- 1Institute of Bacteriology and Mycology, Centre for Infectious Diseases, Faculty of Veterinary Medicine, University of Leipzig, Leipzig, Germany
- 2Institute of Immunology, Centre for Infectious Diseases, Faculty of Veterinary Medicine, University of Leipzig, Leipzig, Germany
Streptococcus suis (S. suis) is an important porcine pathogen, causing severe disease like meningitis and septicemia primarily in piglets. Previous work showed that the IgM-degrading enzyme of S. suis (IdeSsuis) specifically cleaves soluble porcine IgM and is involved in complement evasion. The objective of this study was to investigate IdeSsuis cleavage of the IgM B cell receptor and subsequent changes in B cell receptor mediated signaling. Flow cytometry analysis revealed cleavage of the IgM B cell receptor by recombinant (r) IdeSsuis_homologue as well as IdeSsuis derived from culture supernatants of S. suis serotype 2 on porcine PBMCs and mandibular lymph node cells. Point-mutated rIdeSsuis_homologue_C195S did not cleave the IgM B cell receptor. After receptor cleavage by rIdeSsuis_homologue, it took at least 20 h for mandibular lymph node cells to restore the IgM B cell receptor to levels comparable to cells previously treated with rIdeSsuis_homologue_C195S. B cell receptor mediated signaling after specific stimulation via the F(ab’)2 portion was significantly inhibited by rIdeSsuis_homologue receptor cleavage in IgM+ B cells, but not in IgG+ B cells. Within IgM+ cells, CD21+ B2 cells and CD21- B1-like cells were equally impaired in their signaling capacity upon rIdeSsuis_homologue B cell receptor cleavage. In comparison, intracellular B cell receptor independent stimulation with tyrosine phosphatase inhibitor pervanadate increased signaling in all investigated B cell types. In conclusion, this study demonstrates IdeSsuis cleavage efficacy on the IgM B cell receptor and its consequences for B cell signaling.
Introduction
Streptococcus suis (S. suis) is one of the most important porcine pathogens, causing mainly meningitis, arthritis, endocarditis, serositis, and septicemia, which lead to high economic losses (1). Disease mostly occurs in piglets around 4 to 10 weeks of age. Additionally, S. suis is a successful colonizer of mucosal surfaces in pigs of all ages, especially the nose and tonsils, but also the gastrointestinal and genital tract, without leading to clinical signs (2). S. suis is also an emerging zoonotic pathogen with outbreaks mostly in Asia (3). Currently, it is classified into 29 serotypes (cps), depending on the composition of its polysaccharide capsule, and various sequence types (4). The distribution of serotypes shows great geographical differences. Overall S. suis serotype 2 is the most important worldwide, causing human cases and most frequently isolated from diseased pigs (5). S. suis expresses a variety of virulence factors, e.g. the polysaccharide capsule (6–11).
S. suis also secretes a 124 kDa cysteine protease, designated IgM-degrading enzyme of S. suis (IdeSsuis), with sequence homology to other streptococcal proteases such as IgG proteases IdeS of S. pyogenes, IdeE of S. equi subsp. equi and IdeZ of S. equi subsp. zooepidemicus (12–15). IdeSsuis cleaves exclusively porcine IgM and is the only known bacterial IgM protease so far (12). Cysteine at position 195 in the active center is crucial as point mutation to serine (IdeSsuis_C195S) leads to loss of cleavage activity (16). Cleavage occurs between constant domain C2 and C3 of IgM (17). IgM contains a C1q-binding motif in C3 to activate the classical complement pathway. Therefore cleavage by IdeSsuis abrogates IgM labeling and complement activation on the bacterial surface (16, 17). The deletion mutant S. suis 10ΔIdeSsuis shows attenuated survival in porcine blood in vitro in the presence of increased IgM levels binding to the bacterial surface (16, 17). However, IgM cleavage by IdeSsuis is not crucial for the virulence of S. suis serotype 2 in an intranasal infection model in piglets (16).
IgM is the most important natural antibody produced by B1 cells, and the first to be produced after antigen encounter by conventional B2 cells (18, 19). Levels of serum IgM binding to the surface of a S. suis cps 7 strain were significantly increased in 8-week-old in comparison to 4-week-old piglets (20). At that critical time of increased susceptibility to S. suis infection, specific maternal IgG antibody levels decline (21). Soluble pentameric IgM mediates aggregation and agglutination of antigen and activates the classical complement pathway (18, 22). Furthermore, the transmembrane monomeric IgM B cell receptor (BCR) is typically expressed on naïve mature porcine B cells before isotype switch occurs (23, 24). Upon antigen encounter, activation of B cells leads to somatic hypermutation, VDJ recombination and class switch to IgG, IgA or IgE or cells remain producing IgM (25–27). The IgM monomer as transmembrane protein represents the ligand-binding part of the IgM BCR. For signal transduction, the monomeric membrane-bound immunoglobulin requires association with a glycoprotein dimer of Igα (CD79α) and Igβ (CD79β) which each contain an immunoreceptor tyrosine-based activation motif (ITAM) (26). Receptor activation promotes recruitment of Src-family protein tyrosine kinases (PTK) such as Syk and Lyn which leads to phosphorylation of ITAMs (28). The next step in the signaling cascade is the formation of a membrane-associated signaling complex around B cell adaptor protein SH2-domain-containing leukocyte protein (SLP65, also known as BLNK). This signalosome contains signaling proteins such as phospholipase C-γ2 (PLC-γ2) (29). The following downstream phosphorylation cascade mediates second messenger pathways and eventually leads to B cell activation and differentiation (30). CD45 and CD19 function as positive coregulatory membrane proteins and enhance phosphorylation signals. Negative coregulator CD22 contains immunoreceptor tyrosine-based inhibitory motifs (ITIMs) which facilitate recruitment of protein tyrosine phosphatases (PTPs) like SHP1 and therefore inhibit B cell signaling (30). In contrast to BCR signaling after antigen encounter, tonic signaling occurs ligand-independent (29). It plays an important role in B cell survival and development (30). Treatment of B cells with the PTP inhibitor pervanadate increases tonic signaling (31).
This study was initiated by the finding that IdeSsuis specifically cleaves soluble porcine IgM (12, 16, 17). Hence, we investigated cleavage activity of IdeSsuis on porcine IgM+ B cells and tested the working hypothesis that cleavage of the IgM BCR interferes with IgM BCR-mediated signaling and thereby may modulate early adaptive host immunity in pigs infected with S. suis.
Materials and methods
Bacterial strains and growth conditions
S. suis serotype 2 strain 10 (wildtype, wt), kindly provided by Hilde Smith (Wageningen University and Research, Lelystad, Netherlands), was grown in Todd-Hewitt-Broth (THB) (Bacto, BD, Heidelberg, Germany, catalogue 249240) or on Columbia blood agar plates (Thermo Oxoid, Schwerte, Germany, catalogue PB5039A), at 37°C with 5% CO2. The isogenic in frame deletion mutant S. suis 10ΔideSsuis∇ideSsuis_C195S (∇ideSsuis_C195S) was generated in a previous study (16) and cultivated the same way as the wildtype. Escherichia coli BL21 carrying either pETideSsuis_homologue (12) or pETideSsuis_homologue_C195S (16) were cultured in Luria-Bertani (LB) medium with 100 μg/mL ampicillin.
Expression and purification of recombinant (r) proteins
Recombinant IdeSsuis_homologue (rIdeSsuis_h) and recombinant IdeSsuis_homologue_C195S (rIdeSsuis_h_C195S) were expressed in Escherichia coli BL21 pETideSsuis_homologue and BL21 pETideSsuis_homologue_C195S, respectively, after addition of isopropyl-β-D-thiogalactopyranoside (IPTG) during the exponential growth phase and lastly purified through Ni-affinity chromatography as described previously (12, 16).
Concentration of wt IdeSsuis from culture supernatant
S. suis 10 (wildtype, wt) and 10ΔideSsuis∇ideSsuis_C195S were grown in Iscove′s Modified Dulbecco′s Medium (IMDM, PAN Biotech, Aidenbach, Germany, catalogue P04-20150) supplemented with 5% heat-inactivated fetal calf serum (FCS) until the late exponential growth phase (OD600nm 1.0 – 1.3) and then centrifuged at 3,400 x g for 10 min. The culture supernatant was collected and sterile filtered (0.45 µm, PES-Membrane, Sarstedt via Schubert Laborfachhandel, Leipzig, Germany, catalogue 1826-001). 24-fold concentration of IdeSsuis or IdeSsuis_C195S, respectively, was performed using Vivaspin 20® filters with a 100 kDa cutoff (Sartorius, Göttingen, Germany, catalogue VS2042) according to manufacturer information. Activity of IdeSsuis and IdeSsuis_C195S in concentrated supernatants was verified by Western blot analysis.
SDS-PAGE and Western blot analysis
Western blot analysis was conducted to detect IdeSsuis or its derivatives as well as immunoglobulins and respective cleavage products. To verify cleavage activity of IdeSsuis of S. suis on soluble IgM, 100 µl 24-fold concentrated culture supernatant was preincubated with 1:100 diluted porcine serum for 2 h at 37°C on a rotator. To detect secreted IgM in B cell culture supernatants, porcine mandibular lymph node cells and PBMCs were incubated with rIdeSsuis_homologue or rIdeSsuis_homologue_C195S for 45 min at 37°C, followed by removal of the recombinant proteins and cleavage products by extensive washing. Receptor cleavage was confirmed by flow cytometry as described below. Cells were resuspended in IMDM culture medium and incubated for 21 h. Cell culture supernatant was collected directly, 1 h and 21 h after washing and investigated in an anti-IgM Western blot.
Proteins were separated in SDS-Page under reducing conditions using 4% stacking gels and 10% separating gels, followed by semi-dry blotting onto a nitrocellulose membrane (Roth, Arlesheim, Switzerland, catalogue HP40.1). The membrane was blocked for 1 h at room temperature (RT) or overnight at 4°C under constant shaking in Tris-buffered saline with 0.05% Tween (TBST) supplemented with 5% skim milk powder. All primary and secondary antibodies and corresponding dilutions used are specified in Supplementary Table 1. Antibody incubations were performed in TBST with 1% skim milk powder at RT under constant shaking. Proteins were analysed using WesternBright™ chemiluminescence substrate (Biozym, Hessisch Oldendorf, Germany, catalogue 541014) according to manufacturer’s instructions. Detection was carried out using Fusion SL imaging system and FusionCapt Advance Solo 4 software (both by Vilber Lourmat, Eberhardzell, Germany). Marker bands were overlaid automatically.
Purification of immunoglobulins
All chromatographic purification procedures were carried out with the ÄCTA prime plus chromatography system (GE Healthcare, Little Chalfont, United Kingdom). Size exclusion chromatography (SEC) was performed with the HiLoad 16/600 Superdex 200pg column (GE Healthcare via VWR, Darmstadt, Germany, catalogue 28-9893-35) under the following conditions: flow rate 1 mL/min, 2 mL sample load, collected eluate 2 mL/tube and phosphate buffered saline (PBS, pH 7.35) as running buffer. For affinity chromatography and pre-absorption steps, proteins were covalently bound to CNBr-activated Sepharose 4B (GE Healthcare, Little Chalfont, United Kingdom, catalogue 17-0430-01) according to manufacturer instructions (instruction #71-7086-00 AF). Elution was performed with 0.1 M citric acid/sodium phosphate, pH 2.5 and the eluates were neutralized with 0.5 M trisodium phosphate.
For purification of IgM, porcine serum was precipitated with 6% polyethylenglycole 4000 (PEG4000, Carl Roth, Arlesheim, Switzerland, catalogue 0156), the precipitate was then solubilized in PBS and purified by affinity chromatography with Sepharose columns covalently coupled to Protein G (HiTrap protein G column, GE Healthcare via VWR, Darmstadt, Germany, catalogue 29–0485-81), followed by size exclusion chromatography. The eluates with corresponding size to IgM were pooled and concentrated with 10 kDa cutoff centrifugal filters (VIVASPIN 20®, Sartorius, Göttingen, Germany, catalogue VS2001).
Anti-porcine IgM F(ab')2 polyclonal antibodies were generated as described in (16). Briefly, purified porcine IgM was cleaved by rIdeSsuis(20 µg rIdeSsuis/mg IgM) and thereby generated IgM F(ab')2 fragments were purified by SEC (see above) and covalently coupled to CNBr-activated Sepharose 4B columns. Within a previous study (16), the purified IgM F(ab')2 fragments were also used as antigen for rabbit immunization consisting of one priming and two booster immunizations. A part of the generated rabbit antiserum was precipitated with 40% saturated ammonium sulfate solution and the precipitate was solubilized in 0.1 M sodium acetate buffer, pH 4.5. One milligram pepsin (3829 U/mg, Fluka Analytical via Sigma-Aldrich, Darmstadt, Germany, catalogue 77152) was added to 50 mg gammaglobulin, followed by incubation for 24h at 37°C. Resulting rabbit anti-IgM F(ab')2 antibodies were first purified by affinity chromatography with IgM-F(ab')2-coupled Sepharose 4B columns (see above). After neutralization (pH 7.5), the eluate was preabsorbed with porcine IgG-coupled Sepharose 4B columns (10 mg IgG/mL Sepharose) and the flow through was concentrated with 10 kDa cutoff centrifugal filters to 2 mL (VIVASPIN 20®, Sartorius, Göttingen, Germany, catalogue VS2001). After a second purification step via SEC, a part of the rabbit anti-IgM F(ab')2 antibody preparation was conjugated wit NHS-fluorescein (ThermoFisher Scientific, Schwerte, Germany, catalogue 46410) and purified following manufacturer instructions. The degree of labeling was calculated from spectrophotometric measurements as specified in the manufacturer’s instructions. The other part of the rabbit anti-IgM F(ab')2 antibody preparation remained pure and was used in the stimulation experiments. The final purified polyclonal rabbit anti-porcine IgM F(ab')2 antibody preparation (following referred to as anti-IgM F(ab')2) was stored at 4°C in PBS supplemented with 0.1% ProClin™.
Cells
Mandibular lymph nodes and whole blood was collected from commercially farmed pigs (German Landrace x Pietrain or German saddleback pig) 11 months of age (data shown in Figures 1, 2, and Supplementary Figures 3, 6A) or between 5 and 16 weeks of age (data shown in Figures 3-5, and Supplementary Figures 2, 6B, 7–10).
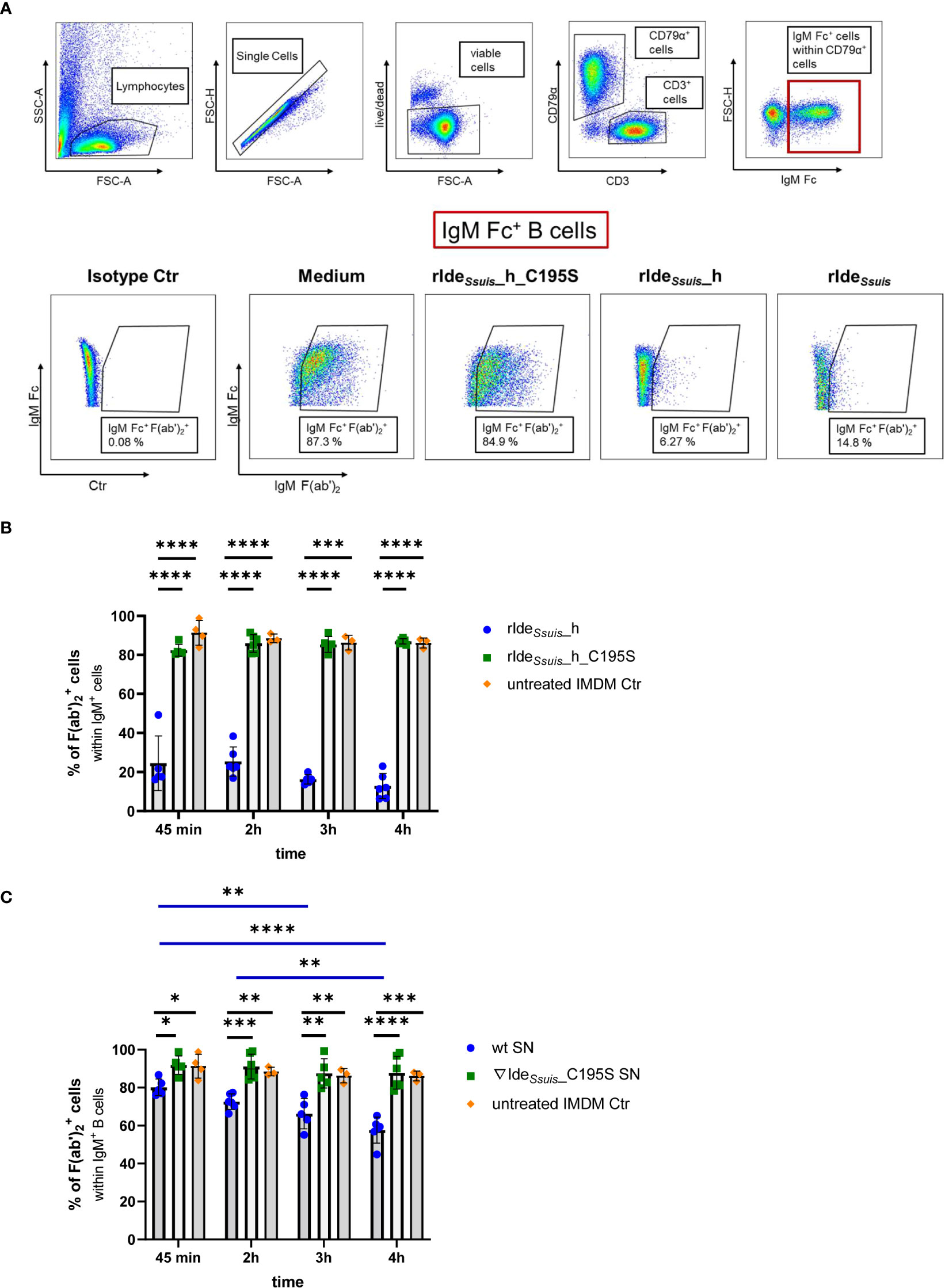
Figure 1 Recombinant and S. suis derived IdeSsuis cleave the B cell receptor on porcine CD79α+IgM+ B cells. (A) Gating strategy to analyze viable IgM+ B cells (IgM+CD79α+CD3-) after treatment with rIdeSsuis_homologue (rIdeSsuis_h), rIdeSsuis or non-functional point-mutated rIdeSsuis_homologue_C195S (rIdeSsuis_h_C195S). Representative pseudocolor plots of mLN cells of 11-month-old pigs are shown. 1 x 106 cells were incubated with 4 µg of each protein for 45 min at 37 °C. Viable cells were gated for the B cell marker CD79α and then for porcine IgM Fc. To detect cleavage, anti-IgM F(ab’)2 was used (lower panels, see Material and Methods). IgM+ B cells were gated for an intact (IgM Fc+ F(ab')2+) BCR. After receptor cleavage, cells lose the signal for IgM F(ab')2. (B) BCR cleavage with recombinant proteins. Significant reduction of IgM F(ab')2+ cells by rIdeSsuis_h in contrast to rIdeSsuis_h_C195S on porcine B cells (gating as described in 1A) is detectable after 45 min and persist until 4 h. Mandibular lymph node cells of 11-month-old pigs (n = 6) were incubated with 4 µg/1x106 cells of rIdeSsuis_h or rIdeSsuis_h_C195S for 45 min, 2 h, 3 h, or 4 h. Cells were analyzed for the percentage of IgM F(ab')2+ cells within IgM Fc+ B cells using flow cytometry. Statistical analysis was conducted with mixed ANOVA with Turkey`s multiple comparisons test. Bars and error bars represent mean and standard deviation, significant differences are indicated (p< 0.001 ***, p < 0.0001 ****). (C) BCR cleavage with culture supernatant (SN). Significant reduction of IgM F(ab')2+ cells by S. suis 10 (wt) supernatant in contrast to S. suis 10ΔideSsuis∇ideSsuis_C195S (10∇ideSsuis_C195S) supernatant on porcine B cells (gating as described in 1A) is detectable after incubation for 45 min and increases until an incubation period of 4 h. Mandibular lymph node cells of 11-month-old pigs (n = 6) were incubated with 100µl/1x106 cells of 24-fold concentrated culture supernatants for 45 min, 2 h, 3 h, or 4 h. Cells were analyzed for the percentage of IgM Fc+ F(ab')2+ cells within IgM+ B cells using flow cytometry. Statistical analysis was conducted with mixed ANOVA with Turkey`s multiple comparisons test. Bars and error bars represent mean and standard deviation, significant differences are indicated (p< 0.1 *, p < 0.01 **, p < 0.001 ***, p < 0.0001 ****). Results of statistical analyses considered not significant are not shown (p > 0.05).
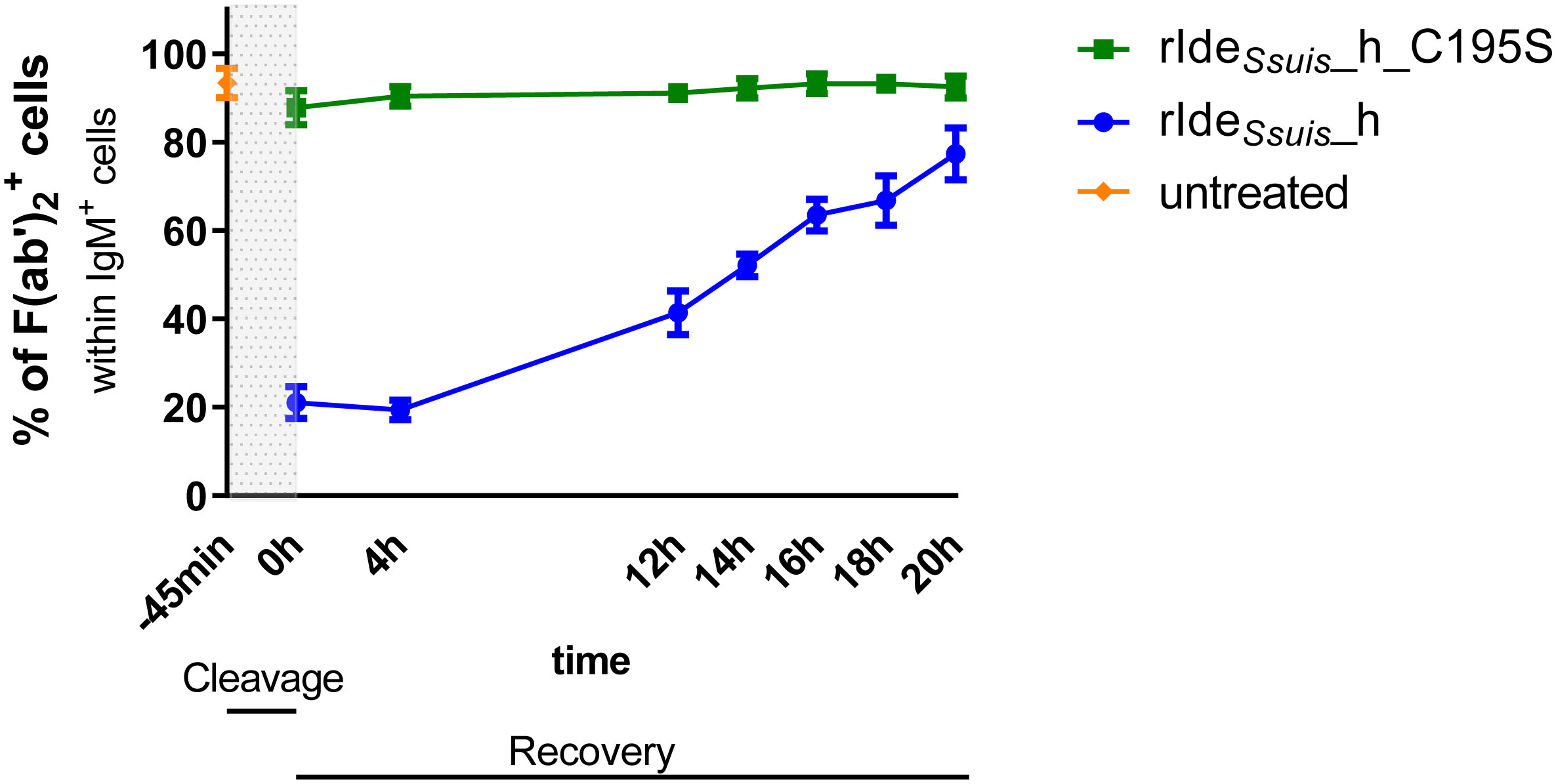
Figure 2 Recovery of IgM B cell receptor expression on porcine lymph node cells takes approximately 20 hours after rIdeSsuis_homologue mediated cleavage. Porcine mandibular lymph node cells of 11-month-old pigs (n = 5 for time points -45 min, 0 h, 4 h, 20 h; n = 3 for time points 12 h, 14 h, 16 h, 18 h) were incubated for 45 min with 4 µg/1x106 cells rIdeSsuis_homologue (rIdeSsuis_h) or non-functional rIdeSsuis_homologue_C195S (rIdeSsuis_h_C195S). After washing, cells were incubated for the given times in culture medium. Cells were stained and analyzed as described in Figure 1A.
For the preparation of peripheral blood mononuclear cells (PBMC) the blood was centrifuged at 400 x g for 10 min. Plasma was removed and the blood was diluted with the same amount of PBS. Density gradient centrifugation was performed over Ficoll (PAN Biotech, Aidenbach, Germany, catalogue P04-601000) at 900 x g for 35 min, followed by gently collecting the interphase with a pipette. Cells were washed with IMDM + 10% heat-inactivated FCS (500 x g, 12 min, RT) and again with PBS (400 x g, 10 min, RT). If necessary, erythrocyte lysis was performed by resuspending the cells in 1-2 mL lysis buffer (PBS supplemented with ammonium chloride, potassium hydrogen carbonate and ethylenediaminetetraacetic acid (EDTA)) and incubating for 2-3 min. Lysis was stopped by adding 1 mL heat-inactivated FCS and filling up with 10 mL IMDM + 10% FCS. After two washing steps (400 x g, 6 min, RT), cells were used directly or resuspended in heat-inactivated FCS supplemented with 10% dimethyl sulfoxide (DMSO, Sigma, Darmstadt, Germany, catalogue D2650-100ML) (freezing medium) and frozen at - 80°C.
Lymph nodes were minced and transferred into digestion medium consisting of IMDM supplemented with 1% penicillin/streptomycin, 50 µM gentamicin and DNase (final concentration 111.1 U/mL). After incubation for 15 min at 37°C, samples were homogenized using gentleMACS™ Dissociator according to manufacturer information. This step was repeated, followed by filtration through sterilized cotton wool and rinsing with PBS. Cells were washed three times with PBS at 400 x g and 4°C for 12 min and then resuspended in freezing medium and stored at -80°C.
BCR cleavage
Cells were thawed, counted with Trypan Blue and adjusted to 1 x 107 cells/mL in IMDM medium supplemented with 10% heat-inactivated FCS and 1% penicillin-streptomycin (PAN Biotech, Aidenbach, Germany, catalogue P06-07100) (IMDM++). Additionally, 10 ng/mL recombinant porcine IL-2 (R&D Systems, Minneapolis, US, catalogue 652-P2) was added to increase cell viability. Four million cells were incubated with 16 µg rIdeSsuis_homologue, 16 µg rIdeSsuis_homologue_C195S or 100 µl 24-fold concentrated culture supernatant from S. suis wt or 10ΔideSsuis∇ideSsuis_C195S, respectively, for 45 min, 2 h, 3 h, or 4 h at 37°C with 5% CO2. Then, cells were transferred into a 96 well plate with 4x105 cells/well on ice, washed two times with cold PBS and stained 20 min at 4°C in 100 µl 1:500 prediluted viability dye. Cells were washed one time with cold PBS supplemented with 3% heat-inactivated FCS and 0.1% sodium acid (FACS buffer) and two times with cold PBS, followed by fixing for 30 min at 4°C with 100 µl 2% paraformaldehyde (PFA) and three times washing with cold FACS buffer.
Next, cells were stained for 15 min at 4°C in 20 µl of staining mix 1 containing anti-IgM Fc and anti-IgM F(ab')2, F(ab')2 control mix containing anti-IgM Fc and anti-rabbit IgG isotype control or IgM Fc control mix containing mouse IgG1 isotype control and anti-IgM F(ab')2, respectively, diluted in FACS buffer. After two washing steps in FACS buffer, cells were blocked for 10 min at 4°C in 20 µl FACS buffer containing 30% porcine heat-inactivated serum (Fc block I). The cells were then stained in 20 µl of staining mix 2 containing anti-mouse IgG1 and anti-CD3 or CD3 control mix containing anti-mouse IgG1 and mouse IgG2a isotype control, respectively, diluted in Fc block I. Following 15 min incubation at 4°C, cells were washed once with FACS buffer and twice with FACS buffer containing 0.5% saponin (saponin buffer) to prepare for intracellular staining. After blocking with saponin buffer containing 30% porcine heat-inactivated serum (Fc block II) for 5 min at 4°C, cells were stained for 30 min at 4°C in 20 µl of staining mix 3 containing anti-CD79α diluted in Fc block II or CD79α fluorescence minus one (FMO) control mix without anti-CD79α. After washing once with saponin buffer and once with FACS buffer, cells were resuspended in 100 µl FACS buffer and measured by flow cytometry (LSRFortessa, BD Biosciences, Heidelberg, Germany). Analyses were carried out using FlowJo™ 10 software (BD Biosciences, Heidelberg, Germany) and gates were placed based on specific isotype controls. First, lymphocytes were gated forward scatter area (FSC-A) versus side scatter area (SSC-A) based on size and granularity. Next, single cells were gated forward scatter height (FSC-H) versus forward scatter area (FSC-A) to exclude doublets. Viable cells were distinguished using CD79α as B cell marker and CD3 as T cell marker in B cells (CD79α+CD3-) and T cells (CD79α-CD3+). IgM Fc+ CD79α+ B cells were analyzed for an intact (IgM Fc+ F(ab')2+) or cleaved (IgM Fc+ F(ab')2−) IgM B cell receptor.
For investigation of receptor cleavage on CD21+ or CD21- cells, staining mix 2 contained anti-mouse IgG1 and anti-CD21 in Fc block I and staining mix 3 contained Streptavidin in Fc block I. All other steps were performed as described above.
All antibodies used are specified in Supplementary Table 2. All washing steps were performed for 4 min at 400 x g or 500 x g after fixation, respectively.
B cell receptor recovery
Cells were thawed, counted and adjusted to 1 x 107 cells/mL in IMDM++ + poIL2 as described above. Four million cells were incubated with 16 µg rIdeSsuis_homologue or rIdeSsuis_homologue_C195S, respectively, for 45 min at 37°C with 5% CO2. After removal of the recombinant proteins by two washing steps with IMDM++, cells were incubated in IMDM++ supplemented with porcine IL-2 to promote cell survival for up to 20 hours. For each investigated time point, 4 x 105 cells/well were transferred into a 96 well plate onto ice and washed two times with cold PBS. All following steps were performed analogous to BCR cleavage experiments.
Incubation of myeloid cells and B cells with soluble IgM
PBMC were thawed, counted and adjusted to 1 x 107 cells/mL in IMDM++ + poIL2 as described above. Four million cells were incubated with 0.5 mg/mL IgM for 30 min at 37°C or remained untreated, respectively. After washing with IMDM++, cells were transferred into a 96 well plate with 4 x 105 cells/well and washed two times with cold PBS. Staining for viability and fixating with 2% PFA were performed as described above. Next, cells were stained for 15 min at 4°C in 20 µl of staining mix 1 containing anti-IgM Fc or IgM Fc control mix containing mouse IgG1 isotype control, respectively, diluted in FACS buffer. After two washing steps in FACS buffer, cells were blocked for 10 min at 4°C in 20 µl FACS buffer containing 30% porcine heat-inactivated serum (Fc block I). The cells were then stained in 20 µl of staining mix 2 containing anti-mouse IgG1 and anti-CD172a or CD172a control mix containing anti-mouse IgG1 and mouse IgG2b isotype control, respectively, diluted in Fc block I. Following 15 min incubation at 4°C, cells were washed once with FACS buffer and twice with saponin buffer. After blocking with saponin buffer containing 30% porcine heat-inactivated serum (Fc block II) for 5 min at 4°C, cells were stained for 30 min at 4°C in 20 µl of intracellular staining mix containing anti-CD79α diluted in Fc block II or CD79α FMO mix without anti-CD79α. All antibodies used are specified in Supplementary Table 2. Cells were measured by flow cytometry as described above.
B cell stimulation
We investigated the impact of BCR cleavage by rIdeSsuis on the stimulatory capacity of anti-IgM F(ab')2 and pervanadate. PBMC were used freshly isolated or thawed and adjusted to 1 x 107 cells/mL in IMDM++ + poIL2 as described above. Four million cells were incubated with 16 µg rIdeSsuis_homologue or rIdeSsuis_homologue_C195S, respectively, for 45 min at 37°C with 5% CO2. After two washing steps with cold PBS, cells were stained for 20 min at 4°C in prediluted viability dye with anti-IgM Fc or mouse IgG1 isotype control, respectively. Then, cells were washed two times with cold IMDM++ medium and resuspended in IMDM++ + poIL-2. Cells were seeded into a 96 well plate with a concentration of 4 x 105 cells/well and incubated for 20 min at 37°C.
To prepare pervanadate, 10 µl 0.1 M sodium orthovanadate (Sigma-Aldrich, Darmstadt, Germany, catalogue 450243-10G) was incubated with the same amount of 1:50 prediluted (0.6% final conc.) hydrogen peroxide (Carl Roth, Arlesheim, Switzerland, catalogue 8070.3) for 15 min at RT, followed by inactivation of surplus H2O2 by adding 30 µl catalase (Sigma-Aldrich, Darmstadt, Germany, catalogue C9322-1G) (20 mg/mL catalase in KH2PO4 buffer). The solution was centrifuged at 5000 x g for 5 min and the supernatant diluted 1:20 in buffer A (20 mM HEPES-NaOH, 120 mM NaCl, pH 7.4).
Cells were stimulated with anti-IgM F(ab')2 (10 µg/mL final concentration) for 1, 2, or 4 min, respectively. Stimulation with 10 µl pervanadate (0.1 mM final concentration) was performed for 5, 10, 15, or 20 min. All agents used for stimulation are specified in Supplementary Table 3. Stimulation was stopped all together by adding the same amount 4% PFA and incubation for 10 min at RT, followed by two washing steps with cold PBS and fixation with 2% PFA for 30 min at 4°C. After two times washing with cold FACS buffer, cells were stained for 15 min at 4°C in 20 µl of staining mix 1 containing anti-porcine IgG or the specific isotype control, diluted in FACS buffer. Next, cells were washed two times with cold FACS buffer, followed by 10 min incubation at 4°C in 20 µl Fc block I. Cells were then stained for 15 min at 4°C in 20 µl of staining mix 2 containing anti-mouse IgG1 and streptavidine, diluted in Fc block I. After two washing steps with cold PBS, cells were permeabilized with 100 µl ice-cold 99% methanol for 30 min on ice, followed by centrifugation, removal of supernatant and two times washing with cold FACS buffer. Next, cells were incubated in 20 µl FACS buffer with 30% murine serum (Fc block III) for 10 min at 4°C and then stained for 30 min at RT in 20 µl staining mix 3 containing anti-phospholipase C-γ2 or mouse IgG1 isotype control, respectively, diluted in Fc block III. After washing with FACS buffer twice, cells were incubated for 15 min at 4°C in 20 µl of staining mix 4 containing anti-CD21 or the respective isotype control, diluted in Fc block I. Cells were measured by flow cytometry as described above.
Cells for rIdeSsuis cleavage control as well as IgM Fc and F(ab')2 isotype controls were stained as described for BCR cleavage. All antibodies used for staining are specified in Supplementary Table 2. All washing steps were performed for 4 min at 400 x g or 500 x g after fixation, respectively.
Statistical analysis
All data represent at least three independent experiments. All statistical analyses were performed with GraphPad Prism 9 (Dotmatics, Boston, Massachusetts, US, www.graphpad.com). Distribution of data was tested both with Shapiro-Wilk and Kolmogorov-Smirnov test. If data achieve normal distribution, unpaired two-tailed t-test was applied. In case of no normal distribution, Mann-Whitney test was conducted. For comparison of more than two measurements, mixed analysis of variance (ANOVA) with subsequent Turkey´s multiple comparisons test was used. Figures show mean and standard deviation or median. Probabilities below 0.05 were considered significant (p< 0.05 *, p< 0.01 **, p< 0.001 ***, p< 0.0001 ****). Flow cytometric data was analyzed with FlowJo™ 10 software (BD Biosciences, Heidelberg, Germany, www.flowjo.com).
Results
Recombinant and S. suis derived IdeSsuis cleaves the porcine IgM B cell receptor
IdeSsuis cleaves soluble porcine IgM and leads to a reduction of porcine IgM bound to the bacterial surface (12, 16). The cysteine protease IdeS of Streptococcus pyogenes is known to cleave soluble IgG as well as the IgG B cell receptor (32). Thus, we hypothesized that IdeSsuis is also able to cleave porcine IgM as a transmembrane protein within the B cell receptor complex. This hypothesis was first investigated with the following recombinant derivatives of IdeSsuis: the intact IgM protease domain (rIdeSsuis_homologue), the point-mutated, inactive IgM protease domain (rIdeSsuis_homologue_C195S) or the intact IgM protease domain together with the large C-terminus of still unknown function (rIdeSsuis) (Supplementary Figure 1). Specifically, porcine mandibular lymph node cells and PBMCs were treated with these recombinant proteins and stained for IgM Fc+ B cells. Representative plots of flow cytometry analyses of mandibular lymph node cells are shown in Figure 1A. Viable lymphocytes were distinguished in B cells (CD79α+CD3-) and T cells (CD79α-CD3+). As IdeSsuis cleaves IgM between constant domain C2 and C3 (16), we chose a monoclonal anti-porcine IgM Fc antibody (K52 1C3), putatively recognizing the membrane bound C3-C4 IgM fragment after IdeSsuis treatment, to detect IgM+ B cells. Polyclonal rabbit anti-IgM F(ab')2- specific antibodies were used to detect the F(ab) part of IgM and therefore an intact IgM BCR (IgM Fc+ F(ab')2+). Lower panels of Figure 1A show representative pseudocolor plots of IgM+ B cells after treatment with the indicated recombinant proteins. We verified the cleavage activity of rIdeSsuis containing the large C-Terminus in a representative number of experiments and found no significant difference in IgM Fc+ F(ab')2+ B cells in comparison to the cleavage activity of rIdeSsuis_homologue (data not shown). Therefore, we continued the following experiments only using rIdeSsuis_homologue.
Indeed, flow cytometry analysis revealed a significant reduction of approximately sixty percent in IgM Fc+ F(ab')2+ cells after treatment with rIdeSsuis_homologue for 45 min, whereas non-functional rIdeSsuis_homologue_C195S had no significant effect on the number of IgM Fc+ F(ab')2+ cells (Figure 1B). After continuous incubation with rIdeSsuis_homologue for 4 h, the reduction in IgM Fc+ F(ab')2+ cells increased to over seventy percent. These findings for the 45 min incubation time period could be reproduced in PBMCs as well as in mLN cells of piglets of a different age (Supplementary Figure 2).
Taken together, these data indicate that rIdeSsuis_homologue as well as rIdeSsuis are able to cleave membrane-bound IgM and that cysteine at position 195 is crucial for this cleavage activity, analogous to cleavage of soluble IgM (16). The large C-terminal domain of IdeSsuis is dispensable for this cleavage activity on the IgM BCR. Both active recombinant variants of IdeSsuis efficiently remove the F(ab')2 part of the IgM BCR. The Fc part remained unaffected and was still detectable on B cells after up to 4 h treatment with rIdeSsuis_homologue (Supplementary Figure 3).
We further assessed whether IdeSsuis secreted by S. suis is able to cleave the IgM BCR. S. suis cps2 strain 10 (wt) and the isogenic S. suis mutant 10ΔideSsuis∇ideSsuis_C195S, which expresses a point-mutated, inactive full-length variant of IdeSsuis (16), were grown in IMDM medium. The supernatant was 24-fold concentrated via 100 kDa cutoff centrifugal filters to retain IdeSsuis or IdeSsuis_C195S (both 124 kDa) while eliminating the largest possible proportion of other secreted bacterial components, especially potential B cell damaging factors like suilysin. Cleavage of soluble IgM by those culture supernatants was confirmed in anti-IgM Western blot analyses under reducing conditions (Supplementary Figure 4).
Concentrated bacterial culture supernatants were incubated for 4 h with mandibular lymph node cells. Anti-IdeSsuis Western blot analysis revealed characteristic IdeSsuis protein bands between 175 and 270 kDa as described by Seele et al. (Supplementary Figure 5) (12), which suggests that IdeSsuis and IdeSsuis_C195S are fairly stable under these conditions. For comparison, recombinant homologue proteins were also incubated with mandibular lymph node cells and examined in parallel. Typical protein bands were detectable between 52 and 66 kDa as described by Rungelrath et al. (16).
Analogous to BCR cleavage with recombinant enzymes, mandibular lymph node cells were incubated with concentrated culture supernatant and analyzed in flow cytometry for an intact (IgM Fc+ F(ab')2+) or cleaved (IgM Fc+ F(ab')2−) IgM BCR (gating described in Figure 1A). A significant reduction of IgM Fc+ F(ab')2+ B cells was detectable after treatment with S. suis wt supernatant for 45 min and increased to approximately thirty percent when incubated for 4 h (Figure 1C). In contrast, there was no detectable effect on IgM Fc+ F(ab')2+ B cells after incubation with concentrated S. suis 10ΔideSsuis∇ideSsuis_C195S culture supernatant. These results demonstrate that not only rIdeSsuis_homologue (as shown above) but also IdeSsuis expressed by S. suis is able to cleave the porcine IgM BCR.
To verify that the measured IgM signals originate from the IgM BCR and not secreted and then surface-bound soluble IgM, i.e. bound to FcµR (18, 33, 34), we investigated B cell culture supernatants in anti-IgM Western blot analysis. Cells were treated with rIdeSsuis_homologue or rIdeSsuis_homologue_C195S, followed by removal of the recombinant proteins and cleavage products and incubation for 21 h. Cell culture supernatant was collected directly, 1 h and 21 h after washing and investigated for soluble IgM. Neither cells preincubated with rIdeSsuis_homologue nor rIdeSsuis_homologue_C195S showed IgM-specific protein bands at any time point (Supplementary Figure 6). These findings indicate that the cells do not produce soluble IgM that could bind to the cell surface. This is in accordance with the interpretation that the detected IgM F(ab')2 signals rely on the expression of the IgM BCR and not on receptor-bound secreted IgM. Additionally, we investigated the capacity of PBMCs to bind soluble IgM to further exclude that soluble and subsequently surface-bound IgM was contributing to the IgM signals measured by flow cytometry. To this end, porcine PBMC were incubated with 0.5 mg/mL soluble porcine IgM and analyzed for IgM Fc+ cells by flow cytometry. Cells positive for myeloid marker CD172a, known to express IgM receptors on the cell surface (18), were used as positive control. No significant effect on the percentage of IgM Fc+ porcine B cells (IgM Fc+ CD79α+) could be measured after IgM preincubation (Supplementary Figure 7). In contrast, CD172a+ cells showed a significant increase in the percentage of IgM Fc+ cells as well as in the median fluorescence intensity of the IgM Fc signal, indicating that porcine CD172a+ cells efficiently bind soluble IgM on the cell surface in contrast to B cells. This is also consistent with our interpretation that the IgM signal of B cells derives from transmembrane IgM as part of the IgM BCR.
In conclusion, these results show for the first time that rIdeSsuis_homologue cleaves the IgM BCR as present on the surface of B cells (CD79α+ CD3-) in porcine mandibular lymph node cells and PBMC.
Complete recovery of IgM B cell receptor expression on the surface of porcine lymph node cells takes approximately 20 hours after rIdeSsuis_homologue-mediated cleavage
After treatment of human PBMC with IgG-degrading enzyme IdeS of S. pyogenes, the number of IgG BCRs takes 16 h to return to pretreatment levels (32). To verify if the porcine IgM BCR is recovered in a similar time course, mandibular lymph node cells were incubated with rIdeSsuis_homologue or non-functional rIdeSsuis_homologue_C195S, followed by incubation for up to 20 h. Cells were stained for CD79α, IgM Fc and IgM F(ab')2 and analyzed in flow cytometry for intact or cleaved BCR as described above (Figure 1A). The number of IgM Fc+ F(ab')2+ B cells in mLN cells increased over time from approximately 20% at 0 h to almost 80% at 20 h after treatment with rIdeSsuis_homologue. After 20 h, numbers of IgM Fc+ F(ab')2+ cells were comparable between rIdeSsuis_homologue and rIdeSsuis_homologue_C195S treated cells (Figure 2). These findings demonstrate that the IgM BCR signal takes approximately 20 h to fully recover. The full recovery of the IgM signal on CD79α+CD3- B cells after rIdeSsuis_homologue cleavage further supports the interpretation that this signal is due to the porcine IgM BCR and not to surface-bound soluble IgM.
Treatment with rIdeSsuis_homologue specifically abolishes IgM B cell receptor mediated signaling
BCR signaling plays a key role in B cell activation and differentiation. BCR ligand-dependent activation results in the recruitment of protein tyrosine kinases Lyn and Syk and downstream tyrosine phosphorylation of phospholipase C-γ2 (PLC-γ2) (35).
We hypothesized that IgM BCR cleavage leads to impaired B cell activation. Therefore, we incubated PBMC with rIdeSsuis_homologue to cleave the BCR or non-functional rIdeSsuis_homologue_C195S for comparison as described above. Cleavage of the IgM BCR was verified by flow cytometry as described in Figure 1A. Next, the cells were treated with different stimuli and analyzed by flow cytometry for tyrosine-phosphorylated PLC-γ2 (pPLC-γ2) as readout parameter for activation of the intracellular downstream signal cascade. IgM BCR specific stimulation was achieved by anti-IgM F(ab')2. The gating strategy of IgM+ and IgG+ cells and representative histograms of IgM+ cells after 2 min stimulation with anti-IgM F(ab')2 in comparison to medium control are shown in Figure 3A. Protein tyrosine phosphatase inhibitor pervanadate intervenes at the level of downstream signal transduction (31) and was used for BCR-independent increase of phosphorylation.
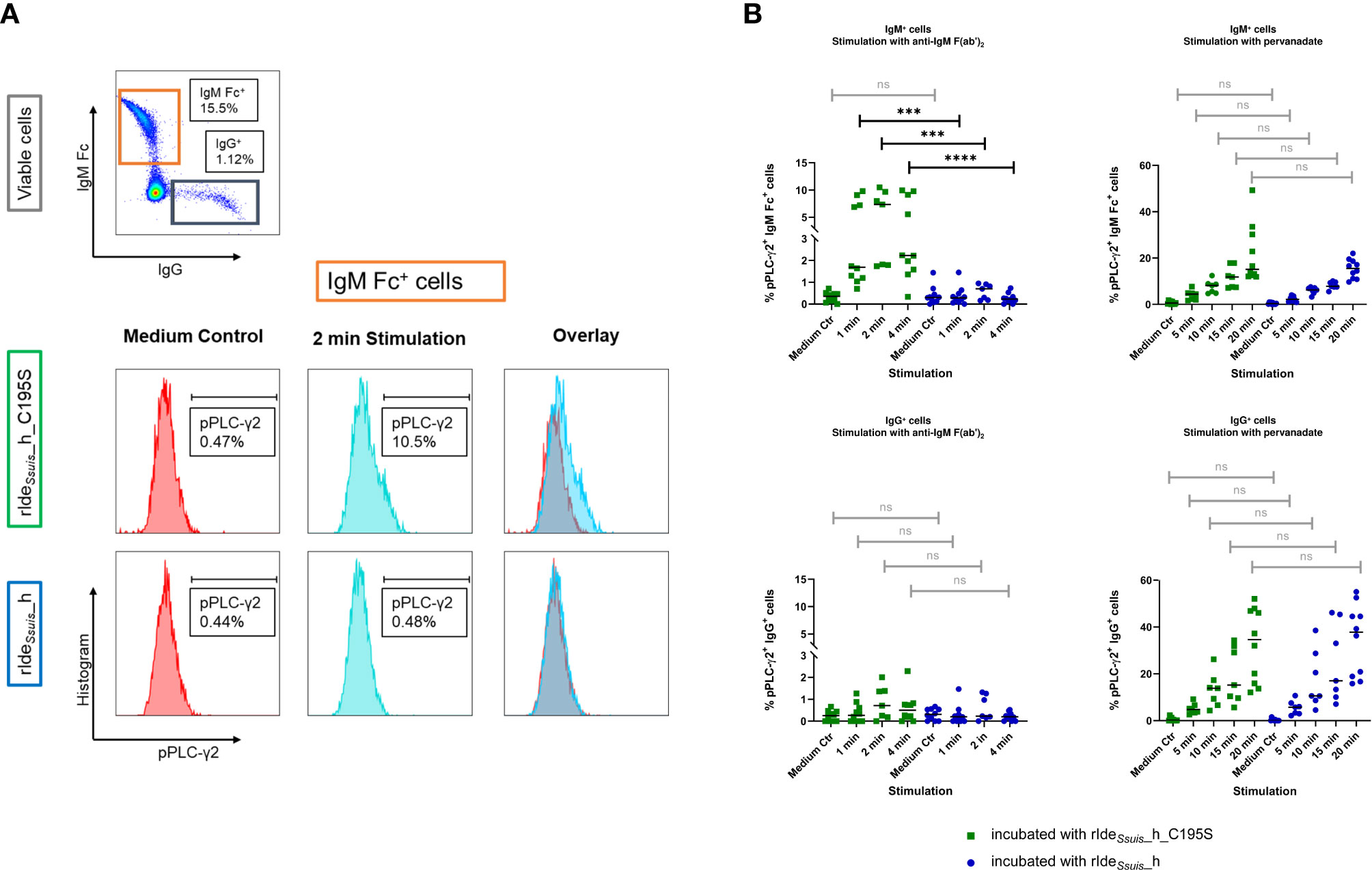
Figure 3 Treatment with rIdeSsuis_homologue abolishes IgM B cell receptor mediated signaling after IgM F(ab’)2-specific stimulation in IgM+, but not IgG+ B cells. (A) Gating strategy to analyze IgM+ and IgG+ cells for phosphorylated phospholipase C-γ2 (pPLC-γ2) after treatment with rIdeSsuis_homologue (rIdeSsuis_h) or non-functional point-mutated rIdeSsuis_homologue_C195S (rIdeSsuis_h_C195S). Representative histograms of IgM Fc+ cells are shown. Cells were kept in culture medium as control (lower panels, left hand side) or were stimulated with anti-IgM F(ab’)2 (lower panels, middle) and analyzed for the percentage of pPLC-γ2+ cells. (B) Stimulation with anti-IgM F(ab’)2 induces tyrosine phosphorylation of phospholipase C-γ2 (PLC-γ2) in IgM+, but not IgG+ lymphocytes. “After cleavage of the IgM BCR, there is no longer an increase in pPLC-γ2+ IgM+ cells detectable. Both cell types can be stimulated with pervanadate, independent of BCR cleavage. Porcine PBMC of 7 to 8-week-old piglets (n = 10) were incubated for 45 min with 4 µg/1x106 cells rIdeSsuis_homologue (rIdeSsuis_h) or rIdeSsuis_homologue_C195S (rIdeSsuis_h_C195S). After washing, cells were stimulated with anti-IgM F(ab’)2 or tyrosine phosphatase inhibitor pervanadate for the indicated times. Cells were stained and analyzed for IgM Fc or IgG and phosphorylated PLC-γ2+ by flow cytometry. Same time points were compared with Mann-Whitney test. Bars represent median, significant differences are indicated (p > 0.5 ns, p < 0.001 ***, p < 0.0001 ****).
We investigated the stimulatory capacity and the consequence of BCR cleavage by rIdeSsuis_homologue on the percentage of IgM Fc+ pPLC-γ2+ cells in PBMC of 7 to 8-week-old piglets and 16-week-old pigs. The cells of the different age groups behaved similarly (Supplementary Figure 8). Hence, we thereupon focused on PBMC from 7 to 8-week old piglets as the typical age period for S. suis disease.
Stimulated with anti-IgM F(ab')2, IgM Fc+ cells preincubated with rIdeSsuis_homologue_C195S showed a significant increase in the percentage of pPLC-γ2+ cells in comparison to IgM+ cells preincubated with rIdeSsuis_homologue (Figure 3B). These findings demonstrate that IgM BCR cleavage prevents recognition and ligand-dependent activation via the F(ab) part and therefore impairs intracellular signaling. As expected, IgG+ cells showed no increased phosphorylation of PLC-γ2 after stimulation with anti-IgM F(ab')2 as well as no effect of preincubation with functional or non-functional rIdeSsuis constructs. In contrast, the median fluorescence intensity (MFI) of pPLC-γ2+ did not increase after stimulation of IgM+ cells preincubated with rIdeSsuis_homologue_C195S in comparison to IgM+ cells preincubated with rIdeSsuis_homologue (Supplementary Figure 9). Taken together, these data indicate that IgM BCR-specific stimulation induces a higher frequency of pPLC-γ2+ IgM+ cells, while the level of phosphorylation per single cell remains restricted to a certain limit when all PTKs are already recruited.
When treated with pervanadate, both IgM+ and IgG+ cells showed a time-dependent increase in pPLC-γ2+ cells independent of enzyme preincubation (Figure 3B). This demonstrates that rIdeSsuis_homologue cleavage activity only affects the IgM BCR-mediated activation. Following 45 min preincubation with either rIdeSsuis_homologue or rIdeSsuis_homologue_C195S, the intracellular downstream signaling cascade appears to be still intact. In contrast to BCR-mediated stimulation, treatment with pervanadate led to a time-dependent increase in frequency as well as in MFI in both IgM+ and IgG+ cells (Supplementary Figure 9). However, IgM+ cells do not reach quite the same high levels of phosphorylation as IgG+ cells. In conclusion, pervanadate stimulation increased the number of pPLC-γ2+ cells as well as phosphorylation levels per cell in the investigated IgM+ and IgG+ cells with no difference between IgM+ cells with intact or cleaved BCR.
Additionally, after stimulation with anti-IgM F(ab')2 or pervanadate, cells were gated for IgM- cells and analyzed for the percentage of pPLC-γ2+ cells as described above. In addition to IgG+ B cells, the IgM- cell population also includes IgA+ and IgD+ B cells. As expected, IgM- cells showed no increase in pPLC-γ2+ cells after IgM-specific stimulation, whereas pPLC-γ2+ cells increased significantly after treatment with pervanadate, independent from pretreatment with rIdeSsuis_homologue or rIdeSsuis_homologue_C195S (Supplementary Figure 10). These results underline the specificity of polyclonal anti-IgM F(ab')2 used for stimulation of IgM+ B cells via the IgM F(ab) part of the BCR.
rIdeSsuis_homologue cleaves the IgM BCR and inhibits IgM B cell receptor mediated signaling on both CD21+ B2 cells and CD21- cells
To investigate IdeSsuis cleavage activity on different IgM-expressing B cell subpopulations, i.e. a CD21+ B2 population and a CD21- population, containing putative B1-like cells (36), PBMC were treated with rIdeSsuis_homologue or rIdeSsuis_homologue_C195S, stained for IgM Fc and CD21 and analyzed in flow cytometry. Both IgM+CD21+ and IgM+CD21- cells showed a significant reduction in IgM F(ab')2+ cells after incubation with rIdeSsuis_homologue in contrast to rIdeSsuis_homologue_C195S treated cells (Figure 4). In conclusion, rIdeSsuis_homologue cleaves the IgM BCR present on B1-like and B2 cell subsets differentiated by CD21 expression.
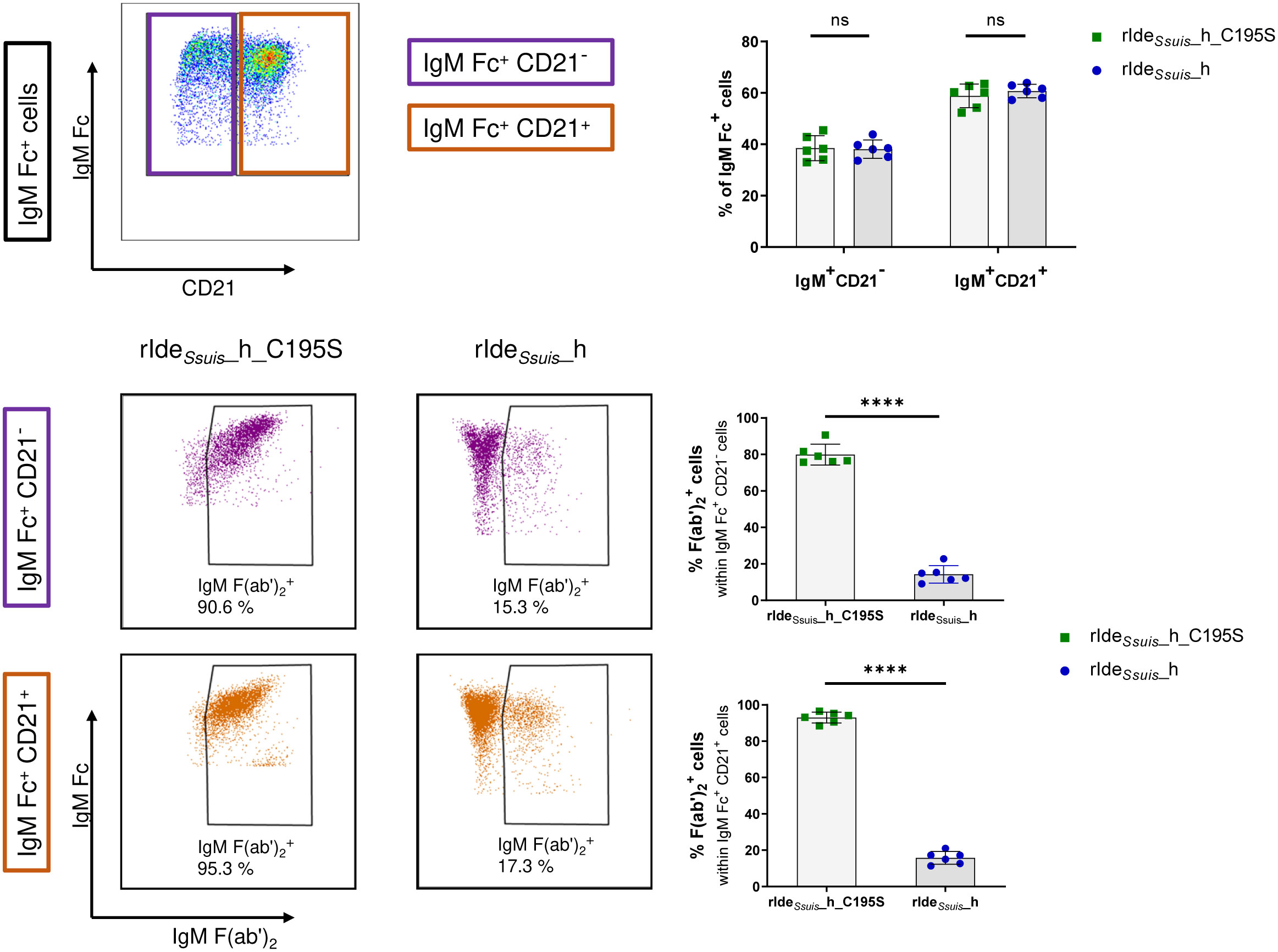
Figure 4 rIdeSsuis_homologue cleaves the IgM B cell receptor on CD21+ B2 cells and CD21- cells. IgM+ cells in porcine PBMCs of 8-week-old piglets can be discriminated by CD21 expression (upper panels). Both IgM+CD21- B1-like cells (middle panels) and IgM+CD21+ B2 cells (lower panels) show significant IgM BCR cleavage by rIdeSsuis_homologue (rIdeSsuis_h) (4 µg/1x106 cells, 45 min, 37°C) compared to rIdeSsuis_homologue_C195S (rIdeSsuis_h_C195S). Pre-gating of IgM Fc+ cells was performed as described in Figure 1A. Statistical analyses were conducted with unpaired t-test (n = 6, p > 0.05 ns, p < 0.0001 ****).
We further assessed the consequence of IgM BCR cleavage on the signaling of CD21+ B2 cells compared to CD21- B cells. Cells were pretreated with rIdeSsuis_homologue or rIdeSsuis_homologue_C195S before stimulation with specific anti-IgM F(ab')2 or protein tyrosine phosphatase inhibitor pervanadate (as described above). Stimulation of both CD21 populations within IgM+ cells with anti-IgM F(ab')2 after preincubation with rIdeSsuis_homologue_C195S resulted in a distinct increase in pPLC-γ2+ cells in contrast to IgM+ cells preincubated with rIdeSsuis_homologue (Figure 5). Significant differences were found comparing CD21+ and CD21- cells preincubated with non-functional rIdeSsuis_homologue_C195S after a stimulation time of 1 min and 2 min, with higher levels of pPLC-γ2+cells within CD21- cells. Phosphorylation of PLC-γ2 was completely abolished by preincubation with rIdeSsuis_homologue, with no significant differences between CD21+ and CD21- cells, indicating that both IgM+ cell subsets are equally impaired in downstream BCR signaling. After stimulation with pervanadate, both cell types showed an increase in pPLC-γ2+ cells independent from preincubation. Statistical analysis found higher percentages of pPLC-γ2+ cells within rIdeSsuis_homologue_C195S-preincubated CD21- cells after a stimulation period of 5 min as well as within rIdeSsuis_homologue-preincubated CD21- cells after a stimulation period of 5, 10, and 15 min in comparison to CD21+ cells. Taken together, significantly more CD21- cells were positive for pPLC-γ2 at specific time points. In summary, these data demonstrate IgM BCR cleavage by rIdeSsuis_homologue and subsequent impairment of intracellular signaling after ligand-dependent receptor activation on both naïve and innate B cell subsets.
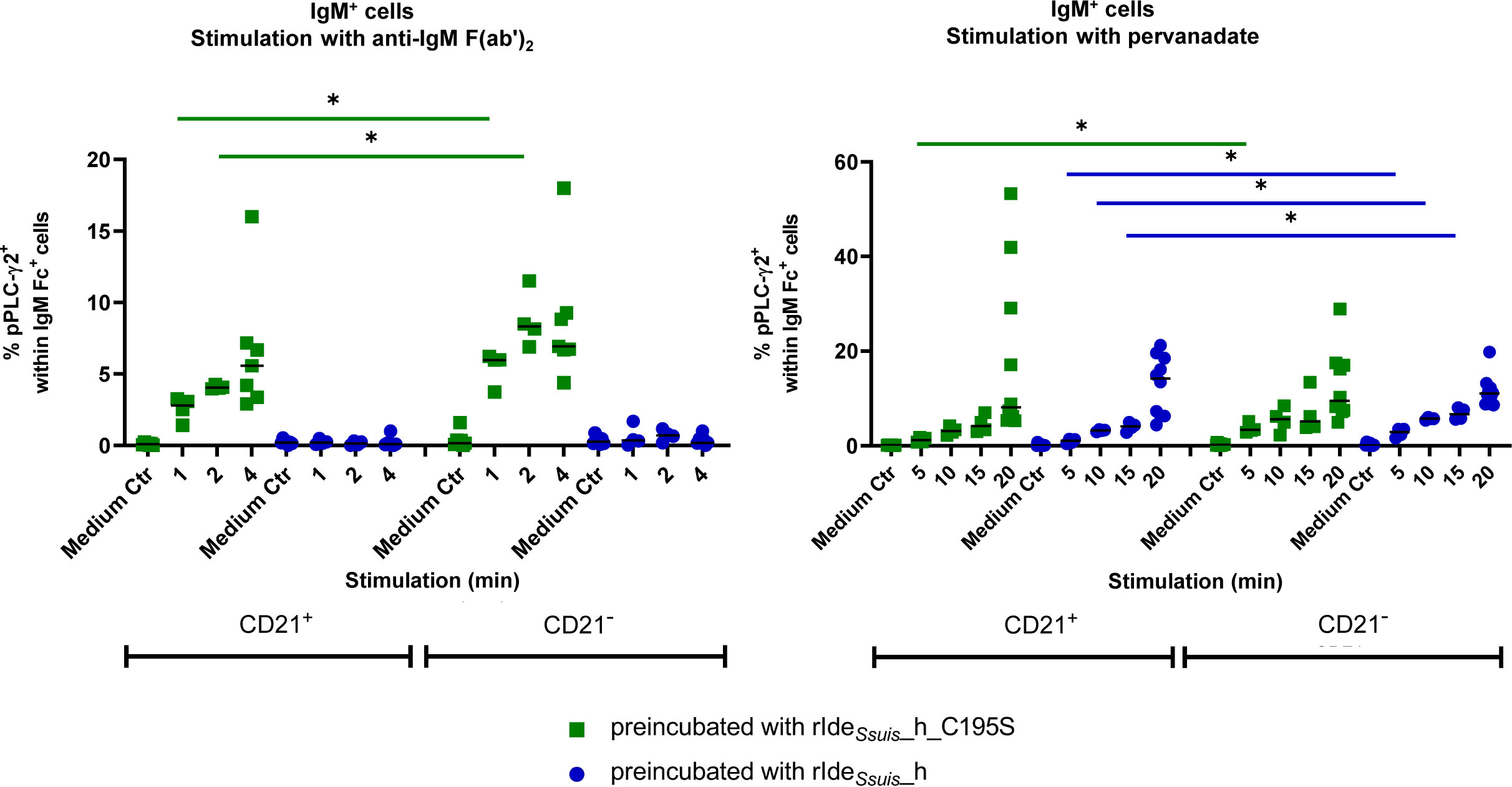
Figure 5 Treatment with rIdeSsuis_homologue abolishes IgM B cell receptor mediated signaling in CD21+ B2 cells and CD21- cells. Porcine PBMC of 7 to 8-week-old piglets (n = 4 - 8) were incubated for 45 min with 4 µg/1x106 cells rIdeSsuis_homologue (rIdeSsuis_h) or rIdeSsuis_homologue_C195S (rIdeSsuis_h_C195S). After washing, cells were stimulated with anti-IgM F(ab’)2 or tyrosine phosphatase inhibitor pervanadate for the indicated times or were kept in cell culture medium without stimulation (Medium Ctr.). Cells were stained and analyzed for IgM Fc, CD21 and phosphorylated PLC-γ2 by flow cytometry. Cells with the same treatment were compared with Mann-Whitney test. Bars represent median, significant differences are indicated (p< 0.1 *). Results of statistical analyses considered not significant are not shown (p > 0.05).
Discussion
Previous research demonstrated that the cysteine protease IdeSsuis specifically cleaves soluble porcine IgM and reduces complement activation (12, 16, 17). Whether IdeSsuis is also able to cleave transmembrane IgM within the BCR complex has not been investigated so far. We were able to show that the IgM BCR is efficiently cleaved by recombinant functional IdeSsuis derivatives as well as by IdeSsuis secreted in culture supernatant (Figure 1).
IgM BCR recovery on the cell surface after cleavage by rIdeSsuis_homologue was investigated over a time period of 21 h. To ensure returning IgM F(ab')2 signals derive from transmembrane IgM within the BCR complex and not secreted and subsequently surface-bound IgM, we investigated soluble IgM in cell culture supernatants after treatment with rIdeSsuis_homologue. Even after an incubation time of 21 h, there was still no soluble IgM detectable, which could potentially bind to the B cell surface via IgM Fc receptors and account for the return of measured IgM F(ab')2 signals (Supplementary Figure 6). This finding is consistent with the study of Braun et al. reporting IgM secretion into culture supernatant only after stimulation, but not spontaneously (36). In addition, we found no increased IgM signal on the surface of porcine B cells after incubation with purified soluble IgM (Supplementary Figure 7). Taken together, our data indicate recovery through expression of new BCRs rather than secretion of soluble IgM and recruitment to the cell surface by Fc receptors (18). Interestingly, after rIdeSsuis_homologue cleavage, recovery of the IgM BCR on the cell surface takes at least 20 h in mandibular lymph node cells (Figure 2). These findings record similarities to the recovery period of B cells after treatment with IdeS of Streptococcus pyogenes, with studies recording that IgG F(ab)+ peripheral blood B cells take 16 h to return to pretreatment levels (32). In comparison, recovery of the IgM BCR takes even longer. The comparatively long recovery period suggests potential biological effects in terms of interfering with the hosts immune response.
IgM BCR cleavage by rIdeSsuis_homologue prevents downstream signaling after F(ab')2-specific receptor stimulation, thus impairing IgM B cell activation following antigen binding (Figure 3B). Similarly, treatment of human B cell lymphoma cells with IdeS inhibits IgG BCR signaling after stimulation via the F(ab) part (32). As IgM is the main BCR on B1 cells and naïve conventional B2 cells (37), IgM BCR cleavage could interfere with the innate immune response as well as the first rapid IgM response in the context of adaptive immunity. On the other hand, the intracellular pathways remain uninfluenced by BCR cleavage. This could be shown by using the tyrosine phosphatase inhibitor pervanadate, where no differences in phosphorylation of PLC-γ2 between B cells with rIdeSsuis_homologue-mediated BCR cleavage and treatment with rIdeSsuis_homologue_C195S were found. Pervanadate intracellularly inhibits PTPs, thereby shifting the balance towards activating tyrosine kinases (29). This demonstrates that IdeSsuis is able to block B cell activation specifically via the BCR.
Mature naïve B cells exit the bone marrow and migrate to secondary lymphoid organs like lymph nodes where they encounter antigen (38, 39). Antigen binding to the BCR of naïve B cells initiates intracellular downstream signaling, antigen internalization and presenting of processed antigen on MHC II molecules (37). As BCR signals and recognition of the antigen-MHC II complex by specific T helper cells lead to proliferation, class switch and differentiation into antibody-secreting or memory B cells, we speculate that infection of lymph nodes with S. suis might lead to impaired B cell differentiation if enough IdeSsuis is expressed to efficiently cleave the IgM BCR. As encapsulated S. suis is also known to modulate MHC-II restricted antigen presentation and cytokine secretion of dendritic cells, leading to an impaired CD4+ T cell activation (40, 41), this important invasive pathogen might substantially interfere with the adaptive immune response of its porcine host. We speculate that this interference with the adaptive immune response contributes to survival of an invasive S. suis strain in the tonsils of convalescent pigs (42).
In B cell development, antigen-independent BCR signaling is important for maintaining tonic signals, which are required for B cell differentiation, maturation and survival at different key checkpoints during development (29, 35). The permanent loss of BCRs on peripheral immature and mature B cells results in cell death (43). It is conceivable that persistent infection of lymph nodes with S. suis, expressing high enough IdeSsuis levels to efficiently cleave the IgM BCR, leads to an impaired germinal center response. This might interfere with the development into long-living plasma cells, memory cells or even result in B cell anergy or death.
Karlsson et al. compared samples from human patients locally or systemically infected with Streptococcus pyogenes to healthy controls and found a specific change in the proteome of the tonsillitis patients (44). The tonsillitis swaps contained much higher levels of IdeS-specific IgG cleavage products compared to plasma from sepsis patients, indicating higher IdeS cleavage efficacy in local infection sites with low IgG levels. Similarly, mucosal surfaces in humans (45) and pigs are characterized by high IgA, but only low IgM levels in comparison to the blood (46). As mucosal surfaces of the upper respiratory tract are the natural environment for S. suis and represent an IgM-low microenvironment, we hypothesize an advantage in colonization and survival for S. suis by efficient IgM cleavage by IdeSsuis on those surfaces.
S. pyogenes is commonly found in the human pharynx, residing as a commensal or causing local infections. Similarly, S. suis frequently colonizes the upper respiratory tract of pigs. Madsen et al. described a model for aerogenous infection, in which pigs were exposed to aerosolized acetic acid followed by aerosolized S. suis (47). After challenge with S. suis serotype 2, bacterial antigen was detectable in the crypts and subepithelial tissue of the tonsils (48). This location is compatible with bacterial colonization and subsequent invasion across the epithelium. Some infected pigs developed clinical signs, with S. suis detectable also in the tracheobronchial lymph node, the mandibular lymph node and other inner organs. These findings led the authors to assume invasion via the tonsils and lymphogenous spread to the regional lymph nodes, but invasion via the lower respiratory tract could not be ruled out completely (49). However, regional lymph nodes seem to be a key point in the process of colonization progressing to invasive disease. As for S. suis, draining lymph nodes of colonized mucosal surfaces, like mandibular or upper cervical lymph nodes, are important sites of encounter with the hosts immune system. Accordingly, we studied the cleavage of the IgM BCR on lymph node cells which are part of the early interaction with the pathogen during local infection and on PBMC which get involved later in infection during bacteremia. Palatine tonsils are an important reservoir for invasive S. suis strains and are discussed as a potential route of entry (49, 50). B cells are naturally present in both tonsils and lymph nodes, so modification of the IgM BCR signaling by secreted IdeSsuis would not only be conceivable but also highly biologically relevant.
Of note, this study demonstrates IgM BCR cleavage on different B cell populations in vitro but it remains to be shown that the IgM BCR is cleaved during infection in vivo and that this is of biological relevance. As S. suis is known to bind efficiently to different porcine immune cells present in porcine blood (51), we consider it likely that IgM BCR cleavage occurs in vivo.
The porcine IgM+CD21+ B cell subset is described to consist mostly of naïve B2 cells, whereas IgM+CD21- B cells represent innate immune cells with B1-like characteristics (36). In this study, we found antigen-mediated BCR signaling in IgM+CD21+ and IgM+CD21- cells to be equally impaired by rIdeSsuis_homologue receptor cleavage (Figure 5).
B1 cells produce IgM as natural antibodies independent from infection and are accountable for most of the circulating IgM, but can also actively respond to T cell-independent antigenic stimulation, especially to encapsulated bacteria (37). B1 cells are mobilized in response to pathogens and migrate to secondary lymphoid organs like lymph nodes, where they secrete IgM antibodies (52). Thus, B1 cells represent the first line of defense in bacterial infection and link innate and adaptive immunity. This underlines the potential important role of IgM in S. suis pathogenesis and the putative advantage of IdeSsuis expression for colonization, when S. suis encounters the host`s innate immune response.
Additionally, IdeSsuis has been demonstrated to be a protective antigen against mortality induced by experimental infection with a S. suis cps2 or a cps9 strain (53, 54). There might be different reasons for the high protective efficacy. As shown by Seele et al., vaccination with rIdeSsuis induces antibodies neutralizing the IgM protease activity (53), which might reduce complement evasion and modification of B cell function as indicated in this paper.
Pathogens have developed a multitude of mechanisms to interfere with B cell functions like presentation of antigens, cell survival or polyclonal B cell activation. Typical examples are Epstein-Barr virus, cytomegalovirus, smallpox virus or Brucella, but also parasites like Trypanosoma ssp. or Plasmodium falciparum (52, 55–57). The modulation of B cells by interfering with BCR signaling seems to be a less common strategy of pathogens. Epstein-Barr Virus induces tyrosine phosphorylation and activation of PLC-γ2 by expression of the latent membrane protein 2A (LMP2A) and therefore mimics BCR signaling events (58). Yersinia pseudotuberculosis expresses tyrosine phosphatase YopH, which blocks the tyrosine phosphorylation cascade in early BCR signaling, leaving B cells unable to upregulate surface costimulatory molecule B7.2 in response to antigenic stimulation (59). However, cleavage of the BCR is only described for the IgG protease IdeS of Streptococcus pyogenes (32). This current study demonstrates that the IgM protease IdeSsuis of S. suis is able to cleave the IgM BCR, resulting in impaired IgM B cell signaling after receptor stimulation. We are not aware of any other host-pathogen interaction directly targeting the IgM BCR despite its crucial role in B cell function. The IdeSsuis gene is highly conserved in S. suis (https://blast.ncbi.nlm.nih.gov/Blast.cgi) and IdeSsuis is expressed by S. suis of different serotypes and sequence types (12). Therefore, we postulate that IdeSsuis plays an important role in persistent local S. suis infection and colonization by modulating the pig’s B cell dependent immune response. However, this hypothesis still needs further in vivo investigations.
Data availability statement
The raw data supporting the conclusions of this article will be made available by the authors, without undue reservation.
Ethics statement
All animal experiments were conducted by veterinarians and in accordance with the principles outlined in the European Convention for the Protection of Vertebrate Animals Used for Experimental and other Scientific Purposes and the German Animal Protection Law (Tierschutzgesetz). All animal experiments (collection of blood and lymph nodes) were either approved by the “Landesdirektion Sachsen” (permit number A09/19 and 25-5131/490/5) or the “Niedersächsisches Landesamt für Verbraucherschutz und Lebensmittelsicherheit” (permit number 25-5131/470/13), which includes review through the registered ethics committees for animal experiments of the two institutions.
Author contributions
Supervision, resources, project administration: CB, GA, UM. Methodology, Investigation, Software, Data analysis: AB, NS, UM. Important preliminary experiments: VR. Production of central experimental tools: WS. Visualization: AB. Writing – first draft: AB. Writing – review: CB, GA, NS, UM, VR. All authors contributed to the article and approved the submitted version.
Funding
This work was funded by the German Research Foundation (Deutsche Forschungsgemeinschaft) grants BA 4730/3-2 and AL 371/10-2. The authors acknowledge the support of the University of Leipzig (open access publication fund).
Acknowledgments
We thank Hilde Smith (Wageningen University and Research, Lelystad, Netherlands) for providing S. suis strain 10. Ingrid Vervuert and Lisa Wahl (University of Leipzig, Faculty of Veterinary Medicine, Germany) kindly provided mandibular lymph nodes of saddle back pigs. Flow cytometry was conducted at the core unit for flow cytometry (CUDZ) of the Faculty of Veterinary Medicine, University of Leipzig.
Conflict of interest
The authors declare that the research was conducted in the absence of any commercial or financial relationships that could be construed as a potential conflict of interest.
Publisher’s note
All claims expressed in this article are solely those of the authors and do not necessarily represent those of their affiliated organizations, or those of the publisher, the editors and the reviewers. Any product that may be evaluated in this article, or claim that may be made by its manufacturer, is not guaranteed or endorsed by the publisher.
Supplementary material
The Supplementary Material for this article can be found online at: https://www.frontiersin.org/articles/10.3389/fimmu.2023.1122808/full#supplementary-material
Abbreviations
BCR, B cell receptor; FCS, fetal calf serum; FMO, fluorescence minus one control; IdeE, Immunoglobulin G-degrading enzyme of S. equi subsp. equi; IdeS, Immunoglobulin G-degrading enzyme of Streptococcus pyogenes; IdeSsuis, Immunoglobulin M-degrading enzyme of Streptococcus suis; IdeZ, Immunoglobulin G-degrading enzyme of S. equi subsp. zooepidemicus; Ig, Immunoglobulin; IMDM, Iscove′s Modified Dulbecco′s Medium; MFI, median fluorescence intensity; mLN, mandibular lymph node; PBMC, peripheral mononuclear blood cells; PBS, phosphate buffered saline; PLC-γ2, phospholipase C-γ2; PTP, protein tyrosine phosphatase; r, recombinant; RT, room temperature; S. suis, Streptococcus suis; wt, wild type.
References
1. Gottschalk M, Segura M. Streptococcosis. In: Zimmerman JJ, editor. Diseases of swine, 11th edition. Hoboken, NJ: Wiley-Blackwell (2019). p. 934–50.
2. Baele M, Chiers K, Devriese LA, Smith HE, Wisselink HJ, Vaneechoutte M, et al. The gram-positive tonsillar and nasal flora of piglets before and after weaning. J Appl Microbiol (2001) 91(6):997–1003. doi: 10.1046/j.1365-2672.2001.01463.x
3. Wertheim HFL, Nghia HDT, Taylor W, Schultsz C. Streptococcus suis: an emerging human pathogen. Clin Infect Dis (2009) 48(5):617–25. doi: 10.1086/596763
4. Okura M, Osaki M, Nomoto R, Arai S, Osawa R, Sekizaki T, et al. Current taxonomical situation of streptococcus suis. Pathogens (2016) 5(3). doi: 10.3390/pathogens5030045
5. Goyette-Desjardins G, Auger J-P, Xu J, Segura M, Gottschalk M. Streptococcus suis, an important pig pathogen and emerging zoonotic agent-an update on the worldwide distribution based on serotyping and sequence typing. Emerg Microbes Infect (2014) 3(6):e45. doi: 10.1038/emi.2014.45
6. Chuzeville S, Auger J-P, Dumesnil A, Roy D, Lacouture S, Fittipaldi N, et al. Serotype-specific role of antigen I/II in the initial steps of the pathogenesis of the infection caused by streptococcus suis. Vet Res (2017) 48(1):39. doi: 10.1186/s13567-017-0443-4
7. Zhang C, Hao H, Yu Y, Kong D, Chen S, Jiang H, et al. Structural basis of the interaction between the meningitis pathogen streptococcus suis adhesin fhb and its human receptor. FEBS Lett (2016) 590(9):1384–92. doi: 10.1002/1873-3468.12174
8. Roy D, Grenier D, Segura M, Mathieu-Denoncourt A, Gottschalk M. Recruitment of factor h to the streptococcus suis cell surface is multifactorial. Pathogens (2016) 5(3). doi: 10.3390/pathogens5030047
9. Vötsch D, Willenborg M, Weldearegay YB, Valentin-Weigand P. Streptococcus suis - the "Two faces" of a pathobiont in the porcine respiratory tract. Front Microbiol (2018) 9:480. doi: 10.3389/fmicb.2018.00480
10. Tanabe S-I, Bonifait L, Fittipaldi N, Grignon L, Gottschalk M, Grenier D. Pleiotropic effects of polysaccharide capsule loss on selected biological properties of streptococcus suis. Can J Vet Res (2009) 73):65–70.
11. Segura M, Gottschalk M, Olivier M. Encapsulated streptococcus suis inhibits activation of signaling pathways involved in phagocytosis. Infect Immun (2004) 72(9):5322–30. doi: 10.1128/IAI.72.9.5322-5330.2004
12. Seele J, Singpiel A, Spoerry C, von Pawel-Rammingen U, Valentin-Weigand P, Baums CG. Identification of a novel host-specific IgM protease in streptococcus suis. J Bacteriol (2013) 195(5):930–40. doi: 10.1128/JB.01875-12
13. Lannergård J, Guss B. IdeE, an IgG-endopeptidase of streptococcus equi ssp. equi. FEMS Microbiol Lett (2006) 262(2):230–5. doi: 10.1111/j.1574-6968.2006.00404.x
14. von Pawel-Rammingen U, Johansson BP, Björck L. IdeS, a novel streptococcal cysteine proteinase with unique specificity for immunoglobulin G. EMBO J (2002) 21(7):1607–15. doi: 10.1093/emboj/21.7.1607
15. Hulting G, Flock M, Frykberg L, Lannergård J, Flock J-I, Guss B. Two novel IgG endopeptidases of streptococcus equi. FEMS Microbiol Lett (2009) 298(1):44–50. doi: 10.1111/j.1574-6968.2009.01698.x
16. Rungelrath V, Weiße C, Schütze N, Müller U, Meurer M, Rohde M, et al. IgM cleavage by streptococcus suis reduces IgM bound to the bacterial surface and is a novel complement evasion mechanism. Virulence (2018) 9(1):1314–37. doi: 10.1080/21505594.2018.1496778
17. Seele J, Beineke A, Hillermann L-M, Jaschok-Kentner B, von Pawel-Rammingen U, Valentin-Weigand P, et al. The immunoglobulin M-degrading enzyme of streptococcus suis, IdeSsuis, is involved in complement evasion. Vet Res (2015) 46:45. doi: 10.1186/s13567-015-0171-6
18. Klimovich VB. IgM and its receptors: structural and functional aspects. Biochem (Mosc) (2011) 76(5):534–49. doi: 10.1134/S0006297911050038
19. Baumgarth N. The double life of a b-1 cell: self-reactivity selects for protective effector functions. Nat Rev Immunol (2011) 11(1):34–46. doi: 10.1038/nri2901
20. Rieckmann K, Seydel A, Szewczyk K, Klimke K, Rungelrath V, Baums CG. Streptococcus suis cps7: an emerging virulent sequence type (ST29) shows a distinct, IgM-determined pattern of bacterial survival in blood of piglets during the early adaptive immune response after weaning. Vet Res (2018) 49(1):48. doi: 10.1186/s13567-018-0544-8
21. Martínez-Boixaderas N, Garza-Moreno L, Sibila M, Segalés J. Impact of maternally derived immunity on immune responses elicited by piglet early vaccination against the most common pathogens involved in porcine respiratory disease complex. Porcine Health Manag (2022) 8(1):11. doi: 10.1186/s40813-022-00252-3
22. Nielsen CH, Fischer EM, Leslie RG. The role of complement in the acquired immune response. Immunology (2000) 100(1):4–12. doi: 10.1046/j.1365-2567.2000.00009.x
23. Sun J, Butler JE. Sequence analysis of pig switch mu, c mu, and c mu m. Immunogenetics (1997) 46(6):452–60. doi: 10.1007/s002510050305
24. Butler JE, Wertz N, Sinkora M. Antibody repertoire development in swine. Annu Rev Anim Biosci (2017) 5:255–79. doi: 10.1146/annurev-animal-022516-022818
25. Butler JE, Wertz N. The porcine antibody repertoire: variations on the textbook theme. Front Immunol (2012) 3:153. doi: 10.3389/fimmu.2012.00153
26. Treanor B. B-cell receptor: from resting state to activate. Immunology (2012) 136(1):21–7. doi: 10.1111/j.1365-2567.2012.03564.x
27. Butler JE, Lager KM, Splichal I, Francis D, Kacskovics I, Sinkora M, et al. The piglet as a model for b cell and immune system development. Vet Immunol Immunopathol (2009) 128(1-3):147–70. doi: 10.1016/j.vetimm.2008.10.321
28. Skrzypczynska KM, Zhu JW, Weiss A. Positive regulation of Lyn kinase by CD148 is required for b cell receptor signaling in B1 but not B2 b cells. Immunity (2016) 45(6):1232–44. doi: 10.1016/j.immuni.2016.10.013
29. Monroe JG. ITAM-mediated tonic signalling through pre-BCR and BCR complexes. Nat Rev Immunol (2006) 6(4):283–94. doi: 10.1038/nri1808
30. Liu W, Tolar P, Song W, Kim TJ. Editorial: BCR signaling and b cell activation. Front Immunol (2020) 11:45. doi: 10.3389/fimmu.2020.00045
31. Keren Z, Diamant E, Ostrovsky O, Bengal E, Melamed D. Modification of ligand-independent b cell receptor tonic signals activates receptor editing in immature b lymphocytes. J Biol Chem (2004) 279(14):13418–24. doi: 10.1074/jbc.M311970200
32. Järnum S, Bockermann R, Runström A, Winstedt L, Kjellman C. The bacterial enzyme IdeS cleaves the IgG-type of b cell receptor (BCR), abolishes BCR-mediated cell signaling, and inhibits memory b cell activation. J Immunol (2015) 195(12):5592–601. doi: 10.4049/jimmunol.1501929
33. Kubagawa H, Oka S, Kubagawa Y, Torii I, Takayama E, Kang D-W, et al. Identity of the elusive IgM fc receptor (FcmuR) in humans. J Exp Med (2009) 206(12):2779–93. doi: 10.1084/jem.20091107
34. Kubagawa H, Oka S, Kubagawa Y, Torii I, Takayama E, Kang D-W, et al. The long elusive IgM fc receptor, FcμR. J Clin Immunol (2014) 34(Suppl 1):S35–45. doi: 10.1007/s10875-014-0022-7
35. Niiro H, Clark EA. Regulation of b-cell fate by antigen-receptor signals. Nat Rev Immunol (2002) 2(12):945–56. doi: 10.1038/nri955
36. Braun RO, Python S, Summerfield A. Porcine b cell subset responses to toll-like receptor ligands. Front Immunol (2017) 8:1044. doi: 10.3389/fimmu.2017.01044
37. Murphy K, Weaver C. "Development of B lymphocytes". In: Janeway's immunobiology. 9th Edition. Garland Science (2017) 296–314.
38. LeBien TW, Tedder TF. B lymphocytes: how they develop and function. Blood (2008) 112(5):1570–80. doi: 10.1182/blood-2008-02-078071
39. Sinkora M, Sinkorova J. B cell lymphogenesis in swine is located in the bone marrow. J Immunol (2014) 193(10):5023–32. doi: 10.4049/jimmunol.1401152
40. Lecours M-P, Letendre C, Clarke D, Lemire P, Galbas T, Benoit-Biancamano M-O, et al. Immune-responsiveness of CD4+ T cells during streptococcus suis serotype 2 infection. Sci Rep (2016) 6:38061. doi: 10.1038/srep38061
41. Letendre C, Auger J-P, Lemire P, Galbas T, Gottschalk M, Thibodeau J, et al. Streptococcus suis serotype 2 infection impairs interleukin-12 production and the MHC-II-Restricted antigen presentation capacity of dendritic cells. Front Immunol (2018) 9:1199. doi: 10.3389/fimmu.2018.01199
42. Baums CG, Kock C, Beineke A, Bennecke K, Goethe R, Schröder C, et al. Streptococcus suis bacterin and subunit vaccine immunogenicities and protective efficacies against serotypes 2 and 9. Clin Vaccine Immunol (2009) 16(2):200–8. doi: 10.1128/CVI.00371-08
43. Kraus M, Alimzhanov MB, Rajewsky N, Rajewsky K. Survival of resting mature b lymphocytes depends on BCR signaling via the igalpha/beta heterodimer. Cell (2004) 117(6):787–800. doi: 10.1016/j.cell.2004.05.014
44. Karlsson CAQ, Järnum S, Winstedt L, Kjellman C, Björck L, Linder A, et al. Streptococcus pyogenes infection and the human proteome with a special focus on the immunoglobulin G-cleaving enzyme IdeS. Mol Cell Proteomics (2018) 17(6):1097–111. doi: 10.1074/mcp.RA117.000525
45. Brandtzaeg P, Farstad IN, Johansen FE, Morton HC, Norderhaug IN, Yamanaka T. The b-cell system of human mucosae and exocrine glands. Immunol Rev (1999) 171:45–87. doi: 10.1111/j.1600-065X.1999.tb01342.x
46. Bourne FJ. The immunoglobulin system of the suckling pig. Proc Nutr Soc (1973) 32(3):205–15. doi: 10.1079/PNS19730041
47. Madsen LW. Experimental infection of conventional pigs with streptococcus suis serotype 2 by aerosolic exposure. Acta Vet Scand (2001) 42:303–6. doi: 10.1186/1751-0147-42-303
48. Madsen LW, Bak H, Nielsen B, Jensen HE, Aalbaek B, Riising HJ. Bacterial colonization and invasion in pigs experimentally exposed to streptococcus suis serotype 2 in aerosol. J Vet Med B Infect Dis Vet Public Health (2002) 49(5):211–5. doi: 10.1046/j.1439-0450.2002.00491.x
49. Segura M, Calzas C, Grenier D, Gottschalk M. Initial steps of the pathogenesis of the infection caused by streptococcus suis: fighting against nonspecific defenses. FEBS Lett (2016) 590(21):3772–99. doi: 10.1002/1873-3468.12364
50. Wilson SM, Norton P, Haverson K, Leigh J, Bailey M. Interactions between streptococcus suis serotype 2 and cells of the myeloid lineage in the palatine tonsil of the pig. Vet Immunol Immunopathol (2007) 117(1-2):116–23. doi: 10.1016/j.vetimm.2007.02.009
51. Öhlmann S, Krieger A-K, Gisch N, Meurer M, de Buhr N, von Köckritz-Blickwede M, et al. D-alanylation of lipoteichoic acids in streptococcus suis reduces association with leukocytes in porcine blood. Front Microbiol (2022) 13:822369. doi: 10.3389/fmicb.2022.822369
52. Castañeda-Sánchez JI, Duarte ARM, Domínguez-López ML, de La Cruz-López JJ, Luna-Herrera J. B lymphocyte as a target of bacterial infections. In: Isvoranu G, editor. Lymphocyte updates - cancer, autoimmunity and infection. InTech IntechOpen (2017) 149–82.
53. Seele J, Hillermann L-M, Beineke A, Seitz M, von Pawel-Rammingen U, Valentin-Weigand P, et al. The immunoglobulin m-degrading enzyme of streptococcus suis, IdeSsuis, is a highly protective antigen against serotype 2. Vaccine (2015) 33(19):2207–12. doi: 10.1016/j.vaccine.2015.03.047
54. Rieckmann K, Seydel A, Klose K, Alber G, Baums CG, Schütze N. Vaccination with the immunoglobulin m-degrading enzyme of streptococcus suis, ide ssuis, leads to protection against a highly virulent serotype 9 strain. Vaccine X (2019) 3:100046. doi: 10.1016/j.jvacx.2019.100046
55. Müller U, Schaub GA, Mossmann H, Köhler G, Carsetti R, Hölscher C. Immunosuppression in experimental chagas disease is mediated by an alteration of bone marrow stromal cell function during the acute phase of infection. Front Immunol (2018) 9:2794. doi: 10.3389/fimmu.2018.02794
56. Reina-San-Martín B, Degrave W, Rougeot C, Cosson A, Chamond N, Cordeiro-Da-Silva A, et al. A b-cell mitogen from a pathogenic trypanosome is a eukaryotic proline racemase. Nat Med (2000) 6(8):890–7. doi: 10.1038/78651
57. Nothelfer K, Sansonetti PJ, Phalipon A. Pathogen manipulation of b cells: the best defence is a good offence. Nat Rev Microbiol (2015) 13(3):173–84. doi: 10.1038/nrmicro3415
58. Fish K, Comoglio F, Shaffer AL, Ji Y, Pan K-T, Scheich S, et al. Rewiring of b cell receptor signaling by Epstein-Barr virus LMP2A. Proc Natl Acad Sci U.S.A. (2020) 117(42):26318–27. doi: 10.1073/pnas.2007946117
Keywords: Streptococcus suis, IdeSsuis, IgM, B cell receptor, receptor cleavage, B cell signalling
Citation: Breitfelder AK, Schrödl W, Rungelrath V, Baums CG, Alber G, Schütze N and Müller U (2023) Immunoglobulin M-degrading enzyme of Streptococcus suis (IdeSsuis) impairs porcine B cell signaling. Front. Immunol. 14:1122808. doi: 10.3389/fimmu.2023.1122808
Received: 13 December 2022; Accepted: 13 January 2023;
Published: 16 February 2023.
Edited by:
Felix Ngosa Toka, Ross University School of Veterinary Medicine, Saint Kitts and NevisReviewed by:
Stefanía P. Bjarnarson, University of Iceland, IcelandFrançois JMA Meurens, Agroalimentaire et de l’alimentation de Nantes-Atlantique (Oniris), France
Zhe Ma, Nanjing Agricultural University, China
Copyright © 2023 Breitfelder, Schrödl, Rungelrath, Baums, Alber, Schütze and Müller. This is an open-access article distributed under the terms of the Creative Commons Attribution License (CC BY). The use, distribution or reproduction in other forums is permitted, provided the original author(s) and the copyright owner(s) are credited and that the original publication in this journal is cited, in accordance with accepted academic practice. No use, distribution or reproduction is permitted which does not comply with these terms.
*Correspondence: Uwe Müller, uwe.mueller@bbz.uni-leipzig.de