- 1Department of Orthopedics and Spine Surgery, The Second Affiliated Hospital of Shantou University Medical College, Shantou, Guangdong, China
- 2Department of Bone and Soft Tissue Oncology, Cancer Hospital of Shantou University Medical College, Shantou, Guangdong, China
- 3Key Laboratory of Biochemistry and Molecular Biology, Hainan Medical University, Haikou, Hainan, China
- 4School of Public Health, Shantou University, Shantou, Guangdong Province, China
- 5Department of Clinical Laboratory, The First Affiliated Hospital of Zhengzhou University, Zhengzhou, Henan, China
- 6Faculty of Psychology, Institute of Educational Science, Huazhong University of Science and Technology, Wuhan, Hubei, China
- 7Department of Clinical Laboratory, State Key Laboratory of Oncology in South China, Collaborative Innovation Center for Cancer Medicine, Guangdong Key Laboratory of Nasopharyngeal Carcinoma Diagnosis and Therapy, Sun Yat-sen University Cancer Center, Guangzhou, Guangdong, China
- 8Department of Clinical Laboratory, The First Affiliated Hospital of Zhengzhou University, Key Clinical Laboratory of Henan Province, Zhengzhou, Henan, China
- 9Department of Pediatrics, The First Affiliated Hospital of Zhengzhou University, Zhengzhou, Henan, China
- 10Department of Hematological Oncology, State Key Laboratory of Oncology in South China, Collaborative Innovation Center for Cancer Medicine, Sun Yat-sen University Cancer Center, Guangzhou, Guangdong, China
- 11Department of General Surgery, Hainan Hospital of People's Liberation Army General Hospital, Sanya, Hainan, China
- 12Department of Pathology, Cancer Hospital of Shantou University Medical College, Shantou, Guangdong, China
- 13Department of Infectious Diseases and Hepatology, The First Affiliated Hospital of Zhengzhou University, Zhengzhou, Henan, China
Introduction: Although there is extended research on the response to severe acute respiratory syndrome coronavirus 2 (SARS-CoV-2) vaccines in adult cancer patients (ACP), the immunogenicity to the variants of concern (VOCs) in childhood cancer patients (CCP) and safety profiles are now little known.
Methods: A prospective, multi-center cohort study was performed by recruiting children with a solid cancer diagnosis and childhood healthy control (CHC) to receive standard two-dose SARS-CoV-2 vaccines. An independent ACP group was included to match CCP in treatment history. Humoral response to six variants was performed and adverse events were followed up 3 months after vaccination. Responses to variants were compared with ACP and CHC by means of propensity score-matched (PSM) analysis.
Results: The analysis included 111 CCP (27.2%, median age of 8, quartile 5.5–15 years), 134 CHC (32.8%), and 163 ACP (40.0%), for a total 408 patients. Pathology included carcinoma, neural tumors, sarcoma, and germ cell tumors. Median chemotherapy time was 7 (quartile, 5–11) months. In PSM sample pairs, the humoral response of CCP against variants was significantly decreased, and serology titers (281.8 ± 315.5 U/ml) were reduced, as compared to ACP (p< 0.01 for the rate of neutralization rate against each variant) and CHC (p< 0.01 for the rate of neutralization against each variant) groups. Chemotherapy time and age (Pearson r ≥ 0.8 for all variants) were associated with the humoral response against VOCs of the CHC group. In the CCP group, less than grade II adverse events were observed, including 32 patients with local reactions, and 29 patients had systemic adverse events, including fever (n = 9), rash (n = 20), headache (n = 3), fatigue (n = 11), and myalgia (n = 15). All reactions were well-managed medically.
Conclusions: The humoral response against VOCs after the CoronaVac vaccination in CCP was moderately impaired although the vaccine was safe. Age and chemotherapy time seem to be the primary reason for poor response and low serology levels.
Introduction
Currently, vaccines against severe acute respiratory syndrome coronavirus 2 (SARS-CoV-2) are being developed as an effective measure against the pandemic (1, 2). Tolerability, safety, and immunogenicity in vaccination for healthy children have been evidenced to be similar to results in vaccine trials of adults and proved effective in building herd immunity (3). Although children who contracted coronavirus disease 2019 (COVID-19) were shown to manifest less severe courses of disease than adults, those with immunocompromised statuses, such as cancers, are still highly vulnerable to virus infection due to altered immune status and care provided (4, 5). Thus, it is pivotal to decrease the risk of COVID-19 contraction in such populations by vaccination if proven effective and safe (6). For adult cancer patients (ACP), prospective trials have demonstrated that various types of vaccines could induce satisfactory immunogenicity without serious adverse events (7–9). Thus, current opinions from a number of organizations have unequivocally recommended cancer patients as the most prioritized group to receive vaccination (8).
Until now, there have been reports of vaccine safety or effectiveness reports in pediatric patients with blood cancer (10), with relatively good tolerability and effectiveness. However, there is a paucity of data concerning SARS-CoV-2 vaccination in the population of childhood solid cancer patients (CCP), probably due to the low incidence in the childhood population (11, 12). Evidence from non-SARS-CoV-2 vaccines may suggest comparable results in terms of safety and immunogenicity (including influenza and pneumococcal vaccines) (13). Studies found sound immunogenicity and safety profiles of such vaccines previously, and immunomodulators in therapeutic regimens may substantially alter seroconversion rates (14, 15). Results can be translated into SARS-CoV-2 vaccination for CCP but direct evidence is still needed in the population. Even though the pathology state may be different, treatment profiles have been shown to substantially influence vaccination outcomes, including chemotherapy common to all solid cancer patients. Additionally, risk factors that contribute to humoral response in CCP should be illustrated and results may be different from those of the adult population, in whom age and immune status were shown to jointly affect outcomes (9). As these questions have seldom been addressed as of now, an observational investigation into the use of inactivated vaccines in this population may provide real-world evidence for future trials.
In this pilot prospective study, we aim to compare humoral response and safety profiles between CCP and healthy children and then between CCP and adult patients.
Methods
Participant enrollment and study design
The multi-center, prospective study recruited CCP and childhood healthy control (CHC) to receive the standard dose SARS-CoV-2 inactivated vaccines (CoronaVac, 4 μg per 0.5 ml per shot) from the pediatric hematology/oncology cancer database (pediatric Vacan cohort, Shan 2021-137) of the five tertiary hospitals (see Supplementary Methods) between 13 December 2021 and 18 July 2022. An independent group of ACP was also retrospectively included to match the CCP by cancer-related treatment and time of receiving vaccines from 1 March 2021 to 1 July 2022. Ethical approval has been gained at the Second Affiliated Hospital of Shantou University Medical College (SAHSU). The study was performed according to the ethical principles of the Declaration of Helsinki and Good Clinical Practice, and all parents of the CCP and CHC provided informed consent before participation.
Inclusion criteria for CCP were as follows (1): age between 3 and 18 years old (2); diagnosis of solid cancers in the past 3 years, irrespective of the current status of disease activities or treatment; and (3) willingness to and ability to have the clinical samples tested by the researchers, including blood, feces, and urine. Key exclusion criteria were as follows (1): contraindications to receiving vaccines, including hypersensitive reactions to the adjuvants of the CoronaVac vaccines (2); the prognosis of the CCP was less than 6 months or equivalent to hospice settings (3); history of severe autoimmune, genetic, or hematological diseases (4); prior infection of SARS-CoV-2 (5); diagnosis of hematological malignancies (which will be published elsewhere); and (6) participants with positive SARS-CoV-2 antibody titers before receiving vaccines.
Participant data
The bio-specimen in the study were per-protocol blood and routine samples of serum from clinical settings collected for downstream analysis. All participant data and samples were de-identified and stored with the analytic ID number in the current investigation. The electronic data capture systems of the SAHSU were applied to save and monitor the de-identified profiles.
The anti-SARS-CoV-2 antibody serology testing was performed prior to receiving vaccines, and 3 months after the second dose, respectively. After collection of the whole blood in Ethylene Diamine Tetraacetic Acid (EDTA) tubes, the serum was carefully collected and centrifuged for 10 min and stored at −80°C until testing. Times of blood drawing, processing, and refrigeration were recorded for each sample. The time from blood drawing to refrigeration was kept to be less than 24 h, and the process was done by the lab technicians blinded to the data. The sera were analyzed in the third-party laboratory in Guangzhou Province for antibody testing. For qualitative and quantitative analysis, the S-specific immunoglobulin G (IgG) was tested using the chemiluminescence kit (Bioscience Technology, Guangzhou, China).
Demographic and therapeutic data were extracted from the Case Record Form of the five tertiary referral hospitals. The demographic information included the gender and the age of participants (CCP, CHC, and ACP). The nutrition status of the CCP was defined with the body mass index of the corresponding growth milestones of the Chinese children and was thus divided into underweight, normal, and overweight by the treating oncologists blinded to the participant data. In the ACP group, the nutrition status was defined by the treating oncologists at follow-up. The treatment details included the cumulative chemotherapy time, steroid use history, tyrosine kinase inhibitor use, and radiotherapy history. The survival time was calculated from the time of cancer diagnosis to the time of receiving vaccines, and the chemotherapy status was also recorded as active or inactive. The pathological types of the CCP and ACP were determined by both histological and radiological evidence from the patient database of the five hospitals.
Included CCP participants received standard, two-dose CoronaVac SARS-CoV-2 vaccines at registered vaccination sites of the Centers for Disease Control and Prevention, with an intervening period of 5 to 7 weeks between the two doses. Participants with severe adverse events after the first doses of SARS-CoV-2 vaccines would not receive the second dose as required by the institutional board.
The primary outcome of the study was to report humoral responses against variants of concern (VOCs) after the COVID-19 vaccination in each group. The secondary outcome was to illustrate follow-up adverse events, or safety, of CCP during 3 months following SARS-CoV-2 vaccination. Solicited adverse events were recorded according to China National Medical Products Administration guidelines (16). Both efficacy and safety outcomes would be compared with ACP and CHC groups by propensity score-matched (PSM) analysis to minimize selection bias that may occur during the study process. Participant data, serology, and neutralization test methods are shown in supplementary details (see Supplementary Methods).
Statistics and analytic protocols
The primary research goal was the humoral response against each variant, including serology conversion, serology conversion rate, and neutralization ability. The secondary goal was adverse events, including local and systemic events graded according to Common Terminology Criteria for Adverse Events. Categorical variables were recorded as numbers and percentages, and continuous variables were recorded as means ± standard deviations (SD) and median (25th–75th quartile). The power (1 − β) of each statistical test was calculated according to the sample size on the PASS software (V.15.0), and each test was based on a pre-specified statistical hypothesis with a type I error of 0.05.
To minimize potential bias, the propensity scores were calculated in the CCP, ACP, and CHC groups. The score was calculated with a logistic conditional regression model (17). Variables included in the regression model included treatment details and baseline demographics. For comparison between CCP and CHC groups, variables included gender and age. After calculation, the nearest neighbor head-to-head (1:1) method was applied to match each comparable participant with the upper acceptable caliper width of 0.2 without replacement (18). To find potential imbalance and to evaluate matching performance, standardized mean difference (SMD) was calculated in both unmatched and matched data (19, 20). According to Austin et al., an SMD over is regarded as imbalanced pairs in the matched samples, where n1 and n2 stand for sample sizes of matching pairs (21). Thus, in matched samples, statistical tests of difference were McNemar test for categorical variables and Wilcoxon signed-rank test for continuous variables (22). Statistics used were carried out in SPSS V.23.0 software.
Results
Baseline characteristics
The study enrolled 121 CCP and 153 gender-matched CHC in a prospective cohort. No participant had ever received SARS-CoV-2 vaccines or been infected by SARS-CoV-2 vaccines, and all patients had negative antibody titers at recruitment. Ten CCP and 19 CHC did not finish the second dose due to concerns about future adverse events. Thus, a total of 111 CCP (73 male and 38 female patients) and 134 CHC (82 male and 52 female patients) were included in the final analysis (Table 1). A total of 163 (88 male and 75 female patients) ACP were enrolled that match the treatment and vaccination details of CCP (Supplementary Table 1). The median age of CCP was 8 (quartile, 5.5–15), and the median age of CHC was 9.5 (quartile, 6–13).
As for pathology types of CCP, 10 CCP (9.0%) had primitive neuroectodermal tumors (PNET), 30 CCP (27.0%) had neuroblastoma, 26 CCP (27.0%) had nasopharyngeal carcinoma, 10 CCP (9.0%) had rhabdomyosarcoma, 21 CCP (18.9%) had osteosarcoma or Ewing sarcoma, 4 CCP (3.6%) had hepatic blastoma, and 10 CCP (9.0%) had germ cell tumors.
Forty-six CCP (41.4%) received chemotherapy for less than 6 months, 21 patients (19.0%) over 1 year, and 44 patients (39.6%) between 6 months and a year. Median chemotherapy time was 7 (quartile, 5–11) months. Twenty CCP were on active chemotherapy when vaccinated. Seventy-eight patients (70.3%) had received radiotherapy in the past year, and 26 patients (23.3%) had received steroid therapies during the past 6 months. The median time of survival of the CCP was 27 (quartile, 20–37) months.
The humoral response was lower in CCP, with younger age combining chemotherapy associated with outcomes
Overall, after receiving two-dose vaccines, 76 of 111 CCP (68.4%) were seropositive and 35 patients had a negative response. Mean antibody titers were 281.8 ± 315.5 U/ml, and 43 (38.7%) participants had antibody levels over 300 U/ml. Univariate analysis of seroconversion failure showed a significant association with the following variables: age, pathology types, nutrition status, and chemotherapy time. Multivariate regression identified the following two variables to be independently significant (Table 2): age (OR = 0.84, 95% CI = 0.71–0.98, p = 0.03) and chemotherapy time (OR = 3.70, 95% CI = 1.71–7.98, p< 0.01). To quantify risk prediction of the serology failure, a nomogram was built that incorporated the two variables (Supplementary Figure 1A). A calibration curve was drawn to compare the actual and predicted risk of seroconversion failure (Supplementary Figure 1B). The concordance index of the nomogram was 0.79.
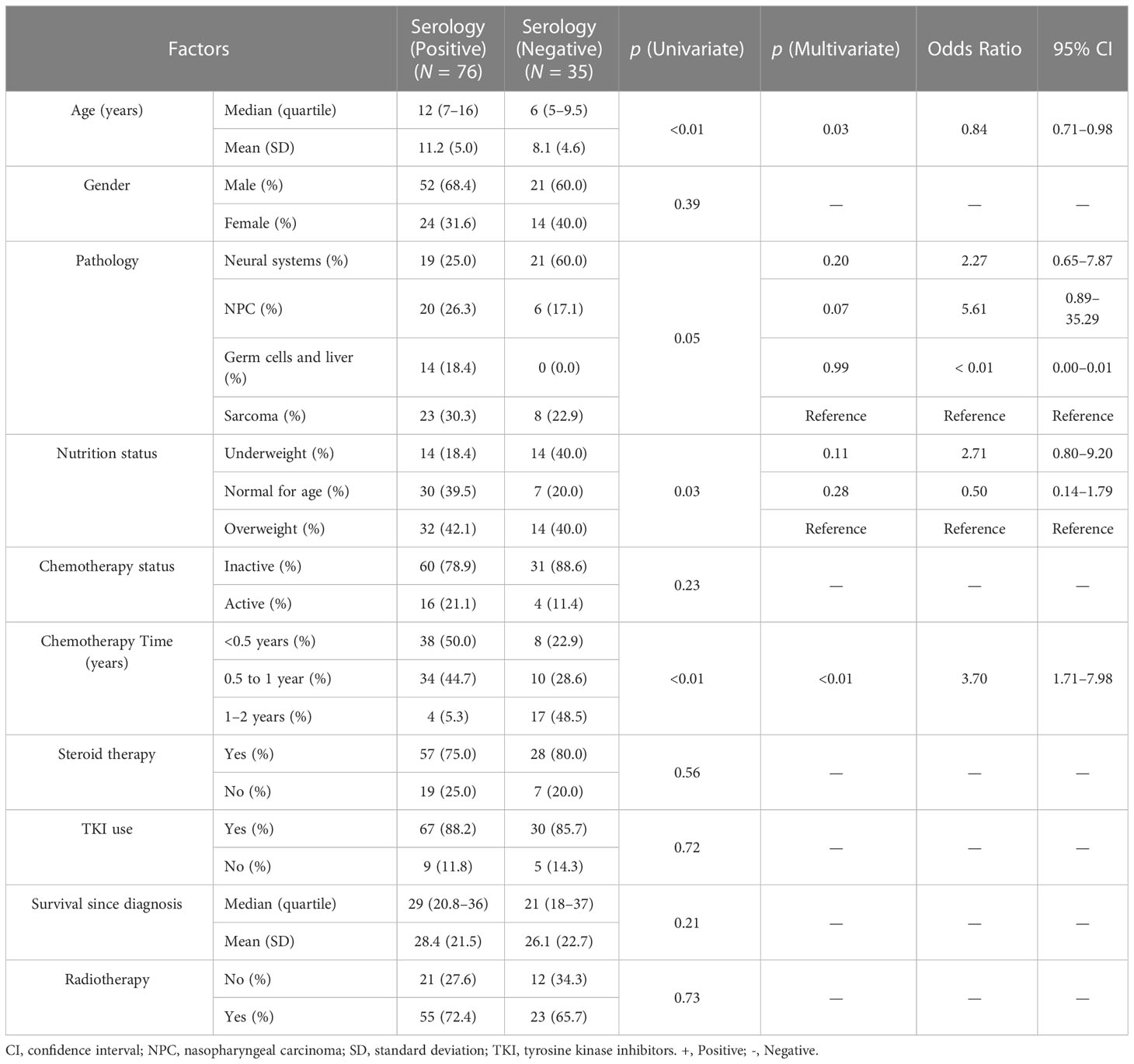
Table 2 Regression analysis for serology response rate of standard vaccines in childhood cancer patients.
In the CHC group, 116 (86.5%) participants had positive serologic responses. Mean serologic titers were 1,210.75 ± 905.04 U/ml, and 100 (74.6%) CHC participants had an adequate response. By PSM comparison (see Supplementary Table 2 for imbalance evaluation results), the rate of seroconversion in the CCP group was significantly less than that of the CHC group (p< 0.01 by paired McNemar test), and the serologic level of the CCP group was significantly less than that of the CHC group (p< 0.01 by Wilcoxon signed-rank test, Figures 1A, B). Antibody response and adverse event comparison are illustrated in Table 3.
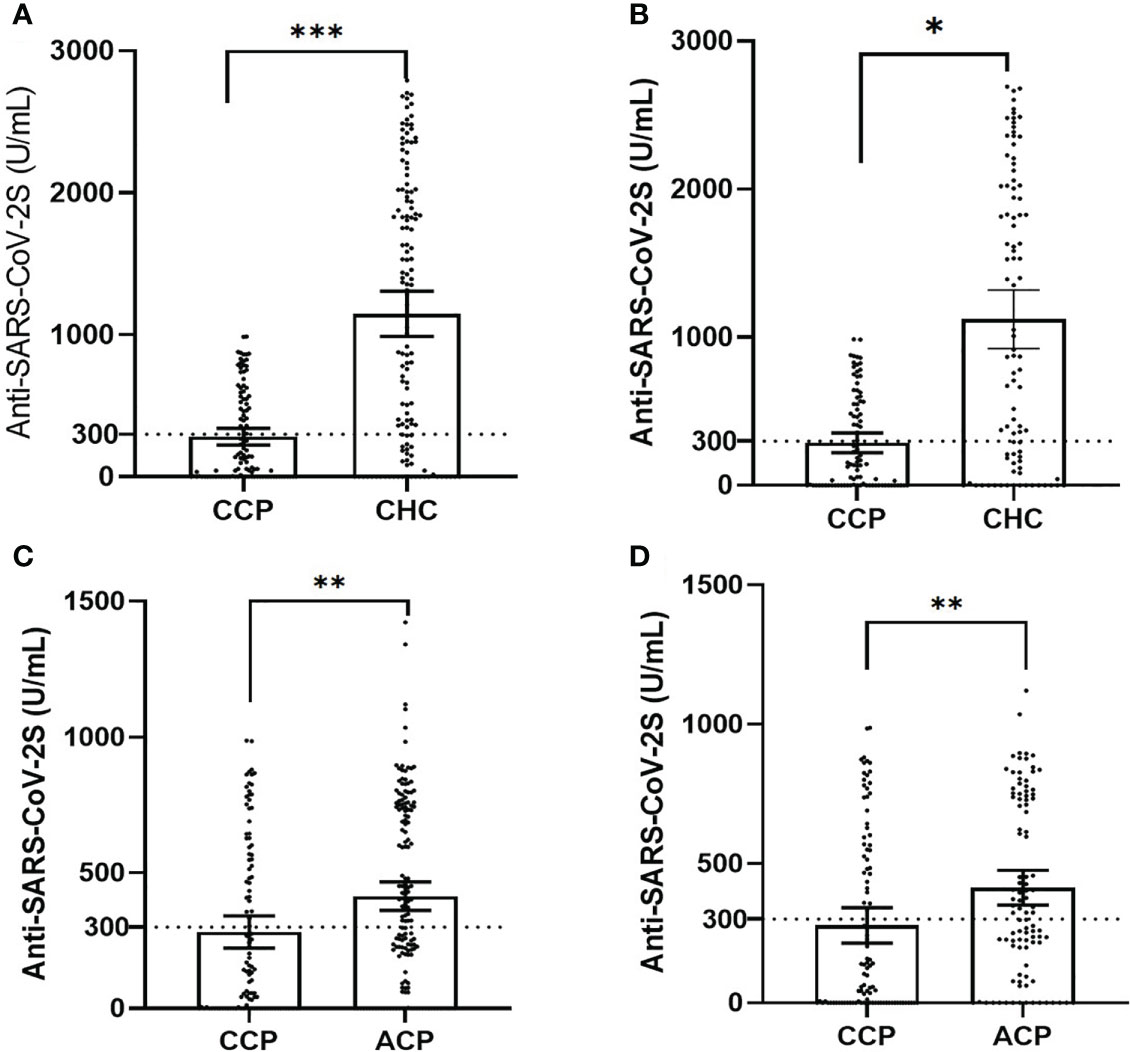
Figure 1 The dot plot of serology titers in unmatched and matched groups, plotted as mean ± 95% confidence intervals (CCP, childhood cancer patients; CHC, childhood healthy control; ACP, adult cancer patients; statistical power calculated to be >0.90 for all tests). (A) The dot plot of unmatched serology titers in the CCP versus the CHC cohort (χ2 test, p< 0.001). (B) The dot plot of matched serology titers in the CCP versus the CHC cohort (Wilcoxon signed-rank test in 1:1 paired samples, p = 0.04). (C) The dot plot of unmatched serology titers in the CCP versus the ACP group (χ2 test, p< 0.01). (D) The dot plot of matched serology titers in the CCP versus the ACP group (Wilcoxon signed-rank test in 1:1 paired samples, p< 0.01). ***p<0.001, **p<0.01, *p<0.05.
In the ACP group, 129 patients had positive serologic responses. Mean serologic titers were 414.41 ± 338.77 U/ml, and 88 patients had an adequate serologic response (53.9%). By PSM comparison between ACP and CCP groups (see Supplementary Table 3 for imbalance evaluation results), there were significantly fewer patients in the CCP group who had positive responses than in the ACP group (p< 0.01 by paired McNemar test), and the titer levels were significantly lower in the CCP group as well (p< 0.01 by Wilcoxon signed-rank test, Figures 1C, D). Antibody response and adverse event comparison are illustrated in Table 4.
Neutralization of VOCs was decreased in CCP, which was associated with longer chemotherapy time
Neutralization test was performed with the spike protein of wild type and VOCs. In the CCP group, percent inhibition was 41.75 ± 25.76 for wild types, 35.68 ± 21.50 for B.1.1.7 (Alfa), 36.32 ± 23.65 for B1.351 (Beta), 34.83 ± 21.13 for P.1 (Gamma), 35.93 ± 24.14 for B.1.617.2 (Delta), and 36.15 ± 24.19 for B.1.1.529 (Omicron). Analysis of variance (ANOVA) showed no significant difference among the six variants (p = 0.28, see Supplementary Figure 3). The Pearson correlation test showed that all subtypes were correlated with serologic titers of the CCP group (Figure 2), and the one-way ANOVA test showed no significance in neutralization ability among VOCs. Longer chemotherapy time was associated with a lower rate of inhibition for vaccines against all variants (p< 0.01, Supplementary Figure 2). In the PSM comparison, there was a significant difference in all VOCs (Alfa, Beta, Gamma, Delta, and Omicron) between the CCP and ACP group, and between the CCP and CHC group (p< 0.05, see Figure 3).
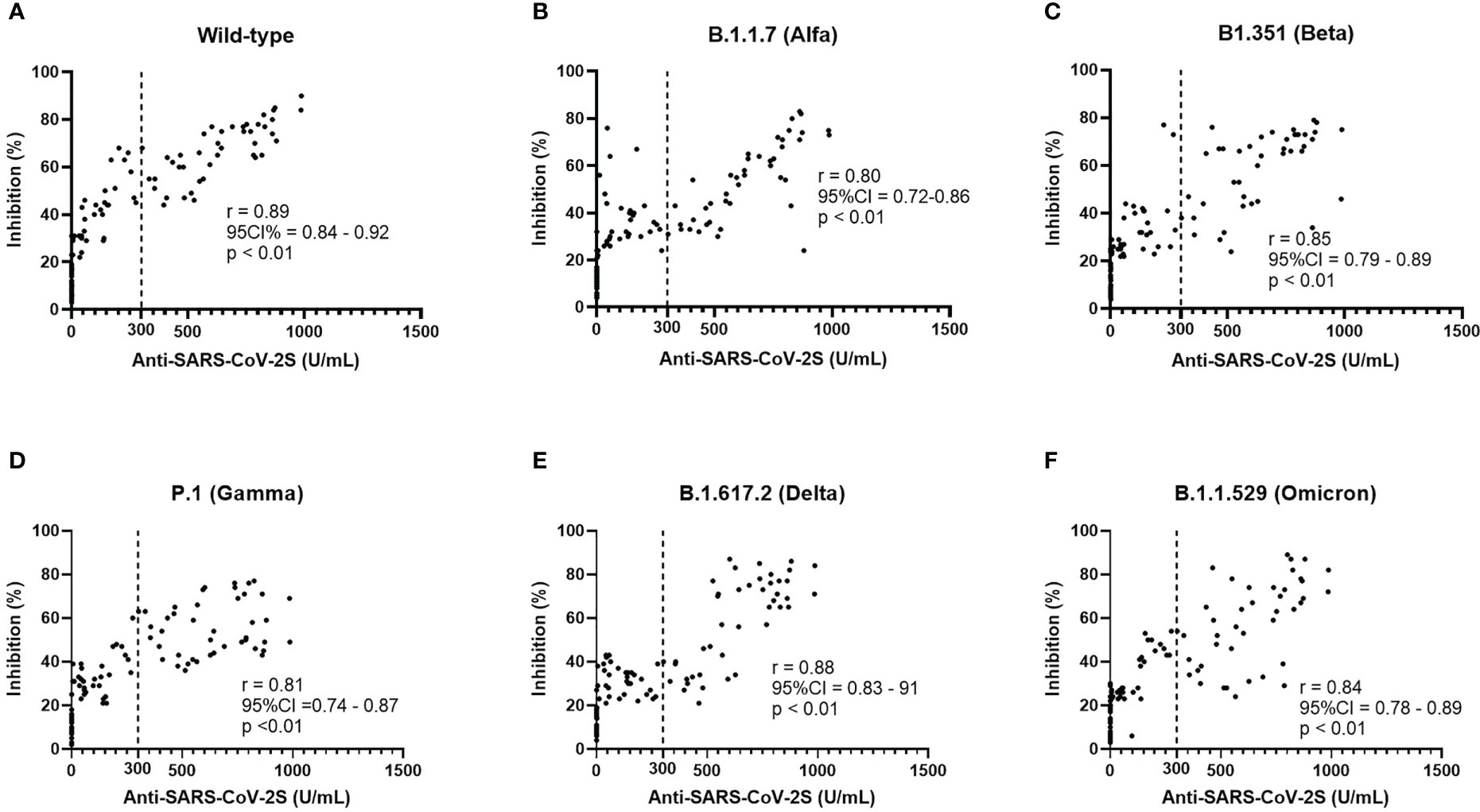
Figure 2 Neutralization ability (A–F) of different variants was found to have a positive correlation with the serologic titers of CoronaVac vaccines and the response to the wild type bears the highest level of correlation.
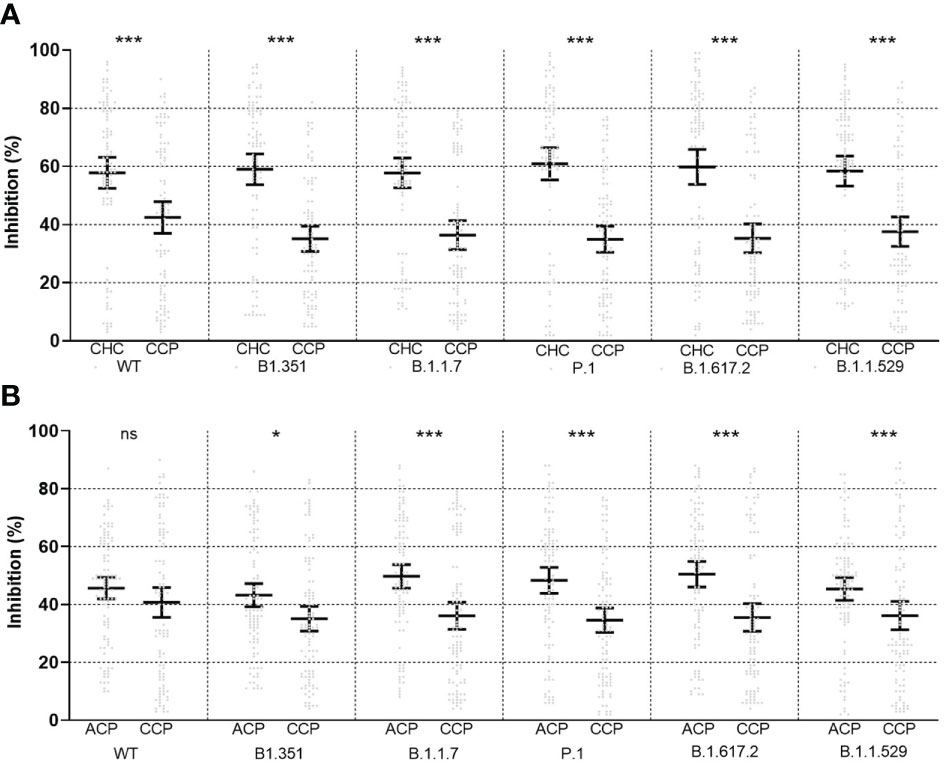
Figure 3 Propensity score-matched comparison of neutralizing ability between childhood healthy control (CHC, left) and childhood cancer patients (CCP, right) (A) and between adult cancer patients (ACP, left) and CCP (right) (B) after vaccination, plotted as mean ± 95% confidence intervals. ns, non-significant; *<0.05; ***<0.001. Statistical test performed with Wilcoxon signed-rank tests in 1:1 paired samples. Statistical power was calculated to be >0.90 for all tests.
Adverse events in unmatched groups
Solicited severe adverse events of CCP reported during follow-up were graded as I and II, and all adverse events were either self-limited or medically well-managed. Thirty-two patients had local reactions following receiving vaccines, including tenderness, rash, and itchiness. Twenty-nine patients had systemic adverse events, including fever (n = 9), rash (n = 20), headache (n = 3), fatigue (n = 11), and myalgia (n = 15). None of the patients had adverse events associated with solid organs or the tumor itself.
Solicited severe adverse events of the CHC group were graded as I and II and were medically managed as well. Thirty-four participants (25.3%) had local reactions, and systemic adverse events were encountered in 31 patients (23.1%). Systemic adverse events included fever (n = 17), rash (n = 16), headache (n = 18), fatigue (n = 19), and myalgia (n = 15).
In the retrospective ACP group, all adverse events were also graded I or II, which were medically managed. Thirty-nine patients (23.9%) reported local reactions, and systemic events were seen in 40 patients (24.5%). Systemic events included fever (n = 22), rash (n = 27), headache (n = 14), fatigue (n = 19), and myalgia (n = 28).
Discussion
We evaluated the safety and immunogenicity of CoronaVac, an inactivated two-dose SARS-CoV-2 vaccine, in pediatric and adult samples of solid cancer patients, and found that vaccines were generally well tolerated without severe adverse events as compared to healthy children. We then investigated humoral response to VOCs and found that the response was moderately impaired in pediatric patients, and even more reduced as compared to adult patients, in the setting of similar adverse event profiles.
VOCs were tested in differential groups, and the vaccine exhibited differential reactive ability. Consistent with response in adult patients, response to Omicron and Delta variant was found to exhibit the lowest level of neutralization, and the result could be further validated or tested in larger-scale samples in the future. Consistent with previous research on adult patients, we found a strong correlation between serology and neutralization tests for all VOCs7. Although belonging to the immuno-compromised population, our study demonstrated that CCP had an even worse level of immune compromise than ACP, suggesting a prolonged period of waned response to VOCs.
Although widely rumored previously in the parents of CCP, the result confirmed that the vaccine was generally safe even in pediatric populations of altered immune status, and may strengthen healthcare messaging of inactivated vaccine promotion to the pediatric population (23–25). As hypothesized previously, the response to vaccines in pediatric populations was different from that of adult patients (26). The age range of the study was relatively young with underdeveloped levels of immune status. After long-term chemotherapy, the response to external stimuli could thus deteriorate due to the sensitivity of the immune system to chemotherapy (27, 28).
It can be noted in the analysis that although only 20 CCP (18%) were on the current regimen of chemotherapy, the therapeutic time range was still independently associated with serology failure (>1 year has the highest risk of failure). This result may suggest that the impact of chemotherapy may have added or chronological effects on vaccine responses even after cessation and was consistent with the Childhood Cancer Survivor Study, which found that significant long-term toxic effects exist for immune systems of post-chemotherapy children and young adolescents (29, 30).
Our study has several limitations. Firstly, longitudinal changes in response have yet to be reported, which will give information on the robustness of vaccine response in such populations. It should be noted that the sample size of the CCP cohort was relatively small, and the ACP group was retrospectively included to match the vaccine response of the CCP cohort. Also, we did not evaluate the cell-mediated response in our study sample, which may give further information on long-term immune response, and further studies are encouraged. These limitations can be best addressed with larger-scale, longitudinal studies and randomized trials on CCP.
Conclusion
Humoral response against VOCs after CoronaVac vaccination in CCP was impaired although the vaccine was safe. Age and chemotherapy time seem to be reasons for poor response and low serology levels.
Data availability statement
The raw data supporting the conclusions of this article will be made available by the authors, without undue reservation.
Ethics statement
The studies involving human participants were reviewed and approved by the Second Affiliated Hospital of Shantou University Medical College (SAHSU). Written informed consent to participate in this study was provided by the participants’ legal guardian/next of kin.
Author contributions
YM, BC and YQW contributed equally to this study. XJW and AZ conceptualized and designed the study, and reviewed and revised the manuscript; YM and NL designed the data collection instruments, carried out the initial analyses, and wrote the first draft of the manuscript; BC and PZ coordinated and supervised data collection and critically reviewed the manuscript for important intellectual content; YQW, ZZ, GZ, GF, DW, LC, SB, YLW, SC, JL, and XLW collected data and reviewed and revised the manuscript. All authors contributed to the article and approved the submitted version.
Funding
This work was supported by grants from the Fellowship of China Postdoctoral Science Foundation (No. 2021M692015) and the National Natural Science Foundation of China Youth Science Fund Project (No. 82102687, No. 82201922, and No. 81902470). All the funders had no role in the design and conduct of the study.
Acknowledgments
The authors would like to acknowledge the volunteers who participated in the study.
Conflict of interest
The authors declare that the research was conducted in the absence of any commercial or financial relationships that could be construed as a potential conflict of interest.
Publisher’s note
All claims expressed in this article are solely those of the authors and do not necessarily represent those of their affiliated organizations, or those of the publisher, the editors and the reviewers. Any product that may be evaluated in this article, or claim that may be made by its manufacturer, is not guaranteed or endorsed by the publisher.
Supplementary material
The Supplementary Material for this article can be found online at: https://www.frontiersin.org/articles/10.3389/fimmu.2023.1110755/full#supplementary-material
References
1. WHO. Coronavirus disease (COVID-19) pandemic: vaccines (2022). Available at: https://www.who.int/emergencies/diseases/novel-coronavirus-2019/covid-19-vaccines.
2. Sterne JAC, Murthy S, Diaz JV, Slutsky AS, Villar J, Angus DC, et al. Association between administration of systemic corticosteroids and mortality among critically ill patients with COVID-19: a meta-analysis. Jama (2020) 324(13):1330–41. doi: 10.1001/jama.2020.17023
3. Han B, Song Y, Li C, Yang W, Ma Q, Jiang Z, et al. Safety, tolerability, and immunogenicity of an inactivated SARS-CoV-2 vaccine (CoronaVac) in healthy children and adolescents: a double-blind, randomised, controlled, phase 1/2 clinical trial. Lancet Infect Dis (2021) 21(12):1645–53. doi: 10.1016/S1473-3099(21)00319-4
4. Mukkada S, Bhakta N, Chantada GL, Chen Y, Vedaraju Y, Faughnan L, et al. Global characteristics and outcomes of SARS-CoV-2 infection in children and adolescents with cancer (GRCCC): a cohort study. Lancet Oncol (2021) 22(10):1416–26. doi: 10.1016/S1470-2045(21)00454-X
5. Jude St Global. Global registry of COVID-19 in pediatric cancer . Available at: https://global.stjude.org/en-us/global-covid-19-observatory-and-resource-center-for-childhood-cancer/registry.html.
6. Kotecha RS. Challenges posed by COVID-19 to children with cancer. Lancet Oncol (2020) 21(5):e235. doi: 10.1016/S1470-2045(20)30205-9
7. Monin L, Laing AG, Muñoz-Ruiz M, McKenzie DR, del Molino del Barrio I, Alaguthurai T, et al. Safety and immunogenicity of one versus two doses of the COVID-19 vaccine BNT162b2 for patients with cancer: interim analysis of a prospective observational study. Lancet Oncol (2021) 22(6):765–78. doi: 10.1016/S1470-2045(21)00213-8
8. Corti C, Crimini E, Tarantino P, Pravettoni G, Eggermont AMM, Delaloge S, et al. SARS-CoV-2 vaccines for cancer patients: a call to action. Eur J Cancer (Oxford Engl 1990). (2021) 148:316–27. doi: 10.1016/j.ejca.2021.01.046
9. Ma Y, Liu N, Wang Y, Zeng J, Hu YY, Hao W, et al. Immune checkpoint blocking impact and nomogram prediction of COVID-19 inactivated vaccine seroconversion in patients with cancer: a propensity-score matched analysis. J Immunother Cancer (2021) 9(11):e003712. doi: 10.1136/jitc-2021-003712
10. Poparn H, Srichumpuang C, Sosothikul D, Jantarabenjakul W, Lauhasurayotin S, Techavichit P, et al. Immune response after 2 doses of BNT162b2 mRNA COVID-19 vaccinations in children and adolescents with cancer and hematologic diseases. Asian Pacific J Cancer Prev APJCP. (2022) 23(6):2049–55. doi: 10.31557/APJCP.2022.23.6.2049
11. Frenck RW, Klein NP, Kitchin N, Gurtman A, Absalon J, Lockhart S, et al. Safety, immunogenicity, and efficacy of the BNT162b2 covid-19 vaccine in adolescents. New Engl J Med (2021) 385(3):239–50. doi: 10.1056/NEJMoa2107456
12. Wimberly CE, Towry L, Davis E, Johnston EE, Walsh KM. SARS-CoV-2 vaccine acceptability among caregivers of childhood cancer survivors. Pediatr Blood cancer. (2021) 69(6):e29443. doi: 10.1002/pbc.29443
13. Choi DK, Strzepka JT, Hunt SR, Tannenbaum VL, Jang IE. Vaccination in pediatric cancer survivors: vaccination rates, immune status, and knowledge regarding compliance. Pediatr Blood cancer. (2020) 67(10):e28565. doi: 10.1002/pbc.28565
14. Hung TY, Kotecha RS, Blyth CC, Steed SK, Thornton RB, Ryan AL, et al. Immunogenicity and safety of single-dose, 13-valent pneumococcal conjugate vaccine in pediatric and adolescent oncology patients. Cancer (2017) 123(21):4215–23. doi: 10.1002/cncr.30764
15. Kotecha RS, Wadia UD, Jacoby P, Ryan AL, Blyth CC, Keil AD, et al. Immunogenicity and clinical effectiveness of the trivalent inactivated influenza vaccine in immunocompromised children undergoing treatment for cancer. Cancer Med (2016) 5(2):285–93. doi: 10.1002/cam4.596
16. China National medical products administration guidelines for grading standards of adverse events in clinical trials of preventive vaccines (2019). Available at: https://www.nmpa.gov.cn/xxgk/ggtg/qtggtg/20191231111901460.html.
17. Bertsekas DP, Tseng P. Relaxation method for minimum cost ordinary network flow problems. Operat Res (1988) 36(1). doi: 10.1287/opre.36.1.93
18. Douglas Bates MM, Bolker B, Walker S. lme4: linear mixed-effects models using eigen and S4 . Available at: http://CRAN.R-project.org/package=lme4.
19. Ho DE, Imai K, King G, Stuart EA eds. Matching as nonparametric preprocessing for reducing model dependence. parametric causal inference. In: Political Analysis (2007) 15:199–236.
20. Iacus S, King G, Porro G. Cem: software for coarsened exact matching. J Stat Software (2009) 30(9):1–27. doi: 10.18637/jss.v030.i09
21. Austin PC. Balance diagnostics for comparing the distribution of baseline covariates between treatment groups in propensity-score matched samples. Stat Med (2009) 28(25):3083–107. doi: 10.1002/sim.3697
22. Ziegel, Eric R. Statistical methods for rates and proportions (Book). Technometrics (2004) 46(2):263–4.
23. Sakuraba A, Luna A, Micic D. Serologic response following SARS-COV2 vaccination in patients with cancer: a systematic review and meta-analysis. J Hematol Oncol (2022) 15(1):15. doi: 10.1186/s13045-022-01233-3
24. Fendler A, Shepherd STC, Au L, Wu M, Harvey R, Schmitt AM, et al. Omicron neutralising antibodies after third COVID-19 vaccine dose in patients with cancer. Lancet. (2022) 399(10328):905–7.
25. Peng X, Gao P, Wang Q, Wu HG, Yan YL, Xia Y, et al. Prevalence and impact factors of COVID-19 vaccination hesitancy among breast cancer survivors: a multicenter cross-sectional study in China. Front Med (2021) 8:741204. doi: 10.3389/fmed.2021.741204
26. Kotecha RS. COVID-19 vaccination for children with cancer. Pediatr Blood cancer. (2022) 69(2):e29340. doi: 10.1002/pbc.29340
27. Carr S, Allison KJ, Van De Velde LA, Zhang K, English EY, Iverson A, et al. Safety and immunogenicity of live attenuated and inactivated influenza vaccines in children with cancer. J Infect Dis (2011) 204(10):1475–82. doi: 10.1093/infdis/jir561
28. Goossen GM, Kremer LC, van de Wetering MD. Influenza vaccination in children being treated with chemotherapy for cancer. Cochrane Database Systemat Rev (2013) 8):Cd006484. doi: 10.1002/14651858.CD006484.pub3
29. Henderson TO, Fowler BW, Hamann HA, Nathan PC, Whitton J, Leisenring WM, et al. Subsequent malignant neoplasms in the childhood cancer survivor study: occurrence of cancer types in which human papillomavirus is an established etiologic risk factor. Cancer (2022) 128(2):373–82. doi: 10.1002/cncr.33922
30. Gibson TM, Mostoufi-Moab S, Stratton KL, Leisenring WM, Barnea D, Chow EJ, et al. Temporal patterns in the risk of chronic health conditions in survivors of childhood cancer diagnosed 1970–99: a report from the childhood cancer survivor study cohort. Lancet Oncol (2018) 19(12):1590–601. doi: 10.1016/S1470-2045(18)30537-0
Keywords: SARS-CoV-2, childhood cancer patients, variants of concern, immune response, propensity score matching
Citation: Ma Y, Chen B, Wang Y, Zhu P, Liu N, Zhang Z, Zhong G, Fu G, Wang D, Cao L, Bai S, Wang Y, Chen S, Wei X, Lv J, Zhang A and Wang X (2023) Reducedhumoral response against variants of concern in childhood solid cancer patients compared to adult patients and healthy children after SARS-CoV-2 vaccination. Front. Immunol. 14:1110755. doi: 10.3389/fimmu.2023.1110755
Received: 29 November 2022; Accepted: 03 April 2023;
Published: 25 May 2023.
Edited by:
Claudia Vanetti, University of Milan, ItalyReviewed by:
Xinghua Song, Sixth Affiliated Hospital of Xinjiang Medical University, ChinaLiang Li, Nanxishan Hospital of Guangxi Zhuang Autonomous Region, China
Copyright © 2023 Ma, Chen, Wang, Zhu, Liu, Zhang, Zhong, Fu, Wang, Cao, Bai, Wang, Chen, Wei, Lv, Zhang and Wang. This is an open-access article distributed under the terms of the Creative Commons Attribution License (CC BY). The use, distribution or reproduction in other forums is permitted, provided the original author(s) and the copyright owner(s) are credited and that the original publication in this journal is cited, in accordance with accepted academic practice. No use, distribution or reproduction is permitted which does not comply with these terms.
*Correspondence: Xinjia Wang, xjwang4@stu.edu.cn; Ao Zhang, zhangao@sysucc.org.cn