- 1Department of Geriatric Psychiatry, Shanghai Mental Health Center, Shanghai Jiao Tong University School of Medicine, Shanghai, China
- 2Alzheimer’s Disease and Related Disorders Center, Shanghai Jiao Tong University, Shanghai, China
Alzheimer’s disease (AD) and COVID-19 share many common risk factors, such as advanced age, complications, APOE genotype, etc. Epidemiological studies have also confirmed the internal relationship between the two diseases. For example, studies have found that AD patients are more likely to suffer from COVID-19, and after infection with COVID-19, AD also has a much higher risk of death than other chronic diseases, and what’s more interesting is that the risk of developing AD in the future is significantly higher after infection with COVID-19. Therefore, this review gives a detailed introduction to the internal relationship between Alzheimer’s disease and COVID-19 from the perspectives of epidemiology, susceptibility and mortality. At the same time, we focused on the important role of inflammation and immune responses in promoting the onset and death of AD from COVID-19.
Introduction
Alzheimer’s disease (AD) is the most common type of dementia and one of the most common neurodegenerative diseases in the elderly, accounting for approximately 90% of dementia cases in this population. AD is characterized by irreversible and progressive loss of function, cognition, and behavior and is often accompanied by various brain disorders such as aphasia, agnosia, amnesia, and, apraxia (1). Its diagnosis is mainly based on clinical presentation as well as fluid and imaging biomarkers that meet several criteria (2), such as “AT(N)” (amyloid, tau, and neurodegeneration) (3, 4). AD is considered as a multifactorial disease, and two main hypotheses have been proposed for the cause of AD: the amyloid hypothesis and the cholinergic hypothesis (5). However, only a limited number of drugs have been developed to address this theory, and only two drugs have been approved for the treatment of AD, including N-methyld-aspartic acid (NMDA) antagonists and cholinesterase inhibitors, which are only effective against the symptoms of AD and do not cure or prevent the disease (5). According to the report of Alzheimer’s Disease International in 2018, the global prevalence of dementia was about 50 million people and was expected to triple by 2050, with two-thirds of them living in low - and middle-income countries (6). Although studies have shown that dementia rates are declining in high-income countries, the evidence is less convincing (7). Since the irreversibility of Alzheimer’s disease, it is extremely important to find out its influencing factors and make early intervention and treatment for its prognosis. The most serious risk factors for Alzheimer’s disease are advanced age and having at least one apolipoprotein E (APOE) epsilon 4 allele (8). Moreover, the Lancet also identifies twelve controllable risk factors such as less education, smoking, excessive alcohol consumption, physical inactivity, low social contact, obesity, depression, diabetes, hypertension, air pollution, hearing impairment and traumatic brain injury, which can affect the progression of dementia in 40 percent of patients (9). By contrast, healthy lifestyle choices such as physical exercise, leisure activities, and Mediterranean diet are considered protective against AD (10). In addition to these factors, the relationship between the novel coronavirus disease (COVID-19) and Alzheimer’s disease is receiving increasing attention. This review will explore the link between COVID-19 and Alzheimer’s disease in detail, including epidemiological investigations, interactions, and possible mechanisms.
The novel coronavirus disease (COVID-19)
COVID-19 is a new infectious disease caused by the severe acute respiratory syndrome coronavirus type 2 (SARS-CoV-2). Since its discovery in December 2019 in mainland China, COVID-19 has swept the world, causing untold losses and casualties (11). On 11 March 2020, the World Health Organization (WHO) declared COVID-19 a “public health Emergency of International concern” (12). As of 27 June 2022, the COVID-19 pandemic has infected more than 540 million people worldwide and caused more than 6 million deaths (13). SARS-CoV-2 is an RNA virus whose genome contains single-stranded positive RNA within a membrane envelope with an average diameter of 75-150 nm (14). It belongs taxonomically to the coronavirus family and the sarbecvirus subgenus, which contains several other species that cause mild to severe human illness (15). SARS-CoV-2 is highly contagious (16), with an estimated reproductive number, R naught (R0), of between 1.4 and 5.6 (17). Once infected with the virus, it can activate innate and adaptive immune responses and lead to large-scale inflammatory responses in the later stages of the disease (18). SARS-CoV-2 is usually spread by respiratory droplets. The average incubation period is 6.4 days. Symptoms after infection usually include cough, fever, myalgia, fatigue and difficulty breathing, and the typical findings of chest computed tomography (CT) images for individuals with COVID-19 are multifocal bilateral patchy ground-glass opacities or consolidation with interlobular septal and vascular thickening in the peripheral areas of the lungs (19). While most patients experience mild illness, a small number develop severe hypoxia requiring hospitalization or mechanical ventilation, and the most severe outcomes even include death (20, 21). Although many treatment options are being explored (e.g., convalescent plasma, and traditional Chinese medicine (TCM)), no large-scale treatment is available. What’s worse, the development of a vaccine for COVID-19 has not been smooth and there have also been some reports of adverse reactions after receiving the vaccine (22). Currently, COVID-19 prevention relies largely on public health policies, such as physical distancing, travel restrictions and city lockdowns, which are also extremely ineffective. Therefore, the future prevention strategies must focus on the target population. The epidemiological evidence suggests that advanced age and complications are the greatest risk factors for poor prognosis in COVID-19 patients (12, 23), and they are also major risk factors for Alzheimer’s disease. There are various indications that COVID-19 and Alzheimer’s disease might be intrinsically related.
COVID-19 increases the risk of developing Alzheimer’s disease in the future: Epidemiological evidence
Yu AT and Absar NM reported two patients diagnosed with COVID-19 who experienced prolonged infection after COVID-19 pneumonia and developed rapidly progressive dementia after a follow-up period of 5-10 months (22). An observational study in France reported that one-third of COVID-19 patients admitted to hospital with acute-respiratory-distress-Syndrome (ARDS) had evidence of cognitive impairment at discharge (24). Mcloughlin BC et al found that hospitalized COVID-19 patients who developed delirium during hospitalization had lower cognitive scores 1 month after discharge (25). Apple AC et al found that cognitive post-acute sequelae of SARS-CoV-2 (PASC) could occur after mild COVID-19 infection (26). Garrigues E et al found that four months after COVID-19 hospitalization, 30‐40% of patients reported problems with memory, attention, and sleep, while 10‐15% reported loss of taste and/or smell (27). Gordon MN et al pointed out that young people were also at risk of developing cognitive symptoms associated with COVID-19, even without severe illness (28). Frontera JA et al. have found that after infection with COVID-19, neurodegenerative biomarkers, such as beta-amyloid protein (aβ 40,42), total tau protein (t-tau), phosphorylated taU-181 (p-tau181), glial fibrillary acid protein (GFAP), neurofilament light chain (NfL), and ubiquitin carboxy-terminal hydrolase L1 (UCHL1), increased to levels observed in AD dementia, and was associated with encephalopathy and worse outcomes in hospitalized COVID-19 patients (29). Wang et al. reviewed 6,245,282 older adults (age ≥65 years), who visited the hospital between February 2020 and May 2021 and found that there was a significant increase in the risk of a new diagnosis of Alzheimer’s within 360 days of the initial diagnosis of COVID-19 (hazard ratio or HR:1.69, 95% CI: 1.53-1.72), especially in patients and women aged 85 years or older (30). A Mendelian randomization study indicated that hospitalization of COVID-19 was significantly associated with a higher risk of Alzheimer’s disease (OR: 1.02, 95% CI: 1.01-1.03, P: 1.19E-03); meanwhile, there was also a significant and positive genetic correlation between hospitalization of COVID-19 and AD (genetic correlation: 0.23, P = 8.36E-07) (31).
Why does COVID-19 increase the risk of Alzheimer’s disease? (possible mechanisms)
Genetic susceptibility
Apolipoprotein E is a major carrier of cholesterol in the central nervous system and an important component of very low-density lipoprotein (VLDL). Of its three alleles (epsilon 2, epsilon 3, and epsilon 4), individuals carrying the epsilon 4 allele are at a higher risk of AD, as APOE E4 genotype will increase fibrinogenesis in the brains of Alzheimer’s patients (32). Genetic correlation analysis showed that there was a significant positive correlation between AD and the heredity of hospitalized COVID-19 (rg = 0.271) (33). Meanwhile, individuals with the APOE E4 gene have also been shown to have more severe cognitive impairment after infecting with AD COVID-19 (34).
Nerve damage
More and more reports have shown that SARS-CoV-2 can alter the dense blood-brain barrier (BBB), enter the brain and damage neurons directly or indirectly, leading to long-term neurological sequelae, such as Alzheimer’s disease (35, 36). Some imaging studies have shown that after infection with COVID-19, patients may have low metabolism in the frontal lobe and cerebellum region, which will eventually lead to the apoptosis of nerve cell (37, 38). Moreover, COVID-19 is also known to infect the hippocampus and further infect the spinal cord, which can lead to further neurodegeneration due to misdirected host immune responses and/or direct damage to nerve cells through replication of viral particles, as in acute encephalitis (39).
Angiotensin-converting enzyme 2 (ACE-2)
The action of ACE-2 is associated with the renin-angiotensin-aldosterone system (RAAS), which is the entry receptor of COVID-19 (40). Once the COVID-19 virus binds to the ACE-2 receptor, it leads to an increased production of the pro-inflammatory phase, which can lead to serious complications known as “cytokine storms.” (41) In the blood-brain barrier and the meninges that cover the spinal cord, the combination of the virus with ACE-2 weakens the enzyme’s ability to protect nerve tissue, thus making them more vulnerable to damage, leading to encephalitis, or myelitis (42). In addition, there has also been reported that the combination of the virus with ACE-2 in the cerebrovascular increased the intracavitary pressure, which eventually led to the patient’s cerebral hemorrhage (43).
Why are people with dementia more susceptible to COVID-19? (epidemiological evidence)
As of September 21, 2021, a series of observational retrospective analyses using electronic health records (EHRs) from Columbia University Irvine Medical Center/New York-Presbyterian Hospital (CUIMC/NYP) have shown that Alzheimer’s disease was a major risk factor for COVID-19 infection (44). A matched-cohort study using primary care electronic records in the UK showed that patients with Down syndrome (DS) were more likely to be diagnosed with COVID-19 than controls (7.4% vs 5.6%, p≤0.001, odds ratio (OR) = 1.35; 95% CI = 1.23-1.48) (45).
A retrospective cohort study of 262,847 vaccinated older adults (age 73.8±6.81 years) in the United States between December 2020 and August 2021 showed that people with dementia had an increased risk of developing breakthrough infections compared to unvaccinated patients, with the highest odds for patients with Lewy body dementia (LBD) (adjusted odds ratio or AOR: 3.06, 95% confidence interval or CI [1.45 to 6.66]) (30). A survey of the Korean National Health Insurance Database suggested that COVID-19 patients were more likely to have a prediagnosed AD (adjusted odds ratio [aOR] = 2.11, 95% confidence interval [CI] = 1.79-2.50, p values < 0.001) compared to the control group (46). An observational cohort study showed that Alzheimer’s disease (OR = 2.29, 95% CI: 1.25-4.16) and dementia (OR = 2.16, 95% CI: 1.36-3.42) were the most significant risk factors for COVID-19 (47). A population-based cohort study conducted in the Lazio region has shown that Alzheimer’s disease was more susceptible to COVID-19, and being males and aged ≥85 years of age were at a higher risk of death from infection (48). A cross-sectional analysis showed that the preexisting cognitive impairment was significantly associated with a higher likelihood of COVID-19 infection (OR, CI: 1.51, 1.35-1.70) (49). A population-based risk assessment model for severe disease outcome from COVID-19 confirmed that Alzheimer’s disease was associated with the highest risk for hospitalization (aHR 3.19, CI: 2.88-3.52) and death (aHR 4.04, CI: 3.32-4.91) (50). A meta-analysis suggested that patients with dementia, including Alzheimer’s disease, had an increased risk of hospitalization and death after contracting COVID-19 (51). Moreover, several reviews have also indicated that neurodegenerative diseases, including Alzheimer’s disease, Parkinson’s disease and epilepsy, are major risk factors for COVID-19 infection, and demand extra care as well as improvised treatment (52).
Why are people with dementia more susceptible to COVID-19? (possible mechanism)
There are several reasons why people with Alzheimer’s disease may be more susceptible to COVID-19. First, people with Alzheimer’s are often older, which is also a major risk factor for COVID-19 (53). It has been speculated that aging may induce the production of reactive oxygen species (ROS), increase neuroinflammation intensify, overproduction of Aβ, which contributes to the pathogenesis of COVID-19 and AD. Since aging is characterized by a progressive loss of blood-brain barrier integrity, older adults may be more susceptible to neuroaggression during the SARS-CoV-2 infection (54); Second, people with dementia often do not have access to accurate information and facts about the COVID-19 pandemic, and they may have difficulty remembering protective procedures or understanding public health messages sent to them. As a result, these people tend to be more susceptible to COVID-19 infection (55). Third, the reduced ability to cope with sudden changes in the social environment makes people with dementia more vulnerable to COVID-19 infection as well as poorer clinical and social outcomes (56). Fourth, people with dementia tend to have a variety of physical conditions, such as hypertension, diabetes, heart diseases, so the consequences of contracting COVID-19 are often worse (57). Fifth, elderly care institutions or medical institutions in many areas, such as China, often receive a large number of dementia patients with a very large population density. Once the COVID-19 infection occurs, the epidemic will spread rapidly. Sixth, some studies have suggested that Alzheimer’s disease and COVID-19 may share a common genetic structure (58), for example, four hub genes (ITPR1, ITPR3, ITPKB, RAPGEF3) were considered as important factors in the development of AD that were affected by COVID-19 (59). Homozygous APOE e4e4 is not only a risk factor for AD, but also increases the susceptibility to severe infection of SARS-CoV-2 (60). Compared to APOE E3 mice, APOE2 and APOE4 mice exhibited increased viral loads as well as suppressed adaptive immune responses early after infection (61). Seventh, serum cholesterol binds to APOE receptors and induces ACE-2 receptor transport to the cell surface. Ultra-resolution imaging studies have shown that high cholesterol levels will confer a 2-fold in increase the entry site of SARS-CoV-2, thus facilitating the entry of the virus (62). At the same time, elevated Aβ levels, specifically Aβ1-42, are associated with SARS-CoV-2, and the binding of Aβ1-42 to the spike protein S1 subunit (S1) of SARS-CoV-2 and ACE2 may negatively affect the course and severity of SARS-CoV-2 infection (63).
Why individuals with Alzheimer’s disease are most likely to die after contracting COVID-19?(epidemiological evidence)
According to relevant reports, people with dementia are more than three times more likely to die from COVID-19 than those without dementia and about 30% of COVID-19 deaths are in people with dementia (64, 65). A cohort study conducted in Tehran, Iran showed that Alzheimer disease were associated with a higher risk of death related to COVID-19 (66). A cohort study conducted in England showed that COVID-19 survivors with pre-existing dementia had a higher risk of hospitalization or death (age and gender adjusted HR 2.47, 1.37 to 4.44, p = 0.002) (67). According to a study by the UK Biobank, older people with Alzheimer’s disease were at the highest risk and mortality from COVID-19 (68). Another UK Biobank study involving 12,863 patients found that all-cause dementia and AD were age-independent risk factors for the severity and death of COVID-19 disease (69). A population-based register study has shown that there was a significant increase in mortality from diabetes and Alzheimer’s disease after infection with COVID-19 (70). In the study by Fathi M et al., they concluded that inpatients with Alzheimer’s disease had an increased risk for 28-day mortality from COVID-19 (71). In the study of Chung SJ et al., they found that AD was not associated with increased susceptibility to COVID-19 infection, but was associated with severe COVID-19 complications, especially mortality (72). Zhao Y et al noted that about 35% of COVID-19 patients present with neurological and neuropsychiatric symptoms, a previous diagnosis of Alzheimer’s disease (AD) predicts the highest risk of COVID-19, and elderly AD patients have the highest mortality rate (73). Wang et al. found a significant association between Alzheimer’s disease (AD) and increased risk of COVID-19 infection and mortality (30). Harb AA et al. found that hospitalized patients with COVID-19 dementia had a higher mortality rate, but dementia was not an independent risk factor for death (74). Moreover, a 93-country study also showed that there was a strong link between AD and COVID-19 deaths (75). Therefore, we are relatively certain that Alzheimer’s disease can increase the risk of death in patients with COVID-19.
Why individuals with Alzheimer’s disease are most likely to die after contracting COVID-19? (immune and inflammatory mechanisms)
In addition to the effects of old age, comorbidities, and genetics described above, immune and inflammatory responses appear to play an important role in promoting AD death. However, there is little evidence to support SARS-CoV-2 infection of central nervous system cells, and most neurological symptoms appear to be due to hypoxia/ischemia and/or damage mediated by inflammatory damage (76). For example, Mao L et al found that COVID-19 can induce uncontrolled cytokine storms (mainly involving IL-6, IL-1β, and TNF), leading to a variety of symptoms including delirium (55). Ziff OJ et al found that there was a significant increased CSF proinflammatory cytokines, such as TNFɑ, IL-6, IL-1β, IL-8, among COVID-19 neurological patients and these proinflammatory cytokines were negatively correlated with sAPPɑ and sAPPβ (31). Poloni TE et al found that the brain infected with COVID-19 would show increased innate immunity and microglia enhancement (77). Ganji R et al. pointed out that COVID-19 could hijack the mitochondria of immune cells, replicate within the mitochondrial structure, disrupt mitochondrial dynamics and lead to cell death (78). Daugherty AM et al suggested that the interaction of inflammation and oxidative stress may initiate a self-propagating cascade that drives subsequent age-related decline (79). Chiricosta L et al. concluded that SARS-CoV-2 worsens AD by increasing neurotoxicity due to elevated levels of inflammation, beta-amyloid, and oxidative stress (80). D’Arrigo JS pointed that immune responses and excessive inflammation may accelerate the progression of inflammatory neurodegeneration in the brain, thereby increasing the likelihood of post-infection memory impairment and accelerating the progression of Alzheimer’s disease (81). Butler MJ found that even in these patients who have recovered from COVID-19 infection, peripheral inflammation may contribute to the progression of neurodegenerative diseases through neuroinflammatory mechanisms (82). Guasp M et al. demonstrated that SARS-CoV-2 infection may promote inflammatory processes by disrupting the blood-brain barrier (83). Reiken S et al. provided evidence linking SARS-CoV-2 infection with TGF-β signaling activation and oxidation overload (84). Naughton SX et al. pointed out that the immune response and excessive inflammation in COVID-19 might accelerate the progression of inflammatory neurodegeneration in the brain, and the elderly were more prone to severe consequences after infection with SARS-CoV-2 (85). An autopsy report also showed that the most typical pathological features of COVID-19 were a large number of t lymphocytes and microthrombus formation in the lung, and a high activation of brain stem-related microglia (86). In conclusion, central nervous system autoimmune cascades triggered by COVID-19 may occur through a variety of pathways, including molecular mimicry, epitope diffusion, bystander activation, autoantibody production, and effector B cell immobilization (87), and ultimately contribute to the death of AD patients. The past studies on Alzheimer’s disease and COVID-19 infection and mortality rate or disease progression and other major findings will be presented in Table 1.
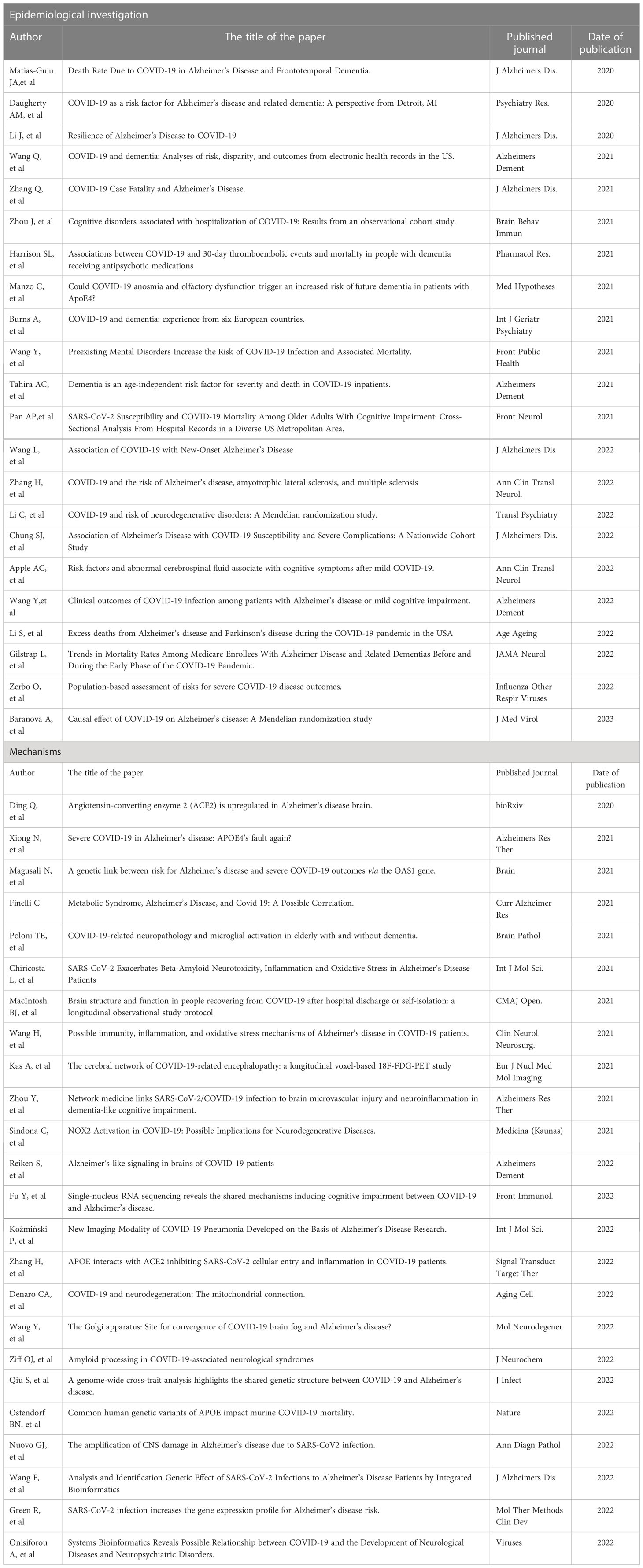
Table 1 The past studies on Alzheimer’s disease and COVID-19 infection and mortality rate or disease progression and other major findings.
Advances in drug treatments for Alzheimer’s disease and COVID-19
Treatments for Alzheimer’s disease include cholinesterase inhibitors (ChEIs) (Donepezil, Rivastin, and Galanthamine) and metaxine. In addition, antidepressants and antipsychotics are also used to control patients’ behavior and psychiatric symptoms (88). Previous studies have shown that disruption of intracellular Ca2+ homeostasis is not only an upstream pathologic pathway for AD, but also for SARS-CoV-2 virus infection and COVID-19 replication (89). Therefore, lithium could be repurposed to treat patients with AD, especially those with COVID-19 (90). Ginkgo biloba extract (GbE) and bobalide (BB) are important bioactive components of Ginkgo biloba extract (GbE), which have been reported to show neuroprotective effects in AD via multiple mechanisms such as anti-oxidative activities, anti-excitotoxicity, and anti-inflammatory. Interestingly, ginkgolides and BB also appear to exhibit antiviral properties against COVID-19 by inhibiting the major proteases of severe acute respiratory syndrome coronavirus type 2 (SARS-CoV-2) (44). Increasing data suggest that Zn2+ metabolism may be involved in neurodegeneration, and the mechanisms may involve modulation of synaptic plasticity through ProSAP/Shank scaffold, neurotransmitter metabolism, and gut microbiota. Moreover, Zn2+ has also been shown to be a potential adjuvant therapy in management of novel coronavirus infection (COVID-19) (91). Myricetin (MYR) is a flavonoid compound widely found in many natural plants, including the bayberry. MYR can enhance immune regulation function, inhibit cytokine storm, improve cardiac dysfunction, and has antiviral potential. So it can be used as an adjuvant treatment for Alzheimer’s disease, cardiovascular injury and other neurological diseases, and may be a potential drug against COVID-19 and other viral infections (92). Flavone luteolin is an important natural polyphenol present in several plants that show antioxidant, anticancer, cytoprotective, anti-inflammatory, and macrophage polarization effects. Recent reports suggest that flavone luteolin can inhibit systemic and neuroinflammatory responses in COVID-19 (93). Other promising treatments for AD and COVID-19 also include (biobased) lipid nanocarrier (81), inflammasome and JaK inhibitors (94), angiotensin converting enzyme (ACE) inhibitors (95), and Metformin (96). However, none of the above drugs have undergone large-scale clinical trials, and the future application prospects are unknown.
Conclusions
There is a strong correlation between Alzheimer’s disease and COVID-19. The two factors influence each other, promote each other and ultimately lead to poor prognosis. In this process, inflammation and immune response are likely to play an extremely important role. In the future epidemic prevention process, we should focus on the protection of the above-mentioned population, so as to reduce the mortality of patients to the greatest extent.
Author contributions
WL and LY contributed to the study concept and design. WL and LS wrote this wrote this article. SX provided the funding support. All authors contributed to the article and approved the submitted version.
Funding
This study was supported by grants from the clinical research center project of Shanghai Mental Health Center (CRC2017ZD02), Shanghai Clinical Research Center for Mental Health (19MC1911100), the Cultivation of Multidisciplinary Interdisciplinary Project in Shanghai Jiaotong University (YG2019QNA10), and the Feixiang Program of Shanghai Mental Health Center (2020-FX-03), the National Natural Science Foundation of China (82101564, 82001123, 82271607), Chinese Academy of Sciences (XDA12040101), Shanghai Clinical Research Center for Mental Health (SCRC-MH, 19MC1911100), the Shanghai Science and Technology Committee (20Y11906800).
Conflict of interest
The authors declare that the research was conducted in the absence of any commercial or financial relationships that could be construed as a potential conflict of interest.
Publisher’s note
All claims expressed in this article are solely those of the authors and do not necessarily represent those of their affiliated organizations, or those of the publisher, the editors and the reviewers. Any product that may be evaluated in this article, or claim that may be made by its manufacturer, is not guaranteed or endorsed by the publisher.
References
1. Yesavage JA, Brooks JO 3rd, Taylor J, Tinklenberg J. Development of aphasia, apraxia, and agnosia and decline in alzheimer’s disease. Am J Psychiatry (1993) 150(5):742–7. doi: 10.1176/ajp.150.5.742
2. Weller J, Budson A. Current understanding of alzheimer’s disease diagnosis and treatment. F1000Research (2018) 7:12688–711. doi: 10.12688/f1000research.14506.1
3. Jack CR Jr., Bennett DA, Blennow K, Carrillo MC, Dunn B, Haeberlein SB, et al. NIA-AA research framework: Toward a biological definition of alzheimer’s disease. Alzheimer’s dementia: J Alzheimer’s Assoc (2018) 14(4):535–62. doi: 10.1016/j.jalz.2018.02.018
4. Nho K, Kueider-Paisley A, MahmoudianDehkordi S, Arnold M, Risacher SL, Louie G, et al. Altered bile acid profile in mild cognitive impairment and alzheimer’s disease: Relationship to neuroimaging and CSF biomarkers. Alzheimer’s dementia: J Alzheimer’s Assoc (2019) 15(2):232–44. doi: 10.1016/j.jalz.2018.08.012
5. Breijyeh Z, Karaman R. Comprehensive review on alzheimer’s disease: Causes and treatment. Molecules (Basel Switzerland) (2020) 25(24):5789–810. doi: 10.3390/molecules25245789
6. Scheltens P, De Strooper B, Kivipelto M, Holstege H, Chételat G, Teunissen CE, et al. Alzheimer’s disease. Lancet (London England) (2021) 397(10284):1577–90. doi: 10.1016/S0140-6736(20)32205-4
7. Wu YT, Beiser AS, Breteler MMB, Fratiglioni L, Helmer C, Hendrie HC, et al. The changing prevalence and incidence of dementia over time - current evidence. Nat Rev Neurology (2017) 13(6):327–39. doi: 10.1038/nrneurol.2017.63
8. van der Lee SJ, Wolters FJ, Ikram MK, Hofman A, Ikram MA, Amin N, et al. The effect of APOE and other common genetic variants on the onset of alzheimer’s disease and dementia: a community-based cohort study. Lancet Neurology (2018) 17(5):434–44. doi: 10.1016/S1474-4422(18)30053-X
9. Livingston G, Huntley J, Sommerlad A, Ames D, Ballard C, Banerjee S, et al. Dementia prevention, intervention, and care: 2020 report of the lancet commission. Lancet (London England) (2020) 396(10248):413–46. doi: 10.1016/S0140-6736(20)30367-6
10. Serrano-Pozo A, Growdon JH. Is alzheimer’s disease risk modifiable? J Alzheimer’s disease: JAD (2019) 67(3):795–819. doi: 10.3233/JAD181028
11. Huang C, Huang L, Wang Y, Li X, Ren L, Gu X, et al. 6-month consequences of COVID-19 in patients discharged from hospital: a cohort study. Lancet (London England) (2021) 397(10270):220–32. doi: 10.1016/S0140-6736(20)32656-8
12. Liu N, Sun J, Wang X, Zhao M, Huang Q, Li H. The impact of dementia on the clinical outcome of COVID-19: A systematic review and meta-analysis. J Alzheimer’s disease: JAD (2020) 78(4):1775–82. doi: 10.3233/JAD-201016
13. Chen F, Chen Y, Wang Y, Ke Q, Cui L. The COVID-19 pandemic and alzheimer’s disease: mutual risks and mechanisms. Trans Neurodegeneration (2022) 11(1):40. doi: 10.1186/s40035-022-00316-y
14. Majumder J, Minko T. Recent developments on therapeutic and diagnostic approaches for COVID-19. AAPS J (2021) 23(1):14. doi: 10.1208/s12248-020-00532-2
15. Yüce M, Filiztekin E, Özkaya KG. COVID-19 diagnosis -a review of current methods. Biosensors Bioelectronics (2021) 172:112752. doi: 10.1016/j.bios.2020.112752
16. Marofi F, Azizi R, Motavalli R, Vahedi G, Nasimi M, Yousefi M, et al. COVID-19: Our current knowledge of epidemiology, pathology, therapeutic approaches, and diagnostic methods. Anti-cancer Agents Medicinal Chem (2021) 21(16):2142–62. doi: 10.2174/1871520621666210201101245
17. Safiabadi Tali SH, LeBlanc JJ, Sadiq Z, Oyewunmi OD, Camargo C, Nikpour B, et al. Tools and techniques for severe acute respiratory syndrome coronavirus 2 (SARS-CoV-2)/COVID-19 detection. Clin Microbiol Rev (2021) 34(3):228–444. doi: 10.1128/CMR.00228-20
18. Anka AU, Tahir MI, Abubakar SD, Alsabbagh M, Zian Z, Hamedifar H, et al. Coronavirus disease 2019 (COVID-19): An overview of the immunopathology, serological diagnosis and management. Scandinavian J Immunol (2021) 93(4):e12998. doi: 10.1111/sji.12998
19. Böger B, Fachi MM, Vilhena RO, Cobre AF, Tonin FS, Pontarolo R. Systematic review with meta-analysis of the accuracy of diagnostic tests for COVID-19. Am J infection control (2021) 49(1):21–9. doi: 10.1016/j.ajic.2020.07.011
20. Kim GU, Kim MJ, Ra SH, Lee J, Bae S, Jung J, et al. Clinical characteristics of asymptomatic and symptomatic patients with mild COVID-19. Clin Microbiol infection: Off Publ Eur Soc Clin Microbiol Infect Diseases (2020) 26(7):948.e941–948.e943. doi: 10.1016/j.cmi.2020.04.040
21. Ochani R, Asad A, Yasmin F, Shaikh S, Khalid H, Batra S, et al. COVID-19 pandemic: from origins to outcomes. a comprehensive review of viral pathogenesis, clinical manifestations, diagnostic evaluation, and management. Le infezioni medicina (2021) 29(1):20–36.
22. Alsharif W, Qurashi A. Effectiveness of COVID-19 diagnosis and management tools: A review. Radiography (London England: 1995) (2021) 27(2):682–7. doi: 10.1016/j.radi.2020.09.010
23. Chaimayo C, Kaewnaphan B, Tanlieng N, Athipanyasilp N, Sirijatuphat R, Chayakulkeeree M, et al. Rapid SARS-CoV-2 antigen detection assay in comparison with real-time RT-PCR assay for laboratory diagnosis of COVID-19 in Thailand. Virol J (2020) 17(1):177. doi: 10.1186/s12985-020-01452-5
24. Helms J, Kremer S, Merdji H, Clere-Jehl R, Schenck M, Kummerlen C, et al. Neurologic features in severe SARS-CoV-2 infection. New Engl J Med (2020) 382(23):2268–70. doi: 10.1056/NEJMc2008597
25. McLoughlin BC, Miles A, Webb TE, Knopp P, Eyres C, Fabbri A, et al. Functional and cognitive outcomes after COVID-19 delirium. Eur geriatric Med (2020) 11(5):857–62. doi: 10.1007/s41999-020-00353-8
26. Apple AC, Oddi A, Peluso MJ, Asken BM, Henrich TJ, Kelly JD, et al. Risk factors and abnormal cerebrospinal fluid associate with cognitive symptoms after mild COVID-19. Ann Clin Trans neurology (2022) 9(2):221–6. doi: 10.1002/acn3.51498
27. Garrigues E, Janvier P, Kherabi Y, Le Bot A, Hamon A, Gouze H, et al. Post-discharge persistent symptoms and health-related quality of life after hospitalization for COVID-19. J infection (2020) 81(6):e4–6. doi: 10.1016/j.jinf.2020.08.029
28. Gordon MN, Heneka MT, Le Page LM, Limberger C, Morgan D, Tenner AJ, et al. Impact of COVID-19 on the onset and progression of alzheimer’s disease and related dementias: A roadmap for future research. Alzheimer’s dementia: J Alzheimer’s Assoc (2022) 18(5):1038–46. doi: 10.1002/alz.12488
29. Frontera JA, Boutajangout A, Masurkar AV, et al, Betensky RA, Ge Y, Vedvyas A.Comparison of serum neurodegenerative biomarkers among hospitalized COVID-19 patients versus non-COVID subjects with normal cognition, mild cognitive impairment, or alzheimer’s dementia. Alzheimer’s dementia: J Alzheimer’s Assoc (2022) 18(5):899–910. doi: 10.1002/alz.12556
30. Wang L, Davis PB, Kaelber DC, Xu R. COVID-19 breakthrough infections and hospitalizations among vaccinated patients with dementia in the united states between December 2020 and august 2021. Alzheimer’s dementia: J Alzheimer’s Assoc (2022) 10:1002–24. doi: 10.1002/alz.12669
31. Ziff OJ, Ashton NJ, Mehta PR, Brown R, Athauda D, Heaney J, et al. Amyloid processing in COVID-19-associated neurological syndromes. J Neurochemistry (2022) 161(2):146–57. doi: 10.1111/jnc.15585
32. Hultman K, Strickland S, Norris EH. The APOE ε4/ε4 genotype potentiates vascular fibrin(ogen) deposition in amyloid-laden vessels in the brains of alzheimer’s disease patients. J Cereb Blood Flow metabolism: Off J Int Soc Cereb Blood Flow Metab (2013) 33(8):1251–8. doi: 10.1038/jcbfm.2013.76
33. Baranova A, Cao H, Zhang F. Causal effect of COVID-19 on alzheimer’s disease: A mendelian randomization study. J Med Virology (2022) 95(1):28107–37. doi: 10.1002/jmv.28107
34. Xiong N, Schiller MR, Li J, Chen X, Lin Z. Severe COVID-19 in alzheimer’s disease: APOE4’s fault again? Alzheimer’s Res Ther (2021) 13(1):111. doi: 10.1186/s13195-021-00858-9
35. Pezzini A, Padovani A. Lifting the mask on neurological manifestations of COVID-19. Nat Rev Neurology (2020) 16(11):636–44. doi: 10.1038/s41582-020-0398-3
36. Tsai LK, Hsieh ST, Chang YC. Neurological manifestations in severe acute respiratory syndrome. Acta neurologica Taiwanica (2005) 14(3):113–9.
37. Guedj E, Million M, Dudouet P, Tissot-Dupont H, Bregeon F, Cammilleri, et al. (18)F-FDG brain PET hypometabolism in post-SARS-CoV-2 infection: substrate for persistent/delayed disorders? Eur J Nucl Med Mol Imaging (2021) 48(2):592–5. doi: 10.1007/s00259-020-04973-x
38. Delorme C, Paccoud O, Kas A, Hesters A, Bombois S, Shambrook P, et al. COVID-19-related encephalopathy: a case series with brain FDG-positron-emission tomography/computed tomography findings. Eur J neurology (2020) 27(12):2651–7. doi: 10.1111/ene.14478
39. Desforges M, Le Coupanec A, Brison É, Meessen-Pinard M, Talbot PJ. Human respiratory coronaviruses : neuroinvasive, neurotropic and potentially neurovirulent pathogens. Virologie (Montrouge France) (2014) 18(1):5–16. doi: 10.1684/vir.2014.0544
40. Ratajczak MZ, Bujko K, Ciechanowicz A, Sielatycka K, Cymer M, Marlicz W, et al. SARS-CoV-2 entry receptor ACE2 is expressed on very small CD45(-) precursors of hematopoietic and endothelial cells and in response to virus spike protein activates the Nlrp3 inflammasome. Stem Cell Rev Rep (2021) 17(1):266–77. doi: 10.1007/s12015-020-10010-z
41. Sun Y, Li J, Xie X, Gu F, Sui Z, Zhang K, et al. Recent advances in osteoclast biological behavior. Front Cell Dev Biol (2021) 9:788680. doi: 10.3389/fcell.2021.788680
42. Baig AM, Khaleeq A, Ali U, Syeda H. Evidence of the COVID-19 virus targeting the CNS: Tissue distribution, host-virus interaction, and proposed neurotropic mechanisms. ACS Chem Neurosci (2020) 11(7):995–8. doi: 10.1021/acschemneuro.0c00122
43. Wu Y, Xu X, Chen Z, Duan J, Hashimoto K, Yang L, et al. Nervous system involvement after infection with COVID-19 and other coronaviruses. Brain behavior immunity (2020) 87:18–22. doi: 10.1016/j.bbi.2020.03.031
44. Niu TT, Yuan BY, Liu GZ. Ginkgolides and bilobalide for treatment of alzheimer’s disease and COVID-19: potential mechanisms of action. Eur Rev Med Pharmacol Sci (2022) 26(24):9502–10. doi: 10.26355/eurrev_202212_30702
45. Baksh RA, Strydom A, Pape SE, Chan LF, Gulliford MC. Susceptibility to COVID-19 diagnosis in people with down syndrome compared to the general population: Matched-cohort study using primary care electronic records in the UK. J Gen Internal Med (2022) 37(8):2009–15. doi: 10.1007/s11606-022-07420-9
46. Kim JH, Chang IB, Kim YH, Min CY, Yoo DM, Choi HG. The association of pre-existing diagnoses of alzheimer’s disease and parkinson’s disease and coronavirus disease 2019 infection, severity and mortality: Results from the Korean national health insurance database. Front Aging Neurosci (2022) 14:821235. doi: 10.3389/fnagi.2022.821235
47. Zhou J, Liu C, Sun Y, Huang W, Ye K. Cognitive disorders associated with hospitalization of COVID-19: Results from an observational cohort study. Brain behavior immunity (2021) 91:383–92. doi: 10.1016/j.bbi.2020.10.019
48. Cascini S, Agabiti N, Marino C, Acampora A, Balducci M, Calandrini E, et al. Incidence and outcomes of SARS-CoV-2 infection in older adults living with dementia: A population-based cohort study. J Alzheimer’s disease: JAD (2022) 89(2):681–93. doi: 10.3233/JAD-220369
49. Pan AP, Meeks J, Potter T, Masdeu JC, Seshadri S, Smith ML, et al. SARS-CoV-2 susceptibility and COVID-19 mortality among older adults with cognitive impairment: Cross-sectional analysis from hospital records in a diverse US metropolitan area. Front neurology (2021) 12:692662. doi: 10.3389/fneur.2021.692662
50. Zerbo O, Lewis N, Fireman B, Goddard K, Skarbinski J, Sejvar JJ, et al. Population-based assessment of risks for severe COVID-19 disease outcomes. Influenza other Respir viruses (2022) 16(1):159–65. doi: 10.1111/irv.12901
51. McAlpine LS, Fesharaki-Zadeh A, Spudich S. Coronavirus disease 2019 and neurodegenerative disease: what will the future bring? Curr Opin Psychiatry (2021) 34(2):177–85. doi: 10.1097/YCO.0000000000000688
52. Sharma S, Batra S, Gupta S, Sharma VK, Rahman MH, Kamal MA. Persons with Co-existing neurological disorders: Risk analysis, considerations and management in COVID-19 pandemic. CNS neurological Disord Drug Targets (2022) 21(3):228–34. doi: 10.2174/1871527320666210308113457
53. Chen Y, Klein SL, Garibaldi BT, Li H, Wu C, Osevala NM, et al. Aging in COVID-19: Vulnerability, immunity and intervention. Ageing Res Rev (2021) 65:101205. doi: 10.1016/j.arr.2020.101205
54. Villa C, Rivellini E, Lavitrano M, Combi R. Can SARS-CoV-2 infection exacerbate alzheimer’s disease? an overview of shared risk factors and pathogenetic mechanisms. J Personalized Med (2022) 12(1):29–72. doi: 10.3390/jpm12010029
55. Mao L, Jin H, Wang M, Hu Y, Chen S, He Q, et al. Neurologic manifestations of hospitalized patients with coronavirus disease 2019 in wuhan, China. JAMA neurology (2020) 77(6):683–90. doi: 10.1001/jamaneurol.2020.1127
56. Li X, Wang W, Zhao X, Zai J, Zhao Q, Li Y, et al. Transmission dynamics and evolutionary history of 2019-nCoV. J Med Virology (2020) 92(5):501–11. doi: 10.1002/jmv.25701
57. Cuffaro L, Di Lorenzo F, Bonavita S, Tedeschi G, Leocani L, Lavorgna L. Dementia care and COVID-19 pandemic: a necessary digital revolution. Neurological sciences: Off J Ital Neurological Soc Ital Soc Clin Neurophysiology (2020) 41(8):1977–9. doi: 10.1007/s10072-020-04512-4
58. Qiu S, Hu Y, Cheng L. A genome-wide cross-trait analysis highlights the shared genetic structure between COVID-19 and alzheimer’s disease. J infection (2022) 84(1):e1–2. doi: 10.1016/j.jinf.2021.08.038
59. Wang F, Xu J, Xu SJ, Guo JJ, Wang F, Wang QW. Analysis and identification genetic effect of SARS-CoV-2 infections to alzheimer’s disease patients by integrated bioinformatics. J Alzheimer’s disease: JAD (2022) 85(2):729–44. doi: 10.3233/JAD-215086
60. Kuo CL, Pilling LC, Atkins JL, Masoli JAH, Delgado J, Kuchel GA, et al. APOE e4 genotype predicts severe COVID-19 in the UK biobank community cohort. journals gerontology Ser A Biol Sci Med Sci (2020) 75(11):2231–2. doi: 10.1093/gerona/glaa131
61. Ostendorf BN, Patel MA, Bilanovic J, Hoffmann HH, Carrasco SE, Rice CM, et al. Common human genetic variants of APOE impact murine COVID-19 mortality. Nature (2022) 611(7935):346–51. doi: 10.1038/s41586-022-05344-2
62. Wang H, Yuan Z, Pavel MA, Jablonski SM, Jablonski J, Hobson R, et al. The role of high cholesterol in age-related COVID19 lethality. bioRxiv: preprint server Biol (2021) 5:86249–61. doi: 10.1101/2020.05.09.086249
63. Hsu JT, Tien CF, Yu GY, Shen S, Lee YH, Hsu PC, et al. The effects of Aβ(1-42) binding to the SARS-CoV-2 spike protein S1 subunit and angiotensin-converting enzyme 2. Int J Mol Sci (2021) 22(15):8226–56. doi: 10.3390/ijms22158226
64. Hariyanto TI, Putri C, Arisa J, Situmeang RFV, Kurniawan A. Dementia and outcomes from coronavirus disease 2019 (COVID-19) pneumonia: A systematic review and meta-analysis. Arch gerontology geriatrics (2021) 93:104299. doi: 10.1016/j.archger.2020.104299
65. Liu KY, Howard R, Banerjee S, Comas-Herrera A, Goddard J, Knapp M, et al. Dementia wellbeing and COVID-19: Review and expert consensus on current research and knowledge gaps. Int J geriatric Psychiatry (2021) 36(11):1597–639. doi: 10.1002/gps.5567
66. Hatamabadi H, Sabaghian T, Sadeghi A, Heidari K, Safavi-Naini SAA, LoohaMA , et al. Epidemiology of COVID-19 in Tehran, Iran: A cohort study of clinical profile, risk factors, and outcomes. BioMed Res Int (2022) 2022:2350063. doi: 10.1155/2022/2350063
67. Bhaskaran K, Rentsch CT, Hickman G, Hulme WJ, Schultze A, Curtis HJ, et al. Overall and cause-specific hospitalisation and death after COVID-19 hospitalisation in England: A cohort study using linked primary care, secondary care, and death registration data in the OpenSAFELY platform. PloS Med (2022) 19(1):e1003871. doi: 10.1371/journal.pmed.1003871
68. Yu Y, Travaglio M, Popovic R, Leal NS, Martins LM. Alzheimer’s and parkinson’s diseases predict different COVID-19 outcomes: A UK biobank study. Geriatrics (Basel Switzerland) (2021) 6(1):10–32. doi: 10.3390/geriatrics6010010
69. Tahira AC, Verjovski-Almeida S, Ferreira ST. Dementia is an age-independent risk factor for severity and death in COVID-19 inpatients. Alzheimer’s dementia: J Alzheimer’s Assoc (2021) 17(11):1818–31. doi: 10.1002/alz.12352
70. Raknes G, Strøm MS, Sulo G, Øverland S, Roelants M, Juliusson PB. Lockdown and non-COVID-19 deaths: cause-specific mortality during the first wave of the 2020 pandemic in Norway: a population-based register study. BMJ Open (2021) 11(12):e050525. doi: 10.1136/bmjopen-2021-050525
71. Fathi M, Taghizadeh F, Mojtahedi H, Zargar Balaye Jame S, Markazi Moghaddam N. The effects of alzheimer’s and parkinson’s disease on 28-day mortality of COVID-19. Rev neurologique (2022) 178(1-2):129–36. doi: 10.1016/j.neurol.2021.08.002
72. Chung SJ, Chang Y, Jeon J, Shin JI, Song TJ, Kim J. Association of alzheimer’s disease with COVID-19 susceptibility and severe complications: A nationwide cohort study. J Alzheimer’s disease: JAD (2022) 87(2):701–10. doi: 10.3233/JAD-220031
73. Zhao Y, Li W, Lukiw W. Ubiquity of the SARS-CoV-2 receptor ACE2 and upregulation in limbic regions of alzheimer’s disease brain. Folia neuropathologica (2021) 59(3):232–8. doi: 10.5114/fn.2021.109495
74. Harb AA, Chen R, Chase HS, Natarajan K, Noble JM. Clinical features and outcomes of patients with dementia compared to an aging cohort hospitalized during the initial new York city COVID-19 wave. J Alzheimer’s disease: JAD (2021) 81(2):679–90. doi: 10.3233/JAD-210050
75. Hashim MJ, Alsuwaidi AR, Khan G. Population risk factors for COVID-19 mortality in 93 countries. J Epidemiol Global Health (2020) 10(3):204–8. doi: 10.2991/jegh.k.200721.001
76. Emmi A, Boura I, Raeder V, Mathew D, Sulzer D, Goldman JE, et al. Covid-19, nervous system pathology, and parkinson’s disease: Bench to bedside. Int Rev neurobiology (2022) 165:17–34. doi: 10.1016/bs.irn.2022.06.006
77. Poloni TE, Medici V, Moretti M, Visonà SD, Cirrincione A, Carlos AF, et al. COVID-19-related neuropathology and microglial activation in elderly with and without dementia. Brain Pathol (Zurich Switzerland) (2021) 31(5):e12997. doi: 10.1111/bpa.12997
78. Ganji R, Reddy PH. Impact of COVID-19 on mitochondrial-based immunity in aging and age-related diseases. Front Aging Neurosci (2020) 12:614650. doi: 10.3389/fnagi.2020.614650
79. Daugherty AM, Chopra T, Korzeniewski SJ, Levy P. COVID-19 as a risk factor for alzheimer’s disease and related dementia: A perspective from Detroit, MI. Psychiatry Res (2020) 294:113557. doi: 10.1016/j.psychres.2020.113557
80. Chiricosta L, Gugliandolo A, Mazzon E. SARS-CoV-2 exacerbates beta-amyloid neurotoxicity, inflammation and oxidative stress in alzheimer’s disease patients. Int J Mol Sci (2021) 22(24):13603–33. doi: 10.3390/ijms222413603
81. D’Arrigo JS. Nanotargeting of drug(s) for delaying dementia: Relevance of covid-19 impact on dementia. Am J Alzheimer’s Dis other dementias (2020) 35:1533317520976761. doi: 10.1177/1533317520976761
82. Butler MJ, Barrientos RM. The impact of nutrition on COVID-19 susceptibility and long-term consequences. Brain behavior immunity (2020) 87:53–4. doi: 10.1016/j.bbi.2020.04.040
83. Guasp M, Muñoz-Sánchez G, Martínez-Hernández E, Santana D, Carbayo Á, Naranjo L, et al. CSF biomarkers in COVID-19 associated encephalopathy and encephalitis predict long-term outcome. Front Immunol (2022) 13:866153. doi: 10.3389/fimmu.2022.866153
84. Reiken S, Sittenfeld L, Dridi H, Liu Y, Liu X, Marks AR. Alzheimer’s-like signaling in brains of COVID-19 patients. Alzheimer’s dementia: J Alzheimer’s Assoc (2022) 18(5):955–65. doi: 10.1002/alz.12558
85. Naughton SX, Raval U, Pasinetti GM. Potential novel role of COVID-19 in alzheimer’s disease and preventative mitigation strategies. J Alzheimer’s disease: JAD (2020) 76(1):21–5. doi: 10.3233/JAD-200537
86. Poloni TE, Moretti M, Medici V, Turturici E, Belli G, Cavriani E, et al. COVID-19 pathology in the lung, kidney, heart and brain: The different roles of T-cells, macrophages, and microthrombosis. Cells (2022) 11(19):3124–54. doi: 10.3390/cells11193124
87. Gupta M, Weaver DF. COVID-19 as a trigger of brain autoimmunity. ACS Chem Neurosci (2021) 12(14):2558–61. doi: 10.1021/acschemneuro.1c00403
88. Balli N, Kara E, Demirkan K. The another side of COVID-19 in alzheimer’s disease patients: Drug-drug interactions. Int J Clin practice (2020) 74(10):e13596. doi: 10.1111/ijcp.13596
89. Ou X, Liu Y, Lei X, Li P, Mi D, Ren L, et al. Characterization of spike glycoprotein of SARS-CoV-2 on virus entry and its immune cross-reactivity with SARS-CoV. Nat Commun (2020) 11(1):1620. doi: 10.1038/s41467-020-15562-9
90. Wei HF, Anchipolovsky S, Vera R, Liang G, Chuang DM. Potential mechanisms underlying lithium treatment for alzheimer’s disease and COVID-19. Eur Rev Med Pharmacol Sci (2022) 26(6):2201–14. doi: 10.26355/eurrev_202203_28369
91. Skalny AV, Aschner M, Tinkov AA. Zinc. Adv Food Nutr Res (2021) 96:251–310. doi: 10.1016/bs.afnr.2021.01.003
92. Song X, Tan L, Wang M, Ren C, Guo C, Yang B, et al. Myricetin: A review of the most recent research. Biomedicine pharmacotherapy = Biomedecine pharmacotherapie (2021) 134:111017. doi: 10.1016/j.biopha.2020.111017
93. Kempuraj D, Thangavel R, Kempuraj DD, Ahmed ME, Selvakumar GP, Raikwar SP, et al. Neuroprotective effects of flavone luteolin in neuroinflammation and neurotrauma. BioFactors (Oxford England) (2021) 47(2):190–7. doi: 10.1002/biof.1687
94. Zalpoor H, Akbari A, Samei A, Forghaniesfidvajani R, Kamali M, Afzalnia A, et al. The roles of eph receptors, neuropilin-1, P2X7, and CD147 in COVID-19-associated neurodegenerative diseases: inflammasome and JaK inhibitors as potential promising therapies. Cell Mol Biol letters (2022) 27(1):10. doi: 10.1186/s11658-022-00311-1
95. Lubbe L, Cozier GE, Oosthuizen D, Acharya KR, Sturrock ED. ACE2 and ACE: structure-based insights into mechanism, regulation and receptor recognition by SARS-CoV. Clin Sci (London England: 1979) (2020) 134(21):2851–71. doi: 10.1042/CS20200899
Keywords: Alzheimer’s disease (AD), COVID-19, epidemiological studies, mechanism, inflammation and immune responses
Citation: Li W, Sun L, Yue L and Xiao S (2023) Alzheimer’s disease and COVID-19: Interactions, intrinsic linkages, and the role of immunoinflammatory responses in this process. Front. Immunol. 14:1120495. doi: 10.3389/fimmu.2023.1120495
Received: 12 December 2022; Accepted: 20 January 2023;
Published: 09 February 2023.
Edited by:
Mario Clerici, University of Milan, ItalyReviewed by:
Shetty Ravi Dyavar, Adicet Bio, Inc, United StatesEgidia Miftode, Grigore T. Popa University of Medicine and Pharmacy, Romania
Copyright © 2023 Li, Sun, Yue and Xiao. This is an open-access article distributed under the terms of the Creative Commons Attribution License (CC BY). The use, distribution or reproduction in other forums is permitted, provided the original author(s) and the copyright owner(s) are credited and that the original publication in this journal is cited, in accordance with accepted academic practice. No use, distribution or reproduction is permitted which does not comply with these terms.
*Correspondence: Ling Yue, bellinthemoon@hotmail.com; Shifu Xiao, xiaoshifu@msn.com
†These authors have contributed equally to this work