- 1Department of Pathology, Grenoble University Hospital, Grenoble, France
- 2Translational Research in Autoimmunity and Inflammation Group (TRAIG), Translational Innovation in Medicine and Complexity (TIMC), University Grenoble-Alpes, CNRS Unité mixte de recherche (UMR) 5525, Grenoble, France
- 3Department of Internal Medicine, Grenoble University Hospital, Grenoble, France
- 4Department of Rheumatology, Grenoble University Hospital, Grenoble, France
- 5Department of Rheumatology, Kanazawa University Hospital, Kanazawa, Japan
- 6Immunology Laboratory, Grenoble University Hospital, Grenoble, France
- 7Department of Nephrology, Grenoble University Hospital, Grenoble, France
- 8B Lymphocytes, Autoimmunity and Immunotherapies, Brest University, INSERM, UMR1227, Brest, France
- 9Odontology Unit, Brest University Hospital, Brest, France
The humoral response is frequently dysfunctioning in autoimmunity with a frequent rise in total serum immunoglobulins, among which are found autoantibodies that may be pathogenic by themselves and/or propagate the inflammatory reaction. The infiltration of autoimmune tissues by antibody-secreting cells (ASCs) constitutes another dysfunction. The known high dependency of ASCs on the microenvironment to survive combined to the high diversity of infiltrated tissues implies that ASCs must adapt. Some tissues even within a single clinical autoimmune entity are devoid of infiltration. The latter means that either the tissue is not permissive or ASCs fail to adapt. The origin of infiltrated ASCs is also variable. Indeed, ASCs may be commonly generated in the secondary lymphoid organ draining the autoimmune tissue, and home at the inflammation site under the guidance of specific chemokines. Alternatively, ASCs may be generated locally, when ectopic germinal centers are formed in the autoimmune tissue. Alloimmune tissues with the example of kidney transplantation will also be discussed own to their high similarity with autoimmune tissues. It should also be noted that antibody production is not the only function of ASCs, since cells with regulatory functions have also been described. This article will review all the phenotypic variations indicative of tissue adaptation described so for at the level of ASC-infiltrating auto/alloimmune tissues. The aim is to potentially define tissue-specific molecular targets in ASCs to improve the specificity of future autoimmune treatments.
1. Introduction
In autoimmune diseases, the immunosuppressive treatments based on steroids and/or anti-mitotic agents used for decades are timely replaced by more immune selective drugs. These new therapies may be in the form of antagonist and cytotoxic antibodies. Alternatively, solubilized receptors may also be used for antagonism purposes. Very recently, T cells transduced with chimeric antigen receptors (CAR) originating from the oncology field has also entered the game. Regarding humoral immunity, three drugs targeting distinct molecules but achieving a quite similar immunosuppressive function have been approved for clinical usage. The first approval concerns rheumatoid arthritis in 2006 with a depleting antibody directed against the CD20 molecule, an ubiquitous receptor expressed on mature B cells (1). The initial discovery came from a study testing a cytotoxic chimeric antibody, rituximab, in patients suffering from B-cell lymphoma. The drug was found highly effective, but most important for our present consideration a patient cosuffering from rheumatoid arthritis (RA) also showed benefits at the autoimmune level (2). Hence, a new therapeutic wave in autoimmunity was launched, the B-cell depletion. This approval was extended to granulomatosis polyangiitis and pemphigus vulgaris in 2014 and 2018, respectively (3, 4). Ocrelizumab, the second generation humanized anti-CD20 was also approved in multiple sclerosis in 2017 (5). The second approval concerns systemic lupus erythematosus (SLE) in 2011, and is based on an antagonist antibody, belimumab, directed against the B-cell activation factor from the TNF superfamily (BAFF) (6). BAFF (TNFSF13b) is one of the latest identified members of this superfamily (7). Precisely, BAFF acts extramedullary on developing B cells at their transitional stage to provide a signal enabling them to reach their final mature stage. It does so by stimulating the BAFF receptor (BAFF-R) (8). BAFF-deficient animals gave a striking phenotype with the almost complete disappearance of the mature B-cell pool. Such a result was the proof of concept to design BAFF antagonism in the B-cell depletion era. Another humanized BAFF, tabalumab, is also under development (9). The third approval concerns neuromyelitis optica (NMO) with a depleting antibody, inebilizumab, against the CD19 receptor (10). Other drugs targeting B cells are also tested in autoimmune diseases such as the depleting antibody ianalumab targeting the BAFF-R, the negatively signaling epratuzumab targeting CD22, and atacicept a soluble form of the TACI receptor antagonizing BAFF and the related member from the TNF superfamily a proliferation inducing ligand (APRIL, TNFSF13) (11–13). Note that a second soluble form of TACI, telitacept, is currently under development with a first recent approval in SLE (14). According to the European Union and United States clinical trial registers, 16 different autoimmune diseases are tested or announced to be with at least one of these drugs in 2022. More precisely, 13 diseases are targeted with one anti-CD20, 7 with one anti-BAFF, 4 with the anti-BAFF-R, 3 with the anti-CD19, 1 with the anti-CD22 and 2 with atacicept. The anti-CD38 antibody, daratumumab, coming from the hemato-oncology field and successfully used to treat multiple myeloma (MM), the most common plasma cell (PC)-derived tumor, has also entered the autoimmunity field for refractory patients (15). The proteasome inhibitor bortezomib has been tested in a large spectrum of antibody-mediated autoimmune diseases (16). The study is now completed and results are awaited. Finally, inhibitors of B-cell specific kinases such as the Bruton tyrosine kinase used to treat B-cell malignancies are also under investigations (17).
Antibody-secreting cells (ASCs) may be of two kinds, the plasmablast (PB) representing the first effector stage during the B-cell differentiation process by its capacity to secrete antibodies and the terminally differentiated plasma cell (PC). PB and PC are short- and long-lived, respectively. The different stages and associated phenotypes of B-cell differentiation has been recently reviewed by others (18). A common marker used to characterize ASCs is the high expression of CD38. The coexpression of CD38 and CD138 is further considered to represent the final PC stage, at least in the human system (19). One important concern regarding CD19, CD20, CD22, BAFF-R and their targeting in autoimmunity is their temporal regulation of expression. Indeed, expression of all these receptors is gradually lost upon differentiation of B cells into effector ASCs. Expression is totally lost on PCs, while they may still be present but at a downregulated level on PBs. This implies that these targets will lead to depletion at best of short-lived PBs, and will leave untouched PC already differentiated in patients before diagnosis and treatment. Notably, these treatments will remove all B cells, precursors of ASCs. The COVID 19 taught us that such profound depletion may be unwanted in autoimmune patients, when an urgent vaccination is needed to face emerging infectious agents (20). The similar expression pattern for CD19, CD20 and CD22 is explained by the fact that they are all under the control of the transcription repressor B lymphocyte-induced maturation protein (Blimp-1), a master activator of PC differentiation (21). It should be noted that CD19 may be considered as an outlier in this family, since CD19 has been observed at the surface of ASCs (22). CD19 expression on PCs may concern a subset of cells considered to represent an immature stage of PC (23). Others reported that CD19 may be lost earlier at the PB stage (24). There are currently no data regarding BAFF-R regulation by Blimp1. However, it is clear that BAFF-R undergoes downregulation in germinal center B cells (25–27). It is very much likely that BAFF-R follows the same transcriptional control considering its highly similar expression pattern compared to CD20 and CD22 and its absence of function in PC biology (28). The two other receptors for BAFF and APRIL, TACI and BCMA, have a different expression pattern. They are not expressed on naive mature B cells, but appear after antigen encounter. BCMA is ubiquitous on PCs and key for their survival as demonstrated in genetically deficient mice (29). TACI has been defined by others as enigmatic (30). This is definitely the case when one considers that TACI has two different isoforms harboring different subcellular localizations, the positive or negative signaling ability reported, and an uncommon ligand-independent activation mode (31, 32). TACI expression has been best studied in MM with a gene expression profile obtained with TACI+ MM cells consistent with terminally differentiated bone marrow (BM) PCs (33, 34). TACI is expressed on healthy ASCs, at least a subset, and has a survival role for these cells (35). BCMA and TACI expression renders BAFF antagonism potentially efficient to target PCs. However, this has not been observed experimentally (36). One explanation to this is the low affinity reported for BAFF with BCMA (37, 38). On the contrary to BAFF, APRIL is a key PC survival factor (28). Hence, atacicept is so far the only biotherapy that may act on established PCs. In an animal model of antibody-mediated autoimmunity, atacicept was found more active than BAFF-only blockade (39). The anti-CD38 daratumumab may look promising. However, one should be aware that CD38 is not only expressed in immune cells including ASCs. In particular, it is expressed by liver stellate cells and anti-CD38 treatments have been associated to a rise in liver enzymes (40, 41). Another important issue regarding PC targeting is their quiescent state rendering them non susceptible to anti-mitotic agents at variance to proliferative PBs (42).
Cell phenotype described above came from studies conducted either in secondary lymphoid organs considered as the generation site, or blood considered as the circulation site, or BM considered as the long-term residency site. Several concerns exist arguing that the phenotype of ASC infiltrating autoimmune tissues may differ. Indeed, one may ask whether terminal differentiation as observed upon homing in the sterile environment of the bone marrow is also similarly occurring after infiltration in an inflamed autoimmune tissue. Autoimmune tissues may harbor ectopic germinal centers (EGCs) commonly defined by an aggregate of proliferating T and B cells intermixed with CD21+ follicular dendritic cells (FDCs) (43). In these cases, the tissue should contain different population of ASCs. All these considerations may highly modulate ASCs susceptibility to current biotherapies targeting B cells discussed above. Here, we will review the literature describing the phenotype and stage of differentiation of ASCs infiltrating autoimmune tissues. We will also review the chemokines engaged in ASC homing (44, 45). Among these chemokines, CXCL-12 has been frequently studied. One should note that this axis is not strictly specific to ASCs, since many leukocytes express the associated receptor, CXCR-4, and that dual pro- and anti-inflammatory functions has been associated to it (46, 47). We selected diseases based on the frequent tissue infiltration by ASCs and/or the description of pathogenic antibodies defined by their ability to transfer/exacerbate the disease in an animal model. We are also discussing kidney allotransplants rejected upon a chronic humoral immune response, since these tissues are displaying high in situ similarities with autoimmune tissues and allotransplanted tissues are also considered for B-cell depletion (48).
2. Systemic diseases
2.1. Systemic lupus erythematosus
SLE is an autoimmune disease characterized by multi-organ involvement and the production of autoantibodies that target nuclear self-antigens of two types. The first type includes antibodies to double-stranded (DS) DNA and nucleosomal components. The second type, named antibodies to extractable nuclear antigens (ENA), includes antibodies to RNA binding proteins. Anti-DS DNA antibodies play a crucial role in the inflammatory and fibrogenic mechanisms of lupus nephritis (LN), while immune complexes with anti-ENA antibodies stimulate interferon production by innate immune cells (49, 50). Pathogenicity of these antibodies was demonstrated in the human system during pregnancy with the neonatal lupus syndrome (51). All B-cell therapies discussed above were tested in SLE (52). However, only the anti-BAFF has been approved to treat patients. This may be explained by the strong correlation observed between serum levels of BAFF and disease severity. Notably, the anti-BAFF in use recognizes only the membrane-bound form of the molecule (53). The anti-CD20 has been disappointing in clinical trials, and a combination of the anti-BAFF and anti-CD20 is currently under evaluation (54). Most recently, telitaciept was approved for the treatment of active SLE in China (14). Epratuzumab failed in SLE, but a post-hoc analysis revealed efficacy in SLE patients with an associated Sjögren syndrome (SS) (55). Of note in SLE is an antibody against the type I IFN receptor recently approved (56). It is believed that such drug may affect ASC differentiation (57). Quite new in the autoimmunity field, a small cohort of refractory SLE patients has been successfully treated with CAR-T cells directed against CD19 (58).
In this systemic disease, tissue lesions with CD138+ ASCs may be observed in kidneys from LN (59). Beyond CD138, their precise phenotype has not been extensively studied. These cells express CXCR4, and CXCL-12 is upregulated in tubules and glomeruli of kidneys from LN patients (60, 61). A single cell RNA analysis recently confirmed the prevalence of the CXCL-12/CXCR4 axis for leukocyte infiltration in LN, and provided evidences that recruited leukocytes may also be a source of CXCL-12 in the inflamed tissue (62). EGC formation may be observed in these inflamed kidneys (63). In addition, expansion of effector B cells of extrafollicular origin may occur during flares (64). Interestingly, the predominance of GCs and extrafollicular B responses may vary among SLE patients (65). Regarding ASC survival factors, BAFF and APRIL have been detected in kidneys from LN (66). However, expression of their receptors has not yet been reported in kidney-infiltrating ASCs.
2.2. Anti-neutrophil cytoplasmic antibody-associated vasculitis
Anti-neutrophil cytoplasmic antibody (ANCA)-associated vasculitis (AAV) are represented by granulomatosis with polyangiitis (GPA) and microscopic polyangiitis (MPA). The antibodies are directed against cytoplasmic antigens, primarily proteinase 3 (PR3) and myeloperoxidase (MPO) from neutrophils. PR3-ANCA is associated with GPA (75%), whereas MPO-ANCA is more commonly associated with MPA (60%). Pathogenicity of ANCA was demonstrated in the human system with the transplacental transfer of MPO-ANCA and induction of renal pathologies (67). The anti-CD20 has been approved in conjunction with corticosteroids (68). Rituximab has also been licensed for remission induction in severe GPA/MPA and refractory/relapsing GPA/MPA (69). At variance, the anti-BAFF was disappointing in this disease (70). It is now tested in association with rituximab (NCT03967925).
Because of the systemic nature of this disease, ASCs have been mostly studied in the circulation. Matsumoto et al. have shown that AAV patients display higher proportions of circulating PBs and PCs as compared to healthy controls, and von Borstel et al. demonstrated that an increased frequency of these cells defined by expression of CD27 and CD38 in GPA patients during remission is related to a higher relapse risk (71, 72). The kidney may be involved in AAV with tubulointerstitial lesions containing inflammatory cells, and a case report showed structures similar to EGCs with an aggregates of T/B cells surrounded by CD138+ PCs (73, 74). To our knowledge, nothing was reported for in situ chemokine production, infiltrating ASCs and BAFF/APRIL expression in kidney lesions from AAV.
2.3. IgG4-related diseases
IgG4-related diseases (IgG4-RDs) are antibody-mediated disorders not considered as strictly of autoimmune origin but rather as a wide spectrum of fibro-inflammatory conditions affecting multiple organs and associated with infiltration of ASCs specifically producing IgG4. Nevertheless, test of patients’serum reactivity defined several autoantigens including prohibitin, annexin A11, laminin 511-E8 and galectin-3 (75). IgG4-RD involves several unrelated organs such as the pancreas, liver, kidney, lung and skin. Despite the fact that the IgG4 isotype is not able to bind complement proteins and activate Fc receptors present on immune cells, pooled IgG4 from patients on their own are nevertheless able to induce pancreatic and salivary gland injuries when injected subcutaneously in neonate mice (76). It is believed that IgG4 pathogenic autoantibodies exert their effect by an antagonism activity of the targeted autoantigen(s). As a relevant example, recombinant antibodies produced from circulating clonally related PBs defined IL-1 receptor antagonist (IL-1 RA) as a new autoantigen, and these antibodies showed an antagonistic activity against IL-1 RA (77). It should be noted that an heterogeneity exist at the level of the isotype of pathogenic autoantibodies produced in this disease, since serum IgG1 from patients also transferred the diseases in neonate mice (76). Rituximab, belimumab associated to prednisone and obexelimab, a second form of anti-CD19, are in trials (NCT01584388, NCT04660565, NCT05662241).
Ducts and acini produce CXCL-12 in IgG4-RDs affecting the pancreas (78). The receptor CXCR4 has also been detected in pancreatic lesions, but the type of cells expressing this receptor were not studied. As for AAV, elevation in total circulating PBs defined in this case by a CD19lowCD20-CD38highCD138- phenotype is a valuable biomarker in IgG4-RDs (79). Among these cells, more than half are IgG4+. EGCs are frequent in IgG4-RDs (80). The precise phenotype of infiltrating IgG4+ ASCs remains to be determined, since only CD138 expression was assessed in kidney and skin lesions (81, 82). While there are no data on BAFF expression in IgG4-RDs, APRIL is produced by CD163+ M2 macrophages (83). There are no data on BAFF-R/TACI/BCMA expression by infiltrating ASCs.
3. Organ-specific diseases
3.1. Sjögren’s syndrome
Primary Sjögren’s syndrome (pSS) is an autoimmune disease affecting among others salivary and lacrimal glands with 2/3 of the patients harboring the so-called anti-Ro/SSA and anti-La/SSB antibodies recognizing ribonucleoproteins (84). Despite their intracellular reactivities, these autoantibodies may be pathogenic, since maternal antibodies transferred via the placenta induce cutaneous lesions in neonates (85) Regarding B-cell therapies, rituximab gave contradictory results but some positive clinical efficacies could be highlighted (86). The anti-BAFF and anti-BAFF-R gave promising results in phase II (87, 88). The anti-BAFF is also currently tested associated to the anti-CD20 (NCT02631538). A trial with telitacicept was recently announced (NCT05673993). A trial with CAR T cells co-targeting CD19 and BCMA has been announced in refractory SS patients (NCT05085431).
pSS has a frequent association with EGCs averaging 30-40% (43). These EGCs have been associated to SS threatening complications with the development of B-cell lymphomas (89). ASCs are well present even in lesions with a low focus score devoid of EGCs (90). Regarding homing, ASCs are found in the vicinity of CXCL-12 expressing cells including epithelial cells, infiltrating leukocytes and adipocytes (91). Notably, CXCL-12 upregulation was also seen in cases with EGCs, implying that it may not only act as a recruitment but also as a retention factor. Another study reported an upregulation of CXCL-9/-10 in pSS compared to healthy salivary glands (92). In EGC+ cases, most ASCs identified by their CD138 expression did not proliferate based on Ki67 reactivity. This observation is of particular importance, and we recently confirmed this finding by imaging mass cytometry with most if not all CD138+ cells outside EGCs negative for Ki67 (Figure 1, data not published). Indeed, it shows that ASCs produced locally differentiate further than the PB stage in the autoimmune tissue. Notably, it has been possible to identify in this disease autoreactive ASCs in lesions by using tagged recombinant autoantigens as detection reagents. By this approach, ASCs binding the Ro52 and Ro60 autoantigens were described among CD20-CD19+ cells (93–95). Regarding survival factors, pSS is one exception among autoimmune tissues characterized by the absence of APRIL expression, whose expression is usually associated with inflammation (96). At variance, BAFF is well expressed, but the expression of BAFF-R on ASCs of pSS has not yet been assessed (97).
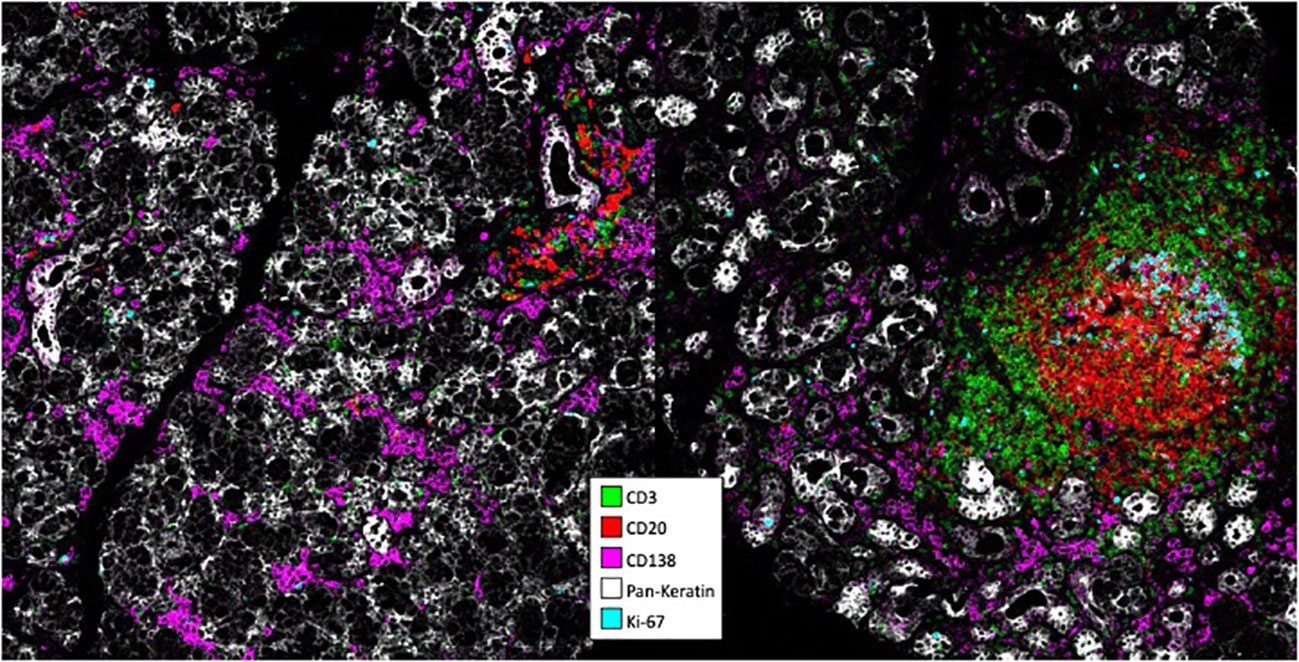
Figure 1 Analysis of pSS lesions with imaging mass cytometry. Formalin-ixed paraffin-embedded minor salivary gland biopsies corresponding to one pSS patient with mild infitration and one patient with an ectopic germinal center were stained with antibodies conjugated to metal tags using the MaxPar® labeling kit. Stainings were visualized on a Hyperion. Imaging system coupled to a Helios mass cytometer.
3.2. Rheumatoid arthritis
RA is an autoimmune disease showing inflammation in the synovial tissue and affecting joints. RA is associated with autoantibodies against citrunillated protein antigens (ACPA). Anti-ACPA are present in more than 80% and 50% in advanced and early RA, respectively. A common mouse model for RA is the transfer of an antibody against the glucose-6-phosphate isomerase into mice (98). As discussed earlier, RA was the first autoimmune disease with an approved B-cell therapy in the form of an anti-CD20. Three phase II trials were performed with atacicept with only one showing a positive clinical response (99). Adding atacicept to rituximab did not ameliorate efficacy (100) A phase II study showed promising results with the anti-BAFF (101). The anti-BAFF-R is in trial (NCT03574545).
As for pSS, EGCs are frequent in RA averaging 40% (43). EGCs are necessarily associated with increased synovium inflammation that may extend systemically, but not necessarily associated to the presence of anti-ACPA (102). ASCs may also be present even in the absence of these EGCs in the early phase of the disease (103). The chemokines CXCL-9/-10 together with expression of their receptor CXCR3 on ASCs have been detected in the synovial tissue of patients (104, 105). CXCL-12 is also upregulated, and CXCR-4 has been reported in ASCS from synovial tissues at the mRNA level (106). Notably, Scheel et al. reported that B cells may differentiate into ASCs within synovial tissues devoid of EGCs (107). In cases positive for EGCs, ASCs present outside EGCs have a non-proliferating phenotype being CD20- and expressing high level of CD38 and CD138 (108, 109). This confirms the observation made in pSS indicating that ASCs may differentiate to the PC stage in an autoimmune tissue. However, a recent single cell RNAseq analysis combined to mass cytometry classifies the majority of ASCs present in synovial tissues as PBs (110). Autoreactivity of ASCs in RA was also probed with tagged-ACPA, and showed that a subset of CD20-CD138+ ASCs residing outside EGCs were producing autoantibodies (111). Autoreactivity against ACPA was further confirmed with the recombinant antibody technology (112). These ASCs in RA lesions were probed t be non-susceptible to rituximab treatment (113). BAFF and APRIL produced by synovial fibroblasts as well as myeloid cells in the case of APRIL are present in inflamed synovial tissues (114–117). Data regarding BAFF-R, TACI and BCMA expression on ASCs at the protein level are missing. However, BCMA and TACI has been described in ASCs from synovial tissues at the mRNA level (112).
3.3. Liver autoimmune diseases
The liver may suffer from an autoimmune attack in mainly two different situations, the autoimmune hepatitis (AIH) and the primary biliary cholangitis (PBC). Two types of AIH have been described according to autoantibody reactivity in patient serum. Type 1 AIH is defined by anti-smooth muscle antibodies directed against actin filaments and associated or not with anti-nuclear antibodies. Type 2 AIH is associated to autoantibodies more specific to the tissue with the so-called liver-kidney microsomal 1 directed against cytochrome P450-2D6, and/or liver-cytosol 1 targeting forminotransferase-cyclodeaminase antibodies. Autoantigens in PBC originate from the mitochondria. AIH-like symptoms were reproduced in mice by passive transfer of an antibody reactive against the hepatocyte surface of IgM isotype and derived from a type 1 AIH patient (118). To our knowledge, induction of PBC by transfer of anti-mitochondrial antibodies from patients in an animal model has not been reported. Quite surprisingly, there is only the anti-BAFF-R in trial for AIH. This is likely explained by the fact that steroid and/or anti-mitotic agents are highly effective in AIH with a limited number of patients estimated around 10% failing. Rituximab and to a lesser extent belimumab were tested with some promising successes in steroid non-responding patients from non-randomized trials (119–122). Notably, rituximab was reported effective in cases of refractory AIH/PBC overlap (123). However, no clinical trials have been announced yet. Rituximab failed in phase II for PBC despite reduction in anti-mitochondrial antibodies (124). To the best of our knowledge, EGCs have not been defined in AIH. Despite this, ASC infiltration is common, ranging to about 2/3 of cases (125). Since seropositivity for autoantibodies is now considered to be superior to 90% in AIH if one considers all autoantibodies described in this disease and not only the type-specific ones (126), seropositive AIH is composed of a least two subtypes, one with and one without ASC infiltration. There are no data regarding ASC specific chemokines in AIH lesions. In addition to the absence of EGCs, this is raising questions regarding ASC homing in AIH livers. CD138+ PCs are observed in AIH lesions (127), but their precise phenotype warrants further investigations. At variance, ASC chemokines including CXCL-9/-10/-12 have been reported in PBC livers (128, 129). As a likely consequence, ASCs also infiltrate PBC livers. ASC infiltration is one common histological hallmark between AIH and PBC. One difference is the more prominent fraction of ASCs secreting switched IgG over IgM antibodies in AIH observed by several independent groups (130–132). Despite the fact that no studies are reporting T/B aggregates with the presence of CD21+ FDCs, EGCs are likely in PBC according to the study reporting the presence of PD-1+ ICOS+ T follicular helper (TFH) aggregated with CD20+ B cells (133). In PBC, a significant fraction of CD38highCD138+ ASCs expresses CD19 (134). These ASCs secrete antibodies against the PDC-E2 mitochondrial antigen. There is currently no data on the production of BAFF and APRIL in autoimmune livers.
3.4. Autoimmune thyroiditis
The thyroid may be subjected to a humoral autoimmune reaction with the Hashimoto thyroiditis (HT) and Graves disease (GD). HT and GD harbor anti-thyroperoxydase/thyroglobulin and anti-thyroid stimulating receptor, respectively. GD may be further associated to orbitopathy. Pathogenicity of these autoantibodies has been clearly established in the human system with spontaneous abortion problems (135, 136). Hypothyroidism in HT is well managed by hormone substitution therapies. Clinical management of GD is more complex, especially in the case of associated orbitopathy. Rituximab was first tested in GD not associated to orbitopathy. A decrease in pathogenic antibodies despite clinical improvement in some patients was not always correlated (137–139). This suggests another role for B cells than the production of autoantibodies in the pathogenesis of GD. Two clinical trials were achieved with rituximab in GD-associated orbitopathy giving controversial effects (140, 141). Rituximab may be effective when administered early in disease active patients, but additional data are needed before any conclusive statement. Two other phase II trials are ongoing.
ASC infiltration and EGC genesis is a very common feature of HT, and they are a source of autoantibodies (142). EGC genesis may also occur but to a lesser extent in GD (143). Regarding ASC chemokines, CXCL-9/-10 and CXCL-9/-10/-11 produced by thyrocytes have been reported in HT and GD, respectively (144–146). CD138 staining was not extensively studied in autoimmune thyroiditis, most likely because it is also expressed at the surface of thyrocytes. One study reported CD138+ PCs in GD (147). 15% of GD associated orbitopathy cases show a selective ASC infiltration in the orbital tissue (148). Notably, upregulation of CXCL-12 in the ocular manifestation of GD has been reported (149). BAFF is produced in the EGCs from HT (150). There is no data regarding APRIL.
3.5. Multiple sclerosis
The presence of oligoclonal immunoglobulin bands in the cerebrospinal fluid (CSF) of patients and the success obtained by B-cell therapies cited in the introduction led to an increased interest in the targeting of humoral immunity in multiple sclerosis (MS). Since the ocrelizumab approval, trial with the anti-CD19 gave trendy results (151). Atacicept failed in MS because of an unexpected disease exacerbation (152). Animals models provided several explanations (153–155). Nevertheless, atacicept failure might not have been directly due to BAFF/APRIL antagonism, since a new trial with telitacicept is ongoing in MS (14). BAFF-only treatment with tabalumab gave no significant results (156). As of today, the disease has never been transferred in an animal with the systemic injection of antibodies directed against myelin-associated products (157). However, systemic injection of these autoantibodies decreased the number of encephalitogenic T cells required to induce demyelinating lesions in the mouse CNS, indicating that the humoral immunity may not induce the disease but participates in its propagation. In patients, one subtype of MS is associated with antibody/complement-mediated demyelination (158). All together, these confirm the potential value to target ASCs in MS.
Non-proliferative terminally differentiated CD138+ PCs have been reported in the CNS parenchyma from MS patients (159). However, most of the reports regarding local ASCs in MS concerns the cerebrospinal fluid (CSF). CXCL-12 was reported upregulated in the parenchyma and CSF of MS patients (160). CSF ASCs expressed CD138, but were nevertheless classified as PBs due to their coexpression of CD19 and HLA-DR (161). While CD19 expression might now be controversial as discussed earlier to distinguish ASC differentiation stages, expression of HLA-DR has been excluded from the PC stage, at least in bone marrow (162). These PBs were detected throughout the disease course in patients, and their number correlated to the level of intrathecal immunoglobulins. This indicates that short-lived PBs are the main ASCs in CSF from MS patients. Arguing in favor of the later, a continuous replenishment of these cells was evidenced by B-cell differentiation into ASCs within the CSF (163, 164). Furthermore, EGCs containing an aggregate of T and B cells, a network of CD21+ FDCs, and surrounded by CD138+ ASCs have also been detected in meninges of MS patients presenting the secondary progressive form of the disease (165, 166). Upregulation of BAFF and APRIL in the CSF of MS patients is controversial (167, 168). While there is no direct data about the expression of BCMA/TACI on the surface of in situ ASCs, the elevated presence in the CSF of protease-cleaved soluble BCMA and TACI, a natural process regulating their surface expression, indicates that they may be expressed on CSF ASCs (169, 170). We and others detected BAFF and APRIL expression produced by astrocytes and myeloid cells, respectively, in MS lesions (155, 171). There, APRIL fulfills an immune function outside humoral immunity by triggering an IL-10-based immunosuppressive response from reactive astrocytes. It should be noted that two independent studies in the gold standard mouse model of MS, the experimental autoimmune encephalitis, revealed ASCs harboring an unexpected immunosuppressive function own to their expression of immunosuppressive cytokines such as IL-10. One group provided strong evidences for migration into the CNS during the disease course of gut-derived IL-10-producing IgA+ ASCs (154). The other group described regulatory ASCs producing IL-10 and IL-35, another cytokine with immunosuppressive function, in secondary lymphoid organs (172). Notably, ASCs producing IL-10 have been detected in CNS lesions from MS patients (173, 174). The phenotype of these cells has not yet been assessed in detail.
3.6. Neuromyelitis optica spectrum disorders
NMO is nowadays considered part of a spectrum disorders (NMOSD) of autoimmune origin affecting the central nervous system, targeting astrocytes and conducting to acute myelitis, optic neuritis and encephalitis. One hallmark of NMO is the presence of IgG autoantibodies to aquaporin 4, a receptor present at the surface of foot processes of astrocytes. Disease transfer by administration of IgG anti-aquaporin 4 from patients was demonstrated by several groups (175). As introduced above, inebilizumab has been approved in NMOSD, and rituximab is in trial (176).
CSF from NMO patients contain elevated levels of CXCL-10 (177, 178). IgG+ ASCs with a PB phenotype according to their expression of CD19 and HLA-DR are present in NMO CSF (179). As in MS, ASC differentiation in patients’ CSF has been proposed. BAFF and APRIL are upregulated in NMO CSFs (167, 180). EGCs in the orbital mass have been reported in two patients (181). These EGCs were defined by an accumulation of CD20+ B lymphocytes, BCL6 expression and surrounding ASCs of IgG and to a lesser extent IgG4 isotypes. As in MS, APRIL targets reactive astrocytes in NMO lesions (182).
3.7. Kidney allotransplants
The main challenge in solid organ allotransplantation including the kidney remains the late humoral rejection. These antibody-mediated rejections (ABMR) are the consequence of the generation of donor-specific antibodies (DSA) against mismatched human leukocyte antigens. Near 20% of the kidney recipients may develop de novo DSA by 5 years post transplantation, which leads to a high risk of graft dysfunction and a low graft survival as compared to transplantation recipient without DSA. Pathogenicity of DSA has been well established when allotransplantations were unsuccessfully performed in recipients with preformed anti-DSA. The later resulted in a mandatory donor sensitization with plasma exchange prior to graft. Correlative studies indicated that IgG3 and IgG4 anti-DSA are the two main isotypes involved in ABMR (183, 184). Rituximab-based regimen were shown to improve graft survival in 4/7 acute ABMR but only 1/7 chronic ABMR (185). The subsequent clinical trial failed for acute ABMR (186). Belimumab is promising with the reduction of de novo DSA antibody formation in a phase II trial (187). Bortezomib has been tested in late/chronic ABMR with some promising results, but the following randomized clinical trial was disappointing (188). Hence, PC targeting in late ABMR is a real concern (189). Recently, a case report with a kidney allograft patient further diagnosed with smoldering myeloma, an early form of MM, reported promising results upon treatment with daratumumab according to levels of anti-DSA and graft survival (190).
Regarding chemokines, CXCR3 with an upregulation of CXCL-11 is thought to be involved in many allograft rejections including kidney (191, 192). CXCL-12 is also elevated in chronic rejection (193). In acute rejection, ASCs are thought to be scarce accounting usually for less than 5% of the cellular infiltrate (194). Nevertheless, presence of these cells has been associated with a poor allograft function and survival (195). One study reported few cases (3%) with numerous CD138+ PCs accounting for up to 30% of the infiltrate (196). ASC-rich infiltrates tend to occur late in the rejection process. ECGs defined by an aggregate of TFH and B cells have been described in acute rejection (197). Longitudinal studies are doable in allograft patients. Proliferative lymphocyte aggregates without FDCs were reported already present in almost 50% of biopsies performed one month after transplant in clinically stable graft (198). EGCs reached 19% at 12 months, and were associated with progressive graft dysfunction. EGCs in kidney allografts appear to be resistant to rituximab treatment (199). In chronic rejection, a local production of DSA against HLA class I and II molecules was evidenced (200, 201). Both BAFF and APRIL are present in situ in ABMR (202).
4. Concluding remarks
It is commonly accepted that human clinical diseases are not single entities but composed of several subtypes. This is well-known also in autoimmune diseases. Table 1A highlights that autoimmune diseases highly differ in their susceptibility to current drugs targeting the humoral immunity. If one assumes that the biodistribution of all these antibody-based biotherapies is similar for a given tissue, Table 1 shows that diseases are biotherapy specific. When ASCs infiltrating the autoimmune tissues are considered, heterogeneity exists at several levels. The first one is the tissue of origin. It could the draining secondary lymphoid organs associated to the production of chemokines within the tissue. Table 1B shows that chemokines may be different according to the tissue considered. Alternatively, it could be inside the pathologic tissue with either clonal expansion of differentiated cells or the differentiation of precursor cells within EGCs. Regarding the latter, their presence appears to be predominant in autoimmune tissues, since only one disease, AIH, has not yet been described to contain EGCs as indicated in Table 1B. It is difficult to state if there is a specific timing of occurrence for EGCs during an autoimmune disease course without the access to longitudinal biopsies from the considered tissues. However, study in kidney transplantation revealed that EGC formation may be frequent in a tissue targeted by an immune reaction.
ASCs infiltrating autoimmune tissues have been convincingly shown to produce antibodies targeting autoantigens in several autoimmune diseases (Table 1B). This highlights their contribution, at least in part, to the pathogenicity, and warrants their targeting to test treatment improvement. However, phenotype of these cells is another level of heterogeneity rendering their targeting complex. Indeed, isotypes of the pathogenic antibodies first vary (Table 1B). More importantly, their stage of differentiation may also vary with the report of PBs and PCs (Table 1B). The latter shows that ASCs have the ability to complete their full differentiation process into PCs in some tissues. In total, an extensive phenotype characterization of ASC infiltrating autoimmune tissues now possible with high throughput technologies is warranted before any efficient ASC-targeting treatment could be designed. This approach is ongoing, and should bring key molecular targets in the near future.
Author contributions
BH designed the study and wrote the manuscript. DG, ABe, ABa, LB, MK, CD-P, GC, JN, J-OP, NS wrote the manuscript. All authors contributed to the article and approved the submitted version.
Funding
NS received a funding from the French hepatology association.
Acknowledgments
The authors are grateful to the Hyperion platform from the laboratory B lymphocytes, autoimmunity and immunotherapies (Brest, France) for imaging mass cytometry facilities.
Conflict of interest
The authors declare that the research was conducted in the absence of any commercial or financial relationships that could be construed as a potential conflict of interest.
Publisher’s note
All claims expressed in this article are solely those of the authors and do not necessarily represent those of their affiliated organizations, or those of the publisher, the editors and the reviewers. Any product that may be evaluated in this article, or claim that may be made by its manufacturer, is not guaranteed or endorsed by the publisher.
References
1. Cohen SB, Emery P, Greenwald MW, Dougados M, Furie RA, Genovese MC, et al. Rituximab for rheumatoid arthritis refractory to anti-tumor necrosis factor therapy: Results of a multicenter, randomized, double-blind, placebo-controlled, phase III trial evaluating primary efficacy and safety at twenty-four weeks. Arthritis Rheum (2006) 54(9):2793−806. doi: 10.1002/art.22025
2. Protheroe A, Edwards JC, Simmons A, Maclennan K, Selby P. Remission of inflammatory arthropathy in association with anti-CD20 therapy for non-hodgkin’s lymphoma. Rheumatol (Oxford) (1999) 38(11):1150−2. doi: 10.1093/rheumatology/38.11.1150
3. Joly P, Maho-Vaillant M, Prost-Squarcioni C, Hebert V, Houivet E, Calbo S, et al. First-line rituximab combined with short-term prednisone versus prednisone alone for the treatment of pemphigus (Ritux 3): a prospective, multicentre, parallel-group, open-label randomised trial. Lancet (2017) 389(10083):2031−40. doi: 10.1016/S0140-6736(17)30070-3
4. Guillevin L, Pagnoux C, Karras A, Khouatra C, Aumaître O, Cohen P, et al. Rituximab versus azathioprine for maintenance in ANCA-associated vasculitis. N Engl J Med (2014) 371(19):1771−80. doi: 10.1056/NEJMoa1404231
5. Hauser SL, Belachew S, Kappos L. Ocrelizumab in primary progressive and relapsing multiple sclerosis. N Engl J Med (2017) 376(17):1694. doi: 10.1056/NEJMc1702076
6. Navarra SV, Guzmán RM, Gallacher AE, Hall S, Levy RA, Jimenez RE, et al. Efficacy and safety of belimumab in patients with active systemic lupus erythematosus: a randomised, placebo-controlled, phase 3 trial. Lancet (2011) 377(9767):721−31. doi: 10.1016/S0140-6736(10)61354-2
7. Schneider P, MacKay F, Steiner V, Hofmann K, Bodmer JL, Holler N, et al. BAFF, a novel ligand of the tumor necrosis factor family, stimulates b cell growth. J Exp Med (1999) 189(11):1747−56. doi: 10.1084/jem.189.11.1747
8. Thompson JS, Bixler SA, Qian F, Vora K, Scott ML, Cachero TG, et al. BAFF-r, a newly identified TNF receptor that specifically interacts with BAFF. Science (2001) 293(5537):2108−11. doi: 10.1126/science.1061965
9. Rovin BH, Dooley MA, Radhakrishnan J, Ginzler EM, Forrester TD, Anderson PW. The impact of tabalumab on the kidney in systemic lupus erythematosus: results from two phase 3 randomized, clinical trials. Lupus (2016) 25(14):1597−601. doi: 10.1177/0961203316650734
10. Cree BAC, Bennett JL, Kim HJ, Weinshenker BG, Pittock SJ, Wingerchuk DM, et al. Inebilizumab for the treatment of neuromyelitis optica spectrum disorder (N-MOmentum): a double-blind, randomised placebo-controlled phase 2/3 trial. Lancet (2019) 394(10206):1352−63. doi: 10.1016/S0140-6736(19)31817-3
11. Samy E, Wax S, Huard B, Hess H, Schneider P. Targeting BAFF and APRIL in systemic lupus erythematosus and other antibody-associated diseases. Int Rev Immunol (2017) 36(1):3−19. doi: 10.1080/08830185.2016.1276903
12. Dörner T, Posch MG, Li Y, Petricoul O, Cabanski M, Milojevic JM, et al. Treatment of primary sjögren’s syndrome with ianalumab (VAY736) targeting b cells by BAFF receptor blockade coupled with enhanced, antibody-dependent cellular cytotoxicity. Ann Rheum Dis (2019) 78(5):641−7. doi: 10.1136/annrheumdis-2018-214720
13. Geh D, Gordon C. Epratuzumab for the treatment of systemic lupus erythematosus. Expert Rev Clin Immunol (2018) 14(4):245−58. doi: 10.1080/1744666X.2018.1450141
14. Dhillon S. Telitacicept: First approval. Drugs (2021) 81(14):1671−5. doi: 10.1007/s40265-021-01591-1
15. Scheibe F, Ostendorf L, Prüss H, Radbruch H, Aschman T, Hoffmann S, et al. Daratumumab for treatment-refractory antibody-mediated diseases in neurology. Eur J Neurol (2022) 29(6):1847−54. doi: 10.1111/ene.15266
16. Kohler S, Märschenz S, Grittner U, Alexander T, Hiepe F, Meisel A. Bortezomib in antibody-mediated autoimmune diseases (TAVAB): study protocol for a unicentric, non-randomised, non-placebo controlled trial. BMJ Open (2019) 9(1):e024523. doi: 10.1136/bmjopen-2018-024523
17. Robak E, Robak T. Bruton’s kinase inhibitors for the treatment of immunological diseases: Current status and perspectives. J Clin Med (2022) 11(10):2807. doi: 10.3390/jcm11102807
18. Hiepe F, Radbruch A. Plasma cells as an innovative target in autoimmune disease with renal manifestations. Nat Rev Nephrol (2016) 12(4):232−40. doi: 10.1038/nrneph.2016.20
19. Jourdan M, Caraux A, Caron G, Robert N, Fiol G, Rème T, et al. Characterization of a transitional preplasmablast population in the process of human b cell to plasma cell differentiation. J Immunol (2011) 187(8):3931−41. doi: 10.4049/jimmunol.1101230
20. Baker D, MacDougall A, Kang AS, Schmierer K, Giovannoni G, Dobson R. Seroconversion following COVID-19 vaccination: can we optimize protective response in CD20-treated individuals? Clin Exp Immunol (2022) 207(3):263−71. doi: 10.1093/cei/uxab015
21. Shaffer AL, Lin KI, Kuo TC, Yu X, Hurt EM, Rosenwald A, et al. Blimp-1 orchestrates plasma cell differentiation by extinguishing the mature b cell gene expression program. Immunity (2002) 17(1):51−62. doi: 10.1016/S1074-7613(02)00335-7
22. Mei HE, Schmidt S, Dörner T. Rationale of anti-CD19 immunotherapy: an option to target autoreactive plasma cells in autoimmunity. Arthritis Res Ther (2012) 14(Suppl 5):S1. doi: 10.1186/ar3909
23. Halliley JL, Tipton CM, Liesveld J, Rosenberg AF, Darce J, Gregoretti IV, et al. Long-lived plasma cells are contained within the CD19(-)CD38(hi)CD138(+) subset in human bone marrow. Immunity (2015) 43(1):132−45. doi: 10.1016/j.immuni.2015.06.016
24. Arumugakani G, Stephenson SJ, Newton DJ, Rawstron A, Emery P, Doody GM, et al. Early emergence of CD19-negative human antibody-secreting cells at the plasmablast to plasma cell transition. J Immunol (2017) 198(12):4618−28. doi: 10.4049/jimmunol.1501761
25. Carrillo-Ballesteros FJ, Oregon-Romero E, Franco-Topete RA, Govea-Camacho LH, Cruz A, Muñoz-Valle JF, et al. B-cell activating factor receptor expression is associated with germinal center b-cell maintenance. Exp Ther Med (2019) 17(3):2053–60. doi: 10.3892/etm.2019.7172
26. Zhang X, Park CS, Yoon SO, Li L, Hsu YM, Ambrose C, et al. BAFF supports human b cell differentiation in the lymphoid follicles through distinct receptors. Int Immunol (2005) 17(6):779−88. doi: 10.1093/intimm/dxh259
27. Ng LG, Sutherland APR, Newton R, Qian F, Cachero TG, Scott ML, et al. B cell-activating factor belonging to the TNF family (BAFF)-r is the principal BAFF receptor facilitating BAFF costimulation of circulating T and b cells. J Immunol (2004) 173(2):807−17. doi: 10.4049/jimmunol.173.2.807
28. Belnoue E, Pihlgren M, McGaha TL, Tougne C, Rochat AF, Bossen C, et al. APRIL is critical for plasmablast survival in the bone marrow and poorly expressed by early-life bone marrow stromal cells. Blood (2008) 111(5):2755−64. doi: 10.1182/blood-2007-09-110858
29. O’Connor BP, Raman VS, Erickson LD, Cook WJ, Weaver LK, Ahonen C, et al. BCMA is essential for the survival of long-lived bone marrow plasma cells. J Exp Med (2004) 199(1):91−8. doi: 10.1084/jem.20031330
30. Mackay F, Schneider P. TACI, an enigmatic BAFF/APRIL receptor, with new unappreciated biochemical and biological properties. Cytokine Growth Factor Rev (2008) 19(3-4):263−76. doi: 10.1016/j.cytogfr.2008.04.006
31. Smulski CR, Zhang L, Burek M, Teixidó Rubio A, Briem JS, Sica MP, et al. Ligand-independent oligomerization of TACI is controlled by the transmembrane domain and regulates proliferation of activated b cells. Cell Rep (2022) 38(13):110583. doi: 10.1016/j.celrep.2022.110583
32. Garcia-Carmona Y, Ting AT, Radigan L, Athuluri Divakar SK, Chavez J, Meffre E, et al. TACI isoforms regulate ligand binding and receptor function. Front Immunol (2018) 9:2125. doi: 10.3389/fimmu.2018.02125
33. Moreaux J, Hose D, Jourdan M, Reme T, Hundemer M, Moos M, et al. TACI expression is associated with a mature bone marrow plasma cell signature and c-MAF overexpression in human myeloma cell lines. Haematologica (2007) 92(6):803−11. doi: 10.3324/haematol.10574
34. Moreaux J, Cremer FW, Reme T, Raab M, Mahtouk K, Kaukel P, et al. The level of TACI gene expression in myeloma cells is associated with a signature of microenvironment dependence versus a plasmablastic signature. Blood (2005) 106(3):1021−30. doi: 10.1182/blood-2004-11-4512
35. Darce JR, Arendt BK, Wu X, Jelinek DF. Regulated expression of BAFF-binding receptors during human b cell differentiation. J Immunol (2007) 179(11):7276−86. doi: 10.4049/jimmunol.179.11.7276
36. Bossen C, Cachero TG, Tardivel A, Ingold K, Willen L, Dobles M, et al. TACI, unlike BAFF-r, is solely activated by oligomeric BAFF and APRIL to support survival of activated b cells and plasmablasts. Blood (2008) 111(3):1004−12. doi: 10.1182/blood-2007-09-110874
37. Miao YR, Thakkar K, Cenik C, Jiang D, Mizuno K, Jia C, et al. Developing high-affinity decoy receptors to treat multiple myeloma and diffuse large b cell lymphoma. J Exp Med (2022) 219(9):e20220214. doi: 10.1084/jem.20220214
38. Day ES, Cachero TG, Qian F, Sun Y, Wen D, Pelletier M, et al. Selectivity of BAFF/BLyS and APRIL for binding to the TNF family receptors BAFFR/BR3 and BCMA. Biochemistry (2005) 44(6):1919−31. doi: 10.1021/bi048227k
39. Haselmayer P, Vigolo M, Nys J, Schneider P, Hess H. A mouse model of systemic lupus erythematosus responds better to soluble TACI than to soluble BAFFR, correlating with depletion of plasma cells. Eur J Immunol (2017) 47(6):1075−85. doi: 10.1002/eji.201746934
40. Daratumumab. In: LiverTox: Clinical and research information on drug-induced liver injury. (Bethesda, MD: National Institute of Diabetes and Digestive and Kidney Diseases). Available at: http://www.ncbi.nlm.nih.gov/books/NBK548759/.
41. March S, Graupera M, Rosa Sarrias M, Lozano F, Pizcueta P, Bosch J, et al. Identification and functional characterization of the hepatic stellate cell CD38 cell surface molecule. Am J Pathol (2007) 170(1):176−87. doi: 10.2353/ajpath.2007.051212
42. Eickenberg S, Mickholz E, Jung E, Nofer JR, Pavenstadt HJ, Jacobi AM. Mycophenolic acid counteracts b cell proliferation and plasmablast formation in patients with systemic lupus erythematosus. Arthritis Res Ther (2012) 14(3):R110. doi: 10.1186/ar3835
43. Bombardieri M, Lewis M, Pitzalis C. Ectopic lymphoid neogenesis in rheumatic autoimmune diseases. Nat Rev Rheumatol (2017) 13(3):141−54. doi: 10.1038/nrrheum.2016.217
44. Cyster JG. Homing of antibody secreting cells. Immunol Rev (2003) 194:48−60. doi: 10.1034/j.1600-065X.2003.00041.x
45. Hauser AE, Debes GF, Arce S, Cassese G, Hamann A, Radbruch A, et al. Chemotactic responsiveness toward ligands for CXCR3 and CXCR4 is regulated on plasma blasts during the time course of a memory immune response. J Immunol (2002) 169(3):1277−82. doi: 10.4049/jimmunol.169.3.1277
46. Song A, Jiang A, Xiong W, Zhang C. The role of CXCL12 in kidney diseases: A friend or foe? Kidney Dis (Basel) (2021) 7(3):176−85. doi: 10.1159/000514913
47. Karin N. The multiple faces of CXCL12 (SDF-1alpha) in the regulation of immunity during health and disease. J Leukoc Biol (2010) 88(3):463−73. doi: 10.1189/jlb.0909602
48. Dragun D, Rudolph B. Novelties in diagnostics and therapy of antibody mediated rejection. Nephrol Dial Transplant (2007) 22(Suppl 8):viii50−3. doi: 10.1093/ndt/gfm647
49. Pisetsky DS. The central role of nucleic acids in the pathogenesis of systemic lupus erythematosus. F1000Res (2019) 8:F1000. doi: 10.12688/f1000research.17959.1
50. Wang X, Xia Y. Anti-double stranded DNA antibodies: Origin, pathogenicity, and targeted therapies. Front Immunol (2019) 10:1667. doi: 10.3389/fimmu.2019.01667
51. Limaye MA, Buyon JP, Cuneo BF, Mehta-Lee SS. A review of fetal and neonatal consequences of maternal systemic lupus erythematosus. Prenat Diagn. (2020) 40(9):1066−76. doi: 10.1002/pd.5709
52. Bryl E. B cells as target for immunotherapy in rheumatic diseases - current status. Immunol Lett (2021) 236:12−9. doi: 10.1016/j.imlet.2021.05.006
53. Kowalczyk-Quintas C, Chevalley D, Willen L, Jandus C, Vigolo M, Schneider P. Inhibition of membrane-bound BAFF by the anti-BAFF antibody belimumab. Front Immunol (2018) 9:2698. doi: 10.3389/fimmu.2018.02698
54. Parodis I, Stockfelt M, Sjöwall C. B cell therapy in systemic lupus erythematosus: From rationale to clinical practice. Front Med (Lausanne). (2020) 7:316. doi: 10.3389/fmed.2020.00316
55. Gottenberg JE, Dörner T, Bootsma H, Devauchelle-Pensec V, Bowman SJ, Mariette X, et al. Efficacy of epratuzumab, an anti-CD22 monoclonal IgG antibody. In: Systemic lupus erythematosus patients with associated sjögren’s syndrome: Post hoc analyses from the EMBODY trials. arthritis rheumatol, (2018). 50:763−73. doi: 10.1002/art.40425
56. Morand EF, Furie R, Tanaka Y, Bruce IN, Askanase AD, Richez C, et al. Trial of anifrolumab in active systemic lupus erythematosus. N Engl J Med (2020) 382(3):211−21. doi: 10.1056/NEJMoa1912196
57. Kiefer K, Oropallo MA, Cancro MP, Marshak-Rothstein A. Role of type I interferons in the activation of autoreactive b cells. Immunol Cell Biol (2012) 90(5):498−504. doi: 10.1038/icb.2012.10
58. Mackensen A, Müller F, Mougiakakos D, Böltz S, Wilhelm A, Aigner M, et al. Anti-CD19 CAR T cell therapy for refractory systemic lupus erythematosus. Nat Med (2022) 28(10):2124−32. doi: 10.1038/s41591-022-02017-5
59. Espeli M, Bökers S, Giannico G, Dickinson HA, Bardsley V, Fogo AB, et al. Local renal autoantibody production in lupus nephritis. J Am Soc Nephrol. (2011) 22(2):296−305. doi: 10.1681/ASN.2010050515
60. Wang A, Guilpain P, Chong BF, Chouzenoux S, Guillevin L, Du Y, et al. Dysregulated expression of CXCR4/CXCL12 in subsets of patients with systemic lupus erythematosus. Arthritis Rheum (2010) 62(11):3436−46. doi: 10.1002/art.27685
61. Ma K, Li J, Wang X, Lin X, Du W, Yang X, et al. TLR4+CXCR4+ plasma cells drive nephritis development in systemic lupus erythematosus. Ann Rheum Dis (2018) 77(10):1498−506. doi: 10.1136/annrheumdis-2018-213615
62. Arazi A, Rao DA, Berthier CC, Davidson A, Liu Y, Hoover PJ, et al. The immune cell landscape in kidneys of patients with lupus nephritis. Nat Immunol (2019) 20(7):902−14. doi: 10.1038/s41590-019-0398-x
63. Bird AK, Meednu N, Anolik JH. New insights into b cell biology in systemic lupus erythematosus and sjögren’s syndrome. Curr Opin Rheumatol (2015) 27(5):461−7. doi: 10.1097/BOR.0000000000000201
64. Tipton CM, Fucile CF, Darce J, Chida A, Ichikawa T, Gregoretti I, et al. Diversity, cellular origin and autoreactivity of antibody-secreting cell population expansions in acute systemic lupus erythematosus. Nat Immunol (2015) 16(7):755−65. doi: 10.1038/ni.3175
65. Malkiel S, Barlev AN, Atisha-Fregoso Y, Suurmond J, Diamond B. Plasma cell differentiation pathways in systemic lupus erythematosus. Front Immunol (2018) 9:427. doi: 10.3389/fimmu.2018.00427
66. Neusser MA, Lindenmeyer MT, Edenhofer I, Gaiser S, Kretzler M, Regele H, et al. Intrarenal production of b-cell survival factors in human lupus nephritis. Mod Pathol (2011) 24(1):98−107. doi: 10.1038/modpathol.2010.184
67. Jennette JC, Xiao H, Falk RJ. Pathogenesis of vascular inflammation by anti-neutrophil cytoplasmic antibodies. J Am Soc Nephrol (2006) 17(5):1235−42. doi: 10.1681/ASN.2005101048
68. Latimer NR, Carroll C, Wong R, Tappenden P, Venning MC, Luqmani R. Rituximab in combination with corticosteroids for the treatment of anti-neutrophil cytoplasmic antibody-associated vasculitis: a NICE single technology appraisal. Pharmacoeconomics (2014) 32(12):1171−83. doi: 10.1007/s40273-014-0189-z
69. Lutalo PMK, D’Cruz DP. Biological drugs in ANCA-associated vasculitis. Int Immunopharmacol (2015) 27(2):209−12. doi: 10.1016/j.intimp.2015.04.023
70. Jayne D, Blockmans D, Luqmani R, Moiseev S, Ji B, Green Y, et al. Efficacy and safety of belimumab and azathioprine for maintenance of remission in antineutrophil cytoplasmic antibody-associated vasculitis: A randomized controlled study. Arthritis Rheumatol (2019) 71(6):952−63. doi: 10.1002/art.40802
71. von Borstel A, Land J, Abdulahad WH, Rutgers A, Stegeman CA, Diepstra A, et al. CD27+CD38hi b cell frequency during remission predicts relapsing disease in granulomatosis with polyangiitis patients. Front Immunol (2019) 10:2221. doi: 10.3389/fimmu.2019.02221
72. Matsumoto K, Suzuki K, Yoshimoto K, Seki N, Tsujimoto H, Chiba K, et al. Significant association between clinical characteristics and immuno-phenotypes in patients with ANCA-associated vasculitis. Rheumatol (Oxford) (2020) 59(3):545−53. doi: 10.1093/rheumatology/kez327
73. Lin ZS, Liu XL, Cui Z, Wang SX, Yu F, Zhou FD, et al. Acute tubulointerstitial nephritis with germinal centers in antineutrophil cytoplasmic antibody-associated vasculitis: A case report and literature review. Med (Baltimore) (2019) 98(48):e18178. doi: 10.1097/MD.0000000000018178
74. Zonozi R, Niles JL, Cortazar FB. Renal involvement in antineutrophil cytoplasmic antibody-associated vasculitis. Rheum Dis Clin North Am (2018) 44(4):525−43. doi: 10.1016/j.rdc.2018.06.001
75. Liu J, Yin W, Westerberg LS, Lee P, Gong Q, Chen Y, et al. Immune dysregulation in IgG4-related disease. Front Immunol (2021) 12:738540. doi: 10.3389/fimmu.2021.738540
76. Shiokawa M, Kodama Y, Kuriyama K, Yoshimura K, Tomono T, Morita T, et al. Pathogenicity of IgG in patients with IgG4-related disease. Gut (2016) 65(8):1322−32. doi: 10.1136/gutjnl-2015-310336
77. Jarrell JA, Baker MC, Perugino CA, Liu H, Bloom MS, Maehara T, et al. Neutralizing anti-IL-1 receptor antagonist autoantibodies induce inflammatory and fibrotic mediators in IgG4-related disease. J Allergy Clin Immunol (2022) 149(1):358−68. doi: 10.1016/j.jaci.2021.05.002
78. Capecchi R, Croia C, Puxeddu I, Pratesi F, Cacciato A, Campani D, et al. CXCL12/SDF-1 in IgG4-related disease. Front Pharmacol (2021) 12:750216. doi: 10.3389/fphar.2021.750216
79. Wallace ZS, Mattoo H, Carruthers M, Mahajan VS, Della Torre E, Lee H, et al. Plasmablasts as a biomarker for IgG4-related disease, independent of serum IgG4 concentrations. Ann Rheum Dis (2015) 74(1):190−5. doi: 10.1136/annrheumdis-2014-205233
80. Maehara T, Moriyama M, Nakashima H, Miyake K, Hayashida JN, Tanaka A, et al. Interleukin-21 contributes to germinal centre formation and immunoglobulin G4 production in IgG4-related dacryoadenitis and sialoadenitis, so-called mikulicz’s disease. Ann Rheum Dis (2012) 71(12):2011−9. doi: 10.1136/annrheumdis-2012-201477
81. Mizushima I, Yamada K, Harada K, Matsui S, Saeki T, Kondo S, et al. Diagnostic sensitivity of cutoff values of IgG4-positive plasma cell number and IgG4-positive/CD138-positive cell ratio in typical multiple lesions of patients with IgG4-related disease. Mod Rheumatol (2018) 28(2):293−9. doi: 10.1080/14397595.2017.1332540
82. Wang R, He D, Zhao L, Liang S, Liang D, Xu F, et al. Role of complement system in patients with biopsy-proven immunoglobulin G4-related kidney disease. Hum Pathol (2018) 81:220−8. doi: 10.1016/j.humpath.2018.07.008
83. Kawakami T, Mizushima I, Yamada K, Fujii H, Ito K, Yasuno T, et al. Abundant a proliferation-inducing ligand (APRIL)-producing macrophages contribute to plasma cell accumulation in immunoglobulin G4-related disease. Nephrol Dial Transplant (2018) 34(6):960–69. doi: 10.1093/ndt/gfy296
84. Mariette X, Criswell LA. Primary sjögren’s syndrome. N Engl J Med (2018) 379(1):97. doi: 10.1056/NEJMcp1702514
85. Miyagawa S, Shinohara K, Kidoguchi K, Fujita T, Fukumoto T, Hashimoto K, et al. Neonatal lupus erythematosus: studies on HLA class II genes and autoantibody profiles in Japanese mothers. Autoimmunity (1997) 26(2):95−101. doi: 10.3109/08916939709003852
86. Chen YH, Wang XY, Jin X, Yang Z, Xu J. Rituximab therapy for primary sjögren’s syndrome. Front Pharmacol (2021) 12:731122. doi: 10.3389/fphar.2021.731122
87. Bowman SJ, Fox R, Dörner T, Mariette X, Papas A, Grader-Beck T, et al. Safety and efficacy of subcutaneous ianalumab (VAY736) in patients with primary sjögren’s syndrome: a randomised, double-blind, placebo-controlled, phase 2b dose-finding trial. Lancet (2022) 399(10320):161−71. doi: 10.1016/S0140-6736(21)02251-0
88. De Vita S, Quartuccio L, Seror R, Salvin S, Ravaud P, Fabris M, et al. Efficacy and safety of belimumab given for 12 months in primary sjögren’s syndrome: the BELISS open-label phase II study. Rheumatol (Oxford) (2015) 54(12):2249−56. doi: 10.1093/rheumatology/kev257
89. Nocturne G, Pontarini E, Bombardieri M, Mariette X. Lymphomas complicating primary sjögren’s syndrome: from autoimmunity to lymphoma. Rheumatol (Oxford) (2019) 60(8):kez052. doi: 10.1093/rheumatology/kez052
90. Mielle J, Tison A, Cornec D, Le Pottier L, Daien C, Pers JO. B cells in sjögren’s syndrome: from pathophysiology to therapeutic target. Rheumatol (Oxford) (2019) key332. doi: 10.1093/rheumatology/key332
91. Szyszko EA, Brokstad KA, Oijordsbakken G, Jonsson MV, Jonsson R, Skarstein K. Salivary glands of primary sjögren’s syndrome patients express factors vital for plasma cell survival. Arthritis Res Ther (2011) 13(1):R2. doi: 10.1186/ar3220
92. Ogawa N, Ping L, Zhenjun L, Takada Y, Sugai S. Involvement of the interferon-gamma-induced T cell-attracting chemokines, interferon-gamma-inducible 10-kd protein (CXCL10) and monokine induced by interferon-gamma (CXCL9), in the salivary gland lesions of patients with sjögren’s syndrome. Arthritis Rheumatol (2002) 46(10):2730−41. doi: 10.1002/art.10577
93. Salomonsson S, Jonsson MV, Skarstein K, Brokstad KA, Hjelmström P, Wahren-Herlenius M, et al. Cellular basis of ectopic germinal center formation and autoantibody production in the target organ of patients with sjögren’s syndrome. Arthritis Rheum (2003) 48(11):3187−201. doi: 10.1002/art.11311
94. Aqrawi LA, Skarstein K, Øijordsbakken G, Brokstad KA. Ro52- and Ro60-specific b cell pattern in the salivary glands of patients with primary sjögren’s syndrome. Clin Exp Immunol (2013) 172(2):228−37. doi: 10.1111/cei.12058
95. Skarstein K, Jensen JL, Galtung H, Jonsson R, Brokstad K, Aqrawi LA. Autoantigen-specific b cells and plasma cells are prominent in areas of fatty infiltration in salivary glands of patients with primary sjögren’s syndrome. Autoimmunity (2019) 52(7−8):242−50. doi: 10.1080/08916934.2019.1684475
96. Lombardi T, Moll S, Youinou P, Pers JO, Tzankov A, Gabay C, et al. Absence of up-regulation for a proliferation-inducing ligand in sjogren’s sialadenitis lesions. Rheumatology (2011) 50(7):1211−5. doi: 10.1093/rheumatology/ker016
97. Carrillo-Ballesteros FJ, Palafox-Sánchez CA, Franco-Topete RA, Muñoz-Valle JF, Orozco-Barocio G, Martínez-Bonilla GE, et al. Expression of BAFF and BAFF receptors in primary sjögren’s syndrome patients with ectopic germinal center-like structures. Clin Exp Med (2020) 20(4):615−26. doi: 10.1007/s10238-020-00637-0
98. Matsumoto I, Staub A, Benoist C, Mathis D. Arthritis provoked by linked T and b cell recognition of a glycolytic enzyme. Science (1999) 286(5445):1732−5. doi: 10.1126/science.286.5445.1732
99. Kaegi C, Steiner UC, Wuest B, Crowley C, Boyman O. Systematic review of safety and efficacy of atacicept in treating immune-mediated disorders. Front Immunol (2020) 11:433. doi: 10.3389/fimmu.2020.00433
100. van Vollenhoven RF, Wax S, Li Y, Tak PP. Safety and efficacy of atacicept in combination with rituximab for reducing the signs and symptoms of rheumatoid arthritis: a phase II, randomized, double-blind, placebo-controlled pilot trial. Arthritis Rheumatol (2015) 67(11):2828−36. doi: 10.1002/art.39262
101. Stohl W, Merrill JT, McKay JD, Lisse JR, Zhong ZJ, Freimuth WW, et al. Efficacy and safety of belimumab in patients with rheumatoid arthritis: a phase II, randomized, double-blind, placebo-controlled, dose-ranging study. J Rheumatol (2013) 40(5):579−89. doi: 10.3899/jrheum.120886
102. Thurlings RM, Wijbrandts CA, Mebius RE, Cantaert T, Dinant HJ, van der Pouw-Kraan TCTM, et al. Synovial lymphoid neogenesis does not define a specific clinical rheumatoid arthritis phenotype. Arthritis Rheumatol (2008) 58(6):1582−9. doi: 10.1002/art.23505
103. Kamogawa J, Takubo N, Arita N, Okada K, Yamamoto H, Yamamoto S, et al. Histopathological characteristics of early rheumatoid arthritis: a case one month after clinical onset. Mod Rheumatol (2000) 10(4):272−5. doi: 10.3109/s101650070016
104. Iwamoto T, Okamoto H, Toyama Y, Momohara S. Molecular aspects of rheumatoid arthritis: chemokines in the joints of patients. FEBS J (2008) 275(18):4448−55. doi: 10.1111/j.1742-4658.2008.06580.x
105. Tsubaki T, Takegawa S, Hanamoto H, Arita N, Kamogawa J, Yamamoto H, et al. Accumulation of plasma cells expressing CXCR3 in the synovial sublining regions of early rheumatoid arthritis in association with production of Mig/CXCL9 by synovial fibroblasts. Clin Exp Immunol (2005) 141(2):363−71. doi: 10.1111/j.1365-2249.2005.02850.x
106. Janssens R, Struyf S, Proost P. Pathological roles of the homeostatic chemokine CXCL12. Cytokine Growth Factor Rev (2018) 44:51−68. doi: 10.1016/j.cytogfr.2018.10.004
107. Scheel T, Gursche A, Zacher J, Häupl T, Berek C. V-Region gene analysis of locally defined synovial b and plasma cells reveals selected b cell expansion and accumulation of plasma cell clones in rheumatoid arthritis. Arthritis Rheumatol (2011) 63(1):63−72. doi: 10.1002/art.27767
108. Rivellese F, Mauro D, Nerviani A, Pagani S, Fossati-Jimack L, Messemaker T, et al. Mast cells in early rheumatoid arthritis associate with disease severity and support b cell autoantibody production. Ann Rheum Dis (2018) 77(12):1773−81. doi: 10.1136/annrheumdis-2018-213418
109. Kim HJ, Berek C. B cells in rheumatoid arthritis. Arthritis Res (2000) 2(2):126−31. doi: 10.1186/ar77
110. Zhang F, Wei K, Slowikowski K, Fonseka CY, Rao DA, Kelly S, et al. Defining inflammatory cell states in rheumatoid arthritis joint synovial tissues by integrating single-cell transcriptomics and mass cytometry. Nat Immunol (2019) 20(7):928−42. doi: 10.1038/s41590-019-0378-1
111. Humby F, Bombardieri M, Manzo A, Kelly S, Blades MC, Kirkham B, et al. Ectopic lymphoid structures support ongoing production of class-switched autoantibodies in rheumatoid synovium. PloS Med (2009) 6(1):e1. doi: 10.1371/journal.pmed.0060001
112. Hardt U, Carlberg K, Af Klint E, Sahlström P, Larsson L, van Vollenhoven A, et al. Integrated single cell and spatial transcriptomics reveal autoreactive differentiated b cells in joints of early rheumatoid arthritis. Sci Rep (2022) 12(1):11876. doi: 10.1038/s41598-022-15293-5
113. Teng YKO, Levarht EWN, Hashemi M, Bajema IM, Toes REM, Huizinga TWJ, et al. Immunohistochemical analysis as a means to predict responsiveness to rituximab treatment. Arthritis Rheum (2007) 56(12):3909−18. doi: 10.1002/art.22967
114. Gabay C, Krenn V, Bosshard C, Seemayer CA, Chizzolini C, Huard B. Synovial tissues concentrate secreted APRIL. Arthritis Res Ther (2009) 11(5):R144. doi: 10.1186/ar2817
115. Ohata J, Zvaifler NJ, Nishio M, Boyle DL, Kalled SL, Carson DA, et al. Fibroblast-like synoviocytes of mesenchymal origin express functional b cell-activating factor of the TNF family in response to proinflammatory cytokines. J Immunol (2005) 174(2):864−70. doi: 10.4049/jimmunol.174.2.864
116. Alsaleh G, Messer L, Semaan N, Boulanger N, Gottenberg JE, Sibilia J, et al. BAFF synthesis by rheumatoid synoviocytes is positively controlled by alpha5beta1 integrin stimulation and is negatively regulated by tumor necrosis factor alpha and toll-like receptor ligands. Arthritis Rheumatol (2007) 56(10):3202−14. doi: 10.1002/art.22915
117. Bombardieri M, Kam NW, Brentano F, Choi K, Filer A, Kyburz D, et al. A BAFF/APRIL-dependent TLR3-stimulated pathway enhances the capacity of rheumatoid synovial fibroblasts to induce AID expression and ig class-switching in b cells. Ann Rheum Dis (2011) 70(10):1857−65. doi: 10.1136/ard.2011.150219
118. Yamauchi K, Yamaguchi N, Furukawa T, Takatsu K, Nakanishi T, Ishida K, et al. A murine model of acute liver injury induced by human monoclonal autoantibody. Hepatology (2005) 42(1):149−55. doi: 10.1002/hep.20726
119. Burak KW, Swain MG, Santodomingo-Garzon T, Santodomino-Garzon T, Lee SS, Urbanski SJ, et al. Rituximab for the treatment of patients with autoimmune hepatitis who are refractory or intolerant to standard therapy. Can J Gastroenterol (2013) 27(5):273−80. doi: 10.1155/2013/512624
120. D’Agostino D, Costaguta A, Álvarez F. Successful treatment of refractory autoimmune hepatitis with rituximab. Pediatrics (2013) 132(2):e526–530. doi: 10.1542/peds.2011-1900
121. Than NN, Hodson J, Schmidt-Martin D, Taubert R, Wawman RE, Botter M, et al. Efficacy of rituximab in difficult-to-manage autoimmune hepatitis: Results from the international autoimmune hepatitis group. JHEP Rep (2019) 1(6):437−45. doi: 10.1016/j.jhepr.2019.10.005
122. Arvaniti P, Giannoulis G, Gabeta S, Zachou K, Koukoulis GK, Dalekos GN. Belimumab is a promising third-line treatment option for refractory autoimmune hepatitis. JHEP Rep (2020) 2(4):100123. doi: 10.1016/j.jhepr.2020.100123
123. Appanna GD, Pembroke TPI, Miners KL, Price DA, Gallimore AM, Ladell K, et al. Rituximab depletion of intrahepatic b cells to control refractory hepatic autoimmune overlap syndrome. QJM (2019) 112(10):793−5. doi: 10.1093/qjmed/hcz161
124. Khanna A, Jopson L, Howel D, Bryant A, Blamire A, Newton JL, et al. Rituximab is ineffective for treatment of fatigue in primary biliary cholangitis: A phase-2 randomised controlled trial. Hepatology (2018) 70(5):1646–57. doi: 10.1002/hep.30099
125. Balitzer D, Shafizadeh N, Peters MG, Ferrell LD, Alshak N, Kakar S. Autoimmune hepatitis: review of histologic features included in the simplified criteria proposed by the international autoimmune hepatitis group and proposal for new histologic criteria. Mod Pathol (2017) 30(5):773−83. doi: 10.1038/modpathol.2016.267
126. Bogdanos DP, Invernizzi P, Mackay IR, Vergani D. Autoimmune liver serology: current diagnostic and clinical challenges. World J Gastroenterol (2008) 14(21):3374−87. doi: 10.3748/wjg.14.3374
127. Abe K, Takahashi A, Nozawa Y, Imaizumi H, Hayashi M, Okai K, et al. The utility of IgG, IgM, and CD138 immunohistochemistry in the evaluation of autoimmune liver diseases. Med Mol Morphol. (2014) 47(3):162−8. doi: 10.1007/s00795-014-0082-z
128. Borchers AT, Shimoda S, Bowlus C, Keen CL, Gershwin ME. Lymphocyte recruitment and homing to the liver in primary biliary cirrhosis and primary sclerosing cholangitis. Semin Immunopathol (2009) 31(3):309−22. doi: 10.1007/s00281-009-0167-2
129. Chuang YH, Lian ZX, Cheng CM, Lan RY, Yang GX, Moritoki Y, et al. Increased levels of chemokine receptor CXCR3 and chemokines IP-10 and MIG in patients with primary biliary cirrhosis and their first degree relatives. J Autoimmun (2005) 25(2):126−32. doi: 10.1016/j.jaut.2005.08.009
130. Cabibi D, Tarantino G, Barbaria F, Campione M, Craxì A, Di Marco V. Intrahepatic IgG/IgM plasma cells ratio helps in classifying autoimmune liver diseases. Dig Liver Dis (2010) 42(8):585−92. doi: 10.1016/j.dld.2009.12.006
131. Lee H, Stapp RT, Ormsby AH, Shah VV. The usefulness of IgG and IgM immunostaining of periportal inflammatory cells (plasma cells and lymphocytes) for the distinction of autoimmune hepatitis and primary biliary cirrhosis and their staining pattern in autoimmune hepatitis-primary biliary cirrhosis overlap syndrome. Am J Clin Pathol (2010) 133(3):430−7. doi: 10.1309/AJCPE93GZSHUNTAI
132. Moreira RK, Revetta F, Koehler E, Washington MK. Diagnostic utility of IgG and IgM immunohistochemistry in autoimmune liver disease. World J Gastroenterol (2010) 16(4):453−7. doi: 10.3748/wjg.v16.i4.453
133. Wang L, Sun Y, Zhang Z, Jia Y, Zou Z, Ding J, et al. CXCR5+ CD4+ T follicular helper cells participate in the pathogenesis of primary biliary cirrhosis. Hepatology (2015) 61(2):627−38. doi: 10.1002/hep.27306
134. Chung BK, Guevel BT, Reynolds GM, Gupta Udatha DBRK, Henriksen EKK, Stamataki Z, et al. Phenotyping and auto-antibody production by liver-infiltrating b cells in primary sclerosing cholangitis and primary biliary cholangitis. J Autoimmun (2017) 77:45−54. doi: 10.1016/j.jaut.2016.10.003
135. Muller AF, Drexhage HA, Berghout A. Postpartum thyroiditis and autoimmune thyroiditis in women of childbearing age: recent insights and consequences for antenatal and postnatal care. Endocr Rev (2001) 22(5):605−30. doi: 10.1210/edrv.22.5.0441
136. Marai I, Carp H, Shai S, Shabo R, Fishman G, Shoenfeld Y. Autoantibody panel screening in recurrent miscarriages. Am J Reprod Immunol (2004) 51(3):235−40. doi: 10.1111/j.1600-0897.2004.00153.x
137. El Fassi D, Nielsen CH, Bonnema SJ, Hasselbalch HC, Hegedüs L. B lymphocyte depletion with the monoclonal antibody rituximab in graves’ disease: a controlled pilot study. J Clin Endocrinol Metab (2007) 92(5):1769−72. doi: 10.1210/jc.2006-2388
138. El Fassi D, Banga JP, Gilbert JA, Padoa C, Hegedüs L, Nielsen CH. Treatment of graves’ disease with rituximab specifically reduces the production of thyroid stimulating autoantibodies. Clin Immunol (2009) 130(3):252−8. doi: 10.1016/j.clim.2008.09.007
139. Erdei A, Paragh G, Kovacs P, Karanyi Z, Berenyi E, Galuska L, et al. Rapid response to and long-term effectiveness of anti-CD20 antibody in conventional therapy resistant graves’ orbitopathy: A five-year follow-up study. Autoimmunity (2014) 47(8):548−55. doi: 10.3109/08916934.2014.939266
140. Salvi M, Vannucchi G, Currò N, Campi I, Covelli D, Dazzi D, et al. Efficacy of b-cell targeted therapy with rituximab in patients with active moderate to severe graves’ orbitopathy: a randomized controlled study. J Clin Endocrinol Metab (2015) 100(2):422−31. doi: 10.1210/jc.2014-3014
141. Deltour JB, d’Assigny Flamen M, Ladsous M, Giovansili L, Cariou B, Caron P, et al. Efficacy of rituximab in patients with graves’ orbitopathy: a retrospective multicenter nationwide study. Graefes Arch Clin Exp Ophthalmol (2020) 258(9):2013−21. doi: 10.1007/s00417-020-04651-6
142. Armengol MP, Juan M, Lucas-Martín A, Fernández-Figueras MT, Jaraquemada D, Gallart T, et al. Thyroid autoimmune disease: demonstration of thyroid antigen-specific b cells and recombination-activating gene expression in chemokine-containing active intrathyroidal germinal centers. Am J Pathol (2001) 159(3):861−73. doi: 10.1016/S0002-9440(10)61762-2
143. Mohr A, Trésallet C, Monot N, Bauvois A, Abiven D, Atif M, et al. Tissue infiltrating LTi-like group 3 innate lymphoid cells and T follicular helper cells in graves’ and hashimoto’s thyroiditis. Front Immunol (2020) 11:601. doi: 10.3389/fimmu.2020.00601
144. Ferrari SM, Ruffilli I, Elia G, Ragusa F, Paparo SR, Patrizio A, et al. Chemokines in hyperthyroidism. J Clin Transl Endocrinol juin (2019) 16:100196. doi: 10.1016/j.jcte.2019.100196
145. García-López MA, Sancho D, Sánchez-Madrid F, Marazuela M. Thyrocytes from autoimmune thyroid disorders produce the chemokines IP-10 and mig and attract CXCR3+ lymphocytes. J Clin Endocrinol Metab (2001) 86(10):5008−16. doi: 10.1210/jcem.86.10.7953
146. Antonelli A, Ferrari SM, Giuggioli D, Ferrannini E, Ferri C, Fallahi P. Chemokine (C-X-C motif) ligand (CXCL)10 in autoimmune diseases. Autoimmun Rev (2014) 13(3):272−80. doi: 10.1016/j.autrev.2013.10.010
147. Hammerstad SS, Jahnsen FL, Tauriainen S, Hyöty H, Paulsen T, Norheim I, et al. Immunological changes and increased expression of myxovirus resistance protein a in thyroid tissue of patients with recent onset and untreated graves’ disease. Thyroid (2014) 24(3):537−44. doi: 10.1089/thy.2013.0287
148. Rotondo Dottore G, Torregrossa L, Lanzolla G, Mariotti S, Menconi F, Piaggi P, et al. Role of the mononuclear cell infiltrate in graves’ orbitopathy (GO): results of a large cohort study. J Endocrinol Invest. (2022) 45(3):563−72. doi: 10.1007/s40618-021-01692-4
149. Fernando R, Atkins SJ, Smith TJ. Intersection of chemokine and TSH receptor pathways in human fibrocytes: Emergence of CXCL-12/CXCR4 cross talk potentially relevant to thyroid-associated ophthalmopathy. Endocrinology (2016) 157(10):3779−87. doi: 10.1210/en.2016-1382
150. Campi I, Tosi D, Rossi S, Vannucchi G, Covelli D, Colombo F, et al. B cell activating factor (BAFF) and BAFF receptor expression in autoimmune and nonautoimmune thyroid diseases. Thyroid (2015) 25(9):1043−9. doi: 10.1089/thy.2015.0029
151. Agius MA, Klodowska-Duda G, Maciejowski M, Potemkowski A, Li J, Patra K, et al. Safety and tolerability of inebilizumab (MEDI-551), an anti-CD19 monoclonal antibody, in patients with relapsing forms of multiple sclerosis: Results from a phase 1 randomised, placebo-controlled, escalating intravenous and subcutaneous dose study. Mult Scler. (2019) 25(2):235−45. doi: 10.1177/1352458517740641
152. Kappos L, Hartung HP, Freedman MS, Boyko A, Radu EW, Mikol DD, et al. Atacicept in multiple sclerosis (ATAMS): a randomised, placebo-controlled, double-blind, phase 2 trial. Lancet Neurol (2014) 13(4):353−63. doi: 10.1016/S1474-4422(14)70028-6
153. Kumar G, Maria Z, Kohli U, Agasing A, Quinn JL, Ko RM, et al. CNS autoimmune responses in BCMA-deficient mice provide insight for the failure of atacicept in MS. Neurol Neuroimmunol Neuroinflamm (2021) 8(3):e973. doi: 10.1212/NXI.0000000000000973
154. Rojas OL, Pröbstel AK, Porfilio EA, Wang AA, Charabati M, Sun T, et al. Recirculating intestinal IgA-producing cells regulate neuroinflammation via IL-10. Cell (2019) 177(2):492−3. doi: 10.1016/j.cell.2019.03.037
155. Baert L, Benkhoucha M, Popa N, Ahmed MC, Manfroi B, Boutonnat J, et al. A proliferation-inducing ligand-mediated anti-inflammatory response of astrocytes in multiple sclerosis. Ann Neurol (2019) 85(3):406−20. doi: 10.1002/ana.25415
156. Krämer J, Wiendl H. What have failed, interrupted, and withdrawn antibody therapies in multiple sclerosis taught us? Neurotherapeutics (2022) 19(3):785−807. doi: 10.1007/s13311-022-01246-3
157. Lassmann H, Bradl M. Multiple sclerosis: experimental models and reality. Acta Neuropathol (2017) 133(2):223−44. doi: 10.1007/s00401-016-1631-4
158. Lucchinetti C, Brück W, Parisi J, Scheithauer B, Rodriguez M, Lassmann H. Heterogeneity of multiple sclerosis lesions: implications for the pathogenesis of demyelination. Ann Neurol (2000) 47(6):707−17. doi: 10.1002/1531-8249(200006)47:6<707::AID-ANA3>3.0.CO;2-Q
159. Pollok K, Mothes R, Ulbricht C, Liebheit A, Gerken JD, Uhlmann S, et al. The chronically inflamed central nervous system provides niches for long-lived plasma cells. Acta Neuropathol Commun (2017) 5(1):88. doi: 10.1186/s40478-017-0487-8
160. Krumbholz M, Theil D, Cepok S, Hemmer B, Kivisäkk P, Ransohoff RM, et al. Chemokines in multiple sclerosis: CXCL12 and CXCL13 up-regulation is differentially linked to CNS immune cell recruitment. Brain (2006) 129(Pt 1):200−11. doi: 10.1093/brain/awh680
161. Cepok S, Rosche B, Grummel V, Vogel F, Zhou D, Sayn J, et al. Short-lived plasma blasts are the main b cell effector subset during the course of multiple sclerosis. Brain (2005) 128(Pt 7):1667−76. doi: 10.1093/brain/awh486
162. Arce S, Luger E, Muehlinghaus G, Cassese G, Hauser A, Horst A, et al. CD38 low IgG-secreting cells are precursors of various CD38 high-expressing plasma cell populations. J Leukoc Biol (2004) 75(6):1022−8. doi: 10.1189/jlb.0603279
163. Ramesh A, Schubert RD, Greenfield AL, Dandekar R, Loudermilk R, Sabatino JJ, et al. A pathogenic and clonally expanded b cell transcriptome in active multiple sclerosis. Proc Natl Acad Sci U S A. (2020) 117(37):22932−43. doi: 10.1073/pnas.2008523117
164. Obermeier B, Mentele R, Malotka J, Kellermann J, Kümpfel T, Wekerle H, et al. Matching of oligoclonal immunoglobulin transcriptomes and proteomes of cerebrospinal fluid in multiple sclerosis. Nat Med (2008) 14(6):688−93. doi: 10.1038/nm1714
165. Magliozzi R, Howell O, Vora A, Serafini B, Nicholas R, Puopolo M, et al. Meningeal b-cell follicles in secondary progressive multiple sclerosis associate with early onset of disease and severe cortical pathology. Brain. avr (2007) 130(Pt 4):1089−104. doi: 10.1093/brain/awm038
166. Serafini B, Rosicarelli B, Magliozzi R, Stigliano E, Aloisi F. Detection of ectopic b-cell follicles with germinal centers in the meninges of patients with secondary progressive multiple sclerosis. Brain Pathol (2004) 14(2):164−74. doi: 10.1111/j.1750-3639.2004.tb00049.x
167. Wang H, Wang K, Zhong X, Qiu W, Dai Y, Wu A, et al. Cerebrospinal fluid BAFF and APRIL levels in neuromyelitis optica and multiple sclerosis patients during relapse. J Clin Immunol (2012) 32(5):1007−11. doi: 10.1007/s10875-012-9709-9
168. Piazza F, DiFrancesco JC, Fusco ML, Corti D, Pirovano L, Frigeni B, et al. Cerebrospinal fluid levels of BAFF and APRIL in untreated multiple sclerosis. J neuroimmunol (2010) 220(1−2):104−7. doi: 10.1016/j.jneuroim.2010.01.011
169. Hoffmann FS, Kuhn PH, Laurent SA, Hauck SM, Berer K, Wendlinger SA, et al. The immunoregulator soluble TACI is released by ADAM10 and reflects b cell activation in autoimmunity. J Immunol (2015) 194(2):542−52. doi: 10.4049/jimmunol.1402070
170. Laurent SA, Hoffmann FS, Kuhn PH, Cheng Q, Chu Y, Schmidt-Supprian M, et al. γ-secretase directly sheds the survival receptor BCMA from plasma cells. Nat Commun 11 juin (2015) 6:7333. doi: 10.1038/ncomms8333
171. Krumbholz M, Theil D, Derfuss T, Rosenwald A, Schrader F, Monoranu CM, et al. BAFF is produced by astrocytes and up-regulated in multiple sclerosis lesions and primary central nervous system lymphoma. J Exp Med (2005) 201(2):195−200. doi: 10.1084/jem.20041674
172. Shen P, Roch T, Lampropoulou V, O’Connor RA, Stervbo U, Hilgenberg E, et al. IL-35-producing b cells are critical regulators of immunity during autoimmune and infectious diseases. Nature (2014) 507(7492):366−70. doi: 10.1038/nature12979
173. Pröbstel AK, Zhou X, Baumann R, Wischnewski S, Kutza M, Rojas OL, et al. Gut microbiota-specific IgA+ b cells traffic to the CNS in active multiple sclerosis. Sci Immunol (2020) 5(53):eabc7191. doi: 10.1126/sciimmunol.abc7191
174. Machado-Santos J, Saji E, Tröscher AR, Paunovic M, Liblau R, Gabriely G, et al. The compartmentalized inflammatory response in the multiple sclerosis brain is composed of tissue-resident CD8+ T lymphocytes and b cells. Brain (2018) 141(7):2066−82. doi: 10.1093/brain/awy151
175. Duan T, Verkman AS. Experimental animal models of aquaporin-4-IgG-seropositive neuromyelitis optica spectrum disorders: progress and shortcomings. Brain Pathol (2020) 30(1):13−25. doi: 10.1111/bpa.12793
176. Jacob A, Weinshenker BG, Violich I, McLinskey N, Krupp L, Fox RJ, et al. Treatment of neuromyelitis optica with rituximab: retrospective analysis of 25 patients. Arch Neurol (2008) 65(11):1443−8. doi: 10.1001/archneur.65.11.noc80069
177. Shimizu F, Nishihara H, Sano Y, Takeshita Y, Takahashi S, Maeda T, et al. Markedly increased IP-10 production by blood-brain barrier in neuromyelitis optica. PloS One (2015) 10(3):e0122000. doi: 10.1371/journal.pone.0122000
178. Narikawa K, Misu T, Fujihara K, Nakashima I, Sato S, Itoyama Y. CSF chemokine levels in relapsing neuromyelitis optica and multiple sclerosis. J Neuroimmunol (2004) 149(1−2):182−6. doi: 10.1016/j.jneuroim.2003.12.010
179. Chihara N, Aranami T, Oki S, Matsuoka T, Nakamura M, Kishida H, et al. Plasmablasts as migratory IgG-producing cells in the pathogenesis of neuromyelitis optica. PloS One (2013) 8(12):e83036. doi: 10.1371/journal.pone.0083036
180. Quan C, Yu H, Qiao J, Xiao B, Zhao G, Wu Z, et al. Impaired regulatory function and enhanced intrathecal activation of b cells in neuromyelitis optica: distinct from multiple sclerosis. Mult Scler. (2013) 19(3):289−98. doi: 10.1177/1352458512454771
181. Chan KH, Lee R, Lau KK, Loong F. Orbital ectopic lymphoid follicles with germinal centers in aquaporin-4-IgG-Positive neuromyelitis optica spectrum disorders. Front Immunol (2017) 8:1947. doi: 10.3389/fimmu.2017.01947
182. Baert L, Marignier R, Lassmann HP, Huard B. Case report: In situ expression of a proliferation-inducing ligand in neuromyelitis optica. Front Neurol (2021) 12:721877. doi: 10.3389/fneur.2021.721877
183. Khovanova N, Daga S, Shaikhina T, Krishnan N, Jones J, Zehnder D, et al. Subclass analysis of donor HLA-specific IgG in antibody-incompatible renal transplantation reveals a significant association of IgG4 with rejection and graft failure. Transpl Int (2015) 28(12):1405−15. doi: 10.1111/tri.12648
184. Lefaucheur C, Viglietti D, Bentlejewski C, Duong van Huyen JP, Vernerey D, Aubert O, et al. IgG donor-specific anti-human HLA antibody subclasses and kidney allograft antibody-mediated injury. J Am Soc Nephrol. (2016) 27(1):293−304. doi: 10.1681/ASN.2014111120
185. Macklin PS, Morris PJ, Knight SR. A systematic review of the use of rituximab for the treatment of antibody-mediated renal transplant rejection. Transplant Rev (Orlando) (2017) 31(2):87−95. doi: 10.1016/j.trre.2017.01.002
186. Sautenet B, Blancho G, Büchler M, Morelon E, Toupance O, Barrou B, et al. One-year results of the effects of rituximab on acute antibody-mediated rejection in renal transplantation: RITUX ERAH, a multicenter double-blind randomized placebo-controlled trial. Transplantation (2016) 100(2):391−9. doi: 10.1097/TP.0000000000000958
187. Banham GD, Flint SM, Torpey N, Lyons PA, Shanahan DN, Gibson A, et al. Belimumab in kidney transplantation: an experimental medicine, randomised, placebo-controlled phase 2 trial. Lancet (2018) 391(10140):2619−30. doi: 10.1016/S0140-6736(18)30984-X
188. Eskandary F, Regele H, Baumann L, Bond G, Kozakowski N, Wahrmann M, et al. A randomized trial of bortezomib in late antibody-mediated kidney transplant rejection. J Am Soc Nephrol (2018) 29(2):591−605. doi: 10.1681/ASN.2017070818
189. Rossi AP, Alloway RR, Hildeman D, Woodle ES. Plasma cell biology: Foundations for targeted therapeutic development in transplantation. Immunol Rev (2021) 303(1):168−86. doi: 10.1111/imr.13011
190. Doberer K, Kläger J, Gualdoni GA, Mayer KA, Eskandary F, Farkash EA, et al. CD38 antibody daratumumab for the treatment of chronic active antibody-mediated kidney allograft rejection. Transplantation (2021) 105(2):451−7. doi: 10.1097/TP.0000000000003247
191. Halloran PF, Venner JM, Madill-Thomsen KS, Einecke G, Parkes MD, Hidalgo LG, et al. Review: The transcripts associated with organ allograft rejection. Am J Transplant. (2018) 18(4):785−95. doi: 10.1111/ajt.14600
192. Dabiri S, Kariminik A, Kennedy D. The role of CXCR3 and its ligands in renal transplant outcome. Eur Cytokine Netw (2016) 27(2):34−40. doi: 10.1684/ecn.2016.0375
193. Hoffmann U, Banas B, Krüger B, Banas M, Bergler T, Böger C, et al. SDF-1 expression is elevated in chronic human renal allograft rejection. Clin Transplant. (2006) 20(6):712−8. doi: 10.1111/j.1399-0012.2006.00540.x
194. Filippone EJ, Farber JL. The implications of b-lineage cells in kidney allografts. Transplantation (2020) 104(10):2011−23. doi: 10.1097/TP.0000000000003163
195. Tsai EW, Wallace WD, Gjertson DW, Reed EF, Ettenger RB. Significance of intragraft CD138+ lymphocytes and p-S6RP in pediatric kidney transplant biopsies. Transplantation (2010) 90(8):875−81. doi: 10.1097/TP.0b013e3181f24e3c
196. Abbas K, Mubarak M, Zafar MN, Aziz T, Abbas H, Muzaffar R, et al. Plasma cell-rich acute rejections in living-related kidney transplantation: a clinicopathological study of 50 cases. Clin Transplant. (2015) 29(9):835−41. doi: 10.1111/ctr.12589
197. de Graav GN, Dieterich M, Hesselink DA, Boer K, Clahsen-van Groningen MC, Kraaijeveld R, et al. Follicular T helper cells and humoral reactivity in kidney transplant patients. Clin Exp Immunol (2015) 180(2):329−40. doi: 10.1111/cei.12576
198. Lee YH, Sato Y, Saito M, Fukuma S, Saito M, Yamamoto S, et al. Advanced tertiary lymphoid tissues in protocol biopsies are associated with progressive graft dysfunction in kidney transplant recipients. J Am Soc Nephrol (2022) 33(1):186−200. doi: 10.1681/ASN.2021050715
199. Thaunat O, Patey N, Gautreau C, Lechaton S, Fremeaux-Bacchi V, Dieu-Nosjean MC, et al. B cell survival in intragraft tertiary lymphoid organs after rituximab therapy. Transplantation (2008) 85(11):1648−53. doi: 10.1097/TP.0b013e3181735723
200. Thaunat O, Patey N, Caligiuri G, Gautreau C, Mamani-Matsuda M, Mekki Y, et al. Chronic rejection triggers the development of an aggressive intragraft immune response through recapitulation of lymphoid organogenesis. J Immunol (2010) 185(1):717−28. doi: 10.4049/jimmunol.0903589
201. Thaunat O, Field AC, Dai J, Louedec L, Patey N, Bloch MF, et al. Lymphoid neogenesis in chronic rejection: evidence for a local humoral alloimmune response. Proc Natl Acad Sci U.S.A. (2005) 102(41):14723−8. doi: 10.1073/pnas.0507223102
Keywords: plasma cells, antibodies, autoimmunity, tissues, treatment
Citation: Giovannini D, Belbezier A, Baillet A, Bouillet L, Kawano M, Dumestre-Perard C, Clavarino G, Noble J, Pers J-O, Sturm N and Huard B (2023) Heterogeneity of antibody-secreting cells infiltrating autoimmune tissues. Front. Immunol. 14:1111366. doi: 10.3389/fimmu.2023.1111366
Received: 29 November 2022; Accepted: 27 January 2023;
Published: 21 February 2023.
Edited by:
Alexander A. Shtil, Russian Cancer Research Center NN Blokhin, RussiaReviewed by:
Betty Diamond, Feinstein Institute for Medical Research, United StatesColleen Mayberry, Jackson Laboratory, United States
Copyright © 2023 Giovannini, Belbezier, Baillet, Bouillet, Kawano, Dumestre-Perard, Clavarino, Noble, Pers, Sturm and Huard. This is an open-access article distributed under the terms of the Creative Commons Attribution License (CC BY). The use, distribution or reproduction in other forums is permitted, provided the original author(s) and the copyright owner(s) are credited and that the original publication in this journal is cited, in accordance with accepted academic practice. No use, distribution or reproduction is permitted which does not comply with these terms.
*Correspondence: Bertrand Huard, QmVydHJhbmQuaHVhcmRAdW5pdi1ncmVub2JsZS1hbHBlcy5mcg==