- 1College of Pulmonary and Critical Care Medicine, 8th Medical Center of Chinese PLA General Hospital, Beijing, China
- 2Department of Pulmonary and Critical Care Medicine, 7th Medical Center of Chinese PLA General Hospital, Beijing, China
- 3Section of Pulmonary and Critical Care and Sleep Medicine, Yale University School of Medicine, New Haven, CT, United States
An unprecedented global pandemic caused by a novel coronavirus named SARS-CoV-2 has created a severe healthcare threat and become one of the biggest challenges to human health and the global economy. As of July 2023, over 767 million confirmed cases of COVID-19 have been diagnosed, including more than 6.95 million deaths. The S protein of this novel coronavirus binds to the ACE2 receptor to enter the host cells with the help of another transmembrane protease TMPRSS2. Infected subjects that can mount an appropriate host immune response can quickly inhibit the spread of infection into the lower respiratory system and the disease may remain asymptomatic or a mild infection. The inability to mount a strong initial response can allow the virus to replicate unchecked and manifest as severe acute pneumonia or prolonged disease that may manifest as systemic disease manifested as viremia, excessive inflammation, multiple organ failure, and secondary bacterial infection among others, leading to delayed recovery, hospitalization, and even life-threatening consequences. The clinical management should be targeted to specific pathogenic mechanisms present at the specific phase of the disease. Here we summarize distinct phases of COVID-19 pathogenesis and appropriate therapeutic paradigms associated with the specific phase of COVID-19.
1 Introduction
The ongoing COVID-19 pandemic is entering its fourth year which began with the identification of a group of patients with unknown pneumonia in Wuhan, China in December 2019 (1). This is the third major coronavirus outbreak preceded by severe acute respiratory syndrome (SARS) and Middle East respiratory syndrome (MERS), however, both of these viruses were contained before causing the global pandemic. Since the emergence of COVID-19, a significant proportion of the human population has been infected with SARS-CoV-2, the causative virus of COVID-19 (2). The rapid spread of the virus allowed the virus to evolve quickly to become more infectious. Multiple variants of the virus named Alpha, Beta, Gamma, Delta, and Omicron, have emerged during this pandemic and repeated infections have become more common. Many of these variants have much higher infectivity compared to the original strain (3, 4). The continuous evolution of SARS-CoV-2 has caused unprecedented devastating effects on human health and the global economy. As of July 2023, more than 767 million cases of confirmed COVID-19 have been reported and the virus has claimed over 6.95 million lives (5). Beyond the acute disease and death, the COVID-19 pandemic has touched every aspect of life including economic well-being, mental health, and impaired services for other diseases leading to an increase in mortalities due to other diseases (6). Additional impacts include impaired learning in children and stress among adults leading to increased rates of suicide and self-harm (7).
Individuals infected by SARS-CoV-2 present with a wide range of disease severity ranging from asymptomatic to severe disease that leads to death. While in most of the subjects, COVID-19 manifests as a mild flu-like disease, a small but significant number of patients develop severe and often life-threatening diseases. A multitude of factors contributes to the disease severity including viral load, inflammation, and immune response equilibrium. The life-threatening condition occurs due to excessive inflammation and/or impaired viral clearance caused by aging, underlying diseases such as diabetes and hypertension, and many other unknown factors (8, 9). Beyond the acute disease, the chronic consequences of COVID-19 are being documented that include severe life-altering changes such as chronic fatigue, impaired memory, and cognitive functions, among others (10, 11).
Significant progress has been made in our understanding of the transmission mechanisms, preventive measures, vaccinations, and therapeutic approaches to treat COVID-19. Despite these advances, COVID-19 remains one of the foremost healthcare challenges with significant morbidity, mortality, and economic costs each day across the world. Further, the continuous emergence of novel strains threatens our progress regarding both preventative and therapeutic approaches as immune evasion and antiviral resistance are likely consequences of viral evolution.
The chronic sequelae of COVID-19, known as long COVID occurs in at least 10% of the infected individuals and poses a global health challenge (12, 13). More than 200 symptoms have been documented affecting multiple organ systems, with many patients experiencing multiple symptoms simultaneously, affecting their quality of life including the ability to return to work (14, 15). Unfortunately, no validated effective treatments currently exist. This review provides updated insights into the pathophysiology of COVID-19 and available therapeutic avenues to treat this deadly disease, with the aim of reducing COVID-19 incidence, hospitalization, mortality, and long COVID.
2 Pathophysiology
2.1 Interactions and entry of the SARS-CoV-2 into the cell
Confirmed COVID-19 patients with symptoms and asymptomatic carriers are the primary source of new infections (16). In addition to the respiratory droplets and contact with contaminated surfaces, infection by fecal-oral route has been speculated (17). When SARS-CoV-2 initially infects people, the viral spike (S) protein binds to the angiotensin-converting enzyme 2(ACE2) receptor, which mediates the entry of SARS-CoV-2 into host cells such as nasal, bronchial epithelial cells and pneumocytes (18). The binding affinity of the S protein of SARS-CoV-2 with ACE2 is 10-20 folds higher than that of SARS-CoV, potentially explaining the quick spread of this pandemic (19). S protein undergoes further priming by type 2 transmembrane serine protease (TMPRSS2), a cellular protease particularly present in alveolar epithelial type II cells, which promotes viral uptake and coronavirus entry. Generally, the ACE2 receptor is expressed in multiple tissue cells, including airways, cornea, esophagus, ileum, colon, liver, gallbladder, heart, kidney, and testis. TMPRSS2 expression has an even broader distribution implicating that ACE2, rather than TMPRSS2, may be a limiting and major factor for viral entry at the early stage of the infection (20, 21). Notably, ACE2 and TMPRSS2 can be targeted for drug intervention to prevent the invasion and transmission of SARS-CoV-2 in host cells (22, 23). To further explain the mechanism of viral entry into host cells, the binding of the S protein to ACE2 in the viral entry process involves several stages:
Attachment: The S protein of SARS-CoV-2 binds to the ACE2 receptor on the surface of the host cell.
Priming: The S protein is then cleaved by a host protease enzyme called TMPRSS2. This cleavage allows the S protein to undergo a conformational change, exposing a fusion peptide that facilitates the fusion of the viral membrane with the host cell membrane.
Fusion: The viral membrane fuses with the host cell membrane, allowing the viral genetic material (RNA) to enter the host cell.
Replication: Once inside the host cell, the viral RNA is used as a template to produce more viral proteins and RNA.
Assembly and Release: The newly produced viral proteins and RNA assemble into new viral particles, which are then released from the host cell to infect other cells. Overall, the binding of the SARS-CoV-2 S protein to the ACE2 receptor on host cells plays a crucial role in the viral entry process and the subsequent development of COVID-19. Understanding the molecular mechanisms underlying this process is crucial for developing effective strategies to prevent and treat COVID-19 (15–18). More recent evidence has indicated ACE2 independent entry of SARS-CoV-2 into the cells, especially in the immune cells (24). The pathological consequences of ACE2-independent entry of the SARS-CoV-2 into host cells are not completely understood. Further, it remains unclear if the extra-pulmonary manifestations such as multi-organ failure are direct consequences of viral infection to those tissues or a consequence of host inflammatory response.
2.2 Early stage of infection
Upon entry into cells in the upper respiratory tract, SARS-CoV-2 starts to replicate and propagate in the nose and upper airways. Although infected subjects may remain asymptomatic at this stage, they are highly infectious with a high viral load, which begins to peak around symptom onset (25, 26). Subsequently, the virus may migrate from the nasal epithelium to the upper respiratory tract via the ciliated cells in the conducting airways (18, 27). The infected individuals can shed viral particles by not only coughing or sneezing but also during their day-to-day activities such as talking, eating, and even exhaling. Pre-symptomatic transmission is considered a significant contributor to viral transmission responsible for 9.1%-62% of positive cases which vary from different literatures among different populations (25, 28, 29).
The viral transmission also occurs during the symptomatic disease and may even continue after symptoms are solved (30). Infected individuals manifest the symptoms of fever, malaise, cough, and sputum production at the early stage. The host mounts an innate response that is mediated by cytokines and antiviral interferons and initiates the adaptive immune response. If the host is able to mount a strong interferon-mediated response at this stage, such as seen in children and adults, they may control the viral replication and limit the disease severity at this stage (26, 31, 32). The precise mediators of early viral clearance are not yet completely understood but given the potent antiviral activity and robust upregulation in those with a mild disease show a critical role of interferons in viral elimination (Figure 1) (32, 33).
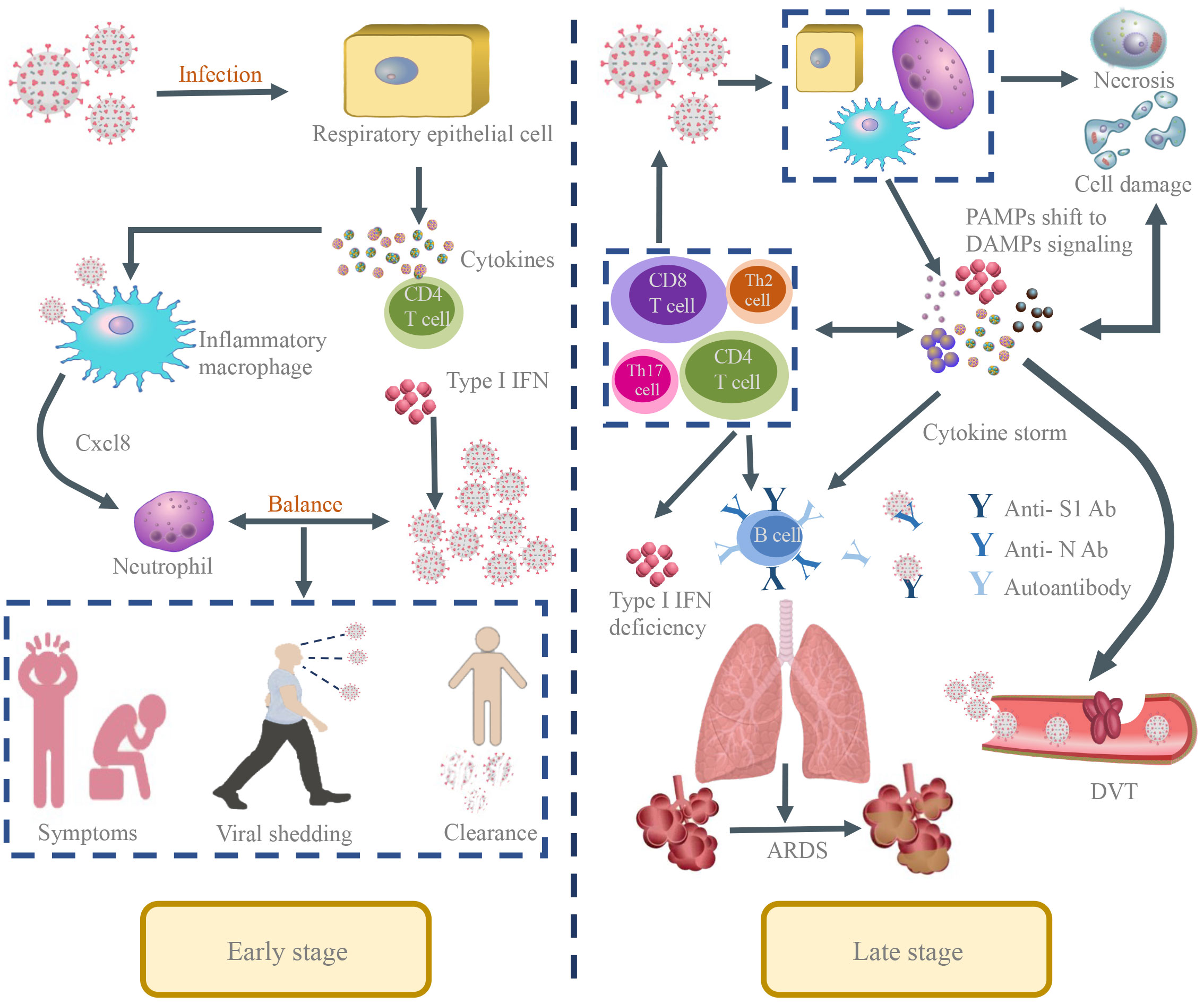
Figure 1 The pathological manifestations of SARS-CoV-2 infection in the human body. Early stage: In the early stage of SARS-CoV-2 infection, the respiratory epithelial cells were targets of the virus and the virus begins to replicate and spread in the nose and upper respiratory tract. The host mounts an innate response mediated by cytokines and antiviral interferons that initiates the adaptive immune response. In the early stages, infected people show the symptoms of fever, malaise, cough and sputum production and can transmit viruses to other people. If the host can mount a strong interferon-mediated response at this stage and appropriate regulation of the host immunity relative to the viral burden, viral replication would be arrested and viral clearance initiated to limit disease severity at this stage. Late stage: If the virus can’t be eradicated in a timely manner likely due to a delayed PAMP-mediated inflammatory/interferon response, the immune response would shift to a nonspecific inflammatory reaction dominated by damage-associated molecular pattern (DAMP) signaling emanating from damaged or dysfunctional virus-infected host cells. Immune cells including CD4 helper T cells, and CD8 cytotoxic T cells are sequestered in the lung tissue. The host cells undergo persistent apoptosis or necrosis or pyroptosis that may amplify the tissue damage. In addition, the inflammatory environment triggers the expression of activated tissue factor on endothelial cells, macrophages, and neutrophils, thereby enhancing activation of the coagulation cascade in the lungs, causing microcirculatory thrombi and ARDS and increasing disease severity and mortality.
2.3 Late stage of infection
Subjects that fail to eradicate the virus in its early stage, may progress to the clinical phase or later stage of the infection, which is manifested by COVID-19 symptoms that may vary in severity and duration (34). It is estimated that 1/5th of the infected patients progresses to the involvement of the lower respiratory tract that involves infection to the alveolar epithelial type II cells, developing severe symptoms like acute respiratory distress syndrome (ARDS), disseminated intravascular coagulation (DIC), and pulmonary embolism. The clinical phase of SARS-CoV-2 can be characterized into three distinct phases: acute or pneumonia phase, viremia phase, and lethal/recovery phase.
The acute phase is characterized by pulmonary disease that is manifested by pulmonary symptoms such as dyspnea, cough, and sputum production with imaging evidence of ground-glass opacity or consolidation in the lung. Diffuse alveolar damage, desquamation of pneumocytes, and hyaline membrane formation are observed during the development of ARDS in COVID-19 (35). The increased permeability of the lung vasculature impairs oxygen diffusion and contributes to the fatal disease. Factors contributing to lung permeability during COVID-19 are multifactorial and may include 1. Direct cytopathic effects of coronavirus in infected endothelium resulting in widespread endothelialitis (36). 2. The reduction of ACE2 activity by SARS-CoV-2 and subsequent increase in angiotensin indirectly boosting the kallikrein-bradykinin pathway which promotes vascular permeability (37). 3. Inflammatory cytokines and vasoactive mediators secreted by immune cells such as activated neutrophils induce the contraction of endothelial cells and loosen endothelial tight junctions. 4. Glycocalyx degradation and hyaluronic acid deposition in the extracellular matrix promote fluid retention (38). The increased lung vascular permeability results in impaired lung function that manifests into decreased blood oxygenation, a marker of disease severity.
The viremic phase begins when the virus enters the peripheral blood. The molecular mechanisms of viremia in COVID-19 are still poorly understood, however, ACE2 independent entry of the virus in peripheral monocytes has been shown to promote pyroptotic cell death and disease severity (24). The viremia and subsequent host response contribute to multiple systemic inflammation and multiorgan failure. The inflammatory response during severe COVID-19 is mediated by a simultaneous increase in the multiple inflammatory cytokines such as IL-1α, IL-1β, IL-6, IL-8, IL-12, IL-17), TNF- α, interferons (IFN- β, IFN- λ), MCP-1, and MIP-1 α, making it difficult to pinpoint the specific mediator of inflammatory response (39). Additionally, the early inflammatory response may help the host to limit viral replication, further complicating the role of cytokines in COVID-19. It is not surprising that therapies targeting specific cytokines such as IL-6 or TNF- α led to mixed results (40, 41).
The lethal phase is mediated by a persistent disease that is manifested both locally and systemically. The inflammatory response in the form of cytokine storm and coagulation factors is significantly elevated in severe patients compared to non-severe patients (42–44). In this phase, neutrophils, CD4 helper T cells, and CD8 cytotoxic T cells are sequestered in the lung tissue (45). The host cells undergo persistent apoptosis, necrosis, or pyroptosis, which may amplify the tissue damage. In addition, the inflammatory environment triggers the expression of activated tissue factor on endothelial cells, macrophages, and neutrophils, enhancing activation of the coagulation cascade in the lungs (46). Markers of the coagulation pathway such as upregulation of D-dimer are clearly visible at this stage. In an autopsy study, Wichmann et al. demonstrated that 58% of COVID-19 patients have a concurrency of DVT, and 1/3 of the deaths were directly caused by pulmonary embolism (PE) (47). The potential role of thrombosis in pulmonary veins distal to the alveolar capillary bed, which should act as clot filters, has been pointed out, suggesting that it could be SARS-CoV-2-related vasculitis responsible for ischemic manifestations in various organs (48). For this reason, severe COVID-19 is not restricted to the respiratory system but is a multisystem disease including the development of various cardiovascular manifestations with myocardial injury, arrhythmia, acute coronary syndrome, and venous thromboembolism. These manifestations are closely related to the disease severity and progression to lethal disease (49). Based on all these features, anticoagulant therapy and immunomodulatory agents are probably necessary to attenuate the hyperinflammatory and prothrombotic states (50). These patients may benefit from immune modulators such as steroids while antiviral agents have limited utility at this stage of the disease. Despite the obvious contribution of coagulation pathways in vascular disease, the use of anticoagulant therapy may be filled with the risk of increased bleeding. On the other hand, microcirculatory thrombi in capillaries and large vessels may already cause extensive damage if administered too late.
2.4 The complexity of immune response during COVID-19
Exacerbated immune response manifested as cytokine storm is a common pathological event in many infectious diseases. However, given the wide range of clinical presentations and extensive studies performed on COVID-19, a clearer picture of beneficial and pathological immune responses started to emerge. Host immune response, initiated by sensing the pathogen-associated molecular patterns (PAMP) present on the pathogen strives to eliminate the invading pathogen. A multitude of evidence suggests that the initial host response is dampened markedly in severe COVID-19 patients, especially those mediated by type I interferon (51, 52). Type I IFNs (IFN- α, IFN- β, IFN- ω) are indispensable in viral clearance, and this impairment is associated with high blood viral load and exacerbated inflammatory response (33). Additionally, the nucleocapsid (N) protein, one of the four structural proteins of CoV, serves as an antagonist of IFN, which appears to be beneficial for viral replication (53, 54). It was speculated that a large spectrum of severe clinical presentations could result from a delayed host response towards a specific pathogen-associated molecular pattern (PAMP), which attenuates the antiviral innate immunity required to eliminate the pathogen. In support of this hypothesis, treatment with type I interferon has shown beneficial effects in COVID-19 (55).
Moreover, the postponement of the PAMP-mediated response shifts the immune response to a nonspecific inflammatory reaction dominated by damage-associated molecular pattern (DAMP) signaling emanating from damaged or dysfunctional virus-infected host cells (56, 57). Although PAMPs and DAMPs can lead to innate and adaptive immune responses, the latter raises additional damage and dysfunction by releasing various pro-inflammatory cytokines by activating dendritic cells and other antigen-presenting cells (APCs). DAMPs initiate innate immune activation and systemic inflammation, which could further upregulate itself by triggering a cytokine storm, providing positive feedback to tissue destruction. It is not surprising that young subjects who remained asymptomatic post-SARS-CoV-2 infection had elevated levels of inflammatory cytokines such as IL-2 while decreasing levels of the anti-inflammatory cytokine IL-10 (57). The current clinical challenge remains to identify and distinguish the DAMP-driven immune response from that driven by PAMPs.
Similar to the innate immune response, the adaptive immune response to SARS-CoV-2 infection has been studied in detail. Overall, it appears that the ability of the host to generate an early humoral response rather than mounting a stronger humoral response is critical in protecting the host against severe disease. Both delayed onset of the humoral response and elevated antibody titers are associated with severe disease in COVID-19 (58, 59). To support this hypothesis, we have shown that the early onset of antibody response was associated with asymptotic disease and patients with severe disease had elevated antibody response (60, 61). Along similar lines, convalescent plasma therapy provided limited benefits in COVID-19, leading the World Health Organization to issue guidelines against plasma therapy for COVID-19 (62).
2.5 Long COVID
Despite the widespread prevalence of long COVID-19, the mechanistic understanding of underlying pathophysiology remains limited. There are several suggested hypotheses for the pathogenesis of long COVID-19 including the persistent exposure of SARS-CoV-2 in tissues, dysregulation of the immune system, reactivation of secondary pathogens (eg. EBV, HHV-6, HCMV, VZV etc.), microbiome dysbiosis in the gastrointestinal system, autoimmunity and immune priming from molecular mimicry, microvascular blood clotting with endothelial abnormalities, and dysfunctional neurological signaling, among others (63–66). Increasing evidence have shown that two third of the population with long COVID is associated with multiple potential risk factors and pre-existing conditions, including female sex, type 2 diabetes, underlying virus reactivation, the presence of specific autoantibodies, and connective tissue disorders (67–69). A higher prevalence of long COVID-19 has been reported in certain ethnicities, including people with Hispanic or Latino heritage (13). Lower income and lack of sufficient rest in the early weeks after SARS-CoV-2 infection are associated with long COVID-19, which is characterized by postexertional malaise, postural orthostatic tachycardia syndrome, pain, fatigue, unrefreshing sleep, brain fog, cognitive dysfunction, gastrointestinal symptoms, neurological symptoms, among others (70, 71).
3 Clinical management
3.1 Prevention and self-protection
The origin of SARS-CoV-2 including the animal host still needs definitive identification (72). Most of the infections occur through human-to-human contact, either directly through droplets emitted from infected objects or indirectly by aerosols suspended in the air (73). Preventive measures such as mask/respirator use are highly effective if used appropriately, although the quality of the mask/respirator is critically important. Although infections in healthcare workers have been reported, but larger outbreaks in the healthcare workers have been rare, even before the availability of vaccines, due to the efficacy of personal protective equipment. A nationwide cross-sectional study in Bangladesh has proved that adequate preventive health measures were associated with a lower risk of infection and death from COVID-19 (74). Among preventive health measures, washing/cleaning hands with soap or hand sanitizer (OR: 0.17, 95% CI: 0.09–0.41), wearing masks properly (OR: 0.02, 95% CI: 0.01–0.43), avoiding crowded places (OR: 0.07, 95% CI: 0.02–0.19), and maintaining social distancing in public places (OR: 0.04, 95% CI: 0.01–0.33), were significantly associated with the reduced number of cases and deaths (74).
3.2 Early isolation and treatment settings
Early isolation and appropriate duration of quarantine are required to stop or slow down the spread of COVID-19 or its newly emerging variants. However, given the ubiquitous spread of COVID-19, the availability of vaccines, effective therapeutic agents, and an overall decrease in the severe disease by new variants, most countries are shifting their focus away from extensive quarantine and isolation of suspected individuals. However, the experience from this pandemic should guide us in future outbreaks to limit both the disease spread and better deal with the social and economic costs associated with the extensive quarantine.
3.3 Vaccines
Vaccines are vital cost-effective tools to prevent the disease and limit the disease severity during the COVID-19 pandemic. Effective vaccine plays a critical role in preventing the viral spread and limiting the disease severity (75). Multiple vaccines are currently available including adenovirus vector vaccines, mRNA, inactivated, and subunit vaccines. The initial data shows that among individuals ≥ 18 years of age, adenoviral vector vaccines were 73% (95% CI = 69- 77) effective and messenger RNA (mRNA) vaccines were 85% (95% CI = 82- 88) (76). Existing adenovirus, mRNAs, and inactivated vaccines can elicit significant immune responses against SARS-CoV-2 RBDs in vaccinated recipients, and individuals develop neutralizing antibodies against the specific area within 30 days of the first and second doses of the vaccine (77). However, the efficacy figures of these vaccines are evaluated within the first 6 months post-vaccination. The immunity gradually decreases over time, and breakthrough infections became common. In addition, mutations in the SARS-CoV-2 can subvert the immunity to cause infections in vaccinated subjects. Boosting host immunity with additional doses of vaccines is effective in limiting disease severity and death, especially among older patients. The beneficial effects of boosters among the young subjects are difficult to decipher given low hospitalizations and mortality in these subjects and potentially due to strong immune response to initial vaccine doses. However, in patients with carcinomas and other immunosuppressive diseases, currently available vaccines have shown to trigger sufficient immune response (78, 79). Further, variant-specific vaccines have been recently developed and approved, however, the precise supremacy of variant-specific vaccines in real-life settings is yet to be determined.
3.4 Diagnostic evaluation
Reliable clinical or laboratory parameters are of great importance to accurately distinguish between COVID-19 and respiratory infections of other origins. Individuals with typical respiratory symptoms such as fever, cough, and myalgia should be tested by respiratory nucleic acid amplification test (NAAT) through the specimen from bronchoalveolar lavage, sputum, and nasopharyngeal swab using real-time fluorescence polymerase chain reaction with reverse transcription (RT-PCR). Since false-negative results occur in the circumstance of low viral load in the initial screening, repeated testing should be performed if the symptoms continue to persist. Other measures such as computed tomography (CT) examination can be performed for the auxiliary diagnosis of COVID-19 which is manifested as patchy or segmental GGOs (93.3%) and reticular markings distributed by peribronchovascular and subpleural (80). Although limitations exist when some imaging signs of COVID-19 were presented as the same as those of other lung diseases. Chest CT is easy to perform and readily available to quickly detect lung lesions and make imaging diagnoses at an early stage. Particular attention should be paid to the final confirmation of a SARS-CoV-2 infection. In addition, patients with fever in the emergency department (ED) should be monitored for the presence of SARS-CoV-2 (81). While nucleic acid-based tests or antigen detection tests are used for diagnostic purposes, antibody detection tests may be used to assess the overall exposure in the population (82).
3.5 Therapy and clinical management
The clinical management and target of currently used therapeutics against SARS-CoV-2 are demonstrated (Tables 1, 2) (96). The clinical management of COVID-19 involves a wide range of antiviral and immune modulatory drugs that are described below (Figure 2).
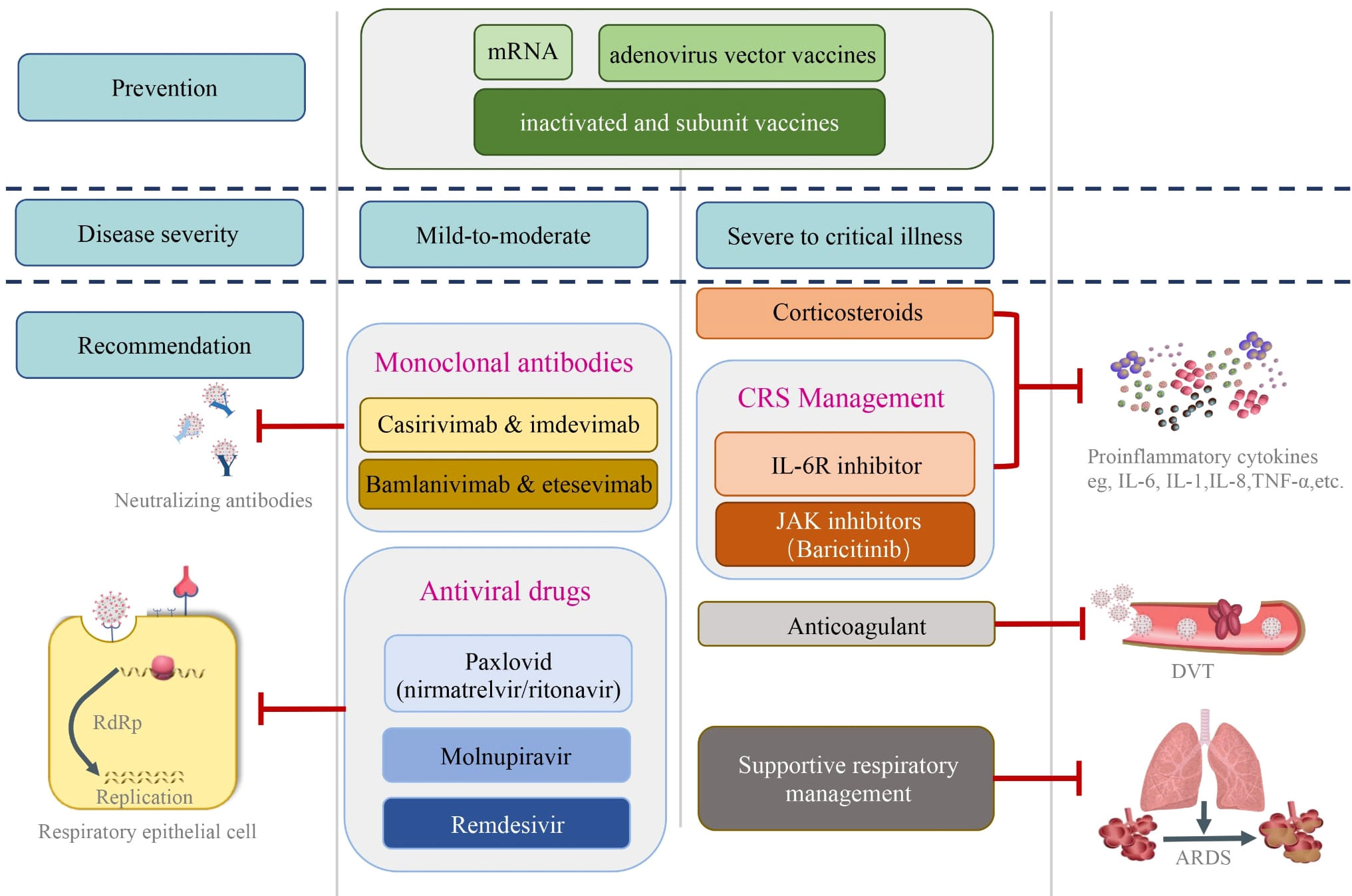
Figure 2 The clinical management and target of currently used therapeutics against SARS-CoV-2. Prior to clinical infection with coronavirus, effective vaccines including adenovirus vector vaccines, mRNA, inactivated, and subunit vaccines play critical roles in preventing virus spread and limiting the severity of the infection. The clinical management and target of currently used therapeutics against SARS-CoV-2 are shown based on the disease severity. Monoclonal antibodies including casirivimab & imdevimab and bamlanivimab & etesevemab have been used in non-severe cases. Likewise, antiviral drugs such as Paxlovid (nirmatrelvir & ritonavir), molnupiravir and remdesivir, which target the virus replication in the infected cells have been used in mild-to-moderate patients. For severe to critically illness, corticosteroids and CRS management including IL-6R inhibitor and JAK inhibitors were administrated to control the excessive inflammation. Anticoagulants have been used to prevent and treat DVT. Supportive respiratory managements have been primary measures to treat patients with low blood oxygenation including ARDS. DVT, deep venous thrombosis; ARDS, acute respiratory distress syndrome.
3.5.1 Antiviral drugs
In the last four years, aggressive research allowed the development of novel antiviral agents in addition to repurposing other antiviral molecules. These agents vary in efficacy and adverse effect profile. Some of the currently available agents are the following:
3.5.1.1 Remdesivir
Remdesivir was originally developed as an antiviral agent against Ebola, however, the clinical trials failed to show any effectiveness in reducing the mortality (83). The mechanism by which remdesivir acts against viruses is by acting as a nucleoside analog and inhibiting RNA-dependent RNA polymerase, which elicits the delay of the chain termination in the replication of the RNA genome (97). The drug was then tested against SARS-CoV-2, where it was found to have potent antiviral effects during in vitro testing (98). These studies led to its approval for emergency use in COVID-19. The initial clinical studies show the clinical benefit of remdesivir in COVID-19 (84). This led to WHO recommending the use of remdesivir for patients without severe or critical illness in April 2022. Subsequent randomized clinical trials failed to show any beneficial effects of remdesivir regardless of the severity of the disease (62, 83, 85). This prompted WHO to recommend against the use of remdesivir in COVID-19 regardless of disease severity (62).
3.5.1.2 Molnupiravir
Molnupiravir is a ribonucleoside prodrug of N-hydroxycytidine (NHC) that effectively inhibits RNA viruses including SARS-CoV-2 (99). Large-scale clinical trials have shown its beneficial effects during COVID-19 including decreasing hospitalizations and mortality (86). Unlike remdesivir, this drug can be used orally, allowing it to be administered early in high-risk subjects. Subsequent studies have confirmed the beneficial findings, but the beneficial effects were limited to a 30% decrease in mortality due to COVID-19 (100, 101). Another major concern is its efficacy against novel variants. Initial data show the clinical benefit of molnupiravir against alpha and beta variants, however, limited clinical data are obtained that molnupiravir exerts activity against delta and omicron variants (86, 102, 103). Recent evidences have indicated the potential benefit of molnupiravir against the omicron variant (104).
3.5.1.3 Paxlovid
Paxlovid is one of the most effective and orally available anticoronaviral medication against SAR-CoV-2. Paxlovid is a combination of nirmatrelvir and ritonavir. Nirmatrelvir targets the main polyprotein protease enzyme of SARS-CoV-2 in the replication cycle, dramatically decreasing the viral loads (105). Ritonavir is a protease inhibitor and a CYP3A4 antagonist, inhibiting nirmatrelvir breakdown and enhancing its pharmacokinetics (106). A double-blind, randomized, controlled trial with 2246 patients who received paxlovid (300mg of nirmatrlvir and 100mg of ritonavir) showed 89% lower incidence of hospitalization and deaths attributed to any cause within 28 days along with decreased viral load (87). Moreover, no obvious safety concerns were observed besides mild generic side effects such as bitter aftertaste, diarrhea, and fatigue. However, one should be careful about drug-drug interactions and should not be co-administrated with other medicines that are metabolized by CYP3A4. Further, this is not indicated in pregnant or breastfeeding patients.
3.5.2 Monoclonal antibodies
Neutralizing antibodies targeting the spike protein of the coronavirus is another important pillar in the fight against SARS-CoV-2 infection, especially in immunocompromised patients (107). Klank et al. reported that monoclonal antibodies (mAbs) such as Bamlanivimab can be used in both preventive and treatment settings (108). In addition, casirivimab and imdevimab also showed a positive protective effects, as SARS-CoV-2 negative individuals who received the specific mAbs appear to have a lower risk of developing COVID-19 after contact with an infected individual (1.5% versus 7.8%; P<0.001). Further, the symptom duration of patients suffering from COVID-19 was shown to be shorter than that of patients on placebo (109). Given these promising protective effects, a combination of bamlanivimab and etesevimab could be a potential treatment option for immunocompromised patients. In a phase 2/3 study, a combination of bamlanivimab and etesevimab was given to patients with malignancies or to those who were in an immunosuppressive status with COVID-19 (88). Compared to the placebo group, patients receiving the drug showed a lower hospitalization rate (2.1% vs. 7.0%; p<0.001) and a significant reduction in viral load at day 7. Other commercially available mAbs such as casirivimab and imdevimab have also shown a positive effect in protecting against reducing viral loads and hospitalization rates and thereby reducing mortality (89, 90). mAbs probably have the greatest beneficial effects in the early phase of coronavirus infection, where viral replication plays an important role (110, 111). Serious adverse effects, including allergic reactions and cardiac issues such as atrial fibrillation, have occurred and require caution following mAbs infusion (91).
In general, monoclonal antibody therapy is recommended for patients with mild to moderate COVID-19 who are at high risk for disease progression. High-risk factors include older age, obesity, diabetes, chronic kidney disease, or immunosuppression. When it comes to the choice of dose, the specific monoclonal antibody and the patient’s weight are taken into consideration. For example, for the monoclonal antibodies bamlanivimab and etesevimab, the recommended dose is 700mg bamlanivimab and 1400mg etesevimab administered together intravenously for patients weighing at least 40 kg (83, 97). The decision to use monoclonal antibodies and the choice of dose should be made by a healthcare professional based on the individual patient’s clinical situation.
3.5.3 Management of cytokine release syndrome
A number of therapies targeting a specific or broad range of cytokines have been tested in COVID-19. Here we describe major approaches to reduce the cytokine storm to alleviate the disease severity.
3.5.3.1 IL-6 receptor blocker
Cytokine storm responses pose a significant risk in infectious diseases including COVID-19. IL-6 is a pleiotropic cytokine stimulating and regulating the immune response during infections. Since IL-6 is identified as the key propagator of the cytokine storm reaction, blocking the two forms of the IL-6 receptor, a membrane-bound and a soluble IL-6 receptor was considered. IL-6 receptor blockers, including tocilizumab and sarilumab, are recommended to be used intravenously for severe or critical illness patients of COVID-19 based on evidence of mortality reduction and decreased requirement of mechanical ventilation (112, 113). However, this is only effective in those with severe disease.
3.5.3.2 Janus (JAK) kinase inhibitors
Janus kinases are important mediators of cytokine storm and inflammation and serve as a potential target for limiting cytokine storm during COVID-19. Baricitinib, ruxolitinib and tofacitinib are three major JAK inhibitors tested for severe or critical COVID-19 patients (92, 93, 114). The three JAK blockers are considered nonspecific despite evident differences. Baricitinib is mainly described as a JAK1/2 inhibitor while ruxolitinib also presents the weak suppression of TYK2 except from JAK1/2, while tofacitinib showed more inhibitory potential on JAK1/3 than JAK2/TYK2 (115, 116). Although large randomized clinical trials have been limited, our meta-analysis demonstrated the overall beneficial effects of JAK inhibitors in COVID-19 (55).
3.5.4 Systemic corticosteroids
Systemic corticosteroids are generally recommended in treating severe and critical COVID-19 patients to control the over-activated inflammatory response despite confounding results (117, 118). Patients younger than 70 years with persistent symptoms for more than seven days and who required mechanical ventilation are shown to benefit from dexamethasone therapy (119). In contrast, no clinical improvement was observed in patients with a shorter duration of symptoms and without supplemental oxygen, even in COVID-19-induced mild to moderate ARDS (120). The optimal timing of the therapy from symptom onset is still a matter of debate. Theoretically, it can be speculated that systemic glucocorticoid use should be avoided until viral replication is under control by the immune system or through effective antivirals. The consensus is arising that corticosteroids should be administered in patients with severe or critical COVID-19, even within seven days of symptoms onset, and non-severe cases should not be treated with corticosteroids even though symptoms occur longer than a week (62).
3.5.5 Convalescent plasma therapy
Convalescent plasma therapy is one of the experimental treatments for SARS-CoV-2 infection. The antiviral neutralizing antibodies collected from the plasma of recovered patients are transfused into the COVID-19 patients with an active infection to enhance the immune response (94, 95, 121). Such plasma therapy has shown a promising recovery rate in H5N1 influenza and Ebola viral disease (122–124). However, due to different methodologies, appropriate antibody titers ranging from 1:100 to 1:2560 have been reported in the existing studies from donor to recipient (125, 126). The range is confusing and the uncertainty of the definitive dose lies in the different limit values (127, 128). WHO living guidelines recommend this therapy applied to patients with severe illness, but only in research settings or clinical trial, for the reasons that convalescent plasma therapy has no significant effect on the indicators such as time to symptom improvement, length of hospital stay, duration of mechanical ventilation, or mortality (62, 129).
3.5.6 Supportive respiratory management
Mechanical ventilation and supplemental oxygen are the primary measures for addressing those who present with low blood oxygenation. High flow nasal cannula (HFNC), Non-invasive ventilation (NIV), and lung-protective invasive mechanical ventilation (IMV) are widely used to support respiration (130, 131). Given the rapid decompensation due to early intubation strategies, HFNC was selected early in the pandemic and used in the populations with hypoxemic respiratory failure and patients often respond well (132, 133). However, HFNC and other NIV devices, including non-invasive bi-level positive pressure ventilation (BPAP) and continuous positive airway pressure (CPAP), may pose a potential risk of aerosolization of the virus. For further safety in HFNC use, evidence has supported the use of a surgical mask (134). CPAP via a mask covering the mouth and nose or helmet NIV is recommended to minimize room air contamination and to better contain aerosol leakage, providing superior oxygenation, pressure, and outcomes (135). In addition, it is recommended that negative pressure rooms be used to reduce the risk of the coronavirus spread in the ambient air (45). A large, international systematic review and meta-analysis was conducted to examine global case fatality rate (CFR) reports in adult patients with COVID-19 who received invasive mechanical ventilation (IMV). The results showed that the overall estimate for the initial CFR in IMV was 45%. Reported CFR was higher in elderly patients and in early pandemic epicenters, which may be impacted by limited ICU resources (136). However, IMV is used for crucial supportive care and to provide additional time for patients with severe hypoxemic respiratory failure in the ICU. Further, invasive mechanical ventilation may increase the risk of secondary bacterial infections in COVID-19 patients by either directly breaching the host defense or through coronavirus-impaired host immunity (137).
4 Conclusion
Our understanding of COVID-19 pathology, clinical management, and treatments has improved significantly in the last four years. However, the persistent emergence of variants poses a serious challenge to the effectiveness of both preventive and therapeutic approaches. The clinical management of these infections faces several problems, including failure to administer early antiviral agents, high false-negative diagnosis rates, mixed reports on certain therapeutic drug efficacy, and rapid progression to severe conditions such as ARDS, pulmonary embolism, disseminated intravascular coagulation, sepsis, and cytokine storm. The global fight against COVID-19 is likely to continue for a long time until we develop effective and clinically proven antiviral therapeutics or vaccines that completely prevent transmission of the disease. Further, as we emerge from the pandemic, special emphasis should be given to the chronic consequences such as long COVID-19, which may become a serious healthcare challenge in upcoming years.
Author contributions
YZ wrote the initial draft, prepared the figures, and tables. LS and DC edited the manuscript and provided guidance. All authors contributed to the article and approved the submitted version.
Funding
This work was supported by funding from The National Key Research and Development Program of China (DC; No.2021YFC2302300), Three Talents One Team Project of The Joint Logistic Support Force (DC; 2021-439), Beijing Nova Program Interdisciplinary Cooperation Project (DC; No. 20220484197), Three and One Innovation Talents from Chinese PLA General Hospital (DC; No. 20230315, The grant of Eight Medical Center of Chinese PLA General Hospital (DC; NO. YQ202211001, No MS202211014, the National Natural Science Foundations of China (No. 81700069), and Major Project of Eighth Medical Center of Chinese PLA General Hospital (No.2021ZD005).
Conflict of interest
The authors declare that the research was conducted in the absence of any commercial or financial relationships that could be construed as a potential conflict of interest.
Publisher’s note
All claims expressed in this article are solely those of the authors and do not necessarily represent those of their affiliated organizations, or those of the publisher, the editors and the reviewers. Any product that may be evaluated in this article, or claim that may be made by its manufacturer, is not guaranteed or endorsed by the publisher.
References
1. Zhou F, Yu T, Du R, Fan G, Liu Y, Liu Z, et al. Clinical course and risk factors for mortality of adult inpatients with COVID-19 in Wuhan, China: a retrospective cohort study. Lancet (2020) 395(10229):1054–62. doi: 10.1016/S0140-6736(20)30566-3
2. Zhu N, Zhang D, Wang W, Li X, Yang B, Song J, et al. A novel coronavirus from patients with pneumonia in China, 2019. N Engl J Med (2020) 382(8):727–33. doi: 10.1056/NEJMoa2001017
4. Thakur V, Ratho RK. OMICRON (B.1.1.529): A new SARS-CoV-2 variant of concern mounting worldwide fear. J Med Virol (2022) 94(5):1821–24. doi: 10.1002/jmv.27541
5. Available at: https://covid19.who.int/?mapFilter=cases.
6. Dang A, Thakker R, Li S, Hommel E, Mehta HB, Goodwin JS. Hospitalizations and mortality from non-SARS-coV-2 causes among medicare beneficiaries at US hospitals during the SARS-coV-2 pandemic. JAMA Netw Open (2022) 5(3)::e221754. doi: 10.1001/jamanetworkopen.2022.1754
7. Sher L. The impact of the COVID-19 pandemic on suicide rates. QJM (2020) 113(10):707–12. doi: 10.1093/qjmed/hcaa202
8. Wu Z, McGoogan JM. Characteristics of and important lessons from the coronavirus disease 2019 (COVID-19) outbreak in China: summary of a report of 72 314 cases from the chinese center for disease control and prevention. JAMA (2020) 323(13):1239–42. doi: 10.1001/jama.2020.2648
9. Zheng Z, Peng F, Xu B, Zhao J, Liu H, Peng J, et al. Risk factors of critical & mortal COVID-19 cases: A systematic literature review and meta-analysis. J Infect (2020) 81(2):e16–25. doi: 10.1016/j.jinf.2020.04.021
10. Al-Aly Z, Xie Y, Bowe B. High-dimensional characterization of post-acute sequelae of COVID-19. Nature (2021) 594(7862):259–64. doi: 10.1038/s41586-021-03553-9
11. Nalbandian A, Sehgal K, Gupta A, Madhavan MV, McGroder C, Stevens JS, et al. Post-acute COVID-19 syndrome. Nat Med (2021) 27(4):601–15. doi: 10.1038/s41591-021-01283-z
12. Ceban F, Ling S, Lui LMW, Lee Y, Gill H, Teopiz KM, et al. Fatigue and cognitive impairment in Post-COVID-19 Syndrome: A systematic review and meta-analysis. Brain Behav Immun (2022) 101:93–135. doi: 10.1016/j.bbi.2021.12.020
13. Davis HE, McCorkell L, Vogel JM, Topol EJ. Author Correction: Long COVID: major findings, mechanisms and recommendations. Nat Rev Microbiol (2023) 21(6):408. doi: 10.1038/s41579-023-00896-0
14. Kedor C, Freitag H, Meyer-Arndt L, Wittke K, Hanitsch LG, Zoller T, et al. A prospective observational study of post-COVID-19 chronic fatigue syndrome following the first pandemic wave in Germany and biomarkers associated with symptom severity. Nat Commun (2022) 13(1):5104. doi: 10.1038/s41467-022-32507-6
15. Klein J, Wood J, Jaycox J, Lu P, Dhodapkar RM, Gehlhausen JR, et al. Distinguishing features of Long COVID identified through immune profiling. medRxiv (2022). doi: 10.1101/2022.08.09.22278592
16. Hoehl S, Rabenau H, Berger A, Kortenbusch M, Cinatl J, Bojkova D, et al. Evidence of SARS-coV-2 infection in returning travelers from wuhan, China. N Engl J Med (2020) 382(13):1278–80. doi: 10.1056/NEJMc2001899
17. National Health Commission Of The People's Republic Of China. Protocol for prevention and control of COVID-19 (Trial edition 6). Available at: http://wwwnhcgovcn/yzygj/s7653p/202203/b74ade1ba4494583805a3d2e40093d88shtml.
18. Hoffmann M, Kleine-Weber H, Schroeder S, Kruger N, Herrler T, Erichsen S, et al. SARS-coV-2 cell entry depends on ACE2 and TMPRSS2 and is blocked by a clinically proven protease inhibitor. Cell (2020) 181(2):271–80 e8. doi: 10.1016/j.cell.2020.02.052
19. Wrapp D, Wang N, Corbett KS, Goldsmith JA, Hsieh CL, Abiona O, et al. Cryo-EM structure of the 2019-nCoV spike in the prefusion conformation. bioRxiv (2020). doi: 10.1101/2020.02.11.944462
20. Sungnak W, Huang N, Becavin C, Berg M, Queen R, Litvinukova M, et al. SARS-CoV-2 entry factors are highly expressed in nasal epithelial cells together with innate immune genes. Nat Med (2020) 26(5):681–87. doi: 10.1038/s41591-020-0868-6
21. Zou X, Chen K, Zou J, Han P, Hao J, Han Z. Single-cell RNA-seq data analysis on the receptor ACE2 expression reveals the potential risk of different human organs vulnerable to 2019-nCoV infection. Front Med (2020) 14(2):185–92. doi: 10.1007/s11684-020-0754-0
22. Chen R, Fu J, Hu J, Li C, Zhao Y, Qu H, et al. Identification of the immunodominant neutralizing regions in the spike glycoprotein of porcine deltacoronavirus. Virus Res (2020) 276:197834. doi: 10.1016/j.virusres.2019.197834
23. Zhao Y, Zhao Z, Wang Y, Zhou Y, Ma Y, Zuo W. Single-cell RNA expression profiling of ACE2, the receptor of SARS-coV-2. Am J Respir Crit Care Med (2020) 202(5):756–59. doi: 10.1164/rccm.202001-0179LE
24. Sefik E, Qu R, Junqueira C, Kaffe E, Mirza H, Zhao J, et al. Inflammasome activation in infected macrophages drives COVID-19 pathology. Nature (2022) 606(7914):585–93. doi: 10.1038/s41586-022-04802-1
25. He X, Lau EHY, Wu P, Deng X, Wang J, Hao X, et al. Temporal dynamics in viral shedding and transmissibility of COVID-19. Nat Med (2020) 26(5):672–75. doi: 10.1038/s41591-020-0869-5
26. Parasher A. COVID-19: Current understanding of its Pathophysiology, Clinical presentation and Treatment. Postgrad Med J (2021) 97(1147):312–20. doi: 10.1136/postgradmedj-2020-138577
27. Wan Y, Shang J, Graham R, Baric RS, Li F. Receptor recognition by the novel coronavirus from wuhan: an analysis based on decade-long structural studies of SARS coronavirus. J Virol (2020) 94(7). doi: 10.1128/JVI.00127-20
28. Bai Y, Yao L, Wei T, Tian F, Jin DY, Chen L, et al. Presumed asymptomatic carrier transmission of COVID-19. JAMA (2020) 323(14):1406–07. doi: 10.1001/jama.2020.2565
29. Zhang J, Tian S, Lou J, Chen Y. Familial cluster of COVID-19 infection from an asymptomatic. Crit Care (2020) 24(1):119. doi: 10.1186/s13054-020-2817-7
30. Ganyani T, Kremer C, Chen D, Torneri A, Faes C, Wallinga J, et al. Estimating the generation interval for coronavirus disease (COVID-19) based on symptom onset data, March 2020. Euro Surveill (2020) 25(17). doi: 10.2807/1560-7917.ES.2020.25.17.2000257
31. Anka AU, Tahir MI, Abubakar SD, Alsabbagh M, Zian Z, Hamedifar H, et al. Coronavirus disease 2019 (COVID-19): An overview of the immunopathology, serological diagnosis and management. Scand J Immunol (2021) 93(4):e12998. doi: 10.1111/sji.12998
32. Yoshida M, Worlock KB, Huang N, Huang N, Lindeboom RGH, Butler CR, Kumasaka N, et al. Local and systemic responses to SARS-CoV-2 infection in children and adults. Nature (2022) 602(7896):321–27. doi: 10.1038/s41586-021-04345-x
33. Hadjadj J, Yatim N, Barnabei L, Corneau A, Boussier J, Smith N, et al. Impaired type I interferon activity and inflammatory responses in severe COVID-19 patients. Science (2020) 369(6504):718–24. doi: 10.1126/science.abc6027
34. Wiersinga WJ, Rhodes A, Cheng AC, Peacock SJ, Prescott HC. Pathophysiology, transmission, diagnosis, and treatment of coronavirus disease 2019 (COVID-19): A review. JAMA (2020) 324(8):782–93. doi: 10.1001/jama.2020.12839
35. Leisman DE, Deutschman CS, Legrand M. Facing COVID-19 in the ICU: vascular dysfunction, thrombosis, and dysregulated inflammation. Intensive Care Med (2020) 46(6):1105–08. doi: 10.1007/s00134-020-06059-6
36. Xu Z, Shi L, Wang Y, Zhang J, Huang L, Zhang C, et al. Pathological findings of COVID-19 associated with acute respiratory distress syndrome. Lancet Respir Med (2020) 8(4):420–22. doi: 10.1016/S2213-2600(20)30076-X
37. van de Veerdonk FL, Netea MG, van Deuren M, van der Meer JW, de Mast Q, Bruggemann RJ, et al. Kallikrein-kinin blockade in patients with COVID-19 to prevent acute respiratory distress syndrome. Elife (2020) 9. doi: 10.7554/eLife.57555
38. Singh SP, Pritam M, Pandey B, Yadav TP. Microstructure, pathophysiology, and potential therapeutics of COVID-19: A comprehensive review. J Med Virol (2021) 93(1):275–99. doi: 10.1002/jmv.26254
39. Fu Y, Cheng Y, Wu Y. Understanding SARS-coV-2-mediated inflammatory responses: from mechanisms to potential therapeutic tools. Virol Sin (2020) 35(3):266–71. doi: 10.1007/s12250-020-00207-4
40. Feldmann M, Maini RN, Woody JN, Holgate ST, Winter G, Rowland M, et al. Trials of anti-tumour necrosis factor therapy for COVID-19 are urgently needed. Lancet (2020) 395(10234):1407–09. doi: 10.1016/S0140-6736(20)30858-8
41. Garbers C, Rose-John S. Genetic IL-6R variants and therapeutic inhibition of IL-6 receptor signalling in COVID-19. Lancet Rheumatol (2021) 3(2):e96–7. doi: 10.1016/S2665-9913(20)30416-1
42. Li H, Liu L, Zhang D, Xu J, Dai H, Tang N, et al. SARS-CoV-2 and viral sepsis: observations and hypotheses. Lancet (2020) 395(10235):1517–20. doi: 10.1016/S0140-6736(20)30920-X
43. Lin L, Lu L, Cao W, Li T. Hypothesis for potential pathogenesis of SARS-CoV-2 infection-a review of immune changes in patients with viral pneumonia. Emerg Microbes Infect (2020) 9(1):727–32. doi: 10.1080/22221751.2020.1746199
44. Ochani R, Asad A, Yasmin F, Shaikh S, Khalid H, Batra S, et al. COVID-19 pandemic: from origins to outcomes. A comprehensive review of viral pathogenesis, clinical manifestations, diagnostic evaluation, and management. Infez Med (2021) 29(1):20–36.
45. Cascella M, Rajnik M, Aleem A, Dulebohn SC, Di Napoli R. Features, evaluation, and treatment of coronavirus (COVID-19). Treasure Island (FL: StatPearls (2022).
46. Helms J, Tacquard C, Severac F, Leonard-Lorant I, Ohana M, Delabranche X, et al. High risk of thrombosis in patients with severe SARS-CoV-2 infection: a multicenter prospective cohort study. Intensive Care Med (2020) 46(6):1089–98. doi: 10.1007/s00134-020-06062-x
47. Wichmann D, Sperhake JP, Lutgehetmann M, Steurer S, Edler C, Heinemann A, et al. Autopsy findings and venous thromboembolism in patients with COVID-19: A prospective cohort study. Ann Intern Med (2020) 173(4):268–77. doi: 10.7326/M20-2003
48. McGonagle D, Bridgewood C, Ramanan AV, Meaney JFM, Watad A. COVID-19 vasculitis and novel vasculitis mimics. Lancet Rheumatol (2021) 3(3):e224–e33. doi: 10.1016/S2665-9913(20)30420-
49. Nishiga M, Wang DW, Han Y, Lewis DB, Wu JC. COVID-19 and cardiovascular disease: from basic mechanisms to clinical perspectives. Nat Rev Cardiol (2020) 17(9):543–58. doi: 10.1038/s41569-020-0413-9
50. Bonaventura A, Vecchie A, Dagna L, Martinod K, Dixon DL, Van Tassell BW, et al. Endothelial dysfunction and immunothrombosis as key pathogenic mechanisms in COVID-19. Nat Rev Immunol (2021) 21(5):319–29. doi: 10.1038/s41577-021-00536-9
51. Blanco-Melo D, Nilsson-Payant BE, Liu WC, Uhl S, Hoagland D, Moller R, et al. Imbalanced host response to SARS-coV-2 drives development of COVID-19. Cell (2020) 181(5):1036–45 e9. doi: 10.1016/j.cell.2020.04.026
52. Sariol A, Perlman S. Lessons for COVID-19 immunity from other coronavirus infections. Immunity (2020) 53(2):248–63. doi: 10.1016/j.immuni.2020.07.005
53. Cui L, Wang H, Ji Y, Yang J, Xu S, Huang X, et al. The nucleocapsid protein of coronaviruses acts as a viral suppressor of RNA silencing in mamMalian cells. J Virol (2015) 89(17):9029–43. doi: 10.1128/JVI.01331-15
54. Hurst KR, Koetzner CA, Masters PS. Characterization of a critical interaction between the coronavirus nucleocapsid protein and nonstructural protein 3 of the viral replicase-transcriptase complex. J Virol (2013) 87(16):9159–72. doi: 10.1128/JVI.01275-13
55. Walz L, Cohen AJ, Rebaza AP, Vanchieri J, Slade MD, Dela Cruz CS, et al. JAK-inhibitor and type I interferon ability to produce favorable clinical outcomes in COVID-19 patients: a systematic review and meta-analysis. BMC Infect Dis (2021) 21(1):47. doi: 10.1186/s12879-020-05730-z
56. Cicco S, Cicco G, Racanelli V, Vacca A. Neutrophil extracellular traps (NETs) and damage-associated molecular patterns (DAMPs): two potential targets for COVID-19 treatment. Mediators Inflammation (2020) 2020:7527953. doi: 10.1155/2020/7527953
57. Day JD, Park S, Ranard BL, Singh H, Chow CC, Vodovotz Y. Divergent COVID-19 disease trajectories predicted by a DAMP-centered immune network model. Front Immunol (2021) 12:754127. doi: 10.3389/fimmu.2021.754127
58. Canaday DH, Oyebanji OA, White E, Keresztesy D, Payne M, Wilk D, et al. Significantly elevated antibody levels and neutralization titers in nursing home residents after SARS-CoV-2 BNT162b2 mRNA booster vaccination. medRxiv (2021). doi: 10.1101/2021.12.07.21267179
59. Lucas C, Klein J, Sundaram ME, Liu F, Wong P, Silva J, et al. Delayed production of neutralizing antibodies correlates with fatal COVID-19. Nat Med (2021) 27(7):1178–86. doi: 10.1038/s41591-021-01355-0
60. Ren L, Zhang L, Chang D, Wang J, Hu Y, Chen H, et al. The kinetics of humoral response and its relationship with the disease severity in COVID-19. Commun Biol (2020) 3(1):780. doi: 10.1038/s42003-020-01526-8
61. Xie C, Li Q, Li L, Peng X, Ling Z, Xiao B, et al. Association of early inflammation with age and asymptomatic disease in COVID-19. J Inflammation Res (2021) 14:1207–16. doi: 10.2147/JIR.S304190
62. Therapeutics and COVID-19: living guideline, 22 april 2022. Geneva: World Health Organization (2022). WHO/2019-nCoV/therapeutics/20223.
63. Pretorius E, Venter C, Laubscher GJ, Kotze MJ, Oladejo SO, Watson LR, et al. Prevalence of symptoms, comorbidities, fibrin amyloid microclots and platelet pathology in individuals with Long COVID/Post-Acute Sequelae of COVID-19 (PASC). Cardiovasc Diabetol (2022) 21(1):148. doi: 10.1186/s12933-022-01579-5
64. Proal AD, VanElzakker MB. Long COVID or post-acute sequelae of COVID-19 (PASC): an overview of biological factors that may contribute to persistent symptoms. Front Microbiol (2021) 12:698169. doi: 10.3389/fmicb.2021.698169
65. Spudich S, Nath A. Nervous system consequences of COVID-19. Science (2022) 375(6578):267–69. doi: 10.1126/science.abm2052
66. Zubchenko S, Kril I, Nadizhko O, Matsyura O, Chopyak V. Herpesvirus infections and post-COVID-19 manifestations: a pilot observational study. Rheumatol Int (2022) 42(9):1523–30. doi: 10.1007/s00296-022-05146-9
67. Merzon E, Weiss M, Krone B, Cohen S, Ilani G, Vinker S, et al. Clinical and socio-demographic variables associated with the diagnosis of long COVID syndrome in youth: A population-based study. Int J Environ Res Public Health (2022) 19(10). doi: 10.3390/ijerph19105993
68. Renz-Polster H, Tremblay ME, Bienzle D, Fischer JE. The pathobiology of myalgic encephalomyelitis/chronic fatigue syndrome: the case for neuroglial failure. Front Cell Neurosci (2022) 16:888232. doi: 10.3389/fncel.2022.888232
69. Su Y, Yuan D, Chen DG, Ng RH, Wang K, Choi J, et al. Multiple early factors anticipate post-acute COVID-19 sequelae. Cell (2022) 185(5):881–95 e20. doi: 10.1016/j.cell.2022.01.014
70. Choutka J, Jansari V, Hornig M, Iwasaki A. Unexplained post-acute infection syndromes. Nat Med (2022) 28(5):911–23. doi: 10.1038/s41591-022-01810-6
71. Williamson AE, Tydeman F, Miners A, Pyper K, Martineau AR. Short-term and long-term impacts of COVID-19 on economic vulnerability: a population-based longitudinal study (COVIDENCE UK). BMJ Open (2022) 12(8):e065083. doi: 10.1136/bmjopen-2022-065083
72. Li X, Zai J, Zhao Q, Nie Q, Li Y, Foley BT, et al. Evolutionary history, potential intermediate animal host, and cross-species analyses of SARS-CoV-2. J Med Virol (2020) 92(6):602–11. doi: 10.1002/jmv.25731
73. van Doremalen N, Bushmaker T, Morris DH, Holbrook MG, Gamble A, Williamson BN, et al. Aerosol and surface stability of SARS-coV-2 as compared with SARS-coV-1. N Engl J Med (2020) 382(16):1564–67. doi: 10.1056/NEJMc2004973
74. Sharif N, Alzahrani KJ, Ahmed SN, Opu RR, Ahmed N, Talukder A, et al. Protective measures are associated with the reduction of transmission of COVID-19 in Bangladesh: A nationwide cross-sectional study. PloS One (2021) 16(11):e0260287. doi: 10.1371/journal.pone.0260287
75. Ahmed SF, Quadeer AA, McKay MR. Preliminary identification of potential vaccine targets for the COVID-19 coronavirus (SARS-coV-2) based on SARS-coV immunological studies. Viruses (2020). doi: 10.3390/v12030254
76. Sharif N, Alzahrani KJ, Ahmed SN, Dey SK. Efficacy, immunogenicity and safety of COVID-19 vaccines: A systematic review and meta-analysis. Front Immunol (2021) 12:714170. doi: 10.3389/fimmu.2021.714170
77. McDonald I, Murray SM, Reynolds CJ, Altmann DM, Boyton RJ. Comparative systematic review and meta-analysis of reactogenicity, immunogenicity and efficacy of vaccines against SARS-CoV-2. NPJ Vaccines (2021) 6(1):74. doi: 10.1038/s41541-021-00336-1
78. Seyahi E, Bakhdiyarli G, Oztas M, Kuskucu MA, Tok Y, Sut N, et al. Antibody response to inactivated COVID-19 vaccine (CoronaVac) in immune-mediated diseases: a controlled study among hospital workers and elderly. Rheumatol Int (2021) 41(8):1429–40. doi: 10.1007/s00296-021-04910-7
79. Sonani B, Aslam F, Goyal A, Patel J, Bansal P. COVID-19 vaccination in immunocompromised patients. Clin Rheumatol (2021) 40(2):797–98. doi: 10.1007/s10067-020-05547-w
80. Dai WC, Zhang HW, Yu J, Xu HJ, Chen H, Luo SP, et al. CT imaging and differential diagnosis of COVID-19. Can Assoc Radiol J (2020) 71(2):195–200. doi: 10.1177/0846537120913033
81. Fistera D, Hartl A, Pabst D, Manegold R, Holzner C, Taube C, et al. What about the others: differential diagnosis of COVID-19 in a German emergency department. BMC Infect Dis (2021) 21(1):969. doi: 10.1186/s12879-021-06663-x
82. Rai P, Kumar BK, Deekshit VK, Karunasagar I, Karunasagar I. Detection technologies and recent developments in the diagnosis of COVID-19 infection. Appl Microbiol Biotechnol (2021) 105(2):441–55. doi: 10.1007/s00253-020-11061-5
83. Spinner CD, Gottlieb RL, Criner GJ, Arribas Lopez JR, Cattelan AM, Soriano Viladomiu A, et al. Effect of Remdesivir vs Standard Care on Clinical Status at 11 Days in Patients With Moderate COVID-19: A Randomized Clinical Trial. JAMA (2020) 324(11):1048–57. doi: 10.1001/jama.2020.16349
84. Beigel JH, Tomashek KM, Dodd LE, Mehta AK, Zingman BS, Kalil AC, et al. Remdesivir for the treatment of covid-19 - final report. N Engl J Med (2020) 383(19):1813–26. doi: 10.1056/NEJMoa2007764
85. Wang Y, Zhang D, Du G, Du R, Zhao J, Jin Y, et al. Remdesivir in adults with severe COVID-19: a randomised, double-blind, placebo-controlled, multicentre trial. Lancet (2020) 395(10236):1569–78. doi: 10.1016/S0140-6736(20)31022-9
86. Jayk Bernal A, Gomes da Silva MM, Musungaie DB, Kovalchuk E, Gonzalez A, Delos Reyes V, et al. Molnupiravir for oral treatment of covid-19 in nonhospitalized patients. N Engl J Med (2022) 386(6):509–20. doi: 10.1056/NEJMoa2116044
87. Hammond J, Leister-Tebbe H, Gardner A, Abreu P, Bao W, Wisemandle W, et al. Oral nirmatrelvir for high-risk, nonhospitalized adults with covid-19. N Engl J Med (2022) 386(15):1397–408. doi: 10.1056/NEJMoa2118542
88. Dougan M, Nirula A, Azizad M, Mocherla B, Gottlieb RL, Chen P, et al. Bamlanivimab plus etesevimab in mild or moderate covid-19. N Engl J Med (2021) 385(15):1382–92. doi: 10.1056/NEJMoa2102685
89. Weinreich DM, Sivapalasingam S, Norton T, Ali S, Gao H, Bhore R, et al. REGN-COV2, a neutralizing antibody cocktail, in outpatients with covid-19. N Engl J Med (2021) 384(3):238–51. doi: 10.1056/NEJMoa2035002
90. Chen P, Nirula A, Heller B, Gottlieb RL, Boscia J, Morris J, et al. SARS-coV-2 neutralizing antibody LY-coV555 in outpatients with covid-19. N Engl J Med (2021) 384(3):229–37. doi: 10.1056/NEJMoa2029849
91. Cohen MS, Nirula A, Mulligan MJ, Novak RM, Marovich M, Yen C, et al. Effect of bamlanivimab vs placebo on incidence of COVID-19 among residents and staff of skilled nursing and assisted living facilities: A randomized clinical trial. JAMA (2021) 326(1):46–55. doi: 10.1001/jama.2021.8828
92. Guimaraes PO, Quirk D, Furtado RH, Maia LN, Saraiva JF, Antunes MO, et al. Tofacitinib in patients hospitalized with covid-19 pneumonia. N Engl J Med (2021) 385(5):406–15. doi: 10.1056/NEJMoa2101643
93. Cao Y, Wei J, Zou L, Jiang T, Wang G, Chen L, et al. Ruxolitinib in treatment of severe coronavirus disease 2019 (COVID-19): A multicenter, single-blind, randomized controlled trial. J Allergy Clin Immunol (2020) 146(1):137–46 e3. doi: 10.1016/j.jaci.2020.05.019
94. Shen C, Wang Z, Zhao F, Yang Y, Li J, Yuan J, et al. Treatment of 5 critically ill patients with COVID-19 with convalescent plasma. JAMA (2020) 323(16):1582–89. doi: 10.1001/jama.2020.4783
95. Simonovich VA, Burgos Pratx LD, Scibona P, Beruto MV, Vallone MG, Vazquez C, et al. A randomized trial of convalescent plasma in covid-19 severe pneumonia. N Engl J Med (2021) 384(7):619–29. doi: 10.1056/NEJMoa2031304
97. Gordon CJ, Tchesnokov EP, Woolner E, Perry JK, Feng JY, Porter DP, et al. Remdesivir is a direct-acting antiviral that inhibits RNA-dependent RNA polymerase from severe acute respiratory syndrome coronavirus 2 with high potency. J Biol Chem (2020) 295(20):6785–97. doi: 10.1074/jbc.RA120.013679
98. Gavriatopoulou M, Ntanasis-Stathopoulos I, Korompoki E, Fotiou D, Migkou M, Tzanninis IG, et al. Emerging treatment strategies for COVID-19 infection. Clin Exp Med (2021) 21(2):167–79. doi: 10.1007/s10238-020-00671-y
99. Menendez-Arias L. Decoding molnupiravir-induced mutagenesis in SARS-CoV-2. J Biol Chem (2021) 297(1):100867. doi: 10.1016/j.jbc.2021.100867
100. Gordon CJ, Tchesnokov EP, SChinazi RF, Gotte M. Molnupiravir promotes SARS-CoV-2 mutagenesis via the RNA template. J Biol Chem (2021) 297(1):100770. doi: 10.1016/j.jbc.2021.100770
101. Kabinger F, Stiller C, Schmitzova J, Dienemann C, Kokic G, Hillen HS, et al. Mechanism of molnupiravir-induced SARS-CoV-2 mutagenesis. Nat Struct Mol Biol (2021) 28(9):740–46. doi: 10.1038/s41594-021-00651-0
102. Abdelnabi R, Foo CS, De Jonghe S, Maes P, Weynand B, Neyts J. Molnupiravir inhibits replication of the emerging SARS-coV-2 variants of concern in a hamster infection model. J Infect Dis (2021) 224(5):749–53. doi: 10.1093/infdis/jiab361
103. Vangeel L, Chiu W, De Jonghe S, Maes P, Slechten B, Raymenants J, et al. Remdesivir, Molnupiravir and Nirmatrelvir remain active against SARS-CoV-2 Omicron and other variants of concern. Antiviral Res (2022) 198:105252. doi: 10.1016/j.antiviral.2022.105252
104. Huang C, Lu TL, Lin L. Real-world clinical outcomes of molnupiravir for the treatment of mild to moderate COVID-19 in adult patients during the dominance of the omicron variant: A meta-analysis. Antibiotics (Basel) (2023). doi: 10.3390/antibiotics12020393
105. Owen DR, Allerton CMN, Anderson AS, Aschenbrenner L, Avery M, Berritt S, et al. An oral SARS-CoV-2 M(pro) inhibitor clinical candidate for the treatment of COVID-19. Science (2021) 374(6575):1586–93. doi: 10.1126/science.abl4784
106. Sevrioukova IF, Poulos TL. Structure and mechanism of the complex between cytochrome P4503A4 and ritonavir. Proc Natl Acad Sci U.S.A. (2010) 107(43):18422–7. doi: 10.1073/pnas.1010693107
107. Ju B, Zhang Q, Ge J, Wang R, Sun J, Ge X, et al. Human neutralizing antibodies elicited by SARS-CoV-2 infection. Nature (2020) 584(7819):115–19. doi: 10.1038/s41586-020-2380-z
108. Klank D, Hoffmann M, Claus B, Zinke F, Bergner R, Paschka P. Monoclonal antibodies for the prevention and treatment of COVID-19 disease in patients with hematological Malignancies: two case reports and a literature review. Hemasphere (2021) 5(11):e651. doi: 10.1097/HS9.0000000000000651
109. O'Brien MP, Forleo-Neto E, Musser BJ, Isa F, Chan KC, Sarkar N, et al. Subcutaneous REGEN-COV antibody combination to prevent covid-19. N Engl J Med (2021) 385(13):1184–95. doi: 10.1056/NEJMoa2109682
110. Bavaro DF, Diella L, Solimando AG, Mocherla B, Gottlieb RL, Chen P, et al. Bamlanivimab and Etesevimab administered in an outpatient setting for SARS-CoV-2 infection. Pathog Glob Health (2022) 116(5):297–304. doi: 10.1080/20477724.2021.2024030
111. Jenks JD, Aslam S, Horton LE, Law N, Bharti A, Logan C, et al. Early monoclonal antibody administration can reduce both hospitalizations and mortality in high-risk outpatients with coronavirus disease 2019 (COVID-19). Clin Infect Dis (2022) 74(4):752–53. doi: 10.1093/cid/ciab522
112. WHO Rapid Evidence Appraisal for COVID-19 Therapies (REACT) Working Group. Anti-interleukin-6 therapies for hospitalized patients with COVID-19: a protocol for a prospective meta-analysis of randomized trials. Available at: https://www.who.int/publications/i/item/WHO-2019-nCoV-PMA_protocols-anti-IL-6-2021.1 (Accessed 10 June 2021).
113. Group WHOREAfC-TW, Shankar-Hari M, Vale CL, Godolphin PJ, Fisher D, Higgins JPT, et al. Association between administration of IL-6 antagonists and mortality among patients hospitalized for COVID-19: A meta-analysis. JAMA (2021) 326(6):499–518. doi: 10.1001/jama.2021.11330
114. Study to assess the efficacy and safety of ruxolitinib in patients with COVID-19 associated cytokine storm (RUXCOVID). Bethesda (MD: ClinicalTrials.gov National Library of Medicine (US. Available at: https://clinicaltrials.gov/ct2/show/results/NCT04362137 (Accessed 4 January 2022).
115. Fragoulis GE, McInnes IB, Siebert S. JAK-inhibitors. New players in the field of immune-mediated diseases, beyond rheumatoid arthritis. Rheumatol (Oxford) (2019) 58(Suppl 1):i43–54. doi: 10.1093/rheumatology/key276
116. Schwartz DM, Kanno Y, Villarino A, Ward M, Gadina M, O'Shea JJ. JAK inhibition as a therapeutic strategy for immune and inflammatory diseases. Nat Rev Drug Discovery (2017) 16(12):843–62. doi: 10.1038/nrd.2017.201
117. Alhazzani W, Moller MH, Arabi YM, Loeb M, Gong MN, Fan E, et al. Surviving Sepsis Campaign: guidelines on the management of critically ill adults with Coronavirus Disease 2019 (COVID-19). Intensive Care Med (2020) 46(5):854–87. doi: 10.1007/s00134-020-06022-5
118. Bai C, Chotirmall SH, Rello J, Alba GA, Ginns LC, Krishnan JA, et al. Updated guidance on the management of COVID-19: from an American Thoracic Society/European Respiratory Society coordinated International Task Force (29 July 2020). Eur Respir Rev (2020) 29(157). doi: 10.1183/16000617.0287-2020
119. Calzetta L, Aiello M, Frizzelli A, Rogliani P, Chetta A. Dexamethasone in patients hospitalized with COVID-19: whether, when and to whom. J Clin Med (2021) 10(8). doi: 10.3390/jcm10081607
120. Jamaati H, Hashemian SM, Farzanegan B, Malekmohammad M, Tabarsi P, Marjani M, et al. No clinical benefit of high dose corticosteroid administration in patients with COVID-19: A preliminary report of a randomized clinical trial. Eur J Pharmacol (2021) 897:173947. doi: 10.1016/j.ejphar.2021.173947
121. Roback JD, Guarner J. Convalescent plasma to treat COVID-19: possibilities and challenges. JAMA (2020) 323(16):1561–62. doi: 10.1001/jama.2020.4940
122. Ankcorn M, Gallacher J, Ijaz S, Taha Y, Harvala H, Maclennan S, et al. Convalescent plasma therapy for persistent hepatitis E virus infection. J Hepatol (2019) 71(2):434–38. doi: 10.1016/j.jhep.2019.04.008
123. van Griensven J, Edwards T, de Lamballerie X, Semple MG, Gallian P, Baize S, et al. Evaluation of convalescent plasma for ebola virus disease in Guinea. N Engl J Med (2016) 374(1):33–42. doi: 10.1056/NEJMoa1511812
124. Zhou B, Zhong N, Guan Y. Treatment with convalescent plasma for influenza A (H5N1) infection. N Engl J Med (2007) 357(14):1450–1. doi: 10.1056/NEJMc070359
125. Lamikanra A, Nguyen D, Simmonds P, Williams S, Bentley EM, Rowe C, et al. Comparability of six different immunoassays measuring SARS-CoV-2 antibodies with neutralizing antibody levels in convalescent plasma: From utility to prediction. Transfusion (2021) 61(10):2837–43. doi: 10.1111/trf.1660
126. O'Donnell MR, Grinsztejn B, Cummings MJ, Justman JE, Lamb MR, Eckhardt CM, et al. A randomized double-blind controlled trial of convalescent plasma in adults with severe COVID-19. J Clin Invest (2021) 131(13). doi: 10.1172/JCI150646
127. Haagmans BL, Noack D, Okba NMA, Li W, Wang C, Bestebroer T, et al. SARS-coV-2 neutralizing human antibodies protect against lower respiratory tract disease in a hamster model. J Infect Dis (2021) 223(12):2020–28. doi: 10.1093/infdis/jiab289
129. Siemieniuk RA, Bartoszko JJ, Diaz Martinez JP, Kum E, Qasim A, Zeraatkar D, et al. Antibody and cellular therapies for treatment of covid-19: a living systematic review and network meta-analysis. BMJ (2021) 374:n2231. doi: 10.1136/bmj.n2231
130. Cheung JC, Ho LT, Cheng JV, Cham EYK, Lam KN. Staff safety during emergency airway management for COVID-19 in Hong Kong. Lancet Respir Med (2020) 8(4):e19. doi: 10.1016/S2213-2600(20)30084-9
131. Meng L, Qiu H, Wan L, Ai Y, Xue Z, Guo Q, et al. Intubation and ventilation amid the COVID-19 outbreak: wuhan's experience. Anesthesiology (2020) 132(6):1317–32. doi: 10.1097/ALN.0000000000003296
132. Frat JP, Thille AW, Mercat A, Girault C, Ragot S, Perbet S, et al. High-flow oxygen through nasal cannula in acute hypoxemic respiratory failure. N Engl J Med (2015) 372(23):2185–96. doi: 10.1056/NEJMoa1503326
133. Gonzalez-Castro A, Fajardo Campoverde A, Medina A, Alapont VMI. Non-invasive mechanical ventilation and high-flow oxygen therapy in the COVID-19 pandemic: the value of a draw. Med Intensiva (Engl Ed) (2021) 45(5):320–21. doi: 10.1016/j.medin.2020.04.017
134. Leonard S, Atwood CW Jr., Walsh BK, DeBellis RJ, Dungan GC, Strasser W, et al. Preliminary findings on control of dispersion of aerosols and droplets during high-velocity nasal insufflation therapy using a simple surgical mask: implications for the high-flow nasal cannula. Chest (2020) 158(3):1046–49. doi: 10.1016/j.chest.2020.03.043
135. Dar M, Swamy L, Gavin D, Theodore A. Mechanical-ventilation supply and options for the COVID-19 pandemic. Leveraging all available resources for a limited resource in a crisis. Ann Am Thorac Soc (2021) 18(3):408–16. doi: 10.1513/AnnalsATS.202004-317CME
136. Lim ZJ, Subramaniam A, Ponnapa Reddy M, Blecher G, Kadam U, Afroz A, et al. Case fatality rates for patients with COVID-19 requiring invasive mechanical ventilation. A Meta-analysis. Am J Respir Crit Care Med (2021) 203(1):54–66. doi: 10.1164/rccm.202006-2405OC
Keywords: pathophysiology, clinical management, SARS-CoV-2, COVID-19, prevention
Citation: Zhu Y, Sharma L and Chang D (2023) Pathophysiology and clinical management of coronavirus disease (COVID-19): a mini-review. Front. Immunol. 14:1116131. doi: 10.3389/fimmu.2023.1116131
Received: 05 December 2022; Accepted: 24 July 2023;
Published: 14 August 2023.
Edited by:
Severino Jefferson Ribeiro da Silva, University of Toronto, CanadaReviewed by:
Shangwen Pan, Huazhong University of Science and Technology, ChinaLuiz Gonzaga Francisco De Assis Barros D’Elia Zanella, University of São Paulo, Brazil
Copyright © 2023 Zhu, Sharma and Chang. This is an open-access article distributed under the terms of the Creative Commons Attribution License (CC BY). The use, distribution or reproduction in other forums is permitted, provided the original author(s) and the copyright owner(s) are credited and that the original publication in this journal is cited, in accordance with accepted academic practice. No use, distribution or reproduction is permitted which does not comply with these terms.
*Correspondence: De Chang, changde@301hospital.com.cn; Lokesh Sharma, lokeshkumar.sharma@yale.edu