- 1Department of Rheumatology and Clinical Immunology, Charité - Universitätsmedizin Berlin, corporate member of Freie Universität Berlin and Humboldt-Universität zu Berlin, Berlin, Germany
- 2Immune Dynamics, Deutsches Rheuma-Forschungszentrum (DRFZ), A Leibniz Institute, Berlin, Germany
- 3Biophysical Analysis, Deutsches Rheuma-Forschungszentrum (DRFZ), A Leibniz Institute, Berlin, Germany
- 4Dynamic and Functional in vivo Imaging, Veterinary Medicine, Freie Universität Berlin, Berlin, Germany
What makes a plasma cell long-lived?
The plasma cell (PC) is the terminal differentiation state of B lymphocytes, phenotypically characterized by an enlarged granular cell body with a highly productive endoplasmic reticulum (ER) and strict energetic requirements to maintain their main function: antibody production. Despite being mitotically quiescent, PC are able to keep up high anabolic activity for months, up to several years. Early studies on “immunoglobulin producing cells” not yet characterized in detail at this point in time suggested an antibody production rate of several ten million molecules per hour and cell (1, 2). When resting B cells are activated in or outside of germinal centers (GC) giving rise to plasma blasts (PB), they rapidly undergo metabolic reprogramming and increase glycolysis and oxidative phosphorylation (OXPHOS) rates (3). From there, they can develop into either short-lived PC (SLPC) or home to the bone marrow (BM), where they become resident (4, 5), long-lived PC (LLPC) (6, 7). However, whilst some cellular and molecular components of the PC niche have been identified (8, 9), the factors maintaining those cells productive at high levels are less well known. Even telling apart SLPC from LLPC is not an easy job. Microanatomical location seems not to be a definite criterion, since affinity-matured PC and LLPC were also found to persist in spleen (10, 11) and in inflamed tissue, in mice and humans alike (12, 13). While one study in mice claimed that LLPCs are produced late during GC responses (14), results from a recent publication indicate that murine LLPC are also formed in early phases of the GC response (15), with some LLPC having undergone no affinity maturation at all (16). Furthermore, evolutionary conserved surface markers for PC, let alone LLPC, are missing. The majority of PC do express B cell maturation antigen (BCMA) and CD138 in mice and humans (17–20), however, there is also substantial CD138 heterogeneity found in human cultured BM aspirates (21). There is further no unique distinctiveness of surface markers for LLPC, which is also true for transcriptional programs (22). Most prominent, the expression of Prdm-1, Mcl1, Irf4 or Xbp1 are common requirements (15, 23). Additionally, microRNA miR-148a was identified in murine LLPC serving metabolic regulation (24). Very recently, after pre-sorting of murine LLPC from SLPC according to scRNA-sequencing-assisted clustering of PC subsets (23), the adhesion molecule EpCAM and a lack of the chemokine receptor CXCR3 were identified as characteristics of IgG LLPC in mice. This approach also illustrates that considerable effort is to be undertaken in order to point out these very rare cells from a heterogeneous parent population.
Investigating metabolic features as an additional deterministic layer of plasma cell development could help to simplify the identification of LLPC. Since the metabolism of a B cell becoming a PC needs to change quite dramatically, we suggest taking the activation of metabolic enzymes, glycolysis and respiration rates and the import of nutrients into account. Metabolic pathway properties at different stages of the maturation and differentiation process may be of an – in part epigenetic – advantage for cell longevity. For example, murine studies in pre-B cells have linked receptor signaling with the regulation of chromatin accesibility (25). In Th17 cells, the accumulation of the citric acid cycle metabolites 2-hydroxyglutarate and acetyl-CoA confers transcriptional regulation via histone modification (26, 27). Whether such cues are able to lastingly imprint the prerequisites of a long-lived phenotype during B cell activation, and if so, for how long, is not known. The fact that serum half-lives of antibodies induced by different viral antigens (Ag) are heterogeneous [e.g., 200 years for measles, 50 years for varicella zoster, 11-19 years for diphtheria and tetanus (28)] is suggesting a dependence of PC longevity from initial triggers (29). Viewed from the B cell side, high-affinity BCRs seem to generate a selection advantage through increasing OXPHOS (30). Similarly, differential CD19 expression could link initial signaling through Ag activation and metabolic rewiring. CD19, on the one hand, is enhancing BCR induced calcium flux to overcome a proposed Ag threshold (31). On the other hand, CD19 in mice is negatively regulating the activity of phospho-inositide-3-kinase (PI3K), an enzyme crucial for initializing metabolic pathways supporting proliferation and survival. In B cells, CD19 deficiency leads to a 50% increase in PI3K activity compared to CD19 sufficient counterparts (32). Since PI3K activity is also a pro-survival factor dependent on stromal cell contact and important for the regulation of mitochondrial integrity and ER-stress in LLPC (33), CD19- PC might have an advantage in coping with metabolic stress. In support of that, the downregulation of CD19 was identified as a hallmark of longevity in human plasma cells (34, 35).
A closer look on PC metabolism
BMPC mostly depend on OXPHOS, using amino acids, especially glutamine, for their carbon demand; despite significant upregulation of the glucose transporter GLUT1 (3). The latter is explained by the fact that glucose in PC is primarily used for antibody glycosylation. However, in times of decreased nutrient availability, PC can also metabolize the imported glucose into pyruvate driving mitochondrial respiration (36). Missing glucose, however, would slow or shut down the hexosamine biosynthesis pathway producing N-Acetyl-glucosamine (GlcNAc), as shown in cancer cells (37, 38). Since it is an essential structural component of protein glycosylation patterns, missing GlcNAc would mean failure in protein modification and trafficking (39). This mechanism has so far been only proven for tumor-associated proteins, but supposedly would have severe functional consequences for antibodies, too (40). Antibody misfolding, hindered degradation of misfolded antibodies or insufficient export from the ER induces ER stress that goes along with calcium efflux, affecting phosphorylation of metabolic gate-keepers like adenosine monophosphate (AMP)-activated protein kinase (AMPK) and mechanistic target of rapamycin (mTOR) (41–43). In fact, LLPC do import larger amounts of the fluorescent glucose analog 2-Deoxy-2-[(7-nitro-2,1,3-benzoxadiazol-4-yl)-amino]-D-glucose in mice, albeit via a still unidentified transport system (44, 45). If glucose does becomes limiting and falsely glycosylated antibodies are produced, then the cells are equipped with a rescue mechanism called the unfolded protein response (UPR). Ultimately, UPR is leading to autophagy that mobilizes additional energy sources by recycling of cellular building blocks from organelles like mitochondria and ER, a key process found indispensable for PC survival (46). The importance of UPR and autophagy in PC is further illustrated by the fact that PC signature genes such as Xbp1, Ire1 and Atg6 are devoted to these processes, though the latter is silenced in terminally differentiated PC (47, 48). Of note, the term UPR is misleading, as this process is primarily a timed orchestration of developmental factors preparing and supporting the B cell- to plasma cell-transition rather than an emergency response to unfolded proteins (49). Still, high-rate antibody output is considerably challenging the cellular machineries of posttranslational modification, folding and trafficking that has to withstand times of crises. Thus, we question a constant secretion rate and instead propose the dynamic regulation of energy metabolism and antibody output as a feature essential for PC longevity (Figure 1).
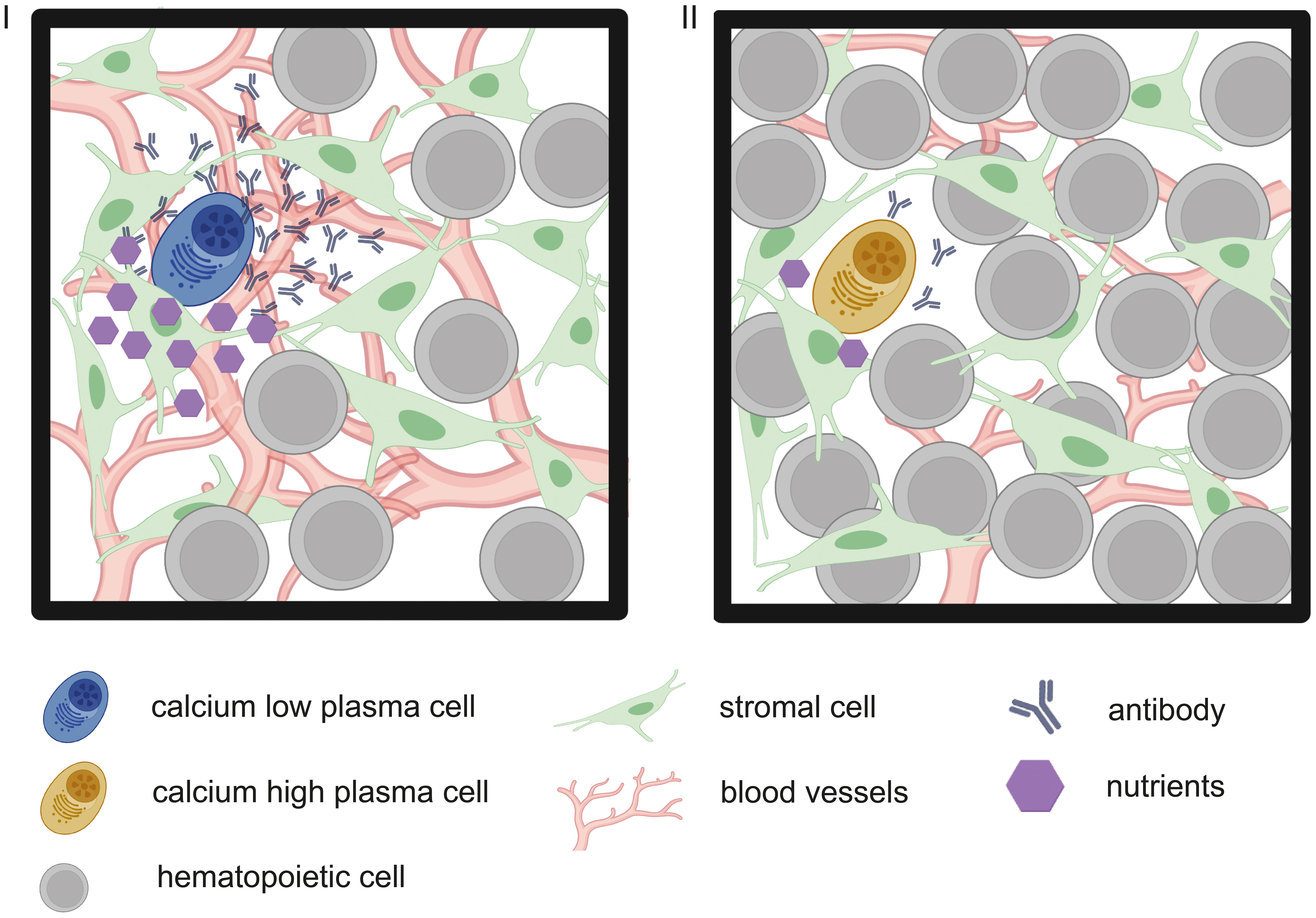
Figure 1 Graphic representation of two hypothetic metabolic states of PC within the BM, depending on niche composition. In this model, PC within their stromal cell niche (green mesenchymal stem cells) are low in cytoplasmic calcium (I, blue PC) in times of sufficient nutrient supply (purple hexagons), e.g. due to a dense vessel network (red branches) and only few other hematopoietic cells (grey), competing for nutrients. Calcium-low cells produce antibodies at high rates. Vice versa, in times of metabolic stress (II) through decreased nutrient supply due to hematopoietic nutrient sinks or vessel remodeling (5), antibody output is decreased.
Intracellular calcium as integrator of stressors
Calcium-mediated BCR signal strength has been known to encode different B cell fates by regulating downstream transcription and mTOR activity for quite some time (50–52). Unexpectedly, employing functional intravital imaging in mice, our group was able to detect also Ag-specific PB displaying vivid calcium fluctuations within a comparatively high concentration range (>500 nM) among otherwise calcium-low (<200 nM) peers (53). Ruling out the possibility of residual BCR signaling or cytokine receptor signaling, we sought for an alternative explanation for the existence of a calcium-high population among PB. Possibly, metabolic stress signals as a consequence of competition for nutrients in the densely packed medullary cords lead to transient calcium increase in the cytoplasm, likely through mitochondrial release (54, 55). Second, high production rates of antibodies induce ER-calcium release alongside ER-stress, initiating calcium replenishment from the extracellular environment. One proposed downstream effector of increased cytoplasmic calcium is AMPK. In times of energy crises, B lineage cells rely on AMPK for survival and functional maintenance (56, 57). AMPK is binding energy-poor AMP when its abundance is high – meaning adenosine triphosphate (ATP) amounts are low. AMP binding leads to AMPK phosphorylation and therefore activation. Studies with fully nutrient-supplied T cells, however, have shown that AMPK phosphorylation can also occur allosterically via calcium-calmodulin/dependent kinase kinase β (CamKKβ) (58, 59). Leakage of calcium ions from mitochondria and/or ER could possibly contribute to reaching a calcium concentration threshold for this pathway to take hold. Importantly, chronic calcium exposure has been found to inhibit AMPK in muscle cells via increased phosphatase activity, in a setting where the cells were additionally treated with 5-aminoimidazole-4-carboxamide ribonucleotide (AICAR), an AMP analog (60, 61). Calcium oscillations, however, activated AMPK. One of the main functions of active AMPK is to inhibit mTOR activity via phosphorylation of adapter molecules (62). Since mTOR inhibition leads to autophagy, stress-induced calcium release into the cytosol would directly lead to switching on mechanisms resolving stress or at least bridging the time until more favorable environmental conditions arise.
But why is it that PC would need such mechanisms in the first place? Given the BM is an organ with high cellular turnover due to hematopoiesis and a tissue undergoing profound age-related changes, it is evident that a certain degree of heterogeneity in the survival niches exists in space and time.
The PC niche and PC turnover models
The BM PC niche fulfills two basic requirements: promoting PC survival and serving as a foundation for adhesion. The stromal cell scaffold of the BM, together with several types of accessory cells, provides the main pro-survival cytokines A proliferation inducing ligand (APRIL), IL6 and CXCL12, the latter being also the chemoattractant guiding PB from secondary lymphoid organs to the BM (63, 64). CXCL12 is sensed by the PC via CXCR4, and tight PC adhesion within the niche is accomplished by VLA4 and LFA1 pairing with VCAM1 and ICAM1 of stromal cells, respectively (65, 66), as well as CD28 with CD80/86 (67, 68). CD28 expression supports LLPC survival by maintaining metabolic fitness via reactive oxygen species (ROS)-dependent signaling and IRF4 activity (69). APRIL directly supports PC survival and can be sensed by the PC via TACI and BCMA (70–72), an interaction also critical for differentiation of B cells into PC and therapeutic target in autoimmunity and multiple myeloma (MM) (73–75). Interestingly, homing can take place also in APRIL-deficient mice (65), so the pro-survival effect is likely independent from direct cell contact. Further, CD138, next to functioning as an adhesion molecule and receptor, can augment the scavenging of APRIL and IL-6 via binding to its heparan sulfate-chains on the cell surface and therefore support survival (76–80).
The general perception of the niche is that it consists of static (the stroma) and dynamic components, i.e. accessory cells providing soluble cytokines, such as granulocytes, megakaryocytes and others (9). Further, the number of niches is finite. PC will home to their niche persistently, unless driven away by inflammation and being outcompeted by newly formed PC (81). Models of PC turnover discussed include the random turnover model, in which newly formed PC replace older ones by chance, and the deterministic model, in which PC originating from certain B cell clones have a greater intrinsic (or imprinted) capability of homing and replacing than others. The problem of both these models is that they assume niches are changing neither in quality nor in number. However, a growing number of studies are reasonably challenging these notions, as discussed below.
Heterogeneity in the BM
The reason that PC are considered static in their survival niche is probably the time scale on which we observe them in in vivo imaging studies. Using time-lapse imaging of up to 12 hours, Benet et al. could show that PC indeed experience phases of increased displacement between marrow regions, interrupted by periods of sessility (82). In addition, they observed clusters of PC in certain areas. While on the one hand this fact disproves the paradigm that PCs always remain alone in the same niche, it also means that the extrinsic conditions in the BM are divided into zones; those that promote movement and those that retain PCs (63). These conditions can also change, presumably on the level of cytokine abundance and/or receptor expression.
For the hematopoietic stem cell (HSC) niche, a heterogeneous architecture has been known for a long time (83). It has been discussed that HSC and leukocytes share in part similar features of their respective interaction with stromal cells (84). Strikingly, they often co-localize with PC and require the same cytokine, CXCL12 (85). Therefore, we would like to argue that the following observations made for HSCs may also apply to the LLPC BM niche, however, this still awaits confirmation. Quiescent HSC reside in endosteal regions, whereas sinusoidal areas are characterized by dynamic turnover and leukocyte trafficking. Local oxygen concentration gradients might in part cause this heterogeneity, as demonstrated by direct oxygen concentration measurements using phosphorescence lifetime imaging (86). These researchers stressed that the BM as a whole is a hypoxic organ despite being highly vascularized, however they found a steep oxygen drop from the endost to perisinosoidal regions, and with growing distance to blood vessels. Notably, areas densely packed with cells appear to be downright oxygen sinks, “reminiscent of solid tumors” (86). Further, variations in the permeability of blood vessels were identified to cause these local oxygenation differences and ROS load of the cells, as indicated by indirect determination with pimonidazole staining and Hif1-a expression in HSC (87). Since the blood flow transports all kinds of nutrients into the highly metabolically active BM, the same might be true for carbon sources needed for PC homeostasis. Further, interstitial calcium concentrations are low in regions with new bone material deposition and hematopoietic progenitors are absent in these regions, leaving the question open to what extent extracellular calcium concentration influences intracellular calcium levels, and therefore cell turnover in vivo (88). These findings illustrate how a microanatomical and metabolite heterogeneity consequently leads to a differential distribution of BM cells. Assuming the same is true for the plasma cell niches is only reasonable. However, up till now, no adequate tools for spatially and temporally resolved metabolic analyses within the BM are available in vivo (8).
Heterogeneity as a result of BM dynamics
One approach to be expanded towards functional metabolic analysis is longitudinal intravital imaging of the BM (LIMB) (5, 89). Using this novel micro-endoscopic technique, our group was able to show that during homeostasis, vessel distribution, volumes and numbers are dynamically changing over time in the femoral marrow. Mice carrying the LIMB endoscope were repeatedly imaged for up to 6 weeks, revealing constant vessel remodeling. This temporal heterogeneity can be explained by several mechanisms: First, life-long exposure to gravity and exercise contribute to bone remodeling and immune cell health, as highlighted by studies under microgravity (90, 91). This effect might very well influence niche dynamics over time. Further, injuries might compromise the integrity of niches, leading to revascularization and cell population redistribution (89). Particularly, mechanosensing in macrophages mediated by the Piezo family of ion channels plays a pivotal role in bone regeneration following irradiation, and mechanosensing also impacts on the HSC niche and number of common lymphoid progenitors (92–94).
Furthermore, the alternation of day and night contributes to temporal heterogeneity of BM microenvironments through circadian gene and protein expression. This very likely affects plasma cell niches, too. The cellularity of the BM in mice and men (although they experience anticyclic rhythms since mice are nocturnal and humans usually not), has been shown to change quite drastically during any 24h. While structural bone remodeling usually peaks 1 hour after daybreak (95), blood replenishment and immune cell circulation even peak two times a day, regulated by two bursts of norepinephrine and tumor necrosis factor (TNF) (96). HSC cell trafficking to the blood was shown to be dependent on rhythmic CXCL12 oscillations from BM stroma, orchestrated by signals from the central nervous system (97). Furthermore, the homeostatic clearance of neutrophils within the marrow provides cues that directly act on the hematopoietic niche (98). That these mechanisms also have functional implications on the adaptive immune response has been demonstrated by the induction of experimental autoimmune encephalomyelitis, a mouse model for multiple sclerosis, at different time points per day (99). When cell counts were high in the periphery (end of day), mice showed dramatically faster disease progression than at the end of the night. It has been argued that this is in part because immune cells are reentering the marrow at night. Such daily turnover of cells in the microenvironment of PC niches is likely contributing to pressure-induced and nutrient supply-induced stress signals, requiring a certain amount of metabolic flexibility. However, longitudinal studies on BM resident cells have yet to be performed. Interestingly, Golan et al. hypothesize that quiescent HSC remaining in the marrow need to “train” their metabolism daily through circadian rhythms, in order to be ready for immediate activation during alarm situations (96). Of note, apart from gene expression and posttranslational modification, phosphorylation is another layer of metabolic regulation readily accessible and rapidly controllable by circadian signal transduction (100). Since many kinases are also calcium dependent enzymes, we expect to see periodic fluctuations in BMPC cytoplasmic calcium as well.
Along with changing daylight also goes diurnal food intake, which influences time-dependent availability of nutrients to the BM. A study investigating the effect of fasting on monocytes found a reduced inflammatory activity as an effect of AMPK-mediated sensing of low energy equivalents, and hence reduced BM egress (101). In the gut, fasting leads to a reduction in B cell numbers in Peyer’s patches, as well as egress of naïve B cells, which, in accordance with the response during the night (or during day in nocturnal animals), retreat to the BM for the time of nutrient deprivation (102). After refeeding, the cell pool is altered. The intracellular consequence of fasting-refeeding, i.e. the transition from low to high glucose levels, is remodeling mitochondria-ER contact sites and calcium ion exchange between the organelles (103). As seen from the circadian oscillatory IgA response in the gut, feeding times also directly impact on PC (104).
To stress the time component of BM heterogeneity, it should also be mentioned, that ageing disrupts bone marrow composition and circadian regulation, as shown for macrophages (105). The phenotypic appearance of BM in old age is characterized by a high proportion of adipocytes, which may well have a significant influence on the number and availability of niches. In fact, the impairment of hematopoiesis by fat deposits has been shown (106). Under this regard, it should also be discussed how results from mouse models can be applied to humans, since the murine BM commonly studied is juvenile and its human counterpart only exists in infancy.
Impact of niche dynamics on humoral immunity
Taken together, BM microenvironments are most likely subject to heterogeneity in space and, perhaps even more important for cell survival, in time. How will this affect plasma cell longevity? LLPC need to be equipped with mechanisms coping with stress signals in a flexible manner. In other words, LLPC will have to translate extracellular stimuli into intracellular responses, eventually affecting metabolism, to a point that ensures reduced energy consumption, but only to an extent not leading to cell death. Stressing this point, the sensitivity of GC B cells and PB to mTOR inhibition by rapamycin stands in contrast to the resistance of LLPC to the drug (107). Instead of dying, LLPC reduce antibody production, as shown in rapamycin-treated mice, ameliorating autoimmunity. After discontinuation of rapamycin injections, antibody levels reinstall to values before treatment, highlighting the reversible capacity of antibody secretion in LLPC. One feature of metabolic flexibility could be for instance a greater spare respiratory capacity seen in LLPC vs SLPC, meaning the difference from basal respiration to the ability to increase mitochondrial electron throughput under stress (36). The manipulation of stress-resolving mechanisms could also have implications for the development of new therapies in the treatment of malignant PC-mediated diseases, like MM or auto-antibody mediated inflammatory diseases (108). These diseases have in common that their causative agents, antibody-producing cells, cannot be eliminated by classical B cell-depleting therapies or immunosuppressive agents (109). The recognition that protein-secreting cells are highly dependent on an intact ER led to the use of the proteasome inhibitor bortezomib in MM and in several autoimmune diseases (110–112). For MM therapy, targeting glutamine metabolism has proven promising (113, 114). However, since PC have developed the aforementioned sophisticated exit strategies to conquer metabolic stress, for complete success of these therapies it will be necessary to identify and target key molecules in the stress-response pathways, probably in combination. For example, in vitro and in mouse models, a synergistic anti-tumor effect of mTOR inhibition with rapamycin and bortezomib has been reported (115).
Vice versa, vaccine development would profit massively from knowing how to trigger longevity in PC and thus ensure lasting protection. Despite a continuously growing global vaccine market that by now has crossed the 100 billion dollar threshold and is expected to gain another 60 billion over the next four years (116), efforts to decipher the true mechanistic nature of durable antibody responses remain low. Advances come from the investigation of immunity induced in response to virus-like particles (117, 118). Schiller et al. argue that presumably the molecular form of Ag decides over induction of LLPC, also discussing an Ag-imprinting mechanism (119, 120). They found that vaccines against human papilloma virus (HPV), gain their remarkable durability from specialized structural features of the protein sequence, naming especially repeats with uniform spacing capable of activating several BCRs of both IgM and IgD subtype, thus inducing an exceptionally strong signal transmitted by IgD (121). Recently, TLR7 signaling has been shown to support the establishment of a favorable vaccination outcome (122). Some time ago, also TLR9 was identified in playing a role in B cell activation leading to stabilization of glycolytic activity, and the circumvention of mitochondrial depolarization by increased intracellular calcium (123, 124). Further, vaccination failure due to a lack of Ag-specific LLPC and an accumulation of SLPC in the BM has been linked to a gain-of-function mutation in Cxcr4 common in warts, hypogammaglobulinemia, infections, and myelokathexis (WHIM) syndrome and Waldenström’s macroglobulinemia (125). This could be explained with enhanced mTOR signaling promoting extrafollicular PC differentiation and BM homing, as seen in a T-independent setting (126). These results are once more stressing a close connection between the sensing of environmental factors by innate immune receptors, BCR- and cytokine signaling and the homeostasis of controlled metabolic properties in establishing LLPC.
Conclusion
In summary, understanding the principles driving differentiation of PC into the either short-lived or long-lived phenotype will be crucial for future therapy as well as vaccine development. Additionally, the factors that maintain LLPC in BM or inflamed tissue survival niches and their variability need to be taken into account (Table 1). Unfortunately, unambiguous identification of LLPC is challenging, requiring multiple analyses of surface markers and transcription factors. Identification by lifetime might be possible by time-stamped fate-mapping (15, 127). To also study the function of LLPC in their natural environment, new longitudinal imaging techniques are urgently needed. One approach to pursue is the monitoring of metabolic parameters and their change over time or in response to stimuli that mimic metabolic stress situations, for example with a combination of LIMB and fluorescence lifetime imaging and enzyme mapping (5, 53, 128, 129). Further, because the BM environment is constantly changing due to time of day, pressure changes in the tissue, aging or diseases and along with it the cellular composition, the niches themselves will likely also change. Thus, LLPC will encounter different environmental challenges, namely supply with nutrients, oxygen or cytokines, among others. Interestingly, though we still need more research on what triggers longevity in LLPC, existing data is pointing toward an imprinting mechanism by which some PC are favoured over others to become LLPC. The imprinted features are most likely metabolic regulators for the resolution of stress. Regulation could for instance be achieved dynamically by periodically cycling processes of autophagy and UPR, in connection with changing intracellular calcium levels and fluctuations in antibody output.
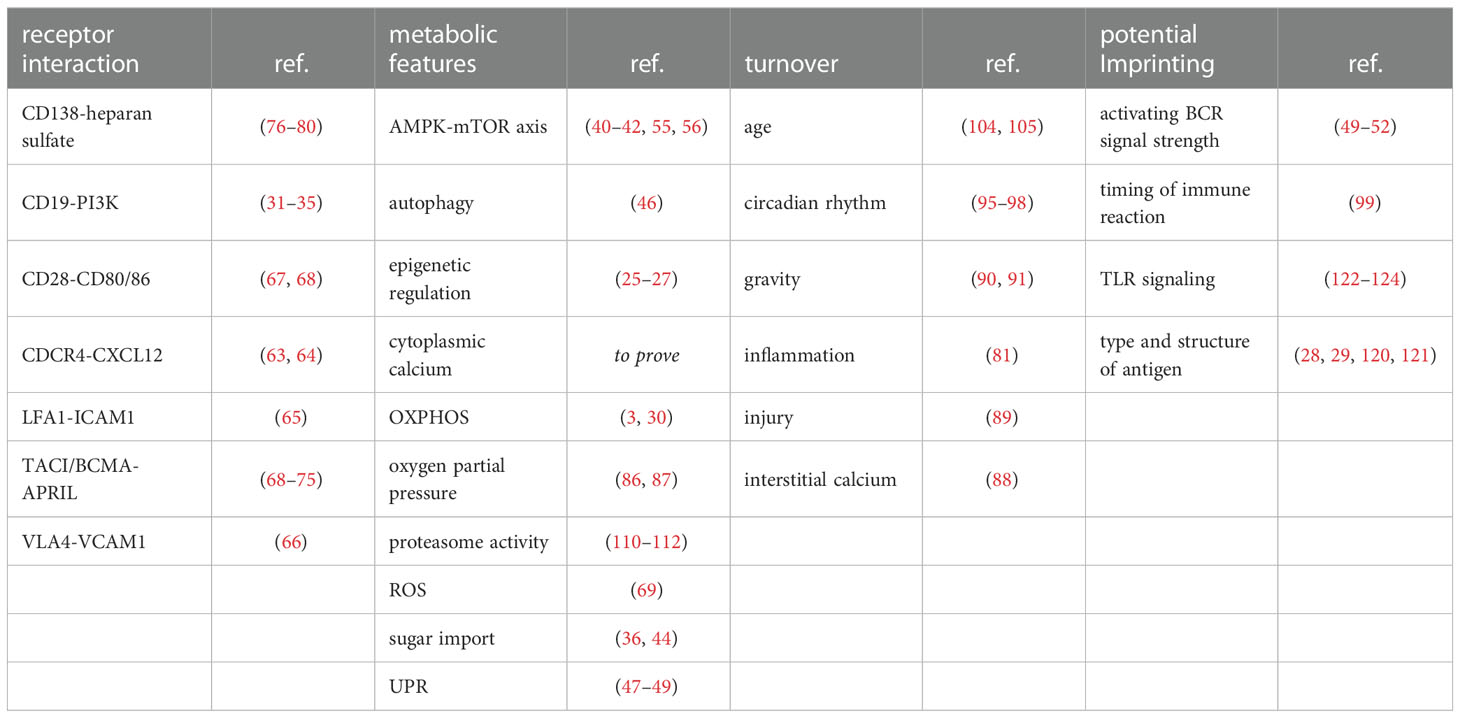
Table 1 Extrinsic and intrinsic factors impacting on survival and longevity of BMPC (alphabetical order).
Author contributions
CU, YC, AH, and RN conceptualized the storyline. CU drafted the manuscript. AH, RN, and YC proof-read and complemented the manuscript. All authors contributed to the article and approved the submitted version.
Funding
This study was supported by funding from the Deutsche Forschungsgemeinschaft, HA5354/10-1, SPP1937 (HA5354/8-2) and DFG HA5354/12-1 to AH, TRR 130 C01 and SFB 1444 P14 (to AH and RN). RN was supported by DFG 1167/5-1.
Acknowledgments
Figure created with BioRender.com.
Conflict of interest
The authors declare that the research was conducted in the absence of any commercial or financial relationships that could be construed as a potential conflict of interest.
Publisher’s note
All claims expressed in this article are solely those of the authors and do not necessarily represent those of their affiliated organizations, or those of the publisher, the editors and the reviewers. Any product that may be evaluated in this article, or claim that may be made by its manufacturer, is not guaranteed or endorsed by the publisher.
References
1. Helmreich E, Kern M, Eisen HN. The secretion of antibody by isolated lymph node cells. J Biol Chem (1961) 236:464–73. doi: 10.1016/s0021-9258(18)64385-6
2. Hibi T, Dosch H-M. Limiting dilution analysis of the b cell compartment in human bone marrow. Eur J Immunol (1986) 16:139–45. doi: 10.1002/eji.1830160206
3. Caro-Maldonado A, Wang R, Nichols AG, Kuraoka M, Milasta S, Sun LD, et al. Metabolic reprogramming is required for antibody production that is suppressed in anergic but exaggerated in chronically BAFF-exposed b cells. J Immunol (2014) 192:3626–36. doi: 10.4049/jimmunol.1302062
4. Ulbricht C, Niesner RA, Hauser AE. Imaging of bone marrow plasma cells and of their niches. Methods Mol Biol (2021) 2308:163–76. doi: 10.1007/978-1-0716-1425-9_14
5. Reismann D, Stefanowski J, Günther R, Rakhymzhan A, Matthys R, Nützi R, et al. Longitudinal intravital imaging of the femoral bone marrow reveals plasticity within marrow vasculature. Nat Commun (2017) 8:2153. doi: 10.1038/s41467-017-01538-9
6. Sze DM, Toellner KM, García de Vinuesa C, Taylor DR, MacLennan IC. Intrinsic constraint on plasmablast growth and extrinsic limits of plasma cell survival. J Exp Med (2000) 192:813–21. doi: 10.1084/jem.192.6.813
7. Manz RA, Löhning M, Cassese G, Thiel A, Radbruch A. Survival of long-lived plasma cells is independent of antigen. Int Immunol (1998) 10(11):1703–11. doi: 10.1093/intimm/10.11.1703
8. Lindquist RL, Niesner RA, Hauser AE. In the right place, at the right time: Spatiotemporal conditions determining plasma cell survival and function. Front Immunol (2019) 10:788. doi: 10.3389/fimmu.2019.00788
9. Zehentmeier S, Roth K, Cseresnyes Z, Sercan Ö, Horn K, Niesner RA, et al. Static and dynamic components synergize to form a stable survival niche for bone marrow plasma cells. Eur J Immunol (2014) 44:2306–17. doi: 10.1002/eji.201344313
10. Slifka MK, Antia R, Whitmire JK, Ahmed R. Humoral immunity due to long-lived plasma cells. Immunity (1998) 8:363–72. doi: 10.1016/S1074-7613(00)80541-5
11. Ellyard JI, Avery DT, Phan TG, Hare NJ, Hodgkin PD, Tangye SG. Antigen-selected, immunoglobulin-secreting cells persist in human spleen and bone marrow. Blood (2004) 103:3805–12. doi: 10.1182/blood-2003-09-3109
12. Starke C, Frey S, Wellmann U, Urbonaviciute V, Herrmann M, Amann K, et al. High frequency of autoantibody-secreting cells and long-lived plasma cells within inflamed kidneys of NZB/W F1 lupus mice. Eur J Immunol (2011) 41:2107–12. doi: 10.1002/eji.201041315
13. Pollok K, Mothes R, Ulbricht C, Liebheit A, Gerken JD, Uhlmann S, et al. The chronically inflamed central nervous system provides niches for long-lived plasma cells. Acta Neuropathol Commun (2017) 5:88. doi: 10.1186/s40478-017-0487-8
14. Weisel FJ, Zuccarino-Catania GV, Chikina M, Shlomchik MJ. A temporal switch in the germinal center determines differential output of memory b and plasma cells. Immunity (2016) 44:116–30. doi: 10.1016/j.immuni.2015.12.004
15. Robinson MJ, Dowling MR, Pitt C, O’Donnell K, Webster RH, Hill DL, et al. Long-lived plasma cells accumulate in the bone marrow at a constant rate from early in an immune response. Sci Immunol (2022) 7:eabm8389. doi: 10.1126/sciimmunol.abm8389
16. Bortnick A, Chernova I, Quinn WJ, Mugnier M, Cancro MP, Allman D. Long-lived bone marrow plasma cells are induced early in response to T cell-independent or T cell-dependent antigens. J Immunol (2012) 188:5389–96. doi: 10.4049/jimmunol.1102808
17. Setiadi AF, Sheikine Y. CD138-negative plasma cell myeloma: a diagnostic challenge and a unique entity. BMJ Case Rep (2019) 12:e232233. doi: 10.1136/bcr-2019-232233
18. Madry C, Laabi Y, Callebaut I, Roussel J, Hatzoglou A, Le Coniat M, et al. The characterization of murine BCMA gene defines it as a new member of the tumor necrosis factor receptor superfamily. Int Immunol (1998) 10:1693–702. doi: 10.1093/intimm/10.11.1693
19. Laâbi Y, Gras MP, Carbonnel F, Brouet JC, Berger R, Larsen CJ, et al. A new gene, BCM, on chromosome 16 is fused to the interleukin 2 gene by a t (4,16)(q26;p13) translocation in a malignant T cell lymphoma. EMBO J (1992) 11:3897–904. doi: 10.1002/j.1460-2075.1992.tb05482.x
20. Laabi Y, Gras MP, Brouet JC, Berger R, Larsen CJ, Tsapis A. The BCMA gene, preferentially expressed during b lymphoid maturation, is bidirectionally transcribed. Nucleic Acids Res (1994) 22:1147–54. doi: 10.1093/nar/22.7.1147
21. Mujtahedi SS, Yigitbilek F, Benavides X, Merzkani MA, Ozdogan E, Abozied O, et al. Bone marrow derived long-lived plasma cell phenotypes are heterogeneous and can change in culture. Transpl Immunol (2022) 75:101726. doi: 10.1016/j.trim.2022.101726
22. Lam WY, Bhattacharya D. Metabolic links between plasma cell survival, secretion, and stress. Trends Immunol (2018) 39:19–27. doi: 10.1016/j.it.2017.08.007
23. Liu X, Yao J, Zhao Y, Wang J, Qi H. Heterogeneous plasma cells and long-lived subsets in response to immunization, autoantigen and microbiota. Nat Immunol (2022) 23:1564–76. doi: 10.1038/s41590-022-01345-5
24. Pracht K, Meinzinger J, Schulz SR, Daum P, Côrte-Real J, Hauke M, et al. miR-148a controls metabolic programming and survival of mature CD19-negative plasma cells in mice. Eur J Immunol (2021) 51:1089–109. doi: 10.1002/eji.202048993
25. Mandal M, Okoreeh MK, Kennedy DE, Maienschein-Cline M, Ai J, McLean KC, et al. CXCR4 signaling directs igk recombination and the molecular mechanisms of late b lymphopoiesis. Nat Immunol (2019) 20:1393–403. doi: 10.1038/s41590-019-0468-0
26. Baixauli F, Piletic K, Puleston DJ, Villa M, Field CS, Flachsmann LJ, et al. An LKB1–mitochondria axis controls TH17 effector function. Nature (2022) 610:555–61. doi: 10.1038/s41586-022-05264-1
27. Hochrein SM, Wu H, Eckstein M, Arrigoni L, Herman JS, Schumacher F, et al. The glucose transporter GLUT3 controls T helper 17 cell responses through glycolytic-epigenetic reprogramming. Cell Metab (2022) 34:516–532.e11. doi: 10.1016/j.cmet.2022.02.015
28. Amanna IJ, Carlson NE, Slifka MK. Duration of humoral immunity to common viral and vaccine antigens. N Engl J Med (2007) 357:1903–15. doi: 10.1056/NEJMoa066092
29. Robinson MJ, Webster RH, Tarlinton DM. How intrinsic and extrinsic regulators of plasma cell survival might intersect for durable humoral immunity. Immunol Rev (2020) 296:87–103. doi: 10.1111/imr.12895
30. Chen D, Wang Y, Manakkat Vijay GK, Fu S, Nash CW, Xu D, et al. Coupled analysis of transcriptome and BCR mutations reveals role of OXPHOS in affinity maturation. Nat Immunol (2021) 22:904–13. doi: 10.1038/s41590-021-00936-y
31. Slifka MK, Amanna IJ. Role of multivalency and antigenic threshold in generating protective antibody responses. Front Immunol (2019) 10:956. doi: 10.3389/fimmu.2019.00956
32. Xu Y, Fairfax K, Light A, Huntington ND, Tarlinton DM. CD19 differentially regulates BCR signalling through the recruitment of PI3K. Autoimmunity (2014) 6934:1–8. doi: 10.3109/08916934.2014.921810
33. Cornelis R, Hahne S, Taddeo A, Petkau G, Malko D, Durek P, et al. Stromal cell-contact dependent PI3K and APRIL induced NF-κB signaling prevent mitochondrial- and ER stress induced death of memory plasma cells. Cell Rep (2020) 32:107982. doi: 10.1016/j.celrep.2020.107982
34. Mei HE, Wirries I, Frölich D, Brisslert M, Giesecke C, Grün JR, et al. A unique population of IgG-expressing plasma cells lacking CD19 is enriched in human bone marrow. Blood (2015) 125:1739–48. doi: 10.1182/blood-2014-02-555169
35. Halliley JL, Tipton CM, Liesveld J, Rosenberg AF, Darce J, Gregoretti IV, et al. Long-lived plasma cells are contained within the CD19-CD38hiCD138+ subset in human bone marrow. Immunity (2015) 43:132–45. doi: 10.1016/j.immuni.2015.06.016
36. Lam WY, Becker AM, Kennerly KM, Wong R, Curtis JD, Llufrio EM, et al. Mitochondrial pyruvate import promotes long-term survival of antibody-secreting plasma cells. Immunity (2016) 45:60–73. doi: 10.1016/j.immuni.2016.06.011
37. Palorini R, Cammarata F, Balestrieri C, Monestiroli A, Vasso M, Gelfi C, et al. Glucose starvation induces cell death in K-ras-transformed cells by interfering with the hexosamine biosynthesis pathway and activating the unfolded protein response. Cell Death Dis (2013) 4:1–14. doi: 10.1038/cddis.2013.257
38. Ding B, Parmigiani A, Divakaruni AS, Archer K, Murphy AN, Budanov AV. Sestrin2 is induced by glucose starvation via the unfolded protein response and protects cells from non-canonical necroptotic cell death. Sci Rep (2016) 6:1–14. doi: 10.1038/srep22538
39. Lauc G, Hirata T, Kizuka Y. (L)The role of glycosylation in health and disease. Springer Nature Switzerland AG: Springer Nature (2021). 3–22 p.
40. Cheng C, Ru P, Geng F, Liu J, Yoo JY, Wu X, et al. Glucose-mediated n-glycosylation of SCAP is essential for SREBP-1 activation and tumor growth. Cancer Cell (2015) 28:569–81. doi: 10.1016/j.ccell.2015.09.021
41. Høyer-Hansen M, Jäättelä M. Connecting endoplasmic reticulum stress to autophagy by unfolded protein response and calcium. Cell Death Differ (2007) 14:1576–82. doi: 10.1038/sj.cdd.4402200
42. Kania E, Pająk B, Orzechowski A. Calcium homeostasis and ER stress in control of autophagy in cancer cells. BioMed Res Int (2015) 2015:352794. doi: 10.1155/2015/352794
43. Decuypere JP, Kindt D, Luyten T, Welkenhuyzen K, Missiaen L, De Smedt H, et al. mTOR-controlled autophagy requires intracellular Ca2+ signaling. PloS One (2013) 8:e61020. doi: 10.1371/journal.pone.0061020
44. D’Souza L, Bhattacharya D. Plasma cells: You are what you eat. Immunol Rev (2019) 288:161–77. doi: 10.1111/imr.12732
45. D’Souza LJ, Wright SH, Bhattacharya D. Genetic evidence that uptake of the fluorescent analog 2NBDG occurs independently of known glucose transporters. PLoS One (2022) 17(8):e0261801. doi: 10.1371/journal.pone.0261801
46. Pengo N, Scolari M, Oliva L, Milan E, Mainoldi F, Raimondi A, et al. Plasma cells require autophagy for sustainable immunoglobulin production. Nat Immunol (2013) 14:298–305. doi: 10.1038/ni.2524
47. Tellier J, Shi W, Minnich M, Liao Y, Crawford S, Smyth GK, et al. Blimp-1 controls plasma cell function through the regulation of immunoglobulin secretion and the unfolded protein response. Nat Immunol (2016) 17:323–30. doi: 10.1038/ni.3348
48. Aragon IV, Barrington RA, Jackowski S, Mori K, Brewer JW. The specialized unfolded protein response of b lymphocytes: ATF6α-independent development of antibody-secreting b cells. Mol Immunol (2012) 51:347–55. doi: 10.1016/j.molimm.2012.04.001
49. Ricci D, Gidalevitz T, Argon Y. The special unfolded protein response in plasma cells. Immunol Rev (2021) 303:35–51. doi: 10.1111/imr.13012
50. Shulman Z, Gitlin AD, Weinstein JS, Lainez B, Esplugues E, Flavell RA, et al. Dynamic signaling by T follicular helper cells during germinal center b cell selection. Science (2014) 345:1058–62. doi: 10.1126/science.1257861
51. Mueller J, Matloubian M, Zikherman J. Cutting edge: An in vivo reporter reveals active b cell receptor signaling in the germinal center. J Immunol (2015) 194:2993–7. doi: 10.4049/jimmunol.1403086
52. Berry CT, Liu X, Myles A, Nandi S, Chen YH, Hershberg U, et al. BCR-induced Ca2+ signals dynamically tune survival, metabolic reprogramming, and proliferation of naive b cells. Cell Rep (2020) 31:107474. doi: 10.1016/j.celrep.2020.03.038
53. Ulbricht C, Leben R, Rakhymzhan A, Kirchhoff F, Nitschke L, Radbruch H, et al. Intravital quantification reveals dynamic calcium concentration changes across b cell differentiation stages. Elife (2021) 10:e56020. doi: 10.7554/eLife.56020
54. Griffiths EJ, Rutter GA. Mitochondrial calcium as a key regulator of mitochondrial ATP production in mammalian cells. Biochim Biophys Acta - Bioenerg (2009) 1787:1324–33. doi: 10.1016/j.bbabio.2009.01.019
55. Raffaello A, Mammucari C, Gherardi G, Rizzuto R. Calcium at the center of cell signaling: Interplay between endoplasmic reticulum, mitochondria, and lysosomes. Trends Biochem Sci (2016) 41:1035–49. doi: 10.1016/j.tibs.2016.09.001
56. Brookens SK, Cho SH, Basso PJ, Boothby MR. AMPKα1 in b cells dampens primary antibody responses yet promotes mitochondrial homeostasis and persistence of b cell memory. J Immunol (2020) 205:3011–22. doi: 10.4049/jimmunol.1901474
57. Brookens SK, Boothby MR. AMPK metabolism in the b lineage modulates humoral responses. Immunometabolism (2021) 3:1–12. doi: 10.20900/immunometab20210011
58. Tamás P, Hawley SA, Clarke RG, Mustard KJ, Green K, Hardie DG, et al. Regulation of the energy sensor AMP-activated protein kinase by antigen receptor and Ca2+ in T lymphocytes. J Exp Med (2006) 203:1665–70. doi: 10.1084/jem.20052469
59. Hawley SA, Pan DA, Mustard KJ, Ross L, Bain J, Edelman AM, et al. Calmodulin-dependent protein kinase kinase-beta is an alternative upstream kinase for AMP-activated protein kinase. Cell Metab (2005) 2:9–19. doi: 10.1016/j.cmet.2005.05.009
60. Park S, Scheffler TL, Gerrard DE. Chronic high cytosolic calcium decreases AICAR-induced AMPK activity via calcium/calmodulin activated protein kinase II signaling cascade. Cell Calcium (2011) 50:73–83. doi: 10.1016/j.ceca.2011.05.009
61. Park S, Scheffler TL, Rossie SS, Gerrard DE. AMPK activity is regulated by calcium-mediated protein phosphatase 2A activity. Cell Calcium (2013) 53:217–23. doi: 10.1016/j.ceca.2012.12.001
62. Gwinn DM, Shackelford DB, Egan DF, Mihaylova MM, Mery A, Vasquez DS, et al. AMPK phosphorylation of raptor mediates a metabolic checkpoint. Mol Cell (2008) 30:214–26. doi: 10.1016/j.molcel.2008.03.003
63. Aaron TS, Fooksman DR. Dynamic organization of the bone marrow plasma cell niche. FEBS J (2022) 289:4228–39. doi: 10.1111/febs.16385
64. Cassese G, Arce S, Hauser AE, Lehnert K, Moewes B, Mostarac M, et al. Plasma cell survival is mediated by synergistic effects of cytokines and adhesion-dependent signals. J Immunol (2003) 171:1684–90. doi: 10.4049/jimmunol.171.4.1684
65. Belnoue E, Tougne C, Rochat A-F, Lambert P-H, Pinschewer DD, Siegrist C-A. Homing and adhesion patterns determine the cellular composition of the bone marrow plasma cell niche. J Immunol (2012) 188:1283–91. doi: 10.4049/jimmunol.1103169
66. DiLillo DJ, Hamaguchi Y, Ueda Y, Yang K, Uchida J, Haas KM, et al. Maintenance of long-lived plasma cells and serological memory despite mature and memory b cell depletion during CD20 immunotherapy in mice. J Immunol (2008) 180:361–71. doi: 10.4049/jimmunol.180.1.361
67. Njau MN, Kim JH, Chappell CP, Ravindran R, Thomas L, Pulendran B, et al. CD28-B7 interaction modulates short- and long-lived plasma cell function. J Immunol (2012) 189:2758–67. doi: 10.4049/jimmunol.1102728
68. Murray ME, Gavile CM, Nair JR, Koorella C, Carlson LM, Buac D, et al. CD28-mediated pro-survival signaling induces chemotherapeutic resistance in multiple myeloma. Blood (2014) 123:3770–9. doi: 10.1182/blood-2013-10-530964
69. Utley A, Chavel C, Lightman S, Holling GA, Cooper J, Peng P, et al. CD28 regulates metabolic fitness for long-lived plasma cell survival. Cell Rep (2020) 31:107815. doi: 10.1016/j.celrep.2020.107815
70. Hahne M, Kataoka T, Schröter M, Hofmann K, Irmler M, Bodmer JL, et al. APRIL, a new ligand of the tumor necrosis factor family, stimulates tumor cell growth. J Exp Med (1998) 188:1185–90. doi: 10.1084/jem.188.6.1185
71. Baert L, Ahmed MC, Manfroi B, Huard B. The number 13 of the family: a proliferation inducing ligand. Curr Opin Immunol (2021) 71:132–7. doi: 10.1016/j.coi.2021.06.018
72. Lee L, Draper B, Chaplin N, Philip B, Chin M, Galas-Filipowicz D, et al. An APRIL-based chimeric antigen receptor for dual targeting of BCMA and TACI in multiple myeloma. Blood (2018) 131:746–58. doi: 10.1182/blood-2017-05-781351
73. Gross JA, Johnston J, Mudri S, Enselman R, Dillon SR, Madden K, et al. TACI and BCMA are receptors for a TNF homologue implicated in b-cell autoimmune disease. Nature (2000) 404:995–9. doi: 10.1038/35010115
74. Marsters SA, Yan M, Pitti RM, Haas PE, Dixit VM, Ashkenazi A. Interaction of the TNF homologues BLyS and APRIL with the TNF receptor homologues BCMA and TACI. Curr Biol (2000) 10:785–8. doi: 10.1016/s0960-9822(00)00566-2
75. Thompson JS, Schneider P, Kalled SL, Wang L, Lefevre EA, Cachero TG, et al. BAFF binds to the tumor necrosis factor receptor-like molecule b cell maturation antigen and is important for maintaining the peripheral b cell population. J Exp Med (2000) 192:129–35. doi: 10.1084/jem.192.1.129
76. Hendriks J, Planelles L, de Jong-Odding J, Hardenberg G, Pals ST, Hahne M, et al. Heparan sulfate proteoglycan binding promotes APRIL-induced tumor cell proliferation. Cell Death Differ (2005) 12:637–48. doi: 10.1038/sj.cdd.4401647
77. Ingold K, Zumsteg A, Tardivel A, Huard B, Steiner Q-G, Cachero TG, et al. Identification of proteoglycans as the APRIL-specific binding partners. J Exp Med (2005) 201:1375–83. doi: 10.1084/jem.20042309
78. Benson MJ, Dillon SR, Castigli E, Geha RS, Xu S, Lam K-P, et al. Cutting edge: the dependence of plasma cells and independence of memory b cells on BAFF and APRIL. J Immunol (2008) 180:3655–9. doi: 10.4049/jimmunol.180.6.3655
79. McCarron MJ, Park PW, Fooksman DR. CD138 mediates selection of mature plasma cells by regulating their survival. Blood (2017) 129:2749–59. doi: 10.1182/blood-2017-01-761643
80. Sarrazin S, Lamanna WC, Esko JD. Heparan sulfate proteoglycans. Cold Spring Harb Perspect Biol (2011) 3:1–33. doi: 10.1101/cshperspect.a004952
81. Radbruch A, Muehlinghaus G, Luger EO, Inamine A, Smith KGC, Dörner T, et al. Competence and competition: The challenge of becoming a long-lived plasma cell. Nat Rev Immunol (2006) 6:741–50. doi: 10.1038/nri1886
82. Benet Z, Jing Z, Fooksman DR. Plasma cell dynamics in the bone marrow niche. Cell Rep (2021) 34:108733. doi: 10.1016/j.celrep.2021.108733
83. Adams GB, Scadden DT. The hematopoietic stem cell in its place. Nat Immunol (2006) 7:333–7. doi: 10.1038/ni1331
84. De Grandis M, Lhoumeau AC, Mancini SJC, Aurrand-Lions M. Adhesion receptors involved in HSC and early-b cell interactions with bone marrow microenvironment. Cell Mol Life Sci (2016) 73:687–703. doi: 10.1007/s00018-015-2064-2
85. Wei Q, Frenette PS. Niches for hematopoietic stem cells and their progeny. Immunity (2018) 48:632–48. doi: 10.1016/j.immuni.2018.03.024
86. Spencer JA, Ferraro F, Roussakis E, Klein A, Wu J, Runnels JM, et al. Direct measurement of local oxygen concentration in the bone marrow of live animals. Nature (2014) 508:269–73. doi: 10.1038/nature13034
87. Itkin T, Gur-Cohen S, Spencer JA, Schajnovitz A, Ramasamy SK, Kusumbe AP, et al. Distinct bone marrow blood vessels differentially regulate haematopoiesis. Nature (2016) 532:323–8. doi: 10.1038/nature17624
88. Yeh SCA, Hou J, Wu JW, Yu S, Zhang Y, Belfield KD, et al. Quantification of bone marrow interstitial pH and calcium concentration by intravital ratiometric imaging. Nat Commun (2022) 13:393. doi: 10.1038/s41467-022-27973-x
89. Stefanowski J, Fiedler AF, Köhler M, Günther R, Liublin W, Tschaikner M, et al. Limbostomy: Longitudinal intravital microendoscopy in murine osteotomies. Cytom Part A (2020) 97:1–13. doi: 10.1002/cyto.a.23997
90. Sarkar R, Pampaloni F. In vitro models of bone marrow remodelling and immune dysfunction in space: Present state and future directions. Biomedicines (2022) 10:766. doi: 10.3390/biomedicines10040766
91. Grimm D, Wehland M, Corydon TJ, Richter P, Prasad B, Bauer J, et al. The effects of microgravity on differentiation and cell growth in stem cells and cancer stem cells. Stem Cells Transl Med (2020) 9:882–94. doi: 10.1002/sctm.20-0084
92. Zhang X, Hou L, Li F, Zhang W, Wu C, Xiang L, et al. Piezo1-mediated mechanosensation in bone marrow macrophages promotes vascular niche regeneration after irradiation injury. Theranostics (2022) 12:1621–38. doi: 10.7150/thno.64963
93. Shen B, Tasdogan A, Ubellacker JM, Zhang J, Nosyreva ED, Du L, et al. A mechanosensitive peri-arteriolar niche for osteogenesis and lymphopoiesis. Nature (2021) 591:438–44. doi: 10.1038/s41586-021-03298-5
94. Xu X, Liu S, Liu H, Ru K, Jia Y, Wu Z, et al. Piezo channels: Awesome mechanosensitive structures in cellular mechanotransduction and their role in bone. Int J Mol Sci (2021) 22:6429. doi: 10.3390/ijms22126429
95. Simmons DJ, Nichols G. Diurnal periodicity in the metabolic activity of bone tissue. Am J Physiol Content (1966) 210:411–8. doi: 10.1152/ajplegacy.1966.210.2.411
96. Golan K, Kollet O, Markus RP, Lapidot T. Daily light and darkness onset and circadian rhythms metabolically synchronize hematopoietic stem cell differentiation and maintenance: The role of bone marrow norepinephrine, tumor necrosis factor, and melatonin cycles. Exp Hematol (2019) 78:1–10. doi: 10.1016/j.exphem.2019.08.008
97. Méndez-Ferrer S, Lucas D, Battista M, Frenette PS. Haematopoietic stem cell release is regulated by circadian oscillations. Nature (2008) 452:442–7. doi: 10.1038/nature06685
98. Casanova-Acebes M, Pitaval C, Weiss LA, Nombela-Arrieta C, Chèvre R, A-González N, et al. XRhythmic modulation of the hematopoietic niche through neutrophil clearance. Cell (2013) 153:1025. doi: 10.1016/j.cell.2013.04.040
99. Druzd D, Matveeva O, Ince L, Harrison U, He W, Schmal C, et al. Lymphocyte circadian clocks control lymph node trafficking and adaptive immune responses. Immunity (2017) 46:120–32. doi: 10.1016/j.immuni.2016.12.011
100. Robles MS, Humphrey SJ, Mann M. Phosphorylation is a central mechanism for circadian control of metabolism and physiology. Cell Metab (2017) 25:118–27. doi: 10.1016/j.cmet.2016.10.004
101. Jordan S, Tung N, Casanova-Acebes M, Chang C, Cantoni C, Zhang D, et al. Dietary intake regulates the circulating inflammatory monocyte pool. Cell (2019) 178:1102–1114.e17. doi: 10.1016/j.cell.2019.07.050
102. Nagai M, Noguchi R, Takahashi D, Takubo K, Dohi T, Hase K, et al. Fasting-refeeding impacts immune cell dynamics and mucosal immune responses. Cell (2019) 178(5):1072–87.e14. doi: 10.1016/j.cell.2019.07.047
103. Castro-Sepúlveda M, Morio B, Tuñón-Suárez M, Jannas-Vela S, Díaz-Castro F, Rieusset J, et al. The fasting-feeding metabolic transition regulates mitochondrial dynamics. FASEB J Off Publ Fed Am Soc Exp Biol (2021) 35:e21891. doi: 10.1096/fj.202100929R
104. Penny HA, Domingues RG, Krauss MZ, Melo-Gonzalez F, Lawson MAE, Dickson S, et al. Rhythmicity of intestinal IgA responses confers oscillatory commensal microbiota mutualism. Sci Immunol (2022) 7:eabk2541. doi: 10.1126/sciimmunol.abk2541
105. Blacher E, Tsai C, Litichevskiy L, Shipony Z, Iweka CA, Schneider KM, et al. Aging disrupts circadian gene regulation and function in macrophages. Nat Immunol (2022) 23:229–36. doi: 10.1038/s41590-021-01083-0
106. Ambrosi TH, Scialdone A, Graja A, Gohlke S, Jank A-M, Bocian C, et al. Adipocyte accumulation in the bone marrow during obesity and aging impairs stem cell-based hematopoietic and bone regeneration. Cell Stem Cell (2017) 20:771–784.e6. doi: 10.1016/j.stem.2017.02.009
107. Jones DD, Gaudette BT, Wilmore JR, Chernova I, Bortnick A, Weiss BM, et al. MTOR has distinct functions in generating versus sustaining humoral immunity. J Clin Invest (2016) 126:4250–61. doi: 10.1172/JCI86504
108. Roman-Trufero M, Auner HW, Edwards CM. Multiple myeloma metabolism – a treasure trove of therapeutic targets? Front Immunol (2022) 13:897862. doi: 10.3389/fimmu.2022.897862
109. Hofmann K, Clauder A-K, Manz RA. Targeting b cells and plasma cells in autoimmune diseases. Front Immunol (2018) 9:835. doi: 10.3389/fimmu.2018.00835
110. Alexander T, Sarfert R, Klotsche J, Kühl AA, Rubbert-Roth A, Lorenz H-M, et al. The proteasome inhibitior bortezomib depletes plasma cells and ameliorates clinical manifestations of refractory systemic lupus erythematosus. Ann Rheum Dis (2015) 74:1474–8. doi: 10.1136/annrheumdis-2014-206016
111. Yates S, Matevosyan K, Rutherford C, Shen Y-M, Sarode R. Bortezomib for chronic relapsing thrombotic thrombocytopenic purpura: A case report. Transfusion (2014) 54:2064–7. doi: 10.1111/trf.12614
112. Neubert K, Meister S, Moser K, Weisel F, Maseda D, Amann K, et al. The proteasome inhibitor bortezomib depletes plasma cells and protects mice with lupus-like disease from nephritis. Nat Med (2008) 14:748–55. doi: 10.1038/nm1763
113. Gonsalves WI, Ramakrishnan V, Hitosugi T, Ghosh T, Jevremovic D, Dutta T, et al. Glutamine-derived 2-hydroxyglutarate is associated with disease progression in plasma cell malignancies. JCI Insight (2018) 3:e94543. doi: 10.1172/jci.insight.94543
114. Thompson RM, Dytfeld D, Reyes L, Robinson RM, Smith B, Manevich Y, et al. Glutaminase inhibitor CB-839 synergizes with carfilzomib in resistant multiple myeloma cells. Oncotarget (2017) 8:35863–76. doi: 10.18632/oncotarget.16262
115. Li J, Liu Z, Li Y, Jing Q, Wang H, Liu H, et al. Everolimus shows synergistic antimyeloma effects with bortezomib via the AKT/mTOR pathway. J Investig Med Off Publ Am Fed Clin Res (2019) 67:39–47. doi: 10.1136/jim-2018-000780
116. Wood L. Vaccines glob mark rep 2022, by type, technol route adm valance, distrib channel (2022) (Accessed November 28, 2022).
117. Brown DR, Garland SM, Ferris DG, Joura E, Steben M, James M, et al. The humoral response to gardasil over four years as defined by total IgG and competitive luminex immunoassay. Hum Vaccin (2011) 7:230–8. doi: 10.4161/hv.7.2.13948
118. Stanley M, Pinto LA, Trimble C. Human papillomavirus vaccines – immune responses. Vaccine (2012) 30:F83–7. doi: 10.1016/j.vaccine.2012.04.106
119. Amanna IJ, Slifka MK. Mechanisms that determine plasma cell lifespan and the duration of humoral immunity. Immunol Rev (2010) 236:125–38. doi: 10.1111/j.1600-065X.2010.00912.x
120. Schiller J, Lowy D. Explanations for the high potency of HPV prophylactic vaccines. Vaccine (2018) 36:4768–73. doi: 10.1016/j.vaccine.2017.12.079
121. Übelhart R, Hug E, Bach MP, Wossning T, Dühren-von Minden M, Horn AHC, et al. Responsiveness of b cells is regulated by the hinge region of IgD. Nat Immunol (2015) 16:534–43. doi: 10.1038/ni.3141
122. Chang X, Krenger P, Krueger CC, Zha L, Han J, Yermanos A, et al. TLR7 signaling shapes and maintains antibody diversity upon virus-like particle immunization. Front Immunol (2022) 12:827256. doi: 10.3389/fimmu.2021.827256
123. Akkaya M, Traba J, Roesler AS, Miozzo P, Akkaya B, Theall BP, et al. Second signals rescue b cells from activation-induced mitochondrial dysfunction and death. Nat Immunol (2018) 19:871–84. doi: 10.1038/s41590-018-0156-5
124. Kwak K, Akkaya M, Pierce SK. B cell signaling in context. Nat Immunol (2019) 20(8):963–9. doi: 10.1038/s41590-019-0427-9
125. Biajoux V, Natt J, Freitas C, Alouche N, Sacquin A, Hemon P, et al. Efficient plasma cell differentiation and trafficking require Cxcr4 desensitization. Cell Rep (2016) 17:193–205. doi: 10.1016/j.celrep.2016.08.068
126. Alouche N, Bonaud A, Rondeau V, Hussein-Agha R, Nguyen J, Bisio V, et al. Hematologic disorder–associated Cxcr4 gain-of-function mutation leads to uncontrolled extrafollicular immune response. Blood (2021) 137:3050–63. doi: 10.1182/blood.2020007450
127. Xu AQ, Barbosa RR, Calado DP. Genetic timestamping of plasma cells in vivo reveals tissue-specific homeostatic population turnover. Elife (2020) 9Le59850. doi: 10.7554/eLife.59850
128. Leben R, Köhler M, Radbruch H, Hauser AE, Niesner RA. Systematic enzyme mapping of cellular metabolism by phasor-analyzed label-free NAD(P)H fluorescence lifetime imaging. Int J Mol Sci (2019) 20:1–19. doi: 10.3390/ijms20225565
Keywords: metabolism, bone marrow, autophagy, long-lived plasma cells (LLPC), calcium, antibody production
Citation: Ulbricht C, Cao Y, Niesner RA and Hauser AE (2023) In good times and in bad: How plasma cells resolve stress for a life-long union with the bone marrow. Front. Immunol. 14:1112922. doi: 10.3389/fimmu.2023.1112922
Received: 30 November 2022; Accepted: 14 February 2023;
Published: 08 March 2023.
Edited by:
Rachel Maurie Gerstein, University of Massachusetts Medical School, United StatesReviewed by:
Karl Balabanian, Institut National de la Santé et de la Recherche Médicale (INSERM), Paris, FranceHans-Martin Jäck, University of Erlangen Nuremberg, Germany
Copyright © 2023 Ulbricht, Cao, Niesner and Hauser. This is an open-access article distributed under the terms of the Creative Commons Attribution License (CC BY). The use, distribution or reproduction in other forums is permitted, provided the original author(s) and the copyright owner(s) are credited and that the original publication in this journal is cited, in accordance with accepted academic practice. No use, distribution or reproduction is permitted which does not comply with these terms.
*Correspondence: Anja E. Hauser, anja.hauser-hankeln@charite.de