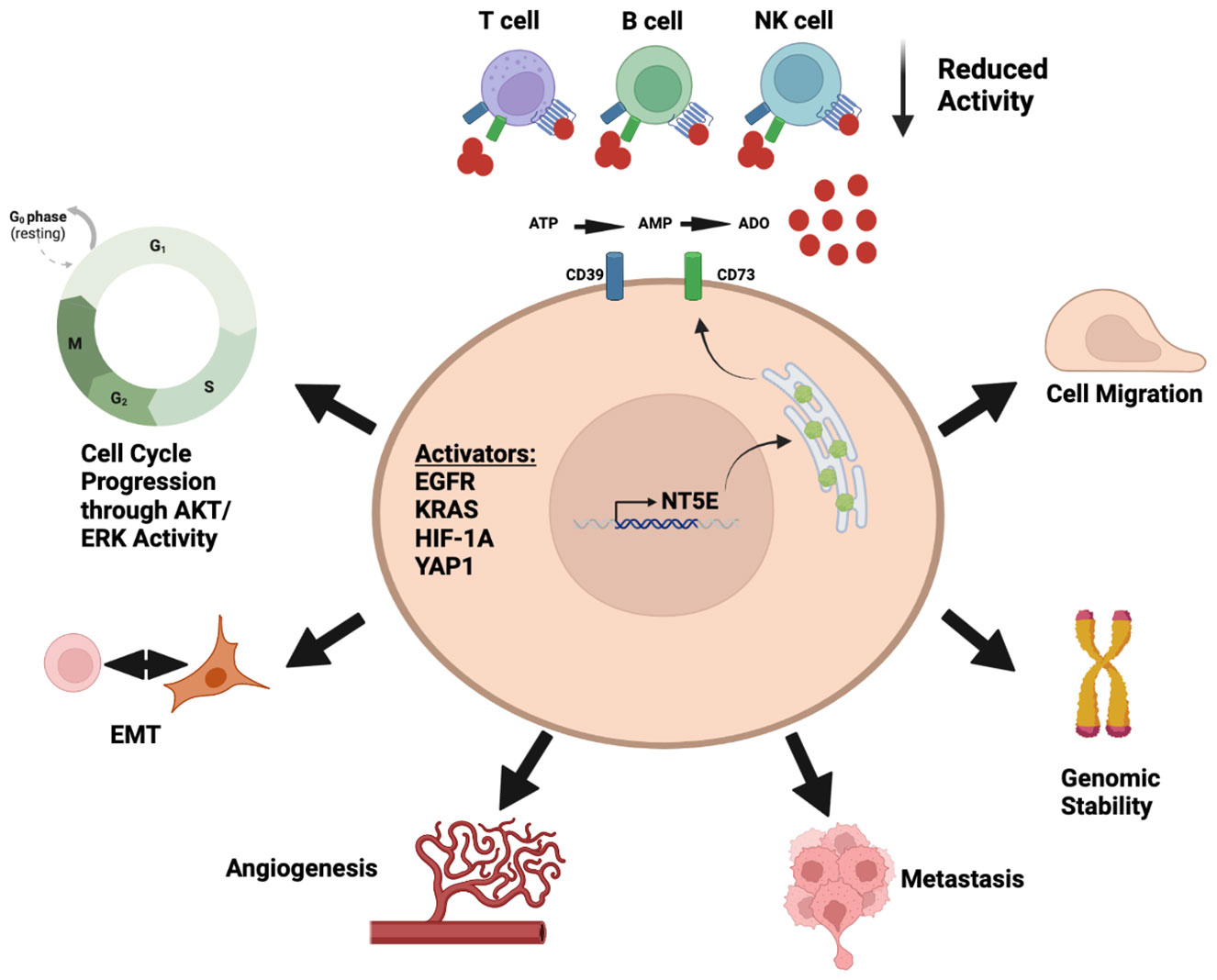
Figure 1 Diverse roles of adenosine pathway in the tumor microenvironment. Illustration was created using BioRender.com.
2 Adenosine production pathway
There is both a canonical and non canonical pathway resulting in adenosine production. Within the canonical pathway, ATP is first released into the tumor microenvironment under various biological conditions such as hypoxia or cellular stress (29, 30). CD39, another ecto-enzyme located on the cell surface, first de-phosphorylates ATP to AMP. CD73 then dephosphorylates AMP resulting in adenosine production (31). Within the non-canoical pathway CD38 and CD203a function to convert NAD+ into AMP which then is converted into adenosine by CD73 (32). An additional pathway through the function of alkaline phosphatase (ALP) can convert ATP, ADP, or AMP into adenosine (33). Furthermore, prostatic acid peptidase (PAP) can convert AMP to adenosine (34). Within the cell, intracellular adenosine levels are controlled by the adenosine kinase (AK) and cyto5’NT or by S adenosyl-homocysteine-hydrolase (SAHH) (35). Intracellular adenosine is then shuttled in and out of the cell by equilibrative nucleoside transporters (36). It is important to note that the main non canonical pathway through CD38 and CD203a cannot bypass CD73 activity (32). Therefore CD73 is integral to the pathway and is responsible for most adenosine accumulation.
2.1 CD39 and CD73 expression in immune cell populations
It is important to note that adenosine pathway related molecules, such as CD39 and CD73, are also expressed by tumor infiltrating lymphocyte (TIL) populations (37, 38). In human colon and lung tumor samples, analysis of TIL populations found that only CD8 T cells that express CD39 represent the stimulated form of T cells whereas those lacking CD39 play a bystander role (39). An in vitro study found similar results that T cells rapidly upregulate CD39 expression upon activation but that CD73 expression remains unchanged (37). In contrast to these findings, another group found that CD39 positive CD8 T cells represent the exhausted phenotype with decreased expression of both TNF and IL-2 through analysis of both human and mouse samples from breast and melanoma tumors (40). It is important to note however that heterogeneity within samples was high with some TIL samples expressing high levels of CD39 and some quite low (39, 40). An additional study utilizing a melanoma mouse model found that both CD73 and CD39 are upregulated on T cells during immune response, but that high CD73 limits effector function through a decrease in mitochondrial capacity (41). This suggests a potential mechanism that T cells control levels of extracellular ATP and AMP to self regulate their activity.
In addition to T cells, CD39 and CD73 function has also been explored on NK cells. A recent report studying both breast and sarcoma tumors found that CD73 positive NK cells within the tumor express higher levels of immune checkpoint molecules such as LAG-3 compared to CD73 negative NK cells found in the peripheral blood. These NK cells have suppressive functions on CD4 T cells (42). An additional study utilizing a mouse model of melanoma found that mice with tumors show modest increase in expression of both CD39 and CD73 on NK cells as compared to tumor naïve mice (38). Furthermore, another study found that CD39 expression on NK cells was not essential for cytotoxic function (43). It is important to note that immune cell populations such as B cells (44), T regulatory cells (45), and MDSC’s (46) have also been shown to express high levels of CD39 and CD73 resulting in the production of adenosine. Interplay between the diverse cells within the tumor microenvironment resulting in adenosine production displays the complexity of this pathway.
3 The adenosine pathway in lung cancer
Lung cancer is the second most diagnosed form of cancer in the United States and nearly 240,000 cases were reported in 2022 (47). Around 85% of lung cancer cases in the United States represent NSCLC histology and the remaining cases are classified as SCLC (48). ICB has become standard of care in recent years due to clinical activity (reviewed in (49) and used as single agent or in combination with chemotherapy. ICB was also explored in the neo-adjuvant setting in early stage NSCLC and due to clinical benefit received FDA approval in combination with chemotherapy in 2022 (50). In contrast, combination of PD-L1 blockade and chemotherapy, while receiving FDA approval, has shown limited clinical benefit in SCLC (51). However, for a subtype of SCLC, known as non-neuroendocrine characterized by a lack of expression of neuroendocrine genes, there has been some benefit with the addition of ICB therapy (52). Treatment for LCNEC and other lung cancers are typically chemotherapy as well (53, 54). The role of ICB in this lung cancer subtype is not yet well studied but there has been some response seen in small clinical trials (55). There is a need to look more extensively at immunosuppressive pathways, such as the adenosine to overcome therapy resistance and tailor treatment options to the individual patient (56–58).
Among all lung cancer subtypes, CD73 has been mostly studied in LUAD. Compared to normal lung, LUAD has significantly increased CD73 expression demonstrating that high CD73 is a tumor specific characteristic (27, 59). CD73 has been also detected in LUSC in some cases but is not universal (59, 60). One study of CD73 in LUSC found that similar to LUAD that knockdown of CD73 results in decreased cell proliferation, migration, and increases response to TKIs (61). In SCLC, adenosine pathway expression was reported in some patient samples and circulating tumor cell explants (CDX) models (62, 63).
Multiple studies have demonstrated across cancer types that expression of genes coding for molecules in adenosine pathway is correlated with poor survival and low overall response rate to cancer therapies (64–66). Recently, adenosine pathway expression in LUAD and LUSC has been investigated and similar results were seen with high CD73 expression on tumor cells correlating with disease progression, treatment relapse, and poor survival (60). Interestingly, in this same study they found that high adenosine receptor expression was associated with increased survival and that correspondingly high CD73 and low adenosine receptor expression resulted in the worst survival outcomes (60).
3.1 Adenosine pathway expression in EGFR mutant LUAD
Recently, multiple groups have focused on targeting the adenosine pathway in EGFR mutant NSCLC leading to swift discoveries in the recent years (26, 67). Standard of care for EGFR mutant tumors are tyrosine kinase inhibitor (TKI)s (68). EGFR mutant tumors are not very responsive to ICB therapy possibly due to their low tumor mutational burden (TMB) as compared to tumors carrying another common oncogenic driver of NSCLC, KRAS, which are more responsive to ICB (69, 70). Somatic mutations can serve as neoantigens and high TMB status is associated with increased sensiviity to ICB therapies on NSCLC (71). Response rates to ICB therapies are between 7-16% depending on EGFR mutation type, compared to 22% in EGFR wild type tumors (72). Acquired resistance to TKIs, such as Osimertinib is common and occurs on average around 18 months after treatment initiation (73). Multiple studies reported that CD73 upregulation was one of the mechanisms of resistance to TKIs (74, 75). We recently analyzed genes related to immune cell function in EGFR mutant versus wild type LUAD and found that NT5E and ADORA1, which encodes for one of the adenosine receptors, were two of the top upregulated immune-suppresive genes in this subtype (26). EGFR mutant NSCLC has a suppressed tumor microenvironment with few NK cells and CD8 T cells, but increased dendritic cell populations, and other immune cell populations remain similar to those seen in EGFR wild type tumors (26). In an EGFR mutant mouse model, we showed that treatment with a CD73 antibody alone resulted in decreased lung tumor growth displaying proof of concept as a treatment option (26). Another group observed similar correlations with EGFR pathway activation and adenosine signaling. Interestingly this group showed Oleclumab is effective in treating EGFR mutant tumors in combination with PD-L1 antibody in a human peripheral blood mononuclear cell (PBMC) transplanted mouse model (76).
Another recent report focused on the connection between MET amplification, CD73 and suppression of STING pathway in EGFR mutant tumors (67). Authors found that MET amplification induces CD73 expression and restrains the function of STING resulting in reduced T cell activation while also causing resistance to 3rd generation EGFR TKIs. MET amplification was found to be specifically associated with CD73 expression in EGFR mutant LUAD. Genetic knockout of MET in lung cancer models resulted in decreased CD73 expression therefore displaying a connection between these two pathways (67). This study links CD73 intrinsic function to extrinsic function through immune cell regulation.
3.2 Adenosine pathway expression in other common oncogenic drivers of LUAD
Although adenosine pathway expression has been studied the most in the EFGR mutant subset of LUAD, other common mutations such as KRAS, BRAF, and MET are just beginning to be explored. A recent study utilizing the TCGA data set found that CD73 expression is significantly elevated in KRAS mutant LUAD (25, 77). Similar induction of CD73 expression was also seen with ALK gene alterations. As proof of concept, they found that pharmacological inhibition of ALK led to a decrease in CD73 mRNA expression (25). This data suggests a link between the adenosine pathway and oncogenic drivers of LUAD. A new study in pancreatic ductal adenocarcinoma (PDAC), where almost 80% of cases are KRAS mutant, demonstrated that activating mutation in KRAS alone is sufficient to elevate levels of CD73 suggesting that CD73 induction with oncogenes extends beyond lung cancer (78). Results from these studies thus far demonstrate oncogenic drivers of NSCLC possibly drive increased CD73 expression and that underling mechanism behind this warrants further investigation.
3.3 Adenosine pathway expression in SCLC
CD73 expression is seen in a subset of SCLC patient CDX models and cell lines and its expression correlated with the non-neuroendocrine or YAP1 positive subtype (62, 63). A recent study focused on the metabolomics of primary SCLC tumors. This study demonstrated that AMP and adenosine were the two significantly upregulated metabolites in primary patient samples in the MYC high subtype versus MYC low subtype (79). This study also examined the link between chemoresistance and purine biosynthesis and found that chemo-resistant SCLC cell lines have increased levels of AMP and ATP compared to parental cell lines. These pre-cursors could result in adenosine accumulation within the tumor microenvironment (79). A similar finding was seen in vivo with chemotherapy relapsed tumors displaying increased purine biosynthesis, including AMP (79). Therefore these interesting findings suggest that purine biosynthesis maybe advantageous for SCLC relapse after treatment.
3.4 Adenosine pathway inhibition in the context of lung cancer tumor transdifferentiation and heterogeneity
Cellular plasticity and evolution of the tumor microenvironment with disease progression has been reported in NSCLCs. Transdifferentiation of EGFR mutant NSCLC to SCLC is one of the reported resistance mechanisms to EGFR TKIs. This occurs in approximately 3-10 percent of EGFR mutated NSCLCs (80). Studies have found that SCLC transformed tumors in these patients no longer respond to TKIs but they do respond to platinum etoposide therapy at least initially, similar to SCLCs (80–82). A recent report found that loss of extracellular-signal regulated kinase (ERK) signaling dependency was the main regulator in this transformation and this resulted in expression of neuroendocrine transcription factors which was normally repressed by ERK signaling (83). SCLC transdifferentiation was also reported in therapy resistant KRAS mutant LUAd (84). It would be interesting to study whether these transformed tumors retain expression of CD73 and can be targeted by CD73 targeting molecules.
In the field of SCLC multiple groups have found that SCLC primarily switches from neuroendocrine to a more non-neuroendocrine like state. This was shown to be driven through NOTCH driven Myc expression (85, 86), while other groups show that this is driven by EMT gene signature including expression of YAP1 (87). Neuroendocrine and non-neuroendocrine tumor types have shown to have different immune microenvironments with neuroendocrine tumors having far less immune cell infilrates (48, 88, 89). A new report examined 146 SCLC patient samples through IHC and found that 2.3% of tumors expressing YAP1 dominantly. Additionally, the authors found that 17.6 percent of tumors expressed 2 of the SCLC lineage markers and 2.8% were positive for 3 different lineage markers. These areas of different lineage marker staining tended to cluster away from one another suggesting that different cell populations were in various parts of the tumor (90). A recent study highlights that the SCLC intertumoral composition is constantly evolving and that there maybe continuous subtype switching (91). Multiple studies have suggested that one of the target genes of the YAP/TAZ axis is CD73, suggesting the idea that induction of CD73 expression may provide a selective advantage to YAP1 expressing SCLC cells under treatment (92–94). This connection must be explored further, but it is possible that the YAP1 positive cells are contributing to adenosine production, with high expression of CD73 seen in YAP1 positive CDX and SCLC cell line models (63, 95).
4 Tumor cell intrinsic functions of CD73
CD73 has been demonstrated to be involved in numerous cancer processes including metastasis (12), increased cell proliferation (96), and tumor invasion (97). In addition to its membrane bound form, CD73 is expressed in soluble forms (98), on extracellular vesicles (99), and localized within different cellular compartments of tumor cells (100). Studies on the intrinsic properties of CD73 have been investigated in numerous cancer types including liver cancer (101), GI cancer (102), Glioblastoma (103), and lung cancer (104) (Table 1). CD73 has been implicated as a target of transcription factors including YAP1 (92), SNAIL (105), HIF1A (106), and TGFB (14, 107, 108). Transcriptional regulation by key drivers of cancer suggests that an increase in CD73 expression is advantageous for cancer cell growth. Therefore, understanding the mechanism of CD73 intracellular function is key to understanding the overall biology of this molecule.
4.1 Role of the CD73 in the EMT program
Recent studies have shown that CD73 contributes to EMT (epithelial mesenchymal transition) and is a novel target beyond the current key genes that are implicated in EMT such as E-cadherin, vimentin, and N-cadherin (109). A recent study utilizing a breast cancer model with genetic loss or pharmacological inhibition of CD73 resulted in far fewer and less invasive organoids in vitro and decreased lung metastasis when injected into an immune competent mouse model. It was reported that when CD73 is knocked down that there is increased E-cadherin expression and that cells appeared to revert from EMT (12). A similar finding was also shown in a preclinical model of ovarian cancer (110). Another recent study in breast cancer found that SNAIL, a key regulator of EMT, bound at promotors of NT5E through chromatin immunoprecipitation (ChIP) sequencing analysis suggesting that there could be interplay between these two genes and the EMT program (111). An additional recent report in NSCLC line A549 showed that CD73 overexpression leads to increased invasion and metastasis both in vitro and in vivo (25). Further studies employing the A549 model demonstrated that CD73 promotes cell proliferation through binding to EGFR which leads to activation of AKT/mTOR pathway (104). Across numerous cancer types genetic knockdown or knockout of CD73 in human cancer cell lines grown in immune deficient mice demonstrated that loss of this molecule reduces tumor growth and metastatic potential even when a functional immune system is not present (12, 110, 112, 113).
4.2 The role of CD73 in cell cycle regulation and treatment resistance
Outside of the scope of the EMT program, studies have demonstrated that CD73 plays a role in other biological processes as well. A few different studies have linked CD73 to control of cell cycle progression (112, 114, 115). A specific study in pancreatic ductal adenocarcinoma (PDAC) found that when CD73 is knocked down, this results in arrest of cells at G1 phase through AKT/ERK/Cyclin D signaling (114). Other studies have focused on how CD73 expression leads to treatment resistance. A recent in vitro study in LUAD demonstrated that knock down of CD73 altered cell cycle progression and sensitized cells to cisplatin chemotherapy treatment (115). Similar results were seen in a model of glioblastoma when they knock down CD73 in their model this resulted in increased temozolomide sensitivity, and that resistance is caused by CD73-A2BAR signaling (116). An additional in vitro study in breast cancer model found that CD73 deficient MDA-MB-231 cells to be significantly more sensitive to Olaparib likely due to decreased PARP activity. They also found that loss of CD73 suppressed mitochondrial respiration and led to increased genomic instability (117).
Studies thus far have demonstrated an interesting link between expression of CD73 and sensitivity to commonly used therapeutic agents. Of note, in the tumors resistant to numerous therapies CD73 expression is increased. These include radiation (118), chemotherapy (119), TKIs (75), as well as monoclonal antibodies (120). This has been shown to occur through different mechanisms across cancer types. A study in melanoma found that there was increased CD73 expression in the tumors resistant to both adoptive T cell transfer and ICB therapy. They found that both in mouse models and human tissues that MAPK signaling resulted in class switching from an epethial to mesenchymal state and that there was clonal selection for a more “invasive” cell population (121). In hepatocellular carcinoma (HCC) CD73 was shown to contribute to resistance to Lenvatinib, a VEGF inhibitor, through AKT overactivity which resulted in increased SOX9 expression and stemness of HCC cells (13). Within the tumor micronevironment, a pre-clinical study in triple negative breast cancer (TNBC) found that after chemotherapy that there was an increase in CD73, CD47, and PD-L1 positive tumor cells (122). Such examples of therapy resistance show that CD73 is tied to multiple mechanisms of therapy resistance.
4.3 Non- enzymatic functions of CD73
CD73 expression correlated with adenosine production in most studies, however it is technically difficult to separate the ectonucleotidase activity from other functions of CD73 in genetic inactivation or antibody blockade studies. One group recently thoroughly studied the non-enyzmatic role of CD73 by blocking the nucleotidase function. They reported a direct physical interaction between CD73 and Src in the ER resulting in Src activation (100). They found that CD73 ectonucleotidase activity did not contribute to Gemcitabine resistance but that resistance was rather caused by AKT pathway activation by CD73. Furthermore they demonstrated that when they mutated the zinc finger binding domain of CD73, which severely inhibits ectonucleotidase activity, that there was still resistance to Gemcitabine suggesting again that it was not the enzymatic activity leading to resistance (100). An additional study in HCC showed that CD73 localizes within the ER of cancer cells and is more abundant in tumors than normal tissue. They propose that there is both a short and and full length of CD73 proteins with the long form only having ectonuclotidase activity and the shorter version found solely localized in the ER (123). To our knowledge these are the only two studies to yet examine proximity of CD73 in the cell and therefore further understanding of the differences between mebrane bound and intracellular CD73 are needed.
5 Tumor cell extrinsic functions of adenosine in the tumor microenvironment
Once adenosine is released into the tumor microenvironment it plays both an immunosuppressive role for some immune subtypes and activates others, although there is less evidence for the latter. There are a total of four adenosine receptors found on the cell surface including A1, A2A, A2B, and A3 (21). These receptors are expressed on cell types throughout the body including both innate and adaptive immune cells. The A2AR receptor is expressed on T, NK, and B cells and when bound to adenosine this results in loss of activity in cell types (125–127). The A2BR receptor is expressed on macrophages, monocyte derived suppressor cells (MDScs) and cancer associated fibroblasts (CAFs) and upon binding of adenosine results in their activation leading to immune suppresion (128). Another important immune cell type that expresses the A2BR are dendritic cells (DC), which are key to antigen presentation (129). The literature demonstrates mixed findings thus far into this cell type with studies showing that adenosine impairs DC migration but does not affect their function (130). In contrast, another study found adenosine impairs DC function and that these impaired DCs release angiogenic cytokines promoting tumor growth (131). Numerous studies have demonstrated that increased adenosine production results in decreased activity of cytotoxic T cell populations and increased activity of regulatory T cell (Treg) populations (125, 132, 133). This combined signaling across immune cell types leads to an immunosuppressive tumor microenvironment.
5.1 Role of adenosine pathway on NK cell function
NK cells are a key component of the innate immune system and display cytotoxic reponse. Recent advances in the field of lung cancer have shown that NK cells play an essential role in controlling tumor growth and response to ICB therapy (134–137). NK cells can target cancer cells independent of antigen presentation on major histocompatibility complex (MHC) making them a valuable therapeutic target as MHC-I expression is lost or reduced in tumors as one of the mechanism of immune evasion (138). Loss of MHC-I is seen across lung cancer subtypes as well (139). However, loss of MHC-I can sensitize tumors to NK cell killing as MHC-I is an immune inhibitory molecule for NK cells (140). Recent advances in the field of lung cancer have shown that NK cells play an essential role in controlling tumor growth and response to ICB therapy (134–137). Recent studies have demonstrated that around 10-20 percent of circulating lymphocytes in the lungs are NK cells, which is higher than NK cell levels found in peripheral blood (141). However, there are numerous reasons why these cells are not able to infiltrate tumors, and reasons include different immune suppressive metabolites and inhibitory molecules. Additionally, tumors have been shown to shed the NK cell activating ligands to escape NK cell tumor killing and antibodies are currently being tested that can prevent this (142).
It was reported that adenosine impedes NK cell function (143–145). A2AR inhibition in a co-culture system resulted in increased NK cell proliferation and signaling response as compared to untreated cultures (146). As a mechanism of tumor resistance researchers found that upon binding of the 4-1BBL domain that tumor cells can hijack NK cells and induce CD73 expression in the NK cells in a breast cancer model. These adenosine producing NK cells additionally had higher levels of immune inhibitory checkpoints such as LAG-3 (42). A similar finding was also found in in vitro co culture methods when cancer cell lines with high CD73 were found to induce CD73 expression in an established NK cell line, NK-92 cells. Cancer cell lines with low CD73 expression did not induce CD73 expression in NK cells (147). A recent study used a chimeric antigen receptor (CAR)-NK cells to target CD73 in a preclinical lung cancer model. Authors observed that both in vitro and in vivo that their CD73 directed CAR inhibited lung cancer growth while not attacking normal tissue (126). These findings demonstrate that adenosine as a cancer target must be explored further.
6 Clinical targeting of adenosine pathway in lung cancer
The first clinical stage antibody developed to target the adenosine pathway was created by Medimmune, compound MEDI9447 (Oleclumab), and began clinical trial testing in 2015 (148). Since then, many studies were initiated to target various components of this pathway including CD73 and CD39 followed by A2AR and combined A2AR/A2BR antibodies (149).
Trials for CD73 targeting agents have progressed the most reaching phase III testing (150). Trials are ongoing for NSCLC (2), PDAC (151), and other advanced solid tumors. A recent phase Ib/2 trial testing Oleclumab in combination with Osimertinib in EGFR mutant NSCLC demonstrated safety and efficacy and is now proceeding to phase II trials (3). The most exciting trial thus far testing Durvalumab alone or in combination with Oleclumab or Monalizumab in stage III unresectable NSCLC recently progressed to phase III testing. At median follow up of 11.5 months the ORR in Durvalumab plus Oleclumab was 30%, and Durvalumab combined with Monalizumab ORR was 35.5%. PFS was also higher in the combination groups as compared to Durvalumab alone (2).
CD39 has also been explored as a therapeutic target. In pre-clinical models, molecules targeting CD39 have shown promising results in colon cancer (152), melanoma (153), and ovarian cancer (154). CD39 antibodies are currently being tested in combination with ICB therapy (NCT04336098) and chemotherapy (NCT03884556) and are currently in phase I stage (155).
Targeting the adenosine receptor has shown modest effect and the trial in combination with Oleclumab in EGFR mutant NSCLC was stopped due to safety issues and lack of efficacy (150). However, in advanced prostate cancer there was some response with single agent AZD4635 (A2AR antagonist) with an overall response rate of 5.1% and when combined with Durvalumab combination, response rate was 16.1% (5).
7 Discussion
The study of the adenosine pathway as a therapeutic target is still in its early stages, however preclinical studies and clinical trial data have demonstrated that targeting this pathway is a viable therapeutic strategy moving forward. Increasing evidence has demonstrated that CD73 has roles independent of its enzymatic function. However only one group thus far has thoroughly studied this mechanism. Even with their findings however it is still thought provoking whether the intracellular function of CD73 is the same as the canonical econucleotidase activity on the cell membrane. This raises an important question as current antibodies targeting CD73 would therefore not prevent activity within the intracellular space. We propose that based on the literature generated thus far that CD73 intracellular activity, whether enzymatic or not, could possibly play an important role in cancer progression as the immune suppression aspect through adenosine production. We note that although we have focused this review around CD73 and adenosine, we highlight that both CD39 as well as the adenosine receptors are also important pieces of this pathway. Importantly studies are needed to understand whether CD39 or CD73 blockade leads to increased compensatory activity of non-canonical pathways adenosine pathways such as CD38 and CD203a.
Targeting the adenosine pathway with recently initiated clinical trials has grown as new combinatory approaches are tested in lung cancer. We believe that CD73 inhbition as a therapeutic target can be applied to other lung cancer types outside of the current scope of EGFR mutant LUAD and unresectable NSCLC. This is especially important surrounding the topic of lineage plasticity and acquired treatment resistance. Therefore we need to better understand as a field what is driving this plasticy and how this is leads to a lack of response to therapeutic agents. We believe an important aspect of further study is understanding how EGFR NSCLCs transdifferentiate to SCLC and whether blockade of the adenosine pathway could be a potential treatment for these tumors.
We also believe there is an unmet need to better understand the role of NK cells and activating this cytotoxic cell type as they have been shown to play critical roles in both NSCLC and SCLC. In regards to the clinical trial data with the ICB and Oleclumab or ICB and Monalizumab COAST study, we suggest a potential further clinical trial could test Monalizumab plus Oleclumab. We believe that based on the current pre-clinical and clinical data thus far that it does not appear that targeting the adenosine pathway alone will result in drastic therapeutic benefit and therefore the best combinatory therapeutics and dosing schedules warrant further investigation. To our knowledge adenosine pathway expression in LCNEC and other thoracic tumors of the lung have not been explored extensively. Several groups have reported that LCNEC and undifferentiated tumors of the lung are becoming more common, and they have not been studied well, but interestingly these tumor types likely arise from therapy resistance (156). Concluding, we see many avenues of further study that are needed both in the pre-clinical and clinical space in order to further understand the role of CD73 and the adenosine pathway overall across not just lung cancer but, also other cancers with an activated adenosine pathway.
Author contributions
Concept and design: RRK and EAA. All authors contributed to the article and approved the submitted version.
Funding
EA is a Cancer Prevention and Research Institute of Texas (CPRIT) Scholar in Cancer Research. EA was supported by CPRIT Scholar Award RR160080, NIH 5P50CA070907, Welch Foundation grant (I-1975-20190330), A Breath of Hope Lung Foundation Fellowship Award (ABOHLF 2020), and NCCN Foundation Young Investigator Award (NCCN 2021). RK was supported by 5T32CA124334.
Conflict of interest
The authors declare that the research was conducted in the absence of any commercial or financial relationships that could be construed as a potential conflict of interest.
Publisher’s note
All claims expressed in this article are solely those of the authors and do not necessarily represent those of their affiliated organizations, or those of the publisher, the editors and the reviewers. Any product that may be evaluated in this article, or claim that may be made by its manufacturer, is not guaranteed or endorsed by the publisher.
References
1. Vaddepally RK, Kharel P, Pandey R, Garje R, Chandra AB. Review of indications of FDA-approved immune checkpoint inhibitors per NCCN guidelines with the level of evidence. Cancers (Basel) (2020) 12(3):738. doi: 10.3390/cancers12030738
2. Herbst RS, Majem M, Barlesi F, Carcereny E, Chu Q, Monnet I, et al. COAST: An open-label, phase II, multidrug platform study of durvalumab alone or in combination with oleclumab or monalizumab in patients with unresectable, stage III non-Small-Cell lung cancer. J Clin Oncol (2022) 40(29):3383–93. doi: 10.1200/JCO.22.00227
3. Lim EA, Bendell JC, Falchook GS, Bauer TM, Drake CG, Choe JH, et al. Phase Ia/b, open-label, multicenter study of AZD4635 (an adenosine A2A receptor antagonist) as monotherapy or combined with durvalumab, in patients with solid tumors. Clin Cancer Res (2022) 38(22):4871–84. doi: 10.1158/1078-0432.CCR-22-0612
4. Chiappori AA, Creelan B, Tanvetyanon T, Gray JE, Haura EB, Thapa R, et al. Phase I study of taminadenant (PBF509/NIR178), an adenosine 2A receptor antagonist, with or without spartalizumab (PDR001), in patients with advanced non-small cell lung cancer. Clin Cancer Res (2022) 28(11):2313–20. doi: 10.1158/1078-0432.CCR-21-2742
5. Kim DW, Kim SW, Camidge DR, Shu CA, Marrone KA, Le X, et al. CD73 inhibitor oleclumab plus osimertinib in previously treated patients with advanced T790M-Negative EGFRm non-small-cell lung cancer: A brief report. J Thorac Oncol (2023) S1556-0864(22)01997-9. doi: 10.1016/j.jtho.2022.12.021
6. Spatola BN, Lerner AG, Wong C, Dela Cruz T, Welch M, Fung W, et al. Fully human anti-CD39 antibody potently inhibits ATPase activity in cancer cells via uncompetitive allosteric mechanism. MAbs (2020) 12(1):1838036. doi: 10.1080/19420862.2020.1838036
7. Johnson ML, Fox W, Lee Y-G, Lee KH, Ahn HK, Kim Y-C, et al. ARC-7: Randomized phase 2 study of domvanalimab + zimberelimab ± etrumadenant versus zimberelimab in first-line, metastatic, PD-L1-high non-small cell lung cancer (NSCLC). J Clin Oncol (2022) 40(36_suppl):397600–. doi: 10.1200/JCO.2022.40.36_suppl.397600
8. Spira AI, Conkling PR, Johnson ML, Gardner O, Gilbert HN, Scharville M, et al. ARC-4 study: Efficacy and safety of AB928 plus carboplatin, pemetrexed and a PD-1 antibody in participants with metastatic non-small cell lung cancer (mNSCLC). J Clin Oncol (2020) 38(15_suppl):e21659–e. doi: 10.1200/JCO.2020.38.15_suppl.e21659
9. Allard B, Longhi MS, Robson SC, Stagg J. The ectonucleotidases CD39 and CD73: Novel checkpoint inhibitor targets. Immunol Rev (2017) 276(1):121–44. doi: 10.1111/imr.12528
10. Allard B, Allard D, Buisseret L, Stagg J. The adenosine pathway in immuno-oncology. Nat Rev Clin Oncol (2020) 17(10):611–29. doi: 10.1038/s41571-020-0382-2
11. Resta R, Yamashita Y, Thompson LF. Ecto-enzyme and signaling functions of lymphocyte CD73. Immunol Rev (1998) 161:95–109. doi: 10.1111/j.1600-065X.1998.tb01574.x
12. Petruk N, Tuominen S, Akerfelt M, Mattsson J, Sandholm J, Nees M, et al. CD73 facilitates EMT progression and promotes lung metastases in triple-negative breast cancer. Sci Rep (2021) 11(1):6035. doi: 10.1038/s41598-021-85379-z
13. Ma XL, Hu B, Tang WG, Xie SH, Ren N, Guo L, et al. CD73 sustained cancer-stem-cell traits by promoting SOX9 expression and stability in hepatocellular carcinoma. J Hematol Oncol (2020) 13(1):11. doi: 10.1186/s13045-020-0845-z
14. Kurnit KC, Draisey A, Kazen RC, Chung C, Phan LH, Harvey JB, et al. Loss of CD73 shifts transforming growth factor-beta1 (TGF-beta1) from tumor suppressor to promoter in endometrial cancer. Cancer Lett (2021) 505:75–86. doi: 10.1016/j.canlet.2021.01.030
15. Boison D, Yegutkin GG. Adenosine metabolism: Emerging concepts for cancer therapy. Cancer Cell (2019) 36(6):582–96. doi: 10.1016/j.ccell.2019.10.007
16. Allard B, Cousineau I, Allard D, Buisseret L, Pommey S, Chrobak P, et al. Adenosine A2a receptor promotes lymphangiogenesis and lymph node metastasis. Oncoimmunology (2019) 8(8):1601481. doi: 10.1080/2162402X.2019.1601481
17. Fredholm BB. Adenosine–a physiological or pathophysiological agent? J Mol Med (Berl) (2014) 92(3):201–6. doi: 10.1007/s00109-013-1101-6
18. Koeppen M, Eckle T, Eltzschig HK. Selective deletion of the A1 adenosine receptor abolishes heart-rate slowing effects of intravascular adenosine in vivo. PloS One (2009) 4(8):e6784. doi: 10.1371/journal.pone.0006784
19. Sperlagh B, Vizi ES. The role of extracellular adenosine in chemical neurotransmission in the hippocampus and basal ganglia: pharmacological and clinical aspects. Curr Top Med Chem (2011) 11(8):1034–46. doi: 10.2174/156802611795347564
20. Vallon V, Muhlbauer B, Osswald H. Adenosine and kidney function. Physiol Rev (2006) 86(3):901–40. doi: 10.1152/physrev.00031.2005
21. Borea PA, Gessi S, Merighi S, Vincenzi F, Varani K. Pharmacology of adenosine receptors: The state of the art. Physiol Rev (2018) 98(3):1591–625. doi: 10.1152/physrev.00049.2017
22. Negrao MV, Skoulidis F, Montesion M, Schulze K, Bara I, Shen V, et al. Oncogene-specific differences in tumor mutational burden, PD-L1 expression, and outcomes from immunotherapy in non-small cell lung cancer. J Immunother Cancer (2021) 9(8):e002891. doi: 10.1136/jitc-2021-002891
23. Herbst RS, Baas P, Kim DW, Felip E, Perez-Gracia JL, Han JY, et al. Pembrolizumab versus docetaxel for previously treated, PD-L1-positive, advanced non-small-cell lung cancer (KEYNOTE-010): a randomised controlled trial. Lancet (2016) 387(10027):1540–50. doi: 10.1016/S0140-6736(15)01281-7
24. Hellmann MD, Callahan MK, Awad MM, Calvo E, Ascierto PA, Atmaca A, et al. Tumor mutational burden and efficacy of nivolumab monotherapy and in combination with ipilimumab in small-cell lung cancer. Cancer Cell (2019) 35(2):329. doi: 10.1016/j.ccell.2019.01.011
25. Han Y, Lee T, He Y, Raman R, Irizarry A, Martin ML, et al. The regulation of CD73 in non-small cell lung cancer. Eur J Cancer (2022) 170:91–102. doi: 10.1016/j.ejca.2022.04.025
26. Le X, Negrao MV, Reuben A, Federico L, Diao L, McGrail D, et al. Characterization of the immune landscape of EGFR-mutant NSCLC identifies CD73/Adenosine pathway as a potential therapeutic target. J Thorac Oncol (2021) 16(4):583–600. doi: 10.1016/j.jtho.2020.12.010
27. Gao ZW, Liu C, Yang L, Chen HC, Yang LF, Zhang HZ, et al. CD73 severed as a potential prognostic marker and promote lung cancer cells migration via enhancing EMT progression. Front Genet (2021) 12:728200. doi: 10.3389/fgene.2021.728200
28. Bendell JC, LoRusso P, Overman MJ, Noonan AM, Kim D-W, Strickler J, et al. Safety and efficacy of the anti-CD73 monoclonal antibody (mAb) oleclumab ± durvalumab in patients (pts) with advanced colorectal cancer (CRC), pancreatic ductal adenocarcinoma (PDAC), or EGFR-mutant non-small cell lung cancer (EGFRm NSCLC). J Clin Oncol (2021) 39(15_suppl):9047–. doi: 10.1200/JCO.2021.39.15_suppl.9047
29. Cai Y, Feng L, Wang X. Targeting the tumor promoting effects of adenosine in chronic lymphocytic leukemia. Crit Rev Oncol Hematol (2018) 126:24–31. doi: 10.1016/j.critrevonc.2018.03.022
30. Carta S, Penco F, Lavieri R, Martini A, Dinarello CA, Gattorno M, et al. Cell stress increases ATP release in NLRP3 inflammasome-mediated autoinflammatory diseases, resulting in cytokine imbalance. Proc Natl Acad Sci U S A (2015) 112(9):2835–40. doi: 10.1073/pnas.1424741112
31. Plesner L. Ecto-ATPases: identities and functions. Int Rev Cytol (1995) 158:141–214. doi: 10.1016/S0074-7696(08)62487-0
32. Horenstein AL, Chillemi A, Zaccarello G, Bruzzone S, Quarona V, Zito A, et al. A CD38/CD203a/CD73 ectoenzymatic pathway independent of CD39 drives a novel adenosinergic loop in human T lymphocytes. Oncoimmunology (2013) 2(9):e26246. doi: 10.4161/onci.26246
33. Sharma U, Pal D, Prasad R. Alkaline phosphatase: an overview. Indian J Clin Biochem (2014) 29(3):269–78. doi: 10.1007/s12291-013-0408-y
34. Zimmermann H. Prostatic acid phosphatase, a neglected ectonucleotidase. Purinergic Signal (2009) 5(3):273–5. doi: 10.1007/s11302-009-9157-z
35. Zhulai G, Oleinik E, Shibaev M, Ignatev K. Adenosine-metabolizing enzymes, adenosine kinase and adenosine deaminase, in cancer. Biomolecules (2022) 12(3):418. doi: 10.3390/biom12030418
36. Boswell-Casteel RC, Hays FA. Equilibrative nucleoside transporters-a review. Nucleosides Nucleotides Nucleic Acids (2017) 36(1):7–30. doi: 10.1080/15257770.2016.1210805
37. Shevchenko I, Mathes A, Groth C, Karakhanova S, Muller V, Utikal J, et al. Enhanced expression of CD39 and CD73 on T cells in the regulation of anti-tumor immune responses. Oncoimmunology (2020) 9(1):1744946. doi: 10.1080/2162402X.2020.1744946
38. Zhang H, Vijayan D, Li XY, Robson SC, Geetha N, Teng MWL, et al. The role of NK cells and CD39 in the immunological control of tumor metastases. Oncoimmunology (2019) 8(6):e1593809. doi: 10.1080/2162402X.2019.1593809
39. Simoni Y, Becht E, Fehlings M, Loh CY, Koo SL, Teng KWW, et al. Bystander CD8(+) T cells are abundant and phenotypically distinct in human tumour infiltrates. Nature (2018) 557(7706):575–9. doi: 10.1038/s41586-018-0130-2
40. Canale FP, Ramello MC, Nunez N, Araujo Furlan CL, Bossio SN, Gorosito Serran M, et al. CD39 expression defines cell exhaustion in tumor-infiltrating CD8(+) T cells. Cancer Res (2018) 78(1):115–28. doi: 10.1158/0008-5472.CAN-16-2684
41. Briceno P, Rivas-Yanez E, Rosemblatt MV, Parra-Tello B, Farias P, Vargas L, et al. CD73 ectonucleotidase restrains CD8+ T cell metabolic fitness and anti-tumoral activity. Front Cell Dev Biol (2021) 9:638037. doi: 10.3389/fcell.2021.638037
42. Neo SY, Yang Y, Record J, Ma R, Chen X, Chen Z, et al. CD73 immune checkpoint defines regulatory NK cells within the tumor microenvironment. J Clin Invest (2020) 130(3):1185–98. doi: 10.1172/JCI128895
43. Yan J, Li XY, Roman Aguilera A, Xiao C, Jacoberger-Foissac C, Nowlan B, et al. Control of metastases via myeloid CD39 and NK cell effector function. Cancer Immunol Res (2020) 8(3):356–67. doi: 10.1158/2326-6066.CIR-19-0749
44. Ziebart A, Huber U, Jeske S, Laban S, Doescher J, Hoffmann TK, et al. The influence of chemotherapy on adenosine-producing b cells in patients with head and neck squamous cell carcinoma. Oncotarget (2018) 9(5):5834–47. doi: 10.18632/oncotarget.23533
45. Mandapathil M, Hilldorfer B, Szczepanski MJ, Czystowska M, Szajnik M, Ren J, et al. Generation and accumulation of immunosuppressive adenosine by human CD4+CD25highFOXP3+ regulatory T cells. J Biol Chem (2010) 285(10):7176–86. doi: 10.1074/jbc.M109.047423
46. Sorrentino C, Miele L, Porta A, Pinto A, Morello S. Myeloid-derived suppressor cells contribute to A2B adenosine receptor-induced VEGF production and angiogenesis in a mouse melanoma model. Oncotarget (2015) 6(29):27478–89. doi: 10.18632/oncotarget.4393
47. Siegel RL, Miller KD, Fuchs HE, Jemal A. Cancer statistics, 2022. CA Cancer J Clin (2022) 72(1):7–33. doi: 10.3322/caac.21708
48. Rudin CM, Poirier JT, Byers LA, Dive C, Dowlati A, George J, et al. Molecular subtypes of small cell lung cancer: a synthesis of human and mouse model data. Nat Rev Cancer (2019) 19(5):289–97. doi: 10.1038/s41568-019-0133-9
49. Ellis PM, Vella ET, Ung YC. Immune checkpoint inhibitors for patients with advanced non-Small-Cell lung cancer: A systematic review. Clin Lung Cancer (2017) 18(5):444–59 e1. doi: 10.1016/j.cllc.2017.02.001
50. Gao S, Li N, Gao S, Xue Q, Wang S, Lv F, et al. Two-year follow-up of single PD-1 blockade in neoadjuvant resectable NSCLC. J Clin Oncol (2021) 39(15_suppl):8522–. doi: 10.1200/JCO.2021.39.15_suppl.8522
51. Spigel DR, Vicente D, Ciuleanu TE, Gettinger S, Peters S, Horn L, et al. Second-line nivolumab in relapsed small-cell lung cancer: CheckMate 331(☆). Ann Oncol (2021) 32(5):631–41. doi: 10.1016/j.annonc.2021.01.071
52. Dowlati A, Abbas A, Chan T, Henick B, Wang X, Doshi P, et al. Immune checkpoint blockade outcome in small-cell lung cancer and its relationship with retinoblastoma mutation status and function. JCO Precis Oncol (2022) 6:e2200257. doi: 10.1200/PO.22.00257
53. Derks JL, van Suylen RJ, Thunnissen E, den Bakker MA, Groen HJ, Smit EF, et al. Chemotherapy for pulmonary large cell neuroendocrine carcinomas: does the regimen matter? Eur Respir J (2017) 49(6):1601838. doi: 10.1183/13993003.01838-2016
54. Gosain R, Mukherjee S, Yendamuri SS, Iyer R. Management of typical and atypical pulmonary carcinoids based on different established guidelines. Cancers (Basel) (2018) 10(12):510. doi: 10.3390/cancers10120510
55. Oda R, Okuda K, Yamashita Y, Sakane T, Tatematsu T, Yokota K, et al. Long-term survivor of pulmonary combined large cell neuroendocrine carcinoma treated with nivolumab. Thorac Cancer (2020) 11(7):2036–9. doi: 10.1111/1759-7714.13471
56. Wu F, Fan J, He Y, Xiong A, Yu J, Li Y, et al. Single-cell profiling of tumor heterogeneity and the microenvironment in advanced non-small cell lung cancer. Nat Commun (2021) 12(1):2540. doi: 10.1038/s41467-021-22801-0
57. Lim ZF, Ma PC. Emerging insights of tumor heterogeneity and drug resistance mechanisms in lung cancer targeted therapy. J Hematol Oncol (2019) 12(1):134. doi: 10.1186/s13045-019-0818-2
58. Aramini B, Masciale V, Samarelli AV, Dubini A, Gaudio M, Stella F, et al. Phenotypic, functional, and metabolic heterogeneity of immune cells infiltrating non-small cell lung cancer. Front Immunol (2022) 13:959114. doi: 10.3389/fimmu.2022.959114
59. Yang H, Yao F, Davis PF, Tan ST, Hall SRR. CD73, tumor plasticity and immune evasion in solid cancers. Cancers (Basel) (2021) 13(2):177. doi: 10.3390/cancers13020177
60. Inoue Y, Yoshimura K, Kurabe N, Kahyo T, Kawase A, Tanahashi M, et al. Prognostic impact of CD73 and A2A adenosine receptor expression in non-small-cell lung cancer. Oncotarget (2017) 8(5):8738–51. doi: 10.18632/oncotarget.14434
61. Zhu J, Zeng Y, Li W, Qin H, Lei Z, Shen D, et al. CD73/NT5E is a target of miR-30a-5p and plays an important role in the pathogenesis of non-small cell lung cancer. Mol Cancer (2017) 16(1):34. doi: 10.1186/s12943-017-0591-1
62. Simpson KL, Stoney R, Frese KK, Simms N, Rowe W, Pearce SP, et al. A biobank of small cell lung cancer CDX models elucidates inter- and intratumoral phenotypic heterogeneity. Nat Cancer (2020) 1(4):437–51. doi: 10.1038/s43018-020-0046-2
63. Cai L, Liu H, Huang F, Fujimoto J, Girard L, Chen J, et al. Cell-autonomous immune gene expression is repressed in pulmonary neuroendocrine cells and small cell lung cancer. Commun Biol (2021) 4(1):314. doi: 10.1038/s42003-021-01842-7
64. Jiang T, Xu X, Qiao M, Li X, Zhao C, Zhou F, et al. Comprehensive evaluation of NT5E/CD73 expression and its prognostic significance in distinct types of cancers. BMC Cancer (2018) 18(1):267. doi: 10.1186/s12885-018-4073-7
65. He X, Gu Y, Cao Y, Hu B, Fang H, Fei Y, et al. Impact of intratumoural CD73 expression on prognosis and therapeutic response in patients with gastric cancer. Eur J Cancer (2021) 157:114–23. doi: 10.1016/j.ejca.2021.08.006
66. Monteiro I, Vigano S, Faouzi M, Treilleux I, Michielin O, Menetrier-Caux C, et al. CD73 expression and clinical significance in human metastatic melanoma. Oncotarget (2018) 9(42):26659–69. doi: 10.18632/oncotarget.25426
67. Yoshida R, Saigi M, Tani T, Springer BF, Shibata H, Kitajima S, et al. MET-induced CD73 restrains STING-mediated immunogenicity of EGFR-mutant lung cancer. Cancer Res (2022) 82(21):4079–92. doi: 10.1158/0008-5472.CAN-22-0770
68. Hsu WH, Yang JC, Mok TS, Loong HH. Overview of current systemic management of EGFR-mutant NSCLC. Ann Oncol (2018) 29(suppl_1):i3–9. doi: 10.1093/annonc/mdx702
69. Offin M, Rizvi H, Tenet M, Ni A, Sanchez-Vega F, Li BT, et al. Tumor mutation burden and efficacy of EGFR-tyrosine kinase inhibitors in patients with EGFR-mutant lung cancers. Clin Cancer Res (2019) 25(3):1063–9. doi: 10.1158/1078-0432.CCR-18-1102
70. Ricciuti B, Wang X, Alessi JV, Rizvi H, Mahadevan NR, Li YY, et al. Association of high tumor mutation burden in non-small cell lung cancers with increased immune infiltration and improved clinical outcomes of PD-L1 blockade across PD-L1 expression levels. JAMA Oncol (2022) 8(8):1160–8. doi: 10.1001/jamaoncol.2022.1981
71. Ready N, Hellmann MD, Awad MM, Otterson GA, Gutierrez M, Gainor JF, et al. First-line nivolumab plus ipilimumab in advanced non-Small-Cell lung cancer (CheckMate 568): Outcomes by programmed death ligand 1 and tumor mutational burden as biomarkers. J Clin Oncol (2019) 37(12):992–1000. doi: 10.1200/JCO.18.01042
72. Hastings K, Yu HA, Wei W, Sanchez-Vega F, DeVeaux M, Choi J, et al. EGFR mutation subtypes and response to immune checkpoint blockade treatment in non-small-cell lung cancer. Ann Oncol (2019) 30(8):1311–20. doi: 10.1093/annonc/mdz141
73. Soria JC, Ohe Y, Vansteenkiste J, Reungwetwattana T, Chewaskulyong B, Lee KH, et al. Osimertinib in untreated EGFR-mutated advanced non-Small-Cell lung cancer. N Engl J Med (2018) 378(2):113–25. doi: 10.1056/NEJMoa1713137
74. Isomoto K, Haratani K, Hayashi H, Shimizu S, Tomida S, Niwa T, et al. Impact of EGFR-TKI treatment on the tumor immune microenvironment in EGFR mutation-positive non-small cell lung cancer. Clin Cancer Res (2020) 26(8):2037–46. doi: 10.1158/1078-0432.CCR-19-2027
75. Ishii H, Azuma K, Kawahara A, Kinoshita T, Matsuo N, Naito Y, et al. Predictive value of CD73 expression for the efficacy of immune checkpoint inhibitors in NSCLC. Thorac Cancer (2020) 11(4):950–5. doi: 10.1111/1759-7714.13346
76. Tu E, McGlinchey K, Wang J, Martin P, Ching SL, Floc’h N, et al. Anti-PD-L1 and anti-CD73 combination therapy promotes T cell response to EGFR-mutated NSCLC. JCI Insight (2022) 7(3):e142843. doi: 10.1172/jci.insight.142843
77. Griesing S, Liao BC, Yang JC. CD73 is regulated by the EGFR-ERK signaling pathway in non-small cell lung cancer. Anticancer Res (2021) 41(3):1231–42. doi: 10.21873/anticanres.14880
78. Faraoni EY, Singh K, Chandra V, Le Roux O, Dai Y, Sahin I, et al. CD73-dependent adenosine signaling through Adora2b drives immunosuppression in ductal pancreatic cancer. Cancer Research (2023) CAN-22-2553. doi: 10.1158/0008-5472.CAN-22-2553
79. Huang F, Huffman KE, Wang Z, Wang X, Li K, Cai F, et al. Guanosine triphosphate links MYC-dependent metabolic and ribosome programs in small-cell lung cancer. J Clin Invest (2021) 131(1):e139929. doi: 10.1172/JCI139929
80. Marcoux N, Gettinger SN, O’Kane G, Arbour KC, Neal JW, Husain H, et al. EGFR-mutant adenocarcinomas that transform to small-cell lung cancer and other neuroendocrine carcinomas: Clinical outcomes. J Clin Oncol (2019) 37(4):278–85. doi: 10.1200/JCO.18.01585
81. Offin M, Chan JM, Tenet M, Rizvi HA, Shen R, Riely GJ, et al. Concurrent RB1 and TP53 alterations define a subset of EGFR-mutant lung cancers at risk for histologic transformation and inferior clinical outcomes. J Thorac Oncol (2019) 14(10):1784–93. doi: 10.1016/j.jtho.2019.06.002
82. Westover D, Zugazagoitia J, Cho BC, Lovly CM, Paz-Ares L. Mechanisms of acquired resistance to first- and second-generation EGFR tyrosine kinase inhibitors. Ann Oncol (2018) 29(suppl_1):i10–i9. doi: 10.1093/annonc/mdx703
83. Inoue Y, Nikolic A, Farnsworth D, Shi R, Johnson FD, Liu A, et al. Extracellular signal-regulated kinase mediates chromatin rewiring and lineage transformation in lung cancer. Elife (2021) 10:e66524. doi: 10.7554/eLife.66524.sa2
84. Iams WT, Beckermann KE, Almodovar K, Hernandez J, Vnencak-Jones C, Lim LP, et al. Small cell lung cancer transformation as a mechanism of resistance to PD-1 therapy in KRAS-mutant lung adenocarcinoma: A report of two cases. J Thorac Oncol (2019) 14(3):e45–e8. doi: 10.1016/j.jtho.2018.11.031
85. Ireland AS, Micinski AM, Kastner DW, Guo B, Wait SJ, Spainhower KB, et al. MYC drives temporal evolution of small cell lung cancer subtypes by reprogramming neuroendocrine fate. Cancer Cell (2020) 38(1):60–78.E12. doi: 10.1016/j.ccell.2020.05.001
86. Mollaoglu G, Guthrie MR, Bohm S, Bragelmann J, Can I, Ballieu PM, et al. MYC drives progression of small cell lung cancer to a variant neuroendocrine subtype with vulnerability to aurora kinase inhibition. Cancer Cell (2017) 31(2):270–85. doi: 10.1016/j.ccell.2016.12.005
87. Wu Q, Guo J, Liu Y, Zheng Q, Li X, Wu C, et al. YAP drives fate conversion and chemoresistance of small cell lung cancer. Sci Adv (2021) 7(40):eabg1850. doi: 10.1126/sciadv.abg1850
88. Dora D, Rivard C, Yu H, Bunn P, Suda K, Ren S, et al. Neuroendocrine subtypes of small cell lung cancer differ in terms of immune microenvironment and checkpoint molecule distribution. Mol Oncol (2020) 14(9):1947–65. doi: 10.1002/1878-0261.12741
89. Gay CM, Stewart CA, Park EM, Diao L, Groves SM, Heeke S, et al. Patterns of transcription factor programs and immune pathway activation define four major subtypes of SCLC with distinct therapeutic vulnerabilities. Cancer Cell (2021) 39(3):346–60 e7. doi: 10.1016/j.ccell.2020.12.014
90. Qu S, Fetsch P, Thomas A, Pommier Y, Schrump DS, Miettinen MM, et al. Molecular subtypes of primary SCLC tumors and their associations with neuroendocrine and therapeutic markers. J Thorac Oncol (2022) 17(1):141–53. doi: 10.1016/j.jtho.2021.08.763
91. Gopal P, Petty A, Rogacki K, Bera T, Bareja R, Peacock CD, et al. Multivalent state transitions shape the intratumoral composition of small cell lung carcinoma. Sci Adv (2022) 8(50):eabp8674. doi: 10.1126/sciadv.abp8674
92. Wang Y, Xu X, Maglic D, Dill MT, Mojumdar K, Ng PK, et al. Comprehensive molecular characterization of the hippo signaling pathway in cancer. Cell Rep (2018) 25(5):1304–17.E5. doi: 10.1016/j.celrep.2018.10.001
93. Kim J, Jang G, Sim SH, Park IH, Kim K, Park C. SMARCA4 depletion induces cisplatin resistance by activating YAP1-mediated epithelial-to-Mesenchymal transition in triple-negative breast cancer. Cancers (Basel) (2021) 13(21):5474. doi: 10.3390/cancers13215474
94. Yang H, Hall SRR, Sun B, Zhao L, Gao Y, Schmid RA, et al. NF2 and canonical hippo-YAP pathway define distinct tumor subsets characterized by different immune deficiency and treatment implications in human pleural mesothelioma. Cancers (Basel) (2021) 13(7):1561. doi: 10.3390/cancers13071561
95. Pearsall SM, Humphrey S, Revill M, Morgan D, Frese KK, Galvin M, et al. The rare YAP1 subtype of SCLC revisited in a biobank of 39 circulating tumor cell patient derived explant models: A brief report. J Thorac Oncol (2020) 15(12):1836–43. doi: 10.1016/j.jtho.2020.07.008
96. Gao ZW, Wang HP, Lin F, Wang X, Long M, Zhang HZ, et al. CD73 promotes proliferation and migration of human cervical cancer cells independent of its enzyme activity. BMC Cancer (2017) 17(1):135. doi: 10.1186/s12885-017-3128-5
97. Yu J, Wang X, Lu Q, Wang J, Li L, Liao X, et al. Extracellular 5'-nucleotidase (CD73) promotes human breast cancer cells growth through AKT/GSK-3beta/beta-catenin/cyclinD1 signaling pathway. Int J Cancer (2018) 142(5):959–67. doi: 10.1002/ijc.31112
98. Gardani CFF, Cappellari AR, de Souza JB, da Silva BT, Engroff P, Moritz CEJ, et al. Hydrolysis of ATP, ADP, and AMP is increased in blood plasma of prostate cancer patients. Purinergic Signal (2019) 15(1):95–105. doi: 10.1007/s11302-018-9642-3
99. Wang M, Jia J, Cui Y, Peng Y, Jiang Y. CD73-positive extracellular vesicles promote glioblastoma immunosuppression by inhibiting T-cell clonal expansion. Cell Death Dis (2021) 12(11):1065. doi: 10.1038/s41419-021-04359-3
100. Yu X, Liu W, Wang Z, Wang H, Liu J, Huang C, et al. CD73 induces gemcitabine resistance in pancreatic ductal adenocarcinoma: A promising target with non-canonical mechanisms. Cancer Lett (2021) 519:289–303. doi: 10.1016/j.canlet.2021.07.024
101. Ma XL, Shen MN, Hu B, Wang BL, Yang WJ, Lv LH, et al. CD73 promotes hepatocellular carcinoma progression and metastasis via activating PI3K/AKT signaling by inducing Rap1-mediated membrane localization of P110beta and predicts poor prognosis. J Hematol Oncol (2019) 12(1):37. doi: 10.1186/s13045-019-0724-7
102. Xu Z, Gu C, Yao X, Guo W, Wang H, Lin T, et al. CD73 promotes tumor metastasis by modulating RICS/RhoA signaling and EMT in gastric cancer. Cell Death Dis (2020) 11(3):202. doi: 10.1038/s41419-020-2403-6
103. Azambuja JH, Gelsleichter NE, Beckenkamp LR, Iser IC, Fernandes MC, Figueiro F, et al. CD73 downregulation decreases In vitro and In vivo glioblastoma growth. Mol Neurobiol (2019) 56(5):3260–79. doi: 10.1007/s12035-018-1240-4
104. Zhang H, Cao Y, Tang J, Wang R. CD73 (NT5E) promotes the proliferation and metastasis of lung adenocarcinoma through the EGFR/AKT/mTOR pathway. BioMed Res Int (2022) 2022:9944847. doi: 10.1155/2022/9944847
105. Hasmim M, Xiao M, Van Moer K, Kumar A, Oniga A, Mittelbronn M, et al. SNAI1-dependent upregulation of CD73 increases extracellular adenosine release to mediate immune suppression in TNBC. Front Immunol (2022) 13:982821. doi: 10.3389/fimmu.2022.982821
106. Synnestvedt K, Furuta GT, Comerford KM, Louis N, Karhausen J, Eltzschig HK, et al. Ecto-5’-nucleotidase (CD73) regulation by hypoxia-inducible factor-1 mediates permeability changes in intestinal epithelia. J Clin Invest (2002) 110(7):993–1002. doi: 10.1172/JCI0215337
107. Xu Y, Wang Y, Yan S, Zhou Y, Yang Q, Pan Y, et al. Intracellular adenosine regulates epigenetic programming in endothelial cells to promote angiogenesis. EMBO Mol Med (2017) 9(9):1263–78. doi: 10.15252/emmm.201607066
108. Garcia-Rocha R, Monroy-Garcia A, Hernandez-Montes J, Weiss-Steider B, Gutierrez-Serrano V, Del Carmen Fuentes-Castaneda M, et al. Cervical cancer cells produce TGF-beta1 through the CD73-adenosine pathway and maintain CD73 expression through the autocrine activity of TGF-beta1. Cytokine (2019) 118:71–9. doi: 10.1016/j.cyto.2018.09.018
109. Nam MW, Kim CW, Choi KC. Epithelial-mesenchymal transition-inducing factors involved in the progression of lung cancers. Biomol Ther (Seoul) (2022) 30(3):213–20. doi: 10.4062/biomolther.2021.178
110. Lupia M, Angiolini F, Bertalot G, Freddi S, Sachsenmeier KF, Chisci E, et al. CD73 regulates stemness and epithelial-mesenchymal transition in ovarian cancer-initiating cells. Stem Cell Rep (2018) 10(4):1412–25. doi: 10.1016/j.stemcr.2018.02.009
111. Dongre A, Rashidian M, Eaton EN, Reinhardt F, Thiru P, Zagorulya M, et al. Direct and indirect regulators of epithelial-mesenchymal transition-mediated immunosuppression in breast carcinomas. Cancer Discovery (2021) 11(5):1286–305. doi: 10.1158/2159-8290.CD-20-0603
112. Wu R, Chen Y, Li F, Li W, Zhou H, Yang Y, et al. Effects of CD73 on human colorectal cancer cell growth in vivo and in vitro. Oncol Rep (2016) 35(3):1750–6. doi: 10.3892/or.2015.4512
113. Stagg J, Divisekera U, McLaughlin N, Sharkey J, Pommey S, Denoyer D, et al. Anti-CD73 antibody therapy inhibits breast tumor growth and metastasis. Proc Natl Acad Sci U S A (2010) 107(4):1547–52. doi: 10.1073/pnas.0908801107
114. Zhou L, Jia S, Chen Y, Wang W, Wu Z, Yu W, et al. The distinct role of CD73 in the progression of pancreatic cancer. J Mol Med (Berl) (2019) 97(6):803–15. doi: 10.1007/s00109-018-01742-0
115. Baghbani E, Noorolyai S, Rahmani S, Shanehbandi D, Shadbad MA, Aghebati-Maleki L, et al. Silencing tumor-intrinsic CD73 enhances the chemosensitivity of NSCLC and potentiates the anti-tumoral effects of cisplatin: An in vitro study. BioMed Pharmacother (2022) 145:112370. doi: 10.1016/j.biopha.2021.112370
116. Yan A, Joachims ML, Thompson LF, Miller AD, Canoll PD, Bynoe MS. CD73 promotes glioblastoma pathogenesis and enhances its chemoresistance via A2B adenosine receptor signaling. J Neurosci (2019) 39(22):4387–402. doi: 10.1523/JNEUROSCI.1118-18.2019
117. Allard D, Cousineau I, Ma E, Allard B, Barèche Y, Fleury H, et al. The CD73 immune checkpoint promotes tumor cell metabolic fitness. bioRxiv (2022), 518350. doi: 10.1101/2022.11.29.518350
118. Nguyen AM, Zhou J, Sicairos B, Sonney S, Du Y. Upregulation of CD73 confers acquired radioresistance and is required for maintaining irradiation-selected pancreatic cancer cells in a mesenchymal state. Mol Cell Proteomics (2020) 19(2):375–89. doi: 10.1074/mcp.RA119.001779
119. Loi S, Pommey S, Haibe-Kains B, Beavis PA, Darcy PK, Smyth MJ, et al. CD73 promotes anthracycline resistance and poor prognosis in triple negative breast cancer. Proc Natl Acad Sci U S A (2013) 110(27):11091–6. doi: 10.1073/pnas.1222251110
120. Turcotte M, Allard D, Mittal D, Bareche Y, Buisseret L, Jose V, et al. CD73 promotes resistance to HER2/ErbB2 antibody therapy. Cancer Res (2017) 77(20):5652–63. doi: 10.1158/0008-5472.CAN-17-0707
121. Reinhardt J, Landsberg J, Schmid-Burgk JL, Ramis BB, Bald T, Glodde N, et al. MAPK signaling and inflammation link melanoma phenotype switching to induction of CD73 during immunotherapy. Cancer Res (2017) 77(17):4697–709. doi: 10.1158/0008-5472.CAN-17-0395
122. Samanta D, Park Y, Ni X, Li H, Zahnow CA, Gabrielson E, et al. Chemotherapy induces enrichment of CD47(+)/CD73(+)/PDL1(+) immune evasive triple-negative breast cancer cells. Proc Natl Acad Sci U S A (2018) 115(6):E1239–E48. doi: 10.1073/pnas.1718197115
123. Snider NT, Altshuler PJ, Wan S, Welling TH, Cavalcoli J, Omary MB. Alternative splicing of human NT5E in cirrhosis and hepatocellular carcinoma produces a negative regulator of ecto-5’-nucleotidase (CD73). Mol Biol Cell (2014) 25(25):4024–33. doi: 10.1091/mbc.e14-06-1167
124. Jeong YM, Cho H, Kim TM, Kim Y, Jeon S, Bychkov A, et al. CD73 overexpression promotes progression and recurrence of papillary thyroid carcinoma. Cancers (Basel) (2020) 12(10):3042. doi: 10.3390/cancers12103042
125. Linnemann C, Schildberg FA, Schurich A, Diehl L, Hegenbarth SI, Endl E, et al. Adenosine regulates CD8 T-cell priming by inhibition of membrane-proximal T-cell receptor signalling. Immunology (2009) 128(1 Suppl):e728–37. doi: 10.1111/j.1365-2567.2009.03075.x
126. Chambers AM, Lupo KB, Wang J, Cao J, Utturkar S, Lanman N, et al. Engineered natural killer cells impede the immunometabolic CD73-adenosine axis in solid tumors. Elife (2022) 11:e73699. doi: 10.7554/eLife.73699.sa2
127. Jeske SS, Brand M, Ziebart A, Laban S, Doescher J, Greve J, et al. Adenosine-producing regulatory b cells in head and neck cancer. Cancer Immunol Immunother (2020) 69(7):1205–16. doi: 10.1007/s00262-020-02535-6
128. Antonioli L, Fornai M, Blandizzi C, Pacher P, Hasko G. Adenosine signaling and the immune system: When a lot could be too much. Immunol Lett (2019) 205:9–15. doi: 10.1016/j.imlet.2018.04.006
129. Wilson JM, Ross WG, Agbai ON, Frazier R, Figler RA, Rieger J, et al. The A2B adenosine receptor impairs the maturation and immunogenicity of dendritic cells. J Immunol (2009) 182(8):4616–23. doi: 10.4049/jimmunol.0801279
130. Hofer S, Ivarsson L, Stoitzner P, Auffinger M, Rainer C, Romani N, et al. Adenosine slows migration of dendritic cells but does not affect other aspects of dendritic cell maturation. J Invest Dermatol (2003) 121(2):300–7. doi: 10.1046/j.1523-1747.2003.12369.x
131. Novitskiy SV, Ryzhov S, Zaynagetdinov R, Goldstein AE, Huang Y, Tikhomirov OY, et al. Adenosine receptors in regulation of dendritic cell differentiation and function. Blood (2008) 112(5):1822–31. doi: 10.1182/blood-2008-02-136325
132. Sorrentino C, Hossain F, Rodriguez PC, Sierra RA, Pannuti A, Osborne BA, et al. Adenosine A2A receptor stimulation inhibits TCR-induced Notch1 activation in CD8+T-cells. Front Immunol (2019) 10:162. doi: 10.3389/fimmu.2019.00162
133. Kinsey GR, Huang L, Jaworska K, Khutsishvili K, Becker DA, Ye H, et al. Autocrine adenosine signaling promotes regulatory T cell-mediated renal protection. J Am Soc Nephrol (2012) 23(9):1528–37. doi: 10.1681/ASN.2012010070
134. Zhu M, Huang Y, Bender ME, Girard L, Kollipara R, Eglenen-Polat B, et al. Evasion of innate immunity contributes to small cell lung cancer progression and metastasis. Cancer Res (2021) 81(7):1813–26. doi: 10.1158/0008-5472.CAN-20-2808
135. Best SA, Hess JB, Souza-Fonseca-Guimaraes F, Cursons J, Kersbergen A, Dong X, et al. Harnessing natural killer immunity in metastatic SCLC. J Thorac Oncol (2020) 15(9):1507–21. doi: 10.1016/j.jtho.2020.05.008
136. Hess JB, Sutherland KD, Best SA. Exploring natural killer cell immunology as a therapeutic strategy in lung cancer. Transl Lung Cancer Res (2021) 10(6):2788–805. doi: 10.21037/tlcr-20-765
137. Hsu J, Hodgins JJ, Marathe M, Nicolai CJ, Bourgeois-Daigneault MC, Trevino TN, et al. Contribution of NK cells to immunotherapy mediated by PD-1/PD-L1 blockade. J Clin Invest (2018) 128(10):4654–68. doi: 10.1172/JCI99317
138. Dhatchinamoorthy K, Colbert JD, Rock KL. Cancer immune evasion through loss of MHC class I antigen presentation. Front Immunol (2021) 12:636568. doi: 10.3389/fimmu.2021.636568
139. Morrison BJ, Steel JC, Morris JC. Reduction of MHC-I expression limits T-lymphocyte-mediated killing of cancer-initiating cells. BMC Cancer (2018) 18(1):469. doi: 10.1186/s12885-018-4389-3
140. Jongsma MLM, Neefjes J, Spaapen RM. Playing hide and seek: Tumor cells in control of MHC class I antigen presentation. Mol Immunol (2021) 136:36–44. doi: 10.1016/j.molimm.2021.05.009
141. Marquardt N, Kekalainen E, Chen P, Kvedaraite E, Wilson JN, Ivarsson MA, et al. Human lung natural killer cells are predominantly comprised of highly differentiated hypofunctional CD69(-)CD56(dim) cells. J Allergy Clin Immunol (2017) 139(4):1321–30 e4. doi: 10.1016/j.jaci.2016.07.043
142. Ferrari de Andrade L, Kumar S, Luoma AM, Ito Y, Alves da Silva PH, Pan D, et al. Inhibition of MICA and MICB shedding elicits NK-Cell-Mediated immunity against tumors resistant to cytotoxic T cells. Cancer Immunol Res (2020) 8(6):769–80. doi: 10.1158/2326-6066.CIR-19-0483
143. Chambers AM, Wang J, Lupo KB, Yu H, Atallah Lanman NM, Matosevic S. Adenosinergic signaling alters natural killer cell functional responses. Front Immunol (2018) 9:2533. doi: 10.3389/fimmu.2018.02533
144. Stagg J, Beavis PA, Divisekera U, Liu MC, Moller A, Darcy PK, et al. CD73-deficient mice are resistant to carcinogenesis. Cancer Res (2012) 72(9):2190–6. doi: 10.1158/0008-5472.CAN-12-0420
145. Raskovalova T, Lokshin A, Huang X, Jackson EK, Gorelik E. Adenosine-mediated inhibition of cytotoxic activity and cytokine production by IL-2/NKp46-activated NK cells: involvement of protein kinase a isozyme I (PKA I). Immunol Res (2006) 36(1-3):91–9. doi: 10.1385/IR:36:1:91
146. Young A, Ngiow SF, Gao Y, Patch AM, Barkauskas DS, Messaoudene M, et al. A2AR adenosine signaling suppresses natural killer cell maturation in the tumor microenvironment. Cancer Res (2018) 78(4):1003–16. doi: 10.1158/0008-5472.CAN-17-2826
147. Chambers AM, Wang J, Dao TN, Lupo KB, Veenhuis P, Ayers MG, et al. Functional expression of CD73 on human natural killer cells. Cancer Immunol Immunother (2022) 71(12):3043–56. doi: 10.1007/s00262-022-03219-z
148. Geoghegan JC, Diedrich G, Lu X, Rosenthal K, Sachsenmeier KF, Wu H, et al. Inhibition of CD73 AMP hydrolysis by a therapeutic antibody with a dual, non-competitive mechanism of action. MAbs (2016) 8(3):454–67. doi: 10.1080/19420862.2016.1143182
149. Thompson EA, Powell JD. Inhibition of the adenosine pathway to potentiate cancer immunotherapy: Potential for combinatorial approaches. Annu Rev Med (2021) 72:331–48. doi: 10.1146/annurev-med-060619-023155
150. Augustin RC, Leone RD, Naing A, Fong L, Bao R, Luke JJ. Next steps for clinical translation of adenosine pathway inhibition in cancer immunotherapy. J Immunother Cancer (2022) 10(2):e004089. doi: 10.1136/jitc-2021-004089
151. Manji GA, Wainberg ZA, Krishnan K, Giafis N, Udyavar A, Quah CS, et al. ARC-8: Phase I/Ib study to evaluate safety and tolerability of AB680 + chemotherapy + zimberelimab (AB122) in patients with treatment-naive metastatic pancreatic adenocarcinoma (mPDAC). J Clin Oncol (2021) 39(3_suppl):404–. doi: 10.1200/JCO.2021.39.3_suppl.404
152. Li XY, Moesta AK, Xiao C, Nakamura K, Casey M, Zhang H, et al. Targeting CD39 in cancer reveals an extracellular ATP- and inflammasome-driven tumor immunity. Cancer Discovery (2019) 9(12):1754–73. doi: 10.1158/2159-8290.CD-19-0541
153. Perrot I, Michaud HA, Giraudon-Paoli M, Augier S, Docquier A, Gros L, et al. Blocking antibodies targeting the CD39/CD73 immunosuppressive pathway unleash immune responses in combination cancer therapies. Cell Rep (2019) 27(8):2411–25 e9. doi: 10.1016/j.celrep.2019.04.091
154. Hayes GM, Cairns B, Levashova Z, Chinn L, Perez M, Theunissen JW, et al. CD39 is a promising therapeutic antibody target for the treatment of soft tissue sarcoma. Am J Transl Res (2015) 7(6):1181–8.
155. Guo S, Han F, Zhu W. CD39 - a bright target for cancer immunotherapy. BioMed Pharmacother (2022) 151:113066. doi: 10.1016/j.biopha.2022.113066
Keywords: adenosine, CD73/NT5E, oleclumab, TKI - tyrosine kinase inhibitor, NSCLC, immune checkppoint blockade, metabolite
Citation: Kowash RR and Akbay EA (2023) Tumor intrinsic and extrinsic functions of CD73 and the adenosine pathway in lung cancer. Front. Immunol. 14:1130358. doi: 10.3389/fimmu.2023.1130358
Received: 23 December 2022; Accepted: 13 March 2023;
Published: 24 March 2023.
Edited by:
Qizhen Shi, Medical College of Wisconsin, United StatesReviewed by:
Russell Jenkins, Massachusetts General Hospital and Harvard Medical School, United StatesAlex Jaeger, Moffitt Cancer Center, United States
Copyright © 2023 Kowash and Akbay. This is an open-access article distributed under the terms of the Creative Commons Attribution License (CC BY). The use, distribution or reproduction in other forums is permitted, provided the original author(s) and the copyright owner(s) are credited and that the original publication in this journal is cited, in accordance with accepted academic practice. No use, distribution or reproduction is permitted which does not comply with these terms.
*Correspondence: Esra A. Akbay, esra.akbay@utsouthwestern.edu